A Case Study to Establish a Basis for Evaluating Geographic Origin Claims of Timber From the Solomon Islands Using Stable Isotope Ratio Analysis
- 1Agroisolab UK Ltd., Welburn, United Kingdom
- 2Agroisolab GmbH, Jülich, Germany
- 3Jodrell Laboratory, Royal Botanic Gardens, Kew, Richmond, United Kingdom
Global demand for low-cost forest products is leading manufacturers and traders to source timber and wood products from vulnerable nations and delicate ecosystems. One small island nation, the Solomon Islands, is seeing exploitation of natural resources accelerating to such a point that its natural forests may be exhausted by 2036. The main causes of natural forest loss on the archipelago are unsustainable or illegal logging practices. Various laws in consumer countries require that members of industry ensure that only legally sourced timber is placed onto their respective national markets. Those that break these laws or fail to act in a way that is compliant may be subject to harsh penalties. This study aims to establish scientific data to evaluate claims that timber has originated from the Solomon Islands. This will enable Operators to carry out due diligence analysis and permit members of Law Enforcement to conduct forensic investigations. Eighty timber core samples comprising 13 different genera of tropical trees were obtained from mature trees in two sites in the Solomon Islands (Guadalcanal and Kolombangara islands) during the period August 2019 to November 2019 using a Pickering Punch sampling device. Homogenised core samples were subject to δ18O, δ2H, δ13C, and δ34S stable isotope analysis using elemental analysis-isotope ratio mass spectrometry. Additional stable isotope data from relevant taxa and geographic origins (elevation, geographic co-ordinates) were also included in this research as an initial assessment of differences in stable isotope ratios between countries. Results show that significant differences are evident in the stable isotope ratios of the sampled taxa within the Solomon Islands (Guadalcanal and Kolombangara Islands) and between other countries. These data can be used as a basis of evaluation to evaluate origin claims of timber or wood products from the Solomon Islands, particularly Kolombangara Island. Furthermore, in the right context, these data can also be used to establish whether timber or wood products declared to be from origins other than the Solomon Islands have stable isotope ratios that are consistent with data from the Solomon Islands. If not, this would suggest foreign timber/forest products are from elsewhere and are being passed-off as originating from the Solomon Islands.
Introduction
The Solomon Islands is an archipelago comprising seven major islands: Guadalcanal, Choiseul, Santa Isabel, New Georgia, Malaita, and Makira (or San Cristobal), and a host of smaller ones. They are extensively forested with 88% of the country under forest cover. More than 80% of the country is under high rainforest with the remaining 8% mainly swamp forest, including mangroves, and upland forests. The rainforest has generally fewer timber species than surrounding countries, with around 60 reaching large sizes. The major species harvested are Pometia pinnata, Calophyllum sp., and a mixture of “whitewoods” (FAO and Pauku, 2009).
The Solomon Islands is China’s second largest source of tropical timber by country (after Papua New Guinea) (Smyth, 2018), yet the landmass of the entire country is only twice the size of Beijing. By comparison, the largest source of tropical logs, Papua New Guinea constitutes roughly half of the landmass of New Guinea, the world’s second largest Island at 785,753 km2. The heavy reliance on such a small territory for timber is rapidly depleting the Solomon Islands’ forest cover, biodiversity, and natural resources at rates several NGOs describe as “unsustainable.” If logging continues at its current rate, the island nation is expected to be deforested by 2036 (Rarawa, 2012). Given the magnitude of the situation, there are serious questions considering how this can be happening in a way that is compliant with law. One NGO report presents evidence that the extraction of timber from the Solomon Islands is not happening legally (Global Witness, 2018).
In 2015 over 2.5 million cubic metres of logs were exported from the Solomon Islands (Global Witness, 2018), this is over 10 times the annual sustainable yield. It is difficult to perceive how such a small nation could place such a large volume of timber on the global market. There is speculation that declared imports from the Solomon Islands may have originated elsewhere, such as Papua New Guinea, and that this occurs to launder illegal timber into the global supply. However, declared import and export data for the Solomon Islands do not support this hypothesis (Global Witness, 2018). Unless timber traders who engage in illegal practices are shipping logs to the Solomon Islands for re-export, it seems the major concern is that timber originating from the Solomon Islands is often unsustainably harvested and possibly illegally harvested rather than mislabelled with respect to its geographic origin.
Much of the timber exported from the Solomon Islands is destined to be turned into plywood or other composite products for export and is not necessarily consumed domestically in China. European Union Timber Regulation (EUTR) (Regulation (EU) No 995/2010, 2016) and the Lacey Act (2008) both demand that timber and timber products are obtained legally before they are placed on the respective EU and United States markets. EUTR places penalties against companies that are the first to place timber products on the EU market if they do not enact a due diligence/due care system to ensure the timber is from a legal source. The Lacey Act may be used in criminal and civil cases to enact penalties against violators that place illegally sourced timber on the United States market.
The main islands/regions for logging in the Solomon Islands are: Western Province (includes the islands of Vella Lavella, Kolombangara, New Georgia, Rendova, Vangunu, and Ngatokae), Choiseul Province, Isabel Province, Guadalcanal Province, Malaita Province, and Makira Province (FAO and Pauku, 2009). Kolombangara Island is the base of operations of Kolombangara Forest Products Ltd. (KFPL) and, to date, this is the only Forest Stewardship Council (FSC) certified source of timber within the Solomon Islands. With FSC’s mandatory chain of custody, sustainability requirements, regular audits, GPS mapping of individual trees amongst many other facets, FSC certified timber from KFPL has negligible risk of illegality. This is contrary to timber from other islands within the Solomon Islands which have elevated risks of illegality. Therefore, being able to verify that timber is from Kolombangara Island is a way to demonstrate that the timber is likely legal.
The ambitions of this research were to define the ranges of stable isotope ratios from multiple species of trees from Kolombangara Island by analysing timber samples extracted from living trees. Data from samples collected from origins other than Kolombangara Island allow for the demonstration of differences which is useful to imply further potential. Analysis of the data helps us to:
• Assess what differences are available in the stable isotope ratios of trees from different provinces within the Solomon Islands and outside of the Solomon Islands.
• Utilise these methods to check whether the declared origin of the Solomon Islands timber is consistent with data from the Solomon Islands.
If significant differences in the stable isotope ratios of trees from different provinces within the Solomon Islands are evident, this will be of great benefit to legal investigation teams in demonstrating that seized timber is illegal or at risk of being illegal. If the isotope ratios of trees from within different provinces of the Solomon Islands are relatively homogenous, this would mean that it may not be necessary to collect reference samples from all provinces to establish that the timber is from the Solomon Islands. However, it could mean that to question its legality, further evidence would be required to demonstrate that timber is not from Kolombangara Island.
Stable isotope analysis is a widely accepted analytical discipline and has a long history, with many laboratories adopting the technology around the world. Since the beginning of the 21st century, the technique has become well established as a means of verifying the origin of food and drink (Boner and Förstel, 2004; Kelly et al., 2005; Heaton et al., 2008; Pilgrim et al., 2010; Li et al., 2015) and is established as an analytical technique that can be used in legal cases (Camin et al., 2017). The same principles used to authenticate food were later applied to timber provenance research (Boner et al., 2007; Keppler et al., 2007; Horacek et al., 2009; Kagawa and Leavitt, 2010; Gori et al., 2012, 2018; Rees, 2015; Watkinson et al., 2020). Verifying the origin of timber typically depends on comparing an unknown sample against an authentic reference database for a region or territory. The technique is used routinely to assess legality, compliance with labelling legislation (DEFRA, 2018; Forest Trends, 2019), and its use to conduct due diligence is advocated by EUTR (Regulation (EU) No 995/2010, 2016). The United Kingdom Office for Product Safety and Standards (OPSS) within the Department for Business, Energy and Industrial Strategy (BEIS) use this technique to ascertain the veracity of timber origin claims.
Materials and Methods
Sampling
Kolombangara Forest Products Ltd., provided FSC with a list of species ranked in importance in terms of their own supply and importance to the Solomon Islands. This list was comparable to the list of economically important species published in the Solomon Islands Forestry Outlook (FAO and Pauku, 2009). The top 10 species intended for sampling were a mix of native and introduced trees including:
• Eucalyptus deglupta
• Gmelina arborea
• Campnosperma brevipetiolatum
• Swietenia macrophylla
• Terminalia calamansanai and Terminalia brassii
• Agathis sp.
• Tectona grandis
• Acacia sp.
• Calophyllum inophyllum
• Palaquium sp.
In addition to this, Solomon Islands Law prohibits the export of unprocessed logs of Vitex cofassus, Intsia bijuga, Gmelina moluccana, and Pterocarpus indicus (Australian Government, 2014; Global Witness, 2018) meaning that samples of these trees would also prove valuable.
Sixty-eight samples of timber were collected on Kolombangara Island in August, September, and November of 2019. Twelve samples of timber were also taken from Guadalcanal Island in September and November 2019 for analysis. Samples were collected by trained collectors who were able to identify the species or genus of the tree in the field. Herbarium voucher material was collected for some specimens, but not all.
A Pickering Punch (Agroisolab UK, Welburn, United Kingdom), a type of hammer-driven bore, was used to collect cores of timber 12–18 cm in length and 1.3 cm wide from living trees. Samples were then transferred from the Pickering Punch into cardboard tubes along with silica gel which in turn, were sealed inside 500 ml evacuated bags to aid drying and protect the sample from the humid air in the local environment. August and September samples were collected using 30 g silica gel per plug and no perforations were made in the acid-free cardboard tubes, however, field tests found this did not adequately facilitate drying, and some cores presented with hyphae upon receipt at the Plant Quarantine Unit at the Royal Botanic Gardens, Kew, United Kingdom. Extensive rot is a concern with respect to stable isotope analysis, Filley et al. (2002) demonstrates that brown rot fungi can cause significant differences in the δ13C in lignin and polysaccharides in spruce sapwood over a sufficient timespan, therefore it was necessary to treat samples to prevent unwanted degradation and re-consider collection packs.
Collecting samples of biological material, such as wood, from the tropics without spoilage is challenging due to the humidity and biodiversity of tropical rainforest. Controlling for mould was therefore equally challenging. To prevent mould growth, collection packs were redesigned for the November collection to allow for better drying without the need to recycle the silica gel, this included 30–40 perforations 6 mm in diameter in each cardboard tube and increasing the mass of silica gel to 100 g per plug, as well as drying and replacing/recharging silica gel prior to sample collection. Samples were shipped to the Royal Botanic Gardens at Kew, destined for the World Forest ID (WFID) collection (Gasson et al., 2020), and were held in quarantine pending approval. To eliminate the risk of pathogenic fungi entering the United Kingdom, samples were heated to 121°C at 15 psi for 30 min before they were released into the WFID collection for storage and analysis.
GPS data, photographs of the trees and leaves, descriptions, and comments about the sampled trees were recorded in a mobile phone application named TreeSnap (Crocker et al., 2019; TreeSnap version 1.15.3 Department of Entomology and Plant Pathology, University of Tennessee, Institute of Agriculture, Knoxville, TN, United States). The data from this collection have since been moved to the WFID application (World-ForestID version 1.2.0, Department of Entomology and Plant Pathology, University of Tennessee, Institute of Agriculture, Knoxville, TN, United States). Figure 1 shows the locations of the samples that were collected from Kolombangara Island.
