- Department of Environmental Science and Studies, DePaul University, Chicago, IL, United States
Urban trees provide numerous benefits, such as cooling from transpiration, carbon sequestration, and street aesthetics. But volatile organic compound emissions from trees can combine with anthropogenic nitrogen oxide emissions to form ozone, a harmful air pollutant. The most commonly-emitted of these compounds, isoprene, negatively impacts air quality and hence is detrimental to human health. In addition to environmental controls such as light and temperature, the quantity of isoprene emitted from a leaf is a genus-specific trait. Leaf isoprene emission is enzymatically controlled, and species are typically classified as emitters or non-emitters (near-zero emission rates). Therefore, the species composition of urban forests affects whole-system isoprene production. The process of plant invasion alters species composition, and invasive tree species can be either emitters or non-emitters. If an invasive, isoprene-emitting tree species displaces native, non-emitting species, then isoprene emission rates from urban forests will increase, with a concomitant deterioration of air quality. We tested a hypothesis that invasive species have higher isoprene emission rates than native species. Using existing tree species inventory data for the Chicago region, leaf-level isoprene emission rates of the six most common invasive and native tree species were measured and compared. The difference was not statistically significant, but this could be due to the variability associated with making a sufficient number of measurements to quantify species isoprene emission rates. The most common invasive species European buckthorn (Rhamnus cathartica, L.) was an emitter. Because European buckthorn often invades the disturbed edges common in urban forests, we tested a second hypothesis that edge-effect isoprene emissions would significantly increase whole-system modeled isoprene emissions. Using Google Earth satellite imagery to estimate forested area and edge length in the LaBagh Woods Forest Preserve of Cook County (Chicago, IL, USA), edge isoprene emission contributed 8.1% compared to conventionally modeled forest emissions. Our results show that the invasion of European buckthorn has increased isoprene emissions from urban forests. This implies that ecological restoration efforts to remove European buckthorn have the additional benefit of improving air quality.
Introduction
Isoprene (2-methyl-1,3-butadiene) is a biogenic volatile organic compound (BVOC) emitted from the biosphere by vegetation and is the most abundant BVOC emitted into the atmosphere (Guenther et al., 2012). Isoprene is emitted from the chloroplasts of plants in conjunction with the process of photosynthesis and the emission rate increases with temperature and light (Sharkey and Monson, 2017). Not all plant species produce isoprene; isoprene emission is a trait that is most often shared at the genus level. Depending on the species, the plant can be a strong or weak emitter of isoprene (Geron et al., 2001). Isoprene affects air quality because when isoprene interacts with oxides of nitrogen and sunlight, ground-level ozone is produced. Ozone harms human and plant health and therefore isoprene emission can negatively affect air quality (Lee et al., 2006). Climate change factors like elevated temperature can led to an increase in isoprene emissions and therefore worsen air quality. The main interaction between climate change and isoprene emission is that an increase in air temperature will increase the production of isoprene, but there are more complicated interactions (e.g., CO2 concentration, Penuelas and Staudt, 2010). Since ecosystem-level isoprene emissions depend on plant composition, invasion by non-native species is another human-mediated impact.
Despite the poorly understood evolutionary origins of isoprene-emitting plants (Sharkey and Monson, 2017), the role of a species in ecological succession may correlate with isoprene emission capacity (Harley et al., 1999). Isoprene emission is hypothesized to be an adaptation in plants that allows species to retain physiological function in extreme heat events (Sharkey and Singsaas, 1995), which would be beneficial for early-stage successional species. Several studies have observed that isoprene emissions are highest in early to middle succession and decrease in late succession. This was observed in different ecosystems: the savanna-rainforest gradient of central Africa (Klinger et al., 1998), the eastern North American temperate forest (Martin and Guenther, 1995) and the Amazon rainforest (Jardine et al., 2016). Early and middle successional plant species have an association with pioneer species, which can also be invasive (Whitmore, 1989; Vila et al., 2011). Invasive species may therefore have a correlation with greater isoprene emission potential because emission is an adaptation that has an advantage in early and middle succession plants. A hypothetical mechanism is that isoprene synthesis is a mechanism to protect the structures responsible for photosynthesis during high temperature extremes (Vickers et al., 2009). Invasive species would benefit from high isoprene emission because they often establish in disturbed areas where high light environments could lead to thermal stress. It is notable that two highly invasive plant species in the United States are both strong isoprene emitters: kudzu (Pueraria montana, Forseth and Innis, 2004) and phragmites (Phragmites australis, Velikova et al., 2004).
Just as climate change affects plant isoprene emission rates, the process of plant invasion is also linked to climate change. Climate change causes greater temperature extremes, and invasive plants are more temperature flexible compared to native plant species (Flanagan et al., 2015). Sheppard and Stanley (2014) also concluded climate change will further promote the competitive advantage of invasive species. They observed that the invasive plants had a more positive growth response to CO2 concentration and were able to better utilize soil nutrients. Because climate change can promote invasive species, there is a three-way linkage between invasion, climate change and ecosystem isoprene emissions.
In addition to invasive species simply changing the emission capacity of an ecosystem through species composition, many invasive species are edge species adapted to disturbance and high light environments (Yates et al., 2004). Invasive species have been shown to be more frequent and diverse on warm (south and east in the northern hemisphere) forest edges (Brothers and Spingarn, 1992). A study conducted by Pauchard and Alaback (2006) found that invasive species richness increased with decreased canopy cover. Transitional landscapes like forest edges are a gateway for invasive species. Forest disturbance creates edges and can increase the potential for invasion (Brothers and Spingarn, 1992; Pauchard and Alaback, 2006). Forest fragmentation associated with urban development like roadways causes an increase in invasive species richness due to edge creation (Pauchard and Alaback, 2006, Dantas de Paul et al., 2016). Linking to BVOCs, invasive species occupying edges are more exposed to high temperature and light extremes, and hence invasive edge species have the potential to drive higher ecosystem isoprene emissions.
European buckthorn (Rhamnus cathartica, L.) is an extremely common invasive species in the Chicago region (Cook, McHenry, Lake, Kane, DuPage, Kendall, and Will counties, as defined by Nowak et al., 2013). In line with the pattern common for invasive species, European buckthorn is frequently found along forest edges (Schulte et al., 2011). For example, modeling studies that incorporate information about forest edges can more successfully predict European buckthorn invasion (Endicott et al., 2017). In growth form, European buckthorn is a tall understory shrub that can grow up to 3 m in height. Fagan and Peart (2004) observed that invasive glossy buckthorn (R. frangula, L.) reduced the growth and survival of shade-tolerant seedlings and saplings of native canopy tree species. European buckthorn has also been shown to alter soil composition, which could be a possible mechanism European buckthorn uses to increase in abundance (Heneghan et al., 2006).
