- 1School of Animal Plant and Environmental Sciences, University of the Witwatersrand, Johannesburg, South Africa
- 2Flora Fauna & Man Ecological Services Ltd, Road Town, British Virgin Islands
- 3Division of Vertebrate Zoology, Department of Ichthyology, American Museum of Natural History, New York, NY, United States
- 4School of Natural Resources, University of Missouri, Columbia, MO, United States
- 5South African Institute for Aquatic Biodiversity, Makhanda, South Africa
- 6Centre for African Ecology, School of Animal, Plant and Environmental Sciences, University of the Witwatersrand, Johannesburg, South Africa
- 7School of Biology, Faculty of Biological Sciences, University of Leeds, Leeds, United Kingdom
Characterizing functional freshwater biodiversity patterns and understanding community determinants can aid in predicting changes and prioritizing specific conservation management actions. Afrotropical freshwater systems support diverse ecological communities, including many taxonomically undescribed species, yet few studies have been undertaken in this region and they remain poorly understood. Here, we use functional trait-based approaches to describe the functional diversity of fish assemblages in the Republic of Congo (west Central Africa) from the headwaters of a large basin (upper basin) to the coastal plains (lower basin). Functional traits were assessed using multivariate ordination, and functional diversity was calculated using functional trait-space occupation. We investigated if fish β diversity is nested regionally and at a basin-scale to understand if species assemblages exhibit non-random or environmental filtering patterns. Trait-environment relationships were modeled to determine the most significant drivers of trait diversity, and functional richness (FRic) was calculated for communities in both basins to identify whether Afrotropical systems conform to expected patterns of biodiversity along a fluvial gradient. Moderate to weak community nestedness and various traits associated with resistance and feeding were significantly correlated with habitat variables in the upper and lower basins respectively. Whilst environmental filtering is an important process of community assembly at basin scale, non-random processes are evident in the upper basin, whereas a high turnover was noted in the lower basin. Trophic traits in larger coastal plain habitats of the lower basin suggest higher trophic diversity is associated with higher spatial heterogeneity and nutrient concentrations. High functional turnover between headwaters and coastal plains suggests different functional strategies in fish assemblages between localities along the fluvial gradient. In contrast to expectations derived from temperate lotic systems, functional richness was comparable across both basinswhich suggests that headwater zones in the region harbor substantial functional diversity, especially when considering upstream catchment size. Functional diversity and environment patterns documented show unique patterns of functional richness that may assist in improving understanding of responses, vulnerabilities and conservation needs in Afrotropical systems.
1 Introduction
General expectations for fish diversity patterns across large river basins are based on documented relationships with climate, physiography, flow conditions, and ecosystem size, but unique biogeographical characteristics in some regions may drive exceptions to predicted patterns (Heino et al., 2013). The conceptual fundamentals of lotic ecology were mainly derived from the study of small forested mesic streams in Europe and North America, (Horwitz, 1978; Vannote et al., 1980; Newbold et al., 1982), with studies in the tropics only appearing more recently (Ward et al., 2002; Minshall, 2007; Dudgeon, 2008). Lotic theory suggests that the environmental continuum changes in predictable ways across the gradient of larger basins, for example the River Continuum Concept suggests that changes in resources along a river continuum dictate the trophic structure of biotic assemblages (Vannote et al., 1980). A central tenet of the concept is that species are replaced along the continuum, and that aquatic biodiversity is lower in the headwaters and is highest in the middle and lower reaches where habitat, flow and riparian cover are optimal, allowing maximum exploitation of resources. In contrast, the Riverine Habitat Template (Townsend and Hildrew, 1994) emphasizes the importance of spatial and temporal heterogeneity for community structure, proposing that they provide the template upon which evolution forges functional species traits. Using this theory, one can predict that in the upper reaches of rivers with steep gradients, high temporal variability and homogenous habitat, a community would show traits that infer resilience and resistance such as firm attachment mechanisms, high mobility, streamlined and flexible bodies and/or dorsoventrally flattened bodies. Conversely, more stable habitats with more habitat heterogeneity such as in the lower parts of river basins will show species with a higher functional trait diversity of less resilient traits (i.e., long lifespans, diverse body forms).
Community ecology has increasingly incorporated the functional trait framework to provide a mechanistic understanding of the structure of communities, ecosystem processes and general patterns shaping communities making it a useful tool for exploring the adaptability and vulnerability of communities to environmental stressors (McGill et al., 2006; Poff et al., 2006; Villéger et al., 2008; Olden and Kennard, 2010; Pease et al., 2012, Pease et al., 2015; Nock et al., 2016; Villéger et al., 2017). In comparison to purely taxonomic studies, a functional trait-based approach allows data to be compared without the constraints of geography, climate or highly divergent taxa (Poff, 1997; Poff et al., 2006; Pease et al., 2012; Bower and Winemiller, 2019a; Bower and Winemiller, 2019b), can support understanding of processes governing regional community assembly (Mouillot et al., 2007; Pease et al., 2015) and guide regionally specific management and conservation of aquatic systems (Sternberg and Kennard, 2013).
The functional trait approach has been used to understand community assemblages in tropical regions at different spatial scales, including environmental filtering and limiting similarity (Bower and Winemiller, 2019a; Bower and Winemiller, 2019b), aquatic-terrestrial linkages (Teresa and Casatti, 2012; Correa and Winemiller, 2014, 2018; Arantes et al., 2019) and environmental gradients (Ibanez et al., 2007; Pease et al., 2012; López-Delgado et al., 2019a, López-Delgado et al., 2019b). Some studies found that gradients of biotic richness and functional diversity in tropical systems conformed with aspects of temperate gradient concepts (Greathouse and Pringle, 2006; Ibanez et al., 2007; Pease et al., 2012). In contrast, numerous other findings suggested environmental filtering as the primary driver of community assemblage (Mouillot et al., 2007; Mouchet et al., 2013; Troia and Gido, 2015; Córdova-Tapia et al., 2018; Bower and Winemiller, 2019b). Boulton et al. (2008) noted in a review that published evidence for consistent differences in ecology and response of tropical streams was generally lacking in literature and argues that the term ‘tropical’ potentially has little significance when applied to stream ecology.
Afrotropical freshwaters support diverse ecological communities, with many species still unknown to science (Darwall et al., 2011; Reid et al., 2018) yet they are significantly under-represented in ecological literature (Dudgeon, 2008; Kessler et al., 2011; di Marco et al., 2017; Titley et al., 2017). Historically the rate of exploitation of natural resources in Afrotropical forests of the Congo Basin has been markedly slower than in other tropical regions (Malhi et al., 2013; Rudel, 2013; van Rooyen et al., 2016; van Rooyen et al., 2019), but over the past decade this pattern has rapidly changed as many central African countries have stabilized politically, resulting in a sharp increase in development, resource extraction, and a range of associated environmental impacts (Abernethy et al., 2013; Megevand et al., 2013; Petrozzi et al., 2016). The rapidly increasing impacts felt in the region and the paucity of freshwater research on Afrotropical biodiversity is a growing obstacle for freshwater conservation (Grill et al., 2019; Leclère et al., 2020; Tickner et al., 2020). In the absence of a broad and data-rich understanding of ecosystems, characterizing functional diversity patterns in freshwater communities has aided in predicting changes, pinpointing vulnerabilities, and prioritizing regional conservation actions (Toussaint et al., 2016).
In this study, we characterize the functional trait-environment and diversity relationships of fish assemblages along an environmental gradient in the Republic of Congo. We characterized functional trait-environment relationships in the upper and lower sections of the basin to understand what is likely driving community assemblage structure. We assessed nestedness of species assemblages at site level to understand if non-random or filtering process of species assemblage underlie patterns of community structure. Lastly, we calculated functional beta-diversity and the volume of functional trait space per basin to understand how functional diversity changes longitudinally. Based on the River Continuum Concept and previous functional studies of fish communities in large tropical basins (Ibanez et al., 2007; Pease et al., 2012) we hypothesize that functional trait diversity would increase along the longitudinal gradient from the upper to lower basin and that feeding traits would reveal the change of food resources along the continuum. Based on the Riverine Habitat Template concept we expected to see evidence of environmental filtering and functional turnover where upper basin assemblages are dominated by traits that are adapted to resistance and hydrodynamics, with the lowland basin containing more diverse feeding traits representative of the increased resources as habitat becomes more heterogenous and stable.
2 Materials and methods
2.1 Study region
The study area is situated in the Republic of Congo over two geographically diverse areas: the upper study area in the Kouilou-Niari River (the Louessé and Mandoro rivers), and the coastal plains of the Kouilou, Loémé and Noumbi basins (Figure 1). The headwaters of the Kouilou-Niari River system originate in the upper basin (Figure 1) and are situated on the Chaillu Massif which consists of incised formations with steep-sloped hills and mountains covered by rare forest types (Mamonekene and Stiassny, 2012; van Rooyen et al., 2019). The upper basin freshwater habitat comprises forest streams on steep slopes, and rivers with varying degrees of forest cover on moderately steep slopes. (Figure 2A–H). The lower basin landscape is crossed by a dense network of drainage channels flowing into larger rivers and natural lakes resulting in high freshwater habitat heterogeneity (Walsh et al., 2014; van Rooyen et al., 2016; Walsh et al., 2022) (Figures 3A–H). The lower basin is situated on gentle slopes and is covered by a mosaic of forest and grassland-savannah.
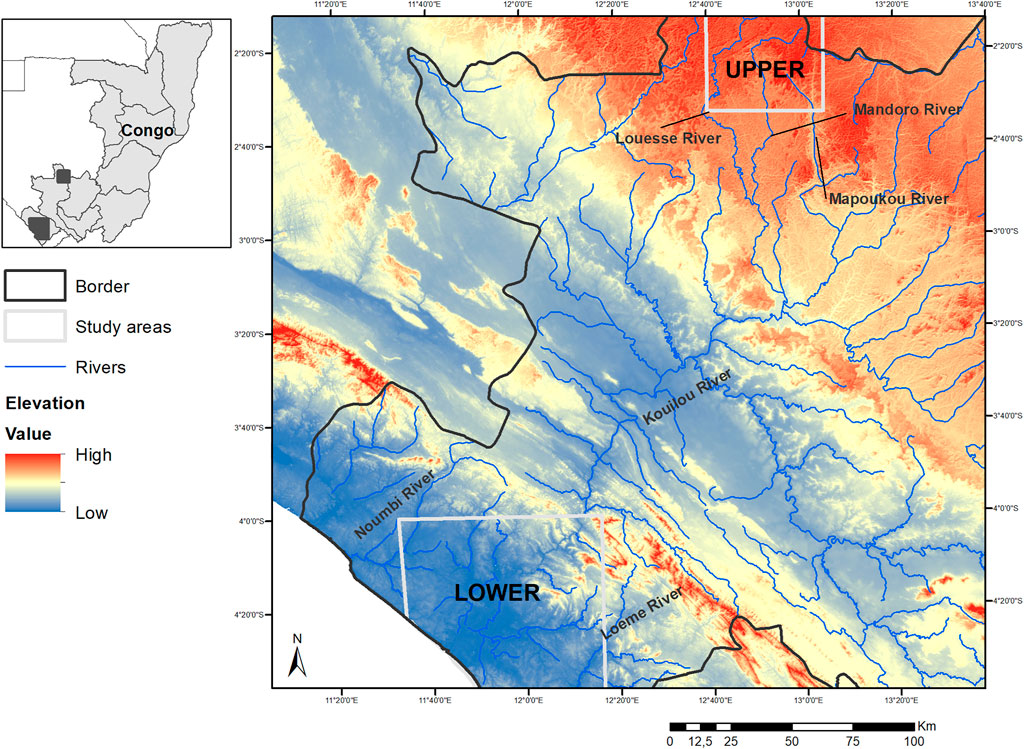
FIGURE 1. Upper and lower basin study areas in the Kouilou, Loémé and Noumbi basins in the Republic of Congo showing major drainages and elevations. The highest point regionally is approximately 750 m above sea level.
