- 1Met Office Hadley Centre, Met Office, Exeter, United Kingdom
- 2School of Biological Sciences, Faculty of Life Sciences, University of Bristol, Bristol, United Kingdom
- 3Natural Sciences, Faculty of Environment Sciences and Economy, University of Exeter, Exeter, United Kingdom
- 4Crop Genetics Department, John Innes Centre, Norwich, United Kingdom
Record-breaking high temperatures were experienced across the United Kingdom during summer 2022. The impacts of these extreme climatic conditions were felt across the food system, including increased energy costs for cold storage, the failure of refrigeration systems in numerous retail facilities, and impacts on livestock including heat stress. Future climate projections indicate an increased likelihood and duration of extreme high temperatures like those experienced in 2022. Learning from the impacts of the 2022 heatwave on the United Kingdom food system can help identify adaptations that build resilience to climate change. We explore the impacts through two case studies (United Kingdom poultry and wheat sectors), discuss potential adaptation options required for a climate-resilient, net-zero United Kingdom food system and consider future research needs. United Kingdom chicken meat production was 9% lower in July 2022 than July 2021; in contrast, energy costs increased for both production and refrigeration. Potential heatwave adaptation measures for poultry include transitioning to heat tolerant chicken breeds, lower stocking density, dehumidification cooling and misting systems, nutritional supplements, and improving retail refrigeration resilience and efficiency. United Kingdom wheat yields were 8% higher in 2022 than the 2017–2021 average. Increases were observed in every United Kingdom region but were least in the South and East where the heatwave intensity was strongest. Future adaptation measures to avoid negative impacts of summer heat stress on winter wheat could include earlier maturing and heat/drought tolerant varieties, earlier autumn sowing, targeted irrigation for drought around anthesis, and soil and water conservation measures.
1 Introduction
Weather and climate extremes have wide-ranging impacts throughout the UK food system (Falloon et al., 2022) and are one of its highest-risk future shocks (Betts et al., 2021). High temperatures cause heat stress to crops, livestock and workforces (Falloon and Betts, 2010; GFS, 2014; Falloon et al., 2015). The hot, dry summers of 2018 and 2020 reduced UK crop yields (Committee on Climate Change, 2018; Berry and Brown, 2021), while high summer temperatures reduce the quality of fruits, brassicas, and tomatoes (Committee on Climate Change, 2018). This can increase supply variability across the food chain which is compounded by a simultaneous increase in consumer demand for barbecue food, salads, and fresh fruit. High temperatures increase food spoilage and safety risks (Bezner-Kerr et al., 2022), demanding different handling, storage and transport practices including increased cold chain use (James and James, 2010).
2022 was a record warm year in the UK observational record since 1884, the first year with an annual mean temperature above 10°C, and the seventh sunniest year since 1910 (Kendon M. et al., 2023a). 2022 rainfall was 6% below the 1991–2020 average, with January-August 2022 being the driest across southern England since 1976, and equal-sixth driest since 1836 (Kendon M. et al., 2023a). The unprecedented 18th-19th July heatwave (Kendon M. et al., 2023a) exceeded previous records, with the highest temperatures occurring in the East of the UK, including temperatures above 40°C for the first time on records (Figure 1).
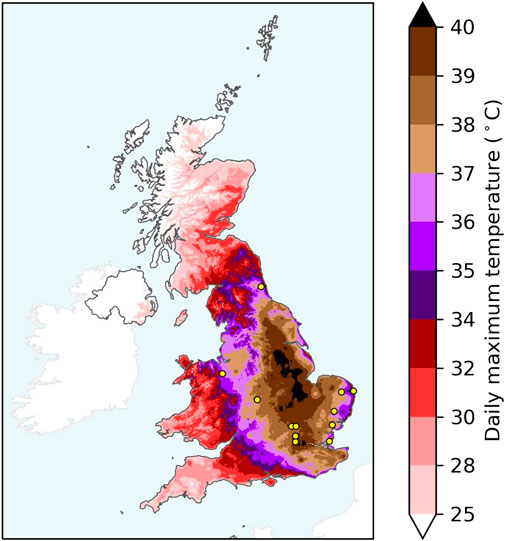
FIGURE 1. UK maximum temperatures on 19 July 2022 (HadUK-Grid 1 km data; Hollis et al., 2018); yellow dots represent supermarket stores that experienced refrigeration system issues during the summer 2022 heatwave.
The impacts of these extreme conditions were felt across the UK food system including:
• Increased energy costs for cold storage1 and refrigeration systems failure in retail facilities2
• Yield losses in fruits and vegetables3
• Increased livestock heat stress (Cooke and Rivero, 2023)
• Limited availability of grass forage for livestock
Human influence has already increased the chance of the UK experiencing temperatures above 40°C, relative to the pre-industrial period (Christidis et al., 2020). Future projections indicate an increasing likelihood and duration of extreme high temperatures at least as severe as those experienced in 2022. By the 2070s under a high emissions scenario, hot summer days increase by 3.8°C–6.8°C, along with a large increase in the frequency of hot spells (Met Office, 2021; Met Office, 2022). The intensity of summer rainfall events is expected to increase, but summers are projected to be drier overall with an increased severity of multi-season droughts (Hanlon et al., 2021).
