- 1Laboratory of Plant Nutrition, Department of Natural Resources and Environment, Agricultural Research Institute, Nicosia, Cyprus
- 2Laboratory of Vegetable Production, Department of Crop Science, Agricultural University of Athens, Athens, Greece
Sustainable use of water and nutrients is fundamental in modern Mediterranean greenhouses and the use of modern decision support systems (DSS) can contribute to this direction. This work focuses on implementing new elements in water and nutrient recycling in cropping systems under saline conditions. Thus, two nutrient solution (NS) control strategies were applied to tomato crops grown in a recirculated NS under low- and high-salinity (NaCl) conditions. According to the first strategy, (i.e., Standard-STD) NS was replenished with water and nutrients based on predetermined “uptake concentrations” (UCs), i.e., nutrient to water uptake ratios, following standard commercial practices. According to the second strategy, NS was replenished by applying recalculated UCs of all nutrients after chemical analysis of a drainage solution sample using the software NUTRISENSE (NTS). Compared with the STD strategy, tomato plants treated with NTS retained drainage nutrient concentrations closer to the optimal values irrespectively of the salinity treatment. This eventually accounted for a smaller deviation from the mean threshold salinity value in the root zone at higher salinity levels, thus plants retained water consumption and crop yield potential at higher stomatal conductance minimizing physiological impacts of salinity on plants. Nutritional control strategies did not impose substantial difference in the UCs for most of the nutrients; however, this was not the case under salinity. Nutrient UCs determined and NTS strategy can be successfully used in DSS to optimize nutrient supply and prolong recirculation, thereby saving precious water and nutrients, in Mediterranean hydroponic crops with particular emphasis on saline environments.
Introduction
Water is a scarce and valuable commodity. Since agriculture is the largest water user, any saving in the water used for irrigation, even the smallest, accounts for a lot. On the other hand, the need to increase the circularity of crop production systems necessitates to keep nutrients in use as long as possible. In this context, recycling water and nutrients in closed soilless cultivation systems (CSCS) offers great opportunities to save precious water and reduce the consumption of fertilizers, thereby minimizing environmental impacts in greenhouse horticulture (Savvas and Neocleous, 2019; Thompson et al., 2020). However, the necessity to control the variability of nutrient concentrations in the fertigation effluents in such systems discourages Mediterranean growers to adopt recycling processes in their greenhouses in most cases (Neocleous et al., 2021). Standard injection of nutrients via stock solutions into the recycling irrigation solution generally forms an efficient fertigation strategy in closed soilless systems with continuous supply of water and nutrients (Savvas and Gruda, 2018). The automated injection of nutrients into the replenishment nutrient solution (NS) is based on the concept of previously determined nutrient “uptake concentrations” (i.e., mass of nutrient per water volume absorbed; Sonneveld and Voogt, 2009) for each specific crop species, with periodic modifications during the cropping period tailored to the developmental stage (Savvas et al., 2013). Nevertheless, matching nutrient supply with special nutritional needs of the crop (Gianquinto et al., 2013; Signore et al., 2016) involves some difficulties with this technique because nutrient addition dosage is not directly related to the nutrient composition of the drainage. An ideal approach should be to continuously monitor individual nutrients in the recycled solution (e.g., ion-selective sensors), however, such techniques are not available in commercial practice up to now (Ahn et al., 2021). The integration of simulation and sensing tools with actual measurements and different management strategies has been documented to be effective in theory and practice in soilless crops (Massa et al., 2020). Yet, the full implementation of decision support systems (DSS) and new emerging technologies (e.g., nanoparticles) in commercial soilless greenhouses remains an open issue (Sambo et al., 2019). In addition, when the primary water contains substantial amounts of NaCl, frequent adjustment of the nutrient injection ratios and concentrations is required to avoid nutrient imbalances and frequent discharge of the solution (Adams, 2002; Katsoulas et al., 2015). This phenomenon depends mainly on the concentration difference of unabsorbed or poorly absorbed ions such as Na+ and Cl− at the inlet (i.e., irrigation water) and the outlet (i.e., consumption by plants) and high levels of unused nutrients. Thus, salt accumulation may impose flushing of circulating water, a diluted nutrient solution supply or an elevated solution osmotic potential. This is a common problem facing Mediterranean regions and a serious drawback for the growers to adopt continuous recirculation systems (Signore et al., 2016). Salinity usually harms crops through osmotic stress, nutrient imbalances, and ion toxicity and to overcome current limits, a research challenge is to develop management tools that reduce salinity in recycled solutions and/or minimize its physiological impacts on plants. Therefore, this study can help to optimize plant nutrition in CSCS establishing mean nutrient UCs (nutrient/water uptake ratios) for the selected crop species making plants less susceptible in salty environments. Additionally, these values can be used as input data in operating DSS as a mean to diminish or even avoid solution runoff under such conditions. NUTRISENSE (https://nutrisense.online/), which was developed by Savvas et al. (2020) operates as a DSS using specific algorithms based on mass balance models (see Savvas and Adamidis, 1999; Savvas, 2000; Savvas, 2002) for the target dosage of each nutrient. Recently an extended version of these algorithms has been proposed (Savvas et al., 2020) which provides an alternative approach capable to automatically make the necessary adaptations whenever needed. Specifically, our objectives were to 1) develop novel elements in the continuous recycling of water and nutrients, 2) establish nutrient UCs for crops facing the issue of salinity in soilless systems, and 3) relate recycling effects to measured plant physiological responses. Tomato (Solanum lycopersicum L.) was selected as a model crop because has the lowest sensitivity to salinity of all fruiting greenhouse vegetables (Maggio et al., 2007), dominates worldwide in greenhouses (FAO, 2018), relies on high addition of fertilizers (Chapagain and Wiesman, 2004) and has different cultivation requirements in soil and soilless systems (Leonardi and Maggio, 2013).
Thus, the potential application of a smart NS replenishment strategy provided by NUTRISENSE in hydroponic tomato crops could lead to a better management of the parameters (i.e., water and nutrients) of hydroponic solution, especially when primary water is of low quality. This is particularly important considering the increasing scarcity of fresh good quality water resources in southern Europe where European greenhouse crops are concentrated (Thompson et al., 2020).
Materials and methods
Plant material—growing conditions
The experiment took place at the Agricultural Research Institute of Cyprus (lat. 34°44′N/long. 33°19′E/altitude 35 m absl) under natural light conditions (daily global solar radiation averaged 10.9 MJ·m−2) in a multi-span greenhouse with plastic coverings equipped with automated climate–control system. Tomato plants (S. lycopersicum L.) of the beefsteak fruit type (Dafnis, Syngenta, Netherlands) were transplanted in twenty NFT (Nutrient Film Technique) hydroponic channels, 6 m long, 0.25 m wide, and 0.1 m deep, achieving a final planting density of 2.5 plants m−2. In each hydroponic channel, nutrient solution (NS) was recirculated day and night enabling a constant solution volume in the system with continuous NS flow from the replenishment tank into the drainage catchment tank. Thus, each hydroponic channel constituted an independent experimental hydroponic unit. The experiment was conducted from November 2020 to February 2021 (Autumn-Winter crop), keeping greenhouse air temperature between 13°C and 28°C and relative humidity between 60%–80%. Plants were trained vertically using a string trellis system attached to a horizontal wire above plant row. For pollination, a bumblebee colony (Bombus terrestris) was introduced into the greenhouse at the flowering stage and pest and diseases were controlled following the practices suggested for sustainability in greenhouse horticulture (FAO, 2013). Plants were topped to facilitate a short production cycle (up to the 6th fruiting truss). A detailed description of the experimental layout has been given by Neocleous and Savvas (2015).
