- 1State Key Laboratory of Cotton Biology, School of Agricultural Sciences, Institute of Cotton Research of Chinese Academy of Agricultural Sciences/Research Base, Zhengzhou University, Anyang, Henan, China
- 2Hebei Academy of Agricultural and Forestry Sciences/Hebei Key Laboratory of Crops Drought Resistance, Dryland Farming Institute, Hengshui, Hebei, China
Drought stress is one of the abiotic stresses that limits crop production and greatly affects crop yield. Enhancement of plant stress resistance by NaCl pretreatment has been reported, but the mechanism by which NaCl pretreatment activates cotton stress resistance remains unclear. In this study, upland cotton (Gossypium hirsutum cv H177) was used as the material to conducted the treatments with three replications: 0 Mm NaCl + 0% PEG6000 (Polyethylene glycol), 0 mM NaCl + 15% PEG6000, 50 mM NaCl + 15% PEG6000 to explore the molecular mechanism by which NaCl improves the drought tolerance of cotton. The results showed that pretreatment with 50 mM NaCl could alleviate the adverse effects of PEG on cotton seeds while promoting the elongation of root length. RNA-seq showed that NaCl specifically induced the expression of carotenoid-related genes. By silencing the upstream gene GHLUT2 of lutein synthesis, it was found that the chlorophyll of silenced plants decreased, and leaf wilting was more sensitive to drought. We found that NaCl enhanced the drought resistance of cotton by regulating genes related to the carotenoid and abscisic acid downstream synthesis pathways. This study provides a new reference for the study of drought resistance in cotton and a theoretical basis for the molecular breeding of cotton.
Introduction
Cotton is the most important fiber crop in the world, and cotton cultivation plays an important role in the national economy. Plants suffer from various environmental stresses during their growth, and drought is one of the main abiotic stress factors affecting crop yield (Rasheed et al., 2020). At present, 43% of the world’s arable land is in arid and semiarid areas, and there is an increasing trend (Shukla et al., 2014). Drought can lead to dramatic changes in the physiological and biochemical activities of most plants, including photosynthesis, increas tion, thus increasing proline and some osmotic substances (Okunlola et al., 2017; Kudla et al., 2018). When crops suffer from severe drought, lipid peroxidation, protein, photosynthetic systems and cell membranes are damaged, leading to a serious decrease in yield (Seki et al., 2007). Previous studies have shown that NaCl pretreatment can improve drought resistance of cotton, but the molecular mechanism is still unclear (Fan et al., 2020). This study provides a new reference for drought resistance mechanism by exploring the molecular mechanism of NaCl pretreatment to improve drought resistance of cotton. At present, transgenic breeding and exogenous application of osmotic regulators are the main ways to improve plant drought resistance (Rajasekaran and Blake, 1999) Due to its simplicity and efficiency, the application of exogenously applied regulators in the early stage of plant growth and development can be regarded as a way to regulate plant stress resistance. Such as α-Linolenic acid (ALA), NaCl and melatonin, have been widely used to improve the drought resistance of crops. (Fan et al., 2020; Li et al., 2020; Li et al., 2021; Zhang et al., 2021).
High concentrations of Na+ inhibit plant growth. However, salt stress is not always negative. Studies have shown that low concentrations of NaCl can act as catalysts to promote auxin synthesis. Gibberellins and endogenous growth regulators were significantly increased in pea seedlings after peas were treated with 50 mM NaCl and eliminated the damage of cell dehydration and ion stress due to reduced water supply (El-Mashad and Kamel, 2001). Low concentrations of NaCl can also alleviate the effects of abiotic stress, and the application of low concentrations of NaCl (5–10 mM) to the foliage can promote the increase in soluble sugar and water content in cucumber seedlings. Low concentrations of NaCl are also stress regulators that increase plant germination. The germination rate, germination potential, and radicle length were significantly improved in the seedling-treated groups after 7 days of germination under water stress compared with the group pretreated with low concentrations of NaCl (Cao et al., 2018). During seed germination, the osmotic potential of the seedlings pretreated with NaCl was significantly lower than that of the untreated seedlings, which may be related to the significantly higher content of soluble sugar and free proline in the seedlings pretreated with NaCl. Low concentrations of NaCl can be used as signaling mediators to activate antioxidants. NaCl induced a higher carotenoid content and improved the antioxidant capacity in yellow maize sprouts (He et al., 2020). Upregulation of carotenoid biosynthesis genes promoted carotenoid accumulation.
Carotenoids are fat soluble pigments found in plant chromoplasts and play an important role in drought resistance in higher plants. Some studies have reported that carotenoids can improve plant tolerance to stress (Khoo et al., 2011). Phytoene synthase (PSY) is the first rate-limiting enzyme in the biosynthetic pathway of carotenoids. Salt stress tolerance occurs due to the joint involvement of the DcPSY2 promoter and the transcription factor AREB (Kevin et al., 2018). Carotenoids are essential in different plant growth processes and are potential antioxidants during plant stress (Zhao et al., 2021). The effects of abiotic stress can be alleviated by NaCl treatment of seeds or regulation of carotenoids. Carotenoids enhanced the resistance of carrot to drought by scavenging ROS, increasing lutein cycle synthesis and dissipating excess light energy. Studies have shown that epoxy carotenoid synthesis can also improve plant tolerance to drought, and the 9-cis-epoxy carotenoid dioxygenase (NCED) gene in multiple species can allow plants to accumulate higher contents. In many species, the NCED gene resulted in higher ABA accumulation in plants (Frey et al., 2006).
In this study, upland cotton (Gossypium hirsutum cv H177) was used as the test material, and 50 mM NaCl pretreatment was carried out to explore the effect of NaCl pretreatment on drought resistance of cotton at germination stage. We then analyzed differentially expressed genes (DEGs) related to drought resistance in cotton pretreated with NaCl by RNA-Seq. NaCl induced the expression of carotenoid and ABA-related genes. A highly differentially expressed gene GHLUT2 was identified in the carotenoid synthesis pathway by RNA-seq. In order to investigate the role of GHLUT2 in response to drought stress, we investigated the function of GHLUT2 using VIGS technique at three-leaf stage of cotton, which method was widely used by researchers. By inhibiting the expression of the lutein synthesis gene GHLUT2, it was found that silenced plants were more sensitive to drought stress, demonstrating that NaCl affects carotenoid-related genes and improves cotton resistance to PEG. This study provides a basis for further study of drought resistance of cotton. Through the transcriptome analysis of NaCl pretreatment to improve drought resistance of cotton, a new understanding of plant drought resistance mechanism was obtained, and a new reference was provided for improving seed germination and drought resistance in arid areas.
Methods and materials
Plant material and NaCl pretreatment conditions at germination stage
To explore the appropriate concentration of NaCl pretreatment to improve the drought resistance of cotton, we carried out experiments using upland cotton drought-resistant cv H177 as the experiment material, a drought-resistant upland cotton strain preserved in our laboratory, which was identified as drought-resistant upland cotton strain. (Lu et al., 2019). Seeds of the same size were selected for gradient experiments with different concentrations (0 mM, 50 mM, 100 mM, 200 mM and 300 mM) of NaCl pretreatment. NaCl concentration of 50 mM was determined as the pretreatment condition. 150 delinted cotton seeds of uniform size were divided into three replicates of 50 seeds each. Cotton seeds of uniform size were placed in 50 mM NaCl solution for 6 h, then treated with 15% PEG 6000 solution and germinated at 25°C for 72 h in the dark. PEG (Polyethylene glycol) is usually used as the means of simulating drought (Lawlor, 1970). PEG (Polyethylene glycol) has been widely used to simulate drought stress with limited metabolic interferences because it is less likely to be absorbed by plants and is not phytotoxic (Shi et al., 2015). To observe the effects of different pretreatments on cotton germination, three biological replicates were set up by soaking in 0 mM and 50 mM NaCl for 6 h. Whole plant samples of germinated seedlings were collected and frozen in liquid nitrogen at −80°C for transcriptome analysis.
cDNA library preparation and transcriptome sequencing
Samples taken from the germination stage were prepared for the next experiment. Visualization and gel recovery on a 1% agarose gel. Advanced molecular biology equipment was used to detect the purity, concentration and integrity of RNA samples to ensure that qualified samples were used for transcriptome sequencing. Total RNA was extracted according to the instructions provided by the RNA-Seq sample preparation kit (Tiangen, Beijing, China), and the RNA concentration was detected using a nanophotometer spectrophotometer (IMPLEN, Westlake Village, CA, United States). First, the mRNA of cotton tissue was enriched with magnetic beads with Oligo (dT); then, Fragmentation Buffer was added to randomly interrupt the mRNA. Using mRNA as a template, the cDNA was purified using AMPure XP beads, the first cDNA chain was synthesized with random hexamers, and the second cDNA chain was synthesized by adding buffer, dNTPs, RNase H and DNA polymerase I. The cDNA was purified by AMPure XP beads, and finally, a cDNA library was constructed by PCR.
