Rising awareness to improve conservation of microorganisms in terrestrial ecosystems: advances and future directions in soil microbial diversity from Chile and the Antarctic Peninsula
- 1HUB Ambiental UPLA, Laboratorio Observatorio de Ecología Microbiana, Laboratory of Aquatic Environmental Research, Laboratorio de Geografía Ambiental & Palinología, Universidad de Playa Ancha, Valparaíso, Chile
- 2Departamento de Ciencias y Geografía, Facultad de Ciencias Naturales y Exactas, Universidad de Playa Ancha, Valparaíso, Chile
- 3Aix-Marseille University, University Toulon, CNRS, IRD, M.I.O. UM 110, Mediterranean Institute of Oceanography, Marseille, France
- 4Millennium Institute Biodiversity of Antarctic and Subantarctic Ecosystems (BASE), Santiago, Chile
- 5Centro de Biotecnología de los Recursos Naturales, Facultad de Ciencias Agrarias y Forestales, Universidad Católica del Maule, Talca, Chile
- 6Doctorado Interdisciplinario en Ciencias Ambientales, Facultad de Ciencias Naturales y Exactas, Universidad de Playa Ancha, Valparaíso, Chile
- 7Laboratorio de Microbiología Aplicada, Centro de Estudios Avanzados en Zonas Áridas, La Serena, Chile
- 8Instituto de Investigación Multidisciplinario en Ciencia y Tecnología, Universidad de La Serena, La Serena, Chile
- 9Grupo Ecología Microbiana de la Rizósfera (EMRi), Laboratorio de Microbiología, Instituto de Biología, Facultad de Ciencias, Pontificia Universidad Católica de Valparaíso, Valparaíso, Chile
- 10Departamento de Ciencias Geológicas, Facultad de Ingeniería y Ciencias Geológicas, Universidad Católica del Norte, Antofagasta, Chile
- 11Laboratory of Microbial Ecology, Department of Ecological Sciences, Faculty of Sciences, Universidad de Chile, Santiago, Chile
- 12Laboratory of Soil Microbial Ecology and Biogeochemistry (LEMiBiS), Institute of Agri-Food, Animal and Environmental Sciences (ICA3), Universidad de O’Higgins, San Fernando, Chile
- 13Center of Applied Ecology and Sustainability (CAPES), Santiago, Chile
Soil ecosystems are important reservoirs of biodiversity, as they are the most diverse habitat on Earth. Microbial biodiversity plays key roles in many ecosystem services, including the support to biogeochemical cycles. However, despite great advances in the understanding of the role of soil microbiota in providing benefits to nature and humankind, there is still much knowledge to be gained from understudied areas across the globe. Indeed, underrepresentation of the Global South in ecological studies has been suggested as an important gap that could compromise global solutions to conservation and the current biodiversity and climate crisis. In the Southern hemisphere, the southwest of South America, which includes Chile, runs behind the rest of the continent on studies related to soil microbial diversity and ecosystem functions. Therefore, to gain a better understanding of the global biodiversity and environment crisis, essential perspectives and knowledge from underrepresented regions need to be acknowledged to avoid biases in the scientific community. The main objective of this work is to understand how soil microbial diversity has been studied in Chile and the Antarctic Peninsula since 1975 to identify main knowledge gaps and funding opportunities for future research. Our survey consists of 343 articles representing 1,335 sampling points from Continental Chile to the Antarctic Peninsula. It revealed a better representation of articles studying bacterial and fungal diversity in the extreme regions of Chile funded by both international funds and the National Agency for Research and Development (ANID). To study microbial diversity, cultivation-based methods are still the most commonly used, whereas molecular studies are increasing but insufficiently applied. We have identified and argued the need to enhance collaborative multi- and interdisciplinary efforts, fundings for sequencing effort, and long-term studies to provide robust and informative knowledge about soil microbial communities.
1 Introduction
Soil ecosystems are important reservoirs of biodiversity, as they are the most diverse habitat on Earth (Anthony et al., 2023). This biodiversity varies from microscopic constituents such as bacteria, archaea, fungi, and viruses, up to macroscopic life, all of which can spend their entire or part of their life cycle within the soil environment (FAO, 2020). Recently, it has been estimated that up to 50% and 90% of all bacteria and fungi identified on Earth are present in soil ecosystems, respectively (Anthony et al., 2023). It is indeed the soil spatial and temporal heterogeneity, along with characteristics missing in other microbial habitats (e.g., rhizosphere environments or spatial isolation within soil aggregate microhabitats), which contributes to this considerable variety of life (Jansson and Hofmockel, 2018). At a local scale, this diversity is mainly driven by soil physicochemical properties (e.g., pH and soil organic carbon) (Fierer, 2017), while spatial patterns at a global scale have been attributed to variations in plant composition and climate conditions, in addition to soil properties (Bardgett and van der Putten, 2014). It has also been pointed out that species richness belowground does not follow the same trend as aboveground biodiversity (Bardgett and van der Putten, 2014).
Microbial biodiversity plays key roles in many soil functions, which in turn results in important soil ecosystem services (Wall et al., 2012). In addition to the contribution to biogeochemical cycles, among others (Bardgett and van der Putten, 2014), soil microorganisms have been recognized as important drivers of ecosystem multifunctionality (i.e., ecosystem functions and services) in terrestrial environments at a global scale (Delgado-Baquerizo et al., 2016). Due to their wide range of functional capacities and their adaptation to diverse (including extreme) conditions, soil microorganisms offer potential biotechnological applications for food or pharmaceutical production. Despite great advances in the understanding of the role of soil microbiota in providing benefits to nature and humankind, much knowledge is needed to better comprehend its specific role in particular soil processes (Jansson and Hofmockel, 2018). Nowadays, global awareness of the soil biodiversity transcendence in ecosystem functioning not only encourages the scientific community to further decipher soil microbial roles in the environment (i.e., from a genetic to a functional diversity level) (Bardgett and van der Putten, 2014; Jansson and Hofmockel, 2018), but also to contribute from academia with appropriate knowledge and technological solutions beyond academia. This is particularly important to support policymakers and stakeholders in their attributions to encourage sustainable soil management practices to preserve such biodiversity, functioning and associated ecosystem services (Cavicchioli et al., 2019).
In a global context, many of the studies conducted on soil biodiversity have taken place either in temperate ecosystems of the northern hemisphere (Guerra et al., 2020) or mainly in agroecosystems (Hartmann and Six, 2022). In ecology, this underrepresentation of the Global South has been suggested as an important gap that could compromise global solutions to conservation and the current biodiversity and climate crisis (Maas et al., 2021). In the Southern hemisphere, the southwest of South America, which includes Chile, particularly lacks studies on soil microbial diversity and ecosystem functions, as opposed to those conducted in the northern part of the continent (Guerra et al., 2020). The ecological significance of this region is beyond local, as Chile has been considered as a “biogeographic island” (Scherson et al., 2017), because it is surrounded by natural barriers (Altiplano, Atacama Desert, Los Andes Mountains, Pacific Ocean, Patagonian ice fields, Southern Ocean and Antarctica) that result in the evolution of a unique biota (Villagrán and Hinojosa, 1997; Villagrán and Armesto, 2005). The large latitudinal gradient characteristic of the Chilean territory, results in biogeographical and climatic conditions favoring the development of a rich and varied biodiversity, with 31,000 native species reported (Ministerio del Medio Ambiente–Chile, 2018) and around 25% of them endemic (up to >50% for fishes, amphibians, reptiles, and plants) (Ministerio del Medio Ambiente–Chile, 2018). Moreover, Chile host one of the five Mediterranean biomes on Earth, which is considered one of the 36 worldwide global hotspots of conservation priority due to its endemism and a high degree of habitat loss, mainly due to the conversion and replacement of the native vegetation (Fuentes et al., 2019). Therefore, to gain a better understanding of the global biodiversity and environment crisis, as it has been previously reinforced (Nielsen et al., 2017; Nuñez et al., 2019), essential perspectives and knowledge from underrepresented regions need to be acknowledged to avoid biases in the scientific community (Maas et al., 2021). This is particularly critical in zone of the globe such as Chile, where unprecedented climatic anomalies such as incomparable droughts are expected to occur sooner than anticipated and increase in frequency in the next 30 years regardless of emission scenarios (Satoh et al., 2022).
In Chile recent efforts documenting the state of knowledge of soil biodiversity have pointed out disparities on soil taxa and ecosystem functions studied (Marín et al., 2022). Other national surveys have reported the distribution of soil organic carbon (Pfeiffer et al., 2020), and provided a gridded database on soil physical and hydraulic properties (Dinamarca et al., 2023), contributing to a better understanding of the environmental variables that could affect microbial diversity. Here, we complement these efforts by presenting a compilation and an in-depth analysis of additional aspects of soil microbial biodiversity studies conducted in Chile and the Antarctic Peninsula. In this systematic review, we have included the Antarctic Peninsula due to the scientific significance for Chile, reflected in specific financing programs developed to support research at this place. Moreover, we considered that it was important to incorporate this area because of administrative, geological, and biological connections with the continental territory. Thus, the area to cover includes diverse biomes, from the Atacama Desert to Antarctica, which adds complexity to the literature search and data analysis. Moreover, we add data generated in the past 50 years, which is challenging due to considerable changes in methodology registered overtime. The present review is the collective effort of a multidisciplinary group of researchers (microbiologists, ecologists, geneticists, soil scientists, and geologists), and the data we present herein may serve as a guide to decision-makers regarding environmental conservation efforts, agroecosystems development, research funding, among others. The main objective of this work is to understand how soil microbial diversity has been studied in Chile and the Antarctic Peninsula since 1975 to identify main knowledge gaps and funding opportunities for future research.
2 Historical context of research and funding development contributing to the study of (soil) microbial diversity in Chile
The formal study of microorganisms in Chile began in the 1880s, with the foundation of the Histology and Pathological Anatomy Laboratories, in the Faculty of Medicine of the University of Chile (Rodríguez, 1992; Osorio, 2010). Microbiology was, at that moment, limited to bacteriological studies focusing on infectious pathologies (Seeger and Espinoza, 2008). In 1927, the Agronomic Institute was transferred to the University of Chile as the Faculty of Agronomy and Veterinary Medicine (Agronomia, 2023) and in 1953, microbiologists from the Bacteriological Institute of Chile created the first Chilean Association of Microbiology (ACHM) now named Chilean Society of Microbiology (SOMICH) (Osorio, 2015). Along with SOMICH, the Society of Ecology of Chile (SOCECOL) and the Chilean Society of Soil Science (SCHCS) founded in the 70’s have greatly contributed to the study of soil biodiversity in the Chilean territory. In terms of documentation of soil studies in Chile, in 1968, a soil map was published as one of the first efforts to show a classification of soils with taxonomic and cartographic units, with the aim to contribute to an adequate agricultural soil management (Alcayaga, 1968). Later, in 1992, the Map of Associations of Large Soil Groups in Chile gathered information from numerous soil studies carried out in Chile since the 1960s (Luzio and Alcayaga, 1992). Related to soils and biodiversity, the work on Mediterranean-type ecosystems (di Castri and Mooney, 1973) and microbial activity in this type of climate (Schaefer, 1973) are among the earliest documented works paving the way to the study of soil microbial diversity in Chile.
Funding formalization to support research, scientific and technological development in Chile is reflected by the creation of several institutions and instruments. The Chilean Economic Development Agency (Corporación de Fomento de la Producción—CORFO) was created in 1939 to link research and productive development (Nazer Ahumada, 2016). Based on the Antarctic Treaty (signed on 1 December 1959, in Washington), the Chilean Antarctic Institute (Instituto Antártico Chileno—INACH) was established in 1963 to promote advances in Antarctic science (Ministerio de Relaciones Exteriores - Chile, 1966). The following year, the Institute of Agricultural Research (Instituto de Investigaciones Agropecuarias—INIA) (Quesada, 2016) was created. In 1953, the United Nations Economic Commission for Latin America and the Caribbean (ECLA, Comisión Económica para América Latina y el Caribe—CEPAL recommended the creation of a coordinating institution for technological research, with fiscal funds available (CEPAL—Comisión Económica para América Latina, 1953). From this, the National Commission of Scientific and Technological Research (Comisión Nacional de Investigación Científica y Tecnológica—CONICYT), currently the National Research and Development Agency (Agencia Nacional de Investigación y Desarrollo—ANID) was founded in 1966 (Ministerio de Educación - Chile, 2018). The latter agency was created in 2018 with the objective of becoming a coordinating and funding hub for the advancement of science and technology in the national context, with a new institutional framework that created the Ministry of Science, Technology, Knowledge and Innovation (MinCTC&I) (Ministerio de Educación - Chile, 2018).