Experimental treatments
Experimental treatments consisted of two nutritional treatments applied in closed hydroponic tomato crops grown under two salinity levels. The plants treated with: 1) a typical replenishment strategy in which replenishing water and nutrients was based on predetermined “uptake concentrations-UCs” values, henceforth named “Standard-STD” and 2) an alternative replenishment nutrient strategy in which, replenishing water and nutrients was based on recalculated UCs of nutrients (henceforth named as “NUTRISENSE-NTS”). Two salinity (NaCl) levels were established by adding NaCl at appropriate amounts in the primary water containing 1.6 mM of NaCl to increase the NaCl concentration to 5.0 mM. The two nutritional treatments and the two salinity levels (i.e., low- and high-salinity) were combined in a 2 × 2 factorial arrangement replicated four times in a randomized complete block design. Each hydroponic channel corresponded to one experimental unit (n = 16) and external plant rows on both sides (n = 4) served as guard plants. Experimental treatments commenced immediately after transplanting and lasted up to crop termination.
Recycling nutrient solution and replenishment strategies
NUTRISENSE utilizes 1) a large database with target concentrations for all nutrients originating from several sources (e.g., Sonneveld and Straver, 1994; Adams, 2002; Sonneveld and Voogt, 2009) amended with relevant data under Mediterranean climatic conditions (e.g., Neocleous and Savvas, 2013, 2015, 2016; Savvas et al., 2013; Neocleous et al., 2017, 2018, 2019; Savvas and Gruda 2018; Savvas and Neocleous, 2019) and 2) current concentrations of the selected nutrients in the supplied and drainage solution to recalculate UCs and readjust the injection rates of nutrients stock solutions in the recovery solution.
According to the first strategy (i.e., Standard-STD) NS was replenished with nutrients and water at predetermined nutrient to water uptake ratios, termed ‘uptake concentrations (UCs) during cropping following standard commercial practices. This technique is based on a programmed addition of nutrients as suggested by Sonneveld and Voogt (2009). Eventually, the input of each ion (Cif) is calculated using its uptake concentration denoted by Ciu and the ionic concentration in the raw water (Ciw) using the equation:
Therefore, blending a NS with nutrient concentrations equal to the expected uptake concentrations with the drain solution resulted in the preparation of the drip irrigation solution aiming at electrical conductivity (EC) values equal to those suggested for this particular crop species and stage of development. According to the second strategy (i.e., NUTRISENSE-NTS), replenishing water and nutrients was based on recalculated UCs of nutrients every 3 weeks which were obtained after introducing compositional characteristics of the drainage NS to NUTRISENSE, as determined through a chemical analysis in the laboratory. As mentioned elsewhere, NTS includes a large database with target NS compositions for different crop species and developmental stages and uses a series of algorithms based on mass balance models (see Savvas and Adamidis, 1999; Savvas, 2000; Savvas, 2002) to calculate the injection rates of stock solutions into the irrigation water. However, a new element (i.e., an additional algorithm) was incorporated in NTS to facilitate the automated modifications of the replenishing NS composition in closed soilless systems. Thus, the actual drainage fraction (α) and current supplied (Cit) and drainage (Cid) nutrient solution concentrations are substituted in the following equation, to recalculate nutrient UCs values (Ciu, Sonneveld, 2000
The recalculation of the actual uptake concentration (Ciu) of the i nutrient (i = K, Ca, Mg, N, P) was based on a mass balance equation taking into consideration the concentration of the i nutrient in the current and previous drainage solution sample, the concentration of this nutrient in the replenishment solution and the water consumption between the two sampling dates.
After the recalculation of Ciu for each nutrient, NTS uses the following
To provide new target nutrient concentrations Cit in the drip solution where now α denotes the target fraction of the recycled solution to the total supplied NS and Cid denotes the target nutrient concentration in the root zone. Accordingly, target EC (ECt, dS m−1) in the drip solution is readjusted by NTS as follows (Savvas et al., 2020):
where ΣCit denotes the sum of the readjusted macrocations (K+, Ca2+, Mg2+) concentrations plus ammonium (NH4+) and sodium (Na+) concentrations.
In both strategies, during the cropping period the drip solution EC was readjusted to higher levels as a function of the measured Na+ concentration in the root-zone solution to facilitate a fully reuse of the NS with zero discharge of fertigation effluents.
Determination of nutrient uptake concentrations
The mean nutrient uptake concentrations (UCi; mmol or μmol·L−1 of nutrient uptake per liter of water absorbed) were estimated based on the removal of water and nutrients from the recirculated nutrient solution at regular time intervals following a mass balance equation previously described (Neocleous and Savvas, 2016):
where, UCi is the mean uptake concentration of the i nutrient (i.e. N_NO3+NH4, H2PO4-P; K, Ca, Mg, mmol·L−1, Fe, Mn, Zn, Cu, μmol·L−1), Vrns is the volume of the recycled nutrient solution; Vadns is the volume of the added nutrient solution (L) on a certain time interval; Cis and Cif (mmol·L−1) are respectively starting and final concentrations of the i nutrient in the recycled solution for the specified interval; and Ci is the concentration of the i nutrient in the added nutrient solution (mmol·L−1). Solution samples were collected from each hydroponic unit (from the replenishment and drainage catchment tank) at regular time intervals (0, 20, 40, 60, 80, 100, and 116 days after transplanting) for elemental analysis and electrical conductivity (EC)/pH measurements (Multimeter MM40+, Crison Instruments, Spain). Water added to the system from calibrated replenishment tank was assumed to be equal to the water consumption by the plants since leakage from the system and evaporation were negligible. UCs for the selected nutrients were calculated and presented based on the stage of development (i.e., Vegetative and Reproductive) to be comparable with published recommendations. The UC of NO3− and NH4+ were added together to calculate the UC of total nitrogen (N).
Measurements
The concentration of nutrients in solution samples was determined as previously reported by Neocleous et al. (2020). Briefly, an atomic absorption spectrophotometer (Solaar M Series, Thermo Elemental, Cambridge, United Kingdom) for Ca, Mg, Fe, Mn, Zn, and Cu, a flame photometer (Model 420, Sherwood Scientific, Cambridge, United Kingdom) for K and Na and a UV/VIS spectrophotometer (Lambda 35, Perkin Elmer; Waltham, MA, United States) for NO3–N, NH4–N, and P determinations were employed. The determination of Cl− was done by silver nitrate titration method in the presence of chromate. The concentration of SO4-S was estimated based on the anion to cation balance. Leaf gas exchange indices (i.e., photosynthesis, stomatal conductance, substomatal CO2 concentration, and transpiration) were recorded in newly developed tomato leaves 60 and 90 days after treatment initiation using a portable photosynthesis system (Li-6400, Li-Cor, Lincoln, NE, United States). Yield parameters (i.e., fresh fruit yield, fruit number per plant, and mean fruit weight) were determined in each experimental unit.