The UK agri-food sector contributed £116.2 billion (6%) to national Gross Value Added in 2020 (Department for Environment Food and Rural Affairs, 2023a), and poultry meat and wheat production were worth £3.1 billion and £4.1 billion in 2022, respectively (Department for Environment Food and Rural Affairs, 2023b). We focus on the impacts of the 2022 UK summer heatwave using two case studies: poultry and wheat. We explore potential adaptations to manage the effects of future heatwaves, contributing to a climate-resilient, net-zero UK food system.
2 Poultry sector
2.1 Heatwave impacts
The impacts of the summer 2022 heatwave were experienced throughout the UK poultry industry supply chain, markedly in broiler farms and retail facilities.
In July 2022, the production of chicken meat by volume in the UK experienced a 9.2% reduction compared to July 2021 (a 2.6% reduction from the 1997–2022 July average, for which the year-to-year standard deviation is 5.6%; Department for Environment Food and Rural Affairs, 2022a). Greater damages were experienced in the East of the UK, which was exposed to the highest temperatures (Figure 1). Animal welfare-reported incidents of heat stress and dead-on-arrival (DOA) at slaughterhouses4 were impacted in July and August 2022, while over 18,500 chickens died in transport due to heat stress—compared to 325 deaths in the same period in 20215.
Poultry meat production is highly energy intensive due to the demand for heating/cooling and lighting (Tsolakis et al., 2018). Retail stores in Eastern UK experienced the strongest impacts. One supermarket experienced issues in the refrigeration systems of 12 stores (Figure 1), while its energy consumption increased to ∼4 GWh above the expected summer value6. Electricity consumption on 19th July 2022 was ∼20% higher than the average daily summer consumption.
2.2 Potential adaptation measures
Currently, the most farmed breeds are selected for large breast size (∼40% total body mass), while heat resistant breeds have smaller breast size (∼35% total body mass). Changing to more heat tolerant breeds such as the Orpington (Cheng, 2010; Li et al., 2013; Fu et al., 2016) could reduce heat stress-related mortality rates. Even accounting for decreased breast size, this could provide a net economic benefit during heatwaves (Sun et al., 2021). Changes in nutrition (e.g., vitamins and feed additives) can mitigate some heat stress impacts but evidence is conflicting regarding their efficacy (Supplementary Table S1).
Broiler housing can be altered to decrease heatwave impacts using misting systems and ventilation (Department for Environment Food and Rural Affairs, 2018). Misting systems are the least expensive method requiring little alteration, but their utility in reducing heat stress is limited since they increase humidity (Khalifa et al., 2018). These issues may be reduced using maximized air flow and/or alternative dehumidification cooling systems to reduce relative humidity levels (Aleem et al., 2022) and overall heat stress impacts.
Lower stocking densities can improve breast meat quality and relieve oxidative stress from high temperatures (Son et al., 2022), with no upfront cost. The impacts of heat stress on poultry during transport (Davie et al., 2021) could be reduced by increasing air-conditioning, increasing airflow and night-time transport (Falloon et al., 2023).
Installing protective systems could prevent existing electricity networks that support refrigeration from collapsing during a heatwave. Auto load shedding switches off selected components at peak demand, preventing power blackouts (Ahsan et al., 2012) but reduces refrigeration capacity.
3 Wheat sector
3.1 Heatwave impacts
In contrast to impacts on poultry in 2022, UK winter wheat production was not adversely affected. 2022 wheat yields were 8% higher than the 2017–2021 average, with increases in every UK region7 (Table 1). The 2022 yield increases were greater in Northern/Western regions of the UK, and least in the South/East where the intensity of the heatwave was strongest. Under long-term climate scenarios, a similar South/East-North/West gradient of wheat yield changes (Cho et al., 2012) and variability (Putelat et al., 2021) has been noted. While high temperatures and drought can negatively impact wheat production (e.g., Machado and Paulsen, 2001; Farooq et al., 2011; 2014; Barlow et al., 2015) the magnitude of impacts depend on the severity of the hazard and the wheat growth stages during which they occur.
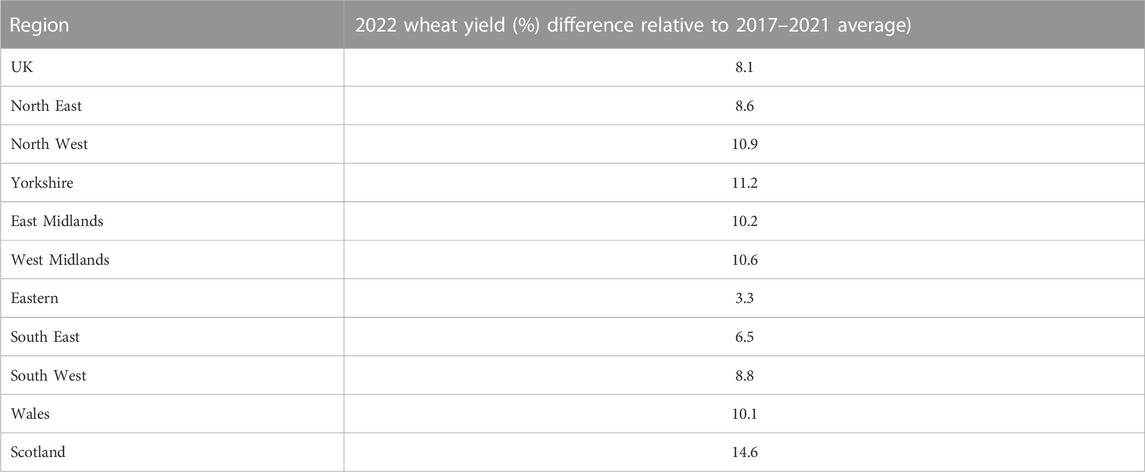
TABLE 1. 2022 wheat yields for UK regions, percentage difference relative to the 2017–2021 average yield for each region. Data source: Department for Environment Food and Rural Affairs (2022b).
