- 1Organic Geochemistry Unit, School of Earth Sciences, Zhejiang University, Hangzhou, China
- 2College of Hydraulic and Environmental Engineering, China Three Gorges University, Yichang, China
- 3School of Marine Sciences, Sun Yat-sen University, Zhuhai, China
- 4Southern Marine Science and Engineering Guangdong Laboratory (Zhuhai), Zhuhai, China
- 5State Key Laboratory of Heavy Oil Processing, China University of Petroleum, Beijing, China
- 6Department of Ocean Science and Hong Kong Branch of the Southern Marine Science and Engineering Guangdong Laboratory (Guangzhou), The Hong Kong University of Science and Technology, Hong Kong, Hong Kong SAR, China
Large-scale reservoirs exhibit complex hydrological conditions and exert a significant alteration on river flowing. Although dissolved organic matter (DOM) is noted to involve in biogeochemical processes, the variation mechanism of DOM chemistry across a large-scale reservoir is not well assessed. Here, we investigated four tributaries across different segments (e.g., the front and non-front areas) of the world’s largest Three Gorges Reservoir (TGR). Optical techniques and ultrahigh-resolution mass spectrometry were used to comprehensively explore the variation of DOM chemistry across TGR in the water-level declining period, and biological incubation experiments were conducted to trace its biogeochemical influences. We found that the variation of DOM composition and property between tributary and river mouth sites show different patterns between front and non-front areas of TGR. In particular, there was more terrestrial derived and biologically recalcitrant DOM in the river mouth than tributaries in the front area, while the opposite variation was observed in the non-front area. Integrated with hydrological information, the results demonstrated that the density current exerts a significant influence on DOM dynamics. Furthermore, the biological incubation experiments suggested that this variation of DOM property among tributaries would involve in the spatial dynamics of carbon dioxide (CO2) was emitted in TGR that more CO2 was emitted in the tributary of the front area than of the non-front area during the water-level declining period.
Introduction
Carbon dioxide (CO2) emission is a hot topic for its significant ecological effects on global climate, especially with carbon neutral promised by national governments in recent years (Ou et al., 2021). As a crucial part of atmospheric carbon budget, CO2 emission from fluvial ecosystem (e.g., reservoir) has been widely focused on (Barros et al., 2011; Weyhenmeyer et al., 2015; Gómez-Gener et al., 2021). Dissolved organic matter (DOM), an ensemble of various kinds of compounds, has been recognized as a key factor, influencing the CO2 emission in reservoirs due to its tight involvement in various carbon cycling processes (Battin et al., 2008). Demonstrating the dynamics of DOM in reservoir is not only crucial to the clarification of biogeochemical processes but also for the constrain of CO2 emission (Aufdenkampe et al., 2011). The unique hydrologic regime, one of the most significant characteristics of a reservoir, has been proven to exert a non-negligible influence on DOM chemistry (Zhang et al., 2015). To be specific, high discharge would increase dissolved organic carbon (DOC) and terrestrial DOM input in the reservoir ecosystem (Chen et al., 2016). Nevertheless, as a complex ecosystem integrated rivers and lakes, reservoirs, especially large-scale reservoirs, showed significant spatial heterogeneity (Kunz et al., 2011), which is crucial in the biogeochemical assessment of reservoirs and could shift the conclusion if it is not adequately considered (Pacheco et al., 2015). Water stratification, transmittance, and nutrient concentrations vary significantly among different segments of the reservoir (Rychteck and Znachor, 2011). For instance, with distance from the dam decreasing, flow rate decreases, whereas water retention time increases, which interfere with the biogeochemical processes (Rueda et al., 2006). Nevertheless, limited research focused on the linkage between the complex spatial heterogeneity and DOM dynamics, which limited the understanding of carbon cycling in reservoirs.
Multiple methods have been applied to draw a comprehensive depiction of DOM properties (e.g., composition, sources, and reactivity) for its complex heterogeneous composition (Dittmar et al., 2021 and references therein). Absorbance spectra (ABS) and excitation–emission matrices (EEMs) provide valuable and efficient insights into the optical characteristics of chromophoric dissolved organic matter (CDOM) (Jaffé et al., 2008). Ultrahigh-resolution electrospray ionization Fourier transform ion cyclotron resonance mass spectrometry (FT-ICR MS) could exhibit the characteristics of DOM formulas (e.g., CHO and N-, S-containing compounds) (Kujawinski, 2002). In recent decades, linkage between optical and molecular features of DOM was also characterized to improve the understanding of DOM behaviors (Stubbins et al., 2014).
In the current work, the large-scale DOM variations in four tributaries of TGR, which covered about half of TGR watershed, was focused on. Although previous studies have been carried out on DOM chemistry in tributaries of TGR and exhibited the bulk and optical characteristics of DOM (Jiang et al., 2018b; Ma and Li, 2020), there are limited studies of the underlying mechanism of DOM dynamics, especially at the molecular level. Our previous studies have been carried out on the influence of hydrological alterations on DOM reactivity, however, these investigations covered one or two tributaries with a watershed area < 100 km2 (He D. et al., 2020; Wang et al., 2021a, b). It remains to be unclear whether the investigations on one or two tributaries would adequately account for spatial heterogeneity and provide a comprehensive assessment of DOM chemistry in the whole TGR, with a watershed area of 1,080 km2 or not. Particularly, spatial heterogeneity-induced variation of DOM dynamics in different segments of reservoir has been elucidated, which highlighted the crucial role of spatial heterogeneity (e.g., hydrological or geomorphological heterogeneity) played in DOM involved biogeochemical processes (Yi et al., 2021). Therefore, a large-scale investigation is needed to resolve the debate and devote to a precise assessment of carbon budget of TGR. By applying multiple approaches, which include optical and molecular techniques, we aim to 1) exhibit optical and molecular features of DOM in large scale of TGR, 2) explore the mechanism of spatial heterogeneity of DOM, and 3) probe the biogeochemical implication (i.e., CO2 emissions) of DOM dynamics.
Methodology
Site description
The TGR (110°25′-111°06′E, 31°04′-31°34′N) is located at the upper Yangtze River, with a subtropical monsoon humid climate. Considering the complex geologic structure settings of the TGR, the front area which covers ca. 100 km from the Three Gorges Dam (TGD) to Badong was introduced and focused on since TGR construction (Liu, 1993; She et al., 2004; Chen et al., 2005). In this work, to assess DOM across a larger scale of TGR, we select tributaries in both the front and non-front areas. In particular, the Xiangxi River (XR) and Shennong River (SNR), which are located in the front area, and the Zhuyi River (ZYR) and Modao River (MDR), which are located in the non-front area, are four representative tributaries in the TGR.
Sample collection
Two sampling sites in each tributary (XR: M01 and T01; SNR: M02 and T02; ZYR: M03 and T03; and MDR: M04 and T04) were chosen to investigate DOM properties variation (Figure 1). M01∼M04 were located in the river mouth of each tributary, while T01∼T04 were located in tributaries. A total number of eight samples were collected in the water-level declining period (28 May 2017).