Statistical analysis
A two-way analysis of variance (ANOVA) was performed on selected data using SAS (ver. 9.2, SAS Institute Inc. Cary, NC, United States) using figures made by GraphPad Prism (ver. 5.0; GraphPad Software; San Diego California, United States).
Results
Mineral nutrients, yield and leaf stomatal responses
Nutritional control strategies (i.e., STD and NTS) did not impose substantial differences in the UCs of most of the nutrients irrespectively of the growing stage. Thus, recombining the data over treatments UCs averaged for the two stages as follow (Vegetative-Reproductive, Table 1): 15.2–14.1 (Nitrogen- NO3− + NH4+), 1.36–1.29 (Phosphorus-P), 8.54–8.32 (Potassium-K), 3.60–3.42 (Calcium-Ca), 1.76–1.70 (Magnesium-Mg, mmol L−1), 14.23–14.33 (Iron-Fe), 8.57–7.61 (Manganese-Mn), 7.24–6.88 (Zinc-Zn), and 0.92–1.12 (Copper-Cu, μmol L−1). However, this was not the case with salinity, which imposed limitations at high NaCl levels in the UCs of some macronutrients (i.e., N, K, and Ca). Micronutrients (Fe, Zn, and Cu) UCs did not show clear and consistent differences with regard to salinity except the UC of Mn, which slightly but significantly increased under high salinity (Table 1). Elemental analysis of the drainage (Figure 1) provides that NTS maintained some macronutrients (N-NO3 and K, Figures 1A,C) and micronutrients (Fe, Mn, and Cu Figures 1F,G,I) levels in the recycled solution closer to the recommended values compared to the STD strategy. On the other hand, salinity boosted drainage sodium chloride (NaCl) concentration regardless the STD or NTS, (Figures 1J, 2F), with only minor effects on the concentration levels of nutrients such as NO3-N and P (Figures 1A,B). Over salinity treatments, NaCl concentration in the drainage ranged between 9–24 mmol·L−1 (Figures 1J, 2F). Concentrations at high range were observed at later stages of growth when crop is considered less sensitive (Sonneveld, 2002; Bar-Yosef, 2008). Concentration of the other selected nutrients in the drainage (Figures 1A–I) ranged as follows: 22–26 (NO3-N), 1.0–1.3 (P), 11–13 (K), 9.5–10.5 (Ca), 4.0–4.7 (Mg, mmol·L−1), 20–24 (Fe), 5.5–7.0 (Mn), 6.4–7.4 (Zn) and 2.0–2.5 (Cu, μmol·L−1). Furthermore, salinity imposed significant differences to the electrical conductivity (EC) in the drainage, plant water uptake, fresh fruit yield, mean fruit weight (Figures 2A–D) and stomatal conductance (Figure 2G) with minor or insignificant effects on transpiration and photosynthetic rates, respectively (Figures 2H,I). In most cases nutritional treatments effect, accounted for a small percentage in the total data variance. However, for some parameters such as the drainage EC, plant water uptake and mean fruit weight (Figures 2A,B,D), the effect was considered significant while for others such as fresh fruit yield (Figure 2C) not significant (p < 0.05). In some other cases, NTS did not have the same effect at all values of NaCl. Thus, a significant interaction effect (p < 0.05) between nutritional treatments and salinity was found, changing the order of the response variable, in the case of the stomatal conductance (Figure 2G). Finally, nutrient concentrations in the refill and drainage solution according to the nutritional strategies tested and the developmental stage are given in Table 2. These recipes for the STD strategy correspond to standard injection rates of nutrients in the recycled solution during the vegetative and reproductive stage following the commercial practice, whereas recipes for the NTS strategy correspond to injection rates of nutrients in the solution only for short periods within each stage (vegetative and reproductive) after chemical analysis of solutions. In absolute values, similar nutrient addition is provided initially at the vegetative stage from both nutritional strategies, whereas nutrient supplementations differ between the two when nutrients levels are accumulated in the recycled solution at later stages of growth (i.e., reproductive stage). The latter entails readjustments in the supply concentrations of nutrients and relevant variations in their ratio (Table 2).
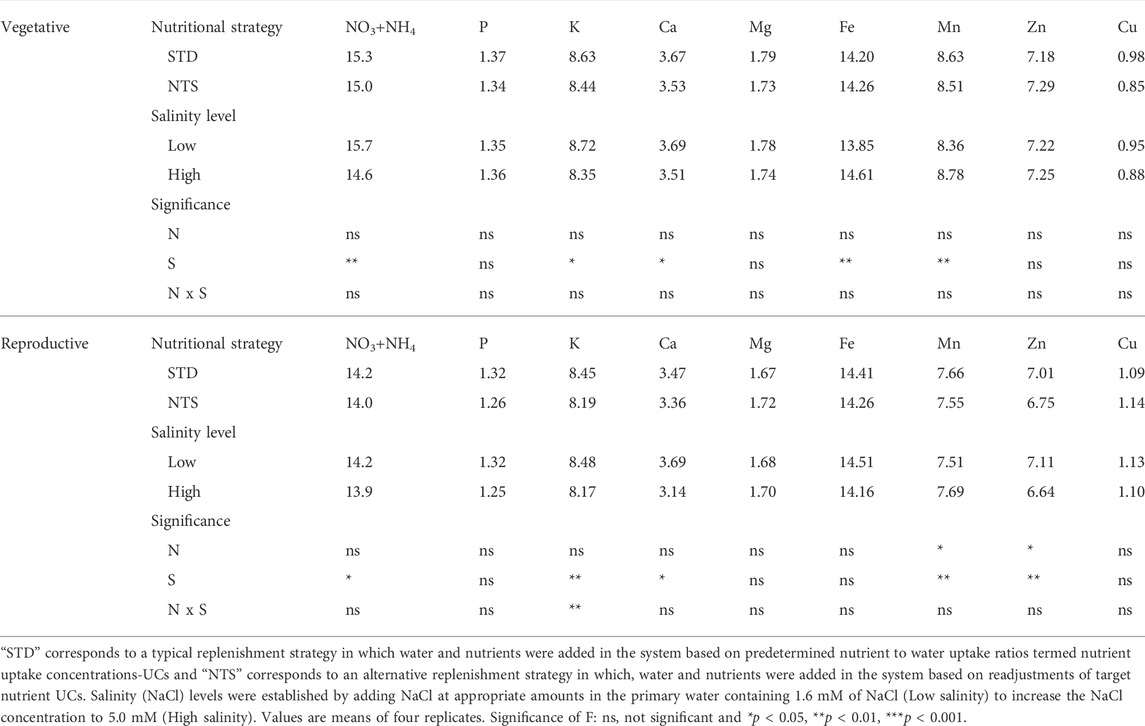
TABLE 1. Nutrient uptake concentrations (NO3+NH4, P, K, Ca, Mg, mmol L−1 and Fe, Mn, Zn, Cu μmol L−1) in the vegetative and reproductive stage of hydroponic tomato crops supplied with nutrient solution compositions provided by two nutritional strategies (i.e., standard-STD and NUTRISENSE-NTS) under low- and high-salinity (NaCl) levels.
