- 1INTERRA Research Institute, Universidad de Extremadura, Cáceres, Spain
- 2School of Water, Energy and Environment, Cranfield University, Bedfordshire, United Kingdom
- 3Sant’Anna School of Advanced Studies, Pisa, Italy
Agroforestry integrates woody vegetation with crop and/or livestock production to benefit from the ecological and economic interactions. The objective of this paper is to systematically determine the spatial distribution of agroforestry in the EU, and changes in the areas and types of agroforestry from 2009 to 2018. This was achieved using the Land Use/Cover Area Statistical (LUCAS) dataset. Agroforestry was categorised into silvopastoral, silvoarable, agrosilvopastoral, grazed permanent crops, intercropped permanent crops and kitchen gardens systems. In our categorisation of ‘agroforestry’, sites combing trees and shrubs with understorey grass or forage were required to show evidence of grazing. In 2018, the total area of agroforestry in the EU28 was 114,621 km2 equivalent to 6.4% of the utilised agricultural area (UAA), and a majority located in the Mediterranean bioregion. Silvopastoral was the most widespread system, representing 81% of the total agroforestry area (5% of UAA), with almost a third of that area present in Spain. An initial analysis of the LUCAS data suggested that the area of agroforestry increased from 2009 to 2012, before declining from 2012 to 2018. However our subsequent analysis suggests that the area of agroforestry in 2009 was underestimated due to a mis-categorisation of some grazing areas. After making corrections, we calculated that the area of agroforestry (using the above definitions) in the EU23 (a full-time sequence for the EU28 is unavailable) declined by 47% between 2009 and 2018. This decline is primarily due to a reduction in outdoor grazing, perhaps driven by reduced livestock numbers and/or permanent livestock housing. The only agroforestry system showing an increase was kitchen gardens (7%). The paper highlights the usefulness of the LUCAS dataset for studying the extent of agroforestry in Europe, but also potential limitations in terms of the consistency of the location of data points and the categorisation of grazing. The paper also argues that although the area of within-field agroforestry may be declining, the drive towards net zero greenhouse gas emissions may be re-establishing the link between increased tree cover and food production at a farm-level.
1 Introduction
Agroforestry has been defined as ‘the practice of deliberately integrating woody vegetation (trees or shrubs) with crop and/or animal systems to benefit from the resulting ecological and economic interactions’ (Burgess and Rosati, 2018). In many places, it is a traditional land use system, and it is estimated that globally there is 1 billion ha of agricultural land with more than 10% tree cover (Zomer et al., 2014), i.e., about 7% of the global land area.
In Europe, agroforestry includes a wide diversity of systems such as silvopastoral, silvoarable, and agrosilvopastoral systems, forest farming, home gardens, improved fallow, grazed forests, and intercropped and grazed orchards (Mosquera-Losada et al., 2009; Nerlich et al., 2013; Pantera et al., 2021). Examples of silvopastoral systems in Mediterranean parts of Europe include the montados in Portugal and the dehesas in Spain where cattle graze beneath cork and holm oaks (Moreno et al., 2018). Other examples include the cork oak forest systems in Sardinia, known as sugherete, and valonia oak systems in Greece (den Herder et al., 2015). The combination of fruit trees with crops or grazing livestock is known as streuobst in central Europe (Herzog, 1998). In the boreal forest and subarctic tundra, reindeer husbandry is widespread, estimated to occupy more than 40 million ha of land in Sweden, Norway and Finland (Jernsletten and Klokov, 2002). In some frameworks, agroforestry also includes the integration of woody landscape features on cropland or grassland, such as hedges, windbreaks, and riparian buffer strips (Mosquera-Losada et al., 2009). For example, hedges and scattered trees occupy more than 400,000 ha in France and parts of the UK and Belgium (den Herder et al., 2015), and windbreaks are a common practice in Hungary (Takács and Frank, 2008).
Trees in arable and grassland systems can be an important source not only of food but also of products such as fuelwood, fibre, timber, gums and resins, and craft products. Agroforestry can also provide recreational opportunities, such as hunting and tourism, and mosaic landscapes are often valued for their diversity and attractiveness of the landscape (Fagerholm et al., 2019; Augére-Granier, 2020). There is also widespread recognition of the environmental benefits of agroforestry compared to conventional agricultural systems. Agroforestry systems can improve the soil health of arable systems, as the trees can increase soil organic matter and retain nutrients (Jose et al., 2009). Integrating trees in crop systems can also reduce soil erosion by increasing the permanence of vegetation cover (Jose et al., 2009) and windbreaks and shelterbelts can reduce windspeed (Bartus et al., 2017; Kučera et al., 2020). Trees on arable land can increase rainfall infiltration rates and soil water retention and reduce nitrate leaching (Siriri et al., 2013). In livestock systems, tree canopies can moderate the temperature with benefits for animal welfare, health, and productivity (Atangana et al., 2014). Trees also provide a habitat for arthropods and some species of birds (Jose et al., 2009; Torralba et al., 2016; Santos et al., 2019; Pantera et al., 2021). Trees and shrubs can increase the amount of soil carbon stored in arable systems (Kirby and Potvin, 2007; Upson et al., 2016; Kay et al., 2019) and the biomass carbon stored in crop and grassland systems (Beka et al., 2023). Tölgyse et al. (2023) reported that trees in temperate deciduous forest wood-pastures in Hungary and Romania reduced herbage growth, but this was offset by positive effects in terms of storing carbon and providing shade and microhabitats. In addition, the presence of management can reduce fire risk relative to shrubland systems (Damianidis et al., 2021). For these reasons, agroforestry is recognised as a sustainable climate-smart agriculture option (FAO and ICRAF, 2019) and hence there is interest in its spatial extent.
Initial reports on the extent of agroforestry in Europe were typically based on literature reviews (Herzog, 1998; Eichhorn et al., 2006; Bergmeier et al., 2010). However, since 2006, Eurostat has carried out the Land Use/Cover Area frame Statistical (LUCAS) survey across the European Union every 3 years, which comprises land cover, land use, agro-environmental and soil data determined by field observation at geographically referenced points (Eurostat, 2009). At each LUCAS point, the land cover (LC) and land use (LU) were recorded. Land cover is the type of physical cover of the land around the point and land use is the socio-economic use. An important feature of LUCAS is that it allows multiple land covers, i.e., where there are multiple layers, such as in agroforestry, the recorder can both report a tree layer and a secondary layer composed of crops or grasses. Thus, the LUCAS surveys was used by den Herder et al. (2017) to determine that the total area under agroforestry in the EU (27 member states) in 2012 was 15.4 million ha, equivalent to about 4% of the territorial area or 9% of the Utilised Agricultural Area (UAA). Den Herder et al. (2017) differentiated three main categories of agroforestry: livestock agroforestry (where livestock production is integrated with trees), high value tree agroforestry (where the primary land use was permanent woody crops such as fruit orchards, olive groves, and nut trees combined with grazing or arable/temporal crops), and arable agroforestry (arable crops integrated with trees). Mosquera-Losada et al. (2018) calculated a larger extent of 19.8 million ha in 2012 by also adding the extent of kitchen gardens. Kitchen gardens, also called home gardens, typically refer to fenced plots in residential areas and allotments where a variety of crops are grown for home consumption. Also using the LUCAS database from 2012, Plieninger et al. (2015a) estimated that pastures in open woodlands, and pastures with sparse or cultivated trees (but not necessarily grazed) in the EU covered 20.3 million ha.
Some authors have suggested that the area of agroforestry is decreasing as farmers remove trees from arable systems to enable easier mechanisation (Eichhorn et al., 2006). In addition, for permanent crops such as olives and apples, there has been a move from high-stem trees (which allowed understorey grazing) to trees grown on dwarf rootstocks (Oosterbaan and Kuiters, 2009; Bergmeier et al., 2010). In Germany, in the last 40 years, streuobst of apple and pear trees have also been converted to grasslands, fields and development areas (Bergmeier et al., 2010), with Küpfer and Balko (2010) reporting a 50% decline. A study of the area of orchard meadows for a region in Southwestern Germany suggested a decline from 16% of the area in 1968 to 12% in 2009, an annual loss rate of 1% (Plieninger er al., 2015b). Other cited reasons for the reduced cover of individual trees include poor tree regeneration due to overgrazing, and new pests (Bergmeier et al., 2010).
To our knowledge, there has been no attempt to use LUCAS data from surveys conducted between 2009 and 2018 to investigate whether the area of agroforestry in Europe is increasing, decreasing, or stable. Hence, the aim of this paper is to evaluate the changes that have occurred in agroforestry in Europe, including the following specific objectives: 1) to determine the current extent of different agroforestry systems in the EU using LUCAS data from 2018; 2) to assess the changes in agroforestry from 2009 to 2018 and examine the implications for future trends; 3) to analyse the causes contributing to variations during the study period; 4) to investigate trends in grazing and their relationship with the changes in the different agroforestry systems; and 5) to evaluate the usefulness of LUCAS data for this type of studies.
2 Material and methods
2.1 Description of the LUCAS database
The extent of agroforestry systems in the EU were determined using LUCAS data, which are based on a two-phases sampling strategy (Buck et al., 2015). In the first phase, points are systematically sampled in a regular grid with a spacing of 2 km in the four cardinal directions covering the whole territory of the EU. This survey contains around 1.1 million different points (the so-called master sample or frame) (Eurostat, 2019a). Each point of the first phase sample is photo-interpreted and assigned a pre-defined land cover class: artificial land, cropland, woodland, shrubland, grassland, bare land, water area or wetland (Buck et al., 2015).
From the first phase sample, a second phase sample of points is drawn randomly and proportionally with respect to the assigned land cover class (more detail on the sampling procedure in Buck et al., 2015; Ballin et al., 2018). These points are visited in the field by surveyors, who record land cover, land use and other environmental parameters. Land cover and land use are recorded according to a harmonised classification system. The surveyor also collects information related to the percentage of land cover within a specific window of observation, including the area size, the width of any specific feature, the height of trees, information on land and water management, such as grazing or irrigation (Eurostat, 2019a). Those points which could not be visited for any reason, for example, due to problems of accessibility, are evaluated using office-based photointerpretation (Ballin et al., 2018). Regarding the geographical location, in the first place the coordinates of the theoretical location are established, and once in the field, the coordinates of the real sampling point are measured with a geographical positioning system (GPS). Those points which could not be visited and that are analysed by photointerpretation are assigned the theoretical coordinates. The LUCAS database includes both coordinates, the real and the theoretical ones.
The basic sampling and survey methods have remained the same throughout all surveys. Nevertheless, the goals of the surveys and their implementation in new countries to the survey have changed and have led to different sample point allocations for different land covers (d’Andrimont et al., 2020). Table 1 presents the number of sample points for each EU member state and for the different survey years. The LUCAS 2006 survey was not included in the study because of its small number of points and the few countries included in the sample (Belgium, Czechia, Germany, France, Italy, Luxembourg, Hungary, Netherlands, Poland and Slovakia). Between 2009 and 2015 the number of sample points increased from 234,484 to 338,725, related mainly with an increment of points per previous countries (+78,269), but also with the inclusion of new countries in the survey (+25,972 points: Bulgaria, Cyprus, Croatia, Malta, Romania). Between 2015 and 2018 the total number of points remained almost unchanged, with some countries registering increases and others decreases. For example, the number of points of the Netherlands almost doubled, whilst Portugal registered a decrease.
