- 1Dauphin Island Sea Lab, Dauphin Island, AL, United States
- 2Department of Marine Sciences, School of Marine and Environmental Sciences, University of South Alabama, Mobile, AL, United States
- 3Nature Coast Biological Station, University of Florida, Cedar Key, FL, United States
- 4Division of Coastal Sciences, School of Ocean Science and Engineering, The University of Southern Mississippi, Ocean Springs, MS, United States
- 5Florida Fish and Wildlife Conservation Commission, Fish and Wildlife Research Institute, St. Petersburg, FL, United States
Drift macroalgae, often found in clumps or mats adjacent to or within seagrass beds, can provide additional food resources and habitat complexity, leading to increased animal abundance, but large concentrations can also inhibit faunal movements, smother benthic communities, and contribute to hypoxia, reducing nekton abundance. Despite its ubiquity, few studies have quantified drift macroalgal prevalence over large spatial scales or its effects on seagrass-associated nekton, hindering our understanding of the functional role of drift macroalgae in ecosystems. We quantified the relationship between drift macroalgal biomass and the seagrass-associated nekton community within five estuaries spanning 2000 km across the northern Gulf of Mexico. Overall, increases in macroalgal biomass within seagrass meadows significantly influenced community structure, increasing shrimp, crab, and fish abundances, but the effect varied by region. Relationships between species richness, diversity, organism size, and macroalgal biomass were not observed, suggesting that drift macroalgae provide additional habitat but not necessarily new niche space. Small nekton play a vital role in many local fisheries, providing valuable food resources for fish and invertebrates. Increased recruitment into macroalgae can benefit local fisheries by providing shelter and increased food resources, which may increase the survival, growth, and population size of recreationally and economically important species. While excess levels of drift macroalgae can negatively impact benthic plant and animal communities, particularly in eutrophic areas, the moderate levels observed during this survey were associated with positive effects on organismal abundance, suggesting that drift algal dynamics should be considered in habitat-based management strategies for coastal estuaries.
Introduction
Despite comprising less than 10% of the ocean area, shallow coastal habitats, such as seagrass meadows, salt marshes, mangroves, and oyster reefs, provide extensive benefits that are both ecologically and economically important. Seagrass habitats alone contribute over $19,000 ha−1 yr−1 to local economies (Costanza et al., 1997; Fourqurean et al., 2012; Tuya et al., 2014). When found within seagrass habitats, drift macroalgae can further increase phytobiomass aboveground by 3- to 100-fold (Morris and Hall, 2001), adding to habitat complexity that can support increased animal diversity (Kingsford, 1995). Drift macroalgae often originate as attached algae on seagrass leaves and other hard substrata before becoming uprooted by various physical disturbances (e.g., currents and waves) (Norton and Mathieson, 1983; Bell and Hall, 1997; Biber, 2002; Lirman et al., 2003). They are classified into three major groups: brown algae (Phaeophyceae), green algae (Chlorophyta), and red algae (Rhodophyta; Norton and Mathieson, 1983; Bell and Hall, 1997; Biber, 2002; Lirman et al., 2003), with rhodophytes being the dominant taxa in the northern Gulf of Mexico (Virnstein and Carbonara, 1985; Bell and Hall, 1997; Holmquist, 1997). Seagrass and associated macroalgae create productive ecosystems relative to neighboring unvegetated substrates, providing valuable nursery habitats (e.g., Carr, 1994; Jackson et al., 2001; Heck et al., 2003), enhancing local fisheries, increasing food resources and fish growth rates, and decreasing predation and competition (e.g., Orth et al., 1984; Fraser and Gilliam, 1987; Rooker et al., 1998; Nagelkerken et al., 2002; Jackson et al., 2015).
Drift macroalgae often increase the structural complexity of seagrass habitats (Morris and Hall, 2001) and provide additional refuge, leading to increases in infauna (Hull, 1987), epifauna (Isaksson and Pihl, 1992), and macrofauna, including small crustaceans and mollusks (Norkko et al., 2000; Raffaelli, 2000; Salovius et al., 2005), juvenile blue crabs (Wilson et al., 1990), and small shrimp and fish (Holmquist, 1994; Holmquist, 1997; Kingsford, 1995; Correia, 2021). Relationships between habitat complexity and nekton abundance and species richness have been the focus of many studies, with more complex habitats increasing niche space and leading to increases in the abundance and diversity of animals found there (e.g., Kingsford, 1995; Rozas and Minello, 1998; Minello, 1999). While macroalgae can enhance seagrass ecosystem services, at sufficiently high biomass, they can also lead to hypoxic conditions with resulting declines in animal abundance and species diversity (Hull, 1987; Bonsdorff, 1992; Raffaelli et al., 1998; Cummins et al., 2004; Zajac and McCarthy, 2015). Whether drift macroalgae have positive or negative impacts on the animal community is largely driven by the quantity and type of algae present, with lower drift algal biomass oftentimes leading to higher nekton abundance and species richness (e.g., Everett, 1994; Holmquist, 1997; Norkko et al., 2000; Arroyo et al., 2006) and high biomass leading to a decline in species richness (e.g., Bonsdorff, 1992; Raffaelli et al., 1998). However, understanding the threshold where the net impact of drift algal biomass transitions from positive to negative and the overall role that drift algae play in structuring animal communities remains an ongoing challenge.