Sequencing by sequencing by synthesis (SBS) technology produces a large amount of high-quality data. After the library was constructed, the q-PCR method was used for detection again, and the effective concentration of the library was 2 nM for accurate quantitative analysis to ensure the quality of the library. The cDNA library was sequenced based on the Illumina high-throughput sequencing platform, which is called raw data (Raw Data). Raw data are usually provided in FASTQ format, and the raw data of each sequenced sample are shown. The sequencing base quality values of the data are usually expressed as Q10, Q20, and Q30. A higher Q value indicates a higher accuracy. After all libraries were qualified, bioinformatics analysis provided by BMKCloud (www.biocloud.net) was used.
The raw data were filtered to obtain clean data, sequence alignment was performed with the designated reference genome to obtain mapped data, and library quality assessments were performed, such as insert fragment randomness tests and data saturation tests. Alternative splicing analysis, new gene analysis and gene structure level analysis were then performed. Subsequently, expression level analyses were performed, such as differential expression calculation, functional annotation and functional enrichment of differentially expressed genes according to the expression level of genes in different treatments or different sample groups. Next, DEseq R software was used to analyze the differential expression of the two treatment groups, and the number of genes obtained by sequencing was tested for saturation and difference. After analyzing the data of the three samples, a set of differentially expressed genes (DEGs) between the two groups was obtained. During the detection of differentially expressed genes, fold change ≥ 2 and FDR < 0.01 were used as screening criteria, and the GOSeq R software package was used to annotate functional genes and correct gene length deviations (Young et al., 2010). As a data repository, KEGG includes the metabolism of carbohydrates, nucleosides, amino acids, etc, and the biodegradation of organic matter. It not only provides advanced functions and utilities of biological systems, such as annotations of cells, organisms, and ecosystems but also contains amino acids. Sequences, links to PDB libraries, etc., are powerful tools for in vivo metabolic analysis and metabolic network research (Minoru et al., 2008). Finally, we used KOBAS software to perform statistical and visual analyses of DEG enrichment (Mao et al., 2005).
Real-time PCR
Fifteen different genes were randomly selected, and the same sample was used to perform real-time PCR to verify the RNA-Seq data. Total RNA was extracted with an EASYspin Plus plant RNA rapid isolation kit (Aidlab Co., Ltd. Beijing, China). The pure RNA was reverse-transcribed using Transcript United States II one-step gDNA removal and cDNA synthesis supermix (TransGen Biotech Co., Ltd. Beijing, China) according to the manufacturer’s instructions. Primer Premier 6 software was used to design gene-specific primers. Details of the primers are shown in Supplementary Table S1. qRT‒PCR assays were performed on the LightCycler 480 Systemast fluorescence quantitative PCR platform with TransStartR top green q-PCR super mix (TransGen Biotech Co., Ltd., Beijing, China) in accordance with the manufacturer’s protocol (Livak and Schmittgen, 2002). Three biological replicates were used, and the 2−ΔΔCT method was used to measure the relative expression level of genes. GhUBQ7 was used as an internal control; it is stably expressed in cotton plants and is not affected by treatment and genotype. Correlation analysis was performed between qRT‒PCR and RNA-seq (Tan et al., 2013).
Virus induced gene silencing analysis of target gene at three-leaf stage
Previous studies have shown that VIGS technology is widely used in cotton (Zhang et al., 2021). In order to investigate the role of GHLUT2 gene under drought stress, we used VIGS technology to silence GHLUT2 atthe three-leaf stage (Xu et al., 2022). Three treatments are designed positive control (CK), positive control (156 + PEG), cotton plants with GHLUT2 gene silenced (LUT + PEG). Using upland cotton cultivar cv H177 as the test material, cotton seeds were planted on a sandy substrate in an incubator at 25°C for 16 h light and 8 h dark. Cotton GHLUT2 (GH_D05G0316) was deduced from AtLCY-ε (AT5G57030) as a reference gene observed in Arabidopsis. The vector selected is the pYL156 vector maintained by our laboratory. After selecting BamHI and SacI restriction enzymes for double digestion, the online tool SGN-VIGS 2 was used to design a 300-bp silenced fragment size. The in-Fusion primers for GHLUT2 were manually designed, while the primer-silencing fragment for GHLUT2 was as follows:
Forward primer AGAAGGCCTCCATGGGGATCCAGGAAACTTGTTTGGCCT; Reverse primer TGCCGGGCCTCGAGACGCGTGAGCTCTAAGCAAGCCATTAGA. Using cotton seedling cDNA as a template, the silent fragment was amplified, and the VIGS expression vector pYL156:GHLUT2 was constructed by in-Fusion ligation technology. The correctly sequenced expression vector was transformed into E. coli and then Agrobacterium using the freeze‒thaw method. The pYL156:PDS and LUT silencing vectors were mixed 1:1 with the helper vector pYL192. Since silencing of the phytoene desaturase gene (PDS) can lead to leaf albinism, the efficiency of silencing can be verified by observing whether VIGS with pYL156:PDS has true leaf albinism and then injecting Agrobacterium suspensions into 7-day-old plants. The cotton was treated with 15% PEG6000 when it grew to the three-leaf stage.
Result
Germination under drought stress after NaCl pretreatment
We observed that both 50 mM NaCl and 100 mM NaCl can improve the drought resistance of cotton seedlings. After careful rescreening of 50, 75, and 100 mM NaCl, the optimal screening concentration and 6-hour duration of 50 mM NaCl were finally determined to be suitable pretreatment conditions. Additionally, the time of pretreatment was screened, and it was observed that the germination potential of seedlings pretreated for 6 h was much higher than that of seedlings pretreated for 3 h and 9 h (Supplementary Figure S1). Different responses to drought stress were observed in NaCl pretreated and control seedlings CK (0 Mm NaCl + 0% PEG6000 Figure 1B), S (50 mM NaCl + 0% PEG6000 Figure 1C) P (0 Mm NaCl +15% PEG6000 Figure 1D), S-P (50 mM NaCl +15% PEG6000 Figure 1E). As a good drought-resistant product, we chose cv H177 as our drought-resistant plant material. Cotton cv H177 was affected by different concentrations of NaCl during germination, and it was found that the seedlings exposed to 50 mM NaCl showed the most pronounced performance after 6 h (Figure 1A). Therefore, we chose 50 mM NaCl to treat cotton seeds at the germination stage for transcriptome sequencing. On the other hand, a treatment with the same concentration of PEG was used to compare the effects of P and S-P. The phenotypic changes of the two treatments are shown. Under PEG stress after 72 h, the radicle was wilted and bent, the hypocotyl and root length were obviously shortened, and some seeds could not germinate. However, under 50 mM NaCl pretreatment, the root length was significantly elongated, and the radicle was not significantly shortened, indicating that the 50 mM NaCl pretreatment seedlings suffered less damage than those under PEG stress.
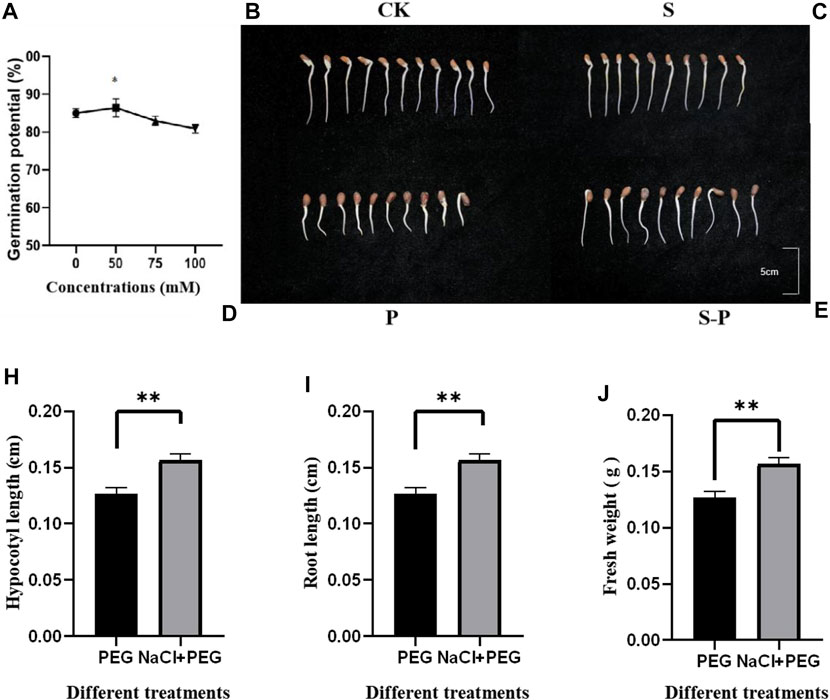
FIGURE 1. Germination potential under four different concentrations and four different treatments. (A) Germination potential after (0, 50, 75 and 100 mM NaCl treatment) (B) CK (0 Mm NaCl + 0% PEG6000 Figure) (C) S 50 mM NaCl + 0% PEG6000 (D) (0 Mm NaCl +15% PEG6000) (E) (50 mM NaCl +15% PEG6000) (H–J) Changes in hypocotyl, root length and fresh weight between treatments.