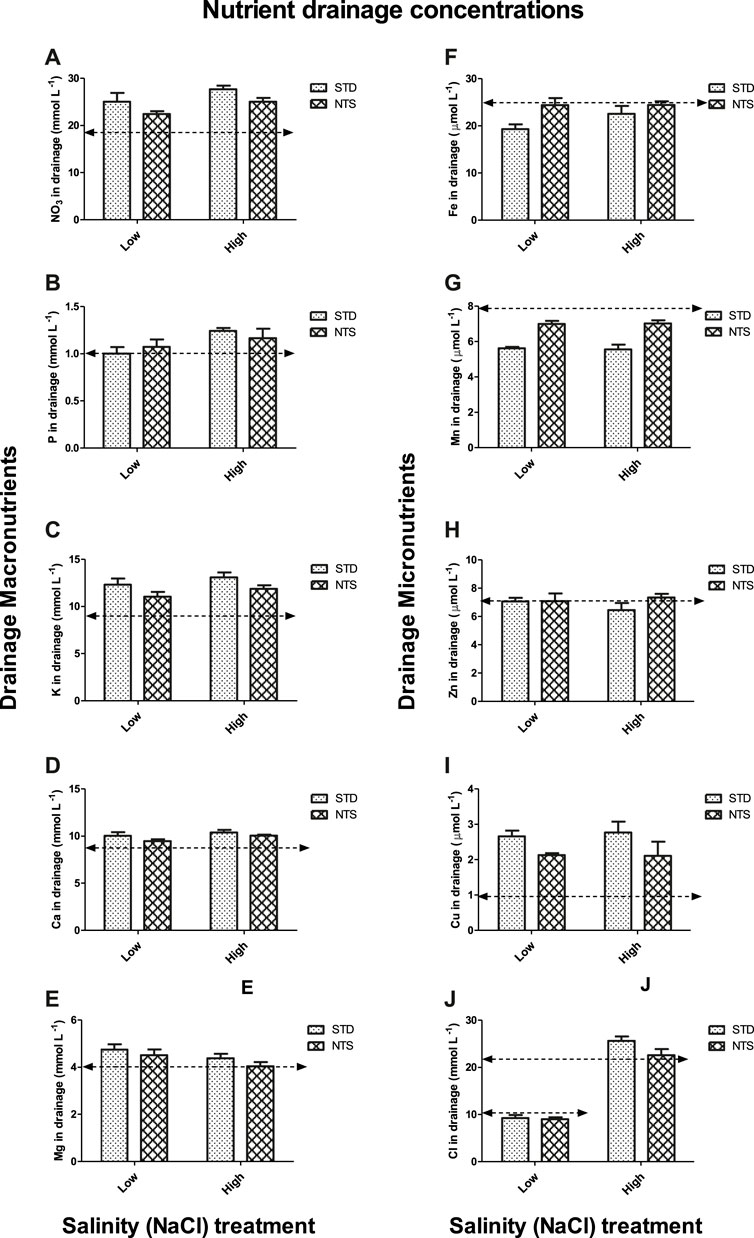
FIGURE 1. Nutrient drainage concentrations of macronutrients (A–E) (NO3, P, K, Ca, and Mg) and micronutrients (F–J) (Fe, Mn, Zn, Cu, and Cl) in hydroponic tomato crops supplied with nutrient solution compositions provided by two nutritional strategies (i.e., standard-STD and NUTRISENSE-NTS) under low- and high-salinity (NaCl) levels. “STD” corresponds to a typical replenishment strategy in which water and nutrients were added in the system based on predetermined nutrient to water uptake ratios termed nutrient uptake concentrations-UCs and “NTS” corresponds to an alternative replenishment strategy in which, water and nutrients were added in the system based on readjustments of target nutrient UCs. Salinity (NaCl) treatments were established by adding NaCl at appropriate amounts in the primary water containing 1.6 mM of NaCl (Low) to increase the NaCl concentration to 5.0 mM (High). Vertical columns in figure parts are means of four replicates ± standard errors. Dotted lines embedded in figure parts represent standard recommendations (based on Savvas and Gruda, 2018).
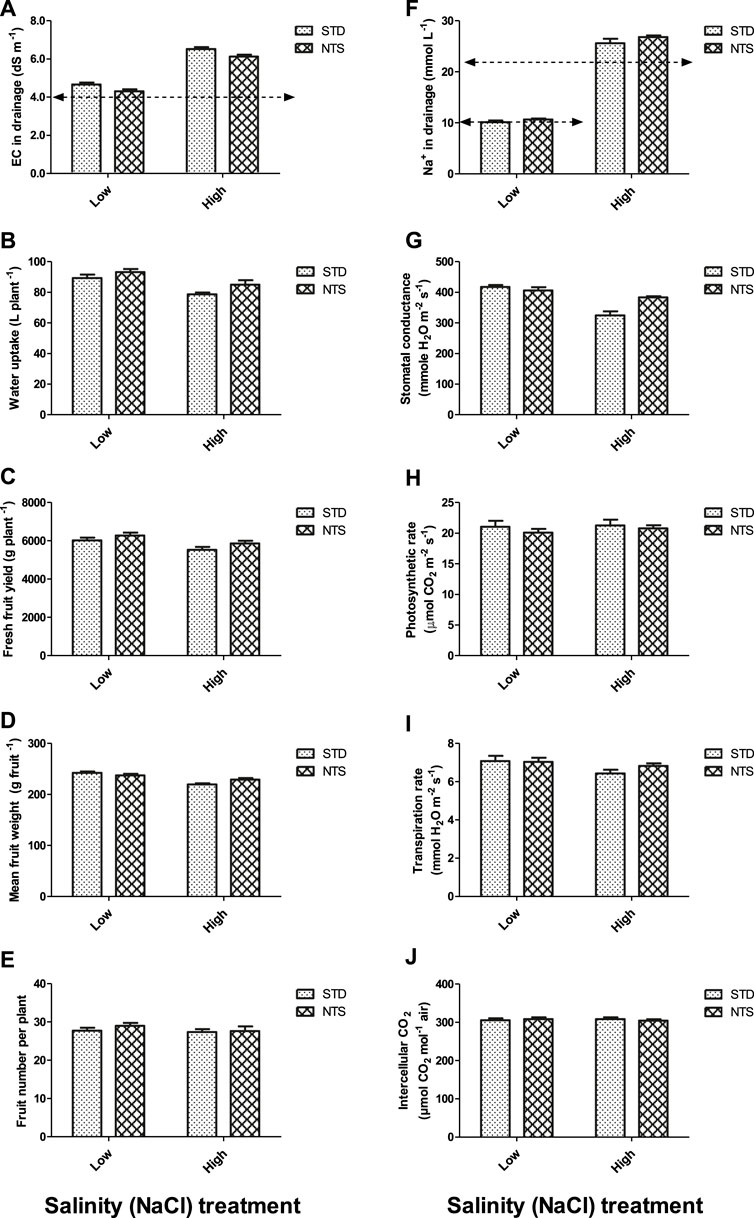
FIGURE 2. Agronomical (A–F) (root zone electrical conductivity-EC, water uptake, fresh fruit yield, mean fruit weight, fruit number per plant, and drainage sodium-Na concentration) and physiological (G–J) (Stomatal conductance, Photosynthetic rate, Transpiration rate, and Intercellular CO2 concentrations) responses in hydroponic tomato crops supplied with nutrient solution compositions provided by two nutritional strategies (i.e., standard-STD and nutrisense-NTS) under low- and high-salinity (NaCl) levels. “STD” corresponds to a typical replenishment strategy in which water and nutrients were added in the system based on predetermined nutrient to water uptake ratios termed nutrient uptake concentrations-UCs and “NTS” corresponds to an alternative replenishment strategy in which, water and nutrients were added in the system based on readjustments of target nutrient UCs. Salinity (NaCl) treatments were established by adding NaCl at appropriate amounts in the primary water containing 1.6 mM of NaCl (Low) to increase the NaCl concentration to 5.0 mM (High). Vertical columns in figure parts are means of four replicates ± standard errors.
