- 1University Rennes, CNRS, ECOBIO—UMR 6553, Rennes, France
- 2DECOD (Ecosystem Dynamics and Sustainability), INRAE, Institut Agro, IFREMER, Rennes, France
The removal of the two dams on the Sélune River since 2019 has led to profound changes in the aquatic ecosystem. Lentic habitats bordered by forest had shifted to new conditions (running water and sparsely vegetated riverbanks) therefore shaping organisms’ assemblies. We studied how the reestablishment of aquatic lotic habitats in interaction with riparian vegetation could mediate the restoration of important ecological functions in the new river. Six stations located along the river continuum were surveyed for 3 years after dam removal: two control stations upstream the former reservoir, three restored stations within the former reservoir, and one control station downstream. We monitored physico-chemical characteristics, phytobenthos biomass and the river’s benthic metabolism, and assessed the functional composition of macroinvertebrate communities. We compared the recorded variables among upstream, downstream and restored sampling stations. We observed a rapid recolonization by invertebrates, but a still low phytobenthic primary production in restored stations. Such a low primary productivity was also reflected in the functional composition of invertebrate communities. Three years after dam removal, there was still a significant time lag between communities recovery and expected ecosystem functioning restoration. We observed a quick colonization by aquatic running-water invertebrate communities of new lotic reaches, but a slower recovery of important ecological functions rates such as those observed in control stations.
1 Introduction
Dams and impoundments have been built for thousands of years for various purposes, including flood control, water supply, irrigation, recreation, navigation, and hydropower generation. However, the number of dams and reservoirs has increased markedly over the past decades, and they are potentially impacting up to 575,900 km of rivers worldwide (Lehner et al., 2011). Even if most of them are small, at least 50,000 of them are large dams higher than 15 m (Berga et al., 2006). Dams and reservoirs are among the main causes of freshwater biodiversity loss (Vörösmarty et al., 2010; Grill et al., 2019; Reid et al., 2019). Decades of research have highlighted the adverse effects of dams on the physical, chemical, and biological characteristics of rivers (Ward and Stanford, 1983; Poff et al., 1997; Rodríguez-Pérez et al., 2021). Consequently, the removal of dams has accelerated significantly in recent decades, and more than 2,000 have been removed in the United States and Europe, mainly low weirs and small dams considered obsolete or abandoned long ago (Bellmore et al., 2017; Habel et al., 2020).
Concomitantly, interest in dam removal as a means of river restoration has driven attention to important new challenges for watershed management and created opportunities for advancing the science of aquatic ecology (Hart et al., 2002; Bellmore et al., 2019). One of the main scientific challenges lies in determining the magnitude, timing, and range of the physical, chemical, and biological responses that can be expected following dam removal (Hart et al., 2002). Therefore, the removal of a dam is a tricky question, and the decision must be taken after careful examination of possible environmental consequences (Doyle et al., 2003; Noda et al., 2018; Habel et al., 2020). Given the relatively small number of studies on the effects of dam removal and the wide range of observed outcomes, the range, magnitude and trajectory of the expected ecological responses are highly uncertain. Environmental responses to dam removal depend on many factors not yet clearly addressed and are highly context dependent (Foley et al., 2017a; Bellmore et al., 2019). For example, improved understanding will require greater focus on how the responses to removal vary with the dam type, the river characteristics, and the watershed setting (Hart et al., 2002). The effects of small dam removal may be much smaller than those of large dam removal (Poff and Hart, 2002; Foley et al., 2017a), notably regarding the physical effects (Major et al., 2017) and their consequences on vegetation colonization and succession (Shafroth et al., 2016) and biological activities (Bellmore et al., 2019). Moreover, the sole responses of specific species (i.e., migratory fish) have been monitored in many studies, and the mechanisms underlying the restoration of the entire ecosystem are still poorly understood (Bellmore et al., 2017). The challenge is greater for the removal of large dams (>15 m high) presenting much larger impacted areas and bigger expected consequences and for which far fewer study cases are available than for small dams and weirs (Wieferich et al., 2021).
Current knowledge on the effects of large dam removal on sediment loss and channel morphology, fish, and riparian vegetation in the former reservoir or in the downstream section are rather limited (Wieferich et al., 2021). Upstream of the dam, a positive and rapid impact of dam removal on sediment storage and geomorphology has been showed (Wilcox et al., 2014; Randle et al., 2015) and also a positive and rapid effect on migratory fish (Bellmore et al., 2019; Duda et al., 2021), with potential indirect effects on non-migratory species (Tabor et al., 2022). The macroinvertebrate community also recovered quickly within 2 years following the removal of dams (Bellmore et al., 2019; Mahan et al., 2021). However, dam removal usually triggers the downstream movement of large amounts of sediment stored in the reservoir that typically increase turbidity, clog the substrate, decrease invertebrate density (Foley et al., 2017b; Mahan et al., 2021) and reduce autotrophic biofilm biomass and activity, at least over the short term (Bellmore et al., 2019; Atristain et al., 2023). In terms of temporal dynamics, most biological and physico-chemical components (Foley et al., 2015) respond much faster than geomorphological ones (Major et al., 2017). In large dam removal case studies, the subsequent responses of the fish and invertebrate communities take between a few months to 1–3 years after dam removal (Mahan et al., 2021; Dézerald et al., 2023), and the same is true for small dam removal (Carlson et al., 2018).
At the ecosystem level, the potential impacts on ecosystem functioning caused by the mobilization of stored sediment, nutrients, and organic matter from aquatic ecosystems and/or riparian vegetation changes are still poorly understood (Bellmore et al., 2019). This is especially true for large dams: no study had focused on the functional responses of their freshwater ecosystems, except the recent short-term study on Enobieta dam removal on biofilm in Spain (Atristain et al., 2023). Some of the potential impacts on ecosystem functioning can be deduced using known chemical, physical, and biological responses to dam removal. After the removal, the environmental conditions in the new channel shift from a lentic (lake) to a lotic (riverine) system that changes the types of organisms in the former impounded reach. Aquatic communities may also shift from pelagic-to benthic-dominated primary producers and invertebrate consumers (Bellmore et al., 2019). As a consequence, we should expect important changes of the river ecosystem functioning, notably its oxygen metabolism. The river metabolism is driven by two fundamental functional processes that regulate carbon and nutrient cycling in river systems (Tank et al., 2010): i) gross primary production (GPP) is the total fixation of inorganic C to organic C by all photoautotrophs, and ii) ecosystem respiration (ER) corresponds to the mineralization of organic C by all organisms in the ecosystem. The GPP/ER ratio can also be used to characterize heterotrophic streams (GPP/ER < 1) often supplied by external inputs of terrestrially derived organic C. Conversely, in autotrophic streams most of organic C is provided by the photosynthetic activity of primary producers. The river metabolism is highly correlated to the stream size (Vannote et al., 1980), turbidity or light (Dodds et al., 2013), or the presence of dams (Chowanski et al., 2020), so that it appears as a good indicator to follow ecosystem-level consequences of dam removal since all these parameters are affected by the removal.
To document the ecosystem responses to large dam removal, we assessed the consequences of the removal of one the Sélune River large dams (36 m high) over 3 years by following the trends in physico-chemical parameters, ecosystem functioning, and community structure shifts. We focused on benthic processes and communities—both producers and consumers—e.g., the benthos metabolism, the phytobenthos community composition, and the macroinvertebrate community trophic structure. By comparing six lotic stations located upstream, downstream and in the new running-water reach that replaced the former reservoirs, we expected (hypothesis H1) a fast homogenization of physico-chemical conditions between the control stations upstream and the stations in the new channel following restoration of water flow, except for turbidity as sediments are exported and affect this parameters downstream. Conversely, the restoration of phytobenthos and of the river metabolism should take longer in the former reservoir and downstream (hypothesis H2) because of higher water turbidity and unstable sediment on the river bottom. Finally, the trophic structure of the macroinvertebrate community should reflect the changes in the availability of feeding resources such as the increase in benthos autochthonous primary production in comparison with a higher contribution of pelagic primary production in the former reservoirs and the decreased sedimentation of fine particles (hypothesis H3).
