- 1Department for Ecology and Ecosystem Modelling, Institute of Biochemistry and Biology, University of Potsdam, Potsdam, Germany
- 2Berlin-Brandenburg Institute of Advanced Biodiversity Research (BBIB), Berlin, Germany
Environmental pollution by microplastics has become a severe problem in terrestrial and aquatic ecosystems and, according to actual prognoses, problems will further increase in the future. Therefore, assessing and quantifying the risk for the biota is crucial. Standardized short-term toxicological procedures as well as methods quantifying potential toxic effects over the whole life span of an animal are required. We studied the effect of the microplastic polystyrene on the survival and reproduction of a common freshwater invertebrate, the rotifer Brachionus calyciflorus, at different timescales. We used pristine polystyrene spheres of 1, 3, and 6 µm diameter and fed them to the animals together with food algae in different ratios ranging from 0 to 50% nonfood particles. As a particle control, we used silica to distinguish between a pure particle effect and a plastic effect. After 24 h, no toxic effect was found, neither with polystyrene nor with silica. After 96 h, a toxic effect was detectable for both particle types. The size of the particles played a negligible role. Studying the long-term effect by using life table experiments, we found a reduced reproduction when the animals were fed with 3 µm spheres together with similar-sized food algae. We conclude that the fitness reduction is mainly driven by the dilution of food by the nonfood particles rather than by a direct toxic effect.
1 Introduction
Scientific and public interest in microplastics (< 5 mm) highly increased in recent years and with it the upcoming concerns of the negative impacts on wildlife and whole ecosystems. Since surveys in marine ecosystems started to evaluate plastic occurrences, first accidentally (Carpenter and Smith, 1972) and later intentionally (Eriksen et al., 2014; Kanhai et al., 2017), alarming results have been reported, and the consequences of mismanaged plastic waste dumping and the associated releases of plastic into the environment have become apparent (Fok et al., 2019). However, (micro)plastics are not only found in aquatic ecosystems. They seem to be ubiquitous; whether in the sediments of fields (Piehl et al., 2018), in the air (Allen et al., 2019), or even in the snow of the Arctic (Bergmann et al., 2019), microplastics (MP) can be found. The associated risk of this pollutant is still unknown.
Many studies reported that a wide range of marine and freshwater organisms are able to ingest MP (Naidu et al., 2018; Windsor et al., 2019). Effects of MP exposure and ingestion can be manifold and likely depend on plastic type (Zimmermann et al., 2020; Phothakwanpracha et al., 2021), particle shape (Frydkjær et al., 2017; Qiao et al., 2019), particle size, and MP concentration (Jeong et al., 2016; Sun et al., 2019). In addition, the feeding strategy (Scherer et al., 2017) and the duration of exposure (Eltemsah and Bøhn, 2019; Cappello et al., 2021) are also determinants of the effect. Results of these laboratory studies are inconclusive, and a general assessment about the effect of MP on animal fitness or the risk of physiological impairments cannot be made yet. Studies reported adverse effects after MP exposure, resulting in increased mortality (Maes et al., 2020; An et al., 2021), decreased reproductive performance (Jeong et al., 2016; Jaikumar et al., 2019), decreased food intake (Cole et al., 2015; An et al., 2021), or even impacts at the molecular level (Gambardella et al., 2017). However, other studies found no or negligible effects after MP exposure on life history traits (Imhof et al., 2017; Wang et al., 2019). These inconsistent MP exposure results may be due to various reasons, such as exposure times, particle types, or test organism. For example, the duration of MP exposure differentially affects life history traits as shown in the study by Eltemsah and Bøhn (2019) with the cladoceran Daphnia magna. While in the short-term experiment, no acute toxic effects could be observed after 48 h, the long-term experiment (up to 77 days) revealed reduced growth and shifts in the timing of reproduction (Eltemsah and Bøhn, 2019). In the study by Cole et al. (2015) with the marine copepod Calanus helgolandicus, it is also evident that the duration of MP exposure plays an important role. Here, increased mortality, reduced egg size, and decreased hatching success were only seen later in the course of the experiment (Cole et al., 2015).
In addition to this unclear picture of the potential effects of MP on aquatic organisms, a shortcoming of current MP research is the frequent lack of an adequate control of inorganic particles (Ogonowski et al., 2018). Aquatic organisms are exposed to a wide range of natural particles, such as particulate organic matter (Wilkinson et al., 2013) or inorganic matter, such as calcite (Stabel, 1986) or clay particles (Tietjen et al., 2005). Therefore, for MP risk assessment distinguishing between changes in life history traits caused by a specific plastic effect or a general particle effect is crucial (Connors et al., 2017). As shown in some studies, increasing concentrations of inorganic particles impair algal uptake by cladocerans (Arruda et al., 1983; Hart, 1988) and result in lower population growth (Kirk, 1991). Furthermore, inorganic particles affect zooplankton groups differentially, as shown in a competition experiment with cladocerans and rotifers by Kirk and Gilbert (1990). There, rotifers were found to be more competitive than cladocerans at high inputs of inorganic particles, likely due to their differing feeding modes (Kirk and Gilbert, 1990). Inorganic particles have rarely been used as reference particles in experiments with cladoceran (Schür et al., 2020; Zimmermann et al., 2020), and for rotifers, only one study recently included inorganic particles in MP experiments in its experimental design (Drago and Weithoff, 2021).
Nevertheless, the uptake of MP at the beginning of the food chain is considered particularly critical due to the risk of bioaccumulation and biomagnification between trophic levels (Setälä et al., 2014). Although the focus changed somewhat in recent years, most MP studies focused on marine environments, so freshwater systems are still underrepresented (Wagner et al., 2014; Blettler et al., 2018). The magnitude of MP concentrations in freshwater ecosystems has been described in several studies for lakes (Fischer et al., 2016; Di and Wang, 2018) and rivers (Mani et al., 2015; Kapp and Yeatman, 2018), demonstrating the need to study the impact of MP on aquatic freshwater organisms. In freshwater systems, rotifers are a pivotal component of zooplankton communities (Arndt, 1993), providing an important link between primary producers and secondary consumers (Walz, 1993). Also, if food resources are adequate, they are widespread and can develop high population densities in freshwaters (Wallace and Snell, 2010). Although rotifers play an important role in limnetic food webs, studies related to the impact of MP to this phylum are still extremely scarce.
The aim of this study was to investigate the effect of polystyrene MP on the life history traits reproduction and survival of the rotifer Brachionus calyciflorus. Therefore, we tested three MP sizes (1, 3, 6 µm) of spherical shape and exposed the rotifer, first, in a short-term acute toxicity test by using increasing MP concentrations and food limitation as additional stressor. In this approach, we used silica beads of the same size range as MP as inorganic reference particles. Second, we tested the impact of the same MP spheres in a long-term chronical toxicity test by using one MP concentration, no reference particles, and food saturation. Thus, we excluded additional stress by food limitation to attribute any effect on life parameters to MP exposure. We chose these two types of particles because polystyrene is one of the most common plastic types found in aquatic ecosystems (Andrady, 2011; Mani et al., 2015), and silica is one of the most abundant minerals of the Earth’s crust and is formed during the weathering of rock formations.
2 Materials and methods
2.1 Test particles
To examine the impact of microplastics and more natural particles on life history traits of an aquatic microconsumer, we used polystyrene (PS) and silica (Si) microsized beads. PS was purchased from Polysciences Europe GmbH, green fluorescently (excitation 441 nm, emission 486 nm) with a density of 1.05 g cm−3. Si was purchased from microParticles GmbH Berlin, nonfluorescent with a density of 1.85 g cm−3. We used the Si particle as a reference particle for PS only in the short-term exposure experiments. Both test particles demonstrated diameters of approximately 1.0, 3.0, and 6.0 µm (detailed information in supplementary material, Supplementary Table S1), respectively. The particle stocks were prepared in MilliQ water in the required concentrations under sterile conditions and were stored in a fridge at 6°C in the dark to prevent bacterial growth. Particle concentrations were microscopically quantified using a hemocytometer (Neubauer improved; CH 30, Olympus Europa SE & Co. KG) and an Olympus (Europa SE & Co. KG) microscope. To avoid particle aggregation, the stocks were sonicated (Sonorex Digitec DT 514 BH, Bandelin electronic GmbH & Co. KG) 30 min before use. For the short-term acute toxicity test (AT, see section 2.4.1), we used the surfactant Tween 20 to prevent the aggregation of particles during the exposure time. Therefore, we added 10 µL of 1% surfactant Tween20-stock to the particle stocks.