We hypothesize that the process of plant invasion increases isoprene emissions from urban forests in the Chicago region (H1). Because isoprene emission is genus-specific, relative abundance of emitting species is a key factor for driving regional isoprene emissions in high-NOx, urban environments (Chameides et al., 1988). This links the process of invasion and species composition change to regional isoprene production which affects air quality. The relative contribution of isoprene produced by invasive and native tree species has not been studied in the Chicago region. In support of H1, we hypothesize that invasive species have higher capacities for isoprene emission compared to native species in Chicago urban forests (H1a). Edge effects in disturbed and fragmented forest edges have been associated with an increase in invasive species. However, the contribution of edge effects on ecosystem isoprene emission is unknown. We hypothesize that the edge effect that promotes the invasive species European buckthorn leads to higher system isoprene emissions (H1b).
Materials and Methods
Three experiments were conducted during the summers of 2016, 2017, and 2019 at the LaBagh Woods Forest Preserve of Cook County, (41.9796° N, 87.7438° W). In the summer of 2016, three native and three invasive tree species were measured for average isoprene emission capacity to test hypothesis H1a. In the summer of 2019, three additional invasive and native tree species were measured, bringing the total number of species measured to 12, in support of better testing hypothesis H1a. In the summer of 2017, European buckthorn edge extent was measured and the impacts on ecosystem isoprene emission were modeled using MEGAN (Guenther et al., 2012) to test hypothesis H1b.
Invasive vs. Native Species Emission Capacity, H1a
Native and non-native woody tree species abundance for the Chicago region was extracted from an existing USDA report (Nowak et al., 2013). The three most abundant native tree species were boxelder (Acer negundo), silver maple (A. saccharinum), and black walnut (Juglans nigra). Note at the time of the study green ash (Fraxinus pennsylvanica) was in the list of most abundant species, but most individuals locally have died or been removed due to the invasion of the Emerald Ash Borer (Agrilus planipennis). The three most abundant invasive tree species were European buckthorn (R. cathartica), Norway maple (A. platanoides), and Siberian elm (Ulmus pumila) (Nowak et al., 2013). Abundance was based on the highest percentage of total leaf area (Table 1). All trees were sampled for isoprene emission capacity from May to August of 2016.
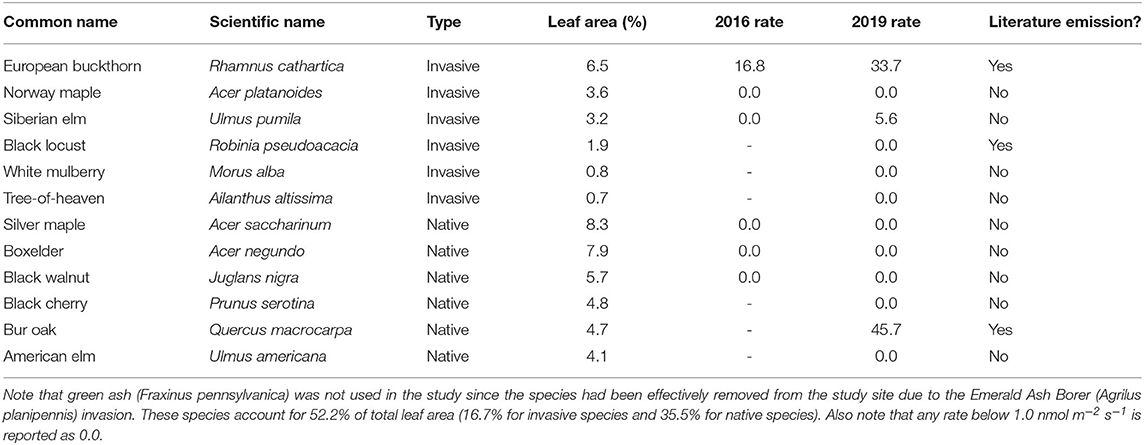
Table 1. The type (Invasive or Native), the percent of total regional leaf area [derived from Nowak et al. (2013)], the mean of the measured isoprene emission rate (nmol m−2 s−1, n = 2 for 2016 and n = 3 for 2019) and the reported presence of isoprene emission from the literature (see text for details) for the tree species used in the experiment.
Two individual trees from each species were located and marked using GPS. A total of at least four isoprene emission samples were collected for each species: two samples from each individual (see Supplementary Table 1). Samples were taken during daylight hours (10:00–16:00 LT) in the field when the average air temperature range was between 23 and 32°C. A LI-6400 leaf-gas exchange system (Licor, Lincoln, NE, USA) was used to measure photosynthesis and control environmental conditions within the leaf cuvette. The cuvette controlled air flow (400 μmol s−1), leaf temperature (30°C), CO2 concentration (400 ppm) and light (PAR = 1,000 μmol m−2 s−1). Photosynthesis rates are not reported but were monitored to ensure the leaf was actively photosynthesizing. Three measurement steps were conducted for each leaf: two with full light exposure and one without light. Isoprene emission is light dependent, and the dark sample was used to ensure that no isoprene was coming from the equipment or incoming air. Sufficient time (15 min) was taken before the dark measurement to avoid the post-illumination burst of isoprene (Li and Sharkey, 2013).
The isoprene was collected by diverting a portion of the air leaving the leaf cuvette through a solid absorbent cartridge (Supelco, Bellefonte, PA USA TENAX) with a portable pump (SKC Inc., Eighty Four, PA, USA Pocket Pump 210-1002, 150 mL min−1) over 5 min for a total of 750 ml. Sample cartridges were kept cool to minimize desorption of isoprene during transportation before analysis. Analysis of the cartridges was done using thermal desorption and a gas chromatograph with a flame ionization detector (GC-FID, model 8610, SRI Instruments, Torrance, CA, USA). Chromatographs produced by the GC-FID identified isoprene by the elution time. The elution time of isoprene and the calibration were determined with a dilution system and a gas-phase standard (Scott-Marrin, Riverside, CA, USA). After averaging the results across the two samples from each species, each species was considered as the experimental unit. A two sample t-test assuming unequal variances was used to analyze the results (n = 3, the number of invasive and native species) and additionally a non-parametric Wilcox test was also employed.
To confirm that European buckthorn was an isoprene emitter, five cuttings were collected Sep 22 2018 from a site near (~5 km distant) LaBagh Woods (North Park Nature Center, 41.9845° N, 87.7252° W) and brought to the laboratory for analysis. Branches 30 – 50 cm in length were cut and the cut end was quickly inserted into water. The branches were brought into the laboratory and placed under an artificial light. The same Licor gas exchange system and gas chromatograph were used for this laboratory analysis as were used in the field. Instead of collecting the isoprene sample onto a portable cartridge, the sample was transferred from the Licor system directly onto the fixed trap of the GC using PTFA tubing and a vacuum pump. The same settings for the leaf gas exchange system were used, except leaf temperature was set to 25°C, because the ambient air temperature was too low to use 30°C. Emission rates at 30°C where then modeled with the Guenther et al. (1993) algorithm.
