The role of zinc to mitigate heavy metals toxicity in crops
- 1Research Center on Ecological Sciences, Jiangxi Agricultural University, Nanchang, China
- 2Department of Agricultural Engineering, Khwaja Fareed University of Engineering and Information Technology, Rahim Yar Khan, Punjab, Pakistan
- 3Department of Agronomy, University of Agriculture Faisalabad, Faisalabad, Pakistan
- 4Department of Botany, Division of Science and Technology, University of Education, Lahore, Pakistan
- 5Cereal Crops section, Agricultural Research Institute, Dera Ismail Khan, Pakistan
- 6College of Plant Science and Technology, Huazhong Agricultural University, Wuhan, China
- 7Key Laboratory of Crops Physiology, Ecology and Genetic Breeding, Ministry of Education/College of Agronomy, Jiangxi Agricultural University, Nanchang, China
- 8Smart-Health Initiative and Red Sea Research Center, Division of Biological and Environmental Sciences and Engineering, King Abdullah University of Science and Technology, Thuwal, Saudi Arabia
- 9Agricultural Botany Department, Faculty of Agriculture (Saba Basha), Alexandria University, Alexandria, Egypt
- 10Bioinformatics Department, Genetic Engineering and Biotechnology Research Institute, University of Sadat City, Sadat City, Egypt
- 11Department of Biology, Al-Jumum University College, Umm Al-Qura University, Makkah, Saudi Arabia
Heavy metal (HM) contamination is a serious concern across the globe, and in recent times, HMs’ intensity has significantly increased, posing a serious threat to crop growth and productivity. Heavy metals pose serious health issues in humans by entering the human food chains. Therefore, it is direly needed to reduce the effects of HMs on plants and humans by adapting appropriate practices. In this context, application of micronutrients can be an essential practice to mitigate the toxic effects of HMs. Zinc (Zn) is a crucial nutrient needed for plant growth, and Zn application reduced the HM-induced toxicity in plants. This review highlights Zn’s role in mitigating the HMs toxicity in plants. We have systematically described the potential mechanisms mediated by Zn to mitigate HMs in plants. Zinc application reduced the HMs uptake and translocation plants, which is considered an essential mechanism of HM stress tolerance. Zn application also improves membrane stability, plant water relationship, nutrient uptake, photosynthetic performance, osmolytes accumulation, anti-oxidant activities, and gene expression. In addition to this, the Zn application substantially improves photosynthesis by enhancing the synthesis of photosynthetic pigments, photosystem activities, enzymatic activities, and maintaining photosynthetic apparatus structure, ensuring better growth under HM stress. Therefore, Zn nutrition could improve the plant performance under HM stress by modulating the plant’s physiological and biochemical functioning, anti-oxidant activities, osmolytes accumulation, and gene expression.
Introduction
Abiotic stresses, including cold, heat, drought, heavy metals, and salinity stress, are significant threats to crop growth and productivity (Kim et al., 2021). Heavy metal (HM) stress is severe stress that negatively affects crop production and human health. Its intensity is continuously soaring due to anthropogenic activities (Umer Chattha et al., 2021; Zainab et al., 2021; Alghrably et al., 2019). Heavy metals are naturally occurring elements with atomic weight and density at least times greater than water (Tchounwou et al., 2012). Different activities, including mining, smelting, application of sewage sludge, fertilizers, and pesticides, are significant sources of HM entry into soil, contaminating Earth (Li et al., 2014; Mapodzeke et al., 2021). Moreover, foundries and leaching of HMs from diverse sources, including landfills, livestock excretion, chicken manure, runoffs, automobiles, and road works, are also significant sources of HMs entry into the environment (Briffa et al., 2020). Heavy metals such as cadmium (Cd), chromium (Cr), mercury (Hg), lead (Pb), arsenic (As), and nickel (Ni) coexist in contaminated soils. Their mobility and bioavailability are a significant concern globally owing to plant uptake and increased human exposure to these HMs by eating contaminated foods (Mapodzeke et al., 2021). Recently, the risks of HMs have significantly increased, and it has created turmoil in the agriculture sector by HMs accumulation in soils and their uptake by plants (Xiao et al., 2017).
HM pollution is a severe problem, and it needs an urgent and practical solution to reduce its hazards to soils and crops (Alengebawy et al., 2021), owing to the fact that these HMs disturb plant’s physiological and biochemical processes and deteriorate soil health and cause adverse impacts on plant growth (Hasanuzzaman et al., 2019; Abdel Salam et al., 2022; Poulson et al., 2021). Moreover, HMs also inhibits plant growth and biomass production, cause chlorosis, water and nutrients imbalance, protein and enzymes denaturation, and produce reactive oxygen species (ROS), which can cause plant death (Raza, 2020; Sultan et al., 2021; Batool et al., 2022). An apparent effect of HM stress is the production of ROS, which causes damage to plant DNA, lipids, and protein. These effects result in a substantial reduction in growth and lead to plant death (Singh et al., 2016; Hasanuzzaman et al., 2020a; Sanjosé et al., 2021). However, plants possess an excellent enzymatic and non-enzymatic anti-oxidant system to counter the effects of these HMs (Hasanuzzaman et al., 2019). These HMs also cause threats to human health by entering the human food chain, a primary global concern (Feng et al., 2021).
Therefore, serious measures must be taken to reduce the impacts of HMs on plants and soils and reduce their health hazards. Developing appropriate technologies and management practices can help reduce the HMs’ uptake by plants in contaminated soils. Using chemical approaches, particularly micronutrients, has become a practical approach globally to mitigate the toxic effects of HMs (Farooq et al., 2020). Zinc (Zn) is a vital micronutrient needed for plant growth (Chattha et al., 2017). Zinc can also significantly increase the tolerance against HMs by increasing the accumulation of potential osmolytes and reducing ROS production (Faran et al., 2019) and HMs uptake and their translocation (Hart et al., 2002; Eckhoff, 2010; Javed et al., 2016; Qaswar et al., 2017; Sharfalddin et al., 2021; Abdelrahman et al., 2021). Zn also maintains membrane integrity and restricts the formation of ROS that cause damage to plant cells (Cakmak, 2000). Moreover, Zn also increases the accumulation of osmolytes (proline) and anti-oxidant activities (APX, ascorbate peroxidase; CAT, catalase; POD, peroxidase; and SOD, superoxide dismutase) and restricts HM uptake. Zinc reduces the HM translocation within plant body, resultantly increasing the plant tolerance against HMs (Rizwan et al., 2019; Li et al., 2020). In addition, Zn triggers the anti-oxidant system and expression of stress-responsive genes, reducing HM-induced oxidative stress in plants (Thounaojam et al., 2014; Zhou et al., 2020).
The excessive concentration of Zn in the plant causes chlorosis and inhibits biomass production, cell division, nutrient uptake, and enzymes, resulting in significant yield loss (Ul-Hassan et al., 2017). However, the toxicity of Zn depends on many factors, including exposure time, plant species, and growth medium. Thus, appropriate studies are needed to optimize the Zn application dose for attaining tolerance against HMs. Therefore, in this review, we have discussed the potential of Zn to mitigate HM stress in plants. We have systematically presented information on mechanisms for Zn-induced HM tolerance in plants. We have also shed light on strategies associated with increasing the Zn accumulation in plants and future research directions to make it an essential practice against HMs.
Heavy metals essentially toxicity
HMs can be classified into non-essential and toxic metals (Cd, Pb, Hg, Cr, As, and Ag) and essential micronutrients (Co, cobalt; Cu, copper; Fe, iron; Mo, molybdenum; and Zn, zinc), which are needed for growth and development (Kalaivanan and Ganeshamurthy, 2016). The essential metals are involved in many physiological and biological processes and enzymatic activities (Bhat et al., 2019). However, deficiency of crucial nutrients causes a substantial growth reduction and leads to plant death under extreme deficiency. Moreover, both essential and non-essential metals at higher concentrations cause a decrease in growth and development by disrupting plant’s function and altering the structure (Ali et al., 2011). The high concentration of HMs also varies the uptake, accretion, and translocation of essential nutrients that cause significant growth and yield loss (Zia-Ur-Rehman et al., 2015). In addition, toxic metals also inhibit growth and photosynthesis. HMs cause chlorosis, disturbing water and nutrient relationship, which resultantly cause death of plants (Ali et al., 2011; Imran K. et al., 2021).
Plant’s responses to heavy metals
HM contamination of agricultural soils is a major concern globally, owing to their unfavorable ecological impacts. The soils used for crop production have been polluted with excess of HMs in different parts of the world, posing a serious threat to crops, humans, and food security (Bhat et al., 2019). Soil polluted with HMs adversely affect plant growth (Table 1), different metabolisms, soil microbial activities, soil fertility and productivity, and health of humans and animals (Foucault et al., 2013; Imran M. et al., 2021). These toxic metals substantially reduce germination and plant growth (Figure 1) and induce several morphological modifications in a plant (Aamer et al., 2018; Ghori et al., 2019). For example, increase in Hg concentration (5–80 mg/L) substantially decreased root and shoot growth and leaves per plant in Jatropha curcas (Marrugo-Negrete et al., 2016). Nickel toxicity significantly reduced seed germination in sunflower, owing to reduction in activities of α amylase and protease (Ashraf et al., 2011). Furthermore, Cd stress adversely affected the leaf area and caused a decrease in root growth and increased root dieback (Rucińska-Sobkowiak, 2016; Aamer et al., 2018), disrupting the structural integrity of membranes which induced undesirable effects including reduced water and nutrient supply (Janicka-Russak et al., 2012).
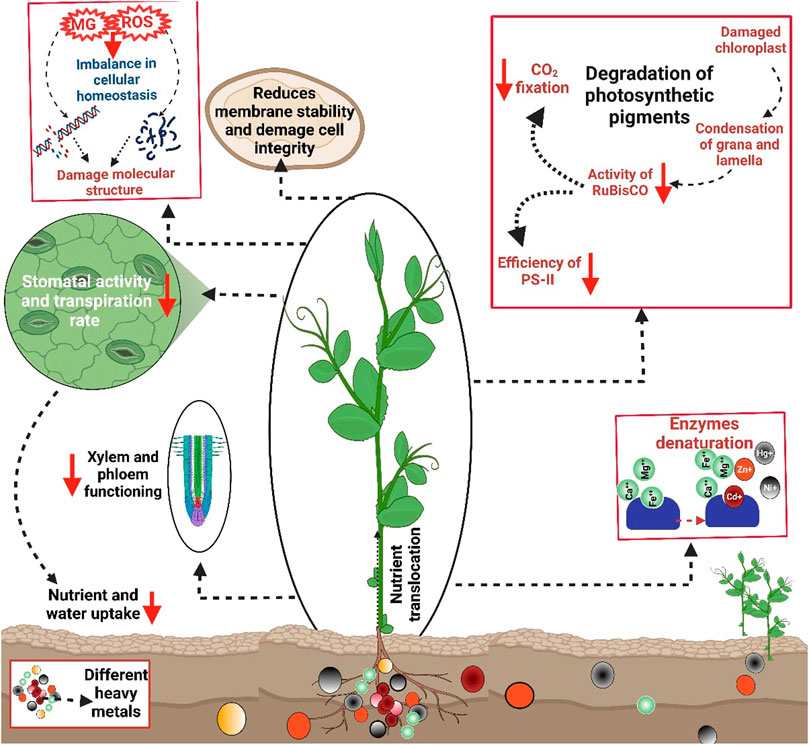
FIGURE 1. Effect of HMs toxicity on plants. The metals toxicity disturbs plant water relations, membrane stability, reduced nutrient water uptake, stomata functioning, xylem and phloem functioning, CO2 fixation, efficiency of PS II, RuBisCO activities, damage chloroplast, and condense grana and lamella, thereby causing significant reduction in growth and development.
HMs toxicity also induces deleterious impacts on plant physiological characteristics, including (Figure 1) chlorophyll contents, photosynthetic rate (Pn), transpiration rate (Tr), and conductance of stomata (gs) and intercellular CO2 concentrations (Ci). For example, Cd and Pb stress significantly inhibited the synthesis of chlorophyll contents in Davidia involucrate (Yang et al., 2020). HMs also damage photosynthetic apparatus, disrupt chlorophyll synthesis and Pn, and significantly reduce assimilate production (Schmidt et al., 2020). Metal toxicity also decreased the leaf water potential of B. oleracea plants grown in Cd-contaminated soils (Shah et al., 2020). Moreover, Cd toxicity, combined with Fe deficiency, affects electron transportation and causes a reduction in CO2 fixation and the efficiency of PS II and Chl synthesis (Le¡ ková et al., 2017). HMs also decrease CO2 fixation by down-regulating the activity of RuBisCO due to damaged chloroplast and condensation of grana and lamella under Cd stress (Guo et al., 2016; Paunov et al., 2018).
HMs also decrease nutrient and water uptake due to altered stomata activity and transpiration rate, causing a significant reduction in growth ((Hussain et al., 2013; Wang et al., 2017). Moreover, HMs reduce transpiration rate (Chandra and Kang, 2016), reduce stomatal activity, and cause significant growth loss (Tavallali, 2017). The functionality of the xylem and phloem is also affected due to HMs. For instance, Cd toxicity causes a significant reduction in the root xylem area of Salix caprea (Vaculík et al., 2012). HMs also competes with nutrient and their translocation within the plant body (Rucińska-Sobkowiak, 2016). Likewise, Cd competes with calcium (Ca), Fe, and magnesium (Mg) and cause a significant reduction in growth and biomass production (Raza, 2020). Toxic metals compete with essential nutrients at enzyme-binding sites, render them denatured, and inhibit their activities (Ghori et al., 2019). The response of Cr stress was studied on Vigna radiata and noted that Cr stress reduced chlorophyll (Chl), protein, and starch contents (Rath et al., 2019).