2 Materials and methods
2.1 Study site
The Sélune River is located in Normandy (France), in a temperate oceanic climate region (Rodríguez-Pérez et al., 2021). It is a 91 km-long coastal stream (mean slope 0.3% to 0.1% in its downstream part) flowing into the Mont Saint Michel Bay after draining a watershed of 1,106 km2 mainly composed of schists and metamorphic rocks. The Sélune River basin lies in a patchy landscape made of forests, pastures, and traditional extensive livestock farming with very few crop areas. The watershed’s climate is oceanic, with a low temperature amplitude and rainfall distributed throughout the year with highest precipitation in December and lowest in July. The Sélune River floods regularly in winter and spring (Fovet et al., 2023). Until recently, the water flow and chemistry of the Sélune River had been profoundly impacted over a 17 km-long section (Fovet et al., 2020; Rodríguez-Pérez et al., 2021) by two large hydropower dams built in 1919 and 1932, respectively and separated by a very short (<1 km) free-flowing section. The amount of fine sediments stored in the 2 dam reservoirs was estimated as 1,800,000 m3 (IDRA, 2012). A small part of the reservoir was contaminated with heavy metals (Andrade et al., 2020), but these sediments were dredged and safely stored outside the riverbed before the removal. The most upstream dam—Vezins Dam (36 m height)—was removed in 2019 after 1 year of regular decrease of the water level in the reservoir, whereas the second dam—Roche-qui-Boit Dam (16 m height)—was completely removed in 2022 (see Dézerald et al., 2023 for details). To keep the massive amount of sediments stored in reservoirs from clogging the downstream section of the river, much of it was dredged and stored in ponds built with gabions and dykes made with local sediments (Berrée, 2019). After the sediments had dried, the gabions were removed.
Six sampling stations were chosen according to their relative positions to the dams (Figure 1). S1u (48°35′56.5″N 0°57′28.5″W) and S2u (48°34′18.0″N 1°06′58.4″W) were located upstream of the two reservoirs, had never been impacted by the dams except for the absence of migratory anadromous fishes, and were used as control stations. These two stations presented slightly anthropized conditions (pasture) but altogether natural river banks with typical riparian tree communities along the rivers of this region (Ravot et al., 2020). S3r (48° 33′ 53.9″N, 1° 09′ 2″W), S4r (48°34′22.1″N 1°10′51.9″W) and S5r (48°34′36.2″N 1°13′11.5″W) were located in the new channel after the recovery of the natural flow regime since 2019 (Figure 2). Finally, S6d was located 4 km downstream of the second dam (48° 35′ 44.9″N, 1° 17′ 35.9″W). The water depth was 80–100 cm maximum and the river was fully wadeable in all stations.
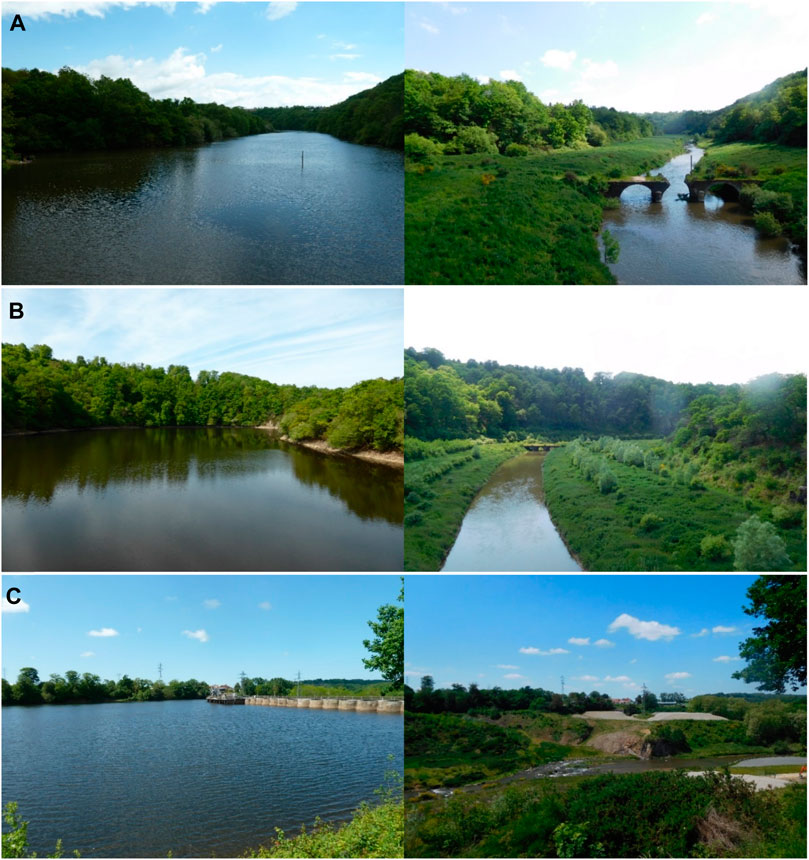
FIGURE 2. Pictures of the stations [(A): S3r; (B) S4r; (C) S5r] in the Vezins Reservoir before (left side; May 2015) and after (right side; May 2023) the dam removal. Photograph credits of “Observatoire photographique des paysages de la Sélune—Université Paris Nanterre et SMBS”.
2.2 Measurement of functional restoration
2.2.1 Physicochemical parameters
Water chemistry was recorded seasonally (four seasons per year) at each station from June 2020 to December 2022. Sampling frequency didn’t allow to highlight short events (e.g., turbidity peak), but this monitoring programme was enough to compare the values between stations. Measurements were always carried out after stabilised water flows (i.e., at least 1 week) and almost simultaneously (time lag of more or less 2 h between S1u and S6d). Water temperature, conductivity, the pH, the dissolved oxygen content (WTW 3320, WTW, Weilheim, Germany) and turbidity (Turb 430T, WTW, Weilheim, Germany) were measured in the field. Filtered-water samples (Syringe filter 0.45 µm, Sarstedt, Nümbrecht, Germany) were analyzed by colorimetry methods for soluble reactive phosphorus, ammonium and nitrate using laboratory test kits for spectrophotometer (PhotoLab 7100 VIS WTW, Weilheim, Germany).
2.2.2 Phytobenthos composition and biomass
The biomass of chlorophyll a in the biofilm (i.e., green algae, diatoms and cyanobacteria), naturally growing on river’s hard substrate, was estimated at each station by the end of June 2020, 2021 and 2022 using a BBE Benthotorch fluorescence probe (bbe moldaenke GmbH, Germany). Twenty-eight to thirty-four measurements were taken randomly at each station upon similarly sized pebbles and boulders.
2.2.3 Benthic metabolism
The benthic metabolism of the river bed was estimated in all stations in spring (mid-May) and summer (end of July) 2022, except at S5r and S6d where the use of equipment and measuring tools was not possible due to high turbidity and unstable sediment on the stream bottom in relation to the breaching of the second dam in June 2022. The metabolism was estimated using optically clear acrylic benthic chambers and dark benthic chambers for estimating benthic fluxes of CO2 (pH values in this section of the river remained stable) (Colas et al., 2021). The 20-cm diameter chambers were buried around 10 cm inside the sediment to reach a volume of around 3 L for the measurement of gas exchanges. The chambers were equipped at the top with a HOBO Temperature/Light sensor (UA-002) data logger, and a hand-held mixer inserted through cable glands. The mixer was used for water homogenization inside the chamber just before water sampling. A PVC tube (6 and 4 mm outer and inner diameters, respectively) with a valve inserted through a cable gland placed at the top of the chamber allowed the water to drain into the chamber during immersion and avoided air bubbles getting into the chamber. The PVC tube also allowed water to get inside the chamber during water sampling in the middle of the chamber at around 10 cm above the sediment. Deployments usually started between 10:30 and 11:00 a.m. by lowering the chamber into the sediment and fixing it using two threaded rod bars (12-mm diameter) sank 40 cm into the sediment and large wing nuts to maintain it in the sediment. Five pairs of dark and clear chambers were placed on soft gravel sediment at each station, at less than 1 m depth and 1-2 m from the bank to avoid shaded areas and vegetation patches. Incubation lasted around 5 h, during which temperature and light were recorded every 10 min to take into account frequent local variations in oceanic weather conditions. At the beginning and at the end of the incubation, 100-mL water samples were collected using 200-mL syringes with three-way stopcocks connected to the end of the PVC tubing equipped with a Luer-lock syringe valve. We created a 100-mL headspace in the syringes with ambient air for each water sample, and then the sample was shaken for 2 minutes to homogenize the concentration of dissolved and air gases. Then, the headspace was stored in Tedlar bags at room temperature (≅ 20°C) for less than 24 h. For each station, additional samples of air were taken at the beginning and at the end of the incubation. As photosynthetic activity was very low at the bottom of the river, the variation in dissolved O2 was small and the probes were not sensitive enough to detect a small variation over only 5 h of incubation and our measurement protocol did not allow us to obtain robust values for O2. Consequently, we preferred to use river metabolism using only dissolved CO2 concentrations. The CO2 concentrations were finally measured by GC-MS (microGC 3000, Agilent Technologies, Inc., Santa Clara, United States).