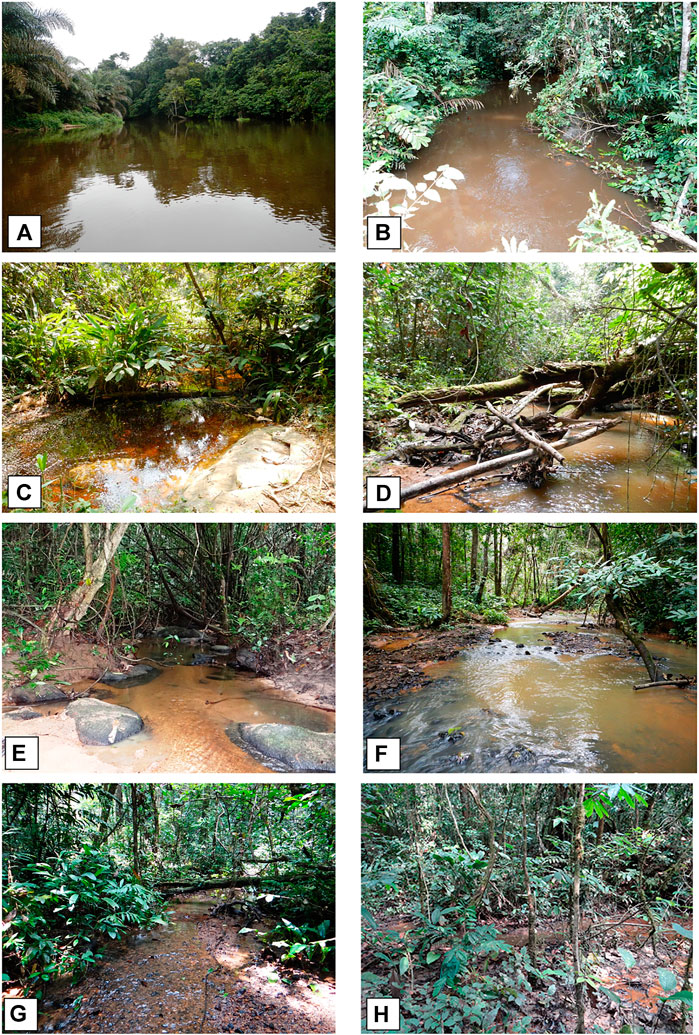
FIGURE 2. Major mesohabitat types sampled in the upper basin in the Louessé and Mandoro river drainages (A)–(B) Rivers; (C)–(G) forest streams, and (H) swamp forests.
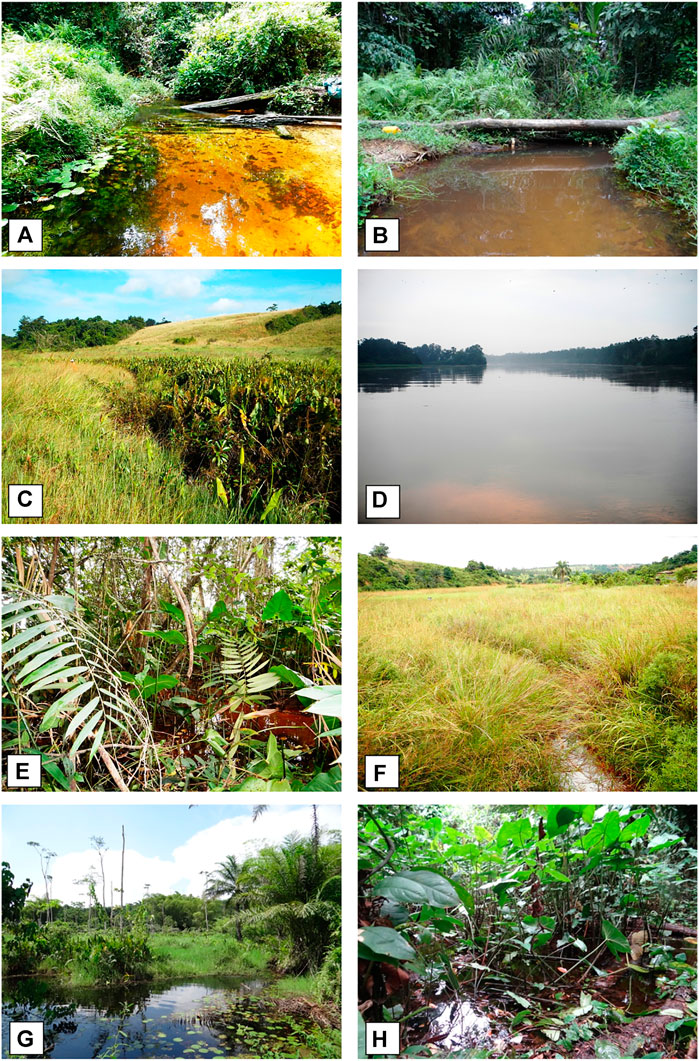
FIGURE 3. Major mesohabitat types sampled in the lower basin coastal study area in the Kouilou, Loémé and Noumbi basins (A)–(B) Forest streams; (C) coastal savannah stream; (D) rivers; (E) swamp forests; (F) savannah wetlands; (G) Mitragyne stipulosa swamp forest; and (H) tall swamp forest.
2.2 Field data collection
The study included a total of 90 sites (48 in the upper and 42 in the lower basin). Study sites included a range of stream orders encompassing swamp forests, headwater tributaries, larger tributaries, and main stem river channels (Figures 2, 3). Sites were sampled under base flow conditions in August 2012 October 2013, and October 2014 where fish capture was most efficient due to manageably low flows.
A 200–400 m stream reach was sampled to representatively include habitat and microhabitat units at each site in the upper and lower basins. Fishes were collected from all habitat and microhabitat types within each reach within each basin position (upper and lower) in a nested design (Smith et al., 2013; Bower and Winemiller, 2019b). Because of the diversity of habitat types in the study region, various fish sampling methods were applied depending on the habitat available at each site, including seine hauls, cast netting, dip-netting, gill netting, fyke netting and backpack electrofishing with a single pass moving in an upstream direction. We assume that survey methods and study design allow for the comparison of communities between sites as site sampling was applied consistently until no new species were collected at a site (Walsh et al., 2014, Walsh et al., 2022). Species accumulation curves were constructed for surveys to verify efficiency of sampling using the Observed richness (Sobs) and mean estimated richness by Chao 1 estimates in Primer 7 (Clarke and Gorley, 2015; Walsh et al., 2022). Fishes were deposited in the Ichthyology Collection of the American Museum of Natural History in New York, United States, with all data accessible online (https://emu-prod.amnh.org/db/emuwebamnh/).
Landscape and local environmental data were collected for all sites and the Spatial Analyst Hydrology package for ArcGIS 10.5 was used to generate river networks and Strahler orders. A total of 47 environmental parameters were measured including physical measures of width, depth and slope, in situ water quality and water samples for basic ion and nutrient analysis. Micro-habitat cover for 8 classes was estimated per reach and velocity-depth class according to Kleynhans (2008). Environmental data were assessed for multicollinearity using the car and caret packages in the R environment (Fox and Weisberg, 2019) where r values of >0.7 were removed from the environmental dataset reducing the total number of variables to 41 (Table 1).
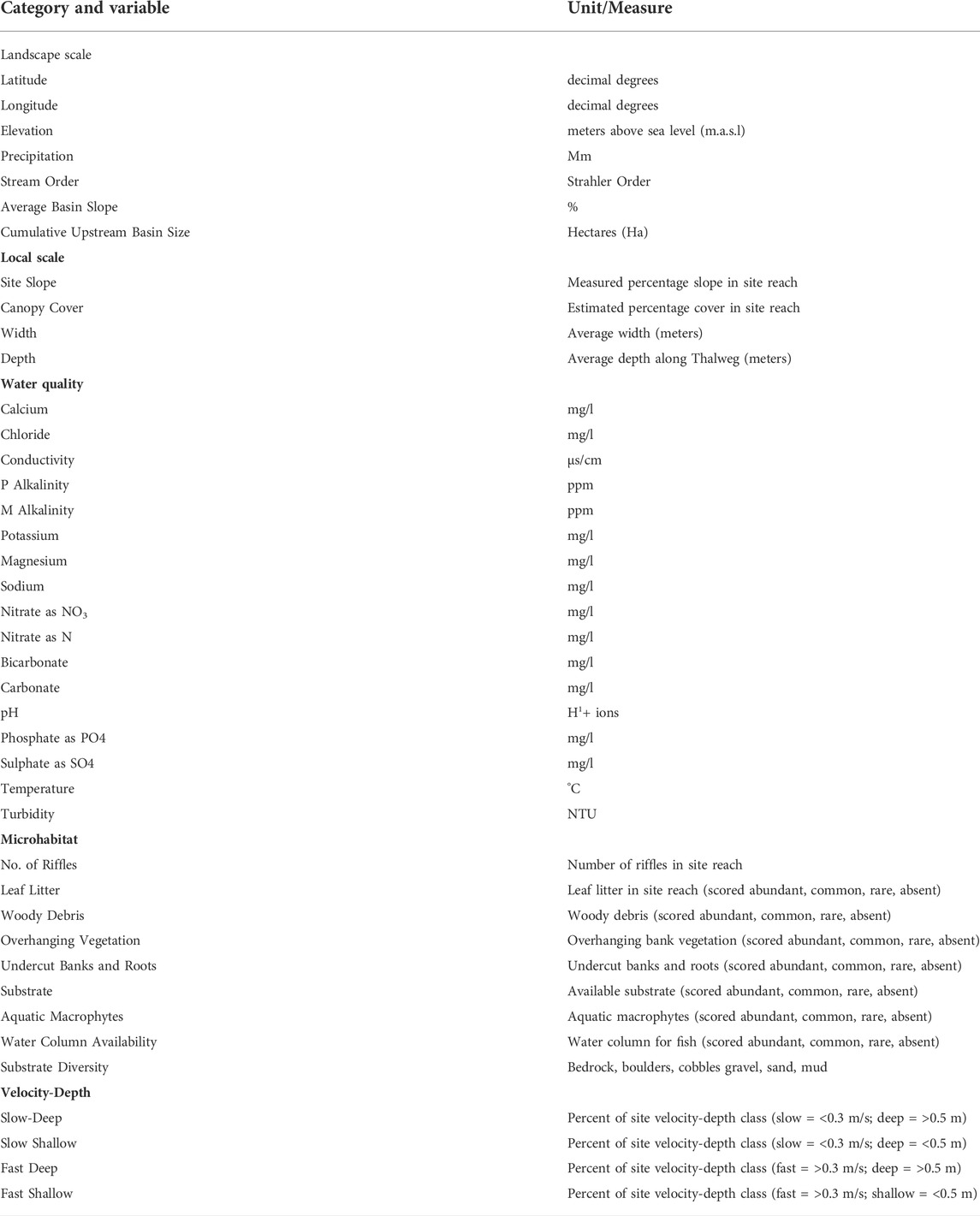
TABLE 1. Landscape and local environmental and physical variables measured at sites in lower and upper basin of the Kouilou-Niari basin.
