- 1Ecology and Genetics Research Unit, University of Oulu, Oulu, Finland
- 2Natural Resources Institute Finland (Luke), Helsinki, Finland
- 3Finnish Food Safety Authority (Ruokavirasto), Veterinary Bacteriology and Pathology Research Unit, Oulu, Finland
- 4UArctic, University of the Arctic, Rovaniemi, Finland
- 5Department of Biological Sciences, University of Alaska Anchorage, Anchorage, AK, United States
Over the last hundred years, many large carnivore species have suffered range contractions, population losses, and habitat alterations that may be influencing their dietary preferences. To identify shifting isotopic niches and reconstruct past and present diets from species of high conservation concern, stable isotope analysis (SIA) of δ13C and δ15N values of tissue from museum collections and ongoing non-invasive monitoring programs offers an effective approach. Here, we assessed the long-term alterations in the trophic structure of a generalist large carnivore over a time of population loss and recovery. Wolverine stable carbon (δ13C) and nitrogen (δ15N) isotopes were measured in hair samples collected from 44 individuals in Finland for the period 1905–2020, and potential dietary were quantified using isotopic niche metrics shifts in two study areas. We provide evidence of a long-term diet shift in Finnish wolverines, with a temporal depletion of hair’s δ13C (β = −0.016‰, R² = 0.46, p < 0.001). Correspondingly, there was a shift in the isotopic niches of the Historical population (before the year 2000) and Recent population (after 2000), with the niche overlap between the Historical and Recent periods being only 33% throughout Finland, and even lower in the two study areas (22% to 24%). The breadth of the isotopic niche, however, did not significantly differ between the two periods, suggesting that despite a change in diet composition, wolverines did not specialize more. The trophic level of wolverines in Finland was stable throughout the study period, with no significant change in δ15N with time (β = 0.0055‰, R² = 0.043, p = 0.17). In summary: A) the strong trophic resilience of this top predator was shown by the stability of its niche breadth and trophic level feeding regime during periods of population decline and recovery as well as during a period of major anthropogenic alterations in their environment; B) the use of SIA methods on historical and recent hairs provided a unique tool to unravel long-term changes in wolverine feeding strategies.
1 Introduction
Terrestrial food webs are often considered to be structured by large carnivores (Wilson and Wolkovich, 2011; Allen et al., 2017; Buskirk, 2023). The top-down control of predators on prey cascades to various taxa through multiple food-web pathways, impacting ecosystem processes at all levels from nutrient cycling to landscape features (Ripple et al., 2014; Winnie and Creel, 2017; Peziol et al., 2023). Despite their crucial ecological function, large carnivore populations have experienced a global decline and range contractions throughout the last century (Wolf and Ripple, 2017; Wolf and Ripple, 2018). Meanwhile, habitat alteration and fragmentation together with climate change have led to modifications in prey species richness and distribution (Christian et al., 2009; Schwensow et al., 2022). The persistence of a predator in an altered ecosystem is then partly predicted by its resilience to changing habitats and prey populations (Hempson et al., 2017). Given the time scale of these alterations and the long generation time of many large carnivore species, the predator’s trophic adaptations to changes in the food web may only be observable in the long term, i.e. several decades (Beckmann and Berger, 2003; Moreno et al., 2013).
Stable Isotope Analysis (SIA) is a valuable tool for studying food web ecology, including reconstructions of large carnivores’ diets (Rogers et al., 2015; Newton, 2016; Stanek et al., 2017; Stanek et al., 2019). As an organism consumes and assimilates resources, the stable isotope ratios of the food sources will be reflected in the consumer’s tissues (DeNiro and Epstein, 1978; DeNiro and Epstein, 1981; Ben-David and Flaherty, 2012). A change in a consumer’s carbon ratio value (δ13C) is a proxy for processes such as changes in carbon source, i.e. a diet shift toward an alternative prey (DeNiro and Epstein, 1978; Kaczensky et al., 2017; Reynolds et al., 2019). The nitrogen ratio value (δ15N) on the other hand is typically enriched relative to its food source, thus a high δ15N value indicates a higher trophic level (DeNiro and Epstein, 1981; Layman et al., 2007; Jennings and Van Der Molen, 2015).
Sequentially grown tissues (e.g. hair, bone, feather) encapsulate long-term information about a consumer’s diet by retaining the δ13C and δ15N isotopic values from the time of growth and remaining metabolically inert afterward (Rogers et al., 2020; Hamstrom et al., 2023). Moreover, a tissue such as hair does not degrade as fast as soft organs after death and can be preserved for decades in museum collections, on naturalized specimens or pelts. Hair also has the advantage of being easily sampled non-invasively on living individuals, e.g. using hair traps (Barja et al., 2016). Yet, only a few diet studies have seized the opportunity of using carbon and nitrogen SIA on museum and recent hair samples in terrestrial ecosystems (Walker et al., 1999; Dalerum and Angerbjörn, 2005).
Isotopic values measured in consumers’ hair samples can be used as a proxy to assess and compare diet breadth at different spatial and temporal scales (Nilsen et al., 2012). The diet breadth of a consumer determines its position on the generalist–specialist continuum, with generalists having a more diverse diet and thus a larger breadth than specialists (Jedrzejewska and Jedrzejewski, 1998). The trophic niche of generalist consumers, i.e. their position in the food web of a community, can be especially dynamic (Balza et al., 2020). Generalists may switch between alternative prey items, e.g. during a seasonal peak in food availability (Ben-David et al., 1997; Djagoun et al., 2016), or shift their diet in the long term to either cope with the loss of resources or take advantage of newly available supplies (Buelow et al., 2018). While long-term dietary studies inferred by SIA have increasingly been used in aquatic ecosystems (Thompson et al., 1995; Farmer and Leonard, 2011; Moreno et al., 2013; Mariano-Jelicich et al., 2017; Reynolds et al., 2019; Carney et al., 2023), it has been sparsely applied on terrestrial food webs (Hilderbrand et al., 1996; English et al., 2018), and essentially at a scale of a few years to three decades in mammalian studies (Galicia et al., 2016; Carbonell Ellgutter et al., 2020).
The wolverine (Gulo gulo) is a large carnivore inhabiting the tundra and boreal forest of the circumpolar north (MacDonald et al., 2017). Like other boreal large carnivores, wolverines disappeared from the southernmost parts of their range due to persecution, overhunting, habitat loss, and human development (Landa et al., 2000; Kyle and Strobeck, 2002). Wolverines are facultative scavengers and generalist predators feeding on diverse prey ranging from small rodents to large ungulates (Myhre and Myrberget, 1975; Magoun, 1987; Koskela et al., 2013a). They adapt to food scarcity by foraging opportunistically, i.e. switching their diet toward occasionally available food sources (Samelius et al., 2002; Shardlow, 2013). In Alaska, for example, Dalerum et al., (2009b) showed that wolverines switched their diet between moose (Alces alces) and caribou (Rangifer tarandus) depending on the ungulate’s population abundance and availability. The diet switch happened on an annual scale and was also observed over six years using SIA of multiple wolverine tissues (Dalerum et al., 2009a).
Despite the global range contraction, local wolverine populations in northern Europe are currently stable or increasing (Abramov, 2016). In Finland for example, wolverines underwent a severe population and genetic bottleneck during the 20th century (Lansink et al., 2020; Sugiyama et al., 2022). After a critical low in 1973, the population entered a slow recovery phase followed by faster growth in the last decade (Mykrä and Pohja-Mykrä, 2015). In 2022, Finland counted 400 individuals split into a Scandinavian and Karelian genetic population (Kojola et al., 2022; Lansink et al., 2022). The species is classified as Endangered, protected nationwide, and therefore qualifies for conservation actions (Nature Conservation Act, 1996; Hyvärinen et al., 2019). However, In Northern Finland where semi-domesticated reindeer (R. t. tarandus) are herded extensively, the wolverines’ predation on livestock results in a typical human–wildlife conflict that negatively affects its conservation (Rasmus et al., 2020). Although previous studies have suggested diet differences between wolverine populations within Finland (Koskela et al., 2013a; Koskela et al., 2013b), little is known about the long-term dynamics of their trophic niches nor about the relevance of potential niche variations for conservation (The Finnish Ministry of Agriculture and Forestry, 2014; Fisher et al., 2022).
In this study, we aimed to detect possible long-term alterations in the diet of a terrestrial generalist predator, the wolverine, by using stable isotope analysis of δ13C and δ15N. We applied SIA on hair samples from modern and museum collections, and assessed changes in carbon source and trophic level over the last 100 years. We specifically quantified isotopic niche position, breadth, and relative overlap of historical and recent wolverine populations, while considering their population structure. Finally, we discussed the overall suitability of SIA for gaining information on the trophic ecology of past and present populations of wolverines, and its implications for large carnivores’ conservation.
