- 1Davey Resource Group, Inc., Urban & Community Forestry Services, Atascadero, CA, United States
- 2Colorado State University, Department of Agricultural Biology, Fort Collins, CO, United States
- 3USDA Forest Service Hardwood Tree Improvement and Regeneration Center, West Lafayette, IN, United States
- 4Colorado State Forest Service Nursery, Fort Collins, CO, United States
Thousand cankers disease (TCD) is caused by the walnut twig beetle (Pityophthorus juglandis) vectoring the fungal canker pathogen Geosmithia morbida, which can result in severe dieback and eventual death to species of walnut (Juglans spp.) and wingnut (Pterocarya spp.). This disease is most devastating to the highly valued species J. nigra (black walnut). This species is primarily grown and harvested for timber production in the Central Hardwood Region of the United States, which comprises part of its native range. Management options for TCD are limited; therefore, finding resistant genotypes is needed. Initial studies on black walnut susceptibility to G. morbida documented some genetic variation and suggested potential resistance. Furthermore, G. morbida is thought to be native to the United States, which may have allowed for co-evolution. To capture the representative genetic diversity and screen for resistance to G. morbida, J. nigra families were collected from across the native range. These wild trees, in conjunction with seedlings developed in a black walnut timber improvement program, were planted in a common garden in Fort Collins, Colorado and repeatedly inoculated with G. morbida over the course of four years and three growing seasons. Improved seedlings exhibited larger cankered areas than wild J. nigra of the same provenance. Cankers induced by G. morbida in wild germplasm were smaller on J. nigra collected from the western and central portions of the native range compared to those collected from the eastern portion. Although trees from the western and central part of the range still incurred cankers, our findings indicate that variation in genetic resistance to G. morbida is present in black walnut. This study was performed with G. morbida independent of the walnut twig beetle, but our results suggest the limited G. morbida resistance observed in J. nigra will prevent the full compromise of black walnut to TCD. Results from this study should be taken into consideration in future black walnut breeding programs.
Introduction
Juglans nigra L., commonly referred to as black walnut, is an ecologically and economically important tree species in the United States, valued for its timber and edible nuts (Michler et al., 2007; Newton et al., 2009). The native range of J. nigra encompasses parts of the midwestern and eastern United States and landscape plantings occur widely throughout the western United States. However, instances of J. nigra mortality were documented in western states in the late 1990s and early 2000s. In 2007, a comprehensive investigation attributed the dieback to the walnut twig beetle (Pityophthorus juglandis Blackman, Coleoptera: Scolytidae) and its fungal associate Geosmithia morbida (Ascomycota: Hypocreales) (Tisserat et al., 2009; Kolařík et al., 2011). This disease was named thousand cankers disease (TCD) for the multitude of fungal cankers that form at beetle wound sites. Beetles mass attack trees while vectoring G. morbida and ultimately, this impedes the flow of resources throughout the tree. TCD impacts all walnut species (Juglans L.) and wingnut (Pterocarya Kunth), but J. nigra is the most susceptible host species (Serdani et al., 2013; Utley et al., 2013; Hishinuma et al., 2016; Hishinuma, 2017; Hefty et al., 2018). Without effective management tools available, TCD has become more widespread and most problematic to planted trees (Yaghmour et al., 2015; Seybold et al., 2019), but also threatening to the $500 million resource of standing J. nigra timber in the United States (Newton et al., 2009).
Management and detection of forest tree diseases are complicated. For example, the first indication of TCD is flagging and dieback in the upper crown, and trees are typically heavily infested when these symptoms appear. In severe cases, trees die within several years (Tisserat et al., 2009). Chemical controls (i.e., insecticides and fungicides) may be effective against TCD (Mayfield et al., 2019) but are impractical in the forest and chemical controls are not viable management options for nut production due to pesticides restrictions (Nix et al., 2013; OFR, 2016). Semiochemical treatments may be effective in an orchard-type setting (Audley et al., 2020) but management is largely focused on quarantine efforts (Daniels et al., 2016) and techniques to sanitize felled trees to prevent the transport of infected logs (Sitz, 2013; Mayfield et al., 2014; Audley et al., 2015; Audley et al., 2016; Elisa et al., 2019; Seabright et al., 2019). Exploration of biological control agents is in the initial stages and may be an option to augment other control tactics in the future (Lambdin et al., 2015; Castrillo et al., 2017; Mayfield et al., 2019). With these limitations, finding naturally resistant genotypes is an important step forward in TCD management (Sniezko and Koch, 2017; Lauritzen, 2018) and ultimately, the most practical solution.
Thousand cankers disease was documented throughout most of the western United States by 2009 (Arizona, California, Colorado, Idaho, New Mexico, Nevada, Oregon, Utah, and Washington) attacking native and planted walnut species (Tisserat et al., 2009, 2011). The initial occurrence of TCD in the western United States is spatially relevant because it overlaps with the native range of the walnut twig beetle. This beetle was described in the southwestern United States, New Mexico and Arizona (Blackman, 1928; Bright, 1981; Wood and Bright, 1992), where Arizona walnut (J. major Torr.) commonly occurs. Historic range expansions show walnut twig beetle in proximity to J. nigra and southern California walnut (J. californica S. Watson) (Cranshaw, 2011), and likely allowed for host shifts (Ploetz et al., 2013). The first record of TCD in the native range of J. nigra, occurred in Tennessee in 2010 (Grant et al., 2011). Since the initial accounts, consistent reports were made throughout the eastern United States (PDA, 2012; VDACS, 2011; ODNR/ODA, 2012; Seybold et al., 2012; NCDACS, 2013; Reed et al., 2013; MDA, 2015; Moore et al., 2019), and abroad (Italy) (Montecchio and Faccoli, 2014; Moricca et al., 2019).
Some genetic continuity exists between the fungal genotypes found in western and eastern United States (Hadziabdic et al., 2014; Zerillo et al., 2014). Therefore, it is possible that TCD has been repeatedly introduced into the eastern United States (Haun et al., 2010); and/or the fungus may be endemic nationwide as suggested by the widespread presence of Geosmithia spp. in other parts of the United States (Huang et al., 2019).
Genetic variability in resistance to the fungal component of TCD has been shown among J. nigra families (i.e., seedlings collected from the same parent tree) under greenhouse screening (Utley et al., 2013), where inoculated branches consistently and repeatedly differed in canker sizes despite similar virulence in G. morbida genetic clades (Sitz et al., 2017). This suggests that resistance to G. morbida is naturally present in black walnut, although to a lesser degree than in J. major, a historical host of the walnut twig beetle (Utley et al., 2013; Hishinuma, 2017). As genetic diversity exists in black walnut, we predicted that resistance should be greatest in J. nigra from the southwestern portion of the range where trees have historically been in closest proximity to TCD where natural selection for resistance would presumably be most intense and also where families are adapted to drought conditions. In the present study, we screened a collection of J. nigra families from across the native range of J. nigra and associated climate factors in order to determine if resistance to G. morbida is present in J. nigra and if locality and connected environmental factors were associated with resistance. A common garden plantation in Fort Collins, CO was used to (1) determine if any J. nigra families exhibited resistance to G. morbida and (2) to assess regional adaptability of any resistant J. nigra families that might aid in future TCD resistance breeding or conservation programs.
