- 1Department of Physical Geography, Trier University, Trier, Germany
- 2Department of Physical Geography, Goethe University Frankfurt am Main, Frankfurt am Main, Germany
- 3Department of Geography, Ibn Zohr University, Agadir, Morocco
The argan woodlands of South Morocco represent an open-canopy dryland forest with traditional silvopastoral usage that includes browsing by goats, sheep and camels, oil production as well as agricultural use. In the past, these forests have undergone extensive clearing, but are now protected by the state. However, the remaining argan woodlands are still under pressure from intensive grazing and illegal firewood collection. Although the argan-forest area seems to be overall decreasing due to large forest clearings for intensive agriculture, little quantitative data is available on the dynamics and overall state of the remaining argan forest. To determine how the argan woodlands in the High Atlas and the Anti-Atlas had changed in tree-crown cover from 1972 to 2018 we used historical black and white HEXAGON satellite images as well as recent WorldView satellite images (see Part A of our study). Because tree shadows can oftentimes not be separated from the tree crown on panchromatic satellite images, individual trees were mapped in three size categories to determine if trees were unchanged, had decreased/increased in crown size or had disappeared or newly grown. The current state of the argan trees was evaluated by mapping tree architectures in the field. Tree-cover changes varied highly between the test sites. Trees that remained unchanged between 1972 and 2018 were in the majority, while tree mortality and tree establishment were nearly even. Small unchanged trees made up 48.4% of all remaining trees, of these 51% showed degraded tree architectures. 40% of small (re-) grown trees were so overbrowsed that they only appeared as bushes, while medium (3–7 m crown diameter) and large trees (>7 m) showed less degraded trees regardless if they had changed or not. Approaches like grazing exclusion or cereal cultivation lead to a positive influence on tree architecture and less tree-cover decrease. Although the woodland was found to be mostly unchanged 1972–2018, the analysis of tree architecture reveals that a lot of (mostly small) trees remained stable but in a degraded state. This stability might be the result of the small trees’ high degradation status and shows the heavy pressure on the argan forest.
1 Introduction
Forest degradation by way of excessive wood exploitation, logging or overgrazing is a serious issue around the world (Lund, 2009; FAO, 2011) and especially in North Africa (Barbero et al., 1990; Wojterski, 1990). These forests have been used by locals for subsistence since the Neolithic, but with an increase in population and intensifying usage the already vulnerable ecosystems cannot regenerate fast enough (Barbero et al., 1990; Médail and Quézel, 1997), leading to forest-density decline (Le Polain de Waroux and Lambin, 2012). A reduced number of trees leaves a part of the soil without cover to shield it from erosion and soil degradation, thus creating less favourable conditions for saplings to develop (Alados and El Aich, 2008; Schnabel et al., 2009; Le Polain de Waroux and Lambin, 2012).
In rural, poorer areas, these dryland forests can provide a source of income for the local population who harvest the wood and turn it into charcoal (Rueda et al., 2015). The impact of charcoal production is still under discussion in the literature and ranges from sustainable forest protection and recovery (Hosier, 1993; Rueda et al., 2015) to forest degradation by selective cutting of suitable trees (Kouami et al., 2009; Ndegwa et al., 2016; Sedano et al., 2016; Kiruki et al., 2017). Charcoal production as a source of income might lead to a financial incentive for preservation of dryland forests (Rueda et al., 2015) but could also lead to excessive logging (Hosier, 1993; Faouzi, 2013). Sustainable solutions of coppicing with subsequent regrowth can be pursued, but an interplay of multiple pressure factors may lead to a degraded system all the same, e.g., failed regrowth due to heavy grazing pressure on young saplings (Hosier, 1993; Le Polain de Waroux and Lambin, 2012).
One such case is the Argania spinosa forest, which covered ∼950,000 ha in South Morocco in 2015 (Lefhaili and Amhajar, 2020). The argan population is seen as strongly degraded (Culmsee, 2004; Le Polain de Waroux and Lambin, 2012), especially due to overgrazing and browsing by goats, sheep and dromedaries but also due to the now illegal wood cutting and the encroachment of agricultural areas into formerly wooded regions (Lybbert et al., 2010; Le Polain de Waroux and Lambin, 2012; Kirchhoff et al., 2019a). The argan tree is the source of the valuable argan oil, which is used for cosmetic and alimentary purposes (Gharby and Charrouf, 2022). It has been produced by the locals for centuries (Charrouf and Guillaume, 2009), today it is produced by women’s cooperatives and marketed all around the world (Lybbert et al., 2010; Defaa et al., 2015). Due to the newly found income, the argan tree is seen as a source of revenue for a part of the population, which in turn helps to preserve the argan forest. In 1998, the Arganeraie was designated a UNESCO Biosphere Reserve (Charrouf and Guillaume, 2018).
However, the argan tree is also known among the local population for its high fuel value, especially when turned into charcoal (Faouzi, 2013). This resulted in the deforestation of 800,000 ha of argan forest in the 12th to 17th century for the use of firewood in the sugarcane industry (Berthier, 1966; Aït Hssaine, 2002). An increase of Argania spinosa forest, probably as pre-steppe scrub forest, in the 19th century could be measured using pollen archives (McGregor et al., 2009). Yet in the beginning of the 20th century, the argan forest was seen as degraded, which is why several protection measures were passed, declaring the argan forest property of the state (1925) and granting several usage rights to the local population (Monnier, 1965; Davis, 2005; Faouzi, 2013). Since the late 1990 s reforestation measures were implemented with grazing exclusion (Defaa et al., 2015) and paid contractors guarding the sites.
Monnier (1965) states that in 1965 700,000 ha of argan on state-owned land remained, with an additional 10,000–20,000 ha on private property. In recent years the argan forest covered between 870,000 (2013) and ∼950,000 ha (2015) (Faouzi, 2013; Lefhaili and Amhajar, 2020), depending on the source; the spatial extent is currently being analysed in a remote-sensing study (Sebbar et al., 2021). In their case study in Awluz in the Province of Taroudant, Le Polain de Waroux and Lambin (2012) compared aerial photographs from 1970 with satellite images from 2007 and revealed a 44.5% decline of forest density during this period. This rapid decline was explained by a multitude of factors, but namely fuelwood extraction and increasing aridity. Browsing and grazing herds of goats, sheep and dromedaries might be partially to blame for the loss of regrowth by feeding on saplings but are mostly responsible for tree degradation due to overbrowsing (Culmsee, 2005; Le Polain de Waroux and Lambin, 2012).
The diverse reports on argan-forest extent as well as argan-density decline show that the current state of the Arganeraie cannot be judged by the covered area alone (Le Polain de Waroux and Lambin, 2012). The knowledge of forest-density change is especially important for sustainable management, since bare land is more susceptible to soil degradation and erosion, leaving less fertile land for natural regrowth or reforestation measures. In this context, the change of the composition of the argan forest (trees/shrubs, canopy cover change, size of the trees) can be an additional factor to determine forest degradation or recovery (Culmsee, 2005; Dendoncker et al., 2020).
Specifically, this study aims to determine the development and state of the remaining argan woodlands on 30 test sites over three study areas in the Souss Basin. To this end, we aim at
1) examining the development of tree cover from 1972 to 2018 by way of tree density and crown size changes;
2) describing the current state of the argan woodlands by investigating tree architecture or browsing and cutting-related growth forms;
3) identifying specific land uses that influence tree-cover changes positively.
Ultimately, the objective of this research is to determine degradation or recovery on the chosen test sites. This is especially necessary because Morocco does not monitor the possible degradation of their forests (Lefhaili and Amhajar, 2020). Modern very high resolution satellite (VHRS) sensors such as WorldView have already proved their potential for individual tree mapping and tree-density and canopy-cover estimation (Hanan and Anchang, 2020). For the historical perspective on the last 50 years, 0.6–1.2 m resolution panchromatic satellite photographs taken 1971–1986 during the American reconnaissance programme HEXAGON have recently become accessible. Field records as well as ultra-high-resolution images taken with an unoccupied aerial system (UAS) are used to validate and give further information, as has been shown before for the Sahel zone (Dendoncker et al., 2020).
This paper is Part B of our research on monitoring dryland trees in South Morocco and uses the argan-tree database resulting from Part A of our study (Marzolff et al., 2022, this issue), where we investigated the potential of historical HEXAGON satellite imagery as a new data source for mapping open-canopy woodlands on the tree level.
2 Materials and methods
2.1 Study areas
The three study areas Ida-Outanane, Taroudant and Aït Baha are situated in the Souss Basin, an alluvial depression between the High Atlas and Anti-Atlas Mountains in Morocco (Figure 1). 30 test sites of 1 ha each were chosen to encompass varying altitudes, climate conditions, soil types and tree densities (see Supplementary Table S1; see also Kirchhoff et al., 2019a). The sites differ in various use-related, tree-cover and relief characteristics. Each test site may have several of these attributes, but we mostly chose neighbouring sites contrasting in one of them; the most salient attribute is recorded as suffix in the test site codes. Sites with code -n have no specific characteristics besides typical silvopastoral usage.
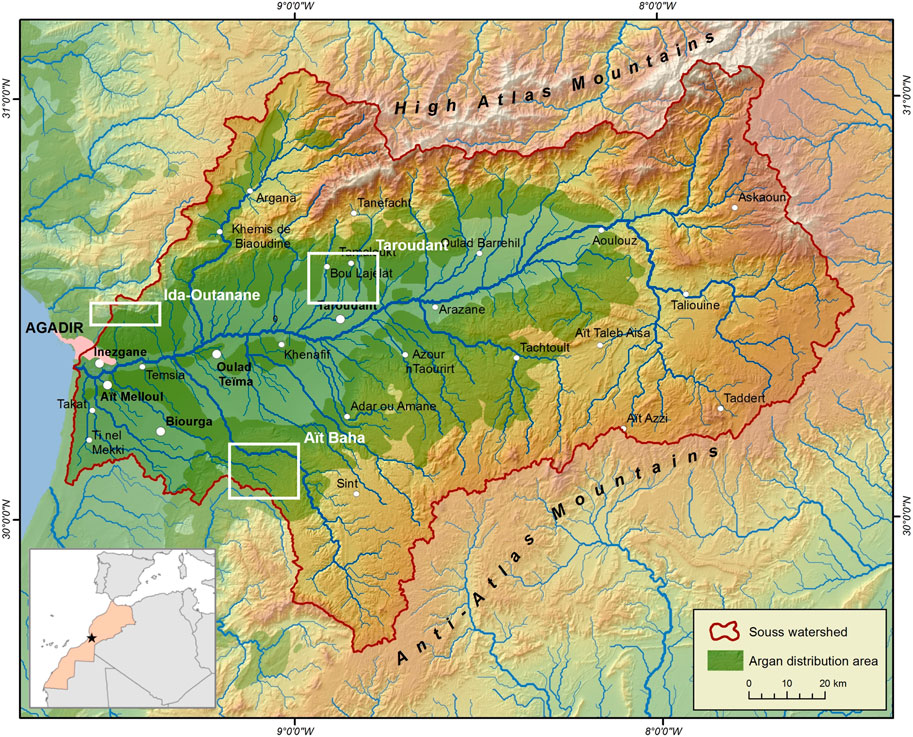
FIGURE 1. The location of the study areas Aït Baha, Ida-Outanane and Taroudant (white boxes) in the Souss Basin, Morocco. The argan distribution area is shown in green shades (see Msanda et al., 2005); the position of the main map is indicated with a star in the smaller location map.