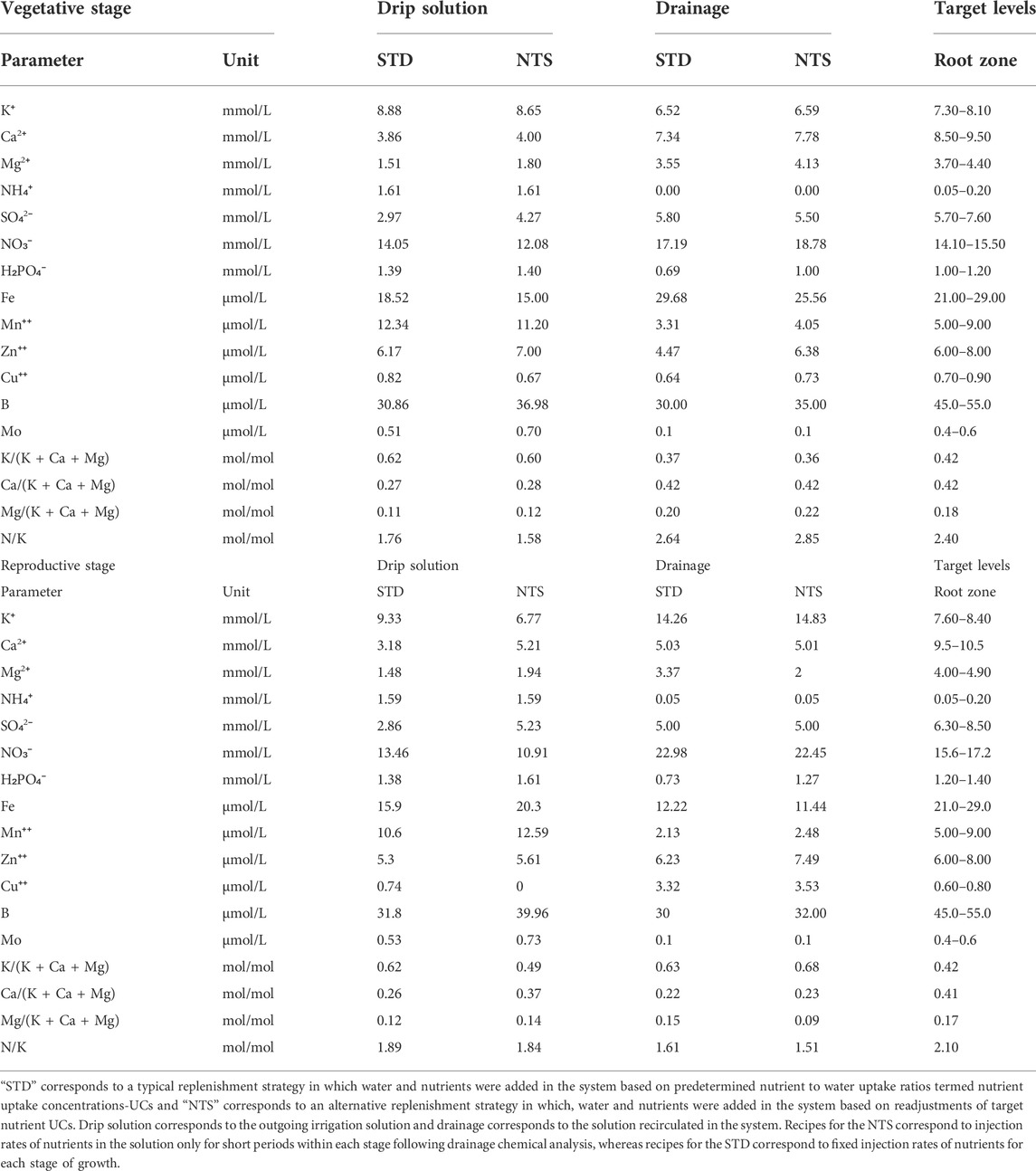
TABLE 2. Nutrient solution compositions provided by two nutritional strategies (i.e., standard-STD and NUTRISENSE-NTS) in hydroponic tomato crops during the vegetative and reproductive stage.
