- Department of Renewable Resources, University of Alberta, Edmonton, AB, Canada
Drought stress associated with warm temperatures is causing increased mortality and reduced growth of trees in drier portions of the boreal forests of western Canada with both warming and drought expected to increase over the coming decades. While thinning is often shown to reduce drought stress, there is little information on its effects in stands comprised of mixtures of trembling aspen and white spruce that are common in the forests of this region. This study examined effects of pre-commercial thinning on aspen and spruce growth, response to drought stress, and stand dynamics for two study sites located in Saskatchewan, Canada. In unthinned plots aspen densities declined from initial densities of 40,000 to 200,000 trees ha−1 to 2,639 trees ha−1 at age 26. Twenty-one years after thinning (i.e., at age 26) diameter of aspen and spruce had increased, with largest trees being found at the lowest aspen densities (200 aspen ha−1 for aspen and 0 aspen ha−1 for spruce). Aspen density affected average height but not height of aspen top height trees. Spruce height decreased significantly with increasing aspen density. Crown width and live crown ratio of both aspen and spruce declined with increasing aspen density. Data from cores collected from aspen and spruce indicate significant positive effects of tree size (basal area at beginning of the year), and CMI (Climate Moisture Index) on basal area increment of both aspen and spruce while increasing aspen basal area (m2ha−1) had negative effects on aspen and spruce growth. Increasing tree size (basal area) and aspen competition (basal area ha−1) both had negative effects on drought resistance and resilience for both aspen and spruce. Yield projections provided by the Mixedwood Growth Model (MGM) suggest that the mixture of 1,500 aspen ha−1 and 1,000 spruce ha−1 has the potential to provide a 23% increase in total mean annual increment (MAI) with a single harvest at age 100, compared to unthinned aspen stands, but with a 44% reduction in spruce MAI compared to pure spruce stands. Thinning of aspen to densities below 4,000 trees ha−1 at age 5 resulted in reduced aspen yields but increased spruce yields.
Introduction
Mixtures of trembling aspen (Populus tremuloides Michx.) and white spruce (Picea glauca (Moench) Voss) are a common natural stand type on upland sites in the western boreal forests of Canada. In this region mixedwood stands represent a range of early- to mid-seral successional stages (Chen and Popadiouk, 2002) and develop most commonly on mesic and subhygric sites (Lieffers et al., 1996). When aspen and white spruce regenerate at approximately the same time following disturbance, aspen will form an overstory above spruce for at least 50 to 60 years (Chen and Popadiouk, 2002; Bergeron et al., 2014). After that time, white spruce begins to grow through the canopy and aspen decreases in dominance and basal area. In the absence of disturbances such as fire or harvesting the mixedwood stand may become a spruce-dominated stand over the ensuing 100 to 200 years (Chen and Popadiouk, 2002; Bergeron et al., 2014). When there are seed trees nearby white spruce may also establish naturally in the understory of mature aspen stands and grow up through the aspen in a similar manner (Kabzems and Garcia, 2004).
Since mixedwoods contribute to improved species and structural diversity, wildlife habitat, and visual quality (Macdonald et al., 2010) managing these stands as mixedwood rather than as pure white spruce or aspen stands is desirable. Overstory aspen is also a nurse crop for young spruce through reducing the incidence of growing season frost, by providing protection from environmental extremes (Filipescu and Comeau, 2011), reducing the vigor of competing vegetation such as bluejoint reedgrass (Calamagrostis canadensis (Michx.) Beauv.) (Lieffers and Stadt, 1994) and reducing damage by white pine weevil (Pissodes strobi Peck) (Taylor et al., 1996). Mixedwoods can potentially provide greater diversity of products (MacDonald, 1996) and higher total yield than single-species stands (Man and Lieffers, 1999; Kabzems et al., 2016).
Historically, mixedwood stands have developed through natural regeneration of both species following fire or other severe disturbances. However, for much of the past four decades white spruce have been planted to regenerate spruce and mixedwood stands following harvesting while aspen is regenerated naturally. Following harvesting of upland mixedwood stands, aspen regenerates vigorously from root sprouts and dominates during early stages of stand development. When conditions are ideal, aspen can regenerate to very high densities, sometimes exceeding 100,000 trees ha−1 at age 2 (Steneker, 1976; Bella, 1986; Frey et al., 2003). However, self-thinning generally results in dramatic reductions in aspen densities during the first 20 years (Peterson and Peterson, 1992; Bokalo et al., 2007).
Early juvenile spacing of aspen can accelerate the growth of residual trees (Bickerstaff, 1946; Steneker, 1976; Perala, 1978; Bokalo et al., 2007; Kabzems et al., 2016), accelerating achievement of merchantable diameter and improving the quality of the stand. Rice et al. (2001) found that, thinning of young aspen (5–15 years old) did not significantly change merchantable stand volume 15 years after treatment but did result in significant increases in diameter of the remaining trees. Reducing aspen densities also increases growth of spruce in mixed stands (Bokalo et al., 2007; Kabzems et al., 2016).
Recent periods of drought and large fires have highlighted potential effects of warm periods and long-term warming trends in Canada's western boreal region. While periodic shifts in temperature, precipitation, and drought have occurred historically, mean annual temperature has increased by up to 3.0°C between 1901 and 2000 (Price et al., 2013). Projections of future climate for the boreal plains of western Canada suggest continuing increases in temperature with increases in winter temperatures of up to 6.5°C and with increases in spring, summer and fall temperatures of up to 4.5°C between 1990 and 2100 (Price et al., 2013). Both growing season and annual precipitation are projected to continue to increase slightly over this period, however increased evapotranspiration is expected to result in reduced soil moisture. While temperature increases and longer growing seasons may be beneficial to forest productivity in Canada's western boreal forest, increasing drought could lead to reductions in growth and to reductions in forest cover in drier portions of the region (Peng et al., 2011; Price et al., 2013; Worrall et al., 2013). In addition, damage by insects such as forest tent caterpillar (Malacosoma disstria Hbn.) (Chen et al., 2017b; Cortini and Comeau, 2020), mountain pine beetle (Dendrochtonus ponderosae Hopkins) (Vore et al., 2020) and spruce beetle (Dendroctonus rufipennis Kirby) (Campbell et al., 2019) are expected to increase as a consequence of increases in both temperature and drought stress. Frequency and magnitude of fire damage (Vore et al., 2020) is also expected to increase due to warming and drought.
The southern extent of stands with more than 60% white spruce content in north America is near the 18°C July temperature isotherm (Burns and Honkala, 1990), suggesting that contraction in spruce distribution may occur in the boreal plains where projected July temperatures may reach 18.5 to 19.8 in 2100 (Price et al., 2013), as also reported by Gray and Hamann (2013). Hogg et al. (2017) report reductions in growth of white spruce in Saskatchewan and Alberta associated with severe drought in 2001–2003. Chen et al. (2017c) also indicate that temperature induced drought is the main climatic factor influencing growth of white spruce in central and northern Alberta. However, competition can also have significant effects on white spruce growth. Alam et al. (2017) report that growth of white spruce in this area is constrained largely by competition with minor but significant effects of drought while Cortini et al. (2012) indicate that competition from aspen can constrain potential benefits of increasing temperature on spruce growth.
Northward and elevational shifts in aspen habitat are expected under climate change (Gray and Hamann, 2013) with declines in aspen associated with severe drought being evident, particularly in marginal habitats (Worrall et al., 2013). Decreases in growth and increases in mortality of aspen have been reported in central Alberta and Saskatchewan associated with severe and prolonged drought events (Hogg et al., 2005, 2008; Chen et al., 2017a) most notably the 2001–2003 drought (Michaelian et al., 2011). Kweon and Comeau (2017) also report that increasing summer dryness appears to be associated with a decrease in the maximum densities that can be carried by aspen stands.
Applying thinning treatments to reduce stand density may reduce drought stress and increase resistance and resilience of stands to drought (Spittlehouse and Stewart, 2003; D'Amato et al., 2013; Keenan, 2015; Sohn et al., 2016; Ammer, 2017; Bottero et al., 2017; del Rio et al., 2017; Holofsky et al., 2018; Andrews et al., 2020; Steckel et al., 2020) through reducing transpiration and rainfall interception and through increasing size of root systems of remaining trees. In aspen stands, thinning has been shown to reduce impacts of drought stress (Bell et al., 2014) and could also increase moisture availability to white spruce in mixed stands. Thinning also accelerates development of stands to merchantable sizes which could provide timber that could be harvested in advance of or salvaged immediately following mortality events.
While growing mixtures can improve ecosystem resistance, recovery and resilience and lead to better growth in response to drought and temperature stresses, outcomes depend on species, site, level of stress, and other factors (Ammer, 2017). Stand density may influence complementary effects, with facilitative effects being weak at very low densities and competitive effects dominating at very high densities (Forrester, 2014). The degree to which facilitation or reductions in intraspecific competition occur in mixtures determine whether mixtures lead to improved ability of stands to deal with drought (Forrester, 2015; Ammer, 2017). Consequently, there are cases where mixed stands may be negatively influenced by drought as well as cases where they are less affected by drought with this resulting from the combined effects of species identity, stand composition, stand structure, stand density, differences in species functional/structural traits, tree size, tree age, soil and site factors, both historical and current climate, drought intensity and drought frequency (Ammer, 2017). There is a lack of published quantitative information on effects of thinning on the dynamics of young aspen-spruce mixtures in the western Canadian boreal forests, including information on how thinning influences the growth of components species and there is little information on how thinning of these mixed stands may influence responses to increasing temperature and drought stress.
In this paper effects of pre-commercial thinning treatments applied at age 5 on aspen and spruce diameter and height, basal area and stand density are examined based on measurements collected at age 26 at two sites located ~30 km north of the town of Big River, Saskatchewan, Canada. In addition, effects of climate, tree size and aspen basal area on annual basal area growth and on resistance, resilience and recovery of the aspen and spruce to drought events in 2003 and 2013 are examined. Working hypotheses are: (1) height and diameter of aspen and white spruce will decline with increasing aspen density; (2) aspen and spruce growth will increase with increases in tree size and climate moisture index (CMI) and decline with increases in aspen density; (3) resistance, recovery and resilience will be increased by thinning, but with resistance, recovery and resilience of spruce being highest when spruce are grown with a low to moderate density of aspen; and, (4) stand yield estimated using the Mixedwood Growth Model (MGM) (assuming no effects of climate change) will be highest in mixed stands with moderate densities of aspen and white spruce while spruce yield will be highest without aspen.
Methods
Study Sites and Study Design
The Western Boreal Growth and Yield (WESBOGY) [now the WESBOGY project of the Forest Growth Organization of Western Canada (FGrOW)] Long Term Study was initiated by the Western Boreal Growth and Yield Association in 1990 to advance knowledge of the dynamics of mixedwood stands and effects of aspen density on spruce and aspen growth (Bokalo et al., 2007). The study involved planting white spruce seedlings in recently clearcut areas where aspen regeneration had already been established. Aspen were thinned to create six densities [0, 200, 500, 1,500, and 4,000 trees ha−1 plus unthinned (natural)] at age 5. Spruce were established at 1,000 and 2,000 trees ha−1 and thinned at age 5 to 500 and 1,000 trees ha−1, respectively in each of six plots in each replicate. Three additional pure aspen treatments with densities of 1,500 and 4,000 trees ha−1 and unthinned were also established. Each installation consists of two replications of the 15 treatments. The study currently includes a total of 615 plots with 20 installations established and maintained in Alberta, British Columbia, Manitoba, Saskatchewan and the Northwest Territories.
