- 1Institute of Marine Biotechnology (IMB), Universiti Malaysia Terengganu (UMT), Kuala Terengganu, Malaysia
- 2Climate Change Adaptation Laboratory, Institute of Marine Biotechnology (IMB), Universiti Malaysia Terengganu (UMT), Kuala Terengganu, Malaysia
- 3Faculty of Business Management, Universiti Teknologi MARA (UiTM), Pahang, Malaysia
- 4Institute for Biodiversity and Sustainable Development, Universiti Teknologi MARA (UiTM), Shah Alam, Malaysia
- 5Faculty of Science and Marine Environment, Universiti Malaysia Terengganu, Kuala Terengganu, Malaysia
- 6Higher Institution Centre of Excellence (HICoE), Institute of Tropical Aquaculture and Fisheries (AKUATROP), Universiti Malaysia Terengganu, Kuala Terengganu, Malaysia
Climate change research on major aquatic species assists various stakeholders (e.g. policymakers, farmers, funders) in better managing its aquaculture activities and productivity for future food sustainability. However, there has been little research on the impact of climate change on aquatic production, particularly in terms of scientometric analyses. Thus, using the bibliometric and scientometric analysis methods, this study was carried out to determine what research exists on the impact of climate change on aquatic production groups. We focused on finfish, crustaceans, and molluscs. Data retrieved from Web of Science was mapped with CiteSpace and used to assess the trends and current status of research topics on climate change associated with worldwide aquatic production. We identified ocean acidification as an important research topic for managing the future production of aquatic species. We also provided a comprehensive perspective and delineated the need for: i) more international collaboration for research activity focusing on climate change and aquatic production in order to achieve the United Nations Sustainable Development Goal by 2030; ii) the incorporation of work from molecular biology, economics, and sustainability.
1 Introduction
The process of climate change is being accelerated due to human activities negatively impacting the environment (e.g., burning fossil fuels, land clearing, and agricultural activities releasing greenhouse gases to the atmosphere), and is currently leading to an overall increase in global temperature. As the ocean absorbs the majority of excess heat (e.g., Sun energy/solar radiation) from this warming, it acts as a buffer for terrestrial life. Consequently, this leads to serious impacts on ocean ecology. This issue has been highlighted in the United Nations Sustainable Development Goals (SDG), where goal 13 states we must “take urgent action to combat climate change and its impacts” (UN SDG, 2021). One aspect that requires urgent action is combating the effects of climate change on oceanic productivity (Steeves et al., 2018; Thakur et al., 2018; Sainz et al., 2019; Heasman et al., 2020; Maulu et al., 2021). This includes many marine fisheries, as decreases in productivity of marine capture, particularly in tropical regions such as Africa and Asia (Cheung et al., 2010; Pauly and Cheung, 2018; Oremus et al., 2020), are increasingly common.
Since 2010, the Food and Agriculture Organization has collected data on the main species produced in global aquaculture, specifically three different groups of finfish, crustaceans, and molluscs (FAO). Previously, the world’s major species production were divided into six different groups including: freshwater fishes, diadromous fishes, marine fishes, crustaceans, molluscs, and other aquatic animals not elsewhere included (FAO, 2011). Aquatic algae were excluded from the list of aquatic species produced because they were regarded as a non-animal item. These lists are managed by The State of World Fisheries and Aquaculture (SOFIA) (FAO, 2021), which aims to provide comprehensive, objective, and global views on fisheries and aquaculture related data to policy-makers, civil society, and those whose livelihoods depend on aquatic resources. The top two major species produced in the world for finfish, crustaceans and molluscs are, respectively: i) grass carp, Ctenopharyngodon idellus and ii) silver carp, Hypophthalmichthys molitrix; i) Whiteleg shrimp, Penaeus vannamei and ii) red swamp crawfish, Procambarus clarkii; and i) cupped oysters nei, Crassostrea spp. and ii) Japanese carpet shell, Ruditapes philippinarum (FAO, 2020). These six species have seen important increases in annual production since 2010, where their total production accounted for almost half of the global production of all 466 managed species in 2018 (47.8%). The importance of managing aquatic production responsibly is thus widely prioritized and recognized. As climate change influences the aquatic environment, understanding these impacts on major species production is truly needed.
Various authors have conducted studies on the impact of climate change on aquaculture activities, mostly in review-based studies (Callaway et al., 2012; Rosa et al., 2012; Froehlich et al., 2018). However, research on the impact of climate change on aquaculture production specifically is limited, especially in terms of scientometric-based analysis. As a result, there is an urgent need to assess the current literature surrounding the impacts of climate change on major aquatic production to improve management and risk assessment of aquaculture activities and its future productivity.
In this study, bibliometric and scientometric analyses were combined to analyze global trends of climate change research regarding the top globally produced finfish, crustacean, and mollusc species. Scientometric study usually refers to the analysis of analyzing and measuring currents patterns of available scientific literature (Azra et al., 2021). Nalimov and Mulcjenko (1971) were first to define scientometrics as “the quantitative methods of research on the development of science as an informational process”. Scientometrics is also known for its ability to synthesis quantitative literatures systematically and comprehensively. Recently, it has also become an essential tool for assessing a researcher’s research and development, collaboration, and academic quality. Various tools can be used to determine relevant subject areas such as VOSviewer, CiteSpace, etc. (Zhou and Song, 2021). To our knowledge, the present article is the first report detailing scientometric analysis on climate change impacts on major aquaculture species produced worldwide. We are specifically interested in the following questions:
RQ1. What are the overall publication trends in terms of publication output?
RQ2. Where is this research area situated on the map of Web of Sciences database?
RQ3. Who are the dominant knowledge carriers in these areas?
RQ4. What are the dominant topics/clusters, and what is their temporal evolution?
RQ5. What are the impactful publications and keywords for these areas?
Answering these research questions will help inform the current body of research and identify important research gaps regarding the impacts of climate change on aquatically produced species.
2 Methods
Scientometric analysis is a date and information visualization analysis tool that can explore important changes along with the development trends of a research domain (Chen and Song, 2019). This analysis has been regarded as an important tool to summarize historical research achievements and reveal what direction future research trends should take. The details for the research framework used in this study is shown in Figure 1.
2.1 Data Source
We used Web of Science (WOS) databases to conduct a literature search for the scientometric analysis. We chose to focus on WOS instead of other databases such as PubMed, Google Scholar, or Scopus, due to the efficiency with which WOS can extract bibliographic data from search results (Azra et al., 2022). According to Meho and Rogers (2008), WOS indexed publications have the highest data quality of literature and the most authenticated information when compared to other database platforms. Furthermore, the result for visual analysis coding using the WOS database is more compatible with the CiteSpace software used for data analysis in this study (Chen 2004). The WOS searches were based on the “topic” (TS) field, which includes article titles, abstracts, keywords, and “KeyWords Plus” (automatically generated terms pulled from the titles of cited articles).
2.2 Article Search
A robust set of search keywords are key for quality literature syntheses (Azzeri et al., 2020). A good search keyword can retrieve the most relevant influential studies. Search keyword formulation begins by applying the PECo method for formulating research objectives/questions, where “P” stands for Population, “E” stands for Exposure and “Co.” for context.
The primary goal of our scientometric analysis was to examine recent trends in research regarding the effects of climate change on the top aquatic production groups, namely finfish, crustaceans, and molluscs. Our “Population” keywords focused on Aquatic Species Production, specifically for animals; keywords on Climate Change were our “Exposure” terms. No “Context” component was included as this criterion is discussed in the result analysis. The search was conducted on 5 September 2021. The search strings are presented below.
