- 1School of Economics and Management, Beihang University, Beijing, China
- 2International School of Economics and Management, Capital University of Economics and Business, Beijing, China
- 3School of Economics and Management, Beijing University of Chemical Technology, Beijing, China
- 4Zhongsheng Environmental Technology Development Co., Ltd., Xi’an, China
- 5National Research Center for Environmental Analysis and Measurement, Beijing, China
- 6Pony Testing International Group Co., Ltd., Beijing, China
Highly toxic pollutants such as dioxins and metals emitted from municipal solid waste (MSW) incineration have caused a serious not-in-my-backyard social phenomenon. However, relatively few studies have comprehensively analyzed the contaminant characteristics, coexistence relationships and potential health risks of dioxins and metals in soils near MSW incinerator. Therefore, this study monitors 17 dioxin homologues and 13 metals in soils around MSW incinerator to evaluate their contaminant and coexistence characteristics as well as associated potential health risks. The empirical study focused on Hainan Province, a relatively pure area with no heavy industry and low population density in southern China. The contamination characteristics analysis shows that the total International Toxic Equivalence Quantity (I-TEQ) concentrations of dioxins are comparatively lower than the risk control standards and that in previous studies, whereas the concentrations of specific metals (e.g., Ni, Cu, Cd and Cr) are higher than the risk control standards. To explore the coexistence relationships, the statistical analysis is conducted and indicates that Sb, Cd, Zn and Pb metals (extracted as principal components, with a variance contribution rate of 30.89%) and most dioxin homologues (accounting for 82.83% of the total I-TEQ values) are clustered into one group, suggesting that they may have similar distribution characteristics and origins. Furthermore, the health risk analysis of two toxic pollutants reveals that the carcinogenic and non-carcinogenic risks of dioxins and most metals (except for Ni and Cr) are all below the acceptable levels, implying that the studied incinerator posed no obvious health effect to the surrounding residents.
1 Introduction
With the enhancement of living standards, China’s municipal solid waste (MSW) production increased rapidly and continuously from 1980 to 2019, with an average annual growth rate of 5.39% (Bo et al., 2022). Notably, more than 242 million tons (Mt) of MSW have been generated in China in 2019, which is 30 times higher than that in 1949 (NBSC, 2020). Such an ever-increasing quantity of MSW has led to nearly two-thirds of Chinese cities facing the dilemma of “waste siege” (Liu et al., 2013; Li et al., 2015). Under such background, MSW incineration, as a promising MSW treatment method (Kang et al., 2022), has been increasingly promoted in China, due to its advantages of fast volume reduction, heat recovery and simultaneous energy generation (Tian et al., 2012; Fu et al., 2022). During 2002–2019, the number of MSW incineration plants increased from 37 to 390, with an annual growth rate of 14.86%. By the end of 2019, the total amount of MSW incineration has boosted more than 60 times from 2002 to be 121.74 million tons, accounting for 50.29% of the total MSW production in China (NBSC, 2020).
However, MSW incineration also has some weaknesses, for example, its emission of toxic pollutants, especially dioxins (known as dibenzo-p-dioxins and dibenzofurans, PCDD/Fs) and metals, could cause high toxicity accumulation in soil and pose health risks to residents living in the vicinity of MSW incinerator (Han et al., 2016; Li Y. et al., 2019). Actually, existing studies have also found a positive correlation between exposure to these toxic pollutants and development of abnormal endocrine, elevated cancer rates, immunity disorders, and increased risk of diabetes (Wang B. et al., 2019; Hashem et al., 2021). This is because of their enrichment, nonbiodegradability, high-persistency half-life of decades, as well as their inherent toxicity, biogeochemical recycling, and ecological risks (Vassura et al., 2011; Wang et al., 2021). Therefore, it is important to monitor these harmful compounds in the soil around MSW incinerator and estimate their health effects on nearby people.
Under such background, many studies have been performed on the characteristics and potential human health risk assessment of dioxins and metals in soils near MSW incinerator. Most studies are confined to a certain type of toxic pollutant, such as metals (Chabukdhara et al., 2016; Li et al., 2017; Ma et al., 2018) or dioxins (Wang et al., 2013; Meng et al., 2016; Deng et al., 2020b; Van Drooge et al., 2021). However, researches that comprehensively considers different types of toxic pollutants are still lacking. In addition, some important metals, such as Al, have not yet been explored, and few, primarily Fe, Sb, and Co, have been explored in existing studies (Rovira et al., 2018; Li Y. et al., 2019; Zhong et al., 2019). Furthermore, two kinds of health risks are normally considered, including carcinogenic risk (CR) (Li P. et al., 2019) and non-carcinogenic risk (NCR) (Xu et al., 2019). However, studies that thoroughly investigating the potential human health risks of multiple hazardous substances (considering both dioxins and metals) in the soil near MSW incinerator are still limited. Therefore, this paper attempts to fill such literature gaps by thoroughly investigating 17 dioxin homologues and 13 metals in the surrounding soils of MSW incinerator to quantitatively assess pollutant characteristics and potential health risks.
To accurately evaluate the effects of MSW incineration to the soil contaminations and human health, a relatively pure area, which is located in Hainan Province, is especially introduced as a case study to eliminate the assessment uncertainty. Hainan Province, which is a recognized unpolluted area in southern China, because there has no heavy industry (Guo et al., 2012; Wang A. T. et al., 2019) and a small population (accounting for only 0.67% of the national population) (NBSC, 2019). The major human activity in Hainan Province is ecological tourism, which is highly dependent on the coastal environment and requires high environmental quality (Zhao et al., 2020). Therefore, it is necessary to evaluate the present pollution situation in the Hainan Province, to provide a scientific reference for the standardized management of MSW incineration plants in China.
Generally, the goals of this study are to 1) explore the contamination characteristics and coexistence relationships of two highly toxics pollutants (dioxins and metals) in soils surrounding MSW incinerator and 2) assess the potential health risks of dioxins and metals emitted from MSW incinerator for local residents.
2 Materials and methods
2.1 Sample collection
Figure 1 shows that the studied MSW incinerator (19°18′21″-19°18′38″N and 110°27′12″-110°27′28″E) is located in Qionghai, Hainan Province, which belongs to the tropical monsoon climatic zone. The prevailing winds are northwest in winter and south in summer. The studied MSW incinerator is surrounded by agricultural land and a highway, and there are no other large industrial factories near the MSW incinerator. As the first MSW incinerator in Hainan, it has been operating for approximately 11 years with an annual disposal capacity of 74,900 tons. The combustion system uses an MSW grate incinerator, and the flue gas treatment system contains a lime slurry preparation system, a rotating spray reactor, an activated carbon adsorption unit, a bag filter and a 60-m-high stack for filtering flue gas emission. The operation information of MSW incinerator in this study are described in Supplementary Table S1.
In September of 2019, 7 surface soil samples (S1-S7, see the geographical coordinates of all sampling sites in Supplementary Table S2) were collected from around the MSW incinerator based on the long-term wind direction of Qionghai city, as illustrated in Figure 1. According to the technical specification for soil environmental monitoring, the soil samples were collected at 20 cm depth from the surface (HJ/T 166–2004). Then, a GPS device was applied to record the location of the sampling points (Garmin eTrex201x, United States). For each soil sample, a total of 1 kg of surface soil was evenly collected across an area of 25 m2, and then five aliquots of soil were evenly mixed into one sample. In particular, one of the samples was taken from the center of the sampling area, and the other were taken in four different directions at a distance of 5 m from the center. After removing debris such as plants and gravel, the soil samples were sealed in polyethylene bags and stored in a −20°C refrigerator for analysis.
2.2 Sample treatment and analysis
The analysis method of dioxins in soil followed HJ 77.4–2008 method (MEPC, 2008). First, a 60-mesh sieve was used to screen all soil samples to filter debris such as gravel, rhizomes and other debris. The decontaminated samples were placed in an ASE 300 system and extracted by selective pressurized liquid extraction, and then the extract was added to a separation funnel and transferred to n-hexane, and acidified to remove grease and organic polymers. Second, 200 ml n-hexane was added into acidified dioxins for elution, followed by silica-dispersed carbon column treatment for further fractionation. Finally, the activated carbon column was inverted, the column was rinsed with 50 ml of toluene, and the toluene solution was collected. All elution’s were concentrated to 20 μL and spiked with 13C12-labelled standards (Wellington, Ontario, Canada) as syringe spike. The used solvents during the extraction and purification processes were gained from CNW (Shanghai, China). Moreover, a method blank control experiment was carried out in each batch of samples, to ensure the accuracy of sample detection. In this study, we performed recovery testing using the analytical method used for the recovery of 13C12-labeled internal quantitative standards. The detailed descriptions of aforementioned methods were listed in Supplementary Table S3.
Thirteen metals were selected according to the actual sampling results and the provisions of the relevant standards (MEEC, 2014; MEEC, 2018a). The collected soil samples were analyzed to determine the quantities of the metals according to the GB/T 22,105.1–2008, GB/T 17,141–1997, HJ 491–2019, HJ 680–2013 and EPA 3050B:1996 and EPA 6010D:2018. In particular, the soil samples were dried and screened with a 100-mesh sieve, then placed in a mixed acid solution of HNO3 and HF for microwave digestion for 10 min, and digested at 165°C for another 20 min. In this study, the Inductively Coupled Plasma Spectrometry with Mass Spectrometry method was used to determine the concentration of metals in soil based on Rh as the internal standard. The detailed descriptions of aforementioned methods were listed in Supplementary Table S4.
In terms of quality assurance and quality control (QA/QC) of dioxins measurements, blank experiments, parallel samples, and recoveries of the sampling standards were performed. The QA/QC data results were listed in Supplementary Tables S5, S6. The blank experiment results showed that the target compound was undetectable, which means that there was no interference during the experiment in the laboratory. The parallel double sample determination method was used to ensure the accuracy of sample determination. Specifically, one parallel double sample was taken for every 20 samples. When the number of samples was less than 10, at least one parallel double sample should be measured. The relative standard deviation of the replicate samples was within 30% of the requirement. The sampling recoveries were controlled in the range of 17–185%, which meets the requirements of testing standards. This was used to estimate the HRGC-HRMS detection limits of the congers, which ranged from 0.001 to 0.005 ng/g. For metals measurement, reference standards and blank experiments were used to verify the analytical procedure. The recoveries for national standard samples met the requirements of testing standards. The recovery rates of the national standard soil sample were 98.11–100.00% for Hg, 98.54–100.00% for As, 98.68% for Cr, 100.00–102.63% for Ni, 106.25% for Cu, 98.84–101.16% for Zn, 100.00–106.25% for Cd, 95.00–102.69% for Pb, 99.36–103.21% for Co, 98.45–100.77% for Mn, 92.59–96.29% for Sb, 101.44% for Al and 190.82% for Fe, respectively.
2.3 Contamination assessment
This section presented a set of assessment methods for the contamination of toxic pollutants in soils near MSW incineration plants. For dioxins, the total International Toxic Equivalence Quantity (I-TEQ) was calculated using the toxic equivalence factor to assess its potential impact on the environment. For metals, the single pollution index and Nemerow pollution index method were introduced to quantify the pollution level. These two metal evaluation methods were carried out according to the pairwise comparison between the measured data of soil samples and the data of the control group in the standard value. In particular, the single pollution index was used to quantify the pollution level of a single element in soil, which can be presented as (Wang et al., 2020):
where Pi represents the single pollution index of metal i in soils; Ci and Si represent the measured average and reference values of each metal, respectively. The background values in soil were obtained from the China National Environmental Monitoring Centre (CNEMC, 1990; Liao et al., 2018), as listed in Supplementary Table S7.
