- 1Department of Natural Resources, Agricultural Research Organization Volcani Center, Institute of Plant Sciences, Rishon Le Tzion, Israel
- 2Division of Biological Sciences, University of Montana, Missoula, MT, United States
- 3Department of Entomology, Cornell University, Ithaca, NY, United States
- 4Plant-Microorganism Interaction Unit, Institute of Natural Resources and Agrobiology of Salamanca (IRNASA-CSIC), Salamanca, Spain
Accumulating evidence supports the importance of belowground interactions for plant performance, ecosystem functioning, and conservation biology. However, studying species interactions belowground has unique challenges relative to the aboveground realm. The structure of the media and spatial scale are among the key aspects that seem to strongly influence belowground interactions. As a consequence, our understanding of species interactions belowground is limited, at least compared to what is known about interactions aboveground. Here we address the general question: Do the ecological concepts that have been developed largely in aboveground systems apply to understanding species interactions in the rhizosphere? We first explore to what extent ecological concepts related to species interactions are considered in rhizosphere studies across various subdisciplines. Next, we explore differences and similarities above- and belowground for fundamental concepts in ecology, choosing topics that are underrepresented in rhizosphere studies but represent a swath of concepts: species diversity, island biogeography, self-organization and ecosystem engineering, trophic cascades, and chemical communication. Finally, we highlight to overcome major challenges of current methodologies to study rhizosphere interactions in order to advance the understanding of belowground interactions in an ecological context. By synthesizing literature related to rhizosphere interactions, we reveal similarities, as well as key differences, in how fundamental ecological concepts are used and tested in above- and belowground studies. Closing the knowledge gaps identified in our synthesis will promote a deeper understanding of the differences above- and belowground and ultimately lead to integration of these concepts.
Introduction
There has been a recent surge in studying species interactions that occur in the rhizospheres of plants (Brussaard, 1997; Hooper et al., 2000; Wardle et al., 2004; Orgiazzi et al., 2016). This surge has opened doors to new and exciting research questions related to how species interactions in the rhizosphere may influence various processes both above- and belowground, that integrate across many subdisciplines in ecology. For example, a recent list of 100 fundamental ecological questions includes several questions on how above- and belowground biodiversity interact and how biotic and abiotic feedbacks between plants and the soil influence plant growth (Sutherland et al., 2013). However, while substantial progress has been made in understanding both above- and belowground ecological processes as well as above-belowground linkages (e.g., Wardle et al., 2004; Lavelle et al., 2016; Coleman et al., 2018), the development of ecological concepts in above- vs. belowground systems have been somewhat disparate (Nobis and Wohlgemuth, 2004; Barot et al., 2007). The disconnect between concepts used in above- and belowground realms is perhaps because these realms differ in many important ways. The scale at which interactions operate between organisms belowground is often much smaller than in aboveground systems. Vertebrate herbivores may explore areas exceeding the size of 1 ha within a day, whereas most soil organisms do not explore more than 1 m2 in their life time (van Der Putten et al., 2016). A further key difference lays in the provision of nutrients and energy, although root exudates provide a significant link of energy flow between the realms (Bais et al., 2006). Nevertheless, belowground, energy flows primarily through the detritus cycle rather than primary production on the terrestrial surface. Moreover, the soil medium differs from the aboveground environment, being a complex and heterogeneous matrix of interconnected spaces. Belowground interactions occur in darkness, where temperature fluctuations are low, relative humidity and CO2 levels are high (Russell and Appleyard, 1915). Such specific characteristics belowground dictates dynamics of organism movement and sensory perception specific to the soil medium. For instance, arthropods adapted to live in deep soil layers show reduced body size, or loss of sight and flight capacity (Andújar et al., 2017). To summarize, fundamental differences between the above- and belowground realms suggest that patterns of interactions above- and belowground are, at least partially, governed by different mechanisms. Reconciling these differences in key ecological processes is critical to developing predictive theory and understanding responses to environmental changes (Wardle et al., 2004; Sutherland et al., 2013).
In this perspective, we address the general question whether ecological concepts that have been developed largely in aboveground systems apply to understanding species interactions in rhizospheres. To answer this question we first performed a literature search to evaluate how widely ecological concepts related to studying species interactions are used in studying belowground interactions. We looked at frequencies of studies related to ecological concepts in general ecology journals compared to sub-discipline journals. Next, we discuss several of the understudied concepts, focusing on concepts that are likely to function fundamentally different in above- and belowground realms. Finally, we outline challenges in studying rhizosphere interactions and some potential solutions. An improved understanding of how ecological concepts are used below- and aboveground should therefore improve research progress of rhizosphere interactions and ultimately enhance understanding of above-belowground linkages.
Addressing the Use of Ecological Concepts in Subdisciplines Related to Rhizosphere Interactions
To examine how widely ecological concepts related to studying species interactions in general ecology vs. sub-discipline journals, we focus on recent usage of ecological concepts in the literature by searching for articles published in the last two decades. We examined general ecology journals and four other subdisciplines related to rhizosphere interactions that cover ecological topics (Supplementary Material 1): soil science, botany, entomology, and microbial ecology. Within each of these five categories, we searched for keywords that represent fundamental concepts in ecology (see Figure 1 and Supplementary Material 1). While our list of key concepts is not exhaustive, it is representative in that it covers topics dealing with species interactions in population, community, landscape, and ecosystem processes. We also included “soil” or “rhizosphere” and “species” to include only articles that were most likely to investigate rhizosphere interactions. Differences between the proportion of articles published in each subdiscipline vs. general ecology journals were tested using proportion tests, corrected for family-wise error rates (Newcombe, 1998).