The first CONICYT (currently ANID) program was created in 1971 (Zárate et al., 2022); later, in 1981 the Fondo Nacional de Desarrollo Científico y Tecnológico (FONDECYT) was launched, hence funding research projects since 1982 (Figure 1). This funding was developed as a competitive grant to support fundamental and applied science (Zárate et al., 2022), with the objective to maintain and strengthen national capacity for high quality research (Benavente et al., 2012). The National Fund for Scientific and Technological Development (Fondo de Fomento al Desarrollo Científico y Tecnológico—FONDEF) was created in 1991 to promote collaboration between academia and private sector by financing research in applied sciences (Benavente et al., 2012). Other relevant sources of research funding in Chile include the Millennium Science Initiative Program (Programa Iniciativa Científica Milenio) created in 1999 to finance research in the areas of Social and Natural Sciences. Since 2011, the Millennium Initiative is part of the MinCTC&I, and until 2013 it has supported 13 Millennium Institutes in the Natural and Exact Sciences and 15 Millennium Nucleus in the same areas (Ministerio de Ciencia Tecnología Conocimiento e Innovación, 2023). It is worth noticing that only two Institutes (Millennium Institute—BASE, Millennium Institute—SECOS) and three Nucleus (Millennium Nucleus—UPWELL, Millennium Nucleus—NUTME, and Millennium Nucleus—LiLi) specially focus on biodiversity, ecology and/or conservation mainly on aquatic related ecosystems (except LiLi) (Ministerio de Ciencia Tecnología Conocimiento e Innovación, 2023). In addition, another instrument created under ANID is the Centros Basales with the aim to strengthen Chile’s scientific capabilities towards greater social and economic development (ANID—Agencia Nacional de Investigación y Desarrollo, 2023). By 2024, out of the 19 Centros Basales, two (CAPES and IEB) concentrate their research on ecology, biodiversity, and sustainability.
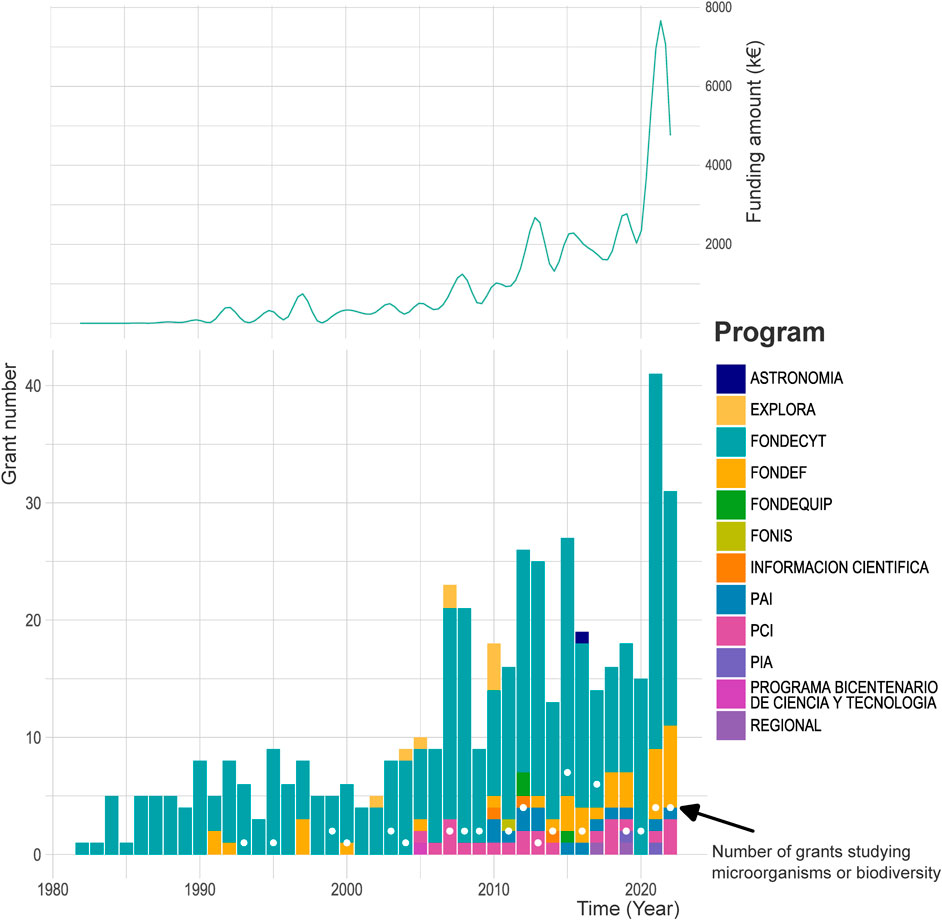
FIGURE 1. Number of soil-related research grants funded by Chilean National Agency for Research and Development (ANID). White points represented the number of grants corresponding to projects focusing on soil microorganisms or biodiversity. Dataset extracted and filtered from https://github.com/ANID-GITHUB/Historico-de-Proyectos-Adjudicados. ASTRONOMIA:Grant for scientific projects in Astronomy; EXPLORA: Programa Nacional de Divulgación y Valoración de la Ciencia y la Tecnología/National program for science and technology outreach; FONDECYT: Fondo Nacional de Desarrollo Científico y Tecnológico; FONDEF: Fondo de Fomento al Desarrollo Científico y Tecnológico/National fund for scientific and technological development; FONDEQUIP: Programa de Equipamiento Científico y Tecnológico/National fund for scientific and technological equipment; FONIS: Fondo Nacional de Investigación y Desarrollo en Salud/National Fund for Research and Development in Health sciences; INFORMACION CIENTIFICA: Fund for strengthening the national scientific journals; PAI: Programa de Atracción e Inserción de Capital Humano Avanzado/Advanced human capital attraction and insertion program; PCI: Programa de cooperación internacional/International cooperation program; PIA: Programa de investigación asociativa/Associative research program; PROGRAMA BICENTENARIO DE CIENCIA Y TECNOLOGIA: Bicentennial program for sciences and technology; REGIONAL = National fund for the implementation of regional science and technology research centers.
Even with all this battery of programs and institutions, FONDECYT has remained the most important horizontal mechanism for funding scientific research in Chile (Benavente et al., 2012) (Figure 1). Despite the undoubted contribution to the national scientific development, it has also been recognized that up to 60% of funding from this program has been granted to only two, out of the 30 Universities belonging to the Consejo de Rectores de las Universidades Chilenas (CRUCH), both in the capital of Chile (Parada, 1999). Since 1981, ANID has funded 473 projects related to soil science (including “terrestrial” or “soil” terms in the title of the project), of which 50 have focused on soil biodiversity and/or microorganism studies (Figure 1, white dots). Of such studies, 46% have been led by the sole University of La Frontera, in the Araucanía administrative region, representing a total of 2,171 k€ accumulated since 1981.
3 Methodology
3.1 Literature search
We created a dataset following the method described by Guerra et al. (2020) adapted to our topic of interest. Briefly, we collected published literature on studies of soil microbial biodiversity. For this literature search, we conducted independent searches in the Web of Knowledge (WOS), the SCOPUS and the PUBMED databases on 23 June 2023, within papers published between 1975 and 2023. For the first search, we used the keywords (soil OR sand) AND (“Antarctic Peninsula” OR Chile*) AND (*bacteria* OR fung* OR *archaea* OR vir* OR microbi* OR metagen* OR metatranscript* OR metataxon* OR genom*) using the “Topic” search option, which includes title, abstract, author keywords, and Keywords Plus. Chile has strong commitments for the conservation and study of the Antarctic Peninsula. In this context, this territory was included in our study because of administrative, geological, and biological connections as well as scientific relevance for the country.
3.2 Rationale of data selection
The initial search returned 1,424 publications, which were further manually selected based on the following inclusion criteria: 1) soil samples excluding biocrusts, marine and lacustrine sediments (the “sand*” keyword being not selective enough to exclude sediments); 2) in situ soil samples were not modified by researchers before analysis (e.g., excluding microcosm or mesocosm incubation); 3) in the case of study after atypical events that may have caused a major change on natural microbial communities (e.g., following a wildfire), the study was only considered if they also provided a control point; 4) study was conducted in the Chilean claimed territory (including Antarctic Peninsula) (the “Chile*” keyword being not selective enough to exclude studies outside Chile); 5) only original studies (i.e., reviews were excluded); 6) only studies with diversity data (i.e., studies with only microbial activity measurements were excluded). The list of selected publications based on the criteria previously described is available in Mendeley data repository at: 10.17632/vxpjcjfvth.1.
3.3 Data handling
Among the 1,424 studies returned by the performed search, a total of 343 articles fitted the scope according to the criteria described in the methodological section. These 343 articles represented 1,335 sampling points where microbial biodiversity was studied in soils from Chile and the Antarctic Peninsula.
All the statistical analysis and figures were performed with the R software version 4.3.1 (R Core Team, 2021) using the R studio version 2023.09.0. The main packages used for the analysis of the dataset related to data frame manipulation, generation of the figures and visual purposes are listed with the corresponding references as supplementary information (see details in the Supplementary Table S1).
The sampling sites were classified into seven macrozones, grouping the administrative regions of Chile, to provide a spatial interpretation of the results (Figure 2): North (17°S–29°S: Arica y Parinacota, Tarapacá, Antofagasta, and Atacama), Central (29°S–34°S: Coquimbo, Valparaíso, and Metropolitana), Central-South (34°S–38°S: O’Higgins, Maule, Ñuble, and Biobío), South (38°S–44°S: Araucanía, Los Ríos, and Los Lagos including Chiloe island), Austral (44°S–56°S: Aysén and Magallanes y la Antártica Chilena including Tierra del Fuego and Cape Horn), Insular (i.e., Chilean islands not considered in other regions including Easter Island, Juan Fernandez Archipelago), and the Antarctic Peninsula; two studies did not exhibit the information and hence were classified as “Non provided”.
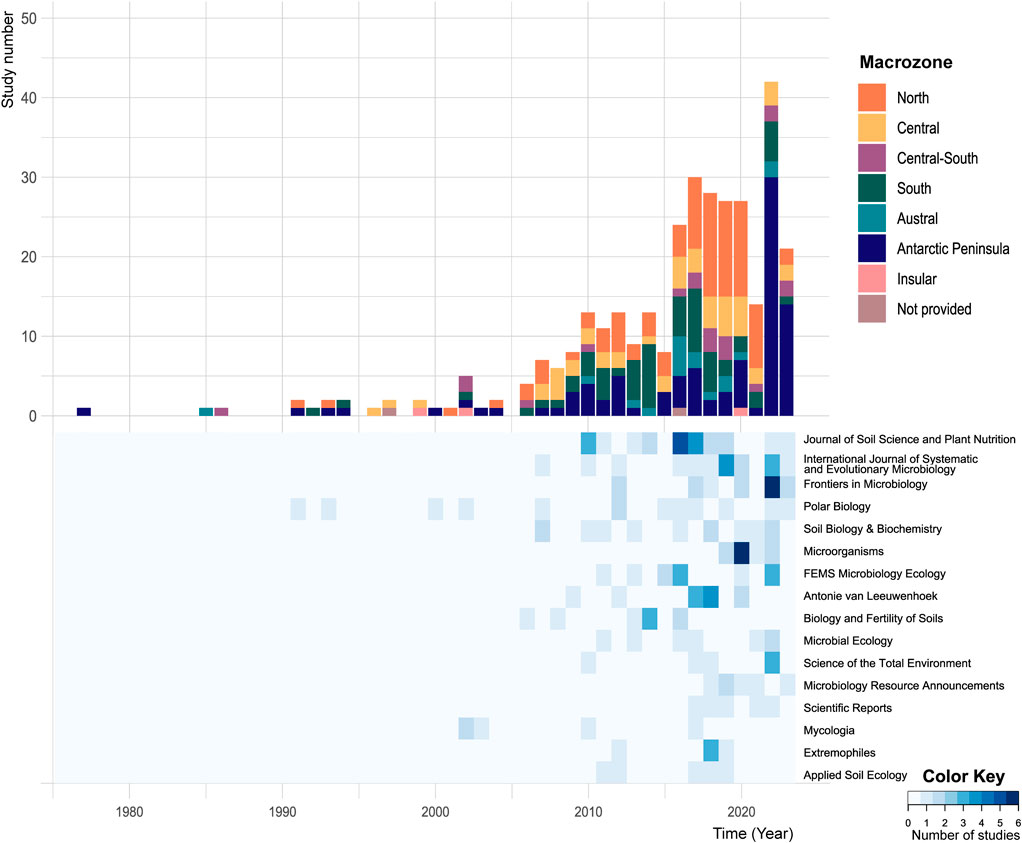
FIGURE 2. Number of studies focusing on soil microbiome diversity in Chile and Antarctic Peninsula. Top panel: number of soil microbial diversity studies per year and per Chilean macrozone. Bottom panel: number of soil microbial diversity studies published per year in the 16 most frequent scientific journals (only showing journals with more than five publications in total).