To test which phenotypic changes were exactly induced by NaCl, the hypocotyls, root length and fresh weight of cotton seedlings were determined. The hypocotyls (Figure 1H), root length (Figure 1I) and fresh weight (Figure 1J) of seedlings pretreated with 0 mM and 50 mM NaCl after determination were significantly different between treatments. The hypocotyl, root length and fresh weight of cotton seedlings were significantly increased under 50 mM NaCl pretreatment. Therefore, 50 mM NaCl was preliminarily determined as the optimal pretreatment concentration according to the phenotype. However, NaCl pretreatment determined which DEGs were induced, and we further analyzed DEGs.
DEGs analysis of drought stress under NaCl pretreatment
RNA-seq data of CK vs. ssP and P vs. S-P samples were compared. Transcriptomic analysis of nine samples was completed, and a total of 55.82 GB of clean data were obtained. Clean data of all samples reached 6.10 GB, and the percentage of Q30 bases was higher than 94.96%. The clean reads of each sample were sequenced with the designated reference genome, and the alignment efficiency ranged from 96.75% to 97.06%. Based on the results of the comparison, we performed alternative splicing prediction analysis, gene structure optimization analysis, and discovery of DEGs. A total of 9,396 new genes were discovered, of which 5,912 were functionally annotated. To evaluate the quality of DEGs, the Pearson correlation coefficient R was used to evaluate the correlation of biological replicates between samples, and the sample replication rates were all above 80% (Figure 2C).
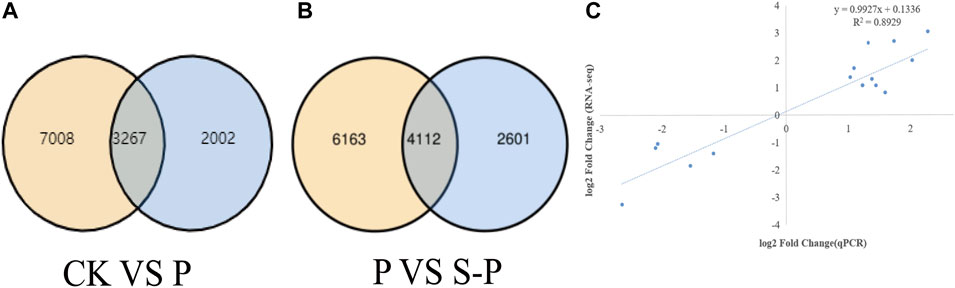
FIGURE 2. Results of RNA-seq data analysis (A,B) Venn diagram of CK VS. P DEG Venn diagram of P VS. P-S DEG. (C) Relationship between RNA-seq and quantitative real-time PCR (Q-PCR) expression data. CK: blank control; P, PEG stress treatment; S-P, PEG stress group after NaCl pretreatment.
During the DEG detection process, the differential gene expression (DEGs) between the two treatments (CK vs. S-P and P vs. S-P) was obtained with a fold change ≥ 2 and FDR < 0.01 as the screening criteria (Figure 2A). In CK vs. P, we detected 10,275 DEGs in response to drought. In the P V S-P, a total of 6,713 DEGs were detected. Figure 2B shows the difference in DEGs between the two treatments. We found that 10,275 genes were DEGs between P and S-P (8,894 genes were specifically expressed in S-P, and 2,601 genes were specifically expressed in S-P). These DEGs were associated with NaCl alleviation of PEG stress. These NaCl-specifically expressed genes were considered important candidate genes to further explain the mechanism by which NaCl pretreatment improves drought resistance.
The function of each part was classified according to the classification method of Gene Ontology (GO). A total of 8,149 DEGs between CK and P were annotated by GO, most of which were enriched in functional categories such as biological process, cellular components, and molecular function. Between P and S-P, a total of 5,392 genes were enriched through GO annotation (Figure 3A). Between CK and P, a total of 8,149 genes were enriched by GO annotation (Figure 3B).
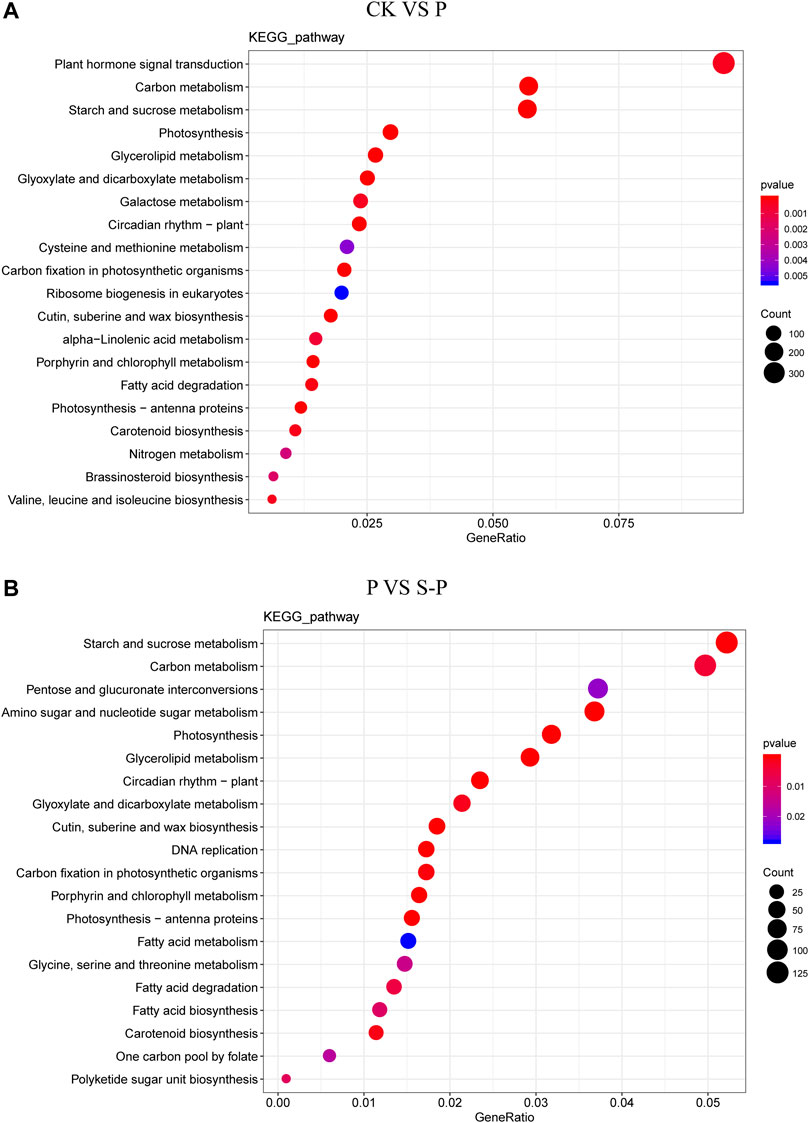
FIGURE 3. KEGG category summary for CK VS. P DEG (A) and P VS. S-P DEG (B). CK: blank control; P, PEG stress treatment; S-P, PEG stress group after NaCl pretreatment.
We performed a KEGG pathway analysis of DEGs to identify the main pathway of enrichment for improved drought resistance in cotton under NaCl pretreatment. Between CK and P, 3725 DEGs were enriched in 132 KEGG pathways, mainly including the following pathways: 355 genes (ko04075) were enriched in plant hormone signal transduction (127 genes upregulated, 228 genes downregulated); 304 genes (ko04626) were enriched in plant-pathoge interactions (76 genes were upregulated, 228 genes were downregulated); and 39 genes (ko00906) were enriched in the carotenoid synthesis pathway (29 downregulated, 10 upregulated). Between P and S-P, 2410 DEGs were enriched in 132 KEGG pathways, 221 genes (ko04075) were enriched in plant hormone signal transduction (114 genes downregulated and 97 genes upregulated), 148 genes (ko04626) were enriched in plant pathogens (117 genes upregulated, 31 genes downregulated), and 27 genes (ko00906) were enriched in the carotenoid synthesis pathway (9 downregulated, 19 upregulated).
To validate the transcriptome data, we used qRT-PCR to explore the expression of 15 randomly selected genes. Correlation analysis was performed on the two sets of data, and qRT-PCR and RNA-seq were used to compare gene fold changes (FCs) between the two treatment groups. As shown in Figures 4A,B, qRT-PCR data were consistent with RNA-seq data, with a significant positive correlation (R2 = 0. 8,929), supporting the reliability of the RNA-seq data.