2.2 Cultivation of rotifer and algae
For the experiments, we used the parthenogenetic monogonont rotifer B. calyciflorus s.s. (Strain USA, Paraskevopoulou et al., 2018) as a microconsumer, which was originally collected at lake Michigan and has been in continuous culture since 2001 in our lab. Rotifer cultures for the short-term AT were fed with the spherical, unicellular green alga Chlorella vulgaris (Chlorophyta; strain 211-11b, Culture Collection of Algae, University of Göttingen, Germany, mean equivalent spherical diameter = 3.4–3.7 µm). To exclude interference of the particle shape between nonfood and food particle in the AT, we decided to use the spherical alga C. vulgaris. For the long-term chronical toxicity test (CT), rotifers were fed with the sickle-shaped chlorophyte alga Monoraphidium minutum (strain 243–1, Culture Collection of Algae, University of Göttingen, Germany, mean equivalent spherical diameter = 3.4 µm). Both algae are in the same size range, of similar nutritional quality for B. calyciflorus, and are frequently used in laboratory experiments (C. vulgaris: Sarma et al., 2000, M. minutum: Schälicke et al., 2019). Rotifers were fed ad libitum every week with the respective algae. Algae and rotifers were kept in modified Woods Hole (WC) Medium (Guillard and Lorenzen, 1972) at 20°C, at a day/night cycle of 16:8 h, and at light intensity of 35 μmol m−2 s−1 photosynthetic active radiation. Algal cultures were grown in semi continuous batch cultures, to keep them in exponential growth phase. Rotifer cultures were regularly fed and transferred into fresh flasks.
2.3 Food concentrations at acute and chronic exposure
Next, we used different food levels for acute and chronic toxicity experiments. For the AT tests, we aimed for a food level that allows for moderate growth. No food would lead to 100% mortality after 4 days making it impossible to estimate toxicity effects of MP in relation to the control. Saturating (high) food concentrations might lead to aggregations interfering with the MP. Therefore, the food concentration at which population growth is half maximal was determined by measuring population growth at cell concentrations of (0.05 × 106, 0.125 × 106, 0.25 × 106, 0.5 × 106, 1 × 106, 2 × 106 cells mL−1) for 4 days. After fitting a saturation curve to the growth rate vs. food concentration data, the food concentration of half-maximal growth of 0.41 days−1 was 1.43 × 105 cells mL−1 (∼2.94 × 106 μm3 ml−1). For the CT, we wanted to test for a sublethal effect in relation to optimal environmental conditions. Thus, nonlimiting food conditions were chosen by using an algal concentration of 5.0 × 105 algae cells mL−1 (∼14.1 × 106 μm3 ml−1) of M. minutum (Fussmann et al., 2005). While MP studies often use mass or particle number as unit, to better compare treatments with the silica beads, we decided to use (bio)volume, because of their high specific weight. Previous studies demonstrated that B. calyciflorus ingests both nonfood particles (Drago et al., 2020; Drago and Weithoff, 2021); however, we did not quantify the actual ingestion in the present study.
2.4 Short-and long-term test approaches
We performed two short-term acute toxicity tests, one for 24 h (AT24) and one for 96 h (AT96), and one life table experiment (CT) to assess the whole life cycle. For the AT24/96 tests, we tested for the effect of three sizes of PS and silica in comparison to a control without artificial particles (only algae). For the life table experiments, we tested only PS, because the experimental set-up did not allow for sufficient turbulence to keep the silica beads in suspension as the PS did (see below).
2.4.1 Acute toxicity test (24 and 96 h)
In order to start the experiment with young animals of the same age, 4 days prior to the experiment, a subsample from the stock culture was transferred in six-well plates, each well containing 2 ml WC-Medium. Two days later, the animals were fed ad libitum with C. vulgaris to synchronize parthenogenetic reproduction. At experimental day 0, to remove remaining algae, adult rotifers were sieved through a 30 µm mesh (Macs SmartStrainer). Thereafter, subitaneous eggs were separated from the adult rotifers by using a vortexer (Vortex Genie 2) at maximum rotation velocity for approximately 1 min. Unattached subitaneous eggs in progressive state of development (Castellanos Paez et al., 1988) were selected to gain neonates of approximately same age for the experiment (∼2 h hatching span). To examine the short-term effects, B. calyciflorus was exposed to varying MP/silica particle concentrations. We applied a substitutive design by keeping the total volume of particles constant and varied the relative contribution of artificial food particle to the total amount of particles. The applied artificial particle concentrations were 0% (control, C. vulgaris: 2.94 × 106 μm3 ml−1), 20%, 33%, 43%, and 50% proportion of artificial particle to total particles, see supplementary material, Supplementary Table S2. Next, the AT24 test was performed with three and the AT96 test with four replicates. To prevent the aggregation of particles during the exposure time, we added 10 µL of 1% surfactant Tween20-stock to the particle stocks. The same amount of Tween was additionally added to MilliQ water stocks. To obtain the same concentration of Tween in each glass vials, these stocks were used for the lower particle volumes. To exclude hidden effects from the surfactant agent on B. calyciflorus, a control with Tween and food algal for both short-term tests were made. Since we found no effect of Tween, we pooled the data from the control and the Tween treatment. This ends up in a total of 78 vials for the 24-h and 104 vials for the 96-h test.
Soda-lime glass vials (Height 24 mm, Diameter 24 mm, Volume 7 ml, Carl Roth) were filled with WC-Medium (5 ml), the appropriate particle concentration, and the food alga. Each vial was shaken for 5 s (Vortex Genie 2), and then, detached subitaneous eggs were added to the vials. To prevent air bubbles, the remaining volume was filled with WC-Medium. Subsequently, glass vials were darkened with duct tape and fixed randomly on a plankton wheel for 24 h or 96 h at a rotational speed of 13 rpm at 20°C (±1°C). The vials were darkened to prevent algal growth during the exposure time. To determine the survival and growth rate of the rotifers after the end of each experiment, the content of each vial was transferred in a well of a six-well plate and rotifers (live and dead), and their eggs were counted using a Stereomicroscope (Stemi 2000 C Carl Zeiss). Animals were declared dead if no cilia nor mastax movement was observed for 5 s. The intrinsic population growth rate r (per day) was calculated according to the equation:
where N0 describes the number of rotifers at the beginning of the experiment, N1 the number of rotifers at the end of the experiment, and ∆t the time interval (4 days). Since in about one-third of the samples, no live rotifers were present after 96 h, we used the N1 + 1 transformation. No growth was detected after 24 h.
2.4.2 Chronical toxicity test: Life table experiment
Three days before the start of the CT, to ensure equal and good physiological conditions, the rotifers were fed daily with approximately 1 × 106 cells mL−1 of the food algal M. minutum. From this stock, 24 egg-bearing females were transferred into 24-well microtiter plates with the target experimental condition. The experiment started with the hatching of the neonates in their respective experimental condition. In all experiments (control and treatments), animals were provided with algal food at a concentration of 5 × 105 cells mL−1 (∼14.1 × 106 μm³ mL−1). For the PS treatment, a fixed particle volume of 9.52 × 105 μm³ mL−1 (∼1 μg ml−1) was used. Thus, the number of beads decreased with increasing bead sizes. The total volume of the algae was ca. 15 times higher than the volume of the beads (Supplementary Table S2).