The study area of Ida-Outanane is located near Agadir and the Atlantic Ocean on the southern foothills of the High Atlas. Its climate is maritime with an annual precipitation of 230–260 mm (data for the suburbs of Agadir, 20 km away, Díaz-Barradas et al., 2010) and a mean annual temperature of 18.4°C (data for Agadir, Saidi, 1995). The Paleozoic, Mesozoic and Cenozoic rocks of the High Atlas (Hssaisoune et al., 2016) are covered by mostly immature soils like Regosols, Leptosols and Fluvisols (Jones et al., 2013). The test sites are either used silvopastorally or for rainfed cultivation of wheat.
Like Ida-Outanane, the study area of Taroudant is situated on the southern foothills of the High Atlas. About 80 km from the coast, its climate is more continental (220 mm annual precipitation, 20°C mean annual temperature; Peter et al., 2014; Saidi, 1995). Four test sites are located in the foothills of the High Atlas, while seven lie on an alluvial fan that originates from the High Atlas and covers the Pliocene and Quaternary fluvial, fluvio-lacustrine and aeolian deposits of the Souss Basin (Aït Hssaine and Bridgland, 2009; Chakir et al., 2014). Besides Argania spinosa, shrubs and bushes such as Launaea arborescens, Ziziphus lotus, Acacia gummifera, Euphorbia spec. and Artemisia spec. make up the vegetation in this study area (Peter et al., 2014; Ain-Lhout et al., 2016; Zunzunegui et al., 2017). The Souss region, and especially the study area of Taroudant, has been dominated by a dynamic land use change, where traditional speculative rainfed agriculture is replaced by more profitable citrus plantations and greenhouses for banana and vegetable cultivation (d’Oleire-Oltmanns et al., 2012; Kirchhoff et al., 2019b; Peter et al., 2014).
The study area of Aït Baha is situated in the northern foothills of the Anti-Atlas Mountains. Closer to the Atlantic Ocean than Taroudant, its precipitation ranges from 250 to 350 mm annually with an average annual temperature of 18.7°C (Seif-Ennasr et al., 2016). Fluvisols, Regosols and Leptosols cover the Precambrian and Palaeozoic rocks of the Anti-Atlas (Jones et al., 2013). At three test sites cereals are cultivated on ploughing terraces, at three test sites argan trees are planted for reforestation purposes.
The 30 test sites listed in Supplementary Table S1 have been subject to extensive research in previous studies by the authors that focussed on the trees’ potential influence on the soil, including infiltration and soil erosion by wind and water (Kirchhoff et al., 2019a; Marzen et al., 2020; Kirchhoff et al., 2021). However, the state and long-term development of the argan woodlands themselves were not subject of these studies.
2.2 Study design
Part A of our study on tree-cover mapping by way of panchromatic (black and white) satellite images showed that results can be prone to errors, because depending on the image viewing geometry and illumination angle, tree shadows cannot be separated visually from the tree crown. With the most unfavourable conditions in the case of our study, shadows can make up over 40% of the mapped tree, which may lead to severe miscalculations and misinterpretations of forest cover if disregarded (Marzolff et al., 2022). To avoid potential errors associated with inaccurate tree-crown delineations, we did not map tree canopy cover on a continuous scale, but opted for measuring tree-cover changes on an ordinal scale. In the following, we will use the term “tree-crown size” for the ordinal crown sizes small, medium and large, and the term “tree-cover change” for the resulting ordinal change classes, which integrate increased or decreased crown size, loss or establishment of trees (see 2.4 as well as Marzolff et al., 2022). Vegetation cover in general may change (positively or negatively) or stay unchanged (Ries, 2005; Sloan et al., 2019). In undisturbed forests, we would expect tree changes in size between the years 1972 and 2018 as well as some dieback and regeneration. When left to grow the trees would increase in size or new trees could grow, therefore increasing tree cover. Since the argan woodlands are a multi-use silvopastoral system, a tree is normally influenced by a multitude of factors unless it is purposely protected. Whole branches may be cut as firewood, diminishing the tree in size and canopy density. The tree may be pollarded or cut completely for charcoal or firewood. If the tree is cut, it will usually resprout with multiple new shoots that need to be protected from browsing to grow. If a small argan tree or saplings from a stump are continually browsed, they will not develop into a tall-stemmed tree but become a dense shrub. Owing to this multitude of factors influencing argan cover, we will determine the tree-crown size change and tree-cover change of all trees on the chosen test sites. We may expect tree sizes and tree cover to have changed on every test site if trees are not hindered from growing. Due to the variety of test sites (usage, distance from settlements etc.) the degree of tree-cover change will also differ between sites. By incorporating knowledge about tree-cover change, tree-crown sizes and plant architecture in 2018 (see Sections 2.3, 2.4), a conclusion about degradation or recovery can be drawn.
2.3 Field mapping and tree architecture classification
We mapped the location of each individual argan tree (2,494 in total) on our 30 test sites in the field in 2018. This enabled us to record small trees as well and also to clearly identify the individual trees in overlapping canopy clusters. Argan trees often have more than one trunk (Culmsee, 2004; Buernor et al., 2021) and form coalescing crowns, but in the field, we could differentiate between multi-stemmed trees (counted as one plant) and single-stemmed trees growing in close groups with clumped canopies. In addition to mapping the trees, each tree was assigned a field number and an architecture class based on its overall appearance (Figure 2), following and modifying the categorisation first described by Culmsee (2004). Tree architecture has been shown to relate strongly to browsing intensities (Culmsee, 2004; Fornara and Du Toit, 2007; Moncrieff et al., 2011) as well as other influences such as cutting, coppicing or pollarding. Trees of architecture class 1–2 are not or little affected by browsing. Trees of classes 3–4 show increasing signs of browsing by climbing goats, but are less limited in their functions (like e.g., fruit production) than trees in architecture classes 5–8, which take the form of shrubs and cushion-like bushes. In addition, we recorded if the trees had multiple trunks, which could be an indication of coppicing in the past.
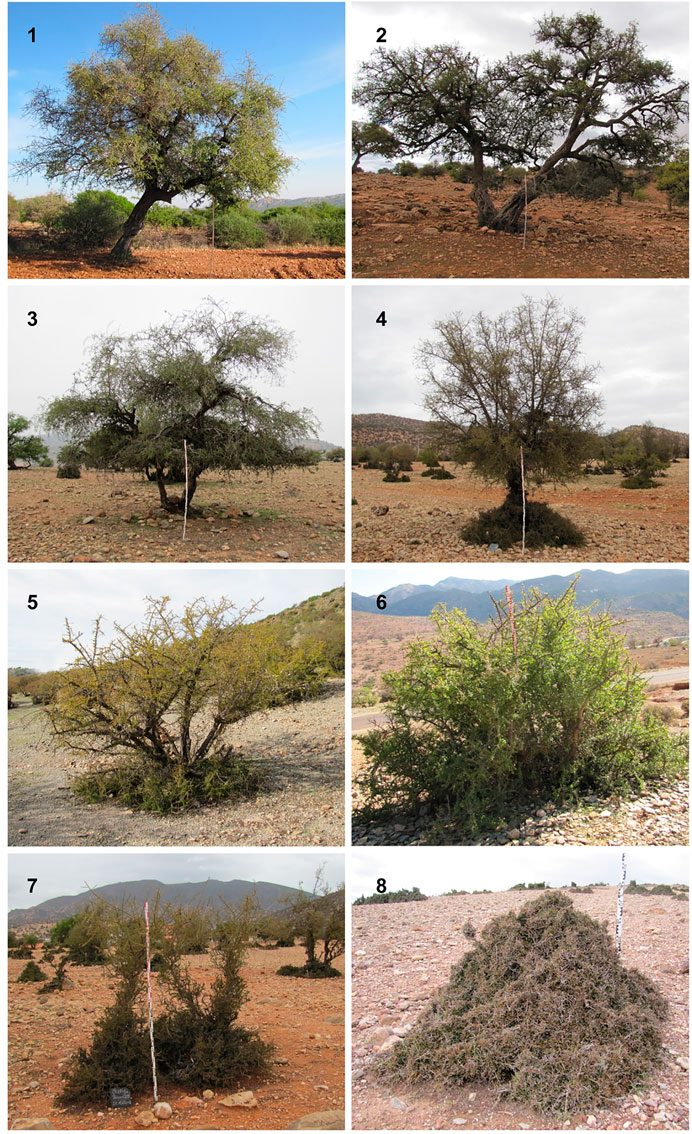
FIGURE 2. Tree architecture classes from 1 to 8 with increasing tree degradation (classification modified and expanded from Culmsee, 2004). 1: Tree with big, round crown, one or multiple trunks easily visible; 2: Tree with multiple, diverging trunks, no densification of branches and leaves; 3: Tree with denser crown, one or multiple trunks are visible despite basal cushions (if present), crown is umbrella-shaped, where branches are pulled down- or upward; 4: Tree with one or multiple trunks, strongly developed basal cushion, possible umbrella-shaped crown; 5: Transition form between tree and shrub with multiple visible trunks, often low in height; 6: Shrub with dense crown, number of trunks due to basal cushion not visible; 7: Shrub with some outgrowing branches, dense, number of trunks not visible (cone-shaped cushion); 8: Low, compact shrub (cone-shaped cushion), dense to very dense.
2.4 Satellite-based tree-cover change mapping
For quantifying tree-cover change between July 1972 and March 2018, we used two types of very high resolution satellite (VHRS) imagery: historical panchromatic satellite photographs taken with the KH-9 camera of the military reconnaissance program HEXAGON (approx. 1 m resolution) and recent multispectral images from the WorldView satellites (pansharpened to 0.5 m resolution); see exemplary subsets in Figure 3. The analogue scanned HEXAGON images were manually georeferenced to the orthorectified WorldView images using a spline transformation. We verified the existence of each field-mapped tree on the HEXAGON images, and trees visually identifiable in 1972 but lost until 2018 were added to the database. First for 2018, then for 1972, the trees were classified into four classes by visual image interpretation of their crown diameters: small (<3 m diameter), medium (3–7 m), large (>7 m) or not present in 1972 or 2018. During this retrospective mapping process, we classified all trees as already existent in 1972 unless they were clearly not recognizable on the HEXAGON image. Any trees where change into another size class was not visually certain were given the same size class as 2018, i.e., unchanged size. For some sites, WorldView images dated from 1 year earlier (July 2017), but tree-crown size or presence could be updated to 2018 with the help of UAS imagery taken in March 2018.
