- 1Department of Forestry Economics & Management, Northeast Forestry University, Harbin, China
- 2Biological Science Research Center, Academy for Advanced Interdisciplinary Studies, Southwest University, Chongqing, China
- 3Pakistan Forest Institute, Peshawar, Pakistan
- 4College of Chemistry, Chemical Engineering and Resource Utilization, Northeast Forestry University, Harbin, China
Riparian buffers and stream channel widths along river networks have extremely significant ecological influences on parameters and stressors associated with riparian health indicators (RHIs). It is imperative for countries that rely heavily on rivers for irrigation to protect RHIs such as habitat, plant cover, regeneration, exotics, and erosion. It is unclear which protection methods are most effective for RHIs in less developed countries, such as Pakistan. This study fills this gap by using a quick field-based technique that includes 273 transects and examines the response of RHIs in the upper and lower Indus River basins (IRB). In the lower Indus basin (LIB), riparian buffer and stream channel widths had the most considerable influence on RHIs using Pearson’s correlations, ranging from ̶ 0.47 < r < 0.71 and ̶ 0.41 < r < 0.32, respectively. There was a significant relationship between stressors and RHIs in the LIB when these widths were changed, and stressors had a significant influence on habitat ̶ 0.37 < r < 0.41, plant cover ̶ 0.32 < r < 0.38, regeneration ̶ 0.29 < r < 0.25, erosion ̶ 0.34 < r < 0.49, and exotics ̶ 0.39 < r < 0.24. In contrast, these stressors in the upper Indus basin (UIB) also adversely affected habitat ̶ 0.28 < r < 0.27, plant cover ̶ 0.34 < r < 0.26, regeneration ̶ 0.19 < r < 0.26, erosion ̶ 0.38 < r < 0.23, and exotics ̶ 0.31 < r < 0.30. It was found from the principal component analysis that the responses of RHIs and stressors varied considerably between the UIB and LIB. Additionally, the agglomerative hierarchical cluster analysis of the RHIs and stressor indices revealed dissimilarities in the UIB and LIB. This study supports the need to examine riparian regions along long rivers, which are subject to the same administrative strategies. Large river ecosystems need revised standards to prevent further degradation based on ecological indicators.
1 Introduction
Riparian zones along rivers are ecologically influenced by several factors (Guo et al., 2021; Yuancai et al., 2022), including the riparian buffer and watercourse widths (Arif et al., 2022b). Similarly, ecological indicators in riparian areas are also influenced by these width factors (Kuglerova et al., 2020; Stutter et al., 2021). Fluvial channel structure varies significantly across the fluvial framework based on the size and location of rivers (Oliveira et al., 2019; Tariq et al., 2021). Streams differ from rivers in gradient, flow, and flora due to their particular terrain and ecology (Chen Z. et al., 2022; Jalayer et al., 2022). In conservation initiatives worldwide, the protection of major rivers takes precedence over the protection of streams. The footprint of a channel is an indication of its proximity to the immediate surroundings (Hu et al., 2022). The rivers that are most vulnerable to the adverse effects of water networks can be identified when riparian ecological characteristics are considered (Jullian et al., 2021). It has been reported that different countries have different policies regarding the definition and implementation of riparian buffer zones and managing ecological indicators in these areas (Dai et al., 2017; Francis et al., 2021). In developed countries, ecological indicators are protected by relatively clear rules, but in less developed or poorer countries, such rules do not exist or are vaguely described.
It has been shown that hydro-fluctuations in the riparian buffer are associated with water body conditions (Ding et al., 2022). There is a great deal of variation in river networks in different regions, just as their rules vary from region to region. Natural and altered river channels have different hydrological characteristics worldwide (Haq et al., 2019), which may adversely affect natural ecosystems (Wang et al., 2020; Fu et al., 2022). Consequently, some developed countries maintain limited riparian widths to ensure their ecological function. River buffer zones in the Nordic-Baltic region of the United States range from 5 to 50 m (Ring et al., 2017). Streams in this area have been conserved as closely as possible to their natural state. Several studies have suggested that the riparian width of streams in Canada should be between 20 and 50 m to minimize habitat degradation (Kuglerova et al., 2020). The study of Borneo, Malaysia has demonstrated that river conservation with a riparian width of 40–100 m lead to greater river services along both riverbanks (Mitchell et al., 2018). There is no limit on the buffer width of rivers and streams in Asian countries, particularly Pakistan. Since each stream and river has unique geomorphological and environmental characteristics (Charles et al., 2018; Rehman et al., 2018), planning for their sustainable use is imperative. Despite this, little is known about the long-term effects of riparian buffers and channel widths on the Indus River. The Indus River originates in the Himalayan range and is one of the longest in Pakistan and a vital lifeline for the people (Ahmed et al., 2018).
There is insufficient room for ecosystem services in stream buffers that are less than 10 m wide on each stream bank. For natural habitats to remain intact, a buffer of 30 m or more is required (Olden et al., 2019; Arif et al., 2022b). It has been found that even small buffer zones are not sufficient to maintain the integrity of waterways in British Columbia, Sweden, and Finland (Kuglerova et al., 2020). There is a need to pay attention to large areas of stream networks worldwide to provide effective service delivery (Rafique et al., 2020; Guo et al., 2021). Water resources can be conserved by improving the environment and hydrological conditions. It is mandated by the UN Sustainable Development Agenda 2030 that everyone pays attention to the state of the environment in terms of conservation (Chen D. et al., 2022). Furthermore, it is still unclear whether riparian and stream channel widths equally affect ecological factors in the upper and lower basins. Considering preliminary findings, international regulations and recommendations for streams and rivers must be re-evaluated. Studies such as those mentioned above may assist us in understanding how river width affects stream ecology (Stutter et al., 2021). Protecting streams and rivers has become increasingly relevant to preserve ecological diversity and function (Mitchell et al., 2018; Bombino et al., 2019). Most recent research has focused on hydrological processes, ecological services, and landscape connectivity. Some politicians and professionals are still skeptical about the reported effects of policy change. As a result of the emergence of sustainable standards, environmentalists and other conservationists are increasingly calling for policy reforms on a global scale.
The environmental authorities have concentrated their efforts on improving the management of riverine areas to address this issue. Riparian regulations must be reviewed based on the results of research and current riparian regulations. The legislation governing riverine areas imposes a minimum width requirement for security. Researchers have also examined buffer widths in tropical locations and North America to determine how they affect the environment (Ring et al., 2017). Generally, Asian countries lack clear policies or define them vaguely. Many guidelines in industrialized nations are unclear, complex, and heavily influenced by local conditions. Such implications must be evaluated for the Indus River Basin (IRB). It was revealed that 50 percent of the studies that assessed riparian buffers and stream channels considered terrestrial ecology, biodiversity, and function when determining their lengths (Luke et al., 2019). Numerous studies have failed to demonstrate the need for an extended buffer or to advance management practices. Several influences on ecosystem function were identified in a review of 453 papers on human activities in riparian ecosystems (Poff et al., 2011). It is apparent that there are no accurate statistical models or theoretical analyses in the existing literature regarding upper and lower basins simultaneously. It may be possible to develop technical data for riparian zones by analyzing the effects of shifting channel widths on riparian zones on a regional scale. Compared to other components of the terrestrial ecosystem, vegetation in riparian buffers appears to have a more significant impact on biodiversity in the terrestrial ecosystem (Tata, 2021; Behzad et al., 2022).
Although there are some differences in biodiversity between those naturally occurring and those that have been somewhat impacted (Muhammad et al., 2022c; Hussain et al., 2022; Islam et al., 2022), habitat loss and fragmentation have contributed to changes in protected species (Haq et al., 2021b; Hu et al., 2022), especially in narrow buffer zones such as streams. Compared to narrow buffer zones, wider buffer zones contain a broader diversity of habitats and plant types (Gundersen et al., 2010; Tata, 2021). Seasonal migrants may also use riverine zones as temporary housing at certain times of the year (Vilchez Mendoza et al., 2014). It has been reported that sufficient birds are found in riparian woody vegetation in wetland settings (Mitchell et al., 2018). Some researchers have concluded that cattle grazing on riparian buffers results in the loss of flora (Lees and Peres, 2008). Landscape exposure in several studies affects the design and implementation of buffer ecosystems. Since river impacts may continue to degrade habitats over time, it is unknown whether terrestrial biodiversity will survive in buffers in the long run (Arif and Changxiao, 2022). In spite of this, it is unclear to what extent long rivers modify riparian functions. It is generally accepted in the literature that the width of the riparian buffer is positively correlated with species diversity (Luke et al., 2019). Due to the broader area and variety of riparian widths found along large streams and rivers, such results are not always effective. The lack of predetermined width limits has prevented ecologists from discovering ecological relationships in streams. Riparian buffers play an influential role in conserving degraded ecosystems (Hira et al., 2022). These buffer zones are also conducive to the spread of invasive species (Hu et al., 2022). Despite this, extensive rivers have not been systematically explored in underdeveloped countries. Some studies have examined the relationship between ecosystems and species in riparian zones (Chen Z. et al., 2022). Research of this type may be of great value to policymakers and requires further investigation. River buffers often share the same stresses as rivers themselves. Since expensive streams and long rivers have narrow widths, researchers tend to focus on buffer zones with wide ranges of width (Arif et al., 2022a). Riparian buffers and other ecological factors are also lack scientific evidence in Pakistan. The buffer zones provided by the IRB provide significant benefits and advantages despite these limitations.