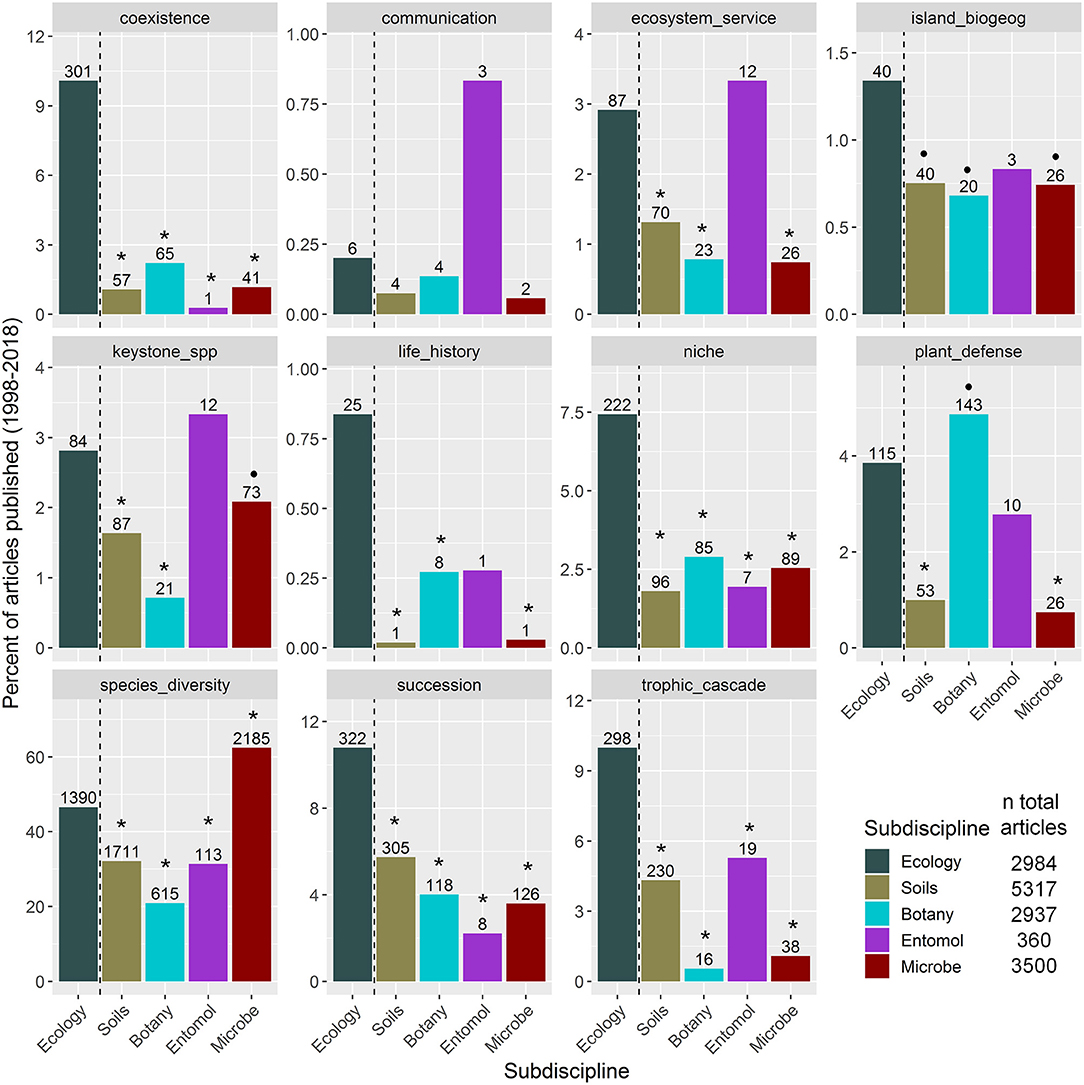
Figure 1. Results of a literature search (1998–2018) for how frequently ecological concept keywords appear in articles that investigated rhizosphere interactions published in general ecology journals by subdiscipline journals. Bars represent the percent of articles related to each concept by subdiscipline. Numbers above the bars indicate the number of articles studying rhizosphere interactions within that subdiscipline that reference the concept, while the total number of articles that investigated rhizosphere interactions per subdiscipline is listed in the key. Subdisciplines that significantly differed in the percent of articles published compared to ecology journals based on pairwise proportion tests, corrected for family-wise error rates, are indicated as follows: •P < 0.1; *P < 0.05.
As we expected, most ecological keywords were more highly referenced within ecology journals compared to other subdisciplines (Figure 1). Rarely, some of the terms were better represented in the sub-discipline journals. For example, compared to their use in ecology journals, “plant defense” is better referenced in the botany literature and ‘species diversity’ is better referenced in the microbial ecology literature. Some concepts also appear to not be well studied in the rhizosphere in any subdiscipline, such as “communication” or “island biogeography”. Nevertheless, we found that most of the concepts were underrepresented in sub-discipline journals compared to ecology journals.
Clearly, there is a limited use of ecological concepts related to studying rhizosphere interactions in the focal subdisciplines. We posit that one reason for that is that researchers are still facing considerable technical challenges studying belowground biology. Hence, roots are still “the hidden half” (Waisel et al., 2002), the soil medium is “the final frontier” (Sugden et al., 2004), and soils are still viewed “through a ped darkly” (Coleman, 2011). Regardless of the reason, a disconnection of concepts among subdisciplines that investigate rhizosphere interactions may ultimately impede progress of understanding of general ecological processes in the rhizosphere and particularly above-belowground linkages.