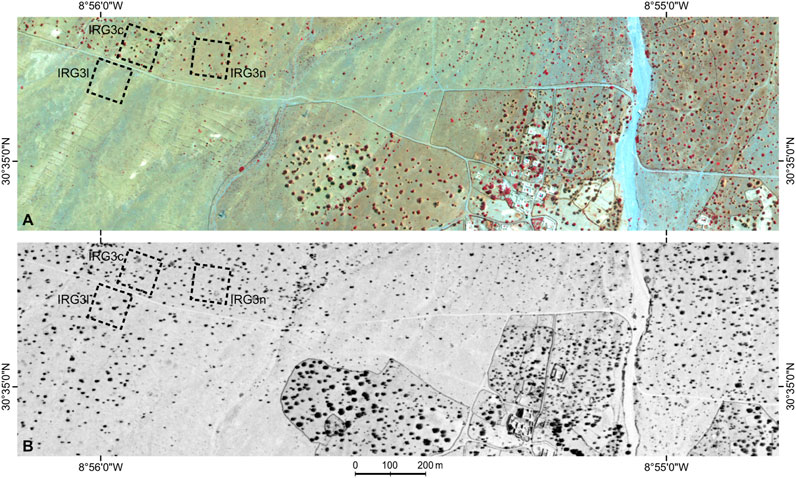
FIGURE 3. Exemplary subsets of the satellite imagery used for tree-cover change mapping. Part of the Taroudant study area, with three test sites marked in dashed lines. The village of Aït Bou Hamida in the lower right was once specialised in charcoal production, which is reflected in the low tree densities in its surroundings. Some remains of charcoal kilns are visible as grey marks on and between IRG3c and IRG2n (see Figure 11B). (A) False-colour infrared WorldView-2 image of 6 July 2017 (B) panchromatic HEXAGON KH-9 stereo-panoramic camera photograph of 16 July 1972. Note the striking comparability of woodland details and other landscape features in the two images in spite of the very different sensor generations and types.
In Part A of our study (Marzolff et al., 2022) we found that HEXAGON imagery has a remarkably similar potential for identifying individual trees compared to the WorldView images, although both have limited ability for mapping small trees and trees in clumped-canopy groups. By using the combination of tree locations known a priori from field mapping with a retrospective mapping approach opting for stability in case of doubt, we could ensure very high accuracy for tree-density and tree-cover measures in 2018 and a conservative tendency towards underestimation rather than overestimation of change. More details on the tree-mapping procedure, especially concerning the complex effects of resolution, viewing and illumination angles in the historical HEXAGON imagery, may be found in Part A of our study (Marzolff et al., 2022).
In order to quantify tree-cover change, we defined seven change classes from −3 to +3 reflecting size-class increase or decrease from 1972 to 2018 (Table 1), and attributed all trees accordingly. The tree-cover change class +3 (from not present 1972 to large tree 2018) could effectively not be observed in this study, and is indeed rather improbable since argan trees grow very slowly (Morton and Voss, 1987), especially under continuing grazing and usage pressure.
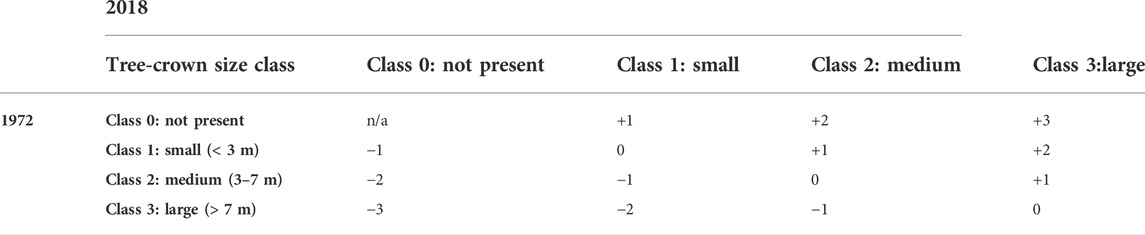
TABLE 1. Tree-cover change classification based on HEXAGON satellite images 1972 and WorldView satellite images 2018. Change-class values indicate ordinal-scaled positive or negative change between the tree-crown size classes.
2.5 Statistical analysis
The tree-cover change classes were analysed using descriptive statistics, and net change sums derived from the tree-cover change classes were calculated to find woodland trends for each test site. Averages for tree mortality and tree establishment for each test site were compared using a Wilcoxon-Test. Furthermore, to determine if there were significant differences between excluded and non-excluded as well as cultivated and non-cultivated test sites (see attributes in Supplementary Table S1), a Mann-Whitney-U-Test was carried out. All statistical analyses were performed with the software IBM SPSS Statistics for Windows, Version 25.0 (IBM Corp., Armonk, NY, United States).
3 Results
3.1 Changes of tree cover and density 1972–2018
As a representative example for the tree-cover change maps 1972–2018, Figure 4 shows the test site MAO1n, where the overall tree density and cover change is quite similar to the average of all 30 test sites (compare with change map Figure 7 in Marzolff et al., 2022). The tree density at MAO1n remained unchanged at 55 trees ha−1, and 33 (or 60%) of the 1972 trees remained in the same size class (yellow circles). 18 trees increased in crown size or were mapped as new (green), while only 14 trees decreased in crown size or were lost (red). However, positive change only reaches class +1, while the negative change classes −2 and −3 occur for 3 and 2 trees, respectively. Thus, in spite of the unchanged total number of trees, this site shows considerable ambiguity in change with a slight tendency towards net decrease of woody cover.
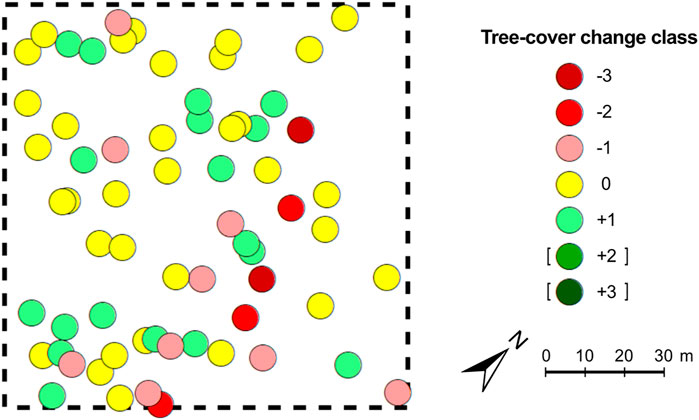
FIGURE 4. Map of tree-cover change 1972–2018 at test site MAO1n (30°04′09″N, 9°07′51″W). Change classes are defined according to Table 1; positive change (green) shows crown-size increase and new trees, negative change (red) shows crown-size decrease and lost trees. Unchanged trees are shown in yellow. This map is a representative example of tree-cover change although there is a high heterogeneity from site to site (see Figure 5).
Figure 5 shows the tree-cover change class distributions on the test sites. Some test sites show a highly dynamic tree-cover change between 1972 and 2018, despite a governmental logging ban, with numerous decreasing and increasing trees. However, unchanged trees are in the majority, with 62.3% of all mapped trees remaining in the same size class as in 1972. The highest percentage of unchanged trees (90.2%) can be found on test site ABH1n. With 20.7% of all mapped trees, tree-cover change +1 is the second most frequent class, indicating that either small new trees have grown or trees have grown to a larger size between 1972 and 2018 (e.g., from small to medium trees, from medium to large trees). 11.6% of all trees have decreased one size class (class −1, from large to medium trees, from medium to small trees, disappeared small trees). Classes −2, +2, and −3 are rarer with 3.5%, 1.4%, and 0.4%, respectively. These classes indicate greater changes, e.g., faster tree growth or logging/coppicing of large or medium trees. Positive and negative changes occurred on nearly every test site. However, one test site, IRG3l, shows 94.1% of negative change: It was specifically chosen as an example of a logged test site, featuring only three remaining argan shrubs in 2018 (see Figure 3).
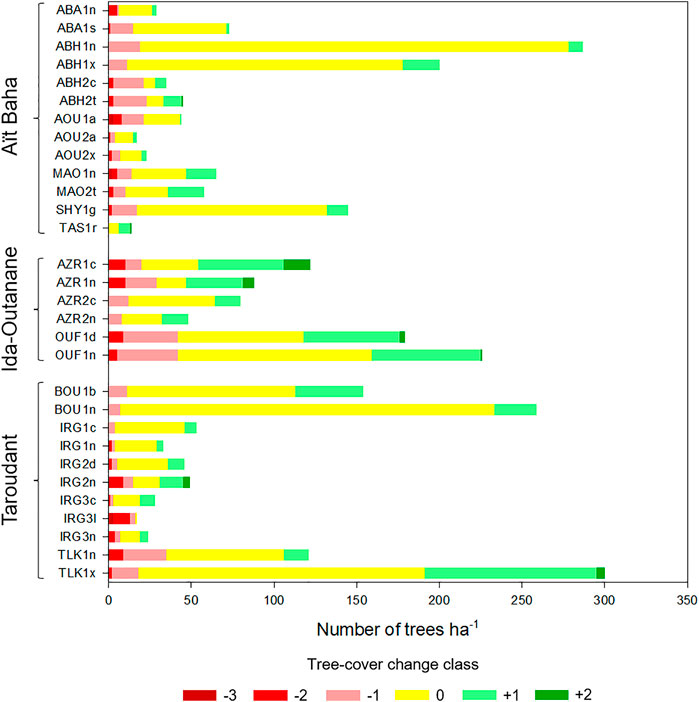
FIGURE 5. Distribution of tree-cover change classes (as defined in Table 1) between 1972 and 2018 at all 30 test sites. The stacked bars show the number of trees with negative changes on the left (crown-size decrease or loss of trees, red shades), unchanged in the middle (yellow) and with positive changes on the right (crown-size increase or new trees, green shades). Tree densities and development on the test sites are very heterogeneous.
Tree establishment (newly grown trees) and tree mortality (lost trees) are similar on the test sites. Overall, 292 trees have newly grown on the 30 test sites, 281 trees disappeared. On average 9.7 trees ha−1 have established themselves, while 9.4 trees ha−1 were lost between 1972 and 2018 (standard deviations: 11.2 and 8.4, respectively). Significant differences using a Wilcoxon-Test could not be found between the means.