Environmental sustainability requires the analysis of riparian zone indicators and stressors in the upper and lower river basins. Additionally, various riparian buffers and channel widths will be investigated for their effects on the aforementioned ecological factors in the upper Indus basin (UIB) and lower Indus basin (LIB) inside the vast Indus basin, i.e., pros and cons. Our purpose was to evaluate the following: We (1) assessed ecological parameters (such as riparian stress and health) using the major sections of the IRB (UIB and LIB); (2) classified the significant components responsible for variability in the riparian zone structures across large areas of the UIB and LIB; and (3) examined the relationship between these ecological parameters when it comes to managing ecological characteristics in river riparian zones with the same patterns. It will provide new insight into how to accomplish this on a large scale.
2 Materials and methods
2.1 Study area
Pakistan has five influential river basins (Indus, Chenab, Ravi, Jhelum, and Sutlej), but the IRB is one of the most famous (Ahmed et al., 2018; Hira et al., 2022). It lies between 29° 30′N and 37° 10′N, and 70° 15′E and 77° 0′E. There is approximately 139,202 km2 of the area within the IRB, of which 8%, 39%, and 53% are within China, India, and Pakistan, respectively (Rehman et al., 2018). This river originates from the Tibetan Plateau’s Lake Mansarovar and is further divided into several branches, which flow through India and Pakistan and empty into the Arabian Sea. Consultants have estimated the annual sediment load at Tarbela Dam, which is the junction of UIB and LIB, to be 242.4 mcm at the time of planning and design (Figure 1). The Indus River produces a great deal of sediment (Habib ur et al., 2017). Kharmong and Panjnad are the points at which the Indus River confluences with other rivers in Pakistan. Due to its geographical characteristics and land use patterns, there are two major components of the IRB: the UIB, which represents 54% of the IRB, and the LIB, which represents 46% of the IRB. Specifically, the LIB specified is the midstream component of the entire IRB (Ahmed et al., 2018). The riparian width range was highest in the LIB at 110.65 ± 8.22 m (mean ± standard deviation) and 95.85 ± 8.68 m in UIB. Similarly, stream channel widths were the uppermost in the LIB and spanned 312.98 ± 12.20 m and 71.25 ± 4.51 m in the UIB (Figure 2). Primarily westerly winds and glaciers feed the UIB, which is between 440 m and 8,361 m above sea level. While LIBs are located between 70 and 440 m asl. Climates in these regions are predominantly arid or semi-arid, with upstream discharge, monsoon rains, and tributaries determining water flow (Rehman et al., 2018). Precipitation in the UIB ranges between 200 and 1,400 mm, while the temperature in the UIB ranges between 5°C and 20°C (Naz et al., 2019; Rafique et al., 2020). LIB temperatures range from 10°C to 32°C and precipitation from 200 to 1,200 mm. The IRB recognizes several major categories of land use (Rashid et al., 2018), including water bodies, forests, shrublands, grasslands, built-up areas, agricultural areas, barren land, and snow and glaciers. There was a difference in the order of dominant major cations between UIB and LIB, reflecting the variety of geological formations. Following the suitability assessment, the river water meets the WHO’s drinking water guidelines. However, it did not conform to the guidelines set by the WHO regarding irrigation. Soil degradation is the primary cause of this problem. Tarbela Dam was constructed along the Indus River approximately 65 km northwest of Islamabad in 1974 and has a capacity of 14,295 mcm at full reservoir capacity. Tarbela reservoir receives approximately 76,274 mcm of water each year. Currently, the dam has 85 years remaining in its useful life (Habib ur et al., 2017). If sediment deposits accumulate in the reservoir, the delta could advance towards the power intakes, interfering with the openings of the outlet and other functions of the dam.
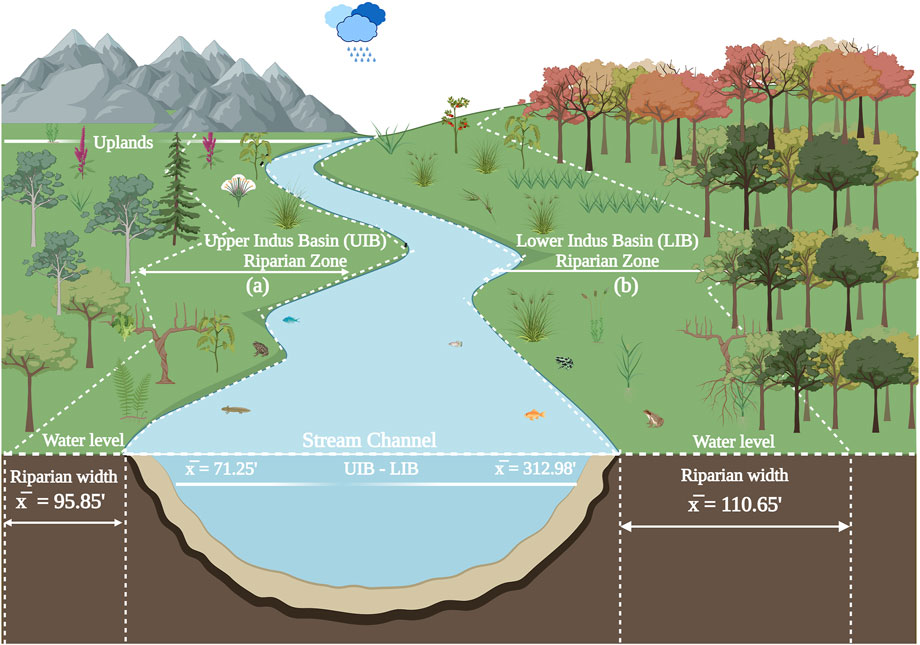
FIGURE 2. An illustration of the riparian zones along the upper and lower Indus basins (A,B). Source: symbols for diagram obtained from (https://app.biorender.com/).
2.2 Conceptualization of sampling
In 2020, data were collected from 273 transects (147 UIB transects and 126 LIB transects, respectively), while accounting for their proportional contribution to the IRB. We adapted a rapid appraisal approach from the literature (see Jansen et al., 2005) for the riparian zone parameters along the river setting in order to obtain real-life data on riparian health indicators (RHIs). Moreover, data on riparian buffers and stream channel widths were collected using the Global Positioning System. Later, Dixon et al. (2006) provide detailed descriptions of the data collection methods for each parameter in a technical guideline report (see Supplementary Figures S1–S13). Jansen et al. (2005) introduced this approach to studying the riparian zone in Australia, and other researchers have adopted it for the riparian zone of the Three Gorges Dam in China (Arif and Changxiao, 2022). Some indicators, such as land-use pattern and farming system, in this study were subject to constraints specific to the IRB. Therefore, the method described by Lanzanova et al. (2019) was utilized to compute new and missing indicators through the process of expert calibration (see Figures 2, 3 in Lanzanova et al., 2019). We quantified each component in the riparian zone by establishing a 100-m-long, 20-m-wide transect parallel to the Indus River (see Supplementary Figure 2). Each indicator scores from 1 to 5 on a five-point scale, with each corresponding to a unique site scenario. Poor conditions are reflected in low scores, while good conditions are reflected in high scores. Once the data has been standardized, an overall score of 100 provides a detailed description of the RHIs performed within a given transect. Two categories of parameters were collected from ecological networks. One category is the RHIs, which have 27 parameters which include sub-indices of habitat, plant cover, regeneration, exotics, and erosion indicators. Stressors are treated independently with 11 parameters in the second category. The index flow chart in Figure 3 illustrates the details of all parameters, including their index and sub-indices. These indicators were depicted as actual indicators that were observed in riparian zones during the sampled period. In this study, riparian buffers and stream channel widths were carefully assessed at the same place along transects to determine their impact on ecological indicators at representative sites. In order to create a viable representation of the entire IRB, the researchers analyzed the maximum number of indicators reported in the literature.