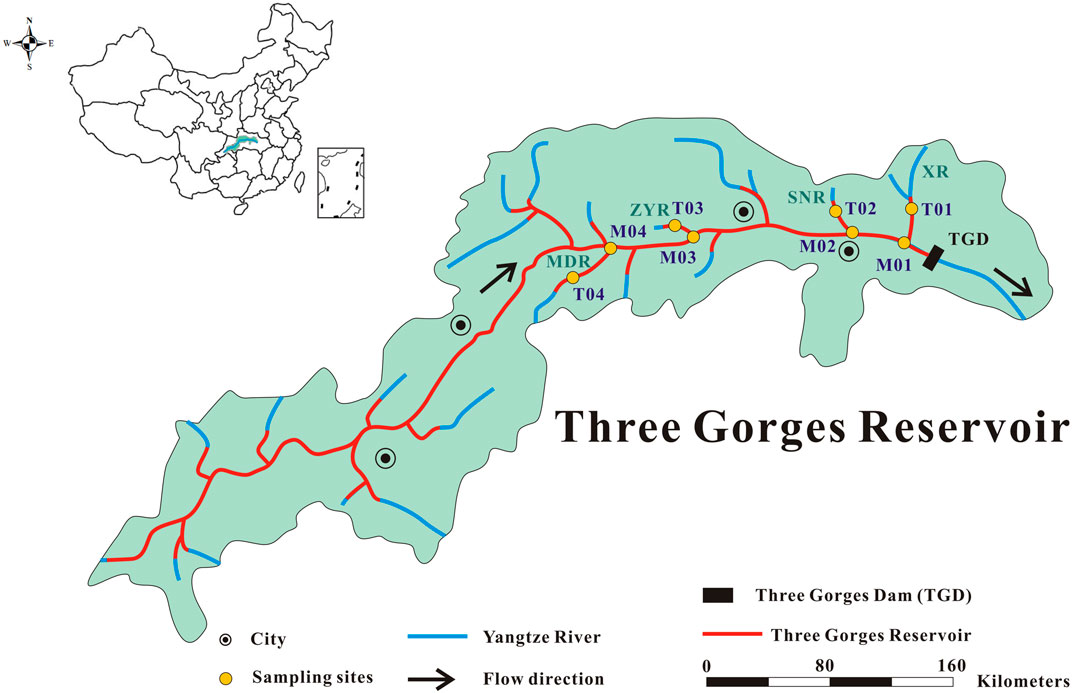
FIGURE 1. Study area and sampling sites in TGR. Sampling sites were selected in XR (M01, T01), SNR (M02, T02), ZYR (M03, T03), and MDR (M04, T04).
Water samples (ca. 1 m deep) were collected in Nalgene bottles, which were prewashed by Millipore-Q water and hydrochloric acid. Then Nalgene bottles with water were transported to laboratory under refrigeration. Water samples for DOM analysis were filtered using glass fiber filters (Whatman GFF, 0.7 μm, precombusted) and polycarbonate membrane (Millipore, 0.22 μm, hydrochloric acid-cleaned).
Bio-incubation experiments were set on water samples obtained from T01, T02, T03, and T04. To be specific, 100-ml unfiltered water samples in precleaned Nalgene bottles were placed under dark condition with aluminum foil coverage for 30 days. Enough headspace (1:1, volume ratio of headspace to water) was maintained to guarantee oxygen. After 30 days, the samples were processed, according to the previous filter procedure.
Analytical procedures
The analytical procedures were conducted with 0.22-μm filtered samples. The values of DOC were determined using a TOC analyzer (Shimadzu), with a coefficient of variation of ca. 2%. Quality control was attributed to a calibration series, deep-sea sample reference (DSR), and low carbon water (LCW) standards from the University of Miami (Dr. D. Hansell).
Optical properties including ABS and EEMs were characterized by using an Aqualog absorption-fluorescence spectrometer (Horiba). The CDOM characteristic parameters (e.g., S275–295 for CDOM spectral slopes, SR for CDOM molecular weight, and SUVA254 for CDOM aromaticity) were obtained based on ABS (Weishaar et al., 2003; Helms et al., 2008; Jaffé et al., 2008). FDOM characteristic parameters (e.g., humification index, HIX) and parallel factor (PARAFAC) analysis were obtained based on EEMs (Ohno, 2002; Huguet et al., 2009). Pre-acidified filtered samples (pH = 2) were processed by solid-phase extraction (SPE) (Agilent Bond Elut PPL filled by styrene–divinylbenzene polymer (SDVB), with recovery efficiencies from 40% to 60% on a DOC basis) (Dittmar et al., 2008). PPL cartridge was dried by N2 gas and eluted by LC-MS MeOH to obtain the SPE-DOM sample for FT-ICR MS analysis. SPE-DOM was analyzed by a 9.4 T Apex-ultra X FT-ICR MS (the Heavy Oil Key Laboratory, China University of Petroleum). A Suwannee River natural organic matter (SRNOM) was used as a standard during the analysis of FT-ICR MS for quality control. The analysis procedure was following He C. et al. (2020), and molecular compounds of DOM were identified. Briefly, formula assignment was performed by selecting a two-mass scale expanded segment near the most abundant peak of the spectrum, followed by detailed identification of each peak within a tolerance of ±0.001 Kendrick mass defect (KMD) (He C. et al., 2020). The relative peak intensities of molecular compounds were calculated following He D. et al. (2020).
The molecular parameters (e.g., element ratio, m/z, modified aromaticity index (AI), and double bond equivalent (DBE)) and composition information (e.g., elemental composition and formula classes (CHO, CHON, and CHOS)) were obtained based on semi-quantitative calculation (Hertkorn et al., 2006; Koch and Dittmar, 2006). For the potential complex composition of DOM in the reservoir, four molecular groups representing different sources were identified as: peptides (H/C: 1.5–2.0, N > 0), polyphenols (AI: 0.50–0.66), highly unsaturated compounds (AI < 0.50 and H/C < 1.5), and unsaturated aliphatic compounds (H/C: 1.5–2.0, N = 0) (Wang et al., 2021a).
Statistical analysis
The association between optical and molecular information was clarified to assess the complex composition of DOM. To be specific, Spearman’s correlation between relative abundance of formulas of FT-ICR MS and optical information was carried out and would be noted as significant as p < 0.05 and r > 0.5 (Stubbins et al., 2014).
Results and discussions
Optical and molecular features of chromophoric dissolved organic matter and solid-phase extraction-biogeochemical processes, respectively
DOC in XR, SNR, ZYR, and MDR varied from 1.4 to 1.7 mg/L, which was similar to the DOC values of samples from upstream of the Yangtze River (Jiang et al., 2018a; Figure 2A). In terms of optical properties, the values of SUVA254 and HIX and humification and aromaticity indicator of CDOM varied between 3.17 and 5.13 L mg-C−1 m−1 and 0.41 and 0.66, respectively, which spanned a similar range with CDOM in river and lake ecosystems (Weishaar et al., 2003). Averaged SUVA254 value was higher than that in upstream of the Yangtze River (ranged from 1.5 to 3.2 L mg-C−1 m−1, with an average of 2.0 L mg-C−1 m−1) (Liu et al., 2021). Moreover, higher values of SUVA254 and HIX were exhibited in tributary than those in river mouth sites of ZYR and MDR, while reverse variations in XR and SNR (Figures 2B,E), and SR and S275–295, parameters related with the averaged molecular weight of CDOM ranged from 1.44 to 1.78 and 0.015 to 0.019 nm−1, respectively. Autochthonous produced CDOM-related indices, BIX and β:α, varied from 0.89 to 1.06 and 0.85 to 0.99, respectively. Higher SR, S275–295, BIX, and β:α were observed in the river mouth than in tributary sites of ZYR and MDR, whereas reverse variations were observed in XR and SNR (Figures 2C,D,F,G). The optical parameters variation of DOM demonstrated that DOM in tributary sites of ZYR and MDR had higher humification and aromaticity degree, higher molecular weight than that in river mouth sites, while opposite variation was observed in XR and SNR (Hansen et al., 2016).