2.2.1 Population Keywords—Selection of Species
Species’ common names were based on the FAO catalogue published in their respective website or referred to on their “Cultured Aquatic Species Information Programmeˮ (Supplementary Appendix S1A). For molluscs, we chose species based on the available fact sheet provided by the FAO, particularly the Cupped oysters nei, Crassostrea spp. According to FAO terminology, the “nei'' is defined as “not elsewhere included,” which is when the species cannot be identified, and more than one species is included in the same group. However, we chose the species based on the FAO Cultured Aquatic Species Information Programme or Introduced Species Fact Sheets in which the species was listed as a cultured species for the genus Crassostrea. For each of the three groups (finfish, crustaceans, molluscs), we list the top two major species produced globally and their associated references in Supplementary Appendix S1A. To identify articles based on these six species, the following key terms were used:
TS= ((“grass carp”) OR (“white amur”) OR (“Ctenopharyngodon idellus”) OR (“silver carp”) OR (“Hypophthalmichthys molitrix”) OR (“whiteleg shrimp”) OR (“white shrimp”) OR (“penaeus vannamei”) OR (“litopenaeus vannamei”) OR (“red swamp crawfish”) OR (“Procambarus clarkii”) OR (“pacific cupped oyster”) OR (“Crassostrea gigas”) OR (“american cupped oyster”) OR (“Crassostrea virginica”) OR (“mangrove cupped oyster”) OR (“crassostrea rhizophorae”) OR (“japanese carpet shell”) OR (“ruditapes philippinarum”))
2.2.2 Exposure Keywords
Keywords associated with our topic “Climate Change” included:
TS=[(climat*) OR (“global warm*”) OR (“seasonal* variat*”) OR (“extrem* event*”) OR (“environment* variab*”) OR (“anthropogenic effect*”) OR (“multiple stres*”) OR (“greenhouse effect*”) OR (“sea level ris*”) OR (erosio*) OR (“agricult* runoff”) OR (“weather* variab*”) OR (“weather* extrem*”) OR (“extreme* climat*”) OR (“environment* impact*”) OR (“environment* chang*”) OR (“anthropogenic stres*”) OR (“temperature ris*”) OR (“temperature effect*”) OR (“warm* ocean”) OR (“sea surface* temperat*”) OR (heatwav*) OR (acidific*) OR (hurrican*) OR (el-nino) OR (“el nino”) OR (“la nina”) OR (la-nina) OR (drought*) OR (flood*) OR (“high precipit*”) OR (“heavy rainfall*”) OR (“CO2 concentrat*”) OR (“melt* of the glacier*”) OR (“melt* ice*”)]
2.3 Eligibility Criteria
2.3.1 Inclusion Criteria
Articles retrieved from WOS searches were included in our subsequent analyses only if they were published in peer-reviewed journals and were written in English.
2.3.2 Exclusion Criteria
We excluded a study if it was not considered original research, if it was published in a journal that did not conduct peer-review, or if it was not written in English. Such studies may have included proceedings papers, reviews, book reviews, abstracts, editorial materials, letters, or news.
2.4 Data Analysis
To address our first research question, we used Excel to visualize descriptive analyses for the number of publications, published journals, authors, universities/institutes, and countries/regions where the authors were affiliated with at time of publication. Then, to answer research questions two through five, we used four visualization analyses in CiteSpace Software. Section 2.4.1 describes CiteSpace techniques and tools in detail. Table 1 summarizes the techniques and tools used to answer the research questions.
2.4.1 Scientometric Analysis
CiteSpace V version 5.2. R 2.3.26.2018 for 64-bit windows was used for visualization and knowledge graph analysis because it allows for the creation of multiple bibliometric networks and the application of multiple methods of analysis (Chen, 2004; Chen and Leydesdorff, 2014). The threshold setting was set at “Top 50 N″ per slice, which allowed the selection of most cited items from each slice to form a network based on the choice of input value and multiple node types. Consequently, the top 50 most cited articles were displayed and ranked accordingly by CiteSpace. The “Time Slicing” was set to 1981–2021 and “Years per slice” at 1 year. To prune the generated network, the “Pruning” parameter was chosen. All term sources in Web of Science, including title, abstract, author keywords, and keywords plus, were chosen for text processing.
2.4.1.1 Dual Map Overlay
We used dual-map overlay to investigate the inter-domain specialty to specialty trends that connect research areas. The citation link trajectory reveals multi-disciplinary relationships. A change in trajectory from one to another indicates that a paper from one discipline was influenced by other articles from another discipline. Ovals represent the number of authors who have published in the field and the number of relevant papers that have been published. The width of the oval represents the author-to-author ratio, while the height of the oval represents the number of papers published. The retrieved literature was divided into two categories: 1) cited journals and 2) citing journals (i.e., the latter cited references from the former) (Chen and Leydesdorff, 2014).
2.4.1.2 Co-Citation Analysis
Co-citation analysis is used to determine the status of scientific development and changes in scientific structure. The co-citation analysis generates a science map with nodes, connections, and density values to show the main structure of selected variables (for this study: i) Author, ii) Journal, iii) Countries, iv) Institutions, and v) Articles) to obtain the cluster of co-citing variables, where a co-citation instance occurs when two sources are cited together in one paper (Chen 2004; Chen and Leydesdorff 2014; Aryadoust and Ang 2019).
A variable’s quality was assessed using degree, centrality, and sigma (Chen et al., 2009; Chen et al., 2010). The degree value represents the number of citations a variable receives from another variable of the same type (e.g., the number of citations one author receives from another author), with a higher degree indicating more citations. Centrality is a measure of influence that shows the degree to which the same variables are close to each other, where variables with high centrality have a greater influence on the network because they connect more variables and thus more information and paths pass through them. Sigma is the sum of the centrality and burstness scores (described below), ranging from 0 to 1 where the highest value is associated with high value research articles (e.g. available raw data, scientifically analyzed data, etc.) (Chen et al. 2009; Chen et al., 2010).
2.4.1.3 Document Cluster Analysis
Based on the documents retrieved, multidimensional clustering was used to identify research clusters in focus areas. Because log-likelihood ratio (LLR) could provide the best results in terms of uniqueness and coverage, it was used to automatically extract the cluster label. “Timeline view” and “cluster view” were in the Document Cluster Analysis was used to visualize the network’s shape and form. The “timeline view” displayed a vertical range of chronological time periods from left to right, whereas the “cluster view” displayed a spatial network of colour-coded and automatically labeled representations in a landscape format (Chen 2004; Chen and Leydesdorff 2014; Aryadoust and Ang 2019).
The modularity Q index, average silhouette metric, and centrality metric were used to assess the quality and homogeneity of the document cluster analysis, as well as the detected clusters (Chen et al., 2009; Chen et al., 2010). The modularity Q index ranges between 0 and 1, with larger index indicating higher reliability. The average silhouette metric has a value between −1 and 1, where values greater than 0 indicate greater homogeneity. Centrality is a measure of influence that shows the degree to which publications or journals stand between each other, where publications with high centrality have higher influence on the network as they connect more publications or journals and therefore more information and paths pass through them.
2.4.1.4 Burstness Analysis
Citation burstness and sigma, both temporal metrics, were used to identify influential publications and top keywords. Burst detection is defined as a sudden increase in the number of citations for a specific article, or “an abrupt elevation of the frequencies [of citations] over a specific time interval,” as indicated by a red ring around the node (Chen et al., 2009; Chen et al., 2010). Sigma is the sum of the centrality and burstness scores, ranging from 0 to 1, with the highest sigma value associated with high-value research articles (Chen et al., 2009; Chen et al., 2010).
2.4.1.5 Knowledge Map Identification
The parameters for knowledge map identification methods include node, degree, burstiness, and centrality. The node is a parameter used to determine the frequency with which a variable has been cited, where a large node indicates a high number of citations, and the line is the connection between the nodes, indicating that these nodes appear in the same articles. Degree refers to the number of connections between variables in the knowledge map, with a higher Degree value indicating more communication and cooperation between the same variables (e.g. institutions, countries). The burstiness of a node is indicated by red rings surrounding it. It shows where the articles begin to “burst” and how strong the “burst” strength is. Purple rings indicate a node’s centrality; a node with a high centrality indicates a strategic position and the ability to bridge different nodes in the chosen networks.
3 Results
3.1 Descriptive Statistics
3.1.1 Evolution of Publications
Between January 1982 and June 2021, 2001 articles were published that contained research about the impact of climate change on aquatic production of one of the six species of interest. The number of publications increased from 1 in 1982 to 205 by 2020 (Figure 2). The number of published articles increased annually, with a 10-years average of 134 publications per year.
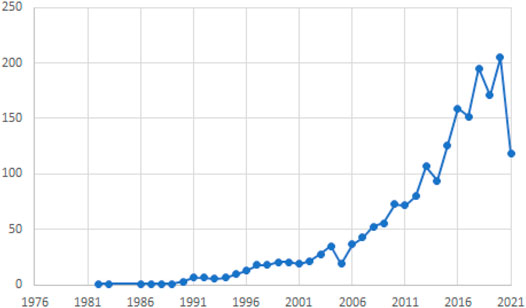
FIGURE 2. Number of research articles regarding climate change impacts on aquatic production of the top six aquaculture species published annually since 1980.
3.1.2 Productive Authors
Since 1980, only five authors produced more than 20 publications related to the effect of climate change on the production of the world’s top six cultured species (Figure 3). Out of a total of 6,762 authors, only 1,507 authors (22.29%) contributed more than one article. Soares AMVM published the most publications with 51, followed by Freitas R (50 publications) and Figueira E (43 publications).