Every 24 h (±1 h), the surviving rotifers were transferred in to a new well with the target conditions, and the number of eggs and neonates was recorded. The experiments were terminated when the initial rotifer died. Rotifers were declared dead when neither cilia nor mastax movement was detected after 5 s. Next, the experiment was run at 20°C (±1°C) in the dark to prevent algal growth, and the plates were placed randomly on a rocker shaker to keep PS in suspension. The experiments were run sequentially, and the plastic-free control was repeated in each run. Since no differences among the different controls were found, these data were pooled. The life table experiments provided data on life span and reproduction: the average life span, the age-specific survival (lx), and the age-specific fecundity (mx) were calculated; x was defined as the age level per day, lx as the proportion of surviving animals at the beginning of an age level, and mx as the number of offspring per surviving animal (Begon et al., 1996). The age-specific net fecundity (lxmx), the net reproduction rate (R0, Eq. (2)), the generation time (T, Eq. 3), and the intrinsic population growth rate r (Eq. 1) were calculated as well.
The intrinsic population growth rate r was calculated using the bootstrapping with 199 iterations (Weithoff and Wacker, 2007). To calculate the standard error, the 95% confidence interval obtained by the bootstrapping method was used (Higgins et al., 2019). For better comparability between results from the AT96 and CT, r was calculated after the first 96 h. To prevent zeros, the data were N1 + 1 transformed.
2.5 Statistical analyses
All data (AT and CT) were tested for normal distribution and homoscedasticity. We used One-way ANOVA for data analysis. If test requirements did not apply, nonparametric Kruskal–Wallis (KW) test or the Scheirer–Ray–Hare (SRH) test (Scheirer et al., 1976) was conducted. When significant results (p ≤ 0.05) of the above tests were obtained, Tukey HSD and Dunn’s test with Bonferroni correction were used as posthoc procedures. In general, we tested the influence of nonfood particles, size of the particles, the concentrations, and the time (only AT) as well as the interaction of these parameters on the life history traits of B. calyciflorus. In the AT, we tested specifically, if differences between both nonfood-particle types could be found.
3 Results
3.1 Survival
Survival (N24) of B. calyciflorus after 24 h (AT24) was neither affected by PS nor by Si particles of any size or any tested particle density (Figure 1A, SRH test: p > 0.05, KW test: p > 0.1, Supplementary Table S3, S4). After 96 h (AT96), we also found no effect of particle type (SRH test: p = 0.9) or particle size (SRH test: p = 0.73) on rotifer survival (Supplementary Table S5). However, a variable decrease in survival was observed in response to artificial particles compared to the control. In detail, exposure to nonfood particles (PS, Si) induced a decrease in survival N96 of the rotifers (Figure 1B) for one size of PS and for all three sizes of Si compared to the control group (KW test: PS: p = 6 µm = 0.01; Si: p = 1, 3, 6 µm = 0.02, Supplementary Table S6). However, no clear trend emerged with respect to the increase of nonfood proportions (Figure 1B). While no significant differences from the control were found for 1 and 3 μm PS with increasing concentrations (KW test: p = 1 µm = 0.1, 3 µm = 0.08), a decrease in rotifers was observed with Si particles for these two sizes: at 50% and 20% food/nonfood ratio (Dunn´s test: p = 1 µm = 0.01, 3 µm = 0.03, respectively). Interestingly, survival was significantly different from control for the 6 µm for both particle types at the lowest (20%) (Dunn’s test: P = PS = 0.03, Si = 0.04) and highest food/nonfood proportion (50%) (Dunn’s test: P = PS/Si = 0.02, Supplementary Table S7).
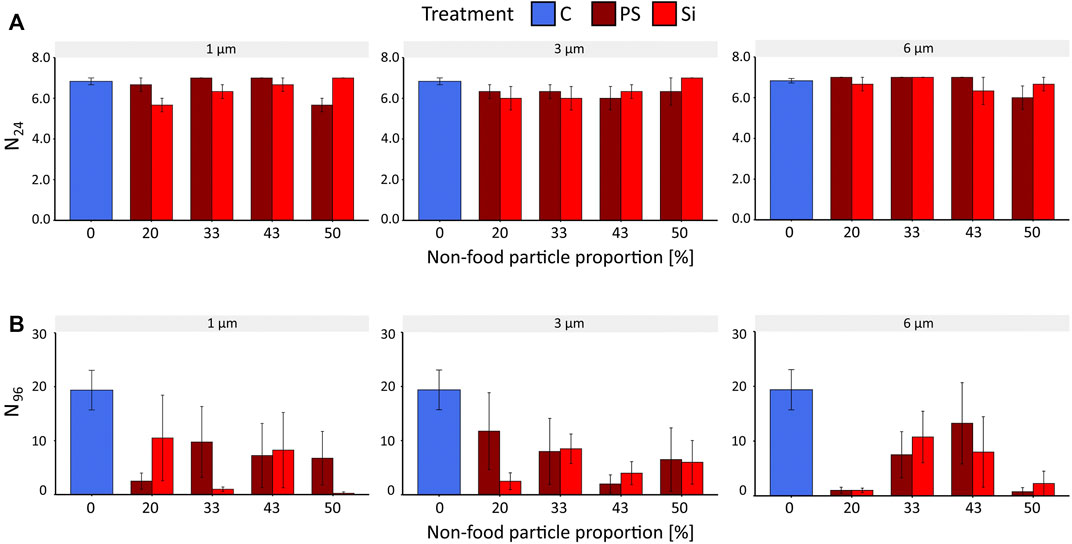
FIGURE 1. Survival of B. calyciflorus after exposure to polystyrene and silica particles of three sizes (1, 3, and 6 µm) and different concentrations (0–50% food/nonfood proportion) and the control (C, food particle only). (A) survival expressed in rotifers alive after 24 h (N24) of the acute toxicity test (AT24). (B) Survival expressed in rotifers alive after 96 h (N96) of the acute toxicity test (AT96). Data were expressed as mean ± standard error.
In CT, instead, no significant difference in survival of B. calyciflorus could be detected in the PS treatments compared to the control (Figures 2A,C). The average life span varied nonsignificantly between 5.0 and 6.6 days among treatments. (One-way ANOVA: for PS presence, p = 0.0957, Supplementary Table S8).