Hotter, drier summers will be more common in the future due to climate change (Met Office, 2022). Understanding the reasons for the higher 2022 wheat yields, despite these conditions, and whether the benefits might be maintained during multi-year hot and dry periods will help underpin future wheat resilience by informing farming adaptations and the development of new varieties.
3.1.1 Temperature
Heat adversely affects wheat yield and quality via prolonged (weeks-months) periods of above optimum temperatures, or short periods (∼1–3 days) of very high temperatures above approximately 35°C (e.g., Harkness et al., 2020). The impacts are greatest in the 2 weeks leading to anthesis (flowering) and grain filling, impacting on grain number and weight respectively (Farooq et al., 2011; Barlow et al., 2015). Impacts on grain filling are most significant in the early stages of this process.
The warm temperatures were distributed unevenly throughout the summer, with anomalies of 0.5°C, 1.3°C, and 1.5°C above the 1991–2020 averages for June, July, and August, respectively (Kendon M. et al., 2023a). There were two significant heatwaves in mid-July and mid-August. UK crop development reports8 for 2022 suggest that anthesis occurred, as normal, around the end of May and early June, with most winter wheat being in growth stage 71 out of 99 (flowering occurs at stage 61). The most critical period of vulnerability to heat stress for most UK winter wheat was late May to early July 2022 when temperatures were above the long-term mean, but there were no significant heat shocks. The exceptionally warm conditions occurred too late in the crop lifecycle to have a significant impact. Winter wheat harvesting9 began during the second week of July and was largely complete by the end of August, associated with the extended warm period during 2022.
3.1.2 Drought
Drought stress primarily affects wheat yields during the reproductive period (Harkness et al., 2020)—in the two-week window before and including anthesis via reduced grain numbers, and after anthesis via a reduction in grain filling which negatively impacts grain weight. Severe crop growth reduction can occur if the entire growing season experiences water stress (Harkness et al., 2020).
Summer 2022 was dry for the UK, receiving 64% of average rainfall versus the 1991–2020 baseline, ranking in the lower third of all years since 1836. Rainfall was not evenly distributed throughout the summer. May, June, July and August recorded 109%, 80%, 59% and 60% of rainfall relative to 1991–2020 respectively. Dry spells occurred in mid-to-late January, late March and April, July, and early August 2022. Crucially, May to early July did not experience significant prolonged dry spells (Kendon M. et al., 2023a).
It appears there was sufficient rainfall during the period when winter wheat is particularly vulnerable to avoid significant yield impacts. The winter and spring dry spells may not have been significant enough to impact crop development, perhaps due to sufficient subsoil water from 2021 rainfall being available for crop growth. Access to subsoil water would have increased resilience to the July-August dry spells when grain filling was taking place but cannot be guaranteed in multi-year hot and dry periods.
3.2 Potential adaptation measures
The 2022 heatwave illustrated that high temperatures may not necessarily have detrimental impacts on winter wheat yields, depending on their timing during the growing season. The regional statistics (Table 1) hint at the potential for adverse impacts in more severe conditions, with smaller yield increases in regions experiencing higher temperatures. This suggests several potential adaptation strategies. Growing earlier maturing varieties would allow anthesis to occur earlier and avoid greater risks from high temperatures, while earlier autumn sowing (Cho et al., 2012) could allow crops longer development time. Putelat et al. (2021) suggest that future wheat crops may escape heat (and drought) impacts through faster development because of warmer year-round temperatures. High temperature impacts could be mitigated by targeted breeding for deeper root systems combined with lower metabolic costs (Li et al., 2022), water storage and irrigation for drought around anthesis, or soil conservation and improvement measures to increase water and nutrient retention. The winter wheat varieties grown in the UK are photoperiod sensitive varieties and require a vernalization period (Sheehan and Bentley, 2021). Earlier planting dates may put crops at greater risk of disease and frost damage during winter. These factors will need to be considered when choosing appropriate adaptation options.
4 Discussion
UK-wide increases in the average number of days per year when livestock heat stress thresholds are exceeded are anticipated (Supplementary Figure S1). This suggests a clear need for effective large-scale adaptation. Adaptation measures for poultry production include transitioning to heat resistant breeds, changing nutritional intake, reducing stocking densities, and improving ventilation and misting systems in poultry housing. At the retail and consumer end, increasing heatwave frequency and intensity will put pressure on refrigeration systems, increasing energy costs and system failures. Efficiency improvements and installation of protective systems for power supply to refrigeration systems could reduce heatwave vulnerability in retail facilities.
The above average wheat yields reported in 2022 support studies that suggest climate change may have different impacts on UK wheat depending on where it is grown (e.g., Harkness et al., 2020; Jägermeyr et al., 2021; Putelat et al., 2021). Wheat is vulnerable to extreme heat and drought in late spring or early summer. The increasing likelihood of these conditions under future climates, particularly in the South/East of England, suggests that inter-annual and spatial yield variability may increase in the absence of effective adaptation such as better water management and heat and drought tolerant varieties.