The CO2 concentration (C, mmol) in the chamber was calculated as follows (Eq. 1):
where Vwater is the volume (mL) of the sample in the syringe and Vatm is the volume of the headspace, Catm and Cwater are the CO2 concentrations (ppm) measured with the micro-GC in the atmosphere and in the water, and β is the Bunsen coefficient of dissolution (L/Latm) computed as follows (Eq. 2):
where a1 = −58.0931; a2 = 90.5069; a3 = 22.294 and b1 = 0.0278; b2 = −0.02589; b3 = 0.00506 are constants for the Bunsen calculation (Weiss, 1970; 1974), T is temperature (K) and S salinity (‰).
The flux of CO2 in chamber (FCO2, mmol.m2.day−1) was calculated as follows (Eq. 3):
where C0 is the CO2 concentration at the beginning of incubation and Ct the concentration at the end, ti is the duration of incubation (h), Vc the volume of the chamber (L) and Sc the surface area of the sediment enclosed in the chamber (m2).
We used FCO2 in dark chambers as a proxy of the ER of the benthos, and the difference between FCO2 in the clear and dark chambers as a proxy of the Net Primary Production (NPP). Contrary to the classical approach of river metabolism with O2, with FCO2 we could not measure the GPP as FCO2 is the result of the photosynthetic activity which consumed the CO2 and the respiration of the benthos that produces CO2. However we could use the NPP as a proxy of the intensity of the net ecosystem production of CO2.
Finally, by using a YSI EXO2 multiparameter sonde (YSI, Yellow Springs, OH, United States) the following parameters were recorded every 10 s during the incubations: temperature (°C), electrical conductivity (µS.cm−1 corrected to 25°C), dissolved oxygen (% and mg.L−1), turbidity (NTU), pH, total algae (chlorophyll + phycocyanin and phycoerythrin, RFU), fluorescent dissolved organic matter (fDOM, QSU), and water depth (m). These measures taken in the water surrounding chambers allowed to record any brief events occurring during the incubations.
2.2.4 Macroinvertebrate sampling
Benthic macroinvertebrates were sampled using a Surber net sampler (0.05 m2, 0.5 mm mesh size) in May 2020 and 2022. Six stratified samples were collected at each date and station in order to cover a similar range of available habitats (cobble, sand-sediment, macrophytes, leaf litter and debris). The samples were immediately fixed with 96° ethanol and stored until sorting under a binocular microscope. Taxa were identified down to the species or genus levels (most crustaceans and Insect larvae), except Nematoda, Hydracarina (not identified further), and most of Diptera (identified down to the family/tribe levels).
We assigned trait values to each invertebrate taxon and each modality according to Tachet et al. (2010) and the freshwaterecology. info database (Schmidt-Kloiber and Hering, 2015). Whenever the taxonomic resolution of our list and that of the databases did not match, we used the next upper taxonomic resolution. Only the feeding habits were retained according to their potential sensitivity to changes in feeding resource availability (light, riparian vegetation) after dam removal. The percentage of each feeding group in the macroinvertebrate community was computed by multiplying the affinity scores of each invertebrate taxon for each feeding group by the abundance.
We also computed the abundances of scrapers/(shredders + collectors) ratio (Ra/h). This ratio highlights the balance between autotrophy and heterotrophy (Merritt et al., 2017). The ratio increases when invertebrates using autotrophic resources (i.e., scrapers) dominate the community in comparison with invertebrates using heterotrophic resources (shredders and collectors).
2.3 Statistical analysis
The seasonal physico-chemical datasets collected from 2020 to 2022 were used to test for differences in water quality between the control (S1u and S2u), restored (S3r, S4r, and S5r) and downstream (S6d) stations using PERMANOVA (Anderson and Walsh, 2013). The data were normalized before generating a similarity matrix (Euclidian distance similarities) to test the factors “station” and “date” with Monte-Carlo tests. We also conducted a similarity percentage analysis (SIMPER) to determine which water quality parameters changed according to the station type (control vs. restored and restored vs. downstream). PERMANOVA and SIMPER analyses were performed using PRIMER 7 software (PRIMER-E, Plymouth, United Kingdom).
Between-station differences in the phytobenthos biomass and benthic flux of CO2 (FCO2) were compared statistically using one-way ANOVAs per date and per type of chamber (dark vs. clear) with “station” as a fixed factor. For the macroinvertebrate feeding groups, we used two-way ANOVAs on square-rooted data for normalization, with “station” and “year” as fixed factors. For all tests, pairwise comparisons were performed using Tukey’s HSD tests. All analyses were carried out using Statistica 7 software (StatSoft).
3 Results
3.1 Physicochemical parameters
Physico-chemical parameters varied between stations along the upstream-downstream gradient and across years (see Supplementary File S1 for annual data). Briefly, we observed an increase in temperature up to 2.6°C and in a lower extend an increase in pH (up to 0.5 UI), conductivity (up to 72 μS.cm−2) and NO3 (up to14.3 mg.L−1), except for turbidity and PO43− which increased from upstream to downstream but decreased at Sd6.
The PERMANOVA on the physico-chemical dataset showed a highly significant “station” effect (Pseudo-F = 2.84; p = 0.001) but a weak “sampling date” effect (Pseudo-F = 2.07; p = 0.047), and no interaction between “station” and “date” (Pseudo-F = 0.23; p = 1.0). Pairwise comparisons highlighted that the “station” effect only concerned S1u, which significantly differed from all other stations (p values <0.02), whereas the other stations did not differ significantly (p values >0.175). A marginal effect of “date” was found between 2021 and 2022 (p = 0.042). SIMPER analyses showed that multiple water quality parameters contributed to the change in overall conditions upstream and downstream (Table 1). Increases in turbidity, nitrate, conductivity, and pH accounted for 60.7% of the dissimilarity in water quality conditions between the two control and the three restored stations. In the downstream station, temperature increased but the pH, turbidity and reactive phosphorus decreased and accounted for 67.7% of the dissimilarity in water quality between the restored (S3r, S4r, and S5r) and the downstream (S6d) stations.
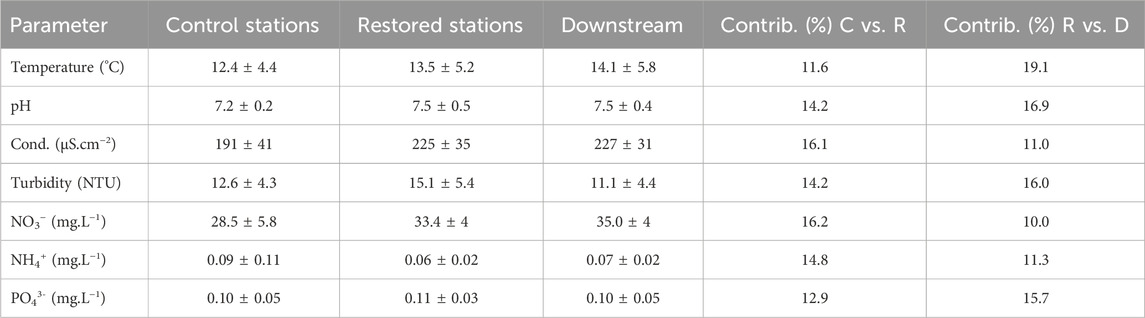
TABLE 1. Mean values (±SD) and results of the similarity percentage (SIMPER) analysis of water quality (normalized values) after the dam removal, and percent contribution to compositional dissimilarity between the control (C: S1u and S2u) and restored (R: S3r, S4r, and S5r) stations and between the restored and downstream (D: S6d) stations.
