- 1Durrell Institute of Conservation and Ecology, School of Anthropology and Conservation, University of Kent, Canterbury, United Kingdom
- 2Fauna & Flora International, Vietnam Programme, Hanoi, Vietnam
- 3Institute for Tropical Biology and Conservation, Universiti Malaysia Sabah, Kota Kinabalu, Malaysia
- 4South East Asia Rainforest Research Partnership, Danum Valley Field Centre, Lahad Datu, Malaysia
Arboreal mammals form a diverse group providing ecologically important functions such as predation, pollination and seed dispersal. However, their cryptic and elusive nature, and the heights at which they live, makes studying these species challenging. Consequently, our knowledge of rainforest mammals is heavily biased towards terrestrial species, limiting our understanding of overall community structure and the possible impacts of human-induced disturbance. We undertook the first in-depth appraisal of an arboreal mammal community in Southeast Asia, using camera-traps set in unlogged and logged tropical rainforest in Sabah, Borneo. Using paired canopy and terrestrial camera-traps at 50 locations (25 in unlogged forest, 25 in logged), we assessed the effectiveness of camera-trapping at characterising the arboreal versus terrestrial community, and tested the influence of strata and forest type on community structure and composition. The paired design detected 55 mammal species across 15,817 camera-trap nights (CTNs), and additional canopy sampling in a subset of trees added a further two arboreal species to the inventory. In total, thirty species were detected exclusively by terrestrial camera-traps, eighteen exclusively by canopy camera-traps, and nine by units set at both heights, demonstrating significant differences between arboreal and terrestrial communities. This pattern was strongest in unlogged forest, reflecting greater structural diversity of this habitat, but held in logged forest as well. Species accumulation curves revealed that canopy camera-trapping significantly boosted species inventories compared to terrestrial-only sampling, and was particularly effective at detecting gliding mammals, rodents and primates. Canopy inventories took longer to reach an asymptote, suggesting that a greater sampling effort is required when deploying canopy camera-traps compared to those set on the ground. We demonstrate that arboreal mammals in Borneo’s rainforest form a diverse and distinct community, and can be sampled effectively using canopy camera-traps. However, the additional costs incurred by sampling in the canopy can be substantial. We provide recommendations to maximise sampling effectiveness, while bringing down costs, to help encourage further study into one of the last frontiers of tropical forest research.
Introduction
Tropical rainforests support exceptional levels of biodiversity, but are highly threatened by anthropogenic activities such as logging (Barlow et al., 2018). Rainforests are structurally complex environments, comprising not only ground-level (i.e., terrestrial) vegetation, but also several interlinked above-ground strata [hereafter the canopy (Moffett, 2000)], and culminating in tree crowns that can reach 30–45 m in height (Dudley and De Vries, 1990). However, due mainly to the difficulties of canopy access (Lowman et al., 2013), most rainforest research is heavily biased towards terrestrial communities and processes (Whitworth et al., 2019a). Consequently, the canopy remains a largely unexplored ecological frontier (Godoy-Guinao et al., 2018). With only a limited understanding of canopies and the wildlife they support, we are missing key insights into the composition, dynamics and functioning of rainforest ecosystems as a whole.
The wildlife utilising the forest canopy plays essential roles in ecosystem functioning, for example by regulating biogeochemical and nutrient cycles, and facilitating forest regeneration via animal-mediated seed dispersal (Nakamura et al., 2017). An estimated 75% of rainforest vertebrates are arboreal or semi-arboreal, spending all or part of their lives in the canopy (Kays and Allison, 2001). Among them, mammals are one of the most diverse and numerous taxonomic groups, filling a wide variety of ecological roles including seed dispersal, pollination, herbivory, and predation (Kays and Allison, 2001; Nakabayashi et al., 2019; Whitworth et al., 2019a). Removal of these key vertebrates may affect the capability of rainforests to recover from disturbance, with potentially cascading consequences for ecosystem stability and resilience (Gardner et al., 2019).