Limited Understanding of Rhizosphere Interactions
According to the Global Soil Biodiversity Atlas (Orgiazzi et al., 2016) the soil is the most biodiversity rich habitat on Earth. It is estimated that 23% of terrestrial animals are soil invertebrates (Decaëns et al., 2006), of which 80% are insects and earthworms (Lavelle et al., 2006). Evidence shows that belowground biodiversity contributes to shaping the functioning of terrestrial ecosystems (Bardgett and Van Der Putten, 2014). Nevertheless, while conceptual frameworks are well developed (Hooper et al., 2000; Wardle et al., 2004; Lavelle et al., 2016) and some interactions are well studied (e.g., mycorrhiza), knowledge gaps remain regarding the diversity of other taxa that are closely associated with plant roots. To demonstrate the paucity in understanding of rhizosphere biota, we pose a simple, but important question: how many of the organisms in a given ecosystem are associated with plant roots for a significant portion of their life cycles? This question is paraphrasing a broader question that several researchers tackled in the last decades: how many species are there on earth (May, 1988; Mora et al., 2011)? Similarly to estimating the total richness of species in an ecosystem based on extrapolations of specified community richness (Erwin, 1982), we could estimate the proportion of species engaging in rhizosphere interactions by looking only at beetles (Supplementary Material 2). The main problem with this approach is twofold: (1) Data scarcity. Exhaustive surveys and studies of belowground arthropod taxa are rare. (2) Conclusions are highly sensitive to model assumptions. Current theory and data are far from being able to provide a meaningful estimate to a fairly simple and fundamental question in ecological theory. It also highlights the gap of knowledge we still have in relation to belowground arthropod interactions as compared to microbes (Heinen et al., 2018). Another fundamental part of ecological research is related to the ways species are spatially arranged in the ecosystem.
Island Biogeography Belowground
The theory of Island Biogeography, first proposed by MacArthur and Wilson (1967), is a foundational concept in ecology that defined species richness on islands as the equilibrium between colonization and extinction. Due to the vast difference in scale, most soil organisms do not conform to the patterns of island biogeography on physical islands (Maraun et al., 2007; Ulrich and Fiera, 2009; Lavelle et al., 2016) or in “islands” created by habitat fragmentation (Mangan et al., 2004; Rantalainen et al., 2008). Ease of dispersal and low space requirements allow belowground biota to escape constraints on colonization faced by larger aboveground organisms (Griffin et al., 2002; Mangan et al., 2004; Rantalainen et al., 2008). However, there is some evidence that belowground communities do conform to the traditional theory of Island Biogeography when plant rhizospheres are considered as ‘islands’, likely because it is a more relevant scale (Peay et al., 2007, 2010; Glassman et al., 2017). The idea of plants as islands for herbivores was proposed as part of the theory of Island Biogeography and expanded to include plants as islands for microbes (Andrews et al., 1987; Martiny et al., 2006). However, studies are lacking assessments of temporal effects and cross taxa biodiversity when looking at plant islands. Thus, understanding belowground spatial ecology would benefit from long-term studies and examination of how species interactions might structure spatial patterns in belowground communities. Specifically, researchers asked how colonization after disturbance is different in rhizosphere interactions. Historically, the phrase ‘succession’ was used, but currently, a wider sense of this process is ‘Self-Organization’ (Lavelle et al., 2016).
Succession in the Rhizosphere
Succession describes predictable and mainly linear shifts or development of communities through time and is one of the earliest ecological concepts (Cowles, 1899). In soil systems however, biological communities are often considered to self-organized across scales of time and space (Lavelle et al., 2016). The processes of soil community self-organization are generally consistent with patterns aboveground. Limited evidence suggests that an area is first colonized by autotrophs such as nitrogen fixing bacteria and algae, followed by the addition of simple heterotrophs, followed by larger and more complex animals and fungal structures (Ohtonen et al., 1999; Maharning et al., 2009). This turnover leads to increased network tightening and more efficient carbon uptake (Morriën et al., 2017). In abandoned agricultural fields, nematode communities shift from herbivorous to fungivorous species in later successional plots as plant productivity decreases and fungal biomass increases (Maharning et al., 2009). Arthropod community turn-over showed less clearly directional and progressive change. Many of the studies of soil self-organization focus on single clades or compare presence of large taxonomic groups, thus missing granular species diversity and turn-over (Maharning et al., 2009). Recent advances in sequencing will make it increasingly easy to monitor these processes in bacterial and fungal soil communities (Fierer et al., 2009, 2010; Hudson et al., 2017). Furthermore, network perspectives can shed light on the assembly and interaction of rhizosphere communities in successional processes (García de León et al., 2016; Morriën et al., 2017;Morriën and Prescott, 2018).
Trophic Cascades and Top-Down Effects on Soil Food Webs
Trophic cascades, where predators indirectly benefit plants by consuming herbivores, is a fundamental concept in community ecology (Hairston et al., 1960; Pace et al., 1999). While the original concept was proposed mainly for aboveground systems, there has been very little consideration of whether trophic cascades might occur in belowground food webs (Denno et al., 2008). Carbon inputs belowground typically occur through detritus, and thus differ markedly from aboveground primary production by plants (Moore et al., 2004; Coleman et al., 2018). As such, soil food webs are considered to be self-organizing systems driven by bottom-up, mutually reinforcing processes (Lavelle et al., 2016). Nevertheless, secondary and tertiary consumers have long been recognized for their role in soil food webs, largely as ecosystem engineers and regulators of nutrient cycles (Lavelle et al., 2016; Coleman et al., 2018). Beginning around the turn of the century, researchers began probing a top-down perspective of soil food webs, asking whether top predators can regulate the abundance of lower trophic levels. While these early studies found evidence of top-down control on invertebrate communities, these impacts did not affect the basal microbial community (e.g., Mikola and Sktälä, 1998; Laakso and Setälä, 1999; Salamon et al., 2006). Other belowground studies, have documented top-down effects of invertebrate predators (e.g., arthropods or nematodes) that have cascading effects on the productivity of plant roots and aboveground tissue (Preisser et al., 2006; Denno et al., 2008; Ali et al., 2013). Collectively, these small but representative examples from the literature suggest a mixture of evidence for belowground trophic cascades. Support for top-down control in belowground trophic interactions comes from cases largely involving the effects of insects and nematodes on plant productivity, whereas lack of support comes from systems with microbes or detritus at the base of the food web. Thus, predicting belowground trophic cascades may require knowledge of population growth rates of organisms interacting in the food webs. Understanding interspecies dynamics may further benefit from studying the way they interact and communicate belowground.
