Identifying global research and conservation priorities for Columbidae: a quantitative approach using random forest models
- 1Laboratoire de Biologie des Organismes et Ecosystèmes Aquatiques (BOREA), Unité Mixte de Recherche 8067, Muséum National d'Histoire Naturelle, Centre National de la Recherche Scientifique, Sorbonne Université, Institut de Recherche et de Développement 207, Université de Caen Normandie, Université des Antilles, Pointe-à-Pitre, Guadeloupe, France
- 2UMR CNRS 6282 Biogéosciences, Université de Bourgogne-Franche Comté, Dijon, France
- 3Caribaea Initiative, Département de Biologie, Université des Antilles, Guadeloupe, France
- 4Institut de Systématique, Evolution, Biodiversité (ISYEB) Muséum National d’Histoire Naturelle, Centre National de la Recherche Scientifique (CNRS), Sorbonne Université, École Pratique des Hautes Études (EPHE), Université des Antilles, Pointe-à-Pitre, Guadeloupe, France
The family of Columbidae, including pigeons and doves, remains understudied despite their patrimonial value and high ecological and conservation relevance. Currently, 353 extant columbid species are listed in the IUCN red list, with about 20% of them being threatened with extinction. However, there has been little effort so far to synthetize the available information on factors influencing extinction risk and the allocation of research effort among columbid species. In this context, using random forest models, the present study aims at quantitatively assessing to what extent environmental, life history and socio-political factors may drive the extinction risk of pigeons and doves and explain differences in scientific attention among species. We found that high risk of extinction in columbids is associated with small historical range, exposure to invasive alien mammals and living in isolated islands and/or at low altitudes, while the probability of population decline is associated with species body size, surrounding human density and narrow habitat breadth. We also evidenced a large disparity between species or population extinction risk and scientific interest. Indeed, most of the studies on columbids have been conducted by scientists from North America and Western Europe on their local species, whereas species from biodiversity hotspots, which are more at risk of extinction, have comparatively received little attention. This unequal acquisition of knowledge creates gaps that deserve to be filled in order to have a good appreciation of extinction risk in columbids and associated threats, through fair transnational cooperation, academic training and regional coordination in conservation-oriented research on columbids.
1 Introduction
The rate of biodiversity loss is currently increasing at an unprecedented rate, as a direct consequence of habitat loss, fragmentation and degradation, biological invasions, and climate change (Balmford et al., 2003; Barnosky et al., 2011). However, the extent and rate of biodiversity loss are not uniform around the globe (Howard et al., 2020), nor across taxa (Pimm et al., 2014). As available funding for conservation research and actions is limited (Evans et al., 2012; Waldron et al., 2013), there is an obvious need to define priorities for optimizing resource allocation (Brooks et al., 2006). Ideally, prioritization mechanisms should be based on sound and reliable scientific evidence (Williams et al., 2020). However, important gaps in knowledge still exist, particularly in several biodiversity-rich countries, such as Haiti or sub-saharan African countries, where ongoing conflicts, political instability and poor access to facilities and funding severely hamper the development of field research and monitoring programs (Hanson et al., 2009; Siddig, 2014; Conteh et al., 2017; dos Santos et al., 2020; Vallès et al., 2021). In addition to spatial bias, the conservation literature tends to give a disproportionate attention to emblematic, charismatic, and flagship species (Andelman and Fagan, 2000; Brambilla et al., 2013; Mammides, 2019; Habel et al., 2021). Consequently, there is a certain mismatch between research efforts and conservation priorities (Christie et al., 2021).
Avian conservation biology research is no exception to the rule (de Lima et al., 2011; Ducatez and Lefebvre, 2014; Murray et al., 2015; Roberts et al., 2016; Buechley et al., 2019). Various factors, such as environmental conditions, phylogeny, life-history traits, levels of anthropogenic pressure, or socio-political factors, independently or jointly, can affect the risk of extinction of avian species (Owens and Bennett, 2000). For instance, extinction risk is not distributed randomly among avian families and in relation to fecundity and body size (Bennett and Owens, 1997). On the other hand, the severity of extinction risk of avian species varies in relation to socioeconomic factors and human pressure (Olah et al., 2016; Chen et al., 2019). Similarly, variation in the level of scientific attention among bird species is significantly influenced by phylogeny, geographic range and ecological life history traits, with non-threatened species being studied twice as much as threatened ones (Ducatez and Lefebvre, 2014). For instance, although the Andean Flamingo, Phoenicoparrus andinus, is the least abundant flamingo in the world and is listed as Vulnerable by the IUCN Species Survival Commission (Derlindati et al., 2014), it has received far less attention than the Least Concern Greater Flamingo, Phoenicopterus roseus (Johnson and Cézilly, 2007; Geraci et al., 2012). In addition, the majority of avian conservation research is conducted in developed countries and/or by North American and western European researchers, confirming the mismatch between priorities and capacity for research and conservation (Brito and Oprea, 2009; Vallès et al., 2021).
In particular, Columbidae, the only extant family of Columbiformes, is particularly understudied, despite its patrimonial value and high ecological and conservation relevance (Walker, 2007; McKenzie and Robertson, 2015; Devenish-Nelson et al., 2019; Yarwood et al., 2019). Pigeons and doves are one of the oldest and most diverse extant lineages of birds. However, their evolutionary radiation and phylogeny remain poorly resolved (Soares et al., 2016), with the creation of new genus or species and the slimming of other genus based on molecular or paleontological evidence (Johnson et al., 2001; Banks et al., 2013; Sangster et al., 2018; Steadman and Takano, 2020). Currently, the family Columbidae includes about 370 species that are distributed on all continents, except for the high Artic and Antarctica (del Hoyo et al., 1997; BirdLife International, 2022). They occur in various habitats (del Hoyo et al., 1997; Gibbs et al., 2001; Carvalho et al., 2015; Fey et al., 2015; Monterrubio-Rico et al., 2016), where they provide important ecosystem services, particularly through seed dispersal (Meehan et al., 2005; Wotton and Kelly, 2012; Costan and Sarasola, 2017; Ando et al., 2021). However, a significant proportion of columbid species are endangered or threatened with extinction (Bennett and Owens, 1997; Walker, 2007; Rivera-Milán et al., 2015; Lees et al., 2021). This results in habitat fragmentation and degradation, introduced predators, competition with invasive columbid species, excessive hunting pressure and ineffective management, and trade (Walker, 2007; Carvalho et al., 2015; Camacho-Cervantes and Schondube, 2018; Lamelas-López et al., 2020; Lormée et al., 2020; Moreno-Zarate et al., 2021; Bacon et al., 2023). The conservation status of several species remains uncertain, mainly due to lack of data (del Hoyo et al., 1997; Walker, 2007). This is particularly true of secretive species or species with very restricted geographical range for which traditional study methods might not be appropriate (Cambrone et al., 2021). More to the point, little effort has been made so far to synthetize the available information on the factors influencing the conservation status of columbid species and research effort. To the best of our knowledge, only Walker (2007) provided a short review of the main drivers of extinction risk for columbid species based on a relatively simple statistical analysis of data available from BirdLife International, emphasizing the vulnerability of insular species. We went further in the present study by quantitatively assessing to what extent environmental, life history traits and socio-political factors may (i) drive the extinction risk of pigeons and doves and (ii) explain differences in scientific attention among species. To that end, we relied on random forest (RF) models and an updated database (including both IUCN conservation status and population trends of columbid species) especially compiled for this study.
2 Materials and methods
2.1 Database creation
2.1.1 Information about columbid species
We obtained data for the 369 species from three main sources: Birdlife international (BirdLife International, 2021), the website Birds of the World (https://birdsoftheworld.org) and Pigeons and Doves: A Guide to the Pigeons and Doves of the World (Gibbs et al., 2001). We extracted information on biological, ecological and environmental traits that are likely to influence extinction risk (see Buechley et al., 2019). We classified explanatory variables into four categories: “environment”, “behavior and life history”, “anthropogenic pressure” and “socio-political indicators” (Table 1). In order to estimate each species’ extinction risk, we relied on IUCN Red List categories and global population trends (BirdLife International, 2021). We classified species as threatened (critically endangered CR, endangered EN and vulnerable VU), non-threatened (near-threatened NT and least-concern LC), extinct (extinct EX and extinct in the wild EW), or data deficient. Because non-threatened species do not necessarily show stable or increasing population trends (Figure 1), we used global population trends of species (Rodrigues et al., 2006) as a second proxy of extinction risk. Species were thus classified as increasing, stable or decreasing according to BirdLife International (2021).
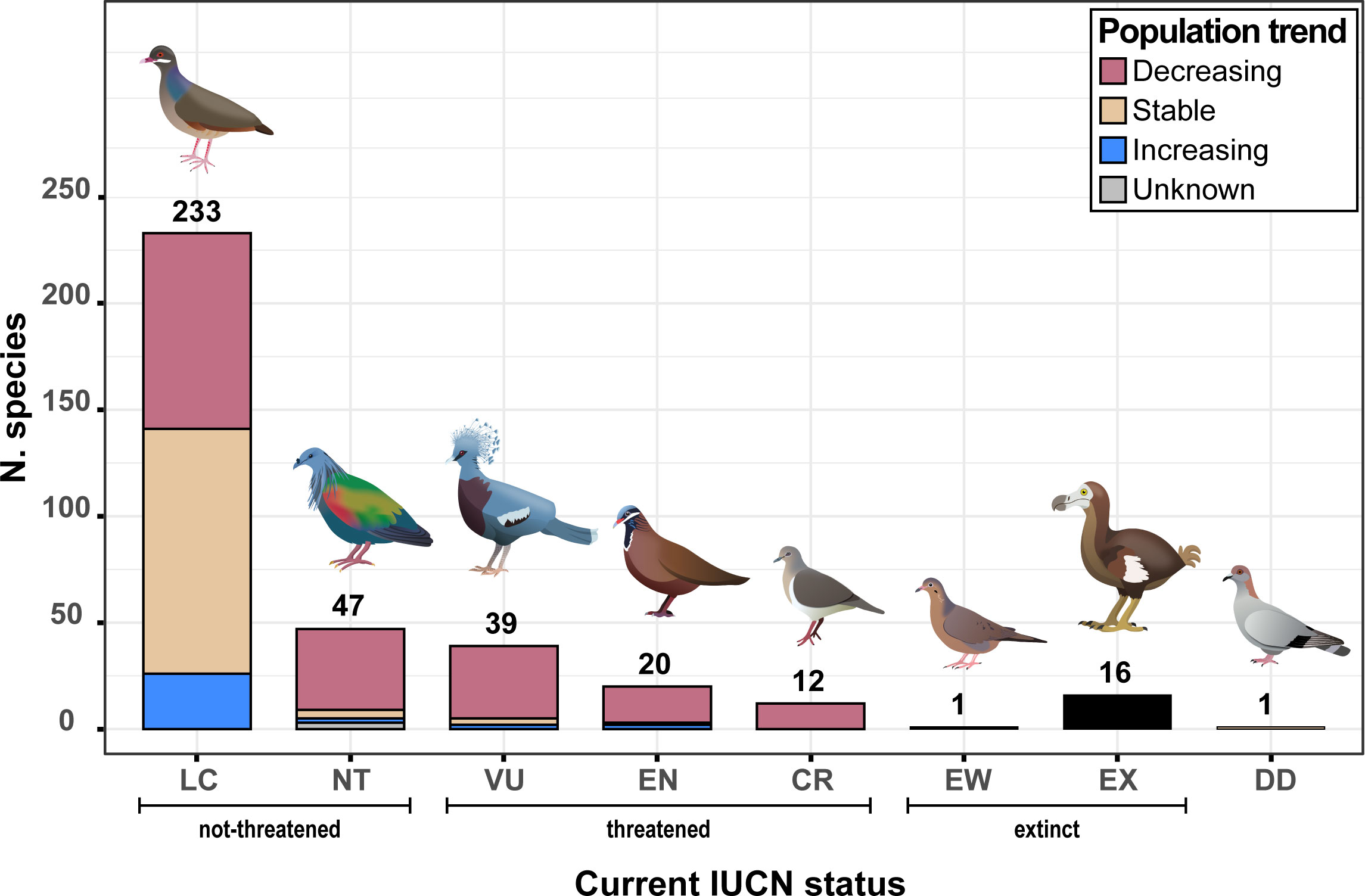
Figure 1 Distribution of columbid species according to their current IUCN status and their current population trend. From left to right, columbid species illustrations correspond to: Bridled Quail-Dove (Geotrygon mystacea), Nicobar Pigeon (Caloenas nicobarica), Victoria Crowned-Pigeon (Goura victoria), Blue-headed Quail-Dove (Starnoenas cyanocephala), Grenada Dove (Leptotila wellsi), Socorro Dove (Zenaida graysoni), Dodo (Raphus cucullatus) and Somali Pigeon (Columba oliviae).
2.1.2 Scientific attention
In order to quantify scientific attention given to each columbid species, we relied on the number of peer-reviewed articles retrieved from an extensive bibliographic search on all databases of the Web of Science (conducted by mid-2021). We performed searches using current and past binomial scientific names, vernacular names in English, French and Spanish and all recognized synonyms. To compile all possible names of species, we used the websites Avibase (https://avibase.bsc-eoc.org) and Birds of the World. We considered several “research areas”, as defined in the Web of Science, as all studies bringing ecological knowledge about a species may be of help for its conservation (Courchamp et al., 2015). Each search was conducted using the following procedure:
“TS=”
(in order to search following terms in title, abstract and keywords of articles)
+
Full binomial scientific names, vernacular and all recognized synonyms in independent quotes, separated by “OR”
+
“AND SU=(“Ecology” OR “Biodiversity and Conservation” OR “Ornithology” OR “Evolutionary Biology” OR “Zoology”)”
(to limit searches to studies related to following science disciplines: Ecology, Conservation Biology, Ornithology, Evolutionary Biology/Ecology and Zoology)
+
“NOT SU=(“Veterinary Sciences” OR “Neurosciences and Neurology” OR “Anthropology”)”
(to exclude any study related to veterinary sciences, neurosciences and neurology, and anthropology).