Materials and Methods
Plant Materials and Plantings
Walnut seeds were collected in the fall of 2012 from wild J. nigra, and each family consisted of open pollinated half-sibling seedlings. Trees included in the study were native, had at least 50 nuts, and harvested from a single tree (Zobel and Talbert, 1984). An emphasis was placed on collecting germplasm from the four corners and the center of the native range (Figure 1). In this study, the geographical provenances were grouped as: northeast (NE), northwest (NW), southeast (SE), southwest (SW), and central (CTR). A mix of several select walnut families that originated from the central provenance and developed by the Hardwood Tree Improvement and regeneration Center (HTIRC), were also included as two provenances [indicated as Forestry and Natural Resources (FNR) and Hardwood Tree Improvement (HTI)]. A number of HTI families were collected in previous years and had been tested in previous screening studies (Utley et al., 2013). Some of the HTI families had been harvested in 2010 and 2011, dried to 8% moisture content, and stored in a freezer at −2.8°C. All seed was fall sown in October of 2012 and seedlings germinated and grew at the Indiana Department of Natural Resources Division of Forestry nursery in Vallonia, IN in 2013. Seedlings were tagged and lifted in January of 2014 before shipment.
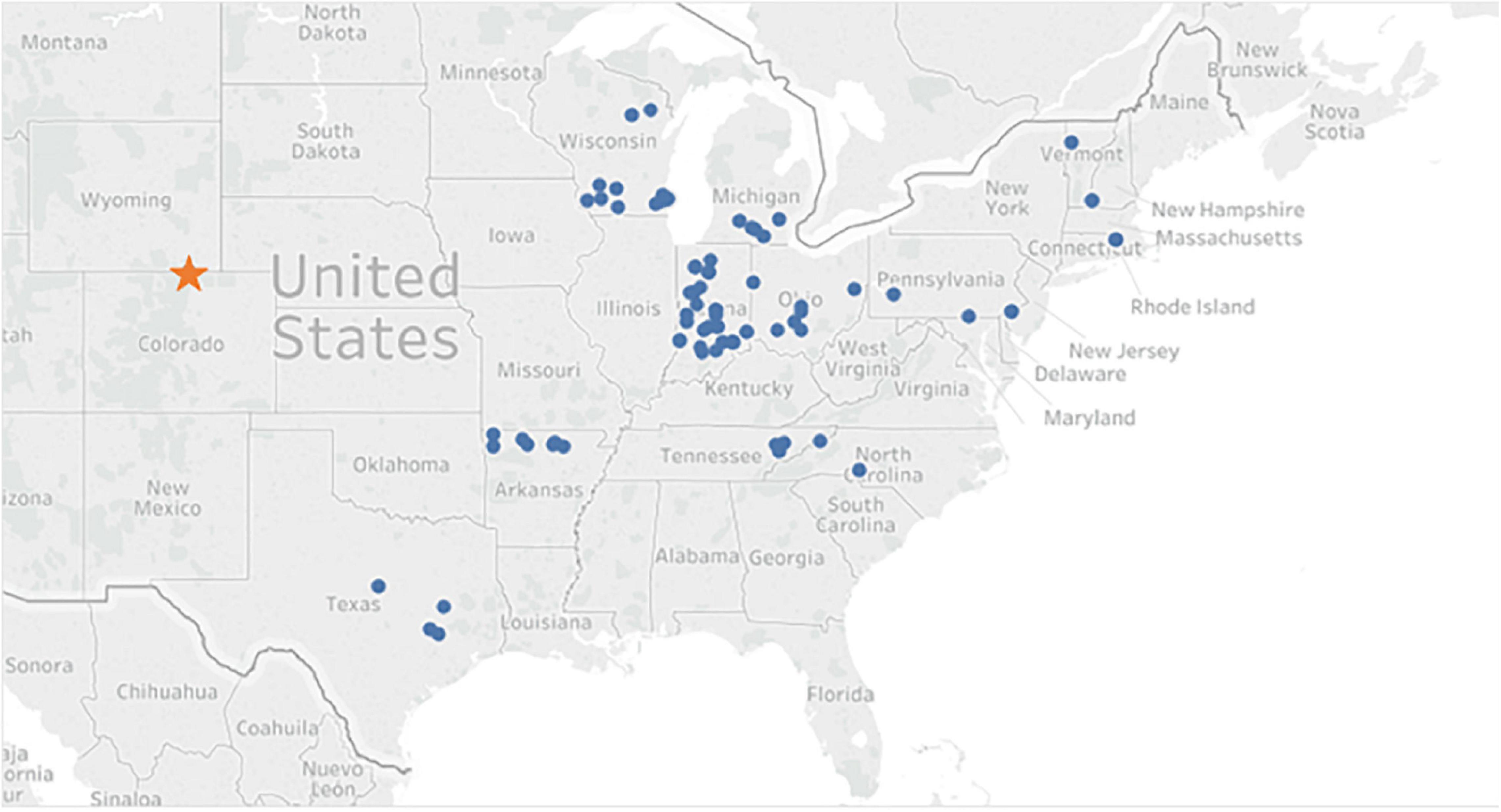
Figure 1. Map of Juglans nigra nut collection locations. Circles represent the location of a parent tree, or the origin of a family and the star indicates the common garden location.
For the TCD resistance screening, the dormant 1-year-old seedlings were shipped to the Colorado State Forest Service Nursery, Fort Collins, CO, on April 23, 2014. The common garden was planted “blind” as each tree was tagged with an arbitrary family code number. Provenance information was not received until the screen was completed. The screening experiment was planted as a completely randomized block design that consisted of nine rows with one tree per family per row because the amount of sunlight varied depending on the proximity to the shelterbelts. In total, 640 trees from 83 families were planted using a 3 ft planting scheme. Although the experimental design strived for 9 trees per family, several of the families had either 6 or 3 saplings due to mortality during storage and/or damaged roots. After planting and during the 2014 growing season, the walnut plot was treated for weed control at the label rate with pendimethalin (BASF Corp., Research Triangle Park, NC), a pre-emergent herbicide, and the plot was irrigated as needed throughout the season to encourage seedling establishment. In other years, the walnut trees did not receive any irrigation and the plot was mechanically weeded. Sapling mortality was recorded at the end of the experiment.
Fungal Isolates
Single-spore isolates of two G. morbida strains were grown on half-strength potato dextrose agar (1/2 PDA) for 10–14 days at 25°C in 100-mm-diameter × 15-mm-deep Petri dishes. Sterile 1/2 PDA was included as a control and G. morbida isolates originally obtained from diseased J. nigra in Colorado G1217 (CBS 124663; haplotype H03, genetic cluster 1) and G2071 (collected from Larimer, County, Fort Collins in 2014) were chosen as inoculum. Methods described by Zerillo et al. (2014) were used to determine haplotype and genetic cluster information for isolate G2071. Mycelium was harvested and lyophilized after 5 days of continual shaking at 70 rpm and 25°C in 1/2 potato dextrose broth. DNA extraction, PCR reaction mixtures, and PCR programs, as described in Zerillo et al. (2014), were used to amplify DNA from the b-tubulin (BT) gene and methionine aminopeptidase (MAP) gene of isolate G2071. DNA was amplified in a thermocycler (MJ Research Inc., Waltham, MA), confirmed by using a Sub-Cell GT Wide Mini (Bio-Rad, Hercules, CA) electrophoresis system, and PCR products were cleaned using a High Pure PCR Product Purification Kit (Roche Life Science). Ten nanograms of DNA per 100 base pairs and 25 pmol of primer were sequenced at Genewiz1, and chromatograms were visualized using Sequence Viewer Software 7.7.1 from Applied Biosystems2. Quality nucleotide sequences were compared to the G. morbida polymorphisms that determine haplotype and cluster as determined by Zerillo et al. (2014).