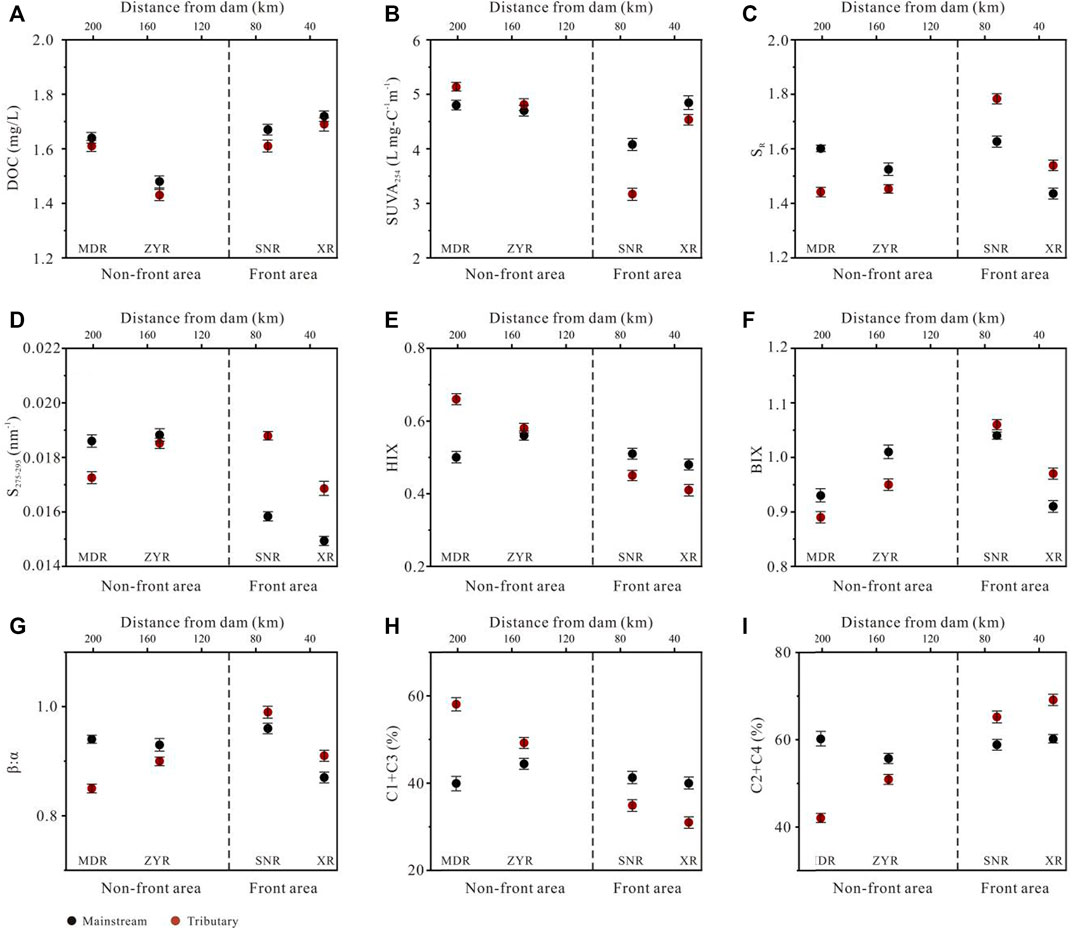
FIGURE 2. Bulk and optical properties variation of DOM. (A) DOC, (B) SUVA254, (C) SR, (D) S275-295, (E) HIX, (F) BIX, (G) β:α, (H) C1+C3, (I) C2+C4.
The optical property variation of DOM was further revealed by EEMs-PARAFAC. Four fluorescent components (humic-like components 1, 3 and protein-like components 2, 4) were identified (Supplementary Figure S1). The characteristics of components were further characterized by OpenFluor comparison. Component 1 (C1) and component 3 (C3) have been proven to be related to CDOM with high aromaticity (Graeber et al., 2012). Component 2 (C2) was associated with tryptophan-like CDOM compounds, while component 4 (C4) was associated with tyrosine-like CDOM compounds (Murphy et al., 2006; Wang et al., 2019). C1 and C3 accounted for 31%–58% (relative proportion) of PARAFAC components, meanwhile C2 and C4 accounted for 42%–69% (Figures 2H,I). The proportion of C2 and C4 was in the high end of protein-like components content, compared to that in other reservoir ecosystems (e.g., Hur et al., 2014). In terms of spatial variation, there were higher C1 and C3 proportions and lower C2 and C4 proportions in tributary than in the river mouth of ZYR and MDR, while opposite variation was observed in XR and SNR (Figures 2H,I).
In FT-ICR MS analysis of SPE-DOM, 7,619 unique formulas were detected in four tributaries by FT-ICR MS (Supplementary Figure S2). The CHO compounds abundance varied from 68.1% to 76.9%, with higher values in the river mouth than in tributary sites of XR, SNR, and lower values in the river mouth than in tributary sites for ZYR and MDR (Supplementary Table S1). CHON, CHOS, and CHONS compounds abundance varied from 12.2 to 18.7%, 9.5 to 14.3%, and 0.5 to 1.0%, respectively (Supplementary Table S1) and were lower than that in the ocean ecosystem, which might be resulted from higher terrestrial input in inland waters than oceans (Medeiros et al., 2015). Heteroatom compounds (e.g., CHON and CHOS compounds) abundance exhibited higher value in tributary than in river mouth sites of XR and SNR, while the opposite distribution was observed in ZYR and MDR (Supplementary Table S1). Molecular parameter calculation demonstrated that the values of AI, DBE, and m/z show higher values in tributary than in river mouth sites of ZYR and MDR, whereas lower values in tributary than in river mouth sites of XR and SNR (Figures 3A,B,C). The molecular property variation hinted that DOM in tributary shows higher aromatic degree and molecular weight than that in river mouth sites of ZYR and MDR and varied oppositely in XR and SNR, which is consistent with the optical property variation (Koch and Dittmar, 2006). Calculations of compound-grouped fractions based on FT-ICR MS exhibited that high aromatic compounds (polyphenols and highly unsaturated compounds) abundance and low aromatic compounds (unsaturated aliphatic compounds and peptides) abundance vary between 89.3 and 92.0% and 7.05 and 9.83%, respectively (Supplementary Table S2). There were higher abundances of high aromatic compounds, and lower relative abundances of low aromatic compounds in tributary than in river mouth sites of ZYR and MDR, and opposite variations in XR and SNR (Figures 3D,E).