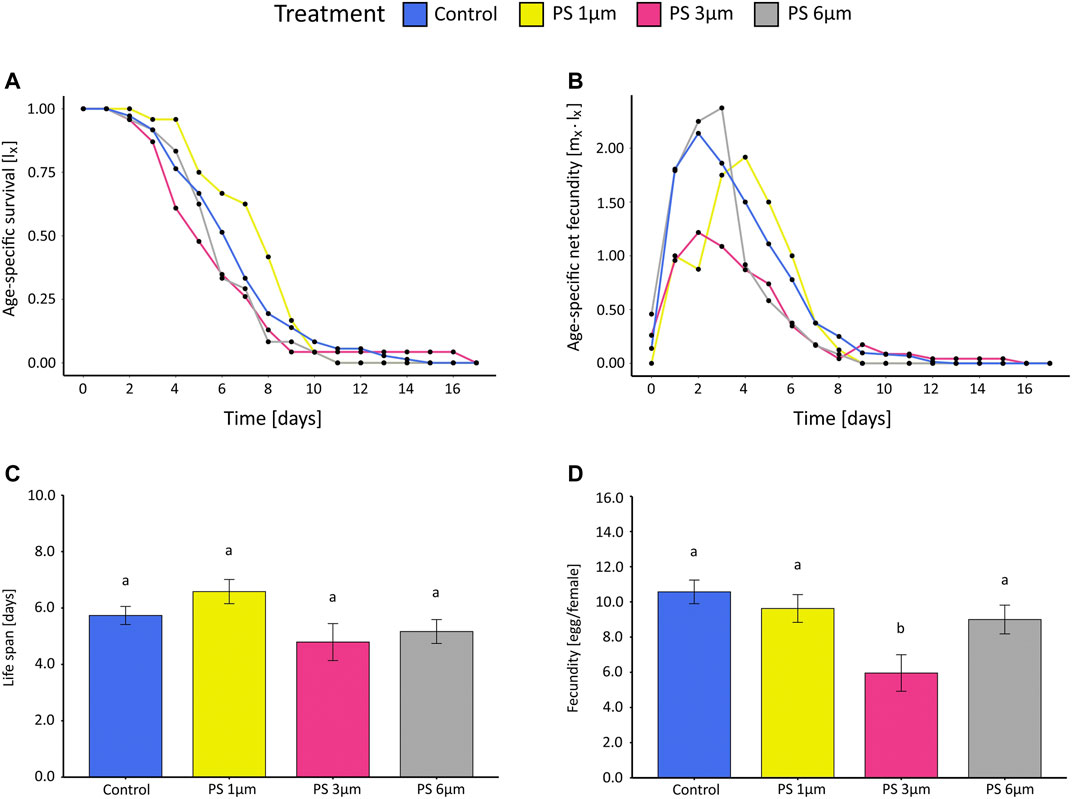
FIGURE 2. Results of the life table experiments (CT) for B. calyciflorus exposed to polystyrene beads of three sizes (1, 3, and 6 µm) and the control (Monoraphidium minutum). (A) age-specific survival (lx), (B) age-specific net fecundity (mx ˑ lx), (C) average life span in days, and (D) averaged fecundity expressed as eggs per female. Data for (C) and (D) were expressed as mean ± standard error. Different letters above columns indicate significant differences (p < 0.05).
3.2 Reproduction and growth rate
No reproduction occurred in the AT24; thus, the intrinsic rate of population growth r was calculated only for AT96, and CT96 (r of the CT was calculated after 96 h for reasons of comparison). While the growth rate for the control of the AT96 was 0.22 (±0.06SE) day−1, the mean growth rate in both particle treatments (AT96) was either negative, or slightly above zero, for the 3 and 6 µm Si treatments (Supplementary Table S13). No effect of the particle sizes (SRH test: p = 0.72), the particle type (SRH test: p = 0.89), the interaction of the two (SRH test: p = 0.57, Supplementary Table S9) was found for either nor for increasing concentrations (SRH test: p = PS = 0.32, Si = 0.12, Supplementary Table S10). We only found effects on the intrinsic population growth rate r compared to the control (Figure 3, Supplementary Table S11). For the AT96 and the CT test, different food levels were tested, and thus, the effects of PS are compared in the light of presence and absence of food limitation (Figure 4). The food levels resulted in a growth rate of 0.71 (±0.07 SE) at high food and of 0.22 (±0.06 SE) at low food, which is comparable to other studies using B. calyciflorus and these two food algae (Rothhaupt, 1990; Weithoff and Walz, 1995; Sarma et al., 2001). In general, growth rates in CT at high food were significant higher as in the AT96 at low food (KW test: p = 0.02, Supplementary Table S12). The age-specific net fecundity (Figure 2B) showed a delayed reproduction for 1 μm PS and a decreased total reproduction for 3 μm PS compared to the control. Averaged egg production (mean eggs per female: 1 µm = 9.6, 3 µm = 6.2, 6 µm = 9.0, control = 10.6) in the CT differed significantly between 3 µm and the control group (TukeyHSD: p = 0.001, Figure 2D, Supplementary Table S8). Detailed results of the CT test are listed in supplementary material (Supplementary Table S14).
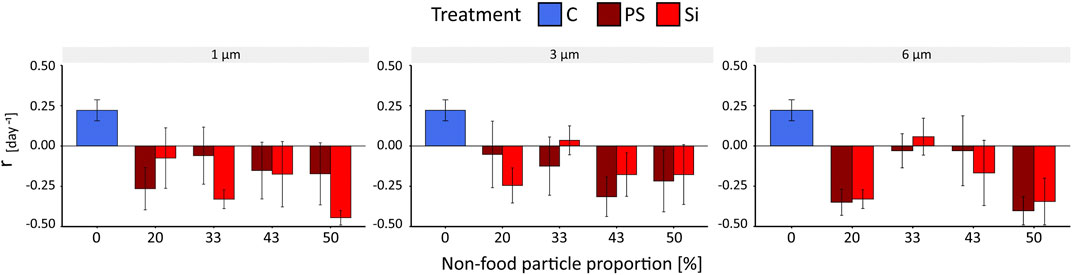
FIGURE 3. Intrinsic rate of population growth r (day−1) of B. calyciflorus after exposure to polystyrene and silica particles of three sizes (1, 3, and 6 µm) and different concentrations (0–50% food/nonfood proportion) and the control (C, food-particle only) for 96 h (AT96). Data were expressed as mean ± standard error.
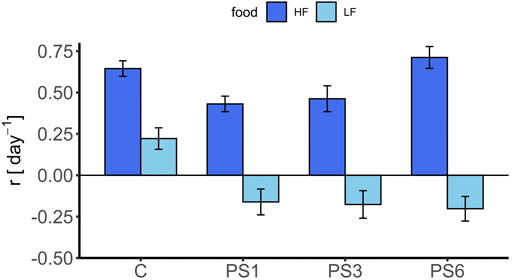
FIGURE 4. Intrinsic rate of population growth after 96 h of B. calyciflorus for the acute toxicity and chronical toxicity test. Shown are the results for the nonfood particle (polystyrene) for 1, 3, and 6 µm beads and the respective control group (C, food-particle only). Data were expressed as mean ± standard error.
4 Discussion
4.1 No acute toxicity of polystyrene
Ourresults indicate that short-term exposure (AT24) to PS in the size classes 1, 3, and 6 µm and at varying concentrations (6, 20, 33, 43 and 50% nonfood-particle proportion) did not demonstrate an acute toxic effect on the rotifer B. calyciflorus. These findings are confirmed by other studies for PS and for a range of aquatic organisms (Gambardella et al., 2018; Eltemsah and Bøhn, 2019). The assumption exists that microplastic, especially of spherical shape, can be easily ingested but also egested (Cole et al., 2013; Scherer et al., 2017). Nevertheless, we found an impact of microplastics on B. calyciflorus at longer timescales. The reproduction decreased in comparison with the control groups both, in the AT96 and the CT test, whereas the survival was only affected in the AT96 test. As reported in other studies, it seems that the reproductive output of aquatic invertebrates is more affected by the uptake of microplastic than their survival (Lee et al., 2013; Jaikumar et al., 2019). The pattern of reduced reproduction along with no clear effect on life span can be explained ecophysiologically. Many animals including rotifers (Weithoff, 2007; Snell, 2014) respond to a reduced food supply with a prolongation in life span on the expense of reproduction. A shortening in life span will only occur at exceptionally severe food limitation close to starvation. Since many studies do not cover the whole life cycle, changes in life span (in particular extensions) cannot be detected. In our study, a tendency from this ecophysiological pattern can be seen in the 1 µm treatment. In the first days, reproduction was slightly delayed, and survival was still relatively high, while at the peak of reproduction, it then continuously decreased.