3.2 Phytobenthos composition and biomass
Whatever the station, the phytobenthos community was composed of a majority of diatoms (mean value 50.0% ± 15% of total biomass) and cyanobacteria (mean value 48.0% ± 16% of total biomass). Green algae represented only a very small share of the phytobenthos (1.0% ± 6% of total biomass). Green algae were detectable in only 4% of the samples, exclusively in summer. Consequently, this group was discarded for further analyses. The biomasses of cyanobacteria and diatoms strongly varied between years (Figures 3A, B; p-values <0.0001), but the overall trends among stations remained similar over time (Figure 3C), except for diatoms in S4r in 2020. S3r and S5r hosted a lower phytobenthic biomass than S1u and S2u (Figure 3C). However, the difference was greater in 2020, especially for diatoms, and was highly reduced in 2021 and 2022, except for in S6d where the biomass of both diatoms (Figure 3A) and cyanobacteria (Figure 3B) strongly declined in 2021 and 2022.
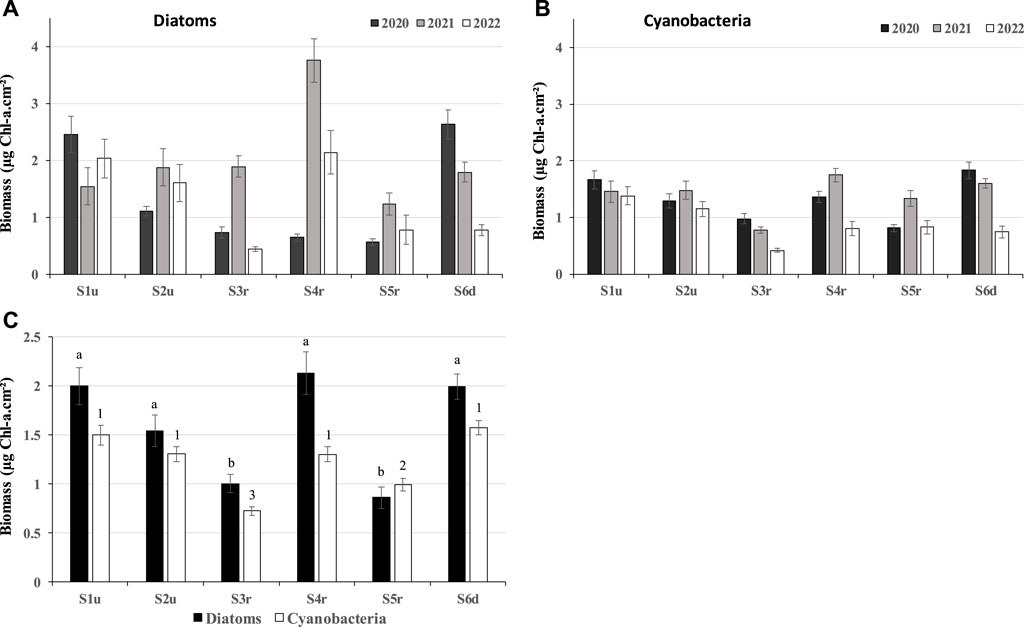
FIGURE 3. Mean chlorophyll biomass values ± SD measured in the summer 2020, 2021 and 2022 at each station for diatoms (A) and cyanobacteria (B) and mean values ± SD per station (C). Small letters (for diatoms) and numbers (for cyanobacteria) indicate significant differences between stations.
3.3 Benthic metabolism
Benthic gas fluxes of CO2 were successfully estimated in the five stations in spring, and only in four stations in July because data from Sr5 were not reliable in summer (Figure 4). Fluxes ranged from −0.08–1.27 g CO2. m−2. d−1 for FCO2. In most cases, a net benthic primary production was recorded in the clear chambers (CO2 consumption), except at S2u in spring and at S4r in summer that exhibited benthic fluxes suggesting a higher sediment respiration.
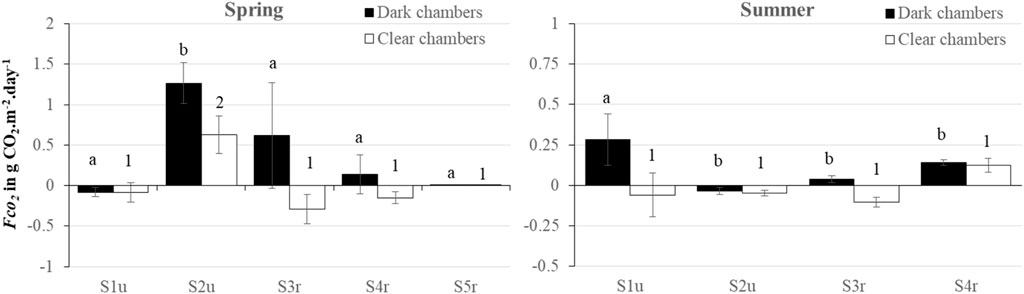
FIGURE 4. Mean values ± SE of benthic flux of CO2 measured in dark chambers (black bars) and clear chambers (white bars) in spring and summer 2022. Small letters (for dark chambers) and numbers (for white bars) indicate significant differences between stations.
The ER of the benthos tended to be lower in the restored stations in spring and in control and restored stations in summer, but the difference were not significant (Fisher exact t-test, p values >0.210). Conversely, GPP was similar in all stations in spring (p = 0.392), but decreased significantly in the restored stations in summer (Table 2, p = 0.029). The GPP/ER ratio (Table 2) increased significantly in restored stations compared with control stations in spring (p = 0.026) and tended to be higher also in summer, even if the difference was not significant in summer (p = 0.472).
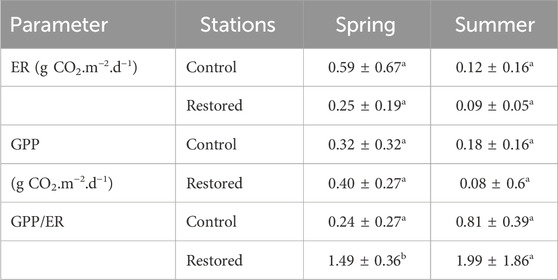
TABLE 2. Mean values (±SE) of ecosystem respiration (ER) and gross primary production (GPP) in the control (S1u and S2u) and restored (S3r, S4r, and S5r) stations in spring and summer 2022. Small letters indicate results of Fisher exact t-tests between stations and seasons for each indicator.
3.4 Macroinvertebrate feeding groups
The proportion of most of the different feeding groups (Figure 5) varied significantly across stations (p values <0.049) and years (p values <0.006), except for deposit feeders that were similar among stations (p = 0.093), and shredders that remained stable across stations and years (Figure 5B, p = 0.283 and p = 0.490, respectively).
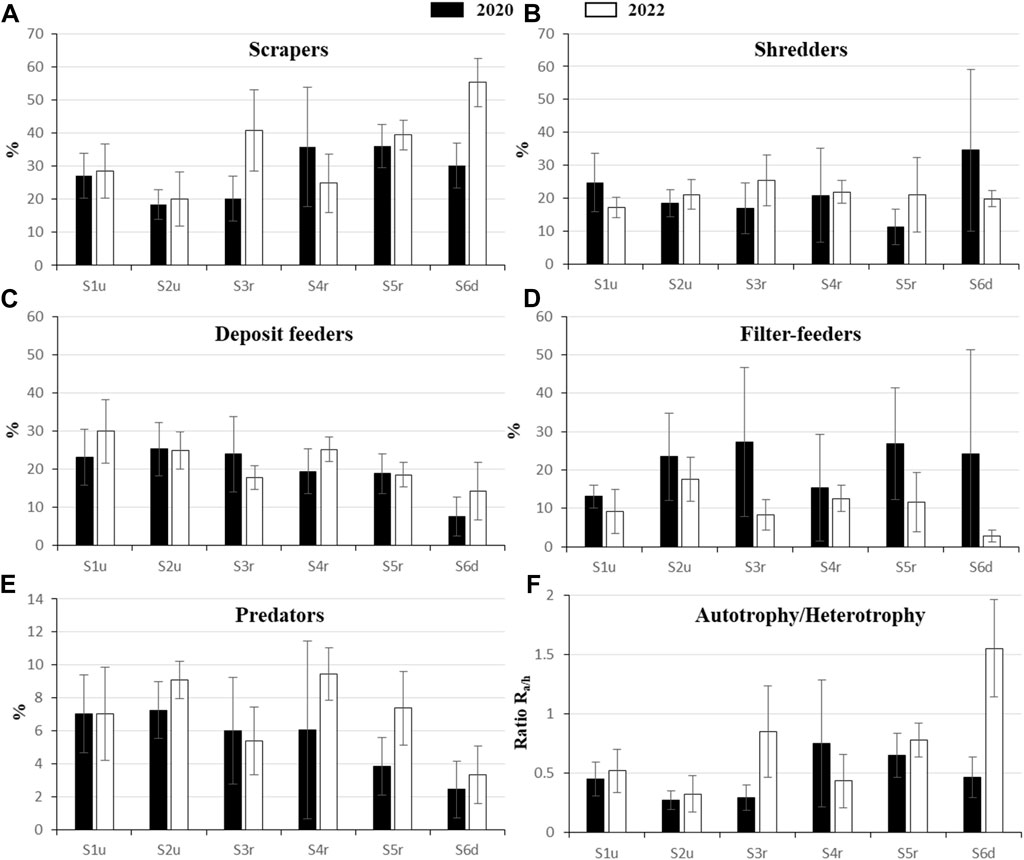
FIGURE 5. Mean percentage values (±SD) of the main feeding groups in the macroinvertebrate community in 2020 (dark bars) and 2022 (white bars): (A), scrapers; (B), shredders; (C), deposit feeders; (D), filter feeders; (E), predators; (F), Ra/h ratio.