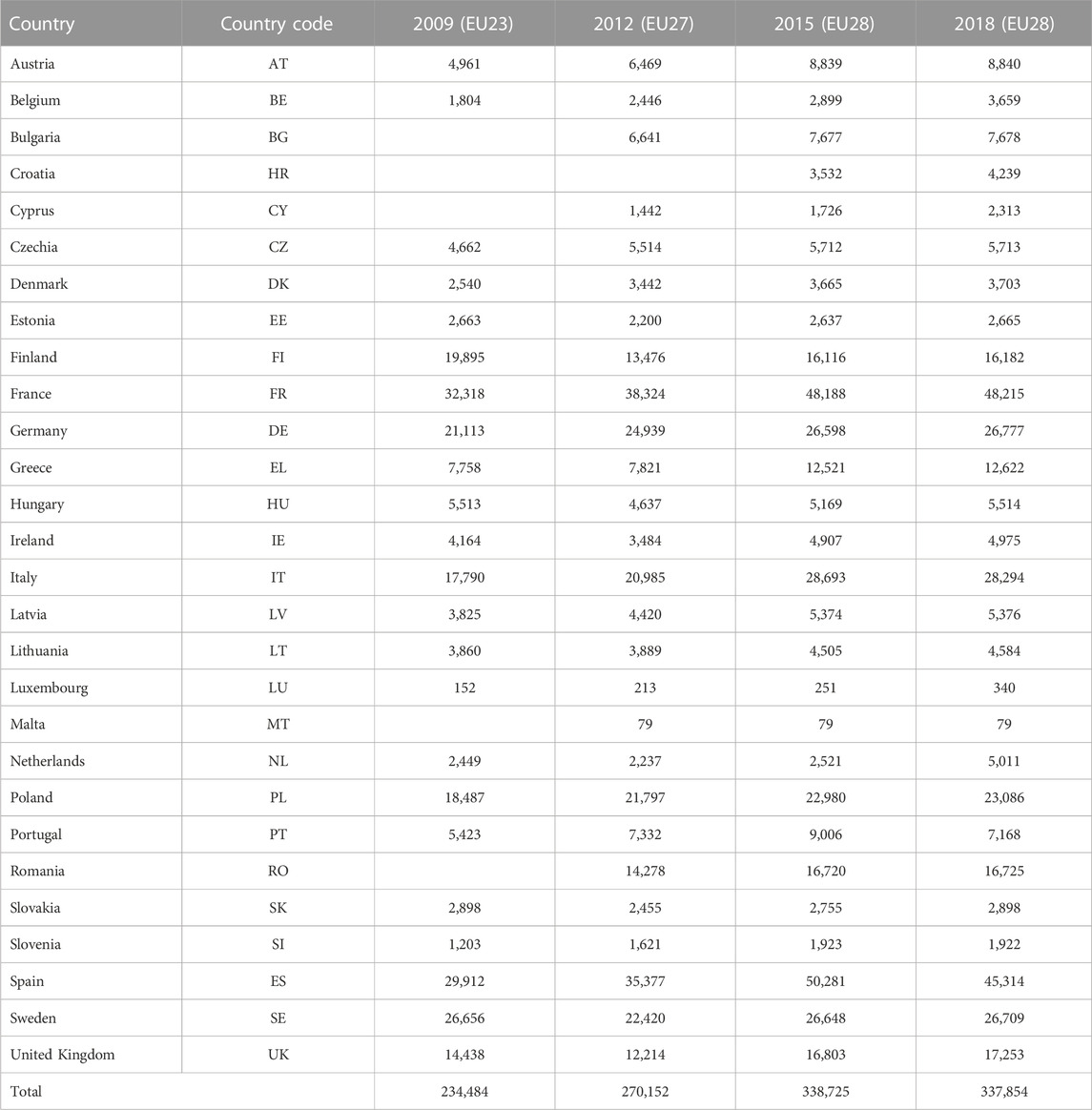
TABLE 1. Number of sample points of each LUCAS survey for each of 28 European countries over 4 years.
LUCAS points provide information about the land cover and land use of the sampled plot. To classify different types of agroforestry, we assumed that these systems always had a primary land cover (LC1) comprising a tree or shrub layer, and a secondary land cover (LC2) including other types of covers, such as crops, grass or, even, tree and shrub layers. The land use component of the LUCAS database includes information on whether the point has agricultural, forestry or other land uses. Kitchen gardens, for example, are considered as an individual land use.
Following instructions for surveyors (Eurostat, 2019a), land management could be classified as ‘signs of grazing’, ‘no signs of grazing’, or ‘grazing not relevant’.‘Signs of grazing’ was recorded when the surveyor observed cattle feeding; cattle infrastructure such as fences, stables, and drinking troughs; and/or dung or cattle trampling. Areas grazed during summer (transhumance) are also considered. If the land cover is suitable for grazing (croplands, woodlands, shrublands, grasslands, wetlands or bare lands) but no signs of grazing are visible, it is marked ‘no signs of grazing’. Finally, if the land cover is not suitable to be grazed (artificial or water areas) it must be assigned as ‘grazing not relevant’. In this way, the selection of certain combinations of primary and secondary land covers, as well as information on land use and management, enabled the identification of agroforestry points and their classification.
The LUCAS surveys in 2009, 2012 and 2015 also included information on woody linear features, which are examined along a 250 m transect running east from the observation point. Agricultural land with woody linear features, although a form of agroforestry, were not included in the present study because the converted length to area requires additional assumptions, and the lack of transect data collected in 2018 prevents a direct comparison between 2009 and 2018.
2.2 Classification of agroforestry systems
Agroforestry systems were classified in six principal classes: kitchen gardens, grazed permanent crops, intercropped permanent crops, silvoarable, silvopastoral, and agrosilvopastoral (Table 2). The classification was completed using the harmonised LUCAS database published by Palmieri et al. (2020). This has the advantage of reducing the complexity and layered nature of the original LUCAS datasets, avoiding inconsistencies and errors between legends and labels from one survey to the next. Furthermore, this harmonization corrected missing internal cross-references in the dataset, which limited the capacity to compute and link the observed variables (d’Andrimont et al., 2020).
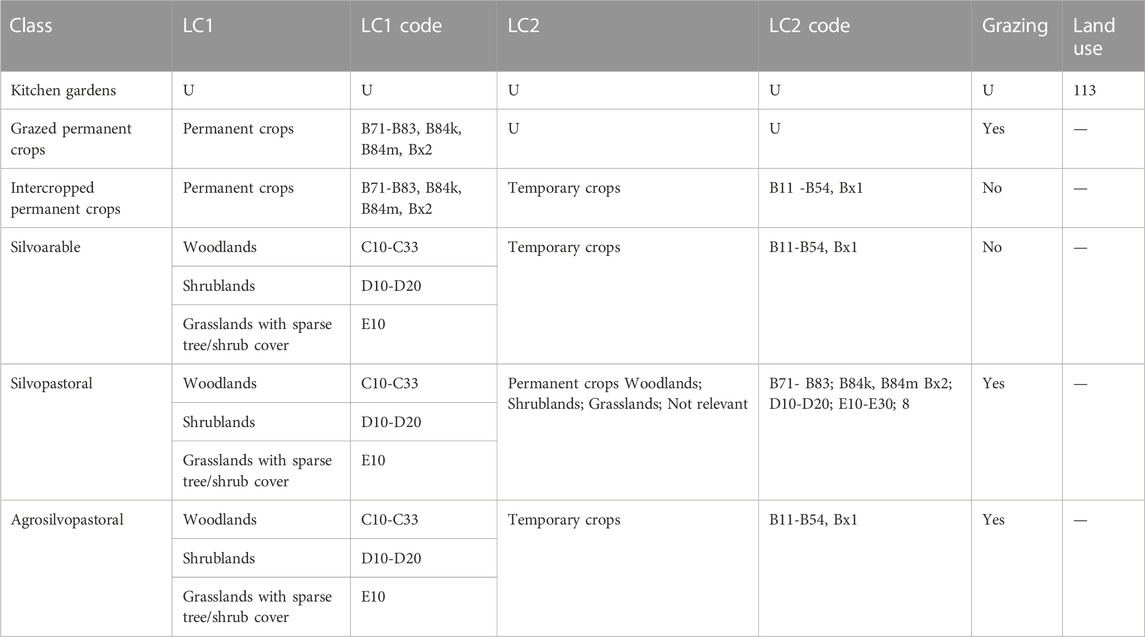
TABLE 2. Criteria used to classify the six agroforestry systems used in the analysis (class), based on the primary land cover (LC1) and the secondary land cover (LC2) defined by LUCAS. U = undetermined which means that any combination is possible but is not relevant for classification.
Classification criteria were based on the combination of land covers LC1 and LC2, as well as on the presence of grazing and of kitchen gardens. The classification was developed so that the agroforestry classes were mutually exclusive, i.e., each point corresponds to only one single class.
Kitchen gardens are considered a form of agroforestry as they commonly combine temporary crops, permanent crops, and, sometimes, shrubs and grass. In order to avoid duplication, kitchen gardens were identified first and did not contribute to any other agroforestry category.
Next, LC1 with woody vegetation were divided in two groups (Table 2): a) permanent crops and b) woodlands, shrublands or grasslands with sparse tree cover.
The permanent crops category includes fruit trees, olive groves, vineyards, nurseries and industrial woody crops. Permanent crops have also been referred to in previous agroforestry studies as high value trees (den Herder et al., 2017) or multipurpose trees (Mosquera-Losada et al., 2018). Names for agroforestry systems involving permanent crops include streuobst, près vergers, and fruit-tree meadows or orchards (Mosquera-Losada et al., 2009). In this study, agroforestry systems incorporating permanent crops were categorised into grazed permanent crops and intercropped permanent crops.
The woodlands1, shrublands2 or grasslands with sparse tree cover3 (LC1) were segregated into one of three categories. Grazed areas were classified as silvopastoral, including systems as forest grazing and wood pasture. Tree cover intercropped with temporary crops (LC2) were classified as silvoarable. Finally, silvopastoral systems combined with arable crops (LC2) were classified as agrosilvopastoral systems.
All of the agroforestry practices defined in the above process represent simultaneous agroforestry systems, where the tree and shrub component is present at the same time as the crop and/or livestock component. Sequential systems where, for example, afforested land is rotated with cropland were not considered.
2.3 Data analysis
The current state and spatial distribution of the agroforestry systems were analysed integrating the classified LUCAS points into a Geographical Information System (ArcGIS software by Esri, 2010) together with a layer of biogeographic regions of Europe. In this way, agroforestry classes were not only characterised by countries, but also by European bioclimatic regions (Alpine, Atlantic, Boreal, Black Sea, Continental, Macaronesia, Mediterranean, Pannonian and Steppic) (European Environment Agency, 2016). In addition, a more in-depth analysis on the dominant land covers for each agroforestry class was carried out.
The area of each agroforestry class for each country (AAF,C; km2) was estimated using the methodology described by den Herder et al. (2017), i.e., the number of classified points for that class in each country (NAF, C) was divided by the total number of LUCAS points surveyed in the country (NC) and multiplied by the surface area of the country (Ac; km2) (Equation 1), using data from Eurostat (2013a):
Additionally, the proportion of Utilised Agricultural Area (UAA) occupied by agroforestry systems was analysed by country, using data from Eurostat (2013b).
The above analysis was completed using data from the survey years 2009, 2012, 2015 and 2018. These results were then used to determine change between surveys using only countries with data from each of the four survey years, i.e., the 23 countries sampled in 2009 (EU23). Data from the new four countries included in the EU27 (Bulgaria, Cyprus, Malta and Romania; Table 1) were also analysed to find out how the extent of agroforestry changed in these territories between 2012 and 2018.
Additional studies were also completed on changes between the ‘grazing’, ‘not grazed’ and ‘grazing not relevant’ classifications and their relationship with the changes in agroforestry systems. It is important to note that grazing is one of the criteria used to classify silvopastoral, agrosilvopastoral and grazed permanent crops systems, and, therefore, it can be helpful in understanding changes.
Furthermore, we conducted a comprehensive analysis of the variation in the number of sampled points among the different LUCAS surveys (refer to Section 2.1) to determine whether these variations could impact the derived area of each agroforestry type. The objective of this analysis was to identify any disparities in the total number of points sampled in each land cover category (including artificial areas, temporary crops, permanent crops, woodlands, shrublands, grasslands, and other land cover types) across the various LUCAS surveys. Additionally, changes in the proportion of sampled points by land cover were also examined by country.
To explore the possible causes of changes, a more detailed analysis was carried out using common datum points. These are points that have the same geographical location between survey years. This analysis resulted in a smaller dataset as not all points are revisited from one survey to the next. The number of common points obtained by comparing the data from consecutive survey years was 184,603 for 2009–2012, 210,658 for 2012–2015, and 107,909 for 2015–2018. An analysis of the common points between the first and the last survey (2009 and 2018) is not reported because the resulting number of points classified as agroforestry was very low.
3 Results
3.1 Extent and spatial distribution of agroforestry in Europe
In 2018, the estimated surface area occupied by agroforestry in EU28 using the specified definitions was 114,621 km2, which represented 3% of the total extent and 6% of utilised agricultural land4 (UAA) (Table 3). In 2018, silvopastoral systems extended over 92,986 km2 (5% of UAA) equivalent to 81% of the agroforestry area. Kitchen gardens occupied 16,687 km2, about 15% of the agroforestry area (1% of UAA), and grazed permanent crops covered 3,434 km2 (about 3% of the agroforestry and 0.2% of UAA). Silvoarable and intercropped permanent crops only covered 676 km2 and 587 km2 respectively, representing less than the 1% of the agroforestry and about 0.04% of UAA. Finally, agrosilvopastoral systems only occupied 251 km2 (0.1% of the agroforestry area) (Table 3).
