- 1Department of Matters and Energy Fluxes, Global Change Research Institute of the Czech Academy of Sciences, Brno, Czechia
- 2Laboratory of Ecological Plant Physiology, Global Change Research Institute of the Czech Academy of Sciences, Brno, Czechia
Agricultural practices applied to increase crop production and secure future food demands can have a large negative impact on the environmental quality. They should be, therefore, also evaluated in terms of an impact on soil organic carbon (C) content and greenhouse gas emissions. In this study, we investigated the combined effects of adaptation technology (AT) based on no-till crop sowing into cover crop mulch, nitrogen (N) fertilization, and external C supply in the form of biochar, compost, and composted biochar on soil CO2 efflux (Rs). Rs was measured using a closed gas-exchange system in biweekly to monthly intervals during 2 consecutive years when winter wheat (first year) and spring barley (second year) were cultivated. Biochar, compost, and composted biochar had no significant effects on Rs. N fertilization with the medium fertilizer dose tended to increase Rs compared to the control at the beginning of the growing season, followed by Rs decrease later in the season. On the other hand, fertilization with the maximum fertilizer dose mostly decreased Rs throughout the whole growing season. AT affected the dynamics of Rs substantially, depending on the year, site, and N fertilization, resulting in significant interactions between technology and date of measurement and also between site and technology. However, the mean effect of AT across the whole season was insignificant. The results show that the C increase in the soil that occurs after the application of compost and the combination of composted biochar application with AT is not accompanied by an increase in Rs and, thus, leads to at least short-term C storage in the soil. The reduction in CO2 emissions from soil to the atmosphere under AT represents a positive aspect of such management practice for mitigating climate change.
Introduction
The world population is constantly rising and is estimated to reach 9.7 billion by 2050 (UN DESA, 2019). That brings up together with the ongoing climate change serious problems, including increasing pressure on food production and security. Intensification of agriculture, however, leads to environmental issues such as soil degradation and water pollution (Chartres and Noble, 2015). Such practices include in particular N fertilization and intensive tillage. Therefore, novel approaches to agricultural production are necessary to meet the growing food demands with minimized negative environmental impact. Moreover, reducing greenhouse gas emissions from soil and efforts to sequester C in the soil in agriculture fields are getting important in the context of climate change mitigation in recent decades.
N is the primary limiting nutrient for plant growth. Its application in agriculture is, therefore, needed for proper growth and development of crops, grain quality, and desired yields. The excessive N application, however, leads to groundwater contamination (Zufiaurre et al., 2019), increased emissions of nitrous oxide (Mosier et al., 2006), which represents an important greenhouse gas, and in the case of NH4+-based fertilizers also to soil acidification (Schroder et al., 2011). To reduce the amount of N fertilizers used and to retain its positive effects at the same time, approaches to increase N use efficiency are employed. Such an effect was reported for biochar amendment (Badu et al., 2019). Biochar application may also lead to increased crop yields, as reported by, e.g., Alburquerque et al. (2013) and Dubey et al. (2020). Another alternative to conventional fertilization is the use of compost that, together with biochar, enables nutrient recycling of organic wastes (Sánchez-Monedero et al., 2019). The combination of biochar and compost appears to be very efficient for soil quality improvement. (Abideen et al., 2020). Biochar has a high cation exchange capacity (Mukherjee and Lal, 2013) and thus promotes the retention of compost nutrients.
For reduction in soil organic matter losses, together with soil protection from erosion and extensive drying, no-till management has been applied (Chaghazardi et al., 2016; Blanco-Canqui and Ruis, 2018). The crop yields in no-till systems seem to be generally lower than in conventional tillage (Xiao et al., 2019; Biberdzic et al., 2020). However, yield improvements were also recorded (Chaghazardi et al., 2016), especially in drier conditions (Angás et al., 2006).
The main goal of effective crop management is to ensure sufficient food production, protect soil from organic C losses and erosion, and finally, also to reduce CO2 emissions to the atmosphere. As agricultural land covers 37% of land area (https://data.worldbank.org/indicator/AG.LND.AGRI.ZS), it represents a substantial source of greenhouse gas emissions, but it also has considerable potential for C sequestration. Therefore, soil CO2 efflux should be one of the parameters considered when evaluating the adoption of innovative management practices in agriculture. CO2 in the soil is produced from the respiration of soil microorganisms and plant roots. They are both driven by micrometeorological conditions, especially temperature and soil water content (Zhang et al., 2013). Microbial respiration is, moreover, affected by soil organic matter availability, while root respiration is driven by crop production (Lohila et al., 2003; Zhang et al., 2013). The effects of management practices on these two processes can not only be additive or even synergistic, but could also lead to compensation to a certain extent because the management effects on soil temperature, microbial community, and crop growth can be variable (e.g., Faust et al., 2019).
All above-listed treatments have a substantial impact on soil properties, quality, microbial biomass, and crop production, which further affects soil C loss through respiration and emissions of CO2 to the atmosphere. The effect of the individual treatments on soil respiration was found to be variable from negative, through zero to positive (Ginting et al., 2003; Zhou et al., 2014; Shah et al., 2017; Li et al., 2018; Wilson et al., 2018; Ge et al., 2020). The effect of different treatments can be site-specific, which may lead to difficulties in comparison of individual management techniques. Therefore, studies with the combination of various treatments are highly needed to create management strategies that maximize the benefits (crop yield) and minimize risks (soil degradation, water pollution, and CO2 emissions).
The aim of the present study was to evaluate the effect of various management practices and their combinations on arable soil CO2 efflux during 2 subsequent years. The main goals were to determine the effect of no-till management with cover crops growing, determine the response of Rs to various amounts of inorganic N fertilizer, and compost, biochar, and composted biochar amendments.
Materials and Methods
Site Description
The experiment was carried out at two nearby sites close to the municipality of Banín in the Czech Republic. The first site was situated to the east of the municipality (E-site; 49°39.9′ N, 16°28.4′ E; 475 m a.s.l.), and the second one to the northwest (NW-site; 49°40.4′ N, 16°27.5′ E; 460 m a.s.l.). The region is characterized by a mean annual temperature 8.0°C and mean sum of precipitation 621 mm (long-term averages 2000–2020). The distance between sites is 1.5 km only, which allows us to consider the differences in climatic parameters to be negligible. The soil type in both sites is Retisol (FAO soil groups). The soil texture is silt loam at the E-site and sandy loam at the NW-site. According to the analyses performed before establishment of the plots, the topsoil at the E-site compared to NW-site was characterized by slightly higher porosity (44.2 vs. 42.5%), higher cation exchange capacity (118.4 meq kg−1 vs. 103.3 meq kg−1), and higher pH/KCl (6.2 vs. 5.5).
Experimental Design
At each site, four experimental blocks (100 × 5 m) were established. Within each block, the plots of a length of 5 m were established with the C amendment (biochar, compost, and composted biochar) or N fertilization. The plots within blocks were randomized. Each block was length-wise split into two strips with conventional (tillage; CT) and adaptation technology (no-till and cover crops; AT), ensuring that each plot got established in both technologies (split-plot design). Each combination of treatment (C and N applications) and technology was thus four times replicated. The plots were separated within blocks by buffering strips of 2 m, sown with the crop, while the individual blocks were separated by 4 m grass strips (Supplementary Figure S1).
All plots were sown with winter wheat (Triticum aestivum var. aestivum) variety Tiguan in October 2018 and with spring barley (Hordeum vulgare) variety Bojos in March 2020. Sowing was carried out with the no-till seeders STP300 (2018) and Vaderstad Rapid 300 (2020). In AT plots, the sowing was carried out directly into the cover crop mulch. In CT plots, the seedbed preparation was done using shallow harrowing after ploughing conducted in 2018, 14 days before sowing, and in 2020, at the beginning of November 2019. Harvest following by ploughing (on the tilled plots) were carried out in August in both years 2019 and 2020. The cover crops were sown on no-till plots immediately after the harvest in August 2018 and 2019. A species-diverse mixture Greening 5, consisting of Phacelia tanacetifolia, Trifolium alexandrinum, Vicia sativa, Pisum sativum, and Fagopyrum esculentum, was sown on the AT plots in sowing rate 35 kg ha−1 with the addition of Camelina sativa (3 kg ha−1), Linum usitatissimum (3 kg ha−1), and Brassica juncea (1 kg ha−1). Sowing of cover crops was done directly into stubble and straw mulch with the STP300 (2018, after winter rye harvest) and Vaderstad Rapid 300 (2019, after winter wheat harvest).