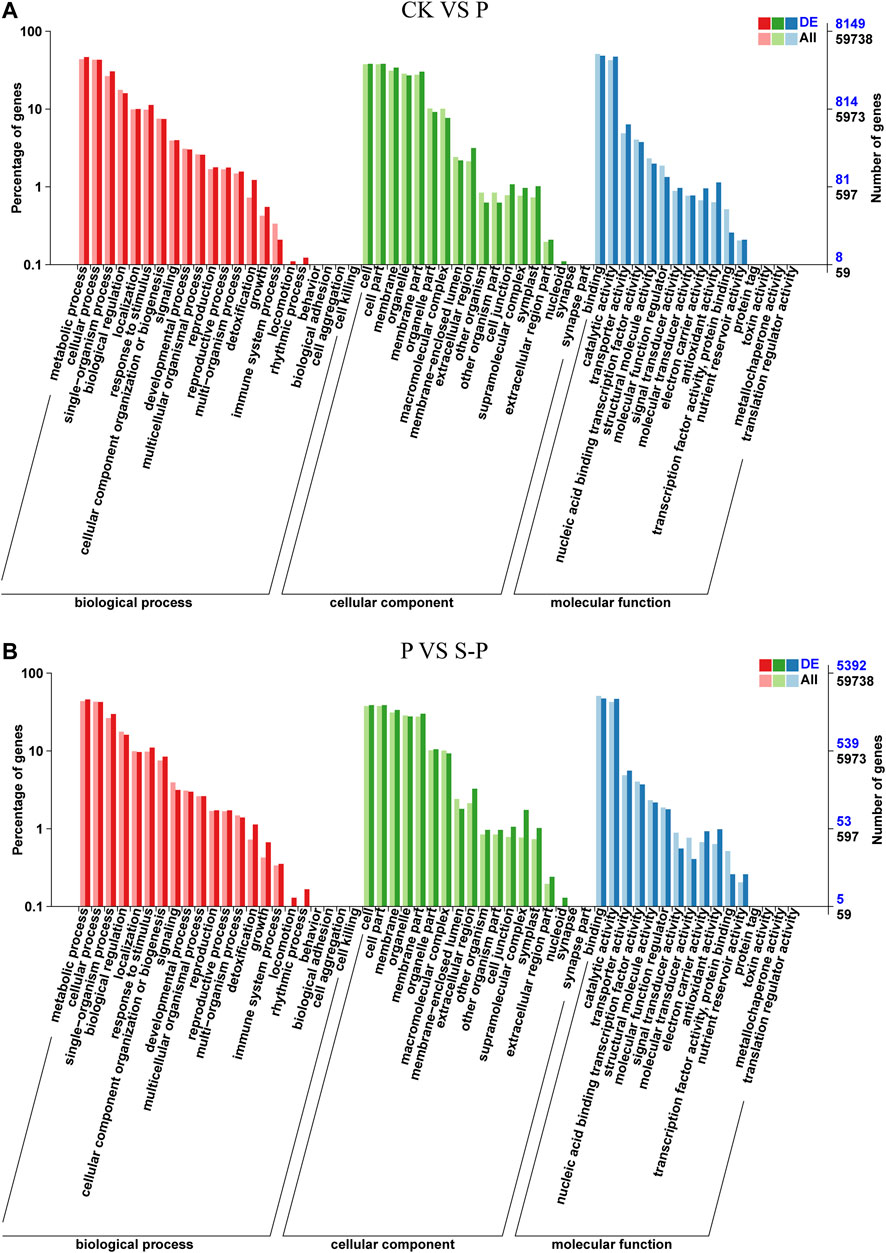
FIGURE 4. Summary of Gene Ontology (GO) categories of the DEGs in CK VS. P (A) and P VS. S-P (B). CK: blank control; P, PEG stress treatment; S-P, PEG stress group after NaCl pretreatment.
Transcription factors regulated by NaCl pretreatment in response to drought stress
Transcription factors play critical roles in regulating plant stress tolerance (Gruber et al., 2009). We found that a total of 1836 transcription factors were differentially expressed between P and CK, and 1413 were differentially expressed between P and S-P. Transcription factors under drought stress were mainly enriched in bHLH, AP2/ERF-ERF, MYB, RLK-Pelle_DLSV, and bZIP, of which 223 were upregulated and 455 were downregulated. After NaCl pretreatment, AP2/ERF-ERF, bHLH, bZIP, MYB, NAC and other transcription factors were mainly enriched, of which 239 were upregulated and 191 were downregulated, as shown in Figure 5. Moreover, it has been shown in the literature that the NAC, AP2/ERF and MYB families in plants are the main transcription factors regulated by carotenoid (Xing et al., 2021). The changes in these transcription factors after NaCl treatment may be related to the drought resistance of cotton.
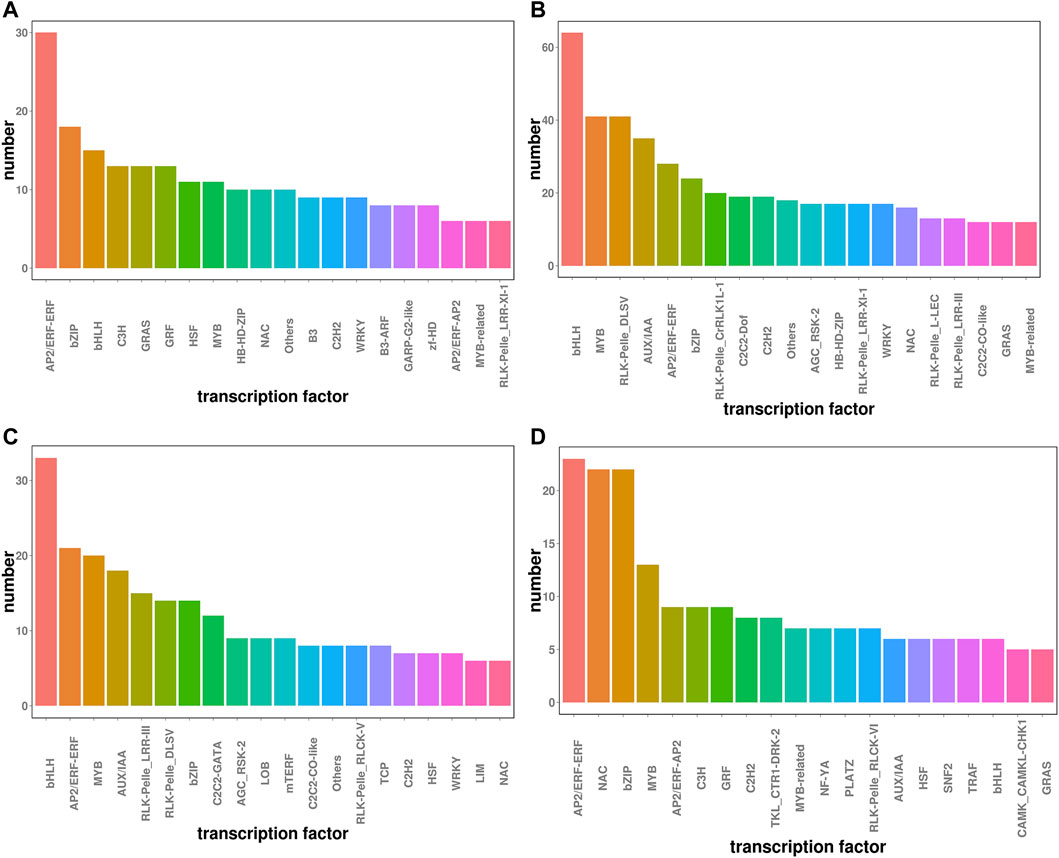
FIGURE 5. Transcription factor statistics under two treatments (A) CK vs. P up regulation (B) Ck vs. P down regulation (C) P vs. S-P up regulation (D) P vs. S-P down regulation.
NaCl pretreatment promoted carotenoid accumulation in cotton under PEG stress
Transcriptome analysis revealed changes in the expression of genes involved in the carotenoid pathway. Figure 6 shows the changes in the expression patterns of nine genes in the carotenoid synthesis pathway induced by NaCl after the PEG stress response in cotton. The carotenoid synthesis pathway is catalyzed by nine enzymes, as shown below (Figure 7). This pathway produces zeaxanthin, violaxanthin, and neoxanthin, the three types of carotenoids that ultimately synthesize ABA. We also observed that 39 related genes were differentially expressed under PEG stress, and 32 genes were downregulated under PEG stress. However, 27 genes (9 downregulated and 19 upregulated) showed different changes after NaCl pretreatment, and the upregulated genes may be involved in enhancing seed stress resistance. Interestingly, the differentially expressed gene GHLUT2 in the carotenoid pathway was observed and selected for functional verification after the bioinformatics of sequencing data. At 3 leaf stage, we used VIGS method to verify the function of this gene, because this stage is the sensitive period to drought and saline-alkali stress, and it is often selected to verify the function of drought and saline-alkali tolerance genes in cotton (Xu et al., 2022).