Over the past few decades, seagrass cover has declined in the northern Gulf of Mexico, while drift algal abundance has remained constant or increased (Benz et al., 1979; Virnstein and Carbonara, 1985; Zieman et al., 1989; Biber and Irlandi, 2006; Kopecky and Dunton, 2006; Fredericq et al., 2009; Correia, 2021). With future climate projections indicating an increase in macroalgae in accordance with higher sea surface temperatures and ocean acidification (Brodie et al., 2014), contemporary studies are needed to assess both the presence and effects of drift macroalgae on seagrass-associated nekton communities. The purpose of this study was to investigate the relationships between drift macroalgal biomass and small nekton communities on a Gulf-wide scale. Previous comparisons across the northern Gulf of Mexico revealed significant differences in algal biomass across the region and sampling period (Correia et al., in press). Because macroalgae were often more abundant during the early summer months, this study primarily focused on data collected during May–June 2018. To determine how drift algal biomass influences nekton community structure, we performed a survey of drift macroalgae across five estuaries spanning 2000 km of the coastline in the northern Gulf of Mexico, measuring macroalgal biomass as well as the nekton community found within the seagrass-macroalgal mosaic. Specifically, we quantified (1) the relationship between algal biomass and nekton community composition (e.g., abundance, diversity, and taxon-specific variations) and (2) regional variation in these relationships across the northern Gulf of Mexico. An epibenthic sled was used during data collection to sample the smaller epibenthic nekton and invertebrates living within the algal-seagrass matrix, which are more likely to be influenced by variations in algal biomass. Because the seagrass percent cover and structure varied across each region, which could affect our interpretation of algal impacts on fauna, a subset of the data was also analyzed to compare nekton community changes across a range of algal weights when the areal coverage of the dominant seagrass turtle grass (Thalassia testudinum) exceeded 75% and canopy heights were between 200 and 400 mm (Correia, 2021). Based on previous findings, we hypothesized that algal biomass would influence the community composition of fauna, increasing the abundance and richness at intermediate algal biomasses.
Materials and methods
Study regions
Five estuaries in the northern Gulf of Mexico, each containing at least 20 sites within seagrass meadows, were surveyed during early (May–June) and late (August–September) summer of 2018 (Supplementary Figure S1). The estuaries sampled included Laguna Madre, TX (LM; n = 20; 26°08′N, 97°14′W), Corpus Christi Bay, TX (CB; n = 20; 27°51′N, 97°08′W), the Chandeleur Islands, LA (LA; n = 20; 29°54′N, 88°50′W), Cedar Key, FL (CK; n = 25; 29°05′N, 83°01′W), and Charlotte Harbor (CH; n = 25; 26°04′N, 82°14′W). These estuaries encompass two semi-enclosed bays with major freshwater inputs (CB and CH), two sites along the open coastline (CK and LA), and one semi-enclosed lagoon with no major freshwater inputs (LM). Sites were selected by overlaying a tessellated hexagonal grid (500 m edge length) on each estuary in ArcGIS (Moore, 2009; Neckles et al., 2012; Wilson and Dunton, 2012). One station was randomly generated within each of the 20–25 grid cells that contained more than 50% seagrass coverage, with a minimum of 500 m distance between sites (Belgrad et al., 2021). Across all regions, turtle grass (T. testudinum) was the dominant macrophyte taxa; however, manatee grass (Syringodium filiforme) and shoal grass (Halodule wrightii) were also common. At each sampling site, abiotic conditions (i.e., temperature, salinity, dissolved oxygen, and depth) were recorded, drift macroalgal biomass was measured, and seagrass cover/abundance and morphometrics [i.e., percent cover (0–100%), shoot height (mm), and shoot density] were assessed. Because we sampled synoptically using the same methods, we were able to evaluate algal biomass and distribution within and among locations across the northern Gulf of Mexico (Supplementary Table S1; Belgrad et al., 2021; Correia, 2021).
Drift algal biomass and nekton community assessment
Within each hexagon, drift algal biomass and nekton abundance within seagrass meadows were measured using an epibenthic sled, consisting of an aluminum frame (0.75 m wide and 0.6 m high) with two skids on either side (0.7 m in length), and a 2 mm stretch mesh net. The sled captured smaller epibenthic fish and invertebrates living within the algal-seagrass matrix. The sled was towed for 13.3 m at approximately 0.3 m sec−1, covering an area of approximately 10 m2. Macroalgae from benthic sled samples were bagged, frozen, and transported to the lab where they were later identified and weighed. Nekton within the sled were identified to the lowest possible classification and counted, and their lengths (i.e., total length for shrimp and fish; carapace width for crabs) were recorded.
Seagrass and algal vegetative sampling (quadrats)
Seagrass structural complexity was assessed using a 1 m2 quadrat divided into 100, 10 cm × 10 cm cells. Four quadrats were haphazardly thrown at each sampling location, and the percent cover, shoot count, and canopy height of each seagrass species present in a quadrat were recorded. The seagrass percent cover by species and the cover of drift algae were measured by counting the number of grid cells within each quadrat that contained a particular vegetation type (0–100 grids quadrat−1). Shoot count was calculated for each seagrass species by counting the number of shoots within a random quadrat grid cell. Canopy height was defined as the mean of three randomly selected shoot height measurements.
Statistical analysis
SAS© and Primer™ 7 were used for all statistical analyses. A combination of univariate and multivariate analyses was performed to assess nekton abundance, biodiversity, animal size, and community structure in relation to drift algal biomass. Variables were tested in SAS© for normality, and abundance was log-transformed to mitigate skewness and to achieve normality. Generalized linear mixed models (GLMMs) were used in SAS© to compare nekton abundance (N), species richness (S), and diversity (H’) across the region (CB, CH, CK, LA, and LM), to algal weight [g wet weight (ww); Horton and Lipsitz, 2012; Sokal and Rohlf, 2013]. The weights of algae collected in the epibenthic sled were then ranked, and multivariate analysis was performed in Primer to compare community differences across these weight classes (0–4), with 0 indicating no algae present (n = 70), 1 indicating 1–300 g ww of algae (n = 77, mean = 115 ± 83 SD), 2 indicating 301–500 g ww (n = 25, mean = 115 ± 83), 3 indicating 501–1,000 g ww (n = 23, mean = 755 ± 172), and 4 indicating over 1,000 g ww (n = 25, mean = 2,560 ± 1,630). The ranking system was necessary for Primer analysis because this software cannot analyze continuous data as a treatment. Species abundance was square-root-transformed to manage skewness and analyzed using a one-way PERMANOVA design with algal weight class (0–4) as the fixed factor. A canonical analysis of principal coordinates (CAP) plot was also performed to illustrate a pattern of difference across algal weight classes.
To account for the positive relationship between drift macroalgal biomass and increased seagrass percent cover (Correia, 2021; Correia et al., in press), a subset of the data was also analyzed to compare the effects of drift algal biomass to nekton community composition while holding seagrass constant (the turtle grass percent cover was greater than 75%, and the canopy heights were between 200 and 400 mm), encompassing 31.8% of the original data set. When analyzing the subset, community or diversity metrics were no longer significant among sampling periods (i.e., early vs. late summer, p > 0.05), and we used samples collected in both early and late summer for this analysis. Generalized linear mixed models (GLMMs) were again performed in SAS© on the data subset to compare nekton abundance (N), species richness (S), and diversity (H’) across the region (CB, CH, CK, LA, and LM) and algal weight (g ww; Horton and Lipsitz, 2012; Sokal and Rohlf, 2013).