In addition, arboreal mammals may be more vulnerable to the effects of logging than their terrestrial counterparts because the large, tall trees that constitute the main structure of their canopy habitat are often also those lost through logging. At present, most tropical research into the effects of logging on wildlife does not include targeted sampling for arboreal mammals, and it is not clear to what extent this group is affected, or whether populations can recover after the cessation of logging activities (Bowler et al., 2017). For many terrestrial taxa, species diversity in logged forest can return to approximately pre-logging levels within a few decades of the cessation of logging (Berry et al., 2010; Brodie et al., 2014). However, it has also been suggested that terrestrial mammal inventories in logged forest may be artificially inflated by increased detections of semi-arboreal species spending more time on the ground (Berry et al., 2010). While this has been demonstrated for some small-bodied arboreal mammals in some areas (Malcolm, 1997; Malcolm and Ray, 2000), other studies have shown post-logging reductions in the abundance of small arboreal rodents (Wells et al., 2007) and occupancy of medium- and large-bodied arboreal mammals (Whitworth et al., 2019a) without an apparent influx effect at ground-level. The current lack of monitoring of the canopy strata is a barrier to our understanding of whether this phenomenon occurs, and highlights the risk that we may be missing declines in arboreal species, and underestimating the true impact of logging.
Traditionally, arboreal mammals have been sampled using ground-based visual surveys, but these tend to be biased towards larger-bodied, diurnal species that can be readily observed and identified from below, and show some degree of tolerance to people (Whitworth et al., 2016; Bowler et al., 2017; Moore et al., 2020). Moreover, the heights at which arboreal species are detected present significant challenges for accurate identification from the ground (Jayasekara et al., 2007; Gregory et al., 2014; Whitworth et al., 2016). Canopy-based live-trapping has also been tested, but is labour-intensive, tends to exclude larger-bodied species, and can result in biased sampling, particularly as bait is used (Caravaggi et al., 2020).
Advances in camera-trapping technology have led to the widespread use of this survey method in the study of terrestrial mammals (Wearn and Glover-Kapfer, 2019), but it is yet to be commonly implemented at canopy-level. To date, applications of camera-trapping in the canopy have focused mainly on documenting animal presence (e.g., Suzuki and Ando, 2019), behaviour (e.g., Godoy-Guinao et al., 2018), or activity in relation to particular habitat features such as fruiting trees (e.g., Jayasekara et al., 2007) or canopy bridges (e.g., Gregory et al., 2017) (see also Supplementary Table 1). Published inventories of arboreal mammal communities based on camera-trap data are limited to five sites (Whitworth et al., 2016, 2019a; Bowler et al., 2017; Hongo et al., 2020; Moore et al., 2020), all in the Neotropics or Africa, and with four of five focusing on medium- and large-bodied mammals. Only three of these studies compared canopy inventories to those generated from camera-traps on the ground (Whitworth et al., 2019a; Hongo et al., 2020; Moore et al., 2020), limiting the inferences that can be made when describing arboreal versus terrestrial communities. Further, almost half of all published canopy-based camera-trap studies that recorded camera-trap height (26 of 54, Supplementary Table 1) placed camera-traps ≤ 10 m above the ground, missing a large portion of the vertical space from their sampling. While canopy camera-trapping has shown great potential as a sampling technique, it has yet to be employed as a standard tool for monitoring arboreal mammal communities. Understanding the advantages and limitations of this method is therefore essential if it is to be more widely adopted.
Here, we provide the first in-depth appraisal of the arboreal mammal community in Southeast Asia, using camera-traps set in unlogged and logged tropical rainforest of Sabah, Malaysian Borneo. The rainforests of Borneo are among the tallest in the world, and are renowned for their arboreal and semi-arboreal mammal fauna, comprising over 70 species and including 14 flying squirrel taxa, representing the global epicentre of gliding mammal diversity (Payne and Francis, 2007; Thorington et al., 2012). We compare the ability of camera-traps to define the arboreal and terrestrial mammal community, and extend our assessment from the medium- and large-sized mammals typically investigated in camera-trap studies to include the numerous smaller-bodied arboreal species, most of which can also be identified by this method (De Bondi et al., 2010). We evaluate the comparative costs of terrestrial versus canopy camera-trapping, and quantify the diversity missed or gained by each technique, revealing how studies of rainforest mammals that focus only on terrestrial species may be overlooking a key component of ecosystem dynamics.