Chemical Communication in the Rhizosphere
Communication among organisms is central to understanding any ecosystem, and the soil environment is no exception. Aboveground, vision and light sensing play an important role for organisms in many ecosystems (Doring, 2014; Kegge et al., 2015), but this option is lacking belowground. Thus, chemical communication is a more effective way for partners to communicate belowground (van Dam, 2009). The soil provides an environment that protects chemical compounds from degradation by oxygen and light, making belowground chemical signals more stable and possibly more reliable than aboveground (Karlovsky, 2008). Plant roots and soil microbes produce large arrays of volatile organic compounds (VOCs) that readily diffuse under atmospheric pressure and travel throughout the air- and liquid-filled pockets of the soil (van Dam et al., 2016). Therefore, they are believed to play important roles in soil interactions at multitrophic level (Schmidt et al., 2015; Venturi and Keel, 2016). Nevertheless, ecological functions of VOCs have mainly been studied for aboveground communities (Dicke and Baldwin, 2010). More recent studies on VOCs released by roots and soil bacteria and fungi found that similarly to the aboveground realm, VOCs also mediate species interactions in the rhizosphere (Rasmann et al., 2005; Martínez-Medina et al., 2017; Ossowicki et al., 2017; Schulz-Bohm et al., 2017). In addition, plants and microbes produce and secrete exudates containing an array of secondary metabolites, which can signal to and interfere with other soil organisms (Venturi and Keel, 2016). However, while the role of plant exudates has received significant attention (e.g., Steinkellner et al., 2007; Toussaint et al., 2012), the extent to which chemical communication in the soil affect interactions and dynamics of networks remains largely unknown.
Research Methodology of Rhizosphere Interaction
We briefly review important methodological gaps that are crucial to advance the understanding of rhizosphere interactions; we point readers to recent reviews for deeper reading into this topic (see McPhee and Aarssen, 2001; Forey et al., 2011). The challenge of studying biotic interactions is tackled by three types of methods: molecular/chemical techniques, field studies, and controlled conditions. Molecular and analytical chemistry tools are providing data on diversity of belowground microbial communities (Hudson et al., 2017). We can see the variation in broad taxonomic lines, but one of the outstanding challenges of the field is connecting species identity, or community composition, to function. Several methodologies such as large-scale and untargeted Gas Chromatography (GC)-mass spectrometry, liquid chromatography (LC)-mass spectrometry platforms, and nuclear magnetic resonance (H-NMR) are being used to decipher the chemical signaling in the rhizosphere (Oburger and Schmidt, 2016; van Dam and Bouwmeester, 2016; van Dam et al., 2016). The large-scale chemical analysis of the rhizomicrobiome, combined metagenomics, metatranscriptomics, metaproteomics and imaging mass spectrometry approaches (MSI) will help to understand the mechanisms involved in the communication between different members of the soil community (Oburger and Schmidt, 2016). Similarly, molecular databases such as Funguild and PICRUSt offer a promising framework to link taxa to function using 16S rRNA gene sequences (Langille et al., 2013; Tedersoo et al., 2014; Hahn et al., 2018). Given the spatial resolution required to investigate rhizosphere interactions (from cm to sub μm), the unpredictability of field conditions, and the instrumental limitations (e.g., immobility) most studies seek to simulate field conditions in semi-artificial experimental conditions (Oburger and Schmidt, 2016). Exploration of belowground organisms could be done with tools as simple as soil sampling and root excavations. For agricultural and plant physiology studies, Trachsel et al. (2011) coined the term “shovelomics” for high-throughput root phenotyping based on root crown architecture. We stress that continuing to apply the principles of shovelomics in ecological research will explore a lot of rhizosphere interactions. While it is difficult to sample soils with minimal disturbances, emerging techniques may allow for quantification of root traits and other belowground measurements using non-destructive techniques such as X-ray tomography (Bardgett et al., 2014). We propose that the integrated use of different molecular and metabolomic methodologies in semi-artificial and field conditions will be the most promising approach in shedding light onto the great number of yet unrevealed processes in the rhizosphere (Ferlian et al., 2018).
Concluding Remarks
The importance of belowground processes in ecology are being increasingly recognized (Wardle et al., 2004; Orgiazzi et al., 2016; Coleman et al., 2018). Still it is uncertain how ecological theories which were developed to describe aboveground interactions apply rhizosphere interactions. The literature shows some similarities between above- and belowground processes. For example, we can cautiously suggest that soil and plant heterogeneity is positively related to species diversity belowground (Kowalchuk et al., 2002). Yet, empirical evidence is still lacking (Scherber et al., 2009). Similarly, temporal development of belowground communities is somewhat predictable, much as it is aboveground (Maharning et al., 2009). Evidence is mixed in other areas; trophic cascades seem to have an important role in community and population dynamics belowground, as they have aboveground. On the other hand, there are major differences between below- and aboveground conditions that affect trophic interactions and dynamics. For instance, the scale at which key ecological processes are examined, such as the strength of trophic cascade linkages, dispersal, and species area curves, all show differences between above- and belowground systems. Differences in media structure can influence the pattern of communication, which is based more on chemicals belowground as compared to a broader use of light perception in the above-ground communities. Furthermore, increased attention to rhizosphere interactions, particularly studies using modern methodological approaches, will allow for more robust tests of many ecological theories. In addition to advancing predictive ecological theory, an improved understanding of rhizosphere interactions will ultimately aid in preserving biodiversity and mitigating negative ecological impacts of global change (Wardle et al., 2004; Sutherland et al., 2013).