Pb stress caused a significant reduction in Pn and membrane stability (Figure 1) and increased osmotic adjustment with increased proline accumulation in ornamental plants (Song et al., 2020). Cu stress damaged plant root and shoot growth due to an increase in electrolyte leakage, malondialdehyde (MDA), and hydrogen peroxide (H2O2) accumulation in kenaf plants. Significant increase in anti-oxidant activities was observed with increasing Cu concentration in gthe rowing medium (Saleem et al., 2020). Fe toxicity in cowpea caused a considerable increase in MDA contents (Ifie et al., 2020). In contrast, in other studies, Cu stress showed a significant increase in MDA contents in Sesuvium portulacastrum (Lokhande et al., 2020). Nitrogen metabolism is critical in plant growth and development under HM stress. HMs significantly reduced nitrate and ammonia assimilation enzymes in Cyamopsis tetragonoloba by increasing their destruction due to increased protease activity (Sangwan et al., 2014). HMs also inhibit meristem mitosis and reduce root elongation, which causes a reduction in nutrient and water uptake (Pavlova, 2017). In another study, it was noted that Cr inhibits the mitotic cell divisions by delaying and extending the cell cycle in rice crop (Sundaramoorthy et al., 2010). Plants possess excellent enzymatic and non-enzymatic anti-oxidant oxidants to prevent the deleterious impacts of oxidative stress (Gill and Tuteja, 2010; Kaur et al., 2019). Plant cells’ anti-oxidant defense system and ROS accumulation uphold a steady-state balance. Therefore, maintaining optimum ROS levels in the cell enables proper redox reactions and regulation of diverse processes needed for plant growth and development (Mittler, 2017). This optimum level can be maintained by balance amid ROS production and ROS scavenging (Hasanuzzaman et al., 2019). Nonetheless, during stress, overproduction of ROS disturbs this balance and causes cellular damage and cell death (Raja et al., 2017). Despite their deleterious impacts, ROS are also known as signaling molecules. ROS transport the signals to redox reactions using the mitogen-activated protein kinase (MAPK) pathway in different cellular mechanisms for increasing plant tolerance against various stresses (Singh et al., 2016).
Methylglyoxal (MG) possesses high cytotoxicity and generates as a result of an imbalance in anti-oxidant cell homeostasis and resultantly leads to oxidation of protein, DNA, and leakage of ions (Syed et al., 2016). The anti-oxidants work to search, neutralize, and remove the ROS (Hasanuzzaman et al., 2020b), and their activities also increase under stress conditions. For instance, alfalfa and spinach plants exposed to Cu and Cd stress increased APX, CAT, and SOD activities (Cota-Ruiz et al., 2020). Populus trichocarpa metal tolerance protein (PtrMTP) gene plays a crucial role in managing HMs through different mechanisms, including homeostasis and detoxification (Gao et al., 2020). Plants also produce diverse binding structures, chelates, and ligands as defense mechanisms against HMs (Raza, 2020). MicroRNAs play an appreciable role in metal tolerance by regulating plant’s anti-oxidant responses, chelations, and auxin and cytokinin signaling (Ding et al., 2020). For example, Casarrubia et al. (2020) noted that microRNA in combination with mycorrhizal plays a significant role in Vaccinium myrtillus in response to Cd stress. MicroRNA expression in the perspective of biosynthesis of secondary metabolites significantly improved the aluminum (Al) and Cd tolerance in tobacco (Cedillo-Jimenez et al., 2020). The quality attributes of the rice crop, including moisture protein content, brown rice rate, and milling degree, were significantly decreased. In contrast, kernel chalkiness increased dramatically in response to Cd (100 mg/kg) stress (Imran K. et al., 2021).
Zinc: A multifaceted nutrient to alleviate heavy metal toxicity
Zinc plays a significant role in the tolerance of HMs, and the beneficial role of Zn in the detoxification of HMs. Zn is linked with changes in plant responses and soil processes. Zn appears to be a suitable choice to reduce the HMs toxicity, which improves the plant tolerance against HMs (Khan et al., 2019; Wu C. et al., 2020). Zn reduces metal accumulation by restricting its uptake and transportation within the plant body (Hart et al., 2002). Zn application improves plant growth by reducing oxidative stress (Wu C. et al., 2020). Zn also improves photosynthetic efficiency (Liu et al., 2015; Zhou et al., 2019), nutrient uptake, and osmolytes accumulation (Rizwan et al., 2017) under HMs. The relationships between Zn and HMs significantly affect plant metal uptake and accumulation (Venkatachalam et al., 2017). The foliar application of Zn mitigates Cr uptake and improves the growth and Zn bio-fortification of Oryza sativa and S. oleracea ((Hussain et al., 2018a; Zaheer et al., 2019).
Zinc application maintains membrane stability and improves plant water relationships under heavy metal stress
The most common effect of HMs is the production of ROS that damages cell membranes and causes electrolyte leakage (Srivastava et al., 2014; Noman et al., 2018). Higher lipid peroxidation under HM stress increases the MDA contents (Medina et al., 2017). Exogenous Zn supplementation decreases MDA and H2O2 contents (Table 2) and protects the membrane integrity under metal stress (Zhou et al., 2020). The reduction in MDA and H2O2 (Figure 3) accumulation following Zn application (Figure 2) is linked with the increase in membrane stability. Zn decrease metal accumulation and increases anti-oxidant activities (Hussain et al., 2018a; Rizwan et al., 2019). Zaheer et al. (2019) noted that Zn chelated with amino acids significantly reduced electrolyte leakage (EL), MDA, and H2O2 accumulation. Zn reduced the ROS production through enhanced anti-oxidant activities (APX, CAT, POD, and SOD). The application of Zn also improved the plant water uptake and relative water content (RWC) in plants grown under metal stress. For instance, Zaheer et al. (2022) reported that the combined application of Zn (10 mg/L) and Fe (5 mg/L) chelated with amino acids significantly improved water use efficiency by 31% under Cr stress. Leaf RWC was decreased by 37% and 48% under As stress (10 and 20 μM). Zn application in from of nanoparticles (50 mg/L) appreciably improved the leaf RWC by 17% and 26% under As stress (10 and 20 μM). In contrast, Zn application (100 mg/L) increased the RWC by almost 8% and 28% under As stress (10 and 20 μM) (Ahmad et al., 2020).
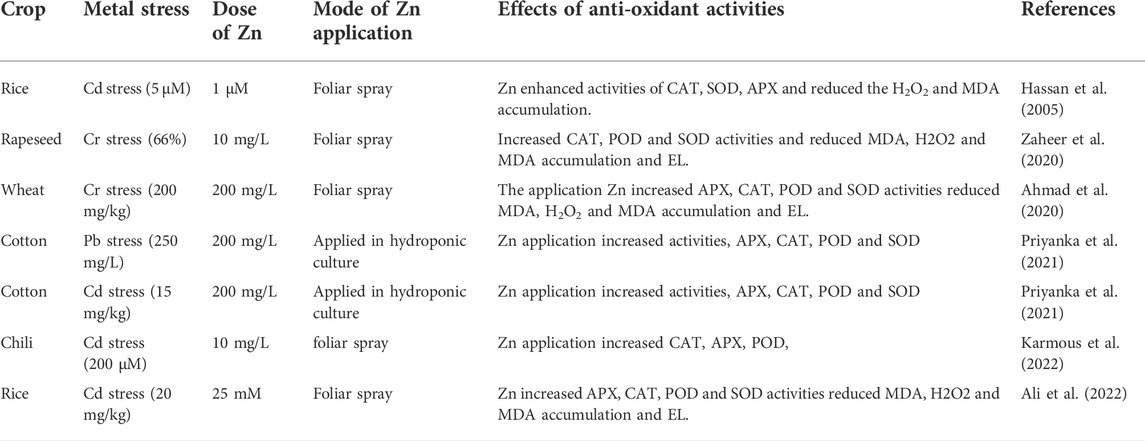
TABLE 2. Effect of Zn on anti-oxidant activities and oxidative stress indicators of different crops under metal stress
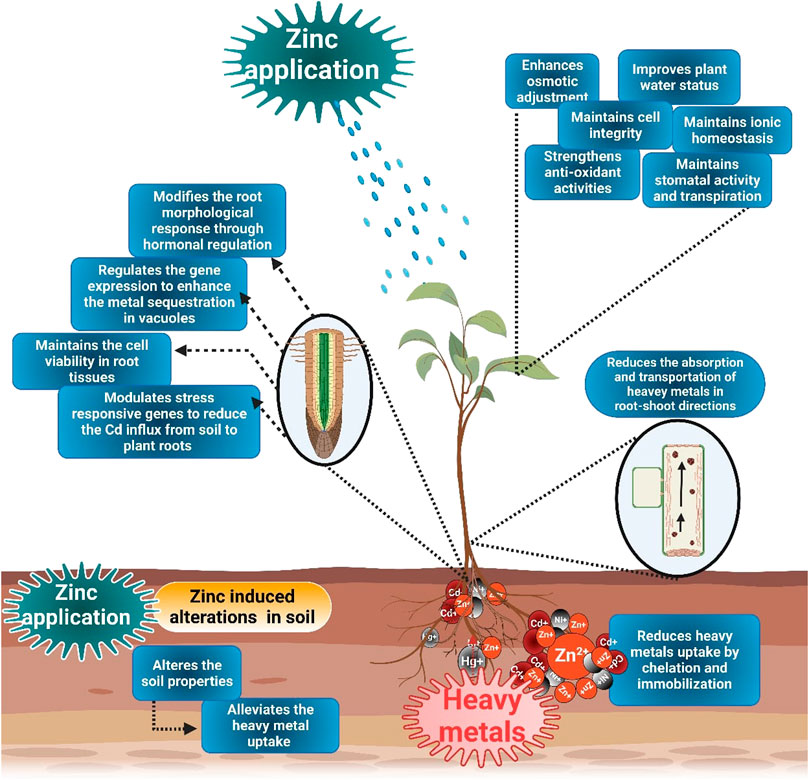
FIGURE 2. Mechanisms of Zn-induced heavy metals toxicity in plants. The application of Zn cause HMs chelation and immobilization in soil and, therefore, reduce their uptake. Zn supply also modifies root architecture, maintain viability of root cells, and regulate the stress-responsive genes to counter HMs toxicity. Moreover, Zn also maintains membrane stability, improve plant water status, osmolytes accumulation, stomata activities, and ionic homeostasis to counter HMs toxicity.
Zinc application improves nutrient uptake under heavy metal stress
Many authors conducted studies to determine the impact of Zn application on nutrient uptake under different HM stresses. Most studies indicated that Zn application improved the uptake of essential nutrients upon exposure to HM stress. The combined application of Zn and Fe chelated with amino acids improved the uptake of both Zn and Fe. Zn and Fe reduced the Cr uptake owing to the fact that amino acids form complexes with HMs and immobilize these metals and cause a reduction in uptake of these metals by plants (Rizwan et al., 2017). The application of Zn also improved the accumulation of Zn in different plants, such as wheat, rice, and spinach (Rizwan et al., 2017; Zaheer et al., 2019). Zaheer et al. (2020) studied the impact of the combined application of Zn and Fe on B. napus grown under Cr stress. They reported that application Fe and Zn increased the shoot Fe and Zn contents by 31% and 33%, whereas root Fe and Zn contents were increased by 28% and 32%, respectively (Zaheer et al., 2020). The combined application of Zn (10 μM) and Si (15 μM) significantly increased the K and Fe contents in roots of rice cultivar Xiushui-110 grown in Cd stress (15 μM) (Mapodzeke et al., 2021).
In contrast, these authors noted a reverse trend in sources of cultivar HIPJ-1 grown under Cd stress (Mapodzeke et al., 2021). In another study, it was observed that Zn supply (50 mg/L) appreciably improved the uptake of nutrients in roots and shoots of rice plants grown under Cd stress (0.8 mM) (Faizan et al., 2021b). In addition, Cao et al. (2013) reported that Zn and glutathione (GSH) application on Cr toxicity. These applications significantly increased the accumulation of Mn, Zn, and Fe by 22%, 14.5%, and 44%, respectively, in rice plants grown under Cr stress (20 μM). All the aforementioned studies show the positive impact of Zn on nutrient uptake under HM stress. More studies are needed to underpin the mechanism involved in Zn-mediated improvement in nutrient uptake under HMs.
Zinc improves plant photosynthetic performance under heavy metals
Heavy metals significantly reduced photosynthetic pigments and photosynthetic efficiency, reducing plant growth and yield. However, Zn possesses an excellent potential to improve plant photosynthetic performance (Figure 2) upon exposure to metal stress (Kolenčík et al., 2019). The application of Zn repairs photosystem II (PS II) and improves the gas exchange parameters. Photosynthetic performance increased under metal stress (Rajiv et al., 2018; Salama et al., 2019). Zn application increased Chl contents, which improved photosynthesis and subsequent plant growth (Altaf et al., 2020; Faizan et al., 2020). The improvement in photosynthetic pigments and stabilization of the photosynthetic apparatus by Zn application under Cd stress is linked with Zn-induced ROS reduction (Rizwan et al., 2019). The application of chelated Fe and Zn increased the Chl contents and gas exchange parameters (Pn, Tr, and gs) in wheat and rice plants grown under Cd (Rizwan et al., 2017; Bashir et al., 2018).
Zaheer et al. (2020) noted that the application of Zn (10 mg/L) improved Chl, Tr, Gs, Pn, and water use efficiency (WUE) by 37%, 36%, 6%, 40%, 6%, and 126%, respectively, in B. napus plants grown under Cr stress (Zaheer et al., 2020). An exogenous supply of Zn has been shown to improve the WUE and nutrient uptake, improving plant growth by governing the process of photosynthesis and transpiration (Hussain et al., 2018a). The combined application of Zn and Si improved the Chl and Pn compared to control in rice plants grown under Cd stress (Mapodzeke et al., 2021). The application of Zn stabilizes the redox homeostasis and improves the photosynthetic performance under HM stress (Mapodzeke et al., 2021). Moreover, Zn, in combination with Si, reduces internal CO2 concentration by utilizing CO2 at a higher rate to counter the effects of Cd-induced reduction in Pn and improves plant stress tolerance (Mapodzeke et al., 2018). The increase in Tr following Zn and Si application is linked with higher transpiration and nutrient uptake (Mapodzeke et al., 2021).
The combined application of Zn and Co improved the plant photosynthetic efficiency and the Fv/Fm ratio (Hussain et al., 2013; Salam et al., 2022). Interestingly, Zn improved the Chl contents and gas exchange parameters, and Zn increased Chl contents by increasing Chl synthesis (Sadak and Bakry, 2020; Del Buono et al., 2021). The excess of Zn ameliorated the photochemistry of PS II by enhancing the fraction of energy utilized for the photochemistry of PS II and restoring the PS II redox state. It also fixed the chloroplast ultra-structure under Cd stress, improving the plant photosynthetic efficiency (Sperdouli et al., 2022).