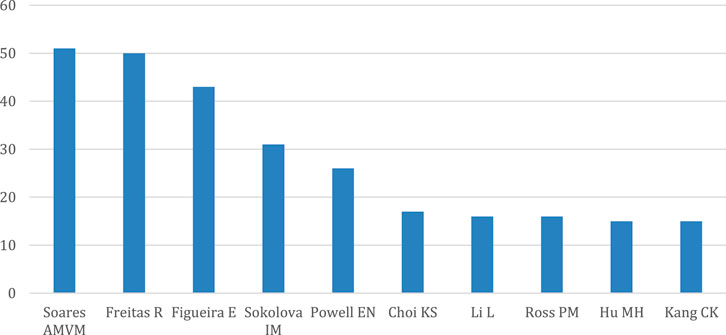
FIGURE 3. Top ten most productive authors for the time period 1980 to 2021, regarding research on climate change impacts on aquatic production of six aquaculture species.
3.1.3 Top Institutions
Figure 4 shows the top ten institutions in terms of total publications. With 146 publications, the Centre National De La Recherche Scientifique Cnrs, France was the leading institution, followed by Ifremer (France) (127 publications) and the Chinese Academy of Sciences (China) (89 publications). Institutions produced 1.1 articles on average.
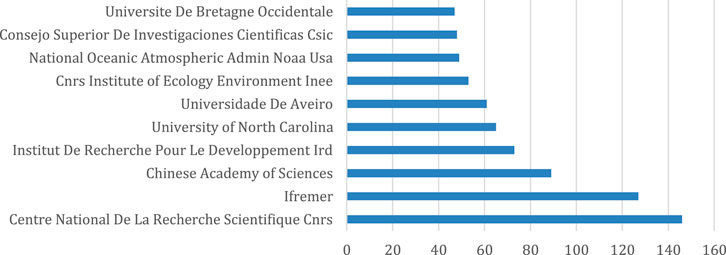
FIGURE 4. Number of publications from the top ten (of 1806) institutions that have researched impacts of climate change on the top six aquaculture species.
3.1.4 Productive Journals
Figure 5 illustrates the top ten journals by number of publications. In total, we identified eligible articles from 466 journals. Of these, Aquaculture had the most publications (105), followed by Journal of Shellfish Research (94 publications), and Marine Ecology Progress Series (74 publications). On average, most journals produced 4.23 articles within the 38 years of publication (1982–2021).
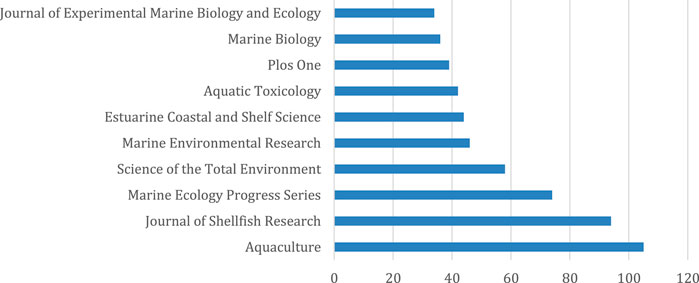
FIGURE 5. Number of publications from the top ten journals containing research about climate change impacts on aquatic production of the top six aquaculture species published between 1980 and 2021.
3.1.5 Regional Distribution
Figure 6 shows the ranking of countries that have published research related to climate change and aquatic species production. The number of publications is scaled from one to 631 on a gradient from white to dark blue. There were 85 countries with relevant publications, where the top five countries accounted for 69.51% of total publications. The United States of America had the most publications (631), followed by China (291 publications), and France (224 publications). The remaining countries had a mean of 10.5 publications.
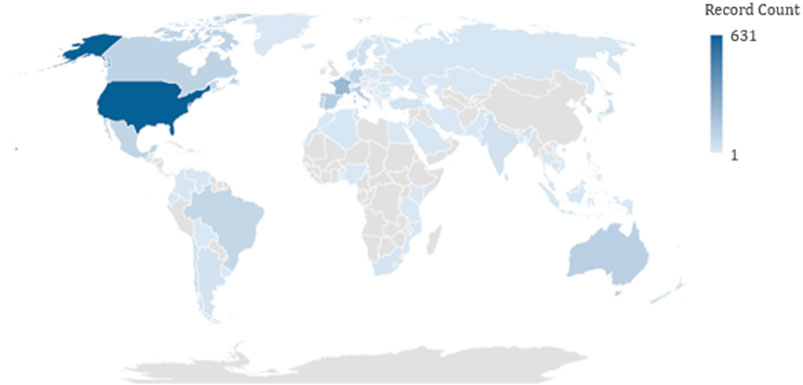
FIGURE 6. Number of publications produced from countries around the world. Dark blue represents the highest total number of publications, whereas lighter shades represent fewer publications.
3.2 Scientometric Analysis
3.2.1 Dual-Map Overlay
Figure 7 illustrates a dual-map overlay for research on the global impact of climate change on major aquatic species production. Dual-map overlay identifies the most productive disciplines using the photovoice method and the intellectual foundation of this domain. Based on the thickness of the blue line in the map, “Ecology, Earth, and Marine” is the most productive discipline. The majority of the publications in “Ecology, Earth, Marine” were cited from four sub-disciplines: i)“ Earth, Geology, Geophysics”; ii)“ Environmental, Toxicology, Nutrition”; (iii) “Plant, Ecology, Zoology” and iv) “Molecular, Biology. Genetics”.
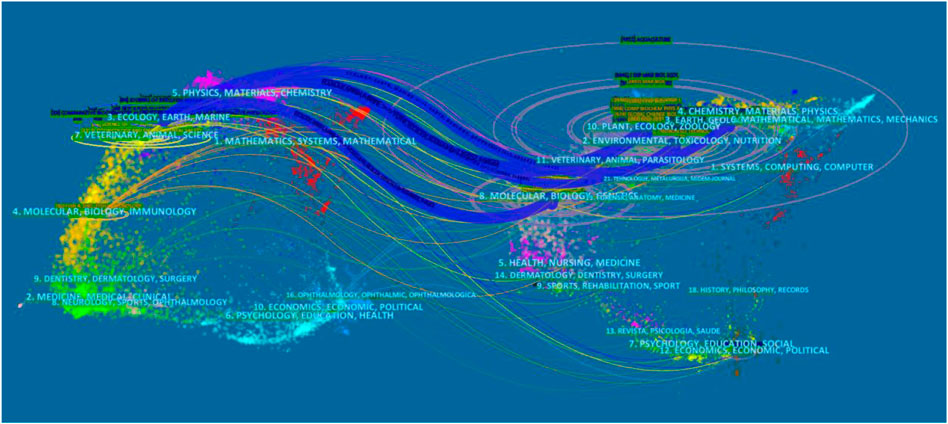
FIGURE 7. Dual map overlay on impact of climate change on major aquatic species production in the world. Points represent literature, ellipses represent groups of subject matter, and directional lines represent connection between subjects. Each unique discipline is represented by a different colour.
3.2.2 Co-Citation Analysis
Co-citation analysis generates a science map that includes nodes, connections, and density values to show the main structure of a selected variable’s development status and changes over time. The results of co-citation analysis for author, journal, country/region, institution, and article document are shown below.
3.2.2.1 Author Co-Citation Analysis
There were 736 nodes and 3,085 connections in the author co-citation network. The co-citation network density value was 0.0114, indicating that there is very little coordination and cooperation between authors. Density values represent the percentage of actual links between authors divided by the probability link. Higher density means higher connectedness between authors in these domain areas. The degree value represents the number of citations an author receives from another author for the same articles, where higher degrees indicate higher citations. Centrality is a measure of influence that shows the degree to which an author has on another’s, where an author with high centrality in the network is also one that has a great influence on the domain area’s behavior (e.g. Author A is cited by author B and author C, thus Author A is the center of information and paths for Author B and Author C must pass through). Sigma value is associated with high value research articles where any paper with sigma value more than 1 has high degree and centrality.
Figure 8 depicts authors that have a centrality score greater than 0.1, where font size increases as centrality score increases. Fu-Lin E. Chu was the most interconnected author, with a centrality score of 0.18, degree of 69, and a sigma of 26.62; in total Chu had 75 network connections (1.16 percent from overall connections). Laurent Barillé was the second most influential author, with a sigma score of 3.77, a centrality score of 0.19, and a degree score of 58. Frédéric Gazeau was the third most influential author (Degree: 32; Centrality: 0.19, Sigma: 3.28). Table 2 shows the details for the top ten authors with the most influence in these fields.