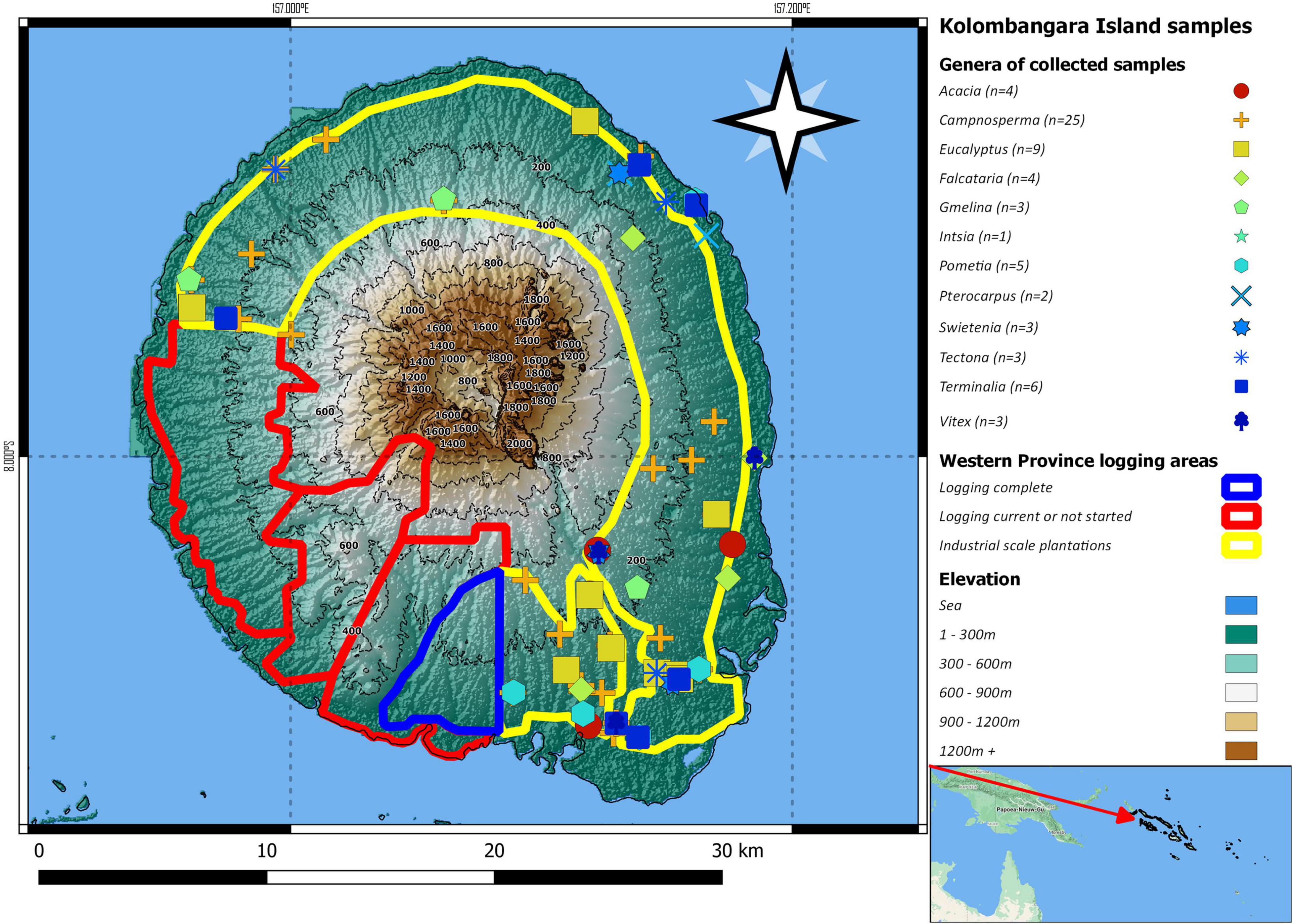
Figure 1. Map of Kolombangara Island with the locations and taxa of samples that were collected during the period September 2019–November 2019. Spatial data sources: Global Administrative Dataset (GADM), ASTER GDEM (ASTER GDEM is a product of METI and NASA), FAO and Pauku (2009), and Google Terrain.
Additionally, this report contains data from: four samples of T. grandis from Papua New Guinea which were collected using an electric drill (Makita BDF 451, Nagoya, Japan) and a 20 cm long 10 mm diameter auger in April 2012 near the Adelbert mountain range, 10 samples of Calophyllum sp. from Kolombangara Island that were collected by KFPL in 2015 as part of an FSC project, 20 samples of Calophyllum sp. from Yang Zai Forestry in southern Taiwan that were collected by FSC in November 2017, two samples of P. pinnata from New Britain (Papua New Guinea, Bismarck Archipelago) in May 2020, and six samples of Pterocarpus soyauxii that were obtained from the Precious Woods Group (PWG) and Compagnie des Bois du Gabon (CBG) concessions in Gabon in June 2019 (Figure 2). Samples from Gabon were collected as part of an effort to define the stable isotope ratios of various species in two FSC concessions and are also evaluated in Watkinson et al. (2021). These data are to be used as a preliminary assessment of differences between geographic origins. At present, the collections of reference samples are not extensive, and any inferences drawn from their evaluation can only be appraised in the context of their current limitations.
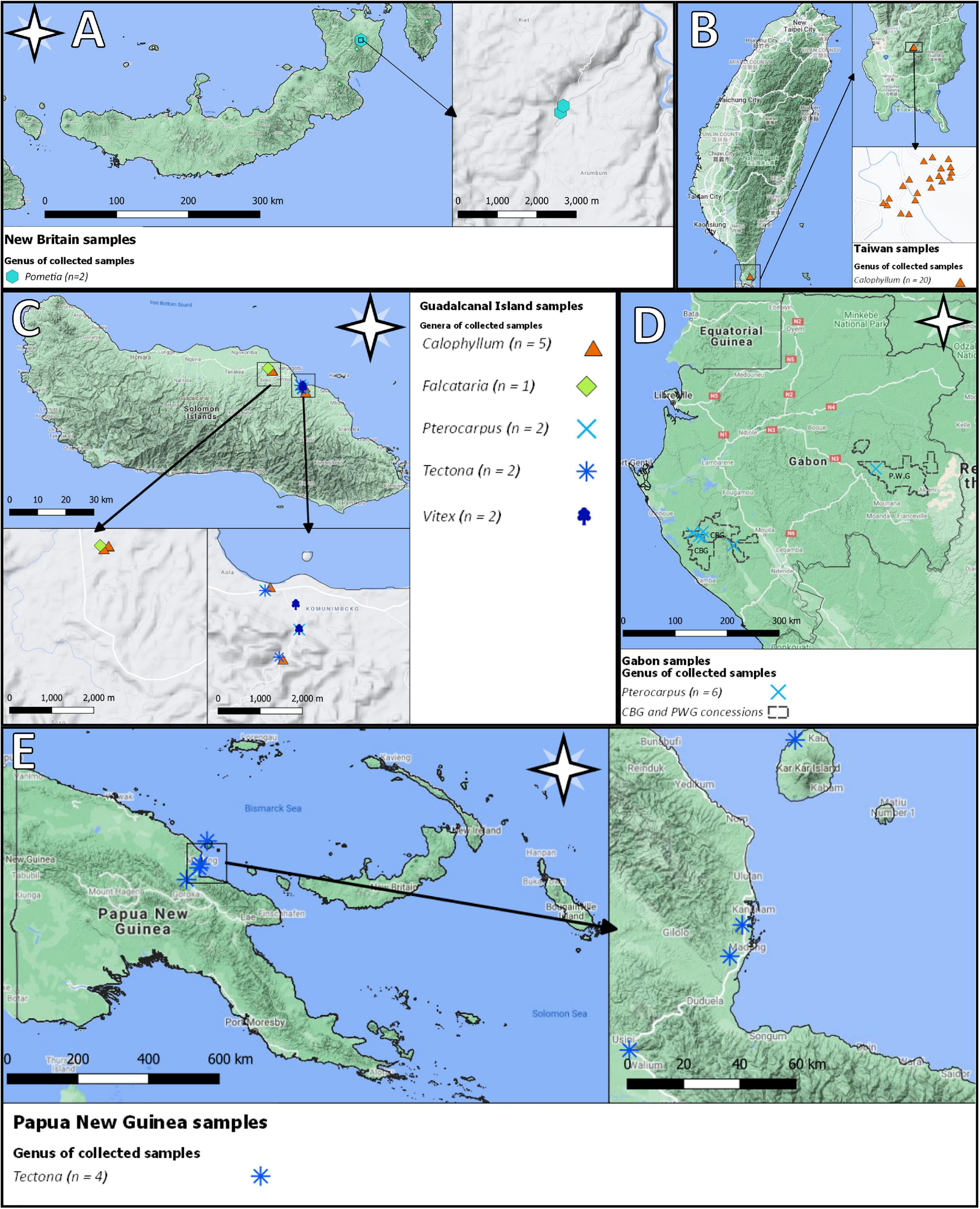
Figure 2. Locations of reference samples in (A) New Britain (Papua New Guinea), (B) Taiwan, (C) Guadalcanal Island (Solomon Islands), (D) Gabon, and (E) Papua New Guinea. Spatial data sources: Global Administrative Dataset (GADM), and Google Terrain.
Measurement
Any samples that were affected by hyphae had the mould removed prior to preparation in accordance with advice in Horacek et al. (2018) and Beeckman et al. (2020). Samples were initially dried at 103°C before grinding/drilling. Samples were drilled using a 7 mm drill bit to the depth of 10–13 cm from the bark side to the pith side to grind them to a coarse powder. All materials were subsequently milled into a fine powder using a ball-mill (Retsch MM220–Haan, Germany). The powder was extracted in a soxhlet apparatus over 6 h with non-polar (dichloromethane) and polar (methanol) solvents which were then dried in a laboratory-type drying cabinet for at least 1 h. Finally, the samples were stored in air-tight sample vials and weighed for analysis.
To avoid equilibration or humidity effects in the oxygen and hydrogen analysis, the weighed-in samples were equilibrated overnight in desiccators with a defined humidity of 10.6%. Afterwards the samples were vacuum dried for at least 2 h.
Sample measurements were corrected against in-house standards at the beginning, middle, and end of each measurement run. In-house standards are traceable back to certified reference materials enabling measurements to be reported relative to an internationally defined standard; for hydrogen and oxygen isotope ratio analysis, Vienna Standard Mean Ocean Water (VSMOW) is used. For carbon isotope ratio analysis, Vienna Pee Dee Belemnite (VPDB) is used. For sulphur isotope ratio analysis Vienna Canyon Diablo Troilite (VCDT) is used. The in-house standards used were 1,4-Dihydroxyanthraquinone (Merch-Schuckhardt) for δ18O and δ2H, L-leucine (Carl Roth GmbH + Co. KG, Karlsruhe, Germany) for δ13C and δ15N, and L-cysteine (Carl Roth GmbH + Co. KG, Karlsruhe, Germany) for δ34S. In-house standards are also controlled in Agroisolab’s free intercomparison testing platform “Agroisolab-KPT” which routinely operates with over 20 isotope laboratories. Measurements are reported in per mil (‰) and were made in accordance with processes outlined in Boner et al. (2007).
δ18O and δ2H measurement was performed using two Isotope Ratio Mass Spectrometers (IRMS) in a master/slave configuration (Isoprime, Elementar, Cheadle, United Kingdom) with each IRMS measuring one isotope ratio; δ18O or δ2H. This configuration provides excellent stability because the magnetic field and accelerating voltage remain constant on each IRMS. The working temperature for pyrolysis is >1530°C and HT-PyrOH is performed with a covalently bonded silicon carbide tube (patented by Agroisolab GmbH, Julich, Germany) filled with glassy carbon chips and coal powder. The mode Combined Uncertainty (uc) for δ18O was 0.2‰ and for δ2H was 1.3‰.