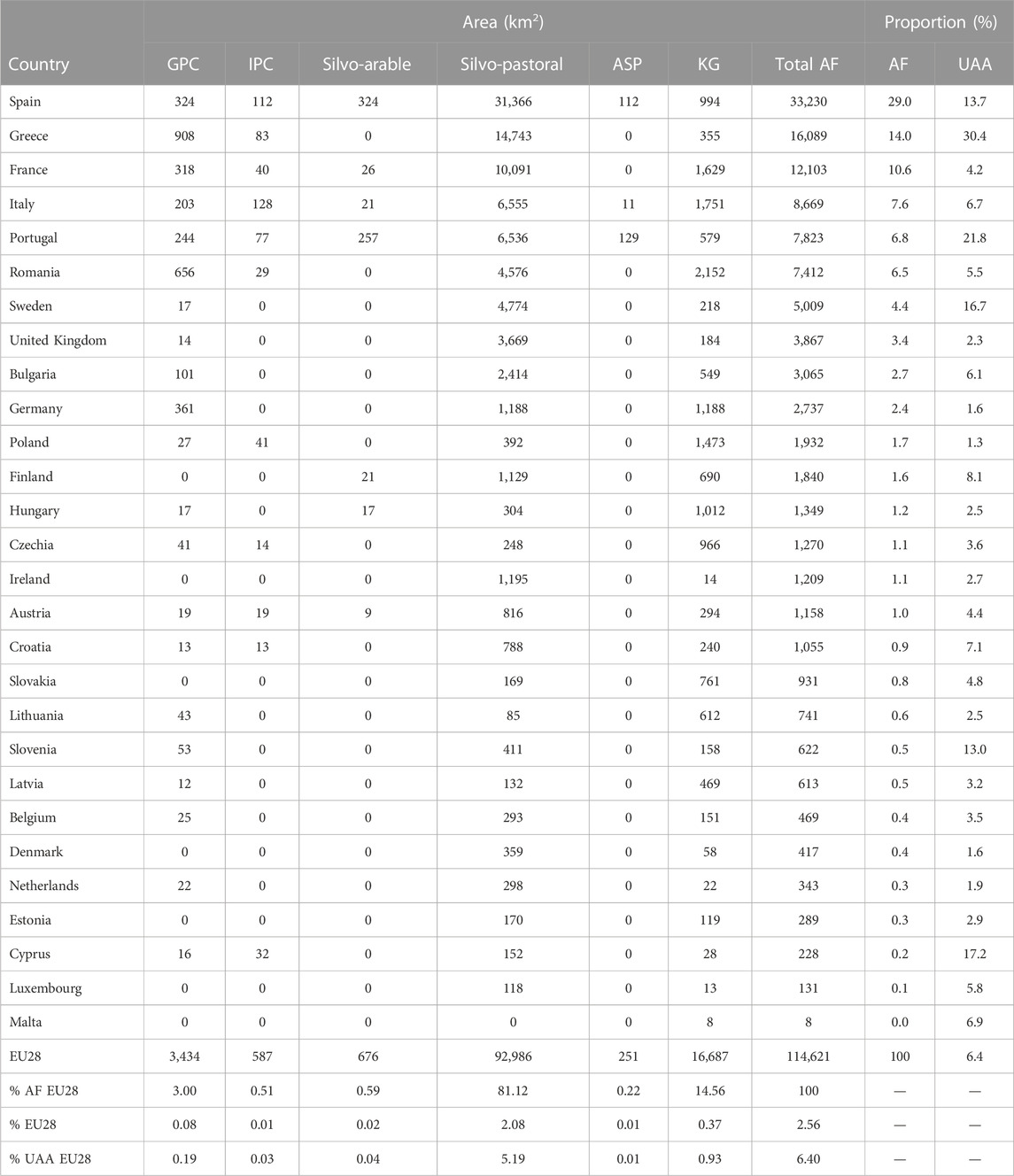
TABLE 3. Area of six agroforestry classes for the survey year 2018, and proportion of agroforestry by country (AF) in relation to total surface area occupied by agroforestry systems in Europe, as well as to utilised agricultural area (UAA) of each country. Data are ordered according to the proportion of AF. GPC = grazed permanent crops, IPC = intercropped permanent crops, ASP = agrosilvopastoral, KG = kitchen gardens.
Spain was the country with the largest area of agroforestry (33,230 km2), followed by Greece (16,089 km2) and France (12,103 km2). These three countries together accounted for 54% of the agroforestry in the EU28 in 2018. The proportions in Italy and Portugal were 8% and 7%, respectively (Table 3). When expressing the surface area occupied by agroforestry systems as a percentage of UAA (Table 3), the highest value of 30% was in Greece. Agroforestry systems occupied more than 20% of UAA in Portugal, and more than 12% in Cyprus, Sweden, Spain, and Slovenia. At the other extreme are countries like Poland, Denmark, Germany, Netherlands, the United Kingdom, Lithuania, and Hungary with less than 3% of their UAA occupied by the six types of agroforestry examined.
3.1.1 Silvopastoral and agrosilvopastoral systems
In 2018, silvopastoral (n = 7,805 points) was the most frequent agroforestry class and Spain was the country with the largest share (34%), followed by Greece (16%), France (11%), Italy (7%) and Portugal (7%). These five countries together represented approximately 61% of the total agroforestry area and 75% of the total silvopastoral area (Table 3). The proportion of European silvopastoral agroforestry in the Atlantic and Continental regions were 14% and 12% respectively (Figure 1; Supplementry Table S1).
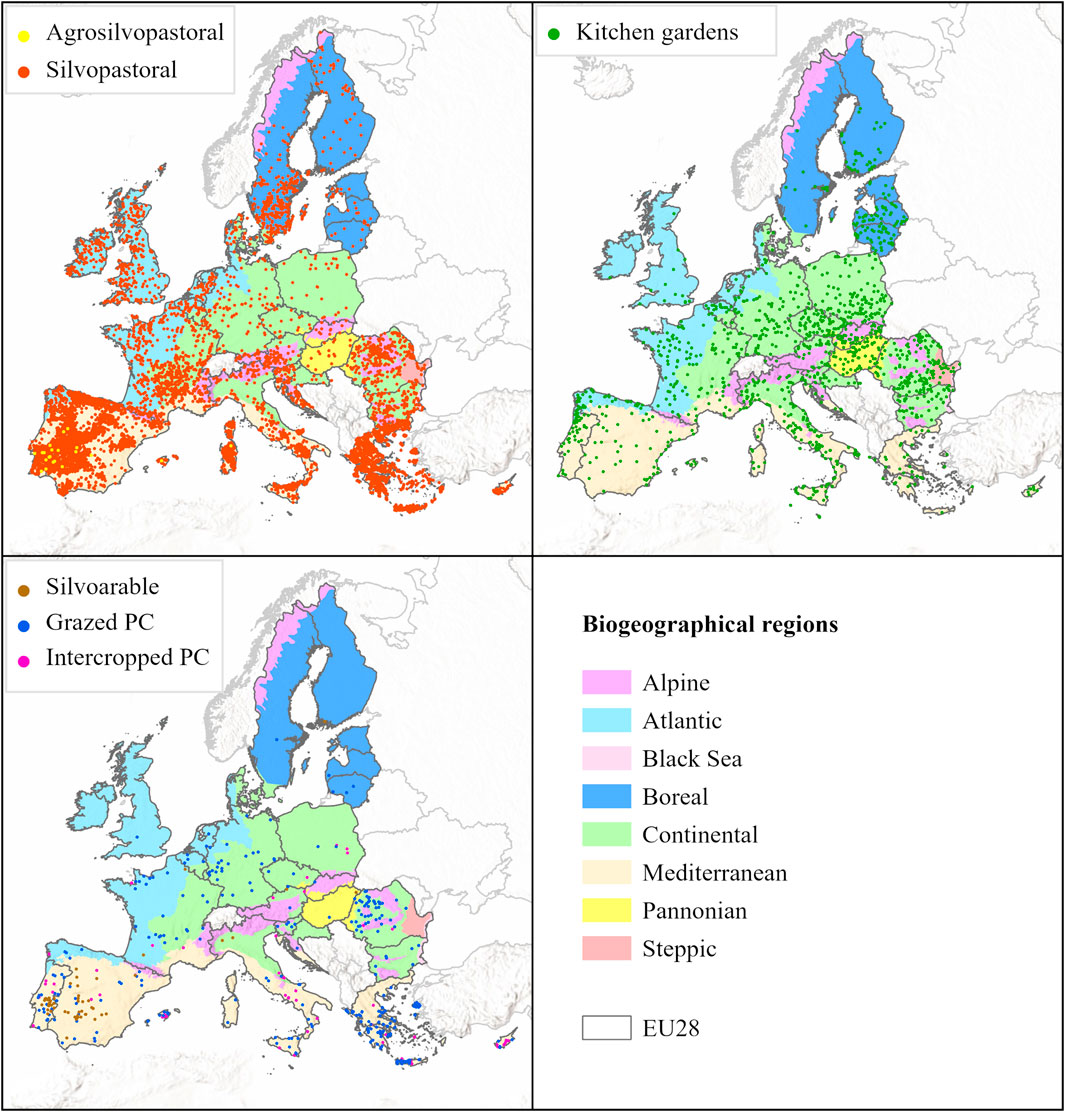
FIGURE 1. Spatial distribution of agroforestry classes in EU28 for the survey year 2018. PC = permanent crops. Bioregion data source: https://www.eea.europa.eu/data-and-maps/data/biogeographical-regions-europe-3.
The main types of land covers found in silvopastoral systems were broadleaved woodland (38%), followed by grasslands with sparse tree or shrub cover (26%) and shrublands without and with sparse tree cover (17% and 10%, respectively) (Supplementry Table S2). In all biogeographical regions, the dominant land cover was grasslands with sparse tree or shrub cover, except for the Mediterranean region, where broadleaved woodland was dominant (45%). Within broadleaf woodland in the Mediterranean region, 68% of the area was broadleaved evergreen forest and 21% was thermophilous deciduous forest (Supplementry Table S2).
Agrosilvopastoral systems (n = 21 points) were only recorded in Mediterranean regions (Supplementry Table S1), split between Portugal (51%), Spain (45%) and Italy (4%) (Table 3). As there were very few points, agrosilvopastoral systems were added to the silvopastoral total in the remaining temporal analyses.
3.1.2 Silvoarable systems
Silvoarable systems (n = 56 points) were only identified in a small number of European countries, with 48% of the area in Spain and 38% in Portugal. Smaller shares were present in France (4%), Italy (3%), Finland (3%), Hungary (3%) and Austria (1%) (Table 3). Silvoarable was mainly found in the Mediterranean region (88%; Figure 1, Supplementry Table S1), where broadleaved woodlands dominate, in combination with cereal crops.
3.1.3 Grazed and intercropped permanent crops
Agroforestry where permanent crops form the main land cover (n = 344 points) could be associated with grazing or with intercrops, with the area associated with grazing being about six-times greater than the area associated with intercrops. The share of grazed permanent crops was unevenly distributed in the EU within Greece and Romania representing 27% and 19% of its total. Moderate shares are found in Germany (11%), Spain (9%), France (9%), Portugal (7%) and Italy (6%). The remaining countries represented less than 13% of total area (Table 3). The highest amounts of intercropped permanent crops were found in Italy (22%), Spain (19%), Greece (14%) and Portugal (13%). The proportions found in Poland, France, Cyprus, Romania, Austria, Czechia and Croatia were less than 7%, and this class was not found in the rest of the EU28 (Table 3).
By biogeographical regions, permanent crops were more characteristic of Mediterranean (57%) and Continental (30%) areas than the rest of the bioregions (Figure 1, Supplementry Table S1). Predominant crops were olive groves in Mediterranean countries; apple orchards in Alpine, Atlantic, Boreal and Continental areas; and nut orchards in the Pannonian region (Supplementry Table S3). Regarding intercropped permanent crops, cereals were the main temporary crop (41%), followed by fodder crops and dry pulses, vegetables, and flowers, representing 25% and 23%, respectively (data not included).
3.1.4 Kitchen gardens
Kitchen gardens (n = 1,266 points) were identified in all countries, with the largest areas found in Romania (13% of total), followed by Italy (11%), France (10%), Poland (9%), Germany (7%), Hungary (6%), Spain (6%) and Czechia (6%). The highest share of kitchen gardens was found in the Continental bioregion (40% of total), followed by the Mediterranean (20%). Smaller proportions were reported in the Atlantic (13%), Boreal (11%), Pannonian (7%) and Alpine (6%) bioregions (Figure 1; Supplementry Table S1).
Kitchen gardens is a land use where different land covers are combined. Among these kitchen gardens, those with permanent crops (mainly apple trees) combined with grasslands, were predominant (45%). They were mostly located in Alpine, Atlantic, Boreal, Continental, Pannonian, and Black Sea bioregions. In contrast, kitchen gardens where temporary crops (mostly dry pulses, vegetables, and flowers) were the predominant land cover, dominated in the Mediterranean and Steppic bioregions (37%). They were followed by kitchen gardens with permanent crops associated with grasslands (25%) (Supplementry Table S4). Only 2% of kitchen gardens were grazed.
3.2 Change in the extent of agroforestry
The estimated area of agroforestry systems, based on our initial analysis of the data, increased by 55% between 2009 and 2012, but decreased by 26% from 2012 to 2015, and by 22% from 2015 to 2018. The overall estimated change between 2009 and 2018 was a decrease of 12,278 km2 or 11% (Table 4). Countries not included in this analysis because they did not belong to the EU23 (Bulgaria, Cyprus, Malta, and Romania) also showed an approximate halving of the agroforestry area from 21,769 km2 in 2012 to 10,713 km2 in 2018.