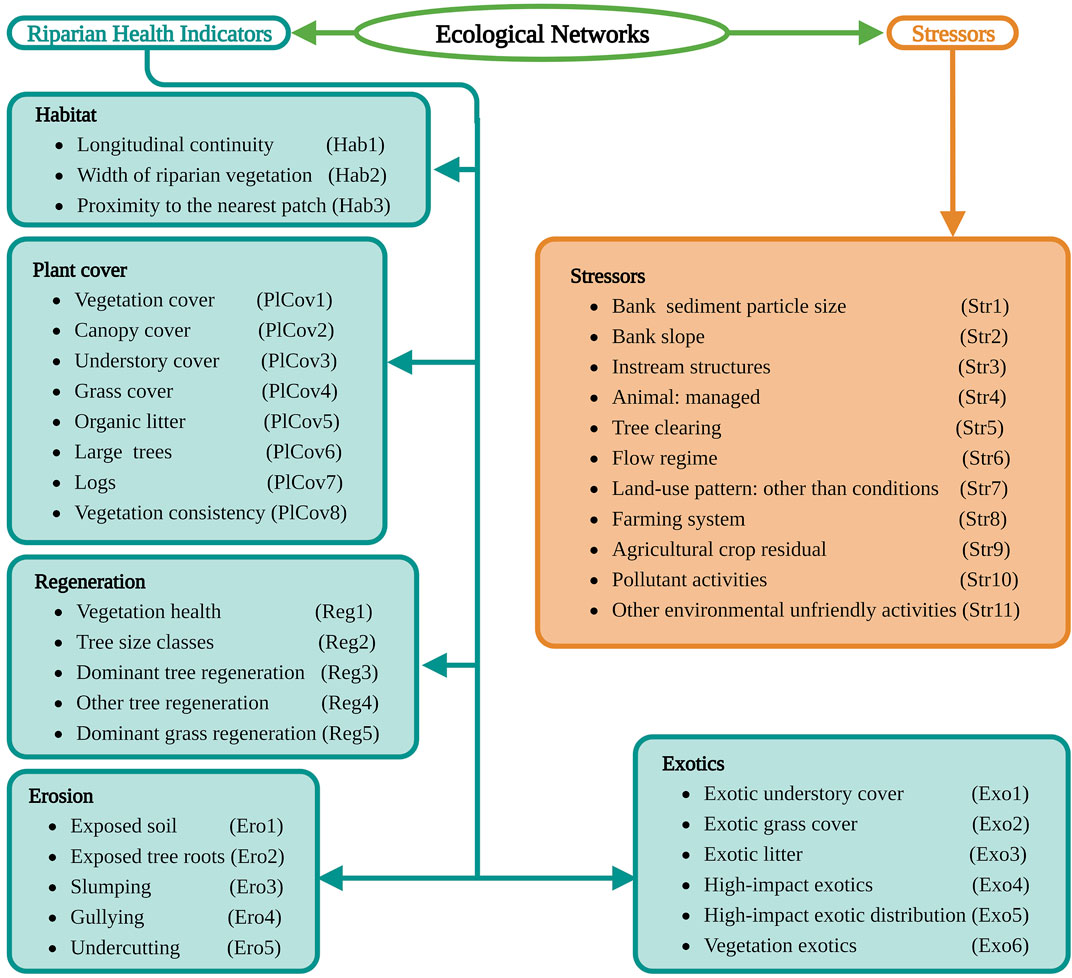
FIGURE 3. List of the riparian health indicators and stressors, along with their respective abbreviations inside the parentheses, used for the upper and lower Indus basin riparian zones of the Indus River in Pakistan. Source: Arif and Changxiao, 2022; Hira et al., 2022.
2.3 Statistical analysis
The statistical analysis was carried out using Origin software, which was released in 2022 (Northampton, MA, USA). It was determined that non-parametric Kruskal–Wallis tests showed significant differences between the RHIs and stressors within the UIB and LIB when the riparian buffer and stream channel widths were changing within the IRB (p < 0.05). This non-parametric test allows comparisons between RHIs and stressor indices or sub-indices on a broad scale (Hira et al., 2022). Principal component analysis (factor analysis) was also used to identify the essential components of both UIBs and LIBs related to RHIs and stressors. In this manner, leading factors are identified (Bombino et al., 2019), thereby lowering potential challenges considering 38 indicators. RHIs and stressors were correlated with riparian buffer and stream channel widths associated with the UIB and LIB river networks by the Pearson correlation coefficient. The correlation coefficient connection in this study represents the connection commonly found in linear models (Arif et al., 2022a). The UIB and LIB index patterns were then analyzed using hierarchical cluster analysis, considering indicators of RHIs and stressors. The thorough analysis permitted the complete combination of similar traits within complex river environments. It is also possible to distinguish between the UIB and LIB river networks using this analysis.
3 Results
3.1 Ecological network responses under changing topographical features
Seven bar graphs (a–g) were created to show the differences in ecological network indices and sub-indices (RHIs and stressors) with 95% confidence levels throughout the IRB’s UIB and LIB regions (Figure 4). The ecological network scored the highest, mostly in the UIB, except for regeneration, which scored well in the LIB. The highest mean scores in the UIB are calculated as follows: 18.31% ± 2.56% for habitat, 8.61% ± 1.21% for plant cover, 16.89% ± 3.91% for erosion, 16.35% ± 2.52% for exotics, 71.08% ± 5.28% for condition, and 34.76% ± 16.99% for stressors. However, regeneration had a mean score of 11.58% ± 2.71% in LIB. These indices were statistically significant at p < 0.01**, except for plant cover, which was significant at p < 0.05* in the UIB. It is important to note that stressors and exotics in LIB were insignificant even at p < 0.05*.
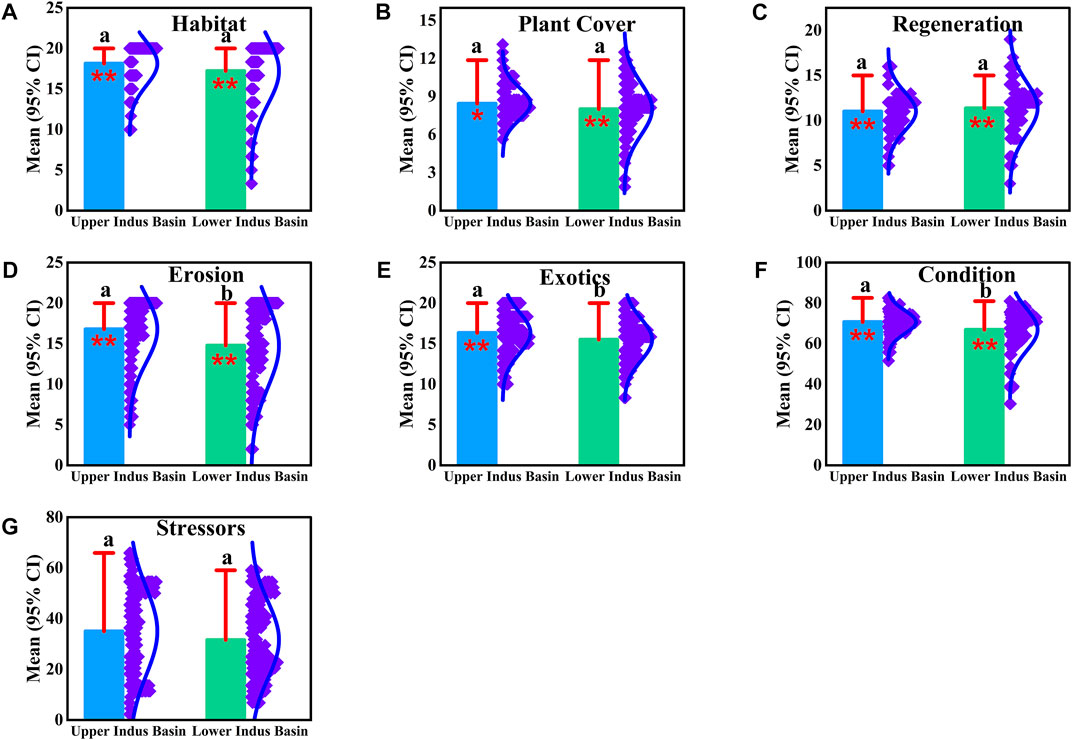
FIGURE 4. Bar graphs (A–G) for ecological networks in the riparian zones of the Indus River in Pakistan. On the y-axis are the mean scores with 95% confidence intervals (CIs). The standard deviation is represented by the red vertical bar. Data distribution is symbolized by the cyan-blue curve, while the purple rhombus represents data distribution. Note: Kruskal-Wallis’s significance levels correspond to p < 0.01 (**) or p < 0.05 (*) for independent samples. By using Dunn-Bonferroni post hoc tests, we use non-identical lowercase letters (A,B) to distinguish pairwise comparisons between the upper and lower Indus basins.