δ13C measurement was performed using an Elemental Analyser (EA3000, Eurovector, Milano, Italy) in combination with IRMS (Nu Horizon, NU-Instruments—Wrexham, Wales). The working temperatures are 1021°C for oxidation and 600°C for reduction. Reduction is carried out in the presence of copper. The mode Combined Uncertainty (uc) for δ13C was 0.1‰.
δ34S measurement was performed using an Elemental Analyser (EA3000, Eurovector, Milano, Italy) with IRMS (Isoprime, Cheadle, United Kingdom). A one tube combustion (oxidation and reduction in one tube) is used to solve issues caused by SO3. Combustion water is directly trapped with magnesium perchlorate at the end of the tube. The working temperature is 1000°C. The mode Combined Uncertainty (uc) for δ34S was 0.2‰.
Data Analysis
As many of the taxa analysed in this project had not been previously analysed by Agroisolab or other laboratories, and the quantities of samples were relatively low, multi-variate analysis methods were not used to help draw conclusions from the data. Instead, univariate analyses were performed to show differences between distributions of means such as Student’s T-test and ANOVA, or to assess co-variance such as regression as well as boxplot visualisations (Microsoft Excel 2016, Microsoft Corporation, Redmond, WA, United States). Three well-sampled taxa (E. deglupta, Terminalia spp., and C. brevipetiolatum) were assessed in SAGA GIS 2.3.2 (Departments for Physical Geography, Hamburg and Göttingen, Germany) for spatially related patterns in their data using variogram analysis (variance/distance) and representing interpolated data using Universal Kriging (Global). This method was used to describe variance/distance related patterns in the data via graphical representation.
Results
In most analysed taxa, oxygen stable isotope ratios ranged between approximately 19.5 and 21.5‰ (Table 1). There are differences in the mean values of the oxygen isotope ratios of the taxa, ANOVA has a low P-value (<0.05) indicating that the differences are significant. However, some results show similar means such as Vitex and Eucalyptus which are not significantly different as the means are nearly identical. Acacia stands out as being significantly enriched in 18O relative to most other sampled timbers in the dataset. The greatest differences in oxygen isotope ratios exist between Acacia, Vitex, and Eucalyptus (2.4‰ difference between means). A wide range of hydrogen stable isotope ratios is evident among the different taxa indicating that there appears to be species effect in the data, i.e., different taxa in the same region may have significantly different stable isotope ratios.
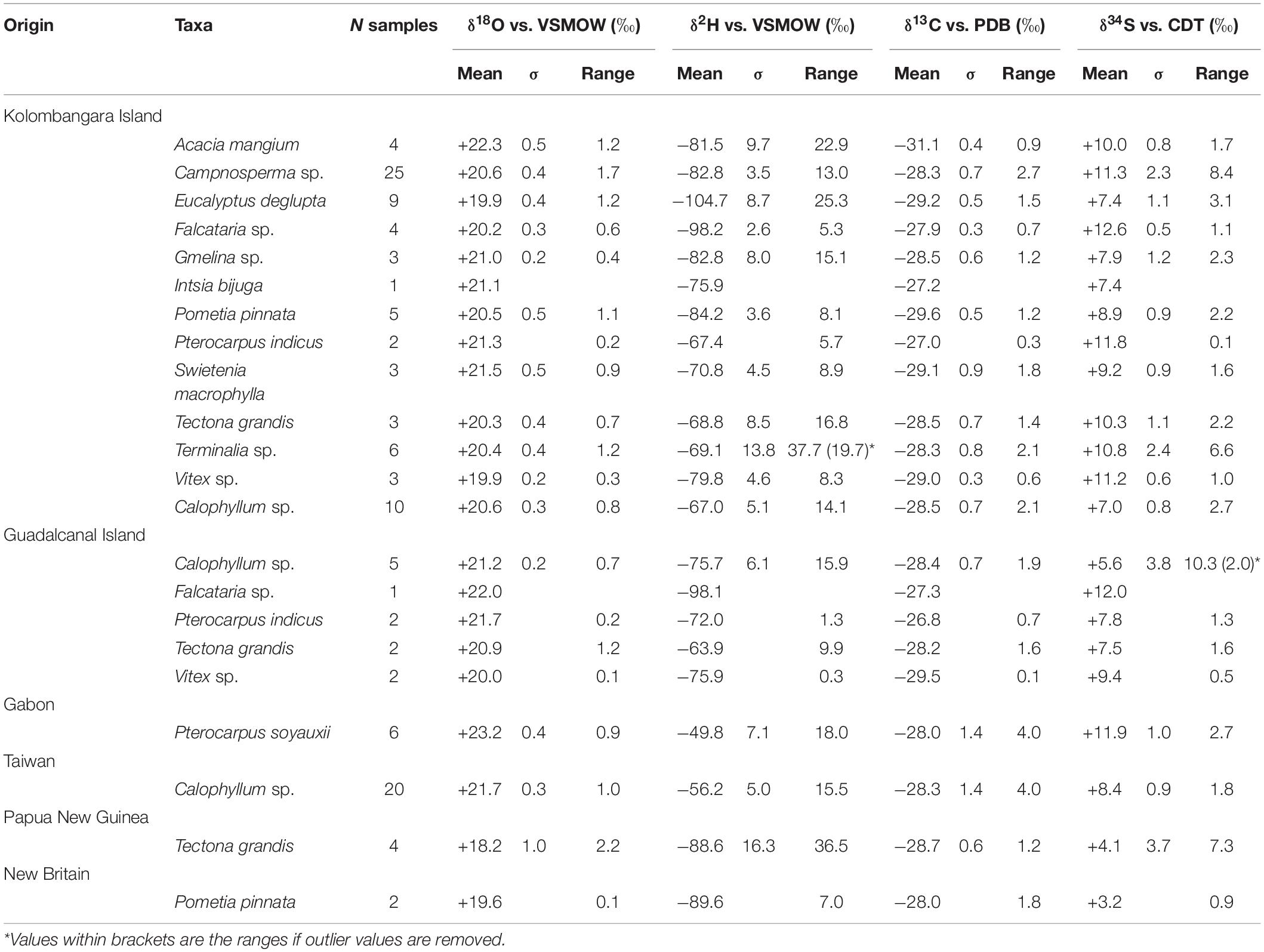
Table 1. Stable isotope ratio data from taxa sampled in the Solomon Islands, Gabon, Taiwan, Papua New Guinea, and New Britain.
Acacia samples from Kolombangara Island had remarkably negative carbon stable isotope ratios in comparison to other analysed taxa from the island. Several ranges of δ13C isotope ratios are evident among the different samples of timber, further supporting the hypothesis that different trees on the same site can have significantly different stable isotope ratios. Reference samples of Acacia show two extremes relative to the dataset, significantly negative δ13C stable isotope ratios and significantly enriched δ18O isotope ratios.
A remarkably wide range of sulphur isotope ratios was evident among the sampled taxa ranging from approximately +6‰ in Calophyllum to +15‰ (11‰ range) in Campnosperma. Even taking the range into context, all samples can be said to be enriched in 34S. The wide ranges of values between different taxa can also be found in the δ2H and δ13C isotope ratios of the samples. ANOVA of Acacia, Pometia, Eucalyptus, Vitex, Calophyllum, Tectona, Terminalia, Campnosperma, and Falcataria δ18O, δ2H, δ13C, and δ34S yielded P values less than 0.05 in all cases and indicates that there is a significant difference between the mean values of each stable isotope ratio of each genus. On its own, this is not conclusive as the sample size for each genus was relatively small but is indicative that different taxa may be expected to have different stable isotope ratio profiles even on an island as small as Kolombangara. It should also be noted that the mean range of sulphur isotope ratios in each genus from Kolombangara Island is not so wide (3.0 ± 2.35‰).
δ18O and δ2H are expected to be well correlated systems where excessive evaporation does not occur Craig (1961). One may reason that this trend should be observable in δ18O and δ2H in wood given that oxygen and hydrogen in timber originate from water. In some sampled taxa this trend is evident, however, in Eucalyptus sp. (Pearson’s R = 0.03), T. grandis (Pearson’s R = −0.40), Falcataria sp. (Pearson’s R = 0.30), and Campnosperma sp. (Pearson’s R = −0.06), this relationship is either weak or not currently evident. This may be due to the low level of sampling, however, 25 samples of Campnosperma sp. were obtained from Kolombangara Island. It is more likely that the relationship cannot be established due to the lack of an adequate range of values being present in the reference samples. To evaluate these relationships further in these taxa, it will be necessary to obtain and analyse more samples from origins that are far from the Solomon Islands (i.e., where different ranges of values are more likely to occur). This may mean sampling timbers from countries where the species is not of concern with respect to legality (e.g., plantation Eucalyptus) to develop better models for evaluation.
Though the values of the stable isotope ratios of the four taxa shown in Figure 3 are different to one another, there appears to be an offset between species in the oxygen and hydrogen stable isotope ratios. This may be of use as it could enable development of statistical models built around a single taxon primarily with lower sampling occurring on other taxa and simply relating the two to one another if this trend is further evaluated. These relationships have been referred to as “Dunbar lines” (Dunbar and Wilson, 1983). If the offset between the taxa can be controlled over a sufficiently wide geographic range and range of values with at least 30 locations per taxa, it should be possible to calculate δ18O and δ2H between different types of wood. This is advantageous as it would alleviate the heavy burden of comprehensively sampling all concerned species in every possible location.
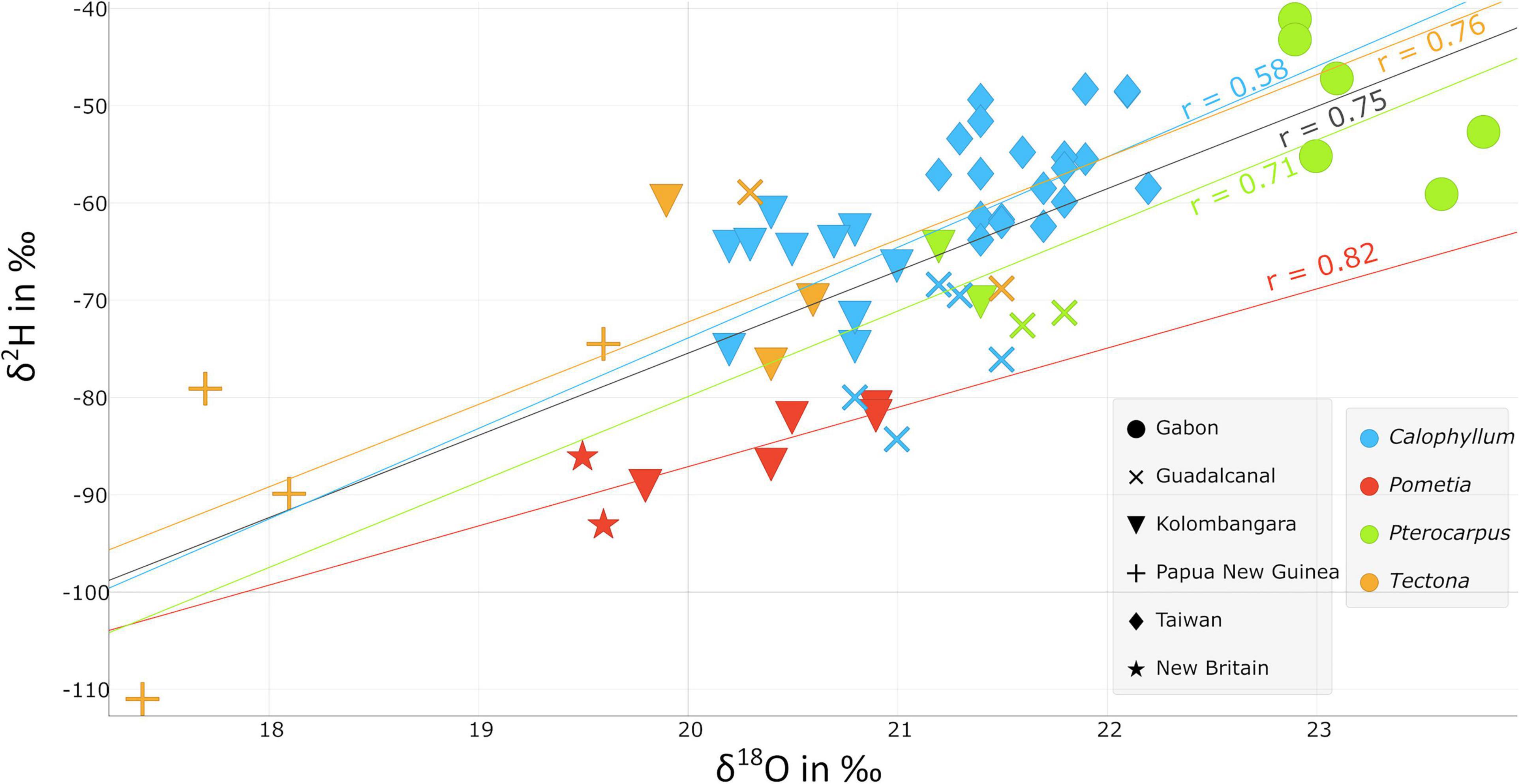
Figure 3. Scatter plots of the δ18O and δ2H stable isotope ratios of Calophyllum spp. (n = 35), Pometia pinnata (n = 7), Pterocarpus spp. (n = 10), and Tectona grandis (n = 9) from the Solomon Islands, Papua New Guinea, Taiwan, and Gabon.