The methodology used in every selected article was clustered into 23 groups (Table 1).
The main objective of every article was reported and clustered into eight categories, named application potential, climate change, extreme environments, new species, effect of disturbance, functionality insights, biogeography, and others (which included descriptive surveys, biotic interactions, bioprospective studies, and paleomicrobiology surveys) (Supplementary Figure S1). A maximum of three categories was attributed to every single study; thus, a single article can contribute to more than one category.
4 Towards a better understanding of soil microbial communities in Chile and the Antarctic Peninsula
4.1 Historical and geographical trends
From a historical point of view, the first study reporting microbial diversity in soils from Chile (and the Antarctic Peninsula), within the time frame of this study (1975–2023), was documented in 1977 (Figure 2). It described two new fungal species from subantarctic Magallanes ecoregion and Antarctic Peninsula (Caretta and Piontelli, 1977). Later, from 1977 to 2005, we found few publications related to the scope of our study, with zero to two reports per year matching our research criteria. The exception to this was observed in 2002 when five studies were conducted in the South of Chile, in the South-Central area (n = 2), Easter Island, and Antarctic Peninsula. Four out of these five studies focused on fungal biodiversity, while the Antarctic study described microalgal communities. From 2005 onwards, the number increased exponentially to reach 11 studies per year between 2010–2015 and 25 per year between 2015–2023. Noticeably, the South and Austral territories were the most studied areas in 1985, 1992, 2011, 2013, 2014, and 2016, accumulating 43 surveys until 2016. This tendency changed between 2017 and 2021, when the focus shifted to the Northern zone of Chile, particularly to the Atacama Desert, recognized as an analog of the soils on Mars since 2003 approximately; thus, as a relevant extreme ecosystem for the discovery of life (Azua-Bustos et al., 2012; Azua-Bustos et al., 2022). Lately, since 2022, studies from the Antarctic Peninsula have increased, both in proportions (60.6% of the studies per year) and absolute number of studies (i.e., n = 30 in 2022 and n = 14 in 2023).
The unique and extreme conditions of the Atacama Desert have been recognized globally due to its ecological niches, resulting in microbial life of scientific, technological, and conservation relevance (Bull et al., 2016; Contador et al., 2020). Our findings add to previous reports at the national scale, highlighting this site as one of the most studied places in Chile in terms of soil microbial diversity (Marín et al., 2022). The Atacama Desert, located within the dry subtropical climate belt, has been recognized as the driest and oldest desert on our planet, where no altitudinal movement has been detected since the end of the Jurassic (150 million years ago) (Hartley et al., 2022) and a cold marine upwelling current since at least the early Cenozoic is present (Azua-Bustos et al., 2012). Descriptions of the Atacama Desert soil microbiota date back to 1966 when NASA sought basic information on desert environments to develop and test instruments for the Viking Mission in 1976 (Cameron et al., 1966). Since this NASA mission provided inconclusive results about the existence of life on Mars, no sites were described during the 1980s (Azua-Bustos et al., 2012). Later, from the early 1990s, we identified two studies reporting the diversity of halophilic bacteria in this extreme environment (Prado et al., 1991; Prado et al., 1993). Then, in 2000, the extreme conditions of the Atacama Desert for the development of life were reported using light and solar UV-B radiation experiments (Dose et al., 2001). A milestone in the investigation of microorganisms from the Atacama Desert was published in 2003 by the group led by Chris McKay, who obtained comparable results as the experiments performed in the Viking Landers missions on Mars, this time with samples taken in the Yungay area (80 km from Antofagasta). They found active decomposition of organic species in these soils and extremely low, but still level of, culturable bacteria in the samples, which validated the Atacama Desert as a Mars analog. (McKay et al., 2003; Navarro-González et al., 2003).
4.2 Main taxa and ecosystems studied
According to our search, out of the targeted microorganisms (i.e., bacteria, archaea, fungi, other microorganisms) studied in Chile and the Antarctic Peninsula, bacteria represent 73% of the studies, followed by fungi with 38% of the studies (Figure 3). Studies focusing on bacteria were common in the North macrozone, representing 30.2% of the study, while articles reporting fungi biodiversity made up 63.6% of the sampling sites at Antarctic and South macrozone. Interestingly, archaeal communities were the main objective of only one study where two archaeal metagenome-assembled genomes (MAGs) were reconstructed (Santos et al., 2021), while two studies focused on the ammonia-oxidizing bacteria and archaea in Central Chile (Bustamante et al., 2012) and the Antarctic Peninsula (Han et al., 2013).
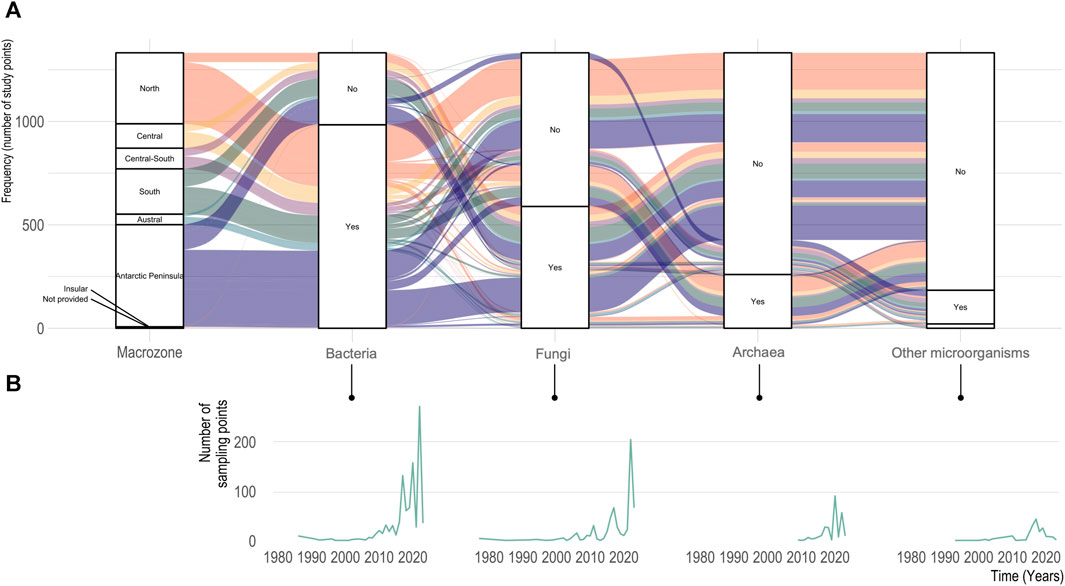
FIGURE 3. Type of microorganisms studied from soils in Chile and the Antarctic Peninsula. (A). Frequency based on the number of sampling points (n = 1,335 sampling points from 343 studies) is given for Bacteria, Archaea, Fungi and Other microorganisms by Chilean Macrozone (first clustering and color). Color for macrozone is similar to Figure 2 and Figure 3. (B). Evolution of the number of sampling points studied per year for every microorganism group considered in this study.
The surveyed ecosystems in the selected publications were representative of the main biomes and/or land use of the region across macrozones (Figure 4). Desert and salt flat reports represented up to 87.8% of the studies conducted in the North of Chile (corresponding to Arica y Parinacota, Tarapacá, Antofagasta, and Atacama administrative regions), which represents the driest territory in the country, with desertic arid to semi-arid climates. The central zone (corresponding to the Coquimbo and Valparaíso regions, and the Metropolitan region, where Santiago, the capital of the country is located), with the highest population density in Chile, encompassed the greatest diversity of ecosystems studied, including scrublands and human-impacted ecosystems (e.g., urban and industrial). In the South-Central area (O’Higgins, Maule, Ñuble and Biobío regions) and Southern territory (La Araucanía, Los Lagos, Los Ríos regions), 86.8% of the selected studies focused on agricultural, forest, and grassland ecosystems, which is consistent with the main land uses at these places. Indeed, the Maule, Ñuble, Biobío, and Araucanía regions have the highest annual and permanent crop area (around 350,000 Ha each) and forest plantation area [500,000–980,000 Ha each; ODEPA—Oficina de Estudios y Políticas Agrarias (2019)] in the country. Finally, forest and scrubland studies dominated in the Austral macrozone (Aysén and Magallanes regions) which is in line with the highest area of native forest reported in the Los Lagos, Aysén, and Magallanes y la Antártica Chilena regions [2,400,000–4,400,000 Ha each; ODEPA—Oficina de Estudios y Políticas Agrarias (2019)].
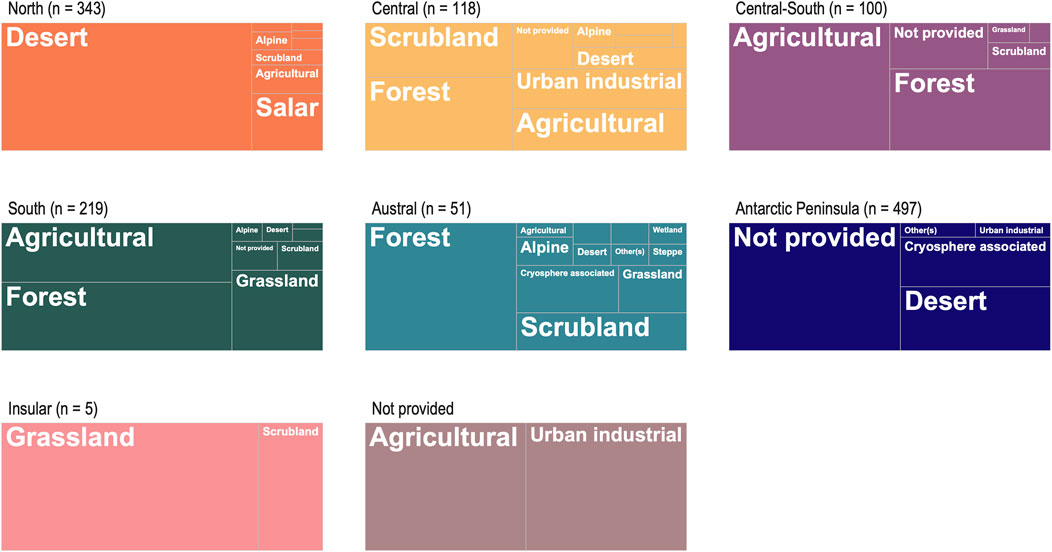
FIGURE 4. Main soil use categories for each macrozone. The number of sampling points per macrozone is indicated between brackets.
5 Main research scope and funding for the study of soil microbial diversity in Chile and the Antarctic Peninsula
5.1 Main scopes of the soil microbial diversity studies
Out of the eight research scopes categories used in this survey (Figure 5), 202 articles focused on studies including descriptive, biotic interactions, bioprospecting studies, and paleomicrobiology surveys (shown as Others in Figure 5). Descriptive studies, focusing mainly on the identification of novel species of bacteria and fungi, represented about 40% of these surveys. The second most abundant category corresponded to biotechnological applications (shown as Application in Figure 4), with 145 out of 343 of the articles identified in our study. Half of the biotechnological applications relate to the agricultural sector, with an important focus on plant growth-promoting rhizobacteria (PGPR), which can enhance plant growth by a wide variety of mechanisms like phosphate solubilization, siderophore production, biological nitrogen fixation, phytohormone production, antifungal activity, induction of resistance to pathogen toxins, promotion of beneficial symbioses, etc. (Bhattacharyya and Jha, 2012). PGPRs offer an attractive way to replace the use of chemical fertilizers or pesticides for the development of sustainable agriculture. In our survey, agricultural application studies focus on crops of interest for Chile or on the adaptation to harsh climatic conditions (e.g., Fuentes et al., 2020; Meza et al., 2022). Another important sector of application is the use of soil microorganisms for bioremediation of contaminated sites, especially by oil (e.g., Serrano and Leiva, 2017; Vázquez et al., 2017), as well as the use of microbial consortia naturally adapted to specific conditions (e.g., low temperature) for bioenergy (methane) production (Aguilar Muñoz et al., 2022). Several extremophilic strains have also been isolated, especially from Antarctic ecosystems, and evaluated for their capacity to produce enzymes of commercial interest, acting as biocatalysts able to carry out reactions at nonstandard conditions (Cabrera and Blamey, 2018; Espina et al., 2022).