Discussion
Recycling the effluent solution in hydroponics can be technically and economically feasible only if it is automatically replenished with the appropriate amounts of water and nutrients in proportions equal to their “uptake concentrations” (masses of nutrients uptake per volume of water uptake, Sonneveld and Voogt, 2009). The nutrient to water uptake ratios relies on two independent physiological processes i.e., photosynthesis and transpiration. Thus, better understanding of plant mineral nutrition-physiology in relation with environmental factors, such as salinity, is very important for establishing optimal plant nutrition and irrigation schemes in Mediterranean greenhouses. This study reveals that, as the crop ages, a stronger increase in water than nutrient demand (Ropokis et al., 2018) can impose reductions in the mean nutrient UCs (Table 1), which entails adjustments in the nutrient solution composition. The exception formed in the UCs of Fe and Cu may indicate increased nutrient concentrations in the fruit biomass, depending upon genotype sensitivity. Furthermore, due to the antagonistic effect of NaCl on the UCs of N, K, and Ca (Table 1), the standard UCs found in the literature (Sonneveld and Straver, 1994; Sonneveld and Voogt, 2009; Savvas et al., 2013) cannot be used straightforward. This study provides UCs of nutrients under salinity conditions and decision support systems such as NUTRISENSE, may include these data in the program libraries, (as an alternative option) enabling optimization of nutrient and water supply in Mediterranean hydroponic tomato crops. An improvement is expected by introducing sensors in conjunction with the software of the program to determine in real-time the variability of nutrient concentration in the fertigation effluents (Kim et al., 2013). This equipment will allow to calculate on-line the exact input doses of individual nutrients. However, it is still challenging to replace conventional nutrient management with sensing technologies (Sambo et al., 2019). On the other hand, modelling approaches on nutrient dynamics and salinity built-up can be extremely helpful to make decisions in soilless cropping systems for controlling the whole process of plant nutrition (Massa et al., 2020). Furthermore, the established UCs in the present study, combined with NTS strategy, may contribute to minimizing discharge of fertigation effluents in short production-cycle hydroponic tomato crops, particularly in semi-arid areas facing saline conditions saving precious water. Fully recycling of the nutrient solution through the entire growing period raised salinity in the drainage to marginal levels (salinity threshold value, Bar-Yosef, 2008) for the low-salinity treatment (avg. 4 dS·m−1) but for the high-salinity, this value rose to substantially higher levels (avg. 6 dS·m−1, Figure 2A). The EC value of 4 dS·m−1 in hydroponic tomato crops is not associated with nutrient restrictions or osmotic stress conditions, allowing plants to render high yield and good quality (Sonneveld and Voogt, 2009). On the other hand, the higher EC derived from rather a gradual and not an abrupt increase of salts in the root zone solution, allowed tomato plants to adapt and maintain crucial metabolic processes when the salinity tolerance threshold is exceeded, such as availability of CO2 and photosynthesis (Figures 2H,J, Parida and Das, 2005), avoiding severe fruit yield losses (avg. 10% decrease, Figure 2C). However, it appears that salinized plants in NTS treatment succeeded even a smaller fruit yield decrease (<10% yield decrease) than plants in STD (Figure 2C) owned to a lower decrease of the salinity-induced mean fruit size (Figure 2D). This allows for a profitable crop production (Cuartero and Fernández-Muñoz, 1998) making the recycling safe under such conditions (when EC > ECthreshold). This was attributed to the fact that salinized plants preserved leaf stomatal conductance at higher levels when treated with NTS than STD strategy with values almost the same as at low-salinity (400 mmol·m2·s−1, Figure 2G), which improved their water status (Figure 2B). It seems that at high-salinity, readjustments in the supply levels and mutual ratios of nutrients with NTS compared to the STD strategy succeeded a smaller deviation from the mean EC threshold (EC increase to a lesser extent by 10%, Figure 2A). In earlier reports, the increase of the EC in the nutrient solution due to high Na concentration in the irrigation water, was directly associated to a decline of stomatal conductance in tomato plants (Maggio et al., 2007). However, under the conditions and the Na levels tested in the current study, the stomatal conductance was not reduced, as mentioned elsewhere. Apparently, the gradual increase of salinity and the moderate level of increase preserved leaf stomatal conductance in salinized tomato plants, while this may also be associated with genotype sensitivity and growing conditions. For example, re-establishing a modified supply cationic ratio through NTS probably minimized competitive interactions occurring among K+, Ca2+, and Mg2+, since the excessive availability of K+ in root zone solution could reasonably inhibit the uptake of the other cations (Sambo et al., 2019; Table 2). Indeed, no nutrient uptake inhibition was observed (Table 1). Since transpiration and photosynthesis remained unaffected (Figures 2H,I), analogous desirable ionic partitioning in epigeous organs may be expected causing the plants eventually to be less susceptible to nutritional disorders (De Pascale et al., 2012). It appears that the above-mentioned nutritional alterations in the root environment (Figure 1), affected positively stomatal functions maintaining a threshold stomatal conductance (Figure 2G) as mentioned elsewhere, which is a key component in metabolic processes such as xylem loading of water and nutrients (Medrano et al., 2002; Taiz and Zeiger, 2002). In fact, stomatal conductance is a reference of many signals (e.g., hormonal factors) associated with plant responding to water stress (Flexas et al., 2004) and most probably a key determinant in the present, retaining the transpiration stream and the availability of water to salinized tomato cells. In this content, stomatal conductance can be considered as a reference parameter reflecting the salinity induced water stress experienced by the plants (Medrano et al., 2002) and a functional response to salinity (Orsini et al., 2013). Indeed, manipulating nutrient to water injection ratios via NTS in salinized plants (Table 2), enabled higher water uptake (85 L/plant) compared with the standard (STD) strategy (78 L/plant, Figure 2B), which finally entails higher mean fruit weight (Figure 2D). Additionally, taking into consideration that a very low number of fruits were considered unmarketable, current data could be used to facilitate a multi-crop production strategy yielding on average 45 kg/m2 per year with minimal need to discharge fertigation effluents to the environment in case of water resources of suboptimal quality. This strategy results final solution NaCl concentrations of about 22–24 mM (Figures 1J, 2F) which is at marginal level for damaging tomatoes (Sonneveld, 2002) and permits some deviation over the mean threshold EC in a closed-loop irrigation system (Bar-Yosef, 2008). This can be explained to some extent by the root Na+ detoxification capacity (Maggio et al., 2007; Orsini et al., 2013), whereas recent findings on biological functions of Cl− in higher plants such as tomato (Franco-Navarro et al., 2016; Colmenero-Flores et al., 2019) suggest its beneficial role in maintaining transpirational fluxes through the regulation of aquaporin (Nieves-Cordones, et al., 2019). In addition, the occurrence of salt stress through a process of gradual accumulation at later stages of growth may allow tomato plants to adjust their physiological mechanisms as a response to salinity (Neocleous et al., 2017). It appears evident from this study that maximizing Na+ and Cl− in nutrient solutions is compatible with the application of fully closed hydroponic systems in short-cycle tomato crops through frequent adjustment of the nutrient injection rates. Finally, considering all the above, a very interesting scenario follows. Assuming a yearly average water consumption of 3 L/m2/day by tomato plants under similar cultivation conditions (Neocleous et al., 2021), these data result a fruit yield of 45 kg per ton of water with relatively high NaCl. This value is comparable with high–ranked water uses efficiency values (product yield per unit water volume consumed) succeeded elsewhere (Nikolaou et al., 2020; Nikolaou et al., 2021) and consistent with the objective of achieving high yields in some plant species under conditions that necessitates the use of saline water. In another case, NTS can facilitate a lower nitrate-N supply, thus causing less leaching losses, which entails higher N use efficiency, and less pollution of aquifers in systems that are not completely closed (see Supplementary Table S1). Thus, developing a smart decision support system to optimize nutrition in soilless culture will eventually contribute to reduction of water and nutrient emissions in Mediterranean greenhouses with a high-water stress index (Gruda, 2019), in line with the EU Green Deal to reduce nutrient losses by 50% in agriculture sector.
Conclusion
In conclusion, this study showed that potential application of a smart fertilizer control tool such as NUTRISENSE (NTS) can help to develop novel elements in the safe recycling of nutrients in Mediterranean greenhouses and to understand better the plant physiological responses behind this, together with establishing nutrient UCs for salinized tomato crops. Plants treated with NTS regulated leaf stomatal conductance and their water status after salinity tolerance threshold, which enables an adequate fruit yield in short-cycle tomato crops. Nutrient UC determined in the present study constitute a sound basis to be used in the libraries of NTS promoting a balanced plant nutrition at high Na+ and Cl− levels in the recycled solution, thus minimizing the need to discharge fertigation effluents. Research is in progress to examine root-derived responses and to give insights related to the nutritional status of the crop, which may provide physiological and productive advantages in tomato crops in regions suffering from scarcity of good-quality water.