There are significant differences in the δ18O, δ2H, and δ34S stable isotope ratios of Calophyllum from regions that were sampled in Kolombangara Island, Guadalcanal Island, and Taiwan as judged by ANOVA (P < 0.05). This is promising as not only can a differentiation be established between Calophyllum spp. from two countries (Taiwan and Solomon Islands), but the data suggest that there is a chance there may be significant differentiation in timbers from different islands within the Solomon Islands (Figure 4). It would only be possible to discover this trend with further sampling of other islands that are important for the logging industry within the Solomon Islands, however, a method that is able to differentiate timber on an island basis would likely be very useful to support questions of legality on timber products that originated from the Solomon Islands.
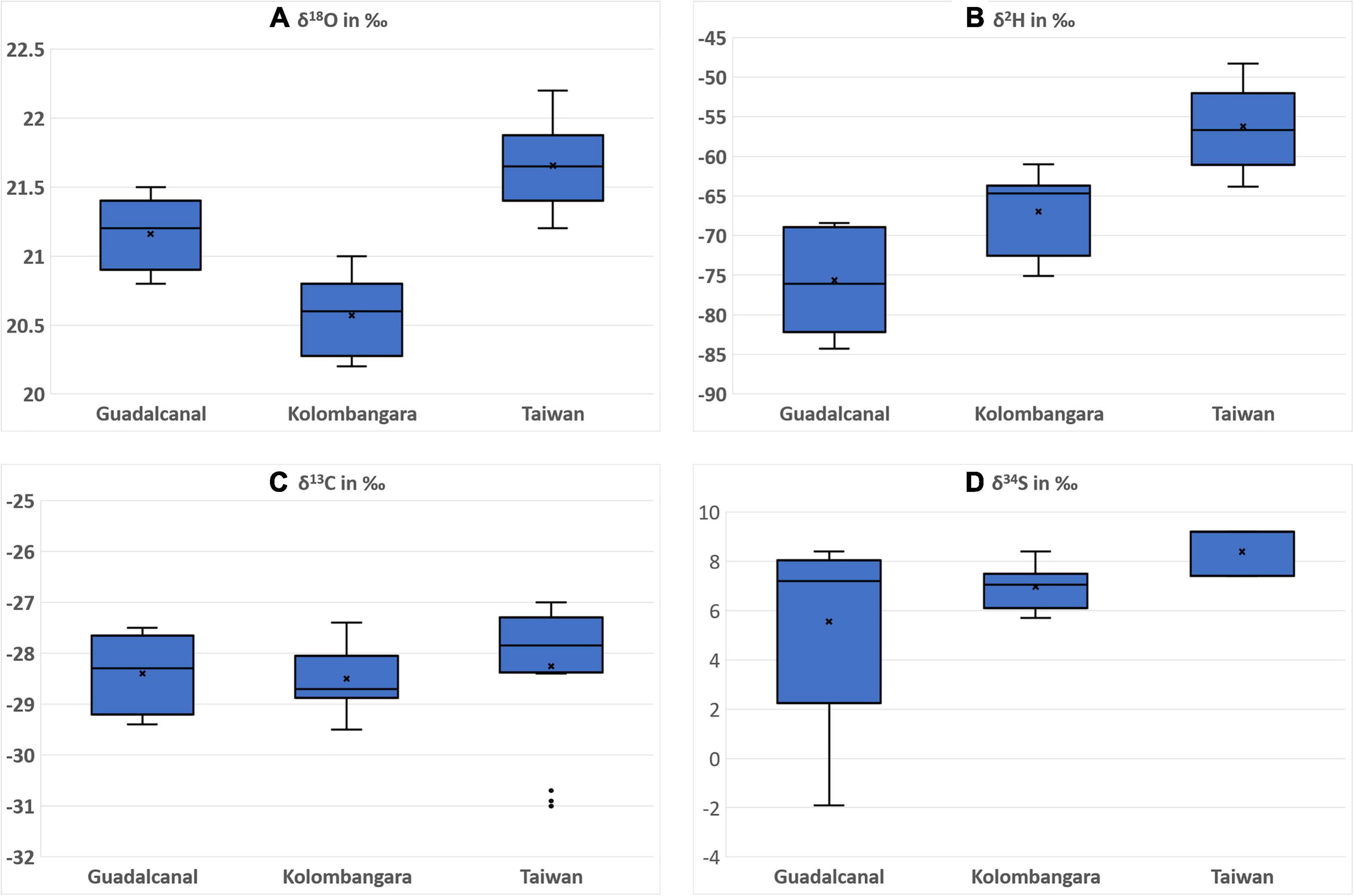
Figure 4. Boxplots of the (A) oxygen stable isotope ratios, (B) hydrogen stable isotope ratios, (C) carbon stable isotope ratios, and (D) sulphur stable isotope ratios of Calophyllum spp. from Guadalcanal Island (SI) (n = 5), Kolombangara Island (SI) (n = 10), and Taiwan (n = 20).
Campnosperma brevipetiolatum Volkens
Campnosperma brevipetiolatum Volkens was the most frequently sampled species in the project with 25 samples collected from different parts of Kolombangara Island. δ18O, δ2H, and δ34S isotope data for Campnosperma sp. have the widest ranges of all sampled taxa. This may be because the data paint a more realistic depiction of the variance that occurs on the island and perhaps should be used as a template in that regard. Figure 5 shows there are very weak trends in the stable isotope ratios of the trees across the island judging by the patterns and ranges evident in the isoscapes. The overall spatial trend in the data as represented by the Universal Kriging models demonstrates that the ranges of δ18O, δ13C, and δ34S are within the Combined Uncertainty (uc). Empirical variogram analysis shows that differences in the values do not appear to be strongly related to distance as regressions of the δ18O and δ2H isotope ratios with the elevation of the samples yield Pearson’s R scores of −0.24 and −0.02, respectively. This means that distance between sampling sites and/or elevation of the sampled trees does not describe much of the observable variation in the Campnosperma data suggesting that the observed variance is due to a combination of random natural variation and measurement uncertainty in Campnosperma sp. from Kolombangara Island. If the variation is due to natural variation, the ranges of values in the δ18O, δ2H, and δ13C isotope ratios of the samples, 1.7, 13, and 2.7‰, respectively, are comparable to ranges of variation in stable isotope ratios between different trees on the same site (intra-site variation) as reviewed by Leavitt (2010). This is of benefit as it illustrates that an n of 25 samples in an area as large as Kolombangara Island appears sufficient to characterise stable isotope variation in accordance with the methods used.
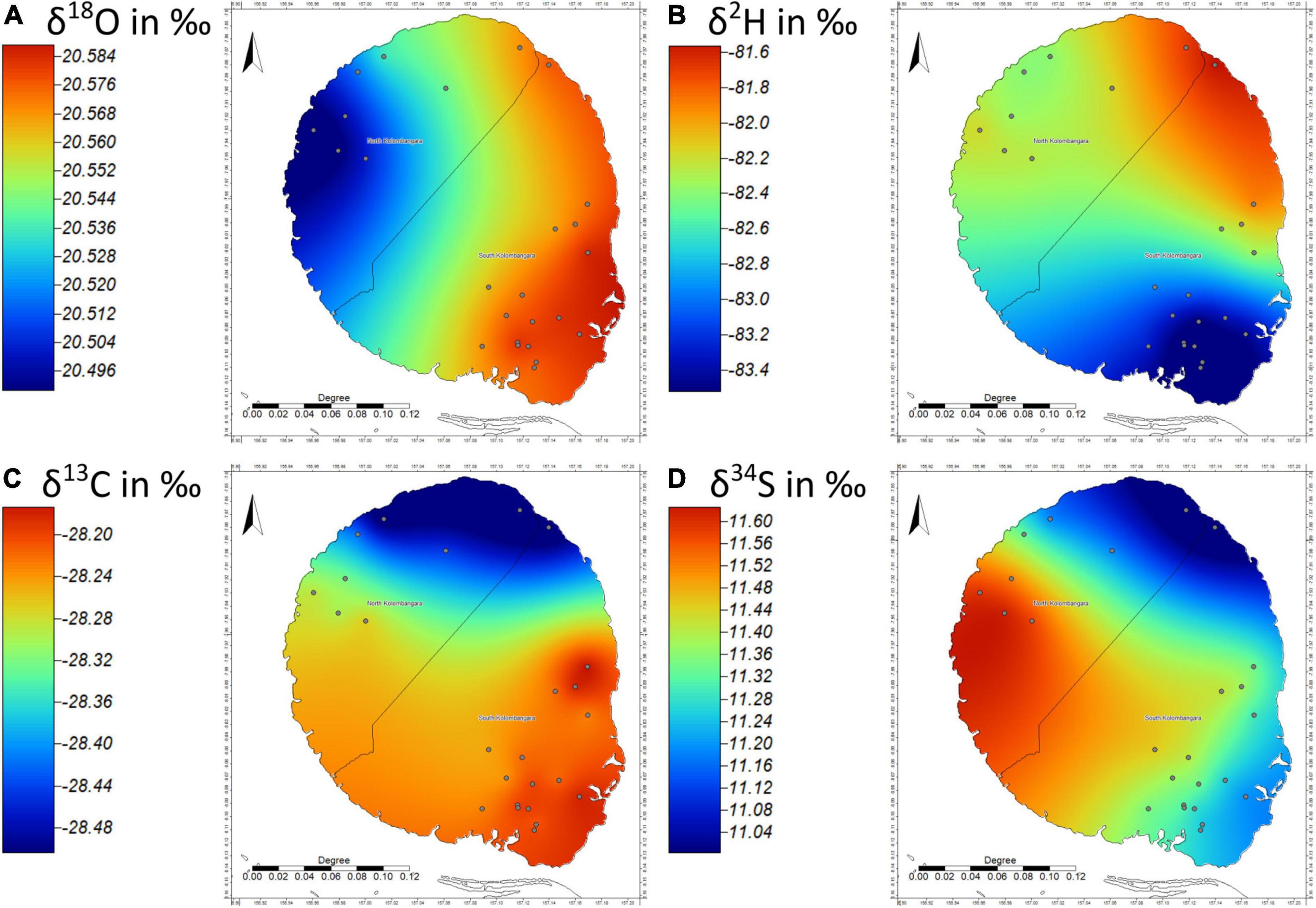
Figure 5. Universal Kriging Isoscapes of (A) δ18O, (B) δ2H, (C) δ13C, (D) and δ34S of Campnosperma brevipetiolatum from Kolombangara Island (n = 25), Solomon Islands. Note that the range displayed in the color scales is within the uc for δ18O, δ13C, and δ34S and is illustrative of a lack of significant difference across the island.