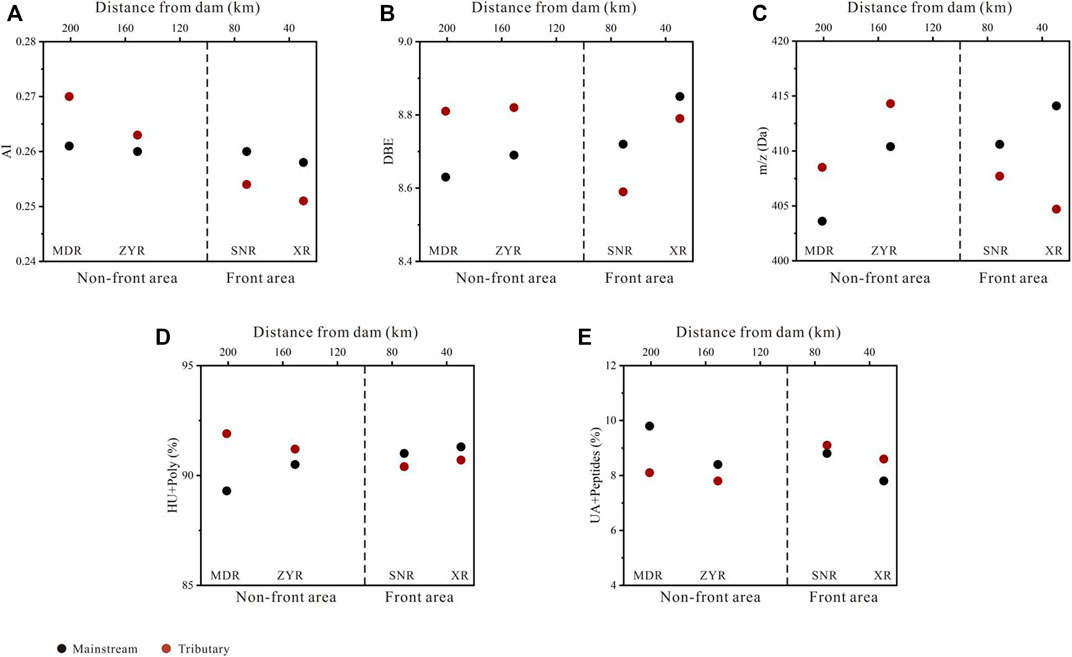
FIGURE 3. Molecular properties variation of DOM. (A) AI, (B) DBE, (C) m/z, (D) HU+Poly, (E) UA+Peptides. HU, Highly unsaturated compounds; Poly, Polyphenols; UA, unsaturated aliphatics.
Spatial variation regime of dissolved organic matter
To provide a comprehensive description of the spatial variation regime of DOM based on CDOM and SPE-DOM analysis, Spearman’s rank correlations between molecular intensities in FT-ICR MS and normalized optical information in EEMs and ABS were conducted.
Formulas showed different tracking behaviors in the correlations with optical indices and PARAFAC components. Compounds with high molecular weight and AI tracked most closely with SUVA254, HIX, C1, and C3, while compounds with low O/C tracked most closely with SR, S275-295, BIX, β:α, C2, and C4 (Figure 4), which turned out that similar optical information likely correlated closely with the similar molecule pool, and the aromaticity and humification degree were in agreement between CDOM and SPE-DOM (Stubbins et al., 2014; Kellerman et al., 2015). Specifically, relatively high m/z, O/C, and AI compounds tracked closely with C1, C3, SUVA254, and HIX, while formulas with high H/C values tracked closely with C2, C4, SR, S275-295, BIX, and β:α. C1 and C3 tracked most closely with terrestrial sourced compounds (polyphenols, which related to organic matter derived from vascular plant, and highly unsaturated compounds, which related to lignin-degradation derived compounds) (Seidel et al., 2015), C4 tracked most closely with autochthonous-sourced compounds (unsaturated aliphatic compounds and peptides, which related to aquatic organisms and autotrophic productivity) (Kellerman et al., 2018), and C2 tracked most closely with anthropogenic-sourced compounds (O3S and O5S classes of compounds, which were associated with wastewater input) (Gonsior et al., 2011; Melendez-Perez et al., 2016). The correlations suggested that optical property represent major of molecular groups in TGR and further supported the optical-molecular linkage of DOM (Wagner et al., 2015).
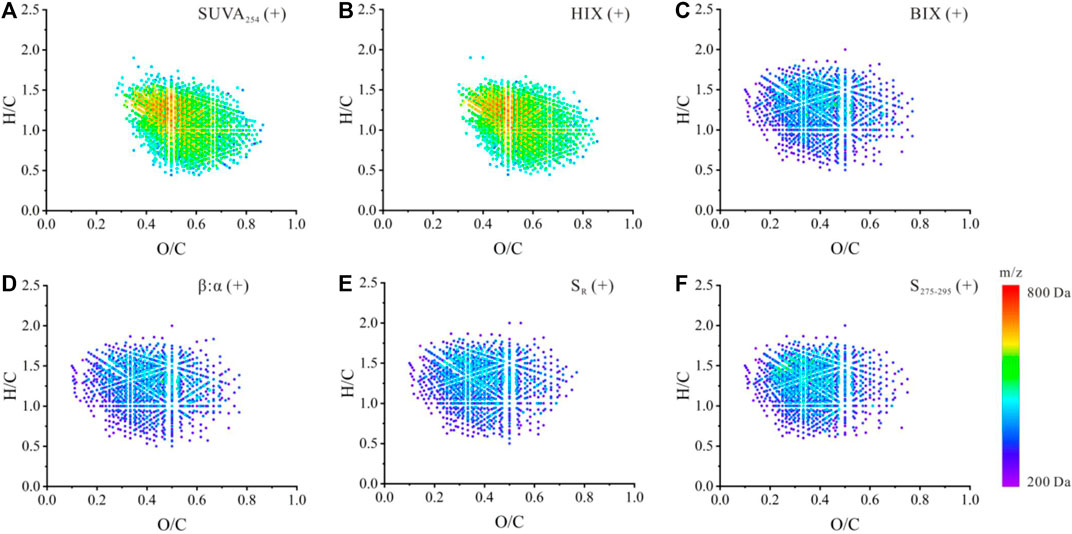
FIGURE 4. Van Krevelen distributions of molecular formulae positively correlated with optical properties. (A) SUVA254, (B) HIX, (C) BIX, (D) β:α, (E) SR (F) S275-295.
Technically, the optical-molecular linkage of DOM among four tributaries were consistent with our previous work which conducted in one tributary (He D. et al., 2020), indicating that optical techniques (e.g., EEMs and ABS) and FT-ICR MS might be equally as effective in tracking the DOM property to some extent in TGR. Considering the simpler, more efficient and lower cost of optical techniques comparing with FT-ICR MS, the correlation analysis might hint on the wide application of optical techniques.
Through the comprehensive analysis of CDOM and SPE-DOM, the variation regime of DOM among tributary and river mouth sites in different tributaries of the TGR was uncovered. Higher abundance of terrestrial DOM and lower abundance of autochthonous and anthropogenic DOM were presented in the river mouth than in tributary sites of XR and SNR, while the opposite variation was observed in ZYR and MDR (Figures 2, 3). In XR and SNR, SUVA254, HIX, and AI exhibited lower values in tributary than in river mouth sites, while the values of SR, S275-295, BIX, and β:α were higher, indicating that DOM in tributary was less aromatic and humified however more microbial or freshly produced than in the tributary (Figures 2, 3). In ZYR and MDR, DOM properties varied oppositely (Figures 2, 3).