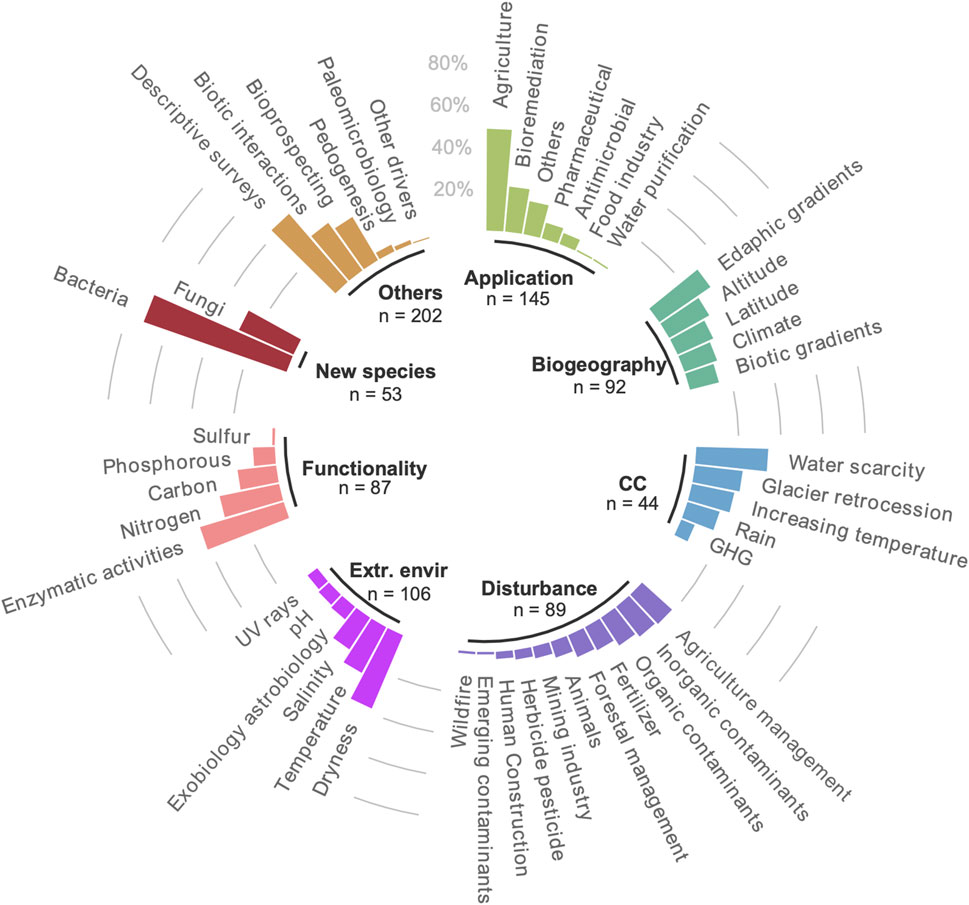
FIGURE 5. Proportion of studies addressing one of the main research scopes identified in this dataset. A single study can match several (sub) categories. CC: climate change; GHG: greenhouse gas; Extr. Env.: extreme environments.
Another important focus of the articles selected was the adaptation to extreme environments with 106 studies. This included conditions including dryness, temperature, and salinity, all of which are typical extreme conditions found in the desert and salt flats of Northern Chile (e.g., Paulino-Lima et al., 2013; Pulschen et al., 2015). These studies are of particular interest for astrobiology and take place in the Atacama Desert, reflecting the relevance of extreme environments at a global scale in the search for life. Indeed, in 2003, the European Science Foundation (ESF) launched support for the Investigating Life in Extreme Environments (ILEE) initiative, highlighting the need for a coordinated, interdisciplinary approach to future “Life in extreme environments” (LEXEN) research. The Coordinating Action for Research on the Study of Life in Extreme Environments (CAREX) was developed between 2008 and 2011 and had four priority areas: 1) contributions of life in extreme environments to biogeochemical cycles and responses to environmental change, 2) stressful environments—responses, adaptation, and evolution, 3) biodiversity, bioenergetics and interactions in extreme environments, and 4) life and habitability (Martins et al., 2017).
The biogeographic distribution of soil microorganisms and how it is related to latitudinal, altitudinal, edaphic, climatic, or biotic (e.g., vegetation cover) gradients also took an important proportion of the studies, with 92 of them focusing on this topic. The peculiar geographic and geomorphological context of Chile, with considerable latitudinal and altitudinal gradients, reflected over more than 4,000 km of expansion from North to South, and few hundred kilometers starting from the Pacific Ocean up to >6,000 m a.s.l in the Andes Cordillera, resulting in more than 20 climatic regimes in the country (ODEPA—Oficina de Estudios y Políticas Agrarias, 2019). These unique natural gradients represent a remarkable diversity of ecosystems to explore soil microbial diversity patterns. In our survey, 20 studies focused on altitudinal gradients (e.g., Renny et al., 2017; Rodríguez-Echeverría et al., 2021) and 17 on the influence of latitudinal variation (e.g., Marín et al., 2017; Knief et al., 2020).
Lastly, studies on disturbance, functionality, and climate change were represented by 89, 87, and 44 studies, respectively. Not surprisingly, within the effect of climate change, in Chile, the main research scope was the effect of water scarcity (linked to increased aridity), on soil microbiomes (Neilson et al., 2017) followed by glacial retreat effects on soil microbial communities (Fernández-Martínez et al., 2017). Within the environmental and anthropogenic disturbances identified in our survey, the majority were related to agriculture management, bringing new insights about how to prevent soil degradation (Ramírez et al., 2020) or leaching (Cardenas et al., 2013). The high proportion of studies assessing the impact of inorganic contaminants (mainly metals) can be related to the importance of the mining activity in Chile (e.g., Altimira et al., 2012; Carvajal et al., 2023).
5.2 Sources of fundings
Out of the reported sources financing research on soil microbial diversity in Chile (23 studies did not provide such information), international funding supported 53% of the studies (Figure 6), of which 59% was dedicated to studies in continental Chile. FONDECYT was the main national financial support, funding 28.7% of the studies (i.e., n = 141). This Chilean research grant represents the earliest national funding instrument supporting soil microbial diversity studies, with publications acknowledging this from 1992 until the present. The second most important pecuniary source with 8.9% of the studies funded is the so-called “Others” category that includes funding from universities or regional government, bilateral cooperation projects between CONICYT and equivalent foreign research agencies (e.g., Argentina, United Kingdom, Spain), programs funded by ANID that favor the insertion into the academia, Education Quality, and Equity Improvement Program (MECESUP Program) and private companies (e.g., Agroenergía Ingeniería Genética S.A, Anglo American Sur Ltda.). Overall, 25.5% of the Antarctic studies involving Chilean institutions were financed or co-financed by the Chilean Antarctic Institute (INACH). Interestingly, although INACH was founded in 1964 and started to finance research in 1995, only in 2016 did the first study on soil microbial diversity in Antarctica receive financial support from this institution (Figure 6).
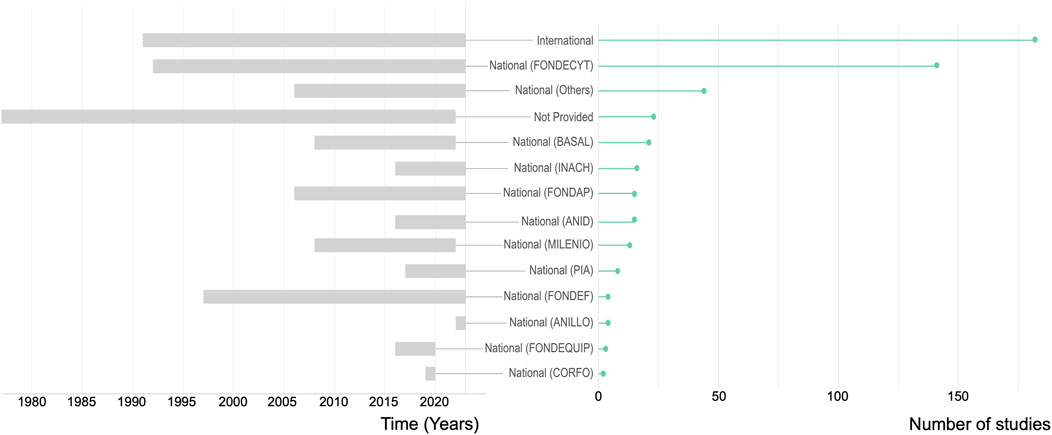
FIGURE 6. Timeline and importance of funding sources. Left panel: timeline of funding sources considering that one single study can be supported by one or more funding sources. Right panel: quantitative importance of funding sources expressed as the number of studies funded.
6 From cultivation to omics techniques
Culture was the most employed methodology (46.4% of the studies; Figure 7), followed by sequencing (27.7% of the studies) and physiological-based approaches (23.3% of the studies). Since 1975, cultivation-based methods have allowed the detection and description of soil microorganisms in Chile. This technique remained the unique approach for microorganism exploration until 1997 and nowadays it is still used, often in combination with other methods (Figure 7). In our survey, 15.5% of the studies (n = 53) allowed the isolation/culture of 61 new microbial taxa (40 bacteria and 21 fungi). This input represents a great contribution to scientific knowledge on soil biodiversity, as these contributed to better comprehend the diversity of Actinobacteria (Idris et al., 2017), Micromonospora (Carro et al., 2018) and Actinomycetes (Okoro et al., 2009). Additionally, they revealed functions still not described in various genus such as biodegradation of chlorinated aromatic compounds (Fulthorpe et al., 1996), metal-chelating compound production (Machuca et al., 2007), tolerance to UV-C (Paulino-Lima et al., 2013), biomineralization of Li-containing nanomaterials by Li-resistant bacteria (Bruna et al., 2022), degradation of PAH (Gran-Scheuch et al., 2020) and antiproliferative activity against a colon cancer cell line (Pavón et al., 2023). The new strains were mainly isolated from the North macrozone (50.9% of the studies, n = 30) and the Antarctic Peninsula (23.7% of the studies, n = 14). In the other macrozones, the remaining 9 studies described mainly new fungal species or genus (n = 10) such as the new genus Nothophytophthora gen. nov. (Jung et al., 2017). or Podospora selenospora sp. nov. discovered from the Ao horizon of a Chilean xerophilic forest soil of the Central-South region (Stchigel et al., 2002).