Inoculations
A total of 640 trees were inoculated on July 7 and 8, 2014, July 5 and 6, 2016, and June 27 and 28, 2017 by creating three wounds with a 5 mm metal punch. Trees were not inoculated in 2015 because stems were cut in 2014 and there was insufficient regrowth. Wounds were made ten centimeters apart on the main stem. The bark layer was removed and a control 1/2 PDA plug was placed onto the bottom wound with a scalpel, then a plug of 1/2 PDA with isolate G1217 into the middle wound, and a plug of 1/2 PDA with G2071 into the top wound. The inoculated area was wrapped with Parafilm for 6 weeks. Stems were harvested on August 18, 2014, August 16, 2016, and August 10 and 11, 2017 and stored at 4°C until processing. The bark was peeled back to expose cankers which were then photographed (Supplementary Figure 1). All cankers were measured within 6 weeks of the harvest date. In 2016, G. morbida was re-isolated from inoculated stems planted in each row, fulfilling Koch’s postulates.
Quantification of Canker Area and Statistics
Cankered areas (cm2) were quantified using image-processing software (Rasband, 1997-2016). Data were log transformed to better approximate assumptions of normality, then analyzed using JMP software v13.1.0 (Sas Institute, 2012). Mixed effects models were fit using log canker area as the response. Branch diameter, G. morbida strain, and provenance were included as fixed effects. To account for blocking in the experimental design, random effects included row, and nested terms. Nested terms included family within provenance. The analysis was run separately for each year. Pairwise comparisons between isolates were considered using a Fisher’s LSD method. Outliers were identified as data points that were outside the 3rd quartile + (1.5∗interquartile range).
Environmental Variables
To account for climatic variation given the broad range of J. nigra in the present study and possible intrinsic adaptive differences among the families, a principal component analysis was performed. Bioclimatic variables, n = 19, were obtained for each seed source location (WorldClim Database v1.4; Hijmans et al., 2005) and analyzed in R v3.5.0 (R Core Team, 2013) using raster, rgdal, foreach, and sp. packages (Dupin et al., 2011; Endriss et al., 2018). In addition, yearly precipitation measurements were obtained from records at the Colorado State University station approximately 1500 m from the common garden site for comparative purposes.
Results
Fungal Isolates
Comparisons of isolates G1217 and G2071 revealed genetic differences. Isolate G1217 was typed as an individual of haplotype 3 within cluster 1 (Zerillo et al., 2014), and we determined that G2071 falls within haplotype 40 within cluster 3. GenBank numbers are MW468403 (BT) and MW468404 (MAP). G1217 produced significantly larger cankers than G2071 in all three years (P < 0.001, df = 1, F = 100.4; 2014 P = 0.0151, df = 1, F = 5.95; 2016 P < 0.001, df = 1, F = 187.21; and 2017 P = 0.0134, df = 1, F = 6.16).
Family Differences
Cankered areas differed by family when analyzed over all 3 years (P < 0.001, df = 2, F = 615.65) and varied each year (2014 P = 0.4441, df = 6, F = 0.98; 2016 P = 0.0117, df = 6, F = 2.99; and 2017 P = 0.1074, df = 6, F = 1.81). In 2014, the establishment year, no significant differences were found either among families or provenances. Furthermore, cankered areas did not exhibit trends similar to those in 2016 and 2017 and were consistently smaller (Table 1). It is likely that the data obtained in 2014 are not indicative of host resistance; not only were the seedlings recently transplanted, but they also received supplementary water throughout the season. Therefore, the data obtained from 2014 were excluded from further analyses.
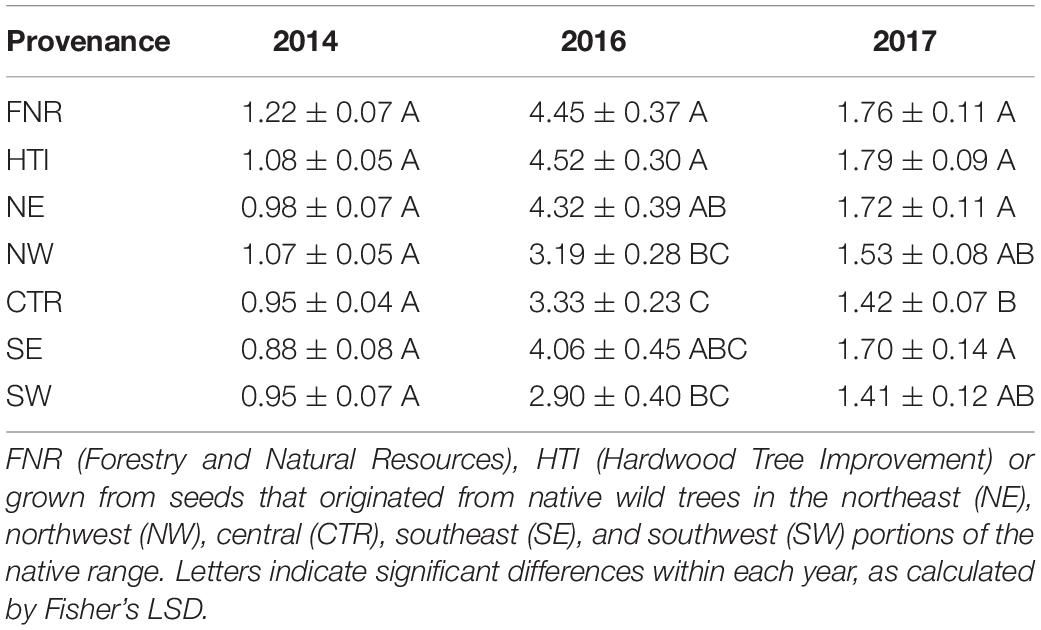
Table 1. Mean and standard error of cankered areas on seedling Juglans nigra selected for enhanced growth and timber quality at the Hardwood Tree Improvement Center.
Mean cankered areas of J. nigra families ranged from 0.81 to 7.88 cm2 in 2016 and from 0.98 to 3.83 cm2 in 2017. Additionally, individuals within a single family had differing responses to inoculation with G. morbida. For example, 2017 canker areas on individual trees from Family 49 (NW provenance) differed by as much as 10.6 cm2.
Provenance Differences
Despite canker areas being largest in 2016, some similarities between provenances were observed when comparing 2016 and 2017. In 2016 and 2017, cankered areas were smaller on trees from the western and central provenances when compared to eastern provenances (Figure 2 and Table 1). On average, the combined cankered areas on trees from the western and central provenances were 14% smaller than those from eastern provenances in 2016 and 8% smaller in 2017. The groups of walnuts in the breeding improvement program that originated from the central provenance (FNR and HTI), both had larger cankered areas than did wild trees from the central provenance (Table 1). In fact, the HTI region included one family (#14-008), previously screened as resistant (Utley et al., 2013), which had substantial cankers and large variation in this study (averaging 3.30 ± 1.69 and 1.02 ± 0.98 cm2 in 2016 and 2017, respectively).