4.1 Barriers to adaptation measures
Current UK wheat varieties are bred for high grain yield. Moving to more heat (or drought) resilient crops may require trade-offs, whereby resilient varieties perform well during stress conditions, but less well than current varieties during ‘normal’ conditions. For poultry breeds, there will be a trade-off between meat quantity per bird and resilience during heatwaves. Further research is required to understand the longer-term production and economic implications of such trade-offs.
Current challenges to the UK poultry sector (e.g., avian influenza and economics) mean that widespread transition may only be feasible with additional support. All farmers are eligible for the UK government’s ‘Basic Payment Scheme (BPS)’ (Department for Environment Food and Rural Affairs, 2019; Rural Payments Agency, 2022), but poultry farmers are currently ineligible for further subsidies. In addition, at least 5 ha of eligible land are required to receive the BPS while approximately 45% of specialist poultry farmers in England have less than this amount (Department for Environment Food and Rural Affairs, 2023c). Financial barriers could impact the uptake of proposed adaptation options, both for poultry and winter wheat. For the refrigeration systems of retail facilities, retrofitting one technique or complete system replacement come at high initial costs. The longer-term economic outcomes therefore need to be assessed, and priority should be given to stores in the most vulnerable regions. Changing poultry breeds or reducing stocking densities may have smaller financial impacts during heatwave conditions. The financial implications of planting earlier maturing and heat/drought tolerant wheat varieties is uncertain and will depend on the balance between lower production during ‘normal’ years and seasons experiencing significant heat impacts. Improved soil and water conservation measures are unlikely to incur significant additional costs, while implementing targeted irrigation would be a significant change for the UK wheat sector.
Seasonal forecasts could support adaptation decision making in the agrifood sector (Falloon et al., 2023), but their usefulness for heatwave resilience in the poultry and wheat sectors will depend on several factors. The timescales of decision making will impact whether effective action can be taken—in wheat farming many decisions relevant to high temperature impacts (e.g., sowing dates, varieties planted) are made the year before harvest, so opportunities to take a different course of action may be limited. The skill of seasonal forecasts is limited during the UK growing season (Falloon et al., 2018), and trust (Pope et al., 2017), user-relevance, and experience with probabilistic information also affect uptake.
Large-scale implementation of effective adaptation measures requires consideration of which actors should drive, fund and facilitate it—which will impact how the risks at different scales (e.g., individual farmers, processors, retailers, or overall UK food security) are balanced.
4.2 Implications of adaptation measures for net zero
The refrigerants used in common hydrofluorocarbon (HFC) refrigeration systems, R404A and R407A, have global warming potentials (GWP) of 3,943 and 2,107, respectively (Mota-Babiloni et al., ¸ 2015; Acha et al., 2016; Gao et al., 2022). With annual leak rates up-to 30%, HFC systems release greenhouse gases that deplete stratospheric ozone (Besher et al., 2015). CO2 has a much lower GWP than HFC, so is the preferred refrigerant for retail in terms of net zero goals (Santosa et al., 2018; Dilshad et al., 2019; Efstratiadi et al., 2019; Yu et al., 2019). However, CO2-based systems are less efficient than HFC systems in hot climates (Fricke et al., 2019), implying a trade-off between the implications for net zero goals and climate mitigation, and for climate adaptation. Performance enhancement technologies for CO2-based systems could improve their high-temperature efficiency (Ge and Tassou, 2009), and contribute to emissions reductions. Increasing poultry housing ventilation and misting and implementing transport measures to mitigate heatwave impacts could conflict with net zero goals by increasing energy consumption (which increases during high temperatures due to lower efficiency) and greenhouse gas emissions (Falloon et al., 2022).
Changing wheat varieties and sowing dates are likely to have negligible impacts on GHG emissions or carbon storage, while irrigation could incur additional energy costs via pumping, and soil and water conservation measures could potentially improve net zero outcomes through increased soil carbon storage and reduced soil carbon losses (e.g., Page et al., 2020).
4.3 Future research needs
The 2022 UK heatwave illustrates the complexity of the impacts of extreme events on the agrifood system; future research should aim to provide detailed studies of them, the resulting needs for adaptation and implications for net-zero goals. A better understanding of the interactions between the timing of extreme weather events and management decisions across the whole food chain could support better resilience. For example, how do antecedent conditions affect impacts, and how do differences in operational decisions affect the overall outcome?
A key challenge for food system resilience is the availability of data to support research studies, and development and evaluation of models and tools (Falloon et al., 2022), both within the food chain, and of local meteorological conditions. Examples include the lack of robust, detailed, public data on poultry mortality and welfare in housing and transport, but also across the broader food chain (e.g., consumer trends, energy use, storage and transport practices/impacts, food safety aspects and workforce heat stress). There is also a lack of readily available, detailed crop yield data in the UK that would support impacts and adaptation assessments, and the development and application of models.
Further research is needed to quantify the effectiveness of adaptation options, both individually and in combination, and to provide cost-benefit analyses. Further research is needed to develop optimum winter wheat varieties for the future UK climate, considering the expected occurrence of extreme events, the potential for compound events and multi-year drought, and vernalisation requirements. In addition, the effectiveness of broader range of adaptation options should be assessed, including diversification and alternative cropping systems.
There is a need to build on experience gained during extreme weather events to help avoid ‘knee-jerk’ responses and maladaptation. For example, for very heavy rainfall events future changes are not expected to unfold as a smooth trend and could be experienced as clusters of record-breaking events followed by decades with none (Kendon E. J. et al., 2023b). Therefore, costly short-term decisions taken to adapt to an experienced weather shock may not prove cost-effective in the mid-term. The timing of extreme events and weather patterns are critical to food system impacts—for example, 2023 UK wheat yields and quality were negatively impacted by high rainfall. In addition, given the projected increases in both the frequency and severity of extreme weather events towards the end of this century, greater levels of adaptation will be needed in the long-term to maintain resilience.