The effect of N nutrition was studied at three levels: control without N fertilizer application (N0), 50% (N50), and 100% (N100) of maximum dose. Maximum dose for winter wheat was 140 kg N ha−1 and for spring barley 100 kg N ha−1. N fertilization in the form of calcium nitrate was conducted in two doses in 2019 (12 March—tillering stages and 29 April—beginning of stem elongation) and in one dose in 2020 (4 April—after sowing). Such N fertilization regime represents the minimum splitting of N applications for typical agronomic practice in winter wheat and spring barley, respectively. Biochar, compost, and composted biochar were incorporated into the soil by split application on 15 March 2017, 26 September 2017, and 10 October 2018 in the total dose of 9, 90, and 90 t ha−1, respectively. The external application of biochar was adjusted to the target amount of 9 Mg ha−1, which is considered to be sufficient for stimulation of crop production without possible negative impacts associated with high doses (Baronti et al., 2010). The target dosage of composted biochar 90 Mg ha−1 was adjusted according to the 10% share of biochar in the resulting composted biochar. The target compost dosage was than derived from the applied composted biochar dosage. The split application aimed to achieve easier soil organisms’ adaptation to external C amendment.
Soil CO2 Efflux Measurements
Soil CO2 efflux was measured between 10 a.m. and 1 p.m. from April till October in monthly intervals in 2019 and biweekly intervals in 2020. The measurements were carried out on three replications (plots) of the selected combination of treatments, summarized in Table 1.
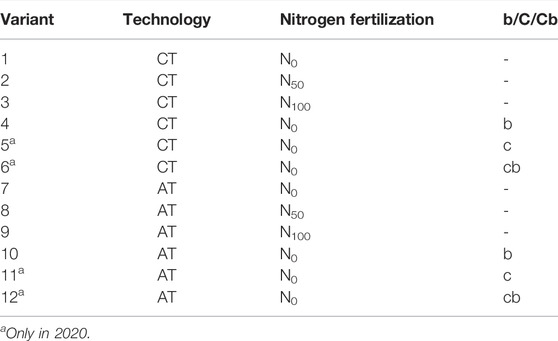
TABLE 1. Combination of treatments chosen for soil CO2 efflux measurements–conventional (CT)/adaptation (AT) technology; doses of 0, 50, and 100% of maximum nitrogen fertilizer dose (N0, N50, and N100), and application of biochar (b), compost (c), or composted biochar (cb).
Soil CO2 efflux (Rs) was measured using two identical non-steady-state through-flow LI-8100 portable systems (Li-Cor, Lincoln, NE, United States) and custom-made cylindrical chambers (diameter 10 cm, height 12 cm) placed on PVC collars. The collars (10 cm in diameter and 5 cm in height) were inserted into the soil to protrude 2.5 cm above the soil. Three collars were installed between crop rows in the middle of each plot in line with the approximate 0.5 m spacing at least 24 h before the first measurement in the spring and after ploughing. Each measurement started 10 s after chamber closure; CO2 concentration was measured repeatedly at intervals of 1 s for 1 min. CO2 efflux was calculated using a linear fit of this continuous increase in CO2 concentration.
The measurement dates were 18 April, 13 May, 6 June, 9 July, 15 August, and 12 September 2019, which corresponds to DOY 108, 133, 157, 190, 227, and 255, respectively; and 17 April, 4 May, 18 May, 1 June, 15 June, 1 July, 15 July, 30 July, 27 August, 8 September, and 8 October 2020, which corresponds to DOY 108, 139, 153, 167, 183, 197, 212, 240, 252, and 282, respectively.
Ancillary Measurements
Soil temperature was measured on each plot during soil CO2 efflux measurement in a depth of 1.5 cm using a penetrate thermometer TPD32 (Omega, Stamford, CT, United States).
During the experiment, soil water content was continually monitored every 60 min using TDR soil moisture sensor (CS650, Campbell Scientific Inc, Logan UT, United States) in depth 0–30 cm connected to the IoT unit. Due to technical problems, SWC measurements started in 2020. Precipitation was measured by a rain gauge Rain-O-Matic Meteorological (Pronamic, Denmark).
Soil Sampling and Analyses
Soil sampling was conducted in September 2020. Five subsamples from the topsoil (0–20 cm) were taken from each plot using Edelman soil auger of 7 cm diameter (Eijkelkamp Soil and Water, Netherland), thoroughly mixed, and dried at room temperature. Dry sample was homogenized using cutting mill SM-200 (Retsch GmbH, Germany) with a sieve of 2 mm. All chemical analyses were done in the laboratories of company Laboratoř Morava, Czech Republic. Cox was determined spectrophotometrically at λ = 590 nm as trivalent chromium, which is formed by the reduction in hexavalent chromium in a mixture of K2Cr2O7 and H2SO4. Total N was analysed using Kjeldahl method by titration of excess H2SO4. pH/H2O was determined potentiometrically in aqueous suspension (for details, see Pospíšilová et al. (2016).
Data Analyses
Effect of individual treatments (no-till, fertilization, biochar, and/or compost amendment, as well as drought) on Rs and parameters from soil analyses were determined by using four-way ANOVA followed by the Fischer’s LSD post hoc test. The analyses were done separately for each year and also for the effect of N fertilization (together with the effect of site, technology, and the date of measurement) and the effect of C amendment (together with the effect of site, technology, and the date of measurement). The analysis was conducted in the software Statistica 12 (StatSoft, Tulsa, OK, United States).
Principal component analysis (PCA) was performed using the software CANOCO 5 (Microcomputer Power, Ithaca, NY, United States) in accordance with Šmilauer and Lepš (2014). The categorical variables (C amendment, site, and crop/year) for the analysis were converted to dummy variables, which enabled the analysis of the direction of the effect on soil CO2 efflux and the interactive effects of factors.
To estimate the effect of studied agricultural practices on the seasonal amount released C through soil CO2 efflux, the measured Rs was considered to represent daily Rs of the measurement date. During this time, the temperature was about 3–4°C higher than daily soil temperature mean at around 10 a.m. (data not shown). Therefore, we may expect some overestimation of the results. Rs was then interpolated between 2 consecutive measurement days. The seasonal amount of released C was calculated for each plot and the effect of individual practices was tested against plots without treatment using paired t-test. Paired t-test was also used to test the effect of N fertilization and C amendment relative to the control.
Results
Micrometeorological Conditions
Total precipitation between April and September was 427 and 585 mm in 2019 and 2020, respectively (Figure 1). The precipitation for this period was higher by 4% in 2019 and by 43% in 2020 in comparison with long-term average 2000–2020 (409 mm). Both years were typical with extremely low precipitation in April (17.5 and 9.5 mm, respectively). In summer 2020, higher precipitation was observed compared to summer 2019. According to SWC measurements at about 25 km distant crop field site with similar SWC dynamic in 2020, it was evident that the low summer precipitation in 2019 resulted in gradual drying of the soil to the levels around 10% (data not shown). The measurements of SWC in 2020 showed no decrease below 25% over the whole period of measurements.
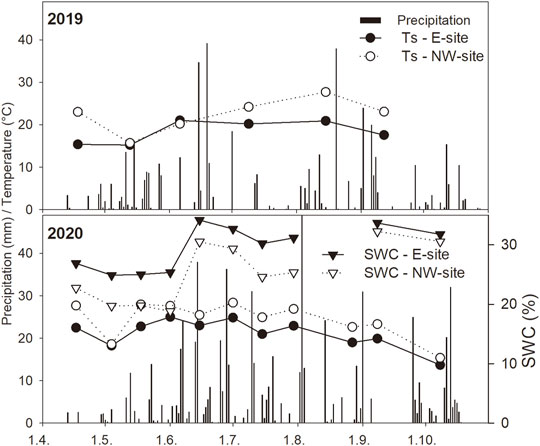
FIGURE 1. Daily precipitation and mean soil temperature (Ts) and soil water content (SWC) measured during soil CO2 efflux measurement campaigns at two sites (E and NW).
The mean soil temperature measured during soil CO2 efflux measurement campaigns ranged between 14 and 25°C at the E-site and between 15 and 28°C at the NW-site. Soil temperature at the NW-site was 0–7.5°C higher compared to the E-site as the measurements at the NW-site were carried out later in the measurement day.
Soil Analyses
Mean values of oxidizable carbon (Cox) ranged between 1.09 and 1.48%, mean total N between 1.28 and 1.73%, and mean pH between 6.1 and 7.0 (Table 2). Although no overall statistically significant effect of AT on the studied soil parameters was found, the technology showed significant interactive effects, particularly when combined with carbon amendment. Statistical analyses revealed significant effects of biochar amendment combined with AT at the E-site and compost and composted biochar with AT at the NW-site on Cox. Compost and composted biochar had also a positive effect on an increase in total N, particularly at the E-site. At the NW-site, there was a significant increase in total N found only when compost application was combined with AT. Similar to Cox and total N, pH/H2O also showed positive effects of compost and composted biochar application, which was observed at both sites. N fertilization increased the total N content and partly also Cox, but the effects of N fertilization were mostly statistically insignificant.