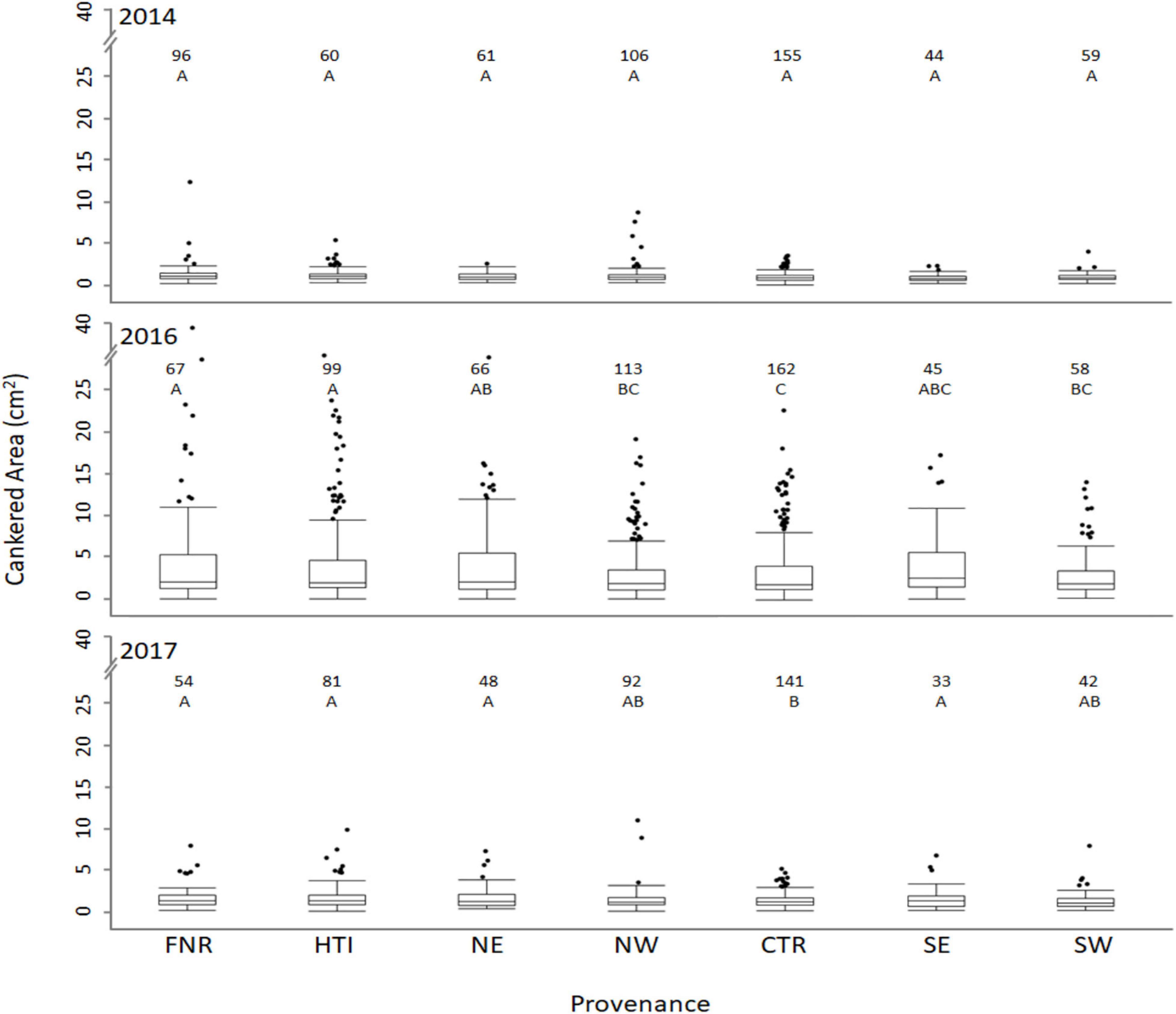
Figure 2. Size of cankered areas (cm2) on Juglans nigra seedlings bred for timber qualities (FNR, HTI) or grown from seed collected from trees that originated from the northeast, northwest, central, southeast, and southwest portion of the native range (NE, NW, CTR, SE, and SW provenances, respectively) planted in a common garden in Fort Collins, CO and inoculated with Geosmithia morbida. The boxes represent the 25th and 75th percentiles, the horizontal line in each box indicates the median. The whiskers signify maximum and minimum values, and circles indicate outliers. Outliers were identified as data points that were outside the 3rd quartile + (1.5 × interquartile range). The numbers above each box plot represent the number of trees tested from the given provenance.
Environmental Variables
Temperature and precipitation in the warmest quarter and in the driest month from seed source locations accounted for 87% of the variation in J. nigra performance (seedling health), as predicted by the seed source location and suitability of the common garden environment. Linear components PC1: temperature accounted for 62% of the variation; PC2: precipitation in the warmest quarter accounted for 17% of the variation; and PC3: precipitation in the driest month accounted for 8% of the variation (Supplementary Figure 2).
Effects of environmental variables associated with temperature and precipitation were also observed in the field. Trees from the southern provenances had higher mortality than trees from the central and northern provenances (Table 2). Over the study period, annual rainfall ranged from 98 to 109% of normal, however 2016 had below normal rainfall, 13–15% less than the other years (Table 3).
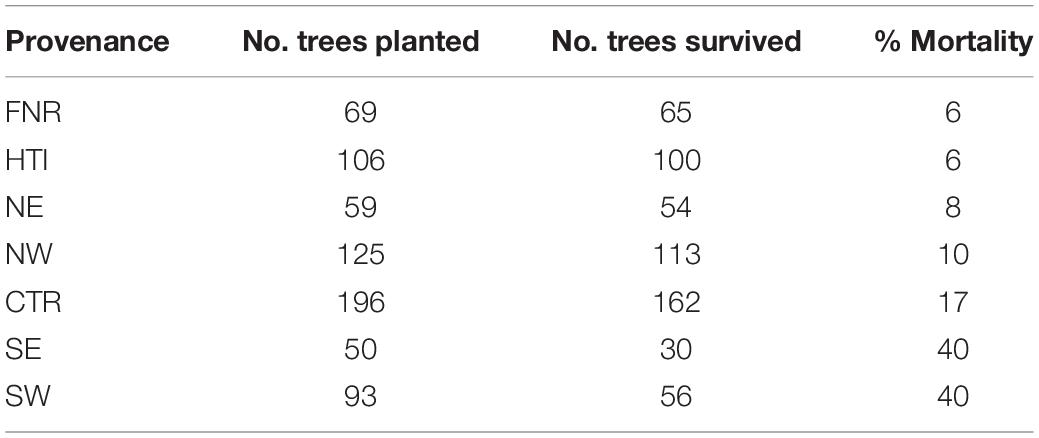
Table 2. Survival by provenance, including the number of trees planted, the number of trees that survived the duration of the experiment, and the percent mortality.
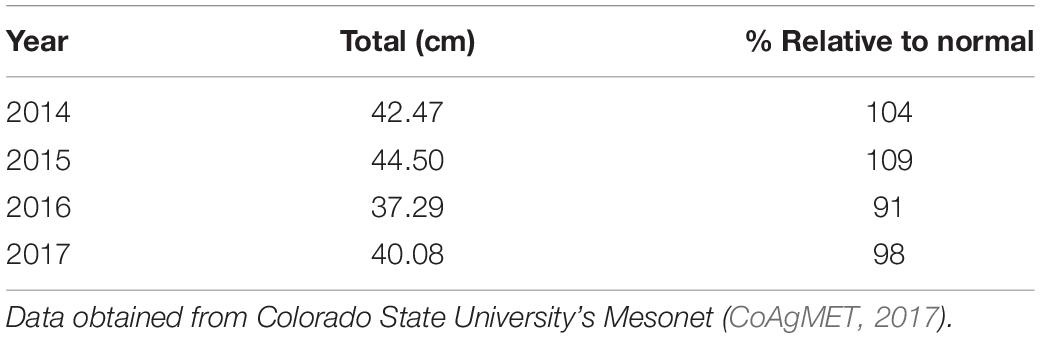
Table 3. Precipitation at the common garden site located at the Colorado State Forest Nursery over the length of the experiment, and the percent precipitation relative to the normal amount (40.8 cm).