The Big River, Saskatchewan installations used in this study were established by Weyerhaeuser Canada in 1992 ~30 km north of the town of Big River, Saskatchewan. The Median (54.09°N 107.07°W, elev. 515 m) and Superior (54.05°N 106.98°W, elev. 505 m) installations were harvested in June of 1992, with aspen allowed to regenerate naturally by root suckering and with spruce planted (at double the final treatment density) in September of 1992. Thinning to treatment target densities was completed in September of 1996. Both installations were level, with moderately well-drained Gray Luvisolic soils and with mesic to subhygric soil moisture regimes.
Data Collection
Measurement of the Median installation was completed during August 2018 and measurement of the Superior was completed during May 2019. Measurement plots are 20 × 20m (0.04 ha) with a 5-m-wide treated buffer on each side of the measurement plot. In thinned plots all spruce and aspen within the 20 × 20 m measurement plot were measured (diameter at breast height (1.3 m) (DBH), height, height to base of live crown, and crown radius). In plots where aspen were not thinned, spruce were measured in the 20 × 20 m plot while aspen were measured in four 5 × 5 m subplots located within the main plot as well as in previously established 2 × 2 m subplots nested within the 5 × 5 m subplots. In unthinned plots previous aspen measurements had used 1 × 1 m (to age 5), and 2 × 2 m (ages 6–23) subplots.
Dendrochronology
Cores were collected at 1.3 m height from two spruce and two aspen in the treated buffer of 13 plots (cores were not collected in plots where aspen density was reduced to 200 trees ha−1) using a 5 mm diameter increment corer. Healthy trees in the tallest size classes in each plot for each species were selected for coring. Cores were scanned and measured using WinDendro software (Regent Instruments Inc., Quebec) and a calibrated EPSON Perfection V700 Photo scanner. Cross dating was visually performed for trees in each plot and across plots guided by wide and narrow rings. Due to the young age and good vigor of the cored trees there were no missing rings and there was no evidence of white rings associated with insect damage in this area. DBH of each sampled tree was measured in the field and previous years tree basal area and basal area increment were calculated based on annual ring widths.
Meteorological Data
Climate data [mean annual temperature, growing degree days above 5C and climate moisture index (CMI)] were obtained from ClimateNA version 6.31 (Wang et al., 2016) which extracts and downscales gridded monthly climate data for North America.
CMI, calculated as the difference between monthly precipitation and monthly evapotranspiration, was used as a measure of drought stress. CMI was calculated based on methods presented by Hogg et al. (2013) using the Penman-Monteith method to calculate evapotranspiration with the exception that CMI calculated by ClimateNA version 6.31 for each calendar year was used. All analysis used CMI averaged over a 3-year period (current year plus the previous 2 years) in order to account for lags associated with water storage in the root zone (Hogg et al., 2005). A 3 year period was used in this study following results from preliminary analysis which indicated that this resulted in better description of growth variation than using the 4 year period suggested by Hogg et al. (2005).
Data Analysis
Mixed models were used to examine effects of prescribed aspen and spruce density on DBH, quadratic mean diameter (QMD), height, slenderness, crown width and live crown ratio at age 26 for this randomized complete block design, using SAS9.4 proc Mixed. Installation, replicate and plot were included as nested random effects in the mixed models.
Annual basal area increment from 1998 to 2018 was calculated using the tree ring data obtained for individual spruce and aspen. A linear mixed model, with repeated measures, was used to examine effects of tree size (basal area at the beginning of each year), aspen basal area, and CMI (CMI3 – which was calculated as average CMI for the current year and for 2 preceeding years) using SAS 9.4 proc Mixed. Aspen basal area (determined at the plot level using the measurement closest in time to the growth year and expressed as m2 ha−1) was used as a measure of aspen competition. Plot level spruce basal area could not be included in the models since it had a strong and significant negative linear relationship with aspen basal area (i.e., strong collinearity). Linear and quadratic terms for mean annual temperature (MAT) and CMI (CMI3) and interactions of these climate variables with aspen basal area were included in the initial models. Backward selection was used to select a parsimonious model which included only significant variables. Installation, replicate, plot and tree were included as nested random effects in the mixed models. Denominator degrees of freedom (DDFM) were calculated using the “Kenward-Rogers” option and a heterogenous autoregressive [ARH(1)] covariance structure was applied. Due to strong and significant (p < 0.001) collinearity with CMI3, mean annual temperature and growing degree days were excluded from these models. Since spruce did not reach 2.0 m height in several plots with aspen densities above 1,500 until 2006, only data from 2007 to 2018 were used for model development. Effects of the severe multi-year drought in 2001–2003 created poor model fits for aspen, consequently data for the 2007–2018 period were also used for aspen.
Short-term growth responses of cored aspen and spruce trees to multiyear drought events in 2003 and 2013 were assessed using Lloret et al. (2011) drought response indices.
Resistance (Rt) indicates a trees' ability to maintain growth during a drought event:
Recovery (Rc) represents a trees' ability to recover to a higher level of growth after drought:
Resilience (Rs) indicates a trees' ability to recover to pre-drought levels:
Where:
Gdr = growth during the year;
Gpre = average annual growth during the 3 preceeding years;
Gpost = average annual growth during the 3 subsequent years.
To accommodate nesting of plots within replicates and installations, linear mixed-effects models were used to examine effects of initial tree size and aspen basal area on resistance, recovery and resilience. Denominator degrees of freedom (DDFM) were calculated using the “BETWITHIN” option.
Results
Temporal Trends of Aspen Density in Unthinned Plots
Aspen densities have declined substantially in the unthinned plots from initial values of between 40,000 and 200,000 trees ha−1 (averaging 97,000 trees ha−1) in 1992 (age 0) to values of between 1,400 and 4,500 trees ha−1 (averaging 2,639 trees ha−1 in 2018 (age 26) (Figure 1). Self-thinning was particularly dramatic during the first 12 years with densities at age 12 ranging between 3,125 and 18,750 trees ha−1.
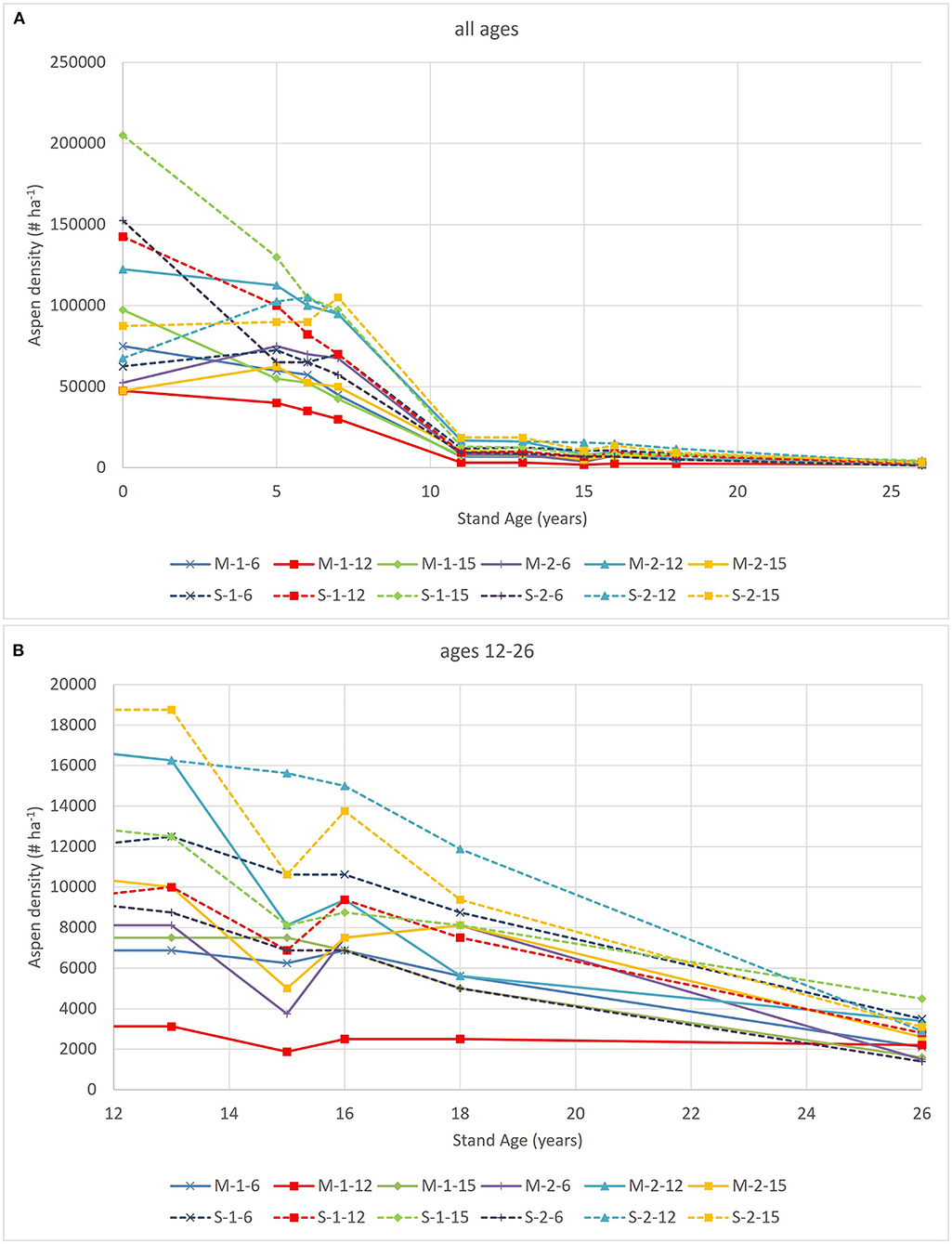
Figure 1. Aspen density trends for the 12 unthinned plots. Panel (A) shows density trends across the full range of ages and panel (B) shows trends from ages 12 to 26. Plot labels refer to installation (M or S for median and superior, respectively), first number refers to replicate (1 or 2) and last number refers to plot number [6 (1,000 spruce ha−1), 12 (500 spruce ha−1) or 15 (0 spruce ha−1)].
Treatment Effects on Densities
While the ranking of treatments in terms of stand densities at age 26 follows treatment order, treatment densities have declined below target densities over the 21 year period following thinning (Table 1). Aspen basal area in the unthinned at age 26 did not differ significantly from basal area in the 4,000 or 1,500 trees ha−1 treatments. Spruce basal area in the treatment with 500 trees ha−1 of spruce was about 40% of that found in the 1,000 spruce trees ha−1 at age 26.
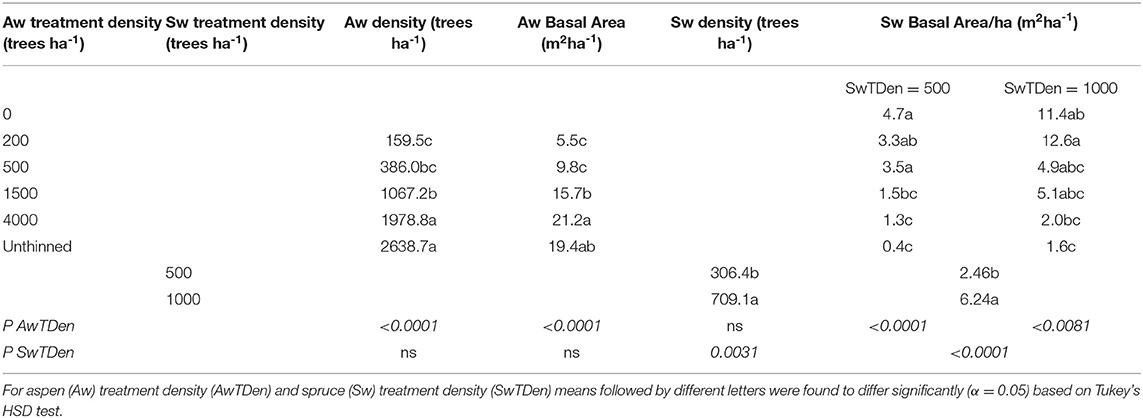
Table 1. Effects of thinning treatments applied at age 5 on stand density and stand basal area at age 26.