Materials and Methods
Study System
Research was undertaken in and around the Stability of Altered Forest Ecosystems Project (Ewers et al., 2011; Figure 1) in Sabah, Malaysian Borneo. We sampled mammals in unlogged forest at Maliau Basin Conservation Area, and in logged forest in the Mt. Louisa Forest Reserve. These areas form part of an extensive contiguous block of dipterocarp forest covering approximately one million hectares in south-central Sabah (Reynolds et al., 2011). Mt. Louisa experienced multiple rounds of logging between 1978 and 2008, but has since been formally protected, whereas the unlogged forest at Maliau Basin has experienced very little disturbance. Our logged forest sampling area was characterised by lower canopy height and reduced canopy cover, with fewer canopy pathways and more canopy gaps than our unlogged forest sampling area (Deere et al., 2020). Camera-trap locations in both unlogged and logged forest covered similar elevations (average 482 m, range 225–933 m).
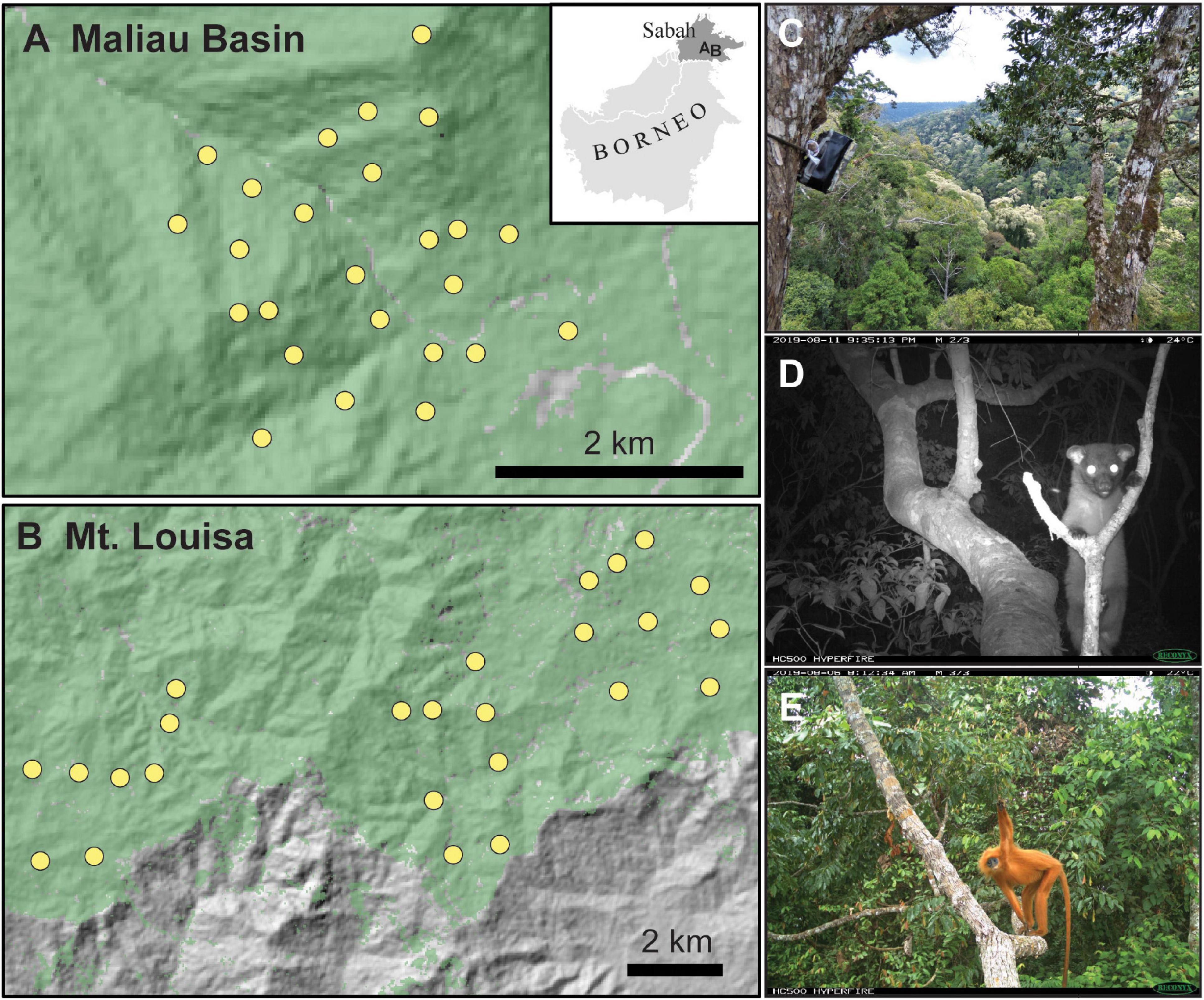
Figure 1. Camera-trap locations in unlogged (A) and logged (B) forest of Sabah, Borneo, with the location of the sampling in relation to Borneo shown on the inset maps. Canopy camera-traps were set on the trunk of trees, facing focal branches (C). Example arboreal species detected included small-toothed palm civet Arctogalidia trivirgata (D) and maroon langur Presbytis rubicunda (E).