We also performed a global search in order to estimate national contributions to research on Columbidae, assessed from the affiliations of authors of peer-reviewed articles. To that end, we used the same search nomenclature, except that we replaced names of species and their synonyms by “Columbidae”, “Columbiformes”, “Pigeon” and “Dove”, separated by the Booleans operator “OR”. In order to exclude all articles on Pigeon pea (Cajanus cajan), we added: NOT TS=(“Pigeon*pea*” OR “Cajanus cajan”).
2.1.3 Spatial analyses (GIS)
We used QGIS (3.10.11) to calculate spatial statistics for explanatory variables used in our analyses (Table 1 and Supplementary Material 1, Method A1). We obtained various raster and polygon files for historical species range from several sources (Table 1), and rasterized polygons representing area distribution of species using scientific attention (log[number of articles + 1]) as value for the raster unit (square of 400km²). Since, polygons of several species may overlap, we beforehand calculated the mean of scientific attention per raster unit.
2.2 Random forest models
We relied on random forest models because this machine-learning technique has been increasingly used in ecological and macro-ecology studies (Cutler et al., 2007; Kampichler et al., 2010) to assess the impact of various drivers on the extinction risks of a given group of species (Davidson et al., 2012; Di Marco et al., 2014; Di Marco et al., 2018; Buechley and Şekercioğlu, 2016; Buechley et al., 2019). In particular, the method is robust to both phylogenetic inertia and outliers, and can include both continuous and categorical variables (Murray et al., 2011; Davidson et al., 2012; Buechley et al., 2019). This technique is therefore particularly suitable for columbids given that their phylogenetic relationships are not fully resolved due to scarce sampling of taxa and limited availability of sequence data (Khan and Arif, 2013; Bruxaux et al., 2018).
We excluded extinct species and data deficient species from our analyses relying on RFs (Figure 1). We performed three different RF models to identify the factors influencing extinction risk, population trends, and scientific attention among columbids, including all traits listed and detailed in Table 1 as explanatory variables. We considered a wider range of threats than the ones considered by IUCN, thus reducing the risk of circularity when using IUCN status as a proxy of extinction risk of columbid species. All models were performed using the R software (v4.1.1, R Core Team, 2021), using RandomForest package (Liaw and Wiener, 2002). Forests consisted of 10,000, 10,000 and 1,000 classification trees for models implying respectively extinction risk, population trends and scientific attention, in order to reach the lowest value of Out Of Bag errors (OOB; i.e., the percentage of error in predicting the class of these statistical individuals across the random forest formation, see Supplementary Material 1, Method A2), which was also stable throughout the formation of the forest. To evaluate the accuracy of our classification models, we relied on the percentage of species correctly classified (i.e., 100% − OOB), Cohen’s kappa statistic that tests the consistency between the actual and predicted classifications, and the sensitivity, specificity and the true skill statistic (TSS = sensitivity + specificity − 1; Allouche et al., 2006; Prasad et al., 2006; Gamer et al., 2019). For the RF addressing scientific attention (quantitative variable), we included the current IUCN category status (threatened/non-threatened) and population trends as explanatory variables. We relied on the percentage of variance explained (R²) as accuracy metrics to assess the performance of the model. The number of variables tested at each split was determined by the program (mtry; root-squared of the total number of explanatory variables). We estimated the relative importance of each explanatory variables implied in RF models based on the mean decrease Gini (when the dependent variable is qualitative or classes) or increase in node purity (when the dependent variable is quantitative), as a measure of how much model accuracy decreases when removing explanatory variables. The calculation is based on the Gini/node impurity value estimated from classification trees composing the forest. Detailed explanations about random forest modelling are provided in Supplementary Material 1, Method A2.
After inspecting partial dependence plots of relationships between explanatory and dependent variables, we restricted the analysis to the eight first most important variables, as the other ones were much less meaningful and did not present enough variation (Supplementary Material 2, Figure A4–A7). In order to compare and statistically assess the relative importance of explanatory variables and partial plots, we calculated 95% confidence interval (95%CI) by performing 1,000 independent bootstraps from the original dataset (Cumming and Finch, 2005). Results of inferential statistical tests (i.e., tests of Wilcoxon, Kruskal-Wallis, Kendall’s correlation or Fisher) are presented in Supplementary Material 2 (Table A1–A3), for all explanatory variables.
Although accuracy metrics were calculated for each RF model, we assessed the prediction capacity of models addressing extinction risk and evaluated overfitting issues by randomly splitting the original dataset into a training dataset (80% of the original dataset) and a dataset that was used to make predictions about extinction risk (i.e., the remaining 20% of the original dataset). Thereafter, we compared predicted results to actual ones by calculating sensitivity, specificity and TSS. This process was renewed 1,000 times in order to calculate the mean and 95% confidence interval of the three performance statistics. Thereafter, we predicted extinction risk for all columbid species by excluding one by one each species from the training dataset (i.e., all species, excepted the species tested). As we found mismatches between predicted and actual extinction risk for some species, we assessed to what extent this was related to levels of scientific attention. To that end, we considered two groups based on the median of scientific attention (i.e., one). The first group then consisted of species for which more than one published scientific article was available whereas the second one corresponded to species for which one or zero scientific article was available. We then tested for differences in the relative proportions of mismatches and correctly evaluated species between the two groups using a Chi-square test. For both analyses, significance level was set at 0.05.
3 Results
Among the 369 described columbid species, 76% are considered by IUCN as non-threatened and 19% as threatened, and 5% as extinct or extinct in the wild (Figure 1). In terms of population trends, 52% of species are declining, while 33% are stable and 9% are increasing. As expected, the relative proportions of declining, stable, and increasing species differed between threatened and non-threatened ones [threatened species: 89%, 6%, and 5%, respectively; non-threatened species: 46%, 43%, and 11%, respectively; Fisher’s exact test, P< 0.0001].
3.1 Extinction risk
3.1.1 Threatened vs. non-threatened species
The RF model classifying species either as non-threatened or threatened correctly classified 87% of the species based on the 38 explanatory variables used (Table 1). RF model sensitivity and specificity respectively indicated that 87% of actual non-threatened species and 82% of actual threatened species were correctly identified. Cohen’s kappa statistics and TSS further indicated that the classification of species significantly differed from a random classification [Cohen’s kappa = 0.51, P< 0.0001; TSS = 0.70], while the OOB error was 13%. The eight most important variables influencing extinction risk were in decreasing order: species historical range size, impact of invasive alien mammals, distance to closest mainland, altitude distribution of the species, HFP, human density, proportion of species historical range in current biodiversity hotspots, and species body mass. Based on the 95% CI comparison, only historical geographic range size had a relative importance significantly higher than the other variables (Figure 2A, see Supplementary Material 2, Figure A4 for all variables importance values). Based on partial dependence plots, species with the smallest historical range size, exposed to invasive alien mammals, living the farthest to mainland and/or at the lowest altitudes were associated to a greater risk of extinction (Figure 3A). Although other variables were relatively important in the prediction of species’ risk extinction based on the RF model, their variation did not seem to be directly associated to extinction risk (Figure 3A; see Supplementary Material 2, Figure A5 for all variables).
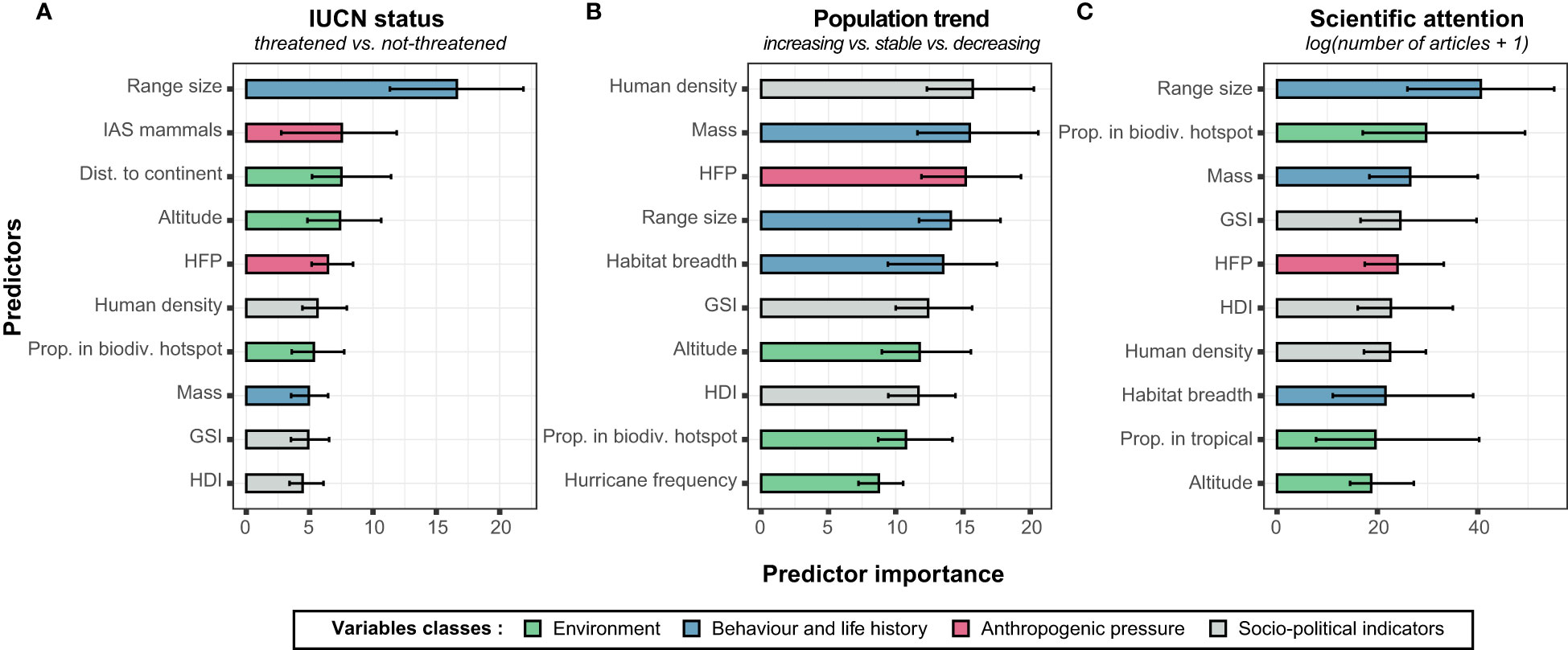
Figure 2 The relative importance of each explanatory variables implied in RF models with (A) IUCN status, (B) population trend and (C) scientific attention as explained variables. Only the ten most important variables are presented here. See Supplementary Material 2, Figure A4 for all explanatory variables.
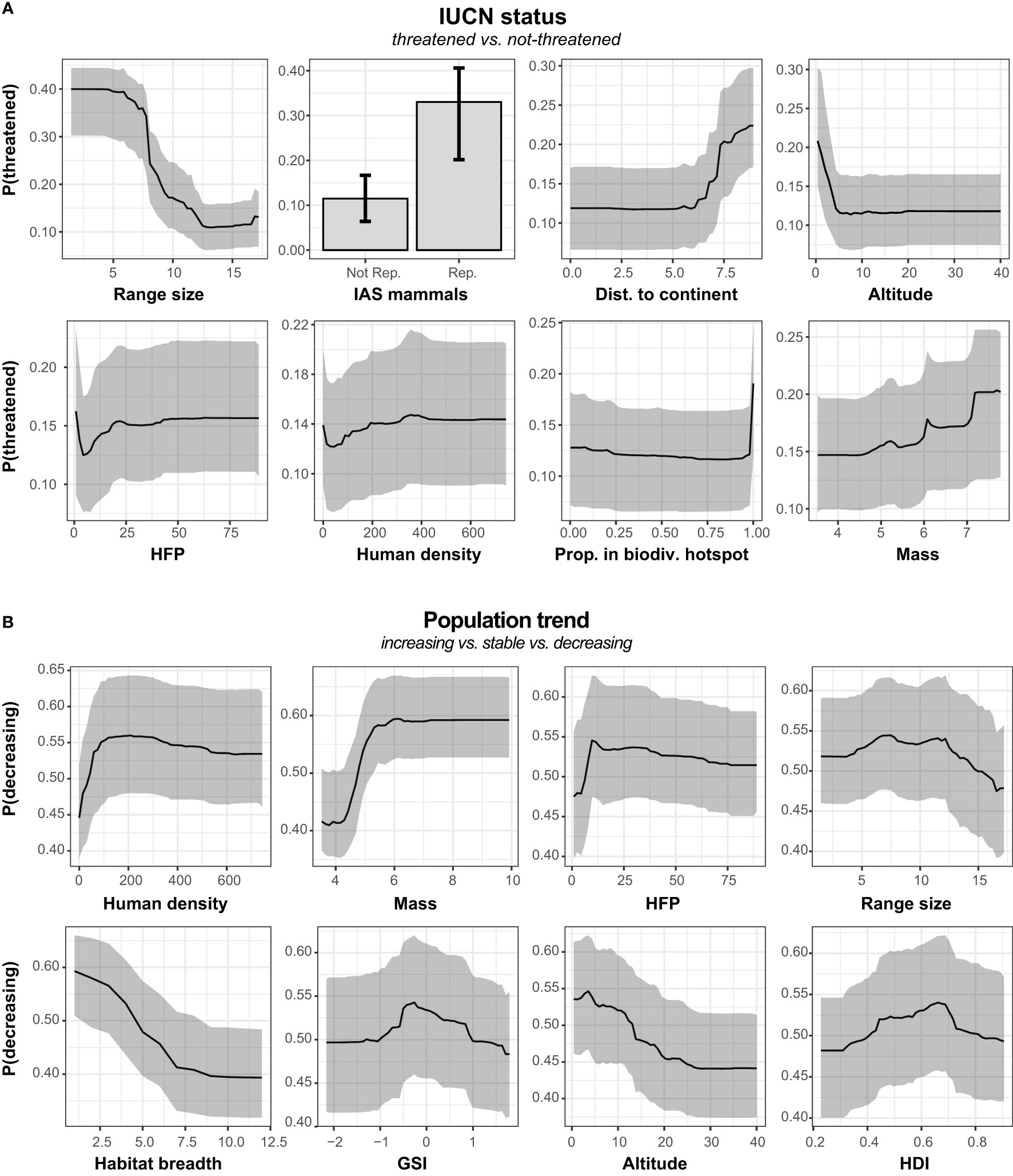
Figure 3 Partial dependence plots illustrating (A) the probability to be threatened and (B) the probability to have decreasing population trend in relation to the top eight predictors according to relative importance (Figures 2A, B). See Supplementary Material 2, Figure A5–A6 for partial dependence plots of all explanatory variables.