Linking spatial heterogeneity induced hydrological variation and dissolved organic matter chemistry, and implications for CO2 emission
Previous studies have exhibited the association between hydrology and DOM property in tributaries of TGR (He D. et al., 2020; Wang et al., 2021a). The variation of DOM property among tributaries and river mouth sites could mostly be controlled by the unique hydrological regime of the reservoir, which has been assessed as a crucial factor shaping DOM characteristics (Cole et al., 2007; Yi et al., 2021). Hydrological management-induced water exchange regime among tributaries, which was characterized as water intrusion from the river mouth to the tributary, has been estimated (Ji et al., 2010; Zhou et al., 2015; Jiang et al., 2017; Ye et al., 2017; Yang et al., 2018; Hu, 2020; Shi et al., 2020) (Supplementary Figure S4). Thus, combined with the hydrological regime, the DOM dynamics model was presented (Figure 5). In XR and SNR, mainstream water with a high terrestrial signal intruded into the tributary and led to the higher terrestrial signal in the river mouth than tributary (Figure 5A). In the meantime, water masses with high concentrations of nutrients in the mainstream of the front area of the TGR were also introduced into the tributary, which would contribute to the algal bloom and devote to the addition of autochthonous DOM in tributaries of the front area (XR and SNR; Figure 5A) (Liu D et al., 2016), which was further supported by the high concentration of total nitrogen (up to ca. 2.2 mg/L in XR and up to ca. 2.3 mg/L in SNR ) and Chla (up to ca. 100 μg/L in XR and up to ca. 70 μg/L in SNR) (Fang et al., 2019; Wang et al., 2020). While in ZYR and MDR, although mainstream water intrudes into the tributary, nutrients with low concentrations and high coverage of farmland in tributaries of the non-front area of TGR might constrain autochthonous DOM input and enhance the terrestrial input (e.g., soil organic matter input through soil erosion in the wet season with substantial rainfall) and result in the relatively higher terrestrial signal of DOM in tributary than in the river mouth (Graeber et al., 2012; Masese et al., 2017) (Figures 5B, Supplementary Figure S5; Supplementary Table S3).
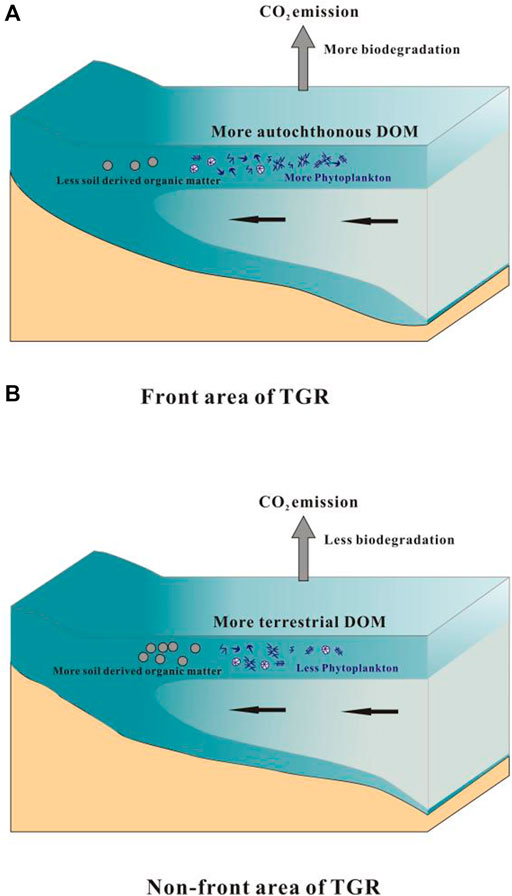
FIGURE 5. A conceptual model relating density current to DOM composition and biodegradation in the (A) front area, (B) non-front area, during the water-level declining period. Note: river bed is only conceptual and arrows in conceptual model do not indicate accurate flow rate but flow direction only.
As a carbon loss hot spot, the underlying mechanism controlling CO2 emission in reservoirs was widely focused on, especially in large-scale reservoirs, which cover a wide area of watershed and hold a large volume of water (Kemenes et al., 2011; Fearnside and Pueyo, 2012; Deemer et al., 2016; Maavara et al., 2017; 2020). DOC is estimated as a crucial point in the CO2 emission of aquatic ecosystems, for the reactive property in carbon dynamic processes (Barros et al., 2011; Lapierre et al., 2013). Theoretically, degradation of labile DOC in aquatic systems will eventually produce CO2, devoting to CO2 partial pressures (PCO2) (Ni et al., 2020). The incubation experiments showed the degradation induced decrease of DOC concentrations by 30% in XR, 27% in SNR, 14% in ZYR, and 16% in MDR, respectively (Supplementary Figure S6A). HIX increased by 53% in XR, 43% in SNR, 12% in ZYR, and 16% in MDR, respectively (Supplementary Figure S6B). The absolute percentages decreased in DOC and increased in HIX in XR and SNR were higher than those in ZYR and MDR. The higher biodegradability of DOM in XR and SNR could be attributed to the high proportion of autochthonous DOM, which could consequently lead to enhanced CO2 emission due to biodegradation (Pérez and Sommaruga, 2006; Lee et al., 2016). While in ZYR and MDR, a high proportion of terrestrial DOM would constrain the biodegradation-related CO2 production (Cataláalet al., 2017; Evans et al., 2017). This was further supported by the uncovering of the key control of organic carbon degradation on PCO2 in TGR (Liu S et al., 2016). Therefore, this density current-induced variation of DOM property needs to be considered when we investigate the spatial dynamics of CO2 emission in TGR and similar large-scale reservoirs.
It should be emphasized that there are also other factors that might influence DOM properties in tributaries (e.g., inflow from the tributary upstream and geomorphology of the tributary). Moreover, although DOM property was investigated in four tributaries, which cover nearly half of the TGR area, the sampling event was only conducted in the water-level declining period, and more seasonal investigation is needed. Limited samples were collected in every tributary. For instance, the degradation of DOM in the water column is also a crucial factor influencing CO2 production, which is not investigated here. Thus, more samples (e.g., water column samples) need to be analyzed in future work. Nevertheless, DOM chemistry across tributaries in both the front and non-front areas of tributaries of the TGR was assessed comprehensively at the bulk and molecular levels in this study for the first time to our best knowledge. A novel insight into the spatial dynamics of CO2 emission was presented, which would be conducive to better interpreting the carbon cycling processes in large-scale reservoirs. With the massive construction of reservoirs globally, the spatial heterogeneity of DOM chemistry across reservoir areas, which tightly links to CO2 emission, should be further explored.
Conclusion
Riverine reservoir construction and operation induced environmental context variations have significant influence on organic matter transportation. However, the relationship between large scaled DOM dynamics among tributaries and reservoir hydrological conditions is not well explored. By the application of multi-techniques (e.g., bulk, optical, and molecular techniques), the different spatial variation regime of DOM property in tributaries among front and non-front areas of the TGR was uncovered during the water-level declining period. We found that there was relatively more autochthonous, less aromatic, and humified DOM in tributary sites than those in river mouth sites in the front area of the TGR, while there was opposite variation in the non-front area of the TGR. This difference in spatial variations of DOM property between the front and non-front areas might result from hydrological variation dominated multi-factors (e.g., soil erosion and algal bloom). Moreover, the incubation experiments of DOC demonstrated that DOM dynamics might contribute to the complex CO2 emission regimes among different areas (e.g., front vs. non-front areas) in the water-level declining period of TGR. This study demonstrated that the investigation on one or two tributaries is crucial, which would provide a new perspective for DOM dynamics in the large-scale reservoir, nevertheless, in order to more accurately clarify the carbon budget of the reservoir, a large-scale study with the consideration of spatial heterogeneity is also necessary. With the dam construction increasing globally, the spatial dynamics of DOM chemistry across large-scale reservoirs should be further investigated.
Data availability statement
The original contributions presented in the study are included in the article/Supplementary Material; further inquiries can be directed to the corresponding author.
Author contributions
KW: investigation, writing—original draft, writing—review, and editing. DH: conceptualization, writing—original draft, writing—review, and editing. SX: data analysis, review, and editing. JL: data analysis, review, and editing. PL: data analysis, review, and editing. CH: review and editing. QS: data analysis, review, and editing.
Funding
This work was supported by China Postdoctoral Science Foundation (No. 2021M701931 to KW), the National Science Foundation of China 41773098 and 41973070 to DH, the Qianjiang talent program to DH, and the Hong Kong Branch of Southern Marine Science and Engineering Guangdong Laboratory (Guangzhou) (SMSEGL20SC01),and the Center for Ocean Research in Hong Kong and Macau (CORE), a joint research center between the Qingdao National Laboratory for Marine Science and Technology, and Hong Kong University of Science and Technology.