Temporal Trends of Aspen and Spruce Size
Figure 2 illustrates trends in aspen and spruce average height and DBH. Thinning of the aspen at age 5 has caused a persistent increase in average aspen height (Figure 2A) over the untreated, although differences between aspen thinning levels are diminishing after age 18. Thinning of the aspen has also resulted in an increase in white spruce height (Figure 2B), with the difference between low aspen densities (0, 200 and 500 trees ha−1) and unthinned increasing over time.
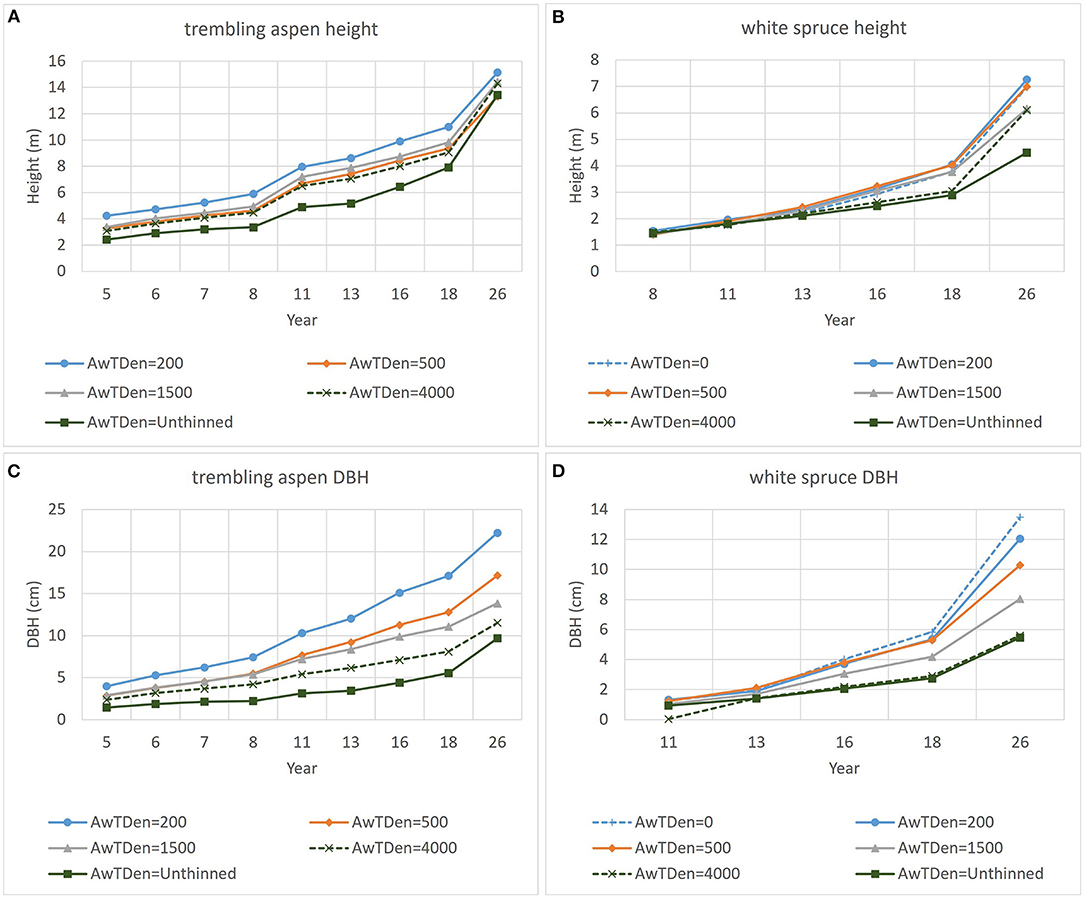
Figure 2. Trends in average height (A,B) and DBH (C,D) for aspen and white spruce for the six aspen densities (AwTDen; values are in trees ha−1).
Average DBH of aspen has consistently decreased with increasing aspen density over the 21 years since thinning (Figure 2C). After age 16, average spruce DBH declined with increasing aspen density, with differentiation between aspen densities increasing with age (Figure 2D).
Treatment Effects on Aspen and Spruce Sizes at Age 26
Analysis of data from age 26 indicate significant (α <0.05) effects of aspen density on average height, DBH, QMD, slenderness (ratio of height: DBH), crown width and live crown ratio (ratio of crown length to height) of aspen (Table 2). Thinning to 200 trees ha−1 resulted in a significant increase in average aspen height over the unthinned, while intermediate densities have intermediate height values. Average DBH of aspen declined with increasing aspen density, with 200 trees ha−1 being larger than 1,500, 4,000, and unthinned, and with 1,500 trees ha−1 being larger than unthinned. QMD shows a similar outcome, although with greater differentiation between individual treatments. Slenderness increased with increasing aspen density with 200, 500 <1,500 <4,000 < unthinned. Crown width decreased with increasing density with 200 trees ha−1 being larger than 1,500, 4,000, and unthinned, and with 1,500 trees ha−1 being larger than unthinned. Live crown ratio decreased with increasing aspen density with 200, 500 > 1,500 > 4,000 >unthinned. To remove the influence of the “chain-saw effect,” where thinning from below causes an increase in average height and diameter due to the removal of small trees, it is common to examine treatment effects for a subsample of dominant trees when analyzing data from thinning experiments. Analysis based on the use of the four top height trees in each plot (Table 2) shows no significant effect of treatment on height of top height trees at age 26. Results from analysis of top height trees for other variables (DBH, slenderness, crown width and live crown ratio) show significant effects of thinning treatments and are similar to results from analysis of overall averages.
Aspen density, but not spruce density, had significant (α <0.05) effects on spruce height, DBH, QMD, Slenderness, crown width and live crown ratio (Table 3). Slenderness increased with increasing aspen density while other variables decreased with increasing aspen density. Although values for height, DBH, crown width and live crown ratio are higher and values for slenderness are lower, similar trends were obtained from analysis using only the four largest DBH spruce in each plot.
Effects of Climate on Basal Area Increment and Interactions With Treatment
Analysis of effects of climate focused on effects of mean annual temperature and climate moisture index (CMI) on basal area increment of cored trees. Variation in key climate variables between 1995 and 2018 is shown in Figure 3. Data for the 2007 to 2017 period included CMI values ranging between −6.36 in 2011 and 12.89 in 2010 and 3 year average CMI (CMI3) values ranging between −1.85 in 2013 and 4.61 in 2010. MAT was tested but found to be non-significant in these models. Tree size (basal area) at the beginning of each year was used as a covariate to account directly for effects of initial tree size on growth and to remove the need for standardization to account for tree size effects. Basal area increment of cored aspen and spruce increased with increasing 3 year average CMI (CMI3) and with increasing size but decreased with increasing aspen basal area ha−1 across the 11 years (2007–2017) included in the analysis presented in Table 4. Figures 4, 5 show growth trends predicted by these models for aspen and spruce.
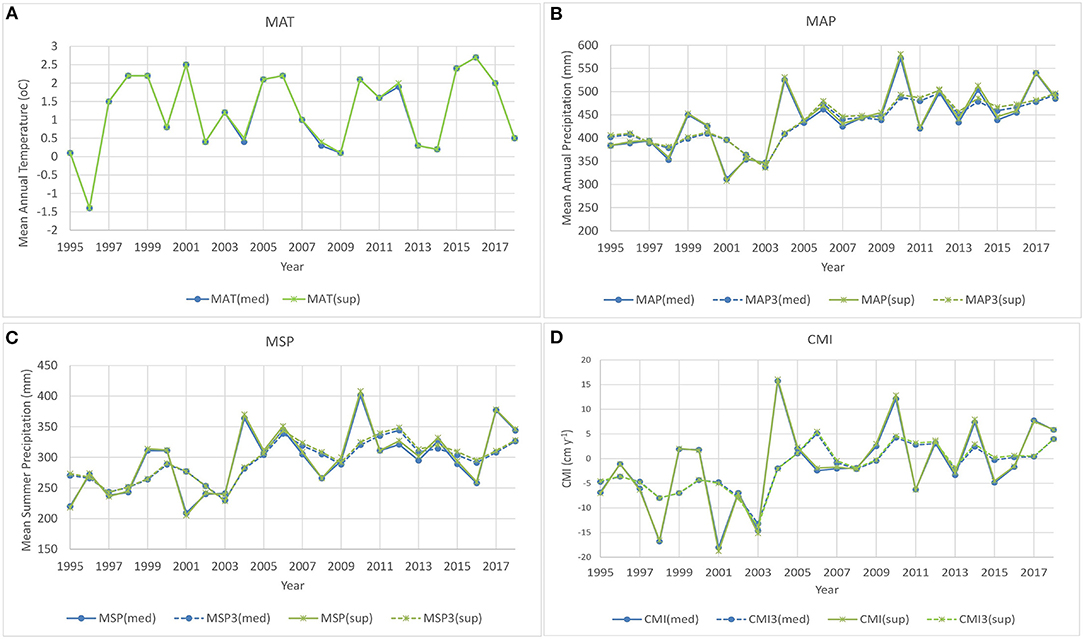
Figure 3. Trends in (A) mean annual temperature (MAT), (B) mean annual precipitation (MAP) and 3 year running average of mean annual precipitation (MAP3), (C) mean summer precipitation (MSP) and 3 year running average of mean summer precipitation (MSP3), and (D) climate moisture index (CMI) and 3 year running average CMI (CMI3) for the two Big River installations (med, median; sup, superior).
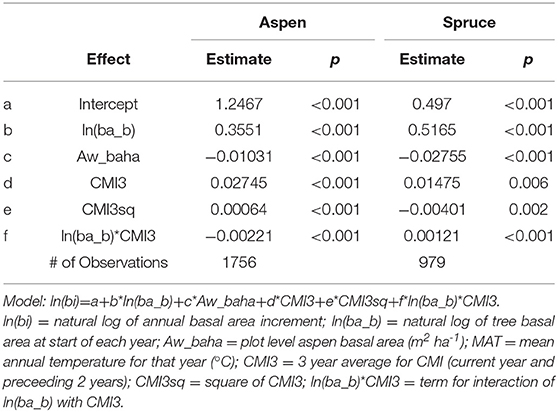
Table 4. Results for models describing variation in annual basal area increment of cored aspen and spruce trees for the 2007–2017 period.