3.2 Selection of main elements by principal component analysis
The PCA plots for the UIB and LIB are displayed in Figure 5 and demonstrate the relationship between RHIs and stressors in the IRB. This study found that PCA component loadings accounted for 62.79% and 55.84% of the variations recorded for UIB (a) and UIB (b), respectively. We tested the validity of these interpretations using Kaiser-Meyer-Olkin sample adequacy tests [0.727 (a) and 0.767 (b)] and Bartlett’s test of sphericity [0.000 for both (a) and (b)]. It was found that only four stressors were significantly associated with the first significant component of the UIB (Str10, Str8, Str7, and Str4), whereas eight mixed variables (Hab1, PlCov1, PlCov4, Reg1, PlCov8, Ero1, Hab2, and Str1) significantly correlated with the first significant component of the LIB. It was evident that the first component exhibited a consistent positive association in both UIB and LIB, with values ranging from 0.942 to 0.689 and 0.942 to 0.483, respectively. The second main component contained four parameters that were combined into UIB (Exo6, Exo2, Exo5, and Exo4) and LIB (Str8, Str10, Str7, and Str4), and their correlations were favorable, ranging from 0.909 to 0.768 (a) and 0.951 to 0.643 (b). The third significant component in UIB consisted of four variables (Ero4, Ero5, Ero3, and Str3) that were inextricably linked (0.885–0.851 and ̶ 0.461), whereas four exotic variables (Exo6, Exo2, Exo5, and Exo4) in LIB ranged from 0.923 to 0.496. In UIB, the fourth significant component consisted of four variables (Hab1, PlCov1, PlCov4, and PlCov8) that were associated with each other (0.830–0.589), while in LIB, three erosion variables were found (Ero4, Ero5, and Ero3) that varied from 0.864 to 0.750. There were three strongly associated parameters for the five main components in the UIB (PlCov6, Reg2, and Str5), each at 0.778, 0.729, and ̶ 0.656. There was a negative correlation between Str3 and the other parameters. The LIB contained only two variables (PlCov2 and Reg3), ranging from 0.869 to 0.813. The sixth component had three parameters in each UIB (PlCov7, Reg3, and Str3) and LIB (Str3, Str11, and Exo3). All of the parameters in component 6 were intricately linked (0.850–0.522 in UIB and 0.808 to 0.525 in LIB).
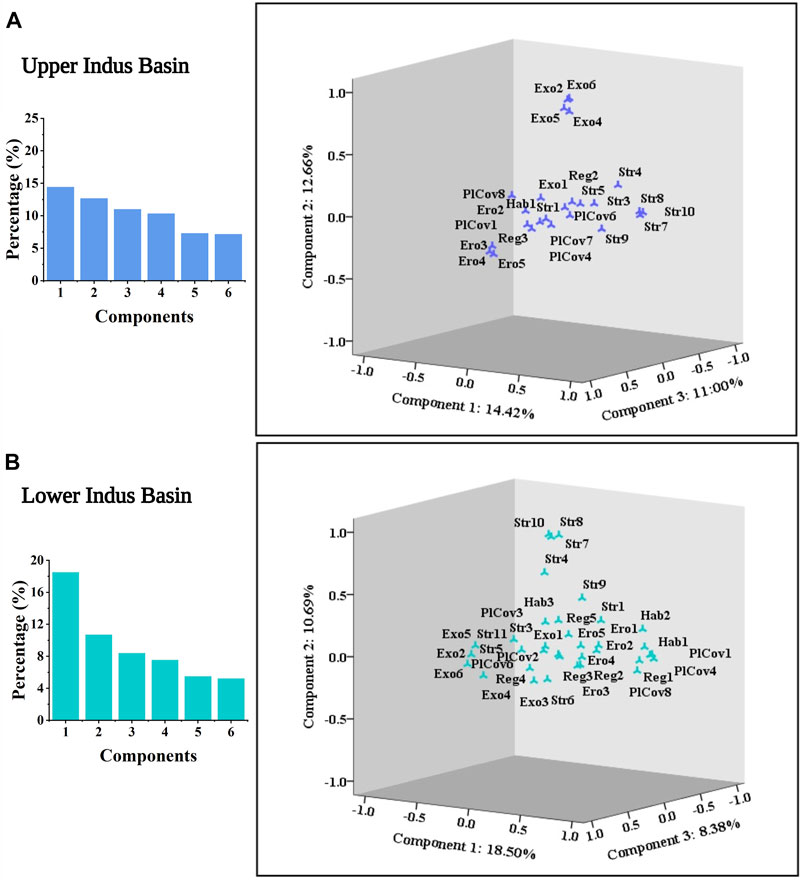
FIGURE 5. Factor analysis (principal component analysis) plots showed the riparian health indicators and stressors along the Indus River in Pakistan’s upper (A) and lower (B) basins. There is a 62.79% and 55.84% explanation for the total variation in the Indus basin riparian zone components according to the PCA factor loadings of the upper (A) and lower (B) riparian zones, respectively. Figure 3 provides further details.
3.3 Relationships of ecological parameters with riparian buffer width and stream channel width
Riparian buffer width and stream channel width–ecological indicators linear relationships at p < 0.001***, p < 0.01**, and p < 0.05* were evaluated using Pearson’s correlations in the IRB’s UIB (a) and LIB (b) sections (Figure 6). It was found that buffer width in the riparian area and stream channel width in the Indus River were positively and negatively correlated with ecological parameters. These ecological parameters were interconnected and had significant relationships with each other (Figure 6). Several ecological parameters were more affected by the changes in riparian buffer width and stream channel width in LIB, and these parameters are associated with higher correlation coefficients among the ecological parameters, ranging from ̶ 0.47*** < r < 0.71*** for riparian width and ̶ 0.41*** < r < 0.32*** for stream channel width. There was a substantial relationship between stressors and RHIs in the LIB under changing these widths, and stressors had a profound influence on plant cover (̶ 0.32*** < r < 0.38***; PlCov3 and PlCov4), habitat (̶ 0.37*** < r < 0.41***; Hab3 and Hab2), regeneration (̶ 0.29** < r < 0.25**; Reg1 and Reg3), exotics (̶ 0.39*** < r < 0.24*; Exo4 and Exo5), and erosion (̶ 0.34*** < r < 0.49***; Ero1). There was a significant correlation between the stressors in the LIB, ranging from ̶ 0.40*** < r < 0.93***; Str1, Str7, and Str8. The RHIs in the LIB also showed strong associations, with highly correlated values, ranging from ̶ 0.51*** < r < 0.93***; Ero1, PlCov1, and PlCov4. Moreover, these stressors in UIB also adversely affected plant cover (̶ 0.34*** < r < 0.26**; PlCov4 and PlCov7), habitat (̶ 0.28*** < r < 0.27**; Hab3 and Hab2), regeneration (̶ 0.19* < r < 0.26**; Reg2), exotics (̶ 0.31*** < r < 0.30***; Exo3 and Exo6), and erosion (̶ 0.38*** < r < 0.23**; Ero2 and Ero1).
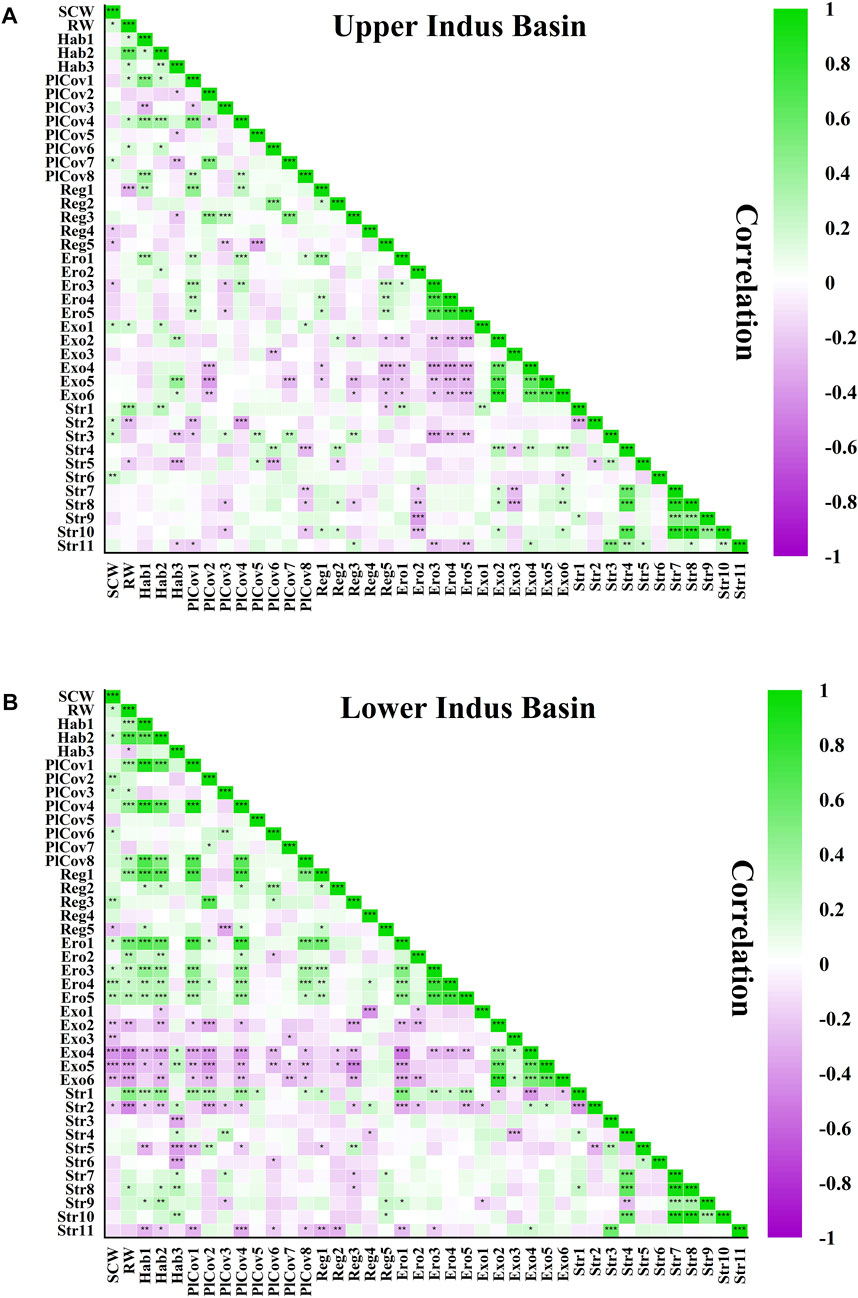
FIGURE 6. Heat maps of Pearson’s correlation for stream channel width (SCW) and riparian width (RW) with the ecological networks (A,B) in the riparian zones of the Indus River in Pakistan. Dark colors indicate strong relationships, whereas light colors indicate weak relationships. The correlations are significant at the 0.001-level (***) (two-tailed); at the 0.01-level (**) (two-tailed); and at the 0.05-level (*) (two-tailed). Figure 3 provides further details.