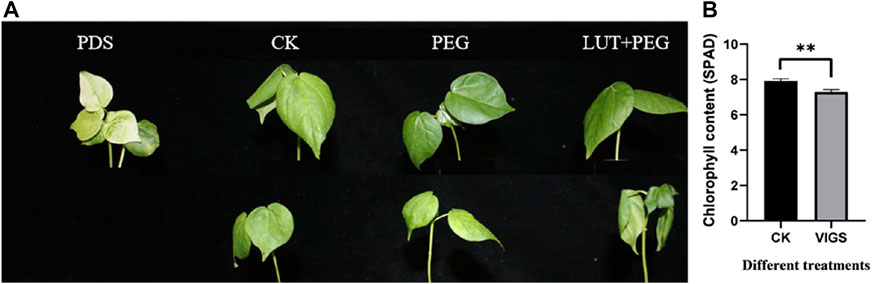
FIGURE 6. Silencing of the upstream synthetic lutein gene GHLUT2 reduced drought tolerance of cotton (A) The upper part was the normal growth group of three-leaf cotton, and the lower part was the drought stress group, divided into three treatment groups, PDS, 156-CK, 156-PEG, LUT-PEG: PDS, 156-CK, 156-PEG, and rut-PEG. (B) Changes of chlorophyll content in cotton at 3 leaf stage.
Changes in gene expression profile of carotenoid pathway after NaCl pretreatment. The expression level was estimated using log 2 (multiple variation) of each transcript. The multiple changes was the ratio of FPKM (fragment number per kilobase transcript per million sequenced fragments) of the NaCl pretreated sample to the unpretreated control sample. Phytoene synthase (PSY), phytoene desaturase (PDS), lycopene isomerase (CRTISO), lycopene ε-cyclase (LCY-ε), carotene ε–Monooxygenase (CYP), lycopen β-cyclase (LCY-β), zeaxanthin epoxidase (ZEP), violaxanthin decyclooxygenase (VDE), Indole-3-acetaldehyde oxidase (AO1), short-chain desaturase reductase (TA1), 9-cis-epoxycarotenoid dioxygenase (NCED).
Function verification of GHLUT2 with VIGS technology at three-leaf stage
Carotenoids are divided into two categories according to their structure: carotenoids and lutein, the most important of which is lutein, accounting for 59% of the total carotenoids (Gong et al., 2021). As an antioxidant, lutein plays an important role in scavenging reactive oxygen species and signal transduction in plants under stress (Vishwanathan et al., 2014). We selected CK treated with water for cotton seedlings at the three-leaf stage as a normal control, and cotton seedlings treated with 15% PEG6000 were used as a blank control. Silenced plants exhibited more severe wilting after 3 h of PEG stress, as shown in their phenotype after PEG stress. To explore the relationship between cotton lutein content and cotton drought resistance, we first compared the chlorophyll content of between (CK and VIGS). The chlorophyll content decreased by approximately 6.4% compared with CK. It has been reported that the ratio between chlorophyll and carotenoids tends to remain more or less constant, in the range of 2.5–3.7 mg total chlorophyll/mg total carotenoids (Roca and Mínguez-Mosquera, 2001). VIGS-silenced plants exhibited more severe wilting after 3 h of PEG stress, as shown in their phenotype after PEG stress. These results indicated that the decrease in lutein content made cotton subjected to more severe PEG stress (Johnson, 2005). This experiment revealed that lutein plays an important role in cotton resistance to PEG stress.
Abscisic acid related genes mitigated the effects of PEG on cotton
Abscisic acid (ABA) is known to be synthesized through two different pathways, one directly occurring in plant pathogenic fungi and the other indirectly synthesized in plants (Kong et al., 2020). Here, we only studied the ABA pathway of carotenoid synthesis. To study the relationship between carotenoids and ABA synthesis in the process of improving the tolerance of cotton to PEG stress, we analyzed the expression of genes related to the ABA pathway (Figure 8). To explore the molecular mechanism by which NaCl regulation improves drought tolerance in cotton, we enriched differential genes between CK, P and S-P in the KEGG pathway, and we found that 31 genes (7 genes up-regulated, 24 genes down-regulated) were enriched in ABA signaling, and 30 genes (21 up-regulated, 9 down-regulated) were enriched in the ABA signaling system. The heatmap showed that the expression levels of these genes were upregulated after PEG stress compared with CK samples, but the expression levels of samples under NaCl pretreatment returned to the same level as CK. The results indicated that these genes may be involved in the resistance of cotton to PEG stress through NaCl-induced expression of ABA downstream genes and related genes in the signaling system.
NaCl pretreatment promoted the changes of ABA downstream-related gene expression profiles in cotton under PEG stress. Expression levels were estimated using the log 2 (fold change) of each transcript. Fold change is the ratio of FPKM (fragments per kilobase transcript per million reads) of NaCl-pretreated samples to untreated control samples, abscisic acid receptor family (PYR), protein phosphatase (PP2C), serine/threonine protein kinase (Sn2RK), ABA response element binding factor (ABF).
Discussion
Due to global warming, drought has become one of the most substantial abiotic stresses (Xu et al., 2011). Drought stress affects crop growth and physiological metabolism, reducing yield. Drought is one of the most important abiotic stresses, severely limiting cotton yield and fiber quality (Sun et al., 2021).
NaCl pretreatment increased the tolerance of cotton
Studies have shown that pre-treating crops with NaCl can increase crop yield, triggering seeds with low concentrations of salt promotes germination of tomato seeds and increases tomato yield (Mahmood, 2020). S. salsa seeds from plants grown in the presence of NaCl were significantly larger and more numerous than seeds from control plants, with increased product, sugar and starch content in the ovules (Guo et al., 2020). It is well known that Na+ is an important inorganic osmotic agent in plants and plays a crucial role in osmoregulation (OA). To investigate the correlation between NaCl pretreatment and drought resistance, cotton seedlings were pretreated with different concentrations of NaCl (0, 50, 75, and 100 mM) before PEG germination. There was a slight increase in the germination rate with increasing pretreatment concentration from 0 to 50 mM but a decrease in germination potential with increasing NaCl concentration from 50 mM to 100 mM, which indicated that a 50 mM NaCl concentration was optimal for enhancing cotton resistance to PEG (Figure 1A). Root length was shortened under PEG stress, while NaCl pretreatment could induce cotton root length and elongation, which was consistent with our results (Ali et al., 2021).
It was found that the root length and hypocotyl length of cv H177 were improved after 50 mM NaCl treatment by comparing the changes in germination potential and hypocotyl length of cotton under the four treatments. These results indicated that NaCl was involved in the regulation of PEG stress in cotton. Higher levels of NaCl (>100 mM) significantly inhibited primary root elongation but slightly increased primary root growth compared to controls treated with low concentrations of NaCl, which is consistent with our results (Dkhil et al., 2014). Exposure of plants to mild abiotic stress can make them resistant to subsequent severe heat, cold, drought or osmotic stress, which was also confirmed by our experiments. In our results, NaCl attenuated the damage of PEG to cotton, probably because pretreatment activated stress memory and phytohormone signal transduction pathways of the plants (Bruce et al., 2007). In this study, it was found that the germination rate of cotton seeds under drought stress could be effectively improved by treating with an appropriate concentration of NaCl, and the resistance of cotton to drought stress was enhanced. The research has certain application value for cotton cultivation in arid regions.
NaCl improves cotton drought resistance by regulating carotenoid biosynthesis
Carotenoids are terpenoids synthesized from the precursor geranylgeranyl diphosphate (GGPP) and play an important role in living organisms (Uarrota et al., 2018). Carotenoids have a variety of antioxidant activities, including the ability to scavenge reactive oxygen species (ROS), such as singlet oxygen, and carotenoids are precursors for the production of plant hormones, such as abscisic acid and strigolactone (SL) (Finkelstein et al., 2002). Endogenous ABA induces root growth, so it is speculated that there is a certain relationship between carotenoids and root elongation (Rowe et al., 2016). Because carotenoids are involved in many plant stress responses, carotenoid levels in plants will vary in different environments. Transcriptome data analysis found that GHPDS1 (GH_D04G1698.gene), GHLUT2 (GH_D05G0316.gene), GHCYP97C1 (GH_A11G3687.gene), GHLCY-1 (GH_A13G0026.gene), GHZEP1 (GH_D05G0767.gene), GhVDE1 (GH_D11G2720.gene), and GhCRTISO (GH_A01G2005.gene) were downregulated under PEG treatment but upregulated under NaCl pretreatment. Phytoene desaturase (PDS) is the first desaturase synthesized in the carotenoid pathway. Studies have shown that excessive phytoene desaturase (PDS) and lycopene isomerase (CRTISO) Expression significantly enhanced plant salt tolerance and photosynthesis (Li et al., 2020).Lycopene ε-cyclase (GHLUT2) is a key gene controlling lutein synthesis in cotton. After overexpression of lycopene ε-cyclase (Lcε-LYC) in Arabidopsis, Arabidopsis low temperature stress resistance was acquired (Song et al., 2015). An independent overexpression line (PgCYP703A4 ox) increased silique size and seed number and was involved in fatty acid hydroxylation affecting keratinization and fruit size (Kim et al., 2022). Controlling carotenoid synthesis can improve tolerance to abiotic stresses such as drought and salinity. Zeaxanthin epoxidase (ZEP) plays an important role in the osmotic stress response, and zeaxanthin epoxidase (ZEP) gene overexpression After expression, Arabidopsis exhibited stronger growth under high-salt and drought treatments (Park et al., 2008). Violaxanthin decyclooxygenase (VDE) is a key enzyme in the lutein cycle and a precursor of abscisic acid (ABA), regulating developmental processes, seed maturation, stomatal closure and stress responses. As one of the key enzymes in carotenoid biosynthesis, lycopene β-cyclase positively regulates downstream ABA synthesis. Transgenic plants overexpressing lycopene cyclase (Ntβ-LCY1) in tobacco exhibited enhanced tolerance to salt and drought stress and strong accumulation of carotenoids. These carotenoids may play an important role in cotton drought resistance.