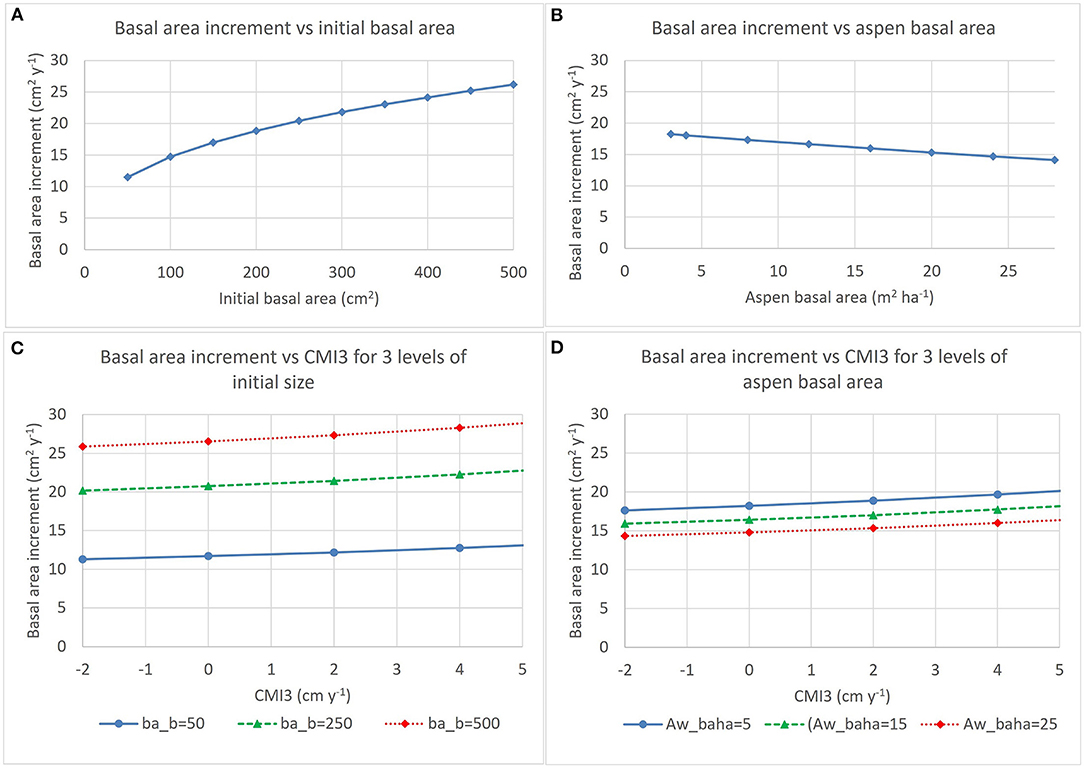
Figure 4. Trends described by the model for annual basal area increment of aspen described in Table 4. Effects of initial basal area and aspen basal area on basal area increment are shown in (A,B), respectively. (C) Shows effects of interactions between CMI3 and initial basal area (ba_b) and (D) shows interaction between CMI3 and aspen basal area (Aw_baha) (for each panel, average values were used for all other variables in the model).
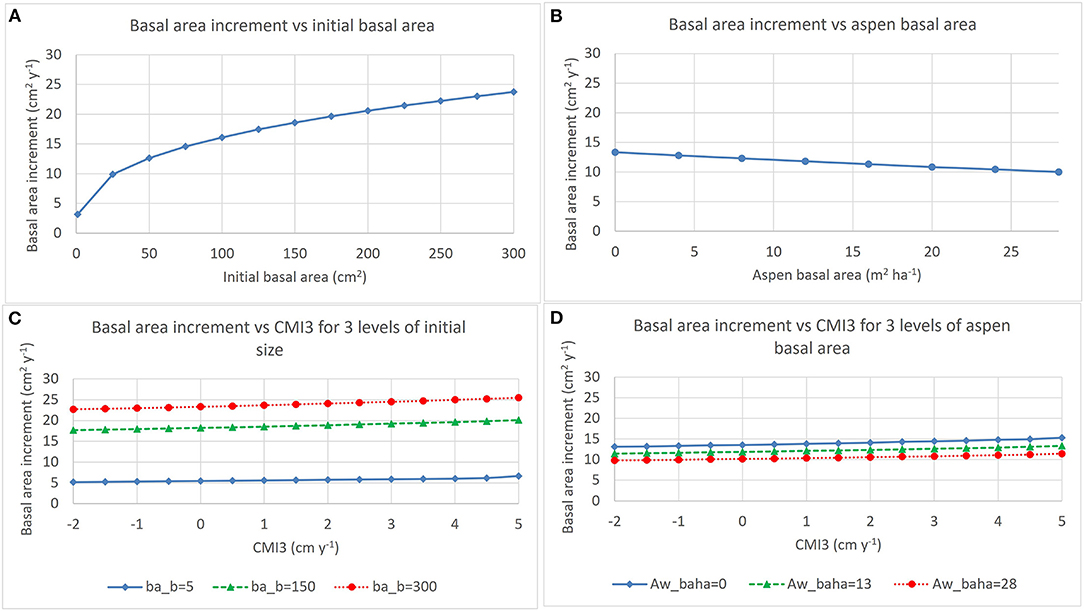
Figure 5. Trends described by the model for annual basal area increment of spruce described in Table 4. Effects of initial basal area and aspen basal area on basal area increment are shown in (A,B), respectively. (C) Shows effects of interactions between CMI3 and initial basal area (ba_b) and (D) shows interaction between CMI3 and aspen basal area (Aw_baha) (for each panel, average values were used for all other variables in the model).
Treatment Effects on Resistance, Recovery and Resilience to Drought
As a result of 3 years of drought, with severe drought in 2001 and 2003, 3 year average CMI (CMI3) for the 2001 to 2003 period was −13.2 for the median installation and −13.7 for the superior installation (Figure 3). A mild drought event associated with low CMI in 2011 and 2013 resulted in 3 year average CMI for 2011-2013 of −2.2 for the median installation and −1.8 for the superior installation. Examination of resistance, recovery and resilience of aspen was conducted for 2003 and 2013.
For the 2003 drought event, aspen resistance was 1.358 at minimum aspen basal area, with tree size and aspen basal area having significant negative effects (Table 5, Figures 6A,B). Aspen recovery was 1.255 and resilience was 1.692 at minimum aspen basal area. While tree size and aspen basal area had significant negative effects on aspen resilience, their effects on recovery were not significant. Figure 6 illustrates the interactions between tree size and aspen density, with resistance dropping below 1.0 when initial tree basal area exceeds 145 cm2 and aspen basal area is 3.28 m2 ha−1, when tree basal area exceeds 100 cm2 and aspen basal area is 17.09 m2 ha−1, and when initial tree basal area exceeds 65 cm2 when aspen basal area is 27.94 m2 ha−1. Resilience remains above 1.0 across the full range of tree sizes when aspen basal area is 3.28 m2 ha−1, but when aspen basal area is 17.09 m2 ha−1 resilience drops below 1.0 when tree basal area exceeds 160 cm2 and when aspen basal area is 27.94 m2ha−1 Rs drops below 1.0 when tree basal area exceeds 140 cm2.
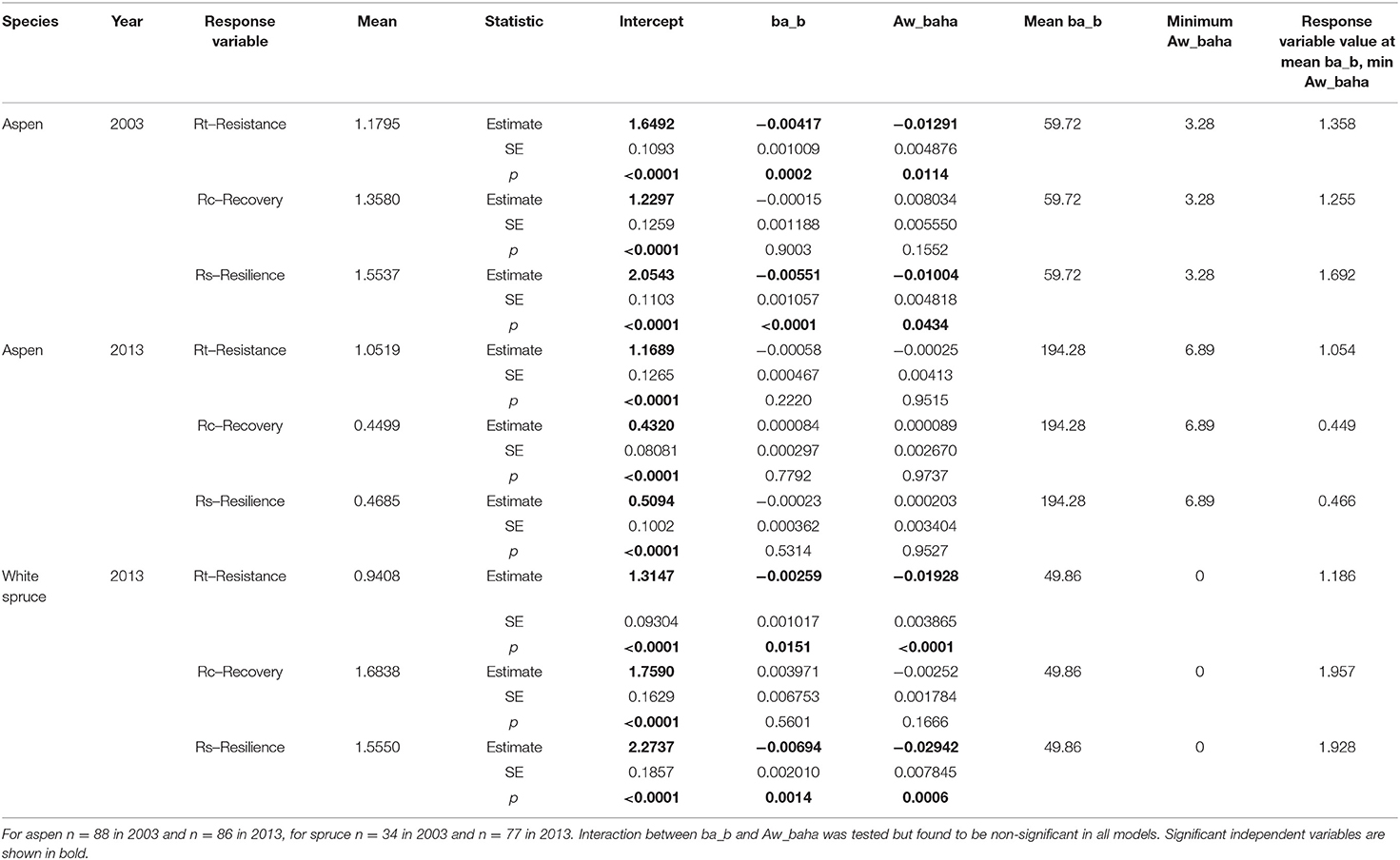
Table 5. Results from linear mixed-effects model examining effects of tree size (basal area) and aspen stand level basal area on tree drought responses in 2003 and 2013 for trembling aspen and white spruce.