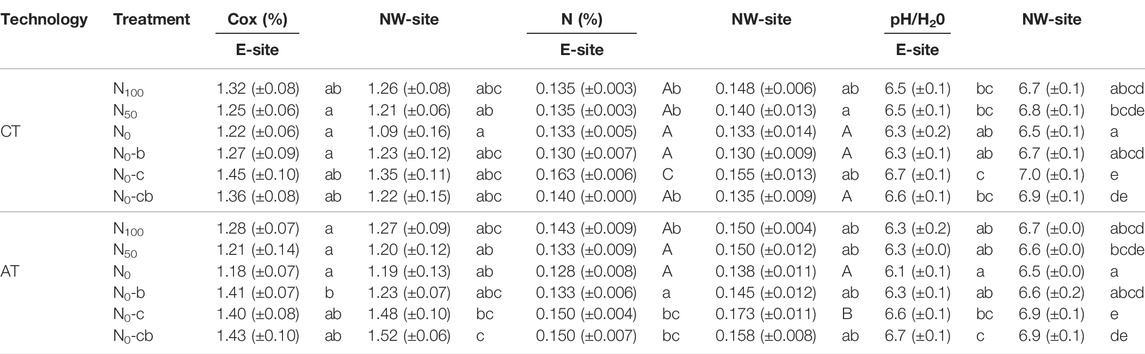
TABLE 2. Means (±SE) of oxidizable carbon (Cox), total nitrogen (N), and pH/H2O in the topsoil (0–20 cm) sampled in September 2020. CT–conventional technology with ploughing, AT–adaptation technology with no-till sowing into cover crop mulch, N0—without nitrogen fertilization, N50—50% of the maximum nitrogen dose, N100—maximum nitrogen dose, b–biochar, c–compost, cb–composted biochar. Letters in columns indicate homogeneous groups of two-way ANOVA post hoc test (Fischer’s LSD, p ≤ 0.05) for each site separately.
Overall Soil CO2 Efflux (Rs)
Rs generally ranged between 0.8 and 6.0 µmolCO2 m−2 s−1 and was highly varied over the two seasons confirmed by four-way ANOVA, presented later in this chapter. This Rs variability was connected to micrometeorological conditions with the lowest Rs in April (Figure 2). Then, Rs increased till June but the following course differed between years. While in 2020, Rs had a gradually decreasing trend till the end of the season, in June 2019 there was a fast drop in Rs as a result of severe lack of precipitation.
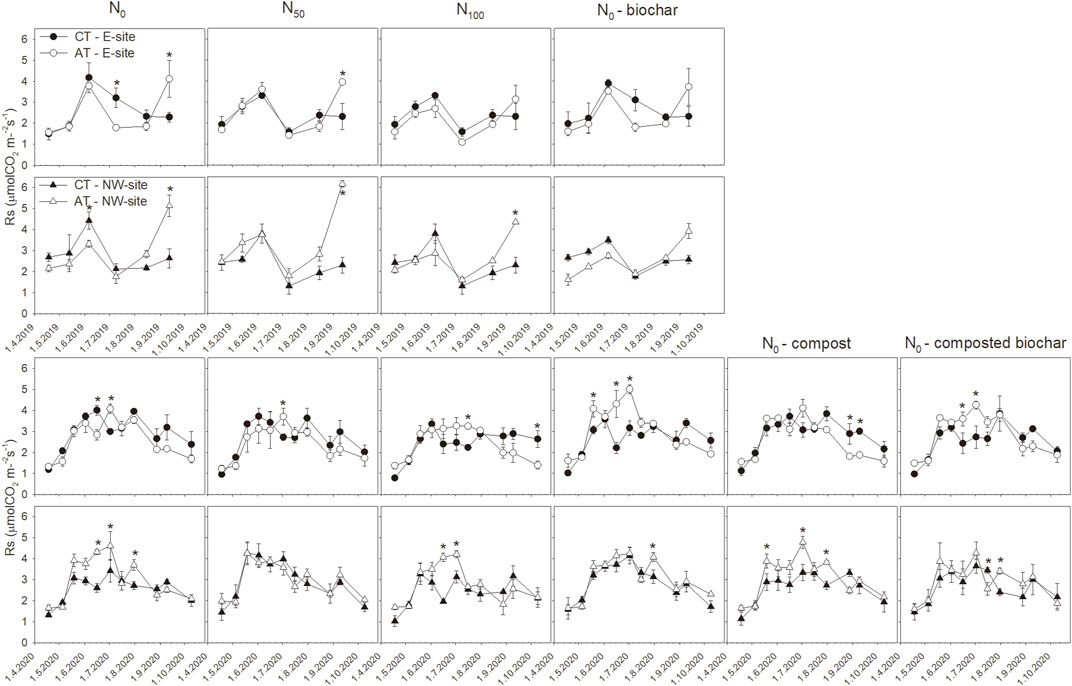
FIGURE 2. Seasonal dynamics of soil CO2 efflux (Rs) in the years 2019 and 2020 at the E-site (circles) and the NW-site (triangles), for variants with different nitrogen fertilization (N0—without nitrogen fertilization, N50—50% of the full nitrogen dose, and N100—full nitrogen dose) and with different carbon amendments (biochar, compost, and composted biochar), and statistical comparison of the technology effect (CT–conventional technology with ploughing–full symbols, and AT–adaptation technology with no-till sowing into cover crop mulch–open symbols). Means (points) and standard errors (error bars) are presented. Asterisks indicate significant differences in Rs for a given date of measurement between CT and AT (Fischer’s LSD post hoc test, p ≤ 0.05).
Estimated seasonal amount of released C from soil on the control plots (under CT, no N fertilization, and without C amendment) was 4.3 and 5.0 t C ha−1 for periods 18 April—12 September and 17 April—8 August in 2019 and 2020, respectively. AT had no effect on seasonal Rs in 2019, while it resulted in an increase in Rs by 5% compared to CT (Figure 3). In contrast to 2020, N50 treatment increased seasonal Rs in 2019 by 3% due to an increase in Rs in the beginning of the season compared to N0. Only N100 treatment resulted in a significant decrease in Rs in both years (p < 0.05) by 13 and 5% in 2019 and 2020, respectively. Despite no clear temporal pattern of the effect on Rs, biochar resulted in a decrease in seasonal Rs in 2019 by 7%. On the contrary, C amendment resulted in an increase in the seasonal Rs by up to 9% in 2020.
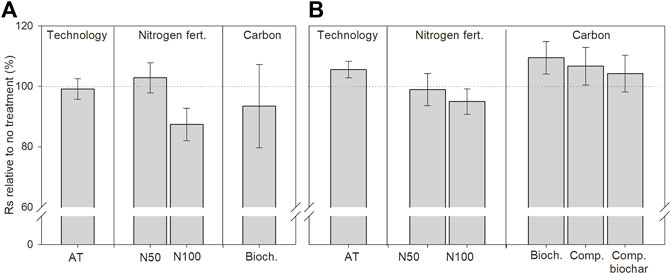
FIGURE 3. Relative effect of agriculture practices on the seasonal sum of CO2 efflux (Rs); (A) season 18 April–12 September 2019; (B) season 7 April–4 October 2020. Effect of technology: adaptation technology with no-till sowing into cover crop mulch (AT) relative to conventional technology with ploughing; effect of nitrogen fertilization: N50 (50% of the full nitrogen dose) and N100 (full nitrogen dose) relative to no nitrogen fertilization; and effect of carbon amendment (biochar, compost, and composted biochar) relative to no carbon amendment treatment.
The four-way ANOVA for the effect of N fertilization (Table 3) revealed besides significant effect of N, site, and date of measurement (DOY) also significant interactions between DOY × site, DOY × technology, site × technology, and DOY × site × technology in both years. The same analysis conducted for the C amendment effect showed a significant effect of DOY and interaction between DOY × technology in both years. For this analysis also, the significant effect of technology was found in 2020.
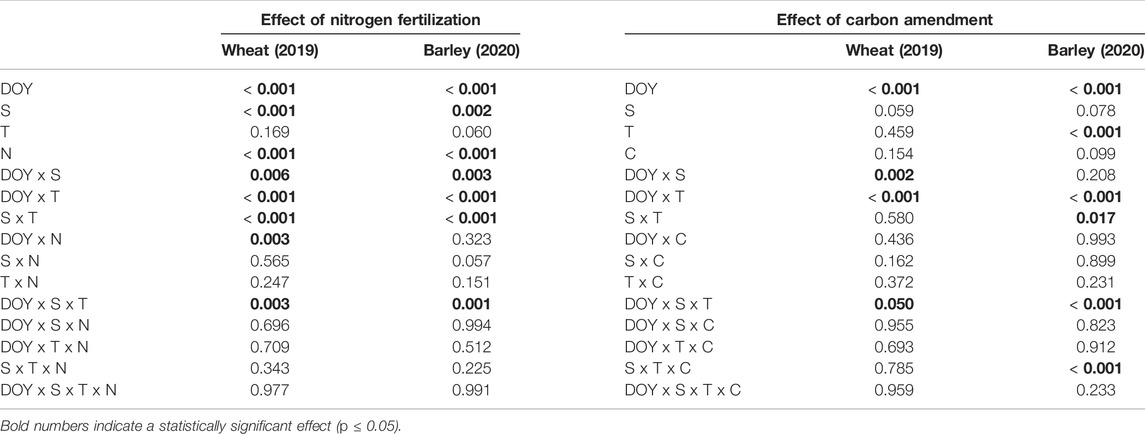
TABLE 3. Results of ANOVA for the effect of different parameters and their interactions: day of year (DOY), site (S), technology (T), nitrogen fertilization (N), and carbon amendment (C). Bold numbers indicate a statistically significant effect (p ≤ 0.05).