3.1.2 Population trends
The RF model correctly classified 73% of species according to population trends, with a OOB error of 27%. In addition, the percentage of true positive was of 74%, 69% and 64% for decreasing, stable and increasing species, respectively. On the other hand, the percentage of true negative was of 80%, 89% and 94% for decreasing, stable and increasing species’ population trends, respectively. Although goodness-of-fit was lower than for the previous model, Cohen’s kappa still indicated a species classification differing from a random classification [Cohen’s kappa = 0.48, P< 0.0001]. The eight most important variables predicting population trends were in decreasing order: human density within the species historical geographic range distribution, species body mass, HFP, species historical geographic range size, species habitat breadth, GSI, species altitude distribution, and HDI (Figure 2B). Based on the comparison of 95%CI, these eight variables did not seem to significantly differ in terms of importance (Figure 2B, see Supplementary Material 2, Figure A4 for all variables importance values). Based on partial dependence plots, risk of population decline was significantly lower for species with a low body weight living in areas characterized by high human density (Figure 3B). In addition, a higher risk of decline was associated with species with narrow habitat breadth and living at low altitudes (Figure 3B). Although other variables were important in predicting species’ risk of decline based on the RF model, partial dependent plots suggest that they are not directly associated to it (Figure 3B; see Supplementary Material 2, Figure A6 for all variables).
3.2 Scientific attention
Our literature survey resulted in 3,537 research articles across the 369 Columbidae species, in scientific disciplines related to environment, ecology and evolution. From these articles, 405 concerned multiple species. Scientific attention (assessed from the number of published articles) differed markedly between columbid species, ranging from 0 to 1363 (median = 1, interquartile = 3). Overall, 10 species (about 3% all species) accounted for 77% of all research articles published since 1966. The most commonly studies species were the Common Wood Pigeon (Columba palumbus, 1363 articles), the Rock Pigeon (Columba livia, 1079), the Mourning Dove (Zenaida macroura, 309), the European Turtle-Dove (Streptopelia turtur, 121), the Eurasian Collared-Dove (Streptopelia decaocto, 117), the Common Ground Dove (Columbina passerina, 115), the Malagasy Turtle Dove (Nesoenas picturatus, 93), the Zenaida Dove (Zenaida aurita, 79), the White-winged Dove (Zenaida asiatica, 65) and the Plain-breasted Ground Dove (Columbina minuta, 46). Among them, only the Eurasian Turtle Dove is listed as vulnerable with a high risk of extinction, whereas the nine other species are considered of least-concern. In contrast, we did not find any article for 151 (41%) species. Overall, scientific attention was negatively associated with IUCN status [Jonckheere-Terpstra test, JT= 13864, P = 0.006].
Research laboratories in North America and Europe contributed the most to research on columbid species, accounting for, respectively, about 43% and 32% of articles published in peer-reviewed journals. Scientific attention by country was positively correlated with GSI and HDI, but negatively with HFP (Supplementary Material 2, Table S5). Based on geographic distribution of species, species inhabiting close to “hotspots” of research on columbid species were the most studied (Figure 4, 5). The geographic distribution of scientific attention was also positively correlated with GSI and HDI, and, contrary to scientific attention per se, to HFP (Figure 4). Accordingly, the vast majority of unstudied species were located in the southern hemisphere, while most of the 10 most studied species were totally or partially located in the northern hemisphere (Figure 5).
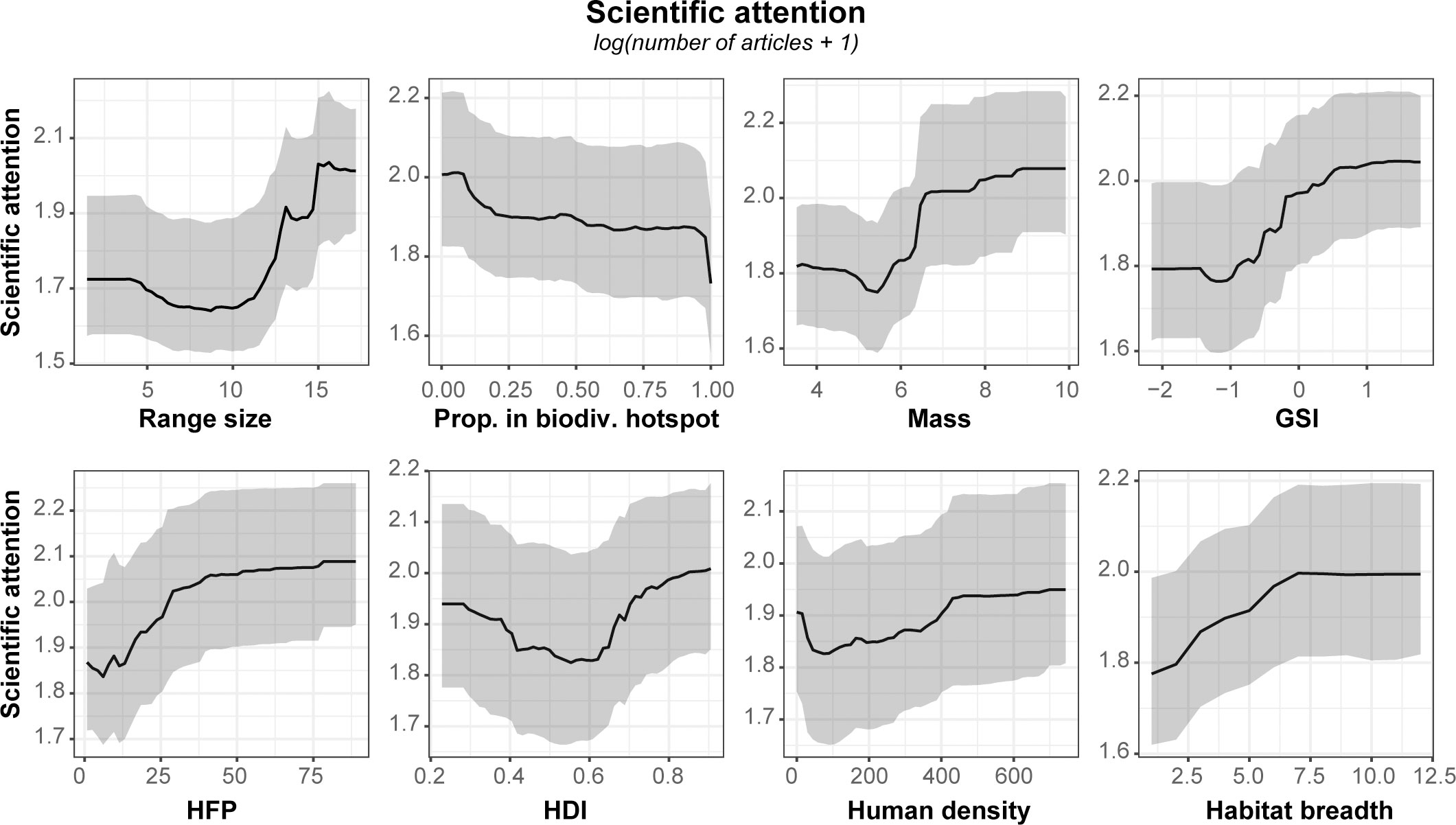
Figure 4 Partial dependence plots illustrating scientific attention in relation to the top eight predictors according to relative importance (Figure 2C). See Supplementary Material 2, Figure A7 for partial dependence plots of all explanatory variables.
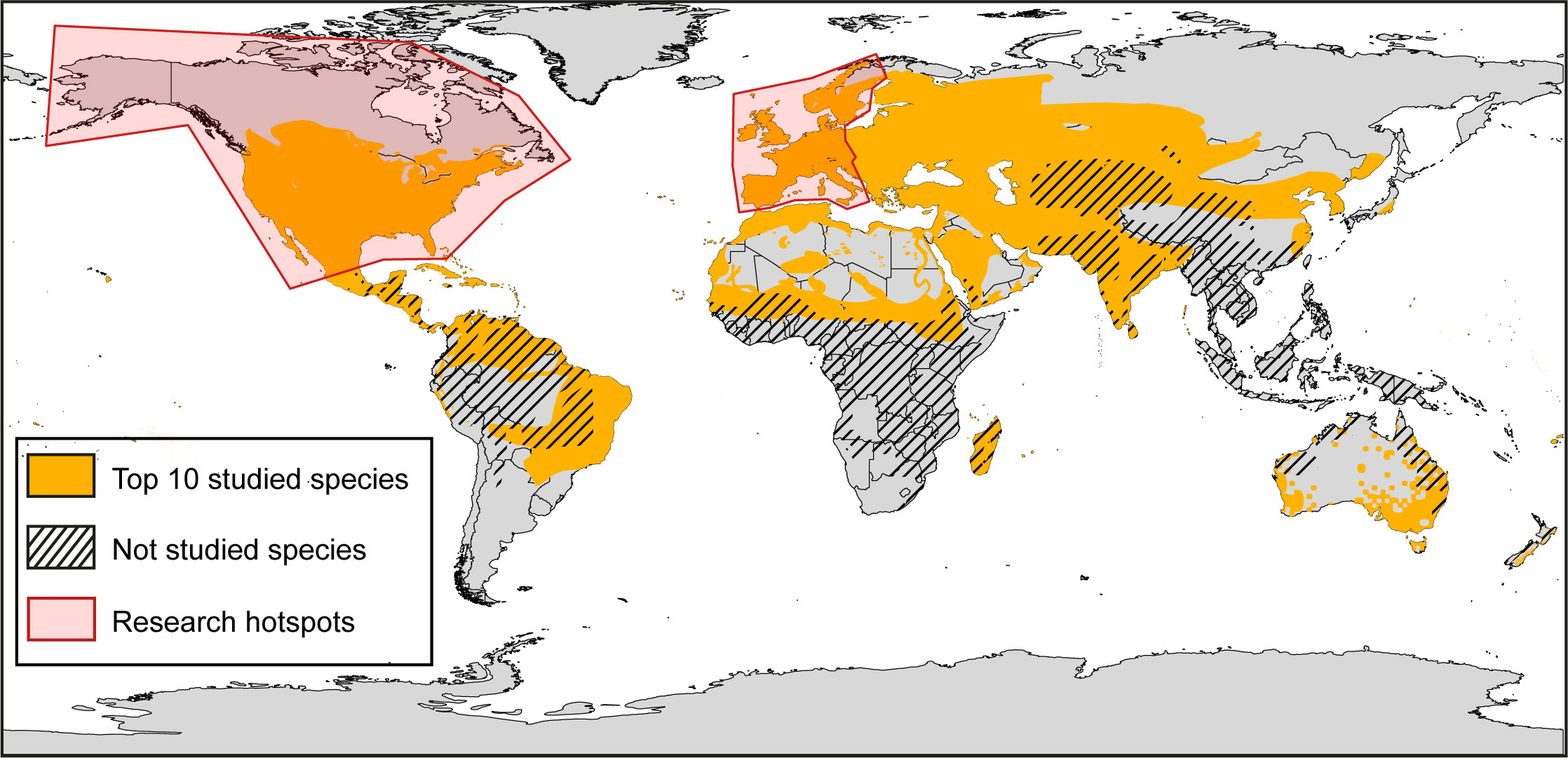
Figure 5 Maps illustrating the geographical distribution of the top 10 studied species and not studied species. The two most important research hotspots correspond to the regions of North America (USA + Canada) in the left, and of Western Europe in the right. They represent about 70% of the scientific production in natural sciences among the first 50 countries according to the Nature index database (https://www.nature.com/nature-index).