2 Materials and methods
2.1 Study area
The study was conducted in Finland, covering the eastern and northern parts of the Finnish wolverine range (Figure 1). The whole study area was defined as Finland and further divided into Northern and Eastern study areas, based on their distinct ecological communities and genetic population structure. The Northern study area is within the reindeer management area (RMA), where about 190,000 semi-domesticated reindeer are extensively herded (The Finnish Reindeer Herding Association, 2021). It consists of various alpine, sub-arctic, and boreal ecosystems, with high plateaus and hills (maximum elevation 1,365 meters above sea level). The snow cover period extends from October/November until May/June (Koskela et al., 2013a). The Eastern study area, outside the RMA, consists mainly of boreal pine forests dominated by Norway spruce (Picea abies) and Scots pine (Pinus sylvestris), though mixed forests can be found as a result of felling and clear-cuttings. There, the rugged terrain reaches an elevation of 300 masl, and snow cover usually lasts from November/December until April, with a peak in March. In contrast to the Northern region, other large carnivores are present in high densities in the Eastern study area (the grey wolf [Canis lupus; Heikkinen et al., 2021a], the Eurasian lynx [Lynx lynx; Holmala et al., 2021], and the brown bear [Ursus arctos; Heikkinen et al., 2021b]).
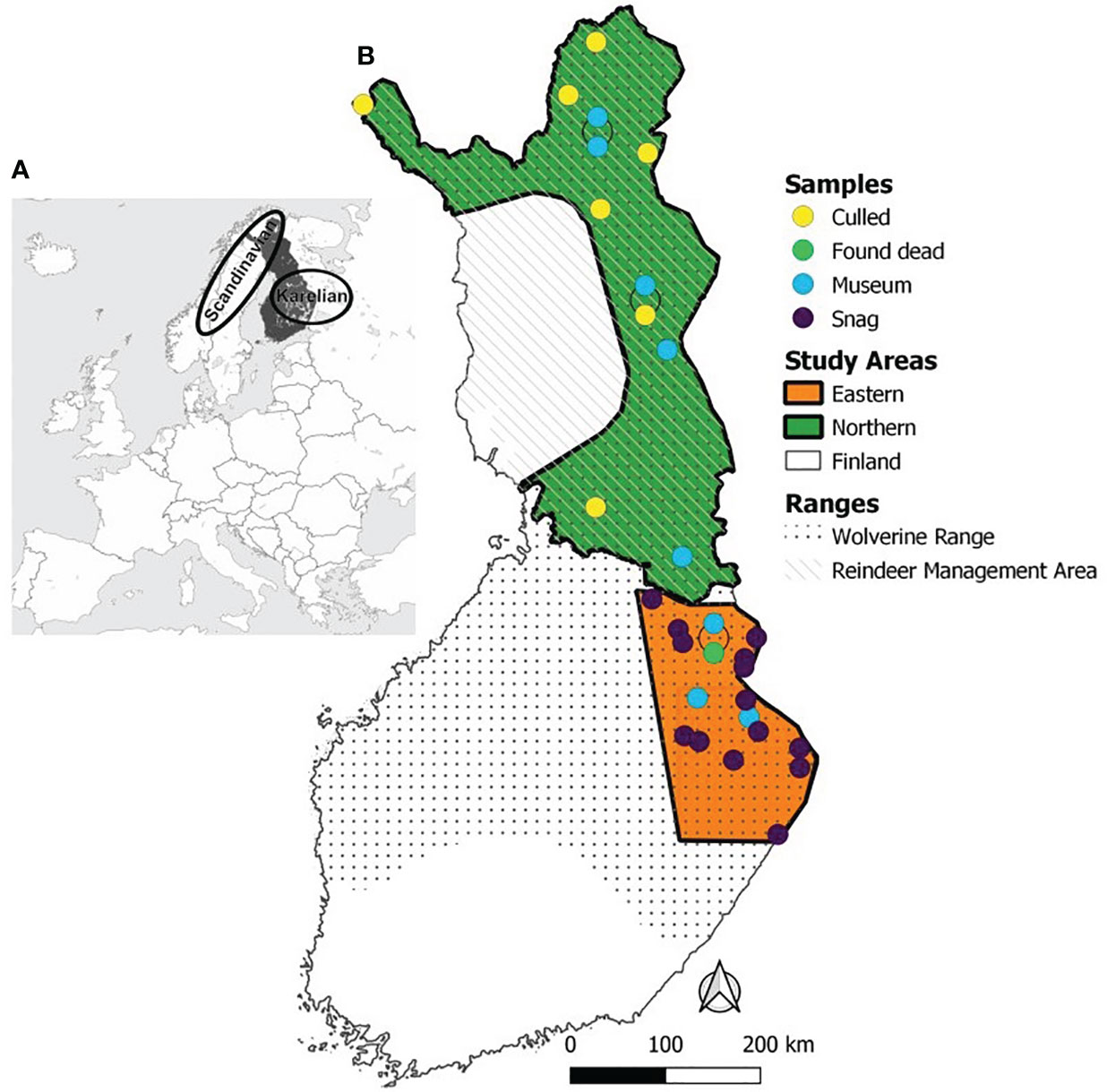
Figure 1 Wolverine sample locations in Finland and the two study areas. (A) In the insert, Finland is coloured dark grey and the two wolverine populations of Fennoscandia (Scandinavian and Karelian; Lansink et al., 2020) are roughly marked with ellipses. (B) Sampling sites were grouped into Northern (green polygon) and Eastern (orange polygon) study areas within the Finnish wolverine range (dots; Abramov, 2016; Kaczensky et al., 2017; The Finnish Natural Resources Institute, 2022). The Northern study area overlaps with the Reindeer Management Area (dashed). Samples (circles) were either collected from museum specimens (blue), hair snags (purple), or from found dead (light green) and legally culled individuals (yellow).
2.2 Sampling
Wolverine hair samples were obtained from 44 individuals from the period 1905–2020. All samples (N = 52) were collected using non-invasive methods, from an ongoing DNA-monitoring project (N = 27), museum specimens (N = 16), and individuals found dead or legally culled independently from our research (N = 9). Baited hair snags were used to collect hair samples over the period 2016–2019 (Lansink et al., 2020). The hairs are plucked by metal brushes, collected in paper envelopes and analyzed for individual identification (see for details on the microsatellite genotyping: Lansink et al., 2020). While most individuals were sampled once, four individuals were sampled on several occasions due to the opportunistic nature of the sampling method. From three individuals sampled three times within the same year, samples were pooled per individual and labeled with the respective collection year. One individual was sampled on three occasions, each in a different year. Given the strong inter-annual variation in wolverines’ diet (Myhre and Myrberget, 1975; Dalerum et al., 2009b), we treated each sample from this individual as independent. Additional hair samples were collected from one individual found dead in 2020 and from eight legally culled individuals in 2019–2020 (Appendix 1). Museum hair samples from similar regions as the other individuals were taken from skins and stuffed specimens from Finnish natural history museums.
2.3 Sample preparation
Hair samples were washed in a solution of 2:1 chloroform/methanol overnight on an orbital shaker at 180 rpm to remove lipids. Samples were rinsed once with fresh 2:1 chloroform/methanol, twice with 96% ethanol, twice with distilled water, and dried in an oven at 50°C for 24 hours. For each hair sample, 15 of the longest guard hairs were cut with a surgical scalpel into 2 cm sections from the follicle to the tip (Careddu et al., 2021). Each hair sample’s section was weighed (0.8–1.2 mg) into a 5 × 9 mm tin capsule (Elemental Microanalysis [UK]).
2.4 Stable isotope analysis
Carbon (13C:12C) and nitrogen (15N:14N) stable isotope ratios were measured in each sample with a mass spectrometer at the Environment and Natural Institute Stable Isotope Laboratory at the University of Alaska Anchorage (Rogers et al., 2020). Ratios were measured as a deviation from international reference standards (Vienna Pee Dee Belemnite for carbon and air for nitrogen), expressed as δ in per mil (‰). The internal laboratory standards were BWBII keratin, peach leaves, moose, and three-spined stickleback (Gasterosteus aculeatus). Measurement errors were found to be typically smaller than ± 0.02‰ for both δ13C and δ15N. In addition, the carbon to nitrogen concentration ratio (C:N) was measured to assess possible contamination or alteration of the samples (DeNiro, 1985).
2.5 Data preparation
2.5.1 Suess effect
Correction of isotopic values is required on large temporal ranges, as changes in the natural occurrence of stable isotopes can be gradually integrated into the tissues of primary producers and may propagate along the food web to higher-level consumers (Long et al., 2005; Kumar, 2011; Newton, 2016). Increasing atmospheric CO2 emission and its associated depletion in 13C, called the ‘‘Suess effect’’, is the main known long-term change in the isotopic baseline (Keeling et al., 2017). As CO2 emissions accelerated after 1960, δ13C measured in hair samples was corrected using equation (1) for samples between 1960 and 2019, and equation (2) for samples prior to 1960 (Francey et al., 1999; Chamberlain et al., 2005; Ben-David and Flaherty, 2012). The most recent sampling year (i.e. 2020) was taken as the reference year and thus not corrected.
Temperature and rainfall could also influence the carbon isotopic baseline of primary producers, but the scale of these changes is far smaller than the Suess effect (Marshall et al., 2007) and becomes negligible when moving up in the food web (English et al., 2018). Values were not corrected as there is no strong evidence of global atmospheric NO3− depletion being integrated into the ecosystem (Felix and Elliott, 2013). Moreover, local processes of soil δ15N depletion due to agricultural fertilization (Bol et al., 2005) are likely irrelevant in the soils of the boreal forest (Liu et al., 2022).