3.4 Analysis of similarities among indices and sub-indices from the upper and lower indus basins
We examine in more detail the statistical variations of the UIB and LIB indices and sub-indices in Figure 7. Agglomerative hierarchical clustering was used to calculate the distance between each cluster, and dendrogram plots were used to illustrate the relationship between the indices and sub-indices. Each situation was divided into seven groups for both UIB (a) and LIB (b). A similar pattern was observed in the UIB between erosion and condition, while the LIB first exhibited habitat and condition together. The second cluster of the UIB included both regeneration and plant cover, while the LIB identified erosion separately. In Cluster 3, habitat is included within the UIB, while plant cover is included within the LIB. UIB’s fourth cluster showed significant differences when stressors and exotics were grouped together, while LIB’s stressors, regeneration, and exotics appeared differently.
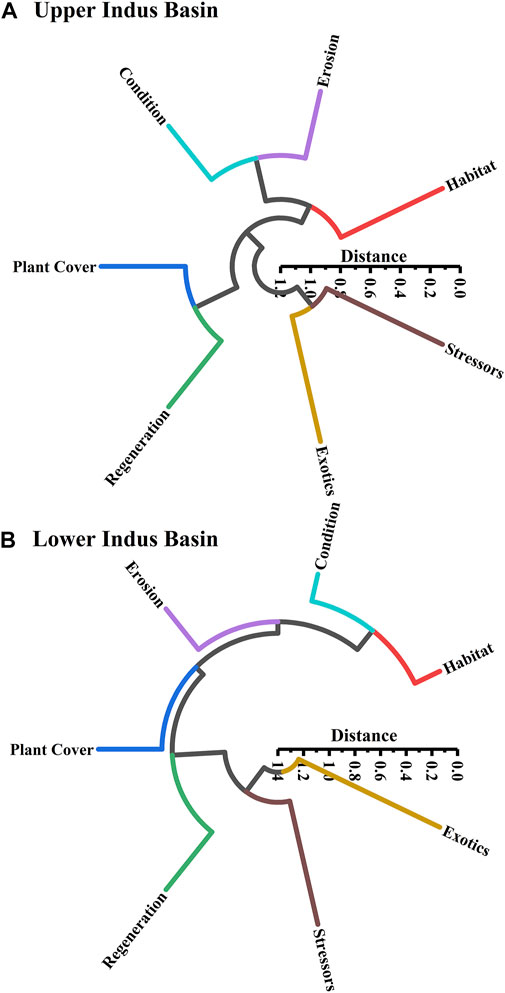
FIGURE 7. Dendrograms produced by agglomerative hierarchical clustering under the influence of channel width and riparian width for ecological network sub-indexes/index for the upper (A) and lower (B) Indus basin riparian zones of the Indus River in Pakistan.
4 Discussion
This study aims to quantify all possible RHIs under the influence of topographical changes in the UIB and LIB of the Indus River in Pakistan. It is widely acknowledged that stream channel length and riparian buffer width must be taken into consideration even in less developed countries such as Pakistan to implement sustainable water resources programs. Modern countries have long recognized this factor (Kuglerova et al., 2017), and as an example, the state of Oregon has developed several guidelines to protect a variety of riverine zones (Stutter et al., 2021). In that area, the riparian buffer varied from 0 to 152 m depending on land use and stream width (Boisjolie et al., 2017), which is consistent with the riparian width in our study area (Figure 2). However, riverine zones are not protected by well-defined policies along the Indus River. Governments are generally responsible for regulating the width of riparian buffers for both conservation and development purposes (Baggaley et al., 2020). Such rules may need to be simplified in order to make the process of maintaining river widths more practical and safer (Biswas et al., 2019). Nevertheless, riparian width should be evaluated according to the magnitude of the river (Francis et al., 2021). It is common practice to overlook stream networks when dams and reservoirs are constructed on rivers to prevent floods (Cartwright and Irivine, 2020).
Figure 4 shows RHIs and stress indices for a wide range of riparian buffer and stream channel widths. There was a significant difference between the mean scores of the UIB and the LIB regarding plant cover and regeneration. In comparison with the LIB, the UIB had higher mean ratings for habitat, exotics, erosion, overall health, and stress. The LIB may be relatively plain, which may explain the higher level of anthropogenic activities there. There is relatively hilly terrain on the UIB where anthropogenic activities have had a limited impact in Pakistan. Previously conducted research has demonstrated that artificial interruptions of rivers during river management have specific impacts (Muhammad et al., 2022a). The study has indicated that administrative procedures need to be modified regarding expanding riparian areas’ administration. Our results also indicate that the same administrative policies are not technically feasible due to the difference in their effects in the UIB and LIB within the IRB. In addition, we have demonstrated for the first time in Pakistan that riparian buffers and stream width significantly impact river ecosystems.
The riparian width retention laws in Pakistan are missing compared to those in industrialized nations such as the United States and European countries. One of the world’s most extensive continuous irrigation networks can be found in Pakistan (Charles et al., 2018). It is estimated that 95% of the country’s water resources are used for agriculture, and the remainder is used for domestic and industrial use (Naz et al., 2019; Rafique et al., 2020). Current buffer width specifications are unavailable, which could compromise the conservation and restoration of IRB’s ecological indicators (Figure 6). In light of our findings, legislators can develop similar state recommendations, but these recommendations need to be tailored to unique river conditions. The development of such policies may assist in reducing the adverse effects of stress factors on river network systems through the modification of strategies and improvement of management practices (Guo et al., 2021). However, there is still a great deal of uncertainty regarding the ecological response of tributaries to rivers and further research is necessary (Yuancai et al., 2022). The Indus River serves as the water source for the world’s largest continuous irrigation system in Pakistan. An environmentalist viewpoint indicates that rivers in Pakistan differ from those in most other countries that feature wild or semi-wild nature (Rashid et al., 2018). Often, riparian buffers are discouraged in limited stream widths where different sets of regulations apply (Hasselquist et al., 2018). Considering a minimum buffer width for each stream when undertaking large-scale projects such as those associated with the Indus River is necessary. As far as their width is concerned, they should comply with the standards in place in other countries such as the United States, Sweden, Finland, and Canada. There may not be any restrictions due to operational impossibilities or lack of knowledge regarding possible consequences. These issues require urgent attention on the part of the Pakistani government.
The use of set-width methods for riparian demarcation combined with automated approaches produces scientific information that covers both sides of the stream network (Stutter et al., 2021). It is possible to quickly characterize riparian areas using this technology and determine the necessary specifications (Tomsett and Leyland, 2019; Qianwen et al., 2022; Zheng et al., 2022). The fixed-width approach defines functions such as bank erosion, vegetation cover, and debris contribution by riverine vegetation (Haq et al., 2022; Hu et al., 2022). The question of whether stressors located in the riparian zone have substantive scientific links to RHIs is also raised by some people (Muhammad et al., 2022b). This problem has been partly addressed by Canadian researchers who compared ground and soil textures within 100-m riparian zones (Graziano et al., 2022). It was found that vegetation characteristics in the upstream area were associated with soil and land management needs at the national level (Arif and Changxiao, 2022). It compared results from a 30-m-wide riparian strip that evaluated 60 catchments in the United States (Arscott et al., 2006). Land-use changes and how streams are connected show that stress forecasts have helped in some places near the water (Deng, 2019). Similarly, there were significant correlations between the topographical and ecological indicators observed under different land uses in the Three Gorges Dam, China (Arif et al., 2022b).