Zinc strengthens anti-oxidant activities under heavy metals stress
Heavy metals induce the production of ROS in plants; however, plants activate an excellent defense system comprising different anti-oxidant enzymes (APX, CAT, POD, and SOD: Table 2) (Afridi et al., 2019). SOD works as the first defense line against the oxidative stress created by ROS (Dos Santos et al., 2017). Zn supplementations enhanced the anti-oxidant activities (Figure 3) and reduced the HMs-induced oxidative damages in maize (Salam et al., 2022). Hussain et al. (2018b) studied the impact of different levels of chelated Zn (0, 10, 20, and 30 mg/L) on growth performance and anti-oxidant activities of rice plants grown under Cd stress (0, 100, and 500 mg/kg). Applying all the Zn levels significantly improved APX, CAT, POD, and SOD (Table 2) and reduced Cr-induced oxidative stress. In another study, Zaheer et al. (2020) also found a substantial increase in gas exchange characteristics with Zn application under Cr stress (50, 100, and 200 mg/kg).
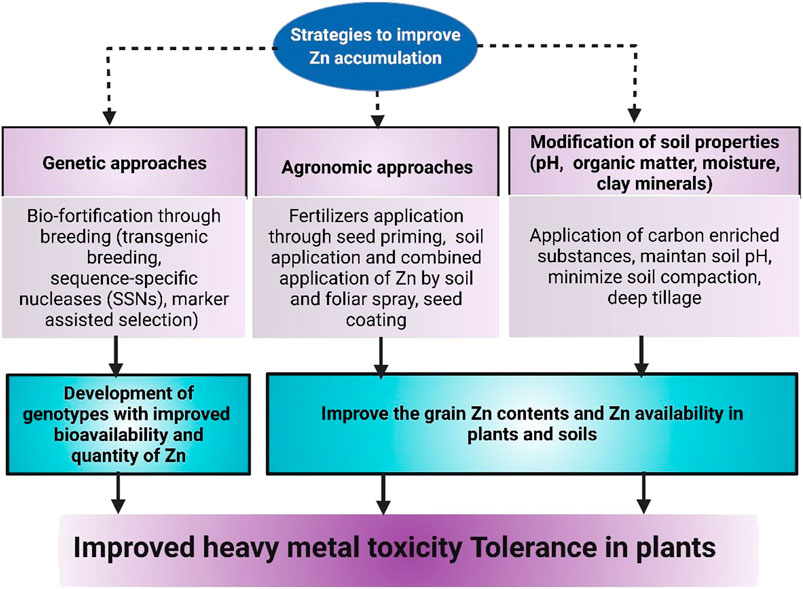
FIGURE 3. Zinc application increases the activities of anti-oxidant activities and osmolytes accumulation that reduces MDA, H2O2, and EL accumulation and improves cell water potential and water use efficiency, thereby improving plant tolerance against HM stress.
Faizan et al. (2021b) tested the impact of ZnO application (50 mg/L) on rice plants grown under Cd stress (0.8 mM). The application of Zn increased the activities of CAT (52%), SOD (59%), and proline accumulation (17%), which in turn appreciably improved the growth of rice by mitigating Cd-induced oxidative stress. In addition, another group of authors noted that Zn application (15 µM) significantly reduced the MDA accumulation while enhancing the photosynthetic performance and activities of CAT and SOD (Mapodzeke et al., 2021). Salam et al. (2022) studied the impact of ZnO application (500 mg/L) on maize plants exposed to Co stress (300 µM). They found that ZnO application appreciably improved the activities of APX, CAT, and SOD by 15%, 16%, and 56%, respectively, and improved the growth and photosynthetic performance under Co stress. Cao et al. (2013) texted the impact of Zn (20 µM) on rice crops grown in hydroponic systems developed under Cr (20 µM) and Cd stresses (20 µM). They noted that Zn application improved the photosynthetic pigments and anti-oxidant activities (APX, CAT, and SOD) under both Cd and Cr stresses.
Calgaroto et al. (2011) exposed P. glomerata plants to Hg stress (50 µM) and supplemented them with Zn application (50 µM). Applying Zn alone or combined with Hg significantly increased shoot APX, CAT. and SOD activities. Soybean plants are treated with diverse As stress levels (0, 10, and 20 uM) under ZnO application (0, 50, and 100 mg/L). Applying Zn (50 µM) increased activities of APX and GR by 37.17% and 16.27%, respectively, under 20-µM As stress (Ahmad et al., 2020). Wu F. et al. (2020) demonstrated that rice seeds primed with Zn (200 mg/L) showed an increase in SOD (22.8%) and CAT (60.705) activities, whereas the reduction in MDA accumulation (30.80%) under As stress (2 mg/L) was observed (Wu F. et al., 2020). Moreover, Yan et al. (2021) also noted an appreciable increase in CAT and SOD activities of rice shoots with Zn application grown under As stress (2 mg/L). Khan et al. (2022) studied the impact of ZnO and PGPR bio-fertilizers on maize plants grown under As stress (50 and 100 ppm). The application of ZnO in combination with plant-growth-promoting rhizobacteria (PGPR) up-regulated the anti-oxidant activities (APX, CAT, POD, and SOD). Anti-oxidants which oxidized the ROS produced in response to As stress (Khan et al., 2022). The glyoxalase cycle consists of glyoxalase I and glyoxalase II (Gly I and Gly II), which remove the methyl glyoxalate (MG) produced during stress conditions (Yadav et al., 2005). The supplementation of ZnO under As stress increased the activities of Gly I and Gly II, reducing MG production and As-induced oxidative stress (Ahmad et al., 2020).
Zinc favors osmolytes accumulation under heavy metals stress
Osmolytes play an imperative role against HMs toxicity in plants, and their accumulation under HM stress improved the anti-oxidant activities and reduced the HMs-induced oxidative stress. For instance, proline (Pro) accumulation has a positive correlation with total anti-oxidant activities (Farooq et al., 2020), and Pro helps in membrane stabilization, removes excessive ROS, and regulates growth under stressful conditions (Torabian et al., 2018). The application of Zn appreciably improved the Pro contents under Cd stress, improving the anti-oxidant activities and plant performance under Cd stress (Farooq et al., 2020). Similarly, Faizan et al. (2021b) noted that ZnO supply increased the Pro contents by increasing the expression of Pro synthesis genes under Cd stress. Moreover, other authors also noted a substantial increase in Pro accumulation with the application of Zn (Helaly et al., 2014; Faizan et al., 2020). Metal toxicity decreases the accumulation of proteins, associated with reduced protein synthesis, and increased protease activity that destroys the protein structure and activity (Balestrasse et al., 2003). Nonetheless, Zn application reduced protein degradation and increased nitrogen uptake, increasing protein accumulation (Laware and Raskar, 2014; Faizan et al., 2021a). Ahmad et al. (2020) exposed the soybean plants to As stress (10 and 20 µM) grown under different levels of ZnO (50 and 100 mg/L). They noted that ZnO application (50 and 100 mg/L) significantly improved the Pro contents by 14.28% and 38.09%, respectively, under As stress (10 and 20 µM). Moreover, these authors also noted that Zn application (100 mg/L) increased the glycine betaine (GB) accumulation by 11.08% and 28.66%, respectively, in rice plants exposed to 10 and 20 μM As stress (Ahmad et al., 2020). These are the limited information available in literature about the effect of Zn application on osmolyte accumulation under HM stress. Therefore, it would be fascinating to conduct more studies on this aspect which will open new window about the role of Zn against HMs toxicity.
Zinc brought ultra-structural changes in plants to induce tolerance against heavy metals
Zn application also brought ultra-structural changes in the plant to induce tolerance against HMs. Metal toxicity (Cd) significantly reduced the viability of cells in plant root tissues; however, the application of ZnO significantly prevented the losses of cell viability in root tissues (Faizan et al., 2021b). The improved photosynthetic efficiency in plants is liked with improved stomatal properties. Co-stress induced damages to guard cells and stomata aperture and significantly reduced plant photosynthetic efficiency. The damage to stomatal aperture and guard cells are the direct effect.
Nonetheless, ZnO application (500 mg/L) significantly protects the guard cells and stomata from damaging impacts of metal stress and improves the plant photosynthetic efficiency (Salam et al., 2022). Moreover, the ZnO application also recovered the chloroplast shape and enhanced the size of grain starch of maize plants grown in metal toxicity (Salam et al., 2022). Zn supply also alleviated the Cd-induced impacts on chloroplast and ensured that the grain starch contents and thylakoid membranes were less dilated (Sperdouli et al., 2022). Metal toxicity also alters the meristematic root cells and induces cellular deformation and thinning of cell walls (Mapodzeke et al., 2021). Zinc application in combination with Si improves the formation of root tip cells, chlorophyll formation, and starch granule formation. Zn improved the photosynthetic performance in crops (Mapodzeke et al., 2021).
Zinc reduces heavy metals uptake and causes immobilization and chelation of heavy metals
Zinc application reduced the HMs uptake (Figure 2), an essential mechanism in plant metal toxicity. Zn application improves Zn accumulation in plants and prevents Cd uptake through the roots and xylem-to-phloem transfer. Zn available in the growth medium has antagonistic effects on Cd uptake and transport (Zhou et al., 2019). Furthermore, Zn also reduced Cd toxicity by decreasing uptake of Cd and promoting biomass production, therefore diluting the metal concentration in plants, also known as the dilution effect (Ur Rehman et al., 2019). Zn supplementation also changes the metal fragmentation in soils and blocks the metal migration toward plant roots (Khan et al., 2019; Zhou et al., 2020). Moreover, Zn application also decreased soil pH, significantly reducing the bioavailability of Cd fractions in soil. Zn application reduced the Cd uptake and subsequent accumulation and its damaging impacts on plants (Khan et al., 2019; Zhou et al., 2020). Likewise, many other authors also noted that Zn application reduced the Cd uptake, its translocation to shoots, and its accumulation in wheat and tomato (Faizan et al., 2020).
ZnO application reduces the absorption and transportation of metals in root–shoot directions, and Zn also functions as a physical barrier to restrict metal uptake by the plant (Faizan et al., 2021a). Zn transporters are assumed to be involved in Hg uptake, and their numbers are significantly affected by Zn presence. The presence of more Zn would occupy more Zn transporters and result in less transport for Hg, reducing the Hg uptake by plants (Liu et al., 2021). In addition, Zn also depicts the anti-oxidant characteristics by diverse pathways, including the metallothionein formation and participates in forming lower molecular weight metallothionein-like proteins (Lane et al., 1987). This MTLP is rich in cysteine, which is famous for binding Hg ions; hence, Zn application alleviates the Hg toxicity by forming the MTLP (Liu et al., 2021). Applying chelated Zn and Fe with amino acids reduced the Cr uptake because amino acids form complexes and reduce their uptake by plants (Rizwan et al., 2017; Zaheer et al., 2022). In another study, it was noted that Zn application reduced the Cd contents in root, stem, and leaf except for grains and substantially reduced the Cd-induced toxicity in wheat plants (Zhou et al., 2020).
Zinc modulates stress-responsive genes under heavy metals stress
The regulation of genes is considered an essential mechanism by which Zn improves plant metal toxicity (Qiao et al., 2019). Natural resistance-associated macrophage protein (Nramp5) is a crucial transporter of Cd and Mn in roots. It is also responsible for the uptake of Cd from the soil (Wu C. et al., 2020). Knockout or knockdown of Nramp5 leads to a significant reduction in Mn and almost complete loss of Cd uptake in roots and shoots (Sasaki et al., 2012). Zinc application significantly reduced the expression of TaNramp5, reducing the Cd influx from soil to plant seeds (Zhou et al., 2020).
Moreover, the knockdown of OsLCT1 by Zn application also decreased the phloem-mediated Cd translocation, whereas it had no impact on xylem-mediated Cd transportation in rice plants (Uraguchi et al., 2011). Zn application also reduced the expression of TaLCT1 in wheat roots, resulting in a significant reduction in Cd accumulation in wheat roots (Zhou et al., 2020). Triticum aestivum transmembrane 20 (TaTM20) is an imperative Cd transporter (Kim et al., 2008). Thus, Zn supplementation increased the expression of TaTM20 and TaHMA3 and decreased the Cd accumulation in roots and leaves, indicating both these genes’ role in Cd efflux (Zhou et al., 2020). HMA2 works as a pump and effluxes Cd and Zn out of the cell. This protein is localized to tonoplast and involves transporting Cd to the xylem (Yang et al., 2020). The foliar application of Zn down-regulated the TaHMA2 expression and reduced the Cd translocation from root to shoots.
Moreover, foliar applied Zn also up-regulated the expression TaHMA3, which enhanced the Cd sequestration in roots’ vacuoles, significantly reducing Cd concentration in plant shoots (Begum et al., 2019; Zhou et al., 2020). These are the limited information available about the role of Zn in increasing the expression of different genes for reducing the effects of HMs. Therefore, a better understanding of gene expression involved in Zn-mediated HMs toxicity is crucial to decipher molecular mechanism behind this phenomenon properly.
Zinc regulates plant growth and yields under heavy metals stress
Plants exposed to HMs significantly reduce growth and development (Table 3). Zn application significantly reduced the HMs toxicity and improved the plant growth and development by triggering anti-oxidant activities and reducing metal accumulation (Table 3). The positive effect of Zn application on plant growth has been confirmed in many studies, which indicate that Zn application significantly improved the increase in metal-contaminated soils (Abbas et al., 2017). The application of Zn significantly improved the biomass of the wheat crop (Khan et al., 2015), which could be attributed to its beneficial role as an essential micronutrient (Shalaby et al., 2017). The time of Zn application is crucial to improve the growth and grain Zn concentration. For instance, Zhou et al. (2020) demonstrated that Zn applied at elongation and heading stages markedly improved wheat crop growth and grain Zn contents. Maize seeds primed with ZnO showed larger plants with more biomass production, linked with reduced metal uptake and translocation from the roots to aerial plant parts (Salam et al., 2022).
Faizan et al. (2021b) studied the impact of ZnO application (50 mg/L) on the growth of rice plants grown under Cd stress (0.8 mM). The application of Zn increased the shoot length (SL; 34%), fresh root weight (RFW; 30%), root dry weight (RDW; 12%), and shoot dry weight (SDW; 23%) as compared to the control. Applying Zn increases the chlorophyll contents, and an increase in Chl contents improves photosynthetic activity and plant growth (Altaf et al., 2020). Zaheer et al. (2020) reported that Zn application (10 mg/L) significantly enhanced the growth and reduced the Cr uptake and accumulation by B. napus plants. Ali et al. (2022) designed a study to determine the impact of Zn–lysine (12.5 and 25 mM) on rice and wheat plants grown under Cd stress (10 and 20 mg/kg). Applying Zn significantly improved crops’ growth and physiological processes under Cd stress (Ali et al., 2022). A thirty-day-old P. glomerata plants exposed to Hg stress (50 μM) showed a significant increase in fresh shoot weight (71%) and new root weight (51%) following the application of Zn (50 μM) (Calgaroto et al., 2011).