Acknowledgments
We acknowledge Yunyun, Li and Qiong, Pan during the analyses.
Conflict of interest
The authors declare that the research was conducted in the absence of any commercial or financial relationships that could be construed as a potential conflict of interest.
Publisher’s note
All claims expressed in this article are solely those of the authors and do not necessarily represent those of their affiliated organizations, or those of the publisher, the editors, and the reviewers. Any product that may be evaluated in this article, or claim that may be made by its manufacturer, is not guaranteed or endorsed by the publisher.
Supplementary material
The Supplementary Material for this article can be found online at: https://www.frontiersin.org/articles/10.3389/fenvs.2022.962706/full#supplementary-material
References
Aufdenkampe, A. K., Mayorga, E., Raymond, P. A., Melack, J. M., Doney, S. C., Alin, S. R., et al. (2011). Riverine coupling of biogeochemical cycles between land, oceans, and atmosphere. Front. Ecol. Environ. 9 (1), 53–60. doi:10.1890/100014
Barros, N., Cole, J. J., Tranvik, L. J., Prairie, Y. T., Bastviken, D., Huszar, V. L. M., et al. (2011). Carbon emission from hydroelectric reservoirs linked to reservoir age and latitude. Nat. Geosci. 4 (9), 593–596. doi:10.1038/ngeo1211
Battin, T. J., Kaplan, L. A., Findlay, S., Hopkinson, C. S., Marti, E., Packman, A. I., et al. (2008). Biophysical controls on organic carbon fluxes in fluvial networks. Nat. Geosci. 1 (2), 95–100. doi:10.1038/ngeo101
Chen, M., He, W., Choi, I., and Hur, J. (2016). Tracking the monthly changes of dissolved organic matter composition in a newly constructed reservoir and its tributaries during the initial impounding period. Environ. Sci. Pollut. Res. 23 (2), 1274–1283. doi:10.1007/s11356-015-5350-5
Chen, S., Yao, Y., Zeng, Z., Liu, L., and Li, X. (2005). Study on numerical simulation of tectonic stress field before and after reservoir impounding in the head area of the Three Gorges. Chin. J. Rock Mech. Eng. 24 (2), 5611–5618. (in Chinese).
Cole, J. J., Prairie, Y. T., Caraco, N. F., Mcdowell, W. H., Tranvik, L. J., Striegl, R. G., et al. (2007). Plumbing the global carbon cycle: Integrating inland waters into the terrestrial carbon budget. Ecosystems 10 (1), 172–185. doi:10.1007/s10021-006-9013-8
Deemer, B. R., Harrison, J. A., Li, S., Beaulieu, J. J., DelSontro, T., Barros, N., et al. (2016). Greenhouse gas emissions from reservoir water surfaces: A new global synthesis. BioScience 66 (11), 949–964. doi:10.1093/biosci/biw117
Dittmar, T., Koch, B., Hertkorn, N., and Kattner, G. (2008). A simple and efficient method for the solid-phase extraction of dissolved organic matter (SPE-DOM) from seawater. Limnol. Oceanogr. Methods 6 (6), 230–235. doi:10.4319/lom.2008.6.230
Dittmar, T., Lennartz, S. T., Buck-Wiese, H., Hansell, D. A., and Hehemann, J. H. (2021). Enigmatic persistence of dissolved organic matter in the ocean. Nat. Rev. Earth Environ. 1, 1–14.
Evans, C. D., Futter, M. N., Moldan, F., Valinia, S., Frogbrook, Z. L., Kothawala, D. N., et al. (2017). Variability in organic carbon reactivity across lake residence time and trophic gradients. Nat. Geosci. 10 (11), 832–835. doi:10.1038/ngeo3051
Fang, H., Song, L., Ji, D., Liu, X., Wang, Y., Zhang, Q., and He, J. (2019). Regional differences and mechanism analysis of summer blooms in Xiangxihe Reservoir Bay. Environ. Sci. Technol. (China) 42 (9), 68–74.
Fearnside, P. M., and Pueyo, S. (2012). Greenhouse-gas emissions from tropical dams. Nat. Clim. Chang. 2 (6), 382–384. doi:10.1038/nclimate1540
Gómez-Gener, L., Rocher-Ros, G., Battin, T., Cohen, M. J., Dalmagro, H. J., Dinsmore, K. J., et al. (2021). Global carbon dioxide efflux from rivers enhanced by high nocturnal emissions. Nat. Geosci. 14, 289–294. doi:10.1038/s41561-021-00722-3
Gonsior, M., Zwartjes, M., Cooper, W. J., Song, W., Ishida, K. P., Tseng, L. Y., et al. (2011). Molecular characterization of effluent organic matter identified by ultrahigh resolution mass spectrometry. Water Res. 45 (9), 2943–2953. doi:10.1016/j.watres.2011.03.016
Graeber, D., Gelbrecht, J., Pusch, M. T., Anlanger, C., and Schiller, D. V. (2012). Agriculture has changed the amount and composition of dissolved organic matter in Central European headwater streams. Sci. Total Environ. 438 (3), 435–446. doi:10.1016/j.scitotenv.2012.08.087
Hansen, A. M., Kraus, T. E., Pellerin, B. A., Fleck, J. A., Downing, B. D., Bergamaschi, B. A., et al. (2016). Optical properties of dissolved organic matter (DOM): Effects of biological and photolytic degradation. Limnol. Oceanogr. 61 (3), 1015–1032. doi:10.1002/lno.10270
He, C., Zhang, Y., Li, Y., Zhuo, X., Li, Y., Zhang, C., et al. (2020a). In-house standard method for molecular characterization of dissolved organic matter by FT-ICR mass spectrometry. ACS Omega 5, 11730–11736. doi:10.1021/acsomega.0c01055
He, D., Wang, K., Pang, Y., He, C., Li, P., Li, Y., et al. (2020b). Hydrological management constraints on the chemistry of dissolved organic matter in the Three Gorges Reservoir. Water Res. 187 (15), 116413. doi:10.1016/j.watres.2020.116413
Helms, J. R., Stubbins, A., Ritchie, J. D., Minor, E. C., Kieber, D. J., Mopper, K., et al. (2008). Absorption spectral slopes and slope ratios as indicators of molecular weight, source, and photobleaching of chromophoric dissolved organic matter. Limnol. Oceanogr. 53, 955–969. doi:10.4319/lo.2008.53.3.0955
Hertkorn, N., Benner, R., Frommberger, M., Schmittkopplin, P., Witt, M., Kaiser, K., et al. (2006). Characterization of a major refractory component of marine dissolved organic matter. Geochimica Cosmochimica Acta 70 (12), 2990–3010. doi:10.1016/j.gca.2006.03.021
Hu, P. (2020). Study on the mechanism of the difference of algal bloom degree between Pengxi and Modao River in the three Gorges reservoir area.. Master thesis. Chongqing: Southwest University.