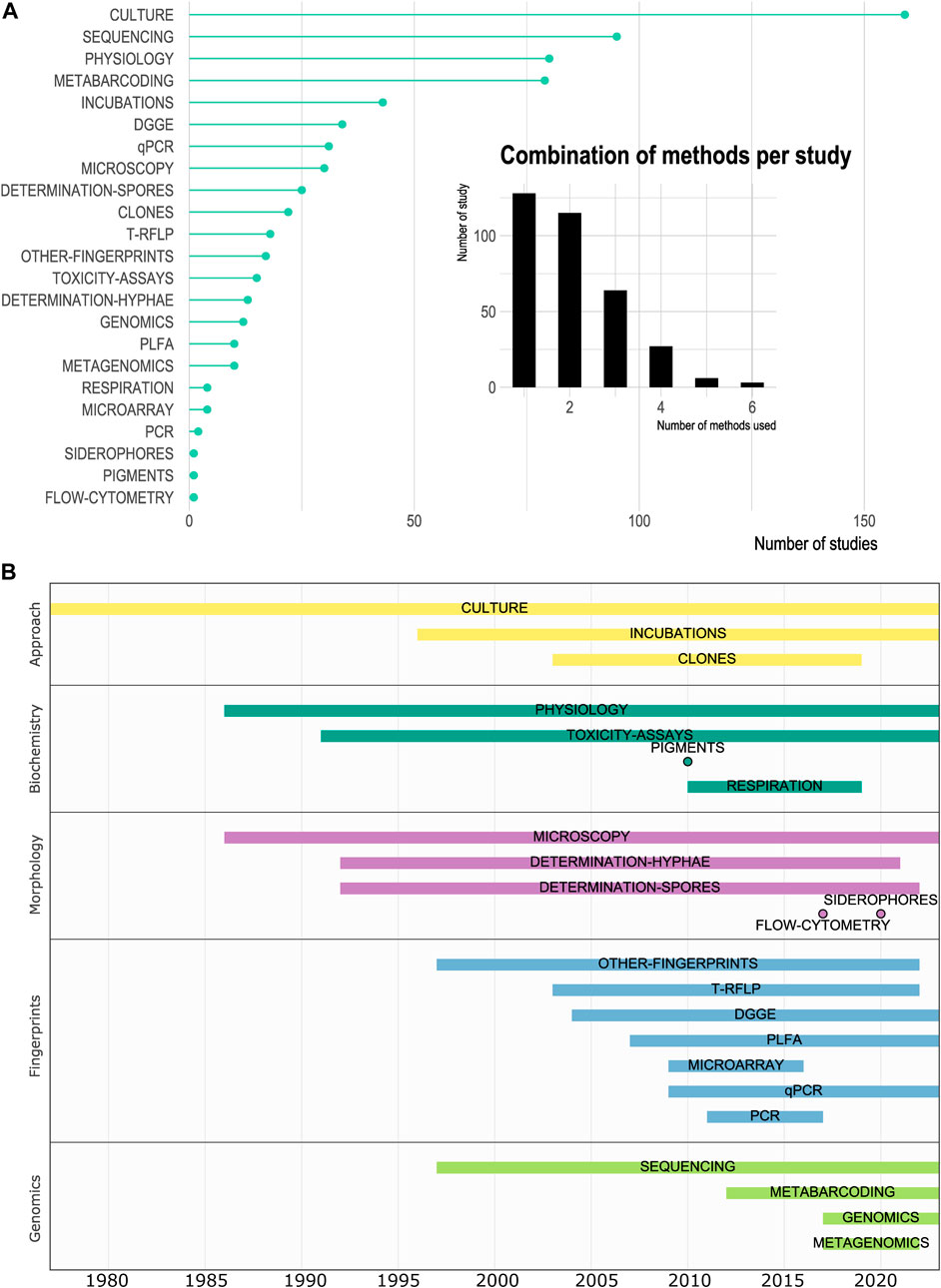
FIGURE 7. Chronological and quantitative analysis of the methods used to study microbial diversity in soils. (A). Identification and frequency of methodologies used in soil microbial diversity studies. Number of studies using at least one of the listed techniques. The inserted barplot presented the number of different methods used per study. (B). Timeline of the methodologies clustered in categories partly defined according to Hatzenpichler et al. (2020).
In 1997, we began to observe the first culture-independent studies using DNA sequencing, cloning, and amino-acid-based fingerprint techniques (Figure 7). The evolution and change of methods allowed to study new aspects of microbial communities. The metabarcoding of a target gene used as a proxy of diversity (mainly 16S rRNA gene for prokaryotes, ITS gene for fungi, and 18S rRNA gene for eukaryotes) began to appear in 2012 in our dataset. Despite the recent incorporation of metabarcoding at the national context, this is the third most used technique with a total of 79 (23%) studies, revealing a very fast spreading of this low-cost high-throughput technique providing access to the whole diversity of a community, overpassing the culture biases and limitations. Indeed, most of the data reported for archaea came from studies using metabarcoding approaches based on sequencing of the 16S rRNA genes. The improvement on sequencing technologies and the decrease of cost resulted in an increase of the use of metagenomic techniques since 2017 (both Illumina MiSeq and Oxford Minion). Metabarcoding, metagenomic or metranscriptomic data were available from the Central, Central-South and Austral regions in a total of no more than 81 sampling points. Continental Chile only accounted for 52.2% of all the sampling points (i.e., 291 sampling points) where high throughput sequencing techniques are applied (Supplementary Figure S4). In our survey, we detected 10 studies using shot-gun sequencing since 2017, with four of them published in 2022 (Figure 7). This technique provides access to the full genomic content of a community, which is useful for functional insights and reveals the metabolic potential of a community, by overpassing the PCR-based biases of metabarcoding. Hence, genomes of uncultured microorganisms can be reconstructed through the generation of MAGs) to explore new putative functions or genes (Hernández et al., 2020). Also, complete genomes of previously isolated strain can be provided (Valenzuela-Heredia et al., 2020; Núñez-Montero et al., 2023). Yet, this approach is based on community DNA, and does not specifically target active microorganisms through their RNA. Based on the publication revised in this survey, no metatranscriptomic study has been reported for the study of microbial diversity in soils from Chile. The difference in soil microbial community representation by culture-dependent and culture-independent approaches has been extensively reported (e.g., Stefani et al., 2015; Hinsu et al., 2021), even in the Chilean Andean highlands (Maza et al., 2019) resulting in the identification of some taxa overrepresented by culture-dependent techniques compared to high throughput sequencing. Also, through cultivation, Hinsu et al. (2021) showed the detection of a large proportion of amplicon sequence variants (ASVs) not retrieved by 16S rRNA gene metabarcoding, suggesting the need to associate different approaches and methodologies. Importantly, 62.7% of the studies combined two or more methodological approaches to provide a more complete picture of microbial diversity. It is interesting to note the efforts to go beyond a sole description, by coupling diversity surveys based on molecular data with functional approaches such as the measurement of diverse enzymatic activities make up 23.3% of the studies (Figure 7).
7 Future directions and opportunities to study soil microorganisms in Chile
There is a widely recognized interest for microorganisms from extreme environments due to their potential biotechnological applications such as enzyme production under extreme conditions, biomass conversion, biobased products, sustainable industrial processes, or prospection of life on extraterrestrial habitats, as reviewed in different parts of the world (e.g., Krüger et al., 2018; Kochhar et al., 2022; Schultz et al., 2023). In Chile, extremophilic microorganisms inhabiting unique environmental conditions in Chilean ecosystems have been studied, together with their potential for biotechnological applications (Azua-Bustos and González-Silva, 2014; Anguita et al., 2018; Orellana et al., 2018; Leiva-Aravena et al., 2019). Indeed, Chile offers a particularly attractive natural laboratory in this perspective due to its multiple extreme ecosystems. The great range of edaphic conditions characterized by alkaline or acidic pH, high salt and metal concentrations, wide temperature gradients, water stress, and strong UV radiation of the high-altitude deserts, salt flats, and geysers in the north of Chile (Altiplano and Atacama Desert) represent a unique microbial reservoir of life of international interest. Soils influenced by high altitude glaciers, geothermal springs, and volcanic activity in the Andes mountains in Central Chile, are subjected to a wide temperature gradient and high metal concentrations. In the southern extreme of Chile microbial life thrives in extremely low temperatures associated with ice fields, cold lakes, and fjords in Patagonia and Antarctica. Therefore, beyond the clear and urgent need to increase the number of studies focusing on soil microbial diversity in the southern hemisphere and extreme ecosystems, through our survey, we identified three main directions for future research: 1) enhance multi- and interdisciplinary collaborations, 2) increase funding support for high-throughput sequencing approaches to study soil microbial diversity in Chile, and, 3) perform long-term studies.
7.1 Collaborative multi- and interdisciplinary studies
Soil ecosystem functioning is complex and depends on the interaction of biological processes, soil physical structure, chemical reactions, and geological context, among others. Therefore, soil microbial diversity can only be fully understood through the integration with these connected disciplines. Despite some efforts to combine soil microbial diversity and geochemical data (e.g., Mandakovic et al., 2018; Aponte et al., 2022; Barret et al., 2022; Aguado-Norese et al., 2023), integrated studies are scarce. As an example, in our survey the type of soil was rarely explicitly indicated, despite the great diversity of soil orders identified in Chile (Casanova et al., 2013). In 73.8% of cases, the type of soil was not described or not identified, which hampers the possibilities of comparisons and raises the need for greater interdisciplinary collaboration among ecologists, microbiologists, and soil scientists to deeper characterize soil ecosystems as living bodies.
Even within biological sciences, interactions are rare, as shown by the low proportion of studies (16.3%) addressing biotic interactions with other components of biodiversity, despite the importance of microorganisms in soil food web both above- and below-ground feedback. Finally, social sciences and humanities are absent from our survey. As a result, the multidisciplinary wide scope journals such as Science of the Total Environment and Scientific Reports only accounted for 6 and 5 studies, respectively (Figure 2). This reinforced a lack of interdisciplinarity as the main journals chosen for publication are highly specialized. Yet, they need to be included in biodiversity studies to provide historical, sociological, or economical perspectives of soil use, as well as to integrate traditional knowledge. Future directions in soil microbial diversity studies should involve tight collaboration with different disciplines to provide a more global picture of ecosystem functioning from different perspectives. Therefore, it seems to us to integrate not only knowledge from natural science related fields but also social science in particular within the new generation of scientists and stakeholders is critical. We hence fully agree with what Timmis et al. (2020) called “the need for microbial literacy” and consider that multidisciplinary teams of microbiologists, microbial ecologists and social scientist should promote, through the collaboration, the access of this knowledge to the society (Bradshaw, 2021). This is critical if complex multi-component concerns such as climate change impact are to be addressed. A possible orientation towards this direction can be encouraged by funding criteria, with the development of interdisciplinary research calls, such as the new “Exploration Call” from Chilean ANID, aiming at contributing to the development and consolidation of disruptive, novel, high-uncertainty scientific-technological research. Finally, as revealed by our survey, the use of soil as a reservoir of microbial resources for potential biotechnological applications is of strong interest in continental Chile, especially in extreme remote regions. However, these prospective studies barely involve traditional knowledge and local communities who live in these places and benefit from ecosystem services provided by uniquely adapted microbial communities. Hence, as a perspective, we consider that a compromise between resource use and conservation should be accounted for in future research, relying on more dialogue and inclusion of local communities.
7.2 Increase and decentralize funding for high-throughput sequencing
As shown in our survey, soil microbial diversity is spatially heterogeneous across Chile, with some highly represented regions (namely, the most extreme ones) and others under-represented, such as the insular and the Austral regions. Funding should promote the development of studies in under-represented ecosystems.
On one hand, at a global scale, south-north collaborations are a way to improve funding availability and stimulate global-impact research (Baker, 2023). However, we observed in our survey that more than 30% of the soil microbial diversity studies conducted in the northern regions of Chile did not involve any Chilean institution (Supplementary Figures S2, S3), highlighting a very strong international interest in the Atacama Desert as an analog for the search for life on Mars and a lack of local collaborations. To improve our knowledge using cutting-edge techniques and propose suitable protection measures/solutions, it is necessary to maintain and strengthen south-north collaborations even between high-income countries in science. Moreover, Chile, as most Latin American countries, is considered a high-income country by the World Bank (World Bank Group, 2020) and the Organization for Economic Co-operation and Development (OECD, 2022). However, in 2020, only 0.33% of the gross domestic product (GDP) of the country was dedicated to research and development, while in Europe, on average 2.23% of the GDP support scientific research (Eurostat, 2023), ranging from 0.46% to 3.44% depending on the country.
On the other hand, at a national scale, we consider that incrementation and decentralization of funding for sequencing is urgently needed to continue supplying the universal microbial genetic archives from Chilean terrestrial ecosystems. In Chile, the FONDEQUIP (Fund for Scientific and Technological Equipment) program alone has allowed for the acquisition and implementation of 10 DNA sequencing platforms, six of which are in Santiago. At a global scale, omic observatories are flourishing, but the global south is generally less covered, and our survey demonstrates that local efforts may be integrated into global networks. For example, shotgun environmental DNA sequencing would provide valuable data combined with culture and functional inputs. Amplicon thoughput sequencing methods are now common in soil microbial studies in Chile, but our review shows that they appeared relatively late in the national context compared to worldwide studies. The same happens with metagenomics reported for the first time in 2017 in our survey, while metatranscriptomics studies are absent despite their rapid development in other parts of the world. This delay could be due to the prohibitively high costs of these techniques, resulting in the need to wait until they become more affordable as occurred previously with amplicon sequencing. This limitation could be overpassed with enhanced specific funding encouraging the use of state-of-the-art techniques.