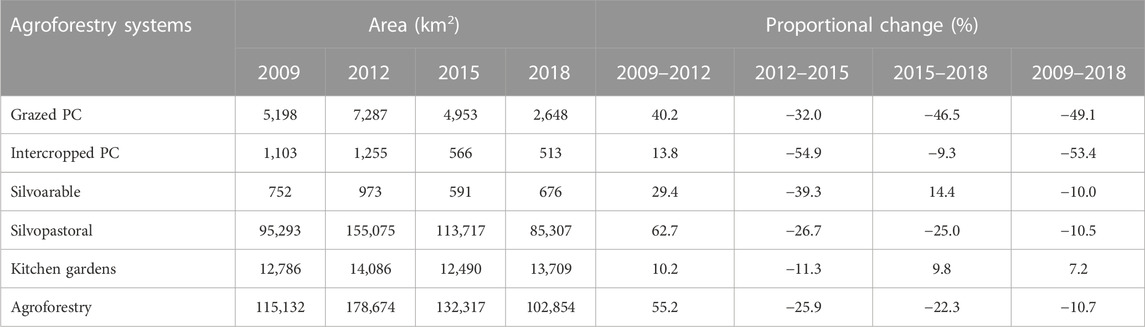
TABLE 4. Area and proportional area changes of five types of agroforestry system for four surveys or four time periods between 2009 and 2018. Data include only countries (EU23) common for each year. The proportional changes are with respect to the initially stated survey year.
The initial data analysis indicated that the areas of silvopastoral systems, the most common type of agroforestry, increased by 59,782 km2, i.e., 63%, between 2009 and 2012 (Table 4). By contrast, there was a 27% reduction between 2012 and 2015, and by 25% from 2015 to 2018. The area of grazed permanent crops also increased by 40% between 2009 and 2012, followed by a 32% decline from 2012 to 2015, and a 47% decline from 2015 to 2018 (Table 4). Ungrazed agroforestry land uses, such as intercropped permanent crops, silvoarable and kitchen gardens, also increased from 2009 to 2012, but less than the two grazed agroforestry types. Intercropped permanent crops showed a decrease of 53% between 2009 and 2018, and the reduction in grazed permanent crops was 49%. Between 2009 and 2018, the area of silvoarable systems was estimated to decline by 10%, whereas the area of kitchen gardens increased by 7% (Table 4).
Figure 2 illustrates the changes which took place between 2009 and 2018 in silvopastoral for selected countries. The surface area occupied by this class in Spain almost doubled between 2009 and 2012 with values of 32,766 and 62,231 km2, respectively (Supplementry Table S5). The resulting difference of 29,465 km2 makes up about half of the total increase of silvopastoral agroforestry between these two survey years (59,782 km2, Table 4). In all other countries silvopastoral agroforestry recorded an increase between 2009 and 2012 and most of them registered a decrease thereafter (2012–2018).
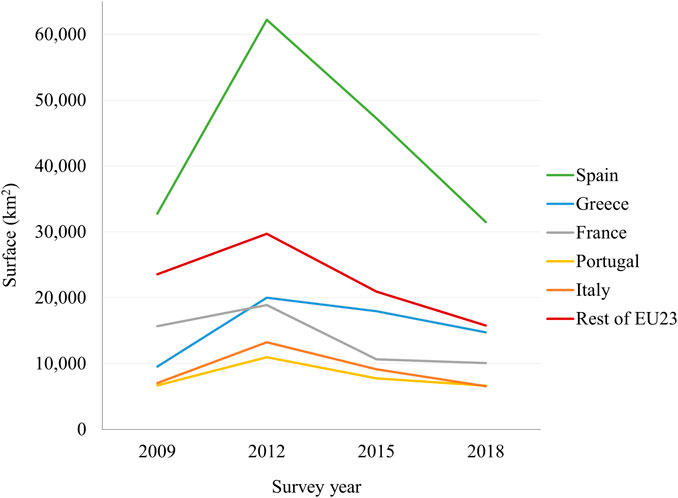
FIGURE 2. Surface extent of silvopastoral systems for survey years 2009, 2012, 2015 and 2018 in EU23.
If the period as a whole is considered, marked differences can be observed between EU23 nations (Figure 2), with Greece being the only Mediterranean country where there was a substantial increase in silvopastoral agroforestry (+54%). Other countries recorded a net increase, but this is not readily apparent in Figure 2 because the total extent of silvopastoral was small in Estonia (+233%), Denmark (+136%), Luxembourg (+101%), Austria (+42%), Netherlands (+30%), Sweden (+32%) and Finland (+21%). Non-Mediterranean countries with notable decreases were United Kingdom (−68%), Hungary (−67%), Lithuania (−58%), Slovakia (−44%), Germany (−38%) and France (−36%) (Supplementry Table S5). Overall, these data indicate an increase of silvopastoral systems in some northern European countries, whereas a decrease was generally observed in southern Europe, except for Greece. New member states included in EU27 (Bulgaria, Cyprus, Romania, Malta) also presented a decrease of silvopastoral systems surface between 2012 and 2018 (−59%; Supplementry Table S5).
Regarding kitchen gardens, the countries with the largest share in 2018 showed diverse changes along the study period, with Italy, Poland and Hungary recording an increase of 9%–21%, whereas France, Germany, Spain and Czechia recorded area losses between 11% and 23% for this type of system. Most countries showed increases (Supplementry Table S6).
In the case of grazed and intercropped permanent crops and silvoarable agroforestry, the main changes between 2009 and 2018 took place in countries with the highest share of the area of each practice. Thus, the calculated area of grazed permanent crops decreased in Mediterranean countries such as Spain, Italy, Portugal and Greece, while in Germany an increase of 18% was estimated. The area of intercropped permanent crops declined in Spain, Italy and Portugal by around 70%, but increased by about 25% in Greece. Finally, silvoarable agroforestry declined by 23% in Spain, whereas an increase of 116% was observed in Portugal.
Overall, in the Mediterranean bioregion, where 64% of agroforestry systems were reported in 2018, between 2009 and 2018 a decrease in agroforestry occurred in countries such as Italy (15%), Spain (7%) and Portugal (5%), while in Greece an increase was registered (44%) (Table 5). Within a country, the greatest proportional declines in agroforestry area were observed in the United Kingdom (−67%), followed by France (−33%), Hungary (−30%), Germany (−24%), Ireland (−20%) and Czechia (−18%) (Table 5). By contrast, the greatest proportional increases in the agroforestry area were recorded in Denmark (174%), Estonia (112%) and Luxembourg (92%) (Table 5), but their overall share of agroforestry systems in 2018 was very low at less than 1% (Table 3). The increase in the agroforestry areas in Sweden, Poland and Finland were associated with an increase in the area of silvopastoral systems and kitchen gardens. Although the area of silvopastoral and silvoarable systems declined in the United Kingdom, France, Germany, Poland and Lithuania, there were increases in the area of grazed or intercropped permanent crops. Spain was the only country that showed a decrease of all types of agroforestry systems.
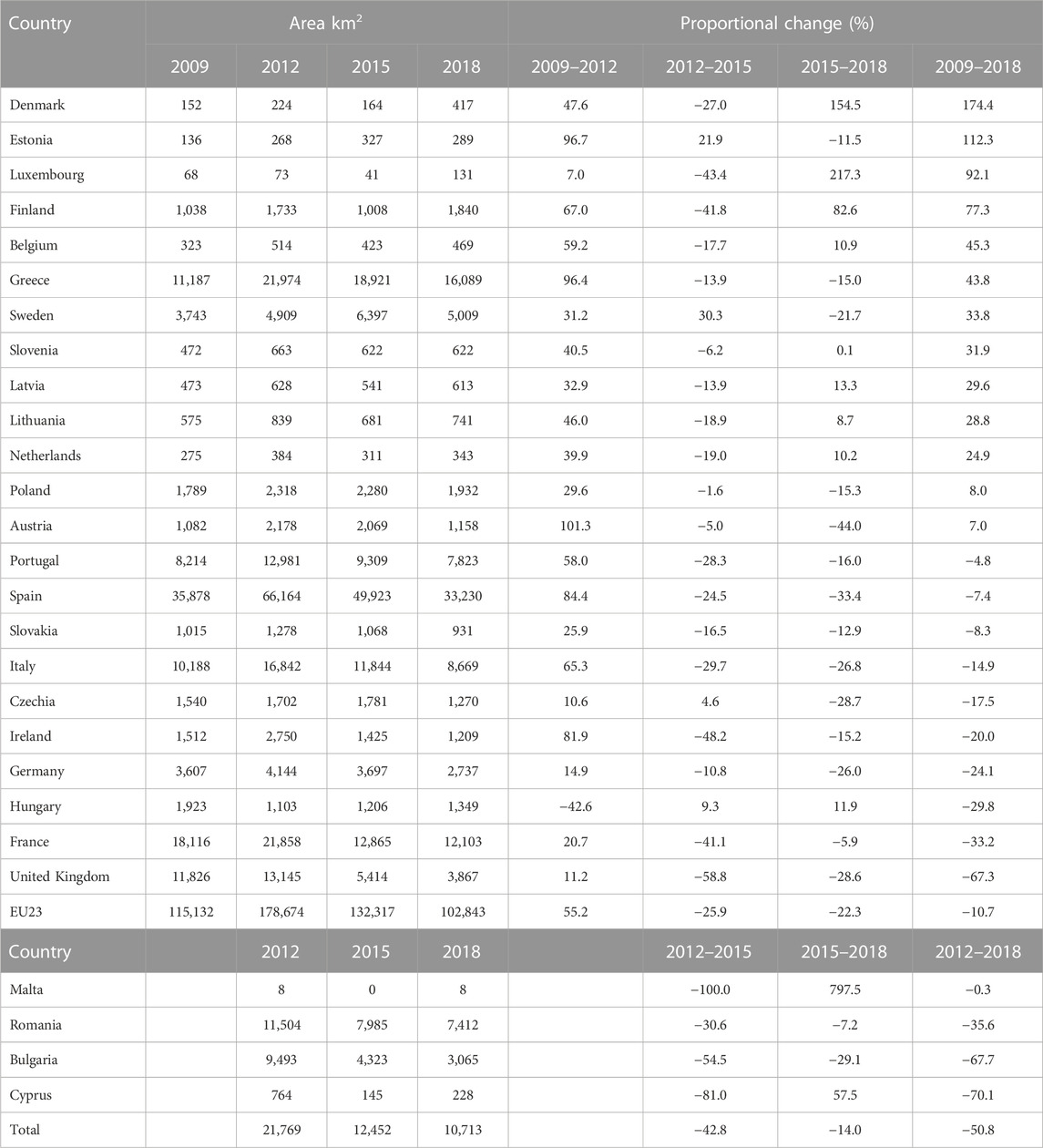
TABLE 5. Area and proportional change in the area of agroforestry systems from 2009 to 2018. Data include the EU23 countries sampled in 2009, and the four new countries included in the EU27 in 2012. The proportional differences presented are with respect to the previous survey year. Data are ordered by the change between 2009 and 2018.
3.2.1 Analysis of the causes of variations
The observed variations in agroforestry area between 2009 and 2018 could be related to a number of causes. They could be due to the implementation of the survey, such as the number of survey points and the criteria used by the observers in the different surveys, and/or real changes of land cover and land use.
The number of sampled points varied depending on the survey year, both the total number of sampled points and the points per country (see Section 2). An additional analysis was completed to determine if this variation could affect the surface estimation of each agroforestry type. Examining high level categories such as ‘woodlands, shrublands and grasslands with sparse tree cover’ indicated point number changes of only −4% to −3%, temporary crops showed a variation of about −3% to 3%, and the number of permanent cropping points varied between −2% and 1% (data not presented). The results suggested that sample point number variations did not explain the land use changes in agroforestry.
To examine the causes that generate changes of land cover/land use in agroforestry systems, an analysis was completed using only common points. Table 6 presents the net balance (difference between percentage gains and losses) for the different agroforestry systems, indicating an increase of 10% for the silvopastoral category between 2009 and 2012, and declines of 12% for 2012–2015 and 11% for 2015–2018. These results follow the same trends as observed with the total number of classified points, i.e., an increase of silvopastoral surface from 2009 to 2012, followed by a decrease from 2012 to 2018 (Table 4). Hence the common points analysis shows that the changes observed with the full dataset were also occurring with the common points. The changes in the area of grazed and intercropped permanent crops also showed similar results with those obtained in the analysis with all data since losses were estimated for 2012–2015 and 2015–2018 periods (Table 6).
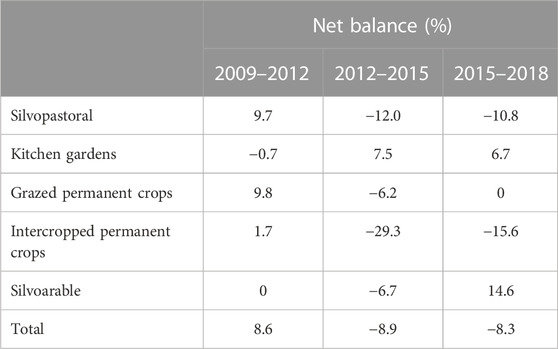
TABLE 6. Net balance of the number of points of the different agroforestry types between survey years.