Huguet, A., Vacher, L., Relexans, S., Saubusse, S., Froidefond, J.-M., Parlanti, E., et al. (2009). Properties of fluorescent dissolved organic matter in the Gironde Estuary. Org. Geochem. 40 (6), 706–719. doi:10.1016/j.orggeochem.2009.03.002
Hur, J., Lee, B. M., Lee, S., and Shin, J. K. (2014). Characterization of chromophoric dissolved organic matter and trihalomethane formation potential in a recently constructed reservoir and the surrounding areas - impoundment effects. J. Hydrology 515 (13), 71–80. doi:10.1016/j.jhydrol.2014.04.035
Jaffé, R., McKnight, D., Maie, N., Cory, R., McDowell, W. H., Campbell, J. L., et al. (2008). Spatial and temporal variations in DOM composition in ecosystems: The importance of long-term monitoring of optical properties. J. Geophys. Res. 113 (4). doi:10.1029/2008jg000683
Ji, D., Liu, D., Yang, Z., and Xiao, S. (2010). Hydrodynamic characteristics of Xiangxi bay in three Gorges reservoir. Sci. China 40 (1), 101–112.
Jiang, T., Chen, X., Wang, D., Liang, J., Bai, W., Zhang, C., et al. (2018b). Dynamics of dissolved organic matter (DOM) in a typical inland lake of the three Gorges reservoir area: Fluorescent properties and their implications for dissolved mercury species. J. Environ. Manag. 206, 418–429. doi:10.1016/j.jenvman.2017.10.048
Jiang, T., Wang, D., Wei, S., Yan, J., Liang, J., Chen, X., et al. (2018a). Influences of the alternation of wet-dry periods on the variability of chromophoric dissolved organic matter in the water level fluctuation zone of the Three Gorges Reservoir area, China. Sci. Total Environ. 636, 249–259. doi:10.1016/j.scitotenv.2018.04.262
Jiang, W., Zhou, C., Ji, D., Liu, D., Ren, Y., Haffner, D., et al. (2017). Comparison of relationship between conduction and algal bloom in Pengxi river and Modao River in three Gorges reservoir. Environ. Sci. 38 (6), 2326–2335. doi:10.13227/j.hjkx.201610183
Kellerman, A. M., Guillemette, F., Podgorski, D. C., Aiken, G. R., Butler, K. D., Spencer, R. G. M., et al. (2018). Unifying concepts linking dissolved organic matter composition to persistence in aquatic ecosystems. Environ. Sci. Technol. 52 (5), 2538–2548. doi:10.1021/acs.est.7b05513
Kellerman, A. M., Kothawala, D. N., Dittmar, T., and Tranvik, L. J. (2015). Persistence of dissolved organic matter in lakes related to its molecular characteristics. Nat. Geosci. 8 (6), 454–457. doi:10.1038/ngeo2440
Kemenes, A., Forsberg, B. R., and Melack, J. M. (2011). CO2 emissions from a tropical hydroelectric reservoir (Balbina, Brazil). J. Geophys. Res. 116, G03004. doi:10.1029/2010jg001465
Koch, B. P., and Dittmar, T. (2006). From mass to structure: An aromaticity index for high-resolution mass data of natural organic matter. Rapid Commun. Mass Spectrom. 20 (5), 926–932. doi:10.1002/rcm.2386
Kujawinski, E. B. (2002). Electrospray ionization fourier transform ion cyclotron resonance mass spectrometry (ESI FT-ICR MS): Characterization of complex environmental mixtures. Environ. Forensics 3 (3-4), 207–216. doi:10.1006/enfo.2002.0109
Kunz, M. J., Wüest, A., Wehrli, B., Landert, J., and Senn, D. B. (2011). Impact of a large tropical reservoir on riverine transport of sediment, carbon, and nutrients to downstream wetlands. Water Resour. Res. 47 (12), 1–16. doi:10.1029/2011wr010996
Lapierre, J. F., Guillemette, F., Berggren, M., and Del Giorgio, P. A. (2013). Increases in terrestrially derived carbon stimulate organic carbon processing and CO2 emissions in boreal aquatic ecosystems. Nat. Commun. 4 (1), 2972. doi:10.1038/ncomms3972
Lee, Y., Lee, B., Hur, J., Min, J. O., Ha, S. Y., Ra, K., et al. (2016). Biodegradability of algal-derived organic matter in a large artificial lake by using stable isotope tracers. Environ. Sci. Pollut. Res. 23 (9), 8358–8366. doi:10.1007/s11356-016-6046-1
Liu, C. (1993). A discussion on the engineering geology environment evolution of surface crust of the Three Gorges Project area of Yangtze River. J. Hebei Coll. Geol. 16 (1), 56–69. (in Chinese).
Liu, D., Yang, Z., Ji, D., Ma, J., Cui, Y., and Song, L. (2016). A review on the mechanism and its controlling methods of the algal blooms in the tributaries of Three Gorges Reservoir. J. Hydraulic Eng. 47 (3), 443–454.
Liu, S., Lu, X. X., Xia, X., Zhang, S., Ran, L., Yang, X., et al. (2016). Dynamic biogeochemical controls on river pCO2 and recent changes under aggravating river impoundment: An example of the subtropical Yangtze River. Glob. Biogeochem. Cycles 30 (6), 880–897. doi:10.1002/2016gb005388
Liu, T., Wang, X., Zhu, E., Liu, Z., Zhang, X., Guo, J., et al. (2021). Evolution of the dissolved organic matter composition along the upper mekong (lancang) river. ACS Earth Space Chem. 5 (2), 319–330. doi:10.1021/acsearthspacechem.0c00292
Ma, Y., and Li, S. (2020). Spatial and temporal comparisons of dissolved organic matter in river systems of the Three Gorges Reservoir region using fluorescence and UV-Visible spectroscopy. Environ. Res. 189, 109925. doi:10.1016/j.envres.2020.109925
Maavara, T., Chen, Q., Meter, K. V., Brown, L. E., Zarfl, C., Ni, J., et al. (2020). River dam impacts on biogeochemical cycling. Nat. Rev. Earth Environ. 1, 103–116. doi:10.1038/s43017-019-0019-0
Maavara, T., Lauerwald, R., Regnier, P., and Cappellen, P. V. (2017). Global perturbation of organic carbon cycling by river damming. Nat. Commun. 8 (1), 15347. doi:10.1038/ncomms15347
Masese, F. O., Salcedo-Borda, J. S., Gettel, G. M., Irvine, K., and McClain, M. E. (2017). Influence of catchment land use and seasonality on dissolved organic matter composition and ecosystem metabolism in headwater streams of a Kenyan river. Biogeochemistry 132 (1), 1–22. doi:10.1007/s10533-016-0269-6
Medeiros, P. M., Seidel, M., Powers, L. C., Dittmar, T., Hansell, D. A., Miller, W. L., et al. (2015). Dissolved organic matter composition and photochemical transformations in the northern North Pacific Ocean. Geophys. Res. Lett. 42 (3), 863–870. doi:10.1002/2014gl062663
Melendez-Perez, J. J., Martínez-Mejía, M. J., Awan, A. T., Fadini, P. S., Mozeto, A. A., Eberlin, M. N., et al. (2016). Characterization and comparison of riverine, lacustrine, marine and estuarine dissolved organic matter by ultra-high resolution and accuracy Fourier transform mass spectrometry. Org. Geochem. 101, 99–107. doi:10.1016/j.orggeochem.2016.08.005
Murphy, K. R., Ruiz, G. M., Dunsmuir, W. T., and Waite, T. D. (2006). Optimized parameters for fluorescence-based verification of ballast water exchange by ships. Environ. Sci. Technol. 40 (7), 2357–2362. doi:10.1021/es0519381
Ni, M., Li, S., Santos, I., Zhang, J., and Luo, J. (2020). Linking riverine partial pressure of carbon dioxide to dissolved organic matter optical properties in a Dry-hot Valley Region. Sci. Total Environ. 704, 135353. doi:10.1016/j.scitotenv.2019.135353
Ohno, T. (2002). Fluorescence inner-filtering correction for determining the humification index of dissolved organic matter. Environ. Sci. Technol. 36 (4), 742–746. doi:10.1021/es0155276
Ou, Y., Roney, C., Alsalam, J., Calvin, K., Creason, J., Edmonds, J., et al. (2021). Deep mitigation of CO2 and non-CO2 greenhouse gases toward 1.5 °C and 2 °C futures. Nat. Commun. 12 (1), 6245. doi:10.1038/s41467-021-26509-z
Pacheco, F. S., Soares, M. C. S., Assireu, A. T., Curtarelli, M. P., Roland, F., Abril, G., et al. (2015). The effects of river inflow and retention time on the spatial heterogeneity of chlorophyll and water-air CO2 fluxes in a tropical hydropower reservoir. Biogeosciences 12 (1), 147–162. doi:10.5194/bg-12-147-2015
Pérez, M. T., and Sommaruga, R. (2006). Differential effect of algal- and soil-derived dissolved organic matter on alpine lake bacterial community composition and activity. Limnol. Oceanogr. 51 (6), 2527–2537. doi:10.4319/lo.2006.51.6.2527
Rueda, F., Moreno-Ostos, E., and Armengol, J. (2006). The residence time of river water in reservoirs. Ecol. Model. 191 (2), 260–274. doi:10.1016/j.ecolmodel.2005.04.030
Rychteck, P., and Znachor, P. (2011). Spatial heterogeneity and seasonal succession of phytoplankton along the longitudinal gradient in a eutrophic reservoir. Hydrobiologia 663 (1), 175–186. doi:10.1007/s10750-010-0571-6
Seidel, M., Yager, P. L., Ward, N. D., Carpenter, E. J., Gomes, H. R., Krusche, A. V., et al. (2015). Molecular-level changes of dissolved organic matter along the Amazon River-to-ocean continuum. Mar. Chem. 177, 218–231. doi:10.1016/j.marchem.2015.06.019
She, C., Sun, S., Liu, S., Xiang, A., Li, H., and Yang, G. (2004). Dynamic variations of gravity field in head area of Three Gorges Reservoir in recent years. J. Geodesy Geodyn. 24 (2), 6–13. (in Chinese).