Discussion
Despite significant differences in canker sizes and hence disease resistance in J. nigra, the cankered areas were substantial on all trees. Importantly, the improved J. nigra selections for enhanced growth and timber quality (Beineke, 1989; McKenna and Coggeshall, 2018) appeared to have been selected against G. morbida resistance, and consistently produced the largest cankers. This phenomenon is most clear when comparing the cankered areas on wild unselected trees from the central provenance. These findings emphasize the need for future breeding programs to consider the trade-offs between TCD resistance and growth rate, as is described by the growth differentiation balance hypothesis. This framework examines the allocation of available resources and the tradeoff between growth and cell differentiation, like those needed for defense mechanisms (Stamp, 2004). Trees in breeding programs selected for growth may inadvertently select against resistance, as observed in our study. Utilizing surviving J. nigra in areas that sustained TCD over many years may be a better breeding approach, as is the case for other systems, such as ash (Fraxinus spp.) and emerald ash borer (Agrilus planipennis). Future TCD screening programs should identify potential mechanisms of resistance, determine if the observed resistance is heritable (Telford et al., 2014), and allow a year or more for seedling establishment before screening, as demonstrated by our 2014 screening data.
Overall, cankered areas on wild J. nigra originating from the western and central provenances were smaller than those from eastern provenances, indicating there is some regional resistance to G. morbida. Underscoring this point, TCD has still not been documented in the western portions of the J. nigra native range. Furthermore, TCD has been seen in the eastern portion of the J. nigra native range (states such as Tennessee, North Carolina, and Pennsylvania, in particular with just several small occurrences in Ohio, and Indiana) causing some mortality, especially following drought (Griffin, 2014).
Outside of the native range, TCD has devastated J. nigra planted in urban landscapes throughout the western United States (Tisserat et al., 2009; Seybold et al., 2019). In contrast, within the native range, TCD has progressed very differently. For example, G. morbida has been detected on a variety of ambrosia beetles, other bark beetles, and weevils in the central and eastern part of J. nigra’s native range (Juzwik et al., 2015; Moore et al., 2019), yet mortality has not been documented with these non-walnut twig beetle associations nor have many species of Pityophthorus been documented.
These observed differences in TCD dynamics between the western United States and the eastern United States, might mean that TCD has not yet spread to the western part of J. nigra’s native range. On the other hand, these differences could also reflect heightened genetic resistance in western J. nigra reflecting co-evolution with G. morbida. Alternatively, co-evolution with a related pathogen species (exaptation) sometime in the past could confer resistance to G. morbida (Budde et al., 2016). Several studies have examined the genetic diversity of populations and examined genomic structure to assess whether G. morbida is native to the southwest or whether this a newly emerged pathogen (Hadziabdic et al., 2014; Schuelke et al., 2017). High genetic diversity and complexity of the pathogen suggests that G. morbida is native and has co-evolved with some Juglans spp. in the past (Daniels et al., 2016). Our results support that the higher resistance of western populations reflects co-evolution of J. nigra and G. morbida which would further support that G. morbida is likely a native organism.
It is not yet well understood if differences in susceptibility to P. juglandis exist in in J. nigra families. This knowledge, however, will be an integral part of understanding TCD epidemiology. Therefore, to more fully understand the impact of TCD, J. nigra identified to exhibit more resistance in this study should be challenged against high populations of P. juglandis naturally vectoring G. morbida. P. juglandis adaptation to the southwestern portion of the United States likely impacted disease progression, but the presence of G. morbida on additional insects (Warmund and van Sambeek, 2014; Juzwik et al., 2015; Chahal et al., 2019; Moore et al., 2019) could complicate TCD epidemiology, especially in areas where P. juglandis is maladapted.
Large variation in J. nigra cankers occurred in all families, similar to what was observed at the provenance level illustrated by numerous outliers in Figure 2. In some other host-pathogen systems such as Dutch Elm Disease and Chestnut Blight, host genetics has shown little variation in pathogen resistance (Gibbs, 1978; Anagnostakis, 1987; Budde et al., 2016). This suggests that J. nigra, and likely other North American black walnut species, may have co-evolved with this fungal pathogen. The host variation we detected, when coupled with the high genetic diversity of G. morbida (Zerillo et al., 2014; Hadziabdic et al., 2014) and P. juglandis (Rugman-Jones et al., 2015) in the western United States, provides further evidence that TCD is native to the United States. This has significant implications since native diseases are not known to have devastating impacts on their hosts. Rather they usually display moderate levels of damage consistent with endemism. The observed disease progression supports this idea, where TCD has been repeatedly found in the east yet mortality has occurred only in landscape trees and not progressed into native eastern forests. Such non-forest sites in the east have behaved much like J. nigra planted outside of the native range in the West (Seybold et al., 2019).
Prior to this screening study, a number of families within the HTI provenance were challenged with G. morbida in the greenhouse (Utley et al., 2013). One family, #14-008, previously recorded as resistant (Utley et al., 2013), incurred large cankers and therefore was not identified as resistant in our field trial. This is not uncommon, and discrepancies between screenings performed in the greenhouse and field have been previously documented (Telford et al., 2014) and show the importance of validating greenhouse trials in the field.
The most important bioclimatic variables obtained from J. nigra seed source locations were associated with temperature and precipitation in the warm or dry months. Winter hardiness is known to correlate to seed source location in J. nigra (Bey, 1979; Bresnan et al., 1993). In this study, it is likely that seedlings from the southern portion of the native range had the highest rates of mortality because they experienced cold temperatures associated with the 2014 polar vortex. The J. nigra common garden site received the least amount of precipitation in 2016, and cankered areas were the largest that year. Thus, drier conditions may have contributed to increased canker sizes in this study and were noted by Griffin (2014), but the correlation between drought and J. nigra susceptibility to G. morbida is a topic that needs further investigation.
G. morbida isolate G1217 produced larger cankered areas in this study and may be slightly more virulent than isolate G2071. Isolate G2071 was consistently used to inoculate the distal end of the main stem, and therefore smaller diameter branches which are known to exhibit larger cankered areas (Sitz et al., 2017), yet G2071 consistently resulted in smaller cankered areas among provenances and from year-to-year. The differences in cankered area between the two fungal isolates was biologically significant due to our robust sample size and replicate years.
This study investigated the relative susceptibility of J. nigra to G. morbida. Importantly, we found that J. nigra seedlings bred for improved growth rate and timber form were the least resistant to G. morbida. Walnuts originating from the northwest and southwest United States edges of the native range of J. nigra exhibited the smallest cankers and were therefore more resistant to G. morbida compared those originating in the eastern United States. The enhanced resistance was consistently exhibited in trees from the western portion of the J. nigra native range. This, coupled with the large variation in cankered areas, provides further evidence that G. morbida is native to the United States, and likely co-evolved with Juglans spp.
Overall, the environmental conditions at the common garden site were drier, and in some cases colder, than those found across the native range of J. nigra. As a result, susceptibility to G. morbida may have been coupled with stress responses of the families. Disease screening studies that include wide-ranging genotypes will have other genetic adaptations that are difficult to control and need to be considered when interpreting data. Results from this study should be taken into consideration in future J. nigra breeding programs, especially since traits ideal for timber production can reduce TCD resistance and may alter resistance to other diseases.
Data Availability Statement
The raw data supporting the conclusions of this article will be made available by the authors, without undue reservation.
Author Contributions
RS designed the study, collected and analyzed the data, and wrote the manuscript. EL designed the study, and collected and analyzed the data. JIC collected and analyzed the data. NT, WC, and JS designed the study and reviewed the drafted manuscript. JM sourced the materials for this study, analyzed the data, and reviewed the drafted manuscript. JS sourced the materials for this study and reviewed and drafted the manuscript. All authors contributed to the article and approved the submitted version.