According to the single pollution index method, the pollution degree of soil environmental quality was divided into four grades (i.e., Not polluted, slight-degree, moderately polluted and strong-degree), as shown in Supplementary Table S8. However, the comprehensive pollution status in the soil cannot be finely explored through single pollution index. Therefore, the Nemerow comprehensive index (Nci), which reflects the pollution degree of multiple metal elements in soils, was used to comprehensively evaluate the impact of high-content substances on the soil environmental quality (Li H. B. et al., 2014). Accordingly, the formula of Nci is as follows:
where
2.4 Statistical analysis
In this section, two popular statistical methods, i.e., principal component analysis (PCA) and hierarchical cluster analysis (HCA), were introduced to explore the different characteristics and coexistence relationships of metals and dioxins in soils. The data analysis was conducted by RStudio, v.4.0.2. In particular, PCA was used to transform a large number of original datasets into a small number of informative and uncorrelated variables (namely principal components (PCs)) (Tang et al., 2020). In this study, PCA was performed to explore the main metal pollutants originating from MSW incineration.
Let the original data X be a m×n data matrix with m observations on n variables (X1, X2,...,Xn) and let C be a n×n sample covariance matrix of original data X. If
where i = 1,2, … ,n,
PCs were arranged in descending order by their contribution to the variance of the original dataset. To select the first k PCs as representatives of the original set of variables, the contribution of the total variance explained by each PC was calculated. When the cumulative contribution rate (
In addition, an effective clustering method, i.e., HCA, was introduced to perform cluster analysis on the homogeneous groups of metals and dioxins in soil samples. The HCA results were usually presented in a tree diagram, which shows the structure of samples and their relationships. There were two main ways to solve the grouping problem, i.e., agglomerative or divisive approaches. In this study, the divisive approach was introduced, which considers all samples as one cluster, and then conducts recursive splits (Granato et al., 2018). In particular, Ward linkage and Kendall rank correlation were used in this study to measure the correlation between different sample clusters. The Ward linkage can be described as:
where
where
2.5 Health risk assessment
Health risk assessment, based on the USEPA standard model, was used for measuring the carcinogenic and non-carcinogenic risks to the public after chemical exposure (Li J,F. et al., 2018). According to existing studies, three major pollutant exposure routes had been identified: 1) chronic daily intake through direct ingestion (CDIing), 2) chronic daily intake via inhalation (CDIinh), and 3) chronic daily intake by dermal exposure (CDIder) (Li J,F. et al., 2018). For the three exposure routes, the CDIs of the three age groups were calculated as follows (USEPA, 2017):
where CDIing, CDIinh and CDIder represent the chronic daily intake doses by ingestion, inhalation and dermal contact exposure route, respectively (mg∙(kg∙day)−1). Cs refers to the concentration of dioxins and metals detected in soils ((dioxins: mg I-TEQ∙kg−1; heavy metals: mg∙kg−1)). IngR and InhR represent the ingestion rate (mg∙day−1) and air inhalation rate (m3∙day−1) for three age groups, respectively. SA means the surface area of skin contact (m2). AF indicates skin adhesion factor (mg?(cm?day)−1). ABS represents the skin absorption factor, being a fixed constant (0.03). EF (day∙year−1) and ED (year) stand for the annual exposure frequency, exposure duration, respectively. PEF is the particulate matter emission factor (m3 kg−1). AT is lifetime skin exposure time (day). In carcinogen risk calculation, the AT value is set at 25,550 days for children, teenagers and adults. In contrast, in non-carcinogenic risk calculation, the values are 4,015, 2,190 and 19,345 days, respectively. BW represents the weight corresponding to each age group (kg). In this study, the related parameters for health risk assessment of dioxins and metals were presented in Supplementary Tables S10, S11.
The CR of dioxins and metals in soil samples for different population groups was measured using Eq. 10 (Li J,F. et al., 2018):
where
The Hazard Index (HI) was used to evaluate the NCR of dioxins and metals in soil samples, and its calculation formula is:
where
In addition, the Monte Carlo method was introduced to address the uncertainty caused by probabilistic parameters for health risk assessment to avoid overestimation or underestimation after using deterministic parameters (Mesa-Frias et al., 2013). The algorithm of the model was implemented using RStudio software, which selects a value of each parameter variable according to its distribution function at random to calculate the health risk, considering 10,000 iterations.
3 Empirical results
3.1 Characteristics of dioxins concentrations in soils
The total I-TEQ of dioxins is calculated by multiplying the measured concentration of dioxin homologues and the corresponding toxic equivalent factors. Accordingly, the measured concentrations and calculated I-TEQ concentrations of dioxins and their congeners in soil samples are shown in Figure 2 and Table 1. The dioxins concentrations and total I-TEQ values of the studied soil samples vary from 162.20 to 1,544.70 ng kg−1 and 1.10–4.21 ng I-TEQ·kg−1, respectively, with average values being 607.96 ng kg−1 and 2.34 ng I-TEQ·kg−1. Overall, the total I-TEQ values of dioxins are comparatively lower than the risk control standard for soil contamination of development land (10 ng I-TEQ·kg−1 screening values and 100 ng I-TEQ·kg−1 control values for Class I construction land) (GB36600-2018) (MEEC, 2018a; see the specific recommended values in Supplementary Table S13). Furthermore, the variations in dioxins levels at each sampling site can also be obtained from Figure 2. First, the highest I-TEQ value of dioxins is found at sample site S6 (4.21 ng I-TEQ·kg−1), which is located in the downwind direction (affected by main wind blowing from east (E)) of the MSW incinerator. Second, at other downwind sampling sites, the I-TEQ value of dioxins at sampling site S5 (affected by wind blowing both from east (E) and south-southeast (SSE)) is higher than those in S1, S2, S3 and S4. Moreover, the CALPUFF simulating results further confirm that the atmospheric diffusion concentration near sampling sites S5 and S6 are higher than those in other regions (as shown in Supplementary Figure S1). Therefore, these results show that the wind direction can affect the dispersion of pollutants (Ghannam and El-Fadel, 2013; Guo et al., 2020; Ruggeri et al., 2020).
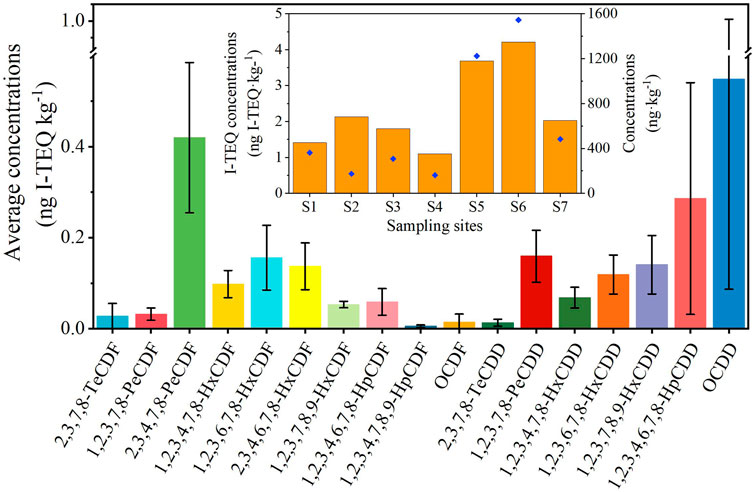
FIGURE 2. I-TEQ Concentrations (unit: ng I-TEQ·kg−1, dry weight) of dioxins congeners in surrounding soils.
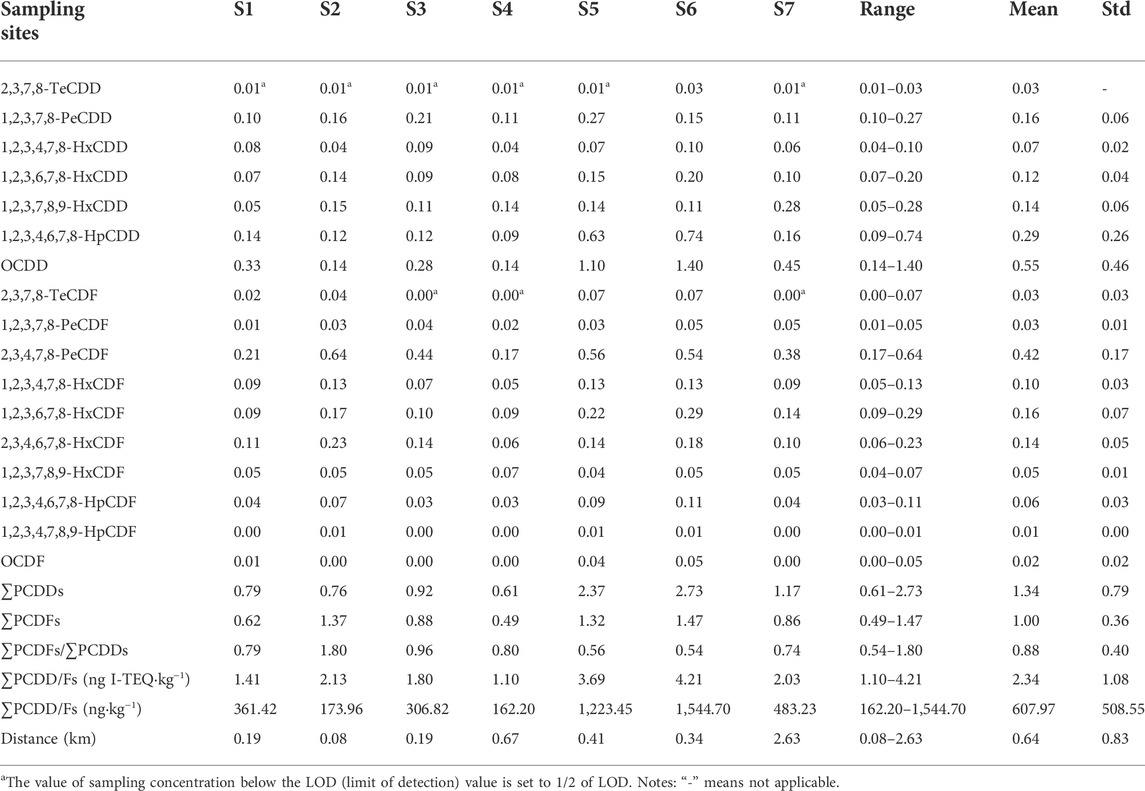
TABLE 1. I-TEQ values (unit: ng I-TEQ·kg−1, dry weight) and concentrations (unit: ng·kg−1, dry weight) of dioxins in the surface soil around the studied MSW incinerator.
Moreover, the proportion of total I-TEQ concentrations of 17 dioxin homologues at each sample is listed in Figure 3. For the I-TEQ contents, the highly chlorinated dioxins, particularly PCDDs are the dominant contributors to the total I-TEQ concentrations in the surrounding soil near MSW incinerator (averagely accounting for 55.00% at each sample), compared with the low-chlorinated dioxins (i.e., PCDFs, 45.00%), which is consistent with existing literature (Xu et al., 2009). In PCDD homologs, OCDD is the top contributor at each sample (with an average of 0.55 I-TEQ·kg−1), accounting for 20.51% of I-TEQ concentration per sample on average. Among PCDF homologs, 2,3,4,7,8-PeCDF is the dominant one (with average of 0.42 I-TEQ·kg−1), and its contribution to the I-TEQ concentration is stable (ranging from 0.17 to 0.64 ng I-TEQ·kg−1 and accounting for 17.96% of I-TEQ concentration per sample on average). In comparison, the concentrations of other PCDF homologs decreased in the order of increase in the levels of chlorination (Pan et al., 2013). Furthermore, the toxic equivalency factor (TEF) of 2,3,4,7,8-PeCDF is 5–500 times higher than those of other homologs.