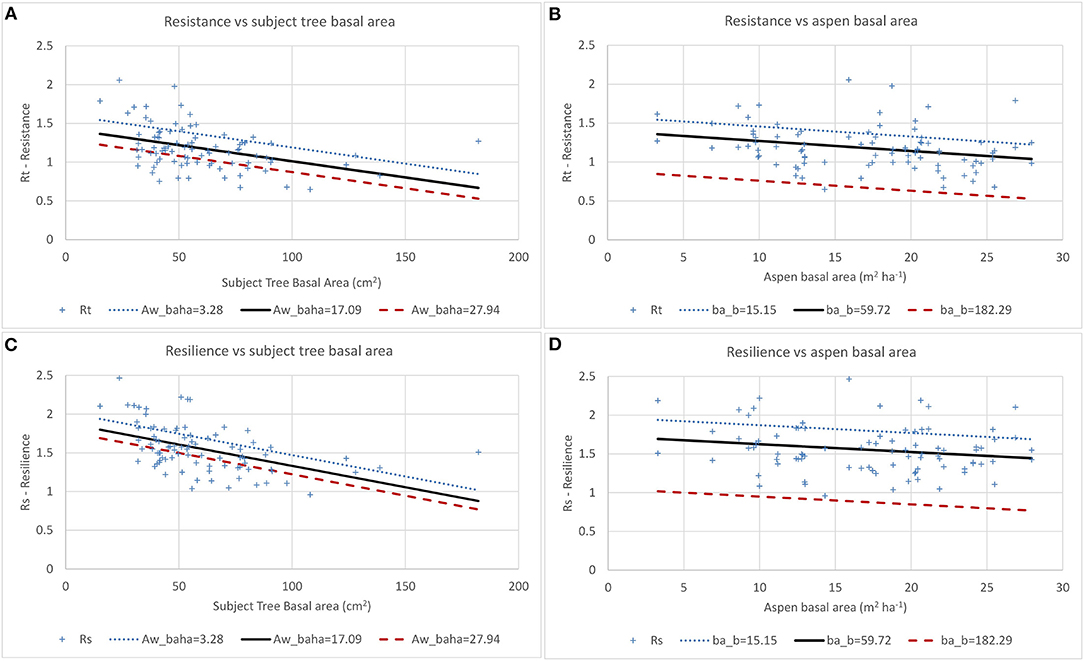
Figure 6. Effects of tree size (A,C) and aspen basal area (B,D) on aspen resistance (Rt) and resilience (Rs) in 2003. + Shows data points and lines show predicted values. Aw_baha = aspen basal area (m2ha−1) and ba_b = initial tree size (m2).
In response to the milder 2013 drought, resistance of aspen was slightly positive but not influenced by aspen basal area or treatments. Recovery and resilience are low (0.449 and 0.466, respectively) which is likely associated with drought stress in 2015 and 2016 (as indicated by low annual CMI and high MAT for these years), but are not influenced by tree size or aspen basal area.
Spruce data were not sufficient to allow analysis for 2003 since <50% of cored spruce had reached 1.3 m height by 2000 and the trees that had passed 1.3 m height by 2003 were growing in plots with very low aspen basal area. Spruce resistance, recovery and resilience to the 2013 drought were all above 1 when aspen basal area was zero (Table 5) with resistance and resilience (but not recovery) showing significant negative effects of tree size and aspen basal area. Interactions between spruce size and aspen density are evident (Figure 7), with resistance dropping below 1.0 when initial tree basal area exceeds 120 cm2 and aspen basal area is 0 m2 ha−1, when spruce basal area exceeds 20 cm2 and aspen basal area is 12.68 m2 ha−1, and is below 1.0 across the full range of spruce sizes when aspen basal area is 27.90 m2 ha−1. Resilience drops below 1.0 when spruce size exceeds 120 cm2 when aspen basal area is 0 m2 ha−1, but when aspen basal area is 12.68 m2 ha−1 Rs drops below 1.0 when spruce basal area exceeds 20 cm2 and when aspen basal area is 27.94 m2 ha−1 Rs drops below 1.0 across the full range of spruce size. The scatter plot (Figures 7B,D) shows points associated with aspen basal areas of between 3 and 8 m2 ha−1 having higher resistance and resilience than points associated with zero aspen basal area which suggests the possibility of some beneficial effects at these low aspen densities. However, a quadratic term was tested in this model but was not significant (p > 0.4).
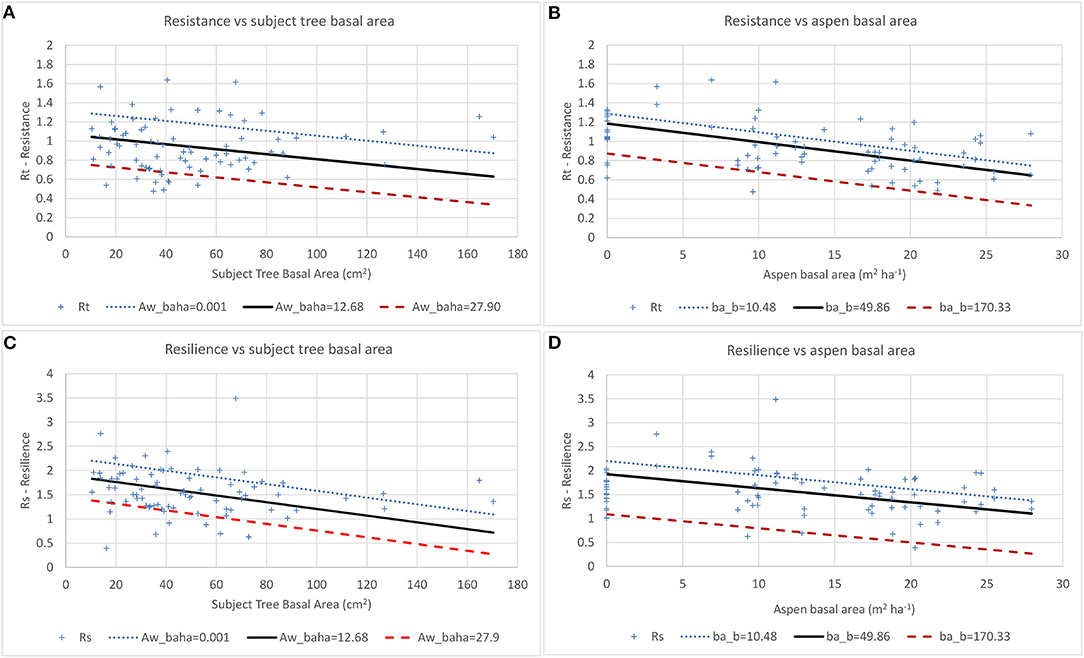
Figure 7. Effects of tree size (A,C) and aspen basal area (B,D) on white spruce resistance (Rt) and resilience (Rs) in 2013. Aw_baha = aspen basal area (m2 ha−1) and ba_b = initial tree size (m2).
MGM Forecasts of Effects of Spruce and Aspen Densities on Yield
The Mixedwood Growth Model (MGM18_VS1_2_18_37_Rev6115, March 2020; https://mgm.ualberta.ca/) was used to examine effects of treatments on growth and yield. MGM was initialized for each plot using treelists that included diameter, height, and tree factor (number of trees ha−1 represented by each tree calculated based on the size of the measurement plot) created from measurements collected in 2018/2019. Site Index was calculated using Alberta Site Index curves (Huang et al., 2009) for each installation based on average spruce top height in replicates of plots 1 and 7 (zero aspen density) and aspen top height in replicates of plots 6, 12, and 15 (natural aspen densities) measured in 2018/2019 (age 26). Site index for the Median installation was calculated to be 17.9 m@age50 for white spruce and 23.1 m@age50 for trembling aspen and site index for the Superior installation was calculated to be 16.8 m@age50 for white spruce and 23.2 m@age50 for trembling aspen.
Figure 8 shows volume-age curves for aspen and spruce in each treatment from age 30 to 150 based on Chapman-Richards curves fit to data from the plot level MGM predictions (Supplementary Figures 1, 2 show volume-age curves for individual plots). Aspen volume increases with increasing aspen density, with the largest aspen volumes being found in plots where aspen were not thinned (plots 6, 12, and 15) or in plots where aspen was thinned to 4,000 trees ha−1 (plots 5, 11, and 14) (Figure 8A, Table 6). At low densities maximum aspen stand volumes are achieved at earlier ages due to accelerated diameter growth of aspen and earlier mortality (DBH is a major driver of aspen mortality in MGM). For plots 2 and 8, where aspen had been thinned to 200 trees ha−1, maximum deciduous volumes of 90 to 105 m3ha−1 occur at ages 50 to 60, while plots where aspen was thinned to 500 trees ha−1 (plots 3 and 9) have maximum deciduous volumes of 175 to 190 m3ha−1 at ages 60 to 70, plots thinned to 1500 aspen ha−1 (plots 4 and 10) have maximum deciduous volumes of 247 to 300 m3ha−1 at age 80, plots thinned to 4,000 aspen ha−1 (plots 5, 11, and 14) reach maximum deciduous volumes of 300 to 330 at age 80 and unthinned (plots 6, 12, and 15) reach maximum deciduous volumes (290 to 325 m3ha−1) at age 90. Spruce volume at any stand age (Figure 8B) declines with both decreasing spruce density and increasing aspen density. With the exception of plot 1 (1000 Sw/0Aw), stand level spruce volumes do not appear to reach a maximum by age 150. Variability in spruce and aspen densities, as well as variation in tree sizes at age 26 (i.e., in the plot data used to initialize MGM) are the primary sources of variation in plot level projections in each treatment.
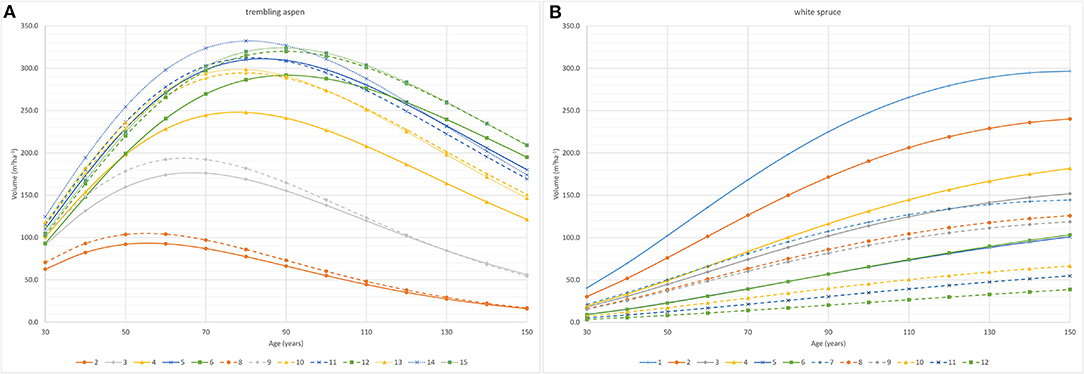
Figure 8. Treatment level volume-age curves for (A) aspen and (B) white spruce based on MGM forecasts of aspen gross stand volumes for each treatment. [Treatments (trees ha−1 at age 5): Plot 1 = 1000Sw/0Aw; plot 2 = 1000Sw/200Aw; plot 3 = 1000Sw/500Aw; plot 4 = 1000Sw/1500Aw; plot 5 = 1000Sw/4000Aw; plot 6 = 1000Sw/unthinned Aw; plot 7 = 500Sw/0Aw; plot8 = 500Sw/200Aw; plot 9 = 500Sw/500Aw; plot 10 = 500Sw/1500Aw; plot 11 = 500Sw/4000Aw; plot 12 = 500Sw/unthinned Aw; plot 13 = 0Sw/1500Aw; plot 14 = 0Sw/4000Aw; plot 15 = 0Aw/unthinned Aw].