2.3 Morphometric traits
Twenty-three functional traits were measured from fish specimens (Table 2). The traits chosen show relationships with habitat-use, locomotion, life history strategy and feeding (Winemiller, 1991; Winemiller and Rose, 1992; Sibbing and Nagelkerke, 2000; Pease et al., 2012). Five individual specimens of each species were measured on a Nikon SMZ800 microscope camera connected to a computer with the NIS-Elements D computer application and a 1× objective lens. Where we encountered less than five individuals of a species, available specimens were measured. Because depth could not be measured accurately using the software, head depth, body depth and oral gape were measured using calipers with measurements taken to the nearest 0.1 mm (Winemiller, 1991; Winemiller and Rose, 1992). To eliminate ontogenetic and sexual dimorphic biases, only adults of all species, and females of sexually dimorphic species (e.g., Alestidae, Cichlidae, and Nothobranchiidae) were included in the analysis. Before proceeding with the functional trait analyses, ANOVAs were performed on trait variance data to confirm that interspecific variation was greater than intraspecific variation (p < 0.05).
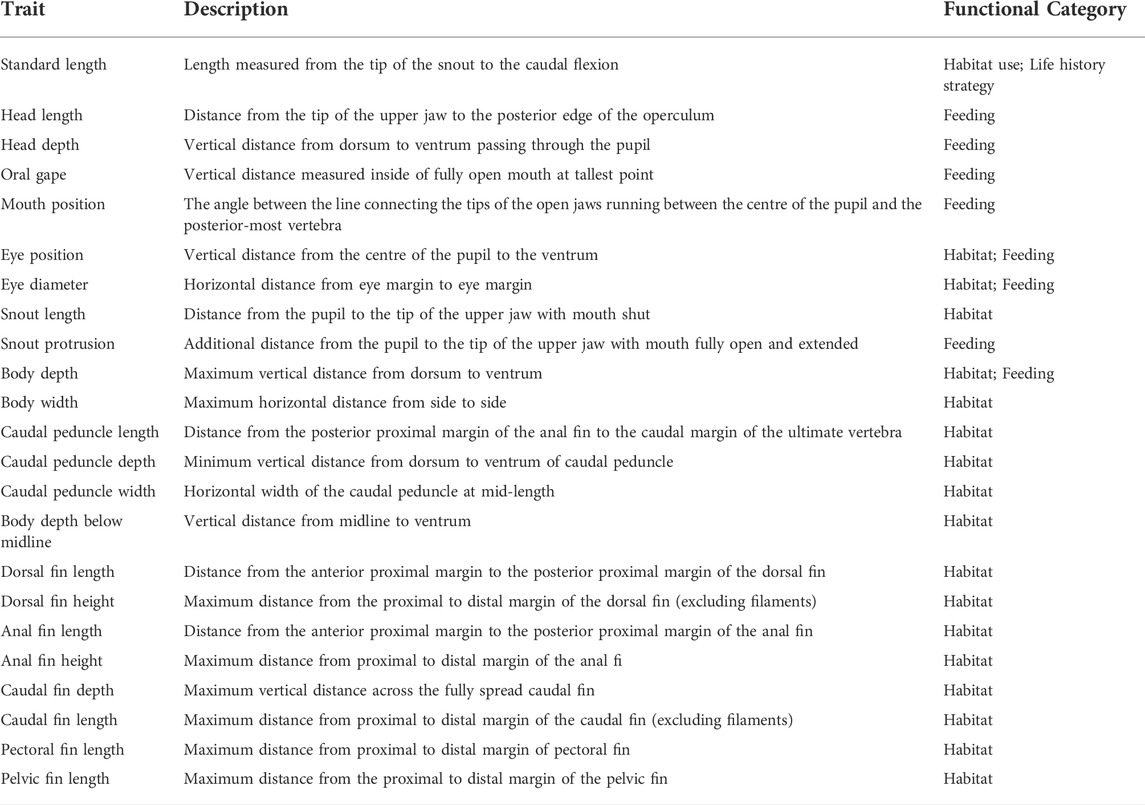
TABLE 2. Functional traits used for upper and lower basin fish assemblages with description of measurement from Pease et al. (2012). Trait definitions and functional categories follow (Gatz, 1979; Webb, 1984; Winemiller, 1991; Winemiller and Rose, 1992; Sibbing and Nagelkerke, 2000).
2.4 Statistical methods
2.4.1 Environmental correlates of fish community structure
The environmental variables matrix was refined to include variables with Z-scores of Spearman’s correlation at a significance of p < 0.05, which reduced the environmental dataset to 29 variables. To show the relationship between sites based on environmental variables, a Principal Component Analyses (PCA) was performed on the refined environmental dataset using normalized environmental data and a matrix based on Euclidean distance. Only variables with a Pearson’s correlation of >0.5 were shown in the PCA.
A stepwise distance-based linear model permutation (DistLM) identified environmental variables which were predictors of fish community structure, considering possible interactions between environmental variables (Clarke and Gorley, 2015). The resemblance matrix was calculated using Log(X+1) Bray-Curtis similarity and transformed. An AIC (Akaike’s Information Criterion) selection criterion was run using 999 permutations with a forward variable selection procedure. Results were represented using a distance-based redundancy analysis (dbRDA). The outcome of the analysis shows vectors representing the combination of environmental variables that were the best predictors of fish community structure at sites. All statistical analyses were completed in Primer 7 and R studio v 4.1.3 (Clarke and Gorley, 2015; RStudio Team, 2021).
Functional traits are phenotypic characteristics that influence ecosystem and community processes. A PCA was performed on the functional trait data collected in Table 2 to show functional trait space and functional traits governing the placement of species in the upper and lower basins. Traits were regressed against standard length (SL), and residuals were used to generate the PCA from a matrix based on Euclidean distance of transformed values (Pease et al., 2012). Mouth position was not correlated with the body size, therefore values for this trait were log transformed and used instead of a residual.
2.4.2 Taxonomic spatial nestedness and species turnover
Βeta diversity can reflect either nestedness or spatial turnover. Nestedness of species assemblages occurs when the species at sites with lower richness are subsets of the species at sites with greater richness (Baselga, 2010) implying a non-random process of species assemblage and structural patterns (Gaston and Blackburn, 2000). Spatial turnover implies the opposite of nestedness and the replacement of some species by others due to environmental filtering and sorting (Qian et al., 2005).
We assessed whether fish β diversity was nested regionally and at a basin scale through the nestedness metric based on overlap and decreasing fill (NODF) (Ulrich and Almeida-Neto, 2012). The NODF quantifies the nestedness among sites or species independently. Values for NODF are between 0 (zero nestedness) and 100 (perfect nestedness). The significance of the NODF was assessed using the CE (proportional) null model which assigns a probability to each matrix cell to be occupied proportional to the corresponding row and column totals (Strona and Fattorini, 2014). To test for the existence of landscape and habitat filtering patterns of β diversity at the regional and basin scale (Townsend, 1989; Townsend and Hildrew, 1994; Poff, 1997; Winemiller, Flecker and Hoeinghaus, 2010), assemblage data were split into upper and lower basin sets, and then combined for the final analysis. The NODF nestedness analysis was performed using NeD software (Strona and Fattorini, 2014).
2.4.3 Functional trait-environment relationships
Fourth-corner analysis was used to test relationships between species traits and environmental variables in the ade4 package in R Studio (Legendre et al., 1997; Dray and Dufour, 2007; Dray et al., 2014). The fourth-corner function measures the predictions by a Pearson correlation coefficient for two quantitative variables (i.e., trait and environmental variable), by Pearson χ2 and G statistic for two qualitative variables and by a Pseudo-F and Pearson r for one quantitative variable and one qualitative variable. The significance of relationships (p value = <0.05) was tested by a permutation of sites and species which avoids inflated Type 1 errors (Dray et al., 2014).
2.4.4 Functional trait space and β-diversity
All functional diversity analyses were computed using the mFD package (Magneville et al., 2022). We compared upper and lower basin trait space using Functional richness (FRic - Cornwell et al., 2006; Villéger et al., 2008) which represents the volume of the convex hull defining the functional space present in each basin assemblage. Each trait was given equal weight in calculating the distance matrix and was computed in a 5-dimensional space which accounted for 91% of the variance.
Βeta functional diversity was calculated for the two basins using mFD: β.fd.multidim, which computes pairwise functional β-diversity, using Jaccard dissimilarity, and resultant components of turnover and nestedness (Villéger et al., 2013). Jaccard dissimilarity indicates the functional dissimilarity between β diversity of the set of functional traits per basin. Click or tap here to enter text.
3 Results
3.1 Environmental predictors and fish community
A total of 80 species in 22 families of the Teleostei were collected over the two study areas, with 41 species in the upper basin and 49 species in the lower basin. Family level classification followed van der Laan et al. (2014). Approximately 50% of the species from the upper and lower basins were members of the following four families: Cyprinidae (23.8%), Mormyridae (10.0%), Cichlidae (8.8%) andClariidae (8.8%). Species richness ranged from three in small forest streams and swamp forests, to 19 in larger river systems and generally increased further downstream along the river gradient.
The first two axes of the environmental PCA explained 31.6% of the variation between sites (Figure 4A). Sites separated strongly between upper and lower basins on the PC1 axis (representing 17.1% of variance) as a function of mostly landscape-scale variables. Lower basin sites had highest variable loadings for water temperature and salt concentrations (Chloride). The upper basin site placement was related to higher precipitation and steeper average catchment slopes according to the eigenvalues. The PC2 axis (representing 14.5% of variance) separated sites based on habitat type (Figure 4B), discriminating between fast velocity sites with larger catchments, diverse substrate and woody cover, and slow-deep sites with dense vegetation cover consisting of various streams and swamp forests.
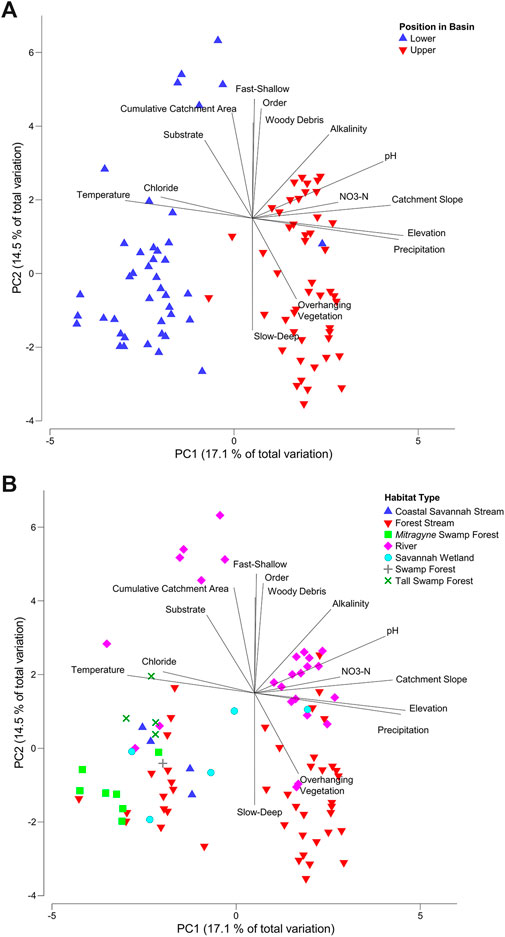
FIGURE 4. PCA with local and landscape scale normalized data using Euclidean distance (A) with position in basin, and (B) habitat type as factor. Vectors were included only from variables with Pearson’s correlation of >0.5. Temp = temperature. M Alk = total alkalinity. Ave = Average.