Camera-Trapping
Camera-traps (Hyperfire HC500, Reconyx, WI, United States) were deployed across 50 locations between October 2017 and September 2019. Locations were divided equally between unlogged and logged forest and identified in advance using a 1.5 km2 grid, whereby every corner of each grid cell comprised a sampling location. Upon navigating to a location either via pre-existing trails or by creating new trails, the nearest tree to the marked point that could be safely climbed (hereafter the focal tree) was rigged with climbing ropes. We did not target a particular species, height or branch architecture type.
Accounting for accessibility and safety constraints, the average distance between sampling locations was 1.26 km (range: 0.5–4 km). Each location comprised one terrestrial camera-trap set approximately 0.3 m above the ground, paired with a canopy camera-trap in the mid- or upper-canopy of the focal tree, which was situated within a 10 m horizontal distance of the terrestrial placement. Canopy camera-traps were set at an average of 25.9 m above ground (range: 9.8–52.3 m), with the average height in unlogged forest (36.0 m) and logged forest (19.3 m) reflecting the differences in average canopy height between the two forest types. To reduce false triggers, camera-traps were attached to trunks or large, stable branches (Figure 1) and any leaves within the detection zone were removed (Gregory et al., 2014). Where possible, canopy camera-traps faced north or south to reduce the likelihood of overexposed images, which is a particular risk in the upper canopy (Otani, 2001). Most camera-traps faced branches of the same tree, were set approximately 0.2–0.3 m above the branch and were angled where necessary using a wooden wedge to account for slope of the branch. Three units faced trunks of adjacent trees (two in unlogged forest, one in logged) where these were judged to be within trigger distance (5–10 m away). Terrestrial camera-traps were also attached to medium-large, stable trunks and any vegetation within the detection zone deemed likely to result in false triggers (e.g., thin herb stems) was cleared. Vegetation disturbance was kept to a minimum in both strata, and canopy orchids and epiphytes in particular were left undisturbed. In order to reduce detection bias for terrestrial species which may either preferentially use or avoid trails (Wearn and Glover-Kapfer, 2017), and in line with our canopy protocol, terrestrial camera-traps did not target any particular habitat feature.
Camera-traps at each location were deployed for a total of 7–8 months. In unlogged forest this occurred continuously with one check mid-deployment to replenish batteries and SD cards, while due to scheduling practicalities, the logged forest deployment occurred in two separate phases. Each camera-trap was set to take three consecutive images per detection with no delay between triggers and no sleep delay before retriggering. The camera-traps we used were equipped with infrared flash for low light conditions to minimise disturbance. This is particularly important for nocturnal species, some of which suffer temporary blindness or may exhibit “trap shyness” when using white flashes (Schipper, 2007).
Sampling comprised a total of 100 camera-trap deployments and, after accounting for malfunction, we obtained data from 99, comprising 49 terrestrial camera-traps (24 in unlogged forest, 25 in logged) and 50 in the canopy (25 in unlogged forest and 25 in logged). Three functioning units did not obtain any mammal captures during deployment (all canopy, two in unlogged forest, one in logged). Thus in total, camera-traps were deployed for 15,817 camera-trap nights (CTNs): 6,661 terrestrial CTN (3,995 in unlogged forest and 2,666 in logged) and 9,156 canopy CTN (6,041 in unlogged forest and 3,115 in logged).
To evaluate whether placing more than one camera-trap in a tree simultaneously could maximise species detection, we set a second canopy camera-trap in 20 of our original focal trees over a period of approximately 3 months. Trees were randomly selected and additional camera-traps were deployed concurrently with the main canopy camera-trap, but positioned at different heights and facing different branches. These second camera-traps (10 in unlogged forest and 9 in logged after excluding one malfunctioning unit) resulted in an additional 1,409 CTN (903 in unlogged forest, 506 in logged).
Data Analysis
Mammal detections were summarised by camera-trap location (per CTN) and species using the R package gtools (R version 4.0.2). Capture events were considered independent if they were separated by a minimum period of 30 min, or if subsequent detections within this threshold contained different individuals or species (Laughlin et al., 2020).