4.2 Particle vs. microplastic effect
Between PS and Si, no significant effects were found neither with increasing particle size nor with increasing concentration. The results of the AT96 suggest a particle rather than a microplastic effect. Similar life history responses were determined in a study by Ogonowski et al. (2016) with D. magna when comparing pristine PE beads with kaolin. Also, Yap et al. (2020), who used red clay as natural particles and PVC to study a potential effect of byssus production, respiration, and survival rate in a marine mussel, found no difference between the two particle types. Next, interestingly, Coady et al. (2020) found, in their study, with D. magna no differences at all in the investigated life history traits between control (laboratory dilution water), the reference particles (fumed nanosized silica), and the used microplastic (nanosized ethylene acryl acid copolymer). Next, contrary, Harris and Carrington (2019) and Zimmermann et al. (2020) reported, in their studies, with the mussel Mytilus trossulus and the cladoceran D. magna that MP, but not the tested natural particles, negatively affected the life parameters of these organisms. The reasons for the different results might be manifold, for example due to the type, size, or shape of the particle tested, the concentration used, the organisms, the duration of the experiment, the observed life history traits, or the experimental design. Especially when conducting experiments with particles, its effect on the organism strongly depends on its bioavailability, which can be modified by aggregation (Drago et al., 2020) and sedimentation (Schür et al., 2020). Until now, only a few studies used reference or natural particles in microplastic ecotoxicology tests with aquatic organisms. However, these and our results show that an urgent need exists to use a reference particle instead of a commonly used particle-free control to evaluate a potential plastic effect and thus a valid risk assessment of MP for aquatic organisms (Connors et al., 2017; Ogonowski et al., 2018).
4.3 High food vs. low food
In the AT24 and AT96, we tested food limitation as an additional stressor, which was excluded in the CT, where sufficient food algae were available. After 24 h, food limitation exhibits no effect on survival, because B. calyciflorus is able to survive starvation during the first 24 h (Kirk, 1997). In the AT96, particle exposure caused comparable effects in the life history traits of B. calyciflorus for all three sizes and food/nonfood-particle proportions, and these differed markedly from the particle-free control. The most obvious cause for this is likely to be a dilution of the food alga (Kong and Koelmans, 2019). As a consequence, the ingestion of food particles decreased, which resulted in declining energy uptake that ultimately affected life history traits (Arruda et al., 1983; McCabe and O’Brien, 1983). When comparing the results of the AT, this process can be seen by monitoring N(t). After 24 h, no difference in survival was observed between particle-free (food algae only) and particle treatments (PS, Si), as sufficient food algae were available for the starting population of 7 rotifers. Only during the course (AT96) of the experiment and increasing population size, subsequently higher algae consumption rate potentially led to a deterioration of the food/nonfood-particle ratio, and the dilution effect became apparent. Further evidence for this explanation came from the life table experiments (CT), where the algae dilution factor (∼6% of the diet) was low. In this case, enough food algae could be ingested to compensate the nutrient deficiency that occurred during the ingestion of nonfood particles. For this reason, the ratio between food/nonfood particles is crucial for life history trait outcomes, as food availability can alleviate nonfood particle (e.g. MPs) effects in B. calyciflorus (Drago and Weithoff, 2021; Xue et al., 2021) and D. magna (Ogonowski et al., 2016). Xue et al. (2021) reported a decrease in net reproduction rate during the exposure to polyethylene microbeads, but this effect could be mitigated by increasing the concentration of food algae. That supports our hypothesis that a diluting effect or the ratio between food/nonfood particles plays a more important role for life history traits of B. calyciflorus than a direct toxic effect of PS microplastics. In general, sufficient food sources often attenuate trait responses to toxic stress stimuli in rotifers (Cecchine and Snell, 1999; Sarma et al., 2001) and daphnids (Reinikainen et al., 1994; Pereira and Goncalves, 2007). Nevertheless, we found a decreased reproduction in the CT, even under high food conditions in the 3 µm treatment, which indicates that 3 μm MP was ingested along with the same sized algae (M. minutum: ∼3.5 µm in diameter; Drago et al., 2020). In a study by DeMott (1986) with B. calyciflorus, researchers showed that no discrimination was found between particles with and without nutritional value; thus, B. calyciflorus does not seem to be able to discriminate between food algae and MP. In light of these findings, a risk of mistakenly ingestion of MPs appears when they are in the same size range as the corresponding food particles. Considering the many studies that demonstrated MP ingestion in zooplankton species and additionally the continuous release of plastic debris into aquatic ecosystems, as well as the continued degradation of plastics, researchers suggested that reproduction affected by MP ingestion could result in implications for entire food webs and associated ecosystem services.
4.4 Environmental relevance of the study
The concentrations of MP used in the experiment are, by far, higher than the ones that have been found in freshwater ecosystems. However, higher MP concentrations compared to MP assessments in water columns of lakes and rivers are quite conceivable near point and nonpoint sources such as wastewater treatment plants (WWTP) or during/after storm or heavy rain events. For example, Hitchcock (2020) showed that the concentration of MP per m3 water was increased 40-fold during a storm event. It should be noted that in most cases for MP concentration surveys, mesh widths of < 300 µm were used (Barrows et al., 2017; Mendoza and Balcer, 2019; Lindeque et al., 2020). However, this does not capture the fraction of small MP that falls within the food size spectrum of a broad range of zooplankton species (Cole et al., 2013; Botterell et al., 2019). The indication of underestimated high concentrations of small MP are shown in studies of Wiggin and Holland (2019) and Enders et al. (2015) for marine and estuarine ecosystems, where the smallest size fraction of MP (3–20 and 10 µm, resp.) accounted for the highest MP proportion. Thus, the expectation exists that the concentrations of small MP found in the future will increase by employing advanced methods and also due to continuous physical fragmentation of larger plastics (Andrady, 2011). However, it should be emphasized that we used pristine PS without additives like plasticizer, flame-retardants, or UV stabilizers, which are bioavailable for animals and are suspected to be toxic when ingested (Rochman, 2015). Microplastics are also known for their potential to adsorb organic pollutants like pesticides (Li et al., 2021), which could also be a threat for aquatic organisms. Their implementation might have altered our results, as shown in a study by Schrank et al. (2019) using D. magna and leaching additives.
5 Conclusion
We conclude that the combination of short- and long-term experiments in ecotoxicology provide a much deeper understanding of the underlying processes of potential toxicity. Further, this appears to be particularly true for sublethal effects such as delayed reproduction or changes in life span.
Data availability statement
The original contributions presented in the study are included in the article/Supplementary Material, and further inquiries can be directed to the corresponding author.
Author contributions
JP, DN, and GW designed the experiments. JP and DN conducted the experiment and performed the analysis of the data. JP, DN, CD, and GW wrote the manuscript. All authors discussed the results and provided extensive comments on the manuscript concerning the analysis, interpretation, and writing. All authors approved the final version and accepted accountability for all aspects of the work.
Funding
This research was supported by the BMBF project MikroPlaTaS (02WPL1448C) granted to GW. Funded by the Deutsche Forschungsgemeinschaft (DFG, German Research Foundation) – Project number 491466077.
Acknowledgments
We thank S. Donath, C. Schirmer, and S. Saumweber for providing us with samples and technical support. We also thank the reviewers for their comments that improved the manuscript.
Conflict of interest
The authors declare that the research was conducted in the absence of any commercial or financial relationships that could be construed as a potential conflict of interest.
Publisher’s note
All claims expressed in this article are solely those of the authors and do not necessarily represent those of their affiliated organizations, or those of the publisher, the editors, and the reviewers. Any product that may be evaluated in this article, or claim that may be made by its manufacturer, is not guaranteed or endorsed by the publisher.
Supplementary material
The supplementary material for this article can be found online at: https://www.frontiersin.org/articles/10.3389/fenvs.2022.955425/full#supplementary-material
References
Allen, S., Allen, D., Phoenix, V. R., Le Roux, G., Durántez Jiménez, P., Simonneau, A., et al. (2019). Atmospheric transport and deposition of microplastics in a remote mountain catchment. Nat. Geosci. 12, 339–344. doi:10.1038/s41561-019-0335-5
An, D., Na, J., Song, J., and Jung, J. (2021). Size-dependent chronic toxicity of fragmented polyethylene microplastics to Daphnia magna. Chemosphere 271, 129591. doi:10.1016/j.chemosphere.2021.129591
Andrady, A. L. (2011). Microplastics in the marine environment. Mar. Pollut. Bull. 62, 1596–1605. doi:10.1016/j.marpolbul.2011.05.030
Arndt, H. (1993). Rotifers as predators on components of the microbial web (bacteria, heterotrophic flagellates, ciliates) – a review. Hydrobiologia 255, 231–246. doi:10.1007/BF00025844
Arruda, J. A., Marzholf, G. R., and Faulk, R. T. (1983). The role of suspended sediments in the nutrition of zooplankton in turbid reservoirs. Ecology 64, 1225–1235. doi:10.2307/1937831
Barrows, A. P. W., Neumann, C. A., Bergera, M. L., and Shaw, S. D. (2017). Grab vs. neuston tow net: a microplastic sampling performance comparison and possible advances in the field. Anal. Methods 9, 1446–1453. doi:10.1039/c6ay02387h
Begon, M., Harper, J. L., and Townsend, C. R. (1996). Ecology: Individuals, populations, and communities. 3rd edition. Cambridge, MA: Blackwell Science Ltd.