PCA analysis showing the associations between factors used in the experiment and Rs showed the major negative effect of N fertilization and generally positive effects of C amendment, which was highest for composted biochar (Figure 4). On the other hand, low effects on Rs were evident from PCA for site and technology.
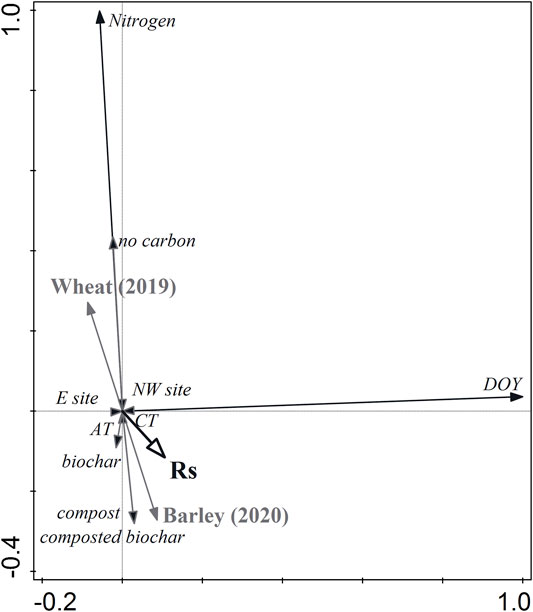
FIGURE 4. Biplot diagram presenting the results of principal component analysis (PCA). The angle between arrows indicates direction of the relationship (positive, negative, or no relationship), and the length of arrows indicates the strength of correlation. The explained cumulative variability by principal components 1 and 2 (axes x and y) was 99.9%. The studied parameters were as follows: soil CO2 efflux (Rs), day of year (DOY) of Rs measurement, adaptation technology (AT) with no-till sowing into cover crop mulch, conventional technology (CT) with ploughing, nitrogen fertilization (nitrogen), external carbon amendment (no carbon, biochar, compost, and composted biochar), the site (E-site and NW-site), and the crop (wheat and barley).
Effect of Adaptation Technology and Interactions With N Fertilization and C Amendment
During the season 2019, significant differences in Rs between CT and AT (Rs was higher in CT compared to AT) were found in N0 treatment in June and July for NW-site and E-site, respectively (Figure 5). The significant differences between AT and CT (Rs was higher in AT compared to CT) were also evident in September (after ploughing) for all N treatments at the NW-site, and also for N0 and N50 treatments at the E-site. In 2020, Rs in AT was significantly higher during June and the beginning of July at the NW-site, especially for N0 and N100 treatments. At the E-site, the differences between CT and AT were variable during this period, showing the shift of the Rs peak for AT to later measurements (July), while in CT the Rs peak was observed mostly at the beginning of June. However, this was significant only for the E-site and the N0 treatment after harvest in 2020 (Figure 2).
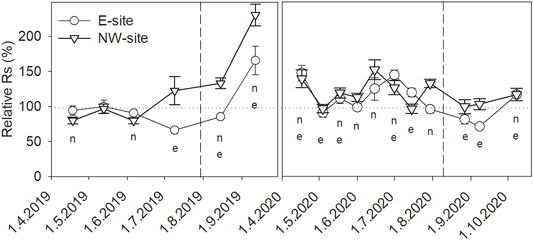
FIGURE 5. Mean (±SE) soil CO2 efflux (Rs) under adaptation technology (with no-till sowing into cover crop mulch) relative to conventional technology with ploughing at two experimental sites (E-site and NW-site) during 2 consecutive years 2019 and 2020. Dashed lines show the approximate timing of harvest. Letters e and n indicate a statistically significant difference (Fischer’s LSD post hoc test, p ≤ 0.05) at the E-site and the NW-site, respectively.
When comparing the effect of C amendment on Rs dynamics, the application of biochar reduced the dynamic fluctuations of Rs during the vegetation, especially on the NW-site in both years. On the contrary, biochar and composted biochar increased the differences between CT and AT during June and July (higher Rs in AT) compared to control at E-site.
When combining all fertilization and C amendment treatments (Figure 5), the largest difference between CT and AT was observed in September 2019 when it amounted to 84 and 136% at the E-site and NW-site, respectively. In 2020, Rs before harvest and shortly after harvest were mostly higher in AT, reaching up to 152% of CT. While no difference in Rs between AT and CT was observed after harvest at the NW-site, tillage significantly increased Rs in CT at the E-site. During this period, Rs in AT reached 81 and 71% of Rs in CT at the end of August and beginning of September, respectively.
Effect of Inorganic N Fertilization
In 2019, wheat fertilization with 70 kg N ha−1 (N50) increased Rs significantly from April to June compared to unfertilized plots (N0) (Figure 6). The largest increase was observed on 13 May when Rs on the N50 plots amounted to 152 and 136% of that on the N0 plots at E-site and NW-site, respectively. Then, we observed a rapid decrease in Rs on the N50 plots, and at the beginning of July, it amounted to only 74 and 87% of that on the N0 plots at the E-site and NW-site, respectively. Later in the season, no differences in Rs between N0 and N50 plots were observed.
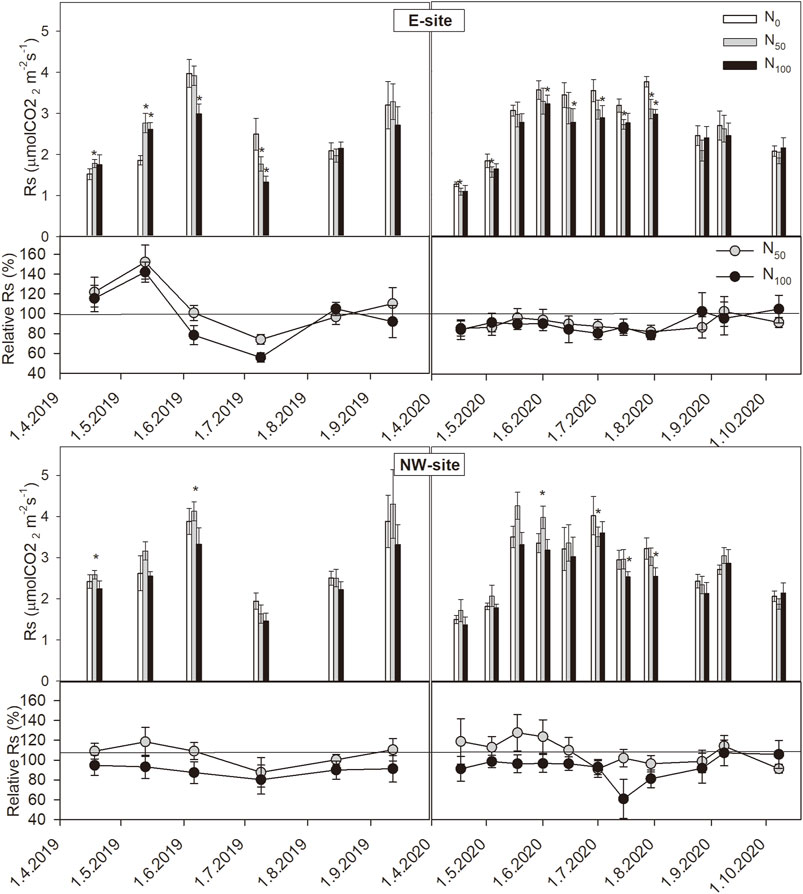
FIGURE 6. Mean (±SE) soil CO2 efflux (Rs) on plots with three variants of added fertilizer (N0—without nitrogen fertilization, N50—50% of the full nitrogen dose, and N100—full nitrogen dose), and soil CO2 efflux in N50 and N100 relative to N0 (mean ± SE) at two experimental sites (E-site and NW-site) during 2 consecutive years 2019 and 2020. Asterisks indicate a significant difference in soil CO2 efflux on plots with fertilizer (N50 or N100) from that on N0 plots (Fischer’s LSD post hoc test, p ≤ 0.05).