To compare species accumulation between canopy and terrestrial strata, we generated sample-based rarefaction curves based on CTNs using the R package iNEXT (Hsieh et al., 2016). This approach accounts for differences in sampling effort between camera-trap locations (i.e., variation in deployment duration due to units being set and collected, or failing, at different times), without needing to discard data. Rather than reducing all locations to the lowest sampling effort, we interpolated species detections up to the maximum observed sample size and then extrapolated detections to a common sample size above this (Smax). Extrapolations were made to approximately double the maximum obtained sample size, as recommended by Hsieh et al. (2016). Where rarefaction results are referred to as statistically significant, this indicates non-overlapping confidence intervals of the relevant species accumulation curves.
Inventory comparisons were made between (a) arboreal and terrestrial communities and (b) unlogged and logged forest habitats, and rarefactions were repeated with subsets of the community data, with species assigned to groupings according to: arboreality, IUCN threat status, body size, and taxonomic group. Arboreality was defined according to the strata in which the species was detected (arboreal = exclusively on canopy camera-traps, terrestrial = exclusively on terrestrial camera-traps, semi-arboreal = on camera-traps in both strata); IUCN threat status was categorised as threatened = categories Vulnerable, Endangered or Critically Endangered; not threatened = Near Threatened, Least Concern or Data Deficient (International Union for Conservation of Nature, 2021); body size was defined as small ≤ 1 kg, medium = 1–5 kg, large ≥ 5 kg; and taxonomic group was divided into Carnivora (viverrids, mustelids, felids, and bear), gliding mammals (flying squirrels and Sunda colugo), non-gliding rodents (murid rodents, non-flying squirrels, and porcupines), Insectivora (treeshrews, moonrat, and Sunda pangolin), Primates (macaques, langurs, gibbon, and orangutan) and Ungulates (deer, mousedeer, pig, and banteng, plus elephant). Information on body size and taxonomic classifications was obtained from Payne and Francis, 2007 and the International Union for Conservation of Nature (2021).
Ordinations were used to explore variation in mammal community composition between terrestrial and canopy camera-trap locations, as well as unlogged versus logged forest. We standardised the species-camera data matrix by maximum values (a Wisconsin double standardisation) to improve detection of community patterns, and calculated pairwise Bray–Curtis dissimilarity coefficients using species detections pooled from the first 91 days of sampling at each camera-trap location (the maximum sampling effort common to a majority of locations, since units functioned for varying time periods). Using the vegan package in R, we then generated a non-metric multidimensional scaling (NMDS) ordination to organise camera-trap locations by similarity in species composition. To examine compositional differences between the habitats and strata, we applied a permutational multivariate analysis of variance (PERMANOVA) using the ADONIS function in vegan. Last, we applied the envfit function with 999 permutations as well as the Pearson’s coefficient function (akin to an indicator species analysis) to the species detections and ordination axis scores to identify the species that contributed the most to the variation in community structure between camera-trap locations.
Costs of Canopy Versus Terrestrial Camera-Trapping
Since we deployed camera-traps at both terrestrial and canopy levels, we calculated the additional costs incurred by our canopy deployments. Calculations assumed a fixed number of camera-traps available for use and compared the cost of setting all units on the ground versus half on the ground and half in the canopy. We assumed cost per camera-trap unit did not vary between strata (damage and repair bills during our study were similar between strata), but this will differ greatly depending on the camera-trap model and battery type used. For our study, cost per unit was USD $464, including one Reconyx Hyperfire HC500 ($450), one 16 GB memory card ($7) and 12 × AA batteries ($7 per camera-trap per deployment) – prices valid for January 2021.
Results
Species Detections and Richness
We recorded 55 mammal species during 8,008 capture events across 15,817 CTNs (Table 1). Of these species, 30 were only detected on terrestrial camera-traps, 16 were restricted to canopy camera-traps, and 9 were detected by camera-traps in both strata (hereafter referred to as semi-arboreal species) (Supplementary Table 2). The 19 experimental second canopy camera-traps added a further 1,409 CTN, with 238 capture events of 18 species. These additional records included 2 arboreal species otherwise undetected (Bornean pygmy squirrel, Excilisciurus exilis, and Temminck’s flying squirrel, Petinomys setosus, both in unlogged forest), and 1 semi-arboreal species otherwise recorded only on terrestrial cameras (banded civet, Hemigalus derbyanus, in logged forest), thus bringing the total number of species recorded exclusively on canopy camera-traps to 18.