To improve the representativeness of our study, the number of both native and invasive species measured was doubled (Table 1). Three additional invasive species (black locust, Robinia pseudoacacia; White mulberry, Morus alba; Tree-of-heaven, Ailanthus altissima) and three more native species (Black cherry, Prunus serotine; Bur oak, Quercus macrocarpa; American elm, Ulmus Americana) were measured and the same six species from summer 2016 were measured again. These 12 species accounted for over half the leaf area in the Chicago region (Nowak et al., 2013). The protocol was similar to above, with the following changes. First, only one leaf per tree was measured, but three individual trees were sampled for each species (n = 3 with no pseudoreplication, see Supplementary Table 1). Second, flexible sampling bags (SamplePro, model 236–001, SKC Inc., Eighty Four, PA) were used to bring collected air from the field to the laboratory for measurement with the same gas chromatograph system described above. The sampling bags were filled with ~1 l of air exiting the Licor gas-exchange system. The bags were analyzed within 36 h of collection. A laboratory analysis of the effect of storage time on isoprene concentration was performed (Supplementary Figure 1), and no sample degradation was observed.
Modeling Edge Effects of European Buckthorn, H1b
A visual quick-screening method was used to estimate the relative amount of European buckthorn leaf area present in forest edges. Three separate edges were surveyed: a tree grove, a bike trail and a parking lot (Figure 1, top). Each survey location represented a different type of edge landscape that is commonly found in urban forests. The grove was a circular patch of forest within a park area consisting of turf grass (247 m in length). The bike path was a straight path bordered by forest (181 m in length). The parking lot had a partially forested perimeter, including a connecting road (217 m in length). The estimates of European buckthorn height and abundance were performed every 3 m along the edge (p = perimeter, i = 1 to p/3). Two one-meter sticks placed end to end were used to visually estimate the height of the highest European buckthorn (hi). If the European buckthorn was higher than 2.5 meters that was not considered edge effect but instead as part of the forest canopy (if hi > 2.5, then hi = 2.5). Then the percentage of European buckthorn leaf cover to that estimated height was visually determined (fi). To calculate the area of edge covered by European buckthorn, the percentage of European buckthorn cover was multiplied by the height of the highest European buckthorn at each survey point (see equation below). The point result was multiplied by the 3-m length of the survey interval and summed for each segment. These areas of edge were associated with the segment compass headings for modeling edge isoprene emissions.
Using a methodology similar to Duhl et al. (2012), vegetation cover was assessed visually with Google Earth imagery. The edges sampled by the surveys were combined with an analysis performed with Google Earth Pro (version 7.2) to estimate the total amount of edge European buckthorn leaf area in LaBagh Woods. The line, path and polygon features of the Google Earth ruler tool were employed. Each sample survey was visually broken up into line segments using Google Earth Pro to find the compass heading (East of North) and length of each line segment. The path feature was used to create a continuous shape from smaller line segments and the total length was compared to each sample survey length to ensure consistency. To determine the total length of edges of wooded areas that were not sampled, the polygon feature was used to calculate wooded area and its perimeter. Google Earth Pro imaginary was used to visually classify the wooded area with understory (Figure 1, bottom). In-person observations were necessary to determine if forest patches had understory edges, since some tree patches had no understory (Figure 2). The wooded area polygons were summed to find the total wooded area with understory. The total area of LaBagh Woods was determined using one polygon. The total forested area was divided by the total area to scale ecosystem isoprene emissions, which assumed a continuous forest canopy. In addition, an edge scale factor was calculated from the ratio of total edge of wooded area with understory (sum of perimeters of wooded polygons) to the measured edge from the surveys.
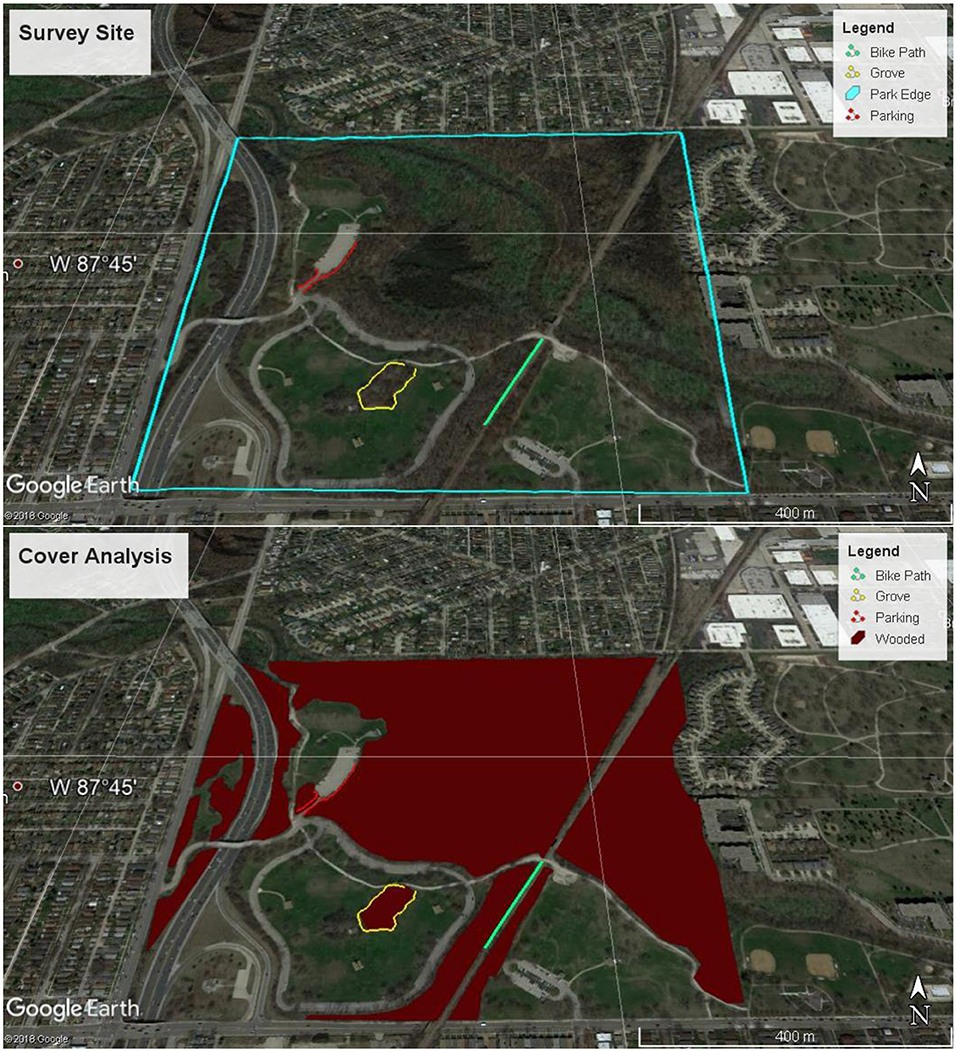
Figure 1. The three locations sampled in LaBagh Woods during summer 2017 and the study boundary (top) and the classified wooded area with an understory at LaBagh Woods (bottom).