Eucalyptus sp.
Eucalyptus was the third most sampled taxon in this project (n = 9). Overall, Eucalyptus samples had the most negative δ2H isotope ratios (mean = −104.7 ± 8.7‰) and the second most negative δ34S isotope ratios (Figure 6). Empirical Variogram analysis of the Eucalyptus data shows that the distance between samples can explain up to 44% of the variation in the data of the δ2H isotope ratios and 12% in the δ18O isotope ratios. Variation in hydrogen isotope ratios is often linked to elevation (Bowen and Revenaugh, 2003), however, the Pearson’s R for δ18O and δ2H with elevation was −0.29 and −0.47, respectively, suggesting that elevation explains only a small portion of the observable variance in the data. The fact that the Pearson’s R had a negative result in both cases is also surprising as this suggests that elevation and δ2H or δ18O are inversely related on Kolombangara Island. This contrasts with expectations as normally δ2H or δ18O become more negative with increasing elevation rather than becoming more enriched.
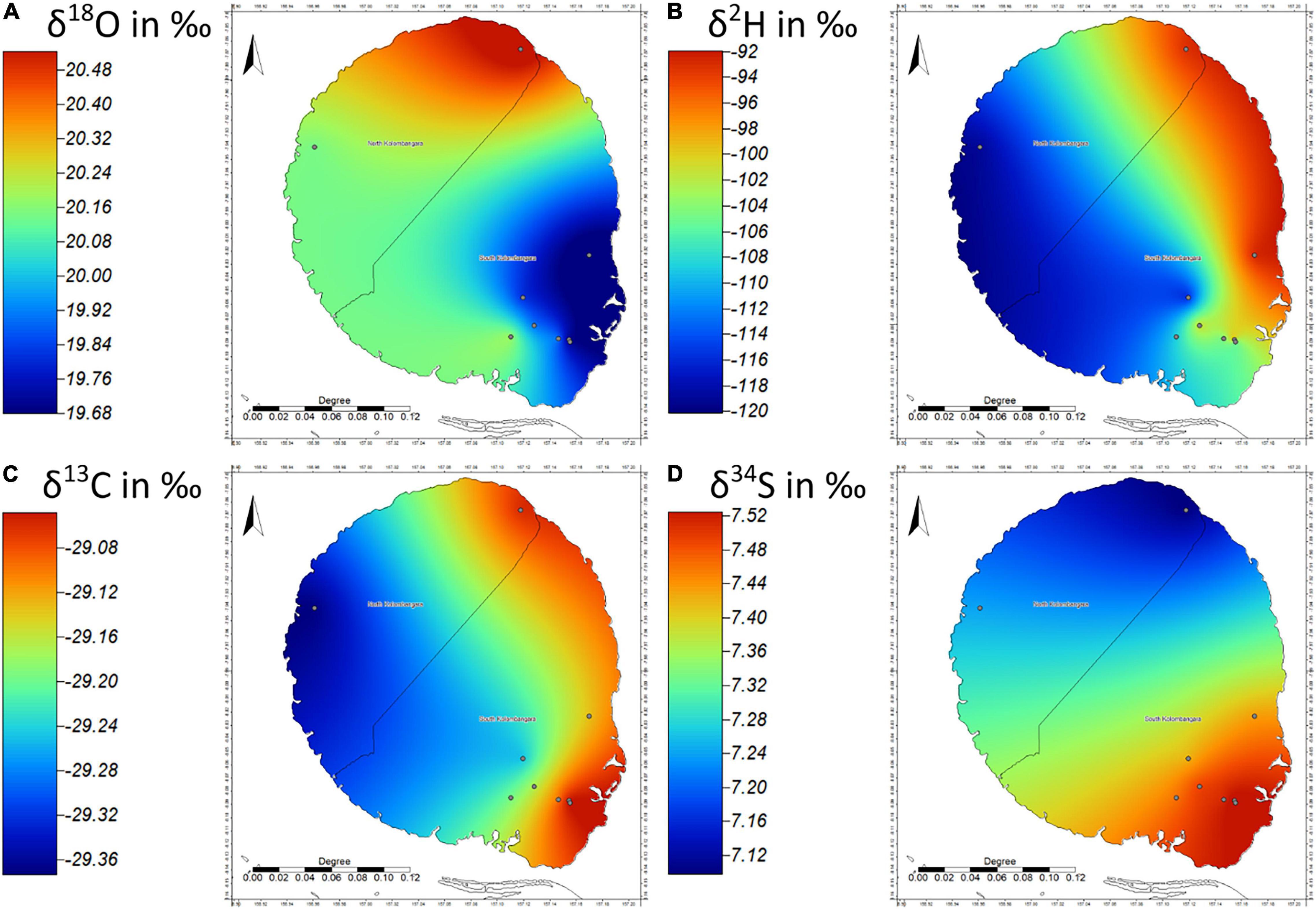
Figure 6. Isoscapes of (A) δ18O, (B) δ2H, (C) δ13C, and (D) δ34S of Eucalyptus sp. from Kolombangara Island (n = 9), Solomon Islands.
Pometia pinnata
The samples of P. pinnata from New Britain are more depleted in 18O and 2H than samples from Kolombangara Island (Figure 7). It does not appear that the reason for this difference is due to the elevation of where the trees grew as the samples from New Britain were collected at approximately 200 m elevation whereas the samples from Kolombangara were collected between 100 and 200 m elevation. The δ18O and δ2H are well-correlated and have a Pearson’s R of 0.82 (Figure 3), this is expected as δ18O and δ2H are correlated in meteoric precipitation. The P. pinnata from New Britain are more enriched in 13C than samples from Kolombangara Island whereas samples from Kolombangara Island are more enriched in 34S than the two samples from New Britain.
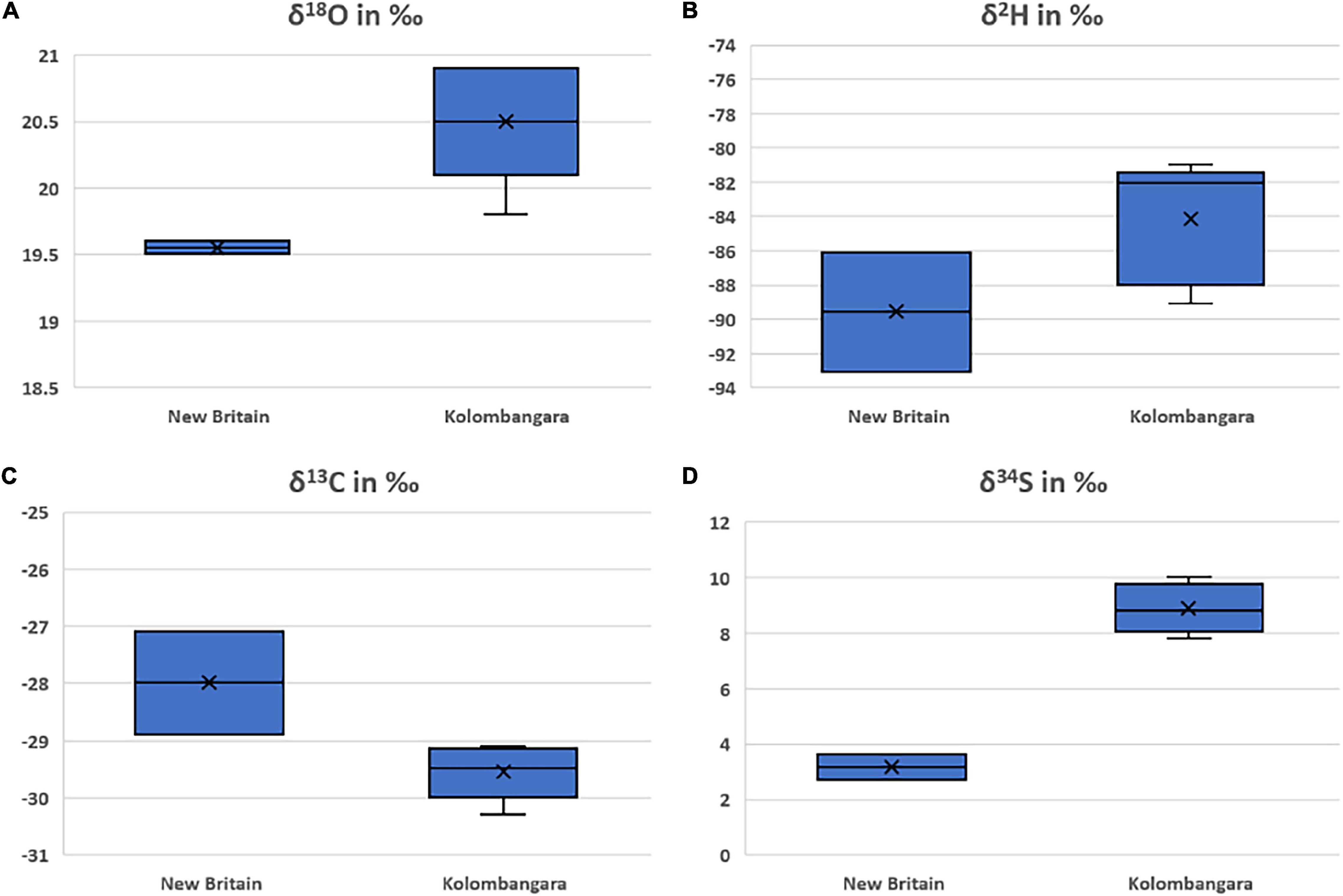
Figure 7. Boxplots of the (A) oxygen stable isotope ratios, (B) hydrogen stable isotope ratios, (C) carbon stable isotope ratios, and (D) sulphur stable isotope ratios of Pometia pinnata from New Britain, Papua New Guinea (n = 2), and Kolombangara Island (SI) (n = 5).
Pterocarpus spp.
Though the overall quantities of Pterocarpus spp. that have been analysed and presented within this report are small, there are significant differences in the δ18O, δ2H, and δ34S (P < 0.05 in all cases) between specimens from locations that were sampled in Kolombangara Island, Guadalcanal Island, and Gabon as judged by ANOVA. This is promising as it demonstrates the potential for differentiation within the Solomon Islands provinces and between different nations that produce Pterocarpus spp. given that it is pantropical and is subject to overharvesting.
Tectona grandis
Significant differences between the δ18O (P < 0.05) and δ2H (P < 0.05) isotope ratios of T. grandis from Guadalcanal Island, Kolombangara Island, and Papua New Guinea are evident (Table 1). This result is promising for future sampling and to be able to address the question of whether exported/imported timber from the Solomon Islands is truly from its declared origin, or whether the timber is from another origin that is a producer of Teak (e.g., Papua New Guinea, Myanmar, and Indonesia). The question of origin is especially important for T. grandis as it is one of the most widely used tropical hardwoods in plantations around the world.