Data Availability
Data from the literature search are deposited in Figshare (10.6084/m9.figshare.7356551).
Author Contributions
All authors listed have made a substantial, direct and intellectual contribution to the work, and approved it for publication. OS initiated the process and lead the writing. PH lead the literature search and contributed to writing and editing. ZG-P and AM contributed to writing and editing.
Conflict of Interest Statement
The authors declare that the research was conducted in the absence of any commercial or financial relationships that could be construed as a potential conflict of interest.
The handling editor is currently co-organizing a Research Topic with three of the authors, OS, PH, and AM, and confirms the absence of any other collaboration.
Acknowledgments
These ideas began at a discussion on belowground ecology at the Plant-Herbivore Interactions Gordon Conference in 2017 and we are grateful to the organizers of the conference and participants of these discussion. We thank A. von Haden, A.L.L Friedman, S. van Nouhuys, and several reviewers for providing helpful feedback on an earlier version of the manuscript. AM acknowledges funding from the program for attracting talent to Salamanca from Fundación Salamanca Ciudad de Cultura y Saberes.
Supplementary Material
The Supplementary Material for this article can be found online at: https://www.frontiersin.org/articles/10.3389/fevo.2019.00058/full#supplementary-material
References
Ali, J. G., Campos-Herrera, R., Alborn, H. T., Duncan, L. W., and Stelinski, L. L. (2013). Sending mixed messages: a trophic cascade produced by a belowground herbivore-induced cue. J. Chem. Ecol. 39, 1140–1147. doi: 10.1007/s10886-013-0332-x
Andrews, J. H., Kinkel, L. L., Berbee, F. M., and Nordheim, E. V. (1987). Fungi, leaves, and the theory of island biogeography. Microb. Ecol. 14, 277–290. doi: 10.1007/BF02012947
Andújar, C., Pérez-González, S., Arribas, P., Zaballos, J. P., Vogler, A. P., and Ribera, I. (2017). Speciation below ground: tempo and mode of diversification in a radiation of endogean ground beetles. Mol. Ecol. 26, 6053–6070. doi: 10.1111/mec.14358
Bais, H. P., Weir, T. L., Perry, L. G., Gilroy, S., and Vivanco, J. M. (2006). The role of root exudates in rhizosphere interactions with plants and other organisms. Annu. Rev. Plant Biol. 57, 233–266. doi: 10.1146/annurev.arplant.57.032905.105159
Bardgett, R. D., Mommer, L., and De Vries, F. T. (2014). Going underground: root traits as drivers of ecosystem processes. Trends Ecol. Evol. 29, 692–699. doi: 10.1016/j.tree.2014.10.006
Bardgett, R. D., and Van Der Putten, W. H. (2014). Belowground biodiversity and ecosystem functioning. Nature 515:505. doi: 10.1038/nature13855
Barot, S., Blouin, M., Fontaine, S., Jouquet, P., Lata, J. C., and Mathieu, J. (2007). A tale of four stories: soil ecology, theory, evolution, and the publication system. PLoS ONE 11:e1248. doi: 10.1371/journal.pone.0001248
Coleman, D. C. (2011). Understanding soil processes: one of the last frontiers in biological and ecological research. Australas. Plant Pathol. 40, 207–214. doi: 10.1007/s13313-011-0041-2
Coleman, D. C., Callaham, Jr. M. A., and Crossley, Jr. D. A. (2018). Fundamentals of Soil Ecology. London: Academic Press.
Cowles, H. C. (1899). The ecological relations of the vegetation on the sand dunes of Lake Michigan. Bot. Gaz. 27, 97–117.
Decaëns, T., Jiménez, J. J., Gioia, C., Measey, G. J., and Lavelle, P. (2006). The values of soil animals for conservation biology. Eur. J. Soil Biol. 42, S23–S38. doi: 10.1016/j.ejsobi.2006.07.001
Denno, R. F., Gruner, D. S., and Kaplan, I. (2008). Potential for entomopathogenic nematodes in biological control. A meta-analysis synthesis and insights from trophic cascade theory. J. Nematol. 40, 61–72. Available online at: https://www.ncbi.nlm.nih.gov/pmc/articles/PMC2586540/pdf/61.pdf
Dicke, M., and Baldwin, I. T. (2010). The evolutionary context for herbivore-induced plant volatiles: beyond the ‘cry for help’. Trends Plant Sci. 15, 167–175. doi: 10.1016/j.tplants.2009.12.002
Doring, T. F. (2014). How aphids find their host plants, and how they don't. Ann. Appl. Biol. 165, 3–26. doi: 10.1111/aab.12142
Erwin, T. L. (1982). Tropical forests: their richness in Coleoptera and other arthropod species. Coleopter. Bull. 36, 74–75.