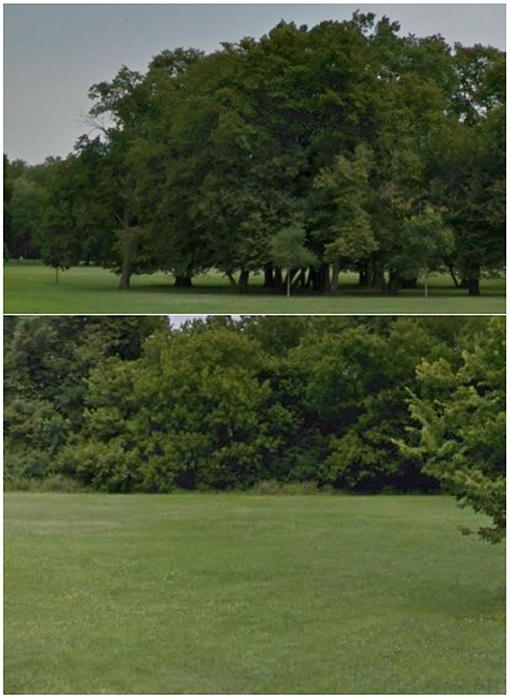
Figure 2. Both images show the difference between trees with and without an understory. The top image shows a wooded area with no understory. The bottom image shows a wooded area with an understory.
In order to model isoprene emissions from the edge segments, the geometric relationship between the face of each edge segment and the sun was calculated hourly. First, the angle solar elevation angle (altitude) and azimuth data (East of North) were determined hourly for June 20, 2017 (U.S. Naval Observatory, 2008). For each line segment of the edge surveys, the sunlit hours of the day for each Google Earth line segment were calculated by comparing the heading from Google Earth Pro and the solar azimuth angle (Table 2). If the solar azimuth was within 90° of the angle perpendicular to the compass heading of the edge segment, the edge was considered sunlit. For each sunlit hour, the sin of the angle (α) between the face of the edge of the sun was calculated for input into the MEGAN model. If the sun was directly perpendicular to the face of the edge, the angle was set to convention at 90° and the sine would be 1 (fully exposed). Also, the face of the edge was assume to be raked back at an angle of 30° (vertical considered 0°). The sine of α was computed by converting the solar azimuth and elevation angles and the edge heading and rake angle into x,y,z vectors of length 1 and using:
Isoprene emissions from both forested areas and the edge segments were estimated with MEGAN (Model of Emissions of Gases and Aerosols from Nature version 2.1, Guenther et al., 2012). On an hourly time step, MEGAN uses meteorological data (solar radiation, air temperature, humidity and wind speed) and information about the canopy (plant functional type, vegetation fraction cover and leaf area index) to calculate isoprene emissions on a per area basis. Because isoprene emissions depend strongly on light and leaf temperature, MEGAN uses a canopy radiation model with layers that requires the solar elevation angle. By default, this is calculated from latitude, day of year and time of day. For the edges, MEGAN was modified to use directly the computed sin(α). The plant function type was set to “broadleaf deciduous temperate tree,” the vegetated fraction cover set to 1 and the leaf area index (LAI) to 3. Urban LAI can vary widely. A nearby US city had LAI values between 0 and 8 (Hardin and Jensen, 2007). Mid-latitude forests typical have LAI values in the range of 3–5 (e.g., Zhao et al., 2011). An LAI of 3 was selected since the forest preserve had a continuous canopy. Because of the relatively limited number of samples collected, we used the emission capacities provided by MEGAN instead of calculating one from the samples. The emission factor for the selected plant functional type was adjusted according to the mix of isoprene-emitting and non-isoprene-emitting species reported by the USDA inventory (Nowak et al., 2013). The inventory for the most common species covered 49.6% of the total leaf area. Of the 49.6%, only 8.4% were from a genus (Quercus) that are expected to emit isoprene. Therefore, the emission factor for broadleaf deciduous temperature trees was scaled by 0.17 (8.4%/49.6%). This assumed that the other ~50% of leaf area had the same mix of emitting and non-emitting genera. Meteorological information for a warm (max air temperature 32.4°C), sunny (max incoming shortwave radiation 414 W m−2) day (Jun 28, 2005) was selected from the historical record at a nearby Ameriflux site [Fermi National Accelerator Laboratory, Batavia (Prairie site), (Matamala, 2017)].
First, total isoprene emissions from the wooded areas of LaBagh Woods for each hour of 1 day were estimated by running MEGAN in its standard mode and then scaling the result by the total wooded area determined from the Google Earth analysis. Next, edge emissions were estimated by running MEGAN for the same day multiple times: once per each line segment of each edge survey. The only change in MEGAN for the edge survey runs was using the calculated sin(α) values calculated for each edge line segment. These runs were scaled up according to the area of European buckthorn determined from the visual surveys. The results were summed across all the line segments from the three surveys. Finally, this result was scaled by the ratio of total edge length from the Google Earth analysis divided by the surveyed edge length. This produced hourly estimates of isoprene emission rates for 1 day from both the wooded areas and the edges.
Results
Invasive vs. Native Species Emission Capacity, H1a
There was no statistical difference between the mean basal isoprene emission rates for measured native and invasive species (Table 1) using either the t-test or the Wilcox test. None of the three native trees emitted significant amounts of isoprene. European buckthorn, the most common invasive species, was a strong emitter while the other two invasive species did not emit isoprene at significant amounts (Table 1). So while hypothesis H1a is rejected due to a lack of statistical significance via the a priori test, it should be noted that European buckthorn was the only significant emitter measured in the summer 2016 experiment. Isoprene emission from European buckthorn was confirmed with the cut-branch experiment conducted during 2018 and again during the summer 2019 experiment. The average of the measured rate from the cut branch experiment was similar (12 nmol m−2 s−1, not shown in Table 1) to the 2016 field result (16.8), unadjusted for measurement leaf temperature. Employing the widely used algorithm from Guenther et al. (1993), the laboratory rate average was 21.9 nmol m−2 s−1.