The PCA plots were extremely useful in identifying key indicators from riparian zones (Figure 5). Pearson correlations have been established between the RHIs and stressors with riparian width and stream channel widths (Figure 6). Stream channel widths and riparian buffers were the most influential factors affecting RHIs and stressors in the LIB. This may be due to more variation in the topographical features in the LIB. Furthermore, we examined the relationships between the indicators and stressors. Stress indices and RHIs were more closely related in the LIB. It was found that the RHIs in LIB showed a moderate correlation with the stress indices in the IRB; however, they had a significant and strong correlation with the RHIs in LIB. There is an agreement between our findings and those of previous studies (Charles et al., 2018; Tariq et al., 2021; Hira et al., 2022).
The RHIs and stressors were correlated clearly with the riparian widths and stream sizes across the IRB riparian zones (Figure 7), considering both UIB and LIB. Differences in location and land use may explain the discrepancy. The results of this study indicate that stream channel management by administrators should not comply with the same sustainable rules (Guo et al., 2021). It is also likely that some discrepancies in buffer activities are due to differences in land use and stream structure between the two jurisdictions (Khalil et al., 2022; Majeed et al., 2022; Monteiro et al., 2022). The UIB and LIB responses in the IRB differ considerably. It is important to recognize that impacts within a single-stream network may differ and that policymakers must establish and implement standards to address these issues. There has been considerable interest in examining techniques for changing widths based on site-specific characteristics since it has long been recognized that fixed-width delimiting is ineffective during riparian transitions (Mac Nally et al., 2008). If data is available, variable-width delimitation may provide insight into the size of critical ecological reasons if fixed-width techniques are scarce. If there is insufficient data at locations, it is difficult to determine the optimal width of riparian buffers to maintain ecological function (Betz et al., 2020). Methods should be specified using data types and outputs for a variety of activities that may be involved.
The interaction between land use management and riparian zones must be considered when identifying and characterizing riparian zones (Luke et al., 2019; Haq et al., 2021a). This consequence influences the predicted role of riparian areas and the management options available to them. It was possible to protect watercourses from pollution by establishing buffer zones; to reduce soil degradation; to maintain ecosystems; and to reduce soil erosion. Failure to focus on buffer zones may be related to the inability to achieve these objectives. Degradation of waterways may be caused by incorrect sustainable conservation methods (Chen Z. et al., 2022; Behzad et al., 2022). The riparian zone can be characterized by plant type, soil type, hydrologic flow regimes, and connectivity with streams through surveys. It is essential to address rivers’ deterioration regardless of their location to prevent further degradation. Ecosystem testing aims to identify important areas, habitats, processes, and functions in a given ecosystem by combining readily available data with statistical analysis and modelling methodologies (Khuroo et al., 2007; Ullah et al., 2022). Therefore, this research discusses assessing influences on riparian zones and adjusting management procedures accordingly.
5 Conclusion
The results of our study indicated that ecological indicators and stress indices differed significantly between the upper and lower Indus basins. Statistically significant variations were found for each parameter index and sub-index based on the Kruskal-Wallis tests. The upper Indus buffer width was lower than the lower Indus buffer width. While both share the same pattern of stream channel width. We found that riparian buffers and stream channel widths had the greatest impacts on these parameters in the lower Indus basin. Compared to the upper Indus basin, the lower Indus basin had a higher correlation coefficient between riparian health indicators (habitat, plant cover, regeneration, exotics, and erosion) and stressors. In order to attain a more comprehensive understanding of the similarities and differences between the upper and lower Indus’s basins, cluster analysis was used to determine discrepancies between each set of indices or sub-indices. It was found that indexing mechanisms in the Indus River responded differently to these factors. There is a need to conduct similar studies on long river riparian areas that adhere to similar management practices. Developing new guidelines is essential to prevent the further degradation of large river ecosystems.
Data availability statement
The original contributions presented in the study are included in the article/Supplementary Material, further inquiries can be directed to the corresponding authors.
Author contributions
AH and MA conceptualized the study and organized the database. AH, MA, NZ, ZG, LX, and CY collected research samples and performed the statistical analysis. AH and MA wrote and revised the manuscript. MA and CY supervised the research. All authors contributed to the article and approved the submitted version.
Funding
This research was funded by the International Young Talents Program (No. QN2022168001L), China, and the Heilongjiang Provincial Key Foundation for Philosophy and Social Sciences (No. 21GLA449), China.
Acknowledgments
Special thanks to the relevant staff of the Terbela Dam Reservoir for providing the basic information about the reservoir.
Conflict of interest
The authors declare that the research was conducted in the absence of any commercial or financial relationships that could be construed as a potential conflict of interest.
Publisher’s note
All claims expressed in this article are solely those of the authors and do not necessarily represent those of their affiliated organizations, or those of the publisher, the editors and the reviewers. Any product that may be evaluated in this article, or claim that may be made by its manufacturer, is not guaranteed or endorsed by the publisher.
Supplementary material
The Supplementary Material for this article can be found online at: https://www.frontiersin.org/articles/10.3389/fenvs.2023.1113482/full#supplementary-material
References
Ahmed, M. F., Rogers, J. D., and Ismail, E. H. (2018). Knickpoints along the upper Indus River, Pakistan: An exploratory survey of geomorphic processes. Swiss J. Geosci. 111 (1), 191–204. doi:10.1007/s00015-017-0290-3
Arif, M., Behzad, H. M., Tahir, M., and Li, C. (2022a). The impact of ecotourism on ecosystem functioning along main rivers and tributaries: Implications for management and policy changes. J. Environ. Manage. 320, 115849. doi:10.1016/j.jenvman.2022.115849
Arif, M., Jiajia, L., Dongdong, D., Xinrui, H., Qianwen, G., Fan, Y., et al. (2022b). Effect of topographical features on hydrologically connected riparian landscapes across different land-use patterns in colossal dams and reservoirs. Sci. Total Environ. 851, 158131. doi:10.1016/j.scitotenv.2022.158131
Arif, M., and Changxiao, L. (2022). Impacts of environmental literacy on ecological networks in the Three Gorges Reservoir, China. Ecol. Indic. 145, 109571. doi:10.1016/j.ecolind.2022.109571
Arscott, D. B., Dow, C. L., and Sweeney, B. W. (2006). Landscape template of New York City's drinking-water-supply watersheds. J. North Am. Benthol. Soc. 25 (4), 867–886. doi:10.1899/0887-3593(2006)025[0867:Ltonyc]2.0.Co;2
Baggaley, N., Lilly, A., Blackstock, K., Dobbie, K., Carson, A., and Leith, F. (2020). Soil risk maps - interpreting soils data for policy makers, agencies and industry. Soil Use Manag. 36 (1), 19–26. doi:10.1111/sum.12541
Behzad, H. M., Arif, M., Duan, S., Kavousi, A., Cao, M., Liu, J., et al. (2022). Seasonal variations in water uptake and transpiration for plants in a karst critical zone in China. Sci. Total Environ. 860, 160424. doi:10.1016/j.scitotenv.2022.160424
Betz, F., Lauermann, M., and Cyffka, B. (2020). Open source riverscapes: Analyzing the corridor of the Naryn River in Kyrgyzstan based on open access data. Remote Sens. 12 (16), 2533. doi:10.3390/rs12162533
Biswas, S. R., Mallik, A. U., Braithwaite, N. T., and Biswas, P. L. (2019). Effects of disturbance type and microhabitat on species and functional diversity relationship in stream-bank plant communities. For. Ecol. Manag. 432, 812–822. doi:10.1016/j.foreco.2018.10.021
Boisjolie, B. A., Santelmann, M. V., Flitcroft, R. L., and Duncan, S. L. (2017). Legal ecotones: A comparative analysis of riparian policy protection in the Oregon coast range, USA. J. Environ. Manage. 197, 206–220. doi:10.1016/j.jenvman.2017.03.075
Bombino, G., Zema, D. A., Denisi, P., Lucas-Borja, M. E., Labate, A., and Zimbone, S. M. (2019). Assessment of riparian vegetation characteristics in Mediterranean headwaters regulated by check dams using multivariate statistical techniques. Sci. Total Environ. 657, 597–607. doi:10.1016/j.scitotenv.2018.12.045
Cartwright, I., and Irivine, D. (2020). The spatial extent and timescales of bank infiltration and return flows in an upland river system: Implications for water quality and volumes. Sci. Total Environ. 743, 140748. doi:10.1016/j.scitotenv.2020.140748
Charles, S. P., Wang, Q. J., Ahmad, M. D., Hashmi, D., Schepen, A., Podger, G., et al. (2018). Seasonal streamflow forecasting in the upper Indus basin of Pakistan: An assessment of methods. Hydrol. Earth Syst. Sci. 22 (6), 3533–3549. doi:10.5194/hess-22-3533-2018
Chen, D., Zhao, Q., Jiang, P., and Li, M. (2022a). Incorporating ecosystem services to assess progress towards sustainable development goals: A case study of the yangtze river economic belt, China. Sci. Total Environ. 806, 151277. doi:10.1016/j.scitotenv.2021.151277
Chen, Z., Song, H., Arif, M., and Li, C. (2022b). Effects of hydrological regime on Taxodium ascendens plant decomposition and nutrient dynamics in the Three Gorges Reservoir riparian zone. Front. Environ. Sci. 10, 990485. doi:10.3389/fenvs.2022.990485
Dai, X., Zhou, Y., Ma, W., and Zhou, L. (2017). Influence of spatial variation in land-use patterns and topography on water quality of the rivers inflowing to Fuxian Lake, a large deep lake in the plateau of southwestern China. Ecol. Eng. 99, 417–428. doi:10.1016/j.ecoleng.2016.11.011
Deng, X. (2019). Correlations between water quality and the structure and connectivity of the river network in the Southern Jiangsu Plain, Eastern China. Sci. Total Environ. 664, 583–594. doi:10.1016/j.scitotenv.2019.02.048
Ding, D., Arif, M., Liu, M., Li, J., Hu, X., Geng, Q., et al. (2022). Plant-soil interactions and C:N:P stoichiometric homeostasis of plant organs in riparian plantation. Front. Plant Sci. 13, 979023. doi:10.3389/fpls.2022.979023
Dixon, I., Douglas, M., Dowe, J., and Burrows, D. (2006). Tropical rapid appraisal of riparian condition version 1 (for use in tropical savannas), River Management technical guideline No. 7. Canberra: Land and Water Australia.