Based on RF models, explanatory variables accounted for 45% of the observed variation in scientific attention among columbid species. The most important variables were species historical geographic range size, proportion of species range in current biodiversity hotspots, species body mass, GSI, HFP, HDI, human density within species range, and species habitat breadth (Figure 2C). In terms of relative importance, the historical range size of species did not differ from the proportion of species range in current biodiversity hotspots, species body mass of species, GSI, and species habitat breadth, as evidenced by their 95%CI (Figure 2C). The importance of other variables was lower (Supplementary Material 2, Figure A4). Partial dependence plots show that species with important range size, high body mass, occurring in countries with highest values of GSI, and located in ecosystems highly impacted by human activities (high HFP value) have been the most studied (Figure 4). Although no clear association was observed with the proportion of species range within hotspots of biodiversity, there was a trend for species exclusively occurring in biodiversity of hotspots to be less studied than others (Figure 4). In addition, we observed a positive trend relating scientific attention to species habitat breadth (Figure 4). Although the others variables were more or less important to explain scientific attention variation based on RF model, their variations were not directly associated to scientific attention (see Supplementary Material 2, Figure A7 for all variables).
3.3 Extinction risk predictions biased by scientific attention
Bootstrapped sensitivity and specificity [95% CI] of the extinction risk model respectively indicated that 78% [46%; 100%] of actual non-threatened species and 87% [78%; 95%] of actual threatened species were correctly identified when randomly using 80% of original dataset as training data, and the remaining 20% as tested data. Moreover, bootstrapped TSS [95% CI] was significantly different from zero (0.649 [0.328; 0.902]) showing that random training datasets classified species better than a random classification. Besides, these values were not significantly different from those calculated from the global model, thus suggesting an absence of overfitting.
Among the 352 extant columbid species, the prediction of extinction risk of 50 species (14%) was inconsistent with their actual status (see Supplementary Material 2, Table A5 for a detailed list). On the one hand, the model predicted a higher extinction risk than that indicated by the IUCN red list for eight of them (16%), with estimated probabilities to be classified as “threatened” by the model being above or equal to 50%. On the other hand, the model suggested to downlist most of these species (42, 84%) as “not threatened”. However, their average estimated probability to be classified as “threatened” by the model was 30% ± 13% (SD), and only ten of them had an estimated probability to be classified as “threatened” inferior to 20%. Importantly, we found that mismatches predictions in term of extinction risk were significantly related to scientific attention. Species with mismatches prediction were in fact less studied than those with predictions consistent with the IUCN red list [Chi-square test, = 7.336, P = 0.007]. The only species classified as data deficient in the IUCN red list, Columba oliviae, was classified as “not threatened” by the model, with a probability of 79%.
4 Discussion
Since Walker (2007), the number of identified species of Columbidae has increased from 317 to 370 species. Meanwhile, the proportions of extinct (4.30% in the present study and 3.70% in Walker, 2007), threatened (19.24% and 18.61%, respectively) or non-threatened species (75.88% and 77.29%, respectively) have however remained stable. Walker (2007) described the geographic distribution of columbid species across the world according to their extinction risk and associated threats, as listed in the IUCN red list. We went further by quantitatively assessing to what extent various biological, ecological, environmental and socio-political drivers influence extinction risk and population trends of columbid species as well as interspecific variation in levels of scientific attention. In addition, we showed, for the first time, that low scientific attention can be responsible for incorrect estimation of the conservation status of columbid species.
4.1 Extinction risk
4.1.1 Ecological and biological drivers
Overall, our results tend to agree with previous findings (Walker, 2007) about the main drivers of extinction risk. However, we show that the use of both IUCN status and population trends are complementary in the identification of these drivers. Using both of them allowed us to identify several biological and ecological traits that make columbid species more prone to extinction. First, range-restricted species appeared more prone to extinction than more geographically widespread ones. Such relationship might seem obvious when using IUCN status as a proxy of extinction risk, because geographic range size is considered in its assessment (Fisher and Owens, 2004). However, geographic range size of columbid species was also positively associated with risk of population decline, thus confirming this factor as an important predictor of global extinction risk. Although the impact of geographic range size on extinction risk is complex, small range size is often associated with restricted ecological niche and small population size. Indeed, the number of habitats or resources required for survival and reproduction of individuals and, hence, population growth, often covary with geographic range size (Gaston, 2003; Peterson et al., 2011; Saupe et al., 2015). Moreover, large-range species are overall less likely to be affected by human disturbance or destruction of their natural environment, or by stochastic events, in their entire geographic range at once (Gaston, 2003; Fisher and Owens, 2004). However, some particular migrating columbid species, despite having a large range size, might be vulnerable to disturbance or damage occurring in their breeding, wintering or stop-over areas, as shown in the case of the Woodpigeon (C. palumba; Schumm et al., 2022; but see Bendjoudi et al., 2015) or the Turtle Dove (S. turtur; Eraud et al., 2013; Schumm et al., 2021). The negative relationship between extinction risk and geographic range size has been evidenced in a large range of terrestrial and marine vertebrate species (Davidson et al., 2012; Di Marco et al., 2014; Böhm et al., 2016; Buechley et al., 2019), and at different geological time scales (Harnik et al., 2012).
Second, island columbid species appeared to be more prone to extinction than continental ones, and even more so the further the distance of islands to mainland. Indeed, in addition to usually having smaller geographic range size than continental species, island-species have evolved in isolation, under particular selection pressures (e.g., relaxed predation and parasitism), making them particularly vulnerable to disturbances such as overexploitation and any kind of negative interactions with invasive species (Purvis et al., 2000a; Purvis et al., 2000b; Boyer, 2010; Loehle and Eschenbach, 2012). Introduced pathogens may also have a significant negative impact on the persistence of isolated populations. For instance, a pathogenic strain of Trichomonas gallinae, which might have been introduced in Mauritius Island, increased adult and nestling mortality in the endangered Pink Pigeon, Neosoenas mayeri (Swinnerton et al., 2005; Bunbury et al., 2007; Bunbury et al., 2008). In mammals and birds, 95% of known extinctions concerned island-species (Loehle and Eschenbach, 2012). However, smaller geographic range size of most insular species might be the most important variable in explaining species extinction risk (Manne et al., 1999). Among columbid species, all known extinct species inhabited islands, excepted for the Passenger Pigeon (Ectopistes migratorius), suggesting that being an island-species is at least as much important as geographic range size, especially when adding other sources of extinction such as overexploitation, invasive species or climate change (Courchamp et al., 2003; Courchamp et al., 2014; Wood et al., 2017). For instance, the two largest known and now extinct columbid species, the iconic Dodo, Raphus cucullatus, and the Rodrigues Solitaire, Pezophaps solitaria, were both endemic to Mauritius islands and flightless. Their extinction was probably due to a combination of factors, including large body size, island endemism, low population growth, small population size and small geographic range size, making them particularly vulnerable to habitat destruction, hunting and invasive species (Duncan et al., 2002; Fisher and Owens, 2004; Hume, 2006; Hilbers et al., 2016). Our results indeed suggest that the extinction risk of columbid species increases with their body mass, as shown in diverse taxa (Gaston and Blackburn, 1995; Davidson et al., 2009; Fritz et al., 2009; Böhm et al., 2016). The influence of body size could actually be multifactorial, and be exacerbated by other external factors like overexploitation. Indeed, larger species are often preferred as game species (Duncan et al., 2002; Whytock et al., 2016; Ripple et al., 2017).
Although hunting has been recognized among the most important threats within Columbidae, concerning 77% of threatened species (Walker, 2007), and that inferential statistics performed in this study (see Supplementary Material 2, Table A1–A2) showed that threatened species are significantly more exposed to it, our models did not identify hunting-related drivers (i.e., subsistence use and sport hunting) as important variables explaining extinction risk. This does not mean that the impact of hunting exposure is null, but that other variables provide a better discriminant classification into RF models to evaluate extinction risk of columbids. It is obvious that hunting has a direct influence on the mortality rate of harvested populations, and, hence, can be a contributing driver to extinction risk for some species (e.g., the European Turtle Dove, Streptopelia turtur, Lormée et al., 2020), especially when it is not regulated or not performed in a sustainable way. However, the assessment of extinction risk requires a more comprehensive approach, considering various drivers and threats, as discussed in management recommendations for the European Turtle Dove (Bacon et al., 2023). The global influence of hunting might thus be lower than that of other threats, such as habitat loss and invasive species. However, its impact on game populations might be exacerbated when combined with such threats (Romero-Muñoz et al., 2019; Romero-Muñoz et al., 2020; Bogoni et al., 2022). For instance, the extinction of the iconic Passenger Pigeon was caused by both unregulated commercial hunting and significant destruction of its breeding habitat (i.e., a decrease estimated about 53%; Stanton, 2014). Furthermore, a more precise metrics of hunting pressure would probably be more informative than mere exposure to hunting (Bellard et al., 2022) and could have enhanced the performance of our RF models. Unfortunately, such information is unavailable for a large majority of game columbids.
Habitat breadth also appeared to influence extinction risk, confirming a general trend (Norris and Harper, 2004; Devictor et al., 2008; Clavel et al., 2011; Callaghan et al., 2019). Although ecological specialization does not always limit the capacity of species to persist (Benito et al., 2020), it might be evolutionary disadvantageous in environments exposed to changes and perturbations (Davies et al., 2004; Colles et al., 2009; Clavel et al., 2011; Ducatez et al., 2014; Morelli et al., 2020). Indeed, specialist species often tend to be associated with small population size, restricted geographic range size and limited use of resources and habitats, what may make them particularly vulnerable when exposed to environmental changes and disturbances (McKinney, 1997; Davies et al., 2004; Colles et al., 2009).
4.1.2 Anthropogenic drivers
Not surprisingly, our analysis points to alien invasive species as an important factor affecting the risk of extinction of columbid species (Walker, 2007). Exotic species are indeed a major threat to biodiversity, as a result of predation, parasitism, competition, hybridization with native species and the disturbance of native ecosystems (Mack et al., 2000; Sax and Gaines, 2008; Walsh et al., 2012). Invasive mammals, especially rodents (e.g., rats Rattus sp.) and cats (Felis catus), are the most damaging group of alien species across all ecosystems. Both are responsible of more than 30% of all known contemporary extinctions, especially on islands (Doherty et al., 2016). Invasive species can predate native columbid species (Rodriguez-Estrella et al., 1991; Tidemann et al., 1994; Zarzoso-Lacoste et al., 2016; Lamelas-López et al., 2020), compete with them for resources (James and Clout, 1996; Bond et al., 2019), or transmit pathogens (Stimmelmayr et al., 2012). Based on IUCN data, 9% of all columbid species have been reported to be exposed to invasive rats, 8% to cats, and 12% to other mammals (IUCN, 2021). Among non-threatened species, 3% of species have been reported to be exposed to invasive mammals, compared to 35% for threatened species and 77% for extinct species. However, the precise impact of invasive mammal species on the demography of columbid species of patrimonial interest in the wild remains to be quantitatively assessed in order to assess to what extent it compares in importance with hunting pressure or habitat loss and thus provide a more comprehensive and integrative approach to the management of species/populations.
Although global warming is supposed to increase extinction risk among species living at high altitude (Williams et al., 2003; Shoo et al., 2005; Sekercioglu et al., 2008; Bässler et al., 2010; La Sorte and Jetz, 2010), we found no evidence for a positive relationship between extinction risk and elevation in columbids. The consequences of global warming on extinction risk at higher elevation might not be felt yet (Bellard et al., 2012) in columbid species, or might have been hidden in our analysis by variation in habitat breadth and more intense human activities at lower elevations. Indeed, landscape heterogeneity tends to decrease with increased elevation, and species found at elevation tend to have a higher degree of ecological specialization than those found at lower elevation (Reif et al., 2013; Rivas-Salvador et al., 2019). Lower elevations are also associated with higher anthropogenic transformation, inducing various negative effects on local biodiversity (Ellis et al., 2010; Reif et al., 2013; White and Bennett, 2015; Smith et al., 2019). Further attention should then be given to the influence of elevation on the demographic trends of columbid species in relation to climate change and habitat modification.
Several other drivers of anthropogenic origin appeared to contribute, directly or indirectly, to increase the extinction risk of columbid species, such as low levels of governmental stability and poor socioeconomics and/or the loss and disturbance of natural habitats through the development of HFP. HFP provides an index of human conversion of natural terrestrial ecosystems based on the measure of eight different anthropogenic pressures related to urbanization, agriculture and human density (Venter et al., 2016), and can therefore be regarded as a proxy of habitat degradation or loss. Yearly variations in HFP have been found to be a good predictor of extinction risk in terrestrial mammals (Di Marco et al., 2018). Although some columbid species flourish in landscapes modified by humans, such as the widespread Feral Pigeon, Columba livia domestica (Carlen et al., 2021), the Zenaida Dove, Zenaida aurita (Wolff et al., 2018), or the invasive Eurasian Collared-Dove, Streptopelia decaocto (Luna et al., 2018), most species rely on their natural habitats to persist. For instance, the occupancy of the Kereru, Hemiphaga novaeseelandiae, an endemic New Zealand Pigeon, has been affected by habitat loss resulting from European settlement in New Zealand (Carpenter et al., 2021). Following Walker (2007), the present study confirms that the loss of natural habitat is the greatest cause of extinction and populations decline in columbid species.