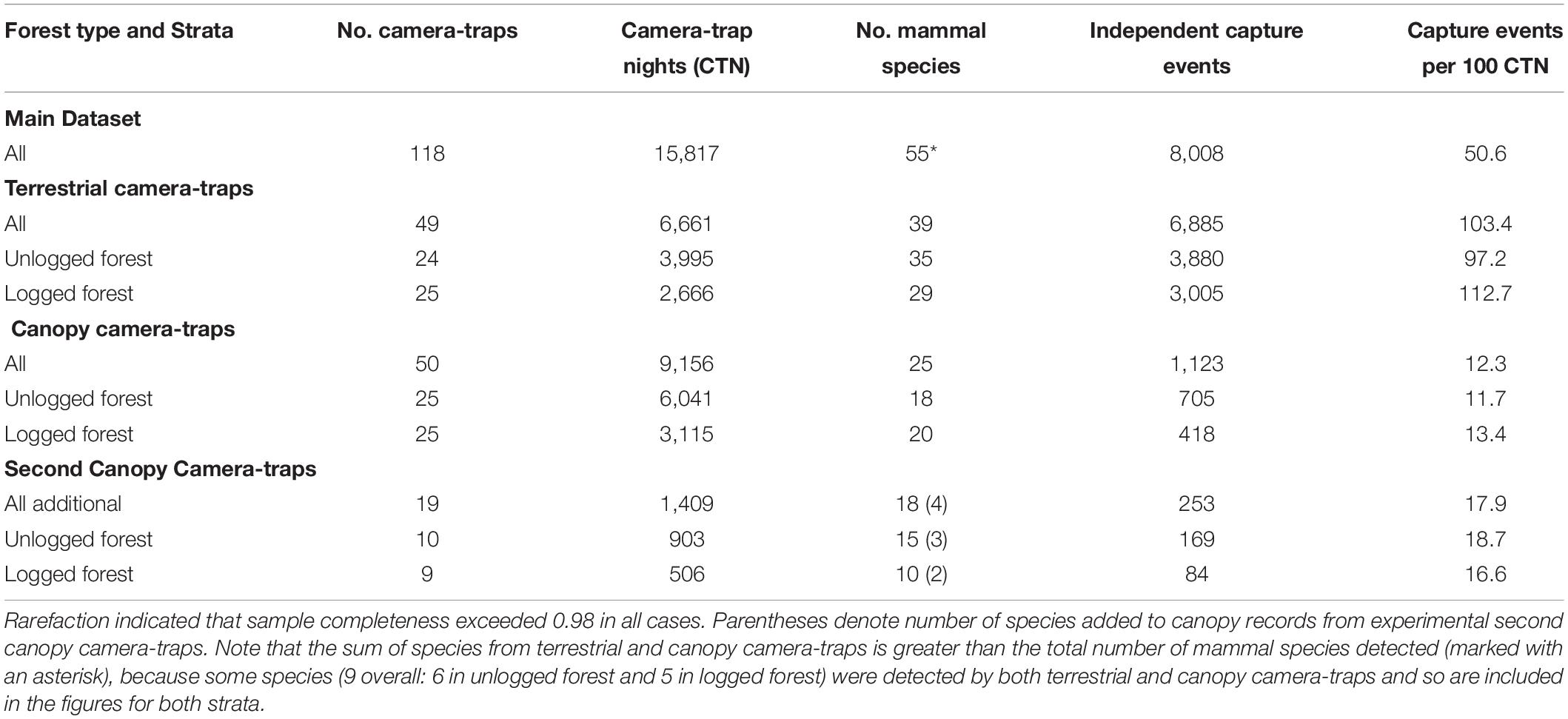
Table 1. Sampling effort, mammal species recorded and number of independent capture events for camera-traps set in terrestrial and canopy strata of unlogged and logged forest in Borneo.