The distribution of tree-cover change classes on each test site reveals the predominant changes on the test sites between 1972 and 2018. Figure 6 shows boxplots of the tree-cover change classes. Since great change in that time is rare, the upper and lower quartiles reach mostly from tree-cover change -1 to +1, while tree-cover change classes −3, −2 and +2 are outliers. For some test sites the upper and lower quartiles are both set on 0 because most trees on these test sites remained unchanged. The means as well as net change sums (NCS) also point in the direction of predominant change on the test site. They are especially low on test site IRG3l (as explained above) due to logging of trees. The length of the whiskers as well as the standard deviation show if the degree of change on the test site was similar (e.g., mostly tree-cover change class −1). For example, test site IRG2n has a mean of −0.06 indicating that nearly no net change has taken place, but a standard deviation of 1.27 due to the co-occurance of positive and negative tree-cover change classes on this site. This indicates a high ambivalence of change, which is also visible on the test sites ABH2t with a slightly more negative trend and on AZR1n. The test site AZR1c shows a high standard deviation (1.08) as well, which is not only due to positive and negative change on the test site but mainly due to the positive change differing (+1, +2). Trends in increasing and decreasing tree-cover can be found; sites with only positive or negative change, however, are rare. This is only the case for the test sites TAS1r (increasing change) and IRG3l (decreasing change). The aforementioned test site MAO1n (Figure 4) has a mean and net change sum which are both negative, although more trees show positive than negative change (Figure 5): This is due to the negative changes reaching the greater classes up to -3, while the positive changes occur only in the lowest class +1—a variability also reflected in the rather high standard deviation of 0.94. The rest of the test sites show mostly no change (10 test sites where upper and lower quartile are both 0), mostly positive change (9 test sites where lower quartile is 0, upper quartile is 1 and mean is in between) or mostly negative change (7 test sites where upper quartile is 0 or below).
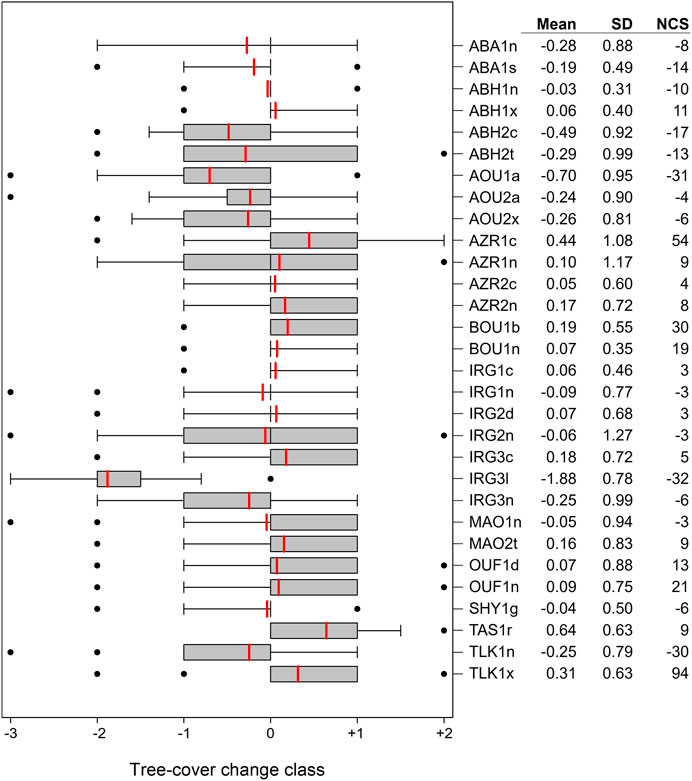
FIGURE 6. Boxplots of tree-cover change classes (as defined in Table 1) showing predominant trends (positive/negative change or no change) on the test sites. Means are shown in red as well as in the first table column; dots show outliers. Where boxes are not displayed, the upper and lower quartile are both 0, showing a predominantly unchanged test site. Standard deviations (SD; second table column) indicate variability of change; net change sums (NCS; third table column) show the weighted dominant change on each test site regardless of unchanged trees.
3.2 Architecture classes and tree-crown sizes as indicators of woodland state in 2018
Using the tree-cover change classes we were able to determine how the trees on our test sites changed between 1972 and 2018. Since we also recorded the architecture class of every tree in 2018 it is possible to give a more complete picture of the state of the argan trees in our study areas and on our test sites. This enables us to determine which trees are most at risk of (further) degradation. In the tree-architecture classification scheme described with Figure 2 (Section 2.3), classes 1 to 4 describe less degraded trees (still recognizable as trees), while classes 5 to 8 characterize trees/shrubs in a higher state of degradation. Trees in architecture classes 7 or 8 are by definition of small size, since they mostly resemble shrubs and are hindered by browsing from growing larger. Trees in architecture classes 5 or 6 are mostly small but might grow to a medium size. Trees in architecture classes 1 to 4 can be of any size, although classes 1 and 2 should mostly be of large or medium size.
Figure 7 shows the distribution of architecture classes for each combination of tree-cover change class and 2018 tree-crown size class. Overall, small trees are in the majority regardless of tree-cover change class, and small unchanged trees (0/small) account for nearly half (48.4%) of all remaining trees. 51% of these trees are in the degraded half of architecture classes; 19.5% of them are in the most degraded architecture class 8. These trees could have been cut, regrown and overbrowsed, or they are stable in a degraded state. Two thirds of newly grown small trees (part of tree-cover change class +1) show the more degraded stages 5–8, most of them are classified as architecture class 8 (40.3%). These are most likely cases where regrowth has been overbrowsed and could not develop into a fully grown tree. The change from medium to small trees—i.e., −1/small in Figures 7 — results in less degraded trees overall (64% in architecture classes 1–4), but also in 21.6% of trees classified as small overbrowsed shrubs (architecture class 8).
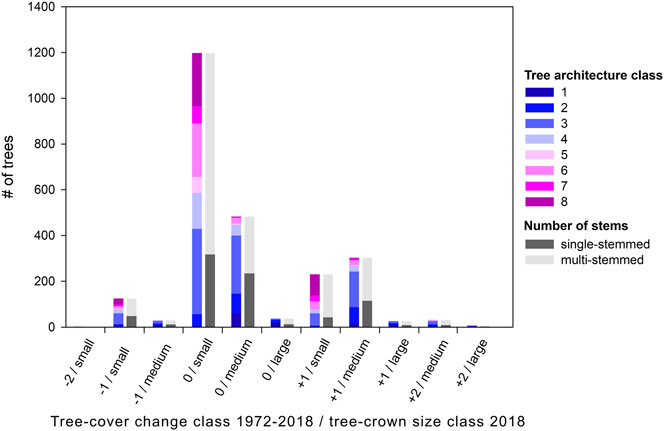
FIGURE 7. Distribution of architecture classes and stem numbers for each combination of tree-cover change class and 2018 tree-crown size class (for trees still present in 2018). Less degraded architecture classes 1 to 4 are shown in blue shades, more degraded classes 5 to 8 in pink shades. The second bar shows the percentage of single- and multi-stemmed trees. The x-axis shows the tree-cover change class 1972-2018 and crown-size class in 2018 (for further details see Table 1), e.g., −1/small thus describes small trees in 2018 that were of medium size in 1972.
34.2% of mapped trees in 2018 are medium-sized trees (3–7 m crown diameter), and most of these have not changed in crown size between 1972 and 2018. They are less degraded than small trees, with most trees classified as architecture class 3. This architecture class shows signs of browsing (goats can climb the trees) but is recognizable as a tree with a typical stem-and-crown tree shape unlike architecture classes 5–8, which only make up 7.5% of medium unchanged trees. This indicates that trees are safer from degradation by browsing when they have reached a certain size, in this case >3 m crown diameter. While large trees are in good condition (none in architecture classes 5, 6, 7 or 8), medium-sized trees are in their best condition when they are in tree-cover change class −1 (3.3% in architecture classes 5–8). This suggests that the change from large trees to medium trees rarely affects the architecture class: this change is possibly caused by the trimming of branches. Large trees only make up 2.9% of all mapped trees in 2018; they mostly have architecture class 2 when increased in size or architecture class 1 when unchanged.
Most of the mapped trees have more than one tree trunk regardless of their size or tree-cover change class. Unchanged medium trees show the highest percentage of single-stemmed trees (48.7%) as well as medium trees that have decreased one size class (−1/medium; 40%). 38.4% of trees changed from medium to small (−1/small) have only one trunk, which makes the interpretation of cutting and regrowth improbable for these trees. Small unchanged trees as well as small newly grown trees (+1/small) have the lowest percentages of single-stemmed trees (26.5% and 18.6%, respectively), indicating that these trees have been cut and (re-)grown, probably several times, and that the supposed new trees are actually regrowth of a cut tree which could not be observed on the Hexagon image of 1972.
3.3 Tree-cover change and woodland state in the different study areas
In order to investigate possible regional differences, we summarized the tree-cover changes per tree-crown size classes for each study area between 1972 and 2018 in Figure 8. The study area of Aït Baha is dominated by mostly unchanged small (53.1%) and medium (17.1%) trees with only little deforestation (8.8%), size changes (12.1% decrease/increase) or new (re-)growth (7.1%). Architecture class 6 is found often in Aït Baha (28.2%). In contrast, Ida-Outanane shows a larger proportion of changed trees. Ida-Outanane has a higher percentage of medium trees than the other two study areas, possibly due to the more maritime climate. The proximity to the city of Agadir also offers other occupation possibilities, whereas the rural population in the study areas of Aït Baha and Taroudant often makes their livelihoods from the herding and sale of argan-browsing goats or sheep. The proportion of newly or re-grown trees is also quite high (15.9%). Although 15.6% of trees have disappeared, a larger part of this study area has positively changed. Most of the trees in Ida-Outanane have changed positively and are in good condition (75.2% in architecture classes 1–4), but many are heavily browsed (16.4% in architecture class 8) or have disappeared completely. As in the other two study areas, most trees in Taroudant are small and have not changed (45.9%). When changed, the trees have only changed little (change class +1: 21.7%) and show more positive change (22.5%) than negative (11.5%) in this study area. The most deforested test site IRG3l is also located in this study area. Taroudant has the highest percentage of trees in architecture classes 7 (6.2%) and 8 (23.5%), which is mostly due to the two test sites BOU1b and BOU1n.
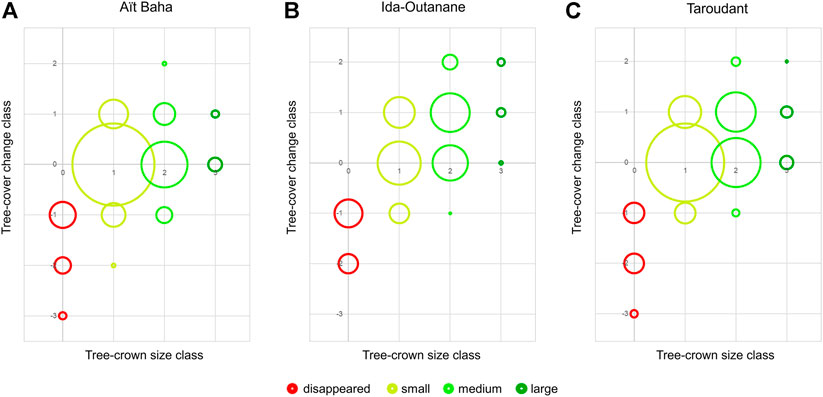
FIGURE 8. Bubble diagrams showing the tree-cover changes and tree-crown size distribution in the study areas Aït Baha (A), Ida-Outanane (B) and Taroudant (C). Tree-crown sizes (as defined in Table 1) are shown on the x-axis and marked in colours (red: 0 = disappeared, yellow: 1 = small, light green: 2 = medium, dark green: 3 = large). The y-axis describes the tree-cover change class from -3 (large tree in 1972, disappeared in 2018) to +2 (regrowth from not present in 1972 to medium tree in 2018 or small tree in 1972 to large tree in 2018), for further details on tree-cover change classes see Table 1. The size of the bubbles is proportional to the percentage of each tree-cover change/tree-size class combination in each study area.