Bergmann, M., Mützel, S., Primpke, S., Tekman, M. B., Trachsel, J., Gerdts, G., et al. (2019). White andwonderful? Microplastics prevail in snow from the alps to the artic. Sci. Adv. 5, eaax1157. doi:10.1126/sciadv.aax1157
Blettler, M. C. M., Abrial, E., Khan, F. R., Sivri, N., and Espinola, L. A. (2018). Freshwater plastic pollution: recognizing research biases and identifying knowledge gaps. Water Res. 143, 416–424. doi:10.1016/j.watres.2018.06.015
Botterell, Z. L. R., Beaumont, N., Dorrington, T., Steinke, M., Thompson, R. C., Lindeque, P. K., et al. (2019). Bioavailability and effects of microplastics on marine zooplankton: a review. Environ. Pollut. 245, 98–110. doi:10.1016/j.envpol.2018.10.065
Cappello, T., De Marco, G., Conti, G. O., Giannetto, A., Ferrante, M., Mauceri, A., et al. (2021). Time-dependent metabolic disorders induced by short-term exposure to polystyrene microplastics in the Mediterranean mussel Mytilus galloprovincialis. Ecotoxicol. Environ. Saf. 209, 111780. doi:10.1016/j.ecoenv.2020.111780
Carpenter, E. J., and Smith, K. L. (1972). Plastics on the sargasso sea surface. Science 175, 1240–1241. doi:10.1126/science.175.4027.1240
Castellanos Paez, M. E., Kurokura, H., and Kasahara, S. (1988). Embryonic development of amictic eggs of a rotifer Brachionus plicatilis. J. Fac. Appl. Biol. Sci. 27, 93–99.
Cecchine, G., and Snell, T. W. (1999). Toxicant exposure increases threshold food levels in freshwater rotifer populations. Environ. Toxicol. 14, 523–530. doi:10.1002/(sici)1522-7278(199912)14:5<523::aid-tox6>3.0.co;2-2
Coady, K. K., Burgoon, L., Doskey, C., and Davis, J. W. (2020). Assessment of transcriptomic and apical responses of Daphnia magna exposed to a polyethylene microplastic in a 21-d chronic study. Environ. Toxicol. Chem. 39, 1578–1589. doi:10.1002/etc.4745
Cole, M., Lindeque, P., Fileman, E., Halsband, C., and Galloway, T. S. (2015). The impact of polystyrenemicroplastics on feeding, function and fecundity in the marine copepod Calanus helgolandicus. Environ. Sci. Technol. 49, 1130–1137. doi:10.1021/es504525u
Cole, M., Lindeque, P., Fileman, E., Halsband, C., Goodhead, R., Moger, J., et al. (2013). Microplastic ingestion by zooplankton. Environ. Sci. Technol. 47, 6646–6655. doi:10.1021/es400663f
Connors, K. A., Dyer, S. D., and Belanger, S. E. (2017). Advancing the quality of environmental microplastic research. Environ. Toxicol. Chem. 36, 1697–1703. doi:10.1002/etc.3829
DeMott, W. R. (1986). The role of taste in food selection by freshwater zooplankton. Oecologia 69, 334–340. doi:10.1007/BF00377053
Di, M., and Wang, J. (2018). Microplastics in surface waters and sediments of the three gorges reservoir, China. Sci. Total Environ. 616-617, 1620–1627. doi:10.1016/j.scitotenv.2017.10.150
Drago, C., Pawlak, J., and Weithoff, G. (2020). Biogenic aggregation of small microplastics alters their ingestion by a common freshwater micro-invertebrate. Front. Environ. Sci. 8, 574274. doi:10.3389/fenvs.2020.574274
Drago, C., and Weithoff, G. (2021). Variable fitness response of two rotifer species exposed to microplastics particles: the role of food quantity and quality. Toxics 9, 305. doi:10.3390/toxics9110305
Eltemsah, Y. S., and Bøhn, T. (2019). Acute and chronic effects of polystyrene microplastics on juvenile and adult Daphnia magna. Environ. Pollut. 254, 112919. doi:10.1016/j.envpol.2019.07.087
Enders, K., Lenz, R., Stedmon, C. A., and Nielson, T. G. (2015). Abundance, size and polymer composition ofmarine microplastics ≥10 μm in the Atlantic Ocean and their modelled vertical distribution. Mar. Pollut. Bull. 100, 70–81. doi:10.1016/j.marpolbul.2015.09.027
Eriksen, M., Lebreton, L. C. M., Carson, H. S., Thiel, M., Moore, C. J., Borerro, J. C., et al. (2014). Plastic pollution in the world’s oceans: more than 5 trillion plastic pieces weighing over 250, 000 tons afloat at sea. PLoS One 9, e111913. doi:10.1371/journal.pone.0111913
Fischer, E. K., Paglialonga, L., Czech, E., and Tamminga, M. (2016). Microplastic pollution in lakes and lake shoreline sediments – A case study on lake bolsena and lake chiusi (central Italy). Environ. Pollut. 213, 648–657. doi:10.1016/j.envpol.2016.03.012
Fok, L., Cheng, N. Y. I., and Yeung, Y.-Y. (2019). “Mismanaged plastic waste: far side of the moon,” in Environmental sustainability and education for waste management: implications for policy and practise. Editors W. W. M. So, C. F. Chow, and J. C. K. Lee (Singapore: Springer), 57–71. doi:10.1007/978-981-13-9173-6_5
Frydkjær, C. K., Iversen, N., and Roslev, P. (2017). Ingestion and egestion of microplastics by the cladoceran Daphnia magna: effects of regular and irregular shaped plastic and sorbed phenanthrene. Bull. Environ. Contam. Toxicol. 99, 655–661. doi:10.1007/s00128-017-2186-3
Fussmann, G. F., Weithoff, G., and Yoshida, T. (2005). A direct, experimental test of resource vs. consumer dependence. Ecology 86, 2924–2930. doi:10.1890/04-1107
Gambardella, C., Morgana, S., Bramini, M., Rotini, A., Manfra, L., Migliore, L., et al. (2018). Ecotoxicological effects of polystyrene microbeads in a battery of marine organisms belonging to different trophic levels. Mar. Environ. Res. 141, 313–321. doi:10.1016/j.marenvres.2018.09.023
Gambardella, C., Morgana, S., Ferrando, S., Bramini, M., Piazza, V., Costa, E., et al. (2017). Effects of polystyrene microbeads in marine planktonic crustaceans. Ecotoxicol. Environ. Saf. 145, 250–257. doi:10.1016/j.ecoenv.2017.07.036
Guillard, R. R. L., and Lorenzen, C. J. (1972). Yellow-green algae with chlorophyllide C12. J. Phycol. 8, 10–14. doi:10.1111/j.1529-8817.1972.tb03995.x
Harris, L. S. T., and Carrington, E. (2019). Impacts of microplastic vs. natural abiotic particles on the clearance rate of a marine mussel. Limnol. Oceanogr. Lett. 5, 66–73. doi:10.1002/lol2.10120
Hart, R. C. (1988). Zooplankton feeding rates in relation to suspended sediment content: potential influences on community structure in a turbid reservoir. Freshw. Biol. 19, 123–139. doi:10.1111/j.1365-2427.1988.tb00334.x
Higgins, J. P. T., Li, T., and Deeks, J. J. (2019). “Choosing effect measures and computing estimates of effect,” in Cochrane handbook for systematic reviews of interventions. Editors J. P. T. Higgins, J. Thomas, J. Chandler, M. Cumpston, T. Li, M. J. Pageet al. (Chichester: John Wiley and Sons) (Cochrane, 2019, version 6.0, updated July 2019).