The results from 2019 largely confirm the results from 2016. For invasive species, there is again no emission from Norway maple, but a relatively low emission rate was detected in 2019 for Siberian elm. European buckthorn again exhibited a relatively high emission rate, but the absolute rate was approximately twice the value in 2019 compared to 2016. None of the three additional invasive species measured in 2019 had detectable emission rates. For three native tree species measured in both years, there were no detected emissions either year. For the three additional native species measured in 2019, there were relatively high emission rates observed for the oak. Considered statistically, neither parametric (t-test) nor non-parametric (Wilcox test) revealed any significant (p < 0.05) difference between native and invasive species using the expanded set of 12 species. Like any experiment that fails to reject the null hypothesis, there is the possibility that a true difference existed and we lacked the statistical power to detect it (false negative or Type II error). Also, isoprene emission rates are highly variable from leaf to leaf and from plant to plant, so our estimates of the mean isoprene emission rate per species have a degree of uncertainty.
Modeling Edge Effects of European Buckthorn, H1b
LaBagh Woods has an estimated total area of 65 ha. Total wooded area determined from the Google Earth survey was 37 ha (Figure 1, bottom), which yielded a forested area fraction of 56%. The maximum rate of hourly isoprene emission from the wooded area of all of LaBagh Woods was 420 g isoprene hour−1. Note that this forested fraction includes wooded areas with no understory (Figure 2). The average European buckthorn coverage was 71% for the parking lot, 50% for the grove and 52% for the bike trail. Table 2 summarizes the total area of European buckthorn, segment heading, hours sunlit and average of the sine of the incident angle for each survey segment of the three transects. The total perimeter of wooded area also gleaned from the Google Earth survey was 7,190 m. The three surveys totaled 645 m in length, so that 9.0% of the wooded perimeter was surveyed. Modeled isoprene emissions across all the segments was summed and scaled by 11 (1/9.0%). The maximum rate of emission from the edges was 52 g isoprene hour−1. Figure 3 compares the diurnal cycle for wooded and edge isoprene emissions from LaBagh Woods. The percentage of isoprene emitted by the edges was 8.1% compared to wooded emissions summed across all daylight hours and varied between 6 and 12% on an hourly basis.
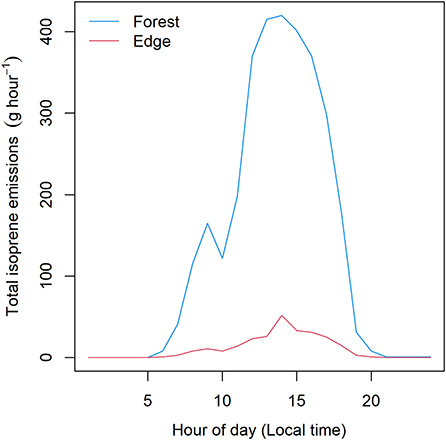
Figure 3. Contributions of edge effect (red line) and forested (blue line) area isoprene emission from the LaBagh Woods study area.
Discussion
Invasive vs. Native Species Emission Capacity, H1a
Leaf- and branch-level measurements of isoprene emission have been conducted for decades and the results from this study can be compared to literature values. The potential for isoprene emission is a trait that typically is expressed at the genus level (Harley et al., 1999). Of the 12 species and nine genera measured in 2019, only three species, each from a different genus, exhibited a significant rate of isoprene emission: European buckthorn (Rhamnus), bur oak (Quercus) and Siberian Elm (Ulmus). For 10 of the 12 species considered in this study, the results are consistent with the literature at the genus level. Previous measurements of the genera Acer, Alianthus, Juglans, Moras, Prunus, and Ulmas revealed very low or negligible isoprene emissions while previous measurements of Robinia, Rhamnus and Quercus found relatively high emission rates (Evans et al., 1982; Lamb et al., 1985; Arey et al., 1991a,b; Hewitt and Street, 1992; Winer et al., 1992; Guenther et al., 1994; Harley et al., 1996; Steinbrecher, 1997; Klinger et al., 2002).
The current study found two contradictions vs. these expectations from the literature: emission from Siberian elm and the lack of emission from black locust (Table 1). In addition to Ulmus being typically noted as a non-emitting genus in the literature, the 2019 result for Siberian elm (Ulmus pumila) is not consistent with (1) a previous literature measurement at the species level (Klinger et al., 2002), (2) the 2016 field measurements nor (3) the other Ulmus species measured in this study, Ulmus americana. We cannot rule out a species misidentification. Interesting, there is one observation that slippery elm (Ulmus rubra) emits isoprene (Guenther et al., 1996), but even this measurement was in contrast to an earlier measurement that found no emission (Guenther et al., 1994). In addition, slippery elm is common in this location (Klimas et al., 2016 for a nearby site and personal communication with C. Klimas that slippery elm is common at LaBagh Woods). This raises the possibility that the 2019 species identification of Siberian elm was incorrect and that slippery elm is an isoprene-emitting species under at least some circumstances. Given the inconsistency with the literature and our own measurements, we are not confident that Ulmus pumila is an isoprene-emitting species. The lack of emission observed for black locust (Robinia pseudoacacia) could be due to tree- and leaf-specific conditions that can lower isoprene emission rates. For example, the capacity to emit isoprene can lag photosynthesis development in leaves and reduced light levels suppress isoprene emission (Harley et al., 1994).
There was no significant difference between the isoprene emission rates from the six native and six invasive species, with tree species as the experimental unit. Leaving aside Ulmus pumila, the results found one emitting species for both the six native and six invasive species. Therefore, there is no evidence to support H1a. But the high observed emission rate from European buckthorn has implications for current and future air quality. Any increase in the relative abundance of European buckthorn through the process of invasion directly increases the leaf area of isoprene-emitting tree species and hence will lead to increases in whole-system isoprene emissions from urban forests.
Some of the projected increases in isoprene emission that result from European buckthorn invasion could be compensated by oak decline. While bur oak is only the fifth-most abundant tree in the Chicago region by leaf area, in the Midwest oaks are a dominant native tree species and are high isoprene emitters (Geron et al., 2001; Schulte et al., 2011). In North American forest and woodlands, oak populations are on the decline. Invasive plants like European buckthorn are competing with oak saplings for soil nutrients, resources and light (Schulte et al., 2011). Invasive plant species have been shown to have a long-term negative impact on oak regeneration (Williams et al., 2006). Despite an abundant population of established oak trees in the over story, oak saplings compete with invasive European buckthorn in the understory (Wyckoff et al., 2012). The European buckthorn plants also may alter the soil conditions which suppresses the regeneration of other species (Heneghan et al., 2006) and the shaded dense shrub layer impacts the establishment of oak saplings (Fagan and Peart, 2004). Because in some cases European buckthorn shrubs are replacing oak trees, the oak decline could offset the increase in European buckthorn since they are both strong isoprene emitters. But there are two reasons that European buckthorn invasion is currently increasing isoprene emissions from this ecosystem. First, while oak regeneration is impeded, the effect on canopy oak area is delayed due to the long growth cycle of oak trees. Second, growth form is important. While there eventually may be compensation in total isoprene-emitting leaf area between oak decline and European buckthorn invasion, currently the additional European buckthorn leaf area is concentrated at forest edges. As the modeling demonstrates, this placement in a high light environment leads to considerable isoprene emissions.