As for the feeding groups, the Ra/h ratio also varied spatially and temporally (p values <0.001). There was not significant interaction between “station” and “year” for the feeding groups (p values >0.077), except for scrapers (p < 0.001) and the Ra/h (p < 0.001) ratio. The proportion of scrapers increased toward the downstream station to become the dominant feeding group (Figure 5A), whereas deposit-feeders (Figure 5C) and predators (Figure 5E) decreased toward the downstream station. The proportion of filter-feeders (Figure 5D) remained stable across stations and years except in D where they significantly dropped down in 2022. As for scrapers, their Ra/h ratio also increased in the restored (S3r, S4r, and S5r) and downstream (S6d) stations (Figure 5F).
4 Discussion
The ecosystem of the Sélune River was strongly modified by the erection of two successive large dams between 1919 and 1932. For almost one century, deep changes occurred in this ecosystem and shaped plankton and macroinvertebrate communities along half of the main stem (Rodríguez-Pérez et al., 2021) and also induced a major discontinuity in the natural process of C flow in aquatic food webs along the river by promoting carbon sequestration (Roussel et al., 2024). The two dams strongly altered the physical, chemical and biological continuity of the river, but their vicinity was not sufficient to create differential impacts: their impact is as that of a single artificial water mass (Rodríguez-Pérez et al., 2021). Overall, our results show that the removal of dams leads to a relatively fast homogenization of habitats and environmental conditions within the former reservoirs, but also important changes downstream since the removal.
4.1 Consequences of dam removal on physico-chemical conditions
In 2021, only 1 year after the removal of Vezins Dam and the decommissioning of Roche-qui-Boit Dam (i.e., the reservoir has been emptied in 2021 before its removal in 2022), only subtle changes in water quality were noticed downstream between the control, restored and downstream stations. This result confirms a very fast restoration of water flows and homogenization of chemical factors following geomorphological restoration, except for turbidity (Fovet et al., 2023) and temperature (hypothesis H1), as observed in previous restorations of large dams (Foley et al., 2015; Atristain et al., 2023) and as expected in conceptual models (Bellmore et al., 2019). Before the dam on the Sélune River was removed, reservoirs were characterized by higher temperature, higher conductivity, a higher total nitrogen concentration but a lower turbidity and lower phosphorus concentrations than in running-water reaches (Rodríguez-Pérez et al., 2021). These differences disappeared for most of the parameters, except temperature and turbidity. Turbidity tended to increase in the restored stations in comparison with the control (S3r, S4r, and S5r) and downstream (S6d) stations, likely explained by the destocking of fine sediment (Wilcox et al., 2014; Bellmore et al., 2019) that was partly deposited downstream in the second reservoir of the decommissioning dam in 2021 and before reaching the downstream station and subsequently the ocean after the removal of the second dam in 2022. This result is corroborated by the continuous monitoring of physico-chemical parameters and sediment transfer from 2017 to 2022 between the upstream part of the reservoir of the former Vezins Dam (between S2u and S3r) and the downstream station S6d (Fovet et al., 2023). Temperature slightly increased between the upstream control stations and downstream stations, probably as a consequence of the lack of riparian vegetation and the reduced the canopy cover in the restored stations (Figure 1) that added heat to the river reach (Webb and Zhang, 2004).
4.2 Phytobenthos and river metabolism restoration
The characteristics of the geomorphic and aquatic habitats changed rapidly after sediments started eroding from the former reservoirs (Fovet et al., 2023). One year after the reservoir emptying (in 2020), the proportion between the two dominant groups in phytobenthos, diatoms and cyanobacteria, remained similar across all stations. However, their biomass was twice as low at the restored sites, especially for diatoms, and had only partly recovered after 2 years (as expected in hypothesis H2). Contrary to restored stations, the biomass of phytobenthos decreased in 2021 and 2022 in the downstream station S6d. The reduction of phytobenthos biomass is likely related to an increase in turbidity and fine sediment concentration following the release of fine sediments into the water column after the dam removal (Fovet et al., 2023). Reduced light associated with increased turbidity is indeed a well-known consequence of dam removal (Bellmore et al., 2019). Sediment release can be very fast in large dams, and most of the stored sediment can be removed only a few months after dam removal (Wilcox et al., 2014; Foley et al., 2015). However, this process occurred throughout the whole study period in the Sélune River, even if it decreased since 2021 (Fovet et al., 2023). This result is mainly due to a long emptying process in several steps, consisting in a high reduction of the water level in the reservoir since 2018 by opening the bottom gates for operations on sediments and bank stabilization before breaching the dam in 2019. The method was selected to avoid a high amount of sediment release in the water downstream in order to protect the Mont St Michel Bay since the Sélune River is one of its main tributaries. This methodological choice diluted the sediment release over time and may explain the lower extent of the increase in turbidity and its persistence over time in the restored stations. The removal process also explains the response in the downstream station S6d since part of the sediment is being retained in the river before reaching the station S6d. The similar phytobenthos biomass observed in control and downstream stations in 2020 and 2021 may be explained by the presence of the Roche-qui-Boit dam, which trapped the sediment released after the Vezins dam breaching. These trapped sediments were then progressively released into the Mont St Michel Bay over time. The quantity of sediments released to the station downstream the former reservoirs was lower in 2020 and 2021, hence lower turbidity and the low impact of dam removal on the phytobenthos during the first 2 years after removal of Vezins Dam. However, the complete removal of the second dam in June 2022 (after its decommissioning in 2021) released a lot of sediment downstream (Fovet et al., 2023), which could have had a significant impact on sediment colonization by phytobenthos this year.
Sediment release does not only affect turbidity, but also sediment stability—a key parameter to allow the colonization by photosynthetic biofilm (Biggs, 1996). Sediment instability in the restored stations likely explains the specific response of station S4r (shows ER = NPP in spring and ER > NPP in summer suggesting biofilms are lacking photosynthetic organisms and likely dominated by non-photosynthetic organisms. S4r was indeed located in a 300-m linear and homogenous stretch with a laminar flow (personal observation) providing more stable conditions for phytobenthos growth (Hondzo and Wang, 2002).
The metabolism of the Sélune River was still weakly but significantly altered in the restored stations as regards phytobenthic biomass 2 years after dam removal (hypothesis H2). The change was mainly driven by ER, which decreased in the restored and control stations both in spring and in summer whereas NPP remained more stable. The spring increase in ER in restored station when discharge was highest suggests that the overall metabolism of the Sélune River may be mainly driven by the seasonal availability of allochthonous C and to a lesser extent by autochthonous processes and light attenuation in the water column (Dodds et al., 2013). The lower ER rates in the restored stations compared with the control stations suggest that a significant proportion of the carbon stored in the fine sediments of the former reservoir may be removed by sediment leaching and may not contribute significantly to sediment respiration. The low contribution of primary producers measured in our study is also partly explained by the location of benthic chambers outside macrophyte beds to avoid their potential impact on the river metabolism (Kupilas et al., 2016) and to focus only on the effect of dam removal on sediment metabolism. The contribution of autotrophy to the river metabolism tends to increase slightly in the restored stations but only in spring. However, the GPP/ER ratio recorded during our short-term incubations increase highly in restored stations both in spring and in summer. The difference observed in the restored stations could result from the increase in available light and a warmer temperature due to the lack of riparian vegetation in the restored stations (Webb and Zhang, 2004).