Lutein helps increase cotton’s resistance to PEG
Lutein is the most important carotenoid, and lutein plays an important role in scavenging ROS (Zhao and Sweet, 2008). As a strong antioxidant, a component of human macular pigment, it prevents macular degeneration. We found that reduced lutein levels resulted in more severe PEG stress in cotton seedlings, with silenced plants becoming more wilted, corroborating our results. It has been reported that after the Ntε-LCY1 mutant was obtained using CRISPR/Cas9 in tobacco, the content of reactive oxygen species in leaves was reduced, and the content of chlorophyll and carotenoid components was increased (Shi et al., 2014). Both chlorophyll and lutein were reduced to varying degrees by VIGS GH.
LUT2 (Gh_D05G0316), making silenced plants more sensitive to PEG. Therefore, it is speculated that lutein plays an important role in cotton under drought stress.
Mechanism of abscisic acid mediated by NaCl on PEG stress resistance
Abscisic acid (ABA) is a phytohormone that plays an important role in plant response and adaptation to various abiotic stresses, including salinity, drought, injury and cold. Abscisic acid (ABA) accumulates in plant cells when plants are stressed, such as drought. Based on transcriptome data analysis, under PEG stress, NaCl could induce the expression of ABA synthesis- and signal transduction-related genes. It was found that GHPYL6 (GH_D10G2740), GHPYL4 (GH_D01G2559), and GHABI2 (GH_D07G0143) were upregulated after PEG stress but were upregulated under NaCl pretreatment. Expression was downregulated after treatment. Studies have shown that abscisic acid receptor (PYL6) is associated with the potential to modulate phytohormone receptors, and overexpression significantly reduces grain yield under nonstress conditions (Mega et al., 2019). Protein phosphatase 2C (ABI1 and ABI2) encode serine/threonine phosphatase 2C (PP2C), which negatively regulates ABA responses, suggesting that ABI1 and ABI2 have negative effects on stress responses (Merlot et al., 2010). Abscisic acid signaling is sensed by PYL proteins and promotes the interaction of PYL proteins with PP2Cs in the cytoplasm or nucleus, resulting in the formation of the ABA-PYL-PP2C ternary complex, resulting in PP2C inactivation and SnRK2 release from PP2C inhibition (Hubbard et al., 2010; Joshi-Saha et al., 2011; Baek et al., 2018). Serine/threonine protein kinase (SnRK2) is then activated by autophosphorylation or phosphorylation by upstream kinases. The expression of ABI1 and PYL6 synthesis-related genes was upregulated under PEG stress, the expression of genes was downregulated by pretreatment, and the level of CK was restored to normal. Additionally, NaCl-induced ABI1 and PYL6 genes promoted ABA production and alleviated the subsequent peg stress. As an osmo-regulator, NaCl improves the stress resistance of cotton by modulating the ABA signal transduction system.
NaCl enhances drought resistance of cotton through a complex network
PEG (Polyethylene glycol) causes plant roots to lack water, reduce water potential and produce reactive oxygen species. As a singlet oxygen quencher, carotenoids have the ability to scavenge peroxy radicals (ROO•, hydroxyl radicals HO•) and other active substances (Eliseu et al., 2012). Condensation of two GGPP molecules to form the first carotenoid phytoene, at which point the carotene pathway bifurcates. Lycopene β cyclase and lycopene ε cyclase channels are opened by upstream signaling. The biosynthesis of carotenoids continues; on the one hand, α-carotene is synthesized through GHLUT2, and lutein is ultimately generated, which plays an important role in scavenging ROS. Lycopene β-cyclase is a key branch point enzyme of another branch. On the other hand, LCY1 is cyclized to generate β-carotene, which is the synthetic precursor of strigolactone, which plays an important role in improving the stress resistance of cotton. Under the catalysis of oxygenase (ZEP) and violaxanthin decyclooxygenase (VDE), violaxanthin is produced. Violaxanthin is regulated by 9-cis-epoxycarotenoid dioxygenase (NCED)-regulated ABA production, ABA increases, downstream transcription factors bHLH, AP2/ERF-ERF, MYB are activated, and plant stress resistance is enhanced. Violaxanthin regulates ABA production by 9-cis-epoxycarotenoid dioxygenase (NCED), which increases ABA and enhances plant stress resistance. As a phytohormone, ABA not only regulates many genes under stress conditions but also mediates other phytohormones, such as salicylic acid (SA) and jasmonic acid (JA), which combine to play a role in the defense response to pathogens and adversity stress (Nakashima and Yamaguchi-Shinozaki, 2013; Tian and Zhang, 2021).
When plants are subjected to abiotic stress, reactive oxygen species (ROS) accumulate, causing severe damage to plant cells. However, plants can gain adaptation to adversity in advance through pretreatment. In this study, we discovered plant stress tolerance pathways, carotenoid biosynthesis and ABA biosynthesis. These secondary metabolic networks interact to regulate plant stress responses. Our transcriptome data support the role of these networks. Our transcriptome data showed that NaCl can regulate carotenoid synthesis. When lycopene senses Na+ stimulation, all pathway-related genes are upregulated, downstream lutein and ABA synthesis are increased, and osmotic potential and stress resistance are improved. This study found that carotenoids play an important role in improving the drought resistance of cotton, thus providing a certain theoretical basis for improving the drought resistance of cotton.
Exogenous application of NaCl can also be used as a method to increase crop osmotic potential, which induces an increase in photosynthetic capacity and osmotic potential (Gholami et al., 2015). NaCl can also induce the expression of some antioxidant enzymes, such as the upregulation of flavonoid and carotenoid synthesis pathways. Combining our results, a mechanistic model summarizes the molecular role of NaCl in enhancing drought resistance in cotton (Figure 9). When plants are stimulated by low concentrations of NaCl, they are first sensed by the cell membrane to activate the Na+/H+ proton pump. At this point, a protein kinase composed of the binding protein SOS3 and the serine/threonine protein kinase SOS2 complex is activated, resulting in an increase in the water potential (Zhu, 2002). In response to NaCl-induced calcium signaling, plants utilize a complex set of signaling mechanisms to influence multiple signaling cascades and play regulatory roles in the regulation of several transcription factors, such as NAC, AP2/ERF, and MYB. These TFs can then bind to the promoters of target genes and regulate genes involved in the carotenoid pathway. The ensuing PEG transients in cotton can be mitigated by promoting lutein and ABA production, thereby increasing intracellular water potential and improving plant stress resistance.
Conclusion
NaCl pretreatment counteracted abiotic stress by regulating some genes related to carotenoids and ABA, suggesting that carotenoids are closely related to drought resistance in cotton. In this treatment, RNA-Seq technology was used to explore the mechanism by which carotenoids regulate drought stress in cotton at the molecular level. After NaCl pretreatment, the drought resistance and germination rate of cotton were improved by controlling the expression of some plant hormones, transcription factors, signal molecule-related genes and other mechanisms, which provided a reference for agronomic breeding. This study helps to establish an effective NaCl pretreatment method to improve cotton stress resistance.
Data availability statement
The original contributions presented in the study are included in the article/Supplementary Material, further inquiries can be directed to the corresponding author.
Author contributions
WY conceived the experiments. KN, MD and YZ performed the experiments. KN, YF, NX, HH, JW, and CR analyzed the data. KN, XL, and XC wrote the initial draft of the manuscript. HZ, YL, XL, TJ, MH, LS, DW, JW, SW, CC, LG, LZ, and WY supervised the project. All the authors read and revised the final manuscript. Part of cotton accessions provided by National Medium-term Gene. Bank of Cotton in China and National cotton germplasm resources platform.
Funding
This project is based on research that was supported by the China Agriculture Research System of MOF and MARA, National natural science foundation of China (general) (31771885) and the HAAFS Science and Technology Innovation Project (2022KJCXZX-HZS-7).
Conflict of Interest
The authors declare that the research was conducted in the absence of any commercial or financial relationships that could be construed as a potential conflict of interest.
Publisher’s note
All claims expressed in this article are solely those of the authors and do not necessarily represent those of their affiliated organizations, or those of the publisher, the editors and the reviewers. Any product that may be evaluated in this article, or claim that may be made by its manufacturer, is not guaranteed or endorsed by the publisher.