Terminalia sp.
Terminalia sp. was the third most sampled timber from Kolombangara Island (n = 6) and thus was subjected to variogram analysis to investigate spatial patterns in the data. Empirical Variogram analysis shows that an insignificant (<1%) amount of variance of the data for all analysed stable isotope ratios can be explained by the distance between sampling sites. This is also evident in Figure 8 as there appears to be very little in terms of the range of values of the stable isotope ratios across the island with the exception of the δ34S (Figure 8D). In this case the Empirical Variogram shows the distance between samples explains 0.13% of the variance in δ34S; this appears to contradict the north to south trend evident in Figure 8D. The reason for the insignificant correlation is that the sample on the north-eastern edge of the island is an outlier relative to the other samples and the Empirical Variogram treats it as such. This suggests that there is either no strong spatial structure to the data for Terminalia sp. on Kolombangara Island, the variance is random, or that there were too few samples to adequately describe the variance across the island. Given the atypical Empirical Variogram result it may be a combination of factors. The ranges for the δ18O, δ2H, δ13C, and δ34S isotope ratios are 1.2, 19.7‰ (after removal of an outlier result), 2.1, and 6.6‰, respectively. The ranges of carbon, oxygen, and hydrogen stable isotope ratios are comparable to the ranges investigated in a multi-species review by Leavitt (2010). Kolombangara Island could be considered as a single “site” as the variation of the stable isotope data is in the natural range and there is no strong spatial structure evident at this time (Figure 8). However, the presence of the outlier could suggest the island may be considered as several sites, though the lack of spatial structure to the data does not strongly support this hypothesis. Further sampling of Terminalia in the region of the outlier sample may answer this question.
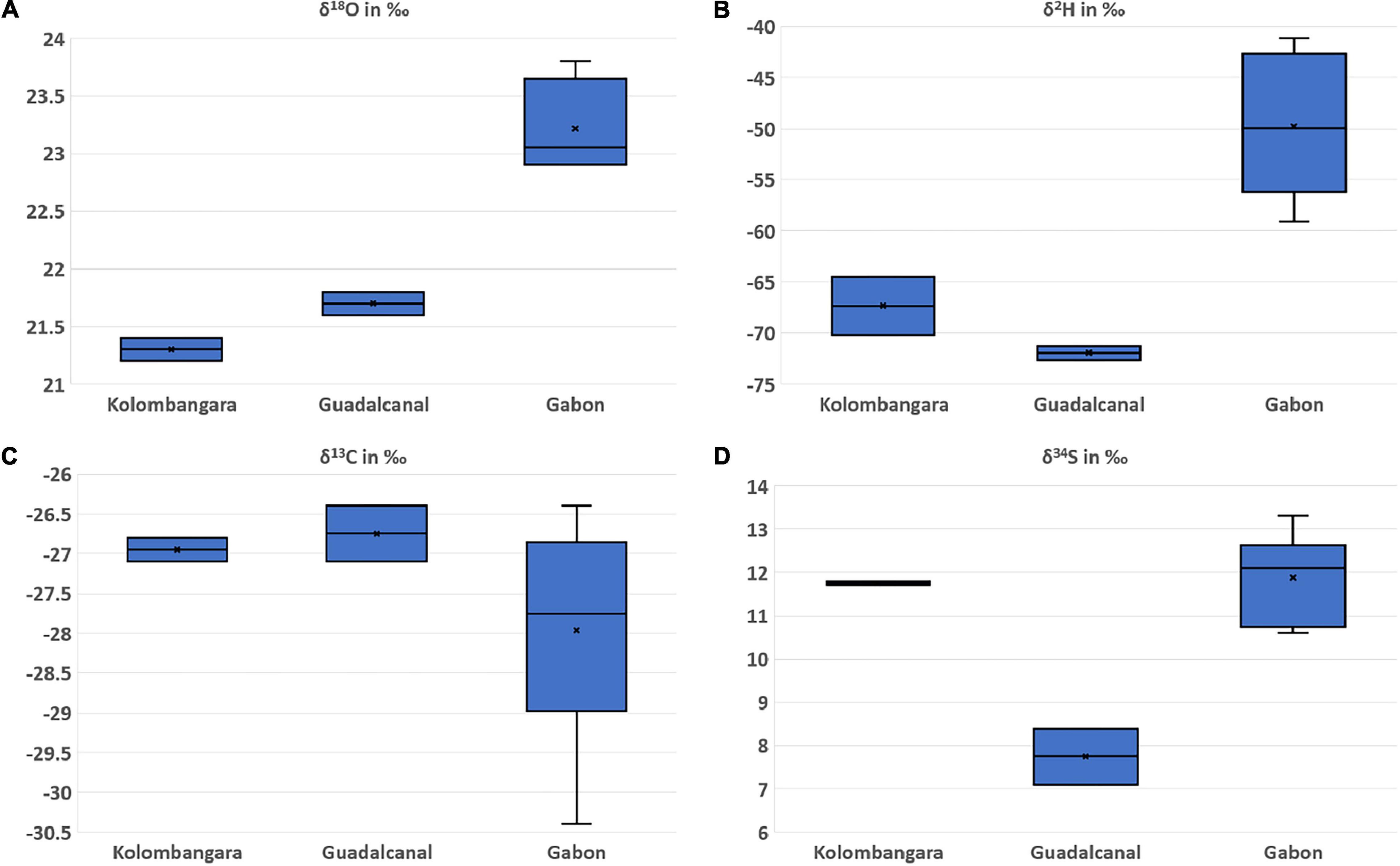
Figure 8. Boxplots of the (A) oxygen stable isotope ratios, (B) hydrogen stable isotope ratios, (C) carbon stable isotope ratios, and (D) sulphur stable isotope ratios of Pterocarpus spp. from Kolombangara Island (SI) (n = 2), Guadalcanal Island (SI) (n = 2), and Gabon (n = 6).
Vitex sp.
Though few samples of this genus were obtained and analysed, there are no significant differences in the δ18O, δ2H, and δ13C isotope ratios of Vitex sp. between Kolombangara and Guadalcanal Islands. Only the δ34S isotope ratios show significant differences between the two origins, with the samples from Kolombangara showing significantly more enrichment in 34S (Student’s T-test P < 0.05) than the samples from Guadalcanal Island. This may be meaningful as other taxa sampled in this project did show significant differences in the δ18O, and δ2H isotope ratios between the two islands (e.g., Calophyllum sp., P. indicus, and T. grandis).
Discussion
Though some taxa have been minimally sampled, we believe it important to include their data so that others may have access to it for use in further dendroprovenancing research using stable isotope analysis. Sampling in tropical regions comes with many challenges, rain makes it hard to collect dry samples, biodiversity is high and important trees are often difficult to find (e.g., P. indicus), tropical wood is often dense, and it is hard to collect many samples in a short space of time. Despite all of this, a great many samples were collected overall, and we hope to build on their data over time throughout the WFID project with this piece of research laying the foundation for data, ideas, and improvements.
We believe that stable isotope data from Campnosperma and Calophyllum characterise Kolombangara Island well. Eucalyptus is characterised well-enough for evaluative purposes, but we are interested in gaining more samples from different parts of the island if Eucalyptus can be found in all areas of interest to further investigate the spatial trend that was observed. An n of 6 for Terminalia, given the outlier we observed, is close to the minimum for characterisation and it may be necessary to either explore the outlier sample more or eliminate it from the dataset. These findings are in keeping with guidelines from the Leavitt (2010) review which shows “sampling 4–6 trees at a site while avoiding juvenile effects in rings near the pith” is often sufficient for characterising site variability. However, the Leavitt (2010) review considers stable isotope variation in sampling sites for the purposes of characterising tree-ring variability and can involve taking many more measurements per tree than in this research. The value of 4–6 trees at a site from studies used by Leavitt (2010) is based on using Expressed Population Signal (EPS) calculations which are prone to misinterpretation according to Buras (2017). EPS is often misunderstood to be a measure for the suitability of tree ring data for climate reconstruction, whereas according to Buras (2017, p. 2) “In theory, EPS is the amount of variance of a population chronology (infinitely replicated) explained by a finite subsample.” Therefore, it will be necessary to produce a more appropriate system for evaluating site variability and characterisation that is directly applicable to the methods presented in this research rather than rely on criteria outlined by Leavitt (2010).
Significant differences were found in numerous parameters of stable isotope data of multiple taxa between islands in the Solomon Islands (Calophyllum sp., Pterocarpus sp., T. grandis, and Vitex sp.). Though the overall quantities of each sampled taxa were relatively low, they were enough to establish ranges of values that can be used for the future evaluation of samples declared to have originated from the Solomon Islands. What is particularly promising is if the trend in the data collection continues, reliable island-level differentiation may be possible if more islands are sampled in the Solomon Islands archipelago. The results demonstrate that there is now a way to perform an evaluation to check whether a sample of FSC timber from the Solomon Islands (which must originate from Kolombangara Island) is from Kolombangara Island or not. Should a tested sample be inconsistent with data from Kolombangara Island, this could indicate that Kolombangara Island was not the origin of the timber and therefore should be subject to further scrutiny.
Differences in the ranges of stable isotope ratios were also evident in different taxa on the same site (assuming Kolombangara Island can be treated as a single site from a stable isotope perspective). On one hand this is disadvantageous because it highlights the necessity to sample as many different taxa as possible to defend any given origin and therefore will increase the cost of projects aimed at protecting the origin of taxa. On the other hand, four taxa (Calophyllum spp., Pometia sp., Pterocarpus spp., and T. grandis) show relationships in their δ18O and δ2H isotope ratios that can be compared to one another. To investigate this further, sampling 10 different taxa in the same site in 30 global locations would allow investigation into these relationships. A practical use of this information would be to establish a “Master” taxon for sampling that other taxa can be compared to in a model on a global scale.
Though there is a wide range in the δ34S of samples collected on Kolombangara Island (11‰) all samples were enriched in 34S. The sulphur in the timber is expected to have originated from soil sulphur sources which in turn are expected to have originated from the volcano. Little has been published on the geology of the Solomon Islands, let alone Kolombangara Island save for “The British Solomon Islands Geological Record 1957 – 1958” (The Government of The British Solomon Islands, 1960) which reduces the ability for detailed interpretation of sulphur isotopes somewhat. Studies related to volcanic rocks have found that volcanic rocks are enriched in 34S relative to CDT (or VCDT) and ocean floor basalts (Sakai et al., 1982; Ueda and Sakai, 1984) which appears to be consistent with the findings from the Kolombangara Island samples.