At the E-site, fertilization with 100% of maximum N dose resulted in an increase in Rs during the first two measurement campaigns in 2019, being statistically significant on 13 May when reaching 142% of that of N0. During June and July, Rs in N100 significantly decreased compared to N0 by 78 and 56%, respectively. Similar to N50, no significant differences between N100 and N0 were observed for the rest of the experimental season. At the NW-site, Rs in N100 plots tended to be lower than in N0 for the whole year 2019. The largest decrease was recorded in June and July (87 and 80% relative to N0, respectively), but the statistical significance was not confirmed at any date.
In 2020, we observed a different trend in Rs on the N50 plots between the two sites. At the E-site, Rs on N50 plots tended to reach up to 83% of Rs on the N0 plots since the beginning of measurements in April. At the NW-site, on the contrary, Rs on N50 plots was higher compared to N0 till the beginning of June, reaching up to 127% on N0. Later in the season, no significant difference between N50 and N0 was confirmed.
Fertilization with the maximum N dose (N100) decreased Rs at both sites since the first measurement in April 2020. The decrease was the highest between the middle of June and end of July at the E-site and between the middle of July and end of July at the NW-site. Rs ranged between 79 and 87%, and between 73 and 81% relatively to N0 at the E-site and NW-site, respectively. No significant effect of fertilization was observed after harvesting on the N100 plots at both sites.
Effect of C Amendment
In both years, the relative effect of C amendment showed high variability (Supplementary Figure S2). Therefore, no significant effect of biochar on Rs was confirmed in 2019. Rs on the plots with biochar amendment amounted to between 96 and 116% and between 81 and 110% of that on untreated plots at the E-site and NW-site, respectively.
Similarly, no significant effect of any investigated treatment (biochar, compost, and composted biochar) was observed in 2020. Rs on the plots with C amendment amounted to between 89 and 119% and between 78 and 124% of that on untreated plots at the E-site and NW-site, respectively.
Discussion
Effect of Adaptation Technology
We observed variable response of Rs to adaptation technology (AT) during the 2 study years, which was particularly evident in the changes in the Rs dynamics during the season. During the first year, Rs in AT tended to be lower before and higher after harvest compared to the CT. In the second year, the trend was the opposite. No-till management is adopted to reduce soil degradation from erosion (Derpsch et al., 2010) and to increase water retention (Blanco-Canqui and Ruis, 2018). According to many previous studies, no-till management increased soil C content and led to a reduction in CO2 emissions (Palm et al., 2014; Ogle et al., 2019). Higher CO2 emissions from tilled fields are related to mechanical changes in the soil profile, creating more favourable conditions for the organic matter oxidation and mineralization processes. This is in accordance with the Rs increase after harvest in the second year of our experiment.
The effect of AT in our experiment, however, combined no-till with cover crop cultivation after main crop harvest. As cover crops may increase Rs through rhizosphere respiration, these two practices may have opposite effects on Rs resulting in mutual compensation. The role in the different responses of Rs to ploughing in the 2 experimental years can also play different water availability in those particular seasons. Zapata et al. (2021) observed a greater SWC decrease in CT during dry weather compared to no-till technology. As Rs highly correlates with SWC under low SWC levels (Yuste et al., 2003), we may assume that the higher soil water retention in AT under dry conditions in 2019 increased Rs compared to CT. On the other hand, in 2020, with relatively better water availability after harvest (Figure 1), AT could reduce Rs because of the reduction in microbial activity and organic C decomposition due to air limitation (Buragienė et al., 2019).
Desired increased C content in AT was expected both through reduced soil respiration and new organic matter input to the soil during cover crop cultivation through the transport of new assimilates to the rhizosphere and leaving plant residues (Cong et al., 2015). This can, however, contribute in turn to the stimulation of microbial decomposition and thus also to increasing CO2 emissions. However, we did not observe any significant increase in soil Cox content during the soil sampling in the autumn of the second year of Rs measurements despite yearly biomass input from the cover crops, but frequently higher Rs in AT during 2020 (Figure 5).
Even in the conditions when AT increases Rs, the effect of AT may be positive for C sequestration (only part of organic C stored by cover crop cultivation is decomposed and released from soil by respiration) and also for making other nutrients available for the following crops. Therefore, also total C balance and crop yields need to be considered when evaluating the adaptation technology effect. Meta-analysis of Pittelkow et al. (2015) showed that no-till reduces yields, yet this response is variable, and under certain conditions, no-till can produce equivalent or greater yields than conventional tillage. The authors also suggested that no-till may become an important climate change adaptation strategy for dry regions.
Effect of N Fertilization
N fertilization is performed to increase the crop yield (Holub et al., 2020). However, N also highly affects the activity, diversity, and composition of microbes in the soil (Zhou et al., 2017), and thus also soil respiration and, subsequently, investigated CO2 emissions to the atmosphere.
We observed that N fertilization resulted in an increase in Rs at the beginning of 2019. Such effect is usually connected with stimulation in microbial activity due to accessible N supply. Moreover, N inputs can stimulate more microbial activity (priming effect) connected with mining for other nutrients (i.e. C), especially in nutrient-poor soils (Dijkstra et al., 2013). An increase in soil CO2 efflux after fertilization was in arable lands observed, for example, by Lamptey et al. (2018) and Liu Q. et al. (2016). However, conflicting results have been reported in other literature (Ni et al., 2012; Li et al., 2018). In addition, a meta-analysis of Zhou et al. (2014) on 138 cropland experiments showed that N fertilization significantly increased soil respiration by 12.4%. This trend was caused mainly by an increase in root respiration, while the response of heterotrophic respiration on N addition was not significant. An increase in root respiration can be connected with plant investment to root growth due to higher water demand for the increased photosynthesis induced by N addition (Qi et al., 2019). According to De Giorgio and Fornaro (2012) and Chen et al. (2020), N addition increases root biomass, especially in the shallow soil layers in wheat and cotton fields, while there was a decrease in deep root biomass. However, we observed higher soil respiration in fertilized plots only at the beginning of the growing season, when the plants and the proportion of root respiration were small, and primarily the microbial respiration was responsible for the observed trend.
Later in the growing season, inhibition of soil CO2 efflux was observed in N fertilized plots during the vegetation period. Such a response to N fertilization was observed also in other studies both in situ (Janssens et al., 2010) and in soil incubation experiments (Ramirez et al., 2012; Zang et al., 2016). Adding N can reduce soil CO2 efflux in several ways. High-level doses of N addition can result in soil acidification, which limits plant and root growth and inhibits the activities of soil microorganisms (Ramirez et al., 2012). We did not observe lower pH on the fertilized plots during the soil sampling in autumn 2020. The decrease in pH, however, can be only temporary as shown by Dong et al. (2022) and can return to an initial state in a few months. N additions can also directly inhibit enzymes needed for the decomposition of recalcitrant C, thereby reducing overall microbial activity (Gallo et al., 2004). Moreover, increased N availability can cause the lack of microorganisms needs to “mine” soil organic matter to obtain N, reducing overall microbial activity (Craine et al., 2007).
It has been confirmed that N fertilization alters microbial composition or decreases microbial biomass (Ramirez et al., 2012; Li et al., 2019). Decrease in microbial biomass or altered communities that are less capable of decomposing more recalcitrant soil C were in previous studies accompanied by lower Rs (Treseder, 2008; Ramirez et al., 2012), but also no effect on Rs was observed (Allison et al., 2008; Li et al., 2010).
The response to fertilization can also depend on the amount of fertilizer. We observed mostly an increase in Rs on N50 plots at the beginning of the growing season compared to N0, while Rs on N100 plots was not affected or the increase was smaller. When Rs inhibition on fertilized plots prevailed, the inhibition was strongest on N100 plots. Similarly, Ramirez et al. (2010), Liu Q. et al. (2016), and Mason-Jones et al. (2018), who found a reduction in Rs after fertilization, observed a more pronounced Rs reduction on plots with a larger dose of mineral N fertilizer. However, a contrary trend, when the N dose increased Rs, was also observed (Lamptey et al., 2018). For example, Peng et al. (2010) found that the amount of N increased Rs in the order medium > high > low.
It is evident that the response of Rs to N fertilization is not uniform throughout the studies. The response may vary with soil properties including, for example, the amount of soil N content before fertilizer application. Janssens et al. (2010) and Sanyal et al. (2021) observed that Rs decreased after fertilization of N non-limited soils, whereas it increased in soils with alow level of N. According to Ding et al. (2010), the stimulatory or inhibitory effect of N fertilization on Rs depends substantially on the concentration of decomposable soil organic C in soils.
Effect of Treatment With Biochar, Compost, and Composted Biochar
In 2019, we did not observe any effect of biochar on Rs. No effect of biochar on soil respiration was also observed by Lu et al. (2014), Liu Q. et al. (2016), and Ge et al. (2020). Biochar is highly resistant to microbial decomposition (Wang et al., 2016); therefore, it remains in the soil over a long period. No effect or even decrease in Rs after biochar application (Case et al., 2014; Shen et al., 2017) is another desired attribute from the perspective of CO2 emissions and losses of soil organic matter. Moreover, biochar amendment can contribute to the enhancement of C stock in the soil.