The proportion of common point numbers relative to the total number of survey points for each survey year and each agroforestry type (Table 7) provides an estimate of the representativeness of the common points analysed. The common points represented more than 62% of the total points for the periods 2009–2012 and 2012–2015. By contrast the common points represented only between 18% and 49% of the total number of points in the 2015–2018 period, suggesting that changes in location could be more significant in this final period. However, even with a lower proportion of common points, the analysis indicates that real changes took place in the area of agroforestry systems.
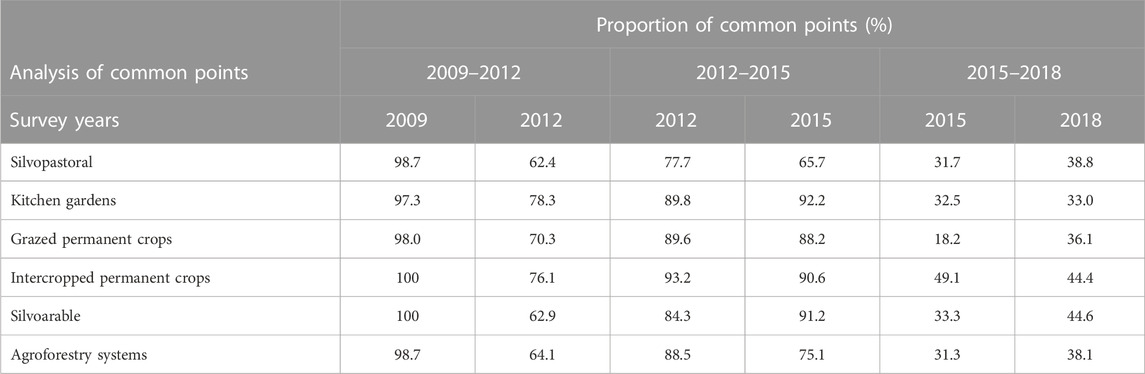
TABLE 7. Proportion of common points with respect to the total number of points for each survey year.
The next step was to examine the reason for changes in the proportion of silvopastoral and grazed permanent crops systems using the common points dataset (Table 8). From 2009 to 2012, for the same land cover, 10% of silvopastoral sites changed from ‘grazing not relevant’ to ‘grazed’ compared to only 0.1% of sites changing from ‘grazed’ to ‘grazing not relevant’, and our analysis indicates that it is this change that is primarily responsible for the increase in the silvopastoral area from 2009 to 2012. By contrast for the period 2012–2015 the proportion of sites changing from ‘grazed’ to ‘non-grazed’ (28%) was greater than the change from ‘non-grazed’ to ‘grazed’ (18%). Similar changes were apparent in 2015–2018. In 2012–2015 and 2015–2018, no changes in ‘grazing not relevant’ were reported for silvopastoral systems. Grazed permanent crops showed similar changes to those for silvopastoral systems, i.e., most gains were linked with changes from ‘non-grazed’ to ‘grazed’ and losses were related with the cessation of grazing (Table 8). In general, losses of silvopastoral or grazed permanent crops due to land cover change were relatively small. The question as to whether there were real changes in grazing or changes in methodological criteria is addressed in the next section.
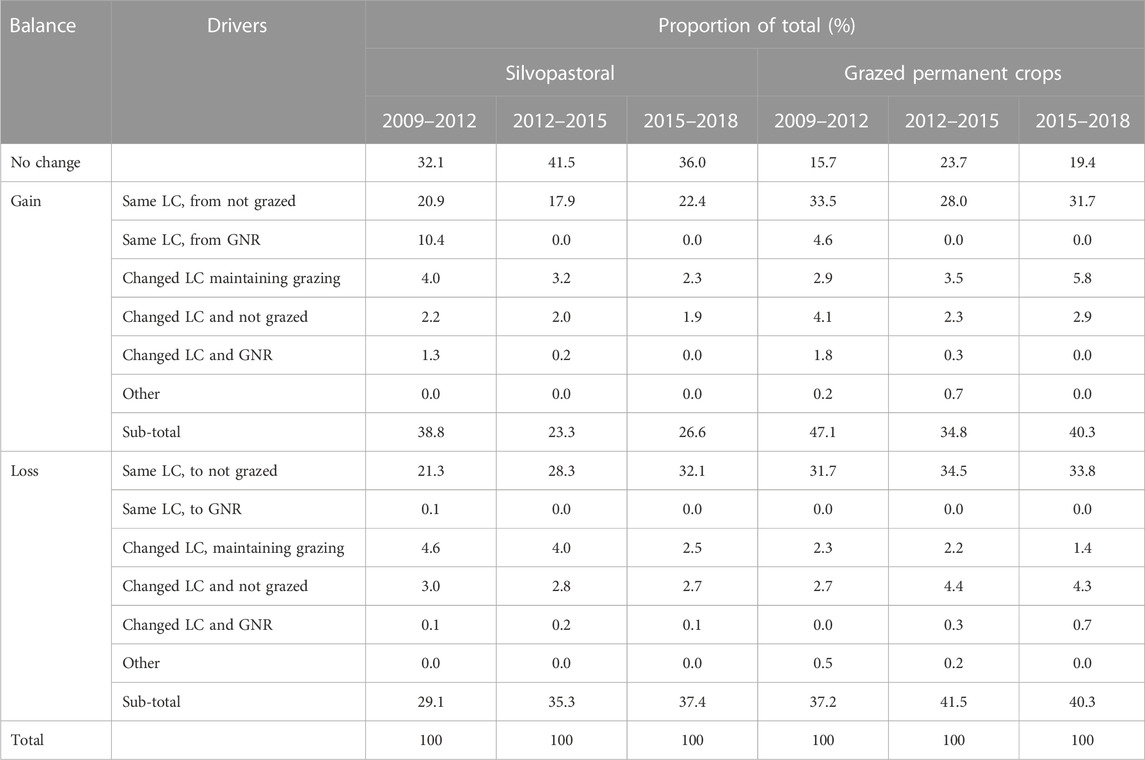
TABLE 8. Proportion of silvopastoral and grazed permanent crops systems in EU23 showing changes between land cover (LC) and grazing status including grazing, grazing not relevant (GNR), and not grazed for three periods.
Regarding the ungrazed intercropped permanent crops and silvoarable systems, the main sources of variation were related with changes of temporary crops, i.e., most gains and losses were associated to changes of temporary crops from/to other land cover (data not presented here). In the case of kitchen gardens, the gains and losses were primarily related to changes between land uses, mainly from/to agricultural use (data not presented here).
3.2.2 Analysis of ‘grazing’ criteria
The area of grazed agroforestry systems (silvopastoral and grazed permanent crops) increased by 73% between 2009 and 2012 and decreased by 43% from 2012 to 2018 (Table 4). In order to determine if the change between 2009 and 2012 was related to the field criteria for detecting grazing, we determined the proportion of points categorised as ‘grazing’, ‘not grazed’ and ‘grazing not relevant’ for each period using the complete LUCAS dataset for the EU23 (Figure 3). Between 2009 and 2012, a reduction on the proportion of ‘grazing not relevant’ (−23%) was associated with an increase in the proportion of points classified as ‘grazing’ (+2%) and ‘not grazed’ (+20%). However after 2012, ‘grazing not relevant’ remained stable, and the ‘grazing’ area declined back to 9%.
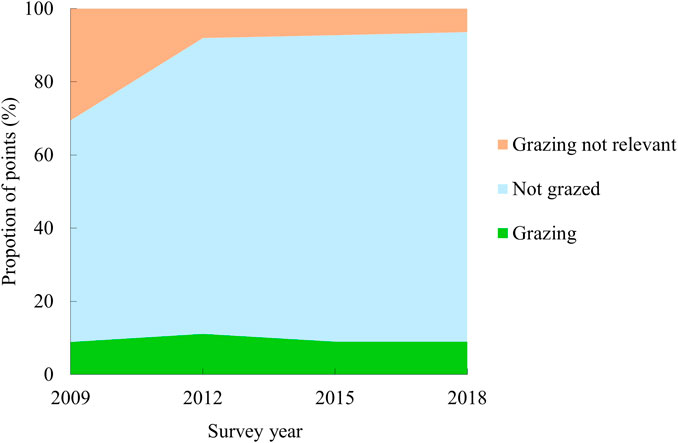
FIGURE 3. Proportion of three grazing classes in the sampled LUCAS data points in 2009, 2012, 2015, and 2018.
Using common points classified as ‘grazing not relevant’ between 2009 and 2018, we observed that 7% and 51% of the points classified in 2009 as ‘grazing not relevant’ changed in 2012 to ‘not grazed’ and ‘grazing’ respectively (Table 9). In the two subsequent periods, the proportions changing from ‘grazing not relevant’ were much lower, and when this occurred, a higher proportion was classified as ‘not grazed’. The percentage of points changing from ‘grazing not relevant’ to ‘grazing’ was less than 0.5% in both periods.
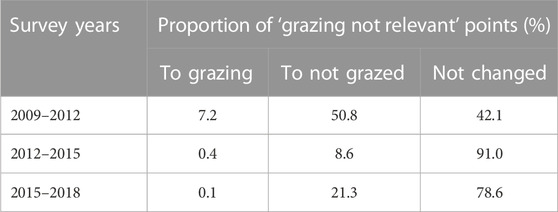
TABLE 9. Changes from ‘grazing not relevant’ to ‘grazing’ and to ‘not grazed’ points based on common points for three survey periods.
Instructions in the LUCAS manuals (Eurostat, 2019a) indicate that only the land covers artificial lands or water areas should be classified as ‘grazing not relevant’, that is, those land covers not susceptible to be grazed or land covers where there is nothing to be grazed (Eurostat, 2019a). However, in 2009 and 2012 there were many points with land covers that could be grazed such as grasslands, permanent crops, shrublands, temporary crops or woodlands, which were incorrectly classified as ‘grazing not relevant’ (n = 60,622 points; Supplementry Table S7). This means that these land covers, which could have been ‘grazing’, ‘not grazed’ and ‘grazing not relevant’ in 2009, were incorrectly classified as ‘grazing not relevant’, and some of these could have been ‘grazed’ points.
Hence there are grounds to argue that our initial estimate for agroforestry in 2009 is an underestimate, specifically for the grazed classes. Adding back in the 7% of all points that changed from ‘grazing not relevant’ to ‘grazing’ between 2009 and 2012 would result in a higher grazed area in 2009 of 570,034 km2 rather than 344,410 km2 (Table 9; Figure 4A). Applying this correction results in a more consistent decline in the area of grazing in Europe between 2009 and 2018 (Figure 4A).
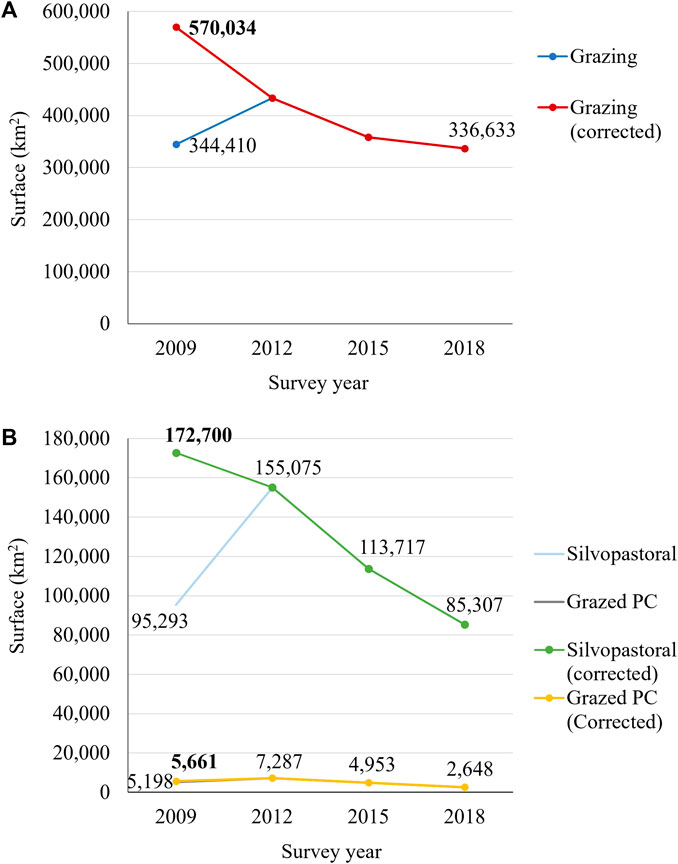
FIGURE 4. Changes of (A) the extent of grazing across the EU23, and (B) the extent of grazed agroforestry systems between 2009 and 2018 applying the correction of the points classified as grazing not relevant. PC = permanent crops.