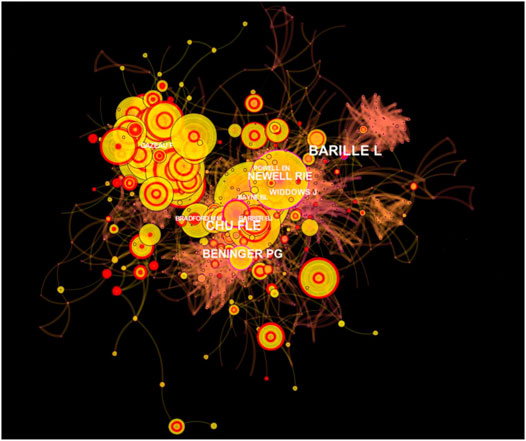
FIGURE 8. Network of authors co-citation, where coloured circles represent author node and the lines connecting them represent connections.
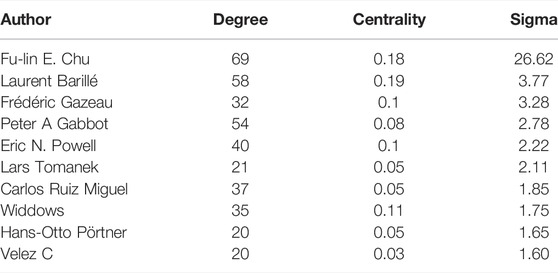
TABLE 2. The co-citation score for the top ten authors regarding research on climate change impacts on aquatic production of the top six aquaculture species.
3.2.2.2 Journal Co-Citation Analysis
Figure 9 illustrates the knowledge map for the journal co-citation analysis, including only journals with centrality scores greater than 0.1. The journal co-citation network had 336 nodes, 1731 connections, and a density of 0.0308. With a sigma score of 36.34, Journal of Shellfish Research (IF:1.396; Q3) was the most influential journal. The second and third most influential journals were Estuaries (IF: 2.133; Q1) and Biology Bulletin US (IF: 0.392; Q4), which had sigma scores of 31.38 and 8.00, respectively. Table 3 shows the details for the top ten journals with the highest influence.
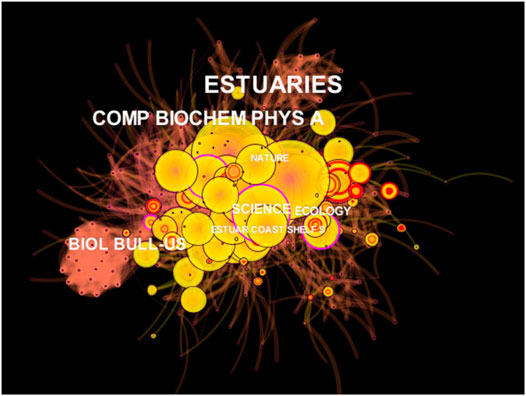
FIGURE 9. Network of journal co-citation. Journal name sizes scales with centrality score (Table 2).
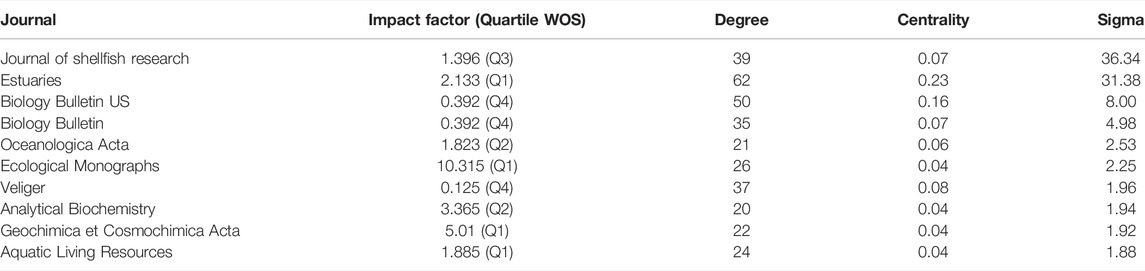
TABLE 3. The co-citation score for the top ten journals publishing articles about climate change impacts on aquatic production of the top six aquaculture species.
3.2.2.3 Countries/Region Co-Citation Analysis
Figure 10 demonstrates a network of co-citations based on the countries/regions of lead authors to obtain a more comprehensive analysis of country distribution and cooperation in field areas. Each node represents a country, and the lines represent each country’s cooperation with another. The size of the nodes reflects the country’s centrality score, and only countries with a centrality score greater than 0.1 are shown in Figure 10. The co-citation network of countries/regions has 52 nodes, 189 connections, and a density of 0.1425.
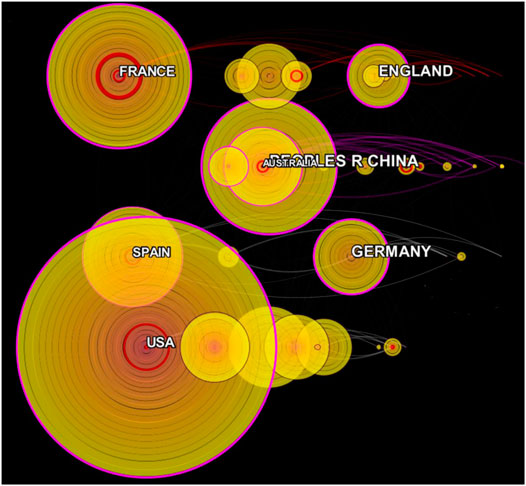
FIGURE 10. Network of country/region co-citation analysis where each node represents a country, and lines represent each country’s cooperation with another. Node size reflects centrality score (Table 4); only countries with centrality scores >0.1 are shown here.
Table 4 lists the top ten countries by co-citation score. The United States of America has the greatest influence among countries focusing on climate change and aquatic production research. This is based on a sigma score of 72.14, while France ranked second with a sigma score of 18.00, and Spain ranked third with a sigma score of 3.81. To improve the sigma score and gain more citations, a country/region should improve paper quality and strengthen collaboration with others.
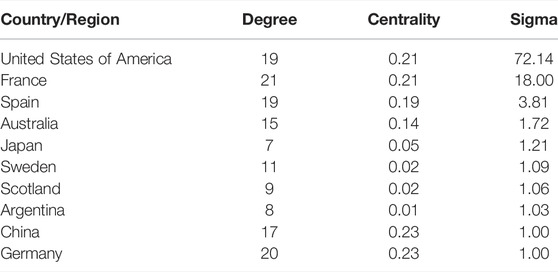
TABLE 4. The co-citation score of the top ten countries involved in publishing research about the impacts of climate change on the top six aquaculture species.
3.2.2.4 Institution Analysis
Figure 11 shows the analysis of cooperation among institutions, where each node represents an institution and the yellow lines between them represents cooperation. For ease of display, we only show institutions with a centrality score greater than 0.1. The network has 283 nodes, 371 connections, and a density of 0.0093. Ifremer from France had the highest sigma score (22.53), followed by The University of North Carolina at Chapel Hill (3.05), and Rutgers University (2.53). Interestingly, although our focus was on global research, the most influential universities were primarily located in France and the United States of America. This suggests the need for increased collaboration from institutions around the world. Table 5 shows the co-citation scores from the top ten institutions.
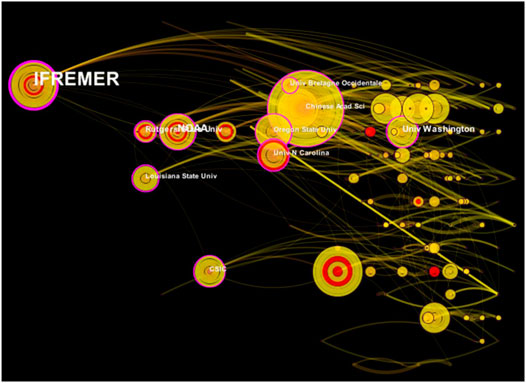
FIGURE 11. Network of institutions co-citation analysis where each node represents an institution and yellow lines represent cooperation. Only institutions with a centrality score greater than 0.1 are shown.