Ferlian, O., Biere, A., Bonfante, P., Buscot, F., Eisenhauer, N., Fernandez, I., et al. (2018). Growing research networks on mycorrhizae for mutual benefits. Trends Plant Sci. 23, 975–984. doi: 10.1016/j.tplants.2018.08.008
Fierer, N., Nemergut, D., Knight, R., and Craine, J. M. (2010). Changes through time: integrating microorganisms into the study of succession. Res. Microbiol. 161, 635–642. doi: 10.1016/j.resmic.2010.06.002
Fierer, N., Strickland, M. S., Liptzin, D., Bradford, M. A., and Cleveland, C. C. (2009). Global patterns in belowground communities. Ecol. Lett. 12, 1–12. doi: 10.1111/j.1461-0248.2009.01360.x
Forey, E., Barot, S., Decaëns, T., Langlois, E., Laossi, K. R., Margerie, P., et al. (2011). Importance of earthworm–seed interactions for the composition and structure of plant communities: a review. Acta Oecologica 37, 594–603. doi: 10.1016/j.actao.2011.03.001
García de León, D., Moora, M., Öpik, M., Neuenkamp, L., and Gerz, M., Jairus, T., et al. (2016). Symbiont dynamics during ecosystem succession: co-occurring plant and arbuscular mycorrhizal fungal communities. FEMS Microbiol. Ecol. 92:fiw097. doi: 10.1093/femsec/fiw097
Glassman, S. I., Lubetkin, K. C., Chung, J. A., and Bruns, T. D. (2017). The theory of island biogeography applies to ectomycorrhizal fungi in subalpine tree “islands” at a fine scale. Ecosphere 8:e01677. doi: 10.1002/ecs2.1677
Griffin, D. W., Kellogg, C. A., Garrison, V. H., and Shinn, E. A. (2002). The global transport of dust: an intercontinental river of dust, microorganisms and toxic chemicals flows through the Earth's atmosphere. Am. Sci. 90, 228–235. doi: 10.1511/2002.3.228
Hahn, P. G., Bullington, L., Larkin, B., LaFlamme, K., Maron, J. L., and Lekberg, Y. (2018). Effects of short- and long-term variation in resource conditions on soil fungal communities and plant responses to soil biota. Front. Plant Sci. 9:1605. doi: 10.3389/fpls.2018.01605
Hairston, N. G., Smith, F. E., and Slododkin, L. B. (1960). Community structure, population control, and competition. Am. Nat. 94, 421–425. doi: 10.1086/282146
Heinen, R., Biere, A., Harvey, J. A., and Bezemer, M. (2018). Effects of soil organisms on aboveground plant-insect interactions in the field: patterns, mechanisms and the role of methodology. Front. Ecol. Evol. 6:106. doi: 10.3389/fevo.2018.00106
Hooper, D. U., Bignell, D. E., Brown, V. K., Brussaard, L., Dangerfield, J. M., Wall, D. H., et al. (2000). Aboveground and belowground biodiversity in terrestrial ecosystems: patterns, mechanisms, and feedbacks. Bioscience 50, 1049–1061. doi: 10.1641/0006-3568(2000)050[1049:IBAABB]2.0.CO;2
Hudson, L. N., Newbold, T., Contu, S., Hill, S. L., Lysenko, I., De Palma, A., et al. (2017). The database of the Predicts (Projecting responses of ecological diversity in changing terrestrial systems) project. Ecol. Evol. 7, 145–188. doi: 10.1002/ece3.2579
Karlovsky, P. (2008). “Secondary metabolites in soil ecology,”in Secondary Metabolites in Soil Ecology. Soil Biology, Vol 14, eds P. Karlovsky (Berlin; Heidelberg:Springer), 1–19.
Kegge, W., Ninkovic, V., Glinwood, R., Welschen, R. A. M., Voesenek, L., and Pierik, R. (2015). Red:far-red light conditions affect the emission of volatile organic compounds from barley (Hordeum vulgare), leading to altered biomass allocation in neighbouring plants. Ann. Botany 115, 961–970. doi: 10.1093/aob/mcv036
Kowalchuk, G. A., Buma, D. S., de Boer, W., Klinkhamer, P. G., and van Veen, J. A. (2002). Effects of above-ground plant species composition and diversity on the diversity of soil-borne microorganisms. Antonie Van Leeuwenhoek 81:509.
Laakso, J., and Setälä, H. (1999). Population- and ecosystem-level effects of predation on microbial-feeding nematodes. Oecologia 120, 279–286. doi: 10.1007/s004420050859
Langille, M. G., Zaneveld, J., Caporaso, J. G., McDonald, D., Knights, D., Reyes, J. A., et al. (2013). Predictive functional profiling of microbial communities using 16S rRNA marker gene sequences. Nat. Biotech. 31, 814–821. doi: 10.1038/nbt.2676
Lavelle, P., Decaëns, T., Aubert, M., Barot, S., Blouin, M., Bureau, F., et al. (2006). Soil invertebrates and ecosystem services. Eur. J. Soil Biol. 42, S3–S15. doi: 10.1016/j.ejsobi.2006.10.002
Lavelle, P., Spain, A., Blouin, M., Brown, G., Decaëns, T., Grimaldi, M., et al. (2016). Ecosystem engineers in a self-organized soil: a review of concepts and future research questions. Soil Sci. 181, 91–109. doi: 10.1097/SS.0000000000000155
MacArthur, R. H., and Wilson, E. O. (1967). The Theory of Island Biogeography, Monographs in Population Biology. Princeton, NJ: Princeton University Press.