3.4 Tree-cover change and woodland state on test sites under different usages
All test sites were chosen for different attributes, mostly based on land use. On some test sites grazing animals are excluded by fences or thorny hedges for preventing damage to young seedlings in reforestation measures or to protect the harvest on cultivated land. Hypothetically, trees on test sites under cultivation—even when easily accessible—are less grazed, since local shepherds lead their herds away from these test sites traditionally used for cultivation by acquainted locals. All test sites can be allocated to cultivated (i.e., showing present or past signs of cultivation) or non-cultivated test sites as well as excluded or non-excluded test sites (Figure 9). Cultivated (Figure 9A) compared to non-cultivated sites (Figure 9B) show both higher negative (cultivated: 21.0% vs. non-cultivated: 14.1%) and higher positive changes (33.9% vs. 18.8%) and less unchanged trees (45.1% vs. 67.1%) using the total number of trees. Most of the negative changes on cultivated test sites (73%) occurred due to the removal of trees. Excluded (Figure 9C) compared to non-excluded sites (Figure 9D) overall showed a slightly higher percentage of unchanged trees (excluded 64.0% vs. non-excluded: 61.1%) but lower negative (10.9% vs. 18.9%) and slightly higher positive changes (25.0% vs. 20.0%), while the difference mostly affected medium trees (size class 2 with tree-cover change classes 1 and 2 are higher for excluded, lower for non-excluded test sites). A Mann-Whitney-U-Test found significant differences between the percentage of positive changes on cultivated and non-cultivated test sites (p < 0.05) and more specifically between the percentage of change class +1 (p < 0.05). There are no significant differences for excluded and non-excluded test sites.
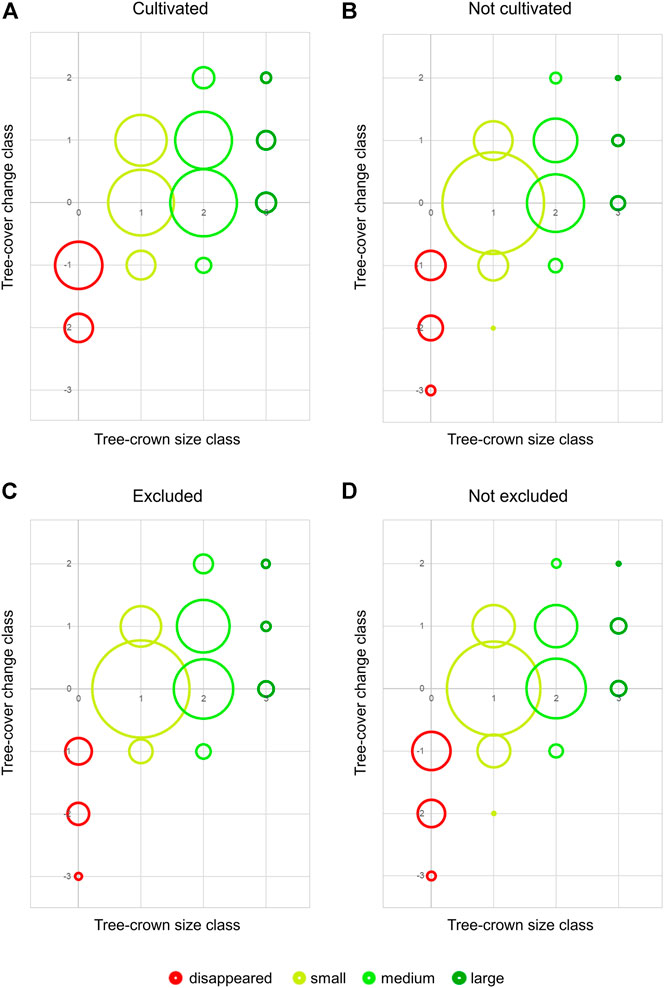
FIGURE 9. Bubble diagrams showing the tree-cover changes and tree-crown size distribution for cultivated/non-cultivated (A,B) and excluded/non-excluded (C,D) test sites. Tree-crown sizes (as defined in Table 1) are shown on the x-axis and marked in colours (red: 0 = disappeared, yellow: 1 = small, light green: 2 = medium, dark green: 3 = large). The y-axis describes the tree-cover change class from −3 (large tree in 1972, disappeared in 2018) to +2 (regrowth from not present in 1972 to medium tree in 2018 or small tree in 1972 to large tree in 2018). The size of the bubbles is proportional to the percentage of each tree-cover change/tree-crown size class combination at cultivated/non-cultivated (A,B) and excluded/non-excluded (C,D) test sites.
Cultivated/excluded and non-cultivated/non-excluded test sites can also be compared according to the distribution of architecture classes. Both cultivated and excluded sites show higher values than their counterparts for architecture classes 1, 2, and 3 and lower values for architecture classes 6, 7, and 8. Significant differences (using a Mann-Whitney-U-Test, p < 0.05) between cultivated and non-cultivated test sites can only be found for architecture class 8 with means of 4.4% and 15% for cultivated and non-cultivated, respectively. For excluded and non-excluded test sites significant differences are present for architecture class 7 (averages of 0.8% and 7.1%, respectively), between the sum of architecture classes 1, 2, 3, and 4 (the less degraded half, averages of 85.7% and 64.1%, respectively) and the sum of architecture classes 5, 6, 7, and 8 (the degraded half, means of 14.3% and 35.9%, respectively).
4 Discussion
The results show the high heterogeneity of tree-cover change in argan woodlands, ranging from mostly positive change (increase of crown sizes, regrowth) and relatively stable growth forms to near-total deforestation (IRG3l) with the remaining trees as degraded shrubs. As is evident from Figures 5, 6 our test sites do not show one direction of evolution but several. Most test sites have a high percentage of unchanged trees, i.e., trees that did not cross size class boundaries, although they might have grown or have been reduced in size. Also, very small trees or trees growing in clumped-canopy groups were more difficult to identify in the panchromatic historical HEXAGON images than the recent multispectral WorldView images. Where in doubt, our retrospective mapping approach therefore opted for unchanged size, and thus slightly overestimated woodland stability (see Marzolff et al., 2022). However, it is clearly evident that on nearly all test sites, many of the analysed trees stayed relatively unchanged over a period of 46 years.
4.1 Tree-density changes of argan woodlands
The degradation of the argan woodlands (in any form) has been discussed previously (Culmsee, 2005; Le Polain de Waroux and Lambin, 2012; Defaa et al., 2015; Kirchhoff et al., 2019a). Regarding tree-density change as a measure of degradation, our results—an average tree-density increase of 0.4%—are in contrast to the argan-forest decline of −44.5% observed by Le Polain de Waroux and Lambin (2012) between 1970 and 2007 some 60 km further east, in the hillslopes of the Anti-Atlas Mountains. However, while our tree counts are based on field mapping 2018 and a retrospective monitoring approach (FB approach; Marzolff et al., 2022), the densities reported by Le Polain de Waroux and Lambin (2012) are based on a remote sensing imagery. Their method is more similar to our WorldView-based (WB) approach, which tends to overestimate negative change. Using this approach, we found an average −8.5% density decrease, with 3 of 30 sites losing 40%–100% of trees. Our study areas also span a larger regional variety with higher heterogeneity in argan woodland evolution, and generally have higher tree densities: Le Polain de Waroux and Lambin report a mean change from 27.4 to 15.2 trees/ha, as compared to our WorldView-based 57.8 to 53 trees/ha (Marzolff et al., 2022, Figure 6B; WB 1972 and WB 2018).
4.2 Factors influencing argan woodland change: forest clearing and wood cutting
For this study, we looked only at the situation on our test sites (wooded in 2018, except most of IRG3l) and explicitly not at historic or recent argan degradation on a large scale, which would have to include the forest clearings in the 1970s to make room for the irrigated cultivation of legumes or citrus plantations (El Alaoui, 1999). Ouassou et al. (2006) state that the loss of forest is mainly due to fuelwood collection while land clearance was secondary, possibly due to the rising awareness regarding deforestation in the 1990s (El Alaoui, 1999) and the subsequent designation of the argan forest as a UNESCO Biosphere Reserve in 1998 (Charrouf and Guillaume, 2018), which could be an explanation for the regrowth on some test sites. In the end of the 1990s the trade with argan charcoal diminished (Le Polain de Waroux, 2013), which had been very popular due to its combustive properties (Rocher, 1926) in some villages that specialised on carbonisation of argan wood between 1970 and 1990. This was also the reason for the change on test site IRG2n (which showed ambivalent tendencies in Figure 6) and the clearing of the test site IRG3l. Since not only old trunks or underbrush were taken but fully intact trees (Rocher, 1926), there were fears that without state intervention the argan forest would disappear completely (Boudy and Chalot, 1935). Figure 10B shows an example for charcoal-production evidences (kilns used by the French military during the colonisation) that can be found on and near our test sites. The test sites where argan trees had once been cut—long before 1972—show different tendencies from increasing, decreasing or ambivalent tree-cover change. Only IRG3l was deforested so irredeemably—after 1972—that it is still bare today. Figure 10A shows forest-clearing activities in 1972∼2 km west of the aforementioned test sites, where trees were pollarded heavily rather than clear-cut and (most) have since regrown. However, cutting the trunk at ground-level was also common, even with removal of the roots (Rocher, 1926) or cutting of regrowth (explanations for disappeared trees), and Figure 10C shows the remains of a root where a tree once stood.
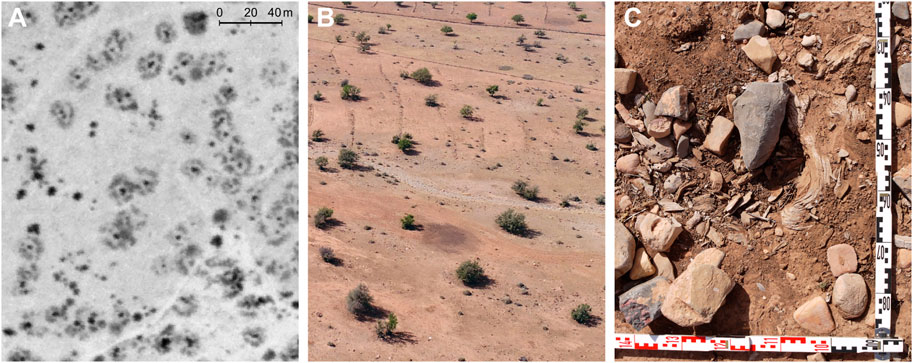
FIGURE 10. Evidences of argan-forest clearing activities. (A) Heavy pollarding and clear-cutting in progress west of IRG3 test sites (8°57′10″W, 30°35′30″N), as seen on HEXAGON satellite imagery of July 1972 (4.5 ha subset of 200 ha clearing area). Cut branches are piled up in rings around the remains of tree stems. (B) Site of a charcoal kiln on test site IRG3n (8°55′54.8″W, 30°35′14.8″N) as seen on oblique UAS image of October 2018 (a second kiln site is visible in the upper right corner). The ash-darkened sites are already present on 1967 CORONA satellite imagery; 98 of them can still be seen today, covering an area of 170 ha. (C) Site of a large tree cut after 1972; parts of the root remain visible in the field (March 2020).