Funding
Funding was provided by US Forest Service State and Private Special Technology Development Program, Project Number R9-2013-01, the Fred M. van Eck Foundation of the HTIRC at Purdue University, USDA Forest Service Northern Research Station, USDA Forest Service Rocky Mountain Research Station, Colorado State University, and the IN-DNR Division of Forestry Vallonia Nursery.
Conflict of Interest
RS was employed by company Davey Resource Group.
The remaining authors declare that the research was conducted in the absence of any commercial or financial relationships that could be construed as a potential conflict of interest.
Acknowledgments
The authors thank volunteers of the American Chestnut Foundation, Indiana Nut and Fruit Growers Association, Walnut Council members, USDA NRCS employees, and Purdue HTIRC staff and students for identifying trees and harvesting seed as well as Orion Aon at the Colorado State Forest Service Nursery. The Colorado State Forest Service Nursery allowed the use of their facilities for these experiments. Field and laboratory technicians including Rasha Al-Akeel, Alison Hall, Allison Serafin, Austin Broberg, Kellie Due, Erika Pierce, Melissa Schreiner, Stephan Miller, Wendlin Burns, Kristen Otto, Peter Forrence, Zach Longtine, Katsi Pena, Ellie Duke, Lysa DuCharme, and Hope Raymond, helped in fungal inoculations, harvesting cankered branches, and quantifying canker areas. Dr. Ann Hess provided statistical consulting through the Franklin Graybill Statistical Laboratory at Colorado State University. Dr. Stacy Endriss and Dr. Carrie Pike also helped with statistical analyses.
Supplementary Material
The Supplementary Material for this article can be found online at: https://www.frontiersin.org/articles/10.3389/ffgc.2021.627911/full#supplementary-material
Supplementary Figure 1 | Inoculation of Geosmithia morbida caused cankers on Juglans nigra.
Supplementary Figure 2 | Principal component biplot of bioclimatic variables to account for the climatic variation in the native range of Juglans nigra. The following 19 bioclimatic variables were obtained for each J. nigra seed source location. Linear components PC1: temperature accounted for 62.5% of the variation, PC2: precipitation in the warmest quarter accounted for 16.7% of the variation, and PC3: precipitation in the driest month accounted for 8% of the variation. The legend indicates the climate range, or GPS location, for each family. Each blue dot represents a family. BIO1 = Annual Mean Temperature; BIO2 = Mean Diurnal Range (Mean of monthly (max temp – min temp)); BIO3 = Isothermality (BIO2/BIO7) (×100); BIO4 = Temperature Seasonality (standard deviation × 100); BIO5 = Max Temperature of Warmest Month; BIO6 = Min Temperature of Coldest Month; BIO7 = Temperature Annual Range (BIO5-BIO6); BIO8 = Mean Temperature of Wettest Quarter; BIO9 = Mean Temperature of Driest Quarter; BIO10 = Mean Temperature of Warmest Quarter; BIO11 = Mean Temperature of Coldest Quarter; BIO12 = Annual Precipitation; BIO13 = Precipitation of Wettest Month; BIO14 = Precipitation of Driest Month; BIO15 = Precipitation Seasonality (Coefficient of Variation); BIO16 = Precipitation of Wettest Quarter; BIO17 = Precipitation of Driest Quarter; BIO18 = Precipitation of Warmest Quarter; BIO19 = Precipitation of Coldest Quarter.
Footnotes
References
Anagnostakis, S. L. (1987). Chestnut blight: the classical problem of an introduced pathogen. Mycologia 79, 23–37. doi: 10.2307/3807741
Audley, J. P., Bostock, R. M., and Seybold, S. J. (2020). Trap assays of the walnut twig beetle, Pityophthorus juglandis blackman (coleoptera: curculionidae: scolytinae), reveal an effective semiochemical repellent combination. J. Chem. Ecol. 46, 1047–1058. doi: 10.1007/s10886-020-01228-9
Audley, J., Mayfield, A. E., Myers, S. W., Taylor, A., and Klingeman, W. E. (2016). Phytosanitation methods influence posttreatment colonization of Juglans nigra logs by Pityophthorus juglandis (coleoptera: curculionidae: scolytinae). J. Eco. Entmol. 109, 213–221. doi: 10.1093/jee/tov252
Audley, J., Taylor, A., Klingeman, W. E., Mayfield, A. E., and Myers, S. W. (2015). Insecticide dip treatments to prevent walnut twig beetle colonization of black walnut logs. For. Prod. J. 66, 233–240. doi: 10.13073/fpj-d-15-000038
Beineke, W. F. (1989). Twenty years of black walnut genetic improvement at purdue university. Northern J. Appl. Forestry 6, 68–71. doi: 10.1093/njaf/6.2.68
Bey, C. F. (1979). Geographic variation in Juglans nigra in the midwestern united states. Silvae Genetica 28, 132–135.
Blackman, M. W. (1928). The genus Pityophthorus Eichh. in North America: A Revisional Study Of The Pityophthori, With Descriptions Of Two New Genera And Seventy-One Species. Syracuse, NY: New York State College of Forestry at Syracuse University.
Bresnan, D. F., Rink, G., Diebel, K., and Geyer, W. A. (1993). 22-year-old plantations. Silvae Genet. 43:4.
Bright, D. E. (1981). Taxonomic monograph of the genus Pitypohthorus eichhoff in north and central america (coleoptera: scolytidae). Mem. Entomol. Soc. Can. 113:378.
Budde, K. B., Nielsen, L. R., Ravn, H. P., and Kjaer, E. D. (2016). The natural evolutionary potential of tree populations to cope with newly introduced pests and pathogens—lessons learned from forest health catastrophes in recent decades. Curr. Forestry Rep. 2, 18–29. doi: 10.1007/s40725-016-0029-9
Castrillo, L. A., Mayfield, A. E. III, Griggs, M. H., Camp, R., Mudder, B., Taylor, A., et al. (2017). Mortality and reduced brood production in walnut twig beetles, Pityophthorus juglandis (coleoptera: curculionidae), following exposure to commercial strains of entomopathogenic fungi Beauveria bassiana and Metarhizium brunneum. Biol. Control 114, 79–86. doi: 10.1016/j.biocontrol.2017.08.007
Chahal, K., Gazis, R., Klingeman, W., Hadziabdic, D., Lambdin, P., Grant, J., et al. (2019). Assessment of alternative candidate subcortical insect vectors from walnut crowns in habitats quarantined for thousand cankers disease. Environ. Entomol. 48, 882–893. doi: 10.1093/ee/nvz064
CoAgMET (2017). Colorado’s Mesonet. Available Online at: http://coagmet.com/
Cranshaw, W. (2011). Recently recognized range extensions of the walnut twig beetle, Pityophthorus juglandis blackman (coleoptera: curculionidae: scolytinae), in the western united states. Coleopterists Bullet. 65, 48–50. doi: 10.1649/0010-065x-65.1.48
Daniels, D., Nix, K., Wadl, P., Vito, L., Wiggins, G., Windham, M., et al. (2016). Thousand cankers disease complex: a forest health issue that threatens Juglans species across the US. Forests 7:260. doi: 10.3390/f7110260
Dupin, M., Reynaud, P., Jarošík, V., Baker, R., Brunel, S., Eyre, D., et al. (2011). Effects of the training dataset characteristics on the performance of nine species distribution models: application to Diabrotica virgifera virgifera. PLoS One 6:e20957. doi: 10.1371/journal.pone.0020957
Elisa, D. A. L., Linaldeddu, B. T., Fanchin, G., Faccoli, M., and Montecchio, L. (2019). The potential for pesticide trunk injections for control of thousand cankers disease of walnut. Phytopathol. Mediterranea 58, 73–79.