Only one of the three investigated taxa (Eucalyptus sp.) showed any spatially predictable trends in variance within Kolombangara Island (Figure 9). While this is useful to make the case that a 30 km diameter area in the Solomon Islands can be considered as a single site for classification, the trends in Eucalyptus should be further investigated. It may be possible that the differences in Eucalyptus on the island can be explained by random chance, or it may be that a prevailing wind may influence the isotopic composition of precipitation even within such a small area. Climate reports for the island state that the northern side of the island is more sheltered whereas the southern side of the island is more exposed. Whitmore (1969) also documented that there is a “10 cm increase in rainfall for every 30 m increase in elevation up to 1500 m” on Kolombangara Island (likely meaning a 100 mm increase in annual rainfall for every 30 m increase in elevation). Gat et al. (2003) discusses that air-sea interactions near the coast in conditions where there is a deficit in humidity can result in a large deuterium-excess. It is possible that the gradient in wind and precipitation is responsible for the difference in stable isotopic composition of Eucalyptus sp. across the island. However, it is also worth considering that while the 25 samples of C. brevipetiolatum that were analysed did not show a spatially predictable trend across the island, the pattern in δ2H is similar across the island (Figure 5B) and may contribute to supporting the prevailing wind and precipitation hypothesis. This poses further questions, for example, why is the range in δ2H only 13‰ for Campnosperma whereas it is 25.3‰ for Eucalyptus? Both woods are diffuse porous and can lack distinct growth ring boundaries. However, C. brevipetiolatum Volkens has 5–20 and 20–40 vessels/mm2 whereas E. deglupta Blume has 5–20 vessels/mm2 suggesting that water may move in greater rates in C. brevipetiolatum, though E. deglupta has vasicentric tracheids which conduct water albeit at lower rates. If the Peclet effect, the mixing of water from xylem and evaporative sites as a place for isotopic fractionation (Farquhar and Lloyd, 1993; Ferrio et al., 2011), is to be considered, these are important considerations for explaining differences in the isotopic composition of cellulose in the two types of tree. Given that there appear to be some differences in the ability of each tree to supply water to its leaves, this may explain some of the differences that have been observed. However, a greater difference between the trees appears to be in terms of the size of the leaves. E. deglupta leaves are approximately 7.5–20 cm long by 5.7–10 cm wide (Chippendale, 1972; Soerianegara and Lemmens, 1993), whereas C. brevipetiolatum leaves are much larger being over 30 cm long and up to 17.5 cm wide (Havel, 1963; Soerianegara and Lemmens, 1993). Perhaps the difference in size of the leaves also reflects that one is less susceptible to changing its stomatal conductance than the other and may explain why one tree has a more homogenous isotopic composition than the other on the same island. Further investigation into this topic would yield results that may greatly inform how the differences in isotopic composition of different taxa can be calculated or considered.
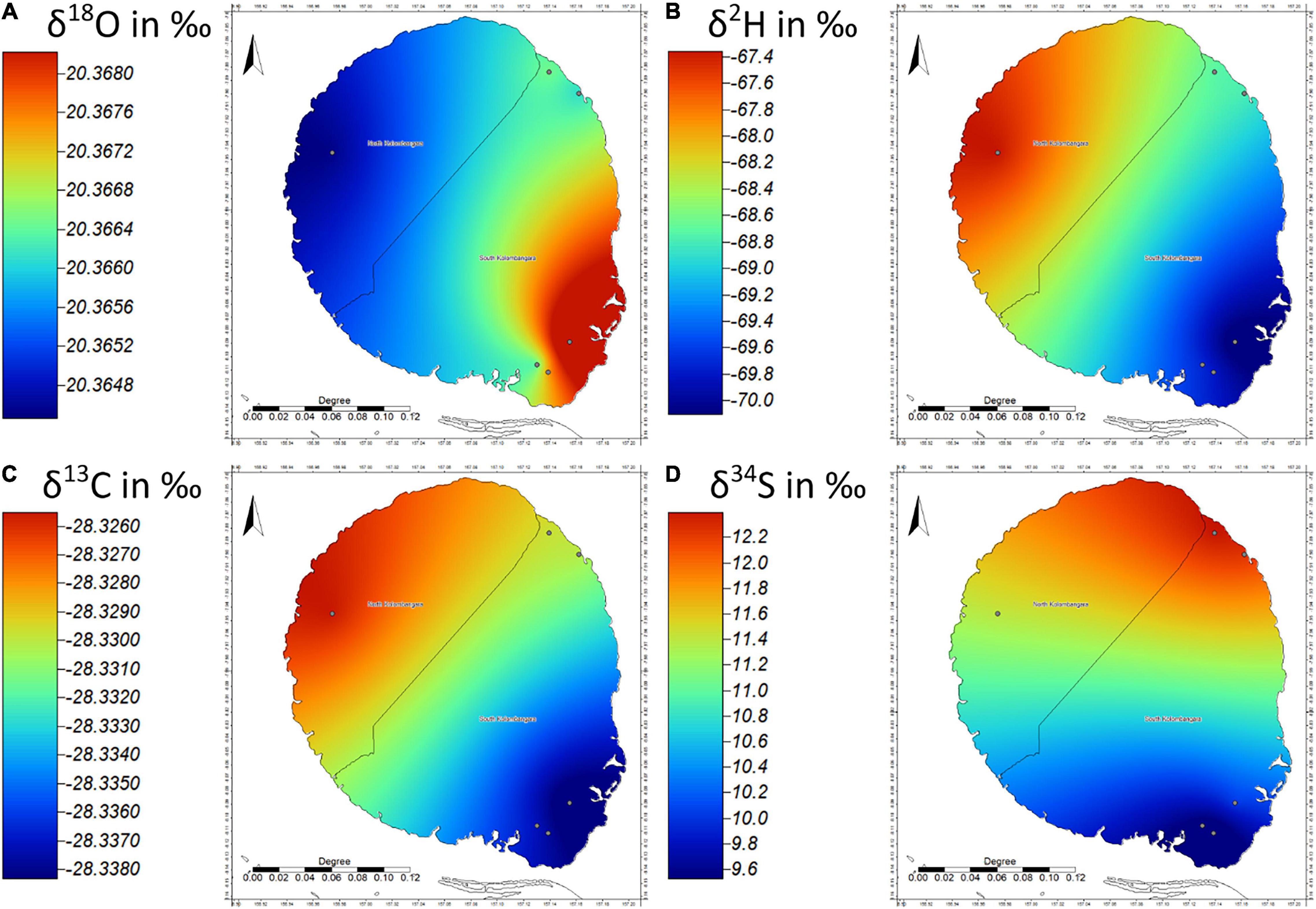
Figure 9. Isoscapes of (A) δ18O, (B) δ2H, (C) δ13C, and (D) δ34S of Terminalia sp. from Kolombangara Island (n = 6), Solomon Islands. Note that the apparent range in δ18O and δ13C are within the range of Combined Uncertainty and exhibit no practical difference across the island.
The method of homogenising a core for analysis is appropriate to real-world testing of timber products. Homogenisation of multiple years of growth of a sample creates a robust mean value that is not heavily influenced by years that may have contained climatic anomalies (Leavitt, 2010). As all measured stable isotope ratios can be influenced by climate in some manner, this must be considered. A large proportion of the logs that are exported from the Solomon Islands to China are manufactured into plywood. As the veneers in plywood are created by rotary peeling of logs, a veneer sample subject to origin analysis may be influenced by climatic anomalies. However, as the data from the core reference sample represents this, the range of values in the reference samples should adequately cover this. However, one disadvantage to this approach appears to be that a degree of information is lost in the homogenisation of the sample and manifests only in terms of model uncertainty. δ13C stable isotope ratios appear to be the most affected by this compromise; Kagawa and Leavitt (2010) demonstrated that high-level resolution of geographic origin assignment is achievable if multiple ring-widths of timber are subject to δ13C stable isotope ratio analysis. δ13C stable isotope ratios are most significantly influenced by water-stress in C3 plants (Farquhar et al., 1989), therefore, building up a profile of years of variation in local climatic conditions related to water-stress can allow for excellent potential for origin assignment. Nevertheless, the disadvantage of this method is that one must know the year corresponding to the ring width of the test sample to make best use of this method for origin assignment. In plywood, or even sawn timber, the way to obtain this information does not seem obvious, hence the compromised utility of carbon isotope ratio analysis that is put forward in this project. The approach used here may be limited in some terms but is designed for practical use.
However, further work to validate the ability of these ranges to verify Kolombangara Island provenance is advisable. Good practice in data analysis often involves building datasets that are large enough to be partitioned into “training” and “test” sections so that an evaluative model can be constructed using the “training” dataset with its performance being assessed by techniques such as k-fold or leave one out cross validation (LOOCV), then subsequently validated using the “test” portion of the dataset. It may also be advisable to collect further samples of known origin and perform a “blind-test” evaluation. This case study has set out to establish a basis for evaluating origin claims of timber from the Solomon Islands using stable isotope analysis and hopefully further work will permit validations of these sorts to be performed.
Data Availability Statement
The raw data supporting the conclusions of this article will be made available by the authors, without undue reservation.
Author Contributions
CW was responsible for data analysis and much of the introduction and discussion. GR was responsible for quality checking the interpretation and the introduction. SH and LM were jointly responsible for sample preparation and data preparation. PG was responsible for discussing the relationship between wood anatomy and the stable isotope results. MB was responsible for the scientific methods, data analysis, and overall management of the project. All authors contributed to the article and approved the submitted version.
Funding
We gratefully acknowledge funding via The US Department of State, US Department of Agriculture, and Defra-ICF: Building and Enhancing the Evidence Base for Nature Based Solutions to Climate Change. Project 29084. Driving innovation in forest protection and enforcement monitoring. Tackling illegal logging: creating a timber reference library to support enforcement (World Forest ID at Kew).
Conflict of Interest
CW and GR were employed by company Agroisolab UK Ltd., Welburn, United Kingdom. SH, LM, and MB were employed by company Agroisolab GmbH.
The remaining author declares that the research was conducted in the absence of any commercial or financial relationships that could be construed as a potential conflict of interest.
Publisher’s Note
All claims expressed in this article are solely those of the authors and do not necessarily represent those of their affiliated organizations, or those of the publisher, the editors and the reviewers. Any product that may be evaluated in this article, or claim that may be made by its manufacturer, is not guaranteed or endorsed by the publisher.
Acknowledgments
A very deep and heartfelt thanks to the following, without whom this work would not have been possible: Elizabeth Lebow, Alex Moad, and their respective colleagues at the United States Forest Service for overseeing the World Forest ID project. FSC, Phil and Tatai Guillery for collecting samples in the Solomon Islands. FSC, Emily Crumley and Cynel Moundounga for collecting samples in Gabon. Dan Raymond, Wilson Tiubule, KFPL and the communities of Kolombangara and North East Guadalcanal for collecting samples and supporting collection efforts in the Solomon Islands. Meaghan Parker-Forney and her respective colleagues at the World Resources Institute for overseeing the World Forest ID project. Jade Saunders of Forest Trends for her advisory and coordination support to the World Forest ID project. Isabella Miles-Bunch and Sara Redstone at the Royal Botanic Gardens at Kew for assisting with sample shipment documentation, receipt of samples in quarantine and their expert help in verifying declared information of field collectors. Tim Fox, Jackie Borrows, and Matt Watkinson for spending hundreds of collective hours assembling sample collection packs. Agroisolab GmbH staff for spending hundred’s of collective hours preparing and measuring samples. Meg Staton, Abdullah Almsaeed et al. for developing the WorldForestID app which tracks samples from the field through Kew to analysis labs.
Supplementary Material
The Supplementary Material for this article can be found online at: https://www.frontiersin.org/articles/10.3389/ffgc.2021.645222/full#supplementary-material
References
Australian Government (2014). Country Specific Guideline for Solomon Islands. Canberra, ACT: Australian Government Department of Agriculture.
Beeckman, H., Blanc-Jolivet, C., Boeschoten, L., Braga, J. W. B., Cabezas, J. A., Chaix, G., et al. (2020). Overview of Current Practices in Data Analysis for Wood Identification A Guide for the Different Timber Tracking Methods, ed. N. Schmitz (Joensuu: Global Timber Tracking Network). doi: 10.13140/RG.2.2.21518.79689
Boner, M., and Förstel, H. (2004). Stable isotope variation as a tool to trace the authenticity of beef. Anal. Bioanal. Chem. 378, 301–310. doi: 10.1007/s00216-003-2347-6
Boner, M., Somner, T., Erven, C., and Förstel, H. (2007). “Fingerprinting methods for the identification of timber origins,” in Proceedings of the International Workshop October 8–9, 2007, Bonn/Germany, (Braunschweig: Johann Heinrich von Thünen-Institut).