In Primer, similarity percentage (SIMPER) analysis identified which species contributed over 70% of the difference across algal weight classes; individual species (or groups of species based upon classification similarities) were compiled and reanalyzed to test for taxon-specific abundance and size differences across the algal weight. During taxon-specific analysis, seagrass variables were held constant and only samples containing greater than 75% turtle grass coverage and canopy heights of 200–400 mm were included. Analysis of taxon-specific abundance was also compared using GLMMs across the region (CB, CH, CK, LA, and LM) and algal weight (g ww) to identify taxon-specific changes across constant seagrass coverage and canopy height.
Results
Overall, the mean animal abundance was significantly different across algal weight (p < 0.001) and was higher when the algal biomass was greater than 500 g (Figure 1A; Table 1). While algal biomass led to increased nekton species abundance, no clear pattern was found with nekton species richness or diversity (p = 0.92; Table 1). Nekton species richness remained relatively stable across algal weight classes, with 49 unique species identified when no algae were present (n = 19), 53 species in 1–300 g ww (n = 37), 52 species in 301–500 g ww (n = 19), 42 species in 501–1,000 g ww (n = 19), and 56 species in areas with over 1,000 g ww of algae (n = 15). Shannon diversity also did not differ significantly across algal weight and only varied by region (p = 0.03; Table 1). When analyzing a subset of the data in turtle grass with a similar coverage and canopy height, similar patterns were observed for animal abundance, with significantly higher animal abundances seen at algal biomasses over 500 g ww, compared to those when no algae were present (Figure 1B; Table 1). Diversity was not significantly different across region (p = 0.14) or algal weight (p = 0.71; Table 1) in the data subset, and the richness again remained stable across all algal weight classes (Correia, 2021).
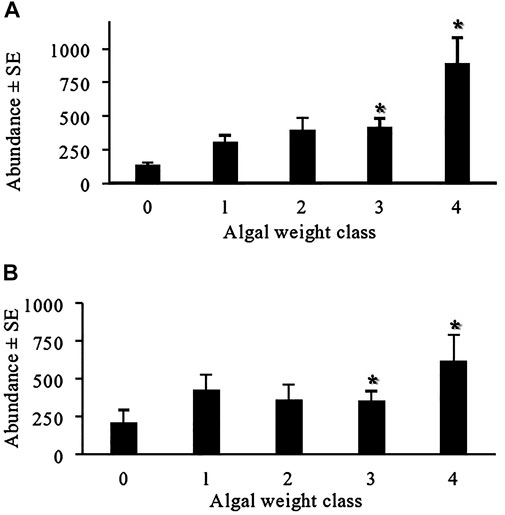
FIGURE 1. Average (+SE) small nekton abundance across drift macroalgae weight classes in the early summer benthic sled samples. Abundance measurements were collected from the epibenthic sled pull, which covered a 10 m2 area. The asterisk (*) indicates that there is a significant difference in the algae weight class compared to that when no algae were present. The full data set (A) and subset in which seagrass was held constant (B) showed a similar pattern of increased abundance at algal concentrations greater than 500 g. In this data set, “0” indicates that no algae were present in the sled sample [n = 19, 22 (full, subset, respectively)], “1” indicates 1–300 g of algae (n = 37, 30), “2” represents 301–500 g of algae (n = 19, 15), “3” represents 501–1,000 g of algae (n = 19, 9), and “4” represents samples containing over 1,001 g of algae (n = 15, 8).
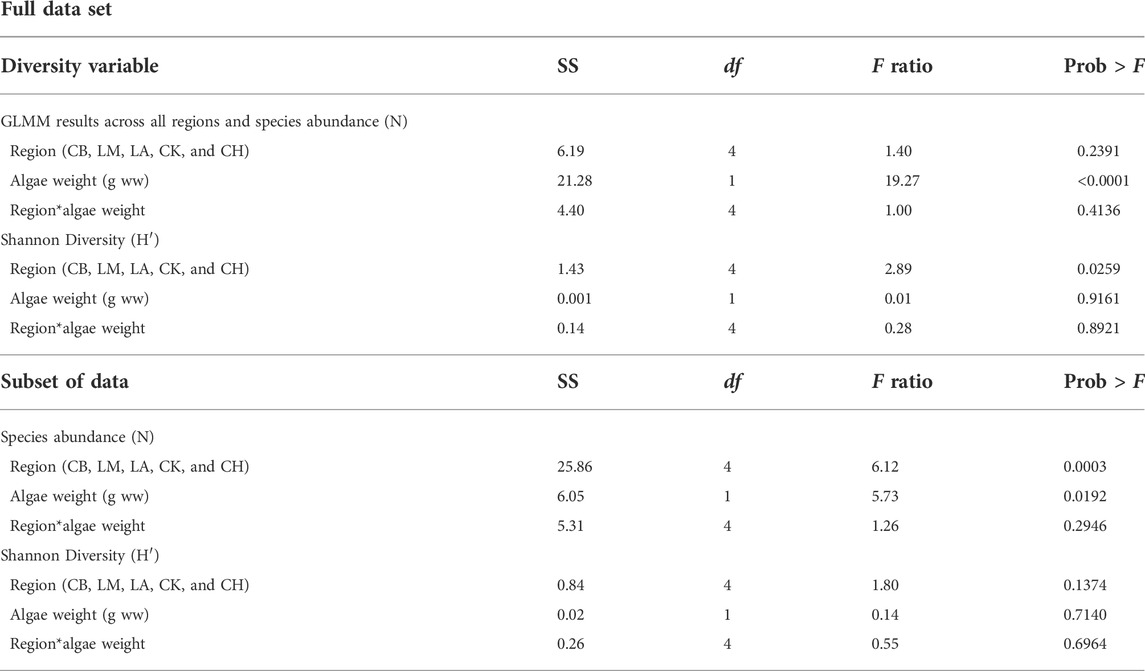
TABLE 1. Animal diversity across region and algae weight. GLMMs were performed in SAS© to compare nekton abundance (N) and diversity (H’) across region (CB, CH, CK, LA, and LM) and algae weight [g wet weight (ww)]. General linear regression models for species abundance across region and algae weight during the summer 2018 sampling season, when seagrass was held constant, was also performed.