Governmental and socioeconomic factors were also found to affect the extinction risk of columbid species, with species inhabiting in developing countries being more exposed to extinction risk. Public policies can have negative or positive influences on biodiversity, through supporting or not reliable environmental and conservation projects and by protecting or not most vulnerable species and/or sensitive ecosystems (Chapron, 2014; Ripple et al., 2016). For instance, the Pink Pigeon, Nesoenas mayeri, have been downlisted twice, in the IUCN red list, from critically-endangered to vulnerable thanks to conservation projects lead by conservationists, private foundations and the Mauritian government (Tatayah, 2019). Globally, the socioeconomic level of countries tends to influence positively the persistence of species (Forester and Machlist, 1996; Dietz and Adger, 2003). For instance, Bösch (2021) showed that the level and speed of countries’ economic-institutional development tend to be negatively associated with illegal logging, and hence, with habitat loss and biodiversity decline. The same is true for wildlife trafficking and hunting pressure, especially in developing countries where wildlife can be the sole source of proteins for isolated and/or low-income communities (Robinson and Bennett, 2002; Fa et al., 2003; Fa et al., 2009; Borgerson et al., 2016; Lescuyer and Nasi, 2016). Legal and illegal hunting can be practiced to obtain food or increased social status, or as a recreational or traditional activity (Phelps et al., 2016; Stirnemann et al., 2018). Indeed, wildlife meat consumption is not always associated with poverty and can also be a sign of wealth (Fa et al., 2009). This is for instance the case in the Pacific islands where poor people hunt both the Pacific Pigeon, Ducula pacifica, and the Tooth-billed Pigeon, Didunculus strigiristris, to sell their meat to high-income populations (Phelps et al., 2016; Stirnemann et al., 2018).
4.2 Scientific attention
In strong contrast with the ubiquity of the family Columbidae throughout the world, the order Columbiformes is among the least studied orders in the Aves class (8th/25, Ducatez and Lefebvre, 2014). The same trend has been observed, at a lower geographical scale, in the Caribbean region (Devenish-Nelson et al., 2019). One reason for the low attention given to columbids could be that this family does not include any charismatic species (Seddon et al., 2005; Devenish-Nelson et al., 2019), excepted for the iconic Dodo and Passenger Pigeon that became textbook cases due to their extinction history (Kyne and Adams, 2017).
Scientific attention on columbid species appeared to be geographically biased and independent of species extinction risk, as shown by the negative association between scientific attention, the proportion of species in biodiversity hotspots and the influence of both HDI and GSI (Livingston et al., 2016; Tydecks et al., 2018; Melles et al., 2019). In addition, we found that island-endemic columbid species were on average less studied than continental species, and even more so the farther they are from mainland (see also Brooks et al., 2008). Contrary to Ducatez and Lefebvre (2014), we did not find any association between scientific attention and clutch size or generation time, probably due to low variability in these two variables among columbids.
Most of the research on columbid species is actually done by North-American and European researchers working on local species, accounting, respectively, for about 43% and 32% of all articles published in peer-reviewed journals, whereas species living between Fiji and Indonesia, the most important hotspot for threatened columbid species and diversity (Walker, 2007), are among the least studied ones. The same geographical bias has been found in several studies focusing on diverse taxa (Ducatez and Lefebvre, 2014; Tydecks et al., 2018; Melles et al., 2019). It can be partly explained by logistic constraints, local poverty, political instability, geographic isolation and lack of local expertise in developing countries (Clark, 1985; de Lima et al., 2011; Amano and Sutherland, 2013; Wilson et al., 2016; Reboredo Segovia et al., 2020; Vallès et al., 2021).
Widespread columbids, in terms of geographic distribution or in terms of number of different habitats used, and relatively large-bodied species (>100g according to Figure 4) seemed more studied than others, as highlighted by Ducatez and Lefebvre (2014), who focused their research on bird Orders. More widespread species are presumably more accessible and have thus more chance to be studied than less widespread ones (Morales and Traba, 2016; Yarwood et al., 2019), while large species are usually easier to locate and observe than more discrete and smaller species (Ripple et al., 2017; Yarwood et al., 2019). Furthermore, we found that scientific attention on columbid species was positively associated with human density and human footprint, suggesting that columbid species inhabiting areas with high human density and highly urbanized were preferentially studied. As explained by Ibáñez-Álamo et al. (2017), species living close to humans, such as feral pigeons (e.g., Perez-Sancho et al., 2020), may receive more attention because of economic and public health issues (Lindahl and Magnusson, 2020) and/or because they are easier to study. Similarly, some applied research has been conducted on columbid species living close to humans in relation to negative impacts on crops (e.g, Murton and Jones, 1973; van Niekerk and van Ginkel, 2009; Firake et al., 2016; Verga et al., 2021). However, columbid species causing damages on crops may also experience higher extinction risk due retaliatory actions, including hunting and poisoning, by farmers (Marrero et al., 2004; Cuesta Hermira and Michalski, 2022).
5 Conclusion and perspectives
Our results quantitatively confirmed earlier findings on the main factors influencing extinction risk and population decline in columbids (Walker, 2007) and revealed large disparity in scientific attention given to columbid species, with the most threatened ones being the least studied. In this respect, species for which predicted extinction risk status were inconsistent with actual status were also the least studied. We therefore recommend to pay more attention to these species, and more generally to specialist species, restricted-range species, particularly insular ones, and/or large species, in relation to habitat disturbance and loss, in order to better estimate direct and specific extinction risk. In addition, investigations on urban populations of columbid species may help understanding interspecific variation in adaptive potential in relation to urbanization and global change.
Secondly, we strongly recommend to investigate the relative importance of sources of mortality of pigeons and doves in the wild in order to gain a more complete picture of the threats weighing on them. In this respect, it would be of interest to assess the relative importance of legal and illegal hunting compared to that of predation by exotic invasive predators in the decline of species. Columbids are indeed particularly appreciated by hunters, but are also exposed to various introduced potential predators in various regions. Although the impact of invasive mammal predators is widely acknowledged as an important threat to native wildlife, precise quantitative assessment is often lacking, particularly for columbid species. To that end, cooperation among hunters, scientists, wildlife officers and agencies should be encouraged, particularly where hunting traditions are an important part of local culture. Although difficult to implement, the regulation of invasive predators or their total eradication may have immediate positive impacts on native populations and ecosystems (Le Corre et al., 2015, Miller-ter Kuile et al., 2020).
Based on the influence of socioeconomic factors revealed here, we also recommend to develop coordinated efforts to better inform governments, public agencies and the general public of the key ecological role played by columbids and of the importance of conserving populations and associated habitats. In that respect, both extinct and extant columbid species could be used more widely in education programs about the erosion of biodiversity. Finally, fair transnational cooperation and capacity building in conservation-oriented research on columbids should be encouraged, especially between small island developing states and developed countries.
Data availability statement
The datasets presented in this study can be found in online repositories. The names of the repository/repositories and accession number(s) can be found below: caribaea.org.
Author contributions
CC: Conceptualization, methodology, formal analysis, data collection, data curation, writing – original draft, visualization. AJ-P: Data collection. EB: Conceptualization, supervision, resources, project administration. FC: Conceptualization, validation, writing – original draft, supervision, project administration. All authors contributed to the article and approved the submitted version.
Funding
CC was funded by a joint-doctoral grant from the Conseil Régional de Guadeloupe and Caribaea Initiative.
Acknowledgments
We thank Caribaea Initiative and the Conseil Régional de Guadeloupe for financial support. We thank Raphaël Pasquier for assistance with the computer cluster at the Centre Commun de Calcul Intensif of the Université des Antilles and two referees for constructive comments. We thank Sophie Labaude for original drawings of columbid species.
Conflict of interest
The authors declare that the research was conducted in the absence of any commercial or financial relationships that could be construed as a potential conflict of interest.
Publisher’s note
All claims expressed in this article are solely those of the authors and do not necessarily represent those of their affiliated organizations, or those of the publisher, the editors and the reviewers. Any product that may be evaluated in this article, or claim that may be made by its manufacturer, is not guaranteed or endorsed by the publisher.
Supplementary material
The Supplementary Material for this article can be found online at: https://www.frontiersin.org/articles/10.3389/fevo.2023.1141072/full#supplementary-material
References
Allouche O., Tsoar A., Kadmon R. (2006). Assessing the accuracy of species distribution models: prevalence, kappa and the true skill statistic (TSS). J. Appl. Ecol. 43, 1223–1232. doi: 10.1111/j.1365-2664.2006.01214.x
Amano T., Sutherland W. J. (2013). Four barriers to the global understanding of biodiversity conservation: wealth, language, geographical location and security. Proc. R. Soc B Biol. Sci. 280, 20122649. doi: 10.1098/rspb.2012.2649
Andelman S. J., Fagan W. F. (2000). Umbrellas and flagships: efficient conservation surrogates or expensive mistakes? Proc. Natl. Acad. Sci. 97, 5954–5959. doi: 10.1073/pnas.100126797
Ando H., Mori Y., Nishihiro M., Mizukoshi K., Akaike M., Kitamura W., et al. (2021). Highly mobile seed predators contribute to interisland seed dispersal within an oceanic archipelago. Oikos 08068. doi: 10.1111/oik.08068
Bacon L., Guillemain M., Arroyo B., Carboneras C., Fay R., Sauser C., et al. (2023). Predominant role of survival on the population dynamics of a threatened species: evidence from prospective analyses and implication for hunting regulation. J. Ornithol 164, 275–285. doi: 10.1007/s10336-022-02038-4
Balmford A., Green R. E., Jenkins M. (2003). Measuring the changing state of nature. Trends Ecol. Evol. 18, 326–330. doi: 10.1016/S0169-5347(03)00067-3
Banks R. C., Weckstein J. D., Remsen J. J. V., Johnson K. P. (2013). Classification of a clade of new world doves (Columbidae: Zenaidini). Zootaxa 3669, 184. doi: 10.11646/Zootaxa.3669.2.11
Barnosky A. D., Matzke N., Tomiya S., Wogan G. O. U., Swartz B., Quental T. B., et al. (2011). Has the earth’s sixth mass extinction already arrived? Nature 471, 51–57. doi: 10.1038/nature09678
Bässler C., Müller J., Hothorn T., Kneib T., Badeck F., Dziock F. (2010). Estimation of the extinction risk for high-montane species as a consequence of global warming and assessment of their suitability as cross-taxon indicators. Ecol. Indic. 10, 341–352. doi: 10.1016/j.ecolind.2009.06.014
Baynham-Herd Z., Amano T., Sutherland W. J., Donald P. F. (2018). Governance explains variation in national responses to the biodiversity crisis. Environ Conserv 45, 407–418. doi: 10.1017/S037689291700056X
Bellard C., Bertelsmeier C., Leadley P., Thuiller W., Courchamp F. (2012). Impacts of climate change on the future of biodiversity. Ecol. Lett. 15, 365–377. doi: 10.1111/j.1461-0248.2011.01736.x
Bellard C., Marino C., Courchamp F. (2022). Ranking threats to biodiversity and why it doesn’t matter. Nat. Commun. 13, 2616. doi: 10.1038/s41467-022-30339-y
Bendjoudi D., Voisin J. F., Doumandji S., Merabet A., Benyounes N., Chenchouni H. (2015). Rapid increase in numbers and change of land-use in two expanding columbidae species (Columba palumbus and Streptopelia decaocto) in Algeria. Avian Res. 6, 1–9. doi: 10.1186/s40657-015-0027-9
Benito B. M., Gil-Romera G., Birks H. J. B. (2020). Ecological memory at millennial time-scales: the importance of data constraints, species longevity and niche features. Ecography 43, 1–10. doi: 10.1111/ecog.04772
Bennett P. M., Owens I. P. F. (1997). Variation in extinction risk among birds: chance or evolutionary predisposition? Proc. R. Soc London. Ser. B Biol. Sci. 264, 401–408. doi: 10.1098/rspb.1997.0057
BirdLife International (2021) Birdlife data zone. Available at: http://datazone.birdlife.org/home (Accessed 4.19.21).
BirdLife International (2022) Birdlife data zone. Available at: http://datazone.birdlife.org/species/search (Accessed 10.24.22).
BirdLife International and Handbook of the Birds of the World (2018) Bird species distribution maps of the world. Available at: http://datazone.birdlife.org/species/requestdis.