Improvements in seasonal forecasting to support agricultural decision making (e.g., Falloon et al., 2018) would help farmers choose appropriate varieties for upcoming seasons and prepare adaptations to farming practices in advance; there is also potential for long-range forecasts to support decision-making across the broader food chain (Falloon et al., 2022). Further developments to process-based models and statistical/machine learning models of wheat yield (e.g., Shirley et al., 2020) will be valuable in identifying indirect impacts on yields (e.g., farming practices and pest and disease pressures).
Data availability statement
The original contributions presented in the study are included in the article/Supplementary Materials, further inquiries can be directed to the corresponding author.
Author contributions
JD: Supervision, Writing–original draft, Writing–review and editing, Conceptualization, Investigation. PF: Conceptualization, Writing–original draft, Writing–review and editing, Supervision. DP: Conceptualization, Investigation, Writing–original draft, Writing–review and editing. TS: Conceptualization, Investigation, Writing–original draft, Writing–review and editing. MH: Conceptualization, Investigation, Writing–original draft, Writing–review and editing. TW: Conceptualization, Investigation, Writing–original draft, Writing–review and editing. TL: Conceptualization, Investigation, Writing–original draft, Writing–review and editing. EG: Conceptualization, Investigation, Writing–original draft, Writing–review and editing. JeS: Conceptualization, Investigation, Writing–original draft, Writing–review and editing. JaS: Conceptualization, Investigation, Writing–original draft, Writing–review and editing. TC: Conceptualization, Writing–original draft, Writing–review and editing. AC: Conceptualization, Writing–original draft, Writing–review and editing. EP: Conceptualization, Writing–original draft, Writing–review and editing. SG: Conceptualization, Writing–original draft, Writing–review and editing.
Funding
The author(s) declare financial support was received for the research, authorship, and/or publication of this article. JD, PF, TC, AC, and EP were supported by the Met Office Food, Farming and Natural Environment Climate Service, funded by the UK’s Department for Environment, Food and Rural Affairs (Defra).
Acknowledgments
The authors would like to thank Chris Brown (ASDA, UK) for assistance with information on the poultry case study.
Conflict of interest
The authors declare that the research was conducted in the absence of any commercial or financial relationships that could be construed as a potential conflict of interest.
The reviewer KT declared a past collaboration with the author PF to the handling editor.
The authors declared that they were an editorial board member of Frontiers, at the time of submission. This had no impact on the peer review process and the final decision.
Publisher’s note
All claims expressed in this article are solely those of the authors and do not necessarily represent those of their affiliated organizations, or those of the publisher, the editors and the reviewers. Any product that may be evaluated in this article, or claim that may be made by its manufacturer, is not guaranteed or endorsed by the publisher.
Supplementary material
The Supplementary Material for this article can be found online at: https://www.frontiersin.org/articles/10.3389/fenvs.2023.1282284/full#supplementary-material
Footnotes
1https://www.foodmanufacture.co.uk/Article/2022/08/15/Extreme-weather-ravaging-the-UK-challenges-food-industry.
2Personal communication—Chris Brown, Asda.
3https://www.theguardian.com/environment/2022/aug/01/uk-farmers-count-cost-as-heatwave-kills-fruit-and-vegetable-crops.
4https://www.food.gov.uk/board-papers/fsa-22-09-18-annual-animal-welfare-main-report-202122.
5https://www.carbonbrief.org/revealed-thousands-of-chickens-in-transit-died-from-heat-stress-on-day-uk-hit-40c/.
6Personal communication—Chris Brown, Asda.
7https://www.gov.uk/government/statistics/cereal-and-oilseed-rape-production/cereal-and-oilseed-production-in-the-united-kingdom-2022. Note that 2022 yield data for Northern Ireland are not available.
8https://ahdb.org.uk/cereals-oilseeds/crop-development-report.
9https://ahdb.org.uk/cereals-oilseeds/gb-harvest-progress.
References
Acha, S., Du, Y., and Shah, N. (2016). Enhancing energy efficiency in supermarket refrigeration systems through a robust energy performance indicator. Intl. J. Refrig. 64, 40–50. doi:10.1016/j.ijrefrig.2015.12.003
Ahsan, M. Q., Chowdhury, A. H., Ahmed, S. S., Bhuyan, I. H., Haque, M. A., and Rahman, H. (2012). Technique to develop auto load shedding and islanding Scheme to prevent power system blackout. IEEE Trans. Power Syst. 27, 198–205. doi:10.1109/tpwrs.2011.2158594
Aleem, M., Sultan, M., Mahmood, M., and Miyazaki, T. (2022). Desiccant dehumidification cooling system for poultry houses in multan (Pakistan). Energy-Efficient Sys. Agric. Applic. 19-42, 19–42. doi:10.1007/978-3-030-86394-4_2
Barlow, K. M., Christy, B. P., O’Leary, G. J., Riffkin, P. A., and Nuttall, J. G. (2015). Simulating the impact of extreme heat and frost events on wheat crop production: a review. Field Crops Res. 171, 109–119. doi:10.1016/j.fcr.2014.11.010
Berry, P., and Brown, I. (2021). “National environment and assets,” in The third UK climate change risk assessment technical report. Editors R. A. Betts, A. B. Haward, and K. V. Pearson (London: Climate Change Committee).