The DistLM analyses showed patterns of fish community structure based on the combination of environmental predictors that contribute most significantly to the species composition of the fish assemblages at sites. The sites were separated by position in the basin (Supplementary Figure S1). The first two dbRDA axes explained 78.96% of the variation out of the fitted model, and 29.59% of the total variation. The dbRDA1 axis explained 69.70% of fitted and 24.8% of total variation and split lower and upper basin fish assemblages based on landscape scale variables, with catchment size, average catchment slope and elevation (p < 0.001) gradients being the most important predictors on this axis. The dbRDA2 axis included site-scale variables along a hydrological (p < 0.001) and NO3-N (p < 0.002) gradient. Most swamp forest and wetland habitat types were split from rivers on the dbRDA2 axis based on these local variables. Due to the clear separation of sites based on environmental and fish community data between basins, the remainder of the analyses were split such that the lower and upper basin data were analyzed separately.
3.2 Taxonomic spatial nestedness
Fish assemblages showed varying degrees of nestedness between upper and lower basins, and across the entire basin (Figures 5A–C). Upper basin sites were moderately nested (NODF = 50.64, z = 14.4, p < 0.001) representing ordered ß diversity and species assemblage structure, where depauperate species assemblages constitute subsets of increasingly richer ones in the landscape (Figure 5A). The lower basin indicated a weak nestedness of assemblages, with NODF values that were lower than the upper basin (Figure 5B- NODF = 20.37, z = 12.57, p < 0.001). Combining the data from upper and lower basin to assess the continuum and turnover at a basin level, species assemblages were weakly nested indicating weak non randomness between ß diversity across the entire basin (Figure 5C - NODF = 24.122, z = 12.57, p < 0.001).
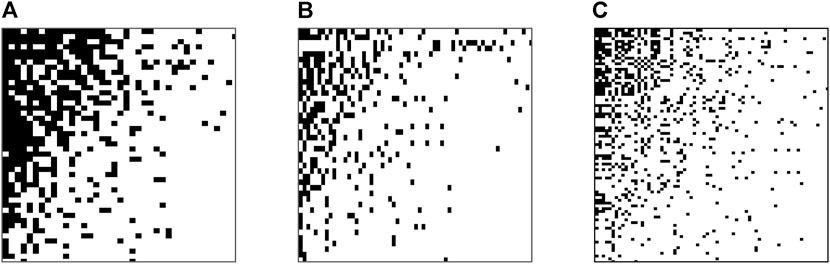
FIGURE 5. Packed nestedness showing distribution patterns of fish assemblages in (A) upper basin and (B) lower basin and (C) upper and lower basin using NODF metrics to overlap and decrease fills with the CE null model.
3.3 Functional trait-environment relationships
Functional trait composition of fish assemblages differed between upper and lower basins (Figures 6A,B); however, primary axes of trait variation were similar in both, with functional traits associated with locomotion strategies (maneuverability vs hydrodynamism) and feeding (position in water column and maximization of food intake) noted as primary drivers of patterns in the fish communities. Phylogenetic influence on functional traits was stronger in the upper basin than in the lower basin.
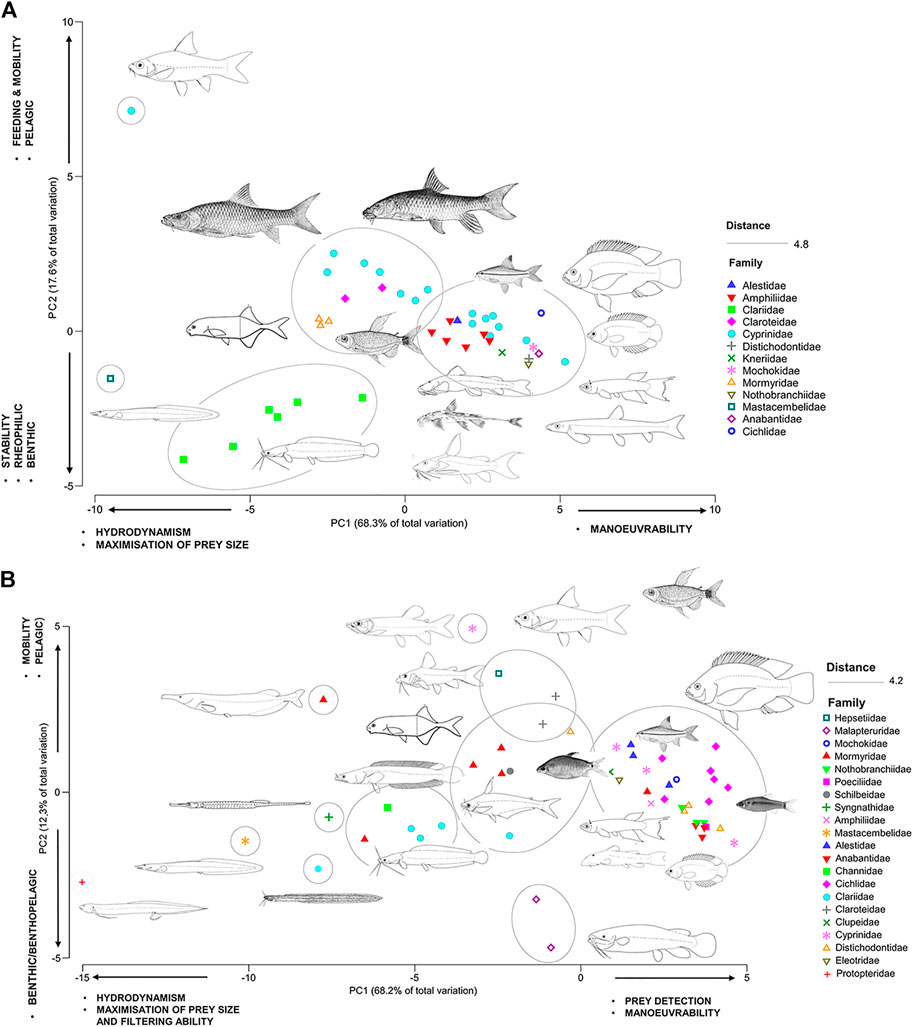
FIGURE 6. Functional traits PCA ordination for (A) Upper basin and (B) Lower basin species showing the position of the fish species in two dimensions of functional trait space. Traits used to characterize the two axes had correlation coefficients ≥0.25.
Upper basin sites with steep slopes contained higher abundances of fusiform and dorso-laterally flattened species and displayed a distinct phylogenetic signature. The PCA of functional traits of the upper basin species explained 85.9% of variation among species (Figure 6A). The PC1 axis (68.3% of variance) showed a gradient of maneuverability/feeding strategy (pectoral fin length, body width, dorsal fin height and eye diameter; positive side of PC1) and hydrodynamics (mouth position, snout protrusion; negative side of PC1). The clariids (Channallabes apus, Clariallabes longicauda, Clarias camerunensis, C. pachynema and C. gabonensis), mastacembelids (Mastacembelus niger) and one algae scraping cyprinid (Labeobarbus axelrodi) had the most negative eigen loadings on PC1. These species are elongate with sub-terminal mouths. The second PC axis (PC2, explaining 17.6% of variance) for the upper basin functional traits separated traits related to water column position where benthopelagic traits (pectoral fin length, head length, snout length, and caudal fin height) showed negative eigen loadings and pelagic traits (eye diameter, dorsal fin height and snout protrusion) were less negative. The elongate clariids (C. apus, C. longicauda, C. camerunensis, C. pachynema, C. longior and C. gabonensis) had the lowest loadings on PC2, with the large-bodied and streamlined cyprinids (Labeobarbus roylii, L. compinei, L. progenys, L. axelrodii and Labeo camerunensis) exhibiting the highest loadings on this axis.
Lower basin sites with more gentle slopes, slow-deep habitat types, higher salt concentrations, and warmer temperatures contained more species with deep compressed and/or elongated bodies and a less distinct phylogenetic influence. The PCA of functional traits of the lower basin species explained 80.5% of variation among species (Figure 6B). The PC1 axis (68.2% of variance) showed a gradient of prey detection and maneuverability (head depth, eye position, pectoral fin length, and body depth) and hydrodynamics and maximization of prey size (mouth position, snout protrusion, and anal fin length). Species of the Cichlidae, Alestidae, Anabantidae, and Cyprinidae with relatively large heads, eyes and body depths had higher positive eigen loadings on PC1, while elongate species of Clariidae, Channidae, Protopteridae, and Syngnathidae had high negative values on PC1. The second PC axis (PC2, explaining 12.3% of variance) separated functional traits related to water column position with benthopelagic traits (head length, caudal fin depth and width and snout length) showing more negative eigen loadings and pelagic species (caudal peduncle width, anal fin height and eye diameter) showing positive loadings. The malapterurids and the elongate clariid C. apus had the lowest loadings on PC2, while the large-bodied and streamlined Hepsetus lineatus, Labeobarbus cardozoi and Oxymormyrus zanclirostris had the highest loadings on this axis.
The fourth-corner analysis (Figure 7) for the upper basin showed two interesting patterns in the species-trait-environment data with a distinct splitting of rhithronic and potamonic species. Only significant trait-environment relationships are reported here. Firstly, inferior mouth position was positively correlated with slope and negatively correlated with Nitrate-Nitrogen (NO3), inferring resistant traits capable of withstanding faster velocities, and benthic feeding patterns in oligotrophic habitat in lower-order systems. This fish group tends to mostly be represented by species in the lower right side of the upper-basin trait PCA from the Mochokidae, Amphilidae and Kneriidae (Figure 6A). Secondly, body width, caudal peduncle width, caudal peduncle length, dorsal fin length, pelvic fin length and anal fin length were all significantly positively correlated with variables related to instream structural cover (stones, algae, woody debris, substrate) and fast-flowing water. These traits were also negatively correlated with vegetation, aquatic macrophytes and slow-deep water which also suggests filtering out of laterally compressed species such as cichlids which generally prefer more lentic and vegetated habitat, and selection for fusiform fishes that require more mobility for feeding (e.g., Cyprinidae).