Applying a similar correction to the area of silvopastoral agroforestry would increase the area estimate of silvopastoral agroforestry in 2009 from 95,293 km2 to 172,700 km2 (Figure 4B). This correction also results in a more consistent decline in the area of silvopastoral agroforestry. The periods after 2012 do not need a correction because no significant changes of ‘grazing not relevant’ to ‘grazing’ were identified. The same correction can be applied to grazed permanent crops. In this case, 5% of the points classified in 2012 were related with changes from ‘grazing not relevant’ to ‘grazing’ (Table 8). Therefore, the updated area estimated for 2009 was 5,661 km2 and the increment between 2009 and 2012 was reduced by 12% (Figure 4B). Finally, the total surface of agroforestry systems would also increase in 2009 if we consider the corrected values, occupying an area of 193,002 km2 instead of 115,132 km2. In this case, the surface loss of agroforestry between 2009 and 2018 would be 47%.
This reduction in the area of grazing is also evident in official statistics from Spain, Greece and the United Kingdom. In Spain, data from the 2009 and 2020 agricultural census (INEbase, 2011; INEbase, 2022) indicated a 21% reduction in the number of farms with extensive livestock (cattle excluding dairy cows, sheep, goats and pigs), while the number of animal heads increased by 14%. This indicates an increase of the stocking rate, as well as farm abandonment, although farms may also have merged. In Greece, according to data from Hellenic Statistical Authority (2019), between 2009 and 2018 livestock farms (cows, sheep, goats and pigs without differentiating between stabled and extensive cattle) and the number of animals decreased by 37% and 13% respectively, suggesting a reduction in grazing. In the United Kingdom, statistics published by the Government’s Department of Environment, Food and Rural Affairs (2022) on land use in agricultural holdings with grazing activities indicated a surface loss between 2009 and 2018 of 2%. These values are not directly comparable with those obtained using LUCAS surveys but they could inform our understanding of the dynamics of the grazed area in Europe between 2009 and 2018.
4 Discussion
The results of this paper are discussed in terms of the challenge of determining the changes in agroforestry area in Europe between 2009 and 2018 and the implications for the use of LUCAS data, as well as the extent of different agroforestry types in 2018 and the implications of previous trends on the anticipated area of agroforestry in Europe in future years. Additionally, the factors that have generated the main changes in the surface area of each agroforestry systems and the current trend to re-establish the link between trees and food production at a farm level are also addressed.
4.1 Changes in the agroforestry area in Europe from 2009 to 2018
An initial analysis of the LUCAS results suggested that the area of agroforestry in the EU23 was 115,132 km2 in 2009, it then increased by 55% to reach 178,674 km2 in 2012, before declining by 26% to reach 132,317 km2 by 2015, and a decline by 22% to reach 102,854 km2 by 2018. However more detailed analysis suggests that some of these large changes are due to methodological problems in the 2009 LUCAS survey. In 2009, some points were incorrectly classified as ‘grazing not relevant’. The guidance for LUCAS indicates that ‘grazing not relevant’ should only correspond to areas that cannot be grazed, such as urban areas or water bodies. However common point analysis showed that points classified in 2009 as ‘grazing not relevant’ represented land covers that could be grazed such as woodland and shrubland, and in the following surveys (2012–2018) such areas were either assigned as ‘grazing’ or ‘not grazed’. If a correction to the 2009 data is made, then the updated predicted area of agroforestry in 2009 is 193,002 km2. This corrected value also results in a more consistent pattern of declining areas of agroforestry between 2009 and 2018, driven primarily by reduced grazing (Figure 4).
The above analysis, suggests that the estimates made by den Herder et al. (2017) for the EU27, using the 2012 data are still valid. Den Herder et al. (2017) estimated the total area occupied by agroforestry (excluding kitchen garden) in the EU27 of 154,000 km2 using LUCAS data from 2012, and Mosquera-Losada et al. (2018) estimated an area (including kitchen gardens and grazed shrublands without sparse tree cover) of 197,700 km2 using the same data. Within this study, for the same survey year and for the same countries we calculate an area of 200,443 km2, including kitchen gardens and grazed shrublands without sparse tree cover in a similar way to Mosquera-Losada et al. (2018). Obviously, both results are similar as identical classes were considered, with the minor variations probably resulting from the use of a harmonised data set in our study which was not previously available.
4.2 Implications for the analysis of LUCAS data
The above results demonstrate the importance of a consistent method for establishing grazing in the LUCAS dataset. If agroforestry areas are defined as areas where there are ecological and economic interactions between trees or shrubs and either crop and/or animals (Burgess and Rosati, 2018), then some criterion is needed to establish if, for example, the level of grazing is significant. Whilst the criterion for ‘grazing not relevant’ is relatively clear, judging between ‘grazing’ and ‘no-grazing’ is not easy and as demonstrated in this paper, the dividing line can have large effects on the estimated area of agroforestry if grazing is considered a necessary part of agroforestry involving livestock. For example, Jensletten and Klokov (2002) estimated that the area of reindeer husbandry in Northern Europe, which could be considered as agroforestry, was 410,000 km2, which is about four-times the total area of agroforestry in the EU28 of 114,621 km2 derived in our study from LUCAS in 2018. In the main, areas of reindeer husbandry in northern Sweden and Finland are not included as the evidence of grazing at individual sample points was insufficient. In addition, as described by den Herder et al. (2017), the level of sampling in mountainous and remote areas tend to be underrepresented in the LUCAS survey.
The analysis highlights that paired samples commonly assessed in 2015 and 2018 represented only 31%–38% of the total number of samples in each year, which means that some of the inter-annual variation could be due to changes in sample point location. This should not be a problem if a land type is common, but it could be important for less abundant land classes. Therefore, whilst analyses of common points are useful, analysis of real land use change could be clearer if LUCAS would maintain consistency in the number and location of the survey points.
4.3 Geographical variations in the total area and change in agroforestry
In 2018, using the LUCAS dataset, we calculated that the six defined agroforestry types in the EU28 (Table 3) occupied 114,621 km2, which represents 6% of utilised agricultural area (UAA). However, this paper demonstrates a consistent decrease in the area of agroforestry in the EU23 between 2009 and 2018. Although we did not recalculate a change in agroforestry per country between 2009 and 2012 to account for the reclassification of ‘grazing not relevant’, Spain which accounted for 40% of the EU28 agroforestry area in 2012, represented about 44% of the reduction that occurred between 2012 and 2018 (Table 5). Other than Spain, the two countries demonstrating the greatest loss in agroforestry between 2012 and 2018 were the United Kingdom (−70%) and Ireland (−56%). By contrast between 2012 and 2018, the calculated area of agroforestry increased in Hungary, Luxembourg, and Denmark. Some of the decline in the United Kingdom and Ireland may be related to the increased use of permanent housing for livestock, driven in part in the dairy sector by the greater use of automated milking (Barkema et al., 2015).
4.4 Explaining the decline in silvopastoral systems
Silvopastoral was the most common agroforestry practice accounting for 81% of the agroforestry area and 5% of UAA of the EU28 in 2018. Silvopastoral agroforestry has different compositions depending on bioclimatic conditions. Thus, in the Atlantic bioregion, about 27% of silvopastoral systems comprise grazed shrublands without sparse tree cover, and 32% comprise grassland with sparse trees or shrubs (Supplementry Table S2). In the Continental bioregion, 54% of silvopastoral systems comprise grazed grassland with sparse trees and shrubs (Supplementry Table S2). In Mediterranean areas, broadleaved woodland is the predominant cover (45%) (Supplementry Table S2), offering fodder reserves for livestock during the dry summer months and the shade provided by the trees can extend the growing season of pastures (San Miguel-Ayanz, 2004; Mosquera-Losada et al., 2018).
Almost all EU23 countries lost silvopastoral surfaces between 2009 and 2018. Moreno and Pulido (2009) report a decrease of the area and tree density of dehesas and montados since the second half of the 20th century. This decline could be related with increased mechanisation, stocking rate, death of trees in over-aged stands, farm abandonment associated with land degradation, water scarcity linked to climate change and depopulation associated with migration from rural to urban areas (Moreno and Pulido, 2009; Rodríguez-Rigueiro et al., 2021). An additional effect of the natural expansion of unmanaged forestlands in this region is the increased frequency of forest fires (Rodríguez-Rigueiro et al., 2021).
In Spain, the reduction in the agroforestry is associated with an abandonment of the farms with grazing livestock. The Spanish National Strategy to Combat Desertification (Ministerio para la Transición Ecológica y el Reto Demográfico, 2022) highlights the process of progressive intensification of livestock farms, including the increased use of housing and less use of grazing. In Greece, the use of nomadic flocks has also been reducing associated with urban expansion, land abandonment and the expansion of industrial crops in pastureland, which have led to a progressive increase of forest and shrubland in farmland and consequently an increase of fire risk (Colantoni et al., 2020). In Romania, Hartel et al. (2013) highlighted changes in traditional grazing systems in the Saxon region of Transylvania, such as the use of fire as a method for clearing pastures. They report that poor fire management perhaps resulted in the burning of half of the silvopastoral systems in this area in 2012. In addition, whilst cattle, horses, buffalo and pigs were the dominant livestock in silvopastoral systems in the last century, the majority of silvopastoral systems in 2012 were grazed only by sheep (60%). In Hungary, Varga (2017) reports that silvopastoral practices have been abandoned in recent decades due to the intensification of agriculture and forestry management. In some EU countries, high stocking densities are still supported by some subsidies from the Common Agriculture Policy (CAP) (Delattre et al., 2020). However, in general, establishing the evidence for the observed reduction in grazing at a national level is difficult due to a lack of official statistics about the area of grazing as opposed to grass cover, and this appears to be an important gap in the available evidence. Further research would be useful to address this issue, which is anticipated to have impacts in terms of biodiversity, fire risk and nutrient management.
4.5 Explaining the decline in agroforestry with permanent and arable crops
Permanent crops predominate in Mediterranean regions, where olive groves are the main form of permanent crop (69%) (Supplementry Table S3). According to Sánchez (1995), the presence of livestock within permanent crops allows cheaper weed control than the use of herbicides, and the vegetation provides fodder for cattle. In addition, in grazed permanent crops, yields can be higher than in ungrazed permanent crops (Sánchez, 1995). However despite these advantages, the area of grazed and intercropped permanent crops in the EU23 decreased by 63% from 8,552 km2 in 2012 to 3,162 km2 in 2018 (Table 4). The decline in the area of streuobst and près vergers in northern Europe, pomaradas in humid parts of northern Spain, joualle in southern France or walnuts intercropped with vegetables in the Italian region of Campania was also reported by Eichhorn et al. (2006). The reasons for the decline include the replacement of high-stem orchards with smaller trees, often on dwarf rootstocks, which supports mechanisation and prevents the integration of livestock. Duarte et al. (2008) highlighted that low profitability is one reason for the abandonment of traditional olive orchards in Mediterranean areas. International competition and imports of fruits and nuts from outside of Europe, such as walnuts from California, changes of land use to artificial areas as well as political measures in favour of more standardised means of production, have also been linked to a decline of permanent crop agroforestry across the EU (Eichhorn et al., 2006). Fungal diseases caused by species in the Botryosphaeriaceae family have also reduced the production of nut crops (such as almond, pistachio and walnut), and olives (Moral et al., 2019).
The reported area of silvoarable agroforestry in Europe is relatively small with a reported decrease from 2012 to 2015, and an increase from 2015 to 2018. This value probably ignores landscape features such a hedgerows and windbreaks which are considered as agroforestry in many frameworks. Historically removal of hedgerows and trees from arable fields have been related to the use of larger arable machinery. Esgalhado et al. (2021) analysed the drivers of land use changes in seven Mediterranean case studies (Portugal, Spain, France, Italy, Malta, Tunisia and Algeria) and found that agricultural intensification was typically associated with the simplification of production systems. The loss of trees has also been associated with the loss of landscape diversity and the loss of some wildlife species (Lasanta et al., 2017), and soil erosion. However, in some locations the need to control soil erosion and minimise water-borne pollution is encouraging farmers to re-integrate trees in cropping systems (Burgess and Rosati, 2018).
4.6 The increase in kitchen gardens
Kitchen gardens account for almost 1% of UAA of the EU28 in 2018 and 15% of the area of agroforestry, being particularly widespread in the Continental and Mediterranean bioregions. This form of agroforestry is associated with urban and peri-urban areas and provides a link between urban areas and local food production (Mosquera-Losada et al., 2018). Unlike other agroforestry practices, kitchen gardens showed a net increase in area in the EU23 from 2009 to 2018. The provision of kitchen gardens can be helpful in allowing local communities to enhance food security particularly during the current context of volatile food prices (Bidar et al., 2020). The large areas of kitchen gardens in central European countries could be related to the traditional use of orchards surrounding houses (Santiago-Freijanes et al., 2018). Urban gardening in cities such as Leipzig in Germany and Lisbon in Portugal is seen as a method to increase socio-ecological resilience (Cabral and Weiland, 2016). By contrast, kitchen gardens are disappearing in Czechia where the practice is negatively associated with the Soviet period (Spilková and Vágner, 2016). Hence the spatial distribution and expansion of this type of agroforestry is clearly related to cultural and political drivers.