Besher, M., Aute, V., Sharma, V., Abdelaziz, O., Fricke, B., and Radermacher, R. (2015). A comparative study on the environmental impact of supermarket refrigeration systems using low GWP refrigerants. Intl. J. Refrig. 56, 154–164. doi:10.1016/j.ijrefrig.2015.03.025
Betts, R. A., Haward, A. B., and Pearson, K. V. (2021). The third UK climate change risk assessment technical report. London: Climate Change Committee.
Bezner Kerr, R., Hasegawa, T., Lasco, R., Bhatt, I., Deryng, D., Farrell, A., et al. (2022). in Food, fibre, and other ecosystem products” in climate change 2022: impacts, adaptation, and vulnerability. Contribution of working group II to the sixth assessment Report of the intergovernmental Panel on climate change. Editors H.-O. Pörtner, D. C. Roberts, M. Tignor, E. S. Poloczanska, K. Mintenbeck, A. Alegríaet al. (Cambridge: Cambridge University Press).
Bollengier-Lee, S., Mitchell, M. A., Utomo, D. B., Williams, P. E. V., and Whitehead, C. C. (1998). Influence of high dietary vitamin E supplementation on egg production and plasma characteristics in hens subjected to heat stress. Br. Poult. Sci 39, 106–112. doi:10.1080/00071669889466
Cheng, H.-W. (2010). Breeding of tomorrow’s chickens to improve well-being. Poult. Sci. 89, 805–813. doi:10.3382/ps.2009-00361
Cho, K., Falloon, P., Gornall, J., Betts, R., and Clark, R. (2012). Winter wheat yields in the UK: uncertainties in climate and management impacts. Clim. Res. 54, 49–68. doi:10.3354/cr01085
Christidis, N., McCarthy, M., and Stott, P. A. (2020). The increasing likelihood of temperatures above 30 to 40 °C in the United Kingdom. Nat. Commun. 11, 3093. doi:10.1038/s41467-020-16834-0
Committee on Climate Change (2018). Land use: reducing emissions and preparing for climate change. Available at: https://www.theccc.org.uk/publication/land-use-reducing-emissions-and-preparing-for-climate-change/ (Accessed August 09, 2023).
Cooke, A. S., and Rivero, M. J. (2023). Livestock heat stress risk in response to the extreme heat event (heatwave) of July 2022 in the UK. bioRxiv. doi:10.1101/2023.05.18.541284
Davie, J. C. S., Garry, F. K., and Pope, E. C. D. (2021). Analysis of heat stress for UK livestock using UKCP18 climate data. Exeter: Met Office Hadley Centre.
Department for Environment Food and Rural Affairs (2022a). United Kingdom poultry and poultry meat statistics – August 2022. Available at: https://www.gov.uk/government/statistics/historical-statistics-notices-on-poultry-and-poultry-meat-production-2022/united-kingdom-poultry-and-poultry-meat-statistics-august-2022 (Accessed August 09, 2023).
Department for Environment, Food and Rural Affairs (2018). Code of practice for the welfare of meat chickens and meat breeding chickens. https://assets.publishing.service.gov.uk/government/uploads/system/uploads/attachment_data/file/694013/meat-chicken-code-march2018.pdf (Accessed August 09, 2023).
Department for Environment Food and Rural Affairs (2019). Guidance: poultry: welfare recommendations. Available at: https://www.gov.uk/government/publications/poultry-on-farm-welfare/poultry-welfare-recommendations (Accessed August 09, 2023).
Department for Environment Food and Rural Affairs (2022b). Cereal and oilseed rape production. Available at: https://www.gov.uk/government/statistics/cereal-and-oilseed-rape-production (Accessed August 09, 2023).
Department for Environment Food and Rural Affairs (2023a). Food statistics in your pocket: food chain. Available at: https://www.gov.uk/government/statistics/food-statistics-pocketbook/food-statistics-in-your-pocket (Accessed August 09, 2023).
Department for Environment Food and Rural Affairs (2023b). Agriculture in the United Kingdom 2022. Available at: https://www.gov.uk/government/statistics/agriculture-in-the-united-kingdom-2022 (Accessed August 09, 2023).
Department for Environment Food and Rural Affairs (2023c). Structure of the agricultural industry in England and the UK at June. Available at: https://www.gov.uk/government/statistical-data-sets/structure-of-the-agricultural-industry-in-england-and-the-uk-at-june (Accessed September 25, 2023).
Dilshad, S., Kalair, A. R., and Khan, N. (2019). Review of carbon dioxide (CO2) based heating and cooling technologies: past, present, and future outlook. Intl. J. Energy Res. 44, 1408–1463. doi:10.1002/er.5024
Efstratiadi, M., Acha, S., Shah, N., and Markides, C. N. (2019). Analysis of a closed-loop water-cooled refrigeration system in the food retail industry: a UK case study. Energy 174, 1133–1144. doi:10.1016/j.energy.2019.03.004
Falloon, P., Bebber, D., Bryant, J., Bushell, M., Challinor, A. J., Dessai, S., et al. (2015). Using climate information to support crop breeding decisions and adaptation in agriculture. World Agric. 5, 25–42.