Supplementary material
The Supplementary Material for this article can be found online at: https://www.frontiersin.org/articles/10.3389/fenvs.2022.998141/full#supplementary-material
References
Ali, N., Seleiman, M., Akmal, M., Alotaibi, M., and Refay, Y. (2021). Drought stress impacts on plants and different approaches to alleviate its adverse effects. Plants 10 (2), 259. doi:10.3390/plants10020259
Baek, W., Lim, C. W., and Lee, S. C. (2018). A DEAD-box RNA helicase, RH8, is critical for regulation of ABA signalling and the drought stress response via inhibition of PP2CA activity. Plant Cell. Environ. 7 (41), 1593–1604. doi:10.1111/pce.13200
Bruce, T. J. A., Matthes, M. C., Napier, J. A., and Pickett, J. A. (2007). Stressful "memories" of plants: Evidence and possible mechanisms. Plant Sci. 173 (6), 603–608. doi:10.1016/j.plantsci.2007.09.002
Cao, Y., Liang, L., Cheng, B., Dong, Y., Wei, J., Tian, X., et al. (2018). Pretreatment with NaCl promotes the seed germination of white clover by affecting endogenous phytohormones, metabolic regulation, and dehydrin-encoded genes expression under water stress. Int. J. Mol. Sci. 19 (11), 3570. doi:10.3390/ijms19113570
Dkhil, B. B., Issa, A., and Denden, M. (2014). Germination and seedling emergence of primed okra (Abelmoschus esculentus L.) seeds under salt stress and low temperature. Am. J. Plant Physiology 9, 38–45. doi:10.3923/ajpp.2014.38.45
El-Mashad, A., and Kamel, A. R. (2001). Amelioration of NaCl stress in Pisum sativum linn. Indian J. Exp. Biol. 39 (5), 469–475.
Eliseu, R., Mariutti, L. R. B., and Mercadante, A. Z. (2012). Scavenging capacity of marine carotenoids against reactive oxygen and nitrogen species in a membrane-mimicking system. Mar. Drugs 10 (8), 1784–1798. doi:10.3390/md10081784
Fan, R., Su, X., Guo, Y., Sun, F., Qu, Y., and Chen, Q. (2020). Cotton seedling drought tolerance is improved via salt preconditioning. Protoplasma 258 (2), 263–277. doi:10.1007/s00709-020-01561-6
Finkelstein, R. R., Gampala, S., and Rock, C. D. (2002). Abscisic acid signaling in seeds and seedlings. Plant Cell. 14, 15–45. doi:10.1105/tpc.010441
Frey, A., Boutin, J. P., Sotta, B., Mercier, R., and Marion-Poll, A. (2006). Regulation of carotenoid and ABA accumulation during the development and germination of Nicotiana plumbaginifolia seeds. Planta 224 (3), 622–632. doi:10.1007/s00425-006-0231-2
Gholami, M., Mokhtarian, F., and Baninasab, B. (2015). Seed halopriming improves the germination performance of black seed (Nigella sativa) under salinity stress conditions. J. Crop Sci. Biotechnol. 18 (1), 21–26. doi:10.1007/s12892-014-0078-1
Gong, J., Zeng, Y., Meng, Q., Guan, Y., Li, C., Yang, H., et al. (2021). Red light-induced kumquat fruit coloration is attributable to increased carotenoid metabolism regulated by FcrNAC22. J. Exp. Bot. 72 (18), 6274–6290. doi:10.1093/jxb/erab283
Gruber, V., Blanchet, S., Diet, A., Zahaf, O., Boualem, A., Kakar, K., et al. (2009). Identification of transcription factors involved in root apex responses to salt stress in Medicago truncatula. Mol. Genet. Genomics 281 (1), 55–66. doi:10.1007/s00438-008-0392-8
Guo, J., Du, M., Lu, C., and Wang, B. (2020). NaCl improves reproduction by enhancing starch accumulation in the ovules of the euhalophyte Suaeda salsa. BMC Plant Biol. 20 (1), 262. doi:10.1186/s12870-020-02468-3
He, W., Wang, Y., Luo, H., Li, D., Liu, C., Song, J., et al. (2020). Effect of NaCl stress and supplemental CaCl2 on carotenoid accumulation in germinated yellow maize kernels. Food Chem. 30 (309), 125779. doi:10.1016/j.foodchem.2019.125779
Hubbard, K. E., Nishimura, N., Hitomi, K., Getzoff, E. D., and Schroeder, J. I. (2010). Early abscisic acid signal transduction mechanisms: Newly discovered components and newly emerging questions. Genes. Dev. 24 (16), 1695–1708. doi:10.1101/gad.1953910
Johnson, E. (2005). The influence of supplemental lutein and docosahexaenoic acid on their serum levels and on macular pigment. Investigative Ophthalmol. Vis. 72, 6274–6290. doi:10.1093/ajcn/87.5.1521
Joshi-Saha, A., Valon, C., and Leung, J. (2011). Abscisic acid signal off the STARTing block. Mol. plant 4 (4), 562–580. doi:10.1093/mp/ssr055
Kevin, S., Paulina, F., Felipe, Q. I. L., Carlos, F. O., Rodrigo, C., Michael, H., et al. (2018). Unraveling the induction of phytoene synthase 2 expression by salt stress and abscisic acid in Daucus carota. J. Exp. Bot. 69 (16), 4113–4126. doi:10.1093/jxb/ery207
Khoo, H. E., Prasad, K. N., Kong, K. W., Jiang, Y., and Ismail, A. (2011). Carotenoids and their isomers: Color pigments in fruits and vegetables. Molecules 16 (2), 1710–1738. doi:10.3390/molecules16021710
Kim, J., Silva, J., Park, C-K., Kim, Y., Park, N., Sukweenadhi, J., et al. (2022). Overexpression of the Panax ginseng CYP703 alters cutin composition of reproductive tissues in Arabidopsis. Plants 11 (3), 383. doi:10.3390/plants11030383
Kong, C., Li, R., Bressan, A., and Song, J. K. (2020). Abscisic acid dynamics, signaling, and functions in plants. J. Integr. plant Biol. 62 (01), 27–56. doi:10.1111/jipb.12899
Kudla, J., Becker, D., Grill, E., Hedrich, R., Schumacher, K., Kummer, U., et al. (2018). Advances and current challenges in calcium signaling. New Phytol. 218 (2), 414–431. doi:10.1111/nph.14966
Lawlor, D. W. (1970). Absorption of polyethylene glycols by plants and their effects on plant growth. New Phytol. 69, 501–513. doi:10.1111/j.1469-8137.1970.tb02446.x
Li, C., Ji, J., Wang, G., Li, Z., Wang, Y., and Fan, Y. (2020). Over-expression of LcPDS, LcZDS, and LcCRTISO, genes from wolfberry for carotenoid biosynthesis, enhanced carotenoid accumulation, and salt tolerance in tobacco. Front. Plant Sci. 11, 119. doi:10.3389/fpls.2020.00119
Li, Z., Su, X., Chen, Y., Fan, X., He, L., Guo, J., et al. (2021). Melatonin improves drought resistance in maize seedlings by enhancing the antioxidant system and regulating abscisic acid metabolism to maintain stomatal opening under PEG-induced drought. J. Plant Biol. 64, 1–14. doi:10.1007s12374/021/09297/3
Livak, K. J., and Schmittgen, T. D. (2002). Analysis of relative gene expression data using real-time quantitative PCR and the 2−ΔΔCT method. Methods 25 (4), 402–408. doi:10.1006/meth.2001.1262
Lu, Z. Y., Wang, J., Chen, X., Wang, D., Wang, S., Guo, L., et al. (2019). Identification and function analysis of drought-specific small RNAs in Gossypium hirsutum L. Plant Sci. 280 (280), 187–196. doi:10.1016/j.plantsci.2018.11.015
Mahmood, S., and Kubra, K. (2020). Effect of different salt concentrations (NaCl) on seed germination and seedling growth of Tomato cv. BINA Tomato-10. Asian Plant Res. J. 5 (3), 38–44. doi:10.9734/aprj/2020/v5i330109
Mao, X., Cai, T., Olyarchuk, J. G., and Wei, L. (2005). Automated genome annotation and pathway identification using the KEGG Orthology (KO) as a controlled vocabulary. Bioinformatics 21 (19), 3787–3793. doi:10.1093/bioinformatics/bti430
Mega, R., Tsujimoto, H., and Okamoto, M. (2019). Genetic manipulation of abscisic acid receptors enables modulation of water use efficiency. Plant Signal. Behav. 14 (10), 1642039. doi:10.1080/15592324.2019.1642039
Merlot, S., Gosti, F., Guerrier, D., Vavasseur, A., and Giraudat, J. (2010). The ABI1 and ABI2 protein phosphatases 2C act in a negative feedback regulatory loop of the abscisic acid signalling pathway. Plant J. 25 (3), 295–303. doi:10.1046/j.1365-313x.2001.00965.x