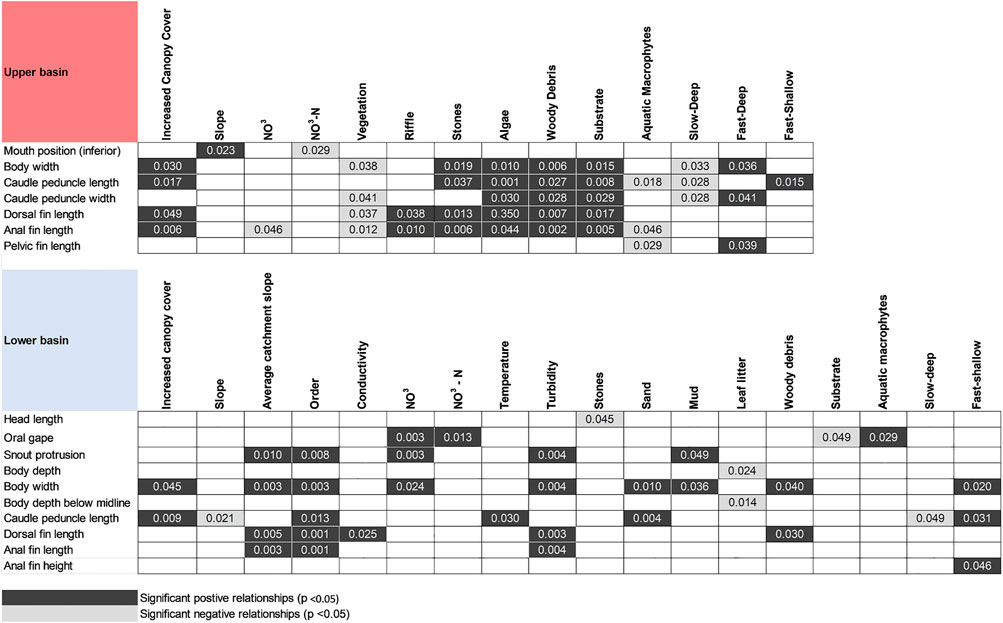
FIGURE 7. Results of the fourth-corner analyses for the upper and lower basin dataset. Black signifies a significant positive relationship (Pearson’s correlation) between environmental variable and trait, and dark grey a significant negative relationship.
A divide associated largely with trophic ecology was noted in the lower basin based on trait-environment relationships (Figure 6B; Figure 7). Trophic traits in the lower basin suggest higher trophic diversity is associated with higher spatial heterogeneity and potentially nutrient concentrations. Maneuverable fish such as cichlids were negatively correlated with leaf litter benthos. Dorsal and anal fin lengths were associated with larger (higher order) systems and higher turbidity and habitat structure from woody debris. Longer head length was negatively correlated with stones, while increased head depth showed a negative correlation with leaf litter. Increased oral gape was positively correlated with increased Nitrate (NO3), NO3-N and aquatic macrophytes, and negatively correlated with substrate which suggests pursuit predators feeding on nekton in midwater habitat. Larger snout protrusion showed a strong positive correlation with catchment slope, order, NO3, turbidity and mud indicating more piscivorous fishes in larger rivers with more nutrient richness. A similar pattern occurred with body width suggesting more mobile, fusiform species that generally consume nekton were strongly positively correlated with the same environmental drivers as snout protrusion, and additionally sand and woody debris. Increased caudal peduncle length and anal fin height, which are associated with mobility, were positively correlated with large, faster-flowing lotic systems with higher salt concentrations, warmer temperatures, and sandy substrate.
3.4 Functional trait space and β-diversity
Functional richness (convex hull trait space) was higher in the lower basin (0.52) than in the upper basin (0.26) (Figure 8) with a large overlap of in trait space between the upper and lower basin in the first two axes. There was also a high β-diversity due to a high turnover (Supplementary Figure S2), with a low similarity of functional β-diversity between basins (Jaccard dissimilarity = 0.80) driven by a high functional turnover resultant (0.62) and a weak functional nestedness (0.17) suggesting that the upper and lower communities have different functional strategies (Villéger et al., 2013).
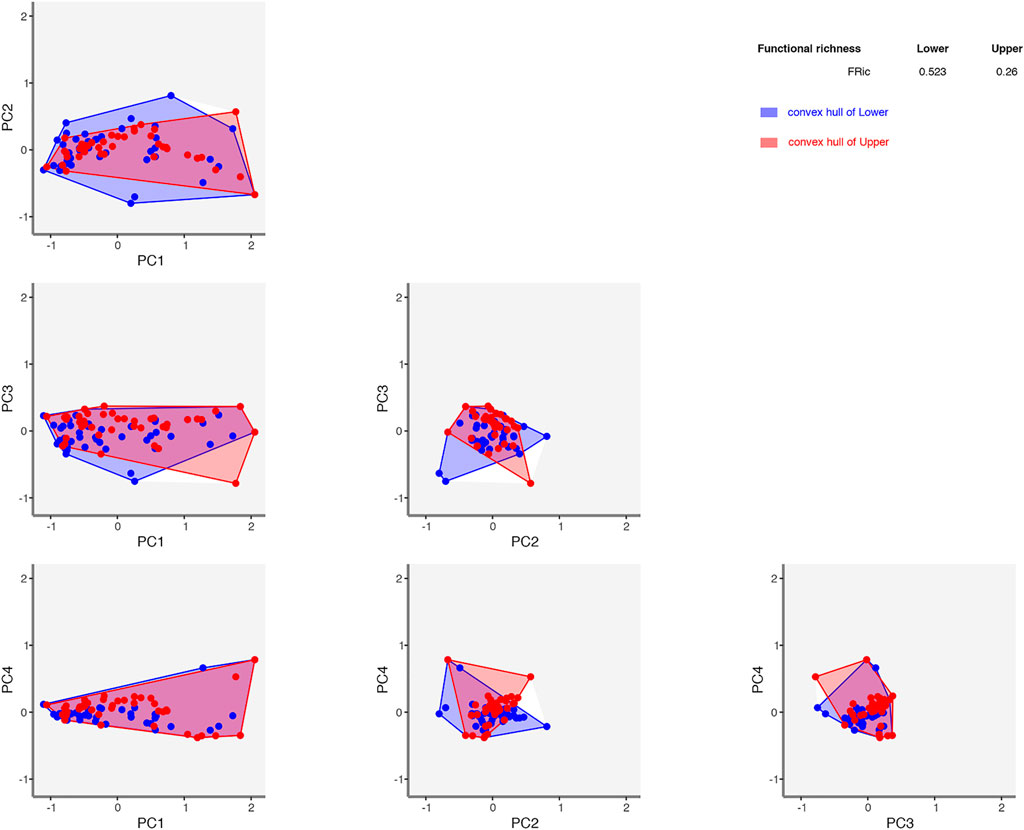
FIGURE 8. Functional richness (FRic) of upper (0.215) and lower basin (0.495) fish communities showing convex hulls and trait space per basin over 5 dimensions of space (accounting for 91% of observed variance in the data). Figure produced in mFD.
4 Discussion
4.1 Environmental variation and fish assemblage patterns
The structure of fish assemblages within the study area presented longitudinal patterns showing species turnover and increased richness from the upper basin in the Chaillu Massif to the lower coastal plains. This pattern has also been documented in large-scale regional studies of fish assemblages in the Neo- and Afrotropics (Kamden Toham and Teugels, 1998; Ibanez et al., 2007; Ibañez et al., 2009; Pease et al., 2012).
In this study, various environmental variables at different spatial scales were correlated with fish assemblage structure with distinct separation of fish assemblages between upper and lower basins. The changes in fish assemblages between basins seem to be primarily predicted by a combination of temperature, hydrology, and geomorphology (driven by average catchment slope), increased productivity (NO3-N), and habitat heterogeneity which are common patterns along fluvial gradients across climatic zones (Horwitz, 1978; Vannote et al., 1980; Ibarra and Stewart, 1989; Kamden Toham and Teugels, 1998; Pease et al., 2012). Fish species richness increased along the fluvial gradient from upper to lower basin; an expected pattern as increasing temperature/productivity, hydrological stability, and habitat structure generally support higher fish richness (Schlosser, 1987; Pease et al., 2012). These observations conform with the River Continuum Concept in that turnover of species and increase in species richness appears to be primarily driven by a combination of temperature, resource availability and habitat heterogeneity (Vannote et al., 1980).
4.2 Taxonomic spatial nestedness
Our study highlights different patterns of taxonomic β diversity at a sub-basin and basin scale. We noted a high turnover component suggesting that environmental filtering and sorting is influential at a basin scale. López-Delgado et al. (2019a) found a similar pattern for a pristine Neotropical river, which is expected due to low dispersal rates at a basin scale where assemblages are strongly influenced by species sorting as organisms are excluded from habitats that reduce their fitness (Soininen, 2014). Our results mirror other studies where sorting and environmental filtering explained lotic fish assemblages at broad scales (Heino et al., 2015a, Heino et al., 2015b; López-Delgado et al., 2019a).
Taxonomic β-diversity patterns differed based on position in the basin. The upper basin displayed a degree of nestedness implying a non-random process of species assemblage and structural patterns (Gaston and Blackburn, 2000). Caetano et al. (2021) showed that steep Neotropical stream assemblages were nested, containing a subset of fish species and traits of the fish metacommunity present in streams with gentle slopes. Slope affects fish diversity patterns by driving other abiotic features at larger scales, such as geomorphology, hydrology, dispersal, and the persistence of species (Eros et al., 2011; Mozzaquattro et al., 2020). Steep average catchment gradients together with high rainfall in the upper basin in our study system influences stream geomorphology and hydrodynamics, often on a daily basis. Headwaters are also relatively isolated within river networks and species dispersal is often limited because of this. These dynamics likely affect the ability of certain species to remain in parts of the river under such conditions, thus reducing alpha diversity and producing nestedness patterns across a steepness gradient. On the contrary, habitats in lower basin reaches are generally less isolated with increased dispersal potential (Caetano et al., 2021). These environments facilitate species sorting and filtering, which is what we found in the lower basin, which had a higher turnover component.
4.3 Functional trait-environment relationships
Functional trait-environment associations showed signals of environmental filtering on functional traits at multiple levels, where habitat and landscape templates structured trait distributions within fish assemblages (Townsend and Hildrew, 1994; Poff, 1997). At regional and global scales, environmental filters appear to have the greatest influence on community structure over other factors such as limiting similarity (Poff, 1997; Blanchet et al., 2014).
Upper basin traits showed strong reach-scale environmental filtering patterns inferring resistance and hydrodynamics. High-energy environmental conditions such as those in fast-flowing streams with increased hydraulic forces and temporally variable hydrology increase the influence of environmental filtering on community assembly (Weiher and Keddy, 1995), and similar relationships have been described for other tropical and sub-tropical areas (Oliveira et al., 2010; Bower and Piller, 2015; Bower and Winemiller, 2019b). Lower basin traits separated out along a feeding and mobility trait gradient. The increased prevalence and diversity of maneuverable deep-bodied fish, such as cichlids and anabantids, indicates filtering of species for habitats that are structurally more complex (Helfman et al., 2009). At the basin scale, the shift from traits important for resistance and hydrodynamics in the upper basin, to traits reflective of more diverse feeding strategies and maneuverability in the lower basin is consistent with the River Continuum Concept where trophic diversity is predicted to be lower in the headwaters, increasing in the lower reaches where a more diverse array of habitat types and food resources are available (Vannote et al., 1980).
Supporting the results of the traits PCA, trait-environment relationships from the fourth corner analysis for the upper basin show functional-trait associations driven by the physical habitat template and hydrology. Filtering of species with traits based on resistance and habitat strategies is consistent with predictions made by the Riverine Habitat Template (Townsend and Hildrew, 1994). Bower and Winemiller (2019b) also found that water velocity, depth and substrate complexity were most important in restricting fish occupation in certain microhabitats across geographical scales, and other studies have observed significant relationships between water velocity and fish assemblage structure (Lamouroux et al., 2002; Willis et al., 2005; Bower and Piller, 2015; Bower and Winemiller, 2019b).
Lower reaches of rivers tend to provide more diverse habitats, food resources and higher aquatic production because of their size and stable hydrology (Thorp et al., 2006). Functional-trait relationships in the lower basin were mostly driven by feeding and maneuverability which is likely driven by increased habitat diversity and increased autochthonous resources (Townsend and Hildrew, 1994). In comparison, trophic traits were not major drivers of the trait-environment associations for the upper basin. The reason for this could be that stochasticity from hydrological flashiness in headwaters may reduce fish populations below carry capacity thereby reducing resource competition (Poff, 1997).