4.3 Functional composition of the macroinvertebrate community
Dam removal strongly modified the availability of the algal primary feeding resources of macroinvertebrates with strong consequences on the relative contribution of the functional feeding groups in the restored (S3r, S4r, S5r) and downstream (S6r) stations since it is the main primary resources available for macroinvertebrates at this part of the Sélune River (personal observation). These effects were still observed 3 years after removal. As for the river metabolism, the contribution of autotrophy increased in the restored stations, mainly driven by the increased abundance of scrapers whereas the abundance of shredders remained stable and the abundance of deposit feeders tended to decrease in the station downstream of the former reservoirs. This result is congruent with our third hypothesis (H3), i.e., a change in the trophic structure of the macroinvertebrate community is expected following changes in the availability of feeding resources. Dam removal, especially for large dams, deeply changes the interactions between riparian and aquatic ecosystems by reducing the abundance of riparian trees (Ravot et al., 2020). As a consequence, we suspect reduced terrestrial inputs into the Sélune River and a higher contribution of autotrophy in the system. An increased percentage of scrapers was observed in restored channels during the first 2 years after the removal of a small dam (Pollard and Reed, 2004; Poulos et al., 2019), but also in woodland harvesting with clear-cutting of riparian trees (Thompson et al., 2009) or agricultural practices (Piscart et al., 2009; 2011). Moreover, Tonin et al. (2017) suggested that variability in decomposition rates between stream reaches was primarily explained by variation in local canopy cover rather than environmental conditions at the catchment scale, indicating the importance of local leaf inputs. Contrary to the percentage of scrapers, the percentage of deposit feeders tended to decrease in the restored channel. Previous studies on small dam removal have highlighted this kind of change (Sullivan and Manning, 2017; Sullivan et al., 2018; Poulos et al., 2019). For large dam removal, the result of the only study available was opposite (Mahan et al., 2021). However, in that case, the increased percentage of deposit feeders was mainly explained by the greater abundance of Chironomidae larvae in the restored channel than in the control reaches, and the percentage of predators declined downstream of the control stations, as the percentage of deposit feeders did. Previous studies have highlighted more idiosyncratic responses with contradictory results making the pattern of predators less predictable and likely site-dependent. In our study, the decreased percentage of predators could be related to an increase in size of predators since predators are mainly represented by small dipterans in control stations and by larger Rhyacophyla sp. larvae in restored and downstream stations. The percentage of shredders remained stable across all stations, whereas the absence of riparian trees in the restored stations might lead to a decreased percentage of shredders in the community. However and surprisingly, the percentage of shredders often remains stable following small or large dam removal (Sullivan et al., 2018; Mahan et al., 2021). This suggests a site effect: for instance, Amphipoda are the dominant shredders in the Sélune River and they are indeed omnivores (Macneil et al., 1997) with opportunistic feeding behavior (Maazouzi et al., 2009; Pellan et al., 2016). A complementary hypothesis, could be the availability of leaf litter material transported longitudinally over a few kilometres from the upstream part of the river and/or laterally over 30–50 m from older riparian trees.
The other parameters (phytobenthos, physico-chemistry) showed that the macroinvertebrate community in the downstream station responded more or less in the same way as in the restored stations, with a higher contribution of autotrophy associated to an increased percentage of scrapers and a decreased percentage of deposit-feeders and predators. Sediment release downstream seems to have had a limited impact on the functional feeding groups even 1 year after removal in 2020. In 2022, the downstream station reacted differently than the other stations, with a very strong increase in the percentage of scrapers and a strong decline in the percentage of filter-feeders, hence a high increase of the autotrophy/heterotrophy ratio. The change in trajectory observed in 2022 may likely be linked to lower turbidity in the station that year.
4.4 Conclusion
Our study shows that, although macroinvertebrate biodiversity recovered rapidly, the ongoing restoration of ecosystem functional responses to the removal of a large dam is still detectable 3 years after the removal. Even if physico-chemical variables reacted quickly, the continuous release of fine sediment and the instability of habitats lead to unfavorable conditions for primary producers. The modification of terrestrial/aquatic linkage inputs of terrestrial carbon and the increase in water temperature in restored stations devoid of riparian trees may also have altered the metabolism of the Sélune River by promoting photoautotrophs. Changes in feeding resources both in the benthic (biofilm, fine sediment) and pelagic (suspended organic matter) areas also impacted the functional groups of macroinvertebrates even after 3 years. These impacts are congruent with impacts observed in small dam removal studies. However, the impact of large dam removal on the riparian area and the huge quantity of sediment trapped in rivers may have functional impacts in the former reservoir and downstream over decades, the time required for trees to grow and stabilize the geomorphology of the river. Finally, our study highlights potential site-specific responses (size of the river, geomorphology, land cover). Delays in restoring the productivity of the restored ecosystems should not affect the recolonization of migratory fishes, since they only have an impact on the restored station of the former reservoirs and the station located downstream. Further studies and long-term monitoring are required to fully understand the functional consequences of large dam removal at the ecosystem level.
Data availability statement
The raw data supporting the conclusion of this article will be made available by the authors, without undue reservation.
Ethics statement
The manuscript presents research on animals that do not require ethical approval for their study.
Author contributions
CP: Writing–original draft, Writing–review and editing. OD: Writing–review and editing. LP: Writing–review and editing. NL: Writing–review and editing. HR-P: Writing–review and editing. TB: Writing–review and editing. DH: Writing–review and editing. J-MR: Writing–review and editing.
Funding
The author(s) declare financial support was received for the research, authorship, and/or publication of this article. This study was funded by the Water Agency (Agence de l’Eau Seine Normandie) under contracts to CNRS (AESN-CNRS 10533864-1 and 1085108-2019) and INRAE (AESN-INRA 1050492).
Acknowledgments
We thank Justine Leruste and Iris Pouliquen for all the help with the fieldwork in the different campaigns. An extra thank to members of the analytical platform EcoChim of the laboratory ECOBIO and Eliot Chatton from the GEOSCIENCES Rennes laboratory, who advised us on the gas analyses.
Conflict of interest
The authors declare that the research was conducted in the absence of any commercial or financial relationships that could be construed as a potential conflict of interest.
Publisher’s note
All claims expressed in this article are solely those of the authors and do not necessarily represent those of their affiliated organizations, or those of the publisher, the editors and the reviewers. Any product that may be evaluated in this article, or claim that may be made by its manufacturer, is not guaranteed or endorsed by the publisher.
Supplementary material
The Supplementary Material for this article can be found online at: https://www.frontiersin.org/articles/10.3389/fenvs.2024.1250810/full#supplementary-material
References
Anderson, M. J., and Walsh, D. C. I. (2013). PERMANOVA, ANOSIM, and the Mantel test in the face of heterogeneous dispersions: what null hypothesis are you testing? Ecol. Monogr. 83, 557–574. doi:10.1890/12-2010.1
Andrade, V. S., Wiegand, C., Pannard, A., Gagneten, A. M., Pédrot, M., Bouhnik-Le Coz, M., et al. (2020). How can interspecific interactions in freshwater benthic macroinvertebrates modify trace element availability from sediment? Chemosphere 254, 125594. doi:10.1016/j.chemosphere.2019.125594
Atristain, M., von Schiller, D., Larrañaga, A., and Elosegi, A. (2023). Short-term effects of a large dam decommissioning on biofilm structure and functioning. Restor. Ecol. 31, e13779. doi:10.1111/rec.13779
Bellmore, J. R., Duda, J. J., Craig, L. S., Greene, S. L., Torgersen, C. E., Collins, M. J., et al. (2017). Status and trends of dam removal research in the United States. WIREs Water 4, e1164. doi:10.1002/wat2.1164
Bellmore, J. R., Pess, G. R., Duda, J. J., O’Connor, J. E., East, A. E., Foley, M. M., et al. (2019). Conceptualizing ecological responses to dam removal: if you remove it, what’s to come? BioScience 69, 26–39. doi:10.1093/biosci/biy152
L. Berga, J. M. Buil, J. C. Bofill, J. C. De Cea, J. A. Garcia Perez, G. Manuecoet al. (2006). Dams and reservoirs, societies and environment in the 21st century, two volume set: proceedings of the international symposium on dams in the societies of the 21st century, 22nd international congress on large dams (ICOLD), barcelona, Spain, 18 June 2006 (London: CRC Press). doi:10.1201/b16818
Berrée, G. (2019). Selune dam removals operation. European Rivers Network international conference on dam removal: selune valley revival Rennes. Available at: https://www.rivernet.org/general/dams/decommissioning_fr_hors_poutes/pdf/2431GBERREE_OperationEffacementsBarragesSelune_colloqueSELUNE2019_bd.pdf.