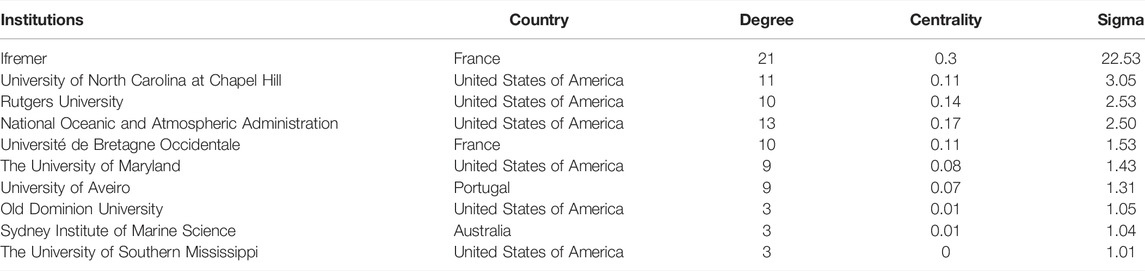
TABLE 5. Co-citation scores from the top ten institutions involved in research on climate change impacts on aquatic production of six aquaculture species.
3.2.2.5 Document Citation Analysis
Figure 12 illustrates document co-citation analysis for research areas; the network had 899 nodes and 2,289 connections, with a density of 0.0057. Only articles with a centrality score greater than 0.1 were listed, alphabetically. A “central” article plays a mediating role in our field of interest.
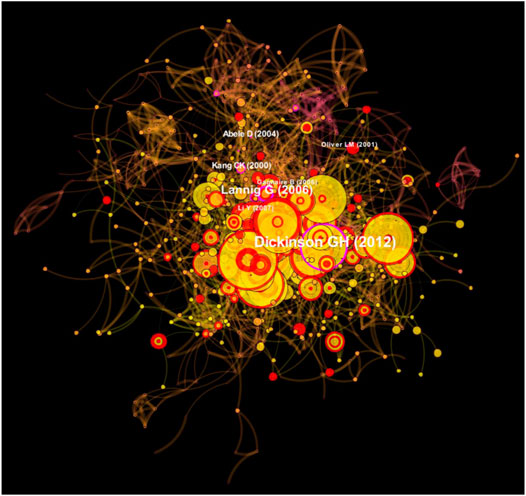
FIGURE 12. Network of document co-citation analysis where only articles with centrality scores greater than 0.1 are shown.
Table 6 displays the top ten most influential scientific publications according to the sigma score. Dickinson et al. (2012) had the most influential article with a sigma score of 4.99, and was titled “Interactive effects of salinity and elevated CO2 levels on juvenile eastern oysters, Crassostrea virginica”. Lannig et al. (2006) had the second highest sigma value. Their study was titled “Temperature-dependent stress response in Crassostrea virginica: Pollution reduces temperature tolerance in oysters” and was published in Aquatic Toxicology. Doney et al. (2009), published in Annual Review of Marine Science, had the third most influential article with a sigma score of 2.08. Their article was titled “Ocean Acidification: The Other CO2 Problem.ˮ
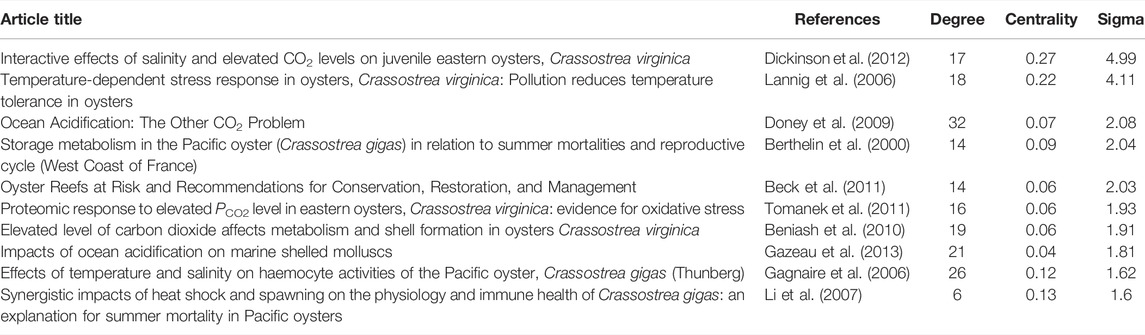
TABLE 6. Co-citation scores for the top ten articles related to research on climate change impacts on the production of the top six aquaculture species.
3.2.3 Document Cluster Analysis
The modularity Q index and the average silhouette metric for the Document Cluster Analysis was 0.8337 and 0.9201, respectively. This suggests a high level of reliability and homogeneity for the network. Our analysis yielded a total of 19 co-citation clusters, of which seven are summarized in Figure 13. Each cluster was labeled and then numbered and ranked according to size, where #0 was the largest cluster. The solid yellow line within each cluster represents the cluster’s lifetime. Text mining and a keyword analysis algorithm in CiteSpace software were used to generate cluster labels, then the loglikelihood ratio (LLR) was used to name these clusters.
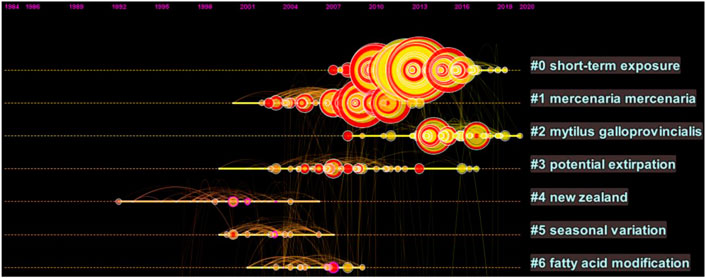
FIGURE 13. Summary of seven of the 19 identified document cluster lifetimes (solid lines). Cluster labels were generated from CiteSpace. Circle size corresponds to cluster size (i.e. number of publications).
Table 7 summarizes the seven major clusters identified by Document Cluster analysis, where each cluster represents a different research topic and its size is equal to the number of publications it has. Each of the seven clusters has more than 30 publications, with Cluster #0 having the most (118 publications). Cluster silhouette score ranged from 0.814 to 0.968, indicating a high degree of homogeneity among publications in each cluster (silhouette score ranges from -1 to 1, where scores higher than 0 are considered homogenous).
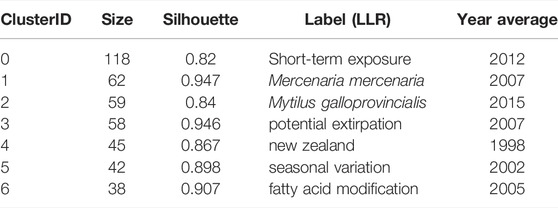
TABLE 7. The seven major clusters that emerged from Document Co-citation Analysis. Size represents the number of publications in a cluster, and silhouette score indicates levels of homogeneity. Labels were derived from log likelihood ratios (LLR).
3.2.4 Burst Analysis
To identify the most influential or landmark publications as well as keywords, we used a burst analysis; trends among studies and keywords are described below.
3.2.4.1 Document Burst
Table 8 shows the top ten publications with the most powerful citation bursts, where the duration of each burst is shown in the rightmost columns. A burst reflects the emergence of a keyword in a publication during a specific time period. The blue line represents the timeline (from 1982 to 2021), while the red line represents the burst period. The publication with the most recent and strongest burst was Gazeau et al. (2013), which is the current hotspot publication (strength = 15.82, 2016–2021).
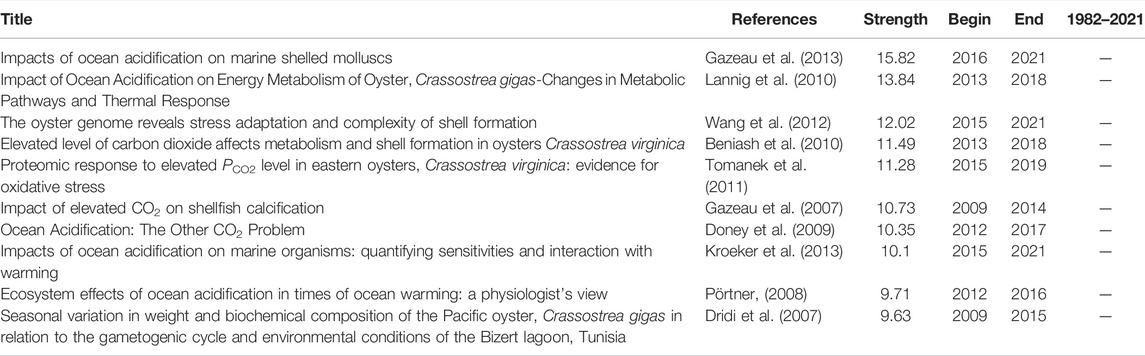
TABLE 8. Top ten publications with the strongest citation bursts between 1982 and 2021. Red sections in the timeline represent the burst period.