The ratios of PCDF to PCDD (abbreviated as F/D) concentrations can be used to distinguish the formation of dioxins by the precursor formation (F/D ratio <1) or de novo synthesis (F/D ratio >1) (Everaert and Baeyens, 2002; Pham et al., 2019). In this study, the values of F/D ratio range from 0.54 to 1.80, with the mean value of 0.88. Among the 7 soil samples, there are the 6 samples, whose F/D ratios are lower than 1, implying the prevailing role of precursor formation in these sampling sites. In addition, similar results were found in soils surrounding MSW incinerator in previous research (Li J,F. et al., 2018; Qiu et al., 2020), suggesting the studied MSW incinerator may have limited effects on dioxins in the surrounding soil. In contrast, the F/D ratio of sampling site S2 was over 1 (i.e., 1.80), indicating that the de novo mechanism as the main source of dioxins formation and more PCDFs being formed because of the long-term deposition of pollutants in sampling site S2 near the MSW incinerator.
To better compare the results with available data, the total dioxins I-TEQ concentrations in soils are summarized in Figure 4. For MSW incinerator emission sources (see Figure 4A), the total I-TEQ values in soils near the studied MSW incinerator are close to or smaller than that in most other studies, such as the research focused on Beijing (Li W. et al., 2014), Hangzhou (Jin et al., 2012), Shanghai (Deng et al., 2011), Northern Taiwan (Wang et al., 2008) in China and Moscow Parks (Shelepchikov et al., 2011) in Russia. The potential reason might be that the studied MSW incinerator is located in the suburb of Qionghai (a city of Hainan Province), which has the low level of industrialization, and small number of large-scale industrial plants (Xu et al., 2014; Wu et al., 2016), therefore largely reducing the impact of other anthropogenic emission sources on the pollutant concentrations in soils (Guo et al., 2012). In addition, Hainan Province required the MSW incineration power generation plants to implement the world’s strictest dioxins emission limit (i.e., 0.05 ng TEQ/m3) since August 2018 (DEEHP 2018), which might be one reason for the lower dioxins level in soils around the studied MSW incinerator.
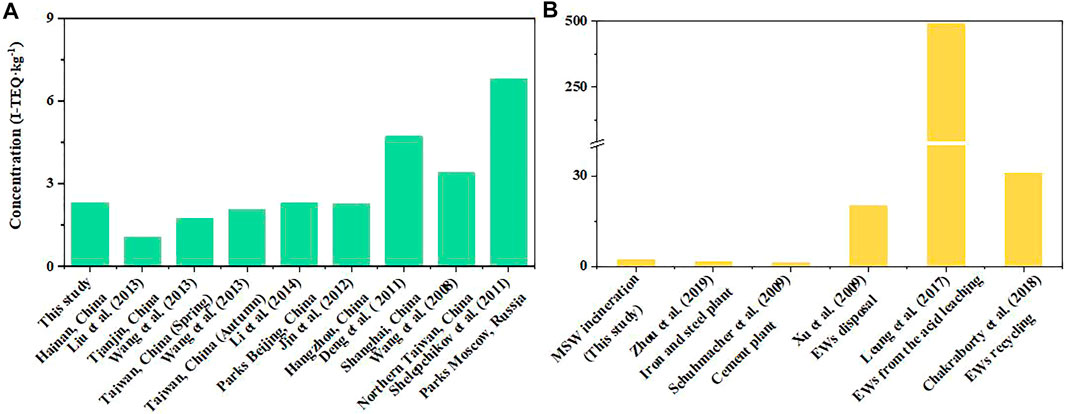
FIGURE 4. Comparative analysis of the literature on the I-TEQ values (unit: ng I-TEQ·kg−1) of PCDD/Fs from MSW incinerator emission source (A) and other emission sources (B).
For other emission sources (see Figure 4B), the value of I-TEQ in the soil around the waste incinerator in this study is significantly lower than the monitoring results in existing studies, such as electronic wastes (EWs) treatment plants (Xu et al., 2013), EWs recycling plants (Chakraborty et al., 2018), and EWs from the acid leaching sites (Leung et al., 2007). The reason might be that compared with the mature treatment equipment of MSW incineration (e.g., flue gas purification systems), the main disposal methods of EWs are more primitive and cruder (e.g., open burning, acid leaching of EWs scrap), which will release excessive toxic substances (including dioxins) into the environment around the EWs disposal areas (Xu et al., 2013; Chakraborty et al., 2018). At the same time, it is very close the value of I-TEQ in soils near the steel and iron plants (Zhou et al., 2019) and cement plants (Schuhmacher et al., 2009). This well confirms that, in most cases, the total I-TEQ concentration of dioxins in soils surrounding of MSW incineration plants is comparable to that of iron and steel industry and cement industry, but is significantly lower than that of emission sources related to the e-waste industry. Furthermore, the WHO-TEQ of dioxins has also been calculated the WHO-TEQ of dioxins in soil for comparison, as described in Supplementary Table S14. The main findings of WHO-TEQ and I-TEQ are similar, but the total WHO-TEQ at each sampling site is 6.2–25.6% lower than the total I-TEQ.
3.2 Characteristics of metals concentrations in soils
The concentrations of thirteen primary metals in soils near MSW incinerator are shown in Table 2. The metal concentrations are ranked as follows: Hg < Cd < Sb < As < Pb < Cu < Co < Ni < Zn < Cr < Mn < Al < Fe. The mean concentrations of Ni, Cu, Cd and Cr exceed the risk control standard for soil contamination of agricultural land by 125.00, 47.42, 33.33 and 6.57%, respectively (GB15618-2018) (MEEC, 2018b). Wang A. T. et al. (2019) also reported high concentrations of Ni, Cu and Cr in the soil, which is consistent with that of the basalt parent material in Hainan (Li J. et al., 2014), as shown in Supplementary Table S15. Moreover, numerous studies found a high concentration of Cd in soils, which largely depends on anthropogenic activities such as rapid urbanization, uncontrolled mining exploration fossil fuel combustion and the application of fertilizers (Wang et al., 2015). For the mean values of the single pollution index (Pi), the study area is strongly polluted by Ni, Co, Cu, and Cd (Pi > 3), moderately polluted by Mn, Cr, Hg and Zn (2 < Pi ≤ 3), slightly polluted by Sb and Pb (1 < Pi ≤ 2) and unpolluted by As (Pi ≤ 1). For the mean values of the Nemerow comprehensive index (Nci), the pollution degrees of most sampling sites (i.e., S1, S2, S3, S4, S5 and S6) belong to the strong level (Nci >3), and only that of sample site S7 is at the moderate pollution level (2 < Nci ≤3). These results indicate that the metal pollution around the studied MSW incinerator is serious, suggesting that these soils are significantly affected by anthropogenic activities.
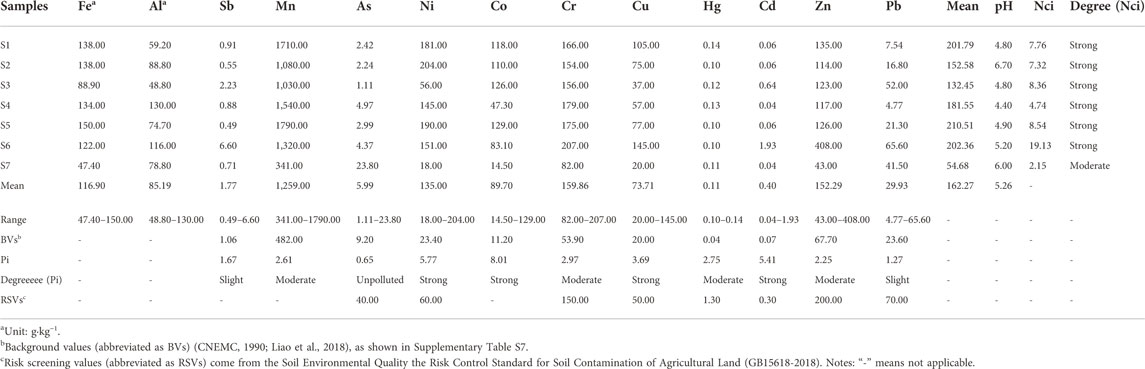
TABLE 2. The concentration (unit: mg.kg−1) and contamination assessment of metals in soils around the MSW incinerator.
To better compare the results with available data, the concentrations of metals around the MSW incinerator are compiled from the existing literature and are listed in Figure 5. Through the result comparisons, two insightful conclusions can be drawn. First, the concentrations of these metals (including As, Cd, Cr, Cu, Hg, Pb, Fe and Zn), are lower than those in existing studies, mainly in Beijing (Han et al., 2016), Guangdong (Li et al., 2017), Zhejiang and Chongqing (Zhong et al., 2019) and London (Rimmer et al., 2006). Possible reason might be that the studied MSW incinerator is located in Qionghai (the industry least developed city), and the soil pollution from other emission sources is quite limited (Guo et al., 2012). Second, the concentrations of Sb, Mn, Ni and Co in this study are higher than those in studies on Zhejiang in China (Zhong et al., 2019), Northern Italy (Capuano et al., 2005) and Catalonia in Spain (Rovira et al., 2014; Rovira et al., 2015; Rovira et al., 2018). These high levels of metals in soils are most likely to be derived from the basaltic parent materials because basalts in Hainan Province had similar high concentrations of Sb (0.11–1.68 mg/kg), Mn (195–1,488 mg/kg), Ni (13.8–208 mg/kg) and Co (0.69–256.6 mg/kg) (Wang A. T. et al., 2019; Bi et al., 2020; Li et al., 2021). In addition, to the best of our knowledge, Al has not yet been studied; therefore, it is excluded from the comparative analysis. Overall, although the mean concentrations of most studied metals are lower than those reported in soils around MSW incinerator, Cu, Cd and Cr are significantly higher than the risk control standard as well as background values.
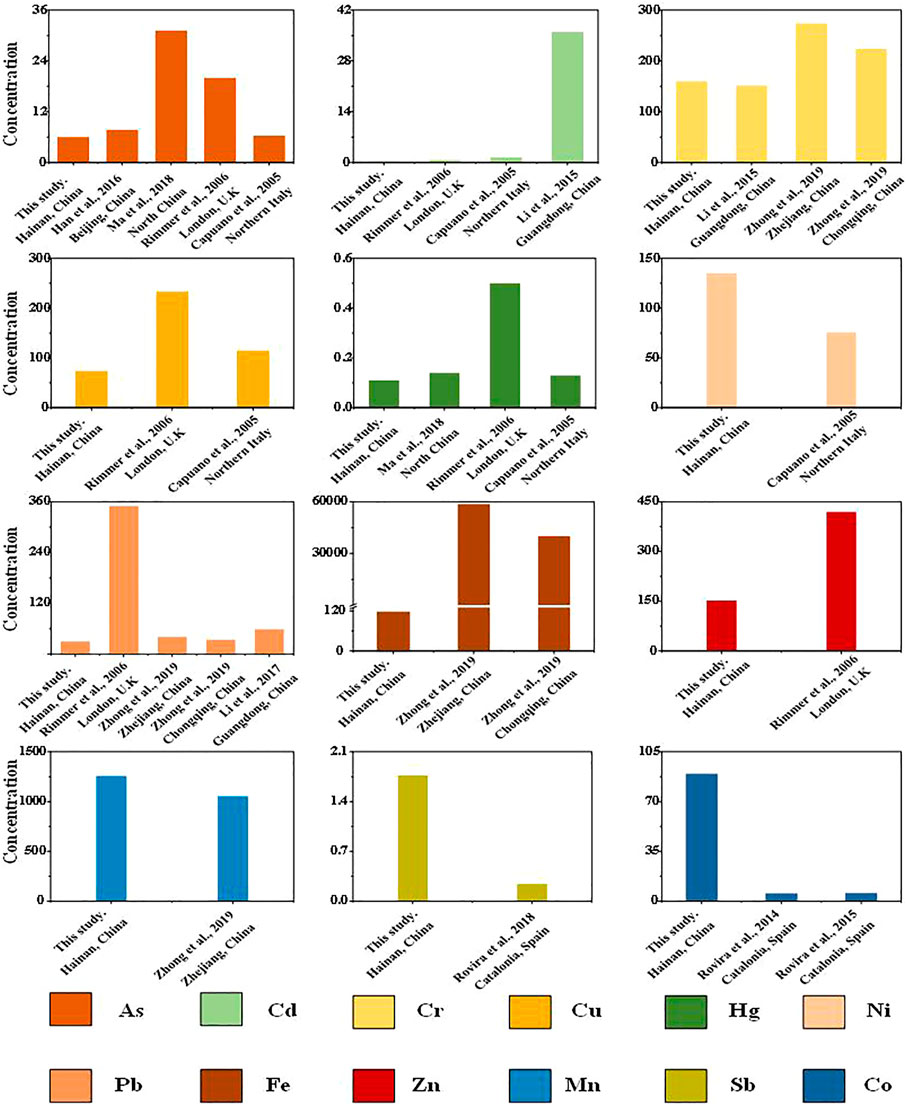
FIGURE 5. Comparative analysis of the literature on concentrations (Fe, unit: g·kg−1; others, unit: mg·kg−1) of metals from MSW incineration.