Bogoni J. A., Ferraz K. M. P. M. B., Peres C. A. (2022). Continental-scale local extinctions in mammal assemblages are synergistically induced by habitat loss and hunting pressure. Biol. Conserv. 272, 109635. doi: 10.1016/j.biocon.2022.109635
Böhm M., Williams R., Bramhall H. R., McMillan K. M., Davidson A. D., Garcia A., et al. (2016). Correlates of extinction risk in squamate reptiles: the relative importance of biology, geography, threat and range size. Glob. Ecol. Biogeogr. 25, 391–405. doi: 10.1111/geb.12419
Bond A. L., Brooke M. D. L., Cuthbert R. J., Lavers J. L., Mcclelland G. T. W., Churchyard T., et al. (2019). Population status of four endemic land bird species after an unsuccessful rodent eradication on Henderson island. Bird Conserv. Int. 29, 124–135. doi: 10.1017/S0959270918000072
Borgerson C., McKean M. A., Sutherland M. R., Godfrey L. R. (2016). Who hunts lemurs and why they hunt them. Biol. Conserv. 197, 124–130. doi: 10.1016/j.biocon.2016.02.012
Bösch M. (2021). Institutional quality, economic development and illegal logging: a quantitative cross-national analysis. Eur. J. For. Res. 140, 1049–1064. doi: 10.1007/s10342-021-01382-z
Boyer A. G. (2010). Consistent ecological selectivity through time in pacific island avian extinctions. Conserv. Biol. 24, 511–519. doi: 10.1111/j.1523-1739.2009.01341.x
Brambilla M., Gustin M., Celada C. (2013). Species appeal predicts conservation status. Biol. Conserv. 160, 209–213. doi: 10.1016/j.biocon.2013.02.006
Brito D., Oprea M. (2009). Mismatch of research effort and threat in avian conservation biology. Trop. Conserv. Sci. 2, 353–362. doi: 10.1177/194008290900200305
Brooks T. M., Collar N. J., Green R. E., Marsden S. J., Pain D. J. (2008). The science of bird conservation. Bird Conserv. Int. 18, S2–S12. doi: 10.1017/S0959270908000427
Brooks T. M., Mittermeier R. A., da Fonseca G. A. B., Gerlach J., Hoffmann M., Lamoreux J. F., et al. (2006). Global biodiversity conservation priorities. Science 313, 58–61. doi: 10.1126/science.1127609
Bruxaux J., Gabrielli M., Ashari H., Prŷs-Jones R., Joseph L., Milá B., et al. (2018). Recovering the evolutionary history of crowned pigeons (Columbidae: Goura): implications for the biogeography and conservation of new guinean lowland birds. Mol. Phylogenet. Evol. 120, 248–258. doi: 10.1016/j.ympev.2017.11.022
Buechley E. R., Şekercioğlu Ç.H. (2016). The avian scavenger crisis: looming extinctions, trophic cascades, and loss of critical ecosystem functions. Biol. Conserv. 198, 220–228. doi: 10.1016/j.biocon.2016.04.001
Buechley E. R., Santangeli A., Girardello M., Neate-Clegg M. H. C., Oleyar D., McClure C. J. W., et al. (2019). Global raptor research and conservation priorities: tropical raptors fall prey to knowledge gaps. Divers. Distrib. 25, 856–869. doi: 10.1111/ddi.12901
Bunbury N., Jones C. G., Greenwood A. G., Bell D. J. (2007). Trichomonas gallinae in mauritian columbids: implications for an endangered endemic. J. Wildl Dis. 43, 399–407. doi: 10.7589/0090-3558-43.3.399
Bunbury N., Jones C. G., Greenwood A. G., Bell D. J. (2008). Epidemiology and conservation implications of Trichomonas gallinae infection in the endangered Mauritian pink pigeon. Biol. Conserv. 141, 153–161. doi: 10.1016/j.biocon.2007.09.008
Callaghan C. T., Major R. E., Wilshire J. H., Martin J. M., Kingsford R. T., Cornwell W. K. (2019). Generalists are the most urban-tolerant of birds: a phylogenetically controlled analysis of ecological and life history traits using a novel continuous measure of bird responses to urbanization. Oikos 128, 845–858. doi: 10.1111/oik.06158
Camacho-Cervantes M., Schondube J. E. (2018). Habitat use by the invasive exotic Eurasian collared-dove (Streptopelia decaocto) and native dove species in the chamela-cuixmala region of West Mexico. Wilson J. Ornithol. 130, 902–907. doi: 10.1676/1559-4491.130.4.902
Cambrone C., Bezault E., Cézilly F. (2021). Efficiency of the call-broadcast method for detecting two Caribbean-endemic columbid game species. Eur. J. Wildl. Res. 67, 65. doi: 10.1007/s10344-021-01507-0
Carlen E. J., Li R., Winchell K. M. (2021). Urbanization predicts flight initiation distance in feral pigeons (Columba livia) across new York city. Anim. Behav. 178, 229–245. doi: 10.1016/j.anbehav.2021.06.021
Carpenter J. K., Walker S., Monks A., Innes J., Binny R. N., Schlesselmann A. K. V. (2021). Factors limiting kererū (Hemiphaga novaeseelandiae) populations across new Zealand. N. Z. J. Ecol. 45, 3441. doi: 10.20417/nzjecol.45.30
Carvalho M., Fa J. E., Rego F. C., Lima R. F. D., Santos G., Palmeirim J. M. (2015). Factors influencing the distribution and abundance of endemic pigeons on são tomé island (Gulf of Guinea). Bird Conserv. Int. 25, 71–86. doi: 10.1017/S0959270914000288
Chapron G. (2014). Challenge the abuse of science in setting policy. Nature 516, 289–289. doi: 10.1038/516289a
Chen C., Zeng D., Zhao Y., Wu Y., Xu J., Wang Y. (2019). Correlates of extinction risk in Chinese endemic birds. Avian Res. 10, 1–10. doi: 10.1186/s40657-019-0147-8
Christie A. P., Amano T., Martin P. A., Petrovan S. O., Shackelford G. E., Simmons B. I., et al. (2021). The challenge of biased evidence in conservation. Conserv. Biol. 35, 249–262. doi: 10.1111/cobi.13577
CIESIN, Columbia University - Center for International Earth Science Information Network (2018). Gridded population of the world, version 4 (GPWv4): population density adjusted to match 2015 revision UN WPP country totals, revision 11 (Palisades, NY: NASA Socioeconomic Data and Applications Center (SEDAC).
Clark D. B. (1985). Ecological field studies in the tropics: geographical origin of reports. Bull. Ecol. Soc Am. 66, 6–9. doi: 10.2307/20166419b
Clavel J., Julliard R., Devictor V. (2011). Worldwide decline of specialist species: toward a global functional homogenization? Front. Ecol. Environ. 9, 222–228. doi: 10.1890/080216
Colles A., Liow L. H., Prinzing A. (2009). Are specialists at risk under environmental change? neoecological, paleoecological and phylogenetic approaches. Ecol. Lett. 12, 849–863. doi: 10.1111/j.1461-0248.2009.01336.x
Conteh A., Gavin M. C., McCarter J. (2017). Assessing the impacts of war on perceived conservation capacity and threats to biodiversity. Biodivers. Conserv. 26, 983–996. doi: 10.1007/s10531-016-1283-7
Costan A. S., Sarasola J. H. (2017). Eared dove (Zenaida auriculata) granivory and its role in seed dispersal in semiarid forests of central Argentina. Ornitol. Neotrop. 28, 43–50. doi: 10.58843/ornneo.v28i0.165
Courchamp F., Chapuis J.-L., Pascal M. (2003). Mammal invaders on islands: impact, control and control impact. Biol. Rev. 78, S1464793102006061. doi: 10.1017/S1464793102006061
Courchamp F., Dunne J. A., Le Maho Y., May R. M., Thébaud C., Hochberg M. E. (2015). Fundamental ecology is fundamental. Trends Ecol. Evol. 30, 9–16. doi: 10.1016/j.tree.2014.11.005
Courchamp F., Hoffmann B. D., Russell J. C., Leclerc C., Bellard C. (2014). Climate change, sea-level rise, and conservation: keeping island biodiversity afloat. Trends Ecol. Evol. 29, 127–130. doi: 10.1016/j.tree.2014.01.001
Cuesta Hermira A. A., Michalski F. (2022). Crop damage by vertebrates in Latin America: current knowledge and potential future management directions. PeerJ 10, e13185. doi: 10.7717/peerj.13185
Cumming G., Finch S. (2005). Inference by eye: confidence intervals and how to read pictures of data. Am. Psychol. 60, 170–180. doi: 10.1037/0003-066X.60.2.170
Cutler D. R., Edwards T. C., Beard K. H., Cutler A., Hess K. T., Gibson J., et al. (2007). Random forests for classification in ecology. Ecology 88, 2783–2792. doi: 10.1890/07-0539.1
Davidson A. D., Boyer A. G., Kim H., Pompa-Mansilla S., Hamilton M. J., Costa D. P., et al. (2012). Drivers and hotspots of extinction risk in marine mammals. Proc. Natl. Acad. Sci. 109, 3395–3400. doi: 10.1073/pnas.1121469109
Davidson A. D., Hamilton M. J., Boyer A. G., Brown J. H., Ceballos G. (2009). Multiple ecological pathways to extinction in mammals. Proc. Natl. Acad. Sci. 106, 10702–10705. doi: 10.1073/pnas.0901956106
Davies K. F., Margules C. R., Lawrence J. F. (2004). A synergistic effect puts rare, specialized species at greater risk of extinction. Ecology 85, 265–271. doi: 10.1890/03-0110
del Hoyo J., Elliott A., Sargatal J., Cabot J. (1997). Handbook of the birds of the world, vol. 4: sandgrouse to cuckoos (Barcelona: Lynx Edicions).
de Lima R. F., Bird J. P., Barlow J. (2011). Research effort allocation and the conservation of restricted-range island bird species. Biol. Conserv. 144, 627–632. doi: 10.1016/j.biocon.2010.10.021
Derlindati E. J., Romano M. C., Cruz N. N., Barison C., Arengo F., Barberis I. M. (2014). Seasonal activity patterns and abundance of Andean flamingo (Phoenicoparrus andinus) at two contrasting wetlands in Argentina. Ornitol. Neotrop. 25, 317–331.
Devenish-Nelson E. S., Weidemann D., Townsend J., Nelson H. P. (2019). Patterns in island endemic forest-dependent bird research: the Caribbean as a case-study. Biodivers. Conserv. 28, 1885–1904. doi: 10.1007/s10531-019-01768-x
Devictor V., Julliard R., Jiguet F. (2008). Distribution of specialist and generalist species along spatial gradients of habitat disturbance and fragmentation. Oikos 117, 507–514. doi: 10.1111/j.0030-1299.2008.16215.x
Dietz S., Adger W. N. (2003). Economic growth, biodiversity loss and conservation effort. J. Environ. Manage. 68, 23–35. doi: 10.1016/S0301-4797(02)00231-1
Di Marco M., Buchanan G. M., Szantoi Z., Holmgren M., Grottolo Marasini G., Gross D., et al. (2014). Drivers of extinction risk in African mammals: the interplay of distribution state, human pressure, conservation response and species biology. Philos. Trans. R. Soc B Biol. Sci. 369, 20130198. doi: 10.1098/rstb.2013.0198
Di Marco M., Venter O., Possingham H. P., Watson J. E. M. (2018). Changes in human footprint drive changes in species extinction risk. Nat. Commun. 9, 4621. doi: 10.1038/s41467-018-07049-5
Doherty T. S., Glen A. S., Nimmo D. G., Ritchie E. G., Dickman C. R. (2016). Invasive predators and global biodiversity loss. Proc. Natl. Acad. Sci. 113, 11261–11265. doi: 10.1073/pnas.1602480113
dos Santos J. W., Correia R. A., Malhado A. C. M., Campos-Silva J. V., Teles D., Jepson P., et al. (2020). Drivers of taxonomic bias in conservation research: a global analysis of terrestrial mammals. Anim. Conserv. 23, 679–688. doi: 10.1111/acv.12586
Ducatez S., Lefebvre L. (2014). Patterns of research effort in birds. PloS One 9, e89955. doi: 10.1371/journal.pone.0089955
Ducatez S., Tingley R., Shine R. (2014). Using species co-occurrence patterns to quantify relative habitat breadth in terrestrial vertebrates. Ecosphere 5, art152. doi: 10.1890/ES14-00332.1
Duncan R. P., Blackburn T. M., Worthy T. H. (2002). Prehistoric bird extinctions and human hunting. Proc. R. Soc London B 269, 517–521. doi: 10.1098/rspb.2001.1918
Ellis E. C., Klein Goldewijk K., Siebert S., Lightman D., Ramankutty N. (2010). Anthropogenic transformation of the biomes 1700 to 2000. Glob. Ecol. Biogeogr. 19, 589–606. doi: 10.1111/j.1466-8238.2010.00540.x
Eraud C., Rivière M., Lormée H., Fox J. W., Ducamp J. J., Boutin J. M. (2013). Migration routes and staging areas of trans-Saharan turtle doves appraised from light-level geolocators. PloS One 8, e59396. doi: 10.1371/journal.pone.0059396
Evans D. M., Barnard P., Koh L. P., Chapman C. A., Altwegg R., Garner T. W. J., et al. (2012). Funding nature conservation: who pays? Anim. Conserv. 15, 215–216. doi: 10.1111/j.1469-1795.2012.00550.x
Fa J. E., Albrechtsen L., Johnson P. J., Macdonald D. W. (2009). Linkages between household wealth, bushmeat and other animal protein consumption are not invariant: evidence from Rio muni, equatorial Guinea. Anim. Conserv. 12, 599–610. doi: 10.1111/j.1469-1795.2009.00289.x
Fa J. E., Currie D., Meeuwig J. (2003). Bushmeat and food security in the Congo basin: linkages between wildlife and people’s future. Environ. Conserv. 30, 71–78. doi: 10.1017/S0376892903000067
Fey K., Vuorisalo T., Lehikoinen A., Selonen V. (2015). Urbanisation of the wood pigeon (Columba palumbus) in finland. landsc. Urban Plan. 134, 188–194. doi: 10.1016/j.landurbplan.2014.10.015
Firake D. M., Behere G. T., Chandra S. (2016). An environmentally benign and cost-effective technique for reducing bird damage to sprouting soybean seeds. Field Crops Res. 188, 74–81. doi: 10.1016/j.fcr.2016.01.008
Fisher D. O., Owens I. P. F. (2004). The comparative method in conservation biology. Trends Ecol. Evol. 19, 391–398. doi: 10.1016/j.tree.2004.05.004
Forester D. J., Machlist G. E. (1996). Modeling human factors that affect the loss of biodiversity. Conserv. Biol. 10, 1253–1263. doi: 10.1046/j.1523-1739.1996.10041253.x
Fritz S. A., Bininda-Emonds O. R. P., Purvis A. (2009). Geographical variation in predictors of mammalian extinction risk: big is bad, but only in the tropics. Ecol. Lett. 12, 538–549. doi: 10.1111/j.1461-0248.2009.01307.x
Gamer M., Lemon J., Ian F., Puspendra S. (2019). Irr: various coefficients of interrater reliability and agreement.