There was substantial overlap of species recorded in unlogged and logged forest, although each forest type included records of a limited number of species not detected in the other (Supplementary Table 2). The number of CTN and capture events varied between unlogged and logged forest and between terrestrial and canopy strata (Table 1). Mammal detections on unlogged forest camera-traps totalled 47 species (29 terrestrial, 12 arboreal, 6 semi-arboreal), while those in logged forest totalled 44 (24 terrestrial, 15 arboreal, and 5 semi-arboreal), including one arboreal squirrel (Callosciurus sp.) whose appearance does not fit the description of any known Bornean species (Supplementary Figure 4). Twenty-one species potentially present within the landscape and likely detectable using camera-traps were not detected at all (Supplementary Table 2). Of these, nine are presumed terrestrial, six arboreal, and six semi-arboreal (Payne and Francis, 2007). Assumption of presence was based on known body size, geographic distribution, elevation range and habitat preferences (Payne and Francis, 2007; International Union for Conservation of Nature, 2021).
Any mammals that could not be identified to species level were excluded from analyses. Of a total of 8,276 capture events of mammal species across all camera-traps, 15 capture events were excluded on this basis, leaving 8,261 for analysis (8,008 from terrestrial and main canopy camera-traps, and 253 from second canopy camera-traps) (Table 1). The 15 exclusions comprised: 1 arboreal squirrel, 1 arboreal murid rodent, and 2 terrestrial squirrels where only a small portion of the body was visible; and 1 arboreal squirrel, and 10 terrestrial murid rodents (likely of 2 species) where identification to species level could be proposed with some confidence, but not with enough certainty to include in analyses.
Effectiveness of Sampling Across Forest Types and Strata
Rarefaction curves for terrestrial communities combined across both forest types reached an asymptote after 3,000–5,000 CTN, suggesting that terrestrial inventories were near complete for this method (Figure 2). In contrast, while canopy inventories also approached an asymptote at 3,000–5,000 CTN, curves still increased gradually (Figure 2), indicating that further sampling effort in the canopy would likely result in further detections of unique (i.e., not previously detected) species. This was corroborated by extrapolated accumulation curves, which predicted that arboreal communities may require more than double the sampling effort of terrestrial communities to generate complete or near-complete inventories (Supplementary Figure 2).
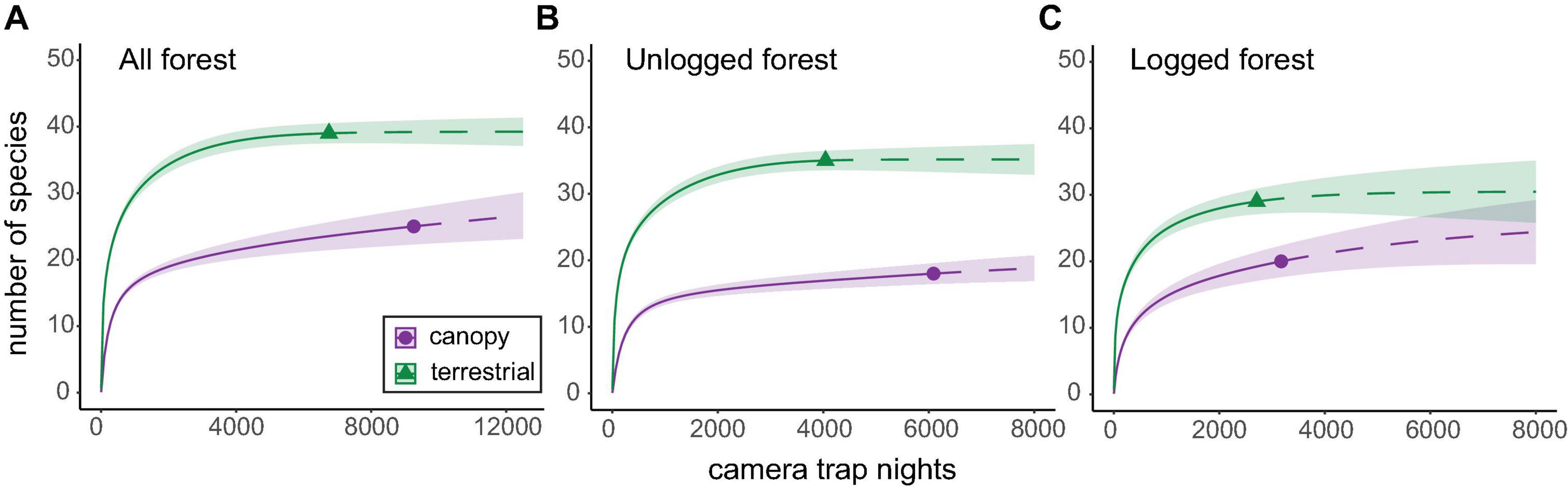
Figure 2. Rarefied species accumulation curves for arboreal and terrestrial mammal communities in panel (A) both forest types combined, (B) unlogged forest only, and (C) logged forest only. Curves were extrapolated (dashed line) to approximately double the minimum observed sample size in each comparison. Confidence intervals (CIs) were set at 84%, which has been demonstrated equivalent to a p value of 0.05 significant differences (MacGregor-Fors and Payton, 2013). CIs are represented by shaded areas around the curves. Additional analyses with CIs at 95% are presented in Supplementary Figure 1 for comparison.