Applying Zn in combination with Si improved the root and shoot length, biomass production, tiller formation, and panicles of rice crop in Cd stress (Mapodzeke et al., 2018). The higher Zn contents alleviated the Cu-induced toxic effects and improved rice plants’ growth and biomass production (Aravind and Prasad, 2005). Moreover, Zn application also helps the Cd-induced oxidative stress and enhances the growth and biomass production by inhibiting the Cd uptake (Akay and Koleli, 2007). The application of Zn (10 mg/kg) significantly reduced the Cd-induced (5 and 10 mg/kg) damages and considerably increased the grain weight, tillers, and grain yield of the wheat crop (Abbas et al., 2017). Javed et al. (2016) applied Zn as a foliar spray (0.05%) to the different stages of wheat crops grown under Cd stress. They noted Zn application at booting and heading stages significantly improved the plant height and biomass production, tillers production (30%), leaves dry weight (19%), and grain production (12%) under Cd stress as compared to Zn applied at tillering and jointing stages (Javed et al., 2016). Moreover, Sarwar et al. (2014) determined the impact of foliar Zn application (0.3% and 0.6%) on wheat plants grown under Cd stress (13 mg/kg). The application of Zn (0.6%) significantly increased the plant height, tillers, grain weight, and grain yield of wheat crop (Sarwar et al., 2014).
Strategies to improve Zinc accumulation in crops
The beneficial impacts of Zn for mitigating HM-induced toxicity are linked with a higher accumulation of Zn in plant parts (Figure 4). The collection of Zn in plants largely depends on Zn availability in soils and plants’ ability to accumulate Zn. The level of Zn in soils can be improved by modifying the soil properties. In contrast, genetic modification and application of Zn fertilizers can enhance plants’ capacity to accumulate more Zn (Figure 4).
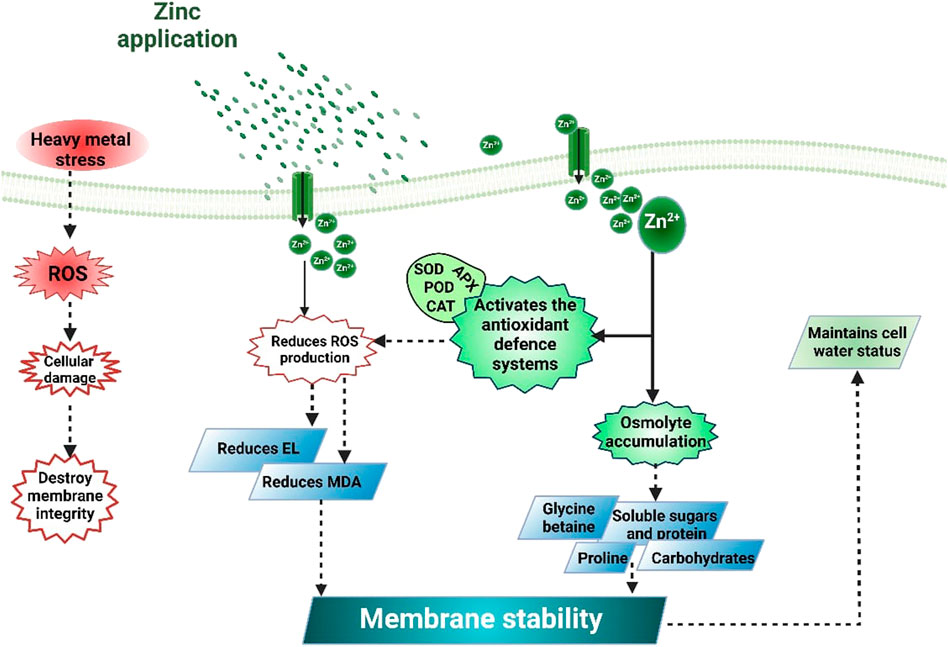
FIGURE 4. Different approaches that can be used to improve Zn accumulation in plants to induce HM stress tolerance.
Genetic approaches
Bio-fortification is a vital global strategy to improve grain nutrient concentration (Miller and Welch, 2013). This approach used breeding and agronomic practices to enhance the accumulation of nutrients in crop plants. The breeding techniques are considered costly and time-consuming, whereas agronomic practice (fertilizers application) is a quick solution to tackle this problem (Bouis et al., 2011; Shahzad et al., 2021). The breeding techniques involve exploring the natural variations amid genotypes for nutrient concentration and changing their genetic makeup to improve the bioavailability and quantity of nutrients (Pfeiffer and McClafferty, 2007). The increase in nutrient (Zn) accumulation in plants substantially improved the plant tolerance against HMs (Farooq et al., 2020). The desired genes are identified from the gene pool of plants and inserted in plants to develop the plants with a more vital ability to accumulate nutrients (Shahzad et al., 2021). Transgenic breeding is an imperative and economical approach to enhancing plant nutrient contents. This technique involves the introduction of genes that increase nutrient availability and decreases anti-nutrient compounds (Shahzad et al., 2021). The increase in grain Zn contents following breeding and agronomic approaches (Shahzad et al., 2021) improved the plant tolerance against Cd and Cr stresses (Ahmad et al., 2020; Faizan et al., 2021b).
Selecting suitable quantitative trait loci (QTLs) linked with higher Zn uptake can also be an essential approach for producing genetically modified crops with higher Zn contents (Dong et al., 2006). The combination of QTL analysis and molecular genetics can help breed improved crop varieties with better efficiency in nutrient use (Verma et al., 2021). Recently, a genome-wide association study (GWAS) has emerged as an essential tool involved in sequencing methods. Bioinformatic tools identify the QTLs and natural variations to characterize the genetic loci controlling the diverse agronomic traits (Huang et al., 2010). Likewise, GWAS is also an effective strategy for identifying QTLs that possess the Zn nutrition trait and is also an efficient way to improve Zn uptake and Zn efficiency in crops (Verma et al., 2021). The transgenic approaches also identified many Zn transporters linked with cations, such as ZRT-IRT-like proteins (ZIP) and cation diffusion facilitator (CDF) families. These play an essential role in the uptake and translocation of Zn in plants (Verma et al., 2021). For example, over-expression of AtZIP1 and HvZIP7 in barley plants has increased the grain Zn contents (Tiong et al., 2014).
Moreover, ligands synthesis gene expression could also be a refreshing approach to Zn solubility , thereby Zn level in crops (Verma et al., 2021). CRISPR/Cas9 has recently become an essential tool for improving crop performance and nutritional quality (Liu et al., 2021). Using CRISPR/Cas9 can substantially increase the concentration of micronutrients in plants. For instance, in rice plants, CRISPR/Cas9-mediated knockdown of vacuolar iron transporter (VIT) genes (OsVIT2) substantially increased the grain Fe contents (Che et al., 2021). Similarly, CRISPR/Cas9-induced disruption of inositol pentakisphosphate 2-kinase 1 (TaIPK1) appreciably improved the grain Zn contents in wheat crop (Ibrahim et al., 2022).
The sequence-specific nucleases (SSNs) can also be used to improve crop nutrient accumulation and bioavailability. This practice involves the modification of desired genes to produce transgenic plants, resulting in a substantial increase in the collection of nutrients in plants (Shahzad et al., 2021). Increasing the breeding bio-fortification of Fe and Zn is complex in plants because the concentrations of nutrients in grains depend on diverse physiological processes (Garcia-Oliveira et al., 2018). Recently a significant genetic variability for these minerals has been reported in six major cereal crops including barley, maize, rice, sorghum, and pearl millet (Teklić et al., 2013; Goudia and Hash, 2015; Gregory et al., 2017). The more precise and reliable phenotyping is also a preference of breeders. However, an extensive study of literature linked with variably Fe and Zn concentrations in cereals suggested that accurate measurement of these nutrients is challenging (Gregory et al., 2017).
Phytic acid (PA) is an essential chelator of Zn, and it affects the Zn availability in plants (Ali et al., 2010). Different mutants of maize, rice, and wheat with low PA have been identified, which can help reduce the PA accumulation in plants to improve Zn availability (Liu et al., 2007). Moreover, proteins significantly increased the nutrient uptake and translocation in plant cells. For instance, ferritin genes transferred from soybean to markedly enhanced grain Zn and Fe contents of rice (Qu et al., 2005). Using molecular markers is also critical. For example, many QTLs associated with Zn have been identified in cereals that can help develop genotypes with higher nutrient accumulation to ensure metal tolerance (Xie et al., 2019; Faizan et al., 2021a). Breeding practices are a sustainable way to develop grains enriched with Zn contents. However, these techniques are costly and need significant resources and time to establish the Zn-enriched grains (Cakmak, 2008). In addition, the success of newly developed genotypes also depends on the Zn pool in soils. Higher Zn protein soil must be maintained to get desired results. In conclusion, developing genotypes with a more dynamic ability to accumulate Zn in grain and other plant parts can help reduce the harmful impacts of HMs on plants and humans.
Zinc fertilization
Fertilizer application is considered a quick and excellent approach (Figure 4) for improving grain Zn concentration and Zn availability in plants. This strategy improves the Zn availability in plants and substantially enhances growth and yield (Yadav et al., 2011). The fertilizer application substantially increased the Zn availability and influences the amount of Zn available to plants (Zaheer et al., 2019). Globally different methods of Zn application are used for improving grain Zn concentration and Zn availability in soil and plants. The Zn application method significantly impacts the grain Zn concentration; therefore, the Zn application method must be economical and farmer friendly (Hussain et al., 2010). Seed priming (SP) is an essential method of Zn application that increases crop production and Zn uptake by plant seeds. This economical and environmentally friendly method appreciably improved grain productivity and grain Zn concentration in plants (Farooq et al., 2012). This method also plays a crucial to improve the HMs tolerance by increasing the Zn concentration in plants. For instance, wheat seeds primed with ZnO showed an appreciable tolerance against Co stress. Results showed a substantial increase in Zn uptake and its translocation to plant plants (Salam et al., 2022).
Soil application of Zn is also an important method used across the globe; however, this method is considered costly because a large quantity of Zn is used in this method (Zaheer et al., 2019). The application of soil-applied Zn effectively increased the grain yield; however, this method is less effective in increasing grain Zn contents (Cakmak et al., 2010). Zn’s soil and foliar application is an efficient approach to improve grain Zn, grain yield, and Zn availability in soil and subsequently to plants (Chattha et al., 2017). This method is also effective in reducing the HMs toxicity in plants. The soil application of Zn effectively increased the Zn concentration in plant parts (root, stem, and leaf). It also reduced the Cd uptake and accumulation, thereby reducing the Cd-induced deleterious impacts on the wheat crop (Farooq et al., 2020; Zhou et al., 2020). Foliar application is another method of Zn application that maintains a higher Zn pool and significantly improves the Zn concentration in grains and other plant parts (Cakmak et al., 2010). This method is considered economical compared to soil application because, in this method, a small quantity of Zn is used. The foliar spray of Zn is also an important method to reduce the harmful impacts of HMs on plants. Many studies indicated that foliar spray of Zn application considerably increased Zn concentration in plant parts and Zn uptake by plants. Zn application also reduced the deleterious impacts of Cd, Cr, and As by restricting their uptake and accumulation in plants (Ahmad et al., 2020; Zaheer et al., 2020; Faizan et al., 2021b). Therefore, in light of the aforementioned findings, agronomic practice (fertilizer application) is an effective and economical approach to improving plant tolerance against HMs.
Modification of soil properties
The concentration of Zn in soil solution is significantly affected by different factors, including soil pH, soil organic matter (SOM), moisture contents, clay minerals, clay minerals, and carbonates (Wang et al., 2017). The carbonates and Fe oxides play a critical role in the dynamics of Zn in soil, affecting Zn availability to plants (Komárek et al., 2018; Moreno-Lora and Delgado, 2020). Therefore, modifying soil Fe oxides by adding organic matter can improve the Zn availability in soil and plants. SOM significantly affects the Zn solubility and its transportation to roots and upper plant parts (Cakmak, 2008). Therefore, applying carbon-enriched substances can substantially increase the soil Zn contents (Chen et al., 2017). Zn contents can help to reduce the HMs’ toxicity in plants (Bashir et al., 2020). Soil pH is an important parameter that significantly affects the Zn availability from the soil solution. The soils with higher pH have lower Zn availability and vice versa (Sadeghzadeh, 2013). However, adding different substances, including sulfuric acid and sulfur, can help reduce soil pH, significantly improving Zn availability and ensuring better tolerance against HMs. Modern agricultural practices have considerably enhanced crop production; however, these practices imposed many disadvantages, such as soil compaction due to the use of heavy machinery. The soil compaction reduced the root’s growth and root penetration and, therefore, reduced the Zn uptake that negatively affects crops’ growth, yield and quality (Parlak and Parlak, 2011). Therefore, minimization of soil compaction and deep tillage practices used intermittently can reduce the soil compaction to ensure better nutrient availability. The higher soil phosphorus (P) contents and application of heavy doses of P fertilizers reduces the Zn availability to plants because Zn co-precipitate with P as ZnO3 (PO4)2, reduces the soil Zn contents. The reduced soil Zn concentration reduced the Zn uptake by plants and resulted in less Zn accumulation in plant parts (Yang et al., 2011). Therefore, a dose of P fertilizers must be chosen precisely to ensure better Zn availability to plants from soil solution to counter the effects of HM stress.
Conclusion and prospects
Anthropogenic activities have significantly increased the concentration of toxic metals in agriculture soils. HMs negatively affects crop growth, development, and yield and pose a severe threat to human health by entering the food chain. The effects of HMs on the environment, agriculture, and humans are critical; therefore, it is direly needed to develop remedial strategies to counter the effects of HMs. In this context, Zn application has become an essential practice to reduce plant metal toxicity. Applying Zn improves membrane stability, plant water uptake, and nutrient uptake. Zn application also maintains photosynthetic performance by improving gas exchange characteristics and photosystem efficiency. Zn supplementation improves osmolytes accumulation and expression of stress-responsive genes and brings ultra-structure changes in the plant body to counter the effects of HMs. At the same time, Zn triggers the anti-oxidant defense system and reduces the ROS that counters HM-induced oxidative stresses. In addition, Zn reduced the HMs uptake and caused chelation and immobilization of HMs in soil, restricting their uptake and entry into the plant body.