However, biochar large surface area and volume of pores may provide a significant habitat for microbes (Quilliam et al., 2013). Moreover, biochar improves also other soil properties, such as soil’s structural quality, nutrient availability, or water retention capacity (Blanco-Canqui, 2017), which can create a favourable environment for soil microorganisms and increase their respiration activity, as observed by, e.g. Smith et al. (2010), Troy et al. (2013), and Shah et al. (2017). Those studies were, however, performed under laboratory incubations when the soil was ground into fine particles. Thereby, the C substrates were not physically protected, and they became highly accessible to soil microorganisms (Liu X. et al., 2016), while under field conditions, the organic substances are physically protected in macroaggregates, which limits their accessibility by soil microbes (Chevallier et al., 2004).
Despite an increase in Cox and N on the plots with compost and composted biochar treatments, we observed no difference in Rs from the control plots in the second year after application, which indicates no long-term effect of compost and composted biochar on Rs at our sites. Compost represents soil enrichment with new organic matter to increase soil quality, soil C content, and amount of released nutrients. Newly available organic C can be rapidly decomposed by microorganisms resulting in an increase in Rs as observed, e.g. by Wilson et al. (2018) or Chen et al. (2019). The effect of compost on Rs also depends on the composition and quality of the compost, which means, e.g. amount of dissolved organic C, lignin, minerals, or C:N ratio (Chen et al., 2019; Ma et al., 2019). The temporality of the compost amendment on Rs was also confirmed in previous studies. For example, Ginting et al. (2003) found no difference in Rs between control and composted plots 4 years after the treatment. The authors, however, confirmed simultaneously residual positive effect on soil quality (higher microbial biomass and mineralizable N). No effect of compost on Rs after 2 years of its application indicates a little effect on CO2 emissions to the atmosphere, but a persistent positive effect on soil quality and subsequently crop production is assumed.
Composted biochar carries all advantages of biochar but with the increased amount of nutrients, which leads to improving soil quality (Schulz et al., 2013). As composting loads biochar with easily bioavailable C and N, it increases their potential fertilizer value and, therefore, might enhance not only soil CO2 emissions but also those of CH4 and N2O (Borchard et al., 2014). As we observed no statistically significant effect of biochar, compost, and composted biochar on Rs accompanied by increase in Cox, we can assume that the three external C amendment treatments contribute to C sequestration in the soil of studied arable fields.
Conclusion
This study examined the effect of different management on soil CO2 efflux from arable soil, which accompanies soil organic matter degradation. As rapid loss of soil organic matter and high CO2 emissions to the atmosphere are undesirable effects, they must be considered when adopting different practices to increase crop yield. Although it was expected that adaptation technology would reduce Rs, the effect was not uniform during the 2 experimental years. We suggest that the effect of no-till can be modified with applied cover crops and actual weather conditions. Inorganic N fertilizer application resulted in some cases in the increase in Rs at the beginning of the growing season. However, we observed mostly no effect or reduction in Rs, especially on the plots with the highest N dose. Biochar, compost, and composted biochar had no effect on Rs. Therefore, we may assume that these management practices might be suitable for preservation or even increase in soil organic matter in the arable soil.
Data Availability Statement
The raw data supporting the conclusion of this article will be made available by the authors, without undue reservation.
Author Contributions
ED: field measurements, data analyses, writing of the original draft, and manuscript editing. HF: field measurements and manuscript writing. PH: meteorological data collection and manuscript writing. KK: statistical analyses and manuscript writing.
Funding
This research was supported by SustES—Adaptation strategies for sustainable ecosystem services and food security under adverse environmental conditions (CZ.02.1.01/0.0/0.0/16_019/0000797), and the used infrastructure belongs under the CzeCOS program (LM2015061) supported by the Ministry of Education, Youth, and Sports of the Czech Republic.
Conflict of Interest
The authors declare that the research was conducted in the absence of any commercial or financial relationships that could be construed as a potential conflict of interest.
Publisher’s Note
All claims expressed in this article are solely those of the authors and do not necessarily represent those of their affiliated organizations, or those of the publisher, the editors, and the reviewers. Any product that may be evaluated in this article, or claim that may be made by its manufacturer, is not guaranteed or endorsed by the publisher.
Supplementary Material
The Supplementary Material for this article can be found online at: https://www.frontiersin.org/articles/10.3389/fenvs.2022.920247/full#supplementary-material
References
Abideen, Z., Koyro, H.-W., Huchzermeyer, B., Gul, B., and Khan, M. A. (2020). Impact of a Biochar or a Biochar-Compost Mixture on Water Relation, Nutrient Uptake and Photosynthesis of Phragmites Karka. Pedosphere 30, 466–477. doi:10.1016/S1002-0160(17)60362-X
Alburquerque, J. A., Salazar, P., Barrón, V., Torrent, J., Del Campillo, M. D. C., Gallardo, A., et al. (2013). Enhanced Wheat Yield by Biochar Addition under Different Mineral Fertilization Levels. Agron. Sustain. Dev. 33, 475–484. doi:10.1007/s13593-012-0128-3
Allison, S. D., Czimczik, C. I., and Treseder, K. K. (2008). Microbial Activity and Soil Respiration under Nitrogen Addition in Alaskan Boreal Forest. Glob. Chang. Biol. 14, 1156–1168. doi:10.1111/j.1365-2486.2008.01549.x
Angás, P., Lampurlanés, J., and Cantero-Martínez, C. (2006). Tillage and N Fertilization: Effects on N Dynamics and Barley Yield under Semiarid Mediterranean Conditions. Soil Tillage Res. 87, 59–71. doi:10.1016/j.still.2005.02.036
Badu, E., Kaba, J. S., Abunyewa, A. A., Dawoe, E. K., Agbenyega, O., and Barnes, R. V. (2019). Biochar and Inorganic Nitrogen Fertilizer Effects on Maize (Zea Mays L.) Nitrogen Use and Yield in Moist Semi-Deciduous Forest Zone of Ghana. J. Plant Nutr. 42, 2407–2422. doi:10.1080/01904167.2019.1659347
Baronti, S., Alberti, G., Delle Vedove, G., di Gennaro, F., Fellet, G., Genesio, L., et al. (2010). The Biochar Option to Improve Plant Yields: First Results from Some Field and Pot Experiments in Italy. Ital. J. Agron. 5, 3–11. doi:10.4081/ija.2010.3
Biberdzic, M., Barac, S., Lalevic, D., Djikic, A., Prodanovic, D., and Rajicic, V. (2020). Influence of Soil Tillage System on Soil Compaction and Winter Wheat Yield. Chil. J. Agric. Res. 80, 80–89. doi:10.4067/s0718-58392020000100080
Blanco-Canqui, H. (2017). Biochar and Soil Physical Properties. Soil Sci. Soc. Am. J. 81, 687–711. doi:10.2136/sssaj2017.01.0017
Blanco-Canqui, H., and Ruis, S. J. (2018). No-Tillage and Soil Physical Environment. Geoderma 326, 164–200. doi:10.1016/j.geoderma.2018.03.011
Borchard, N., Spokas, K., Prost, K., and Siemens, J. (2014). Greenhouse Gas Production in Mixtures of Soil with Composted and Noncomposted Biochars is Governed by Char-Associated Organic Compounds. J. Environ. Qual. 43, 971–979. doi:10.2134/jeq2013.07.0290
Buragienė, S., Šarauskis, E., Romaneckas, K., Adamavičienė, A., Kriaučiūnienė, Z., Avižienytė, D., et al. (2019). Relationship between CO2 Emissions and Soil Properties of Differently Tilled Soils. Sci. Total Environ. 662, 786–795. doi:10.1016/j.scitotenv.2019.01.236
Case, S. D. C., Mcnamara, N. P., Reay, D. S., and Whitaker, J. (2014). Can Biochar Reduce Soil Greenhouse Gas Emissions from a Miscanthus Bioenergy Crop? GCB Bioenergy 6, 76–89. doi:10.1111/gcbb.12052