Maharning, A. R., Mills, A. A. S., and Adl, S. M. (2009). Soil community changes during secondary succession to naturalized grasslands. Appl. Soil Ecol. 41, 137–147. doi: 10.1016/j.apsoil.2008.11.003
Mangan, S. A., Eom, A. H., Adler, G. H., Yavitt, J. B., and Herre, E. A. (2004). Diversity of arbuscular mycorrhizal fungi across a fragmented forest in Panama: insular spore communities differ from mainland communities. Oecologia 141, 687–700. doi: 10.1007/s00442-004-1684-2
Maraun, M., Schatz, H., and Scheu, S. (2007). Awesome or ordinary? Global diversity patterns of oribatid mites. Ecography 30, 209–216. doi: 10.1111/j.0906-7590.2007.04994.x
Martínez-Medina, A., Van Wees, S. C. M., and Pieterse, C. M. J. (2017). Airborne signals from Trichoderma fungi stimulate iron uptake responses in roots resulting in priming of jasmonic acid-dependent defences in shoots of Arabidopsis thaliana and Solanum lycopersicum. Plant Cell Environ. 40, 2691–2705. doi: 10.1111/pce.13016
Martiny, J. B., Bohannan, B. J. M., Brown, J. H., Colwell, R. K., Fuhrman, J. A., Green, J. L., et al. (2006). Microbial biogeography: putting microorganisms on the map. Nat. Rev. Microbiol. 4, 102–112. doi: 10.1038/nrmicro1341
May, R. M. (1988). How many species are there on earth? Science, 241, 1441–1449. doi: 10.1126/science.241.4872.1441
McPhee, C. S., and Aarssen, L. W. (2001). The separation of above-and below-ground competition in plants a review and critique of methodology. Plant Ecol. 152, 119–136. doi: 10.1023/A:1011471719799
Mikola, J., and Sktälä, H. (1998). No evidence of trophic cascades in an experimental microbial-based soil food web. Ecology 79, 153–164. doi: 10.1890/0012-9658(1998)079[0153:NEOTCI]2.0.CO;2
Moore, J. C., Berlow, E. L., Coleman, D. C., De Suiter, P. C., Dong, Q., Hastings, A., et al. (2004). Detritus, trophic dynamics and biodiversity. Ecol. Lett. 7, 584–600. doi: 10.1111/j.1461-0248.2004.00606.x
Mora, C., Tittensor, D. P., Adl, S., Simpson, A. G., and Worm, B. (2011). How many species are there on Earth and in the ocean? PLoS Biol. 9:e1001127. doi: 10.1371/journal.pbio.1001127
Morriën, E., Hannula, E., Snoek, L., Helmsing, N., Zweers, H., Hollander, M., et al. (2017). Soil networks become more connected and take up more carbon as nature restoration progresses. Nat. Commun. 8:14349. doi: 10.1038/ncomms14349
Morriën, E., and Prescott, C. E. (2018). Pellets or particles? How can we predict the effect of soil macro-arthropods on litter decomposition? Funct. Ecol. 32, 2480–2482. doi: 10.1111/1365-2435.13217
Newcombe, R. G. (1998). Two-sided confidence intervals for the single proportion: comparison of seven methods. Stat. Med. 17, 857–872.
Nobis, M., and Wohlgemuth, T. (2004). Trend words in ecological core journals over the last 25 years (1978-2002). Oikos 106, 411–420. doi: 10.1111/j.0030-1299.2004.13496.x
Oburger, E., and Schmidt, H. (2016). New methods to unravel rhizosphere processes. Trends Plant Sci. 21, 243–255. doi: 10.1016/j.tplants.2015.12.005
Ohtonen, R., Fritze, H., Pennanen, T., Jumpponen, A., and Trappe, J. (1999). Ecosystem properties and microbial community changes in primary succession on a glacier forefront. Oecologia 119, 239–246. doi: 10.1007/s004420050782
Orgiazzi, A., Bardgett, R. D., and Barrios, E. (eds.). (2016). Global Soil Biodiversity Atlas. Luxemburg: European Commission.
Ossowicki, A., Jafra, S., and Garbeva, P. (2017). The antimicrobial volatile power of the rhizospheric isolate Pseudomonas donghuensis P482. PloS ONE 12:e0174362. doi: 10.1371/journal.pone.0174362
Pace, M. L., Cole, J. J., Carpenter, S. R., and Kitchell, J. F. (1999). Trophic cascades revealed in diverse ecosystems. Trends Ecol. Evol. 14, 483–488. doi: 10.1016/S0169-5347(99)01723-1
Peay, K. G., Bruns, T. D., Kennedy, P. G., Bergemann, S. E., and Garbelotto, M. (2007). A strong species–area relationship for eukaryotic soil microbes: island size matters for ectomycorrhizal fungi. Ecol. Lett. 10, 470–480. doi: 10.1111/j.1461-0248.2007.01035.x
Peay, K. G., Garbelotto, M., and Bruns, T. D. (2010). Evidence of dispersal limitation in soil microorganisms: isolation reduces species richness on mycorrhizal tree islands. Ecology 91, 3631–3640. doi: 10.1890/09-2237.1
Preisser, E. L., Dugaw, C. J., Dennis, B., and Strong, D. R. (2006). Plant facilitation of a belowground predator. Ecology 87, 1116–1123. doi: 10.1890/0012-9658(2006)87[1116:PFOABP]2.0.CO;2
Rantalainen, M.-L., Haimi, J., Fritze, H., Pennanen, T., and Setälä, H. (2008). Soil decomposer community as a model system in studying the effects of habitat fragmentation and habitat corridors. Soil Biol. Biochem. 40, 853–863. doi: 10.1016/j.soilbio.2007.11.008
Rasmann, S., Köllner, T. G., Degenhardt, J., Hiltpold, I., Toepfer, S., Kuhlmann, U., et al. (2005). Recruitment of entomopathogenic nematodes by insect-damaged maize roots. Nature 434, 732–737. doi: 10.1038/nature03451