Shi, S., Cui, Y., Song, L., Liu, D., Ji, D., Huo, J., et al. (2020). Characteristics and causes of density flow in the Shennong bay of the three Gorges reservoir. Resour. Environ. Yangtze Basin 29 (8), 1847–1857. (in Chinese).
Stubbins, A., Lapierre, J.-F., Berggren, M., Prairie, Y. T., Dittmar, T., Giorgio, P. A. del., et al. (2014). What’s in an EEM? Molecular signatures associated with dissolved organic fluorescence in boreal Canada. Environ. Sci. Technol. 48 (18), 10598–10606. doi:10.1021/es502086e
Wagner, S., Jaffé, R., Cawley, K., Dittmar, T., and Stubbins, A. (2015). Associations between the molecular and optical properties of dissolved organic matter in the Florida Everglades, a model coastal wetland system. Front. Chem. 3, 66. doi:10.3389/fchem.2015.00066
Wang, K., Li, P., He, C., Shi, Q., and He, D. (2021a). Hydrologic heterogeneity induced variability of dissolved organic matter chemistry among tributaries of the Three Gorges Reservoir. Water Res. 201, 117358. doi:10.1016/j.watres.2021.117358
Wang, K., Pang, Y., Gao, C., Chen, L., Jiang, X., Li, P., et al. (2021b). Hydrological management affected dissolved organic matter chemistry and organic carbon burial in the Three Gorges Reservoir. Water Res. 199, 117195. doi:10.1016/j.watres.2021.117195
Wang, K., Pang, Y., He, C., Li, P., Xiao, S., Sun, Y., et al. (2019). Optical and molecular signatures of dissolved organic matter in Xiangxi Bay and mainstream of Three Gorges Reservoir, China: Spatial variations and environmental implications. Sci. Total Environ. 657, 1274–1284. doi:10.1016/j.scitotenv.2018.12.117
Wang, Y., Xu, T., Cui, Y., Ji, D., Huang, J., Fang, H., et al. (2020). Seasonal thermal stratification in Shennong bay and its effect on algal blooms. J. Hydroecology 41 (4), 19–26.
Weishaar, J. L., Aiken, G. R., Bergamaschi, B. A., Fram, M. S., Fujii, R., Mopper, K., et al. (2003). Evaluation of specific ultraviolet absorbance as an indicator of the chemical composition and reactivity of dissolved organic carbon. Environ. Sci. Technol. 37 (20), 4702–4708. doi:10.1021/es030360x
Weyhenmeyer, G. A., Kosten, S., Wallin, M. B., Tranvik, L. J., Jeppesen, E., Roland, F., et al. (2015). Significant fraction of CO2 emissions from boreal lakes derived from hydrologic inorganic carbon inputs. Nat. Geosci. 8, 933–936. doi:10.1038/ngeo2582
Yang, Z., Cheng, B., Xu, Y., Liu, D., Ma, J., Ji, D., et al. (2018). Stable isotopes in water indicate sources of nutrients that drive algal blooms in the tributary bay of a subtropical reservoir. Sci. Total Environ. 634, 205–213. doi:10.1016/j.scitotenv.2018.03.266
Yang, Z. (2014). Wuhan University. Doctoral thesis(in Chinese).The mechanisms of algal blooms and its operation method through water level fluctuation under the situation of the bidirectional density currents in tributaries of the Three Gorges Reservoir.
Ye, Z., Wang, Y., Hu, M., Wang, G., Liang, S., Wang, Q., et al. (2017). Hydrogen and oxygen stable isotope tracing of eco-hydrological processes in water bodies mixing the mainstream and a tributary of the Three Gorges Reservoir. Environ. Sci. Technol. 36 (8), 2358–2366.
Yi, Y., Zhong, J., Bao, H., Mostofa, K. M. G., Xu, S., Xiao, H., et al. (2021). The impacts of reservoirs on the sources and transport of riverine organic carbon in the karst area: A multi-tracer study. Water Res. 194, 116933. doi:10.1016/j.watres.2021.116933
Zhang, Y., Wu, Z., Liu, M., He, J., Shi, K., Zhou, Y., et al. (2015). Dissolved oxygen stratification and response to thermal structure and long-term climate change in a large and deep subtropical reservoir (Lake Qiandaohu, China). Water Res. 75, 249–258. doi:10.1016/j.watres.2015.02.052
Keywords: Three Gorges Reservoir, dissolved organic matter, carbon cycling, water-level declining period, spatial dynamics
Citation: Wang K, Xiao S, Liu J, Li P, He C, Shi Q and He D (2022) Spatial dynamics of dissolved organic matter among different segments of a large-scale reservoir in the water-level declining period. Front. Environ. Sci. 10:962706. doi: 10.3389/fenvs.2022.962706
Received: 06 June 2022; Accepted: 04 July 2022;
Published: 08 August 2022.
Edited by:
Peng Zhang, China University of Geosciences Wuhan, ChinaCopyright © 2022 Wang, Xiao, Liu, Li, He, Shi and He. This is an open-access article distributed under the terms of the Creative Commons Attribution License (CC BY). The use, distribution or reproduction in other forums is permitted, provided the original author(s) and the copyright owner(s) are credited and that the original publication in this journal is cited, in accordance with accepted academic practice. No use, distribution or reproduction is permitted which does not comply with these terms.
*Correspondence: Ding He, ZGluZ2hlQHVzdC5oaw==