Gaston K. J. (2003). The structure and dynamics of geographic ranges (New York, NY: Oxford University Press).
Gaston K. J., Blackburn T. M. (1995). Birds, body size and the threat of extinction. Philos. Trans. R. Soc London. Ser. B Biol. Sci. 347, 205–212. doi: 10.1098/rstb.1995.0022
Geraci J., Béchet A., Cézilly F., Ficheux S., Baccetti N., Samraoui B., et al. (2012). Greater flamingo colonies around the Mediterranean form a single interbreeding population and share a common history. J. Avian Biol. 43, 341–354. doi: 10.1111/j.1600-048X.2012.05549.x
Gibbs D., Barnes E., Cox J. (2001). Pigeons and doves: a guide to the pigeons and doves of the world (New Haven, CT: Yale University Press).
Habel J. C., Gossner M. M., Schmitt T. (2021). Just beautiful?! what determines butterfly species for nature conservation. Biodivers. Conserv. 30, 2481–2493. doi: 10.1007/s10531-021-02204-9
Hanson T., Brooks T. M., Da Fonseca G. A. B., Hoffmann M., Lamoreux J. F., Machlis G., et al. (2009). Warfare in biodiversity hotspots. Conserv. Biol. 23, 578–587. doi: 10.1111/j.1523-1739.2009.01166.x
Harnik P. G., Simpson C., Payne J. L. (2012). Long-term differences in extinction risk among the seven forms of rarity. Proc. R. Soc B Biol. Sci. 279, 4969–4976. doi: 10.1098/rspb.2012.1902
Hilbers J. P., Schipper A. M., Hendriks A. J., Verones F., Pereira H. M., Huijbregts M. A. J. (2016). An allometric approach to quantify the extinction vulnerability of birds and mammals. Ecology 97, 615–626. doi: 10.1890/14-2019.1
Hoffman M., Koenig K., Bunting G., Costanza J., Williams K. J. (2016). Biodiversity hotspots. doi: 10.5281/zenodo.3261807
Howard C., Flather C. H., Stephens P. A. (2020). A global assessment of the drivers of threatened terrestrial species richness. Nat. Commun. 11, 993. doi: 10.1038/s41467-020-14771-6
Hume J. P. (2006). The history of the dodo Raphus cucullatus and the penguin of Mauritius. Hist. Biol. 18, 69–93. doi: 10.1080/08912960600639400
Ibáñez-Álamo J. D., Rubio E., Bitrus Zira K. (2017). The degree of urbanization of a species affects how intensively it is studied: a global perspective. Front. Ecol. Evol. 5, 1–8. doi: 10.3389/fevo.2017.00041
IUCN (2021) The IUCN red list of threatened species. version 2021-3). Available at: https://www.iucnredlist.org.
James R. E., Clout M. N. (1996). Nesting success of new Zealand pigeons (Hemiphaga novaeseelandiae) in response to a rat (Rattus rattus) poisoning programme at wenderholm regional park. N. Z. J. Ecol., 45–51.
Johnson K. P., Mateman A. C., Cate C.t., Lessells C. M., Clayton D. H. (2001). A molecular phylogeny of the dove genera Streptopelia and Columba. Auk 118, 874–887. doi: 10.1093/auk/118.4.874
Kampichler C., Wieland R., Calmé S., Weissenberger H., Arriaga-Weiss S. (2010). Classification in conservation biology: a comparison of five machine-learning methods. Ecol. Inform. 5, 441–450. doi: 10.1016/j.ecoinf.2010.06.003
Khan H. A., Arif I. A. (2013). COI barcodes and phylogeny of doves (Columbidae family). Mitochondrial DNA 24, 689–696. doi: 10.3109/19401736.2013.773319
Knapp K. R., Kruk M. C., Levinson D. H., Diamond H. J., Neumann C. J. (2010). The international best track archive for climate stewardship (IBTrACS). Bull Am Meteorol Soc 91, 363–376. doi: 10.1175/2009BAMS2755.1
Kyne P. M., Adams V. M. (2017). Extinct flagships: linking extinct and threatened species. Oryx 51, 471–476. doi: 10.1017/S0030605316000041
Lamelas-López L., Fontaine R., Borges P. A. V., Gonçalves D. (2020). Impact of introduced nest predators on insular endemic birds: the case of the Azores woodpigeon (Columba palumbus azorica). Biol. Invasions 22, 3593–3608. doi: 10.1007/s10530-020-02343-0
La Sorte F. A., Jetz W. (2010). Projected range contractions of montane biodiversity under global warming. Proc. R. Soc B Biol. Sci. 277, 3401–3410. doi: 10.1098/rspb.2010.0612
Le Corre M., Danckwerts D. K., Ringler D., Bastien M., Orlowski S., Morey Rubio C., et al. (2015). Seabird recovery and vegetation dynamics after Norway rat eradication at tromelin island, western Indian ocean. Biol. Conserv. 185, 85–94. doi: 10.1016/j.biocon.2014.12.015
Lees A. C., Devenish C., Areta J. I., de Araújo C. B., Keller C., Phalan B., et al. (2021). Assessing the extinction probability of the purple-winged ground dove, an enigmatic bamboo specialist. Front. Ecol. Evol. 9. doi: 10.3389/fevo.2021.624959
Lescuyer G., Nasi R. (2016). Financial and economic values of bushmeat in rural and urban livelihoods in Cameroon: inputs to the development of public policy. Int. For. Rev. 18, 93–107. doi: 10.1505/146554816819683726
Lindahl J., Magnusson U. (2020). Zoonotic pathogens in urban animals: enough research to protect the health of the urban population? Anim. Heal. Res. Rev. 21, 50–60. doi: 10.1017/S1466252319000100
Livingston G., Waring B., Pacheco L. F., Buchori D., Jiang Y., Gilbert L., et al. (2016). Perspectives on the global disparity in ecological science. Bioscience 66, 147–155. doi: 10.1093/biosci/biv175
Loehle C., Eschenbach W. (2012). Historical bird and terrestrial mammal extinction rates and causes. Divers. Distrib. 18, 84–91. doi: 10.1111/j.1472-4642.2011.00856.x
Lormée H., Barbraud C., Peach W., Carboneras C., Lebreton J., Moreno-Zarate L., et al. (2020). Assessing the sustainability of harvest of the European turtle-dove along the European western flyway. Bird Conserv. Int. 30, 506–521. doi: 10.1017/S0959270919000479
Luna Á., Romero-Vidal P., Hiraldo F., Tella J. L. (2018). Cities favour the recent establishment and current spread of the Eurasian collared dove Streptopelia decaocto (Frivaldszky 1838) in Dominican republic. BioInvasions Rec. 7, 95–99. doi: 10.3391/bir.2018.7.1.15
Mack R. N., Simberloff D., Lonsdale M. W., Evans H., Clout M., Bazzaz F. A. (2000). Biotic invasions: causes, epidemiology, global consequences, and control. Ecol. Appl. 10, 689–710. doi: 10.1890/1051-0761(2000)010[0689:BICEGC]2.0.CO;2
Mammides C. (2019). European Union’s conservation efforts are taxonomically biased. Biodivers. Conserv. 28, 1291–1296. doi: 10.1007/s10531-019-01725-8
Manne L. L., Brooks T. M., Pimm S. L. (1999). Relative risk of extinction of passerine birds on continents and islands. Nature 399, 258–261. doi: 10.1038/20436
Marrero P., Oliveira P., Nogales M. (2004). Diet of the endemic Madeira laurel pigeon Columba trocaz in agricultural and forest areas: implications for conservation. Bird Conserv. Int. 14, 165–172. doi: 10.1017/S0959270904000218
McKenzie A. J., Robertson P. A. (2015). Which species are we researching and why? a case study of the ecology of British breeding birds. PloS One 10, e0131004. doi: 10.1371/journal.pone.0131004
McKinney M. L. (1997). Extinction vulnerability and selectivity: combining ecological and paleontological views. Annu. Rev. Ecol. Syst. 28, 495–516. doi: 10.1146/annurev.ecolsys.28.1.495
Meehan H. J., Mcconkey K. R., Drake D. R. (2005). Early fate of Myristica hypargyraea seeds dispersed by Ducula pacifica in Tonga, Western Polynesia. Austral Ecol. 30, 374–382. doi: 10.1111/j.1442-9993.2005.01479.x
Melles S. J., Scarpone C., Julien A., Robertson J., Levieva J. B., Carrier C., et al. (2019). Diversity of practitioners publishing in five leading international journals of applied ecology and conservation biology 1987–2015 relative to global biodiversity hotspots. Écoscience 26, 323–340. doi: 10.1080/11956860.2019.1645565
Miller-ter Kuile A., Orr D., Bui A., Dirzo R., Klope M., McCauley D., et al. (2020). Impacts of rodent eradication on seed predation and plant community biomass on a tropical atoll. Biotropica 53, 232–242. doi: 10.1111/btp.12864
Monterrubio-Rico T. C., Villaseñor-Gómez J. F., Álvarez-Jara M., Escalante-Pliego P. (2016). Ecología y situación actual de la familia columbidae en la reserva de la biosfera los tuxtlas, veracruz. Ornitol. Neotrop. 27, 17–26. doi: 10.58843/ornneo.v27i0.124
Morales M. B., Traba J. (2016). Prioritising research in steppe bird conservation: a literature survey. Ardeola 63, 137. doi: 10.13157/arla.63.1.2016.rp6
Morelli F., Benedetti Y., Callaghan C. T. (2020). Ecological specialization and population trends in European breeding birds. Glob. Ecol. Conserv. 22, e00996. doi: 10.1016/j.gecco.2020.e00996
Moreno-Zarate L., Arroyo B., Peach W. (2021). Effectiveness of hunting regulations for the conservation of a globally-threatened species: the case of the European turtle-dove in Spain. Biol. Conserv. 256, 109067. doi: 10.1016/j.biocon.2021.109067
Murray H., Green E., Williams D., Burfield I., Brooke M de L. (2015). Is research effort associated with the conservation status of European bird species? Endanger. Species Res. 27, 193–206. doi: 10.3354/esr00656
Murray K. A., Rosauer D., McCallum H., Skerratt L. F. (2011). Integrating species traits with extrinsic threats: closing the gap between predicting and preventing species declines. Proc. R. Soc B Biol. Sci. 278, 1515–1523. doi: 10.1098/rspb.2010.1872
Murton R. K., Jones B. E. (1973). The ecology and economics of damage to brassicae by wood-pigeons Columba palumbus. Ann. Appl. Biol. 75, 107–122. doi: 10.1111/j.1744-7348.1973.tb01342.x
Norris K., Harper N. (2004). Extinction processes in hot spots of avian biodiversity and the targeting of pre–emptive conservation action. Proc. R. Soc London. Ser. B Biol. Sci. 271, 123–130. doi: 10.1098/rspb.2003.2576
Olah G., Butchart S. H. M., Symes A., Guzmán I. M., Cunningham R., Brightsmith D. J., et al. (2016). Ecological and socio-economic factors affecting extinction risk in parrots. Biodiversity Conserv. 25, 205–223. doi: 10.1007/s10531-015-1036-z
Owens I. P. F., Bennett P. M. (2000). Ecological basis of extinction risk in birds: habitat loss versus human persecution and introduced predators. Proc. Natl. Acad. Sci. 97, 12144–12148. doi: 10.1073/pnas.200223397
Perez-Sancho M., García-Seco T., Porrero C., García N., Gomez-Barrero S., Cámara J. M., et al. (2020). A ten-year-surveillance program of zoonotic pathogens in feral pigeons in the city of Madrid, (2005–2014): the importance of a systematic pest control. Res. Vet. Sci. 128, 293–298. doi: 10.1016/j.rvsc.2019.12.006
Peterson A. T., Soberón J., Pearson R. G., Anderson R. P., Martínez-Meyer E., Nakamura M., et al. (2011). Ecological niches and geographic distributions (MPB-49) (Princeton, NJ: Princeton University Press).