3.2.4.2 Keyword Burst
Table 9 displays the keywords with the highest citation burst. Keyword analysis has been used to detect emerging trends and research area hotspots over time. The burst reflects the emergence of a keyword in a particular subject area during a specific time period. As above, the blue line represents the timeline (from 1982 to 2021), while the red line represents the burst period. Beginning in 1992 and ending in 2005, the word “seasonal variation” had the highest burst strength (16.17). The next top two keywords were “perkinsus marinus” (Strength = 11.48, 1994–2012) and “seawater acidification” (Strength = 10.32, 2016–2021). Although one of the shorter bursts (5 years), “seawater acidification” had the most recent burst time period, continuing into 2021, indicating a more recent emerging interest for this topic.
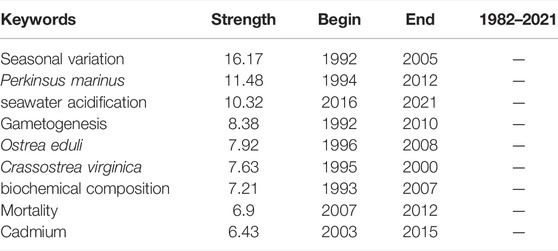
TABLE 9. Top ten keywords with strongest citation burst for the time period 1982 to 2021. Red section on timelines represents the period of the burst.
4 Discussion
Our study aimed to present a scientometric analysis for research on the effects of climate change on the top major aquatic production. We discuss our results in detail below.
4.1 Research Question Discussion
4.1.1 RQ1: What Are the Overall Publication Trends in Terms of Publication Output?
To answer our first research question, we performed a descriptive analysis on the number of publications, published journals, authors, universities/institutes, and the countries/regions of affiliation. The number of articles published increased annually, with a 10-years average of 134 publications per year. The United States of America had the greatest number of papers published in the field, while France had the second highest number of publications. With 13.64 total publications, Centre National De La Recherche Scientifique Cnrs and Ifremer from France together had the highest publication rate for institutions. This demonstrates that developed countries such as the United States of America and France are leading in this field. While other countries may have a relatively large number of publications, such as China, they have yet to emerge as prominent research institutions and/or scholars. This result may be due to France and the United States having more resources to conduct scientific analyses. As such, we strongly suggest that more international scientific research exchanges and collaboration be conducted in the future.
4.1.2 RQ2: Where is This Research Area Situated on the Map of Web of Sciences Database?
Publications were largely situated within the discipline “Ecology, Earth, and Marine” and there were few publications in other disciplines. While this result may not be surprising given the nature of the research, we suggest that multidisciplinary studies would be beneficial to fully understand the impact of climate change on aquatic production. For example, research joining ecology and economics would give deeper insights on the economic effects of climate change.
4.1.3 RQ3: Who Are the Dominant Knowledge Carriers in These Areas?
To answer our third research question, a co-citation analysis of the author, journal, country, institutions, and articles was performed. We found that Fu-Lin E. Chu was the most influential author, with a focus on nutrition and host/parasite interactions in shellfish and fish. Laurent Barillé was the second most influential author, with a background in multi- and hyperspectral remote sensing of intertidal areas. Frédéric Gazeau, the third most influential author, studied coastal metabolism, the effects of ocean acidification and warming on various marine organisms, and the use of techniques to study the effects of ocean acidification on marine communities. The work from these top three researchers demonstrates the multi-disciplinarity of climate change and aquatic production research. It is critical for the experts in these fields to engage with others in different focus areas to build connections between sub-disciplines. For example, work on host/parasite interactions would greatly benefit from research on ocean acidification–does increasing acidification change host/parasite interactions? Increased inter-disciplinary cooperation would help address such questions and give a better picture of climate change impacts on aquacultured species.
Our analysis on scientific journals revealed that ecology and biology research dominated the research areas. Some of the most prestigious journals in biology science have published articles in this area. For example, the Journal of Shellfish Research, Estuaries, and Biology Bulletin US were among the most influential journals. For influential institutions, Ifremer from France, the University of North Carolina, and Rutgers University from the United States of America were the most influential. As a result, it is not surprising that France and the United States ranked first and second in the co-citation analysis results discussed above. However, this again demonstrates that research affiliations are unbalanced, with most research conducted by France and the United States. Because research on climate change impacts affects the entire world, it is clear that collaboration within this research domain would be beneficial.
Of the top ten most influential scientific publications, Matoo’s (2013) article was the most influential. Matoo (2013) investigated the interactions of salinity and partial pressure of CO2 (PCO2) on biomineralization and energy homeostasis in juvenile Crassostrea virginica, a common estuarine bivalve. According to the study, the combined effects of elevated PCO2 and fluctuating salinity may endanger the survival of eastern oysters by weakening their shells and increasing energy consumption. Their results suggest that the production of these bivalves may be at risk as climate change may affect both PCO2 and salinity. Lannig et al. (2006) had the article with the second highest co-citation score. Their research assessed how temperature and a toxic metal, cadmium (Cd), affects energy metabolism. They found that oysters exposed to elevated temperature, but not Cd, showed no significant change in condition, survival rate, or lipid peroxidation. However, those exposed to both Cd and temperature stress died quickly. Doney, Fabry, Feely, and Kleypas (2009) had the third highest co-citation score. Their work discussed the impact of decreased calcium carbonate saturation on shell-forming marine organisms ranging from plankton to benthic molluscs, echinoderms, and corals. Together, these three works demonstrate the impacts of continued ocean warming and ocean acidification on aquatic organisms, especially for shell-forming species. It is clear there are interactive effects of ocean temperature, acidification, and environmental metals that warrant further investigation. Regardless, their results support the increasing need to decrease effects of climate change to maintain current production of shelled organisms.
4.1.4 RQ4: What Are the Dominant Topics/Clusters, and What is Their Temporal Evolution?
To answer our fourth question, we used a document cluster analysis which yielded a total of 19 co-citation clusters. Publications were assigned to each cluster because they were cited by a similar group of publications, indicating a co-citation relationship. According to our results, the dominant research topics and directions were “short-term exposure”; “Mercenaria mercenaria”; “Mytilus galloprovincialis”; “potential extirpation”; “New Zealand”; “seasonal variation”; and “fatty acid modification.” This indicates that the majority of research focuses on two species, particularly from New Zealand, and on the effects of relatively short time periods of exposure. While it is important to study the short-term exposure to stressors in marine organisms, climate change is likely to have longer term impacts, and as such there is a need to understand how long-term stress will impact species.
4.1.5 RQ5: What Are the impactful Publications and Keywords for These Areas?
The document burst analysis showed a pattern of new research topics emerging, with previous burst publications gradually being replaced by more recent publications. Gazeau et al. (2007) investigated the calcification rates of edible mussels (Mytilus edulis) and the Pacific oyster (Crassostrea gigas), which decreased linearly as pCO2 increased. Gazeau et al. (2013) had the most recent burst citation, and studied pteropods, which have a negative relationship with the level of ocean acidification. The blood of shelled molluscs may have lower pH, which can affect several physiological processes (e.g., respiration, excretion, etc.) and, in some cases, increase their mortality over time. The shift in focus from the previous study, which focused on impact, to the current study, which focused on resilience, demonstrates an increased understanding of the issues in the research areas. It also indicates that the current body of research is focusing on understanding acidification effects. This is important to understand for future management of important shelled species that are cultured. Again, the most important papers identify effects of ocean acidification as being significant and important to mitigate.
5 Conclusion
In general, ocean acidification was identified as a reoccurring important climate change impact to understand, particularly with respect to the production of aquatic species. With the world still struggling to find effective solutions to combat climate change, research on climate action and aquatic production is increasingly important to mitigate or minimize environmental effects on the aquaculture industry. Here, we have compiled a cluster of related research from different disciplines and noted important research gaps and future directions. Most of the research focused on ocean acidification effects, but next we need to understand how to mitigate such effects. There is a significant gap that requires further investigation and international collaboration.
Our study only obtained papers from Web of Science (WOS) databases, which may have resulted in publication bias. However, WOS is regarded as having higher publication standards because its database is geared towards science and social sciences, and because it has larger databases with a broader scope than other available datasets (Bar-Ilan, 2008; Adriaanse and Rensleigh, 2013). Future research could compare other databases with WOS to map these research areas and perhaps identify missing links. Additionally, we used CiteSpace software rather than collecting data manually, thus some irrelevant subjects may have been included in the analysis. Still, CiteSpace allows our analysis to be reproducible, and striking a balance between stringent criteria and over-excluding specific studies is difficult. Future research with a high precision goal should consider using more stringent keyword searches or reviewing exported data manually to reduce the likelihood of irrelevant studies.