Macroalgal biomass significantly influenced overall community structure (Figure 2A; Pseudo-F = 3.124; p = 0.001). Pairwise comparisons across the different algal weight classes indicated that small benthic communities were significantly different when algae were present. When comparing algal weight classes to one another, all areas containing algae were significantly different from areas with no algae (Supplementary Table S2). Areas containing smaller concentrations of algae (i.e., Class 1–3) were also significantly different from areas containing over 1,000 g of algae (Class 4; Supplementary Table S2). When analyzing these relationships using a subset of the data to control for seagrass cover and canopy height, macroalgal biomass still significantly influenced community structure (Figure 2B; Pseudo-F = 2.24; p = 0.002). Pairwise comparisons across algal weight class indicated that all areas containing algae were significantly different from areas with no algae (Supplementary Table S2). When comparing algal weight classes to one another, areas containing 300–500 g (Class 2) of macroalgae were significantly different from areas containing over 500 g (Classes 3 and 4). Macroalgal concentrations below 300 g showed the most variability in the nekton community and were not significantly different from the other macroalgal classes (Supplementary Table S2).
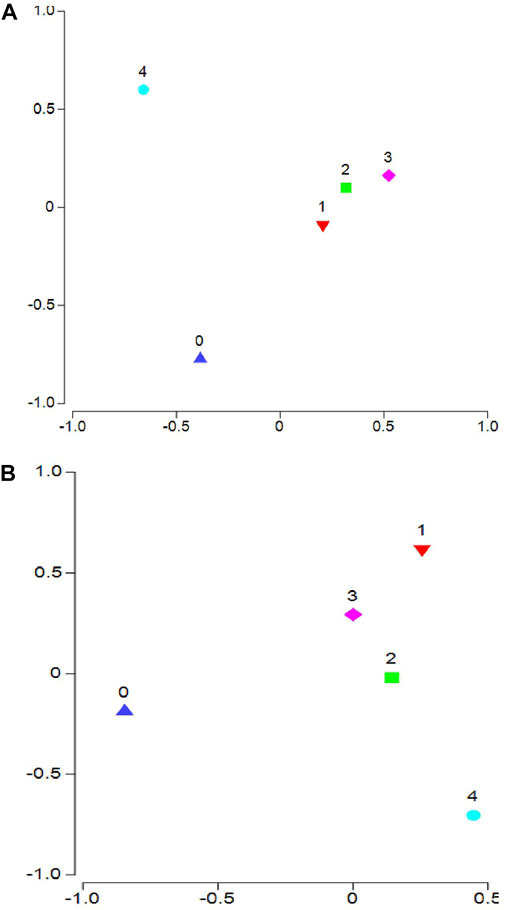
FIGURE 2. CAP plot of community structure across algae weight class. CAP plot comparing communities during the early summer across averaged algae weight classes. Comparisons were run using the complete data set (A) and a subset of the data, in which the turtle grass percent cover was >75% cover and the average canopy height was between 200 and 400 mm (B). Classifications included microbenthic sled samples where no algae were present (0), along with areas containing up to 300 g (1), 301–500 g (2), 501–1,000 g (3), and over 1,000 g (4) of macroalgae.
Taxon-specific responses to macroalgae
The largest sources of dissimilarity between communities across the different algal classes, as identified by SIMPER analysis, were nonpenaeid shrimp (Hippolytidae, Palaemonidae, Processidae), penaeid shrimp (Penaeidae), gobies (Gobiidae), pipefish (Syngnathidae), and pinfish (Lagodon rhomboides), which collectively made up over 70% of the dissimilarity. Hippolytidae (hippolytid shrimp) were the most abundant organisms collected, with over 1,000 individuals present in some samples. Because of this, Hippolytidae was analyzed separately from other nonpenaeid shrimp. All other nonpenaeid shrimp (Palaemonidae, Palaemonetes sp., Periclimenes sp., Processa sp., Periclimenes americanus, Periclimenes longicaudatus, Palaemon floridanus, Palaemonetes pugio, Palaemonetes vulgaris, Tozeuma carolinense, Thor sp., and Thor dobkini) were grouped together. Penaeid shrimp included Penaeidae, Farfantepenaeus sp., Rimapenaeus sp., Farfantepenaeus duorarum, Farfantepenaeus aztecus, and Litopenaeus setiferus. Gobies included Gobiidae, Gobiosoma sp., Microgobius sp., Bathygobius sp., Ctenogobius sp., Gobionellus sp., Gobiosoma robustum, Ctenogobius boleosoma, Microgobius gulosus, Microgobius thalassinus, Gobiosoma bosc, Evothodus lyricus, and Bathygobius soporator. Pipefish included Syngnathidae, Syngnathus sp., Syngnathus floridae, Syngnathus louisiana, Syngnathus scovelli, and Anarchopterus criniger.
There was a significant interaction between region and weight class for Hippolytidae shrimp abundance at α = 0.1 (p = 0.09; Table 2). Other nonpenaeid shrimp abundances were also significantly different across region (p < 0.001) and algal weight class (p = 0.03; Table 2). The abundances of penaeid shrimp and gobies, however, only differed across region and were not related to algal biomass (Table 2). An overall positive trend in Hippolytidae shrimp was observed in Texas and Florida regions with increasing algal biomass, with a slight negative trend in the Chandeleur Islands, LA. At the highest algal biomass, a slight decline in Hippolytidae abundance was also observed in Laguna Madre, TX, Corpus Christi Bay, TX, and Charlotte Harbor, FL (Supplementary Figure S2). Other nonpenaeid shrimp followed a similar pattern, with an increase in shrimp abundance at all locations except Cedar Key, FL (Supplementary Figure S4). The degree of shrimp increase was regionally dependent (Supplementary Figure S4, S5). While organismal abundance increased, the overall length of shrimp and fish did not change with algal weight (p > 0.05; Supplementary Table S3, S4), indicating that the same organismal size classes were accessing structured habitats at all drift algal biomasses.