2.5.2 Periods
Samples from before the year 2000 were grouped as Historical (N = 13), and samples taken after 2000 as Recent (N = 33). The Historical-Recent division reflected the wolverine population history in Finland (i.e. the bottleneck and a period of low population densities vs. population recovery and growth; Lansink et al., 2020; Kojola et al., 2022) as well as the carnivores guild recolonization history in Finland (i.e. absent or in low numbers during the Historical, and rapidly increasing or stabilizing during the Recent period; Pohja-Mykrä et al., 2005; Heikkinen et al., 2021b; Holmala et al., 2021). Moreover, the groups ensured that the sample sizes were large enough for the later analysis (N ≥ 3; Jackson et al., 2011). The sample size of Recent individuals was larger than the Historical one in the Eastern region (N = 26 and N = 6, respectively). Any bias attributed to this difference in sample size was ruled out by running all analyses with equal Historical and Recent sample sizes (N = 6). Six Recent individuals from the Eastern study region were randomly selected, with replacement, and the process was repeated 10 times. Results from the random samplings were similar to the original results (Appendix 2).
2.6 Data analysis
Long-term shifts in isotopic niches between the Recent and Historical wolverine populations were evaluated for the study areas (Northern and Eastern) and pooled together as Finland. The isotopic niches were reconstructed for each group by fitting ellipses encompassing 95% of the data using the R package SIBER (Stable Isotope Bayesian Ellipses in R package; version 2.1.6; Jackson et al., 2011). The similarity in isotopic niches between the two groups was quantified by their overlap, expressed as a percentage of the overlapping area over the total area covered by the two ellipses (Krumsick and Fisher, 2019). Niche breadths were quantified by their Bayesian standard ellipse area (SEAB), calculated by fitting Bayesian multivariate normal distributions to the data (Catry et al., 2016). We used JAGS (Just Another Gibbs Sampler) parameters as: iterations = 130.000, burn-in = 5.000, thin = 50, chains = 4, and applied a correction for small sample size (Jackson et al., 2011). To compare the niche breadth extent between the two periods, the posterior probability of Historical’s SEAB being larger than Recent’s SEAB was calculated (PPH>R). Historical’s SEAB was considered significantly larger where PPH>R ≥ 0.95.
3 Results
The δ13C stable isotopes values of wolverine hairs in Finland ranged from −24.7 to −21.3 ‰ (mean= −23.3‰, SD = 0.8, Suess corrected) and δ15N values fell between 5.1 and 8.8 ‰ (mean = 6.5 ‰, SD = 0.9). The carbon to nitrogen ratio was consistent throughout the study period (max = 3.1, min = 2.8, mean = 2.9, SD = 0.1), falling in the expected range for unaltered tissue samples (DeNiro, 1985). Detailed isotopic values are given for each sample in Appendix 1.
During the last century (1905–2020), the δ13C values of wolverine hairs became depleted in Finland (Figure 2A; estimated slope β = −0.016‰, R² = 0.46, p-value < 0.001), and within both study areas (Figure 2B; Northern: β = −0.015‰, R² = 0.76, p < 0.001; Eastern: β = −0.012‰, R² = 0.17, p = 0.031). For the δ15N, although the Eastern study area showed a slight temporal enrichment with a minor correlation (β = −0.013‰, R² = 0.12, p = 0.046), no significant correlation between years and the δ15N values was found in the Northern study area (Figure 2D, R² = 0.085, p = 0.31) nor overall in Finland (Figure 2C, R² = 0.043, p = 0.17).
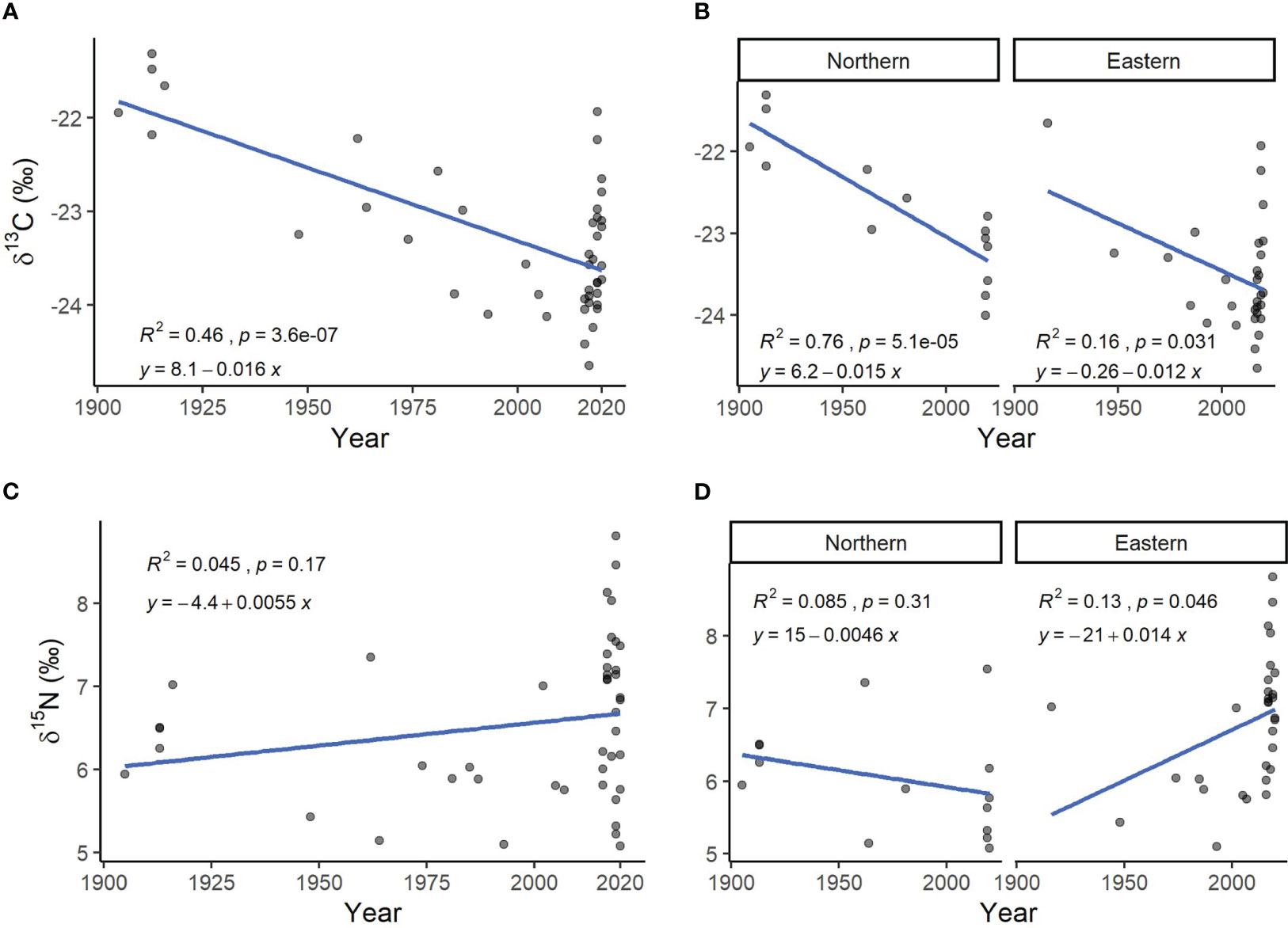
Figure 2 δ13C (A, B) and δ15N (C, D) isotopic values over the years 1905–2020 of hair samples (N = 44) from wolverines in Finland (A, C) and per study region (B, D). Linear regression equations are given ‘y = α+ βx’ and shown as a blue line, the coefficient of determination is given as ‘R²’, and the p-values as ‘p’. Overlapping points are shown as darker grey. δ13C values prior to 2020 were corrected to account for the Suess effect.
The isotopic niche of the overall Historical population was significantly different from the Recent populations (OverlapFinland = 33%, Figure 3A), though there was no significant difference in the niche breadth extent (PPH>R = 0.32, Figure 3B). When divided between study areas, isotopic niche overlaps between Historical and Recent populations were small, ranging from 22% in the Eastern to 24% in the Northern region. In the Northern region, the SEAB of the Historical population was 1.40 ‰² (95%CI: 1.08–1.95 ‰², N = 7) and of the Recent population 2.00‰² (95%CI: 1.43–2.82‰², N = 7). SEAB values of a similar range were found in the Eastern region (Historical: 1.37‰², 95%CI: 1.00–1.97‰², N = 6; Recent: 1.35‰², 95%CI: 1.17–2.57‰², N = 26). Differences in SEAB between the Recent and Historical populations were not statistically significant in Finland or in the regions (Northern: PPH>R = 0.60; Eastern: PPH>R = 0.33).