In our co-citation analyses, only the names of the primary (first) authors were used. Although citing publications did not have such a restriction, databases of cited publications downloaded from WoS did not include the names of other contributing authors. If these databases made more author names available, the co-citation analysis could produce different results, possibly identifying more collaboration than we detected. Regardless, our study offers a comprehensive view on the current body of research studying the effects of climate change on the production of top aquatic species. We clearly demonstrate the need for more international collaboration. Addressing climate change impacts is important to the sustainable research agenda in conjunction with achieving the SDGs goal by 2030. We also suggest that studies in disciplines other than “Ecology, Earth, and Marine” should be encouraged in the future, as they may offer important insights that would otherwise be overlooked (e.g. economic perspectives).
Author Contributions
All authors conceptualized this study. MNA and MM formulated the research objectives, whereas MNA pointed out the inclusion and exclusion criteria under the supervision of other two authors MAG and YS The expert in the subject is MNA and MAG Then, MM performed the searches. YS proof-read the article and reviewed the overall manuscript scientifically. All authors have read and agreed to the published version of the manuscript.
Funding
The Department of Higher Education, Ministry of Higher Education Malaysia, provided funding through the Long Term Research Grant Scheme (LRGS) program (LRGS/1/2020/UMT/01/1; LRGS UMT Vot No. 56040) titled ‘Ocean climate change: potential risk, impact, and adaptation towards marine and coastal ecosystem services in Malaysia.’
Conflict of Interest
The authors declare that the research was conducted in the absence of any commercial or financial relationships that could be construed as a potential conflict of interest.
Publisher’s Note
All claims expressed in this article are solely those of the authors and do not necessarily represent those of their affiliated organizations, or those of the publisher, the editors and the reviewers. Any product that may be evaluated in this article, or claim that may be made by its manufacturer, is not guaranteed or endorsed by the publisher.
Acknowledgments
The first author wishes to express his gratitude to the Sustainable Ocean Alliance (SOA) and the Environmental Defense Fund (EDF) in the United States of America (United States) for his inaugural fellowship on Leadership for Climate Resilient Fisheries (LCRF).
Supplementary Material
The Supplementary Material for this article can be found online at: https://www.frontiersin.org/articles/10.3389/fenvs.2022.905428/full#supplementary-material
References
Aryadoust, V., and Ang, B. H. (2019). Exploring the Frontiers of Eye Tracking Research in Language Studies: A Novel Co-Citation Scientometric Review. Comput. Assist. Lang. Learn. 34, 898–933. doi:10.1080/09588221.2019.1647251
Azra, M. N., Noor, M. I. M., Ikhwanuddin, M., and Ahmed, N. (2021). “Global Trends on Covid-19 and Food Security Research: A Scientometric Study,” in Advances in Food Security and Sustainability. Editor M. Cohen. 6th Edition, 1–33. Cambridge, Massachusetts, Academic Press. Available at: (https://www.ncbi.nlm.nih.gov/pmc/articles/PMC8426152/pdf/main.pdf). doi:10.1016/bs.af2s.2021.07.005
Azra, M. N., Noor, M. I. M., Sung, Y. Y., Dawood, M. A. O., and Ghaffar, M. A. (2022). Trends and Developments in Thermal Tolerance: A Scientometric Research Study. J. Ther. Biol. 106, 103234. doi:10.1016/j.jtherbio.2022.103234
Azzeri, A., Ching, G. H., Jaafar, H., Mohd Noor, M. I., Razi, N. A., Then, A. Y.-H., et al. (2020). A Review of Published Literature Regarding Health Issues of Coastal Communities in Sabah, Malaysia. Ijerph 17, 1533. doi:10.3390/ijerph17051533
Bar-Ilan, J. (2008). Which H-Index? - A Comparison of WoS, Scopus and Google Scholar. Scientometrics 74, 257–271. doi:10.1007/s11192-008-0216-y
Beck, M. W., Brumbaugh, R. D., Airoldi, L., Carranza, A., Coen, L. D., Crawford, C., et al. (2011). Oyster Reefs at Risk and Recommendations for Conservation, Restoration, and Management. BioScience 61, 107–116. doi:10.1525/bio.2011.61.2.5
Beniash, E., Ivanina, A., Lieb, N., Kurochkin, I., and Sokolova, I. (2010). Elevated Level of Carbon Dioxide Affects Metabolism and Shell Formation in Oysters Crassostrea Virginica (Gmelin). Mar. Ecol. Prog. Ser. 419, 95–108. doi:10.3354/meps08841
Berthelin, C., Kellner, K., and Mathieu, M. (2000). Storage Metabolism in the Pacific Oyster (Crassostrea gigas) in Relation to Summer Mortalities and Reproductive Cycle (West Coast of France). Comp. Biochem. Physiology Part B Biochem. Mol. Biol. 125, 359–369. doi:10.1016/S0305-0491(99)00187-X
Callaway, R., Shinn, A. P., Grenfell, S. E., Bron, J. E., Burnell, G., Cook, E. J., et al. (2012). Review of Climate Change Impacts on Marine Aquaculture in the UK and Ireland. Aquat. Conserv. Mar. Freshw. Ecosyst. 22, 389–421. doi:10.1002/aqc.2247
Chen, C., Chen, Y., Horowitz, M., Hou, H., Liu, Z., and Pellegrino, D. (2009). Towards an Explanatory and Computational Theory of Scientific Discovery. J. Inf. 3, 191–209. doi:10.1016/j.joi.2009.03.004
Chen, C., Ibekwe-SanJuan, F., and Hou, J. (2010). The Structure and Dynamics of Cocitation Clusters: A Multiple-Perspective Cocitation Analysis. J. Am. Soc. Inf. Sci. 61, 1386–1409. doi:10.1002/asi.21309
Chen, C., and Leydesdorff, L. (2014). Patterns of Connections and Movements in Dual-Map Overlays: A New Method of Publication Portfolio Analysis. J. Assn Inf. Sci. Tec. 65, 334–351. doi:10.1002/asi.22968
Chen, C. (2004). Searching for Intellectual Turning Points: Progressive Knowledge Domain Visualization. Proc. Natl. Acad. Sci. U.S.A. 101, 5303–5310. doi:10.1073/pnas.0307513100
Chen, C., and Song, M. (2019). Visualizing a Field of Research: A Methodology of Systematic Scientometric Reviews. Plos One 14, e0223994. doi:10.1371/journal.pone.0223994
Cheung, W. W. L., Lam, V. W. Y., Sarmiento, J. L., Kearney, K., Watson, R., Zeller, D., et al. (2010). Large-Scale Redistribution of Maximum Fisheries Catch Potential in the Global Ocean Under Climate Change. Glob. Change Biol. 16, 24–35. doi:10.1111/j.1365-2486.2009.01995.x
Dickinson, G. H., Ivanina, A. V., Matoo, O. B., Pörtner, H. O., Lannig, G., Bock, C., et al. (2012). Interactive Effects of Salinity and Elevated CO2 Levels on Juvenile Eastern Oysters, Crassostrea Virginica. J. Exp. Biol. 215, 29–43. doi:10.1242/jeb.061481
Doney, S. C., Fabry, V. J., Feely, R. A., and Kleypas, J. A. (2009). Ocean Acidification: The Other CO2 Problem. Annu. Rev. Mar. Sci. 1, 169–192. doi:10.1146/annurev.marine.010908.163834
Dridi, S., Romdhane, M. S., and Elcafsi, M. h. (2007). Seasonal Variation in Weight and Biochemical Composition of the Pacific Oyster, Crassostrea gigas in Relation to the Gametogenic Cycle and Environmental Conditions of the Bizert Lagoon, Tunisia. Aquaculture 263, 238–248. doi:10.1016/j.aquaculture.2006.10.028
FAO (2020). Food and Agriculture Organization of the United Nation (FAO), 2020 Edition of the State of World Fisheries and Aquaculture: Sustainability in Action. Rome, Italy: The Publishing Group (OCCP). 244. doi:10.4060/ca9229en
FAO (2021). Publications: The Collection of the State of World Fisheries and Aquaculture (SOFIA). Available at: http://www.fao.org/fishery/publications/sofia/en (Assessed at 1st September, 2021).
FAO (2011) World Aquaculture 2010. FAO Fisheries and Aquaculture Technical Paper: 500/1. Aquaculture Service: Fisheries and Aquaculture Resources Use and Conservation Division. Rome: FAO Fisheries and Aquaculture Department, Food And Agriculture Organization Of The United Nations. Available at: http://www.fao.org/3/ba0132e/ba0132e.pdf.