Phelps J., Biggs D., Webb E. L. (2016). Tools and terms for understanding illegal wildlife trade. Front. Ecol. Environ. 14, 479–489. doi: 10.1002/fee.1325
Pimm S. L., Jenkins C. N., Abell R., Brooks T. M., Gittleman J. L., Joppa L. N., et al. (2014). The biodiversity of species and their rates of extinction, distribution, and protection. Science 344, 1246752. doi: 10.1126/science.1246752
Prasad A. M., Iverson L. R., Liaw A. (2006). Newer classification and regression tree techniques: bagging and random forests for ecological prediction. Ecosystems 9, 181–199. doi: 10.1007/s10021-005-0054-1
Purvis A., Gittleman J. L., Cowlishaw G., Mace G. M. (2000a). Predicting extinction risk in declining species. Proc. R. Soc London. Ser. B Biol. Sci. 267, 1947–1952. doi: 10.1098/rspb.2000.1234
Purvis A., Jones K. E., Mace G. M. (2000b). Extinction. BioEssays 22, 1123–1133. doi: 10.1002/1521-1878(200012)22:12<1123::AID-BIES10>3.0.CO;2-C
Reboredo Segovia A. L., Romano D., Armsworth P. R. (2020). Who studies where? boosting tropical conservation research where it is most needed. Front. Ecol. Environ. 18, 159–166. doi: 10.1002/fee.2146
Reif J., Marhoul P., Koptík J. (2013). Bird communities in habitats along a successional gradient: divergent patterns of species richness, specialization and threat. Basic Appl. Ecol. 14, 423–431. doi: 10.1016/j.baae.2013.05.007
Ripple W. J., Chapron G., López-Bao J. V., Durant S. M., Macdonald D. W., Lindsey P. A., et al. (2016). Saving the world’s terrestrial megafauna. Bioscience 66, 807–812. doi: 10.1093/biosci/biw092
Ripple W. J., Wolf C., Newsome T. M., Hoffmann M., Wirsing A. J., McCauley D. J. (2017). Extinction risk is most acute for the world’s largest and smallest vertebrates. Proc. Natl. Acad. Sci. 114, 10678–10683. doi: 10.1073/pnas.1702078114
Rivas-Salvador J., Hořák D., Reif J. (2019). Spatial patterns in habitat specialization of European bird communities. Ecol. Indic. 105, 57–69. doi: 10.1016/j.ecolind.2019.05.063
Rivera-Milán F. F., Bertuol P., Simal F., Rusk B. L. (2015). Distance sampling survey and abundance estimation of the critically endangered Grenada dove (Leptotila wellsi). Condor 117, 87–93. doi: 10.1650/CONDOR-14-131.1
Roberts B. E. I., Harris W. E., Hilton G. M., Marsden S. J. (2016). Taxonomic and geographic bias in conservation biology research: a systematic review of wildfowl demography studies. PloS One 11, e0153908. doi: 10.1371/journal.pone.0153908
Robinson J. G., Bennett E. L. (2002). Will alleviating poverty solve the bushmeat crisis? Oryx 36, 332. doi: 10.1017/S0030605302000662
Rodrigues A., Pilgrim J., Lamoreux J., Hoffmann M., Brooks T. (2006). The value of the IUCN red list for conservation. Trends Ecol. Evol. 21, 71–76. doi: 10.1016/j.tree.2005.10.010
Rodríguez-Estrella R., Arnaud G., Alvarez Cardenas S., Rodriguez A. (1991). Predation by feral cats on birds at isla Socorro, Mexico. West. Birds 22, 141–143.
Romero-Muñoz A., Benítez-López A., Zurell D., Baumann M., Camino M., Decarre J., et al. (2020). Increasing synergistic effects of habitat destruction and hunting on mammals over three decades in the gran chaco. Ecography 43, 954–966. doi: 10.1111/ecog.05053
Romero-Muñoz A., Torres R., Noss A. J., Giordano A. J., Quiroga V., Thompson J. J., et al. (2019). Habitat loss and overhunting synergistically drive the extirpation of jaguars from the gran chaco. Divers. Distrib 25, 176–190. doi: 10.1111/ddi.12843
Sangster G., Sweet A. D., Johnson K. P. (2018). Paraclaravis, a new genus for the purple-winged and maroon-chested ground-doves (Aves: columbidae). Zootaxa 4461, 134–140. doi: 10.11646/zootaxa.4461.1.10
Saupe E. E., Qiao H., Hendricks J. R., Portell R. W., Hunter S. J., Soberón J., et al. (2015). Niche breadth and geographic range size as determinants of species survival on geological time scales. Glob. Ecol. Biogeogr. 24, 1159–1169. doi: 10.1111/geb.12333
Sax D. F., Gaines S. D. (2008). Species invasions and extinction: the future of native biodiversity on islands. Proc. Natl. Acad. Sci. 105, 11490–11497. doi: 10.1073/pnas.0802290105
Sayre R., Noble S., Hamann S., Smith R., Wright D., Breyer S., et al. (2019). A new 30 meter resolution global shoreline vector and associated global islands database for the development of standardized ecological coastal units. J. Oper. Oceanogr. 12, S47–S56. doi: 10.1080/1755876X.2018.1529714
Schumm Y. R., Masello J. F., Cohou V., Mourguiart P., Metzger B., Rösner S., et al. (2022). Should I stay or should I fly? migration phenology, individual-based migration decision and seasonal changes in foraging behaviour of common woodpigeons. Sci. Nat. 109, 1–16. doi: 10.1007/s00114-022-01812-x
Schumm Y. R., Metzger B., Neuling E., Austad M., Galea N., Barbara N., et al. (2021). Year-round spatial distribution and migration phenology of a rapidly declining trans-Saharan migrant–evidence of winter movements and breeding site fidelity in European turtle doves. Behav. Ecol. Sociobiol 75, 1–16. doi: 10.1007/s00265-021-03082-5
Seddon P. J., Soorae P. S., Launay F. (2005). Taxonomic bias in reintroduction projects. Anim. Conserv. 8, 51–58. doi: 10.1017/S1367943004001799
Sekercioglu C. H., Schneider S. H., Fay J. P., Loarie S. R. (2008). Climate change, elevational range shifts, and bird extinctions. Conserv. Biol. 22, 140–150. doi: 10.1111/j.1523-1739.2007.00852.x
Shoo L. P., Williams S. E., Hero J.-M. (2005). Climate warming and the rainforest birds of the Australian wet tropics: using abundance data as a sensitive predictor of change in total population size. Biol. Conserv. 125, 335–343. doi: 10.1016/j.biocon.2005.04.003
Siddig A. A. H. (2014). Biodiversity of Sudan: between the harsh conditions, political instability and civil wars. Biodiversity J. 5, 545–555.
Smith F. A., Elliott Smith R. E., Lyons S. K., Payne J. L., Villaseñor A. (2019). The accelerating influence of humans on mammalian macroecological patterns over the late. Quaternary. Quat. Sci. Rev. 211, 1–16. doi: 10.1016/j.quascirev.2019.02.031
Soares A. E. R., Novak B. J., Haile J., Heupink T. H., Fjeldså J., Gilbert M. T. P., et al. (2016). Complete mitochondrial genomes of living and extinct pigeons revise the timing of the columbiform radiation. BMC Evol. Biol. 16, 230. doi: 10.1186/s12862-016-0800-3
Stanton J. C. (2014). Present-day risk assessment would have predicted the extinction of the passenger pigeon (Ectopistes migratorius). Biol. Conserv. 180, 11–20. doi: 10.1016/j.biocon.2014.09.023
Steadman D. W., Takano O. M. (2020). A new genus and species of pigeon (Aves, columbidae) from the kingdom of Tonga, with an evaluation of hindlimb osteology of columbids from Oceania. Zootaxa 4810, 401–420. doi: 10.11646/zootaxa.4810.3.1
Stimmelmayr R., Stefani L. M., Thrall M. A., Landers K., Revan F., Miller A., et al. (2012). Trichomonosis in free-ranging eurasian collared doves (Streptopelia decaocto) and african collared dove hybrids (Streptopelia risorii) in the Caribbean and description of ITS-1 region genotypes. Avian Dis. 56, 441–445. doi: 10.1637/9905-082311-Case.1
Stirnemann R. L., Stirnemann I. A., Abbot D., Biggs D., Heinsohn R. (2018). Interactive impacts of by-catch take and elite consumption of illegal wildlife. Biodivers. Conserv. 27, 931–946. doi: 10.1007/s10531-017-1473-y
Swinnerton K. J., Greenwood A. G., Chapman R. E., Jones C. G. (2005). The incidence of the parasitic disease trichomoniasis and its treatment in reintroduced and wild pink pigeons Columba mayeri. Ibis 147, 772–782. doi: 10.1111/j.1474-919X.2005.00466.x
Tatayah V. (2019). How did the pink pigeon bounce back from just nine birds? (Birdlife Int). Available at: https://www.birdlife.org/worldwide/news/how-did-pink-pigeon-bounce-back-just-nine-birds.
Tidemann C., Yorkston H., Russack A. (1994). The diet of cats, Felis catus, on Christmas island, Indian ocean. Wildl. Res. 21, 279. doi: 10.1071/WR9940279
Tydecks L., Jeschke J. M., Wolf M., Singer G., Tockner K. (2018). Spatial and topical imbalances in biodiversity research. PloS One 13, e0199327. doi: 10.1371/journal.pone.0199327
Vallès H., Labaude S., Bezault E., Browne D., Deacon A., Guppy R., et al. (2021). Low contribution of Caribbean-based researchers to academic publications on biodiversity conservation in the insular Caribbean. Perspect. Ecol. Conserv. 19, 443–453. doi: 10.1016/j.pecon.2021.07.006
van Niekerk J., van Ginkel C. (2009). The feeding behaviour of pigeons and doves on sown grain crops on the south African highveld. Ostrich 75, 39–43. doi: 10.2989/00306520409485410
Venter O., Sanderson E. W., Magrach A., Allan J. R., Beher J., Jones K. R., et al. (2016). Global terrestrial human footprint maps for 1993 and 2009. Sci. Data 3, 160067. doi: 10.1038/sdata.2016.67
Venter O., Sanderson E. W., Magrach A., Allan J. R., Beher J., Jones K. R., et al. (2018). Last of the wild project, version 3 (LWP-3): 2009 human footprint 2018 release. doi: 10.7927/H46T0JQ4
Verga E. G., Huais P. Y., Herrero M. L. (2021). Population responses of pest birds across a forest cover gradient in the chaco ecosystem. For Ecol. Manage 491, 119174. doi: 10.1016/j.foreco.2021.119174
Waldron A., Mooers A. O., Miller D. C., Nibbelink N., Redding D., Kuhn T. S., et al. (2013). Targeting global conservation funding to limit immediate biodiversity declines. Proc. Natl. Acad. Sci. 110, 12144–12148. doi: 10.1073/pnas.1221370110
Walker J. S. (2007). Geographical patterns of threat among pigeons and doves (Columbidae). Oryx 41, 289–299. doi: 10.1017/S0030605307001016
Walsh J. C., Venter O., Watson J. E. M., Fuller R. A., Blackburn T. M., Possingham H. P. (2012). Exotic species richness and native species endemism increase the impact of exotic species on islands. Glob. Ecol. Biogeogr. 21, 841–850. doi: 10.1111/j.1466-8238.2011.00724.x
White R. L., Bennett P. M. (2015). Elevational distribution and extinction risk in birds. PloS One 10, e0121849. doi: 10.1371/journal.pone.0121849
Whytock R. C., Buij R., Virani M. Z., Morgan B. J. (2016). Do large birds experience previously undetected levels of hunting pressure in the forests of central and West Africa? Oryx 50, 76–83. doi: 10.1017/S0030605314000064
Williams D. R., Balmford A., Wilcove D. S. (2020). The past and future role of conservation science in saving biodiversity. Conserv. Lett. 13, 1–7. doi: 10.1111/conl.12720
Williams S. E., Bolitho E. E., Fox S. (2003). Climate change in Australian tropical rainforests: an impending environmental catastrophe. Proc. R. Soc London. Ser. B Biol. Sci. 270, 1887–1892. doi: 10.1098/rspb.2003.2464
Wilson K. A., Auerbach N. A., Sam K., Magini A. G., Moss A. S. L., Langhans S. D., et al. (2016). Conservation research is not happening where it is most needed. PloS Biol. 14, e1002413. doi: 10.1371/journal.pbio.1002413
Wolff P. J., DeGregorio B. A., Rodriguez-Cruz V., Mulero-Oliveras E., Sperry J. H. (2018). Bird community assemblage and distribution in a tropical, urban ecosystem of Puerto Rico. Trop. Conserv. Sci. 11, 1–10. doi: 10.1177/1940082918754777
Wood J. R., Alcover J. A., Blackburn T. M., Bover P., Duncan R. P., Hume J. P., et al. (2017). Island extinctions: processes, patterns, and potential for ecosystem restoration. Environ. Conserv. 44, 348–358. doi: 10.1017/S037689291700039X
Wotton D. M., Kelly D. (2012). Do larger frugivores move seeds further? body size, seed dispersal distance, and a case study of a large, sedentary pigeon. J. Biogeogr. 39, 1973–1983. doi: 10.1111/jbi.12000
Yarwood M. R., Weston M. A., Symonds M. R. E. (2019). Biological determinants of research effort on Australian birds: a comparative analysis. Emu - Austral Ornithol. 119, 38–44. doi: 10.1080/01584197.2018.1501274
Keywords: biogeography, doves, endangered species, extinction risk, pigeons, scientific attention, threatened species
Citation: Cambrone C, Jean-Pierre A, Bezault E and Cézilly F (2023) Identifying global research and conservation priorities for Columbidae: a quantitative approach using random forest models. Front. Ecol. Evol. 11:1141072. doi: 10.3389/fevo.2023.1141072
Received: 09 January 2023; Accepted: 28 June 2023;
Published: 24 July 2023.
Edited by:
Ming-Chih Chiu, Chinese Academy of Sciences (CAS), ChinaReviewed by:
Hervé Lormee, French Office for Biodiversity (OFB), FranceJhih-Rong Liao, Tokyo Metropolitan University, Japan
Copyright © 2023 Cambrone, Jean-Pierre, Bezault and Cézilly. This is an open-access article distributed under the terms of the Creative Commons Attribution License (CC BY). The use, distribution or reproduction in other forums is permitted, provided the original author(s) and the copyright owner(s) are credited and that the original publication in this journal is cited, in accordance with accepted academic practice. No use, distribution or reproduction is permitted which does not comply with these terms.
*Correspondence: Christopher Cambrone, christopher.cambrone@caribaea.org
†ORCID: Christopher Cambrone, orcid.org/0000-0001-9928-4178
Aurélie Jean-Pierre, orcid.org/0000-0001-8414-7859
Etienne Bezault, orcid.org/0000-0001-7172-6480
Frank Cézilly, orcid.org/0000-0003-2673-6713