Hitchcock, J. N. (2020). Storm events as key moments of microplastic contamination in aquatic ecosystems. Sci. Total Environ. 734, 139436. doi:10.1016/j.scitotenv.2020.139436
Imhof, H. K., Rusek, J., Thiel, M., Wolinska, J., and Laforsch, C. (2017). Do microplastic particles affect Daphnia magna at the morphological, life history and molecular level? PLoS One 12, e0187590. doi:10.1371/journal.pone.0187590
Jaikumar, G., Brun, N. R., Vijver, M. G., and Bosker, T. (2019). Reproductive toxicity of primary and secondary microplastics to three cladocerans during chronic exposure. Environ. Pollut. 249, 638–646. doi:10.1016/j.envpol.2019.03.085
Jeong, C.-B., Won, E.-J., Kang, H.-M., Lee, M.-C., Hwang, D.-S., Hwang, U.-K., et al. (2016). Microplastic size-dependent toxicity, oxidative stress induction, and p-JNK and p-p38 activation in the monogonont rotifer (Brachionus koreanus). Environ. Sci. Technol. 50, 8849–8857. doi:10.1021/acs.est.6b01441
Kanhai, L. D. K., Officer, R., Lyashevska, O., Thompson, R. C., and O´Connor, I. (2017). Microplastic abundance, distribution and composition along a latitudinal gradient in the Atlantic Ocean. Mar. Pollut. Bull. 115, 307–314. doi:10.1016/j.marpolbul.2016.12.025
Kapp, K. J., and Yeatman, E. (2018). Microplastic hotspots in the snake and lower columbia rivers: a journey from the greater yellowstone ecosystem to the pacific ocean. Environ. Pollut. 241, 1082–1090. doi:10.1016/j.envpol.2018.06.033
Kirk, K. L., and Gilbert, J. J. (1990). Suspended clay and the population dynamics of planktonic rotifers and cladocerans. Ecology 71, 1741–1755. doi:10.2307/1937582
Kirk, K. L. (1997). Life-history response to variable environments: starvation and reproduction in planktonic rotifers. Ecology 78, 434–441. doi:10.1890/0012-9658(1997)078[0434:LHRTVE]2.0.CO;2
Kirk, L. K. (1991). Suspended clay reduces Daphnia feeding rate: behavioural mechanisms. Freshw. Biol. 25, 357–365. doi:10.1111/j.1365-2427.1991.tb00498.x
Kong, X., and Koelmans, A. A. (2019). Modeling decreased resilience of shallow lake ecosystems toward eutrophication due to microplastic ingestion across the food web. Environ. Sci. Technol. 53, 13822–13831. doi:10.1021/acs.est.9b03905
Lee, K.-W., Shim, W. J., Kwon, O. Y., and Kang, J.-H. (2013). Size-dependent effects of micro polystyrene particles in the marine copepod Trigriopus japonicas. Environ. Sci. Technol. 47, 11278–11283. doi:10.1021/es401932b
Li, H., Wang, F., Li, J., Deng, S., and Zhang, S. (2021). Adsorption of three pesticides on polyethylene microplastics in aqueous solutions: kinetics, isotherms, thermodynamics, and molecular dynamics simulation. Chemosphere 264, 128556. doi:10.1016/j.chemosphere.2020.128556
Lindeque, P. K., Cole, M., Coppock, R. L., Lewis, C. N., Miller, R. Z., Watts, A. J. R., et al. (2020). Are we underestimating microplastic abundance in the marine environment? A comparison of microplastic capture with nets of different mesh-size. Environ. Pollut. 265, 114721. doi:10.1016/j.envpol.2020.114721
Maes, T., Barry, J., Stenton, C., Roberts, E., Hicks, R., and Bignell, J. (2020). The world is your oyster: low-dose, long-term microplastic exposure of juvenile oysters. Heliyon 6, e03103. doi:10.1016/j.heliyon.2019.e03103
Mani, T., Hauk, A., Walter, U., and Burkhardt-Holm, P. (2015). Microplastics profile along the rhine river. Sci. Rep. 5, 17988. doi:10.1038/srep17988
McCabe, G. D., and O’Brien, W. J. (1983). The effects of suspended silt on feeding and reproduction of Daphnia pulex. Am. Midl. Nat. 110, 324. doi:10.2307/2425273
Mendoza, L. M. R., and Balcer, M. (2019). Microplastics in freshwater environments: a review of quantification assessment. TrAC Trends Anal. Chem. 113, 402–408. doi:10.1016/j.trac.2018.10.020
Naidu, S. A., Rao, V. R., and Ramu, K. (2018). Microplastics in the benthic invertebrates from the coastal waters of kochi, southeastern arabian sea. Environ. Geochem. Health 40, 1377–1383. doi:10.1007/s10653-017-0062-z
Ogonowski, M., Gerdes, Z., and Gorokhova, E. (2018). What we know and what we think we know about microplastic effects – a critical perspective. Curr. Opin. Environ. Sci. Health 1, 41–46. doi:10.1016/j.coesh.2017.09.001
Ogonowski, M., Schür, C., Jarsen, A., and Gorokhova, E. (2016). The effects of natural and anthropogenic microparticles on individual fitness in Daphnia magna. PLoS One 11, e0155063. doi:10.1371/journal.pone.0155063
Paraskevopoulou, S., Tiedemann, R., and Weithoff, G. (2018). Differential response to heat stress among evolutionary lineages of an aquatic invertebrate species complex. Biol. Lett. 14, 20180498. doi:10.1098/rsbl.2018.0498
Pereira, J. L., and Goncalves, F. (2007). Effects of food availability on the acute and chronic toxicity of the insecticide methomyl to Daphnia spp. Sci. Total Environ. 386, 9–20. doi:10.1016/j.scitotenv.2007.07.040
Phothakwanpracha, J., Lirdwitayaprasit, T., and Pairohakul, S. (2021). Effects of sizes and concentrations of different types of microplastics on bioaccumulation and lethality rate in the green mussel, Perna viridis. Mar. Pollut. Bull. 173, 112954. doi:10.1016/j.marpolbul.2021.112954
Piehl, S., Leibner, A., Löder, M. G. J., Dris, R., Bogner, C., Laforsch, C., et al. (2018). Identification andquantification of macro- and microplastics on an agricultural farmland. Sci. Rep. 8, 17950. doi:10.1038/s41598-018-36172-y
Qiao, R., Deng, Y., Zhang, S., Borri Wolosker, M., Zhu, Q., Ren, H., et al. (2019). Accumulation of different shapes of microplastics initiates intestinal injury and gut microbiota dysbiosis in the gut of zebrafish. Chemosphere 236, 124334. doi:10.1016/j.chemosphere.2019.07.065
Reinikainen, M., Ketola, M., and Walls, M. (1994). Effects of the concentration of toxic Microcystis aeruginosa and alternative food on the survival of Daphnia pulex. Limnol. Oceanogr. 39, 424–432. doi:10.4319/lo.1994.39.2.0424
Rochman, C. M. (2015). “The complex mixture, fate and toxicity of chemicals associated with plastic debris in the marine environment,” in Marine anthropogenic litter. Editors M. Bergmann, L. Gutow, and M. Klages (Cham: Springer). doi:10.1007/978-3-319-16510-3_5
Rothhaupt, K. O. (1990). Population growth rates of two closely related rotifer species: effects of food quantity, particle size, and nutritional quality. Freshw. Biol. 23, 561–570. doi:10.1111/j.1365-2427.1990.tb00295.x
Sarma, S. S., Nandini, S., and Flores, J. L. (2001). Effect of methyl parathion on the population growth of the rotifer Brachionus patulus (O.F. Müller) under different algal food (Chlorella vulgaris) densities. Ecotoxicol. Environ. Saf. 48, 190–195. doi:10.1006/eesa.2000.2014