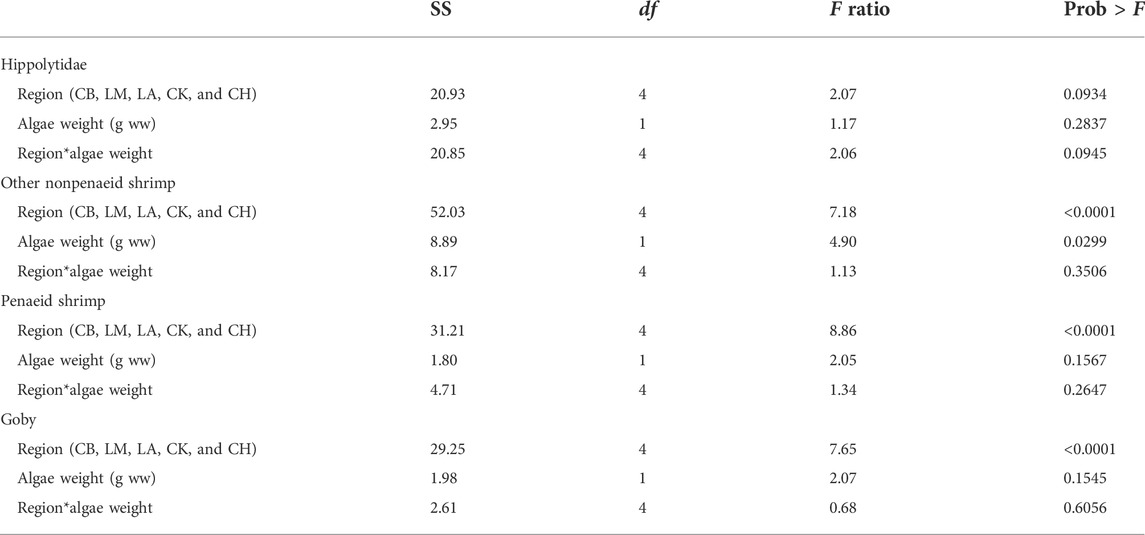
TABLE 2. Selected species-specific abundance comparisons across region and algae weight when seagrass metrics were held constant. Only samples with greater than 75% turtle grass cover and canopy heights of 200–400 mm were used in this analysis.
Discussion
Across the northern Gulf of Mexico, the abundance of small epibenthic nekton were positively related to drift algal biomass, but the strength of the effect varied regionally. When the seagrass cover and canopy height were held constant, the same patterns were observed, strengthening our conclusion that changes in macroalgal biomass were the primary driver of organismal patterns. Diversity and species-specific size, however, did not change with macroalgal biomass, indicating an additive role within the seagrass landscape rather than a distinct habitat type, at least for the nekton sampled. Red and brown drift algae, which are common in the Gulf of Mexico and accounted for most of the drift algae in this study, are known to increase faunal abundances (Virnstein and Carbonara, 1985; Bell and Hall, 1997; Holmquist, 1997; Correia, 2021), and thus, our results are consistent with previous findings. In some instances, however, drift algae can smother seagrass beds, cause hypoxia, and ultimately reduce faunal abundance and diversity (Hull, 1987; Isaksson and Pihl, 1992; Norkko and Bonsdorff, 1996a; Norkko and Bonsdorff, 1996b; Raffaelli, 2000). This is commonly seen when nutrient enrichment leads to drastic increases in drift macroalgal biomass, reducing local dissolved oxygen and light concentrations. This effect appears to be dependent on algal biomass and may be species-specific (Hull, 1987; Raffaelli, 2000; Young, 2009). A slight decline in nonpenaeid shrimp was observed at the highest algal biomasses (exceeding 2,000 g ww) in Corpus Christi Bay, TX, Laguna Madre, TX, and Charlotte Harbor, FL, which could be an indicator of algal-mediated habitat degradation (Supplementary Figure S2). Our data are limited at the higher end of algal biomass, although making it difficult to identify a threshold at which algae might begin to have negative effects. Future studies should quantify abundance changes across a larger range of algal biomass to pinpoint such thresholds. With few exceptions, in this study, drift algae were associated with higher animal abundance, driven largely by shrimp species. We believe that this represents an effect of added three-dimensional structure within the seagrass-macroalgal mosaic, allowing more individuals to occupy a given area of seagrass.
Shallow vegetated habitats are commonly associated with increased abundance and diversity of organisms compared to adjacent bare substrates, leading to inferences that structure, regardless of type, is important when determining species occupancy per given area (Heck et al., 2003; Minello et al., 2003; Humphries et al., 2011). Previously, a positive relationship between increased turtle grass percent cover and drift macroalgae percent cover was found (Correia, 2021; Correia et al., in press), which could be influencing the results of this study, due to the overall increase in habitat complexity. However, by analyzing a subset of the data over a narrow range of turtle grass percent cover and canopy heights and coming to similar conclusions, it seems unlikely that seagrass percent cover alone explains the patterns noted between algal biomass and nekton abundance. The added complexity provided by drift macroalgae likely leads to positive changes in nekton abundance by the same mechanism. However, quantification of algal influences on flow, temperature, dissolved oxygen, and recruitment of small nekton organisms would provide additional information regarding the nature of the habitat provided by drift algae relative to seagrass alone.
Macroalgal blooms are more variable than other complex habitats found along the coast (e.g., seagrass meadow, coral reef, and mangrove forest) and are largely dependent on local flow, season, and water quality. Generally, red and brown macroalgae in the Gulf of Mexico are found at peak abundance during the summer (Pihl et al., 1996). Although algae can grow rapidly, storms and currents can displace them quickly (Pihl et al., 1999). Not surprisingly, the ephemeral nature of macroalgal communities makes this a good habitat for smaller, motile, and more opportunistic organisms that can successfully find and use the habitat type when available (Norkko et al., 2000; Camp et al., 2014).
Variation in the positive association between algal biomass and nekton abundance could be related to differences in algal species composition across each region or differences in life history characteristics of both penaeid and nonpenaeid shrimp found in these areas. Changes in macroalgae species composition significantly influenced local animal abundances, with some algal species containing higher abundances than others (Correia, 2021). Spyridia, for example, contained approximately 757 ± 335 individuals when listed as the primary algal taxon in Charlotte Harbor, FL, with 23 unique species identified. Conversely, locations primarily containing Hypnea had 57 ± 39.5 individuals and nine unique species present (Correia, 2021). However, regardless of region and macroalgal composition, which likely contributed to the variation in our study, higher algal abundances were associated with increased nekton abundances.