However, the role of Zn under HMs toxicity is not fully explored, and many questions need to be answered in future studies. It is a dire need to optimize the rates of Zn application for different crops under different climatic conditions and cropping systems before it is recommended for the farming community. Most studies are conducted in control conditions; limited field studies are available about the role of Zn under HMs toxicity. Therefore, it is a dire need to conduct long-term studies in soils affected by HMs to explore the potential of Zn in mitigating the harmful impacts of HMs. Moreover, the rate of Zn application must also be optimized by conducting long-term studies keeping the soil and climatic conditions in mind. The crosstalk of Zn with osmolytes and different hormones is poorly studied. Most authors studied the crosstalk of Zn with proline and glycine betaine under HMs. Therefore, more studies are needed to explore the crosstalk of Zn with abscisic acid, auxin, cytokinin, ethylene, and gibberellins under HMs, as these hormones play a significant role in tolerance against HMs.
In addition, the effect of Zn application on the accumulation of metabolites, plant nutrients, and other osmolytes needs more studies. The role of Zn in gene expression under HMs is also poorly studied; therefore, it is needed to explore the role of Zn in gene expression under different HMs. The molecular mechanism of Zn-induced HMs tolerance is poorly studied. In-depth studies are direly needed to explore molecular mechanisms of Zn-induced HMs toxicity in plants. The role of Zn is studied well with only a few HMs (Cd, Cr, As, and Hg). Hence, more studies are needed to explore the role of Zn against lead, nickel, cobalt, and copper stresses. Enhancing the plant’s genetic potential to uptake Zn would also be a promising avenue to improve plant tolerance against HMs. Recent development in genomics, computational biology, genetic engineering, and high-throughput phenotyping can facilitate to understand the basis of Zn accumulation in plants. These findings increase the development of high Zn accumulating plant species. All these efforts will undoubtedly increase our understanding about the zinc role in mitigating the harmful impacts of HMs in plants.
Author contributions
MUH, MN, and ANS conceptualized the study. MUH prepared the manuscript. MN, AM, ANS, AAS, FM, MB, AR, MJ, NA, MH, and SQ reviewed and edited the manuscript. All authors contributed to the article and approved the submitted version.
Acknowledgments
Authors would like to acknowledge Alexandria University, Alexandria, Egypt and King Abdullah University of Science and Technology (KAUST), Saudi Arabia for support.
Conflict of interest
The authors declare that the research was conducted in the absence of any commercial or financial relationships that could be construed as a potential conflict of interest.
Publisher’s note
All claims expressed in this article are solely those of the authors and do not necessarily represent those of their affiliated organizations, or those of the publisher, the editors, and the reviewers. Any product that may be evaluated in this article, or claim that may be made by its manufacturer, is not guaranteed or endorsed by the publisher.
References
Aamer, M., Muhammad, U., Li, Z., Abid, A., Su, Q., Liu, Y., et al. (2018). Foliar application of glycinebetaine (GB) alleviates the cadmium (Cd) toxicity in spinach through reducing Cd uptake and improving the activity of anti-oxidant system. Appl. Ecol. Environ. Res. 16, 7575–7583. doi:10.15666/aeer/1606_75757583
Abbas, M., Akmal, M., Ullah, S., Hassan, M., and Farooq, S. (2017). Effectiveness of zinc and gypsum application against cadmium toxicity and accumulation in wheat (Triticum aestivum L.) Commun. Soil Sci. Plant Anal. 48, 1659–1668. doi:10.1080/00103624.2017.1373798
Abdelrahman, S., Alghrably, M., Campagna, M., Hauser, C. A. E., Jaremko, M., and Lachowicz, J. I. (2021). Metal complex formation and anticancer activity of cu (I) and cu (ii) complexes with metformin Molecules 26 (16), 4730.
Abdel Salam, M., Mokhtar, M., Albukhari, S. M., Baamer, D. F., Palmisano, L., and Jaremko, M. (2022). Synthesis and characterization of green ZnO@ polynaniline/bentonite tripartite structure (G. Zn@ PN/BE) as adsorbent for as (V) ions: Integration, steric, and energetic properties Polymers 14 (12), 2329.
Afridi, M. S., Mahmood, T., Salam, A., Mukhtar, T., Mehmood, S., Ali, J., et al. (2019). Induction of tolerance to salinity in wheat genotypes by plant growth promoting endophytes: Involvement of ACC deaminase and antioxidant enzymes. Plant Physiol. biochem. 139, 569–577. doi:10.1016/j.plaphy.2019.03.041
Ahmad, P., Alyemeni, M. N., Al-Huqail, A. A., Alqahtani, M. A., Wijaya, L., Ashraf, M., et al. (2020). Zinc oxide nanoparticles application alleviates arsenic (As) toxicity in soybean plants by restricting the uptake of as and modulating key biochemical attributes, antioxidant enzymes, ascorbate-glutathione cycle and glyoxalase system. Plants 9, 825. doi:10.3390/plants9070825
Ahmed, T., Noman, M., Manzoor, N., Shahid, M., Abdullah, M., Ali, L., et al. (2021). Nanoparticle-based amelioration of drought stress and cadmium toxicity in rice via triggering the stress responsive genetic mechanisms and nutrient acquisition. Ecotoxicol. Environ. Saf. 209, 111829. doi:10.1016/j.ecoenv.2020.111829
Akay, A., and Koleli, N. (2007). Interaction between cadmium and zinc in barley (< i> Hordeum vulgare< i> L.) grown under field conditions. Bangladesh J. Bot. 36, 13–19. doi:10.3329/bjb.v36i1.1543
Alam, P., Balawi, T. H., Altalayan, F. H., Hatamleh, A. A., Ashraf, M., and Ahmad, P. (2021). Silicon attenuates the negative effects of chromium stress in tomato plants by modifying antioxidant enzyme activities, ascorbate–glutathione cycle and glyoxalase system. Acta Physiologiae Plantarum 43, 1–17.
Alengebawy, A., Abdelkhalek, S. T., Qureshi, S. R., and Wang, M.-Q. (2021). Heavy metals and pesticides toxicity in agricultural soil and plants: Ecological risks and human health implications. Toxics 9, 42. doi:10.3390/toxics9030042
Alghrably, M., Czaban, I., Jaremko, T., and Jaremko, M. (2019). Interaction of amylin species with transition metals and membranes. J. .inorganic biochem. 191, 69–76.
Ali, M., Shuja, M. N., Zahoor, M., and Qadri, I. (2010). Phytic acid: How far have we come? Afr. J. Biotechnol. 9, 1551–1554. doi:10.5897/ajb10.045
Ali, S., Bai, P., Zeng, F., Cai, S., Shamsi, I. H., Qiu, B., et al. (2011). The ecotoxicological and interactive effects of chromium and aluminum on growth, oxidative damage and antioxidant enzymes on two barley genotypes differing in Al tolerance. Environ. Exp. Bot. 70, 185–191. doi:10.1016/j.envexpbot.2010.09.002
Ali, S., Mfarrej, M. F. B., Hussain, A., Akram, N. A., Rizwan, M., Wang, X., et al. (2022). Zinc fortification and alleviation of cadmium stress by application of lysine chelated zinc on different varieties of wheat and rice in cadmium stressed soil. Chemosphere 295, 133829. doi:10.1016/j.chemosphere.2022.133829
Altaf, M. M., Diao, X.-P., Imtiaz, M., Shakoor, A., Altaf, M. A., Younis, H., et al. (2020). Effect of vanadium on growth, photosynthesis, reactive oxygen species, antioxidant enzymes, and cell death of rice. J. Soil Sci. Plant Nutr. 20, 2643–2656. doi:10.1007/s42729-020-00330-x
Aravind, P., and Prasad, M. N. V. (2005). Modulation of cadmium-induced oxidative stress in Ceratophyllum demersum by zinc involves ascorbate–glutathione cycle and glutathione metabolism. Plant Physiol. biochem. 43, 107–116. doi:10.1016/j.plaphy.2005.01.002
Ashraf, M. Y., Sadiq, R., Hussain, M., Ashraf, M., and Ahmad, M. (2011). Toxic effect of nickel (Ni) on growth and metabolism in germinating seeds of sunflower (Helianthus annuus L.) Biol. Trace Elem. Res. 143, 1695–1703. doi:10.1007/s12011-011-8955-7
Balestrasse, K. B., Benavides, M. P., Gallego, S. M., and Tomaro, M. L. (2003). Effect of cadmium stress on nitrogen metabolism in nodules and roots of soybean plants. Funct. Plant Biol. 30, 57–64. doi:10.1071/fp02074
Bariş, Ç. Ç., and Muammer, Ü. N. A. L. (2021). Nikel’in Brokoli (Brassica oleracea L. var. italica) Tohumlarının Çimlenmesi ve Fide Gelişimi Üzerine Etkileri. Düzce Üniversitesi Bilim ve Teknoloji Dergisi 9 (3), 226–261. doi:10.29130/dubited.798455
Bashir, A., Rizwan, M., Ali, S., Adrees, M., and Qayyum, M. F. (2020). Effect of composted organic amendments and zinc oxide nanoparticles on growth and cadmium accumulation by wheat; a life cycle study. Environ. Sci. Pollut. Res. 27, 23926–23936. doi:10.1007/s11356-020-08739-8
Bashir, A., Rizwan, M., Ali, S., Zia Ur Rehman, M., Ishaque, W., Atif Riaz, M., et al. (2018). Effect of foliar-applied iron complexed with lysine on growth and cadmium (Cd) uptake in rice under Cd stress. Environ. Sci. Pollut. Res. 25, 20691–20699. doi:10.1007/s11356-018-2042-y
Batool, M., El-Badri, A. M., Hassan, M. U., Haiyun, Y., Chunyun, W., Zhenkun, Y., et al. (2022). Drought stress in Brassica napus: Effects, tolerance mechanisms, and management strategies. J. Plant Growth Regul., 1–25. doi:10.1007/s00344-021-10542-9
Begum, N., Hu, Z., Cai, Q., and Lou, L. (2019). Influence of PGPB inoculation on HSP70 and HMA3 gene expression in switchgrass under cadmium stress. Plants 8, 504. doi:10.3390/plants8110504
Bhat, J. A., Shivaraj, S., Singh, P., Navadagi, D. B., Tripathi, D. K., Dash, P. K., et al. (2019). Role of silicon in mitigation of heavy metal stresses in crop plants. Plants 8, 71. doi:10.3390/plants8030071
Bidast, S., Golchin, A., Baybordi, A., and Naidu, R. (2022). Effects of Fe oxide-based nanoparticles on yield and nutrient content of corn in Cobalt-contaminated soils. Environ. Technol. Innovat. 26, 102314. doi:10.1016/j.eti.2022.102314
Bouis, H. E., Hotz, C., Mcclafferty, B., Meenakshi, J., and Pfeiffer, W. H. (2011). Biofortification: A new tool to reduce micronutrient malnutrition. Food Nutr. Bull. 32, S31–S40. doi:10.1177/15648265110321s105
Briffa, J., Sinagra, E., and Blundell, R. (2020). Heavy metal pollution in the environment and their toxicological effects on humans. Heliyon 6 (9), e04691. doi:10.1016/j.heliyon.2020.e04691
Cakmak, I. (2008). Enrichment of cereal grains with zinc: Agronomic or genetic biofortification? Plant Soil 302, 1–17. doi:10.1007/s11104-007-9466-3
Cakmak, I., Kalayci, M., Kaya, Y., Torun, A., Aydin, N., Wang, Y., et al. (2010). Biofortification and localization of zinc in wheat grain. J. Agric. Food Chem. 58, 9092–9102. doi:10.1021/jf101197h
Cakmak, I. (2000). Tansley review No. 111: Possible roles of zinc in protecting plant cells from damage by reactive oxygen species. New Phytol. 146, 185–205. doi:10.1046/j.1469-8137.2000.00630.x
Calgaroto, N. S., Cargnelutti, D., Rossato, L. V., Farias, J. G., Nunes, S. T., Tabaldi, L. A., et al. (2011). Zinc alleviates mercury-induced oxidative stress in Pfaffia glomerata (Spreng.) Pedersen. Biometals 24, 959–971. doi:10.1007/s10534-011-9457-y
Cao, F., Wang, N., Zhang, M., Dai, H., Dawood, M., Zhang, G., et al. (2013). Comparative study of alleviating effects of GSH, Se and Zn under combined contamination of cadmium and chromium in rice (Oryza sativa). Biometals 26, 297–308. doi:10.1007/s10534-013-9611-9
Casarrubia, S., Martino, E., Daghino, S., Kohler, A., Morin, E., Khouja, H.-R., et al. (2020). Modulation of plant and fungal gene expression upon cd exposure and symbiosis in ericoid mycorrhizal Vaccinium myrtillus. Front. Microbiol. 11, 341. doi:10.3389/fmicb.2020.00341
Cedillo-Jimenez, C. A., García-Ortega, L. F., Martínez-Reséndiz, M., Arné-Robles, D., Cruz-Hernandez, A., and Guevara-Gonzalez, R. G. (2020). Response of transgenic tobacco overexpressing the CchGLP gene to cadmium and aluminium: phenotypic and microRNAs expression changes. Physiol. Mol. Biol. Plant 26, 3–13. doi:10.1007/s12298-019-00716-x
Chandra, R., and Kang, H. (2016). Mixed heavy metal stress on photosynthesis, transpiration rate, and chlorophyll content in poplar hybrids. Forest Sci. Technol. 12 (2), 55–61. doi:10.1080/21580103.2015.1044024
Chatterjee, C., Gopal, R., and Dube, B. (2006). Impact of iron stress on biomass, yield, metabolism and quality of potato (Solanum tuberosum L.). Scientia horticulturae 108, 1–6. doi:10.1016/j.scienta.2006.01.004
Chattha, M. U., Hassan, M. U., Khan, I., Chattha, M. B., Mahmood, A., Nawaz, M., et al. (2017). Biofortification of wheat cultivars to combat zinc deficiency. Front. Plant Sci. 8, 281. doi:10.3389/fpls.2017.00281
Che, P., Chang, S., Simon, M. K., Zhang, Z., Shaharyar, A., Ourada, J., et al. (2021). Developing a rapid and highly efficient cowpea regeneration, transformation and genome editing system using embryonic axis explants. Plant J. 106 (3), 817–830. doi:10.1111/tpj.15202
Chen, Y., Cui, J., Tian, X., Zhao, A., Li, M., Wang, S., et al. (2017). Effect of straw amendment on soil Zn availability and ageing of exogenous water-soluble Zn applied to calcareous soil. PLoS One 12, e0169776. doi:10.1371/journal.pone.0169776
Cota-Ruiz, K., Ye, Y., Valdes, C., Deng, C., Wang, Y., Hernández-Viezcas, J. A., et al. (2020). Copper nanowires as nanofertilizers for alfalfa plants: Understanding nano-bio systems interactions from microbial genomics, plant molecular responses and spectroscopic studies. Sci. Total Environ. 742, 140572. doi:10.1016/j.scitotenv.2020.140572
Del Buono, D., Di Michele, A., Costantino, F., Trevisan, M., and Lucini, L. (2021). Biogenic ZnO nanoparticles synthesized using a novel plant extract: Application to enhance physiological and biochemical traits in maize. Nanomaterials 11, 1270. doi:10.3390/nano11051270
Ding, Y., Ding, L., Xia, Y., Wang, F., and Zhu, C. (2020). Emerging roles of microRNAs in plant heavy metal tolerance and homeostasis. J. Agric. Food Chem. 68, 1958–1965. doi:10.1021/acs.jafc.9b07468
Dos Santos, J. O., De Fária, M. E., Da Silva, D. M., De Oliveira Silveira, H. R., Campos, C. N., and Alves, J. D. (2017). Copper (Cu) stress affects carbon and antioxidant metabolism in'Coffea arabica'seedlings. Aust. J. Crop Sci. 11, 960–967. doi:10.21475/ajcs.17.11.08.pne445
Faizan, M., Bhat, J. A., Chen, C., Alyemeni, M. N., Wijaya, L., Ahmad, P., et al. (2021a). Zinc oxide nanoparticles (ZnO-NPs) induce salt tolerance by improving the antioxidant system and photosynthetic machinery in tomato. Plant Physiol. biochem. 161, 122–130. doi:10.1016/j.plaphy.2021.02.002
Faizan, M., Bhat, J. A., Hessini, K., Yu, F., and Ahmad, P. (2021b). Zinc oxide nanoparticles alleviates the adverse effects of cadmium stress on Oryza sativa via modulation of the photosynthesis and antioxidant defense system. Ecotoxicol. Environ. Saf. 220, 112401. doi:10.1016/j.ecoenv.2021.112401
Faizan, M., Faraz, A., Mir, A. R., and Hayat, S. (2021c). Role of zinc oxide nanoparticles in countering negative effects generated by cadmium in Lycopersicon esculentum. J. Plant Growth Regul. 40 (1), 101–115. doi:10.1007/s00344-019-10059-2
Faizan, M., Sehar, S., Rajput, V.D., Faraz, A., Afzal, S., Minkina, T., et al. (2021d). Modulation of cellular redox status and antioxidant defense system after synergistic application of zinc oxide nanoparticles and salicylic acid in rice (Oryza sativa) plant under arsenic stress. Plants 10 (11), 2254. doi:10.3390/plants10112254
Faizan, M., Hayat, S., and Pichtel, J. (2020). “Effects of zinc oxide nanoparticles on crop plants: A perspective analysis,” in Sustainable agriculture reviews 41 (Berlin Germany: Springer), 83–99.