Chaghazardi, H. R., Jahansouz, M. R., Ahmadi, A., and Gorji, M. (2016). Effects of Tillage Management on Productivity of Wheat and Chickpea under Cold, Rainfed Conditions in Western Iran. Soil Tillage Res. 162, 26–33. doi:10.1016/j.still.2016.04.010
Chartres, C. J., and Noble, A. (2015). Sustainable Intensification: Overcoming Land and Water Constraints on Food Production. Food Sec. 7, 235–245. doi:10.1007/s12571-015-0425-1
Chen, J., Liu, L., Wang, Z., Zhang, Y., Sun, H., Song, S., et al. (2020). Nitrogen Fertilization Increases Root Growth and Coordinates the Root-Shoot Relationship in Cotton. Front. Plant Sci. 11, 880. doi:10.3389/fpls.2020.00880
Chen, Z., Xu, Y., Castellano, M. J., Fontaine, S., Wang, W., and Ding, W. (2019). Soil Respiration Components and Their Temperature Sensitivity under Chemical Fertilizer and Compost Application: The Role of Nitrogen Supply and Compost Substrate Quality. J. Geophys. Res. Biogeosci. 124, 556–571. doi:10.1029/2018JG004771
Chevallier, T., Blanchart, E., Albrecht, A., and Feller, C. (2004). The Physical Protection of Soil Organic Carbon in Aggregates: A Mechanism of Carbon Storage in a Vertisol under Pasture and Market Gardening (Martinique, West Indies). Agric. Ecosyst. Environ. 103, 375–387. doi:10.1016/j.agee.2003.12.009
Cong, W.-F., Hoffland, E., Li, L., Six, J., Sun, J.-H., Bao, X.-G., et al. (2015). Intercropping Enhances Soil Carbon and Nitrogen. Glob. Chang. Biol. 21, 1715–1726. doi:10.1111/gcb.12738
Craine, J. M., Morrow, C., and Fierer, N. (2007). Microbial Nitrogen Limitation Increases Decomposition. Ecology 88, 2105–2113. doi:10.1890/06-1847.1
De Giorgio, D., and Fornaro, F. (2012). Nitrogen Fertilization and Root Growth Dynamics in Durum Wheat. Ital. J. Agron. 7, 207–213. doi:10.4081/ija.2012.e29
Derpsch, R., Friedrich, T., Kassam, A., and Hongwen, L. (2010). Current Status of Adoption of No-Till Farming in the World and Some of its Main Benefits. Int. J. Agric. Biol. Eng. 3, 1–25. doi:10.3965/j.issn.1934-6344.2010.01.001-025
Dijkstra, F. A., Carrillo, Y., Pendall, E., and Morgan, J. A. (2013). Rhizosphere Priming: A Nutrient Perspective. Front. Microbiol. 4, 216. doi:10.3389/fmicb.2013.00216
Ding, W., Yu, H., Cai, Z., Han, F., and Xu, Z. (2010). Responses of Soil Respiration to N Fertilization in a Loamy Soil under Maize Cultivation. Geoderma 155, 381–389. doi:10.1016/j.geoderma.2009.12.023
Dong, Y., Yang, J.-L., Zhao, X.-R., Yang, S.-H., Mulder, J., Dörsch, P., et al. (2022). Seasonal Dynamics of Soil pH and N Transformation as Affected by N Fertilization in Subtropical China: An In Situ15N Labeling Study. Sci. Total Environ. 816, 151596. doi:10.1016/j.scitotenv.2021.151596
Dubey, R. K., Dubey, P. K., Chaurasia, R., Singh, H. B., and Abhilash, P. C. (2020). Sustainable Agronomic Practices for Enhancing the Soil Quality and Yield of Cicer Arietinum L. Under Diverse Agroecosystems. J. Environ. Manage. 262, 110284. doi:10.1016/j.jenvman.2020.110284
Faust, S., Koch, H.-J., and Joergensen, R. G. (2019). Respiration Response to Different Tillage Intensities in Transplanted Soil Columns. Geoderma 352, 289–297. doi:10.1016/j.geoderma.2019.05.023
Gallo, M., Amonette, R., Lauber, C., Sinsabaugh, R. L., and Zak, D. R. (2004). Microbial Community Structure and Oxidative Enzyme Activity in Nitrogen-Amended North Temperate Forest Soils. Microb. Ecol. 48, 218–229. doi:10.1007/s00248-003-9001-x
Ge, X., Cao, Y., Zhou, B., Xiao, W., Tian, X., and Li, M.-H. (2020). Combined Application of Biochar and N Increased Temperature Sensitivity of Soil Respiration but Still Decreased the Soil CO2 Emissions in Moso Bamboo Plantations. Sci. Total Environ. 730, 139003. doi:10.1016/j.scitotenv.2020.139003
Ginting, D., Kessavalou, A., Eghball, B., and Doran, J. W. (2003). Greenhouse Gas Emissions and Soil Indicators Four Years after Manure and Compost Applications. J. Environ. Qual. 32, 23–32. doi:10.2134/jeq2003.2300
Holub, P., Klem, K., Tůma, I., Vavříková, J., Surá, K., Veselá, B., et al. (2020). Application of Organic Carbon Affects Mineral Nitrogen Uptake by Winter Wheat and Leaching in Subsoil: Proximal Sensing as a Tool for Agronomic Practice. Sci. Total Environ. 717, 137058. doi:10.1016/j.scitotenv.2020.137058
Janssens, I. A., Dieleman, W., Luyssaert, S., Subke, J.-A., Reichstein, M., Ceulemans, R., et al. (2010). Reduction of Forest Soil Respiration in Response to Nitrogen Deposition. Nat. Geosci. 3, 315–322. doi:10.1038/ngeo844
Lamptey, S., Li, L., and Xie, J. (2018). Impact of Nitrogen Fertilization on Soil Respiration and Net Ecosystem Production in Maize. Plant Soil Environ. 64, 353–360. doi:10.17221/217/2018-PSE
Li, J., Jian, S., Koff, J. P., Lane, C. S., Wang, G., Mayes, M. A., et al. (2018). Differential Effects of Warming and Nitrogen Fertilization on Soil Respiration and Microbial Dynamics in Switchgrass Croplands. GCB Bioenergy 10, 565–576. doi:10.1111/gcbb.12515
Li, L.-J., Zeng, D.-H., Yu, Z.-Y., Fan, Z.-P., and Mao, R. (2010). Soil Microbial Properties under N and P Additions in a Semi-arid, Sandy Grassland. Biol. Fertil. Soils 46, 653–658. doi:10.1007/s00374-010-0463-y
Li, W., Wang, J., Li, X., Wang, S., Liu, W., Shi, S., et al. (2019). Nitrogen Fertilizer Regulates Soil Respiration by Altering the Organic Carbon Storage in Root and Topsoil in Alpine Meadow of the North-Eastern Qinghai-Tibet Plateau. Sci. Rep. 9, 13735. doi:10.1038/s41598-019-50142-y
Liu, Q., Wang, R., Li, R., Hu, Y., and Guo, S. (2016a). Temperature Sensitivity of Soil Respiration to Nitrogen Fertilization: Varying Effects between Growing and Non-Growing Seasons. PLoS One 11, e0168599. doi:10.1371/journal.pone.0168599
Liu, X., Zheng, J., Zhang, D., Cheng, K., Zhou, H., Zhang, A., et al. (2016b). Biochar Has No Effect on Soil Respiration across Chinese Agricultural Soils. Sci. Total Environ. 554-555, 259–265. doi:10.1016/j.scitotenv.2016.02.179
Lohila, A., Aurela, M., Regina, K., and Laurila, T. (2003). Soil and Total Ecosystem Respiration in Agricultural Fields: Effect of Soil and Crop Type. Plant Soil 251, 303–317. doi:10.1023/A:1023004205844
Lu, N., Liu, X.-R., Du, Z.-L., Wang, Y.-D., and Zhang, Q.-Z. (2014). Effect of Biochar on Soil Respiration in the Maize Growing Season after 5 Years of Consecutive Application. Soil Res. 52, 505–512. doi:10.1071/SR13239
Ma, M., Zang, Z., Xie, Z., Chen, Q., Xu, W., Zhao, C., et al. (2019). Soil Respiration of Four Forests along Elevation Gradient in Northern Subtropical China. Ecol. Evol. 9, 12846–12857. doi:10.1002/ece3.5762
Mason-Jones, K., Schmücker, N., and Kuzyakov, Y. (2018). Contrasting Effects of Organic and Mineral Nitrogen Challenge the N-Mining Hypothesis for Soil Organic Matter Priming. Soil Biol. Biochem. 124, 38–46. doi:10.1016/j.soilbio.2018.05.024
Mosier, A. R., Halvorson, A. D., Reule, C. A., and Liu, X. J. (2006). Net Global Warming Potential and Greenhouse Gas Intensity in Irrigated Cropping Systems in Northeastern Colorado. J. Environ. Qual. 35, 1584–1598. doi:10.2134/jeq2005.0232
Mukherjee, A., and Lal, R. (2013). Biochar Impacts on Soil Physical Properties and Greenhouse Gas Emissions. Agronomy 3, 313–339. doi:10.3390/agronomy3020313
Ni, K., Ding, W., Cai, Z., Wang, Y., Zhang, X., and Zhou, B. (2012). Soil Carbon Dioxide Emission from Intensively Cultivated Black Soil in Northeast China: Nitrogen Fertilization Effect. J. Soils Sediments 12, 1007–1018. doi:10.1007/s11368-012-0529-6
Ogle, S. M., Alsaker, C., Baldock, J., Bernoux, M., Breidt, F. J., McConkey, B., et al. (2019). Climate and Soil Characteristics Determine where No-Till Management Can Store Carbon in Soils and Mitigate Greenhouse Gas Emissions. Sci. Rep. 9, 11665. doi:10.1038/s41598-019-47861-7
Palm, C., Blanco-Canqui, H., DeClerck, F., Gatere, L., and Grace, P. (2014). Conservation Agriculture and Ecosystem Services: An Overview. Agric. Ecosyst. Environ. 187, 87–105. doi:10.1016/j.agee.2013.10.010
Pittelkow, C. M., Liang, X., Linquist, B. A., Van Groenigen, K. J., Lee, J., Lundy, M. E., et al. (2015). Productivity Limits and Potentials of the Principles of Conservation Agriculture. Nature 517, 365–368. doi:10.1038/nature13809
Pospíšilová, L., Vlček, V., Hybler, V., Hábová, M., and Jandák, J. (2016). “Standard Analytical Methods and Evaluation Criteria of Soil Physical, Agrochemical, Biological and Hygienic Parameters,” in Folia Universitatis Agriculturae et Silviculturae Mendelianae Brunensis (Brno: Mendelova univerzita v Brně), 123.