4.3 Factors influencing argan woodland change: charcoal production and overbrowsing
Since there are no active charcoal kilns in the region today due to bottled gas being readily available as fuel, recent deforestation for the transformation to charcoal is not visible on our test sites. Faouzi (2013) states, however, that many households can still not afford gas or charcoal for cooking and thus use wood taken from the forest or the cuttings of citrus plantations, which could be a reason for trees decreased in size (parts of tree-cover change classes −1 and −2). Wood cutting is acknowledged by locals as part of the problem (Le Polain et Waroux and Lambin, 2012) while grazing/browsing of argan trees by local herds is seen as less of a problem for tree survival but rather for the short-term harvest (Lybbert et al., 2010). Excessive browsing/grazing as well as soil compaction by trampling may make regrowth from seedlings exceedingly difficult (c.f. Fornara and Du Toit, 2007; Rasmussen et al., 2018), which could also explain trees decreased in size (cutting and regrowth) or unchanged trees. Since natural regeneration is very rarely possible, coppicing can be a method of rejuvenation for mature argan forests (Morton and Voss, 1987; Nouaim et al., 2002). If the argan tree is cut, it can rejuvenate with multiple saplings from the old wood (Morton and Voss, 1987), thus resulting in multiple trunks (see Figure 7). This regrowth must, however, be protected against overgrazing/overbrowsing. A tree’s recovery after cutting could be delayed or halted if grazing pressure continued during the growing phase (Hosier, 1993). A low growth rate could also indicate that the tree has been degraded in the past (by cutting and/or overbrowsing), is now in a stable state where it cannot degrade much further but is also prevented from growing (by, e.g., overbrowsing). The stability of the trees can be caused by their high degradation status. This is probably the case on the test sites BOU1b and BOU1n (>66% change class 0, mostly architecture classes 7 and 8). Both sites have obviously been clear-cut prior to 1972, and stump regrowth has since been contained by extreme grazing pressure to the slow development of highly densified cone-shaped cushions (see Figure 2, architecture class 8). Culmsee (2005) makes the argument that cone-shaped cushions (architecture classes 7 and 8) are maximally browsed and are thus shaped only by grazing pressure. This is possible if young trees are continually browsed, but it can also be a combination of felled trees that are now hindered from regrowing. Small unchanged trees are the majority of trees mapped for this study, of these 51.0% of trees are in the degraded half of architecture classes suggesting stability in a degraded state. Medium unchanged trees do not show this tendency and are clearly recognizable as trees. If a tree has reached a certain crown size, in this case >3 m diameter, they seem to be secure from degradation to a shrub, although still browsed (as seen in the high percentage of architecture class 3). Tree architecture is in general shaped by the selective pressures of their environment (Archibald and Bond, 2003; Moncrieff et al., 2014), which are numerous for the argan tree. Although argan trees are resilient in their harsh environment, the high demand for charcoal coupled with high grazing pressure led to a degradation described by many authors (Boudy and Chalot, 1935; Nouaim et al., 2002; Le Polain de Waroux and Lambin, 2012; Le Polain de Waroux, 2013). Since land-usage rights in argan woodlands are complex, this could lead to a behaviour focusing on overexploitation in the short-term (Ouassou et al., 2006), especially in parts of the forest that are free to use for everyone (Lavauden, 1941). A study by Lybbert et al. (2011), analysing vegetation-index (NDVI) time series from before and after 1998, found that the argan woodlands had not visibly improved since being designated a Biosphere Reserve, especially in proximity to argan-oil markets, which corresponds to our results, since most mapped trees are unchanged. This also means that the space between trees has not changed, the soil is unprotected and vulnerable to water and wind erosion making a rehabilitation on the test sites very improbable (Kirchhoff et al., 2019a; Marzen et al., 2020; Kirchhoff et al., 2021).
4.4 Influence of land use: grazing exclusion and cultivation
Fencing-off test sites to exclude grazing and browsing herds has a slightly positive effect on the argan trees in our study. However, many trees still remain unchanged, possibly due to the test sites having been excluded for only a short time. However, this could be due to one test site (TLK1×), which has the highest tree density and which has been fenced in since at least October 2004. Other excluded test sites are AOU1a and AOU2×, which showed decreases in woody cover. Yet, trees on excluded test sites are mostly in less degraded architecture classes than trees on non-excluded sites. Cultivation, in turn, leads to the removal of some trees but leads to an increase in tree size and density over time. Since cultivated sites are not grazed, younger trees are protected and less trees are fully degraded to cone-shaped cushions (architecture class 8). Grazing exclusion without the cooperation of the local population is not necessarily the best way to protect, since top-down approaches like installing fences around areas used by the local population might result in break-ins of herds (Defaa et al., 2015). Cultivation on (parts of) a test site, however, seems to protect most of the existing trees, at least on our test sites, since the population is able to use the land and overgrazing is prevented. Blanco et al. (2015) found similar results for acacia trees in Southwestern Morocco, with high regeneration and recruitment on cultivated lands.
4.5 Degradation or recovery?
Degraded forests are generally connected with a loss of function and ecosystem services (Chazdon, 2008; Vásquez-Grandón et al., 2018). The identification as a degraded forest can vary depending on the definition of forest and forest degradation (Lund, 2009; Vásquez-Grandón et al., 2018; Gao et al., 2020). While Lund (2009) proposes a human-induced tree loss in the forest, Vásquez-Grandón et al. (2018) also mention loss of canopy cover but add changes in structure, composition as well as low or a lack of regeneration. These factors are found on most of our test sites, although we unfortunately have no data about tree architecture in 1972. A change in structure or composition is, however, not improbable (see Figure 7). Since we found a recovery of argan woodlands on some test sites and a degradation on others, a clear trend in one direction cannot be identified.
4.6 Future possibilities and challenges
Our results, which combine tree-cover and plant-architecture data, confirm the importance of forest structure estimations emphasised by Vásquez-Grandón et al. (2018) and Gao et al. (2020). In our study, tree-cover change was analysed from very high resolution satellite images, and tree architecture was mapped in the field. Data on tree-canopy cover on a continuous scale—rather than ordinal size classes—may reliably be mapped from VHRS images for larger trees and from UAS imagery also for small trees. However, the historical perspective, i.e., precise canopy-cover change, is hindered by the difficulties of delineating and measuring exact sizes of tree crowns in the HEXAGON images (Marzolff et al., 2022). A time-effective alternative to field surveys of every tree can be tree-structural information obtained from UAS imagery or 3D point clouds from LiDAR or photogrammetric analysis (Gao et al., 2020; Gobbi et al., 2020; Marzolff et al., 2020). Even a simple visual interpretation of shadows on UAS images can give important clues to the shape of a three-dimensional object (Aber et al., 2019:53), in this case the tree’s architecture. During our field campaigns, we also conducted aerial surveys with a quadcopter UAS (see Part A of our study; Marzolff et al., 2022, and Marzolff et al., 2020), and one example for a late-afternoon image is shown in Figure 11. The different shadow shapes, densities and lengths distinguish more shrub-like compact plants of architecture classes 6 and 7 from larger and taller single-stemmed and multi-stemmed trees of architecture classes 1–4. Insight about the height of the tree is given by different lengths of shadows, about the crown density by the compactness or fragmentation of the shadow; both can help to interpret a tree’s state not solely by its crown size. Despite the shadows’ distortion, many plant-architectural characteristics familiar from the normal ground-perspective (Figure 2) are recognizable and classifiable (possibly automatically). In the near future it is probable that UAS images will be increasingly available via data repositories and social networks; a shadow-based analysis of dryland tree cover and state could thus become possible worldwide by using such imagery from random sample sites. Citizen-science approaches could help gather UAS images where none are available and could be an efficient alternative to the more detailed but very time-consuming field-based approach for large-area forest structure estimation.
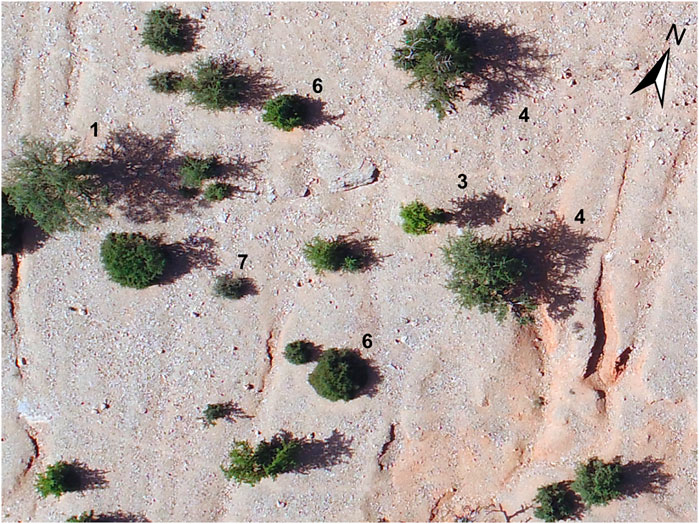
FIGURE 11. Tree shadows as indicator of tree architecture on test site SHY1g. Vertical UAS image taken on 8 March 2020, 16:15 local time. Note the different shadow shapes and lengths as well as the appearance of the crown (dense, sparse), which give insight into the growth form and architecture class affiliation of the tree (see Figure 2). Small and dense shadows are architecture classes 5–8, while large, fragmented shadows occur for architecture classes 1–4. Exemplary architecture classes are shown as numbers next to shadows.
5 Conclusion
In conclusion, we have shown that the tree-cover change between 1972 and 2018 showed a high heterogeneity on the argan test sites. Only few sites showed mostly positive or mostly negative tree-cover changes with few unchanged trees. However, 48% of all studied trees remained small (<3 m diameter) and unchanged with half of them being in a degraded state. Due to the pressure on the argan forest by wood cutting, overgrazing etc. these trees are hindered from growing but remain stable in a degraded state. The degradation of the tree can thus cause the stability found in the tree-cover change classes. The trees’ protection of the soil is therefore limited, with bare intertree areas degrading and leaving a less fertile base for young seedlings. Since overgrazing is a problem in southern Morocco, trees on sites with protection from browsing (however achieved) can grow relatively undisturbed. Some trees were cut to make room for cultivation or—before the ban on cutting—due to charcoal production, and regrowth seemed to be mostly protected from overbrowsing on these test sites. With financial incentives focused on specific sites they can be protected while afforestation sites (not planned with involvement of the local population) are subject to break-ins and further overgrazing. Herds of goats, sheep and sometimes dromedaries can roam the argan woodlands leaving little chance for regrowth. However, our data show an increase in tree density and tree size on few test sites, demonstrating that regrowth is possible under some circumstances. A regeneration of the argan forest could therefore be achievable with the support of the local population if grazing is controlled. Additional monitoring at regional scale could show argan woodland dynamics and would be an important resource to further understand their current status and future trends.