Similar to the analysis results of dioxins, the effect of wind directions on the distribution of metals can also be obtained from Figure 6. First, it can be seen that Sb, Cr, Cu, Cd, Zn and Pb have the highest concentrations in sampling site S6, which is the downwind direction of the MSW incinerator. Notably, the maximum concentrations of Cd, Pb and Sb in soil samples are 50.78, 13.75 and 13.47 times of the corresponding minimum concentrations, respectively. Second, Al, As and Hg have the highest or second highest concentrations at sampling site S4, which is also in the downwind direction. These results indicate that the soils in the downwind area around the MSW incinerator are vulnerable to the atmospheric deposition of metal-containing pollutants (Rovira et al., 2015).
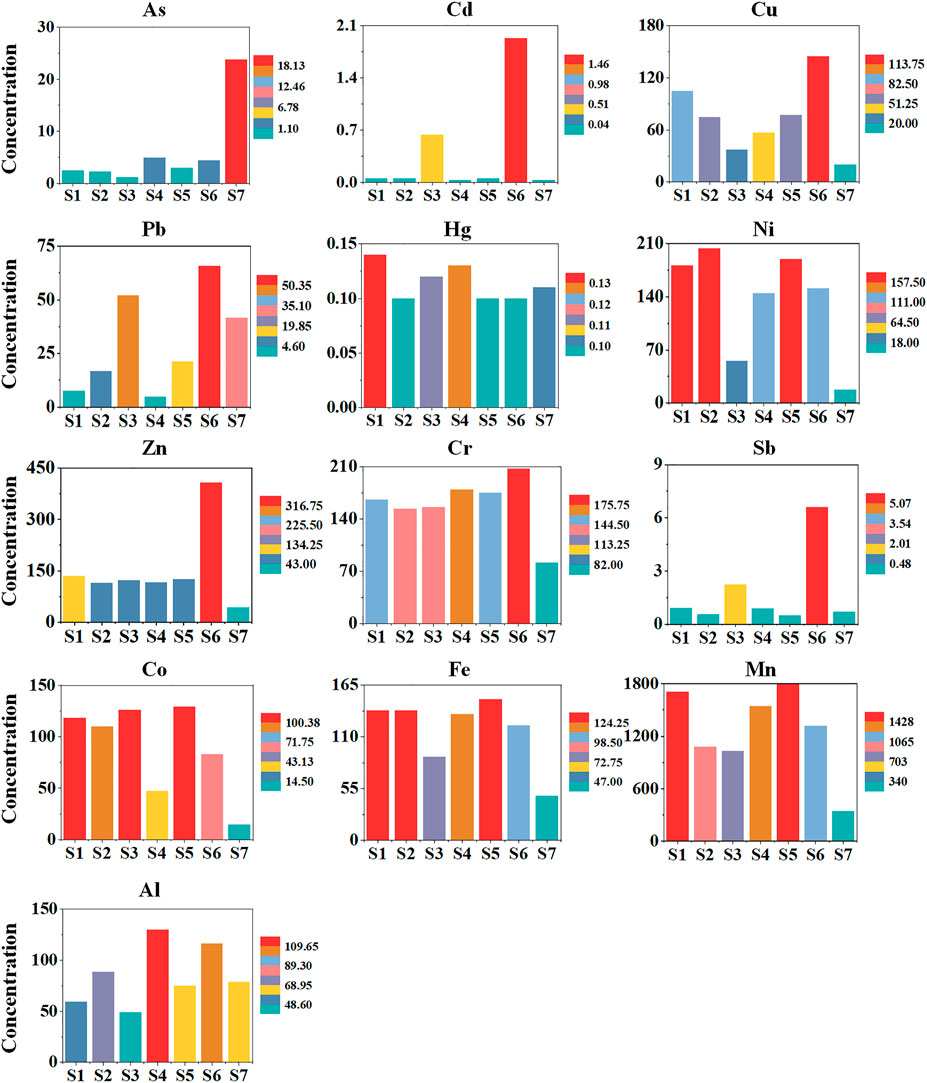
FIGURE 6. Metal concentration distribution at each sampling site (Al and Fe, unit: g·kg−1; others, unit: mg·kg−1; dry weight).
3.3 Source apportionment and relationship analysis
To further explore the source analysis of metals in soils, an effective tool of PCA is conducted, and the analysis results are presented in Supplementary Table S16. According to the variance contribution rate (≥85%) and the gravel chart (eigenvalue>1), three principal components, PC1, PC2 and PC3, can be extracted. The first principal component PC1 mainly includes metal elements such as Fe, Ni, Mn, Cr, Co, and Cu, with the largest variance contribution rate (VCR) of 44.95%. Among these species, most are identified as hyperaccumulators of Ni, Cu, Co and Mn (Chen et al., 2021). Moreover, Fe, Ni, Mn, Cr and Co can be also controlled by soil parent material, soil texture, soil erosion and ground source, and come from natural sources (Jin et al., 2019). The level of Cu in this study soils is also consistent with the composition of soil basalt parent material in Hainan reported in previous studies (Li J. et al., 2014; Wang A. T. et al., 2019). In summary, the metals in PC1 are not susceptible to external environmental influences. In particular, external environmental influences mainly refer to anthropogenic activities, including vehicle exhaust, waste disposal, sewage discharge, industrial emissions, the use of pesticides and fertilizers, livestock manure and atmospheric deposition (Jin et al., 2019).
The prevailing metals in principal component PC2 (with a variance contribution rate of 30.89%) include Sb, Cd, Zn and Pb, which are regarded as anthropogenic emission sources. In particular, it has also been reported in existing literature that Cd and Pb were the particular pollutants emitted by MSW incineration (Bretzel and Calderisi, 2011). The principal components PC3, largely loaded with Al (with a VCR of 12%), is regarded as a natural source. Al is one of the abundant trace elements in soil and is derived from the parent materials (Wang P. et al., 2019). In general, the sources of metals in soils might be associated with complex natural conditions (e.g., soil loading, deposition, and drainage rate) and unnatural anthropogenic emission sources (e.g., waste disposal activities, industrial discharges and automobile exhaust) (Wang et al., 2020).
Furthermore, the correlation analysis is performed on 17 dioxin homologues and 13 metals in soils, and the results are displayed in Figure 7. According to the Pearson correlation coefficient (r), the correlations between dioxins and metals can be divided into three categories. For dioxin homologues, 1,2,3,6,7,8-HxCDD, 1,2,3,6,7,8-HxCDF, 1,2,3,4,6,7,8-HpCDF and OCDF are highly positively correlated with each other (r > 0.82, p < 0.05). For metals, Zn and Pb are both significantly correlated with Sb and Cd (r = 0.56, p < 0.05). For metals and dioxins, As and Pb show highly positive correlations with 1,2,3,7,8,9-HxCDD and 1,2,3,7,8-PeCDF, respectively (r = 0.89, p < 0.05; r = 0.83, p < 0.05; respectively). Existing studies have found that Pb, as a good tracer of combustion processes, mainly comes from anthropogenic emission sources, such as fuel combustion and MSW incineration (Yu et al., 2016; Ma et al., 2018; Li P. et al., 2019). There is a good correlation between metals and dioxin homologues, indicating the coexistence and enrichment of the two substances, which provides a new idea for further identification and monitoring of dioxins in soils.
To further verify the correlation results between dioxins and metals, HCA method is used to classify each pollutant and identified similar groups. Supplementary Figure S2 plots a phylogenetic tree of dioxins and metals, in which the pollutants are grouped into three clusters based on the same emission source. Cluster 1 includes As, Al, Hg, 1,2,3,7,8,9-HxCDD and 1,2,3,7,8,9-HxCDF. It has been reported that As is mainly derived from anthropogenic and industrial activities, such as sewage sludge and industrial discharge (Ma et al., 2018), while Hg is an important pollutant in industrial coal combustion (Duan et al., 2017). Therefore, the species in Cluster 1 might have the same origin, such as anthropogenic and industrial emission sources. Cluster 2 includes Fe, Ni, Mn, Cr, Co, Cu (PC1) and 1,2,3,7,8-PeCDD, which is consistent with the results of principal component analysis. According to the existing studies, the metals in cluster 2 may come from natural sources, such as Ni, Cr and Co (Chabukdhara and Nema, 2013; Tepanosyan et al., 2017; Jin et al., 2019) or may be components of domestic waste, such as Fe and Mn (Peng et al., 2008; Hanc et al., 2011). Therefore, cluster 2 may originate from natural sources or MSW combustion emissions. In addition, the remaining elements are joined together in Cluster 3, including Sb, Cd, Zn and Pb as well as most dioxins homologs (representing 82.83% of the total I-TEQ values). It has been reported that common wastes, such as batteries, paints, colored glass, waste tire, plastic and paper inks, contain abundant metals of Cd, Zn, and Pb (Yu et al., 2016), while MSW incineration is an important source of Sb emissions (Tian et al., 2012; Li P. et al., 2019). This result suggests that the MSW incineration may be one source of dioxins in the soil of the study area. Furthermore, the findings indicate that the coexisting metals and dioxin homologs may have similar distribution characteristics and sources. Therefore, monitoring the specific metals (e.g., Sb, Cd, Zn and Pb) may help describe the distribution of dioxins in soils (Bo et al., 2022) and address the difficulties of traditional dioxin monitoring methods (e.g., high measurement costs and technical requirements) (Peng et al., 2020). However, this finding needs to be further confirmed by laboratory mechanism analysis, comprehensively considering the chemical and biological interactions of pollutants, and the effects of other factors (e.g., soil organic matter content and MSW type) (Zhang et al., 2020; Yuan et al., 2021).
3.4 Human risk assessment
3.4.1 Health risks of dioxins
For carcinogenic risk, the carcinogenic risk (CR) of dioxins in different population groups under three exposure routes are described in Figures 8A–C. For three age groups, the total CR values of dioxins for children, teenagers and adults are 3.76E-07, 9.60E-08 and 3.50E-07, respectively. Carcinogenic risk is significantly higher in children than in other population groups, probably because the ingestion rate and exposure duration of children are significantly higher than teenagers and adults. According to existing literature, the carcinogenic risks of three population groups are less than the acceptable limit of 1.00E-06, implying that no apparent cancer risk developed (USEPA, 2018). For different exposure routes, ingestion is the dominant exposure route of dioxins, representing 78.51, 64.80 and 66.41% of the total carcinogenic risk through all exposure routes for three different population groups, respectively.