The presence of fusiform, wide-bodied fish at steeper sites in the lower basin shows some conformance with environmental filtering concepts as such body forms are more resistant to variable hydrology and swifter flow velocities (Townsend and Hildrew, 1994). Although no strong relationships were elucidated for deep-bodied fish, diversity of the Cichlidae, Anabantidae, and Alestidae was much higher compared to the upper basin (Supplementary Figure S3). While some of this increased diversity could be linked to ancient taxonomic radiations of secondary freshwater fishes in the coastal regions, the present-day environment provides no evidence for salinity acting as a diversity filtering factor in the lower basin. Increased fin lengths are associated with woody debris allowing maneuverability in more structurally complex habitats such as those in the lower basin (Lamouroux et al., 2002; Wainwright et al., 2002; Dala-Corte et al., 2016). In the lentic microhabitats of the lower basin community there is less environmental filtering acting on body form and species with various body shapes often coexist (Winemiller, 1991). The prominent environmental-feeding trait patterns in the lower basin suggest that more specialist feeders (pelagic pursuit predators and piscivores) are present in larger river assemblages (Vannote et al., 1980; Sibbing and Nagelkerke, 2000). Similarly, fish assemblages in larger, deeper, lower-velocity streams in the Amazon Basin were dominated by nektobenthic species that feed on fish and invertebrates and require cover (Santos et al., 2019).
Although phylogenetic relationships were not explicitly tested in this study, the morphospace of the upper basin assemblage showed a regional phylogenetic pattern in functional groups. Other communities assembled through habitat filtering have shown similar phylogenetic clustering containing closely related species (Cavender-Bares et al., 2009). The upper basin pool of species also shows patterns of phylogenetic underdispersion where assemblages are strongly driven by environmental filtering and have a narrower set of traits that are suited for their environmental conditions (Weiher and Keddy, 1995; Cornwell et al., 2006). Similar signals of phylogenetic underdispersion were prevalent in structuring regional fish assemblages in West Africa, South America, and Southeast Asia, with environmental filtering as the primary driver of regional community assemblage in these regions (Bower and Winemiller, 2019b). Weak phylogenetic patterns were seen in functional traits of the lower basin morphospace, except for the Cichlidae. This could possibly indicate underdispersion in the Cichlidae, and a higher degree of resource partitioning for other species in the lower basin community. This pattern could also be attributed to the larger size of systems sampled in the lower basin, which contributed to more species being sampled locally.
4.4 Functional trait space and β-diversity
Functional richness increased along the longitudinal gradient from the upper to the lower parts of the basin. Longitudinal functional changes in fish assemblages are common and usually attributable to differences in local environments (Schlosser, 1991; Welcomme et al., 2006; Ferreira et al., 2019). The stress-dominance hypothesis (Coyle et al., 2014) posits that functional trait diversity decreases as environmental stress increases, whereas interspecific trait variation is expected to be greater in less stressful environments. The energy requirements of inhabiting high-velocity, temporally variable habitats restricts functional trait diversity and thus FRic is normally higher in more stable systems such as those in the lower basin (Webb, 1984). Relative to the lower basin, the upper basin’s fish assemblages appear to be significantly richer in terms of functional diversity per unit of upstream catchment area.
The high β-diversity and functional turnover longitudinally suggest that different functional strategies characterize fish assemblages in the upper and lower portions of the basin. High functional turnover can occur if unique species from each community are functionally different, indicating niche differentiation between communities (Villéger et al., 2013). In our study, fish assemblages were characterized either by locomotion/resistance functional strategies likely driven by higher slopes and flashier hydrology in the upper basin, or by functional strategies reflecting more trophic diversity in the lower basin. This indicates that the upper basin community is driven more by environmental filtering and ability to deal with abiotic stress, where the lower basin functionality appears to be driven by resource availability. Small-bodied insectivorous species predominated in the upper basin and were replaced by an increase in large-bodied herbivores, detritivores and piscivores in the lower basin showing an increased trophic diversity along the river gradient. This agrees with other studies were lower basin assemblages are driven more by diversity of resources whereas the upper basin assemblages seem to be more influenced by proximal environmental conditions (Dunning et al., 1992; Datry et al., 2016).
5 Conclusion
Whilst our results provide preliminary support for conceptual models such as the River Continuum Concept and the River Habitat Template in this Afrotropical system, we also found unique patterns of functional diversity. High β diversity turnover indicates that functional strategies driving communities are dependent on the position in the basin. At a sub-basin scale however, there is moderate nestedness in communities of the upper basin, and these headwater zones harbor substantial functional diversity, especially when considering upstream catchment size. Improved understanding of other mechanisms driving functional diversity in Afrotropical systems will be important for efforts to conserve freshwater systems, especially in light of increasing development and widespread loss of habitat in the region (van Rooyen et al., 2016). For instance, if the spatial distribution of fishes originates mainly from environmental filtering as is indicated in our study, then approaches to conserve fish diversity should focus on the conservation of habitat heterogeneity at suitable spatial and temporal scales (López-Delgado et al., 2019a). The distinction between the turnover and nestedness resultants is also important, as they require opposing conservation approaches (Wright and Reeves, 1992). Functionally nested communities such as those in the headwaters of our study would allow for the prioritization of a small amount of the functionally richest areas for conservation, whereas communities displaying high turnover resultants such as the lower basin would require conservation strategies that consider larger areas and connectivity (Baselga, 2010).
Data availability statement
The raw data supporting the conclusions of this article will be made available by the authors, without undue reservation.
Ethics statement
The animal study was reviewed and approved by Animal Ethics Screening Committee, University of the Witwatersrand.
Author contributions
GW conceived the ideas, collected field data, designed the methods, statistically analyzed data and wrote the first draft. JS and AP contributed to the statistical analyses. All authors contributed critically to the drafts and gave final approval for publication.
Funding
This work was supported by the University of the Witwatersrand under the University of the Witwatersrand, Johannesburg National Research Foundation Rated Researchers Incentive Grant 103581 and the Axelrod Curatorship of the American Museum of Natural History.
Acknowledgments
We gratefully acknowledge Jean de Dieu Mountou, Frédéric Avoukou, Gislain Nakoundzi Taty and the local authorities of Koutou for their invaluable assistance during our field collections, and the following colleagues: Valdie Nina Boukaka Mikembi, Victor Mamonekene (University of Marien Ngouabi, Republic of Congo), Michiel Jonker and Marco Alexandre (Ecotone Freshwater Consultants) for their contributions to the field expeditions. We thank Jonathan Levin (University of the Witwatersrand) for statistical support. Our thanks to the collections staff at the American Museum of Natural History (Chloe Lewis, Thomas Vigliotta, Radford Arrindell and Kimberly Bernotas) and to the following taxonomists: Jouke van der Zee (Royal Museum for Central Africa), Rainer Sonnenberg (Zoologisches Forschungsmuseum Alexander Koenig), Anton Lamboj (University Vienna) and Pedro H.N Bragança (South African Institute for Aquatic Biodiversity). All fishes were collected and exported with permission of the Congolese Direction Générale de l’Economie Forestière, Direction dela Faune et des Aires Protégées (permits on file at the AMNH).
Conflict of interest
Authors GW and JG are employed by Flora Fauna and Man Ecological Services Ltd.
The remaining authors declare that the research was conducted in the absence of any commercial or financial relationships that could be construed as a potential conflict of interest.
Publisher’s note
All claims expressed in this article are solely those of the authors and do not necessarily represent those of their affiliated organizations, or those of the publisher, the editors and the reviewers. Any product that may be evaluated in this article, or claim that may be made by its manufacturer, is not guaranteed or endorsed by the publisher.
Supplementary material
The Supplementary Material for this article can be found online at: https://www.frontiersin.org/articles/10.3389/fenvs.2022.981960/full#supplementary-material
References
Abernethy, K. A., Coad, L., Taylor, G., Lee, M. E., and Maisels, F. (2013). Extent and ecological consequences of hunting in Central African rainforests in the twenty-first century. Phil. Trans. R. Soc. B 368, 20120303. doi:10.1098/rstb.2012.0303
Arantes, C. C., Winemiller, K. O., Petrere, M., and Freitas, C. E. C. (2019). Spatial variation in aquatic food webs in the Amazon River floodplain. Freshw. Sci. 38, 213–228. doi:10.1086/701841
Baselga, A. (2010). Partitioning the turnover and nestedness components of beta diversity. Glob. Ecol. Biogeogr. 19, 134–143. doi:10.1111/j.1466-8238.2009.00490.x
Blanchet, S., Helmus, M. R., Brosse, S., and Grenouillet, G. (2014). Regional vs local drivers of phylogenetic and species diversity in stream fish communities. Freshw. Biol. 59, 450–462. doi:10.1111/fwb.12277
Boulton, A. J., Boyero, L., Covich, A. P., Dobson, M., Lake, S., and Pearson, R. (2008). “Are tropical streams ecologically different from temperate streams?,” in Tropical stream ecology. Editor D. Dudgeon (London: Academic Press), 273–294.
Bower, L. M., and Piller, K. R. (2015). Shaping up: A geometric morphometric approach to assemblage ecomorphology. J. Fish. Biol. 87, 691–714. doi:10.1111/jfb.12752
Bower, L. M., and Winemiller, K. O. (2019a). Fish assemblage convergence along stream environmental gradients: An intercontinental analysis. Ecography 42, 1691–1702. doi:10.1111/ecog.04690
Bower, L. M., and Winemiller, K. O. (2019b). Intercontinental trends in functional and phylogenetic structure of stream fish assemblages. Ecol. Evol. 9, 13862–13876. doi:10.1002/ece3.5823
Caetano, V., Camana, M., Dala-Corte, R. B., and Melo, A. S. (2021). Scale-sensitive stream slope drives nested fish trait-based diversity. Aquat. Ecol. 55, 1051–1063. doi:10.1007/s10452-021-09881-2
Cavender-Bares, J., Kozak, K. H., Fine, P. V. A., and Kembel, S. W. (2009). The merging of community ecology and phylogenetic biology. Ecol. Lett. 12, 693–715. doi:10.1111/j.1461-0248.2009.01314.x
Córdova-Tapia, F., Hernández-Marroquín, V., and Zambrano, L. (2018). The role of environmental filtering in the functional structure of fish communities in tropical wetlands. Ecol. Freshw. Fish. 27, 522–532. doi:10.1111/eff.12366
Cornwell, W. K., Schwilk, D. W., and Ackerly, D. D. (2006). A trait-based test for habitat filtering: Convex hull volume. Ecology 87, 1465–1471. doi:10.1890/0012-9658(2006)87[1465:ATTFHF]2.0.CO;2
Correa, S. B., and Winemiller, K. O. (2014). Niche partitioning among frugivorous fishes in response to fluctuating resources in the Amazonian floodplain forest. Ecology 95, 210–224. doi:10.1890/13-0393.1
Correa, S. B., and Winemiller, K. (2018). Terrestrial–aquatic trophic linkages support fish production in a tropical oligotrophic river. Oecologia 186, 1069–1078. doi:10.1007/s00442-018-4093-7
Coyle, J. R., Halliday, F. W., Lopez, B. E., Palmquist, K. A., Wilfahrt, P. A., and Hurlbert, A. H. (2014). Using trait and phylogenetic diversity to evaluate the generality of the stress-dominance hypothesis in eastern North American tree communities. Ecography 37, 814–826. doi:10.1111/ecog.00473
Dala-Corte, R. B., Giam, X., Olden, J. D., Becker, F. G., Guimarães, T. de F., and Melo, A. S. (2016). Revealing the pathways by which agricultural land-use affects stream fish communities in South Brazilian grasslands. Freshw. Biol. 61, 1921–1934. doi:10.1111/fwb.12825
Darwall, W. R. T., Smith, K. G., Allen, D. J., Holland, R. A., Harrison, I. J., and Brooks, E. G. E. (2011). The diversity of life in African freshwaters: Underwater, under threat: An analysis of the status and distribution of freshwater species throughout mainland Africa. Gland, Switzerland and Cambridge, United Kingdom: IUCN.