Francis, T. B., Abbott, K. C., Cuddington, K., Gellner, G., Hastings, A., Lai, Y.-C., et al. (2021). Management implications of long transients in ecological systems. Nat. Ecol. Evol. 5 (3), 285–294. doi:10.1038/s41559-020-01365-0
Fu, L., Ren, Y., Lu, L., and Chen, H. (2022). Relationship between ecosystem services and rural residential well-being in the Xin’an river Basin, China. Ecol. Indic. 140, 108997. doi:10.1016/j.ecolind.2022.108997
Graziano, M. P., Deguire, A. K., and Surasinghe, T. D. (2022). Riparian buffers as a critical landscape feature: Insights for riverscape conservation and policy renovations. Diversity 14 (3), 172. doi:10.3390/d14030172
Gundersen, P., Lauren, A., Finer, L., Ring, E., Koivusalo, H., Saetersdal, M., et al. (2010). Environmental services provided from riparian forests in the nordic countries. Ambio 39 (8), 555–566. doi:10.1007/s13280-010-0073-9
Guo, Z., Boeing, W. J., Borgomeo, E., Xu, Y., and Weng, Y. (2021). Linking reservoir ecosystems research to the sustainable development goals. Sci. Total Environ. 781, 146769. doi:10.1016/j.scitotenv.2021.146769
Habib ur, R., Rehman, M. A., Naeem, U. A., Hashmi, H. N., and Shakir, A. S. (2017). Possible options to slow down the advancement rate of Tarbela delta. Environ. Monit. Assess. 190 (1), 39. doi:10.1007/s10661-017-6376-6
Haq, S. M., Malik, Z. A., and Rahman, I. U. (2019). Quantification and characterization of vegetation and functional trait diversity of the riparian zones in protected forest of Kashmir Himalaya, India. Nord. J. Bot. 37 (11), njb.02438. doi:10.1111/njb.02438
Haq, S. M., Hamid, M., Lone, F. A., and Singh, B. (2021a). Himalayan hotspot with alien weeds: A case study of biological spectrum, phenology, and diversity of weedy plants of high altitude mountains in district kupwara of J&K himalaya, India. Proc. Natl. Acad. Sci. India, Sect. B Biol. Sci. 91, 139–152. doi:10.1007/s40011-020-01219-6
Haq, S. M., Shah, A. A., Yaqoob, U., and Hassan, M. (2021b). Floristic quality assessment index of the dagwan stream in dachigam national park of Kashmir himalaya. Proc. Natl. Acad. Sci. India, Sect. B Biol. Sci. 91, 657–664. doi:10.1007/s40011-021-01247-w
Haq, S. M., Calixto, E. S., Rashid, I., Srivastava, G., and Khuroo, A. A. (2022). Tree diversity, distribution and regeneration in major forest types along an extensive elevational gradient in Indian Himalaya: Implications for sustainable forest management. For. Ecol. Manag. 506, 119968. doi:10.1016/j.foreco.2021.119968
Hasselquist, E. M., Lidberg, W., Sponseller, R. A., Agren, A., and Laudon, H. (2018). Identifying and assessing the potential hydrological function of past artificial forest drainage. Ambio 47 (5), 546–556. doi:10.1007/s13280-017-0984-9
Hira, A., Arif, M., Zarif, N., Gul, Z., Liu, X., and Cao, Y. (2022). Impacts of stressors on riparian health indicators in the upper and lower Indus River Basins in Pakistan. Int. J. Environ. Res. Public Health 19 (20), 13239. doi:10.3390/ijerph192013239
Hu, X., Arif, M., Ding, D., Li, J., He, X., and Li, C. (2022). Invasive plants and species richness impact litter decomposition in riparian zones. Front. Plant Sci. 13, 955656. doi:10.3389/fpls.2022.955656
Hussain, S., Qin, S., Nasim, W., Bukhari, M. A., Mubeen, M., Fahad, S., et al. (2022). Monitoring the dynamic changes in vegetation cover using spatio-temporal remote sensing data from 1984 to 2020. Atmosphere 13, 1609. doi:10.3390/atmos13101609
Islam, F., Riaz, S., Ghaffar, B., Tariq, A., Shah, S. U., Nawaz, M., et al. (2022). Landslide susceptibility mapping (LSM) of Swat district, Hindu Kush Himalayan region of Pakistan, using GIS-based bivariate modeling. Front. Environ. Sci. 10, 1027423. doi:10.3389/fenvs.2022.1027423
Jalayer, S., Sharifi, A., Abbasi-Moghadam, D., Tariq, A., and Qin, S. (2022). Modeling and predicting land use land cover spatiotemporal changes: A case study in chalus watershed, Iran. IEEE J. Sel. Top. Appl. Earth Obs. Remote Sens. 15, 5496–5513. doi:10.1109/JSTARS.2022.3189528
Jansen, A., Robertson, A., Leigh, T., and Andrea, W. (2005). Rapid Appraisal of Riparian Condition, Vol. 4A, 16p.