Data availability statement
The HEXAGON images used in this study are freely available from the USGS EarthExplorer. The argan-tree database may be obtained from the corresponding author on reasonable request.
Author contributions
MK, IM, MS, and JR designed the study; AAH provided resources and logistical support; IM, MS, AAH, and JR supervised the research activities; MK, IM and RS acquired the data; MK, IM, MS, and JR performed the analysis and interpretation; JR and IM acquired funding; MK and IM wrote the original draft. All authors performed review and editing of the final version of the manuscript.
Funding
Funding of this study by the Deutsche Forschungsgemeinschaft (DFG) as part of the ARGAN research project with project no. RI 835/24 and MA 2549/6-1 is gratefully acknowledged. WorldView and GeoEye satellite data were provided by the European Space Agency (ESA) under the Third Party’s Mission scheme, project no. 36256. TanDEM-X elevation data (used in orthorectification) were provided by the German Space Agency (DLR) under project no. DEM_HYDR1131. The publication was funded by the Open Access Fund of Universität Trier and the German Research Foundation (DFG) within the Open Access Publishing funding programme.
Acknowledgments
We thank the responsible local, regional and national Moroccan authorities for supporting our studies and allowing access to their territories. We are also grateful to Abdellatif Hanna and Khalil Hamouch for their considerable help with our fieldwork.
Conflict of interest
The authors declare that the research was conducted in the absence of any commercial or financial relationships that could be construed as a potential conflict of interest.
Publisher’s note
All claims expressed in this article are solely those of the authors and do not necessarily represent those of their affiliated organizations, or those of the publisher, the editors and the reviewers. Any product that may be evaluated in this article, or claim that may be made by its manufacturer, is not guaranteed or endorsed by the publisher.
Supplementary material
The Supplementary Material for this article can be found online at: https://www.frontiersin.org/articles/10.3389/fenvs.2022.896703/full#supplementary-material
References
Aber, J. S., Marzolff, I., Ries, J. B., and Aber, S. E. W. (2019). Small-format aerial photography and UAS imagery – principles, techniques and geoscience applications. Amsterdam, Netherlands: Elsevier.
Ain-Lhout, F., Boutaleb, S., Diaz-Barradas, M. C., Jauregui, J., and Zunzunegui, M. (2016). Monitoring the evolution of soil moisture in root zone system of Argania spinosa using electrical resistivity imaging. Agric. Water Manag. 164 (1), 158–166. doi:10.1016/j.agwat.2015.08.007
Aït Hssaine, A., and Bridgland, D. (2009). Pliocene-quaternary fluvial and aeolian records in the Souss Basin, southwest Morocco: A geomorphological model. Glob. Planet. Change 68 (4), 288–296. doi:10.1016/j.gloplacha.2009.03.002
Aït Hssaine, A. (2002). L’érosion des sols à Taroudant entre nature et societé. Bull. Réseau Eros. 21, 295–300.
Alados, C. L., and El Aich, A. (2008). Stress assessment of argan (Argania spinosa (L.) Skeels) in response to land uses across an aridity gradient. Translational asymmetry and branch fractal dimension. J. Arid Environ. 72 (4), 338–349. doi:10.1016/j.jaridenv.2007.06.015
Archibald, S., and Bond, W. J. (2003). Growing tall vs growing wide: Tree architecture and allometry of Acacia karoo in forest, savanna, and arid environments. Oikos 102, 3–14. doi:10.1034/j.1600-0706.2003.12181.x
Barbero, M., Bonin, G., Loisel, R., and Quézel, P. (1990). Changes and disturbances of forest ecosystems caused by human activities in the Western part of the Mediterranean Basin. Vegetatio 87, 151–173. doi:10.1007/bf00042952
Benismail, M. C., Bouzit, Z., Zabir, K., and Bouchaouch, S. (2013). “Eléments de conduite des arganiers en culture intensive: La "taille" des arbres,” in Actes du 2ème Congrès International de l’Arganier Agadir 9-11 décembre 2013 (Agadir, Morocco: ANDZOA).
Berthier, P. (1966). Les anciennes sucreries du Maroc et leurs réseaux hydrauliques. Rabat, Morocco: L’Université Mohammed V de Rabat. [dissertation thesis].
Blanco, J., Genin, D., and Carrière, S. M. (2015). The influence of Saharan agro-pastoralism on the structure and dynamics of acacia stands. Agric. Ecosyst. Environ. 213, 21–31. doi:10.1016/j.agee.2015.07.013
Boudy, P., and Chalot, J. P. (1935). Le rôle économique de la forêt marocaine. Bull. Soc. Éco. Maroc. II 8, 98–104.
Buernor, A., Amri, A., Birouk, A., Analy, C., Kehel, Z., and Aitlhaj, A. (2021). Contribution to the identification of morphological descriptors for the genetic diversity of argan tree (Argania spinosa L. Skeels). Glob. J. Ecol. 6 (1), 051–061. doi:10.17352/gje.000044
Chakir, L., Aït Hssaine, A., and Bridgland, D. (2014). Morphogenesis and morphometry of alluvial fans in the high Atlas, Morocco: A geomorphological model of the fans of the wadi beni mhammed, Souss valley. Int. J. Environ. 3 (3), 294–311. doi:10.3126/ije.v3i3.11090
Charrouf, Z., and Guillaume, D. (2009). Sustainable development in northern Africa: The argan forest case. Sustainability 1 (4), 1012–1022. doi:10.3390/su1041012
Charrouf, Z., and Guillaume, D. (2018). The argan oil project: Going from utopia to reality in 20 years. OCL 25 (2), D209. doi:10.1051/ocl/2018006
Chazdon, R. L. (2008). Beyond deforestation: Restoring forests and ecosystem services on degraded lands. Science 320, 1458–1460. doi:10.1126/science.1155365
Culmsee, H. (2005). “An assessment of sustainability from the geobotanical perspective,” in Vegetation and pastoral use in the Western High Atlas Mountains (Morocco)Publications de la Faculté des Lettres et des Sciences Humaines de Rabat. Série: Colloques et Séminairesd. Editors M. Aït Hamza, and H. Popp (Rabat, Morocco: Actes du 7eme Colloque Maroco-Alleman), 67–80.
Culmsee, H. (2004). Vegetation und Weidenutzung im Westlichen Hohen Atlas (Marokko). Eine Nachhaltigkeitsbewertung aus geobotanischer Sicht. Diss. Bot. 389, 1–244.
d'Oleire-Oltmanns, S., Marzolff, I., Peter, K. D., and Ries, J. B. (2012). Unmanned aerial vehicle (UAV) for monitoring soil erosion in Morocco. Remote Sens. 4, 3390–3416. doi:10.3390/rs4113390
Davis, D. K. (2005). Potential forests: Degradation narratives, science, and environmental policy in protectorate Morocco, 1912-1956. Environ. Hist. 10 (2), 211–238. doi:10.1093/envhis/10.2.211
Dendoncker, M., Brandt, M., Rasmussen, K., Taugourdeau, S., Fensholt, R., Tucker, C. J., et al. (2020). 50 years of woody vegetation changes in the Ferlo (Senegal) assessed by high-resolution imagery and field surveys. Reg. Environ. Change 20, 137. doi:10.1007/s10113-020-01724-4
Defaa, C., Elantry, S., El Alami, S.L., Achour, A., El Mousadik, A., and Msanda, F. (2015). Effects of Tree Shelters on the Survival and Growth of Argania spinosa Seedlings in Mediterranean Arid Environment. International Journal of Ecology 1, 6. doi:10.1155/2015/124075
Díaz-Barradas, M. C., Zunzunegui, M., Ain-Lhout, F., Jáuregui, J., Boutaleb, S., Álvarez-Cansino, L., et al. (2010). Seasonal physiological responses of Argania spinosa tree from Mediterranean to semi-arid climate. Plant Soil 337, 217–231. doi:10.1007/s11104-010-0518-8
El Alaoui, N. (1999). Paysages, usages et voyages d’Argania spinosa (L.) Skeels (IXe-Xe siècles). jatba. 41 (2), 45–79. doi:10.3406/jatba.1999.3711
FAO (2011). “Assessing forest degradation. Towards the development of globally applicable guidelines,” in Forest resources assessment (Rome: FAO).
Faouzi, H. (2013). L’exploitation du bois-énergie dans les arganeraies: Entre soutenabilité et degradation (région des haha, haut-atlas Occidental, maroc). com. 262, 155–182. doi:10.4000/com.6832
Fornara, D. A., and Du Toit, J. T. (2007). Browsing lawns? Responses of Acacia nigrescens to ungulate browsing in an african savanna. Ecology 88 (1), 200–209. doi:10.1890/0012-9658(2007)88[200:BLROAN]2.0.CO;2
Gao, Y., Skutsch, M., Paneque-Gálvez, J., and Ghilardi, A. (2020). Remote sensing of forest degradation: A review. Environ. Res. Lett. 15, 103001. doi:10.1088/1748-9326/abaad7
Gharby, S., and Charrouf, Z. (2022). Argan oil: Chemical composition, extraction process, and quality control. Front. Nutr. 8, 804587. doi:10.3389/fnut.2021.804587
Gobbi, B., van Rompaey, A., Loto, D., Gasparri, I., and Vanacker, V. (2020). Comparing forest structural attributes derived from UAV-based point clouds with conventional forest inventories in the Dry Chaco. Remote Sens. 12, 4005. doi:10.3390/rs12234005
Hanan, N. P., and Anchang, J. Y. (2020). Satellites could soon map every tree on Earth. Nature 587, 42–43. doi:10.1038/d41586-020-02830-3
Hosier, R. H. (1993). Charcoal production and environmental degradation: Environmental history, selective harvesting, and post-harvest management. Energy Policy 21 (5), 491–509. doi:10.1016/0301-4215(93)90037-g
Hssaisoune, M., Boutaleb, S., Benssaou, M., Bouaakkaz, B., and Bouchaou, L. (2016). in Physical geography, geology, and water resource availability of the souss-massa river BasinThe souss-massa river basin, Morocco. Editors R. Choukr-Allah, R. Ragab, L. Bouchaou, and D. Barceló (Basel, Switzerland: Springer International Publishing), 27–56. doi:10.1007/698_2016_68
Jones, A., Breuning-Madsen, H., Brossard, M., Dampha, A., Deckers, J., Dewitte, O., et al. (2013). Soil Atlas of Africa. Luxembourg: Publications Office of the European Union.