Russell, E. J., and Appleyard, A. (1915). The atmosphere of the soil: its composition and the causes of variation. J. Agric. Sci. 7, 1–48. doi: 10.1017/S0021859600002410
Salamon, J. A., Alphei, J., Ruf, A., Schaefer, M., Scheu, S., Schneider, K., et al. (2006). Transitory dynamic effects in the soil invertebrate community in a temperate deciduous forest: effects of resource quality. Soil Biol. Biochem. 38, 209–221. doi: 10.1016/j.soilbio.2005.04.033
Scherber, C., Strauss, S. Y., Vos, M., and Wardle, D. A. (2009). Empirical and theoretical challenges in aboveground–belowground ecology. Oecologia 161, 1–14. doi: 10.1007/s00442-009-1351-8
Schmidt, R., Cordovez, V., de Boer, W., Raaijmakers, J., and Garbeva, P. (2015). Volatile affairs in microbial interactions. ISME J. 9, 2329–2335. doi: 10.1038/ismej.2015.42
Schulz-Bohm, K., Geisen, S., Wubs, E. J., Song, C., de Boer, W., and Garbeva, P. (2017). The prey's scent–volatile organic compound mediated interactions between soil bacteria and their protist predators. ISME J. 11, 817–820. doi: 10.1038/ismej.2016.144
Steinkellner, S., Lendzemo, V., Langer, I., Schweiger, P., Khaosaad, T., Toussaint, J. P., et al. (2007). Flavonoids and strigolactones in root exudates as signals in symbiotic and pathogenic plant-fungus interactions. Molecules 12, 1290–1306. doi: 10.3390/12071290
Sugden, A., Stone, R., and Ash, C. (2004). Ecology in the underworld. Science 304:1613. doi: 10.1126/science.304.5677.1613
Sutherland, W. J., Freckleton, R. P., Godfray, H. C. J., Beissinger, S. R., Benton, T., Cameron, D. D., et al. (2013). Identification of 100 fundamental ecological questions. J. Ecol. 101, 58–67. doi: 10.1111/1365-2745.12025
Tedersoo, L., Bahram, M., Polme, S., Koljalg, U., Yorou, N. S., Wijesundera, R., et al. (2014). Global diversity and geography of soil fungi. Science 346:1256688. doi: 10.1126/science.1256688
Toussaint, J. P., Pham, T. T. M., Barriault, D., and Sylvestre, M. (2012). Plant exudates promote PCB degradation by a rhodococcal rhizobacteria. Appl. Microbiol. Biotechnol. 95, 1589–1603. doi: 10.1007/s00253-011-3824-z
Trachsel, S., Kaeppler, S. M., Brown, K. M., and Lynch, J. P. (2011). Shovelomics: high throughput phenotyping of maize (Zea mays L.) root architecture in the field. Plant Soil 341, 75–87. doi: 10.1007/s11104-010-0623-8
Ulrich, W., and Fiera, C. (2009). Environmental correlates of species richness of European springtails (Hexapoda: Collembola). Acta Oecologica 35, 45–52. doi: 10.1016/j.actao.2008.07.007
van Dam, N. M. (2009). Belowground herbivory and plant defenses. Ann. Rev. Ecol. Evol. Syst. 40, 373–392. doi: 10.1146/annurev.ecolsys.110308.120314
van Dam, N. M., and Bouwmeester, H. J. (2016). Metabolomics in the rhizosphere: tapping into belowground chemical communication. Trends Plant Sci. 21, 256–265. doi: 10.1016/j.tplants.2016.01.008
van Dam, N. M., Weinhold, A., and Garbeva, P. (2016). “Calling in the dark: the role of volatiles for communication in the rhizosphere,” in Deciphering Chemical Language of Plant Communication. Signaling and Communication in Plants, eds J. Blande, and R. Glinwood (Cham: Springer), 175–210. doi: 10.1007/978-3-319-33498-1_8
van Der Putten, W. H., Bardgett, R. D., De Ruiter, P. C., Hol, W. H. G., Meyer, K. M., Bezemer, T. M., et al. (2016). Signaling in the rhizosphere. Trends Plant Sci. 21, 187–198.
Venturi, V., and Keel, C. (2016). Signaling in the rhizosphere. Trends Plant Sci. 21 187–198. doi: 10.1016/j.tplants.2016.01.005
Waisel, Y., Eshel, A., and Kafkafi, U. (eds.). (2002). Plant Roots: The Hidden Half. 3rd Edn. New York, NY: Marcel Dekker Inc.
Keywords: belowground, biodiversity, community ecology, ecological theory, rhizosphere, species interaction
Citation: Shelef O, Hahn PG, Getman-Pickering Z and Martinez Medina A (2019) Coming to Common Ground: The Challenges of Applying Ecological Theory Developed Aboveground to Rhizosphere Interactions. Front. Ecol. Evol. 7:58. doi: 10.3389/fevo.2019.00058
Received: 11 June 2018; Accepted: 18 February 2019;
Published: 14 March 2019.
Edited by:
Mysore V. Tejesvi, University of Oulu, FinlandReviewed by:
Deirdre McClean, University of Edinburgh, United KingdomRobin Heinen, Netherlands Institute of Ecology (NIOO-KNAW), Netherlands
Copyright © 2019 Shelef, Hahn, Getman-Pickering and Martinez Medina. This is an open-access article distributed under the terms of the Creative Commons Attribution License (CC BY). The use, distribution or reproduction in other forums is permitted, provided the original author(s) and the copyright owner(s) are credited and that the original publication in this journal is cited, in accordance with accepted academic practice. No use, distribution or reproduction is permitted which does not comply with these terms.
*Correspondence: Oren Shelef, c2hlbGVmQHZvbGNhbmkuYWdyaS5nb3YuaWw=
†These authors have contributed equally to this work