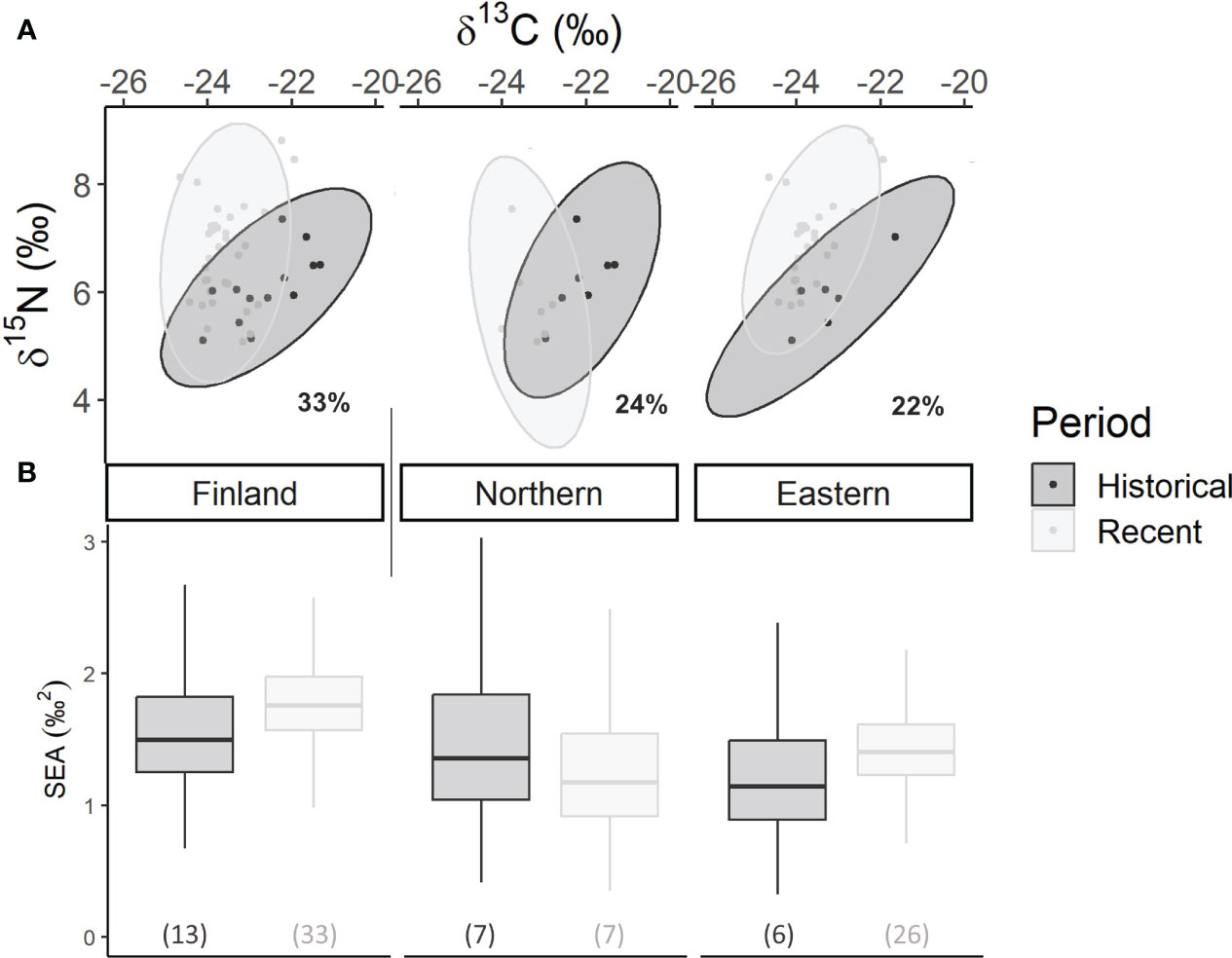
Figure 3 Reconstructed isotopic niches for ellipses and standard ellipses area (SEA) of Historical and Recent wolverine hair samples throughout Finland and divided over two study areas. (A) Bivariate plots of δ13C and δ15N showing the SIBER 95% standard ellipse areas surrounding the reconstructed isotopic niche spaces of Historical (dark grey) and Recent (light grey) wolverines in Finland and in the study areas. Overlap between Historical and Recent population’s ellipses are given as percentages. δ13C values prior to 2020 were corrected to account for the Suess effect. (B) Boxplots of the posterior predictive estimates of the Bayesian standard ellipse areas (SEAB) modeled in SIBER comparing Historical (dark grey) and Recent (light grey) wolverines in the study areas. Sample sizes are given in brackets for each period and study area.
4 Discussion
4.1 Long-term diet shift of wolverines in Finland
The δ13C values of wolverine hairs became depleted during the last 100 years in both Eastern and Northern study areas of Finland. δ13C shifts in generalist predators are often linked with the predator shifting its diet toward an alternative prey type, e.g. from planktivorous to benthic-feeding prey (Hempson et al., 2017), or herbivorous to detritivorous prey (Wise et al., 2006). Interestingly, our results are consistent with Dalerum et al. (2009a), who observed similar patterns of isotopic values from various tissues of Alaskan wolverines over a shorter period (6 years). In Alaska, this change in δ13C values was associated with a shift in their diet composition from caribou toward moose (Dalerum et al., 2009b). Our results suggest a possible change in the diet composition of Finnish wolverines, shifting towards an alternative prey, though without a change in trophic level.
Our results further support a possible diet shift by the small overlap between the Historical and Recent wolverine population’s reconstructed isotopic niches (Figure 3). Small overlaps in isotopic niches have been shown to reflect for example long-term shifts in the diet of aquatic consumers (Secchi et al., 2017; Reynolds et al., 2019). For another boreal large carnivore, Hilderbrand et al. (1996) found a long-term shift (1916–1994) in the diet of grizzly bears (Ursus arctos horribilis) in North America by comparing the isotopic values of tissues from a historical and a modern population. In this case, the difference in isotopic niches between the temporal populations was linked to a change in the grizzly’s diet from salmon toward terrestrial prey, as increased damming negatively affected salmon availability.
In the current wolverine study, the enriched δ15N values and the variance observed were typical of a generalist consumer at a high trophic level (DeNiro and Epstein, 1981). Nonetheless, the absence of a clear trend in δ15N values indicates a lack of a significant diet switch towards an alternative trophic level during the study period (Stanek et al., 2017). Thus, the trophic level of wolverines in Finland during the last century was likely stable despite an alteration of habitat, climate and an associated change in prey community. This is consistent with SIA of Alaskan wolverines (Dalerum et al., 2009a) and has also been observed in other generalist consumers, such as marine birds or fur seals (Ceia et al., 2012; Vales et al., 2020).
Despite the shift in the isotopic and dietary niche, there was no significant temporal variation in the niche breadth of wolverines. In Norway, Van Dijk et al. (2008) suggested that the niche breadth of wolverines would decrease with grey wolf recolonization, as wolves provide moose carrion which the wolverine can specialize on. However, in our Eastern study area, where wolves recolonized their former range after 1990 (Kojola et al., 2006; Mykrä et al., 2017; Heikkinen et al., 2021b), we did not observe a significant difference between the isotopic niche breadth of the Historical and Recent wolverine populations. A possibility is that coexistence with wolves might not be the sole driver of the wolverine niche breadth extant, especially in a community of several other large carnivores. The Eurasian lynx also recolonized its former range and acts as a carrion provider throughout Fennoscandia, making carcasses of diverse prey species available for wolverines (Sunde and Kvam, 1997; Andrén et al., 2011; Mattisson et al., 2011). Thus, the overall long-term stability of the wolverine niche breadth might be partly caused by wolverines scavenging from carrion made available by several coexisting large carnivores (Magoun, 1987).
Considering other generalist consumers and predators, long-term diet shifts have typically been associated with changes in prey communities (Farmer and Leonard, 2011; Secchi et al., 2017) resulting from multiple factors such as overharvesting (Reynolds et al., 2019), habitat degradation (Hempson et al., 2017), or anthropogenic disturbances (Hilderbrand et al., 1996). The Finnish boreal forest in our study areas is known to have largely lost its ecosystem integrity over the past century (Grantham et al., 2020). Factors such as climate change (Fraixedas et al., 2015), the intensification of forestry practices (Järvinen et al., 1977; Vasander, 2006), urbanization (Scrafford et al., 2018), and fluctuation in ungulate populations (Nygrén, 1987; Luoma, 2002) could all have led to changes in the wolverine’s prey communities, resulting in the observed diet shift. In any case, the remarkable stability of the Finnish wolverine’s niche breadth through a century of habitat modification and dietary shift may indicate the resilience to trophic alteration (Jenkins and Davoren, 2021), with subsequent conservation implications for wolverines.
4.2 Implications for wolverine conservation
Generalists often respond the most successfully to trophic disturbances (Berumen and Pratchett, 2008; Peers et al., 2014), especially in cases of alterations of their prey community (Wilmers and Post, 2006; Christian et al., 2009). As the persistence of a consumer in a changing ecosystem is partly conditioned to its dietary plasticity (Silver and Marsh, 2003; Dunham, 2017), the wolverine might be particularly resilient to fluctuations in prey abundance and availability. The diet shift of wolverines in Finland over the last century confirms the versatility of this generalist consumer to adapt to long-term changes in its environment. Although our results indicate trophic resilience, preserving the boreal ecosystem remains highly important as food-web alterations can reach a tipping point after which the survival of consumers is put at risk (Llope et al., 2011; Griffith et al., 2019).