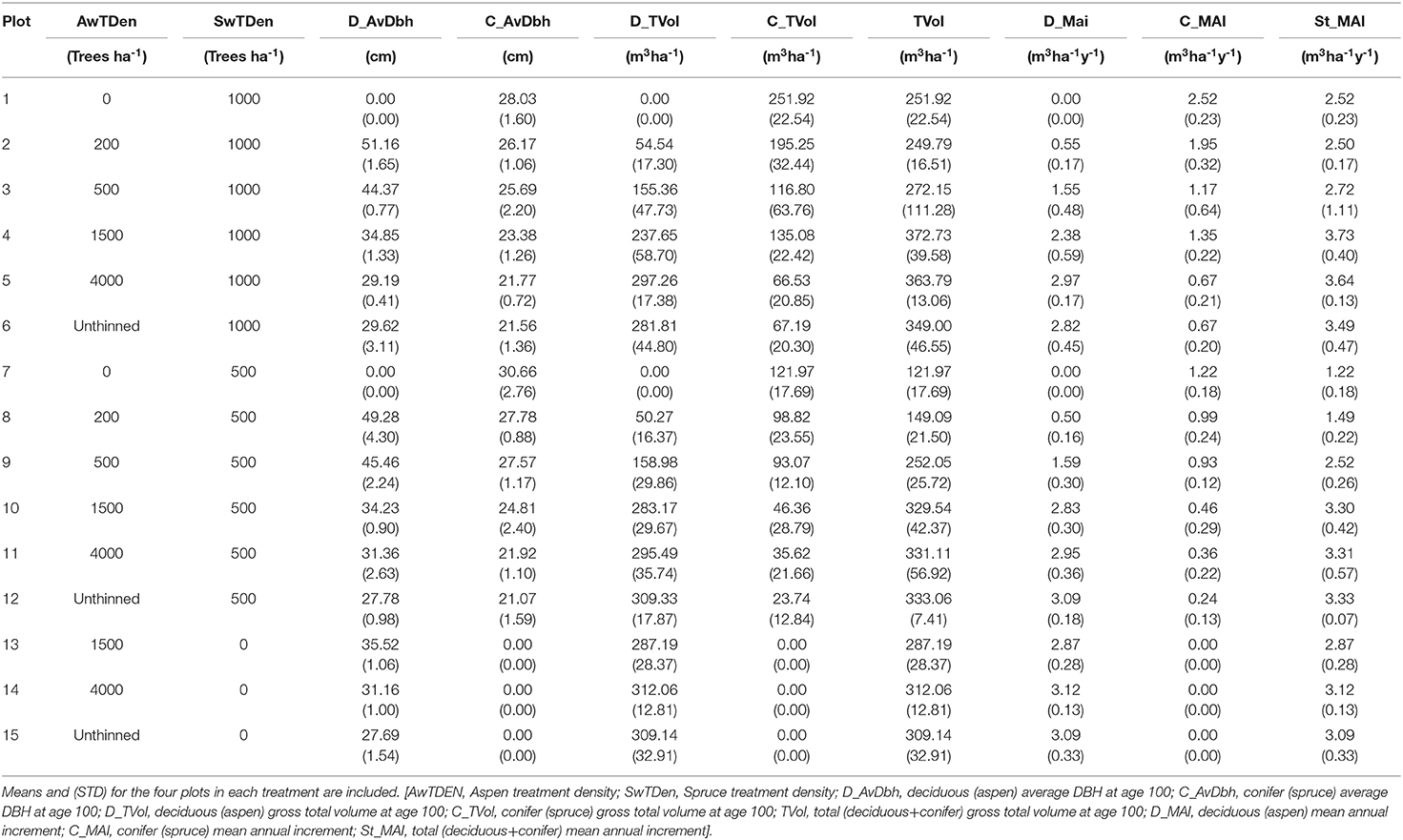
Table 6. MGM forecasts of aspen and spruce diameters, yields and MAI at age 100 for the 15 treatments.
Spruce DBH at age 100, as predicted by MGM declines with increases in either aspen or spruce density (Table 6). For aspen, DBH at age 100 is reduced primarily by aspen density, with the largest diameters being evident in plots 2 and 8 which were both thinned to 200 aspen ha−1 at age 5.
Aspen yields (gross total volume) at age 100 and aspen MAI range up to a maximum of 312 m3ha−1 and 3.1 m3ha−1y−1, respectively. Yield and MAI of aspen generally increase with increases in initial aspen density (Table 6). However, in some cases the 4,000 aspen ha−1 treatment has slightly higher aspen yield and MAI than the unthinned. Spruce yield at age 100 shows effects of both aspen and spruce density and decreases with increasing aspen density while increasing with increased spruce density (Table 6). Spruce MAI was 2.5 m3ha−1 y−1 with 0 aspen and 1,000 spruce (plot 1), 1.2 m3ha−1y−1 with 0 aspen and 500 spruce (plot 7), while with natural aspen densities (unthinned) 1000 spruce (plot 6) had a spruce MAI of 0.7 m3ha−1y−1 and 500 spruce (plot 12) spruce MAI was 0.2 m3ha−1y−1 (Table 6). Total, aspen + spruce, MAI was higher in mixed than in pure stands, with the highest MAI (3.73 m3ha−1y−1) evident in plot 4 with 1,500 aspen and 1,000 spruce ha−1. This contrasts with pure spruce plots, plots 1 and 7, with MAI's of 2.5 and 1.2 m3ha−1y−1, respectively, and with pure aspen plots, plots 14 and 15, with MAI of 3.1 m3ha−1y−1. MAI of these stands is at the higher end of values observed for natural stands and reflects selection of good aspen sites, with good initial stocking for the study as well as planting of spruce.
Culmination age increases with increasing aspen density. In addition, volume at culmination increases with increasing density. Small effects of increasing spruce density on aspen volumes are evident for the two lowest aspen densities (200 and 500 aspen ha−1). Both spruce and aspen densities influence spruce yields, with culmination of spruce occurring later with increasing aspen density.
Discussion
Do Thinning Treatments Influence Aspen and Spruce Growth?
Effects of aspen and spruce densities on aspen and spruce size at age 26 at Big River are consistent with other published studies which show that thinning in young, healthy aspen stands can accelerate the growth of residual trees (Bickerstaff, 1946; Steneker, 1976; Perala, 1978; Peterson and Peterson, 1992; Rice et al., 2001), accelerating achievement of merchantable diameter and improving the quality of the stand. These results support hypothesis 1 (height and diameter of aspen and white spruce decline with increasing aspen density). Height and height growth of aspen were increased by thinning in three of the seven stands that Bella and Yang (1991) studied. In contrast, Penner et al. (2001) found no benefit to thinning a stand with 4000 to 5000 trees ha−1 at age 20, due to the fact that unspaced plots had self-thinned to densities similar to those of thinned plots by age 36. Results for Big River also indicate that substantial self-thinning has occurred in the unthinned plots, with aspen densities in the unthinned plots averaging 2,639 trees ha−1 at age 26.
Positive effects of thinning of aspen on spruce growth (Bokalo et al., 2007; Kabzems et al., 2016) are also evident for Big River. Reducing aspen densities using manual, mechanical, or chemical spacing treatments or removal of aspen around crop conifers, gives consistent improvements in growing conditions for white spruce when aspen has established at high densities.
Both intra- and inter- specific competition have immediate effects on diameter growth of trees, while impacts on height growth do not generally appear until severe competition levels are reached or competition remains at sufficient intensity for a period of time. As a result, the ratio of height to root collar diameter (HDR) or height to DBH (slenderness) increase in response to increases in competition (Bokalo et al., 2007; Kabzems et al., 2016), as also found for the Big River installations. In addition, crown width and live crown ratio are strongly influenced by social status of a tree and by both intra- and inter-specific competition (Groot and Schneider, 2011; Kabzems et al., 2016). At Big River, increases in aspen density led to increases in slenderness and decreases in crown width and live crown ratio of white spruce, with the strongest differences being evident between the 0 aspen and unthinned treatments. Variation in plots where aspen had been thinned reflects variation in degree and duration of competition reduction. As spruce crown closure develops in some treatments, effects of intraspecific competition on crown width and live crown ratio are expected. In addition, self-shading is expected to influence crown width and live-crown ratio as spruce become larger (Kershaw et al., 1990).
How Do Tree Size, Competition and Climate Influence Growth of Spruce and Aspen?
Results from analysis of effects of tree size, aspen basal area, and CMI on annual growth of cored aspen and spruce are consistent with observations from other studies. Similar increases in growth with size, particularly within the range of sizes encountered in these young stands, have been reported by other studies (Comeau et al., 2003; Filipescu and Comeau, 2007). Positive effects of increasing CMI and negative effects of competition on growth of these species in western Canada have also been reported by other studies (Alam et al., 2017; Chen et al., 2017b,c; Jiang et al., 2018). Despite expectations to the contrary, the regression models fit to the annual basal area growth data at these sites highlight competitive effects of aspen on spruce growth over facilitative effects and support hypothesis 2.
Does Thinning Influence Resistance, Recovery and Resilience of These Stands to Drought?
Tree size, species, competition, and magnitude and duration of drought influence resistance, recovery, and resilience of stands to drought (Sohn et al., 2016; Schmitt et al., 2020; Steckel et al., 2020). In this study, conducted in young mixtures of trembling aspen and white spruce, tree size and aspen basal area have negative effects on resistance and resilience of both trembling aspen and white spruce and support hypothesis 3 (resistance, recovery and resilience will be increased by thinning) in relation to resistance and resilience but not recovery. Data were not sufficient to allow detailed analysis of effects of magnitude or duration of drought, although outcomes for aspen differ between the severe 2001–2003 drought and the milder 2011–2013 drought and suggest that aspen may be well-equipped for handling mild short-term drought events on these two sites.
Results indicate that resistance and resilience of spruce was best at low aspen basal areas (3 to 7 m2ha−1) although these could not be shown to be significantly better than at an aspen basal area of 0 m2ha−1. Resistance and resilience of aspen was also highest at the lowest stand basal area and declined with increasing basal area, as reported for other species (e.g., Sohn et al., 2016). Benefits of thinning are still evident 21 years after treatment, due to the long duration of their effects on aspen basal area, consistent with Giuggiola et al. (2013). In their meta-analysis Sohn et al. (2016) found that benefits of thinning decrease with time since thinning, highlighting a need for continuing measurement of these stands to document whether the benefits of thinning decrease. Declining resistance and resilience with increasing tree size has been reported for some, but not all, previous studies (Ammer, 2017; Andrews et al., 2020).
Acceleration of growth and associated increases in tree size and water demand following thinning can result in older thinned stands having lower resistance and resilience than unthinned stands (D'Amato et al., 2013) which could be due to drought sensitivity increasing with tree size due to increased leaf area (Ammer, 2017). Other studies indicate long-term benefits of thinning to drought resistance (Giuggiola et al., 2013; Ammer, 2017) due to reductions in stand level transpiration and increases in soil water availability. Further examination of effects of thinning of these mixed stands on resistance, recovery, and resilience including data covering a longer time period, severe drought events, and including multiple sites with a range of drought stress is needed to improve our understanding of consequences of drought, and effects of drought severity and duration. Examination of effects of a broader range of spruce densities, and of key processes would also be useful.
How Does Thinning Influence Stand Volume and MAI?
Yield outcomes for Big River are similar to results reported by Kabzems et al. (2016), with increasing aspen densities leading to increased aspen yield and decreased spruce yield and delayed culmination age for spruce volume. These findings are in general agreement with hypothesis 4 (stand yield will be highest in mixed stands with moderate densities of aspen and white spruce while spruce yield will be highest without aspen). Thinning of aspen to densities below 4,000 trees ha−1 resulted in reduced volumes at harvest as reported by Bella and Yang (1991). Total (aspen plus spruce) mean annual increment (MAI) for the two Big River installations averaged 2.52 m3ha−1y−1 for the 1,000 spruce ha−1 treatment, 3.15 m3ha−1y−1 in the stands with 4,000 aspen ha−1 or unthinned, and 3.73 m3ha−1y−1 for the mixture with 1,500 aspen ha−1 and 1,000 spruce ha−1. The 1,500 aspen ha−1 and 1,000 spruce ha−1 mixture provided a 23% increase in total (aspen plus spruce) MAI over the pure aspen stands, with little reduction in aspen MAI and with a 44% reduction in spruce MAI. Kabzems et al. (2016) indicate, based on results from MGM, that the highest total stand yields were achieved with an aspen density of 2,000 to 5,000 trees ha−1 and a spruce density of 1,300 trees ha−1. Differences between Kabzems et al. (2016) and this study reflect differences in treatments, site, climate and other factors.