7.3 Long term studies
Another gap that has been identified during our survey is the lack of long-term studies. As pointed out by Cavicchioli et al. (2019) long-term monitoring is needed as they allow to identify patterns between biological parameters and climatic variables or gradual changes that cannot be detected with single sampling events. As an example, Hartmann et al. (2015) demonstrated the effect of conventional versus organic agriculture on soil microbial diversity (bacteria and fungi) over 20 years of monitoring in Switzerland. Also, along a 7-year survey, Wu et al. (2022) evaluated the effect of warming temperatures on bacterial, fungal and protistan diversity and interactions in an old-field tall-grass prairie in Oklahoma (United States). In our survey, we retrieved only one long-term study, reported by Aguilera et al. (2016). It conducted a 25-year study bringing new insights about the effect of precipitation regime changes and presence of small mammals on bacterial and fungal communities in a semi-arid shrubland of the Bosque Fray (Jorge National Park). One factor that limits the realization of long-term studies is the scarcity of mid- to long-term fundings almost nonexistent in Chile. The ANID BASAL and FONDAP are the two funding tools available for research centers in Chile for 5 years projects (renewable for 5 more years). Two centers with BASAL fundings (CAPES and IEB) focus on biodiversity and ecology at the time of writing and may have the capacity to produce long-term dataset, hence filling the important gap for terrestrial microbial ecology in Chile.
8 Conclusion
Through a comprehensive review of 343 articles studying soil microbial diversity from Chile and the Antarctic Peninsula, we revealed a strong interest in applied microbiology to improve agriculture practices and the study of extreme environments. The deep analysis of the main fundings and the evolution of methodologies used to study microbial community diversity in this territory, provided recommendations for future research in this field. We emphasized the need for multi- and interdisciplinary efforts to increase knowledge on soil microbial diversity not only for academia but also to bring this to local communities and stakeholders. We suggested some future funding directions adapted to the use of cutting-edge techniques. Finally, we argue the urgent need to begin long-term studies, crucial in a global change context. Our work contributes both to global and local awareness on the importance of soil microbial biodiversity in Chile and the Antarctic Peninsula. Indeed, the geographic region we cover here, with its unique biodiversity and variety of biomes, might serve as a natural laboratory where, for example, the effect of climate anomalies on soil microbial diversity can be evaluated sooner than elsewhere.
Author contributions
CL: Conceptualization, Data curation, Writing–original draft, Formal Analysis, Funding acquisition, Investigation, Visualization. LC: Conceptualization, Data curation, Investigation, Writing–original draft, Writing–review and editing. SC-O: Conceptualization, Data curation, Investigation, Writing–original draft, Writing–review and editing, Visualization. CQ-U: Conceptualization, Data curation, Investigation, Writing–original draft. AS: Conceptualization, Data curation, Writing–review and editing. CY: Conceptualization, Data curation, Investigation, Writing–review and editing. JO: Conceptualization, Data curation, Writing–review and editing. JT: Conceptualization, Data curation, Writing–review and editing. CR: Conceptualization, Data curation, Writing–original draft, Writing–review and editing, Funding acquisition.
Funding
The author(s) declare financial support was received for the research, authorship, and/or publication of this article. CL has been supported by ANID FONDECYT 11201072 and ANID InES I + D 2021 (INID210013). S. Cuadros-Orellana acknowledges ANID FONDECYT 1201692, ANID FONDEQUIP EQM210185 and ANID FONDEF ID23I10049 support. CQ-U has been funded by ANID BECA DOCTORADO NACIONAL 21221130, FONDECYT 11170566, FONDECYT 1211977, NODO 220002 and Fondo UPLA: CNE 23-20. CR acknowledges ANID FONDECYT 11180869 and ANID PIA/BASAL FB0002. LC acknowledges support from ANID FONDECYT 1211672 and INACH RT09-12. JO and LC acknowledged support from ANID–Millennium Science Initiative Program–ICN 2021_002.
Acknowledgments
Authors particularly acknowledge the preliminary discussions with the founding members of the first Chilean Microbial Ecology Network (Red Chilena de Ecología Microbiana, RECHEM). We are also grateful to Constanza Soto for her help in the dataset generation. CL and CR also acknowledge the support frame under the project URO RED 21992 [Plan de Fortalecimiento de Universidades del Estado (CUECH)].
Conflict of interest
The authors declare that the research was conducted in the absence of any commercial or financial relationships that could be construed as a potential conflict of interest.
Publisher’s note
All claims expressed in this article are solely those of the authors and do not necessarily represent those of their affiliated organizations, or those of the publisher, the editors and the reviewers. Any product that may be evaluated in this article, or claim that may be made by its manufacturer, is not guaranteed or endorsed by the publisher.
Supplementary material
The Supplementary Material for this article can be found online at: https://www.frontiersin.org/articles/10.3389/fenvs.2024.1326158/full#supplementary-material
References
Agronomia (2023). Facultad de Ciencias Agronómicas Universidad de Chile. Historia. Available: https://agronomia.uchile.cl/facultad/la-facultad/historia (Accessed October 14, 2023).
Aguado-Norese, C., Cárdenas, V., Gaete, A., Mandakovic, D., Vasquez-Dean, J., Hodar, C., et al. (2023). Topsoil and subsoil bacterial community assemblies across different drainage conditions in a mountain environment. Biol. Res. 56 (1), 35–15. doi:10.1186/s40659-023-00445-2
Aguilar-Muñoz, P., Lavergne, C., Chamy, R., and Cabrol, L. (2022). The biotechnological potential of microbial communities from Antarctic soils and sediments: application to low temperature biogenic methane production. J. Biotechnol. 351, 38–49. doi:10.1016/j.jbiotec.2022.04.014
Aguilera, L. E., Armas, C., Cea, A. P., Gutiérrez, J. R., Meserve, P. L., and Kelt, D. A. (2016). Rainfall, microhabitat, and small mammals influence the abundance and distribution of soil microorganisms in a Chilean semi-arid shrubland. J. Arid. Environ. 126, 37–46. doi:10.1016/j.jaridenv.2015.11.013
Alcayaga, S. (1968). “Los mapas de suelos,” in Publicación técnica N°1. Series 1968 (Santiago de Chile: CORFO).
Altimira, F., Yáñez, C., Bravo, G., González, M., Rojas, L. A., and Seeger, M. (2012). Characterization of copper-resistant bacteria and bacterial communities from copper-polluted agricultural soils of central Chile. BMC Microbiol. 12, 193. doi:10.1186/1471-2180-12-193
Anguita, J. M., Rojas, C., Pastén, P. A., and Vargas, I. T. (2018). A new aerobic chemolithoautotrophic arsenic oxidizing microorganism isolated from a high Andean watershed. Biodegradation 29, 59–69. doi:10.1007/s10532-017-9813-x
ANID—Agencia Nacional de Investigación y Desarrollo (2023). Centros Basales. Available: https://anid.cl/centros-e-investigacion-asociativa/centros-basales/ (Accessed October 14, 2023).
Anthony, M. A., Bender, S. F., and van der Heijden, M. G. A. (2023). Enumerating soil biodiversity. Proc. Natl. Acad. Sci. U.S.A. 120 (33), e2304663120. doi:10.1073/pnas.2304663120
Aponte, H., Galindo-Castañeda, T., Yáñez, C., Hartmann, M., and Rojas, C. (2022). Microbial community-level physiological profiles and genetic prokaryotic structure of burned soils under Mediterranean sclerophyll forests in central Chile. Front. Microbiol. 13, 824813. doi:10.3389/fmicb.2022.824813
Averill, C., Anthony, M. A., Baldrian, P., Finkbeiner, F., van den Hoogen, J., Kiers, T., et al. (2022). Defending earth's terrestrial microbiome. Nat. Microbiol. 7 (11), 1717–1725. doi:10.1038/s41564-022-01228-3
Azua-Bustos, A., and González-Silva, C. (2014). Biotechnological applications derived from microorganisms of the Atacama Desert. BioMed Res. Int. 2014, 1–7. doi:10.1155/2014/909312
Azua-Bustos, A., González-Silva, C., and Fairén, A. G. (2022). The Atacama Desert in northern Chile as an analog model of Mars. Front. Astron. Space Sci. 8. doi:10.3389/fspas.2021.810426
Azua-Bustos, A., Urrejola, C., and Vicuña, R. (2012). Life at the dry edge: microorganisms of the Atacama Desert. FEBS Lett. 586 (18), 2939–2945. doi:10.1016/j.febslet.2012.07.025
Baker, S. (2023). North-south publishing data show Stark inequities in global research. Nature 624 (7991), S1. doi:10.1038/d41586-023-03901-x
Bardgett, R. D., and van der Putten, W. H. (2014). Belowground biodiversity and ecosystem functioning. Nature 515 (7528), 505–511. doi:10.1038/nature13855
Barret, M., Gandois, L., Thalasso, F., Martinez Cruz, K., Sepulveda Jauregui, A., Lavergne, C., et al. (2022). A combined microbial and biogeochemical dataset from high-latitude ecosystems with respect to methane cycle. Sci. Data 9 (1), 674. doi:10.1038/s41597-022-01759-8
Benavente, J. M., Crespi, G., Figal Garone, L., and Maffioli, A. (2012). The impact of national research funds: a regression discontinuity approach to the Chilean FONDECYT. Res. Policy 41 (8), 1461–1475. doi:10.1016/j.respol.2012.04.007
Bhattacharyya, P. N., and Jha, D. K. (2012). Plant growth-promoting rhizobacteria (PGPR): emergence in agriculture. World J. Microbiol. Biotechnol. 28 (4), 1327–1350. doi:10.1007/s11274-011-0979-9
Bradshaw, A. (2021). Microbiological literacy and the role of social science: a response to Timmis et al. Environ. Microbiol. 23 (11), 6350–6354. doi:10.1111/1462-2920.15808
Bruna, N., Galliani, E., Oyarzún, P., Bravo, D., Fuentes, F., and Pérez-Donoso, J. M. (2022). Biomineralization of lithium nanoparticles by Li-resistant Pseudomonas rodhesiae isolated from the Atacama salt flat. Biol. Res. 55 (1), 12. doi:10.1186/s40659-022-00382-6
Bull, A. T., Asenjo, J. A., Goodfellow, M., and Gómez-Silva, B. (2016). The Atacama Desert: technical resources and the growing importance of novel microbial diversity. Annu. Rev. Microbiol. 70, 215–234. doi:10.1146/annurev-micro-102215-095236
Bustamante, M., Verdejo, V., Zúñiga, C., Espinosa, F., Orlando, J., and Carú, M. (2012). Comparison of water availability effect on ammonia-oxidizing bacteria and archaea in microcosms of a Chilean semiarid soil. Front. Microbiol. 3, 282. doi:10.3389/fmicb.2012.00282
Cabrera, M. Á., and Blamey, J. M. (2018). Biotechnological applications of archaeal enzymes from extreme environments. Biol. Res. 51, 37. doi:10.1186/s40659-018-0186-3
Cameron, R. E., Gensel, D. R., and Blank, C. B. (1966). “Soil studies—desert microflora. Xii. Abundance of microflora in soil samples from the Chile Atacama Desert,” in Supporting research and advanced developments, space programs summary no. 37–38 (Pasadena, CA: Jet Propulsion Lab, NASA), 140–147.
Cardenas, L. M., Hatch, D. J., Scholefield, D., Jhurreea, D., Clark, I. M., Hirsch, P. R., et al. (2013). Potential mineralization and nitrification in volcanic grassland soils in Chile. Soil Sci. Plant Nutr. 59 (3), 380–391. doi:10.1080/00380768.2013.789395
Caretta, G., and Piontelli, E. (1977). Microsporum magellanicum and Cunninghamella Antarctica, new species isolated from Australia and Antarctic soil of Chile. Sabouraudia 15 (1), 1–10. doi:10.1080/00362177785190021
Carro, L., Razmilic, V., Nouioui, I., Richardson, L., Pan, C., Golinska, P., et al. (2018). Hunting for cultivable Micromonospora strains in soils of the Atacama Desert. Antonie Leeuwenhoek 111 (8), 1375–1387. doi:10.1007/s10482-018-1049-1
Carvajal, M., Jeldres, P., Vergara, A., Lobaina, E., Olivares, M., Meza, D., et al. (2023). Bioremoval of copper by filamentous fungi isolated from contaminated soils of Puchuncaví-Ventanas Central Chile. Environ. Geochem. Health 45 (7), 4275–4293. doi:10.1007/s10653-023-01493-z
Casanova, M., Salazar, O., Seguel, O., and Luzio, W. (2013). The soils of Chile. Dordrecht, Netherlands: Springer.
Cavicchioli, R., Ripple, W. J., Timmis, K. N., Azam, F., Bakken, L. R., Baylis, M., et al. (2019). Scientists' warning to humanity: microorganisms and climate change. Nat. Rev. Microbiol. 17 (9), 569–586. doi:10.1038/s41579-019-0222-5
CEPAL—Comisión Económica para América Latina (1953). Investigación tecnológica y formación de técnicos en América Latina. Estudio preliminar de prueba referente a Chile preparado por la Secretaría Ejecutiva con la cooperación de un grupo de expertos. Rio de Janeiro: Naciones Unidas.