Endriss, S. B., Alba, C., Norton, A. P., Pyšek, P., and Hufbauer, R. A. (2018). Breakdown of a geographic cline explains high performance of introduced populations of a weedy invader. J. Ecol. 106, 699–713. doi: 10.1111/1365-2745.12845
Gibbs, J. N. (1978). Intercontinental epidemiology of dutch elm disease. Ann. Rev. Phytopathol. 16, 287–307. doi: 10.1146/annurev.py.16.090178.001443
Grant, J. F., Windham, M. T., Haun, W. G., Wiggins, G. J., and Lambdin, P. L. (2011). Initial assessment of thousand cankers disease on black walnut, Juglans nigra, in eastern tennessee. Forests 2, 741–748. doi: 10.3390/f2030741
Griffin, G. J. (2014). Status of thousand cankers disease on eastern black walnut in the eastern united states at two locations over 3 years. Forest Pathol. 45, 203–214. doi: 10.1111/efp.12154
Hadziabdic, D., Vito, L. M., Windham, M. T., Pscheidt, J. W., Trigiano, R. N., and Kolarik, M. (2014). Genetic differentiation and spatial structure of Geosmithia morbida, the causal agent of thousand cankers disease in black walnut (Juglans nigra). Curr. Genet. 60, 75–87. doi: 10.1007/s00294-013-0414-x
Haun, G., Powell, S., Strohmeier, C., and Kirksey, J. (2010). State of Tennessee thousand cankers disease action plan. Tennessee Department of Agriculture, Division of Regulatory Services and Division of Forestry, Nashville, Tennessee. Available Online at: https://www.tn.gov/content/dam/tn/agriculture/documents/planthealth/AgBusTCDActionPlan.pdf
Hefty, A. R., Aukema, B. H., Venette, R. C., Coggeshall, M. V., McKenna, J. R., and Seybold, S. J. (2018). Reproduction and potential range expansion of walnut twig beetle across the juglandaceae. Biol. Invasions 20, 2141–2155. doi: 10.1007/s10530-018-1692-5
Hijmans, R. J., Cameron, S. E., Parra, J. L., Jones, P. G., and Jarvis, A. (2005). Very high resolution interpolated climate surfaces for global land areas. Int. J. Climatol. 25, 1965–1978. doi: 10.1002/joc.1276
Hishinuma, S. M., Dallara, P. L., Yaghmour, M. A., Zerillo, M. M., Parker, C. M., Roubtsova, T. V., et al. (2016). Wingnut (juglandaceae) as a new generic host for Pityophthorus juglandis (coleoptera: curculionidae) and the thousand cankers disease pathogen, Geosmithia morbida (ascomycota: hypocreales). Can. Entomol. 148, 83–91. doi: 10.4039/tce.2015.37
Hishinuma, S. (2017). Interactions Among The Walnut Twig Beetle, Pityophthorus Juglandis, The Pathogenic Fungus, Geosmithia Morbida, And Host Species In Thousand Cankers Disease In California. Davis: University of California.
Huang, Y. T., Skelton, J., Johnson, A. J., Kolařík, M., and Hulcr, J. (2019). Geosmithia species in southeastern USA and their affinity to beetle vectors and tree hosts. Fungal Ecol. 39, 168–183. doi: 10.1016/j.funeco.2019.02.005
Juzwik, J., Banik, M. T., Reed, S. E., English, J. T., and Ginzel, M. D. (2015). Geosmithia morbida found on weevil species Stenomimus pallidus in indiana. Plant Health Prog. 16, 7–10. doi: 10.1094/php-rs-14-0030
Kolařík, M., Freeland, E., Utley, C., and Tisserat, N. (2011). Geosmithia morbida sp. nov., a new phytopathogenic species living in symbiosis with the walnut twig beetle (Pityophthorus juglandis) on Juglans in USA. Mycologia 103, 325–332. doi: 10.3852/10-124
Lambdin, P., Nix, K., Grant, J., Pauslen, D., and Merten, P. (2015). Natural enemies of the walnut twig beetle in eastern tennessee. Int. J. Agric. Res. 2, 31–39.
Lauritzen, J. E. (2018). Characterization of black walnut genotypes for resistance to thousand cankers disease, frost hardiness and other desirable horticultural traits. Master’s Thesis. Logan, UT: Utah State University.
Mayfield, A. E. III, Fraedrich, S. W., Taylor, A., Merten, P., and Myers, S. W. (2014). Efficacy of heat treatment for the thousand cankers disease vector and pathogen in small black walnut logs. J. Eco. Entomol. 107, 174–184. doi: 10.1603/ec13390
Mayfield, A. E., Juzwik, J., Scholer, J., Vandenberg, J. D., and Taylor, A. (2019). Effect of bark application with Beauveria bassiana and permethrin insecticide on the walnut twig beetle (coleoptera: curculionidae) in black walnut bolts. J. Eco. Entomol. 112, 2493–2496. doi: 10.1093/jee/toz150
McKenna, J. R., and Coggeshall, M. V. (2018). The genetic improvement of black walnut for timber production. in Plant Breeding Reviews. (ed) I. Goldman New Jersy: John Wiley & Sons, Inc. 41, 263–290. doi: 10.1002/9781119414735.ch6
MDA (2015). Walnut twig beetle and thousand cankers disease. Available Online at: https://mda.maryland.gov/plants-pests/Pages/tcd.aspx.
Michler, C., Woeste, K., and Pijut, P. (2007). Black walnut. USDA Forest Service/UNL Faculty Publications. 45. Available Online at: http://digitalcommons.unl.edu/usdafsfacpub/45
Montecchio, L., and Faccoli, M. (2014). First record of thousand cankers disease Geosmithia morbida and walnut twig beetle Pityophthorus juglandis on Juglans nigra in europe. Plant Dis. 98, 696–696. doi: 10.1094/pdis-10-13-1027-pdn
Moore, M., Juzwik, J., Miller, F., Roberts, L., and Ginzel, M. D. (2019). Detection of Geosmithia morbida on numerous insect species in four eastern states. Plant Health Progr. 20, 133–139. doi: 10.1094/php-02-19-0016-rs
Moricca, S., Bracalini, M., Benigno, A., Ginetti, B., Pelleri, F., and Panzavolta, T. (2019). Thousand cankers disease caused by Geosmithia morbida and its insect vector Pityophthorus juglandis first reported on Juglans nigra in Tuscany, central Italy. Plant Dis. 103, 369–369. doi: 10.1094/pdis-07-18-1256-pdn
Newton, L., Fowler, G., Neeley, A. D., Schall, R. A., and Takeuchi, Y. (2009). Pathway assessment: geosmithia sp. And Pityophthorus Juglandis Blackman Movement From The Western Into The Eastern United States. Washington, DC: US Department of Agriculture, Animal and Plant Health Inspection Service.
Nix, K., Lambdin, P., Grant, J., Coots, C., and Merten, P. (2013). Concentration levels of imidacloprid and dinotefuran in five tissue types of black walnut. Juglans nigra. Forests 4, 887–897. doi: 10.3390/f4040887
NCDACS (2013). Haywood County wood products under NCDA&CS quarantine for thousand cankers disease. Available Online at: http://www.ncagr.gov/paffairs/release/2013/1-13-Thousand-Cankers-Quarantine-Haywood-County.htm
ODNR/ODA (2012). Walnut twig beetle now in Ohio County. Available Online at: http://thevwindependent.com/?p=32540
OFR (2016). Tolerances and exemptions for pesticide chemicals residues in food—specific tolerances. Available Online at: https://www.law.cornell.edu/cfr/text/40/part-180/subpart-C
PDA (2012). Thousand cankers disease description. Available Online at: https://www.agriculture.pa.gov/Plants_Land_Water/PlantIndustry/plant-health/TCD/Pages/default.aspx
Ploetz, R. C., Hulcr, J., Wingfield, M. J., and De Beer, Z. W. (2013). Destructive tree diseases associated with ambrosia and bark beetles: black swan events in tree pathology? Plant Disease 97, 856–872. doi: 10.1094/pdis-01-13-0056-fe
R Core Team (2013). R: A language and environment for statistical computing. Vienna, Austria: R Foundation for Statistical Computing.