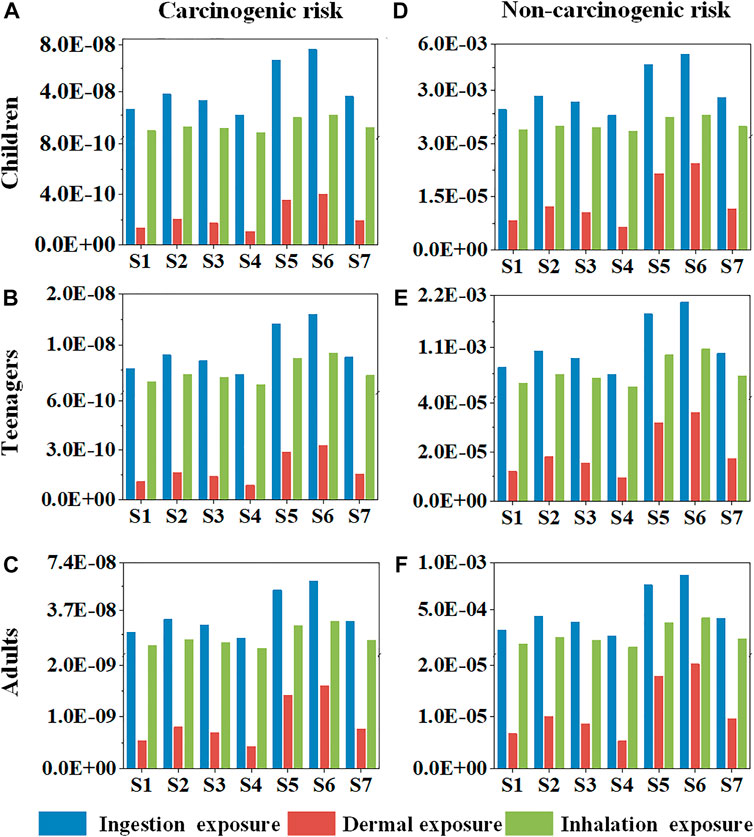
FIGURE 8. Carcinogenic risk (A‐C) and non-carcinogenic risk (D‐F) of dioxins for different population groups under three exposure pathways.
For non-carcinogenic risk, Figures 8D–F shows the HI of dioxins for different population groups under three exposure routes. For different age groups, the total HI values of dioxins are in the order of children (2.63E-02) > teenagers (1.23E-02) > adults (5.06E-03), which is different from the order of CR for three population groups. The reason for the difference is that the exposure time parameters (AT) in CR and NCR calculations for three population groups are different, as shown in Supplementary Table S10. Moreover, the HI values of all sample points are far below 1, indicating that there is no obvious non-carcinogenic risk. For different exposure routes, ingestion has also been shown to be a major contributor to non-carcinogenic risk, representing 78.55, 64.91 and 66.57% of the total HI values via three exposure routes for children, teenagers and adults, respectively.
Generally, these results suggest that dioxins in soils of the study area do not pose carcinogenic and non-carcinogenic risks to nearby populations. Furthermore, the health risk level of dioxins in the study area are close to those areas focused on eastern China (Li J. et al., 2018), and far lower than those in Beijing (Hao et al., 2018) and Shanghai (Deng et al., 2020a) in China and Mataró (Rovira et al., 2015), Campdorà (Rovira et al., 2018) and Barcelona (Domingo et al., 2017) in Spain. A comparison of the results is shown in Supplementary Table S17.
3.4.2 Health risks of metals
For the health risk assessment for metals, the population groups are broadly composed of children, male adults and female adults. Accordingly, the carcinogenic and non-carcinogenic risks to these three age groups exposed to metals through three pathways are shown in Figure 9. For carcinogenic risk, due to the lack of slope factors, some metals (i.e., Fe, Al, Sb, Mn, Cu, Hg and Zn) are not considered in the carcinogenic risk calculation. Regarding different population groups, the total CRs of children, male adults and female adults are 9.51E-03, 2.38E-02 and 2.52E-02, respectively (see Figures 9A–C), which are higher than the acceptable level (CR < 10–4) (Ma et al., 2018). This result indicates that people living around MSW incinerator have much higher carcinogenic risks. Regarding three exposure routes, dermal exposure is the largest contributor to total carcinogenic risk for three population groups (representing approximately 100% of CR). Regarding different metals, Ni and Cr have the highest CR values for the three age groups, exceeding the threshold of acceptable level by one or two orders of magnitude.
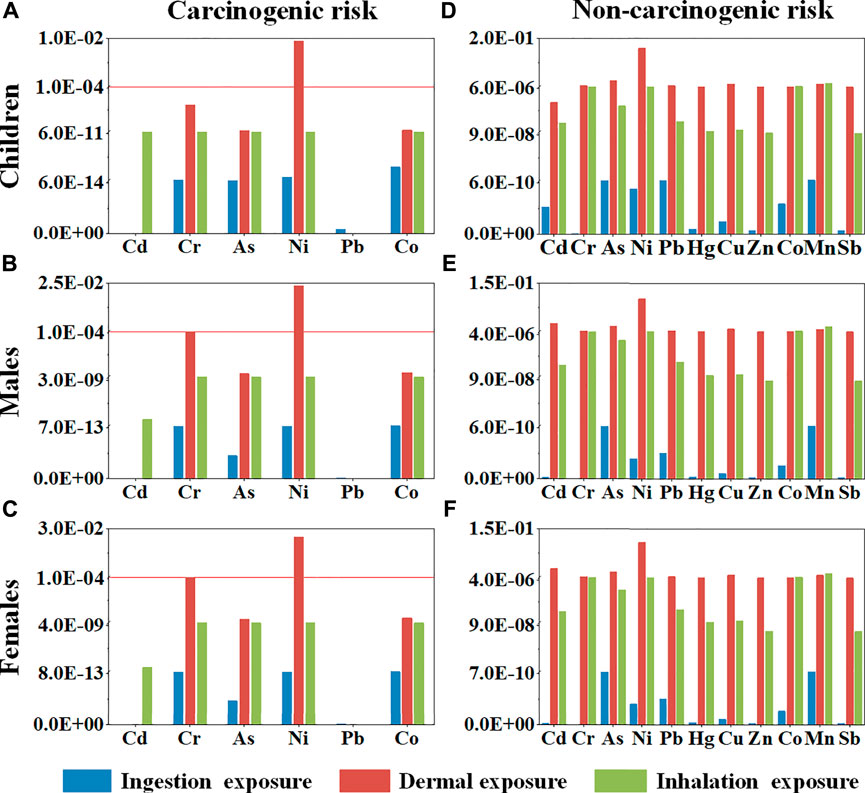
FIGURE 9. Carcinogenic risk (A–C) and non-carcinogenic risk (D–F) of metals for different population groups under three exposure pathways.
For non-carcinogenic risk, the NCR for 11 metals (excluding Fe and Al, without slope factors) are calculated, as shown in Figures 9D–F and the Fe and Al metal slope factors are not mentioned in previous studies. The results reveal that the HI values of metals are far below the acceptable level (HI < 1), which indicates that the metals in soils do not pose significant non-carcinogenic health risks to surrounding population. Regarding different population groups, the total HI values of metals in descending order are as follows: male adults (1.90E-01) < female adults (1.99E-01) < children (2.86E-01). In comparison, children are more susceptible to contaminants of metals, due to their high respiration rate, high hand-mouth activity to the soil and higher absorption of specific toxic metals in gastrointestinal tract (Li Z. et al., 2014). Regarding different exposure routes, dermal exposure is also the largest contributor to population health risks, accounting for 92.88, 89.92 and 91.27% of the total HI values for three population groups, respectively. Regarding different metals, children, male adults and female adults are most vulnerable to the harm of Ni, As and Cd, whose contribution rates are 79.57, 78.02 and 76.88%, respectively.
To further explore the probabilities of exceeding risk, the Monte Carlo simulation method is introduced, and the model performed 10,000 iterations, as the results listed in Table 3. Only As, Ni, Cr and Co exceed the limit of carcinogenic risk, with probability of 0.15, 0.20, 20.66 and 2.55% above the limit, respectively. In addition, only Ni, Cr, Co and Mn exceed the limit of non-carcinogenic risk, with exceeding probabilities of 0.31, 1.43, 4.86 and 24.25%, respectively. Accordingly, the cumulative probabilities of total carcinogenic and non-carcinogenic risks for the three age groups are displayed in Figures 10, 11.
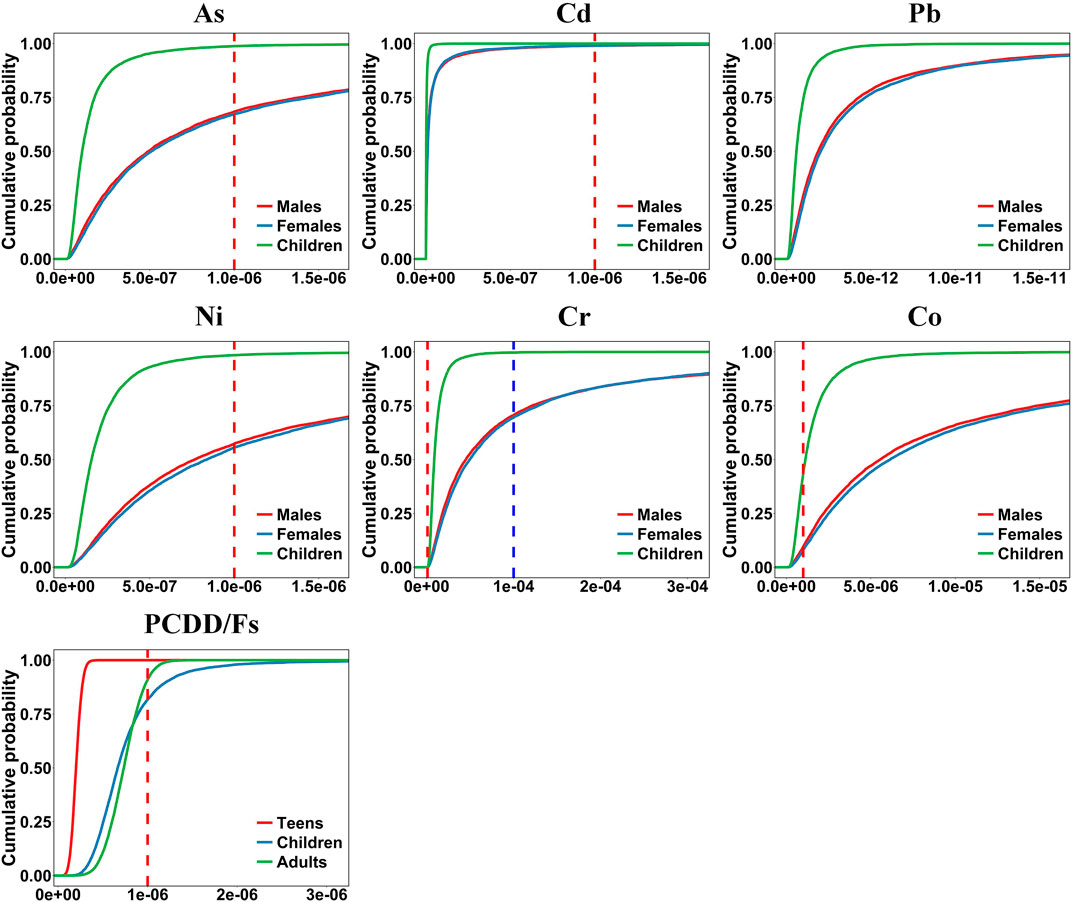
FIGURE 10. Cumulative probabilities of the total carcinogenic risk for different population groups (Note: dotted lines represent acceptable levels).