Kirchhoff, M., Engelmann, L., Zimmermann, L. L., Seeger, M., Marzolff, I., Aït Hssaine, A., et al. (2019a). Geomorphodynamics in argan woodlands, South Morocco. Water 11, 2193. doi:10.3390/w11102193
Kirchhoff, M., Peter, K. D., Aït Hssaine, A., and Ries, J. B. (2019b). Land use in the Souss region, South Morocco and its influence on wadi dynamics. Zfg_suppl. 62, 137–160. doi:10.1127/zfg_suppl/2019/0525
Kirchhoff, M., Romes, T., Marzolff, I., Seeger, M., Aït Hssaine, A., and Ries, J. B. (2021). Spatial distribution of argan tree influence on soil properties in southern Morocco. SOIL 7, 511–524. doi:10.5194/soil-7-511-2021
Kiruki, H. M., van der Zanden, E. H., Malek, Ž., and Verburg, P. H. (2017). Land cover change and woodland degradation in a charcoal producing semi-arid area in Kenya. Land Degrad. Dev. 28, 472–481. doi:10.1002/ldr.2545
Kouami, K., Yaovi, N., and Honan, A. (2009). Impact of charcoal production on woody plant species in west Africa: A case study in Togo. Sci. Res. Essays 4 (9), 881–893.
Lavauden, L. (1941). Les forêts de la France. Mémoire couronné par l’Académie des Sciences colonials. jatba. 21 (239-240), 285–365. doi:10.3406/jatba.1941.2133
Le Polain de Waroux, Y. (2013). Environmental degradation and economic development in the argan woodlands of Aoulouz (Morocco). Sécheresse 24, 29–38. doi:10.1684/sec.2013.0373
Le Polain de Waroux, Y., and Lambin, E. F. (2012). Monitoring degradation in arid and semi-arid forests and woodlands: The case of the argan woodlands (Morocco). Appl. Geogr. 32, 777–786. doi:10.1016/j.apgeog.2011.08.005
Lefhaili, A., and Amhajar, M. (2020). Évaluation des ressources forestières mondiales 2020. Rome: FAO.
Lund, H. G. (2009). What is a degraded forest? White paper prepared for FAO. Gainesville, Virginia, USA: Forest Information Services, 39. AvaliableAt: https://www.researchgate.net/publication/280921178_What_is_a_degraded_forest (Accessed 09 13, 2021).
Lybbert, T. J., Aboudrare, A., Chaloud, D., Magnan, N., and Nash, M. (2011). Booming markets for Moroccan argan oil appear to benefit some rural households while threatening the endemic argan forest. Proc. Natl. Acad. Sci. U. S. A. 108 (34), 13963–13968. doi:10.1073/pnas.1106382108
Lybbert, T. J., Magnan, N., and Aboudrare, A. (2010). Household and local forest impacts of Morocco’s argan oil bonanza. Environ. Dev. Econ. 15, 439–464. doi:10.1017/S1355770X10000136
Marzen, M., Kirchhoff, M., Marzolff, I., Aït Hssaine, A., and Ries, J. B. (2020). Relative quantification of wind erosion in argan woodlands in the Souss Basin, Morocco. Earth Surf. Process. Landf. 45, 3808–3823. doi:10.1002/esp.5002
Marzolff, I., Kirchhoff, M., Stephan, R., Seeger, M., Aït Hssaine, A., and Ries, J. B. (2022). Monitoring dryland trees with remote sensing. Part A: Beyond CORONA—historical HEXAGON satellite imagery as a new data source for mapping open-canopy woodlands on the tree level. Front. Environ. Sci. 10, 896702. doi:10.3389/fenvs.2022.896702
Marzolff, I., Stephan, R., Kirchhoff, M., Seeger, M., Aït Hssaine, A., and Ries, J. B. (2020). UAV-based classification of tree-browsing intensity in open woodlands. EGU General Assem. doi:10.5194/egusphere-egu2020-10301
McGregor, H. V., Dupont, L., Stuut, J.-B. W., and Kuhlmann, H. (2009). Vegetation change, goats, and religion: A 2000-year history of land use in southern Morocco. Quat. Sci. Rev. 28, 1434–1448. doi:10.1016/j.quascirev.2009.02.012
Médail, F., and Quézel, P. (1997). Hot-spots analysis for conservation of plant biodiversity in the mediterranean basin. Ann. Mo. Botanical Gard. 84 (1), 112–127. doi:10.2307/2399957
Moncrieff, G. R., Chamaillé-Jammes, S., Higgins, S. I., O’Hara, R. B., and Bond, W. J. (2011). Tree allometries reflect a lifetime of herbivory in an African savanna. Ecology 92 (12), 2310–2315. doi:10.1890/11-0230.1
Moncrieff, G. R., Lehmann, C. E. R., Schnitzler, J., Gambiza, J., Hiernaux, P., Ryan, C. M., et al. (2014). Contrasting architecture of key African and Australian savanna tree taxa drives intercontinental structural divergence. Glob. Ecol. Biogeogr. 23, 1235–1244. doi:10.1111/geb.12205
Monnier, Y. (1965). Les problèmes actuels de l'arganeraie marocaine. Rev. For. Fr. 11, 750–767. doi:10.4267/2042/24697
Morton, J. F., and Voss, G. L. (1987). The argan tree (Argania sideroxylon, sapotaceae), A desert source of edible oil. Econ. Bot. 41 (2), 221–233. doi:10.1007/bf02858970
Msanda, F., El Aboudi, A., and Peltier, J-P. (2005). Biodiversité et biogéographie de l’arganeraie marocaine. Cah. Agric. 14 (4), 357–364.
Ndegwa, G. M., Nehren, U., Grüninger, F., Iiyama, M., and Anhuf, D. (2016). Charcoal production through selective logging leads to degradation of dry woodlands: A case study from mutomo district, Kenya. J. Arid. Land 8 (4), 618–631. doi:10.1007/s40333-016-0124-6
Nouaim, R., Mangin, G., Breuil, M. C., and Chaussod, R. (2002). The argan tree (Argania spinosa) in Morocco: Propagation by seeds, cutting and in-vitro techniques. Agrofor. Syst. 54, 71–81. doi:10.1023/a:1014236025396
Ouassou, A., Amziane, T. H., and Lajouad, L. (2006). “State of natural resources. Degradation in Morocco and plan of action for desertification and drought control,” in Desertification in the mediterranean region: A security issue. Editors W. G. Kepner, J. L. Rubio, D. A. Mouat, and F. Pedrazzini (Netherlands: Springer), 251–268.
Peter, K. D., d’Oleire-Oltmanns, S., Ries, J. B., Marzolff, I., and Aït Hssaine, A. (2014). Soil erosion in gully catchments affected by land-levelling measures in the Souss basin, Morocco, analysed by rainfall simulation and UAV remote sensing data. Catena 113, 24–40. doi:10.1016/j.catena.2013.09.004
Rasmussen, K., Brandt, M., Tong, X., Hiernaux, P., Diouf, A. A., Assouma, M. H., et al. (2018). Does grazing cause land degradation? Evidence from the sandy ferlo in northern Senegal. Land Degrad. Dev. 29, 4337–4347. doi:10.1002/ldr.3170
Ries, J. B. (2005). Soil erosion on abandoned fields in mediterranean Mountains – monitoring of processes and development. J. Mediterr. Ecol. 6 (1), 43–52.
Rueda, C. V., Baldi, G., Gasparri, I., and Jobbágy, E. G. (2015). Charcoal production in the Argentine Dry Chaco: Where, how and who? Energy Sustain. Dev. 27, 46–53. doi:10.1016/j.esd.2015.04.006
Saidi, M. E. M. (1995). Contribution à l’hydrologie profonde et superficielle du bassin du Souss (Maroc). Climatologie, Hydrogéologie, crues et bilans hydrologiques en milieu sub-aride. Paris, France: Université Paris IV, Sorbonne. [dissertation thesis].
Schnabel, S., Gómez Gutiérrez, A., and Lavado Contador, J. F. (2009). “Grazing and soil erosion in dehesas of SW Spain,” in Advances in studies on desertification. Editors A. Romero Diaz, F. Belmonte Serrato, F. Alonso Sarria, and F. Lopez Bermudez (Murcia, Spain: Ediciones de la Universidad de Murcia), 725–728.
Sebbar, B., Moumni, A., Lahrouni, A., Chehbouni, A., Belghazi, T., and Maksoudi, B. (2021). Remotely sensed phenology monitoring and land-cover classification for the localization of the endemic argan tree in the southern-west of Morocco. J. Sustain. For. 1, 15. doi:10.1080/10549811.2021.1897018
Sedano, F., Silva, J. A., Machoco, R., Meque, C. H., Sitoe, A., Ribeiro, N., et al. (2016). The impact of charcoal production on forest degradation: A case study in tete, Mozambique. Environ. Res. Lett. 11, 094020. doi:10.1088/1748-9326/11/9/094020
Seif-Ennasr, M., Zaaboul, R., Hirich, A., Caroletti, G. N., Bouchaou, L., El Abidine El Morjani, Z., et al. (2016). Climate change and adaptive water management measures in Chtouka Aït Baha region (Morocco). Sci. Total Environ. 573, 862–875. doi:10.1016/j.scitotenv.2016.08.170
Sloan, S., Meyfroidt, P., Rudel, T. K., Bongers, F., and Chazdon, R. (2019). The forest transformation: Planted tree cover and regional dynamics of tree gains and losses. Glob. Environ. Change 59, 101988. doi:10.1016/j.gloenvcha.2019.101988
Vásquez-Grandón, A., Donoso, P. J., and Gerding, V. (2018). Forest degradation: When is a forest degraded? Forests 9, 726. doi:10.3390/f9110726
Wojterski, T. W. (1990). Degradation stages of the oak forests in the area of Algiers. Vegetatio 87, 135–143. doi:10.1007/bf00042950
Keywords: open-canopy woodland, tree density, woody cover, plant architecture, forest degradation, argan tree, change mapping, HEXAGON
Citation: Kirchhoff M, Marzolff I, Stephan R, Seeger M, Aït Hssaine A and Ries JB (2022) Monitoring dryland trees with remote sensing. Part B: Combining tree cover and plant architecture data to assess degradation and recovery of Argania spinosa woodlands of South Morocco. Front. Environ. Sci. 10:896703. doi: 10.3389/fenvs.2022.896703
Received: 15 March 2022; Accepted: 21 September 2022;
Published: 07 October 2022.
Edited by:
Niall Patrick Hanan, New Mexico State University, United StatesReviewed by:
Salma Sai Kachout, Institut National de la Recherche Agronomique de Tunisie (INRAT), TunisiaAbdoul Aziz Diouf, Centre de Suivi Ecologique, Senegal
Niall Patrick Hanan, New Mexico State University, United States
Copyright © 2022 Kirchhoff, Marzolff, Stephan, Seeger, Aït Hssaine and Ries. This is an open-access article distributed under the terms of the Creative Commons Attribution License (CC BY). The use, distribution or reproduction in other forums is permitted, provided the original author(s) and the copyright owner(s) are credited and that the original publication in this journal is cited, in accordance with accepted academic practice. No use, distribution or reproduction is permitted which does not comply with these terms.
*Correspondence: Mario Kirchhoff, a2lyY2hob2ZmQHVuaS10cmllci5kZQ==