Jullian, C., Nahuelhual, L., and Laterra, P. (2021). The Ecosystem Service Provision Index as a generic indicator of ecosystem service supply for monitoring conservation targets. Ecol. Indic. 129, 107855. doi:10.1016/j.ecolind.2021.107855
Khalil, U., Imtiaz, I., Aslam, B., Ullah, I., Tariq, A., and Qin, S. (2022). Comparative analysis of machine learning and multi-criteria decision making techniques for landslide susceptibility mapping of Muzaffarabad district. Front. Environ. Sci. 10, 1028373. doi:10.3389/fenvs.2022.1028373
Khuroo, A. A., Rashid, I., Reshi, Z., Dar, G. H., and Wafai, B. A. (2007). The alien flora of Kashmir Himalaya. Biol. Invasions 9, 269–292. doi:10.1007/s10530-006-9032-6
Kuglerova, L., Hasselquist, E. M., Richardson, J. S., Sponseller, R. A., Kreutzweiser, D. P., and Laudon, H. (2017). Management perspectives on Aqua incognita: Connectivity and cumulative effects of small natural and artificial streams in boreal forests. Hydrol. Process. 31 (23), 4238–4244. doi:10.1002/hyp.11281
Kuglerova, L., Jyvasjarvi, J., Ruffing, C., Muotka, T., Jonsson, A., Andersson, E., et al. (2020). Cutting edge: A comparison of contemporary practices of riparian buffer retention around small streams in Canada, Finland, and Sweden. Water Resour. Res. 56 (9). doi:10.1029/2019wr026381
Lanzanova, D., Whitney, C., Shepherd, K., and Luedeling, E. (2019). Improving development efficiency through decision analysis: Reservoir protection in Burkina Faso. Environ. Model. Softw. 115, 164–175. doi:10.1016/j.envsoft.2019.01.016
Lees, A. C., and Peres, C. A. (2008). Conservation value of remnant riparian forest corridors of varying quality for Amazonian birds and mammals. Conserv. Biol. 22 (2), 439–449. doi:10.1111/j.1523-1739.2007.00870.x
Luke, S. H., Slade, E. M., Gray, C. L., Annammala, K. V., Drewer, J., Williamson, J., et al. (2019). Riparian buffers in tropical agriculture: Scientific support, effectiveness and directions for policy. J. Appl. Ecol. 56 (1), 85–92. doi:10.1111/1365-2664.13280
Mac Nally, R., Molyneux, G., Thomson, J. R., Lake, P. S., and Read, J. (2008). Variation in widths of riparian-zone vegetation of higher-elevation streams and implications for conservation management. Plant Ecol. 198 (1), 89–100. doi:10.1007/s11258-007-9387-5
Majeed, M., Lu, L., Haq, S. M., Waheed, M., Sahito, H. A., Fatima, S., et al. (2022). Spatiotemporal distribution patterns of climbers along an abiotic gradient in Jhelum district, Punjab, Pakistan. Forests 13, 1244. doi:10.3390/f13081244
Mitchell, S. L., Edwards, D. P., Bernard, H., Coomes, D., Jucker, T., Davies, Z. G., et al. (2018). Riparian reserves help protect forest bird communities in oil palm dominated landscapes. J. Appl. Ecol. 55 (6), 2744–2755. doi:10.1111/1365-2664.13233
Monteiro, L. d. S., Oliveira-Júnior, J. F. d., Ghaffar, B., Tariq, A., Qin, S., Mumtaz, F., et al. (2022). Rainfall in the urban area and its impact on climatology and population growth. Atmosphere 13, 1610. doi:10.3390/atmos13101610
Muhammad, A., Behzad, H. M., Tahir, M., and Changxiao, L. (2022a). Nature-based tourism influences ecosystem functioning along waterways: Implications for conservation and management. Sci. Total Environ. 842, 156935. doi:10.1016/j.scitotenv.2022.156935
Muhammad, A., Jiajia, L., Tahir, M., Jie, Z., and Changxiao, L. (2022b). Environmental literacy scenarios lead to land degradation and changes in riparian zones: Implications for policy in China. Land Degrad. Dev. 4450, 156–172. doi:10.1002/ldr.4450
Muhammad, A., Qi, Y., Dong, Z., and Wei, H. (2022c). Rapid retrieval of cadmium and lead content from urban greenbelt zones using hyperspectral characteristic bands. J. Clean. Prod. 374, 133922. doi:10.1016/j.jclepro.2022.133922
Naz, S., Ahsanuddin, M., Inayatullah, S., Siddiqi, T. A., and Imtiaz, M. (2019). Copula-based bivariate flood risk assessment on Tarbela dam, Pakistan. Hydrology 6 (3), 79. doi:10.3390/hydrology6030079
Olden, A., Selonen, V. A. O., Lehkonen, E., and Kotiaho, J. S. (2019). The effect of buffer strip width and selective logging on streamside plant communities. Bmc Ecol. 19, 9. doi:10.1186/s12898-019-0225-0
Oliveira, C. F., do Valle Junior, R. F., Valera, C. A., Rodrigues, V. S., Sanches Fernandes, L. F., and Leal Pacheco, F. A. (2019). The modeling of pasture conservation and of its impact on stream water quality using Partial Least Squares-Path Modeling. Sci. Total Environ. 697, 134081. doi:10.1016/j.scitotenv.2019.134081
Poff, B., Koestner, K. A., Neary, D. G., and Henderson, V. (2011). Threats to riparian ecosystems in western North America: An analysis of existing literature. J. Am. Water Resour. Assoc. 47 (6), 1241–1254. doi:10.1111/j.1752-1688.2011.00571.x
Qianwen, G., Arif, M., Zhongxun, Y., Jie, Z., Xinrui, H., Dongdong, D., et al. (2022). Plant species composition and diversity along successional gradients in arid and semi-arid regions of China. For. Ecol. Manag. 524, 120542. doi:10.1016/j.foreco.2022.120542
Rafique, A., Burian, S., Hassan, D., and Bano, R. (2020). Analysis of operational changes of Tarbela reservoir to improve the water supply, hydropower generation, and flood control objectives. Sustainability 12 (18), 7822. doi:10.3390/su12187822
Rashid, M. U., Latif, A., and Azmat, M. (2018). Optimizing irrigation deficit of multipurpose cascade reservoirs. Water Resour. Manag. 32 (5), 1675–1687. doi:10.1007/s11269-017-1897-x
Rehman, Q. F. U., Zhang, F., Pant, R. R., Wang, G., Khan, S., and Zeng, C. (2018). Spatial variation, source identification, and quality assessment of surface water geochemical composition in the Indus River Basin, Pakistan. Environ. Sci. Pollut. Res. 25 (13), 12749–12763. doi:10.1007/s11356-018-1519-z
Ring, E., Johansson, J., Sandstrom, C., Bjarnadottir, B., Finer, L., Libiete, Z., et al. (2017). Mapping policies for surface water protection zones on forest land in the Nordic-Baltic region: Large differences in prescriptiveness and zone width. Ambio 46 (8), 878–893. doi:10.1007/s13280-017-0924-8
Sliva, L., and Williams, D. D. (2001). Buffer zone versus whole catchment approaches to studying land use impact on river water quality. Water Res. 35 (14), 3462–3472. doi:10.1016/s0043-1354(01)00062-8
Stutter, M., Baggaley, N., Huallachain, D. O., and Wang, C. (2021). The utility of spatial data to delineate river riparian functions and management zones: A review. Sci. Total Environ. 757, 143982. doi:10.1016/j.scitotenv.2020.143982
Tariq, A., Shu, H., Kuriqi, A., Siddiqui, S., Gagnon, A. S., Lu, L., et al. (2021). Characterization of the 2014 Indus River flood using hydraulic simulations and satellite images. Remote Sens. 13 (11), 2053. doi:10.3390/rs13112053
Tata, L. R. R. (2021). Biodiversity impact assessment of two large dam projects in India under long term multi-scenarios simulation. Impact Assess. Proj. apprais. 39 (4), 335–347. doi:10.1080/14615517.2021.1916263
Tomsett, C., and Leyland, J. (2019). Remote sensing of river corridors: A review of current trends and future directions. River Res. Appl. 35 (7), 779–803. doi:10.1002/rra.3479
Ullah, I., Aslam, B., Shah, S. H. I. A., Tariq, A., Qin, S., Majeed, M., et al. (2022). An integrated approach of machine learning, remote sensing, and GIS data for the landslide susceptibility mapping. Land 11, 1265. doi:10.3390/land11081265
Vilchez Mendoza, S., Harvey, C. A., Saenz, J. C., Casanoves, F., Pablo Carvajal, J., Gonzalez Villalobos, J., et al. (2014). Consistency in bird use of tree cover across tropical agricultural landscapes. Ecol. Appl. 24 (1), 158–168. doi:10.1890/13-0585.1
Wang, Y., Zhang, N., Wang, D., and Wu, J. (2020). Impacts of cascade reservoirs on Yangtze River water temperature: Assessment and ecological implications. J. Hydrol. 590, 125240. doi:10.1016/j.jhydrol.2020.125240
Yuancai, Q., Arif, M., Dong, Z., Ting, W., Qin, Y., Bo, P., et al. (2022). The effect of hydrological regimes on the concentrations of nonstructural carbohydrates and organic acids in the roots of Salix matsudana in the Three Gorges Reservoir, China. Ecol. Indic. 142, 109176. doi:10.1016/j.ecolind.2022.109176
Keywords: Indus River, Terbela Dam Reservoir, ecosystem function, riparian health indicators, plant cover, stressors
Citation: Hira A, Arif M, Zarif N, Gul Z, Xiangyue L and Yukun C (2023) Effects of riparian buffer and stream channel widths on ecological indicators in the upper and lower Indus River basins in Pakistan. Front. Environ. Sci. 11:1113482. doi: 10.3389/fenvs.2023.1113482
Received: 01 December 2022; Accepted: 14 February 2023;
Published: 23 February 2023.
Edited by:
Arnaldo Marín, University of Murcia, SpainReviewed by:
Amit Kumar Tiwari, Banaras Hindu University, IndiaShiekh Marifatul Haq, University of Kashmir, India
Pir Mohammad, Hong Kong Polytechnic University, Hong Kong SAR, China
Copyright © 2023 Hira, Arif, Zarif, Gul, Xiangyue and Yukun. This is an open-access article distributed under the terms of the Creative Commons Attribution License (CC BY). The use, distribution or reproduction in other forums is permitted, provided the original author(s) and the copyright owner(s) are credited and that the original publication in this journal is cited, in accordance with accepted academic practice. No use, distribution or reproduction is permitted which does not comply with these terms.
*Correspondence: Muhammad Arif, bXVoYW1tYWRhcmlmQHN3dS5lZHUuY24=; Cao Yukun, Y3lrbGtAMTYzLmNvbQ==
†These authors have contributed equally to this work