Falloon, P., Bebber, D. P., Dalin, C., Ingram, J., Mitchell, D., Hartley, T. N., et al. (2022). What do changing weather and climate shocks and stresses mean for the UK food system? Env. Res. Lett. 17, 051001. doi:10.1088/1748-9326/ac68f9
Falloon, P., Bruno Soares, M., Manzanas, R., San-Martin, D., Liggins, F., Taylor, I., et al. (2018). The land management tool: developing a climate service in Southwest UK. Clim. Serv. 9, 86–100. doi:10.1016/j.cliser.2017.08.002
Falloon, P., Hodge, K., Barrow, A., New, S., Garry, F., Oakes, R., et al. (2023). Impacts of weather and climate extremes on the UK food chain – scoping adaptation and policy responses and their consequences: workshop report. Met Office UK: Report to Defra, Met Office Food, Farming and Natural Environment Climate Service.
Falloon, P. D., and Betts, R. A. (2010). Climate impacts on European agriculture and water management in the context of adaptation and mitigation - the importance of an integrated approach. Sci. Tot. Environ. 408, 5667–5687. doi:10.1016/j.scitotenv.2009.05.002
Farooq, M., Bramley, H., Palta, J. A., and Siddique, K. H. M. (2011). Heat stress in wheat during reproductive and grain-filling phases. Crit. Rev. Plant Sci. 30, 491–507. doi:10.1080/07352689.2011.615687
Farooq, M., Hussain, M., and Siddique, K. H. M. (2014). Drought stress in wheat during flowering and grain-filling periods. Crit. Rev. Plant Sci. 33, 331–349. doi:10.1080/07352689.2014.875291
Faye, B., Foster, I., Folberth, C., Franke, J. A., Fuchs, K., Guarin, J. R., et al. (2021). Climate impacts on global agriculture emerge earlier in new generation of climate and crop models. Nat. Food. 2, 873–885. doi:10.1038/s43016-021-00400-y
Fricke, B. A., Nawaz, K., Elatar, A. F., and Sharma, V. (2019). “Increasing the efficiency of a carbon dioxide refrigeration system using a pressure exchanger,” in Proceedings of the 25th IIR International Congress of Refrigeration: Montréal, Canada, August 24-30, 2019, 1775–1781. doi:10.18462/iir.icr.2019.1598
Fu, W., Lee, W. R., and Abasht, B. (2016). Detection of genomic signatures of recent selection in commercial broiler chickens. BMC Genet. 17, 122. doi:10.1186/s12863-016-0430-1
Gao, E., Zhang, Z., Deng, Q., Jing, H., Wang, X., and Zhang, X. (2022). Techno-economic and environmental analysis of low-GWP alternative refrigerants in cold storage unit under year-round working conditions. Intl. J. Refrig. 134, 197–206. doi:10.1016/j.ijrefrig.2021.11.007
Ge, Y. T., and Tassou, S. A. (2009). Control optimisation of CO2 cycles for medium temperature retail food refrigeration systems. Intl. J. Refrig. 32, 1376–1388. doi:10.1016/j.ijrefrig.2009.01.004
GFS (2014). GFS Insight Issue 2: severe weather and UK food resilience. Available at: https://www.foodsecurity.ac.uk/publications/insight-issue-two-severe-weather-and-uk-food-resilience.pdf (Accessed August 09, 2023).
Habibian, M., Ghazi, S., Moeini, M. M., and Abdolmohammadi, A. (2013). Effects of dietary selenium and vitamin E on immune response and biological blood parameters of broilers reared under thermoneutral or heat stress conditions. Intl. J. Biometeorol. 58, 741–752. doi:10.1007/s00484-013-0654-y
Hanlon, H. M., Bernie, D., Carigi, G., and Lowe, J. A. (2021). Future changes to high impact weather in the UK. Clim. Change 166, 50–23. doi:10.1007/s10584-021-03100-5
Harkness, C., Semenov, M. A., Areal, F., Senapati, N., Trnka, M., Balek, J., et al. (2020). Adverse weather conditions for UK wheat production under climate change. Agric. For. Meteorol. 282–283, 107862. doi:10.1016/j.agrformet.2019.107862
Hollis, D., McCarthy, M., Kendon, M., Legg, T., and Simpson, I. (2018). HadUK-Grid gridded and regional average climate observations for the UK. Exeter: Centre for Environmental Data Analysis. Available at: http://catalogue.ceda.ac.uk/uuid/4dc8450d889a491ebb20e724debe2dfb.
James, S., and James, C. (2010). The food cold-chain and climate change. Food Res. Intern. 43, 1944–1956. doi:10.1016/j.foodres.2010.02.001
Jägermeyr, J., Müller, C., Ruane, A. C., et al. (2021). Climate impacts on global agriculture emerge earlier in new generation of climate and crop models. Nat. Food. 2, 873–885. doi:10.1038/s43016-021-00400-y
Kendon, E. J., Fischer, E. M., and Short, C. J. (2023b). Variability conceals emerging trend in 100yr projections of UK local hourly rainfall extremes. Nat. Commun. 14, 1133. doi:10.1038/s41467-023-36499-9
Kendon, M., McCarthy, M., Jevrejeva, S., Matthews, A., Williams, J., Sparks, T., et al. (2023a). State of the UK climate 2022. Intl. J. Climatol. 43, 1–83. doi:10.1002/joc.8167
Khalifa, E., AbouZaher, S., and Elmoghazy, H. (2018). Effectiveness of two different evaporative cooling systems in a synthetic warmed air space. Misr J. Agric. Eng. 35, 1473–1492. doi:10.21608/mjae.2018.95356
Li, B., Zhang, X., Morita, S., Sekiya, N., Araki, H., Gu, H., et al. (2022). Are crop deep roots always beneficial for combating drought: a review of root structure and function, regulation and phenotyping. Agric. Water Manag. 271, 107781. doi:10.1016/j.agwat.2022.107781
Li, Q., Xu, Z., Liu, L., Yu, H., Rong, H., Tao, L., et al. (2013). Effects of breeds and dietary protein levels on the growth performance, energy expenditure and expression of avUCP mRNA in chickens. Mol. Biol. Rep. 40, 2769–2779. doi:10.1007/s11033-012-2030-0
Machado, S., and Paulsen, G. M. (2001). Combined effects of drought and high temperature on water relations of wheat and sorghum. Plant Soil 233, 179–187. doi:10.1023/A:1010346601643
Met Office (2021). Update to UKCP local (2.2km) projections. Available at: https://www.metoffice.gov.uk/pub/data/weather/uk/ukcp18/science-reports/ukcp18_local_update_report_2021.pdf (Accessed August 17, 2023).