Data availability statement
The original contributions presented in the study are included in the article/Supplementary Material, further inquiries can be directed to the corresponding authors.
Author contributions
All authors listed have made a substantial, direct, and intellectual contribution to the work and approved it for publication.
Funding
This work was supported by the Agricultural Research Institute of Cyprus and the Hellenic Foundation for Research and Innovation (HFRI) within the project “NUTRISENSE: Development of an innovative technology using special ion electrodes and suitable software for hydroponic production with emphasis on recycling of the drainage solution in closed systems” (HFRI-FM17-3196).
Conflict of interest
The authors declare that the research was conducted in the absence of any commercial or financial relationships that could be construed as a potential conflict of interest.
Publisher’s note
All claims expressed in this article are solely those of the authors and do not necessarily represent those of their affiliated organizations, or those of the publisher, the editors and the reviewers. Any product that may be evaluated in this article, or claim that may be made by its manufacturer, is not guaranteed or endorsed by the publisher.
Supplementary material
The Supplementary Material for this article can be found online at: https://www.frontiersin.org/articles/10.3389/fenvs.2022.965964/full#supplementary-material
References
Adams, P. (2002). “Nutritional control in hydroponics,” in Hydroponic production of vegetables and ornamentals. Editors D. Savvas, and H. C. Passam (Athens: Embryo Publications), 211–261.
Ahn, T. I., Park, J-E., Jung, J. H., Kim, S. M., Yoo, G., Kim, H. S., et al. (2021). Nutrient dosing framework for an emission-free urban hydroponic production. Front. Plant Sci. 12, 768717. doi:10.3389/fpls.2021.768717
Bar-Yosef, B. (2008). “Fertigation management and crops response to solution recycling in semi-closed greenhouses,” in Soilless culture: Theory and practice. Editors M. Raviv, and H. J. Lieth (Amsterdam, Netherlands: Elsevier), 341–424.
Chapagain, B. P., and Wiesman, Z. (2004). Effect of Nutri-Vant-PeaK foliar spray on plant development, yield, and fruit quality in greenhouse tomatoes. Sci. Hortic. 102, 177–188. doi:10.1016/j.scienta.2003.12.010
Colmenero-Flores, J. M., Franco-Navarro, J. D., Cubero-Font, P., Peinado-Torrubia, P., and Rosales, M. A. (2019). Chloride as a beneficial macronutrient in higher plants: New roles and regulation. Int. J. Mol. Sci. 20, 4686–4732. doi:10.3390/ijms20194686
Cuartero, J., and Fernández-Muñoz, R. (1998). Tomato and salinity. Sci. Hortic. 78, 83–125. doi:10.1016/S0304-4238(98)00191-5
De Pascale, S., Orsini, F., Caputo, R., Palermo, M. A., Barbieri, G., and Maggio, A. (2012). Seasonal and multiannual effects of salinisation on tomato yield and fruit quality. Funct. Plant Biol. 39, 689–698. doi:10.1071/FP12152
FAO (2013). Good agricultural practices for greenhouse vegetable crops – principles for mediterranean climate areas. Rome: FAO.
Flexas, J., Bota, J., Cifre, J., Mariano Escalona, J., Galmés, J., Gulías, J., et al. (2004). Understanding down‐regulation of photosynthesis under water stress: Future prospects and searching for physiological tools for irrigation management. Ann. Appl. Biol. 144, 273–283. doi:10.1111/j.1744-7348.2004.tb00343.x
Franco-Navarro, J. D., Brumós, J., Rosales, M. A., Cubero-Font, P., Talón, M., and Colmenero-Flores, J. M. (2016). Chloride regulates leaf cell size and water relations in tobacco plants. J. Exp. Bot. 67, 873–891. doi:10.1093/jxb/erv502
Gianquinto, G., Muñoz, P., Pardossi, A., Ramazzotti, S., and Savvas, D. (2013). Soil fertility and plant nutrition. Good agricultural practices for greenhouse vegetable crops principles for mediterranean climate areas. Rome: FAO.
Gruda, N. S. (2019). Increasing sustainability of growing media constituents and stand-alone substrates in soilless culture systems. Agronomy 9, 298. doi:10.3390/agronomy9060298
Katsoulas, N., Savvas, D., Kitta, E., Bartzanas, T., and Kittas, C. (2015). Extension and evaluation of a model for automatic drainage solution management in tomato crops grown in semi-closed hydroponic systems. Comput. Electron. Agric. 113, 61–71. doi:10.1016/j.compag.2015.01.014
Kim, H. J., Kim, W. K., Roh, M. Y., Kang, C. I., Park, J. M., and Sudduth, K. A. (2013). Automated sensing of hydroponic macronutrients using a computer-controlled system with an array of ion-selective electrodes. Comput. Electron. Agric. 93, 46–54. doi:10.1016/j.compag.2013.01.011
Leonardi, C., and Maggio, A. (2013). “Choice of species and cultivars for protected cultivation,” in Good agricultural practices for greenhouse vegetable crops principles for mediterranean climate areas (Rome: FAO).