Contador, C. A., Veas-Castillo, L., Tapia, E., Antipan, M., Miranda, N., Ruiz-Tagle, B., et al. (2020). Atacama database: a platform of the microbiome of the Atacama Desert. Antonie Leeuwenhoek 113 (2), 185–195. doi:10.1007/s10482-019-01328-x
Delgado-Baquerizo, M., Maestre, F. T., Reich, P. B., Jeffries, T. C., Gaitan, J. J., Encinar, D., et al. (2016). Microbial diversity drives multifunctionality in terrestrial ecosystems. Nat. Commun. 7, 10541. doi:10.1038/ncomms10541
di Castri, F., and Mooney, H. (1973). Mediterranean type ecosystems, ecological studies 7. Berlin, Germany: Springer-Verlag.
Dinamarca, D. I., Galleguillos, M., Seguel, O., and Faúndez Urbina, C. (2023). CLSoilMaps: a national soil gridded database of physical and hydraulic soil properties for Chile. Sci. Data 10 (1), 630. doi:10.1038/s41597-023-02536-x
Dose, K., Bieger-Dose, A., Ernst, B., Feister, U., Gómez-Silva, B., Klein, A., et al. (2001). Survival of microorganisms under the extreme conditions of the Atacama Desert. Orig. Life Evol. Biosph. 31 (3), 287–303. doi:10.1023/a:1010788829265
Espina, G., Muñoz-Ibacache, S. A., Cáceres-Moreno, P., Amenabar, M. J., and Blamey, J. M. (2022). From the discovery of Extremozymes to an enzymatic product: roadmap based on their applications. Front. Bioeng. Biotechnol. 9, 752281. doi:10.3389/fbioe.2021.752281
Eurostat (2023). Gross domestic expenditure on research and development 2022. Statistic Explained. Available: https://ec.europa.eu/eurostat/statistics-explained/index.php?title=R%26D_expenditure&oldid=551418#Gross_domestic_expenditure_on_R.26D (Accessed December 21, 2023).
FAO (2020). State of knowledge of soil biodiversity – status, challenges and potentialities, summary for policymakers. Rome: FAO.
Fernández-Martínez, M. A., Pérez-Ortega, S., Pointing, S. B., Allan Green, T. G., Pintado, A., Rozzi, R., et al. (2017). Microbial succession dynamics along glacier forefield chronosequences in Tierra del Fuego (Chile). Polar Biol. 40 (10), 1939–1957. doi:10.1007/s00300-017-2110-7
Fierer, N. (2017). Embracing the unknown: disentangling the complexities of the soil microbiome. Nat. Rev. Microbiol. 15 (10), 579–590. doi:10.1038/nrmicro.2017.87
Fuentes, A., Herrera, H., Charles, T. C., and Arriagada, C. (2020). Fungal and bacterial microbiome associated with the rhizosphere of native plants from the Atacama Desert. Microorganisms 8 (2), 209. doi:10.3390/microorganisms8020209
Fulthorpe, R. R., Rhodes, A. N., and Tiedje, J. M. (1996). Pristine soils mineralize 3-chlorobenzoate and 2,4-dichlorophenoxyacetate via different microbial populations. Appl. Environ. Microbiol. 62 (4), 1159–1166. doi:10.1128/aem.62.4.1159-1166.1996
Gran-Scheuch, A., Ramos-Zúñiga, J., Fuentes, E., Bravo, D., and Pérez-Donoso, J. M. (2020). Effect of co-contamination by PAHs and heavy metals on bacterial communities of diesel contaminated soils of South Shetland Islands, Antarctica. Microorganisms 8 (11), 1749. doi:10.3390/microorganisms8111749
Guerra, C. A., Heintz-Buschart, A., Sikorski, J., Chatzinotas, A., Guerrero-Ramírez, N., Cesarz, S., et al. (2020). Blind spots in global soil biodiversity and ecosystem function research. Nat. Commun. 11 (1), 3870. doi:10.1038/s41467-020-17688-2
Han, J., Jung, J., Park, M., Hyun, S., and Park, W. (2013). Short-term effect of elevated temperature on the abundance and diversity of bacterial and archaeal amoA genes in Antarctic soils. J. Microbiol. Biotechnol. 23 (9), 1187–1196. doi:10.4014/jmb.1305.05017
Hartley, A. J., Chong, G., Houston, J., and Mather, A. E. (2022). 150 million years of climatic stability: evidence from the Atacama Desert, northern Chile. J. Geol. Soc. 162 (3), 421–424. doi:10.1144/0016-764904-071
Hartmann, M., Frey, B., Mayer, J., Mader, P., and Widmer, F. (2015). Distinct soil microbial diversity under long-term organic and conventional farming. ISME J. 9 (5), 1177–1194. doi:10.1038/ismej.2014.210
Hartmann, M., and Six, J. (2022). Soil structure and microbiome functions in agroecosystems. Nat. Rev. Earth Environ. 4 (1), 4–18. doi:10.1038/s43017-022-00366-w
Hatzenpichler, R., Krukenberg, V., Spietz, R. L., and Jay, Z. J. (2020). Next-generation physiology approaches to study microbiome function at single cell level. Nat. Rev. Microbiol. 18 (4), 241–256. doi:10.1038/s41579-020-0323-1
Hernández, M., Vera-Gargallo, B., Calabi-Floody, M., King, G. M., Conrad, R., and Tebbe, C. C. (2020). Reconstructing genomes of carbon monoxide oxidisers in volcanic deposits including members of the class Ktedonobacteria. Microorganisms 8 (12), 1880. doi:10.3390/microorganisms8121880
Hinsu, A., Dumadiya, A., Joshi, A., Kotadiya, R., Andharia, K., Koringa, P., et al. (2021). To culture or not to culture: a snapshot of culture-dependent and culture-independent bacterial diversity from peanut rhizosphere. PeerJ 9, e12035. doi:10.7717/peerj.12035
Idris, H., Goodfellow, M., Sanderson, R., Asenjo, J. A., and Bull, A. T. (2017). Actinobacterial rare biospheres and dark matter revealed in habitats of the Chilean Atacama Desert. Sci. Rep. 7 (1), 8373. doi:10.1038/s41598-017-08937-4
Jansson, J. K., and Hofmockel, K. S. (2018). The soil microbiome-from metagenomics to metaphenomics. Curr. Opin. Microbiol. 43, 162–168. doi:10.1016/j.mib.2018.01.013
Jorquera, M. A., Inostroza, N. G., Lagos, L. M., Barra, P. J., Marileo, L. G., Rilling, J. I., et al. (2014). Bacterial community structure and detection of putative plant growth-promoting rhizobacteria associated with plants grown in Chilean agro-ecosystems and undisturbed ecosystems. Biol. Fertil. Soils 50 (7), 1141–1153. doi:10.1007/s00374-014-0935-6
Jung, T., Scanu, B., Bakonyi, J., Seress, D., Kovacs, G. M., Duran, A., et al. (2017). Nothophytophthora gen. nov., a new sister genus of Phytophthora from natural and semi-natural ecosystems. Persoonia 39, 143–174. doi:10.3767/persoonia.2017.39.07
Knief, C., Bol, R., Amelung, W., Kusch, S., Frindte, K., Eckmeier, E., et al. (2020). Tracing elevational changes in microbial life and organic carbon sources in soils of the Atacama Desert. Glob. Planet. Change 184, 103078. doi:10.1016/j.gloplacha.2019.103078
Kochhar, N., Shrivastava, S., Ghosh, A., Rawat, V. S., Sodhi, K. K., Kumar, M., et al. (2022). Perspectives on the microorganism of extreme environments and their applications. Curr. Res. Microb. Sci. 3, 100134. doi:10.1016/j.crmicr.2022.100134
Krüger, A., Schäfers, C., Schröder, C., and Antranikian, G. (2018). Towards a sustainable biobased industry–highlighting the impact of extremophiles. New Biotechnol. 40, 144–153. doi:10.1016/j.nbt.2017.05.002
Leiva-Aravena, E., Leiva, E., Zamorano, V., Rojas, C., Regan, J. M., and Vargas, I. T. (2019). Organotrophic acid-tolerant microorganisms enriched from an acid mine drainage affected environment as inoculum for microbial fuel cells. Sci. Total Environ. 678, 639–646. doi:10.1016/j.scitotenv.2019.05.003
Luzio, W., and Alcayaga, S. (1992). Mapa de asociaciones de grandes grupos de suelos de Chile. Agric. Téc. 52 (4), 347–353.
Maas, B., Pakeman, R. J., Godet, L., Smith, L., Devictor, V., and Primack, R. (2021). Women and global south strikingly underrepresented among top-publishing ecologists. Conserv. Lett. 14 (4). doi:10.1111/conl.12797
Machuca, A., Pereira, G., Aguiar, A., and Milagres, A. M. (2007). Metal-chelating compounds produced by ectomycorrhizal fungi collected from pine plantations. Lett. Appl. Microbiol. 44 (1), 7–12. doi:10.1111/j.1472-765X.2006.02046.x
Mandakovic, D., Rojas, C., Maldonado, J., Latorre, M., Travisany, D., Delage, E., et al. (2018). Structure and co-occurrence patterns in microbial communities under acute environmental stress reveal ecological factors fostering resilience. Sci. Rep. 8 (1), 5875. doi:10.1038/s41598-018-23931-0
Marín, C., Aguilera, P., Oehl, F., and Godoy, R. (2017). Factors affecting arbuscular mycorrhizal fungi of Chilean temperate rainforests. J. Soil Sci. Plant Nutr. 17 (4), 966–984. doi:10.4067/s0718-95162017000400010
Marín, C., Rubio, J., and Godoy, R. (2022). Chilean blind spots in soil biodiversity and ecosystem function research. Austral Ecol. 47 (7), 1372–1381. doi:10.1111/aec.13232
Martins, Z., Cottin, H., Kotler, J. M., Carrasco, N., Cockell, C. S., de la Torre Noetzel, R., et al. (2017). Earth as a tool for astrobiology—a European perspective. Space Sci. Rev. 209 (1-4), 43–81. doi:10.1007/s11214-017-0369-1
Maza, F., Maldonado, J., Vásquez-Dean, J., Mandakovic, D., Gaete, A., Cambiazo, V., et al. (2019). Soil bacterial communities from the Chilean Andean highlands: taxonomic composition and culturability. Front. Bioeng. Biotechnol. 7, 10. doi:10.3389/fbioe.2019.00010
McKay, C. P., Friedmann, E. I., Gómez-Silva, B., Cáceres-Villanueva, L., Andersen, D. T., and Landheim, R. (2003). Temperature and moisture conditions for life in the extreme arid region of the Atacama desert: four years of observations including the el niño of 1997-1998. Astrobiology 3 (2), 393–406. doi:10.1089/153110703769016460
Meza, C., Valenzuela, F., Echeverría-Vega, A., Gomez, A., Sarkar, S., Cabeza, R. A., et al. (2022). Plant-growth-promoting bacteria from rhizosphere of Chilean common bean ecotype (Phaseolus vulgaris L.) supporting seed germination and growth against salinity stress. Front. Plant. Sci. 13, 1052263. doi:10.3389/fpls.2022.1052263
Ministerio de Ciencia Tecnología Conocimiento e Innovación (2023). Iniciativa científica Milenio. Available: https://www.iniciativamilenio.cl/institutos-y-nucleos-milenio [Accessed 14 October 2023].
Ministerio de Educación - Chile (2018). Ley 21105, de 13 de agosto de 2018. Crea el Ministerio de Ciencia, Tecnología, Conocimiento e Innovación. Available: https://www.bcn.cl/leychile/navegar?idNorma=1121682 [Accessed 5 September 2023].
Ministerio del Medio Ambiente - Chile (2018). “Biodiversidad de Chile,” in Patrimonio y desafíos (Santiago de Chile: Ministerio del Medio Ambiente - Chile), 430.
Ministerio de Relaciones Exteriores - Chile (1966). Ley 16592, de 21 de diciembre de 1966. Crea Dirección de Fronteras y Límites del Estado. Available: https://www.bcn.cl/leychile/navegar?idNorma=28558 (Accessed October 18, 2023).