Across the northern Gulf of Mexico, the presence of drift macroalgae may have a large, positive effect on smaller nekton organisms, such as shrimp and gobies, with the timing of peak algal abundance often coinciding with the peak in the recruitment of juvenile nekton (Turner and Brody, 1983; Rogers et al., 1993). This increase in recruitment can be beneficial to local fisheries by providing additional food resources, increasing the survival, growth rates, and development of important prey and forage species. It is important, however, to continue to monitor changes in drift macroalgae biomass since they often indicate eutrophication and can quickly transition to nuisance species with well-documented negative effects on the seagrass ecosystems in which they are found.
Data availability statement
The data sets presented in this study can be found in online repositories. The names of the repository/repositories and accession number(s) can be found below: http://www.darnellseagrassecologylab.com/turtlegrass.
Author contributions
KD and MD conceived and proposed the project, and all authors assisted in planning, coordination, and execution of the project. KC, BB, and DS took samples in the Texas region. KD, MD, and CH collected samples in the Louisiana region, and SA, BF, MH, CM, and AM collected samples in the Florida regions. Data were QA/QCed by CH and KC and analyzed by KC. KC coordinated the first draft with writing input by DS. All co-authors contributed to the editing of the subsequent drafts of the manuscript.
Funding
This paper is a result of research funded by the National Oceanic and Atmospheric Administration’s RESTORE Science Program award # NA17NOS4510093 to the University of Southern Mississippi, Dauphin Island Sea Lab, the University of Florida, and Florida Fish and Wildlife Conservation Commission.
Acknowledgments
The authors would like to thank Samantha Smith, Michael Wheeler, and Donna O’Dell for assistance in the field and laboratory, Manuel Merello for identifying macroalgal samples, and the reviewers who provided helpful edits to the manuscript prior to publication.
Conflict of interest
The authors declare that the research was conducted in the absence of any commercial or financial relationships that could be construed as a potential conflict of interest.
Publisher’s note
All claims expressed in this article are solely those of the authors and do not necessarily represent those of their affiliated organizations or those of the publisher, the editors, and the reviewers. Any product that may be evaluated in this article or claim that may be made by its manufacturer is not guaranteed or endorsed by the publisher.
Supplementary material
The Supplementary Material for this article can be found online at: https://www.frontiersin.org/articles/10.3389/fenvs.2022.939296/full#supplementary-material
References
Arroyo, N. L., Aarnio, K., and Bonsdorff, E. (2006). Drifting algae as a means of Re-colonizing defaunated sediments in the baltic sea. A short-term microcosm study. Hydrobiologia 554, 83–95. doi:10.1007/s10750-005-1008-5
Belgrad, B. A., Correia, K. M., Darnell, K. M., Darnell, M. Z., Hayes, C. T., Hall, M. O., et al. (2021). Environmental drivers of seagrass-associated nekton abundance across the northern Gulf of Mexico. Estuaries Coasts 44, 2279–2290. doi:10.1007/s12237-021-00927-0
Bell, S. S., and Hall, M. O. (1997). Drift macroalgal abundance in seagrass beds:investigating large-scale associations with physical and biotic attributes. Mar. Ecol. Prog. Ser. 147, 277–283. doi:10.3354/meps147277
Benz, M., Eiseman, N. J., and Gallaher, E. E. (1979). Seasonal occurrence and variation in standing crop of a drift algal community in the Indian river, Florida. Bot. Mar. 22, 413–420. doi:10.1515/botm.1979.22.7.413
Biber, P. D., and Irlandi, E. A. (2006). Temporal and spatial dynamics of macroalgal communities along an anthropogenic salinity gradient in Biscayne Bay (Florida, USA). Aquat. Bot. 85 (1), 65–77. doi:10.1016/j.aquabot.2006.02.002
Biber, P. D. (2002). The effects of environmental stressors on the dynamics of 3 functional groups of algae in Thalassia testudinum habitats of biscayne bay, Florida: A modeling approach. PhD dissertation. Coral Gables, FL: University of Miami.
Bonsdorff, E. (1992). Drifting algae and zoobenthos - effects on settling and community structure. Neth. J. Sea Res. 30, 57–62. doi:10.1016/0077-7579(92)90045-g
Brodie, J., Williamson, C. J., Smale, D. A., Kamenos, N. A., Mieszkowska, N., Santos, R., et al. (2014). The future of the northeast Atlantic benthic flora in a high CO2 world. Ecol. Evol. 4 (13), 2787–2798. doi:10.1002/ece3.1105
Camp, E. V., Staudhammer, C. L., Pine, W. E., Tetzlaff, J. C., and Frazer, T. K. (2014). Replacement of rooted macrophytes by filamentous macroalgae: Effects on small fishes and macroinvertebrates. Hydrobiologia 722 (1), 159–170. doi:10.1007/s10750-013-1694-3
Carr, M. H. (1994). Effects of macroalgal dynamics on recruitment of a temperate reef fish. Ecology 75, 1320–1333. doi:10.2307/1937457
Correia, K. M. (2021). What doesn’t kill us makes us stronger: Seagrass community resilience in the northern Gulf of Mexico. PhD dissertation. Mobile, AL: University of South Alabama.
Correia, K. M., Alford, S. B., Belgrad, B. A., Darnell, K. M., Darnell, M. Z., et al. Drift macroalgal distribution in northern Gulf of Mexico seagrass meadows. PeerJ. in press.