4.7 Re-establishing the link between trees and food production at a farm-level
The results demonstrate that reductions in the area of grazing is reducing the area of agroforestry (where there are direct tree-animal interactions). However, as national governments and food producers set increasing priority on achieving net zero greenhouse gas emissions (Costa et al., 2022), there is increasing interest in increasing the area of tree cover on farms to ‘inset’ (as opposed to offsetting) the greenhouse gas emissions related to food production within the farm (Burgess and Graves, 2022). Because many countries across Europe have targets to increase the area of tree cover, whilst the direct link between trees and crop and/or animal production may be reducing in at a field level, the indirect links between trees and food production may be strengthening at farm level.
5 Conclusion
The analysis in this paper provides to our knowledge the first systematic analysis of how the area of agroforestry in Europe has changed over time, using data collected between 2009 and 2018. It uses a similar definition of the area of agroforestry to that used by den Herder et al. (2017), which specifies that there should be evidence of grazing in silvopastoral areas. The results demonstrate that agroforestry occurs throughout Europe, but the proportion of land occupied by the combination of woody perennials with crops and/or animals is greatest in the Mediterranean region. Silvopastoral systems are the most common form of agroforestry in Europe, accounting for about 81% of the agroforestry area in the EU28 area in 2018. The second most common category was kitchen gardens. An initial analysis of the changes in the area of agroforestry in the EU23 area from 2009 to 2018 suggested that the area of agroforestry increased from 2009 to 2012, and then declined from 2012 to 2018. However more detailed analysis suggests that our initial estimate of the area of agroforestry in 2009 was underestimated to an inconsistent use of the criterion for the ‘grazing not relevant’ category in LUCAS. With this correction, we propose that the actual pattern is of a consistent decline in the area of agroforestry in the EU23 area from 193,002 km2 in 2009 to 102,854 km2 in 2018, primarily related to a decline in silvopastoral systems from 172,700 km2 to 85,307 km2 over the same period. Moreover, we argue that a reason for this decline is a reduction in the outdoor grazing of livestock across Europe, partly caused by reduced ruminant numbers in some countries and the increasing permanent housing of livestock indoors. These reported changes in the level of grazing across Europe are worthy of further research, and could have other implications for biodiversity, fire-risk, and nutrient management.
In addition there has been a reduction in the area of grazed permanent crops due to the replacement of high-stem cultivars with short-stem trees which are more suited for mechanical harvesting and less suitable for the integration of livestock. By contrast, the area of kitchen gardens between 2009 and 2018 showed a net increase. The results demonstrate the usefulness of a consistent and regularly collected land cover and land use dataset, including grazing data, across a wide range of countries to understand the changes occurring in European agriculture. The results also demonstrate the importance in establishing clear criteria for the categorisation of different land uses and cover, and that it may be useful to minimise the inter-annual variation in the geographical location of sampling points. Although the reduction in the area of grazing is reducing the area of in-field agroforestry in Europe, the increasing interest in increasing tree cover on farms to mitigate and adapt to the effects of climate change, may be increasing the interactions between trees and food production at a farm-scale.
Data availability statement
Publicly available datasets were analyzed in this study. This data can be found here: https://ec.europa.eu/eurostat/web/lucas/data/primary-data.
Author contributions
JR-D: Conceptualization, Formal Analysis, Investigation, Methodology, Writing–original draft, Writing–review and editing. SS: Conceptualization, Funding acquisition, Investigation, Methodology, Project administration, Supervision, Writing–original draft, Writing–review and editing. PB: Writing–review and editing. SB: Funding acquisition, Project administration, Supervision, Writing–review and editing.
Funding
The authors declare financial support was received for the research, authorship, and/or publication of this article. This work was supported by the European Union’s Horizon 2020 Research and Innovation Programme (grant number 862993) in the framework of the Agroforestry and Mixed farming systems—Participatory research to drive the transition to a resilient and efficient land use in Europe (AGROMIX) project.
Acknowledgments
We also thank the two reviewers for their helpful comments and suggestions that helped us in improving the article.
Conflict of interest
The authors declare that the research was conducted in the absence of any commercial or financial relationships that could be construed as a potential conflict of interest.
Publisher’s note
All claims expressed in this article are solely those of the authors and do not necessarily represent those of their affiliated organizations, or those of the publisher, the editors and the reviewers. Any product that may be evaluated in this article, or claim that may be made by its manufacturer, is not guaranteed or endorsed by the publisher.
Supplementary material
The Supplementary Material for this article can be found online at: https://www.frontiersin.org/articles/10.3389/fenvs.2023.1258697/full#supplementary-material
Footnotes
1Areas covered by trees with a canopy of at least 10% (Eurostat, 2019b).
2Areas dominated (at least 10% of the surface) by shrubs and low woody plants normally not able to reach more than 5 m of height. It may include sparsely occurring trees with a canopy below 10% (Eurostat, 2019b).
3Land predominantly covered by communities of grassland, grass-like plants and forbs including sparsely occurring trees (the tree canopy is between 5% and 10% and the total of the tree + shrub canopy is between 5% and 20% of the area) (Eurostat, 2019b).
4In 2018, following data of Eurostat, the total extent of utilised agricultural land for the EU28 was 1,791,446 km2.
References
ArcGIS, , and ArcMap, (2010). ArcGIS® and ArcMap™ (software GIS) version 10.0. Redlands, CA: Environmental Systems Research Institute, Inc.
Atangana, A., Khasa, D., Chang, S., and Degrande, A. (2014). “Agroforestry for soil conservation,” in Tropical agroforestry. Editors A. Atangana, D. Khasa, S. Chang, and A. Degrande (Netherlands: Springer), 203–216.
Augére-Granier, M. L. (2020). Agroforestry in the European union. European Parliament Research Service. Available at: https://policycommons.net/artifacts/1336911/agroforestry-in-the-european-union/1944452/ (Accessed March 14, 2023).
Ballin, M., Barcaroli, G., Masseli, M., and Scarnó, M. (2018). Redesign sample for land use/cover area frame survey (LUCAS) 2018. Luxembourg: Publication office of the European Union. doi:10.2785/132365
Barkema, H. W., von Keyserlingk, M. A. G., Kastelic, J. P., Lam, T. J. G. M., Luby, C., Roy, J. P., et al. (2015). Invited review: changes in the dairy industry affecting dairy cattle health and welfare. J. Dairy Sci. 98, 7426–7445. doi:10.3168/jds.2015-9377
Bartus, M., Barta, K., Szatmári, J., and Farsang, A. (2017). Modeling wind erosion hazard control efficiency with an emphasis on shelterbelt system and plot size planning. Z. für Geomorphol. 61, 123–133. doi:10.1127/zfg/2017/0406
Beka, S., Burgess, P. J., and Corstanje, R. (2023). Robust spatial estimates of biomass carbon on farms. Sci. Total Environ. 861, 160618. doi:10.1016/j.scitotenv.2022.160618
Bergmeier, E., Petermann, J., and Schröder, E. (2010). Geobotanical survey of wood-pasture habitats in Europe: diversity, threats and conservation. Biodivers. Conserv. 19, 2995–3014. doi:10.1007/s10531-010-9872-3
Bidar, G., Pelfrêne, A., Schwartz, C., Waterlot, C., Sahmer, K., Marot, F., et al. (2020). Urban kitchen gardens: effect of the soil contamination and parameters on the trace element accumulation in vegetables – a review. Sci. Total Environ. 738, 139569. doi:10.1016/j.scitotenv.2020.139569
Buck, O., Haub, C., Woditsch, S., Lindemann, D., Kleinwillinghöfer, L., Hazeu, G., et al. (2015). Analysis of the LUCAS nomenclature and proposal for adaptation of the nomenclature in view of its use by the Copernicus land monitoring services. EEA/EFTAS. Contract No 3436/B2015/RO-COPERNICUS/EEA.56195. EEA - European Environment Agency. Available at: https://land.copernicus.eu/user-corner/technical-library/LUCAS_Copernicus_Report_v2-2.pdf.
Burgess, P. J., and Graves, A. (2022). The potential contribution of agroforestry to net zero objectives. Report for the woodland trust. Bedfordshire: Cranfield University. Available at: https://dspace.lib.cranfield.ac.uk/handle/1826/18664.
Burgess, P. J., and Rosati, A. (2018). Advances in European agroforestry: results from the AGFORWARD project. Agrofor. Syst. 92, 801–810. doi:10.1007/s10457-018-0261-3
Cabral, I., and Weiland, U. (2016). “Urban gardering in Leipzig and Lisbon: A comparative study on governance,” in Growing in cities: interdisciplinary perspectives on urban gardening. Conference proceedings. Editor S. Tappert (Basel: University of Applied Science), 66–79.
Colantoni, A., Egidi, G., Quaranta, G., D’Alessandro, R., Vinci, S., Turco, R., et al. (2020). Sustainable land management, wildfire risk and the role of grazing in Mediterranean urban-rural interfaces: a regional approach from Greece. Land 9, 21. doi:10.3390/land9010021
Costa, C., Wollenberg, E., Benitez, M., Newman, R., Garner, N., and Bellone, F. (2022). Roadmap for achieving net-zero emissions in global food systems by 2050. Sci. Rep. 12, 15064. doi:10.1038/s41598-022-18601-1
Damianidis, C., Santiago-Freijanes, J. J., den Herder, M., Burgess, P. J., Mosquera Losada, M. R., Graves, A., et al. (2021). Agroforestry as a sustainable land use option to reduce wildfires risk in European Mediterranean areas. Agrofor. Syst. 95. doi:10.1007/s10457-020-00482-w
d’Andrimont, R., Yordanov, M., Martinez-Sanchez, L., Eiselt, B., Palmieri, A., Dominici, P., et al. (2020). Harmonised LUCAS in-situ land cover and use database for field surveys from 2006 to 2018 in the European Union. Sci. Data 7, 352. doi:10.1038/s41597-020-00675-z
Delattre, L., Debolini, M., Paoli, J. C., Napoleone, C., Moulery, M., Leonelli, L., et al. (2020). Understanding the relationships between extensive livestock systems, land-cover changes, and CAP support in less-favored mediterranean areas. Land 9, 518. doi:10.3390/land9120518
den Herder, M., Burgess, P. J., Mosquera-Losada, M. R., Herzog, F., Hartel, T., Upson, M., et al. (2015). Preliminary stratification and quantification of agroforestry in europe. Milestone report 1.1 for EU FP7 AGFORWARD research project (613520). Available at: https://www.repository.utl.pt/bitstream/10400.5/9194/1/REP-CEF-1-M1_Stratification%20of%20agroforestry.pdf den Herder, M
den Herder, M., Moreno, G., Mosquera-Losada, R. M., Palma, J. H. N., Sidiropoulou, A., Santiago Freijanes, J. J., et al. (2017). Current extent and stratification of agroforestry in the European Union. Agri. Ecosyst. Environ. 241, 121–132. doi:10.1016/j.agee.2017.03.005
Department of Environment, (2022). Food and rural Affairs of United Kingdom government. Structure of the Agricultural Industry. Available at: https://www.gov.uk/government/collections/structure-of-the-agricultural-industry (Accessed January 30, 2023).
Duarte, F., Jones, N., and Fleskens, L. (2008). Traditional olive orchards on sloping land: sustainability or abandonment? J. Environ. Manage. 89, 86–98. doi:10.1016/j.jenvman.2007.05.024
Eichhorn, M. P., Paris, P., Herzog, F., Incoll, L. D., Liagre, F., Mantzanas, K., et al. (2006). Silvoarable systems in europe - past, present and future prospects. Agrofor. Syst. 67, 29–50. doi:10.1007/s10457-005-1111-7
Esgalhado, C., Guimaraes, M. H., Lardon, S., Debolini, M., Balzan, M. V., Gennai-Schott, S. C., et al. (2021). Mediterranean land system dynamics and their underlying drivers: stakeholder perception from multiple case studies. Lands. Urban Plan. 213, 104134. doi:10.1016/j.landurbplan.2021.104134
European Environment Agency, (2016). Data from: biogeographical regions. Available at: https://www.eea.europa.eu/data-and-maps/data/biogeographical-regions-europe-3.
Eurostat, (2013a). Data from: data browser. Available at: https://ec.europa.eu/eurostat/databrowser/view/reg_area3/default/table?lang=en.
Eurostat, (2013b). Data from: data browser. Available at: https://ec.europa.eu/eurostat/databrowser/view/TAG00025/default/table.
Eurostat, (2009). LUCAS 2009 (land use/cover area frame survey). Technical reference document C-1. Instructions for surveyors. European Commission. Available at: https://ec.europa.eu/eurostat/documents/205002/208938/LUCAS+2009+Instructions.