At a local scale, our results show that the current diet niche of wolverines in the Northern area is not smaller than the niche of the Eastern area. These results indicate that wolverines living within the reindeer herding area are as generalist predators as the wolverines living outside of it. Somewhat surprisingly the degree of specialization of wolverines in the Northern study area did not significantly change over time, even though the abundance of semi-domestic reindeer varied greatly (Helle and Kojola, 2006; Uboni et al., 2016). Previously, Koskela et al. (2013a) detected that reindeer accounts as a significant part of the wolverine diet. Additional wolverine diet studies in the RMA are thus necessary to assess if the current generalist population is composed of a few individuals that specialize on reindeer, i.e. specific individuals taking a disproportionate reindeer toll, or if the majority of wolverines are generalists (Mcaulay et al., 2021).
4.3 SIA for diet studies of large carnivores
While the causality between diet shifts and observed long-term shift in stable isotopes values is supported by many studies (Chamberlain et al., 2005; Kaczensky et al., 2017; Mariano-Jelicich et al., 2017; Reynolds et al., 2019), there are other factors that could explain the changes in isotopic values. Metabolic processes, for example, can cause short-term and seasonal variation in isotopic values (Ben-David and Flaherty, 2012; Newton, 2016). However, our study encompasses 44 individuals sampled over a century, so the short-term and individual-specific variations are most likely not affecting the observed long-term trend (Post, 2002; Dalerum and Angerbjörn, 2005). Given this long study period, our results could be affected by background fluctuations in isotopic ratios. For example, the observed trend in carbon isotopic values of the wolverine tissues could be impacted by long-term changes in the isotopic values of the prey items themselves (Dalerum et al., 2009a). Nevertheless, the scale of this shift at a prey species level would not significantly impact the isotopic values of a predator at a higher trophic level (Calandra et al., 2015). Hypothetically, even if there were a strong shift in the isotopic values of a dietary source, its effect on the consumer’s values would likely be reduced by the diverse diet composition of wolverines. Although a unidirectional shift in isotopic values across all dietary sources cannot be ruled out, this explanation is of little parsimony and is not supported by long-term SIA studies to our knowledge (Balčiauskas and Balčiauskienė, 2022). Therefore, the observed shift in isotopic niche and trend in hair isotopic values are best explained by the hypothesis of a long-term diet shift of the wolverine.
Our study further demonstrates the potential of using SIA on museum and recent samples to infer changes in the trophic ecology of large carnivores, particularly in the long term. Fisher et al. (2022) noted that as research on wolverines started decades after their range collapse, ecological investigations are limited to the remaining present populations. Our results nonetheless suggest that SIA can shed light on the trophic ecology of past and extinct populations of wolverines, and could easily be extended to other species with key trophic roles. For extinct populations with only museum samples left, SIA provides the only record of dietary change over historical time scales of range contraction and population collapse. Remarkably, the samples collected for SIA studies also open up ways for complementary studies, e.g. on population genetics (Lansink et al., 2020).
5 Conclusion
We were able to identify a long-term diet shift of wolverines in Finland. There was, however, no change in the degree of specialization nor in the trophic level of the wolverine, thus suggesting a high dietary resilience and adaptation to multiple alterations in their food web. Overall, SIA proved to be a suitable tool to study long-term diet trends in top consumers of terrestrial food webs, especially when hair samples from both museum collections and modern studies are available. We thus encourage further long-term stable isotope analysis on large carnivores to assess their past diet niches and to provide a better understanding of their ecological context for the conservation of present populations. Finally, as the diet of carnivores is considered an indicator of the overall status of biodiversity within an area (Sergio et al., 2008; Reynolds et al., 2019), reconstructing their past and present trophic niches could help to detect signs of temporal changes in biodiversity.
Data availability statement
The original contributions presented in the study are included in the article/Supplementary Material, further inquiries can be directed to the corresponding author/s.
Ethics statement
Ethical approval was not required for the study involving animals in accordance with the local legislation and institutional requirements because we used historical hair samples from museum skins and stuffed specimens. I used modern samples by re-using leftover hair samples from a previous study on wolverine genetics, and from individuals found dead or legally culled independently from our research. As no animal was handled or killed in link with this research, no ethical approval was required.
Author contributions
CM: Conceptualization, Formal analysis, Investigation, Methodology, Software, Visualization, Writing – original draft. TH: Investigation, Methodology, Software, Writing – original draft. GL: Conceptualization, Investigation, Resources, Writing – original draft. KH: Resources, Writing – review & editing. MI: Resources, Writing – review & editing. IK: Resources, Writing – review & editing. JA: Project administration, Supervision, Writing – review & editing. JW: Conceptualization, Funding acquisition, Investigation, Project administration, Resources, Supervision, Writing – review & editing.
Funding
The author(s) declare that no financial support was received for the research, authorship, and/or publication of this article.
Acknowledgments
This project would not have been possible without the permission to collect museum samples from the Natural History Museums of Helsinki, Kuopio, and Oulu. We want to thank the field technicians of the Finnish Natural Resources Institute for their work in the field to collect modern samples. Thanks to the ENRI (Environment and Natural Resource Institute) Stable Isotope Lab, University of Alaska Anchorage, for conducting the δ13C and δ15N measures and analysis.
Conflict of interest
The authors declare that the research was conducted in the absence of any commercial or financial relationships that could be construed as a potential conflict of interest.
Publisher’s note
All claims expressed in this article are solely those of the authors and do not necessarily represent those of their affiliated organizations, or those of the publisher, the editors and the reviewers. Any product that may be evaluated in this article, or claim that may be made by its manufacturer, is not guaranteed or endorsed by the publisher.
Supplementary material
The Supplementary Material for this article can be found online at: https://www.frontiersin.org/articles/10.3389/fevo.2023.1284901/full#supplementary-material
References
Abramov A. V. (2016). Gulo gulo. The IUCN Red List of Threatened Species 2016. T9561A45198537. doi: 10.2305/IUCN.UK.2016-1.RLTS.T9561A45198537.en
Allen B. L., Allen L. R., Andrén H., Ballard G., Boitani L., Engeman R. M., et al. (2017). Can we save large carnivores without losing large carnivore science? Food Webs 12 (1), 64–75. doi: 10.1016/j.fooweb.2017.02.008
Andrén H., Persson J., Mattisson J., Danell A. C. (2011). Modelling the combined effect of an obligate predator and a facultative predator on a common prey: Lynx (Lynx lynx) and wolverine (Gulo gulo) predation on reindeer (Rangifer tarandus). Wildl. Biol. 17 (1), 33–43. doi: 10.2981/10-065
Balčiauskas L., Balčiauskienė L. (2022). Long-term changes in a small mammal community in a temperate zone meadow subject to seasonal floods and habitat transformation. Integr. Zool. 17 (3), 443–455. doi: 10.1111/1749-4877.12571
Balza U., Lois N. A., Polito M. J., Pütz K., Salom A., Raya Rey A. (2020). The dynamic trophic niche of an island bird of prey. Ecol. Evol. 10 (21), 12264–12276. doi: 10.1002/ece3.6856
Barja I., Navarro-Castilla Á., Pérez L. (2016). Effectiveness and applications of hair traps for the study of wild mammal populations. Polish J. Ecol. 64 (3), 409–419. doi: 10.3161/15052249PJE2016.64.3.010
Beckmann J. P., Berger J. (2003). Rapid ecological and behavioural changes in carnivores: The responses of black bears (Ursus americanus) to altered food. J. Zool. 261 (2), 207–212. doi: 10.1017/S0952836903004126
Ben-David M., Flaherty E. A. (2012). Stable isotopes in mammalian research: A beginner’s guide. J. Mammal. 93 (2), 312–328. doi: 10.1644/11-MAMM-S-166.1
Ben-David M., Flynn R. W., Schell D. M. (1997). Annual and seasonal changes in diets of martens: Evidence from stable isotope analysis. Oecologia 111 (2), 280–291. doi: 10.1007/s004420050236
Berumen M. L., Pratchett M. S. (2008). Trade-offs associated with dietary specialization in corallivorous butterflyfishes (Chaetodontidae: Chaetodon). Behav. Ecol. Sociobiol. 62 (6), 989–994. doi: 10.1007/s00265-007-0526-8
Bol R., Eriksen J., Smith P., Garnett M. H., Coleman K., Christensen B. T. (2005). The natural abundance of 13C, 15N, 34S and 14C in archived, (1923-2000) plant and soil samples from the Askov long-term experiments on animal manure and mineral fertilizer. Rapid Commun. Mass Spectrom. 19 (22), 3216–3226. doi: 10.1002/rcm.2156
Buelow C. A., Reside A. E., Baker R., Sheaves M. (2018). Stable isotopes reveal opportunistic foraging in a spatiotemporally heterogeneous environment: Bird assemblages in mangrove forests. PloS One 13 (11). doi: 10.1371/journal.pone.0206145
Buskirk S. W. (2023). Carnivoran Ecology: The Evolution and Function of Communities (Oxford, United Kingdom: Oxford Press). doi: 10.1093/oso/9780192863249.001.0001
Calandra I., Labonne G., Mathieu O., Henttonen H., Lévêque J., Milloux M. J., et al. (2015). Isotopic partitioning by small mammals in the subnivium. Ecol. Evol. 5 (18), 4132–4140. doi: 10.1002/ece3.1653
Carbonell Ellgutter J. A., Ehrich D., Killengreen S. T., Ims R. A., Unnsteinsdóttir E. R. (2020). Dietary variation in Icelandic arctic fox (Vulpes lagopus) over a period of 30 years assessed through stable isotopes. Oecologia 192 (2), 403–414. doi: 10.1007/s00442-019-04580-0
Careddu G., Ciucci P., Mondovì S., Calizza E., Rossi L., Costantini M. L. (2021). Gaining insight into the assimilated diet of small bear populations by stable isotope analysis. Sci. Rep. 11 (1), 1–16. doi: 10.1038/s41598-021-93507-y
Carney B., Tessler D., Coletti H., Welker J. M., Causey D. (2023). Stable isotope-determined diets of black oystercatchers (Haematopus bachmani) in the Northern gulf of Alaska. Marine Ornithology, 51, 125–135.