Faran, M., Farooq, M., Rehman, A., Nawaz, A., Saleem, M. K., Ali, N., et al. (2019). High intrinsic seed Zn concentration improves abiotic stress tolerance in wheat. Plant Soil 437, 195–213. doi:10.1007/s11104-019-03977-3
Farooq, M., Ali, S., Hameed, A., Bharwana, S., Rizwan, M., Ishaque, W., et al. (2016). Cadmium stress in cotton seedlings: physiological, photosynthesis and oxidative damages alleviated by glycinebetaine. S. Afr. J. Bot. 104, 61–68. doi:10.1016/j.sajb.2015.11.006
Farooq, M., Ullah, A., Usman, M., and Siddique, K. H. (2020). Application of zinc and biochar help to mitigate cadmium stress in bread wheat raised from seeds with high intrinsic zinc. Chemosphere 260, 127652. doi:10.1016/j.chemosphere.2020.127652
Farooq, M., Wahid, A., and Siddique, K. H. (2012). Micronutrient application through seed treatments: A review. J. Soil Sci. Plant Nutr. 12, 125–142. doi:10.4067/s0718-95162012000100011
Feng, R., Wang, L., Yang, J., Zhao, P., Zhu, Y., Li, Y., et al. (2021). Underlying mechanisms responsible for restriction of uptake and translocation of heavy metals (metalloids) by selenium via root application in plants. J. Hazard. Mater. 402, 123570. doi:10.1016/j.jhazmat.2020.123570
Foucault, Y., Lévèque, T., Xiong, T., Schreck, E., Austruy, A., Shahid, M., et al. (2013). Green manure plants for remediation of soils polluted by metals and metalloids: Ecotoxicity and human bioavailability assessment. Chemosphere 93, 1430–1435. doi:10.1016/j.chemosphere.2013.07.040
Gao, Y., Yang, F., Liu, J., Xie, W., Zhang, L., Chen, Z., et al. (2020). Genome-wide identification of metal tolerance protein genes in Populus trichocarpa and their roles in response to various heavy metal stresses. Int. J. Mol. Sci. 21, 1680. doi:10.3390/ijms21051680
Garcia-Oliveira, A. L., Chander, S., Ortiz, R., Menkir, A., and Gedil, M. (2018). Genetic basis and breeding perspectives of grain iron and zinc enrichment in cereals. Front. Plant Sci. 9, 937. doi:10.3389/fpls.2018.00937
Georgiadou, E. C., Kowalska, E., Patla, K., Kulbat, K., Smolińska, B., Leszczyńska, J., et al. (2018). Influence of heavy metals (Ni, Cu, and Zn) on nitro-oxidative stress responses, proteome regulation and allergen production in basil (Ocimum basilicum L.) plants. Fronti. Plant Sci. 9, 862. doi:10.3389/fpls.2018.00862
Ghori, N.-H., Ghori, T., Hayat, M., Imadi, S., Gul, A., Altay, V., et al. (2019). Heavy metal stress and responses in plants. Int. J. Environ. Sci. Technol. (Tehran). 16, 1807–1828. doi:10.1007/s13762-019-02215-8
Gill, S. S., and Tuteja, N. (2010). Reactive oxygen species and antioxidant machinery in abiotic stress tolerance in crop plants. Plant Physiol. biochem. 48 (12), 909–930. doi:10.1016/j.plaphy.2010.08.016
Goudia, B. D., and Hash, C. T. (2015). Breeding for high grain Fe and Zn levels in cereals. Int. J. Innovation Appl. Stud. 12, 342–354.
Gregory, P. J., Wahbi, A., Adu-Gyamfi, J., Heiling, M., Gruber, R., Joy, E. J., et al. (2017). Approaches to reduce zinc and iron deficits in food systems. Glob. Food Secur. 15, 1–10. doi:10.1016/j.gfs.2017.03.003
Guo, H., Hong, C., Chen, X., Xu, Y., Liu, Y., Jiang, D., et al. (2016). Different growth and physiological responses to cadmium of the three Miscanthus species. PloS one 11, e0153475. doi:10.1371/journal.pone.0153475
Hart, J. J., Welch, R. M., Norvell, W. A., and Kochian, L. V. (2002). Transport interactions between cadmium and zinc in roots of bread and durum wheat seedlings. Physiol. Plant. 116, 73–78. doi:10.1034/j.1399-3054.2002.1160109.x
Hasanuzzaman, M., Alhaithloul, H. a. S., Parvin, K., Bhuyan, M., Tanveer, M., Mohsin, S. M., et al. (2019). Polyamine action under metal/metalloid stress: Regulation of biosynthesis, metabolism, and molecular interactions. Int. J. Mol. Sci. 20, 3215. doi:10.3390/ijms20133215
Hasanuzzaman, M., Bhuyan, M. B., Raza, A., Hawrylak-Nowak, B., Matraszek-Gawron, R., Al Mahmud, J., et al. (2020b). Selenium in plants: Boon or bane? Environ. Exp. Bot. 178, 104170. doi:10.1016/j.envexpbot.2020.104170
Hasanuzzaman, M., Bhuyan, M., Zulfiqar, F., Raza, A., Mohsin, S. M., Mahmud, J. A., et al. (2020a). Reactive oxygen species and antioxidant defense in plants under abiotic stress: Revisiting the crucial role of a universal defense regulator. Antioxidants 9, 681. doi:10.3390/antiox9080681
Hassan, M. J., Zhang, G., Wu, F., Wei, K., and Chen, Z. (2005). Zinc alleviates growth inhibition and oxidative stress caused by cadmium in rice. J. Plant Nutrit. Soil Sci. 168, 255–261. doi:10.1002/jpln.200420403
Helaly, M. N., El-Metwally, M. A., El-Hoseiny, H., Omar, S. A., and El-Sheery, N. I. (2014). Effect of nanoparticles on biological contamination of'in vitro'cultures and organogenic regeneration of banana. Aust. J. Crop Sci. 8, 612–624.
Hou, M., Li, M., Yang, X., and Pan, R. (2019). Responses of nonprotein thiols to stress of vanadium and mercury in maize (Zea mays L.) seedlings. Bull. Environ. Contam. Toxicol. 102, 425–431. doi:10.1007/s00128-019-02553-w
Hussain, A., Ali, S., Rizwan, M., Zia Ur Rehman, M., Hameed, A., Hafeez, F., et al. (2018a). Role of zinc–lysine on growth and chromium uptake in rice plants under Cr stress. J. Plant Growth Regul. 37, 1413–1422. doi:10.1007/s00344-018-9831-x
Hussain, A., Ali, S., Rizwan, M., Zia Ur Rehman, M., Javed, M. R., Imran, M., et al. (2018b). Zinc oxide nanoparticles alter the wheat physiological response and reduce the cadmium uptake by plants. Environ. Pollut. 242, 1518–1526. doi:10.1016/j.envpol.2018.08.036
Hussain, M. B., Ali, S., Azam, A., Hina, S., Farooq, M. A., Ali, B., et al. (2013). Morphological, physiological and biochemical responses of plants to nickel stress: A review. Afr. J. Agric. Res. 8, 1596–1602. doi:10.5897/ajar12.407
Hussain, S., Maqsood, M. A., and Rahmatullah, M. (2010). Increasing grain zinc and yield of wheat for the developing world: A review. Emir. J. Food Agric., 22, 326–339. doi:10.9755/ejfa.v22i5.4821
Ibrahim, S., Saleem, B., Rehman, N., Zafar, S. A., Naeem, M. K., and Khan, M. R. (2022). CRISPR/Cas9 mediated disruption of Inositol Pentakisphosphate 2-Kinase 1 (TaIPK1) reduces phytic acid and improves iron and zinc accumulation in wheat grains. J. Advanc. Res. 37, 33–41. doi:10.1016/j.jare.2021.07.006
Ifie, J. E., Ifie-Etumah, S. O., and Ikhajiage, B. (2020). Physiological and biochemical responses of selected cowpea (Vigna unguiculata (L.) Walp.) accessions to iron toxicity. Acta Agric. Slov. 115 (1), 25–38.
Imran K, K., Seleiman, M. F., Chattha, M. U., Jalal, R. S., Mahmood, F., Hassan, F. A., et al. (2021). Enhancing antioxidant defense system of mung bean with a salicylic acid exogenous application to mitigate cadmium toxicity. Not. Bot. Horti Agrobot. Cluj. Napoca. 49, 12303. doi:10.15835/nbha49212303
Imran M, M., Hussain, S., He, L., Ashraf, M. F., Ihtisham, M., Warraich, E. A., et al. (2021). Molybdenum-induced regulation of antioxidant defense-mitigated cadmium stress in aromatic rice and improved crop growth, yield, and quality traits. Antioxidants 10, 838. doi:10.3390/antiox10060838
Janicka-Russak, M., Kabała, K., and Burzyński, M. (2012). Different effect of cadmium and copper on H+-ATPase activity in plasma membrane vesicles from Cucumis sativu s roots. J. Exp. Bot. 63, 4133–4142. doi:10.1093/jxb/ers097
Javed, H., Naeem, A., Rengel, Z., and Dahlawi, S. (2016). Timing of foliar Zn application plays a vital role in minimizing Cd accumulation in wheat. Environ. Sci. Pollut. Res. 23, 16432–16439. doi:10.1007/s11356-016-6822-y
Kalaivanan, D., and Ganeshamurthy, A. N. (2016). “Mechanisms of heavy metal toxicity in plants,” in Abiotic stress physiology of horticultural crops (Berlin Germany: Springer), 85–102.
Karmous, I., Gammoudi, N., and Chaoui, A. (2022). Assessing the potential role of zinc oxide nanoparticles for mitigating cadmium toxicity in Capsicum annuum L. under in vitro conditions. J. Plant Growth Regul. 1–16. doi:10.1007/s00344-022-10579-4
Kaur, N., Jagmeet, K., Grewal, S. K., and Inderjit, S. (2019). Effect of heat stress on antioxidative defense system and its amelioration by heat acclimation and salicylic acid pre-treatments in three pigeonpea genotypes. Indian J. Agric. Biochem. 32 (1), 106–110. doi:10.5958/0974-4479.2019.00014.5
Khan, M. A., Yasmin, H., Shah, Z. A., Rinklebe, J., Alyemeni, M. N., and Ahmad, P. (2022). Co application of biofertilizer and zinc oxide nanoparticles upregulate protective mechanism culminating improved arsenic resistance in maize. Chemosphere 294, 133796. doi:10.1016/j.chemosphere.2022.133796
Khan, M. I. R., Nazir, F., Asgher, M., Per, T. S., and Khan, N. A. (2015). Selenium and sulfur influence ethylene formation and alleviate cadmium-induced oxidative stress by improving proline and glutathione production in wheat. J. plant physiology 173, 9–18. doi:10.1016/j.jplph.2014.09.011
Khan, Z. S., Rizwan, M., Hafeez, M., Ali, S., Javed, M. R., and Adrees, M. (2019). The accumulation of cadmium in wheat (Triticum aestivum) as influenced by zinc oxide nanoparticles and soil moisture conditions. Environ. Sci. Pollut. Res. 26, 19859–19870. doi:10.1007/s11356-019-05333-5
Kim, H., Seomun, S., Yoon, Y., and Jang, G. (2021). Jasmonic acid in plant abiotic stress tolerance and interaction with abscisic acid. Agronomy 11, 1886. doi:10.3390/agronomy11091886
Kim, Y.-Y., Kim, D.-Y., Shim, D., Song, W.-Y., Lee, J., Schroeder, J. I., et al. (2008). Expression of the novel wheat gene TM20 confers enhanced cadmium tolerance to bakers' yeast. J. Biol. Chem. 283, 15893–15902. doi:10.1074/jbc.m708947200
Kolenčík, M., Ernst, D., Komár, M., Urík, M., Šebesta, M., Dobročka, E., et al. (2019). Effect of foliar spray application of zinc oxide nanoparticles on quantitative, nutritional, and physiological parameters of foxtail millet (Setaria italica l.) under field conditions. Nanomaterials 9, 1559. doi:10.3390/nano9111559
Komárek, M., Antelo, J., Králová, M., Veselská, V., Číhalová, S., Chrastný, V., et al. (2018). Revisiting models of Cd, Cu, Pb and Zn adsorption onto Fe (III) oxides. Chem. Geol. 493, 189–198. doi:10.1016/j.chemgeo.2018.05.036
Lane, B., Kajioka, R., and Kennedy, T. (1987). The wheat-germ Ec protein is a zinc-containing metallothionein. Biochem. Cell Biol. 65, 1001–1005. doi:10.1139/o87-131
Laware, S., and Raskar, S. (2014). Influence of zinc oxide nanoparticles on growth, flowering and seed productivity in onion. Int. J. Curr. Microbiol. Sci. 3, 874–881.