Rasband, W.S. (1997-2016). ImageJ, U. S. National Institutes of Health, Bethesda, Maryland, USA. http://imagej.nih.gov/ij/
Reed, S., English, J. T., Juzwik, J., and Ginzel, M. (2013). Bark and ambrosia beetles and their associated fungi colonizing stressed walnut in missouri and indiana. Walnut Council Bull. 40, 17–19.
Rugman-Jones, P. F., Seybold, S. J., Graves, A. D., and Stouthamer, R. (2015). Phylogeography of the walnut twig beetle, Pityophthorus juglandis, the vector of thousand cankers disease in north american walnut trees. PLoS One 10:e0118264. doi: 10.1371/journal.pone.0118264
Schuelke, T. A., Guangxi, W., Westbrook, A., Woeste, K., Plachetzki, D. C., Broders, K., et al. (2017). Comparative genomics of pathogenic and non-pathogenic beetle-vectored fungi in the genus Geosmithia. Genom. Biol. Evol. 9, 3312–3327. doi: 10.1093/gbe/evx242
Seabright, K. W., Myers, S. W., Fraedrich, S. W., Mayfield, A. E., Warden, M. L., and Taylor, A. (2019). Methyl bromide fumigation to eliminate thousand cankers disease causal agents from black walnut. Forest Sci. 98:1911.
Serdani, M., Vlach, J. J., Wallis, K. L., Zerillo, M., McCleary, T., Romero-Severson, J., et al. (2013). First report of Geosmithia morbida and Pityophthorus juglandis causing thousand cankers disease in butternut. Plant Health Progr. 14:38. doi: 10.1094/php-2013-1018-01-br
Seybold, S. J., Coleman, T. W., Dallara, P. L., Dart, N. L., Graves, A. D., Pederson, L. A., et al. (2012). Recent collecting reveals new state records and geographic extremes in the distribution of the walnut twig beetle, Pityophthorus juglandis blackman (coleoptera: scolytidae), in the united states. Pan. Pac. Entomol. 88, 277–280. doi: 10.3956/2012-32.1
Seybold, S. J., Kilingeman III, W. E., Hishinuma, S. M., Coleman, T. W., and Graves, A. D. (2019). Status and impact of walnut twig beetle in urban forest, orchard, and native forest ecosystems. J. For. 117, 152–163. doi: 10.1093/jofore/fvy081
Sitz, R. (2013). Management Options For The Walnut Twig Beetle, Pityophthorus Juglandis Blackman, Vector Of The Fungal Canker Pathogen Geosmithia Morbida. Master’s Thesis. Fort Collins, USA: Colorado State University.
Sitz, R. A., Luna, E. K., Caballero, J. I., Tisserat, N. A., Cranshaw, W. S., and Stewart, J. E. (2017). Virulence of genetically distinct Geosmithia morbida isolates to black walnut and their response to coinoculation with Fusarium solani. Plant Dis. 101, 116–120. doi: 10.1094/pdis-04-16-0535-re
Sniezko, R. A., and Koch, J. (2017). Breeding trees resistant to insects and diseases: putting theory into application. Biol. Invasions 19, 3377–3400. doi: 10.1007/s10530-017-1482-5
Stamp, N. (2004). Can the growth-differentiation balance hypothesis be tested rigorously? Oikos. 107, 439–448. doi: 10.1111/j.0030-1299.2004.12039.x
Tisserat, N., Cranshaw, W., Leatherman, D., Utley, C., and Alexander, K. (2009). Black walnut mortality in colorado caused by the walnut twig beetle and thousand cankers disease. Plant Health Progr. 10:10. doi: 10.1094/PHP-2009-0811-01-RS
Telford, A., Cavers, S., Ennos, R. A., and Cottrell, J. E. (2014). Can we protect forests by harnessing variation in resistance to pests and pathogens? Forestry An Int. J. Forest Res. 88, 3–12. doi: 10.1093/forestry/cpu012
Tisserat, N., Cranshaw, W., Putnam, M. L., Pscheidt, J., Leslie, C. A., Murray, M., et al. (2011). Black walnut mortality in colorado caused by the walnut twig beetle and thousand cankers disease. Plant Health Prog. 10:10. doi: 10.1094/PHP-2011-0630-01-BR
Utley, C., Nguyen, T., Roubtsova, T., Coggeshall, M., Ford, T. M., Grauke, L. J., et al. (2013). Susceptibility of walnut and hickory species to Geosmithia morbida. Plant Dis. 97, 601–607. doi: 10.1094/pdis-07-12-0636-re doi: 10.1094/pdis-07-12-0636-re
VDACS (2011). VDACS - Plant and pest pervices - Thousand cankers disease. Available Online at: http://www.vdacs.virginia.gov/plant&pest/disease-tcd.shtml
Warmund, M., and van Sambeek, J. (2014). Thousand cankers disease: Geosmithia morbida spores isolated from a weevil. Mo. Environ. Gard. 20:3.
Wood, S. L., and Bright, D. E. Jr. (1992). A catalogue of scolytidae and platypodidae (coleoptera). part 2, volumes A & B. Great. Basin Nat. Mem. 13:1005.
Yaghmour, M. A., Nguyen, T. L., Roubtsova, T. V., Hasey, J. K., Fichtner, E. J., DeBuse, C., et al. (2015). First report of Geosmithia morbida on english walnut and its paradox rootstock in california. Plant Dis. 99:1183.
Zerillo, M. M., Caballero, J. I., Woeste, K., Graves, A. D., Hartel, C., Pscheidt, J. W., et al. (2014). Population structure of Geosmithia morbida, the causal agent of thousand cankers disease of walnut trees in the united states. PloS One 9:e112847. doi: 10.1371/journal.pone.0112847
Keywords: thousand cankers disease, Geosmithia morbida, resistance screening, black walnut, common garden
Citation: Sitz RA, Luna EK, Ibarra Caballero J, Tisserat NA, Cranshaw WS, McKenna JR, Stolz J and Stewart JE (2021) Eastern Black Walnut (Juglans nigra L.) Originating From Native Range Varies in Their Response to Inoculation With Geosmithia morbida. Front. For. Glob. Change 4:627911. doi: 10.3389/ffgc.2021.627911
Received: 10 November 2020; Accepted: 12 February 2021;
Published: 09 March 2021.
Edited by:
Mariangela N. Fotelli, Hellenic Agricultural Organization, GreeceReviewed by:
Jackson Audley, USDA Forest Service, United StatesPierluigi (Enrico) Bonello, The Ohio State University, United States
Copyright © 2021 Sitz, Luna, Ibarra Caballero, Tisserat, Cranshaw, McKenna, Stolz and Stewart. This is an open-access article distributed under the terms of the Creative Commons Attribution License (CC BY). The use, distribution or reproduction in other forums is permitted, provided the original author(s) and the copyright owner(s) are credited and that the original publication in this journal is cited, in accordance with accepted academic practice. No use, distribution or reproduction is permitted which does not comply with these terms.
*Correspondence: Rachael A. Sitz, UmFjaGFlbC5TaXR6QGRhdmV5LmNvbQ==; Jane E. Stewart, SmFuZS5TdGV3YXJ0QGNvbG9zdGF0ZS5lZHU=