Bowen, G. J., and Revenaugh, J. (2003). Interpolating the isotopic composition of modern meteoric precipitation. Water Resour. Res. 1299:39. doi: 10.1029/2003WR002086
Buras, A. (2017). A comment on the expressed population signal. Dendrochronologia 44, 130–132. doi: 10.1016/j.dendro.2017.03.005
Camin, F., Boner, M., Bontempo, L., Fauhl-Hassek, C., Kelly, S. D., Riedl, J., et al. (2017). Stable isotope techniques for verifying the declared geographical origin of food in legal cases. Trends Food Sci. Technol. 61, 176–187. doi: 10.1016/j.tifs.2016.12.007
Chippendale, G. M. (1972). Available online at: http://specimens.kew.org/herbarium/K000279434 (accessed December 22, 2020).
Craig, H. (1961). Isotopic variations in meteoric waters. Science 133, 1702–1703. doi: 10.1126/science.133.3465.1702
Crocker, E., Condon, B., Almsaeed, A., Jarret, B., Dana Nelson, C., Abbott, A. G., et al. (2019). TreeSnap: a citizen science app connecting tree enthusiasts and forest scientists. Plants People Planet 2, 47–52. doi: 10.1002/ppp3.41
DEFRA (2018). International Forestry Team, PB14501 Timber and Timber Products (Placing on the Market) Regulations 2013 Post Implementation Review §. London: DEFRA.
Dunbar, J., and Wilson, A. T. (1983). Oxygen and hydrogen isotopes in fruit and vegetable juices. Plant Physiol. 72, 725–727. doi: 10.1104/pp.72.3.725
FAO, and Pauku, R. L. (2009). ASIA-Pacific Forestry Sector Outlook Study II Solomon Islands Forestry Outlook Study §Working Paper Series. Working Paper No: APFSOS/WP/27. Bangkok: FAO.
Farquhar, G. D., Ehleringer, J. R., and Hubick, K. T. (1989). Carbon isotope discrimination and photosynthesis. Annu. Rev. Plant Physiol. Plant Mol. Biol. 40, 503–537. doi: 10.1146/annurev.pp.40.060189.002443
Farquhar, G. D., and Lloyd, J. (1993). “Carbon and oxygen isotope effects in the exchange of carbon dioxide between terrestrial plants and the atmosphere,” in Stable Isotopes in Plant Carbon-Water Relations, eds J. R. Ehleringer, A. E. Hall, and G. D. Farquhar (San Diego, CA: Academic Press), 47–70. doi: 10.1016/b978-0-08-091801-3.50011-8
Ferrio, J. P., Pou, A., Florez-Sarasa, I., Gessler, A., Kodama, N., Flexas, J., et al. (2011). The péclet effect on leaf water enrichment correlates with leaf hydraulic conductance and mesophyll conductance for CO2. Plant Cell Environ. 35, 611–625. doi: 10.1111/j.1365-3040.2011.02440.x
Filley, T. R., Cody, G. D., Goodell, B., Jellison, J., Noser, C., and Ostrofsky, A. (2002). Lignin demethylation and polysaccharide decomposition in spruce sapwood degraded by brown rot fungi. Org. Geochem. 33, 111–124. doi: 10.1016/s0146-6380(01)00144-9
Forest Trends (2019). Meeting Summary: Timber Regulation Enforcement Exchange. London: Forest Trends.
Gasson, P. E., Lancaster, C. A., Young, R., Redstone, S., Miles-Bunch, I. A., Rees, G., et al. (2020). WorldForestID: addressing the need for standardized wood reference collections to support authentication analysis technologies; a way forward for checking the origin and identity of traded timber. Plants People Planet 3, 130–141. doi: 10.1002/ppp3.10164
Gat, J. R., Klein, B., Kushnir, Y., Roether, W., Wernli, H., Yam, R., et al. (2003). Isotope composition of air moisture over the Mediterranean Sea: an index of the air-sea interaction pattern. Tellus B Chem. Phys. Meteorol. 55B, 953–965. doi: 10.3402/tellusb.v55i5.16395
Global Witness (2018). Paradise Lost: How China Can Help the Solomon Islands Protect its Forests. Available online at: https://www.globalwitness.org/documents/19474/Paradise_Lost_English_Final_Report.pdf (accessed 22.08.2020).
Gori, Y., Stradiotti, A., and Camin, F. (2018). Timber isoscapes. a case study in a mountain area in the Italian Alps. PLoS One 13:e0192970. doi: 10.1371/journal.pone.0192970
Gori, Y., Wehrens, R., Greule, M., Keppler, F., Ziller, L., La Porta, N., et al. (2012). Carbon, hydrogen and oxygen stable isotope ratios of whole wood, cellulose and lignin methoxyl groups of Picea abies as climate proxies. Rapid Commun. Mass Spectrom. 27, 265–275. doi: 10.1002/rcm.6446
Havel, J. J. (1963). NGF17192. Morobe: Bends Area Bulolo-Lae Road LAE BRI BM NSW UH PNH US BISH BUL.
Heaton, K., Kelly, S. D., Hoogewerff, J., and Woolfe, M. (2008). Verifying the geographical origin of beef: the application of multi-element isotope and trace element analysis. Food Chem. 107, 506–515. doi: 10.1016/j.foodchem.2007.08.010
Horacek, M., Jakusch, M., and Krehan, H. (2009). Control of origin of larch wood: discrimination between European (Austrian) and Siberian Origin by stable isotope analysis. Rapid Commun. Mass Spectrom. 23, 3688–3692. doi: 10.1002/rcm.4309
Horacek, M., Rees, G., Boner, M., and Zahnen, J. (2018). Comment on: developing forensic tools for an African Timber: […], by Vlam Et Al., 2018. Biol. Conserv. 226, 333–334. doi: 10.1016/j.biocon.2018.06.037
Kagawa, A., and Leavitt, S. W. (2010). Stable carbon isotopes of tree rings as a tool to pinpoint the geographic origin of timber. J. Wood Sci. 56, 175–183. doi: 10.1007/s10086-009-1085-6
Kelly, S., Heaton, K., and Hoogewerff, J. (2005). Tracing the geographical origin of food: the application of multi-element and multi-isotope analysis. Trends Food Sci. Technol. 16, 555–567. doi: 10.1016/j.tifs.2005.08.008
Keppler, F., Harper, D. B., Kalin, R. M., Meier-Augenstein, W., Farmer, N., Davis, S., et al. (2007). Stable hydrogen isotope ratios of lignin methoxyl groups as a paleoclimate proxy and constraint of the geographical origin of wood. New Phytol. 176, 600–609. doi: 10.1111/j.1469-8137.2007.02213.x
Lacey Act (2008). 16 U.S.C. §§3371-3378. Available online at: https://uscode.house.gov/view.xhtml?path=/prelim@title16/chapter53&edition=prelim (accessed April 30, 2021).
Leavitt, S. W. (2010). Tree-ring C–H–O isotope variability and sampling. Sci. Total Environ. 408, 5244–5253. doi: 10.1016/j.scitotenv.2010.07.057
Li, A., Keely, B., Chan, S. H., Baxter, M., Rees, G., and Kelly, S. (2015). Verifying the provenance of rice using stable isotope ratio and multi-element analyses: a feasibility study. Qual. Assur. Saf. Crops Foods 7, 343–354. doi: 10.3920/qas2013.0378
Pilgrim, T. S., Watling, R. J., and Grice, K. (2010). Application of trace element and stable isotope signatures to determine the provenance of tea (Camellia sinensis) samples. Food Chem. 118, 921–926. doi: 10.1016/j.foodchem.2008.08.077
Rarawa, D. H. (2012). Central Bank of the Solomon Islands Rep. Annual Report. Available online at: http://www.cbsi.com.sb/wp-content/uploads/2016/09/AR-2011.pdf (accessed April 27, 2012).
Rees, G. O. (2015). Verifying the Declared Origin of Timber Using Stable Isotope Ratio and Multi-Element Analyses. Available online at: http://etheses.whiterose.ac.uk/9522/ (accessed August 22, 2020).
Regulation (EU) No 995/2010 (2016). Guidance Document for the EU Timber Regulation. Brussels: EU timber regulation.
Sakai, H., Casadevall, T. J., and Moore, J. G. (1982). Chemistry and isotope ratios of sulfur in basalts and volcanic gases at Kilauea Volcano, Hawaii. Geochim. Cosmochim. Acta 46, 729–738. doi: 10.1016/0016-7037(82)90024-2
Smyth, J. (2018). China’s Demand for Timber Threatens Forests in Solomon Islands. Financial Times. Available online at: https://www.ft.com/content/b8f237ee-d26c-11e8-a9f2-7574db66bcd5 (accessed October 18, 2018).
Soerianegara, I., and Lemmens, R. H. M. J. (1993). PROSEA Plant Resources of South-East Asia 5(1) Timber Trees: Major Commercial Timbers. Wageningen: Pudoc Scientific Publishers.
The Government of The British Solomon Islands (1960). The British Solomon Islands Geological Record 1957 – 1958. Reports on Investigations into the Geology and Mineral Resources of the Protectorate. London: Crown Agents For Oversea Governments and Administrations.
Ueda, A., and Sakai, H. (1984). Sulfur isotope study of quaternary volcanic rocks from the Japanese Islands Arc.”. Geochim. Cosmochim. Acta 48, 1837–1848. doi: 10.1016/0016-7037(84)90037-1
Watkinson, C. J., Gasson, P., Rees, G. O., and Boner, M. (2020). The development and use of isoscapes to determine the geographical origin of Quercus Spp. in the United States. Forests 11:862. doi: 10.3390/f11080862
Watkinson, C. J., Rees, G. O., Moundounga, C. G., Gasson, P., Hofem, S., and Boner, M. (2021). Stable isotope ratio analysis of timber to protect two forest concessions in Gabon. Front. For. Glob. Change For. Ecophysiol. (in press).
Keywords: stable isotopes, Solomon Islands, tropical timber, EUTR, Lacey Act amendment, EA-IRMS
Citation: Watkinson CJ, Rees GO, Hofem S, Michely L, Gasson P and Boner M (2022) A Case Study to Establish a Basis for Evaluating Geographic Origin Claims of Timber From the Solomon Islands Using Stable Isotope Ratio Analysis. Front. For. Glob. Change 4:645222. doi: 10.3389/ffgc.2021.645222
Received: 22 December 2020; Accepted: 27 May 2021;
Published: 08 February 2022.
Edited by:
Micha Horacek, HBLFA Francisco Josephinum, BLT Wieselburg, AustriaReviewed by:
Jessica Young-Robertson, University of Alaska Fairbanks, United StatesBernd Degen, Thünen Institute of Forest Genetics, Germany
Copyright © 2022 Watkinson, Rees, Hofem, Michely, Gasson and Boner. This is an open-access article distributed under the terms of the Creative Commons Attribution License (CC BY). The use, distribution or reproduction in other forums is permitted, provided the original author(s) and the copyright owner(s) are credited and that the original publication in this journal is cited, in accordance with accepted academic practice. No use, distribution or reproduction is permitted which does not comply with these terms.
*Correspondence: Charles J. Watkinson, charlie.watkinson@agroisolab.com