Met Office (2022). UK Climate Projections headline findings. Available at: https://www.metoffice.gov.uk/research/approach/collaboration/ukcp/summaries/headline-findings (Accessed August 09, 2023).
Mota-Babiloni, A., Navarro-Esbri, J., Peris, B., Moles, F., and Verdu, G. (2015). Experimental Evaluation of R448A as R404A lower-GWP alternative in refrigeration systems. Energy conver. Manag. 105, 756–762. doi:10.1016/j.enconman.2015.08.034
Page, K. L., Dang, Y. P., and Dalal, R. C. (2020). The ability of conservation agriculture to conserve soil organic carbon and the subsequent impact on soil physical, chemical, and biological properties and yield. Front. Sustain. Food Sys. 4. doi:10.3389/fsufs.2020.00031
Pope, E. C. D., Buontempo, C., and Economou, T. (2017). Quantifying how user-interaction can modify the perception of the value of climate information: a Bayesian approach. Clim. Serv. 6, 41–47. doi:10.1016/j.cliser.2017.06.006
Putelat, T., Whitmore, A. P., Senapati, N., and Semenov, M. A. (2021). Local impacts of climate change on winter wheat in Great Britain. R. Soc. Open Sci. 8, 201669. doi:10.1098/rsos.201669
Rural Payments Agency (2022). Basic payment Scheme (BPS) 2022. Available at: https://www.gov.uk/government/collections/basic-payment-scheme-bps-2022 (Accessed August 09, 2023).
Santosa, I. M. C., Sudirman, , and Waisnawa, I. S. (2018). Performance analysis of transcritical CO2 refrigeration system for supermarket application. Intl. J. GEOMATE 15, 70–75. doi:10.21660/2018.50.IJCST1
Sheehan, H., and Bentley, A. (2021). Changing times: opportunities for altering winter wheat phenology. Plants, People, Planet 3, 113–123. doi:10.1002/PPP3.10163
Shirley, R., Pope, E., Bartlett, M., Oliver, S., Quadrianto, N., Hurley, P., et al. (2020). An empirical, Bayesian approach to modelling crop yield: maize in USA. Environ. Res. Commun. 2, 025002. doi:10.1088/2515-7620/ab67f0
Son, J., Kim, H.-J., Hong, E.-C., and Kang, H.-K. (2022). Effects of stocking density on growth performance, antioxidant status, and meat quality of finisher broiler chickens under high temperature. Antioxidants 11, 871. doi:10.3390/antiox11050871
Sun, X., You, J., Xu, L., Zhou, D., Cai, H., Maynard, C. J., et al. (2021). Quality properties of chicken meatballs prepared with varying proportions of Woody Breast Meat. J. Food Sci. Technol. 59, 666–676. doi:10.1007/s13197-021-05060-1
Tsolakis, N., Srai, J. S., and Aivazidou, E. (2018). Blue water footprint management in a UK poultry supply chain under environmental regulatory constraints. Sustainability 10, 625. doi:10.3390/su10030625
Keywords: heatwave, agriculture, food, poultry, wheat, adaptation, climate change, resilience
Citation: Davie JCS, Falloon PD, Pain DLA, Sharp TJ, Housden M, Warne TC, Loosley T, Grant E, Swan J, Spincer JDG, Crocker T, Cottrell A, Pope ECD and Griffiths S (2023) 2022 UK heatwave impacts on agrifood: implications for a climate-resilient food system. Front. Environ. Sci. 11:1282284. doi: 10.3389/fenvs.2023.1282284
Received: 23 August 2023; Accepted: 01 November 2023;
Published: 15 November 2023.
Edited by:
Adam Schlosser, Massachusetts Institute of Technology, United StatesReviewed by:
Kairsty Topp, Scotland’s Rural College, United KingdomCopyright © 2023 Davie, Falloon, Pain, Sharp, Housden, Warne, Loosley, Grant, Swan, Spincer, Crocker, Cottrell, Pope and Griffiths. This is an open-access article distributed under the terms of the Creative Commons Attribution License (CC BY). The use, distribution or reproduction in other forums is permitted, provided the original author(s) and the copyright owner(s) are credited and that the original publication in this journal is cited, in accordance with accepted academic practice. No use, distribution or reproduction is permitted which does not comply with these terms.
*Correspondence: Jemma C. S. Davie, jemma.davie@metoffice.gov.uk