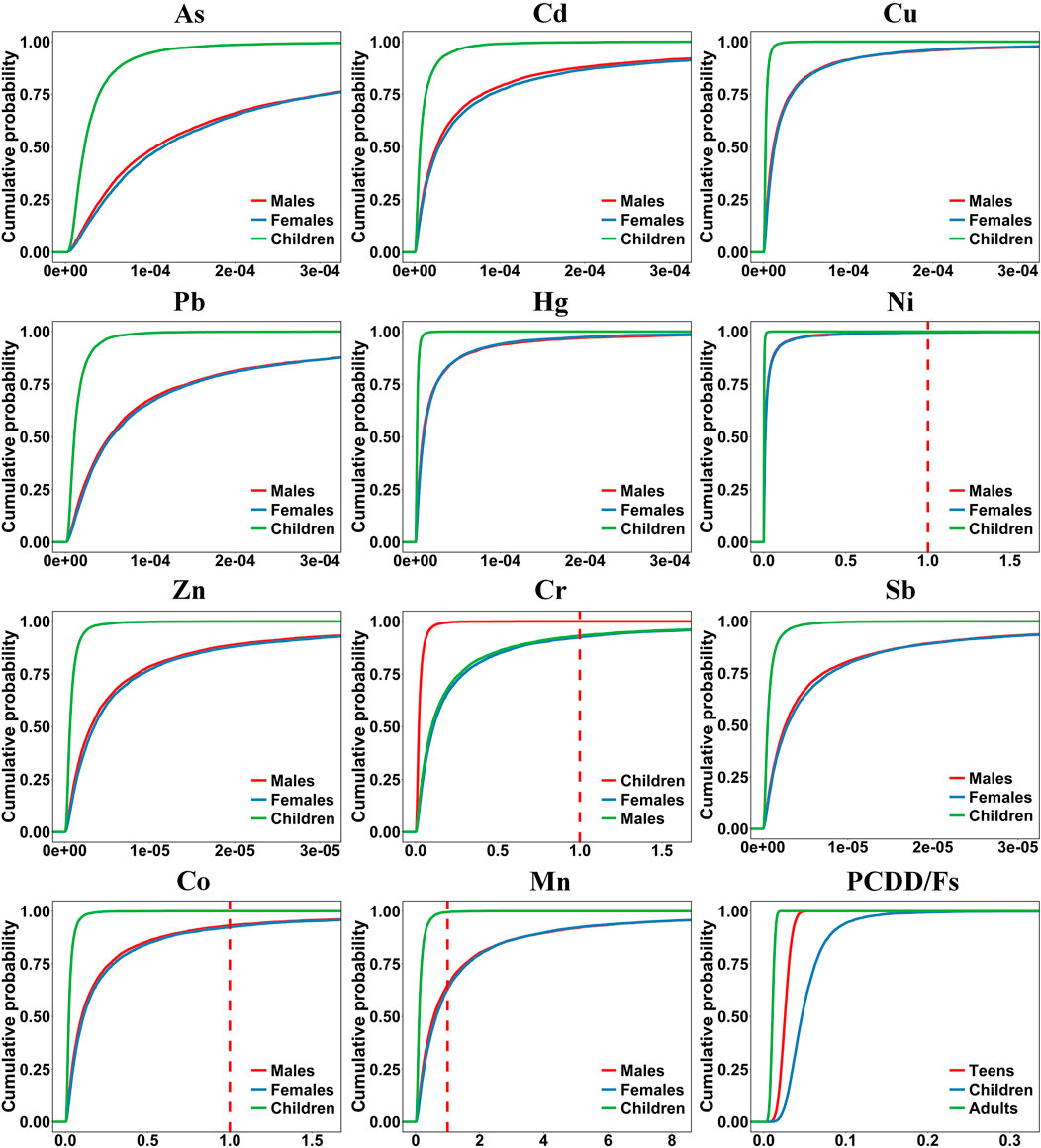
FIGURE 11. Cumulative probabilities of the total non-carcinogenic risk for different population groups (Note: dotted lines represent acceptable levels).
In general, the carcinogenic and non-carcinogenic risks of metals for three population groups are all below the acceptable levels, indicating that metals in soils have weak negative effect on human health. Nevertheless, it is also desirable to pay more attention to minimizing the health risks of metals, particularly for Ni and Cr, as well as through the dermal exposure route.
4 Conclusion
Taking Hainan Province as a case study, this paper comprehensively explores the contamination characteristics, coexistence relationship and health risks of 17 dioxin homologues and 13 metals in soils around the MSW incinerator. For contamination characteristics, the total I-TEQ concentrations of dioxins are comparatively lower than the risk control standards for soil contamination of development land (GB36600-2018) and that in previous studies focusing on Beijing, Shanghai and Hangzhou. Notably, the concentrations of specific metals (e.g., Ni, Cu, Cd and Cr) are higher than the risk control standards for soil contamination of agricultural land (GB15618-2018). For coexistence relationships, the result shows that Sb, Cd, Zn and Pb in soils and most dioxin homologues (accounting for 82.83% of the total I-TEQ values) are clustered into one group (with r > 0.60, p < 0.05, in most cases), implying that they may have similar distribution characteristics and origins. For health risks, the CR and HI of dioxins and most metals (except for Ni and Cr) are all below the acceptable limits, suggesting that there are no significant health risks to surrounding population.
Data availability statement
The original contributions presented in the study are included in the article/Supplementary Material, further inquiries can be directed to the corresponding author.
Author contributions
LT: Conceptualization, Methodology, Writing - Review & Editing. JG: Methodology, Writing - Original draft, Writing - Review & Editing. LL: Data curation, Investigation, Writing - Review & Editing. RW: Visualization, Sample validation. LC: Sample collection, Investigation. AL: Conceptualization, Supervision. YL: Sample testing, Sample validation.
Funding
This work is supported by grants from the National Natural Science Foundation of China (NSFC Nos. 71971007; 72004144).
Conflict of interest
Authors LC and YL are employed by Zhongsheng Environmental Technology Development Co., Ltd. and Pony Testing International Group Co., Ltd., respectively.
The remaining authors declare that the research was conducted in the absence of any commercial or financial relationships that could be construed as a potential conflict of interest.
Publisher’s note
All claims expressed in this article are solely those of the authors and do not necessarily represent those of their affiliated organizations, or those of the publisher, the editors and the reviewers. Any product that may be evaluated in this article, or claim that may be made by its manufacturer, is not guaranteed or endorsed by the publisher.
Supplementary material
The Supplementary Material for this article can be found online at: https://www.frontiersin.org/articles/10.3389/fenvs.2022.898934/full#supplementary-material
References
Bi, X., Zhang, M., Wu, Y., Fu, Z., Sun, G., Shang, L., et al. (2020). Distribution patterns and sources of heavy metals in soils from an industry undeveloped city in Southern China. Ecotoxicol. Environ. Saf. 205, 111115. doi:10.1016/j.ecoenv.2020.111115 | |
Bo, X., Guo, J., Wan, R., Jia, Y., Yang, Z., Lu, Y., et al. (2022). Characteristics, correlations and health risks of PCDD/Fs and heavy metals in surface soil near municipal solid waste incineration plants in Southwest China. Environ. Pollut. 298. doi:10.1016/j.envpol.2022.118816 | |
Bretzel, F. C., and Calderisi, M. (2011). Contribution of a municipal solid waste incinerator to the trace metals in the surrounding soil. Environ. Monit. Assess. 182 (1-4), 523–533. doi:10.1007/s10661-011-1894-0 | |
Capuano, F., Cavalchi, B., Martinelli, G., Pecchini, G., Renna, E., Scaroni, I., et al. (2005). Environmental prospection for PCDD/PCDF, PAH, PCB and heavy metals around the incinerator power plant of Reggio Emilia town (Northern Italy) and surrounding main roads. Chemosphere 58 (11), 1563–1569. doi:10.1016/j.chemosphere.2004.11.065 | |
Chabukdhara, M., Munjal, A., Nema, A. K., Gupta, S. K., and Kaushal, R. K. (2016). Heavy metal contamination in vegetables grown around peri-urban and urban-industrial clusters in Ghaziabad, India. Hum. Ecol. Risk Assess. Int. J. 22 (3), 736–752. doi:10.1080/10807039.2015.1105723 |
Chabukdhara, M., and Nema, A. K. (2013). Heavy metals assessment in urban soil around industrial clusters in ghaziabad, India: Probabilistic health risk approach. Ecotoxicol. Environ. Saf. 87, 57–64. doi:10.1016/j.ecoenv.2012.08.032 | |
Chakraborty, P., Selvaraj, S., Nakamura, M., Prithiviraj, B., Cincinelli, A., and Bang, J. J. (2018). PCBs and PCDD/Fs in soil from informal e-waste recycling sites and open dumpsites in India: Levels, congener profiles and health risk assessment. Sci. Total Environ. 621, 930–938. doi:10.1016/j.scitotenv.2017.11.083 | |
Chen, L., Liu, J., Zhang, W., Zhou, J., Luo, D., and Li, Z. (2021). Uranium (U) source, speciation, uptake, toxicity and bioremediation strategies in soil-plant system: A review. J. Hazard. Mat. 413, 125319. doi:10.1016/j.jhazmat.2021.125319 |
CNEMC (China National Environmental Monitoring Centre) (1990). The background values of elements in Chinese soils. Beijing: China Environmental Science Press.
DEEHP (Department of Ecology and Environment of Hainan Province) (2018). Letter of opinions on implementation standards for pollutant emissions of New and expanded MSW Incineration Power Generation Projects. Availablet: http://hnsthb.hainan.gov.cn/xxgk/0200/0202/hjywgl/hjyxpj/201903/t20190308_2401715.html.