Potential overyielding at Big River is indicated by estimated total stand MAI being higher in mixtures with 1,500 or 4,000 aspen ha−1 and with either 500 or 1,000 spruce ha−1. Consistent with the evidence of overyielding indicated by MGM estimates of higher MAI of mixed stands with aspen density above 1,500 trees ha−1 compared to pure stands, Kweon and Comeau (2019) also found that overyielding was strongest in plots with 1,500 aspen ha−1, based on analysis of data from seven similar WESBOGY installations in Alberta. Overyielding is thought to result from differences in growth rates and shade tolerance of aspen and white spruce which leads to niche separation, and the ability of the mixed stand to use more light and carry a larger total number of trees than is the case for pure stands (Kabzems et al., 2016; Kweon and Comeau, 2019). Alternative management strategies could see removal of aspen at earlier ages while leaving spruce to grow to culmination. The application of this “understory protection” approach has the potential to increase yields of both aspen and spruce.
Study Limitations
Analysis of effects of climate on growth and on resistance, recovery and resilience to drought stress was limited by sampling of only the largest trees of each species across the plots used in this study, and by the short time period over which useable tree ring data were available (most notably for white spruce). Collection of cores from or annual diameter measurement for a larger sample of trees representing the full range of tree sized and spanning a longer time period, would be beneficial. Using average CMI over a 3-year period to examine effects of drought stress requires further testing based on the dynamics of soil water storage on these and on other sites. Data from sites covering a broader range of climatic conditions are needed to provide more general inferences regarding effects of stand density and composition on responses of mixedwood stands to drought. In addition, supplemental studies are required to improve understanding of the mechanisms associated with these responses. Since MGM18 does not consider potential effects of changing climate on yield, the yield forecasts need to be interpreted cautiously.
Ongoing remeasurement of this study at 5-year intervals is planned and will provide valuable long-term information on treatment effects and stand dynamics. Coring and/or annual measurement of diameter increment, coupled with field measurements of soil moisture across several sites with similar treatments over a 20 year or longer time period would contribute substantially to understanding of the effects of thinning treatments on resilience of boreal aspen-white spruce mixtures to drought and increasing temperatures.
Conclusions
Results from analysis of data collected at age 26 (21 years after pre-commercial thinning) for the Big River WESBOGY LTS installations indicate:
1. Thinning lead to significant increases in aspen average height, average and top DBH and reductions in average and top slenderness but did not alter top height at age 26;
2. Spruce height and diameter at age 26 increased and spruce slenderness decreased with reductions in aspen density;
3. Crown width and live crown ratio of both aspen and spruce at age 26 increased following thinning, with values being highest at the lowest aspen densities;
4. Tree size and CMI had significant positive effects while aspen basal area had significant negative effects on the growth of individual trees cored in 10 (spruce) or 11 (aspen) plots in each of the two installations;
5. Resistance and resilience of aspen and spruce to drought events were negatively affected by increases in tree size (basal area) and aspen density (basal area). Results suggest that thinning of the aspen had enduring effects on growth, and on drought resistance and resilience of both species;
6. Aspen yield (at age 100) is potentially reduced but aspen DBH at age 100 is increased by thinning of the aspen while spruce yield was increased by thinning the aspen. Spruce yield was higher for 1,000 trees ha−1 than for 500 trees ha−1 initial densities.
7. Yield projections suggest that the mixture of 1,500 aspen and 1,000 spruce per hectare has the potential to provide a 23% increase in total MAI with a single harvest at age 100, compared to unthinned aspen stands, but with a 44% reduction in spruce MAI compared to pure spruce stands.
Data Availability Statement
The raw data supporting the conclusions of this article will be made available by the authors, without undue reservation.
Author Contributions
PC analyzed data and wrote this paper.
Funding
Funding to support the 2018 and 2019 remeasurement and data entry for the Big River LTS installations was provided by the Forest Innovation Program – Canadian Wood Fibre Centre 2018–2019.
Conflict of Interest
The author declares that the research was conducted in the absence of any commercial or financial relationships that could be construed as a potential conflict of interest.
Acknowledgments
I gratefully acknowledge the involvement of several agencies and individuals in the establishment, management and remeasurement of the Big River Wesbogy long-term study installations. These installations were established by Weyerhaeuser Canada in 1992 under the direction of Mr. Paul Leblanc and maintained by Weyerhaeuser through 2008. The Canadian Wood Fibre Centre of the CFS accepted responsibility for maintenance and measurement of these installations from 2010 through 2016, and we are grateful to Derrek Sidders, Dan MacIsaac and Tim Keddy for their assistance. We also gratefully acknowledge assistance from Saskatchewan Environment with monitoring and maintaining these installations. I am particularly grateful to Susah Humphries, Rachel Keglowitsch, Felix Oboite and Kayla Frankiw for their assistance with remeasuring these installations and with data entry and preparation and to Kirk Johnson for his assistance with MGM initialization and simulations. I am also grateful to two reviewers and to Dan Kneeshaw for their useful comments on the draft manuscript.
Supplementary Material
The Supplementary Material for this article can be found online at: https://www.frontiersin.org/articles/10.3389/ffgc.2020.621752/full#supplementary-material
References
Alam, S. A., Huang, J.-G., Stadt, K. J., Comeau, P. G., Dawson, A., Gea-Izquierdo, G., et al. (2017). Effects of competition, drought stress and photosynthetic productivity on the radial growth of white spruce in western Canada. Front. Plant Sci. 8:1915. doi: 10.3389/fpls.2017.01915
Ammer, C. (2017). “Unraveling the importance of inter- and intraspecific competition for the adaptation of forests to climate change,” in Progress in Botany, Vol. 78, eds F. M. Canovas, U. Lüttge, and R. Matyssek (Berlin: Springer), 345–367. doi: 10.1007/124_2016_14
Andrews, C. M., D'Amato, A. W., Fraver, S., Palik, B., Battaglia, M. A., and Bradford, J. B. (2020). Low density moderates growth declines during hot droughts in semi-arid forests. J. Appl. Ecol. 57, 1089–1102. doi: 10.1111/1365-2664.13615
Bell, D. M., Bradford, J. B., and Lauenroth, W. K. (2014). Forest stand structure, productivity, and age mediate climatic effects on aspen decline. Ecology 95, 2040–2046. doi: 10.1890/14-0093.1
Bella, I. E. (1986). Logging practices and subsequent development of aspen stands in east-central Saskatchewan. For. Chron. 62, 81–83. doi: 10.5558/tfc62081-2
Bella, I. E., and Yang, R. C. (1991). “Should we thin young aspen stands?” in Aspen Management for the 21st Century, eds S. Navratil, and P. B. Chapman (Edmonton, AB: Forestry Canada, Northern Forestry Centre), 135–139.
Bergeron, Y., Chen, H. Y. H., Kenkel, N. C., Leduc, A. L., and Macdonald, S. E. (2014). Boreal mixedwood stand dynamics: ecological processes underlying multiple pathways. For. Chron. 90, 202–213. doi: 10.5558/tfc2014-039
Bickerstaff, A. (1946). The effect of thinning upon the growth and yield of aspen stands. Project P-19. Silviculture Research Note. Ottawa, ON: Department of Mines and Resources, Lands, Parks, and Forests Branch, Dominion Forest Service.
Bokalo, M., Comeau, P. G., and Titus, S. J. (2007). Early development of tended mixtures of aspen and spruce in western Canadian boreal forests. For. Ecol. Manage. 242, 175–184. doi: 10.1016/j.foreco.2007.01.038
Bottero, A., D'Amato, A. W., Palik, B. J., Bradford, J. B., Fraver, S., Battaglia, M. A., et al. (2017). Density-dependent vulnerability of forest ecosystems to drought. J. Appl. Ecol. 54, 1605–1614. doi: 10.1111/1365-2664.12847
Burns, R. M., and Honkala, B. H. (1990). Silvics of North America. Washington, DC: US Dept. of Agriculture, Forest Service, Agricultural Handbook 654. Available online at: https://www.srs.fs.usda.gov/pubs/misc/ag_654/table_of_contents.htm
Campbell, E. M., Antos, J. A., and van Akker, L. (2019). Resilience of southern Yukon boreal forests to spruce beetle outbreaks. For. Ecol. Manage. 433, 52–63. doi: 10.1016/j.foreco.2018.10.037
Chen, H. Y. H., and Popadiouk, R. V. (2002). Dynamics of North American boreal mixedwoods. Environ. Rev. 10, 137–166. doi: 10.1139/a02-007
Chen, L., Huang, J.-G., Alam, S. A., Zhai, L., Dawson, A., Stadt, K. J., et al. (2017a). Drought causes reduced growth of trembling aspen in western Canada. Glob. Change Biol. 23, 2887–2902. doi: 10.1111/gcb.13595
Chen, L., Huang, J.-G., Dawson, A., Zhai, L., Stadt, K. J., Comeau, P. G., et al. (2017b). Contributions of insects and droughts to growth decline of trembling aspen mixed boreal forest of western Canada. Glob. Change Biol. 24, 655–667. doi: 10.1111/gcb.13855
Chen, L., Huang, J.-G., Stadt, K. J., Comeau, P. G., Zhai, L., Dawson, A., et al. (2017c). Drought explains variation in the radial growth of white spruce in western Canada. Agric. For. Met. 233, 133–142. doi: 10.1016/j.agrformet.2016.11.012
Comeau, P. G., Wang, J. R., and Letchford, T. (2003). Influences of paper birch competition on growth of understory white spruce and subalpine fir following spacing. Can. J. For. Res. 33, 1962–1973. doi: 10.1139/x03-117
Cortini, F., and Comeau, P. G. (2020). Pests, climate and competition effects on survival and growth of trembling aspen in western Canada. N. For. 51, 175–190. doi: 10.1007/s11056-019-09726-9
Cortini, F., Comeau, P. G., and Bokalo, M. (2012). Trembling aspen competition and climate affects white spruce growth in boreal mixtures of western Canada. For. Ecol. Manage. 277, 67–73. doi: 10.1016/j.foreco.2012.04.022
D'Amato, A. W., Bradford, J. B., Fraver, S., and Palick, B. J. (2013). Effects of thinning on drought vulnerability and climate response in north temperate forest ecosystems. Ecol. Appl. 23, 1735–1742. doi: 10.1890/13-0677.1
del Rio, M., Bravo-Oviedo, A., Pretzsch, H., Lof, M., and Puiz-Peinado, R. (2017). A review of thinning effects on Scots pine stands: from growth and yield to challenges under global change. For. Syst. 26:eR03S. doi: 10.5424/fs/2017262-11325
Filipescu, C. N., and Comeau, P. G. (2007). Aspen competition affects light and white spruce growth across several boreal sites in western Canada. Can. J. For. Res. 37, 1701–1713. doi: 10.1139/X07-011
Filipescu, C. N., and Comeau, P. G. (2011). Influence of Populus tremuloides density on air and soil temperature. Scand. J. For. Res. 26, 421–428. doi: 10.1080/02827581.2011.570784
Forrester, D. I. (2014). The spatial and temporal dynamics of species interactions in mixed-species forests: from pattern to process. For. Ecol. Manage. 312, 282–292. doi: 10.1016/j.foreco.2013.10.003
Forrester, D. I. (2015). Transpiration and water-use efficiency in mixed-species forests versus monocultures: effects of tree size, stand density and season. Tree Physiol. 35, 289–304. doi: 10.1093/treephys/tpv011
Frey, B. R., Lieffers, V. J., Landhausser, S. M., Comeau, P. G., and Greenway, K. J. (2003). An analysis of sucker regeneration of trembling aspen. Can. J. For. Res. 33, 1169–1179. doi: 10.1139/x03-053
Giuggiola, A., Bugmann, H., Zingg, A., Dobbertin, M., and Rigling, A. (2013). Reduction of stand density increases drought resistance in xeric Scots pine forests. For. Ecol. Manage. 310, 827–835. doi 10.1016/j.foreco.2013.09.030
Gray, L. K., and Hamann, A. (2013). Tracking suitable habitat for tree populations under climate change in western North America. Clim. Change 117, 289–303. doi: 10.1007/s10584-012-0548-8
Groot, A., and Schneider, R. (2011). Predicting maximum branch diameter from crown dimensions, stand characteristics and tree species. For. Chron. 87, 542–551. doi: 10.5558/tfc2011-053
Hogg, E. H., Barr, A. G., and Black, T. A. (2013). A simple soil moisture index for representing multi-year drought impacts on aspen productivity in the western Canadian Interior. Agric. For. Meteorol. 178–179, 173–182. doi: 10.1016/j.agrformet.2013.04.025
Hogg, E. H., Brandt, J. P., and Kochtubajda, B. (2005). Factors affecting interannual variation in growth of western Canadian aspen forests during 1951-2000. Can. J. For. Res. 35, 610–622. doi: 10.1139/x04-211
Hogg, E. H., Brandt, J. P., and Michaelian, M. (2008). Impacts of a regional drought on the productivity, dieback and biomass of western Canadian aspen forests. Can. J. For. Res. 38, 1373–1384. doi: 10.1139/X08-001
Hogg, E. H., Michaelian, M., Hook, T. I., and Undershultz, M. E. (2017). Recent climatic drying leads to age-independent growth reductions of white spruce stands in western Canada. Glob. Change Biol. 23, 5297–5308. doi: 10.1111/gcb.13795
Holofsky, J. E., Andrews-Key, S. A., Edwards, J. E., Johnston, M. H., Nelson, H. W., Peterson, D. L., et al. (2018). Adapting forest management to climate change: the state of science and applications in Canada and the United States. For. Ecol. Manage. 421, 84–97. doi: 10.1016/j.foreco.2018.02.037
Huang, S., Meng, S. X., and Yang, Y.. (2009). A Growth and Yield Projection System (GYPSY) for Natural and Post-harvest Stands in Alberta. Alberta Sustainable Resource Development, Forest Management Branch, Edmonton, AB Publication, 22. Available online at: https://open.alberta.ca/publications/9780778584865 (accessed May 28, 2020).