Maggio, A., Raimondi, G., Martino, A., and De Pascale, S. (2007). Salt stress response in tomato beyond the salinity tolerance threshold. Environ. Exp. Bot. 59, 276–282. doi:10.1016/j.envexpbot.2006.02.002
Massa, D., Magán, J. J., Montesano, F. F., and Tzortzakis, N. (2020). Minimizing water and nutrient losses from soilless cropping in southern Europe. Agric. Water Manag. 241, 106395. doi:10.1016/j.agwat.2020.106395
Medrano, H., Escalona, J. M., Bota, J., Gulías, J., and Flexas, J. (2002). Regulation of photosynthesis of C3 plants in response to progressive drought: Stomatal conductance as a reference parameter. Ann. Bot. 89, 895–905. doi:10.1093/aob/mcf079
Neocleous, D., Nikolaou, G., Ntatsi, G., and Savvas, D. (2020). Impact of chelated or inorganic manganese and zinc applications in closed hydroponic bean crops on growth, yield, photosynthesis, and nutrient uptake. Agronomy 10, 881. doi:10.3390/agronomy10060881
Neocleous, D., Nikolaou, G., Ntatsi, G., and Savvas, D. (2021). Nitrate supply limitations in tomato crops grown in a chloride-amended recirculating nutrient solution. Agric. Water Manag. 258, 107163. doi:10.1016/j.agwat.2021.107163
Neocleous, D., Ntatsi, G., and Savvas, D. (2017). Physiological, nutritional and growth responses of melon (Cucumis melo L.) to a gradual salinity built-up in recirculating nutrient solution. J. Plant Nutr. 40, 2168–2180. doi:10.1080/01904167.2017.1346673
Neocleous, D., and Savvas, D. (2015). Effect of different macronutrient cation ratios on macronutrient and water uptake by melon (Cucumis melo) grown in recirculating nutrient solution. J. Plant Nutr. Soil Sci. 178, 320–332. doi:10.1002/jpln.201400288
Neocleous, D., and Savvas, D. (2018). Modelling Ca2+ accumulation in soilless zucchini crops: Physiological and agronomical responses. Agric. Water Manag. 203, 197–206. doi:10.1016/j.agwat.2018.03.017
Neocleous, D., and Savvas, D. (2016). NaCl accumulation and macronutrient uptake by a melon crop in a closed hydroponic system in relation to water uptake. Agric. Water Manag. 165, 22–32. doi:10.1016/j.agwat.2015.11.013
Neocleous, D., and Savvas, D. (2013). Response of hydroponically-grown strawberry (Fragaria×ananassaDuch.) plants to different ratios of K:Ca:Mg in the nutrient solution. J. Hortic. Sci. Biotechnol. 88, 293–300. doi:10.1080/14620316.2013.11512968
Neocleous, D., and Savvas, D. (2019). The effects of phosphorus supply limitation on photosynthesis, biomass production, nutritional quality, and mineral nutrition in lettuce grown in a recirculating nutrient solution. Sci. Hortic. 252, 379–387. doi:10.1016/j.scienta.2019.04.007
Nieves-Cordones, M., García-Sánchez, F., Pérez-Pérez, J. G., Colmenero-Flores, J. M., Rubio, F., and Rosales, M. A. (2019). Coping with water shortage: An update on the role of K+, Cl-, and water membrane transport mechanisms on drought resistance. Front. Plant Sci. 10, 1619. doi:10.3389/fpls.2019.01619
Nikolaou, G., Neocleous, D., Christou, A., Kitta, E., and Katsoulas, N. (2020). Implementing sustainable irrigation in water-scarce regions under the impact of climate change. Agronomy 10, 1120. doi:10.3390/agronomy10081120
Nikolaou, G., Neocleous, D., Christou, A., Polycarpou, P., Kitta, E., and Katsoulas, N. (2021). Energy and water related parameters in tomato and cucumber greenhouse crops in semiarid mediterranean regions. A review, part II: Irrigation and fertigation. Horticulturae 7, 548. doi:10.3390/horticulturae7120548
Orsini, F., Sanoubar, R., Oztekin, G. B., Kappel, N., Tepecik, M., Quacquarelli, C., et al. (2013). Improved stomatal regulation and ion partitioning boosts salt tolerance in grafted melon. Funct. Plant Biol. 40, 628–636. doi:10.1071/FP12350
Parida, A. K., and Das, A. B. (2005). Salt tolerance and salinity effects on plants: A review. Ecotoxicol. Environ. Saf. 60, 324–349. doi:10.1016/j.ecoenv.2004.06.010
Ropokis, A., Ntatsi, G., Kittas, C., Katsoulas, N., and Savvas, D. (2018). Impact of cultivar and grafting on nutrient and water uptake by sweet pepper (Capsicum annuum l.) grown hydroponically under mediterranean climatic conditions. Front. Plant Sci. 9, 1244. doi:10.3389/fpls.2018.01244
Sambo, P., Nicoletto, C., Giro, A., Pii, Y., Valentinuzzi, F., Mimmo, T., et al. (2019). Hydroponic solutions for soilless production systems: Issues and opportunities in a smart agriculture perspective. Front. Plant Sci. 10, 923. doi:10.3389/fpls.2019.00923
Savvas, D., and Adamidis, K. (1999). Automated management of nutrient solutions based on target electrical conductivity, pH, and nutrient concentration ratios. J. Plant Nutr. 22, 1415–1432. doi:10.1080/01904169909365723
Savvas, D., Gianquinto, G., Tuzel, Y., and Gruda, N. (2013). “Soilless culture. Good agricultural practices for greenhouse vegetable crops,” in Principles for mediterranean climate areas (Rome: FAO).
Savvas, D., and Gruda, N. (2018). Application of soilless culture technologies in the modern greenhouse industry - a review. Eur. J. Hortic. Sci. 83, 280–293. doi:10.17660/eJHS.2018/83.5.2
Savvas, D., and Neocleous, D. (2019). “Developments in soilless/hydroponic cultivation of vegetables,” in Achieving sustainable cultivation of vegetables. Editor G. Hochmuth (Cambridge: Burleigh Dodds Science Publishing Limited), 1–33.
Savvas, D., Ntatsi, G., and Drakatos, S. (2020). A decision support system to automatically calculate and readjust nutrient solutions in commercial soilless cultivations. Acta Hortic. 1271, 293–300. doi:10.17660/ActaHortic.2020.1271.40
Savvas, D. (2002). “Nutrient solution recycling,” in Hydroponic production of vegetables and ornamentals. Editors D. Savvas, and H. C. Passam (Athens: Embryo Publications), 299–343.
Savvas, D. (2000). Nutritional management of gerbera (Gerbera jamesonii) grown in a closed soilless culture system. Acta Hortic. 554, 175–182. doi:10.17660/ActaHortic.2001.554.18
Signore, A., Serio, F., and Santamaria, P. (2016). A targeted management of the nutrient solution in a soilless tomato crop according to plant needs. Front. Plant Sci. 7, 391. doi:10.3389/fpls.2016.00391
Sonneveld, C. (2002). “Composition of nutrient solution,” in Hydroponic production of vegetables and ornamentals. Editors D. Savvas, and H. C. Passam (Athens: Embryo Publications), 179–210.
Sonneveld, C. (2000). Effects of salinity on substrate grown vegetables and ornamentals in greenhouse horticulture. Wageningen: Wageningen Agricultural University.
Sonneveld, C., and Straver, N. (1994). Nutrient solutions for vegetables and flowers grown in water or substrates. Ser. Voedingsoploss. Glastuinb. 8, 45.
Sonneveld, C., and Voogt, W. (2009). Plant nutrition of greenhouse crops. Dordrecht: Springer. doi:10.1007/978-90-481-2532-6
Keywords: recycling drainage, fertilizers, water, NUTRISENSE, salinity, greenhouses, tomato crops
Citation: Neocleous D and Savvas D (2022) Validating a smart nutrient solution replenishment strategy to save water and nutrients in hydroponic crops. Front. Environ. Sci. 10:965964. doi: 10.3389/fenvs.2022.965964
Received: 10 June 2022; Accepted: 18 August 2022;
Published: 07 September 2022.
Edited by:
Maria Elisa Magri, Federal University of Santa Catarina, BrazilReviewed by:
Hayssam M. Ali, King Saud University, Saudi ArabiaNicola Dal Ferro, University of Padua, Italy
Copyright © 2022 Neocleous and Savvas. This is an open-access article distributed under the terms of the Creative Commons Attribution License (CC BY). The use, distribution or reproduction in other forums is permitted, provided the original author(s) and the copyright owner(s) are credited and that the original publication in this journal is cited, in accordance with accepted academic practice. No use, distribution or reproduction is permitted which does not comply with these terms.
*Correspondence: Damianos Neocleous, ZC5uZW9jbGVvdXNAYXJpLmdvdi5jeQ==; Dimitrios Savvas, ZC5zYXZ2YXNAYXVhLmdy
†These authors have contributed equally to this work