Minoru, K., Michihiro, A., Susumu, G., Masahiro, H., Mika, H., Masumi, I., et al. (2008). KEGG for linking genomes to life and the environment. Nucleic Acids Res. 36, 480–484. doi:10.1093/nar/gkm882
Nakashima, K., and Yamaguchi-Shinozaki, K. (2013). ABA signaling in stress-response and seed development. Plant Cell. Rep. 32 (7), 959–970. doi:10.1007/s00299-013-1418-1
Okunlola, G. O., Olatunji, O. A., Akinwale, R. O., Tariq, A., and Adelusi, A. A. (2017). Physiological response of the three most cultivated pepper species (Capsicum spp.) in Africa to drought stress imposed at three stages of growth and development. Sci. Hortic. 224, 198–205. doi:10.1016/j.scienta.2017.06.020
Park, H. Y., Seok, H. Y., Park, B. K., Kim, S. H., Goh, C. H., Moon, Y. H., et al. (2008). Overexpression of Arabidopsis ZEP enhances tolerance to osmotic stress. Biochem. Biophys. Res. Commun. 375 (1), 80–85. doi:10.1016/j.bbrc.2008.07.128
Rajasekaran, L. R., and Blake, T. J. (1999). New plant growth regulators protect photosynthesis and enhance growth under drought of Jack Pine seedlings. J. Plant Growth Regul. 18 (4), 175–181. doi:10.1007/pl00007067
Rasheed, A., Hassan, M. U., Aamer, M., Batool, M., Huijie, L. I., Wu, Z., et al. (2020). A critical review on the improvement of drought stress tolerance in rice (Oryza sativa L.) Not. Bot. Horti Agrobot. Cluj. Napoca. 48 (4), 1756–1788. doi:10.15835/nbha48412128
Roca, M., and Mínguez-Mosquera, M. (2001). Changes in chloroplast pigments of olive varieties during fruit ripening. J. Agric. Food Chem. 49 (2), 832–839. doi:10.1021/jf001000l
Rowe, J. H., Topping, J. F., Liu, J., and Lindsey, K. (2016). Abscisic acid regulates root growth under osmotic stress conditions via an interacting hormonal network with cytokinin, ethylene and auxin. New Phytol. 211 (1), 225–239. doi:10.1111/nph.13882
Seki, M., Umezawa, T., Kim, J-M., Matsui, A., To, T. K., and Shinozaki, K. (2007). Transcriptome analysis of plant drought and salt stress response. Plant gene. 12, 261–283. doi:10.1007/978-1-4020-5578-2_11
Shi, G., Xia, S., Ye, J., Huang, Y., Liu, C., and Zhang, Z. (2015). PEG-simulated drought stress decreases cadmium accumulation in castor bean by altering root morphology. Environ. Exp. Bot. 111, 127–134. doi:10.1016/j.envexpbot.2014.11.008
Shi, Y., Wang, R., Luo, Z., Jin, L., Liu, P., Chen, Q., et al. (2014). Molecular cloning and functional characterization of the Lycopene ε-Cyclase ene via virus-induced gene silencing and its expression pattern in Nicotiana tabacum. Int. J. Mol. Sci. 15 (8), 14766–14785. doi:10.3390/ijms150814766
Shukla, S., Mcnally, A., Husak, G., and Funk, C. (2014). Seasonal drought forecast system for food-insecure regions of East Africa. Hydrology Earth Syst. Sci. 11 (3), 3049–3081. doi:10.5194/hessd-11-3049-2014
Song, X., Diao, J., Ji, J., Wang, G., Li, Z., Wu, J., et al. (2015). Overexpression of lycopene ε-cyclase gene from lycium chinense confers tolerance to chilling stress in Arabidopsis thaliana. Gene 15 (576), 395–403. doi:10.1016/j.gene.2015.10.051
Sun, F. L., Chen, Q., Chen, Q. J., Jiang, M., Gao, W., and Qu, Y. Y. (2021). Screening of key drought tolerance indices for cotton at the flowering and boll setting stage using the dimension reduction method. Front. Plant Sci. 12, 619926. doi:10.3389/fpls.2021.619926
Tan, J., Tu, L., Deng, F., Hu, H., Nie, Y., and Zhang, X. (2013). A genetic and metabolic analysis revealed that cotton fiber cell development was retarded by flavonoid naringenin. Plant Physiol. 162 (1), 86–95. doi:10.1104/pp.112.212142
Tian, Y., and Zhang, T. (2021). MIXTAs and phytohormones orchestrate cotton fiber development. Curr. Opin. Plant Biol. 59, 101975. doi:10.1016/j.pbi.2020.10.007
Uarrota, V. G., Stefen, D. L. V., Leolato, L. S., Gindri, D. M., and Nerling, D. (2018). Antioxidants and antioxidant enzymes in higher plants. Springer International Publishing, 207–232. doi:10.1007/978-3-319-75088-0
Vishwanathan, R., Kuchan, M. J., Sen, S., and Johnson, E. J. (2014). Lutein and preterm infants with decreased concentrations of brain carotenoids. J. Pediatr. Gastroenterol. Nutr. 59, 659–665. doi:10.1097/mpg.0000000000000389
Xing, J. L., Li, W., Lam, S. M., Yuan, H., Shui, G., Yang, J., et al. (2021). AP2/ERF and r2r3-MYB family transcription factors: Potential associations between temperature stress and lipid metabolism in auxenochlorella protothecoides. Biotechnol. Biofuels 14 (1), 22–16. doi:10.1186/s13068-021-01881-6
Xu, L., Han, L., and Huang, B. (2011). Antioxidant enzyme activities and gene expression patterns in leaves of Kentucky Bluegrass in response to drought and post-drought recovery. J. Am. Soc. Hortic. Sci. 136 (4), 247–255. doi:10.21273/jashs.136.4.247
Xu, N., Zhang, H., Lu, X., Wang, X., Zhang, Y., Fan, Y., et al. (2022). ER whorls triggered by GhCNX6 were discovered involved in drought stress on cotton. Environ. Exp. Bot. 203, 105062. doi:10.1016/j.envexpbot.2022.105062
Young, M., Wakefield, M. J., Smyth, G. K., and Oshlack, A. (2010). Gene ontology analysis for RNA-seq: Accounting for selection bias. Genome Biol. 11 (2), 14. doi:10.1186/gb-2010-11-2-r14
Zhang, Y., Fan, Y., Rui, C., Zhang, H., Xu, N., Dai, M., et al. (2021). Melatonin improves cotton salt tolerance by regulating ROS scavenging system and Ca2+ signal transduction. Front. Plant Sci. 12, 693690. doi:10.3389/fpls.2021.693690
Zhang, H., Sun, X., and Dai, M. (2021). Improving crop drought resistance with plant growth regulators and rhizobacteria: Mechanisms, applications, and perspectives. Plant Commun. 3 (1), 100228. doi:10.1016/j.xplc.2021.100228
Zhao, L., and Sweet, B. V. (2008). Lutein and zeaxanthin for macular degeneration. Am. J. Health. Syst. Pharm. 65 (13), 1232–1238. doi:10.2146/ahjp080052
Zhao, S., Zhang, Q., Liu, M., Zhou, H., Ma, C., and Wang, P. (2021). Regulation of plant responses to salt stress. Int. J. Mol. Sci. 22 (9), 4609. doi:10.3390/ijms22094609
Keywords: NaCl pretreatment, carotenoid metabolism, cotton, drought resistance, abscisic acid
Citation: Ni K, Dai M, Lu X, Zhang Y, Fan Y, Xu N, Feng X, Huang H, Wang J, Rui C, Zhang H, Lei Y, Liu X, Jiang T, Han M, Sun L, Chen X, Wang D, Wang J, Wang S, Chen C, Guo L, Zhao L and Ye W (2022) Pretreatment of NaCl enhances the drought resistance of cotton by regulating the biosynthesis of carotenoids and abscisic acid. Front. Environ. Sci. 10:998141. doi: 10.3389/fenvs.2022.998141
Received: 19 July 2022; Accepted: 31 October 2022;
Published: 23 November 2022.
Edited by:
Niall Patrick Hanan, New Mexico State University, United StatesReviewed by:
Amit Kumar Mishra, Mizoram University, IndiaNasim Ahmad Yasin, University of the Punjab, Pakistan
Jinfa Zhang, New Mexico State University, United States
Copyright © 2022 Ni, Dai, Lu, Zhang, Fan, Xu, Feng, Huang, Wang, Rui, Zhang, Lei, Liu, Jiang, Han, Sun, Chen, Wang, Wang, Wang, Chen, Guo, Zhao and Ye. This is an open-access article distributed under the terms of the Creative Commons Attribution License (CC BY). The use, distribution or reproduction in other forums is permitted, provided the original author(s) and the copyright owner(s) are credited and that the original publication in this journal is cited, in accordance with accepted academic practice. No use, distribution or reproduction is permitted which does not comply with these terms.
*Correspondence: Wuwei Ye, eWV3MTU4QDE2My5jb20=
†These authors have contributed equally to this work