Deng, Y. Y., Jia, L. J., Li, K., Rong, Z. Y., and Yin, H. W. (2011). Levels of PCDD/Fs in agricultural soils near two municipal waste incinerators in Shanghai, China. Bull. Environ. Contam. Toxicol. 86, 65–70. doi:10.1007/s00128-010-0168-9 | |
Deng, Y. Y., Peng, P. A., Jia, L. J., Mao, W. L., Hu, J. F., and Yin, H. W. (2020a). Environmental exposure-associated human health risk of dioxin compounds in the vicinity of a municipal solid waste incinerator in Shanghai, China. Bull. Environ. Contam. Toxicol. 105 (1), 173–179. doi:10.1007/s00128-020-02903-z | |
Deng, Y. Y., Peng, P. A., Jia, L. J., Yin, H. W., Hu, J. F., and Mao, W. L. (2020b). Atmospheric bulk deposition of polychlorinated dibenzo-p-dioxins and dibenzofurans (PCDD/Fs) in the vicinity of MSWI in Shanghai, China. Ecotox. Ecotoxicol. Environ. Saf. 196, 110493. doi:10.1016/j.ecoenv.2020.110493 | |
Domingo, J. L., Rovira, J., Nadal, M., and Schuhmacher, M. (2017). High cancer risks by exposure to PCDD/fs in the neighborhood of an integrated waste management facility. Sci. Total Environ. 607, 63–68. doi:10.1016/j.scitotenv.2017.06.272 | |
Duan, L., Wang, X., Wang, D., Duan, Y., Cheng, N., and Xiu, G. (2017). Atmospheric mercury speciation in Shanghai, China. Sci. Total Environ. 578, 460–468. doi:10.1016/j.scitotenv.2016.10.209 | |
Everaert, K., and Baeyens, J. (2002). The formation and emission of dioxins in large scale thermal processes. Chemosphere 46 (3), 439–448. doi:10.1016/s0045-6535(01)00143-6 | |
Fu, Z., Lin, S., Tian, H., Hao, Y., Wu, B., Liu, S., et al. (2022). A comprehensive emission inventory of hazardous air pollutants from municipal solid waste incineration in China. Sci. Total Environ. 826, 154212. doi:10.1016/j.scitotenv.2022.154212 | |
Ghannam, K., and El-Fadel, M. (2013). Emissions characterization and regulatory compliance at an industrial complex: An integrated MM5/CALPUFF approach. Atmos. Environ. X. 69, 156–169. doi:10.1016/j.atmosenv.2012.12.022 |
Granato, D., Santos, J. S., Escher, G. B., Ferreira, B. L., and Maggio, R. M. (2018). Use of principal component analysis (PCA) and hierarchical cluster analysis (HCA) for multivariate association between bioactive compounds and functional properties in foods: A critical perspective. Trends Food Sci. Technol. 72, 83–90. doi:10.1016/j.tifs.2017.12.006 |
Guo, D., Wang, R., and Zhao, P. (2020). Spatial distribution and source contributions of PM2.5 concentrations in Jincheng, China. Atmos. Pollut. Res. 11 (8), 1281–1289. doi:10.1016/j.apr.2020.05.004 |
Guo, Y., Fu, Y., Yan, S., Yang, Y., Guo, Y., and Bao, Z. (2012). Heavy metal distribution between parent soil and pepper in an unpolluted area, Hainan Island, China. Environ. Earth Sci. 66 (4), 1083–1089. doi:10.1007/s12665-011-1314-3 |
Hao, Y., Li, Y., Wang, T., Hu, Y., Sun, H., Matsiko, J., et al. (2018). Distribution, seasonal variation and inhalation risks of polychlorinated dibenzo-p-dioxins and dibenzofurans, polychlorinated biphenyls and polybrominated diphenyl ethers in the atmosphere of Beijing, China. Environ. Geochem. Health 40 (5), 1907–1918. doi:10.1007/s10653-017-9961-2 | |
Han, Y., Xie, H., Liu, W., Li, H., Wang, M., Chen, X., et al. (2016). Assessment of pollution of potentially harmful elements in soils surrounding a municipal solid waste incinerator, China. Front. Environ. Sci. Eng. 10 (6), 7–17. doi:10.1007/s11783-016-0873-7 |
Hanc, A., Novak, P., Dvorak, M., Habart, J., and Svehla, P. (2011). Composition and parameters of household bio-waste in four seasons. Waste Manag. 31 (7), 1450–1460. doi:10.1016/j.wasman.2011.02.016 | |
Hashem, M., Hasan, M., Nayan, A. H., Payel, S., Hasan, M., and Sahen, M. (2021). The environmental impacts of heavy metals in soil, certain plants and wastewater near industrial area of Brahmanbaria, Bangladesh. Environ. Monit. Assess. 193 (10), 1–11. doi:10.1007/s10661-021-09497-x | |
Jin, Y., O'Connor, D., Ok, Y. S., Tsang, D. C., Liu, A., and Hou, D. (2019). Assessment of sources of heavy metals in soil and dust at children’s playgrounds in Beijing using GIS and multivariate statistical analysis. Environ. Int. 124, 320–328. doi:10.1016/j.envint.2019.01.024 | |
Jin, Y. Q., Liu, H. M., Li, X. D., Ma, X. J., Lu, S. Y., Chen, T., et al. (2012). Health risk assessment of PCDD/F emissions from municipal solid waste incinerators (MSWIs) in China. Environ. Technol. 33 (22-24), 2539–2545. doi:10.1080/09593330.2012.696714 | |
Kang, Y., Yang, Q., Wang, L., Chen, Y., Lin, G., Huang, J., et al. (2022). China's changing city-level greenhouse gas emissions from municipal solid waste treatment and driving factors. Resour., Conserv. Recycl. 180Spatial distribution of polybrominated diphenyl ethers and polychlorinated dibenzo-p-dioxins and dibenzofurans in soil and combusted residue at Guiyu, an electronic waste recycling site in southeast China. Environ. Sci. Technol. 41 (8), 1061682730–1061682737. doi:10.1021/es0625935 |
Li, T., Cai, G., Zhang, M., Li, S., and Nie, X. (2021). The response of benthic foraminifera to heavy metals and grain sizes: A case study from hainan island, China. Mar. Pollut. Bull. 167, 112328. doi:10.1016/j.marpolbul.2021.112328 | |
Li, H. B., Tang, Z. H., Deng, Q. J., and Wang, J. (2014a). The comprehensive environmental quality evaluation and pollution analysis about the north area of Guangdong. Appl. Mech. Mat. 507, 798–805. doi:10.4028/www.scientific.net/amm.507.798 |
Li, J, F., Zhang, Y., Sun, T., Hao, H., Wu, H., Wang, L., et al. (2018a). The health risk levels of different age groups of residents living in the vicinity of municipal solid waste incinerator posed by PCDD/Fs in atmosphere and soil. Sci. Total Environ. 631, 81–91. doi:10.1016/j.scitotenv.2018.03.009 | |
Li, J., Yuan, G. L., Li, P., Duan, X. C., Yu, H. H., Qiu, J. L., et al. (2018b). Insight into the local source of polybrominated diphenyl ethers in the developing Tibetan Plateau: The composition and transport around the Lhasa landfill. Environ. Pollut. 237, 1–9. doi:10.1016/j.envpol.2018.02.029 | |
Li, J., Zhang, G., and Gong, Z. (2014b). Mobilization and redistribution of elements in soils developed from extreme weathering basalt on Hainan Island. Chin. J. Geochem. 33 (3), 262–271. doi:10.1007/s11631-014-0686-y |
Li, N., Kang, Y., Pan, W., Zeng, L., Zhang, Q., and Luo, J. (2015). Concentration and transportation of heavy metals in vegetables and risk assessment of human exposure to bioaccessible heavy metals in soil near a waste-incinerator site, South China. Sci. Total Environ. 521, 144–151. doi:10.1016/j.scitotenv.2015.03.081 | |
Li, P., Wang, X., Zou, X., Yu, Z., Li, J., Yang, Y., et al. (2019b). Metallic contamination in soils around a municipal solid waste incineration site: A case study in northeast China. Environ. Sci. Pollut. Res. 26 (25), 26339–26350. doi:10.1007/s11356-019-05763-1 | |
Li, T., Wan, Y., Ben, Y., Fan, S., and Hu, J. (2017). Relative importance of different exposure routes of heavy metals for humans living near a municipal solid waste incinerator. Environ. Pollut. 226, 385–393. doi:10.1016/j.envpol.2017.04.002 | |
Li, W., Li, C., Chen, Z., Ding, N., and Cai, Z. (2014c). Levels of polychlorinated dibenzo-p-dioxins and dibenzofurans in mountainous and park soils in Beijing, China. Int. J. Environ. Anal. Chem. 94 (7), 691–711. doi:10.1080/03067319.2014.891106 |
Li, Y., Zhang, H., Shao, L., Zhou, X., and He, P. (2019a). Impact of municipal solid waste incineration on heavy metals in the surrounding soils by multivariate analysis and lead isotope analysis. J. Environ. Sci. 82, 47–56. doi:10.1016/j.jes.2019.02.020 | |
Li, Z., Ma, Z., van der Kuijp, T. J., Yuan, Z., and Huang, L. (2014d). A review of soil heavy metal pollution from mines in China: Pollution and health risk assessment. Sci. Total Environ. 468, 843–853. doi:10.1016/j.scitotenv.2013.08.090 | |
Liao, X., Zhang, C., Sun, G., Li, Z., Shang, L., Fu, Y., et al. (2018). Assessment of metalloid and metal contamination in soils from Hainan, China. Int. J. Environ. Res. Public Health 15 (3), 454. doi:10.3390/ijerph15030454 | |
Liu, W., Li, H., Tian, Z., Xie, H., and Hu, J. (2013). Spatial distribution of polychlorinated biphenyls in soil around a municipal solid waste incinerator. J. Environ. Sci. 25 (8), 1636–1642. doi:10.1016/s1001-0742(12)60234-4 | |
Ma, W., Tai, L., Qiao, Z., Zhong, L., Wang, Z., Fu, K., et al. (2018). Contamination source apportionment and health risk assessment of heavy metals in soil around municipal solid waste incinerator: A case study in north China. Sci. Total Environ. 631, 348–357. doi:10.1016/j.scitotenv.2018.03.011 | |
MEEC (Ministry of Ecology and Environment of China) (2018b). Soil environmental quality risk control standard for soil contamination of agricultural land. GB15618-2018. (Beijing: China Statistics Press). (in Chinese).
MEEC (Ministry of Ecology and Environment of China) (2018a). Soil environmental quality risk control standard for soil contamination of development land. GB36600-2018. (Beijing: China Statistics Press). (in Chinese).
MEEC (Ministry of Ecology and Environment of China) (2014). Standard for pollution control on the municipal solid waste incineration. GB 18485–2014. (Beijing: China Statistics Press). (in Chinese).
Meng, B., Ma, W. L., Liu, L. Y., Zhu, N. Z., Song, W. W., Lo, C. Y., et al. (2016). PCDD/Fs in soil and air and their possible sources in the vicinity of municipal solid waste incinerators in northeastern China. Atmos. Pollut. Res. 7 (2), 355–362. doi:10.1016/j.apr.2015.10.014 |
MEPC (Ministry of Environmental Protection of the People’s Republic of China) (2008). National environmental protection standard of the people’s Republic of China. HJ77.4-2008. Soil and sediment determination of polychlorinated dibenzo-p-dioxin (PCDDs) and polychlorinated dibenzofurans (PCDFs) isotope dilution HRGC-HRMS.
Mesa-Frias, M., Chalabi, Z., Vanni, T., and Foss, A. M. (2013). Uncertainty in environmental health impact assessment: Quantitative methods and perspectives. Int. J. Environ. Health Res. 23 (1), 16–30. doi:10.1080/09603123.2012.678002 | |
NBSC (National Bureau of Statistics of China) (2019). China statistical yearbook. Beijing: China Statistical Publishing House.
NBSC (National Bureau of Statistics of China) (2020). China statistical yearbook. Beijing, China: China Statistics Press.