Datry, T., Melo, A. S., Moya, N., Zubieta, J., de la Barra, E., and Oberdorff, T. (2016). Metacommunity patterns across three Neotropical catchments with varying environmental harshness. Freshw. Biol. 61, 277–292. doi:10.1111/fwb.12702
di Marco, M., Chapman, S., Althor, G., Kearney, S., Besancon, C., Butt, N., et al. (2017). Changing trends and persisting biases in three decades of conservation science. Glob. Ecol. Conservation 10, 32–42. doi:10.1016/j.gecco.2017.01.008
Dray, S., Choler, P., Dolédec, S., Peres-Neto, P. R., Thuiller, W., Pavoine, S., et al. (2014). Combining the fourth-corner and the RLQ methods for assessing trait responses to environmental variation. Ecology 95, 14–21. doi:10.1890/13-0196.1
Dray, S., and Dufour, A. B. (2007). The ade4 package: Implementing the duality diagram for ecologists. J. Stat. Softw. 22, 1–20. doi:10.18637/jss.v022.i04
Dunning, J. B., Danielson, B. J., Pulliam, H. R., and Ecology, I. (1992). Ecological processes that affect populations in complex landscapes. Oikos 65, 169–175. doi:10.2307/3544901
Eros, T., Schmera, D., and Schick, R. S. (2011). Network thinking in riverscape conservation - a graph-based approach. Biol. Conserv. 144, 184–192. doi:10.1016/j.biocon.2010.08.013
Ferreira, F. C., Souza, U. P., Cetra, M., and Petrere, M. (2019). Rhithronic and potamonic fishes coexist in wadeable streams under distinct metacommunity processes. Ecol. Freshw. Fish. 28, 85–96. doi:10.1111/eff.12433
Fox, J., and Weisberg, S. (2019). An R companion to applied regression. Third edition (Thousand Oaks, CA: SAGE Publications Inc.). Available at: https://socialsciences.mcmaster.ca/jfox/Books/Companion/.
Gaston, K. J., and Blackburn, T. M. (2000). Pattern and process in macroecology. United Kingdom: Blackwell Publishing. doi:10.1002/9780470999592
Gatz, A. J. (1979). Ecological morphology of freshwater stream fishes. Tulane Stud. Zoology Bot. 21, 91–124. doi:10.2307/1936608
Greathouse, E. A., and Pringle, C. M. (2006). Does the River continuum concept apply on a tropical island? Longitudinal variation in a Puerto Rican stream. Can. J. Fish. Aquat. Sci. 63, 134–152. doi:10.1139/f05-201
Grill, G., Lehner, B., Thieme, M., Geenen, B., Tickner, D., Antonelli, F., et al. (2019). Mapping the world’s free-flowing rivers. Nature 569, 215–221. doi:10.1038/s41586-019-1111-9
Heino, J., Melo, A. S., and Bini, L. M. (2015a). Reconceptualising the beta diversity-environmental heterogeneity relationship in running water systems. Freshw. Biol. 60 (2), 223–235. doi:10.1111/fwb.12502
Heino, J., Melo, A. S., Siqueira, T., Soininen, J., Valanko, S., and Bini, L. M. (2015b). Metacommunity organisation , spatial extent and dispersal in aquatic systems : Patterns , processes and prospects. Freshw. Biol. 60 (5), 845–869. doi:10.1111/fwb.12533
Heino, J., Schmera, D., and Eros, T. (2013). A macroecological perspective of trait patterns in stream communities. Freshw. Biol. 58, 1539–1555. doi:10.1111/fwb.12164
Helfman, G., Collette, B. B., Facey, D. E., and Bowen, B. W. (2009). The diversity of fishes: Biology, evolution, and ecology. 2nd Edition. New Jersey, NY: John Wiley & Sons.
Horwitz, R. J. (1978). Temporal variability patterns and the distributional patterns of stream fishes. Ecol. Monogr. 48, 307–321. doi:10.2307/2937233
Ibañez, C., Belliard, J., Hughes, R. M., Irz, P., Kamdem-Toham, A., Lamouroux, N., et al. (2009). Convergence of temperate and tropical stream fish assemblages. Ecography 32, 658–670. doi:10.1111/j.1600-0587.2008.05591.x
Ibanez, C., Oberdorff, T., Teugels, G., Mamononekene, V., Lavoué, S., Fermon, Y., et al. (2007). Fish assemblages structure and function along environmental gradients in rivers of Gabon (Africa). Ecol. Freshw. Fish. 16, 315–334. doi:10.1111/j.1600-0633.2006.00222.x
Ibarra, M., and Stewart, D. J. (1989). Longitudinal zonation of sandy beach fishes in the napo river basin, eastern Ecuador. Copeia 2, 364–381. doi:10.2307/1445433
Kamden Toham, A., and Teugels, G. G. (1998). Diversity patterns of fish assemblages in the Lower Ntem River Basin (Cameroon), with notes on potential effects of deforestation. Fundam. Appl. Limnol. 141, 421–446. doi:10.1127/archiv-hydrobiol/141/1998/421
Kessler, M., Abrahamczyk, S., Bos, M., Buchori, D., Putra, D. D., Robbert Gradstein, S., et al. (2011). Cost-effectiveness of plant and animal biodiversity indicators in tropical forest and agroforest habitats. J. Appl. Ecol. 48, 330–339. doi:10.1111/j.1365-2664.2010.01932.x
Kleynhans, C. (2008). Fish response assessment index in river EcoClassification: Manual for EcoStatus determination (version 2). Pretoria, South Africa: Water Research Commission and Department of Water and Forestry. doi:10.13140/RG.2.1.1859.4000
Lamouroux, N., Poff, N. L. R., and Angermeier, P. L. (2002). Intercontinental convergence of stream fish community traits along geomorphic and hydraulic gradients. Ecology 83, 1792–1807. doi:10.1890/0012-9658(2002)083[1792:ICOSFC]2.0.CO;2
Leclère, D., Obersteiner, M., Barrett, M., Butchart, S. H. M., Chaudhary, A., de Palma, A., et al. (2020). Bending the curve of terrestrial biodiversity needs an integrated strategy. Nature 585, 551–556. doi:10.1038/s41586-020-2705-y
Legendre, P., Galzin, R. G., and Harmelin-Vivien, M. L. (1997). Relating behavior to habitat: Solutions to the fourth-corner problem. Ecology 78, 547–562. doi:10.2307/2266029
López-Delgado, E. O., Winemiller, K. O., and Villa-Navarro, F. A. (2019a). Do metacommunity theories explain spatial variation in fish assemblage structure in a pristine tropical river? Freshw. Biol. 64, fwb.13229–379. doi:10.1111/fwb.13229
López-Delgado, E. O., Winemiller, K. O., and Villa-Navarro, F. A. (2019b). Local environmental factors influence beta‐diversity patterns of tropical fish assemblages more than spatial factors. Ecology 101 (2), e02940. doi:10.1002/ecy.2940
Magneville, C., Loiseau, N., Albouy, C., Casajus, N., Claverie, T., Escalas, A., et al. (2022). mFD: an R package to compute and illustrate the multiple facets of functional diversity. Ecography 2022, 1–15. doi:10.1111/ecog.05904
Malhi, Y., Adu-Bredu, S., Asare, R. A., Lewis, S. L., and Mayaux, P. (2013). African rainforests: Past, present and future. Phil. Trans. R. Soc. B 368, 20120312. doi:10.1098/rstb.2012.0312
Mamonekene, V., and Stiassny, M. L. J. (2012). Fishes of the du Chaillu Massif, niari depression, and mayombe Massif (republic of Congo, west-central Africa): A list of species collected in tributaries of the upper ogowe and middle and upper Kouilou-Niari River basins. Check List. 8, 1172–1183. doi:10.15560/8.6.1172
McGill, B. J., Enquist, B. J., Weiher, E., and Westoby, M. (2006). Rebuilding community ecology from functional traits. Trends Ecol. Evol. 21, 178–185. doi:10.1016/j.tree.2006.02.002
Megevand, C., Mosnier, A., Hourticq, J., Sanders, K., Doetinchem, N., and Streck, C. (2013). Deforestation trends in the Congo basin. Washington D.C: World Bank Group. doi:10.1596/978-0-8213-9742-8
Minshall, G. W. (2007). Stream ecosystem theory : A global perspective. J. North Am. Benthol. Soc. 7, 263–288. doi:10.2307/1467294
Mouchet, M. A., Burns, M. D. M., Garcia, A. M., Vieira, J. P., and Mouillot, D. (2013). Invariant scaling relationship between functional dissimilarity and co-occurrence in fish assemblages of the Patos Lagoon estuary (Brazil): Environmental filtering consistently overshadows competitive exclusion. Oikos 122, 247–257. doi:10.1111/j.1600-0706.2012.20411.x
Mouillot, D., Dumay, O., and Tomasini, J. A. (2007). Limiting similarity, niche filtering and functional diversity in coastal lagoon fish communities. Estuar. Coast. Shelf Sci. 71, 443–456. doi:10.1016/j.ecss.2006.08.022
Mozzaquattro, L. B., Dala-Corte, R. B., Becker, F. G., and Melo, A. S. (2020). Effects of spatial distance, physical barriers, and habitat on a stream fish metacommunity. Hydrobiologia 847, 3039–3054. doi:10.1007/s10750-020-04309-8
Newbold, J. D., O’Neill, R. V., Elwood, J. W., and van Winkle, W. (1982). Nutrient spiralling in streams : Implications for nutrient limitation and invertebrate activity. Am. Nat. 120, 628–652. doi:10.1086/284017
Nock, C. A., Vogt, R. J., and Beisner, B. E. (2016). Functional traits. In: eLS. Chichester: John Wiley & Sons, Ltd, 1–8. doi:10.1002/9780470015902.a0026282
Olden, J. D., and Kennard, M. J. (2010). Intercontinental comparison of fish life history strategies along a gradient of hydrologic variability. Am. Fish. Soc. Symposium 73, 83–107. Available at: http://depts.washington.edu/oldenlab/wordpress/wp-content/uploads/2013/03/AFS_Chapter_2010.pdf.