Catry T., Lourenço P. M., Lopes R. J., Carneiro C., Alves J. A., Costa J., et al. (2016). Structure and functioning of intertidal food webs along an avian flyway: A comparative approach using stable isotopes. Funct. Ecol. 30 (3), 468–478. doi: 10.1111/1365-2435.12506
Ceia F. R., Phillips R. A., Ramos J. A., Cherel Y., Vieira R. P., Richard P., et al. (2012). Short- and long-term consistency in the foraging niche of wandering albatrosses. Mar. Biol. 159 (7), 1581–1591. doi: 10.1007/s00227-012-1946-1
Chamberlain C. P., Waldbauer J. R., Fox-Dobbs K., Newsome S. D., Koch P. L., Smiths D. R., et al. (2005). “Pleistocene to recent dietary shifts in California condors,” in Proceedings of the National Academy of Sciences of the United States of America, Vol. 102. 16707–16711. doi: 10.1073/pnas.0508529102
Christian K., Isabelle L. V., Frédéric J., Vincent D. (2009). More species, fewer specialists: 100 years of changes in community composition in an island biogeographical study. Diversity Distrib. 15 (4), 641–648. doi: 10.1111/j.1472-4642.2009.00569.x
Dalerum F., Angerbjörn A. (2005). Resolving temporal variation in vertebrate diets using naturally occurring stable isotopes. Oecologia 144 (4), 647–658. doi: 10.1007/s00442-005-0118-0
Dalerum F., Angerbjörn A., Kunkel K., Shults B. S. (2009a). Pattern of C13 and N15 in wolverine Gulo gulo tissues from the Brooks Range, Alaska. Curr. Zool. 55 (3), 188–192. doi: 10.1093/czoolo/55.3.188
Dalerum F., Kunkel K., Angerbjörn A., Shults B. S. (2009b). Diet of wolverines (Gulo gulo) in the western Brooks Range, Alaska. Polar Res. 28 (2), 246–253. doi: 10.1111/j.1751-8369.2008.00090.x
DeNiro M. J. (1985). Postmortem preservation and alteration of in vivo bone collagen isotope ratios in relation to palaeodietary reconstruction. Nature 317, 806–809. doi: 10.1038/317806a0
DeNiro M. J., Epstein S. (1978). Influence of diet on the distribution of carbon isotopes in animals. Geochimica Cosmochimica Acta 42 (5), 495–506. doi: 10.1002/mop.25285
DeNiro M. J., Epstein S. (1981). Influence of diet on the distribution of nitrogen isotopes in animals. Geochimica Cosmochimica Acta 45 (3), 341–351. doi: 10.1016/0016-7037(81)90244-1
Djagoun C. A. M. S., Codron D., Sealy J., Mensah G. A., Sinsin B. (2016). Isotopic niche structure of a mammalian herbivore assemblage from a West African savanna: Body mass and seasonality effect. Mamm. Biol. 81 (6), 644–650. doi: 10.1016/j.mambio.2016.09.001
Dunham N. T. (2017). Feeding Ecology and Dietary Flexibility of Colobus angolensis palliatus in Relation to Habitat Disturbance. Int. J. Primatol. 38 (3), 553–571. doi: 10.1007/s10764-017-9965-x
English P. A., Green D. J., Nocera J. J. (2018). Sable isotopes from museum specimens may provide evidence of long-term change in the trophic ecology of a migratory aerial insectivore. Front. Ecol. Evol. 6 (14). doi: 10.3389/fevo.2018.00014
Farmer R. G., Leonard M. L. (2011). Long-term feeding ecology of great black-backed Gulls (Larus marinus) in the northwest Atlantic: 110 years of feather isotope data. Can. J. Zool. 89 (2), 123–133. doi: 10.1139/Z10-102
Felix J. D., Elliott E. M. (2013). The agricultural history of human-nitrogen interactions as recorded in ice core δ15N-NO3-. Geophys. Res. Lett. 40 (8), 1642–1646. doi: 10.1002/grl.50209
Fisher J. T., Murray S., Barrueto M., Carroll K., Clevenger A. P., Hausleitner D., et al. (2022). Wolverines (Gulo gulo) in a changing landscape and warming climate: A decadal synthesis of global conservation ecology research. Global Ecol. Conserv. 34. doi: 10.1016/j.gecco.2022.e02019
Fraixedas S., Lindén A., Lehikoinen A. (2015). Population trends of common breeding forest birds in southern Finland are consistent with trends in forest management and climate change. Ornis Fennica 92 (4), 187–203.
Francey R. J., Allison C. E., Etheridge D. M., Trudinger C. M., Enting I. G., Leuenberger M., et al. (1999). A 1000-year high precision record of δ13C in atmospheric CO2. Tellus B: Chem. Phys. Meteorol. 51 (2), 170–193. doi: 10.3402/tellusb.v51i2.16269
Galicia M. P., Thiemann G. W., Dyck M. G., Ferguson S. H., Higdon J. W. (2016). Dietary habits of polar bears in Foxe Basin, Canada: possible evidence of a trophic regime shift mediated by a new top predator. Ecol. Evol. 6 (16), 6005–6018. doi: 10.1002/ece3.2173
Grantham H. S., Duncan A., Evans T. D., Jones K. R., Beyer H. L., Schuster R., et al. (2020). Anthropogenic modification of forests means only 40% of remaining forests have high ecosystem integrity. Nat. Commun. 11 (1). doi: 10.1038/s41467-020-19493-3
Griffith G. P., Hop H., Vihtakari M., Wold A., Kalhagen K., Gabrielsen G. W. (2019). Ecological resilience of Arctic marine food webs to climate change. Nat. Climate Change 9 (11), 868–872. doi: 10.1038/s41558-019-0601-y
Hamstrom N., Tomco P., Welker J. M. (2023). Strontium isotope analysis, the neonatal line, and archaeological caribou herd identity in northwest Alaska. Arctic Sci. (in Press).
Heikkinen S., Kojola I., Mäntyniemi S. (2021a). Bear population in Finland 2021 (Natural Resources Institute Finland). Luonnonvara-ja biotalouden tutkimus 32/2022 (in Finnish only).
Heikkinen S., Valtonen M., Härkälä A., Helle I., Mäntyniemi S., Kojola I. (2021b). Wolf population in Finland 2021 (Natural Resources Institute Finland). Luonnonvara- ja biotalouden tutkimus 39/2021 (in Finnish only).
Helle T., Kojola I. (2006). “Population Trends of Semi-domesticated Reindeer in Fennoscandia – Evaluation of Explanations,” in Reindeer Management in Northernmost Europe. Ecological Studies, vol. 184 . Eds. Forbes B. C., et al (Berlin, Heidelberg: Springer). doi: 10.1007/3-540-31392-3_16
Hempson T. N., Graham N. A. J., MacNeil M. A., Williamson D. H., Jones G. P., Almany G. R. (2017). Coral reef mesopredators switch prey, shortening food chains, in response to habitat degradation. Ecol. Evol. 7 (8), 2626–2635. doi: 10.1002/ece3.2805
Hilderbrand G. V., Farley S. D., Robbins C. T., Hanley T. A., Titus K., Servheen C. (1996). Use of stable isotopes to determine diets of living and extinct bears. Can. J. Zool. 74 (11), 2080–2088. doi: 10.1139/z96-236
Holmala K., Valtonen M., Herrero A. (2021). Lynx population in Finland 2021 (Natural Resources Institute Finland). Luonnonvara- ja biotalouden tutkimus 70/2021 (in Finnish only).
Hyvärinen E., Juslén A., Kemppainen E., Uddström A., Liukko U.-M. (2019). The 2019 Red List of Finnish Species Vol. 704 (Helsinki: The Finnish Ministry of Environment and the Finnish Environment Institute). Available at: http://hdl.handle.net/10138/299501.
Jackson A. L., Inger R., Parnell A. C., Bearhop S. (2011). Comparing isotopic niche widths among and within communities: SIBER - Stable Isotope Bayesian Ellipses in R. J. Anim. Ecol. 80 (3), 595–602. doi: 10.1111/j.1365-2656.2011.01806.x
Järvinen O., Kuusela K., Väisänen R. (1977). Effects of modern forestry on the numbers of breeding birds in Finland in 1945-1975. Silva Fennica 11 (4), 284–294.