Le¡ Ková, A., Giehl, R. F., Hartmann, A., Farga¡ Ová, A., and Von Wirén, N. (2017). Heavy metals induce iron deficiency responses at different hierarchic and regulatory levels. Plant Physiol. 174, 1648–1668. doi:10.1104/pp.16.01916
Li, L., Zhang, Y., Ippolito, J. A., Xing, W., Qiu, K., and Wang, Y. (2020). Cadmium foliar application affects wheat Cd, Cu, Pb and Zn accumulation. Environ. Pollut. 262, 114329. doi:10.1016/j.envpol.2020.114329
Li, Z., Ma, Z., Van Der Kuijp, T. J., Yuan, Z., and Huang, L. (2014). A review of soil heavy metal pollution from mines in China: Pollution and health risk assessment. Sci. Total Environ. 468, 843–853. doi:10.1016/j.scitotenv.2013.08.090
Liu, Q.-L., Xu, X.-H., Ren, X.-L., Fu, H.-W., Wu, D.-X., and Shu, Q.-Y. (2007). Generation and characterization of low phytic acid germplasm in rice (Oryza sativa L.) Theor. Appl. Genet. 114, 803–814. doi:10.1007/s00122-006-0478-9
Liu, T., Man, Y., Li, P., Zhang, H., and Cheng, H. (2021). A hydroponic study on effect of zinc against mercury uptake by triticale: Kinetic process and accumulation. Bull. Environ. Contam. Toxicol. 108, 359–365.
Liu, W., Shang, S., Feng, X., Zhang, G., and Wu, F. (2015). Modulation of exogenous selenium in cadmium‐induced changes in antioxidative metabolism, cadmium uptake, and photosynthetic performance in the 2 tobacco genotypes differing in cadmium tolerance. Environ. Toxicol. Chem. 34, 92–99. doi:10.1002/etc.2760
Lokhande, V. H., Patade, V. Y., Srivastava, S., Suprasanna, P., Shrivastava, M., and Awasthi, G. (2020). Copper accumulation and biochemical responses of Sesuvium portulacastrum (L.) Mater. Today Proc. 31, 679–684. doi:10.1016/j.matpr.2020.07.117
Mapodzeke, J. M., Adil, M. F., Wei, D., Joan, H. I., Ouyang, Y., and Shamsi, I. H. (2021). Modulation of key physio-biochemical and ultrastructural attributes after synergistic application of zinc and silicon on rice under cadmium stress. Plants 10, 87. doi:10.3390/plants10010087
Mapodzeke, J. M., Sagonda, T., Sehar, S., Zhang, X., Huang, Y., Zvobgo, G., et al. (2018). Effects of zinc and silicon on cadmium toxicity and mineral element translocation in two rice (Oryza sativa) genotypes. J. Zhejiang Univ. 44, 294–310. doi:10.3785/j.issn.1008-9209.2017.12.131
Marrugo-Negrete, J., Durango-Hernández, J., Pinedo-Hernández, J., Enamorado-Montes, G., and Díez, S. (2016). Mercury uptake and effects on growth in Jatropha curcas. J. Environ. Sci. 48, 120–125. doi:10.1016/j.jes.2015.10.036
Medina, S., Collado-González, J., Ferreres, F., Londoño-Londoño, J., Jiménez-Cartagena, C., Guy, A., et al. (2017). Quantification of phytoprostanes–bioactive oxylipins–and phenolic compounds of Passiflora edulis Sims shell using UHPLC-QqQ-MS/MS and LC-IT-DAD-MS/MS. Food Chem. 229, 1–8. doi:10.1016/j.foodchem.2017.02.049
Mei, L., and Zhu, Y., Zhang, X., Zhou, X., Zhong, Z., Li, H., et al. (2021). Mercury-induced phytotoxicity and responses in upland cotton (Gossypium hirsutum L.) seedlings. Plants 10, 1494. doi:10.3390/plants10081494
Miller, D. D., and Welch, R. M. (2013). Food system strategies for preventing micronutrient malnutrition. Food Policy 42, 115–128. doi:10.1016/j.foodpol.2013.06.008
Mittler, R. (2017). ROS are good. Trends Plant Sci. 22 (1), 11–19. doi:10.1016/j.tplants.2016.08.002
Moreno-Lora, A., and Delgado, A. (2020). Factors determining Zn availability and uptake by plants in soils developed under Mediterranean climate. Geoderma 376, 114509. doi:10.1016/j.geoderma.2020.114509
Noman, A., Ali, Q., Maqsood, J., Iqbal, N., Javed, M. T., Rasool, N., et al. (2018). Deciphering physio-biochemical, yield, and nutritional quality attributes of water-stressed radish (Raphanus sativus L.) plants grown from Zn-Lys primed seeds. Chemosphere 195, 175–189. doi:10.1016/j.chemosphere.2017.12.059
Parlak, M., and Parlak, A. Ö. (2011). Effect of soil compaction on root growth and nutrient uptake of forage crops. J. Food Agric. Environ. 9, 275–278.
Poulson, B. G., Alsulami, Q. A., Sharfalddin, A., El Agammy, E. F., Mouffouk, F., Emwas, A. H., et al. (2021). Cyclodextrins: Structural, chemical, and physical properties, and applications. Polysaccharides 3 1, 1–31.
Paunov, M., Koleva, L., Vassilev, A., Vangronsveld, J., and Goltsev, V. (2018). Effects of different metals on photosynthesis: Cadmium and zinc affect chlorophyll fluorescence in durum wheat. Int. J. Mol. Sci. 19, 787. doi:10.3390/ijms19030787
Pavlova, D. (2017). Nickel effect on root-meristem cell division in Plantago lanceolata (Plantaginaceae) seedlings. Aust. J. Bot. 65, 446–452. doi:10.1071/bt17054
Pfeiffer, W. H., and Mcclafferty, B. (2007). HarvestPlus: Breeding crops for better nutrition. Crop Sci. 47, S-88–S-105. doi:10.2135/cropsci2007.09.0020ipbs
Priyanka, N., Geetha, N., Manish, T., Sahi, S., and Venkatachalam, P. (2021). Zinc oxide nanocatalyst mediates cadmium and lead toxicity tolerance mechanism by differential regulation of photosynthetic machinery and antioxidant enzymes level in cotton seedlings. Toxicology Rep. 8, 295–302. doi:10.1016/j.toxrep.2021.01.016
Qaswar, M., Hussain, S., and Rengel, Z. (2017). Zinc fertilisation increases grain zinc and reduces grain lead and cadmium concentrations more in zinc-biofortified than standard wheat cultivar. Sci. Total Environ. 605, 454–460. doi:10.1016/j.scitotenv.2017.06.242
Qiao, K., Liang, S., Wang, F., Wang, H., Hu, Z., and Chai, T. (2019). Effects of cadmium toxicity on diploid wheat (Triticum urartu) and the molecular mechanism of the cadmium response. J. Hazard. Mater. 374, 1–10. doi:10.1016/j.jhazmat.2019.04.018
Qu, L. Q., Yoshihara, T., Ooyama, A., Goto, F., and Takaiwa, F. (2005). Iron accumulation does not parallel the high expression level of ferritin in transgenic rice seeds. Planta 222, 225–233. doi:10.1007/s00425-005-1530-8
Raja, V., Majeed, U., Kang, H., Andrabi, K. I., and John, R. (2017). Abiotic stress: interplay between ROS, hormones and MAPKs. Environ. Exp. Bot. 137, 142–157. doi:10.1016/j.envexpbot.2017.02.010
Rajiv, P., Vanathi, P., and Thangamani, A. (2018). An investigation of phytotoxicity using Eichhornia mediated zinc oxide nanoparticles on Helianthus annuus. Biocatal. Agric. Biotechnol. 16, 419–424. doi:10.1016/j.bcab.2018.09.017
Rath, B. P., Hota, S., Subhadarshini, S., Dash, D., and Das, P. K. (2019). Consequence of chromium-tainted soil on physical and biochemical responses of Vigna radiata L. J. Appl. Biol. Biotechnol. 7, 3–1. doi:10.7324/JABB.2019.70107
Raza, A. (2020). Metabolomics: A systems biology approach for enhancing heat stress tolerance in plants. Plant Cell Rep. 41, 741–763. doi:10.1007/s00299-020-02635-8
Rizwan, M., Ali, S., Ali, B., Adrees, M., Arshad, M., Hussain, A., et al. (2019). Zinc and iron oxide nanoparticles improved the plant growth and reduced the oxidative stress and cadmium concentration in wheat. Chemosphere 214, 269–277. doi:10.1016/j.chemosphere.2018.09.120
Rizwan, M., Ali, S., Hussain, A., Ali, Q., Shakoor, M. B., Zia-Ur-Rehman, M., et al. (2017). Effect of zinc-lysine on growth, yield and cadmium uptake in wheat (Triticum aestivum L.) and health risk assessment. Chemosphere 187, 35–42. doi:10.1016/j.chemosphere.2017.08.071
Rucińska-Sobkowiak, R. (2016). Water relations in plants subjected to heavy metal stresses. Acta Physiol. Plant. 38, 257–313. doi:10.1007/s11738-016-2277-5
Sadak, M. S., and Bakry, B. A. (2020). Zinc-oxide and nano ZnO oxide effects on growth, some biochemical aspects, yield quantity, and quality of flax (Linum uitatissimum L.) in absence and presence of compost under sandy soil. Bull. Natl. Res. Cent. 44, 98–12. doi:10.1186/s42269-020-00348-2
Sadeghzadeh, B. (2013). A review of zinc nutrition and plant breeding. J. soil Sci. plant Nutr. 13, 905–927. doi:10.4067/S0718-95162013005000072
Salam, A., Khan, A. R., Liu, L., Yang, S., Azhar, W., Ulhassan, Z., et al. (2022). Seed priming with zinc oxide nanoparticles downplayed ultrastructural damage and improved photosynthetic apparatus in maize under cobalt stress. J. Hazard. Mater. 423, 127021. doi:10.1016/j.jhazmat.2021.127021
Salama, D. M., Osman, S. A., Abd El-Aziz, M., Abd Elwahed, M. S., and Shaaban, E. (2019). Effect of zinc oxide nanoparticles on the growth, genomic DNA, production and the quality of common dry bean (Phaseolus vulgaris). Biocatal. Agric. Biotechnol. 18, 101083. doi:10.1016/j.bcab.2019.101083
Saleem, M. H., Fahad, S., Rehman, M., Saud, S., Jamal, Y., Khan, S., and Liu, L. (2020). Morpho-physiological traits, biochemical response and phytoextraction potential of short-term copper stress on kenaf (Hibiscus cannabinus L.) seedlings. PeerJ, 8, e8321. doi:10.7717/peerj.8321
Sangwan, P., Kumar, V., and Joshi, U. (2014). Effect of chromium (VI) toxicity on enzymes of nitrogen metabolism in clusterbean (Cyamopsis tetragonoloba L.) Enzyme rResearch 2014, 784036. doi:10.1155/2014/784036
Sanjosé, I., Navarro-Roldán, F., Infante-Izquierdo, M. D., Martínez-Sagarra, G., Devesa, J. A., Polo, A., et al. (2021). Accumulation and effect of heavy metals on the germination and growth of Salsola vermiculata L. Seedlings. Diversity 13, 539. doi:10.3390/d13110539
Sarwar, N., Bibi, S., Ahmad, M., and Ok, Y. S. (2014). Effectiveness of zinc application to minimize cadmium toxicity and accumulation in wheat (Triticum aestivum L.) Environ. Earth Sci. 71, 1663–1672. doi:10.1007/s12665-013-2570-1
Sasaki, A., Yamaji, N., Yokosho, K., and Ma, J. F. (2012). Nramp5 is a major transporter responsible for manganese and cadmium uptake in rice. Plant Cell 24, 2155–2167. doi:10.1105/tpc.112.096925
Schmidt, S. B., Eisenhut, M., and Schneider, A. (2020). Chloroplast transition metal regulation for efficient photosynthesis. Trends Plant Sci. 25, 817–828. doi:10.1016/j.tplants.2020.03.003
Shah, A. A., Khan, W. U., Yasin, N. A., Akram, W., Ahmad, A., Abbas, M., et al. (2020). Butanolide alleviated cadmium stress by improving plant growth, photosynthetic parameters and antioxidant defense system of Brassica oleracea. Chemosphere 261, 127728. doi:10.1016/j.chemosphere.2020.127728
Shahzad, R., Jamil, S., Ahmad, S., Nisar, A., Khan, S., Amina, Z., et al. (2021). Biofortification of cereals and pulses using new breeding techniques: Current and future perspectives. Front. Nutr. 665, 721728. doi:10.3389/fnut.2021.721728
Shalaby, T., Bayoumi, Y., Alshaal, T., Elhawat, N., Sztrik, A., and El-Ramady, H. (2017). Selenium fortification induces growth, antioxidant activity, yield and nutritional quality of lettuce in salt-affected soil using foliar and soil applications. Plant Soil 421, 245–258. doi:10.1007/s11104-017-3458-8
Sharfalddin, A. A., Emwas, A. H., Jaremko, M., and Hussien, M. A. (2021). Transition metal complexes of 6–mercaptopurine: Characterization, Theoretical calculation, DNA–Binding, molecular docking, and anticancer activity. Appl. Organometallic Chem. 35 (1), e6041.