Biggs, B. J. F. (1996). “2 - patterns in benthic algae of streams,” in Algal ecology aquatic ecology. Editors R. J. Stevenson, M. L. Bothwell, and R. L. Lowe (San Diego: Academic Press), 31–56. doi:10.1016/B978-012668450-6/50031-X
Carlson, P. E., Donadi, S., and Sandin, L. (2018). Responses of macroinvertebrate communities to small dam removals: implications for bioassessment and restoration. J. Appl. Ecol. 55, 1896–1907. doi:10.1111/1365-2664.13102
Chowanski, K., Kunza, L., Hoffman, G., Genzoli, L., and Stickney, E. (2020). River management alters ecosystem metabolism in a large oligotrophic river. Freshw. Sci. 39, 534–548. doi:10.1086/710082
Colas, F., Baudoin, J.-M., Bonin, P., Cabrol, L., Daufresne, M., Lassus, R., et al. (2021). Ecosystem maturity modulates greenhouse gases fluxes from artificial lakes. Sci. Total Environ. 760, 144046. doi:10.1016/j.scitotenv.2020.144046
Dézerald, O., Roussel, J. M., Lanoë, E., Beauverger, T., Bazin, A., Rodríguez-Pérez, H., et al. (2023). Fast but transient recovery of aquatic and terrestrial communities after a large dam removal. Front. Ecol. Evol. 11, 1254462. doi:10.3389/fevo.2023.1254462
Dodds, W. K., Veach, A. M., Ruffing, C. M., Larson, D. M., Fischer, J. L., and Costigan, K. H. (2013). Abiotic controls and temporal variability of river metabolism: multiyear analyses of Mississippi and Chattahoochee River data. Freshw. Sci. 32, 1073–1087. doi:10.1899/13-018.1
Doyle, M. W., Harbor, J. M., and Stanley, E. H. (2003). Toward policies and decision-making for dam removal. Environ. Manag. 31, 0453–0465. doi:10.1007/s00267-002-2819-z
Duda, J. J., Hoy, M. S., Chase, D. M., Pess, G. R., Brenkman, S. J., McHenry, M. M., et al. (2021). Environmental DNA is an effective tool to track recolonizing migratory fish following large-scale dam removal. Environ. DNA 3, 121–141. doi:10.1002/edn3.134
Foley, M. M., Bellmore, J. R., O’Connor, J. E., Duda, J. J., East, A. E., Grant, G. E., et al. (2017a). Dam removal: listening in. Water Resour. Res. 53, 5229–5246. doi:10.1002/2017WR020457
Foley, M. M., Duda, J. J., Beirne, M. M., Paradis, R., Ritchie, A., and Warrick, J. A. (2015). Rapid water quality change in the Elwha River estuary complex during dam removal. Limnol. Oceanogr. 60, 1719–1732. doi:10.1002/lno.10129
Foley, M. M., Warrick, J. A., Ritchie, A., Stevens, A. W., Shafroth, P. B., Duda, J. J., et al. (2017b). Coastal habitat and biological community response to dam removal on the Elwha River. Ecol. Monogr. 87, 552–577. doi:10.1002/ecm.1268
Fovet, O., Meric, F., Crave, A., Cador, J.-M., and Rollet, A.-J. (2023). Early assessment of effects of dam removal on abiotic fluxes of the Selune River, France. Front. Environ. Sci. 11, 1231721. doi:10.3389/fenvs.2023.1231721
Fovet, O., Ndom, M., Crave, A., and Pannard, A. (2020). Influence of dams on river water-quality signatures at event and seasonal scales: the Sélune River (France) case study. River Res. Appl. 36, 1267–1278. doi:10.1002/rra.3618
Grill, G., Lehner, B., Thieme, M., Geenen, B., Tickner, D., Antonelli, F., et al. (2019). Mapping the world’s free-flowing rivers. Nature 569, 215–221. doi:10.1038/s41586-019-1111-9
Habel, M., Mechkin, K., Podgorska, K., Saunes, M., Babiński, Z., Chalov, S., et al. (2020). Dam and reservoir removal projects: a mix of social-ecological trends and cost-cutting attitudes. Sci. Rep. 10, 19210. doi:10.1038/s41598-020-76158-3
Hart, D. D., Johnson, T. E., Bushaw-Newton, K. L., Horwitz, R. J., Bednarek, A. T., Charles, D. F., et al. (2002). Dam removal: challenges and opportunities for ecological research and river restoration. BioScience 52, 669–682. doi:10.1641/0006-3568(2002)052[0669:DRCAOF]2.0.CO;2
Hondzo, M., and Wang, H. (2002). Effects of turbulence on growth and metabolism of periphyton in a laboratory flume. Water Resour. Res. 38, 1–9. doi:10.1029/2002WR001409
IDRA (2012). Étude IDRA environnement pour la préfecture de la manche. Effacement des barrages de la Sélune, gestion des sédiments contaminés et plan de gestion – phase 1 synthèse des données et état des lieux du site.
Kupilas, B., Friberg, N., McKie, B. G., Jochmann, M. A., Lorenz, A. W., and Hering, D. (2016). River restoration and the trophic structure of benthic invertebrate communities across 16 European restoration projects. Hydrobiologia 769, 105–120. doi:10.1007/s10750-015-2569-6
Lehner, B., Liermann, C. R., Revenga, C., Vörösmarty, C., Fekete, B., Crouzet, P., et al. (2011). High-resolution mapping of the world’s reservoirs and dams for sustainable river-flow management. Front. Ecol. Environ. 9, 494–502. doi:10.1890/100125
Maazouzi, C., Piscart, C., Pihan, J.-C., and Masson, G. (2009). Effect of habitat-related resources on fatty acid composition and body weight of the invasive Dikerogammarus villosus in an artificial reservoir. Fundam. Appl. Limnol. 175, 327–338. doi:10.1127/1863-9135/2009/0175-0327
Macneil, C., Dick, J. T. A., and Elwood, R. W. (1997). The trophic ecology of freshwater gammarus spp. (crustacea:amphipoda): problems and perspectives concerning the functional feeding group concept. Biol. Rev. 72, 349–364. doi:10.1111/j.1469-185X.1997.tb00017.x
Mahan, D. C., Betts, J. T., Nord, E., Van Dyke, F., and Outcalt, J. M. (2021). Response of benthic macroinvertebrates to dam removal in the restoration of the Boardman River, Michigan, USA. PLoS One 16, e0245030. doi:10.1371/journal.pone.0245030
Major, J. J., East, A. E., O’Connor, J. E., Grant, G. E., Wilcox, A. C., Magirl, C. S., et al. (2017). Geomorphic responses to dam removal in the United States – a two-decade perspective. Gravel-Bed Rivers Process. Disasters 2017, 355–383. doi:10.1002/9781118971437.ch13
Merritt, R. W., Cummins, K. W., and Berg, M. B. (2017). “Chapter 20 - trophic relationships of macroinvertebrates,” in Methods in stream ecology, volume 1. Editors F R Hauer, and G A Lamberti. 3rd (Boston: Academic Press), 413–433. doi:10.1016/B978-0-12-416558-8.00020-2
Noda, K., Hamada, J., Kimura, M., and Oki, K. (2018). Debates over dam removal in Japan. Water Environ. J. 32, 446–452. doi:10.1111/wej.12344
Pellan, L., Medoc, V., Renault, D., Spataro, T., and Piscart, C. (2016). Feeding choice and predation pressure of two invasive gammarids, Gammarus tigrinus and Dikerogammarus villosus, under increasing temperature. Hydrobiologia 781, 43–54. doi:10.1007/s10750-015-2312-3
Piscart, C., Genoel, R., Dolédec, S., Chauvet, E., and Marmonier, P. (2009). Effects of intense agricultural practices on heterotrophic processes in streams. Environ. Pollut. 157, 1011–1018. doi:10.1016/j.envpol.2008.10.010
Piscart, C., Navel, S., Maazouzi, C., Montuelle, B., Cornut, J., Mermillod-Blondin, F., et al. (2011). Leaf litter recycling in benthic and hyporheic layers in agricultural streams with different types of land use. Sci. Total Environ. 409, 4373–4380. doi:10.1016/j.scitotenv.2011.06.060
Poff, N. L., Allan, J. D., Bain, M. B., Karr, J. R., Prestegaard, K. L., Richter, B. D., et al. (1997). The natural flow regime. BioScience 47, 769–784. doi:10.2307/1313099