Froehlich, H. E., Gentry, R. R., and Halpern, B. S. (2018). Global Change in Marine Aquaculture Production Potential Under Climate Change. Nat. Ecol. Evol. 2, 1745–1750. doi:10.1038/s41559-018-0669-1
Gagnaire, B., Frouin, H., Moreau, K., Thomas-Guyon, H., and Renault, T. (2006). Effects of Temperature and Salinity on Haemocyte Activities of the Pacific Oyster, Crassostrea gigas (Thunberg). Fish Shellfish Immunol. 20, 536–547. doi:10.1016/j.fsi.2005.07.003
Gazeau, F., Parker, L. M., Comeau, S., Gattuso, J.-P., O’Connor, W. A., Martin, S., et al. (2013). Impacts of Ocean Acidification on Marine Shelled Molluscs. Mar. Biol. 160, 2207–2245. doi:10.1007/s00227-013-2219-3
Gazeau, F., Quiblier, C., Jansen, J. M., Gattuso, J.-P., Middelburg, J. J., and Heip, C. H. R. (2007). Impact of Elevated CO2 on Shellfish Calcification. Geophys. Res. Lett. 34, L07603. doi:10.1029/2006GL028554
Heasman, K. G., Scott, N., Ericson, J. A., Taylor, D. I., and Buck, B. H. (2020). Extending New Zealand's Marine Shellfish Aquaculture into Exposed Environments - Adapting to Modern Anthropogenic Challenges. Front. Mar. Sci. 7, 565686. doi:10.3389/fmars.2020.565686
Kroeker, K. J., Kordas, R. L., Crim, R., Hendriks, I. E., Ramajo, L., Singh, G. S., et al. (2013). Impacts of Ocean Acidification on Marine Organisms: Quantifying Sensitivities and Interaction with Warming. Glob. Change Biol. 19, 1884–1896. doi:10.1111/gcb.12179
Lannig, G., Eilers, S., Portner, H. O., Sokolova, I. M., and Bock, C. (2010). Impact of Ocean Acidification on Energy Metabolism of Oyster, Crassostrea gigas—Changes in Metabolic Pathways and Thermal Response. Marine Drugs 8, 2318–2339. doi:10.3390/md8082318
Lannig, G., Flores, J. F., and Sokolova, I. M. (2006). Temperature-Dependent Stress Response in Oysters, Crassostrea Virginica: Pollution Reduces Temperature Tolerance in Oysters. Aquat. Toxicol. 79, 278–287. doi:10.1016/j.aquatox.2006.06.017
Li, Y., Qin, J. G., Abbott, C. A., Li, X., and Benkendorff, K. (2007). Synergistic Impacts of Heat Shock and Spawning on the Physiology and Immune Health of Crassostrea Gigas: An Explanation for Summer Mortality in Pacific Oysters. Am. J. Physiology-Regulatory, Integr. Comp. Physiology 293, R2353–R2362. doi:10.1152/ajpregu.00463.2007
Matoo, O. B. (2013). Interactive Effects of Ocean Acidification and Multiple Stressors on Physiology of Marine Bivalves. Diss. Abstr. Int. 75 (Suppl. B), 248. Available at: https://ninercommons.uncc.edu/islandora/object/etd%3A1255/datastream/PDF/download/citation.pdf.
Maulu, S., Hasimuna, O. J., Haambiya, L. H., Monde, C., Musuka, C. G., Makorwa, T. H., et al. (2021). Climate Change Effects on Aquaculture Production: Sustainability Implications, Mitigation, and Adaptations. Front. Sustain. Food Syst. 5, 609097. doi:10.3389/fsufs.2021.609097
Meho, L. I., and Rogers, Y. (2008). Citation Counting, Citation Ranking, and h -Index of Human-Computer Interaction Researchers: A Comparison of Scopus and Web of Science. J. Am. Soc. Inf. Sci. Technol. 59, 1711–1726. doi:10.1002/asi.20874
Nalimov, V., and Mulcjenko, B. (1971). Measurement of Science: Study of the Development of Science as an Information Process. Washington DC: Foreign Technology Division, 1–210. Available at: https://eric.ed.gov/?id=ED065286.
Oremus, K. L., Bone, J., Costello, C., García Molinos, J., Lee, A., Mangin, T., et al. (2020). Governance Challenges for Tropical Nations Losing Fish Species Due to Climate Change. Nat. Sustain 3, 277–280. doi:10.1038/s41893-020-0476-y
Pauly, D., and Cheung, W. W. L. (2018). Sound Physiological Knowledge and Principles in Modeling Shrinking of Fishes Under Climate Change. Glob. Change Biol. 24, e15–e26. doi:10.1111/gcb.13831
Pörtner, H. (2008). Ecosystem Effects of Ocean Acidification in Times of Ocean Warming: A Physiologist's View. Mar. Ecol. Prog. Ser. 373, 203–217. doi:10.3354/meps07768
Rosa, R., Marques, A., and Nunes, M. L. (2012). Impact of Climate Change in Mediterranean Aquaculture. Rev. Aquac. 4, 163–177. doi:10.1111/j.1753-5131.2012.01071.x
S. Adriaanse, L., and Rensleigh, C. (2013). Web of Science, Scopus and Google Scholar a Content Comprehensiveness Comparison. Electron. Libr. 31, 727–744. doi:10.1108/EL-12-2011-0174
Sainz, J. F., Di Lorenzo, E., Bell, T. W., Gaines, S., Lenihan, H., and Miller, R. J. (2019). Spatial Planning of Marine Aquaculture Under Climate Decadal Variability: A Case Study for Mussel Farms in Southern California. Front. Mar. Sci. 6, 253. doi:10.3389/fmars.2019.00253
Steeves, L. E., Filgueira, R., Guyondet, T., Chassé, J., and Comeau, L. (2018). Past, Present, and Future: Performance of Two Bivalve Species Under Changing Environmental Conditions. Front. Mar. Sci. 5, 184. doi:10.3389/fmars.2018.00184
Thakur, K. K., Vanderstichel, R., Barrell, J., Stryhn, H., Patanasatienkul, T., and Revie, C. W. (2018). Comparison of Remotely-Sensed Sea Surface Temperature and Salinity Products with In Situ Measurements from British Columbia, Canada. Front. Mar. Sci. 5, 121. doi:10.3389/fmars.2018.00121
Tomanek, L., Zuzow, M. J., Ivanina, A. V., Beniash, E., and Sokolova, I. M. (2011). Proteomic Response to Elevated PCO2 Level in Eastern Oysters, Crassostrea Virginica: Evidence for Oxidative Stress. J. Exp. Biol. 214, 1836–1844. doi:10.1242/jeb.055475
UN SDG (2021). United Nation Sustainable Development Goals, Goal 13: Take Urgent Action to Combat Climate Change and its Impacts (Updates as COVID-19 Response). Available at: https://www.un.org/sustainabledevelopment/climate-change/ (Assessed at 4th September, 2021).
Zhang, G., Fang, X., Guo, X., Li, L., Luo, R., Xu, F., et al. (2012). The Oyster Genome Reveals Stress Adaptation and Complexity of Shell Formation. Nature 490, 49–54. doi:10.1038/nature11413
Keywords: aquaculture production, climate action, crustaceans, environment, finfish, molluscs, sustainable development goals (SDG), state of world fisheries and aquaculture
Citation: Azra MN, Mohd Noor MI, Sung YY and Abd Ghaffar M (2022) Visualizing a Field of Research With Scientometrics: Climate Change Associated With Major Aquatic Species Production in the World. Front. Environ. Sci. 10:905428. doi: 10.3389/fenvs.2022.905428
Received: 27 March 2022; Accepted: 25 May 2022;
Published: 13 July 2022.
Edited by:
Huopo Chen, Institute of Atmospheric Physics (CAS), ChinaReviewed by:
Luigi Aldieri, University of Salerno, ItalyGuo Donglin, Institute of Atmospheric Physics (CAS), China
Copyright © 2022 Azra, Mohd Noor, Sung and Abd Ghaffar. This is an open-access article distributed under the terms of the Creative Commons Attribution License (CC BY). The use, distribution or reproduction in other forums is permitted, provided the original author(s) and the copyright owner(s) are credited and that the original publication in this journal is cited, in accordance with accepted academic practice. No use, distribution or reproduction is permitted which does not comply with these terms.
*Correspondence: Mohamad N. Azra, YXpyYW1uQHVtdC5lZHUubXk=; Mazlan Abd Ghaffar, bWFnQHVtdC5lZHUubXk=
†These authors share first authorship