Jiang, X., Huang, J.-G., Cheng, J., Dawson, A., Stadt, K. J., Comeau, P. G., et al. (2018). Interspecific variation in growth responses to tree size, competition and climate of western Canadian boreal mixed forests. Sci. Total Environ. 631–632, 1070–1078. doi: 10.1016/j.scitotenv.2018.03.099
Kabzems, R., Bokalo, M., Comeau, P. G., and MacIsaac, D. A. (2016). Managed mixtures of aspen and white spruce 21 to 25 years after establishment. Forests 7:5. doi: 10.3390/f7010005
Kabzems, R., and Garcia, O. (2004). Structure and dynamics of trembling aspen – white spruce mixed stands near Fort Nelson, B.C. Can. J. For. Res. 34, 384–395. doi: 10.1139/x03-254
Keenan, R. J. (2015). Climate change impacts and adaptation in forest management: a review. Ann. For. Sci. 72, 145–167. doi: 10.1007/s13595-014-0446-5
Kershaw, J. A., Maguire, C. A., and Hann, D. W. (1990). Longevity and duration of radial growth in Douglas-fir branches. Can. J. For. Res. 20, 1690–1695. doi: 10.1139/x90-225
Kweon, D., and Comeau, P. G. (2017). Effects of climate on maximum size-density relationships in western Canadian trembling aspen stands. For. Ecol. Manage. 406, 281–289. doi: 10.1016/j.foreco.2017.08.014
Kweon, D., and Comeau, P. G. (2019). Factors influencing overyielding in young boreal mixedwood stands in western Canada. For. Ecol. Manage. 432, 546–557. doi: 10.1016/j.foreco.2018.09.053
Lieffers, V. J., Macmillan, R. B., MacPherson, D., Branter, K., and Stewart, J. D. (1996). Semi-natural and intensive silvicultural systems for the boreal mixedwood forest. For. Chron. 72, 286–292. doi: 10.5558/tfc72286-3
Lieffers, V. J., and Stadt, K. J. (1994). Growth of understory Picea glauca, Calamagrostis canadensis and Epilobium angustifolium in relation to overstory light. Can. J. For. Res. 24, 1193–1198. doi: 10.1139/x94-157
Lloret, F., Keeling, E. G., and Sala, A. (2011). Components of tree resilience: effects of successive low-growth episodes in old ponderosa pine forests. Oikos 120, 1909–1920. doi: 10.1111/j.1600-0706.2011.19372.x
MacDonald, G. B. (1996). “Mixed wood management research and practice in Ontario,” in Silviculture of temperate and boreal broadleaf-conifer mixtures, eds P. G. Comeau and K. D. Thomas, Vol. 36 (Victoria, BC: Province of British Columbia; Ministry of Forests Research Program), 102–113. Available online at: https://www.for.gov.bc.ca/hfd/pubs/Docs/Lmh/Lmh36.htm (accessed May 28, 2020).
Macdonald, S. E., Lecomte, N., Bergeron, Y., Brais, S., Chen, H., Comeau, P., et al. (2010). Ecological Implications of Changing the Composition of Boreal Mixedwood Forests. A State of Knowledge Report. Edmonton, AL: Sustainable Forest Management Network, 48. Available online at: https://sfmn.ualberta.ca/publications/state-of-knowledge-reports/ (accessed May 28, 2020).
Man, R., and Lieffers, V. J. (1999). Are mixtures of aspen and white spruce more productive than single species stands? For. Chron. 75, 505–513. doi: 10.5558/tfc75505-3
Michaelian, M., Hogg, E. H., Hall, R. J., and Arsenault, E. (2011). Massive mortality of aspen following severe drought along the southern edge of the Canadian boreal forest. Glob. Change Biol. 17, 2084–2094. doi: 10.1111/j.1365-2486.2010.02357.x
Peng, C., Ma, Z., Lei, X., Zhu, Q., Chen, H., Wang, W., et al. (2011). A drought-induced pervasive increase in tree mortality across Canada's boreal forests. Nat. Clim. Change 1, 467–471. doi: 10.1038/nclimate1293
Penner, M., Robinson, C., and Woods, M. (2001). The response of good and poor aspen clones to thinning. For. Chron. 77, 874–884. doi: 10.5558/tfc77874-5
Perala, D. A. (1978). Thinning Strategies for Aspen: A Predictive Model. USDA Forest Service Research Paper NC-RP-161.
Peterson, E. B., and Peterson, N. M. (1992). Ecology, Management and Use of Aspen and Balsam Poplar in the Prairie Provinces, Canada, Vol. 1. Edmonton, AL: Forestry Canada, Northwest Region, Northern Forestry Centre, 252.
Price, D. T., Alfaro, R. I., Brown, K. J., Flannigan, M. D., Fleming, R. A., Hogg, E. H., et al. (2013). Anticipating the consequences of climate change for Canada's boreal forest ecosystems. Environ. Rev. 21, 322–365. doi: 10.1139/er-2013-0042
Rice, J. A., MacDonald, G. B., and Weingartner, D. H. (2001). Precommercial thinning of trembling aspen in northern Ontario: part 1 – growth responses. For. Chron. 77, 893–901. doi: 10.5558/tfc77893-5
Schmitt, A., Trouver, R., Seynave, I., and Lebourgeois, F. (2020). Decreasing stand density favors resistance, resilience and recovery of Quercus petraea trees to severe drought, particularly on dry sites. Ann. For. Sci. 77:52. doi: 10.1007/s13595-020-00959-9
Sohn, J. A., Saha, S., and Bauhus, J. (2016). Potential of forest thinning to mitigate drought stress: a meta-analysis. For. Ecol. Manage. 380, 261–273. doi: 10.1016/j.foreco.2016.07.046
Spittlehouse, D. L., and Stewart, R. B. (2003). Adapting to climate change in forest management. BC J. Ecosyst. Manage. 4, 7–17. Available online at: https://jem-online.org/index.php/jem/article/view/254/173 (accessed May 30, 2020).
Steckel, M., Moser, W. K., del Rio, M., and Pretzsch, H. (2020). Implications of reduced stand density on tree growth and drought susceptibility: a study of three species under varying climate. Forests 11:627. doi: 10.3390/f11060627
Steneker, G. A. (1976). Guide to the silvicultural management of trembling aspen in the prairie provinces. Information Report NOR-X-164. Edmonton, AB: Environment Canada Forestry Service, Northern Forest Research Centre.
Taylor, S. P., Alfaro, R. I., DeLong, C., and Rankin, L. (1996). The effects of overstory shading on white pine weevil damage to white spruce and its effect on spruce growth rates. Can. J. For. Res. 26, 306–312. doi: 10.1139/x26-034
Vore, M. E., Dery, S. J., Hou, Y., and Wei, X. (2020). Climatic influences on forest fire and mountain pine beetle outbreaks and resulting runoff effects in large watersheds in British Columbia, Canada. Hydrol. Proc. 2020, 1–16. doi: 10.1002/hyp.13908
Wang, T., Hamann, A., Spittlehouse, D. L., and Carroll, C. (2016). Locally downscaled and spatially customizable climate data for historical and future periods for North America. PLoS ONE 11:e0156720. doi: 10.1371/journal.pone.0156720
Keywords: boreal mixedwoods, thinning, trembling aspen, white spruce, drought, resistance, resilience
Citation: Comeau PG (2021) Effects of Thinning on Dynamics and Drought Resistance of Aspen-White Spruce Mixtures: Results From Two Study Sites in Saskatchewan. Front. For. Glob. Change 3:621752. doi: 10.3389/ffgc.2020.621752
Received: 26 October 2020; Accepted: 16 December 2020;
Published: 15 January 2021.
Edited by:
Dan Kneeshaw, Université du Québec à Montréal, CanadaReviewed by:
Rongzhou Man, Ontario Ministry of Natural Resources and Forestry, CanadaDaniel Dumais, Ministry of Forests, Wildlife and Parks, Canada
Copyright © 2021 Comeau. This is an open-access article distributed under the terms of the Creative Commons Attribution License (CC BY). The use, distribution or reproduction in other forums is permitted, provided the original author(s) and the copyright owner(s) are credited and that the original publication in this journal is cited, in accordance with accepted academic practice. No use, distribution or reproduction is permitted which does not comply with these terms.
*Correspondence: Philip G. Comeau, phil.comeau@ualberta.ca