The abundance and spread of European buckthorn is projected to negatively impact future air quality because of interactions with climate change. Because invasive plant species have a wider range of tolerance, climate change will favor the regeneration and growth of invasive plants (Dukes et al., 2009). Warmer temperatures are also predicted to increase invasive plant diversity (Schulte et al., 2011). In addition to these effects on species composition, warmer temperatures directly drive more isoprene emissions (Sharkey and Monson, 2014). These two factors would combine to increase isoprene emissions from urban forests and worsen air quality as the climate warms.
Modeling Edge Effects of European Buckthorn, H1b
The modeling results support H1b. Because European buckthorn can dominate forest edges, its invasion into an urban landscape can increase isoprene emissions by a greater amount than predicted from a simple increase in isoprene-emitting leaf area. Our survey results support the previous observations discussed in the Introduction: European buckthorn was very common along the forest edges found in LaBagh Woods. Across the three surveys, European buckthorn was observed to be >50% of edge cover. This supports previous literature that European buckthorn invasion occurs along edges (Schulte et al., 2011). Further, the edge location of European buckthorn promotes high leaf-level isoprene emission rates, due to direct sunlight exposure. Sunlit leaves can have greatly elevated leaf temperatures if there is insufficient cooling by transpiration. Because of the sharp response of isoprene emission to temperature, this leads to high emission rates, for example under drought stress (Potosnak et al., 2014). Modeling the impact of edge-located European buckthorn increased whole-system emissions by 8%. While the effect on air quality will depend on local conditions of atmospheric chemistry (Papiez et al., 2009), this can lead to negative human health impacts through the production of ground-level ozone. This links the management of invasive species in urban forests to improvements in air quality.
Google Earth can be a useful tool for assessing canopy cover in urban areas (Duhl et al., 2012). Also, using Google Earth to determine the forested area of LaBagh Woods had some of the same promise as using Google Street View to determine urban greenery (Li et al., 2015). But there are some necessary cautions. While it is relatively easy to distinguish tree canopies from turf grass visually, there was no way to distinguish tree canopy areas with and without understory plants and in particular edges with European buckthorn (Figure 2). An in-person assessment of the forest preserve was necessary to make this distinction, but Google Street View is also helpful for this task, if available.
Management Implications
In urban forests like LaBagh Woods, forested areas are dominated by slowing growing climax tree species surrounded by edges created by fragmentation. While features like roadways, bike trails, and paved recreation paths may reduce soil compaction and provide recreational access, they create more edges. These forest edges promote the invasion of European buckthorn and increase whole-system isoprene emissions. This leads to one of two potential strategies to reduce European buckthorn invasion. The first is eliminating edges and the second is directly removing the plant. While reducing edge area can be challenging in established urban forests, creating a transitional ecosystem that provides a border between the edge and the forest can reduce invasion. Both of these strategies can reduce the abundance of European buckthorn and lower isoprene emissions. Importantly, efforts to remove European buckthorn would not only help restore native species but would also improve air quality.
Invasive Species and Isoprene Emissions
European buckthorn, kudzu, and phragmites are all major invasive species in the United States and are also isoprene emitters. This is similar to the finding noted above that pioneer species have a tendency to emit isoprene. We speculate that isoprene emission confers a competitive advantage to these invasive species.
Data Availability Statement
The original contributions presented in the study are included in the article/Supplementary Material, further inquiries can be directed to the corresponding author/s.
Author Contributions
AM, AS, and MP contributed to all field measurements. AM and MP jointly wrote the paper. MP performed the isoprene emission modeling with MEGAN and is the academic advisor for AM. All authors contributed to the article and approved the submitted version.
Conflict of Interest
The authors declare that the research was conducted in the absence of any commercial or financial relationships that could be construed as a potential conflict of interest.
Acknowledgments
The authors would like to thank DePaul University for internal funding sources for this research. The authors would like to thank the Cook County Forest Preserve for permission to perform field research at LaBagh Woods. The authors also acknowledge the contributions of Christine Wiedinmyer for creating a database of isoprene emission enclosure measurements. Portions of this work first appeared in DePaul Discoveries. This is a departmental requirement for the undergraduate senior thesis in Environmental Science.
Supplementary Material
The Supplementary Material for this article can be found online at: https://www.frontiersin.org/articles/10.3389/ffgc.2020.601678/full#supplementary-material
References
Arey, J., Winer, A. M., Atkinson, R., Aschmann, S. M., Long, W. D., and Morrison, C. L. (1991a). The emission of (z)-3-hexen-1-ol; (z)-3-hexenylacetate and other oxygenated hydrocarbons from agricultural plant species. Atmos. Environ. 25A, 1063–1075. doi: 10.1016/0960-1686(91)90148-Z
Arey, J., Winer, A. M., Atkinson, R., Aschmann, S. M., Long, W. D., Morrison, C. L., et al. (1991b). Terpenes emitted from agricultural species found in California's central valley. J. Geophys. Res. Atmos. 96, 9329–9336. doi: 10.1029/91JD00447