Navarro-González, R., Rainey, F. A., Molina, P., Bagaley, D. R., Hollen, B. J., de la Rosa, J., et al. (2003). Mars-like soils in the Atacama Desert, Chile, and the dry limit of microbial life. Science 302 (5647), 1018–1021. doi:10.1126/science.1089143
Nazer Ahumada, R. (2016). La Corporación de Fomento a la Producción y la modernización económica de Chile. 1939-1970. Rev. Gest. Pública 5 (2), 283–316. doi:10.22370/rgp.2016.5.2.2223
Neilson, J. W., Califf, K., Cardona, C., Copeland, A., van Treuren, W., Josephson, K. L., et al. (2017). Significant impacts of increasing aridity on the arid soil microbiome. mSystems 2 (3), e00195-16. doi:10.1128/mSystems.00195-16
Nielsen, M. W., Alegria, S., Börjeson, L., Etzkowitz, H., Falk-Krzesinski, H. J., Joshi, A., et al. (2017). Opinion: gender diversity leads to better science. Proc. Natl. Acad. Sci. U.S.A. 114 (8), 1740–1742. doi:10.1073/pnas.1700616114
Nuñez, M. A., Barlow, J., Cadotte, M., Lucas, K., Newton, E., Pettorelli, N., et al. (2019). Assessing the uneven global distribution of readership, submissions and publications in applied ecology: obvious problems without obvious solutions. J. Appl. Ecol. 56 (1), 4–9. doi:10.1111/1365-2664.13319
Núñez-Montero, K., Rojas-Villalta, D., Hernández-Moncada, R., Esquivel, A., and Barrientos, L. (2023). Genome sequence of Pseudomonas sp. strain So3.2b, isolated from a soil sample from robert island (antarctic specially protected area 112), antarctic. Antarct. Microbiol. Resour. Announce. 12 (3), e0116722. doi:10.1128/mra.01167-22
ODEPA—Oficina de Estudios y Políticas Agrarias (2019). Panorama de la agricultura chilena. Santiago, Chile: Ministerio de Agricultura. ODEPA.
OECD (2022). OECD economic surveys: Chile. https://www.oecd-ilibrary.org/economics/oecd-economic-surveys-chile_19990847.
Okoro, C. K., Brown, R., Jones, A. L., Andrews, B. A., Asenjo, J. A., Goodfellow, M., et al. (2009). Diversity of culturable actinomycetes in hyper-arid soils of the Atacama Desert, Chile. Antonie Leeuwenhoek 95 (2), 121–133. doi:10.1007/s10482-008-9295-2
Orellana, R., Macaya, C., Bravo, G., Dorochesi, F., Cumsille, A., Valencia, R., et al. (2018). Living at the frontiers of life: extremophiles in Chile and their potential for bioremediation. Front. Microbiol. 9, 2309. doi:10.3389/fmicb.2018.02309
Osorio, C. (2015). Historia de la ensenanza de la microbiologia en Chile: Centros formadores. Rev. Chil. Infectol. 32 (4), 447–452. doi:10.4067/S0716-10182015000500012
Osorio, C. G. (2010). The history of microbiology in Chile: about the origin of experimental bacteriology. Rev. Med. Chile 138 (7), 913–919. doi:10.4067/s0034-98872010000700019
Paulino-Lima, I. G., Azua-Bustos, A., Vicuña, R., González-Silva, C., Salas, L., Teixeira, L., et al. (2013). Isolation of UVC-tolerant bacteria from the hyperarid Atacama Desert, Chile. Microb. Ecol. 65 (2), 325–335. doi:10.1007/s00248-012-0121-z
Pavón, A., Corsini, G., Orellana, P., Calisto, N., Navarro, L., Wiese, G., et al. (2023). Identification of Antarctic soil bacteria exhibiting antiproliferative activity against a colon cancer cell line. Int. J. Morphol. 41 (1), 286–296. doi:10.4067/s0717-95022023000100286
Pfeiffer, M., Padarian, J., Osorio, R., Bustamante, N., Olmedo, G. F., Guevara, M., et al. (2020). CHLSOC: the Chilean soil organic carbon database, a multi-institutional collaborative effort. Earth Syst. Sci. Data 12 (1), 457–468. doi:10.5194/essd-12-457-2020
Prado, B., del Moral, A., and Campos, V. (1993). Distribution and types of heterotrophyc halophilic flora from Salar de Atacama, Chile. Toxicol. Environ. Chem. 38 (3-4), 163–166. doi:10.1080/02772249309357887
Prado, B., Del Moral, A., Quesada, E., Ríos, R., Monteoliva-Sanchez, M., Campos, V., et al. (1991). Numerical taxonomy of moderately halophilic Gram-negative rods isolated from the Salar de Atacama, Chile. Syst. Appl. Microbiol. 14 (3), 275–281. doi:10.1016/s0723-2020(11)80381-4
Pulschen, A. A., Rodrigues, F., Duarte, R. T., Araujo, G. G., Santiago, I. F., Paulino-Lima, I. G., et al. (2015). UV-resistant yeasts isolated from a high-altitude volcanic area on the Atacama Desert as eukaryotic models for astrobiology. MicrobiologyOpen 4 (4), 574–588. doi:10.1002/mbo3.262
Quesada, C. F. (2016). Institucionalización científica y financiamiento filantrópico. El Convenio Universidad de Chile-Universidad de California y la Fundación Ford (1965-1975). Fronteras 3 (1), 125–148.
Ramírez, P. B., Fuentes-Alburquenque, S., Díez, B., Vargas, I., and Bonilla, C. A. (2020). Soil microbial community responses to labile organic carbon fractions in relation to soil type and land use along a climate gradient. Soil Biol. biochem. 141, 107692. doi:10.1016/j.soilbio.2019.107692
R Core Team (2021). R: a language and environment for statistical computing. Vienna, Austria: R Foundation for Statistical Computing.
Renny, M., Acosta, M. C., Cofré, N., Domínguez, L. S., Bidartondo, M. I., and Sersic, A. N. (2017). Genetic diversity patterns of arbuscular mycorrhizal fungi associated with the mycoheterotroph Arachnitis uniflora Phil. (Corsiaceae). Phil. (Corsiaceae). Ann. Bot. 119 (8), 1279–1294. doi:10.1093/aob/mcx023
Rodríguez, M. (1992). La microbiología en Chile: su desarrollo a la luz de un siglo de existencia. Rev. Med. Chile 120, 463–470.
Rodríguez-Echeverría, S., Delgado-Baquerizo, M., Morillo, J. A., Gaxiola, A., Manzano, M., Marquet, P. A., et al. (2021). Azorella cushion plants and aridity are important drivers of soil microbial communities in Andean ecosystems. Ecosystems 24 (7), 1576–1590. doi:10.1007/s10021-021-00603-1
Santos, A., Bruna, P., Martinez-Urtaza, J., Solís, F., Valenzuela, B., Zamorano, P., et al. (2021). Two archaeal metagenome-assembled genomes from El Tatio provide new insights into the Crenarchaeota phylum. Genes 12 (3), 391. doi:10.3390/genes12030391
Satoh, Y., Yoshimura, K., Pokhrel, Y., Kim, H., Shiogama, H., Yokohata, T., et al. (2022). The timing of unprecedented hydrological drought under climate change. Nat. Commun. 13 (1), 3287. doi:10.1038/s41467-022-30729-2
Schaefer, P. (1973). “Microbial activity under seasonal conditions of drought in Mediterranean climates,” in Mediterranean type ecosystems, ecological studies 7. Editors F. Di Castri, and H. Mooney (Berlin, Germany: Springer-Verlag), 191–198.
Scherson, R. A., Thornhill, A. H., Urbina-Casanova, R., Freyman, W. A., Pliscoff, P. A., and Mishler, B. D. (2017). Spatial phylogenetics of the vascular flora of Chile. Mol. Phylogenet. Evol. 112, 88–95. doi:10.1016/j.ympev.2017.04.021
Schultz, J., dos Santos, A., Patel, N., and Rosado, A. S. (2023). Life on the edge: bioprospecting extremophiles for astrobiology. J. Indian Inst. Sci. 103, 721–737. doi:10.1007/s41745-023-00382-9
Seeger, M., and Espinoza, F. (2008). Highlights of Latin American microbiology: the 19th ALAM congress. Int. Microbiol. 11, 289–292. doi:10.2436/20.1501.01.74
Serrano, J., and Leiva, E. (2017). Removal of arsenic using acid/metal-tolerant sulfate reducing bacteria: a new approach for bioremediation of high-arsenic acid mine waters. Water 9 (12), 994. doi:10.3390/w9120994
Stchigel, A. M., Calduch, M., Guarro, J., and Zaror, L. (2002). A new species of Podospora from soil in Chile. Mycologia 94 (3), 554–558. doi:10.1080/15572536.2003.11833221
Stefani, F. O., Bell, T. H., Marchand, C., De La Providencia, I. E., El Yassimi, A., St-Arnaud, M., et al. (2015). Culture-dependent and-independent methods capture different microbial community fractions in hydrocarbon-contaminated soils. PloS one 10 (6), e0128272. doi:10.1371/journal.pone.0128272
Timmis, K., Timmis, J., and Jebok, F. (2020). The urgent need for microbiology literacy in society: children as educators. Microb. Biotechnol. 13 (5), 1300–1303. doi:10.1111/1751-7915.13619
Valenzuela-Heredia, D., Henríquez-Castillo, C., Donoso, R., Lavín, P., Pavlov, M. S., Franchi, O., et al. (2020). Complete genome sequence of Pseudomonas chilensis strain ABC1, isolated from soil. Microbiol. Resour. Announce. 9 (39), e00775-20. doi:10.1128/MRA.00775-20
Vázquez, S., Monien, P., Pepino Minetti, R., Jürgens, J., Curtosi, A., Villalba Primitz, J., et al. (2017). Bacterial communities and chemical parameters in soils and coastal sediments in response to diesel spills at Carlini Station, Antarctica. Sci. Total Environ. 605-606, 26–37. doi:10.1016/j.scitotenv.2017.06.129
Villagrán, C., and Armesto, J. J. (2005). “Fitogeografía histórica de la cordilera de la costa de Chile,” in Historia, biogeografía y ecología de los bosques costeros de Chile. Editors C. Smith-Ramírez, J. J. Armesto, and C. Valdovinos (Santiago: Editorial Universitaria), 99–115.
Villagrán, C., and Hinojosa, F. (1997). Historia de los bosques del sur de Sudamérica, II: análisis fitogeográfico. Rev. Chil. Hist. Nat. 70, 241–267.
D. H. Wall, R. D. Bardgett, V. Behan-Pelletier, J. E. Herrick, T. H. Jones, K. Ritzet al. (2012). Soil ecology and ecosystem services (New York, United States of America: Oxford University Press).
World Bank Group (2020). Economy profile of Chile. Available: https://Archive.Doingbusiness.Org/En/Data/Exploreeconomies/Chile (Accessed December 21, 2023).
Wu, L., Zhang, Y., Guo, X., Ning, D., Zhou, X., Feng, J., et al. (2022). Reduction of microbial diversity in grassland soil is driven by long-term climate warming. Nat. Microbiol. 7 (7), 1054–1062. doi:10.1038/s41564-022-01147-3
Keywords: global south, biogeography, microbial ecology, biodiversity, soil diversity, Chile, bibliometrics
Citation: Lavergne C, Cabrol L, Cuadros-Orellana S, Quinteros-Urquieta C, Stoll A, Yáñez C, Tapia J, Orlando J and Rojas C (2024) Rising awareness to improve conservation of microorganisms in terrestrial ecosystems: advances and future directions in soil microbial diversity from Chile and the Antarctic Peninsula. Front. Environ. Sci. 12:1326158. doi: 10.3389/fenvs.2024.1326158
Received: 22 October 2023; Accepted: 29 January 2024;
Published: 15 February 2024.
Edited by:
Marta Sebastian, Institut de Ciències del Mar, CSIC, SpainReviewed by:
Antti Juhani Rissanen, Tampere University, FinlandYi Zhai, Berkeley Lab (DOE), United States
Copyright © 2024 Lavergne, Cabrol, Cuadros-Orellana, Quinteros-Urquieta, Stoll, Yáñez, Tapia, Orlando and Rojas. This is an open-access article distributed under the terms of the Creative Commons Attribution License (CC BY). The use, distribution or reproduction in other forums is permitted, provided the original author(s) and the copyright owner(s) are credited and that the original publication in this journal is cited, in accordance with accepted academic practice. No use, distribution or reproduction is permitted which does not comply with these terms.
*Correspondence: Claudia Rojas, claudia.rojas@uoh.cl