Pan, Y., Yang, L., Zhou, J., Liu, J., Qian, G., Ohtsuka, N., et al. (2013). Characteristics of dioxins content in fly ash from municipal solid waste incinerators in China. Chemosphere 92 (7), 765–771. doi:10.1016/j.chemosphere.2013.04.003 | |
Peng, C. H., Bai, B. S., and Chen, Y. F. (2008). Study on the preparation of Mn-Zn soft magnetic ferrite powders from waste Zn–Mn dry batteries. Waste Manag. 28 (2), 326–332. doi:10.1016/j.wasman.2007.03.020 | |
Peng, Y., Lu, S., Li, X., Yan, J., and Cen, K. (2020). Formation, measurement, and control of dioxins from the incineration of municipal solid wastes: Recent advances and perspectives. Energy fuels. 34 (11), 13247–13267. doi:10.1021/acs.energyfuels.0c02446 |
Pham, M. T. N., Anh, H. Q., Nghiem, X. T., Tu, B. M., Dao, T. N., and Nguyen, M. H. (2019). Characterization of PCDD/fs and dioxin-like PCBs in flue gas from thermal industrial processes in vietnam: A comprehensive investigation on emission profiles and levels. Chemosphere 225, 238–246. doi:10.1016/j.chemosphere.2019.03.024 | |
Qiu, J., Tang, M., Peng, Y., Lu, S., Li, X., and Yan, J. (2020). Characteristics of PCDD/Fs in flue gas from MSWIs and HWIs: Emission levels, profiles and environmental influence. Aerosol Air Qual. Res. 20 (10), 2085–2097. doi:10.4209/aaqr.2019.11.0610 |
Rimmer, D. L., Vizard, C. G., Pless-Mulloli, T., Singleton, I., Air, V. S., and Keatinge, Z. A. (2006). Metal contamination of urban soils in the vicinity of a municipal waste incinerator: One source among many. Sci. Total Environ. 356 (1-3), 207–216. doi:10.1016/j.scitotenv.2005.04.037 | |
Rovira, J., Nadal, M., Schuhmacher, M., and Domingo, J. L. (2018). Concentrations of trace elements and PCDD/Fs around a municipal solid waste incinerator in Girona (Catalonia, Spain). Human health risks for the population living in the neighborhood. Sci. Total Environ. 630, 34–45. doi:10.1016/j.scitotenv.2018.02.175 | |
Rovira, J., Nadal, M., Schuhmacher, M., and Domingo, J. L. (2014). Environmental levels of PCDD/Fs and metals around a cement plant in Catalonia, Spain, before and after alternative fuel implementation. Assessment of human health risks. Sci. Total Environ. 485, 121–129. doi:10.1016/j.scitotenv.2014.03.061 | |
Rovira, J., Vilavert, L., Nadal, M., Schuhmacher, M., and Domingo, J. L. (2015). Temporal trends in the levels of metals, PCDD/Fs and PCBs in the vicinity of a municipal solid waste incinerator. Preliminary assessment of human health risks. Waste Manag. 43, 168–175. doi:10.1016/j.wasman.2015.05.039 | |
Ruggeri, M. F., Lana, N. B., Altamirano, J. C., and Puliafito, S. E. (2020). Spatial distribution, patterns and source contributions of POPs in the atmosphere of Great Mendoza using the WRF/CALMET/CALPUFF modelling system. Emerg. Contam. 6, 103–113. doi:10.1016/j.emcon.2020.02.002 |
Schuhmacher, M., Nadal, M., and Domingo, J. L. (2009). Environmental monitoring of PCDD/Fs and metals in the vicinity of a cement plant after using sewage sludge as a secondary fuel. Chemosphere 74, 1502–1508. doi:10.1016/j.chemosphere.2008.11.055 | |
Shelepchikov, A. A., Brodskii, E. S., Feshin, D. B., Zhil'Nikov, V. G., Mir-Kadyrova, E. Y., and Balashova, S. P. (2011). Polychlorinated dibenzo-p-dioxins, dibenzofurans, and biphenyls in soils of Moscow. Eurasian Soil Sc. 44, 286–296. doi:10.1134/s1064229311030124 |
Tang, L., Zhang, C., Li, L., and Wang, S. (2020). A multi-scale method for forecasting oil price with multi-factor search engine data. Appl. Energy 257, 114033. doi:10.1016/j.apenergy.2019.114033 |
Tepanosyan, G., Maghakyan, N., Sahakyan, L., and Saghatelyan, A. (2017). Heavy metals pollution levels and children health risk assessment of Yerevan kindergartens soils. Ecotoxicol. Environ. Saf. 142, 257–265. doi:10.1016/j.ecoenv.2017.04.013 | |
Tian, H., Gao, J., Lu, L., Zhao, D., Cheng, K., and Qiu, P. (2012). Temporal trends and spatial variation characteristics of hazardous air pollutant emission inventory from municipal solid waste incineration in China. Environ. Sci. Technol. 46 (18), 10364–10371. doi:10.1021/es302343s | |
USEPA (2017). Mid atlantic risk assessment. Regional screening level (RSL) summary table. United States Environmental Protection Agency.
USEPA (2018). Office of air quality planning and standards, research triangle park, North Carolina technical support document EPA’s 2014 national air toxics assessment, 2014 NATA TSD. United States Environmental Protection Agency.
Van Drooge, B. L., Abalos, M., Abad, E., Adrados, M. A., Gomez, A., Gallés, P., et al. (2021). Qualitative and quantitative changes in traffic and waste incineration PCDD/Fs in urban air and soils under different seasonal conditions (Metropolitan Area of Barcelona). Sci. Total Environ. 753, 142149. doi:10.1016/j.scitotenv.2020.142149 | |
Vassura, I., Passarini, F., Ferroni, L., Bernardi, E., and Morselli, L. (2011). PCDD/Fs atmospheric deposition fluxes and soil contamination close to a municipal solid waste incinerator. Chemosphere 83 (10), 1366–1373. doi:10.1016/j.chemosphere.2011.02.072 | |
Wang, X., Dan, Z., Cui, X., Zhang, R., Zhou, S., Wenga, T., et al. (2020). Contamination, ecological and health risks of trace elements in soil of landfill and geothermal sites in Tibet. Sci. Total Environ. 715, 136639. doi:10.1016/j.scitotenv.2020.136639 | |
Wang, A. T., Wang, Q., Li, J., Yuan, G. L., Albanese, S., and Petrik, A. (2019a). Geo-statistical and multivariate analyses of potentially toxic elements’ distribution in the soil of hainan island (China): A comparison between the topsoil and subsoil at a regional scale. J. Geochem. Explor. 197, 48–59. doi:10.1016/j.gexplo.2018.11.008 |
Wang, B., Wang, P., Xie, L. H., Lin, R. B., Lv, J., Li, J. R., et al. (2019b). A stable zirconium based metal-organic framework for specific recognition of representative polychlorinated dibenzo-p-dioxin molecules. Nat. Commun. 10 (1), 3861–3868. doi:10.1038/s41467-019-11912-4 | |
Wang, D., Dang, Z., Feng, H., and Wang, R. (2015). Distribution of anthropogenic cadmium and arsenic in arable land soils of Hainan, China. Toxicol. Environ. Chem. 97 (3-4), 402–408. doi:10.1080/02772248.2015.1050193 |
Wang, H., Fan, Z., Kuang, Z., Yuan, Y., Liu, H., and Huang, H. (2021). Heavy metals in marine surface sediments of Daya Bay, Southern China: Spatial distribution, sources apportionment, and Ecological risk Assessment. Front. Environ. Sci. 9, 559. doi:10.3389/fenvs.2021.755873 |
Wang, J. B., Wang, M. S., Wu, E. M. Y., Chang-Chien, G. P., and Lai, Y. C. (2008). Approaches adopted to assess environmental impacts of PCDD/F emissions from a municipal solid waste incinerator. J. Hazard. Mat. 152 (3), 968–975. doi:10.1016/j.jhazmat.2007.07.090 | |
Wang, P., Li, Z., Liu, J., Bi, X., Ning, Y., Yang, S., et al. (2019c). Apportionment of sources of heavy metals to agricultural soils using isotope fingerprints and multivariate statistical analyses. Environ. Pollut. 249, 208–216. doi:10.1016/j.envpol.2019.03.034 | |
Wang, Y. H., Rao, G. W., Chen, M. L., Yu, J. Y., and Chang-Chien, G. P. (2013). Investigation on dioxin level of flue gas, ambient air, vegetation and soil nearby large-scale MSWI in Taiwan. Adv. Mat. Res. 781-784, 2508–2516. doi:10.4028/www.scientific.net/amr.781-784.2508 |
Wu, Q., Zhou, H., Tam, N. F., Tian, Y., Tan, Y., Zhou, S., et al. (2016). Contamination, toxicity and speciation of heavy metals in an industrialized urban river: Implications for the dispersal of heavy metals. Mar. Pollut. Bull. 104 (1-2), 153–161. doi:10.1016/j.marpolbul.2016.01.043 | |
Xu, M. X., Yan, J. H., Lu, S. Y., Li, X. D., Chen, T., Ni, M. J., et al. (2009). Agricultural soil monitoring of PCDD/Fs in the vicinity of a municipal solid waste incinerator in Eastern China: Temporal variations and possible sources. J. Hazard. Mat. 166 (2-3), 628–634. doi:10.1016/j.jhazmat.2008.11.072 | |
Xu, P., Chen, Z., Wu, L., Chen, Y., Xu, D., Shen, H., et al. (2019). Health risk of childhood exposure to PCDD/Fs emitted from a municipal waste incinerator in Zhejiang, China. Sci. Total Environ. 689, 937–944. doi:10.1016/j.scitotenv.2019.06.425 | |
Xu, P., Tao, B., Li, N., Qi, L., Ren, Y., Zhou, Z., et al. (2013). Levels, profiles and source identification of PCDD/Fs in farmland soils of Guiyu, China. Chemosphere 91, 824–831. doi:10.1016/j.chemosphere.2013.01.068 | |
Xu, X., Zhao, Y., Zhao, X., Wang, Y., and Deng, W. (2014). Sources of heavy metal pollution in agricultural soils of a rapidly industrializing area in the Yangtze Delta of China. Ecotoxicol. Environ. Saf. 108, 161–167. doi:10.1016/j.ecoenv.2014.07.001 | |
Yu, J., Sun, L., Wang, B., Qiao, Y., Xiang, J., Hu, S., et al. (2016). Study on the behavior of heavy metals during thermal treatment of municipal solid waste (MSW) components. Environ. Sci. Pollut. Res. 23 (1), 253–265. doi:10.1007/s11356-015-5644-7 | |
Yuan, Q., Sallach, J. B., Rhodes, G., Bach, A., Crawford, R., Li, H., et al. (2021). Natural organic matter does not diminish the mammalian bioavailability of 2, 3, 7, 8-tetrachlorodibenzo-p-dioxin. Chemosphere 264, 128420. doi:10.1016/j.chemosphere.2020.128420 | |
Zhang, J., Zhang, S., and Liu, B. (2020). Degradation technologies and mechanisms of dioxins in municipal solid waste incineration fly ash: A review. J. Clean. Prod. 250, 119507. doi:10.1016/j.jclepro.2019.119507 |
Zhao, L., Liu, J., Cai, G., Huang, L., and Luo, W. (2020). Distribution, source, and pollution assessment of heavy metals in Sanya offshore area, south Hainan Island of China. Mar. Pollut. Bull. 160, 111561. doi:10.1016/j.marpolbul.2020.111561 | |
Zhong, S., Wei, Z., Zhang, L., Lei, C., Gao, H., Feng, J., et al. (2019). Spatial distribution of heavy metals in soil around municipal solid waste incinerators at coastal plain and inland mountainous region in China. IOP Conf. Ser. Earth Environ. Sci. 358 (2), 022032. doi:10.1088/1755-1315/358/2/022032 |
Keywords: contaminant characteristics, coexistence relationships, toxic pollutants, municipal solid waste incineration, health risks, surface soil
Citation: Tang L, Guo J, Li L, Wan R, Cui L, Liu A and Lu Y (2022) Contamination characteristics, coexistence relationships and health risk assessment of dioxins and metals in topsoil around municipal solid waste incinerator in Hainan, China. Front. Environ. Sci. 10:898934. doi: 10.3389/fenvs.2022.898934
Received: 18 March 2022; Accepted: 22 August 2022;
Published: 15 September 2022.
Edited by:
Juying Li, Shenzhen University, ChinaReviewed by:
Wei Chen, China University of Geosciences Wuhan, ChinaPaula S. Tourinho, Masaryk University, Czechia
Meihua Deng, Zhejiang Academy of Agricultural Sciences, China
Xiaoqing Lin, Zhejiang University, China
Copyright © 2022 Tang, Guo, Li, Wan, Cui, Liu and Lu. This is an open-access article distributed under the terms of the Creative Commons Attribution License (CC BY). The use, distribution or reproduction in other forums is permitted, provided the original author(s) and the copyright owner(s) are credited and that the original publication in this journal is cited, in accordance with accepted academic practice. No use, distribution or reproduction is permitted which does not comply with these terms.
*Correspondence: Ling Li, bGluZ2xpQGN1ZWIuZWR1LmNu