Brothers, T. S., and Spingarn, A. (1992). Forest fragmentation and alien plant invasion of central indiana old-growth forests. Conserv. Biol. 6, 91–100. doi: 10.1046/j.1523-1739.1992.610091.x
Chameides, W., Lindsay, R., Richardson, J., and Kiang, C. (1988). The role of biogenic hydrocarbons in urban photochemical smog: Atlanta as a case study. Science 241, 1473–1475. doi: 10.1126/science.3420404
Dantas de Paul, M., Groeneveld, J., and Huth, A. (2016). The extent of edge effects in fragmented landscapes: insights from satellite measurements of tree cover. Ecol. Indic. 69, 196–204. doi: 10.1016/j.ecolind.2016.04.018
Duhl, T. R., Guenther, A., and Helmig, D. (2012). Estimating urban vegetation cover fraction using google earth® images. J. Land Use Sci. 7, 311–329. doi: 10.1080/1747423X.2011.587207
Dukes, J. S., Pontius, J., Orwig, D., Garnas, J. R., Rodgers, V. L., Brazee, N., et al. (2009). Responses of insect pests, pathogens and invasive plant species to climate change in the forests of northeastern North America: what can we predict? NRC Res. Press. 39, 231–248. doi: 10.1139/X08-171
Endicott, S., Drescher, M., and Brenning, A. (2017). Modelling the spread of European buckthorn in the region of waterloo. Biol. Invasions 19, 2993–3011. doi: 10.1007/s10530-017-1504-3
Evans, R. C., Tingey, D. T., Gumpertz, M., and Burns, W. F. (1982). Estimates of isoprene emissions rates in plants. Bot. Gaz. 143, 301–310. doi: 10.1086/botanicalgazette.143.3.2474826
Fagan, M. E., and Peart, D. R. (2004). Impact of the invasive shrub glossy buckthorn (Rhamnus frangula L.) on juvenile recruitment by canopy trees. For. Ecol. Manage. 193, 95–107. doi: 10.1016/j.foreco.2004.02.015
Flanagan, N. E., Richardson, C. J., and Ho, M. (2015). Connecting differential response of native and invasive riparian plants to climate change and environmental alteration. Ecol. Appl. 25, 753–767. doi: 10.1890/14-0767.1
Forseth, I. N., and Innis, A. F. (2004). Kudzu (Pueraria montana): history, physiology, and ecology combine to make a major ecosystem threat. CRC. Crit. Rev. Plant Sci. 23, 401–413. doi: 10.1080/07352680490505150
Geron, C., Harley, P., and Guenther, A. (2001). Isoprene emission capacity for US tree species. Atmos. Environ. 35, 3341–3352. doi: 10.1016/S1352-2310(00)00407-6
Guenther, A., Greenberg, J., Harley, P., Helmig, D., Klinger, L., Vierling, L., et al. (1996). Leaf, branch, stand and landscape scale measurements of volatile organic compound fluxes from U.S. woodlands. Tree Physiol. 16, 17–24. doi: 10.1093/treephys/16.1-2.17
Guenther, A., Zimmerman, P., and Wildermuth, M. (1994). Natural volatile organic-compound emission rate estimates for united-states woodland landscapes. Atmos. Environ. 28, 1197–1210. doi: 10.1016/1352-2310(94)90297-6
Guenther, A. B., Jiang, X., Heald, C., L., Sakulyanontvittaya, T., Duhl, T., et al. (2012). The model of emissions of gases and aerosols from nature version 2.1 (MEGAN2.1): an extended and updated framework for modeling biogenic emissions. Geosci. Model Dev. 5, 1471–1492. doi: 10.5194/gmd-5-1471-2012
Guenther, A. B., Zimmerman, P. R., Harley, P. C., Monson, R., K., and Fall, R. (1993). Isoprene and monoterpene emission rate variability—model evaluations and sensitivity analyses. J. Geophys. Res. Atmos. 98, 12609–12617. doi: 10.1029/93JD00527
Hardin, P. J., and Jensen, R. R. (2007). The effect of urban leaf area on summertime urban surface kinetic temperatures: a Terre Haute case study. Urban For. Urban Green. 6, 63–72. doi: 10.1016/j.ufug.2007.01.005
Harley, P., Deem, G., Flint, S., and Caldwell, M. (1996). Effects of growth under elevated UV-B on photosynthesis and isoprene emission in Quercus gambelii and Mucuna pruriens. Glob. Chang. Biol. 2, 149–154. doi: 10.1111/j.1365-2486.1996.tb00060.x
Harley, P. C., Litvak, M. E., Sharkey, T. D., and Monson, R. K. (1994). Isoprene emission from velvet bean leaves—interactions among nitrogen availability, growth photon flux-density, and leaf development. Plant Physiol. 105, 279–285. doi: 10.1104/pp.105.1.279
Harley, P. C., Monson, R. K, and Lerdau, M. T. (1999). Ecological and evolutionary aspects of isoprene emission from plants. Oecologia. 118, 109–123. doi: 10.1007/s004420050709
Heneghan, L., Fatemi, F., Umek, L., Grady, K., Fagen, K., and Workman, M. (2006). The invasive shrub European buckthorn (Rhamnus cathartica, L.) alters soil properties in Midwestern U.S. woodlands. Appl. Soil Ecol. 32, 142–148. doi: 10.1016/j.apsoil.2005.03.009
Hewitt, C. N., and Street, R. A. (1992). A qualitative assessment of the emission of nonmethane hydrocarbon compounds from the biosphere to the atmosphere in the UK—present knowledge and uncertainties. Atmos. Environ. A Gen. Topics 26, 3069–3077. doi: 10.1016/0960-1686(92)90463-U
Jardine, K. J., Jardine, A. B., Souza, V., Carneiro, V., Ceron, J., Gimenez, B., et al. (2016). Methanol and isoprene emissions from the fast growing tropical pioneer species Vismia Guianensis (aubl.) pers. (hypericaceae) in the central amazon forest. Atmos. Chem. Phys. 16, 6441–6452. doi: 10.5194/acp-16-6441-2016
Klimas, C., Williams, A., Hoff, M., Lawrence, B., Thompson, J., and Montgomery, J. (2016). Sustainability 8:853. doi: 10.3390/su8090853
Klinger, L. F., Greenburg, J., Guenther, A., Tyndall, G., Zimmerman, P., M'Bangui, M., et al. (1998). Patterns in volatile organic compound emissions along a savanna-rainforest gradient in Central Africa. J. Geophys. Res. Atmos. 103, 1443–1454. doi: 10.1029/97JD02928
Klinger, L. F., Li, Q.-J., Guenther, A. B., Greenberg, J. P., Baker, B., and Bai, J.-H. (2002). Assessment of volatile organic compound emissions from ecosystems of China. J. Geophys. Res. 107:4603. doi: 10.1029/2001JD001076
Lamb, B., Westberg, H., and Allwine, G. (1985). Biogenic hydrocarbon emissions from deciduous and coniferous trees in the United States. J. Geophys. Res. 90, 2380–2390. doi: 10.1029/JD090iD01p02380
Lee, J. D., Lewis, A. C., Monks, P. S., Jacob, M., Hamilton, J. F., Hopkins, J. R., et al. (2006). Ozone photochemistry and elevated isoprene during the UK heatwave of august 2003. Atmos. Environ. 40, 7598–7613. doi: 10.1016/j.atmosenv.2006.06.057