Singh, S., Parihar, P., Singh, R., Singh, V. P., and Prasad, S. M. (2016). Heavy metal tolerance in plants: Role of transcriptomics, proteomics, metabolomics, and ionomics. Front. Plant Sci. 6, 1143. doi:10.3389/fpls.2015.01143
Song, X., Zhang, C., Chen, W., Zhu, Y., and Wang, Y. (2020). Growth responses and physiological and biochemical changes in five ornamental plants grown in urban lead‐contaminated soils. Plant-Environment Interact. 1, 29–47. doi:10.1002/pei3.10013
Sperdouli, I., Adamakis, I.-D. S., Dobrikova, A., Apostolova, E., Hanć, A., and Moustakas, M. (2022). Excess zinc supply reduces cadmium uptake and mitigates cadmium toxicity effects on chloroplast structure, oxidative stress, and photosystem II photochemical efficiency in salvia sclarea plants. Toxics 10, 36. doi:10.3390/toxics10010036
Srivastava, R. K., Pandey, P., Rajpoot, R., Rani, A., and Dubey, R. (2014). Cadmium and lead interactive effects on oxidative stress and antioxidative responses in rice seedlings. Protoplasma 251, 1047–1065. doi:10.1007/s00709-014-0614-3
Sultan, I., Khan, I., Chattha, M. U., Hassan, M. U., Barbanti, L., Calone, R., et al. (2021). Improved salinity tolerance in early growth stage of maize through salicylic acid foliar application. Ital. J. Agron. 16. 1810. doi:10.4081/ija.2021.1810
Sundaramoorthy, P., Chidambaram, A., Ganesh, K. S., Unnikannan, P., and Baskaran, L. (2010). Chromium stress in paddy:(i) nutrient status of paddy under chromium stress; (ii) phytoremediation of chromium by aquatic and terrestrial weeds. Comptes rendus Biol. 333, 597–607. doi:10.1016/j.crvi.2010.03.002
Syed, M., Iftekharuddaula, K., Mian, M., Rasul, M., Rahmam, G., Panaullah, G. M., et al. (2016). Main effect QTLs associated with arsenic phyto-toxicity tolerance at seedling stage in rice (Oryza sativa L.) Euphytica 209, 805–814. doi:10.1007/s10681-016-1683-5
Tavallali, V. (2017). Interactive effects of zinc and boron on growth, photosynthesis, and water relations in pistachio. J. Plant Nutr. 40, 1588–1603. doi:10.1080/01904167.2016.1270308
Tchounwou, P. B., Yedjou, C. G., and Patlolla, A. K. and Sutton, D. J. (2012). Heavy metal toxicity and the environment. Mol. Clin. Environ. Toxicol. 101, 133–164. doi:10.1007/978-3-7643-8340-4_6
Teklić, T., Lončarić, Z., Kovačević, V., and Singh, B. R. (2013). Metallic trace elements in cereal grain–a review: How much metal do we eat? Food Energy secur. 2, 81–95. doi:10.1002/fes3.24
Thakur, N., Chrungoo, S., Rana, S., Kaur, S., Kaur, S., and Pathak, A. (2021). Effect Of Copper Nanoparticles On In-Vitro Seed Germination Of Wheat (Triticum Aestivum L.) Varieties. Ijpsr. Int. J. Pharmaceut. Sci. Res. 12 (8), 4307–4313. doi:10.13040/IJPSR.0975-8232
Thounaojam, T. C., Panda, P., Choudhury, S., Patra, H. K., and Panda, S. K. (2014). Zinc ameliorates copper-induced oxidative stress in developing rice (Oryza sativa L.) seedlings. Protoplasma 251, 61–69. doi:10.1007/s00709-013-0525-8
Torabian, S., Zahedi, M., and Khoshgoftarmanesh, A. (2018). Effect of foliar spray of zinc oxide on some antiox idant enzymes activity of sunflower under salt stress. J. Agric. Sci. Technol. 18 (4), 1013–1025. https://jast.modares.ac.ir/article-23-5061-en.html
Turan, V., Ramzani, P. M. A., Ali, Q., Abbas, F., Iqbal, M., Irum, A., et al. (2018). Alleviation of nickel toxicity and an improvement in zinc bioavailability in sunflower seed with chitosan and biochar application in pH adjusted nickel contaminated soil. Archi. Agron. Soil Sci. 64, 1053–1067. doi:10.1080/03650340.2017.1410542
Ul-Hassan, Z., Ali, S., Rizwan, M., Hussain, A., Akbar, Z., Rasool, N., et al. (2017). Role of zinc in alleviating heavy metal stress. Berlin/Heidelberg, Germany: Springer.
Umer Chattha, M., Arif, W., Khan, I., Soufan, W., Bilal Chattha, M., Hassan, M. U., et al. (2021). Mitigation of cadmium induced oxidative stress by using organic amendments to improve the growth and yield of mash beans [Vigna mungo (L.)]. Agronomy 11, 2152. doi:10.3390/agronomy11112152
Ur Rehman, M. Z., Rizwan, M., Rauf, A., Ayub, M. A., Ali, S., Qayyum, M. F., et al. (2019). Split application of silicon in cadmium (Cd) spiked alkaline soil plays a vital role in decreasing Cd accumulation in rice (Oryza sativa L.) grains. Chemosphere 226, 454–462. doi:10.1016/j.chemosphere.2019.03.182
Uraguchi, S., Kamiya, T., Sakamoto, T., Kasai, K., Sato, Y., Nagamura, Y., et al. (2011). Low-affinity cation transporter (OsLCT1) regulates cadmium transport into rice grains. Proc. Natl. Acad. Sci. U. S. A. 108, 20959–20964. doi:10.1073/pnas.1116531109
Vaculík, M., Konlechner, C., Langer, I., Adlassnig, W., Puschenreiter, M., Lux, A., et al. (2012). Root anatomy and element distribution vary between two Salix caprea isolates with different Cd accumulation capacities. Environ. Pollut. 163, 117–126. doi:10.1016/j.envpol.2011.12.031
Venkatachalam, P., Priyanka, N., Manikandan, K., Ganeshbabu, I., Indiraarulselvi, P., Geetha, N., et al. (2017). Enhanced plant growth promoting role of phycomolecules coated zinc oxide nanoparticles with P supplementation in cotton (Gossypium hirsutum L.) Plant Physiol. biochem. 110, 118–127. doi:10.1016/j.plaphy.2016.09.004
Verma, V., Vishal, B., Kohli, A., and Kumar, P. P. (2021). Systems-based rice improvement approaches for sustainable food and nutritional security. Plant Cell Rep. 40 (11), 2021–2036. doi:10.1007/s00299-021-02790-6
Wa-Lwalaba, J. L., Zvogbo, G., Mulembo, M., Mundende, M., and Zhang, G. (2017). The effect of cobalt stress on growth and physiological traits and its association with cobalt accumulation in barley genotypes differing in cobalt tolerance. J. Plant Nutrit. 40, 2192–2199. doi:10.1080/01904167.2017.1346676
Wang, Q., Kong, X.-P., Zhang, B.-H., and Wang, J. (2017). Adsorption of Zn (II) on the kaolinite (001) surfaces in aqueous environment: A combined dft and molecular dynamics study. Appl. Surf. Sci. 414, 405–412. doi:10.1016/j.apsusc.2017.04.062
Wu C, C., Dun, Y., Zhang, Z., Li, M., and Wu, G. (2020). Foliar application of selenium and zinc to alleviate wheat (Triticum aestivum L.) cadmium toxicity and uptake from cadmium-contaminated soil. Ecotoxicol. Environ. Saf. 190, 110091. doi:10.1016/j.ecoenv.2019.110091
Wu F, F., Fang, Q., Yan, S., Pan, L., Tang, X., and Ye, W. (2020). Effects of zinc oxide nanoparticles on arsenic stress in rice (Oryza sativa L.): Germination, early growth, and arsenic uptake. Environ. Sci. Pollut. Res. 27, 26974–26981. doi:10.1007/s11356-020-08965-0
Xiao, R., Wang, S., Li, R., Wang, J. J., and Zhang, Z. (2017). Soil heavy metal contamination and health risks associated with artisanal gold mining in Tongguan, Shaanxi, China. Ecotoxicol. Environ. Saf. 141, 17–24. doi:10.1016/j.ecoenv.2017.03.002
Xie, X., Hu, W., Fan, X., Chen, H., and Tang, M. (2019). Interactions between phosphorus, zinc, and iron homeostasis in nonmycorrhizal and mycorrhizal plants. Front. Plant Sci., 10, 1172. doi:10.3389/fpls.2019.01172
Yadav, R., Patel, A., Dodia, I., Aglodiya, A., Patel, G., and Augustine, N. (2011). Agronomic bio-fortification of wheat (Triticum aestivum L.) through iron and zinc enriched organics. J. Wheat Res. 3, 46–51.
Yadav, S. K., Singla-Pareek, S. L., Reddy, M., and Sopory, S. (2005). Methylglyoxal detoxification by glyoxalase system: A survival strategy during environmental stresses. Physiology Mol. Biol. Plants 11, 1.
Yan, S., Wu, F., Zhou, S., Yang, J., Tang, X., and Ye, W. (2021). Zinc oxide nanoparticles alleviate the arsenic toxicity and decrease the accumulation of arsenic in rice (Oryza sativa L.) BMC Plant Biol. 21, 150–211. doi:10.1186/s12870-021-02929-3
Yang, X. W., Tian, X. H., Lu, X. C., Cao, Y. X., and Chen, Z. H. (2011). Impacts of phosphorus and zinc levels on phosphorus and zinc nutrition and phytic acid concentration in wheat (Triticum aestivum L.) J. Sci. Food Agric. 91, 2322–2328. doi:10.1002/jsfa.4459
Yang, Y., Zhang, L., Huang, X., Zhou, Y., Quan, Q., Li, Y., et al. (2020). Response of photosynthesis to different concentrations of heavy metals in Davidia involucrata. PLoS One 15, e0228563. doi:10.1371/journal.pone.0228563
Zaheer, I. E., Ali, S., Rizwan, M., Bareen, F.-E., Abbas, Z., Bukhari, S. a. H., et al. (2019). Zinc-lysine prevents chromium-induced morphological, photosynthetic, and oxidative alterations in spinach irrigated with tannery wastewater. Environ. Sci. Pollut. Res. 26, 28951–28961. doi:10.1007/s11356-019-06084-z
Zaheer, I. E., Ali, S., Saleem, M. H., Arslan Ashraf, M., Ali, Q., Abbas, Z., et al. (2020). Zinc-lysine supplementation mitigates oxidative stress in rapeseed (Brassica napus L.) by preventing phytotoxicity of chromium, when irrigated with tannery wastewater. Plants 9, 1145. doi:10.3390/plants9091145
Zaheer, I. E., Ali, S., Saleem, M. H., Yousaf, H. S., Malik, A., Abbas, Z., et al. (2022). Combined application of zinc and iron-lysine and its effects on morpho-physiological traits, antioxidant capacity and chromium uptake in rapeseed (Brassica napus L.) PloS one 17, e0262140. doi:10.1371/journal.pone.0262140
Zainab, N., Khan, A. A., Azeem, M. A., Ali, B., Wang, T., Shi, F., et al. (2021). PGPR-mediated plant growth attributes and metal extraction ability of Sesbania sesban L. In industrially contaminated soils. Agronomy 11, 1820. doi:10.3390/agronomy11091820
Zhou, J., Zhang, C., Du, B., Cui, H., Fan, X., Zhou, D., et al. (2020). Effects of zinc application on cadmium (Cd) accumulation and plant growth through modulation of the antioxidant system and translocation of Cd in low-and high-Cd wheat cultivars. Environ. Pollut. 265, 115045. doi:10.1016/j.envpol.2020.115045
Zhou, Z., Zhang, B., Liu, H., Liang, X., Ma, W., Shi, Z., et al. (2019). Zinc effects on cadmium toxicity in two wheat varieties (Triticum aestivum L.) differing in grain cadmium accumulation. Ecotoxicol. Environ. Saf. 183, 109562. doi:10.1016/j.ecoenv.2019.109562
Zia-Ur-Rehman, M., Sabir, M., Rizwan, M., Saifullah Ahmed, H., Nadeem, M. H., and Ahamed, H. R. (2015). “Remediating cadmium-contaminated soils by growing grain crops using inorganic amendments,”. Editors K. R. Hakeem, M. Sabir, M. Ozturk, and A. Murmet, Soil remediation plants: Prospects challenges, 367–396. doi:10.1016/B978-0-12-799937-1.00013-9
Keywords: anti-oxidants, breeding techniques, gene expression, heavy metals, stress, nutrient uptake, photosynthetic performance, zinc fertilization
Citation: Hassan MU, Nawaz M, Mahmood A, Shah AA, Shah AN, Muhammad F, Batool M, Rasheed A, Jaremko M, Abdelsalam NR, Hasan ME and Qari SH (2022) The role of zinc to mitigate heavy metals toxicity in crops. Front. Environ. Sci. 10:990223. doi: 10.3389/fenvs.2022.990223
Received: 09 July 2022; Accepted: 26 August 2022;
Published: 06 October 2022.
Edited by:
Muhammad Azeem, Institute of Urban Environment (CAS), ChinaReviewed by:
Sandeep K. Malyan, National Institute of Hydrology, IndiaPankaj Kumar, Gurukul Kangri Vishwavidyalaya, India
Copyright © 2022 Hassan, Nawaz, Mahmood, Shah, Shah, Muhammad, Batool, Rasheed, Jaremko, Abdelsalam, Hasan and Qari. This is an open-access article distributed under the terms of the Creative Commons Attribution License (CC BY). The use, distribution or reproduction in other forums is permitted, provided the original author(s) and the copyright owner(s) are credited and that the original publication in this journal is cited, in accordance with accepted academic practice. No use, distribution or reproduction is permitted which does not comply with these terms.
*Correspondence: Adnan Noor Shah, ans.786@yahoo.com
†These authors have contributed equally to this work