Poff, N. L., and Hart, D. D. (2002). How Dams Vary and Why it Matters for the Emerging Science of Dam Removal: an ecological classification of dams is needed to characterize how the tremendous variation in the size, operational mode, age, and number of dams in a river basin influences the potential for restoring regulated rivers via dam removal. BioScience 52, 659–668. doi:10.1641/0006-3568(2002)052[0659:HDVAWI]2.0.CO;2
Pollard, A. I., and Reed, T. (2004). Benthic invertebrate assemblage change following dam removal in a Wisconsin stream. Hydrobiologia 513, 51–58. doi:10.1023/B:hydr.0000018164.17234.4f
Poulos, H. M., Miller, K. E., Heinemann, R., Kraczkowski, M. L., Whelchel, A. W., and Chernoff, B. (2019). Dam removal effects on benthic macroinvertebrate dynamics: a new england stream case study (Connecticut, USA). Sustainability 11, 2875. doi:10.3390/su11102875
Randle, T. J., Bountry, J. A., Ritchie, A., and Wille, K. (2015). Large-scale dam removal on the Elwha River, Washington, USA: erosion of reservoir sediment. Geomorphology 246, 709–728. doi:10.1016/j.geomorph.2014.12.045
Ravot, C., Laslier, M., Hubert-Moy, L., Dufour, S., Le Coeur, D., and Bernez, I. (2020). Large dam removal and early spontaneous riparian vegetation recruitment on alluvium in a former reservoir: lessons learned from the pre-removal phase of the Sélune River project (France). River Res. Appl. 36, 894–906. doi:10.1002/rra.3535
Reid, A. J., Carlson, A. K., Creed, I. F., Eliason, E. J., Gell, P. A., Johnson, P. T. J., et al. (2019). Emerging threats and persistent conservation challenges for freshwater biodiversity. Biol. Rev. 94, 849–873. doi:10.1111/brv.12480
Rodríguez-Pérez, H., Pannard, A., Gorzerino, C., Pellan, L., Massé, S., Bouger, G., et al. (2021). Ecological consequences of consecutive river damming for three groups of bioindicators. Ecol. Indic. 131, 108103. doi:10.1016/j.ecolind.2021.108103
Roussel, J. M., Fraisse, S., Dézerald, O., Fovet, O., Pannard, P., Rodríguez-Pérez, H., et al. (2024). Effects of large dams on the aquatic food web along a coastal stream with high sediment loads. Front. Ecol. Evol. 11, 1254462. doi:10.3389/fevo.2023.1250892
Schmidt-Kloiber, A., and Hering, D. (2015). www.freshwaterecology.info – an online tool that unifies, standardises and codifies more than 20,000 European freshwater organisms and their ecological preferences. Ecol. Indic. 53, 271–282. doi:10.1016/j.ecolind.2015.02.007
Shafroth, P. B., Perry, L. G., Rose, C. A., and Braatne, J. H. (2016). Effects of dams and geomorphic context on riparian forests of the Elwha River, Washington. Ecosphere 7, e01621. doi:10.1002/ecs2.1621
Sullivan, S. M. P., and Manning, D. W. P. (2017). Seasonally distinct taxonomic and functional shifts in macroinvertebrate communities following dam removal. PeerJ 5, e3189. doi:10.7717/peerj.3189
Sullivan, S. M. P., Manning, D. W. P., and Davis, R. P. (2018). Do the ecological impacts of dam removal extend across the aquatic–terrestrial boundary? Ecosphere 9, e02180. doi:10.1002/ecs2.2180
Tabor, R. A., Johnson, J. R., Peters, R. J., Mahan, R., McHenry, M. L., Brenkman, S. J., et al. (2022). Distribution, relative abundance, and length of sculpins in the elwha river watershed following the removal of two hydroelectric dams. nwsc 95, 292–306. doi:10.3955/046.095.0305
Tachet, H., Richoux, P., Bournaud, M., and Usseglio-Polatera, P. (2010). Invertébrés d’eau douce: systématique, biologie, écologie. Paris: CNRS édition.
Tank, J. L., Rosi-Marshall, E. J., Griffiths, N. A., Entrekin, S. A., and Stephen, M. L. (2010). A review of allochthonous organic matter dynamics and metabolism in streams. J. N. Am. Benthol. Soc. 29, 118–146. doi:10.1899/08-170.1
Thompson, R. M., Phillips, N. R., and Townsend, C. R. (2009). Biological consequences of clear-cut logging around streams—moderating effects of management. For. Ecol. Manag. 257, 931–940. doi:10.1016/j.foreco.2008.10.025
Tonin, A. M., Hepp, L. U., and Gonçalves, J. F. (2017). Spatial variability of plant litter decomposition in stream networks: from litter bags to watersheds. Ecosystems 21, 567–581. doi:10.1007/s10021-017-0169-1
Vannote, R. L., Minshall, G. W., Cummins, K. W., Sedell, J. R., and Cushing, C. E. (1980). The River continuum concept. Can. J. Fish. Aquat. Sci. 37, 130–137. doi:10.1139/f80-017
Vörösmarty, C. J., McIntyre, P. B., Gessner, M. O., Dudgeon, D., Prusevich, A., Green, P., et al. (2010). Global threats to human water security and river biodiversity. Nature 467, 555–561. doi:10.1038/nature09440
Ward, J., and Stanford, J. (1983). “The serial discontinuity concept of lotic ecosystems,” in Dynamics of lotic ecosystems, 29–42.
Webb, B. W., and Zhang, Y. (2004). Intra-annual variability in the non-advective heat energy budget of Devon streams and rivers. Hydrol. Process. 18, 2117–2146. doi:10.1002/hyp.1463
Weiss, R. F. (1970). The solubility of nitrogen, oxygen and argon in water and seawater. Deep Sea Res. Oceanogr. Abstr. 17, 721–735. doi:10.1016/0011-7471(70)90037-9
Weiss, R. F. (1974). Carbon dioxide in water and seawater: the solubility of a non-ideal gas. Mar. Chem. 2, 203–215. doi:10.1016/0304-4203(74)90015-2
Wieferich, D. J., Duda, J., Wright, J. J., Uribe, R. D., and Beard, J. W. (2021). Dam removal information portal dashboard Python dash software. USGS Organization. Available at: https://data.usgs.gov/drip-dashboard. doi:10.5066/P9UNIWKF
Keywords: functional integrity, river restoration, phytobenthos, macroinvertebrate feeding groups, river metabolism, gas exchange, benthic chambers
Citation: Piscart C, Dézerald O, Pellan L, Le Bris N, Rodríguez-Pérez H, Beauverger T, Huteau D and Roussel J-M (2024) Persistent disconnect between flow restoration and restoration of river ecosystem functions after the removal of a large dam on the Sélune River. Front. Environ. Sci. 12:1250810. doi: 10.3389/fenvs.2024.1250810
Received: 19 October 2023; Accepted: 09 February 2024;
Published: 27 February 2024.
Edited by:
Francisca C. Aguiar, University of Lisbon, PortugalReviewed by:
Diego Rodrigues Macedo, Federal University of Minas Gerais, BrazilXianfu Zhao, Ministry of Water Resources and Chinese Academy of Sciences, China
Copyright © 2024 Piscart, Dézerald, Pellan, Le Bris, Rodríguez-Pérez, Beauverger, Huteau and Roussel. This is an open-access article distributed under the terms of the Creative Commons Attribution License (CC BY). The use, distribution or reproduction in other forums is permitted, provided the original author(s) and the copyright owner(s) are credited and that the original publication in this journal is cited, in accordance with accepted academic practice. No use, distribution or reproduction is permitted which does not comply with these terms.
*Correspondence: Christophe Piscart, Y2hyaXN0b3BoZS5waXNjYXJ0QHVuaXYtcmVubmVzLmZy
†Present address: Héctor Rodríguez-Pérez, Office Français de la Biodiversité, Pôle R&D ECLA, Aix-en-Provence, France