Li, X., Zhang, C., Li, W., Ricard, R., Meng, Q., and Zhang, W. (2015). Assessing street-level urban greenery using Google Street View and a modified green view index. Urban For. Urban Green. 14, 675–685. doi: 10.1016/j.ufug.2015.06.006
Li, Z., and Sharkey, T. D. (2013). Metabolic profiling of the methylerythritol phosphate pathway reveals the source of post-illumination isoprene burst from leaves. Plant Cell Environ. 36, 429–437. doi: 10.1111/j.1365-3040.2012.02584.x
Martin, P. H., and Guenther, A. B. (1995). Insights into the dynamics of forest succession and non-methane hydrocarbon trace gas emissions. J. Biogeogr. 22, 493–499. doi: 10.2307/2845946
Matamala, R. (2017). AmeriFlux US-IB2 Fermi National Accelerator Laboratory- Batavia (Prairie site). doi: 10.17190/AMF/1246066
Nowak, D. J., Hoehn, R. E. III, Bodine, A. R., Crane, D. E., Dwyer, J. F., Bonnewell, V., et al. (2013). Urban Trees and Forests of the Chicago Region. Newton Square: U.S. Forest Service. doi: 10.2737/NRS-RB-84
Papiez, M. R., Potosnak, M. J., Goliff, W. S., Guenter, A. B., Matsunaga, S. N., and Stockell, W. R. (2009). The impacts of reactive terpene emissions from plants on air quality in Las Vegas, Nevada. Atmos. Environ. 43, 4109–4123. doi: 10.1016/j.atmosenv.2009.05.048
Pauchard, A., and Alaback, P. B. (2006). Edge type defines alien plant species invasions along Pinus contorta burned, highway and clearcut forest edges. For. Ecol. Manage 223, 327–335. doi: 10.1016/j.foreco.2005.11.020
Penuelas, J., and Staudt, M. (2010). BVOCs and global change. Trends Plant Sci. 15, 133–144. doi: 10.1016/j.tplants.2009.12.005
Potosnak, M. J., LeStourgeon, L., Pallardy, S. G., Hosman, K. P., Gu, L., Karl, T., et al. (2014). Observed and modeled ecosystem isoprene fluxes from an oak-dominated temperate forest and the influence of drought stress. Atmos. Environ. 84, 314–322. doi: 10.1016/j.atmosenv.2013.11.055
Schulte, L. A., Mottl, E. C., and Palik, B. J. (2011). The association of two invasive shrubs, common buckthorn (Rhamnus cathartica) and Tartarian honeysuckle (Lonicera tatarica), with oak communities in the midwestern United States. Can. J. For. Res. 41, 1981–1992. doi: 10.1139/x11-112
Sharkey, T. D., and Monson, R. K. (2014). The future of isoprene emission from leaves, canopies and landscapes. Plant Cell Environ. 37, 1727–1740. doi: 10.1111/pce.12289
Sharkey, T. D., and Monson, R. K. (2017). Isoprene research – 60 years later, the biology is still enigmatic. Plant Cell Environ. 40, 1671–1678. doi: 10.1111/pce.12930
Sharkey, T. D., and Singsaas, E. L. (1995). Why plants emit isoprene. Nature 374, 769–769. doi: 10.1038/374769a0
Sheppard, C. S., and Stanley, M. C. (2014). Does elevated temperature and doubled CO2 increase growth of three potentially invasive plants? Invasive Plant Sci. Manag. 7, 237–246. doi: 10.1614/IPSM-D-13-00038.1
Steinbrecher, R. (1997). “Isoprene: production by plants and ecosystem-level estimates” in Biogenic Volatile Organic Compounds in the Atmosphere. eds G. Helas Slanina, J. Steinbrecher, and R. Kugler (Amsterdam: Academic Publishing), 101–114.
U.S. Naval Observatory (2008). Astronomical Applications Dept. Available online at: https://www.esrl.noaa.gov/gmd/grad/solcalc/azel.html (accessed December 15, 2020).
Velikova, V., Edreva, A., and Loreto, F. (2004). Endogenous isoprene protects Phragmites australis leaves against singlet oxygen. Physiol. Plant 122, 219–225. doi: 10.1111/j.0031-9317.2004.00392.x
Vickers, C., Possell, M., Cojocariu, C., Velikova, V., Laothawornkitkul, J., Ryan, A., et al. (2009). Isoprene synthesis protects transgenic tobacco plants from oxidative stress. Plant Cell Environ. 32, 520–531. doi: 10.1111/j.1365-3040.2009.01946.x
Vila, M., Espinar, J. L., Hejda, M., Hulme, P. E., Jarosik, V., Maron, J. L., et al. (2011). Ecological impacts of invasive alien plants: a meta-analysis of their effects on species, communities and ecosystems Ecol. Lett. 14, 702–708. doi: 10.1111/j.1461-0248.2011.01628.x
Whitmore, T. C. (1989). Canopy gaps and the two major groups of forest trees. Ecology 70, 536–538. doi: 10.2307/1940195
Williams, K., Westrick, L. J., and Williams, B. J. (2006). Effects of blackberry (Rubus discolor) invasion oak population dynamics in a California savanna. For. Ecol. Manag. 228, 187–196. doi: 10.1016/j.foreco.2006.03.002
Winer, A. M., Arey, J., Atkinson, R., Aschmann, S. M., Long, W. D., Morrison, C. L., et al. (1992). Emission rates of organics from vegetation in California's central valley. Atmos. Environ. 26A, 2647–2659. doi: 10.1016/0960-1686(92)90116-3
Wyckoff, P. H., Greiman, R., Krueger, A., and Logan, L. (2012). Forest dynamics at Minnesota's prairie-forest border driven by invasive buckthorn (Rhamnus cathartica) and native bur oak (Quercus macrocarpa). J. Torrey Bot. Soc. 139, 311–322. doi: 10.3159/TORREY-D-11-00051.1
Yates, E. D., Levia, D. F., and Williams, C. L. (2004). Recruitment of three non-native invasive plants into a fragmented forest in Southern illinois. For. Ecol. Manage. 190, 119–130. doi: 10.1016/j.foreco.2003.11.008
Keywords: isoprene, invasive species, biogenic volatile organic compound emissions, urban forests, edge effects
Citation: Mistry AP, Steffeck AWT and Potosnak MJ (2021) Edge Growth Form of European Buckthorn Increases Isoprene Emissions From Urban Forests. Front. For. Glob. Change 3:601678. doi: 10.3389/ffgc.2020.601678
Received: 01 September 2020; Accepted: 08 December 2020;
Published: 06 January 2021.
Edited by:
Iolanda Filella, Consejo Superior de Investigaciones Científicas (CSIC), SpainReviewed by:
Miguel Portillo-Estrada, University of Antwerp, BelgiumRita Baraldi, Italian National Research Council, Italy
Copyright © 2021 Mistry, Steffeck and Potosnak. This is an open-access article distributed under the terms of the Creative Commons Attribution License (CC BY). The use, distribution or reproduction in other forums is permitted, provided the original author(s) and the copyright owner(s) are credited and that the original publication in this journal is cited, in accordance with accepted academic practice. No use, distribution or reproduction is permitted which does not comply with these terms.
*Correspondence: Mark J. Potosnak, bWFyay5wb3Rvc25ha0BkZXBhdWwuZWR1