Jedrzejewska B., Jedrzejewski W. (1998). “Predation in Vertebrate Communities,” in Ecological Studies (Heidelberg: Springer Berlin). doi: 10.1007/978-3-662-35364-6
Jenkins E. J., Davoren G. K. (2021). Seabird species- and assemblage-level isotopic niche shifts associated with changing prey availability during breeding in coastal Newfoundland. Ibis 163 (1), 183–196. doi: 10.1111/ibi.12873
Jennings S., Van Der Molen J. (2015). Trophic levels of marine consumers from nitrogen stable isotope analysis: Estimation and uncertainty. ICES Journal of Marine Science 72 (8), 2289–2300. doi: 10.1093/icesjms/fsv120
Kaczensky P., Burnik Šturm M., Sablin M. V., Voigt C. C., Smith S., Ganbaatar O., et al. (2017). Stable isotopes reveal diet shift from pre-extinction to reintroduced Przewalski’s horses. Sci. Rep. 7 (1), 1–9. doi: 10.1038/s41598-017-05329-6
Keeling R. F., Graven H. D., Welp L. R., Resplandy L., Bi J., Piper S. C., et al. (2017). “Atmospheric evidence for a global secular increase in carbon isotopic discrimination of land photosynthesis,” in Proceedings of the National Academy of Sciences of the United States of America, Vol. 114. 10361–10366. doi: 10.1073/pnas.1619240114
Kojola I., Aspi J., Hakala A., Heikkinen S., Ilmoni C., Ronkainen S. (2006). Dispersal in an expanding wolf population in Finland. Journal of Mammalogy 87 (2), 281–286. doi: 10.1644/05-MAMM-A-061R2.1
Kojola I., Heikkinen S., Mäntyniemi S., Ollila T. (2022). Wolverine population in Finland 2022 (Natural Resources Institute Finland). Luonnonvara- ja biotalouden tutkimus 101/2022 (in Finnish only).
Koskela A., Kojola I., Aspi J., Hyvärinen M. (2013a). The diet of breeding female wolverines (Gulo gulo) in two areas of Finland. Acta Theriol. 58 (2), 199–204. doi: 10.1007/s13364-012-0111-z
Koskela A., Kojola L., Aspi J., Hyvärinen M. (2013b). Effect of reproductive status on the diet composition of wolverines (Gulo gulo) in boreal forests of eastern Finland. Annales Zoologici Fennici 50 (1–2), 100–106. doi: 10.5735/086.050.0109
Krumsick K. J., Fisher J. A. D. (2019). Spatial and ontogenetic variation in isotopic niche among recovering fish communities revealed by Bayesian modeling. PloS One 14 (4), 1–21. doi: 10.1371/journal.pone.0215747
Kumar B. (2011). Stable isotopes. (Dordrecht: Springer). 1078–1079. doi: 10.1007/978-90-481-2642-2_536
Kyle C. J., Strobeck C. (2002). Connectivity of peripheral and core populations of North American wolverines. J. Mammal. 83 (4), 1141–1150. doi: 10.1644/1545-1542(2002)083<1141:COPACP>2.0.CO;2
Landa A., Lindén M., Kojola I. (2000). Action Plan for the conservation of Wolverines (Gulo gulo) in Europe. Nat. Environ. 115 (115), 44. Council of Europe Publishing.
Lansink G., Esparza-Salas R., Joensuu M., Koskela A., Bujnáková D., Kleven O., et al. (2020). Population genetics of the wolverine in Finland: the road to recovery? Conserv. Genet. 21 (3), 481–499. doi: 10.1007/s10592-020-01264-8
Lansink G., Kleven O., Ekblom R., Spong G., Kopatz A., Mattisson J., et al. (2022). Potential for increased connectivity between differentiated wolverine populations. Biol. Conserv. 272 (August), 109601. doi: 10.1016/j.biocon.2022.109601
Layman C. A., Arrington D. A., Montaña C. G., Post D. M. (2007). Can stable isotope ratios provide for community-wide measures of trophic structure? Ecology 88 (1), 42–48. doi: 10.1890/0012-9658(2007)88[42:CSIRPF]2.0.CO;2
Liu G., Yin Z., Yan G., Liu S., Wang X., Xing Y., et al. (2022). Effects of long-term nitrogen addition on the δ15N and δ13C of Larix gmelinii and soil in a boreal forest. Ecol. Processes 11 (1). doi: 10.1186/s13717-022-00382-0
Llope M., Daskalov G. M., Rouyer T. A., Mihneva V., Chan K. S., Grishin A. N., et al. (2011). Overfishing of top predators eroded the resilience of the Black Sea system regardless of the climate and anthropogenic conditions. Global Change Biol. 17 (3), 1251–1265. doi: 10.1111/j.1365-2486.2010.02331.x
Long E. S., Sweitzer R. A., Diefenbach D. R., Ben-David M. (2005). Controlling for anthropogenically induced atmospheric variation in stable carbon isotope studies. Oecologia 146 (1), 148–156. doi: 10.1007/s00442-005-0181-6
Luoma A. (2002). Moose hunting in Finland: management of a heavily harvested population (Helsinki, Finland: Publications of the University of Helsinki). Available at: http://ethesis.helsinki.fi/julkaisut/mat/ekolo/vk/luoma/.
MacDonald D. W., Harrington L. A., Newman C. (2017). Dramatis personae: an introduction to the wild musteloids. Biology and Conservation of Musteloids. Oxford: Oxford Academic, 39–40.
Magoun A. J. (1987). Summer and winter diets of wolverines, gulo gulo, in Arctic Alaska. Can. Field-Natural. 101 (3), 392–393.
Mariano-Jelicich R., Copello S., Seco Pon J. P., Favero M. (2017). Long-term changes in Black-browed albatrosses diet as a result of fisheries expansion: an isotopic approach. Mar. Biol. 164 (6). doi: 10.1007/s00227-017-3176-z
Marshall J. D., Renée Brooks J., Lajtha K. (2007). Sources of variation in the stable isotopic composition of plants. In. Stable Isotopes Ecol. Environ. Sci., 22–60. doi: 10.1002/9780470691854
Mattisson J., Andrén H., Persson J., Segerström P. (2011). Influence of intraguild interactions on resource use by wolverines and Eurasian lynx. J. Mammal. 92 (6), 1321–1330. doi: 10.1644/11-MAMM-A-099.1
Mcaulay J. R., Monks J. M., Wilson D. J., Seddon P. J. (2021). Individual specialists within a generalist niche: variable diet of stoats and implications for conservation. New Z. J. Ecol. 45 (2), 1–10. doi: 10.20417/nzjecol.45.29
Moreno R., Jover L., Diez C., Sardà F., Sanpera C. (2013). Ten years after the prestige oil spill: seabird trophic ecology as indicator of long-term effects on the coastal marine ecosystem. PloS One 8 (10). doi: 10.1371/journal.pone.0077360
Myhre R., Myrberget S. (1975). Diet of wolverines (Gulo gulo) in Norway. J. Mammal. 56 (4), 752–757. doi: 10.2307/1379650
Mykrä S., Pohja-Mykrä M. (2015). Back-calculation of large carnivore populations in Finland in 1865–1915. Annales Zoologici Fennici 52 (5–6), 285–300. doi: 10.5735/086.052.0504
Mykrä S., Pohja-Mykrä M., Vuorisalo T. (2017). Hunters’ attitudes matter: diverging bear and wolf population trajectories in Finland in the late nineteenth century and today. European Journal of Wildlife Research 63(5). doi: 10.1007/s10344-017-1134-1
Nature Conservation Act (1996) The Finnish Ministry of Environment. Available at: https://www.finlex.fi/en/laki/kaannokset/1996/en19961096.