Qi, D., Hu, T., Song, X., and Zhang, M. (2019). Effect of Nitrogen Supply Method on Root Growth and Grain Yield of Maize under Alternate Partial Root-Zone Irrigation. Sci. Rep. 9, 8191. doi:10.1038/s41598-019-44759-2
Quilliam, R. S., Glanville, H. C., Wade, S. C., and Jones, D. L. (2013). Life in the 'Charosphere' - Does Biochar in Agricultural Soil Provide a Significant Habitat for Microorganisms? Soil Biol. Biochem. 65, 287–293. doi:10.1016/j.soilbio.2013.06.004
Ramirez, K. S., Craine, J. M., and Fierer, N. (2012). Consistent Effects of Nitrogen Amendments on Soil Microbial Communities and Processes across Biomes. Glob. Chang. Biol. 18, 1918–1927. doi:10.1111/j.1365-2486.2012.02639.x
Ramirez, K. S., Craine, J. M., and Fierer, N. (2010). Nitrogen Fertilization Inhibits Soil Microbial Respiration Regardless of the Form of Nitrogen Applied. Soil Biol. Biochem. 42, 2336–2338. doi:10.1016/j.soilbio.2010.08.032
Sánchez-Monedero, M. A., Cayuela, M. L., Sánchez-García, M., Vandecasteele, B., D’Hose, T., López, G., et al. (2019). Agronomic Evaluation of Biochar, Compost and Biochar-Blended Compost across Different Cropping Systems: Perspective from the European Project FERTIPLUS. Agronomy 9, 225. doi:10.3390/agronomy9050225
Sanyal, D., Wolthuizen, J., and Bly, A. (2021). Influence of Nitrogen Fertilization Rate on Soil Respiration: A Study Using a Rapid Soil Respiration Assay. Nitrogen 2, 218–228. doi:10.3390/nitrogen2020014
Schroder, J. L., Zhang, H., Girma, K., Raun, W. R., Penn, C. J., and Payton, M. E. (2011). Soil Acidification from Long-Term Use of Nitrogen Fertilizers on Winter Wheat. Soil Sci. Soc. Am. J. 75, 957–964. doi:10.2136/sssaj2010.0187
Schulz, H., Dunst, G., and Glaser, B. (2013). Positive Effects of Composted Biochar on Plant Growth and Soil Fertility. Agron. Sustain. Dev. 33, 817–827. doi:10.1007/s13593-013-0150-0
Shah, T., Khan, S., and Shah, Z. (2017). Soil Respiration, pH and EC as Influenced by Biochar. Soil Environ. 36, 77–83. doi:10.25252/se/17/51184
Shen, Y., Zhu, L., Cheng, H., Yue, S., and Li, S. (2017). Effects of Biochar Application on CO2 Emissions from a Cultivated Soil under Semiarid Climate Conditions in Northwest China. Sustainability 9, 1482. doi:10.3390/su9081482
Smith, J. L., Collins, H. P., and Bailey, V. L. (2010). The Effect of Young Biochar on Soil Respiration. Soil Biol. Biochem. 42, 2345–2347. doi:10.1016/j.soilbio.2010.09.013
Treseder, K. K. (2008). Nitrogen Additions and Microbial Biomass: A Meta-Analysis of Ecosystem Studies. Ecol. Lett. 11, 1111–1120. doi:10.1111/j.1461-0248.2008.01230.x
Troy, S. M., Lawlor, P. G., O' Flynn, C. J., and Healy, M. G. (2013). Impact of Biochar Addition to Soil on Greenhouse Gas Emissions Following Pig Manure Application. Soil Biol. Biochem. 60, 173–181. doi:10.1016/j.soilbio.2013.01.019
Wang, J., Xiong, Z., and Kuzyakov, Y. (2016). Biochar Stability in Soil: Meta‐analysis of Decomposition and Priming Effects. GCB Bioenergy 8, 512–523. doi:10.1111/gcbb.12266
Wilson, C., Zebarth, B. J., Burton, D. L., and Goyer, C. (2018). Short-Term Effects of Diverse Compost Products on Soil Quality in Potato Production. Soil Sci. Soc. Am. J. 82, 889–900. doi:10.2136/sssaj2017.10.0345
Xiao, L., Zhao, R., and Kuhn, N. J. (2019). Straw Mulching is More Important Than No Tillage in Yield Improvement on the Chinese Loess Plateau. Soil Tillage Res. 194, 104314. doi:10.1016/j.still.2019.104314
Yuste, J. C., Janssens, I. A., Carrara, A., Meiresonne, L., and Ceulemans, R. (2003). Interactive Effects of Temperature and Precipitation on Soil Respiration in a Temperate Maritime Pine Forest. Tree Physiol. 23, 1263–1270. doi:10.1093/treephys/23.18.1263
Zang, H., Wang, J., and Kuzyakov, Y. (2016). N Fertilization Decreases Soil Organic Matter Decomposition in the Rhizosphere. Appl. Soil Ecol. 108, 47–53. doi:10.1016/j.apsoil.2016.07.021
Zapata, D., Rajan, N., Mowrer, J., Casey, K., Schnell, R., and Hons, F. (2021). Long-Term Tillage Effect on With-In Season Variations in Soil Conditions and Respiration from Dryland Winter Wheat and Soybean Cropping Systems. Sci. Rep. 11, 2344. doi:10.1038/s41598-021-80979-1
Zhang, Q., Lei, H.-M., and Yang, D.-W. (2013). Seasonal Variations in Soil Respiration, Heterotrophic Respiration and Autotrophic Respiration of a Wheat and Maize Rotation Cropland in the North China Plain. Agric. For. Meteorol. 180, 34–43. doi:10.1016/j.agrformet.2013.04.028
Zhou, J., Jiang, X., Wei, D., Zhao, B., Ma, M., Chen, S., et al. (2017). Consistent Effects of Nitrogen Fertilization on Soil Bacterial Communities in Black Soils for Two Crop Seasons in China. Sci. Rep. 7, 3267. doi:10.1038/s41598-017-03539-6
Zhou, L., Zhou, X., Zhang, B., Lu, M., Luo, Y., Liu, L., et al. (2014). Different Responses of Soil Respiration and its Components to Nitrogen Addition Among Biomes: A Meta-Analysis. Glob. Chang. Biol. 20, 2332–2343. doi:10.1111/gcb.12490
Keywords: biochar, compost, conservation technology, no-till, soil respiration, tillage
Citation: Darenova E, Findurova H, Holub P and Klem K (2022) Soil CO2 Efflux Response to Combined Application of Adaptation Technologies, Nitrogen Fertilization, and External Carbon Amendment in Wheat and Barley Field. Front. Environ. Sci. 10:920247. doi: 10.3389/fenvs.2022.920247
Received: 14 April 2022; Accepted: 24 May 2022;
Published: 30 June 2022.
Edited by:
Ilan Stavi, Dead Sea and Arava Science Center, IsraelReviewed by:
Youchao Chen, Zhejiang A&F University, ChinaJianqiu Zheng, Pacific Northwest National Laboratory (DOE), United States
Copyright © 2022 Darenova, Findurova, Holub and Klem. This is an open-access article distributed under the terms of the Creative Commons Attribution License (CC BY). The use, distribution or reproduction in other forums is permitted, provided the original author(s) and the copyright owner(s) are credited and that the original publication in this journal is cited, in accordance with accepted academic practice. No use, distribution or reproduction is permitted which does not comply with these terms.
*Correspondence: Eva Darenova, ZGFyZW5vdmEuZUBjemVjaGdsb2JlLmN6