Costanza, R., d'Arge, R., de Groot, R., Farber, S., Grasso, M., Hannon, B., et al. (1997). The value of the world's ecosystem services and natural capital. Nature 387, 253–260. doi:10.1038/387253a0
Cummins, S. P., Roberts, D. E., and Zimmerman, K. D. (2004). Effects of the green macroalga Enteromorpha intestinalis on macrobenthic and seagrass assemblages in a shallow coastal estuary. Mar. Ecol. Prog. Ser. 266, 77–87. doi:10.3354/meps266077
Everett, R. A. (1994). Macroalgae in marine soft-sediment communities: Effects on benthic faunal assemblages. J. Exp. Mar. Biol. Ecol. 150, 223–247. doi:10.1016/0022-0981(94)90030-2
Fourqurean, J. W., Duarte, C. M., Kennedy, H., Marbà, N., Holmer, M., Mateo, M. A., et al. (2012). Seagrass ecosystems as a globally significant carbon stock. Nat. Geosci. 5, 505–509. doi:10.1038/ngeo1477
Fraser, D. F., and Gilliam, J. F. (1987). Feeding under predation hazard: Response of the guppy and hart's rivulus from sites with contrasting predation hazard. Behav. Ecol. Sociobiol. 21, 203–209. doi:10.1007/bf00292500
Fredericq, S., Cho, T. O., Earle, S. A., Gurgel, F. G. C., Krayesky, D. M., Mateo-Cid, L. E., et al. (2009). “Seaweeds of the Gulf of Mexico,” in Gulf of Mexico- origins, waters, and biota biodiversity. Editors D. L. Felder, and D. K. Camp (College Station, Texas: Texas A&M Press), 187–259.
Heck, K. L., Hays, G., and Orth, R. J. (2003). Critical evaluation of the nursery role hypothesis for seagrass meadows. Mar. Ecol. Prog. Ser. 253, 123–136. doi:10.3354/meps253123
Holmquist, J. G. (1994). Benthic macroalgae as a dispersal mechanism for fauna: Influence of a marine tumbleweed. J. Exp. Mar. Biol. Ecol. 180, 235–251. doi:10.1016/0022-0981(94)90069-8
Holmquist, J. G. (1997). Disturbance and gap formation in a marine benthic mosaic:influence of shifting macroalgal patches on seagrass structure and mobile invertebrates. Mar. Ecol. Prog. Ser. 158, 121–130. doi:10.3354/meps158121
Horton, N. J., and Lipsitz, S. R. (2012). Review of software to ft generalized estimating equation regression models. Am. Stat. 53, 160–169. doi:10.1080/00031305.1999.10474451
Hull, S. C. (1987). Macroalgal mats and species abundance: A field experiment. Estuar. Coast. Shelf Sci. 25, 519–532. doi:10.1016/0272-7714(87)90112-0
Humphries, A. T., La Peyre, M. K., Kimball, M. E., and Rozas, L. P. (2011). Testing the effect of habitat structure and complexity on nekton assemblages using experimental oyster reefs. J. Exp. Mar. Biol. Ecol. 409, 172–179. doi:10.1016/j.jembe.2011.08.017
Isaksson, I., and Pihl, L. (1992). Structural changes in benthic macrovegetation and associated epibenthic faunal communities. Neth. J. Sea Res. 30, 131–140. doi:10.1016/0077-7579(92)90052-g
Jackson, E. L., Attrill, M., Rowden, A. A., Bossey, S. J., and Jones, M. B. (2001). The importance of seagrass beds as a habitat for fishery species. Oceanogr. Mar. Biol. Annu. Rev. 39, 269–303.
Jackson, E. L., Rees, C., Wilding, M. J., and Attrill, M. J. (2015). Use of a seagrass residency index to apportion commercial fishery landing values and recreation fisheries expenditure to seagrass habitat service. Conserv. Biol. 29, 899–909. doi:10.1111/cobi.12436
Kingsford, M. J. (1995). Drift algae: A contribution to near-shore habitat complexity in the pelagic environment and an attractant for fish. Mar. Ecol. Prog. Ser. 116, 297–301. doi:10.3354/meps116297
Kopecky, A. L., and Dunton, K. H. (2006). Variability in drift macroalgal abundance in relation to biotic and abiotic factors in two seagrass dominated estuaries in the western Gulf of Mexico. Estuaries Coasts 29, 617–629. doi:10.1007/bf02784286
Lirman, D., Orlando, B., Maciá, S., Manzello, D., Kaufman, L., Biber, P., et al. (2003). Coral communities of biscayne bay, Florida and adjacent offshore areas: Diversity, abundance, distribution, and environmental correlates. Aquat. Conserv. Mar. Freshw. Ecosyst. 13, 121–135. doi:10.1002/aqc.552
Minello, T. J., Able, K. W., Weinstein, M. P., and Hays, C. G. (2003). Salt marshes as nurseries for nekton: Testing hypotheses on density, growth and survival through meta-analysis. Mar. Ecol. Prog. Ser. 246, 39–59. doi:10.3354/meps246039
Minello, T. J. (1999). “Nekton densities in shallow estuarine habitats of Texas and Louisiana and Identification of essential fish habitat (EFH),”. Fish habitat: Essential fish habitat and rehabilitation. Editor L. Benaka (Bethesda, Maryland: Am. Fish. Soc. Symp.), 22, 43–75.
Moore, K. (2009). NERR SWMP biomonitoring protocol. MD: Natl. Est. Res. Syst. NOAA Ocean Service. Silver Spring.Long-term monitoring for estuarine submersed and emergent vegetation communities
Morris, L. J., and Hall, L. M. (2001). Estimating drift algae abundance in the Indian River Lagoon, FL. St. Palatka, FL: Johns River Water Management District Technical Memorandum, 10pp.
Nagelkerken, I., Roberts, C. M., van der Velde, G., Dorenbosch, M., van Riel, E., Cocheret de la Moriniere, E., et al. (2002). How important are mangroves and seagrass beds for coral-reef fish? The nursery hypothesis tested on an island scale. Mar. Ecol. Prog. Ser. 244, 299–305. doi:10.3354/meps244299
Neckles, H. A., Kopp, B. S., Peterson, B. J., and Pooler, P. S. (2012). Integrating scales of seagrass monitoring to meet conservation needs. Estuaries Coasts 35, 23–46. doi:10.1007/s12237-011-9410-x
Norkko, A., and Bonsdorff, E. (1996a). Altered benthic prey-availability due to episodic oxygen deficiency caused by drifting algal mats. Mar. Ecol. Berl. 17, 355–372. doi:10.1111/j.1439-0485.1996.tb00514.x
Norkko, A., and Bonsdorff, E. (1996b). Rapid zoobenthic community responses to accumulations of drifting algae. Mar. Ecol. Prog. Ser. 131, 143–157. doi:10.3354/meps131143
Norkko, J., Bonsdorff, E., and Norkko, A. (2000). Drifting algal mats as an alternative habitat for benthic invertebrates:. J. Exp. Mar. Biol. Ecol. 248, 79–104. doi:10.1016/s0022-0981(00)00155-6
Norton, T. A., and Mathieson, A. C. (1983). The biology of unattached seaweeds. Editor F. Round, and D. Chapman (Amsterdam: Elsevier Sci. Publ), Prog. Phycol. Res. 2, 333–386.