Newton J. (2016). Stable isotopes as tools in ecological research. ELS 1–8. doi: 10.1002/9780470015902.a0021231.pub2
Nilsen E. B., Christianson D., Gaillard J.-M., Halley D., Linnell J. D. C., Odden M., et al. (2012). “Describing food habits and predation: field methods and statistical considerations,” in Carnivore Ecology and Conservation: A Handbook of Techniques (Oxford, 2012: Oxford Press), 256–272. doi: 10.1093/acprof:oso/9780199558520.003.0011
Peers M. J. L., Wehtje M., Thornton D. H., Murray D. L. (2014). Prey switching as a means of enhancing persistence in predators at the trailing southern edge. Global Change Biol. 20 (4), 1126–1135. doi: 10.1111/gcb.12469
Peziol M., Elbroch L. M., Shipley L. A., Evans R. D., Thornton D. H. (2023). Large carnivore foraging contributes to heterogeneity in nutrient cycling. Landscape Ecol. 38 (6), 1497–1509. doi: 10.1007/s10980-023-01630-0
Pohja-Mykrä M., Vuorisalo T., Mykrä S. (2005). Hunting bounties as a key measure of historical wildlife management and game conservation: Finnish bounty schemes 1647-1975. Oryx 39(3), 284–291. doi: 10.1017/S0030605305000785
Post D. M. (2002). Using stable isotopes to estimate trophic position: Models, methods, and assumptions. Ecology 83 (3), 703–718. doi: 10.1890/0012-9658(2002)083[0703:USITET]2.0.CO;2
Rasmus S., Kojola I., Turunen M., Norberg H., Kumpula J., Ollila T. (2020). Mission impossible? Pursuing the co-existence of viable predator populations and sustainable reindeer husbandry in Finland. J. Rural Stud. 80, 135–148. doi: 10.1016/j.jrurstud.2020.08.017
Reynolds S. J., Hughes B. J., Wearn C. P., Dickey R. C., Brown J., Weber N. L., et al. (2019). Long-term dietary shift and population decline of a pelagic seabird—A health check on the tropical Atlantic? Global Change Biol. 25 (4), 1383–1394. doi: 10.1111/gcb.14560
Ripple W. J., Estes J. A., Beschta R. L., Wilmers C. C., Ritchie E. G., Hebblewhite M., et al. (2014). Status and ecological effects of the world’s largest carnivores. Science 343, 1241484. doi: 10.1126/science.1241484
Rogers M. C., Hilderbrand G. V., Gustine D. D., Joly K., Leacock W. B., Mangipane B. A., et al. (2020). Splitting hairs: dietary niche breadth modelling using stable isotope analysis of a sequentially grown tissue. Isotopes Environ. Health Stud., 358–369. doi: 10.1080/10256016.2020.1787404
Rogers M. C., Peacock E., Simac K., O’Dell M. B., Welker J. M. (2015). Diet of female polar bears in the southern Beaufort Sea of Alaska: evidence for an emerging alternative foraging strategy in response to environmental change. Polar Biol. 38 (7), 1035–1047. doi: 10.1007/s00300-015-1665-4
Samelius G., Alisauskas R. T., Larivière S., Bergman C., Hendrickson C. J., Phipps K., et al. (2002). Foraging behaviours of wolverines at a large arctic goose colony. Arctic 55 (2), 148–150. doi: 10.14430/arctic699
Schwensow N. I., Heni A. C., Schmid J., Montero B. K., Brändel S. D., Halczok T. K., et al. (2022). Disentangling direct from indirect effects of habitat disturbance on multiple components of biodiversity. J. Anim. Ecol. 91 (11), 2220–2234. doi: 10.1111/1365-2656.13802
Scrafford M. A., Avgar T., Heeres R., Boyce M. S. (2018). Roads elicit negative movement and habitat-selection responses by wolverines (Gulo gulo luscus). Behav. Ecol. 29 (3), 534–542. doi: 10.1093/beheco/arx182
Secchi E. R., Botta S., Wiegand M. M., Lopez L. A., Fruet P. F., Genoves R. C., et al. (2017). Long-term and gender-related variation in the feeding ecology of common bottlenose dolphins inhabiting a subtropical estuary and the adjacent marine coast in the western South Atlantic. Mar. Biol. Res. 13 (1), 121–134. doi: 10.1080/17451000.2016.1213398
Sergio F., Caro T., Brown D., Clucas B., Hunter J., Ketchum J., et al. (2008). Top predators as conservation tools: Ecological rationale, assumptions, and efficacy. Annu. Rev. Ecol. Evol. System. 39, 1–19. doi: 10.1146/annurev.ecolsys.39.110707.173545
Shardlow T. F. (2013). Isotopic evidence of salmon, Oncorhynchus spp., in the diet of the Wolverine, Gulo gulo, on Princess Royal Island, British Columbia. Can. Field-Natural. 127 (4), 338–342. doi: 10.22621/cfn.v127i4.1515
Silver S. C., Marsh L. K. (2003). Dietary flexibility, behavioral plasticity, and survival in fragments: lessons from translocated howlers. Primates Fragments, 251–265. doi: 10.1007/978-1-4757-3770-7_16. Springer.
Stanek A., Mangipane B., Wolf N., Hildebrand G. V., Mangipane B., Causey D. C., et al. (2017). Seasonal foraging strategies of Alaskan gray wolves (Canis lupus) in an ecosystem subsidized by Pacific salmon (Oncorhynchus spp.). Can. J. Zool. 95, 555–563. doi: 10.1139/cjz-2016-0203
Stanek A. E., Wolf N., Welker J. M., Jensen S. (2019). Experimentally derived incorporation rates and diet-to-tissue discrimination values for carbon and nitrogen stable isotopes in gray wolves (Canis lupus) fed a marine diet. Can. J. Zool. 97 (12), 1225–1230. doi: 10.1139/cjz-2019-0049
Sugiyama Y., Nishita Y., Lansink G. M. J., Holmala K., Aspi J., Masuda R. (2022). Diversity of the MHC class II DRB gene in the wolverine (Carnivora: Mustelidae: Gulo gulo) in Finland. PloS One 17 (5 May). doi: 10.1371/journal.pone.0267609
Sunde P., Kvam T. (1997). Diet patterns of Eurasian lynx Lynx lynx: what causes sexually determined prey size segregation? Acta Theriol. 42 (2), 189–201. doi: 10.4098/AT.arch.97-21
The Finnish Ministry of Agriculture and Forestry (2014) Finland’s wolverine population management plan. Available at: http://urn.fi/URN.
The Finnish Natural Resources Institute. (2022). Database of large carnivores’ observation in Finland 2022. Available at: https://Luonnonvaratieto.Luke.Fi/Kartat?Panel=suurpedot.
The Finnish Reindeer Herding Association. (2021). Statistics on Reindeer population (in Finnish only), Paliskuntain Yhdistys. Available at: https://paliskunnat.fi/py/materiaalit/tilastot/poromaarien-kehitys/.
Thompson D. R., Furness R. W., Lewis S. A. (1995). Diets and long-term changes in d15N and d13C values in northern fulmars Fulmarus glacialis from two northeast Atlantic colonies. Mar. Ecol. Prog. Ser. 125, 3–11. doi: 10.3354/meps125003
Uboni A., Horstkotte T., Kaarlejärvi E., Sévêque A., Stammler F., Olofsson J., et al. (2016). Long-term trends and role of climate in the population dynamics of Eurasian reindeer. PloS One 11 (6), 1–20. doi: 10.1371/journal.pone.0158359
Vales D. G., Cardona L., Loizaga R., García N. A., Crespo E. A. (2020). Long-term stability in the trophic ecology of a pelagic forager living in a changing marine ecosystem. Front. Mar. Sci. 7. doi: 10.3389/fmars.2020.00087
Van Dijk J., Gustavsen L., Mysterud A., May R., Flagstad Ø., Brøseth H., et al. (2008). Diet shift of a facultative scavenger, the wolverine, following recolonization of wolves. J. Anim. Ecol. 77 (6), 1183–1190. doi: 10.1111/j.1365-2656.2008.01445.x
Vasander H. (2006). “The use of mires for agriculture and forestry,” in Finland – Land of mires (The Finnish Environment Institute), 173–178. Available at: https://helda.helsinki.fi/bitstream/handle/10138/37961/FE_23_2006.pdf?sequence=6#page=175.
Walker J. L., Potter C. W., Macko S. A. (1999). The diets of modern and historic bottlenose dolphin populations reflected through stable isotopes. Mar. Mammal Sci. 15 (2), 335–350. doi: 10.1111/j.1748-7692.1999.tb00805.x
Wilmers C. C., Post E. (2006). Predicting the influence of wolf-provided carrion on scavenger community dynamics under climate change scenarios. Global Change Biol. 12 (2), 403–409. doi: 10.1111/j.1365-2486.2005.01094.x
Wilson E. E., Wolkovich E. M. (2011). Scavenging: How carnivores and carrion structure communities. Trends Ecol. Evol. 26 (3), 129–135. doi: 10.1016/j.tree.2010.12.011
Winnie J., Creel S. (2017). The many effects of carnivores on their prey and their implications for trophic cascades, and ecosystem structure and function. Food Webs 12, 88–94. doi: 10.1016/j.fooweb.2016.09.002
Wise D. H., Moldenhauer D. M., Halaj J. (2006). Using stable isotopes to reveal shifts in prey consumption by generalist predators. Ecol. Appl. 16 (3), 865–876. doi: 10.1890/1051-0761(2006)016[0865:USITRS]2.0.CO;2
Wolf C., Ripple W. J. (2017). Range contractions of the world’s large carnivores. R. Soc. Open Sci. 4 (7). doi: 10.1098/rsos.170052
Keywords: stable isotope analysis, SIA, large carnivores, diet switch, diet niche, isotopic niche
Citation: Massé CF, Hiltunen TA, Lansink GMJ, Holmala K, Isomursu M, Kojola I, Aspi J and Welker JM (2023) Long-term dietary shifts in a generalist predator, the wolverine (Gulo gulo). Front. Ecol. Evol. 11:1284901. doi: 10.3389/fevo.2023.1284901
Received: 29 August 2023; Accepted: 31 October 2023;
Published: 15 November 2023.
Edited by:
Aurelio F. Malo, University of Alcalá, SpainReviewed by:
Antoinette J Piaggio, Animal and Plant Health Inspection Service (USDA), United StatesPedro Miguel Araújo, Coimbra, Portugal
Copyright © 2023 Massé, Hiltunen, Lansink, Holmala, Isomursu, Kojola, Aspi and Welker. This is an open-access article distributed under the terms of the Creative Commons Attribution License (CC BY). The use, distribution or reproduction in other forums is permitted, provided the original author(s) and the copyright owner(s) are credited and that the original publication in this journal is cited, in accordance with accepted academic practice. No use, distribution or reproduction is permitted which does not comply with these terms.
*Correspondence: Clément F. Massé, Y2xlbWVudC5tYXNzZUBvdWx1LmZp