Oliveira, E. F., Goulart, E., Breda, L., Minte-vera, C. V., Ricardo, L., Paiva, D. S., et al. (2010). Ecomorphological patterns of the fish assemblage in a tropical floodplain : Effects of trophic , spatial and phylogenetic structures. Neotrop. Ichthyol. 8, 569–586. doi:10.1590/S1679-62252010000300002
Pease, A. A., González-Díaz, A. A., Rodiles-Hernández, R., and Winemiller, K. O. (2012). Functional diversity and trait-environment relationships of stream fish assemblages in a large tropical catchment. Freshw. Biol. 57, 1060–1075. doi:10.1111/j.1365-2427.2012.02768.x
Pease, A. A., Taylor, J. M., Winemiller, K. O., and King, R. S. (2015). Ecoregional, catchment, and reach-scale environmental factors shape functional-trait structure of stream fish assemblages. Hydrobiologia 753, 265–283. doi:10.1007/s10750-015-2235-z
Petrozzi, F., Amori, G., Franco, D., Gaubert, P., Pacini, N., Eniang, E. A., et al. (2016). Ecology of the bushmeat trade in west and central Africa. Trop. Ecol. 57, 545–557.
Poff, N. L. (1997). Landscape filters and species traits: Towards mechanistic understanding and prediction in stream ecology. J. North Am. Benthol. Soc. 16, 391–409. doi:10.2307/1468026
Poff, N. L. R., Olden, J. D., Vieira, N. K. M., Finn, D. S., Simmons, M. P., and Kondratieff, B. C. (2006). Functional trait niches of North American lotic insects: Traits-based ecological applications in light of phylogenetic relationships. J. North Am. Benthol. Soc. 25, 730–755. doi:10.1899/0887-3593(2006)025[0730:FTNONA]2.0.CO;2
Qian, H., Ricklefs, R. E., and White, P. S. (2005). Beta diversity of angiosperms in temperate floras of eastern Asia and eastern North America. Ecol. Lett. 8, 15–22. doi:10.1111/j.1461-0248.2004.00682.x
Reid, A. J., Carlson, A. K., Creed, I. F., Eliason, E. J., Gell, P. A., Johnson, P. T. J., et al. (2018). Emerging threats and persistent conservation challenges for freshwater biodiversity. Biol. Rev. 94, 849–873. doi:10.1111/brv.12480
RStudio Team (2021). RStudio: Integrated development environment for R. RStudio. Boston, MA: PBC Title. Available at: http://www.rstudio.com.
Rudel, T. K. (2013). The national determinants of deforestation in sub-Saharan Africa. Phil. Trans. R. Soc. B 368, 20120405. doi:10.1098/rstb.2012.0405
Santos, L. L., Benone, N. L., Soares, B. E., Barthem, R. B., and Montag, L. F. A. (2019). Trait–environment relationships in Amazon stream fish assemblages. Ecol. Freshw. Fish. 1, 424–433. doi:10.1111/eff.12465
Schlosser, I. J. (1987). “A conceptual framework for fish communities in small warmwater streams,” in Community and evolutionary ecology in North American stream fishes. Editors W. J. Matthews, and D. C. Heins ( Norman, OK: University of Oklahoma Press), 17–24.
Schlosser, I. J. (1991). Stream fish ecology: A landscape perspective. BioScience 14, 704–712. doi:10.2307/1311765
Sibbing, F. A., and Nagelkerke, L. A. J. (2000). Resource partitioning by Lake Tana barbs predicted from fish morphometrics and prey characteristics. Rev. Fish. Biol. Fish. 10, 393–437. doi:10.1023/A:1012270422092
Smith, A. B., Sandel, B., Kraft, N. J. B., and Carey, S. (2013). Characterizing scale-dependent community assembly using the functional-diversity-area relationship. Ecology 94, 2392–2402. doi:10.1890/12-2109.1
Soininen, J. (2014). A quantitative analysis of species sorting across organisms and ecosystems. Ecology 95, 3284–3292. doi:10.1890/13-2228.1
Sternberg, D., and Kennard, M. J. (2013). Environmental, spatial and phylogenetic determinants of fish life-history traits and functional composition of Australian rivers. Freshw. Biol. 58, 1767–1778. doi:10.1111/fwb.12166
Strona, G., and Fattorini, S. (2014). On the methods to assess significance in nestedness analyses. Theory Biosci. 133, 179–186. doi:10.1007/s12064-014-0203-1
Teresa, F. B., and Casatti, L. (2012). Influence of forest cover and mesohabitat types on functional and taxonomic diversity of fish communities in Neotropical lowland streams. Ecol. Freshw. Fish. 21, 433–442. doi:10.1111/j.1600-0633.2012.00562.x
Thorp, J. H., Thoms, M. C., and Delong, M. D. (2006). The riverine ecosystem synthesis: Biocomplexity in river networks across space and time. River Res. Appl. 22, 123–147. doi:10.1002/rra.901
Tickner, D., Opperman, J. J., Abell, R., Acreman, M., Arthington, A. H., Bunn, S. E., et al. (2020). Bending the curve of global freshwater biodiversity loss: An emergency recovery plan. BioScience 70, 330–342. doi:10.1093/biosci/biaa002
Titley, M. A., Snaddon, J. L., and Turner, E. C. (2017). Scientific research on animal biodiversity is systematically biased towards vertebrates and temperate regions. PLoS ONE 12, e0189577–14. doi:10.1371/journal.pone.0189577
Toussaint, A., Charpin, N., Brosse, S., and Villéger, S. (2016). Global functional diversity of freshwater fish is concentrated in the Neotropics while functional vulnerability is widespread. Sci. Rep. 6, 22125. doi:10.1038/srep22125
Townsend, C. R., and Hildrew, A. G. (1994). Species traits in relation to a habitat templet for river systems. Freshw. Biol. 31, 265–275. doi:10.1111/j.1365-2427.1994.tb01740.x
Townsend, C. R. (1989). The patch dynamics concept of stream community ecology. J. North Am. Benthol. Soc. 8, 36–50. doi:10.2307/1467400
Troia, M. J., and Gido, K. B. (2015). Functional strategies drive community assembly of stream fishes along environmental gradients and across spatial scales. Oecologia 177, 545–559. doi:10.1007/s00442-014-3178-1
Ulrich, W., and Almeida-Neto, M. (2012). On the meanings of nestedness: Back to the basics. Ecography 35, 865–871. doi:10.1111/j.1600-0587.2012.07671.x
van der Laan, R., Eschmeyer, W. N., and Fricke, R. (2014). Family-group names of Recent fishes. Zootaxa 3882, 1–230. doi:10.11646/zootaxa.3882.1.1
van Rooyen, M. W., van Rooyen, N., Miabangana, E. S., Nsongola, G., Vasicek Gaugris, C., and Gaugris, J. Y. (2019). Floristic composition, diversity and structure of the rainforest in the mayoko district, republic of Congo. Open J. For. 9, 16–69. doi:10.4236/ojf.2019.91002
van Rooyen, M. W., van Rooyen, N., Orban, B., Nsongola, G., Miabangana, E. S., and Gaugris, J. (2016). Floristic composition, diversity and structure of the forest communities in the Kouilou département, Republic of Congo. Trop. Ecol. 57, 805–824.
Vannote, R. L., Minshall, G. W., Cummins, K. W., Sedell, J. R., and Cushing, C. E. (1980). the River continuum concept. Can. J. Fish. Aquat. Sci. 37, 130–137. doi:10.1139/f80-017
Villéger, S., Brosse, S., Mouchet, M., Mouillot, D., and Vanni, M. J. (2017). Functional ecology of fish: Current approaches and future challenges. Aquat. Sci. 79, 783–801. doi:10.1007/s00027-017-0546-z
Villéger, S., Grenouillet, G., and Brosse, S. (2013). Decomposing functional β-diversity reveals that low functional β-diversity is driven by low functional turnover in European fish assemblages. Glob. Ecol. Biogeogr. 22, 671–681. doi:10.1111/geb.12021
Villéger, S., Mason, N. W. H., and Mouillot, D. (2008). New multidimensional functional diversity indices for a multifaceted framework in functional ecology. Ecology 89, 2290–2301. doi:10.1890/07-1206.1
Wainwright, P. C., Bellwood, D. R., and Westneat, M. W. (2002). Ecomorphology of locomotion in labrid fishes. Environ. Biol. Fishes 65, 47–62. doi:10.1023/A:1019671131001
Walsh, G., Alexandre, M. P., Boukaka Mikembi, V. N., Jonker, M. N., Mamonekene, V., Henri, A. J., et al. (2022). Fishes of the Loémé river system, Republic of the Congo, lower Guinea, west‐central Africa. Afr. J. Ecol., 1–9. doi:10.1111/aje.13016
Walsh, G., Jonker, M. N., and Mamonekene, V. (2014). A collection of fishes from tributaries of the lower Kouilou, Noumbi and smaller coastal basin systems, Republic of the Congo, Lower Guinea, west-central Africa. Check List. 10, 900–912. doi:10.15560/10.4.900
Ward, J. V., Robinson, C. T., and Tockner, K. (2002). Applicability of ecological theory to riverine ecosystems. SIL Proc. 1922-2010 28, 443–450. doi:10.1080/03680770.2001.11902621
Webb, P. W. (1984). Body form , locomotion and foraging in aquatic vertebrates. Am. Zool. 24, 107–120. doi:10.1093/icb/24.1.107
Weiher, E., and Keddy, P. A. (1995). Assembly rules , null models , and trait dispersion : New questions from old patterns. Oikos 74, 159–164. doi:10.2307/3545686
Welcomme, R. L., Winemiller, K. O., and Cowx, I. G. (2006). Fish environmental guilds as a tool for assessment of ecological condition of rivers. River Res. Appl. 22, 377–396. doi:10.1002/rra.914
Willis, S. C., Winemiller, K. O., and Lopez-Fernandez, H. (2005). Habitat structural complexity and morphological diversity of fish assemblages in a Neotropical floodplain river. Oecologia 142, 284–295. doi:10.1007/s00442-004-1723-z
Winemiller, K. O. (1991). Ecomorphological diversification in lowland freshwater fish assemblages from five biotic regions. Ecol. Monogr. 61, 343–365. doi:10.2307/2937046
Winemiller, K. O., Flecker, A. S., and Hoeinghaus, D. J. (2010). Patch dynamics and environmental heterogeneity in lotic ecosystems. J. North Am. Benthol. Soc. 29, 84–99. doi:10.1899/08-048.1
Winemiller, K. O., and Rose, K. A. (1992). Patterns of life-history diversification in North American fishes: Implications for population regulation. Can. J. Fish. Aquat. Sci. 49, 2196–2218. doi:10.1139/f92-242
Keywords: functional traits, ecomorphology, freshwater, forest, environmental filtering, river continuum, gradient
Citation: Walsh G, Pease AA, Woodford DJ, Stiassny MLJ, Gaugris JY and South J (2022) Functional diversity of afrotropical fish communities across river gradients in the Republic of Congo, west central Africa. Front. Environ. Sci. 10:981960. doi: 10.3389/fenvs.2022.981960
Received: 29 June 2022; Accepted: 02 August 2022;
Published: 06 September 2022.
Edited by:
Carla Simone Pavanelli, State University of Maringá, BrazilReviewed by:
Fabio Di Dario, Federal University of Rio de Janeiro, BrazilLucia Rapp Py-Daniel, National Institute of Amazonian Research (INPA), Brazil
Copyright © 2022 Walsh, Pease, Woodford, Stiassny, Gaugris and South. This is an open-access article distributed under the terms of the Creative Commons Attribution License (CC BY). The use, distribution or reproduction in other forums is permitted, provided the original author(s) and the copyright owner(s) are credited and that the original publication in this journal is cited, in accordance with accepted academic practice. No use, distribution or reproduction is permitted which does not comply with these terms.
*Correspondence: Gina Walsh, Zy53YWxzaEBmbG9yYWZhdW5hbWFuLmNvbQ==