Orth, R. J., Heck, K. L., and van Montfrans, J. (1984). Faunal communities in seagrass beds: A review of the influence of plant structure and prey characteristics on predator: Prey relationships. Estuaries 7, 339. doi:10.2307/1351618
Pihl, L., Magnusson, G., Isaksson, I., and Wallentinus, I. (1996). Distribution and growth dynamics of ephemeral macroalgae in shallow bays on the Swedish west coast. J. Sea Res. 35, 169–180. doi:10.1016/s1385-1101(96)90744-3
Pihl, L., Sverson, A., Moksnes, P., and Wennhage, (1999). Distribution of green algal mats throughout shallow soft bottoms of the Swedish Skagerrak archipelago in relation to nutrient sources and wave exposure. J. Sea Res. 41, 281–294. doi:10.1016/S1385-1101(99)00004-0
Raffaelli, D. (2000). Interactions between macro-algal mats and invertebrates in the Ythan estuary, Aberdeenshire, Scotland. Helgol. Mar. Res. 54, 71–79. doi:10.1007/s101520050004
Raffaelli, D., Raven, J. A., and Poole, L. J. (1998). Ecological impact of green macroalgal blooms. Oceanogr. Mar. Biol. Annu. Rev. CRC Press 36, 97–125.
Rogers, B. D., Shaw, R. F., Herke, W. H., and Blanchet, R. H. (1993). Recruitment of postlarval and juvenile Brown shrimp (Penaeus aztecus ives) from offshore to estuarine waters of the northwestern Gulf of Mexico. Estuar. Coast. Shelf Sci. 36, 377–394. doi:10.1006/ecss.1993.1023
Rooker, J. R., Holt, G. J., and Holt, S. A. (1998). Vulnerability of newly settled red drum ( sciaenops ocellatus ) to predatory fish: Is early-life survival enhanced by seagrass meadows? Mar. Biol. 131, 145–151. doi:10.1007/s002270050305
Rozas, L. P., and Minello, T. J. (1998). Nekton use of salt marsh, seagrass, and nonvegetated habitats in the south Texas (USA) estuary. Bull. Mar. Sci. 63 (3), 481–501. doi:10.3354/meps096147
Salovius, S., Nyqvist, M., and Bonsdorff, E. (2005). Life in the fast lane: Macrobenthos use temporary drifting algal habitats. J. Sea Res. 53, 169–180. doi:10.1016/j.seares.2004.05.001
Sokal, R., and Rohlf, F. J. (2013). Biometry: The principles and practice of statistics in biological research. 3rd edition. New York, New York, USA: B WH Freeman.
Turner, R. E., and Brody, M. S. (1983). Habitat suitability index models: Northern Gulf of Mexico brown shrimp and white shrimp. Baton Rouge, LA: National Coastal Ecosystems Team Division of Biological Services Research and Development Fish and Wildlife Service, 35.
Tuya, F., Haroun, R., and Espino, F. (2014). Economic assessment of ecosystem services: Monetary value of seagrass meadows for coastal fisheries. Ocean. Coast. Manag. 96, 1–7. doi:10.1016/j.ocecoaman.2014.04.032
Virnstein, R. W., and Carbonara, P. A. (1985). Seasonal abundance and distribution of drift algae and seagrasses in the mid-Indian River lagoon, Florida. Aquat. Bot. 23, 67–82. doi:10.1016/0304-3770(85)90021-x
Wilson, C. J., and Dunton, K. H. (2012). Assessment of seagrass habitat quality and plant condition in Texas coastal waters. Report submitted to the coastal bend bays and estuaries Program. Project number 1201. 46pp.
Wilson, K. A., Able, K. W., and Heck, K. L. (1990). Habitat use by juvenile blue crabs: A comparison among habitats in southern New Jersey. Bull. Mar. Sci. 46, 105–114.
Young, D. R. (2009). “Macroalgal interactions with the seagrasses zostera spp. and Thalassia testudinum,” in Nelson wg. 2009. Seagrasses and protective criteria: A review and assessment of research status (Newport, OR: National Health and Environmental Effects Research Laboratory U.S. Environmental Protection Agency Newport OR 97365).
Zajac, R., and McCarthy, A. (2015). Drift algae in new haven harbor and impacts on benthic communities communities. West Haven Connecticut: University of New Haven, 17.
Keywords: habitat complexity, diversity, abundance, Texas, Louisiana, Florida
Citation: Correia KM, Alford SB, Belgrad BA, Darnell KM, Darnell MZ, Furman BT, Hall MO, Hayes CT, Martin CW, McDonald AM and Smee DL (2022) Drift macroalgae positively influence seagrass-associated nekton communities of the northern Gulf of Mexico. Front. Environ. Sci. 10:939296. doi: 10.3389/fenvs.2022.939296
Received: 09 May 2022; Accepted: 04 July 2022;
Published: 23 August 2022.
Edited by:
Jun Hou, Hohai University, ChinaReviewed by:
Jianxiang Feng, Sun Yat-sen University, ChinaPaulo Sergio Salomon, Federal University of Rio de Janeiro, Brazil
Copyright © 2022 Correia, Alford, Belgrad, Darnell, Darnell, Furman, Hall, Hayes, Martin, McDonald and Smee. This is an open-access article distributed under the terms of the Creative Commons Attribution License (CC BY). The use, distribution or reproduction in other forums is permitted, provided the original author(s) and the copyright owner(s) are credited and that the original publication in this journal is cited, in accordance with accepted academic practice. No use, distribution or reproduction is permitted which does not comply with these terms.
*Correspondence: Kelly M. Correia, ZHJrbWNvcnJlaWFAZ21haWwuY29t
†Present Address: Christian T. Hayes, Department of Biology, Environmental Science, and Health Science, Waynesburg University, Waynesburg, PA, United States