Eurostat, (2019a). LUCAS 2018 (land use/cover area frame survey). Technical reference document C1. Instructions for surveyors. European Commission. Available at: https://jeodpp.jrc.ec.europa.eu/ftp/jrc-opendata/LUCAS/LUCAS_harmonised/3_supporting/LUCAS2018-C1-Instructions.pdf.
Eurostat, (2019b). LUCAS 2018 (land use/cover area frame survey). Technical reference document C3 classification (land cover and land use). Available at: https://ec.europa.eu/eurostat/documents/205002/8072634/LUCAS2018-C3-Classification.pdf.
Fagerholm, N., Torralba, M., Moreno, G., Girardello, M., Herzog, F., Aviron, S., et al. (2019). Cross-site analysis of perceived ecosystem service benefits in multifunctional landscapes. Glob. Environ. Change 56, 134–147. doi:10.1016/j.gloenvcha.2019.04.002
Hartel, T., Dorresteijn, I., Klein, C., Máthé, O., Moga, C. I., Öllerer, K., et al. (2013). Wood-pastures in a traditional rural region of Eastern Europe: characteristics, management and status. Biol. Conserv. 166, 267–275. doi:10.1016/j.biocon.2013.06.020
Hellenic Statistical Authority, (2019). Livestock surveys. Available at: https://www.statistics.gr/en/statistics/-/publication/SPK13/- (Accessed January 30, 2023).
Herzog, F. (1998). Streuobst: a traditional agroforestry system as a model for agroforestry development in temperate Europe. Agrofor. Syst. 42, 61–80. doi:10.1023/a:1006152127824
Inebase, (2011). Data from: censo agrario 2009. Available at: https://www.ine.es/dyngs/INEbase/es/operacion.htm?c=Estadistica_C&cid=1254736176851&menu=resultados&idp=1254735727106#!tabs-1254736194950 (Accessed January 30, 2023).
Inebase, (2022). Data from: censo agrario 2020. Available at: https://www.ine.es/dyngs/INEbase/es/operacion.htm?c=Estadistica_C&cid=1254736176851&menu=resultados&idp=1254735727106#!tabs-1254736194950 (Accessed January 30, 2023).
Jernsletten, J. L., and Klokov, K. (2002). Sustainable reindeer husbandry. Arctic Council 2000-2002. Centre for Saami Studies: University of TromsØ. Available at: http://www.reindeer-husbandry.uit.no/online/Final_Report/final_report.pdf.
Jose, S. (2009). Agroforestry for ecosystem services and environmental benefits: an overview. Agrofor. Syst. 76, 1–10. doi:10.1007/s10457-009-9229-7
Kay, S., Rega, C., Moreno, G., den Herder, M., Palma, J. H., Borek, R., et al. (2019). Agroforestry creates carbon sinks whilst enhancing the environment in agricultural landscapes in Europe. Land use policy 83, 581–593. doi:10.1016/j.landusepol.2019.02.025
Kirby, K. R., and Potvin, C. (2007). Variation in carbon storage among tree species: implications for the management of a small-scale carbon sink project. For. Ecol. Manag. 246, 208–221. doi:10.1016/j.foreco.2007.03.072
Kučera, J., Podhrázská, J., Karásek, P., and Papaj, V. (2020). The effect of windbreak parameters on the wind erosion risk assessment in agricultural landscape. J. Ecol. Eng. 21, 150–156. doi:10.12911/22998993/116323
Küpfer, C., and Balko, J. (2010). Streuobstwiesen in Baden-Württemberg-Wie viele Obstbaüme wachsen im Land und in welchem Zustand sind sie? Horizonte 35, 38–41.
Lasanta, T., Arnáez, J., Pascual, N., Ruiz-Flaño, P., Errea, M. P., and Lana-Renault, N. (2017). Space–time process and drivers of land abandonment in Europe. Catena 149, 810–823. doi:10.1016/j.catena.2016.02.024
Ministerio para la Transición Ecológica y el Reto Demográfico, (2022). Estrategia nacional de Lucha contra la Desertificación. Madrid: Ministerio para la Transición Ecológica y el Reto Demográfico.
Moral, J., Morgan, D., Trapero, A., and Michailides, T. J. (2019). Ecology and epidemiology of diseases of nut crops and olives caused by Botryosphaeriaceae fungi in California and Spain. Plant Dis. 103, 1809–1827. doi:10.1094/pdis-03-19-0622-fe
Moreno, G., Aviron, S., Berg, S., Crous-Duran, J., Franca, A., García de Jalón, S., et al. (2018). Agroforestry systems of high nature and cultural value in Europe: provision of commercial goods and other ecosystem services. Agrofor. Syst. 92, 877–891. doi:10.1007/s10457-017-0126-1
Moreno, G., and Pulido, F. J. (2009). “The functioning, management and persistence of dehesas,” in Agroforestry in Europe: current status and future prospects. Editors A. Rigueiro-Rodríguez, J. McAdam, and M. Mosquera-Losada (Dordrecht: Springer), 127–160.
Mosquera-Losada, M. R., McAdam, J., Romero-Franco, R., Santiago-Freijanes, J. J., and Riguero-Rodríquez, A. (2009). “Definitions and components of agroforestry practices in Europe,” in Agroforestry in Europe: current status and future prospects. Editors A. Rigueiro-Rodríguez, J. McAdam, and M. Mosquera-Losada (Dordrecht: Springer), 3–19.
Mosquera-Losada, M. R., Santiago-Freijanes, J. J., Rois-Díaz, M., Moreno, G., den Herder, M., Aldrey-Vázquez, J. A., et al. (2018). Agroforestry in Europe: a land management policy tool to combat climate Change. Land Use Policy 78, 603–613. doi:10.1016/j.landusepol.2018.06.052
Nerlich, K., Graeff-Hönninger, S., and Claupein, W. (2013). Agroforestry in Europe: a review of the disappearance of traditional systems and development of modern agroforestry practices, with emphasis on experiences in Germany. Agrofor. Syst. 87, 475–492. doi:10.1007/s10457-012-9560-2
Oosterbaan, A., and Kuiters, A. T. (2009). “Agroforestry in The Netherlands,” in Agroforestry in Europe: current status and future prospects. Editors A. Rigueiro-Rodríguez, J. McAdam, and M. R. Mosquera-Losada (Dordrecht: Springer), 331–341.
Palmieri, A., Reuter, H. I., Joebges, C., Eiselt, B., d'Andrimont, R., Lemoine, G., et al. (2020). Data from: harmonised LUCAS in-situ land cover and use database for field surveys from 2006 to 2018 in the European Union. European Commission, Joint Research Centre JRC. Available at: https://jeodpp.jrc.ec.europa.eu/ftp/jrc-opendata/LUCAS/LUCAS_harmonised/.
Pantera, Α., Mosquera-Losada, M. R., Herzog, F., and den Herder, M. (2021). Agroforestry and the environment. Agrofor. Syst. 95, 767–774. doi:10.1007/s10457-021-00640-8
Plieninger, T., Hartel, T., Martín-López, B., Beaufoy, G., Bergmeier, E., Kirby, K., et al. (2015a). Wood-pastures of Europe: geographic coverage, social–ecological values, conservation management, and policy implications. Biol. Conser. 190, 70–79. doi:10.1016/j.biocon.2015.05.014
Plieninger, T., Levers, C., Mantel, M., Costa, A., Schaich, H., and Kuemmerle, T. (2015b). Patterns and drivers of scattered tree loss in agricultural landscapes: orchard meadows in Germany (1968-2009). PLoS ONE 10, e0126178. doi:10.1371/journal.pone.0126178
Rodríguez-Rigueiro, F. J., Santiago-Freijanes, J. J., Mosquera-Losada, M. R., Castro, M., Silva-Losada, P., Pisanelli, A., et al. (2021). Silvopasture policy promotion in European Mediterranean areas. PLoS ONE 16, e0245846. doi:10.1371/journal.pone.0245846
San Miguel-Ayanz, A. (2004) ‘Mediterranean European silvopastoral systems’, in Silvopastoralism and sustainable land management. Proceedings of an international congress on silvopastoralism and sustainable management held in Lugo, Spain, eds. R. M. Mosquera-Losada, A. Rigueiro-Rodríguez, and J. McAdam (Wallingford UK: CABI Publishing), 36–40.
Santiago-Freijanes, J. J., Mosquera-Losada, M. R., Aldrey-Vázquez, J. A., and Rigueiro-Rodríguez, A. (2018). “Homegardens: agriculture in the city as an agroforestry practice,” in Agroforestry as sustainable land use, proceedings of the 4th European agroforestry conference. Editors R. M. Mosquera-Losada, and N. Ferreiro-Domínguez (Spain: European Agroforestry Federation and University of Santiago de Compostela in Lugo), 122–126.
Santos, P. Z. F., Crouzeilles, R., and Sansevero, J. B. B. (2019). Can agroforestry systems enhance biodiversity and ecosystem service provision in agricultural landscapes? A meta-analysis for the Brazilian Atlantic Forest. For. Ecol. Manag. 433, 140–145. doi:10.1016/j.foreco.2018.10.064
Schwartz, N. B., Uriarte, M., Gutiérrez-Vélez, V. H., Baethgen, W., Defries, R., Fernandes, K., et al. (2015). Climate, landowner residency, and land cover predict local scale fire activity in the Western Amazon. Glob. Environ. Change. 31, 144–153. doi:10.1016/j.gloenvcha.2015.01.009
Siriri, D., Wilson, J., Coe, R., Tenywa, M. M., Bekunda, M. A., Ong, C. K., et al. (2013). Trees improve water storage and reduce soil evaporation in agroforestry systems on bench terraces in SW Uganda. Agrofor. Syst. 87, 45–58. doi:10.1007/s10457-012-9520-x
Spilková, J., and Vágner, J. (2016). The loss of land devoted to allotment gardening: the context of the contrasting pressures of urban planning, public and private interests in Prague, Czechia. Land Use Policy 52, 232–239. doi:10.1016/j.landusepol.2015.12.031
Takács, V., and Frank, N. (2008). “The traditions, resources and potential of forest growing and multipurpose shelterbelts in Hungary,” in Agroforestry in Europe: current status and future prospects. Editors A. Rigueiro-Rodríguez, J. McAdam, and M. R. Mosquera-Losada (Dordrecht: Springer), 415–433.
Tölgyesi, C., Kelemen, A., Bátori, Z., Kiss, R., Hábenczyus, A. A., Havadtői, K., et al. (2023). Maintaining scattered trees to boost carbon stock in temperate pastures does not compromise overall pasture quality for the livestock. Agric. Ecosyst. Environ. 351, 108477. doi:10.1016/j.agee.2023.108477
Torralba, M., Fagerholm, N., Burgess, P. J., Moreno, G., and Plieninger, T. (2016). Do European agroforestry systems enhance biodiversity and ecosystem services? A meta-analysis. Agric. Ecosyst. Environ. 230, 150–161. doi:10.1016/j.agee.2016.06.002
Upson, M. A., Burgess, P. J., and Morison, J. I. L. (2016). Soil carbon changes after establishing woodland and agroforestry trees in a grazed pasture. Geoderma 283, 10–20. doi:10.1016/j.geoderma.2016.07.002
Varga, A. (2017). ‘Innovation from the past.’ silvopastoral systems in Hungary in the light of Hungarian ethnographic literature. Acta Ethnogr. Hung. 62 (1), 135–162. doi:10.1556/022.2017.62.1.7
Zomer, R. J., Trabucco, A., Coe, R., Place, F., van Noordwijk, M., and Xu, J. C. (2014). “Trees on farms: an update and reanalysis of agroforestry’s global extent and socio-ecological characteristics,” in Working paper 179. Bogor, Indonesia, world agroforestry Centre (ICRAF) southeast asia regional program. doi:10.5716/WP14064.PDF
Keywords: agroforestry, European Union, land use changes, spatial analysis, LUCAS data
Citation: Rubio-Delgado J, Schnabel S, Burgess PJ and Burbi S (2023) Reduced grazing and changes in the area of agroforestry in Europe. Front. Environ. Sci. 11:1258697. doi: 10.3389/fenvs.2023.1258697
Received: 14 July 2023; Accepted: 12 October 2023;
Published: 02 November 2023.
Edited by:
Hualin Xie, Jiangxi University of Finance and Economics, ChinaReviewed by:
László Erdős, Hungarian Academy of Science, HungaryMaria Villa, Instituto Politécnico de Bragança, Portugal
Copyright © 2023 Rubio-Delgado, Schnabel, Burgess and Burbi. This is an open-access article distributed under the terms of the Creative Commons Attribution License (CC BY). The use, distribution or reproduction in other forums is permitted, provided the original author(s) and the copyright owner(s) are credited and that the original publication in this journal is cited, in accordance with accepted academic practice. No use, distribution or reproduction is permitted which does not comply with these terms.
*Correspondence: Judit Rubio-Delgado, anVkaXRyZEB1bmV4LmVz
†These authors have contributed equally to this work