Sarma, S. S., Ramírez Pérez, T., and Nandini, S. (2000). Comparison of the sensitivity of Brachionus calyciflorus and Brachionus patulus (Rotifera) to selected heavy metals under low and high food (Chlorella vulgaris) levels. Bull. Environ. Contam. Toxicol. 64, 735–739. doi:10.1007/s001280000065
Schälicke, S., Sobisch, L.-Y., Martin-Creuzburg, D., and Wacker, A. (2019). Food quantity–quality co-limitation: interactive effects of dietary carbon and essential lipid supply on population growth of a freshwater rotifer. Freshw. Biol. 64, 903–912. doi:10.1111/fwb.13272
Scheirer, C. J., Ray, W. S., and Hare, N. (1976). The analysis of ranked data derived from completely randomized factorial designs. Biometrics 32, 429. doi:10.2307/2529511
Scherer, C., Brennholt, N., Reifferscheid, G., and Wagner, M. (2017). Feeding type and development drive the ingestion of microplastics by freshwater invertebrates. Sci. Rep. 7, 17006. doi:10.1038/s41598-017-17191-7
Schrank, I., Trotter, B., Dummert, J., Scholz-Böttcher, B. M., Löder, M. G. J., Laforsch, C., et al. (2019). Effects of microplastic particles and leaching additive on the life history and morphology of Daphnia magna. Environ. Pollut. 255, 113233. doi:10.1016/j.envpol.2019.113233
Schür, C., Zipp, S., Thalau, T., and Wagner, M. (2020). Microplastics but not natural particles induce multigenerational effects in Daphnia magna. Environ. Pollut. 260, 113904. doi:10.1016/j.envpol.2019.113904
Setälä, O., Fleming-Lehtinen, V., and Lehtiniemi, M. (2014). Ingestion and transfer of microplastics in theplanktonic food web. Environ. Pollut. 185, 77–83. doi:10.1016/j.envpol.2013.10.013
Snell, T. W. (2014). Rotifers as models for the biology of aging. Int. Rev. Hydrobiol. 99, 84–95. doi:10.1002/iroh.201301707
Stabel, H.-H. (1986). Calcite precipitation in Lake Constance: Chemical equilibrium, sedimentation, and nucleation by algae1. Limnol. Oceanogr. 31, 1081–1094. doi:10.4319/lo.1986.31.5.1081
Sun, Y., Xu, W., Gu, Q., Chen, Y., Zhou, Q., Zhang, L., et al. (2019). Small-sized microplastics negatively affect rotifers: changes in the key life-history traits and rotifer - phaeocystis population dynamics. Environ. Sci. Technol. 53, 9241–9251. doi:10.1021/acs.est.9b02893
Tietjen, T., Vähätalo, A. V., and Wetzel, R. G. (2005). Effects of clay mineral turbidity on dissolved organic carbon and bacterial production. Aquat. Sci. 67, 51–60. doi:10.1007/s00027-004-0753-2
Wagner, M., Scherer, C., Alvarez-Muñoz, D., Brennholt, N., Bourrain, X., Buchinger, S., et al. (2014). Microplastics in freshwater ecosystems: what we know and what we need to know. Environ. Sci. Eur. 26, 12. doi:10.1186/s12302-014-0012-7
Wallace, R. L., and Snell, T. W. (2010). “Rotifera,” in Ecology and classification of north American Freshwater invertebrates. Editors J. H. Thorp, and A. P. Covich (London: Academic Press 2010, Elsevier Inc.), 173–235. doi:10.1016/B978-0-12-374855-3.00008-X
Walz, N. (1993). Plankton regulation dynamics: Experiments and models in rotifer continuous cultures. Heidelberg: Springer-Verlag Berlin.
Wang, Y., Zhang, D., Zhang, M., Mu, J., Ding, G., Mao, Z., et al. (2019). Effects of ingested polystyrene microplastics on brine shrimp, Artemia parthenogenetica. Environ. Pollut. 244, 715–722. doi:10.1016/j.envpol.2018.10.024
Weithoff, G. (2007). Dietary restriction in two rotifer species: the effect of the length of food deprivation on life span and reproduction. Oecologia 153, 303–308. doi:10.1007/s00442-007-0739-6
Weithoff, G., and Wacker, A. (2007). The mode of nutrition of mixotrophic flagellates determines the food quality for their consumers. Funct. Ecol. 21, 1092–1098. doi:10.1111/j.1365-2435.2007.01333.x
Weithoff, G., and Walz, N. (1995). Influence of filamentous cyanobacterium Planktothrix agardhii on population growth and reproductive pattern of the rotifer Brachionus calyciflorus. Hydrobiologia 313, 381–386. doi:10.1007/BF00025974
Wiggin, K. J., and Holland, E. B. (2019). Validation and application of cost and time effective methods forthe detection of 3–500 μm sized microplastics in the urban marine and estuarine environments surrounding Long Beach, California. Mar. Pollut. Bull. 143, 152–162. doi:10.1016/j.marpolbul.2019.03.060
Wilkinson, G. M., Carpenter, S. R., Cole, J. J., Pace, M. L., and Yang, C. (2013). Terrestrial support of pelagic consumers: patterns and variability revealed by a multilake study. Freshw. Biol. 58, 2037–2049. doi:10.1111/fwb.12189
Windsor, F. M., Tilley, R. M., Tyler, C. R., and Ormerod, S. J. (2019). Microplastic ingestion by riverine macroinvertebrates. Sci. Total Environ. 646, 68–74. doi:10.1016/j.scitotenv.2018.07.271
Xue, Y.-H., Sun, Z.-X., Feng, L.-S., Jin, T., Xing, J.-C., Wen, X.-L., et al. (2021). Algal density affects the influences of polyethylene microplastics on the freshwater rotifer Brachionus calyciflorus. Chemosphere 270, 128613. doi:10.1016/j.chemosphere.2020.128613
Yap, V. H. S., Chase, Z., Wright, J. T., Hurd, C. L., Lavers, J. L., Lenz, M., et al. (2020). A comparison with natural particles reveals a small specific effect of PVC microplastics on mussel performance. Mar. Pollut. Bull. 160, 111703. doi:10.1016/j.marpolbul.2020.111703
Keywords: microplastics, rotifer, freshwater, natural particle, toxicity, environmental pollution
Citation: Pawlak J, Noetzel DC, Drago C and Weithoff G (2022) Assessing the toxicity of polystyrene beads and silica particles on the microconsumer Brachionus calyciflorus at different timescales. Front. Environ. Sci. 10:955425. doi: 10.3389/fenvs.2022.955425
Received: 28 May 2022; Accepted: 28 June 2022;
Published: 09 August 2022.
Edited by:
Anita Mukherjee, University of Calcutta, IndiaReviewed by:
S.S.S. Sarma, Universidad Nacional Autónoma de México, MexicoMaranda Esterhuizen, University of Helsinki, Finland
Copyright © 2022 Pawlak, Noetzel, Drago and Weithoff. This is an open-access article distributed under the terms of the Creative Commons Attribution License (CC BY). The use, distribution or reproduction in other forums is permitted, provided the original author(s) and the copyright owner(s) are credited and that the original publication in this journal is cited, in accordance with accepted academic practice. No use, distribution or reproduction is permitted which does not comply with these terms.
*Correspondence: Julia Pawlak, julia.pawlak.1@uni-potsdam.de
†These authors have contributed equally to this work and share first authorship