Arboreal mammal species diversity in both unlogged and logged forest types was significantly lower than that characterised at the terrestrial level (Figure 2). The signal was stronger in unlogged forest (35 species across terrestrial camera-trap locations versus 18 species across canopy camera-trap locations) than logged forest (29 species across terrestrial camera-trap locations versus 20 species across canopy camera-trap locations) and this was driven by lower detections of terrestrial species in logged forest. Arboreal communities in both forest types largely comprised different species than those found at ground level, with a majority of species (46 of 55; 84%) detected exclusively by camera-traps in one strata, and only nine species (16%) captured on camera-traps at both heights (Supplementary Table 2).
Canopy camera-traps were particularly effective at detecting gliding mammals and primates, with non-gliding rodents also well-sampled (Figure 3), reflecting the main taxa present in the canopy. Canopy camera-traps matched terrestrial camera-traps in their ability to detect semi-arboreal species (Figure 3). On the other hand, terrestrial camera-traps detected more viverrids, mustelids, and felids. Terrestrial camera-traps were also effective at sampling non-gliding rodents, although examination of species identity (Supplementary Table 2) reveals there to be little overlap with non-gliding rodent species detected in the canopy, reflecting the high diversity within this group. Significantly more threatened arboreal mammals were detected in unlogged forest than in logged forest, although there was no significant difference in detection of threatened terrestrial mammals between forest types (Figure 3).
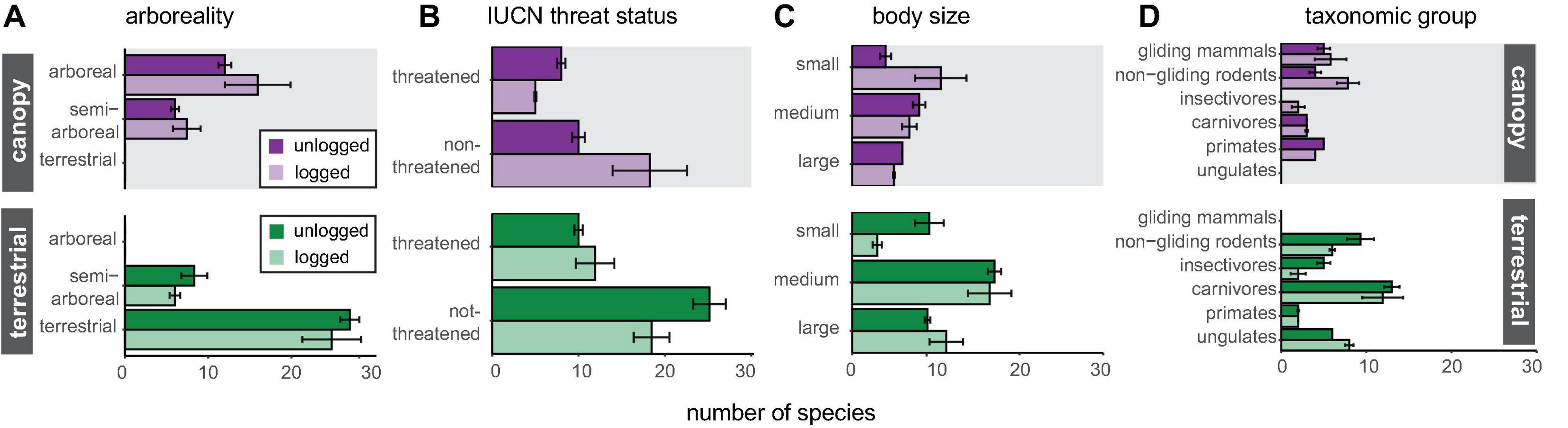
Figure 3. Number of species detected by canopy and terrestrial camera-traps in logged and unlogged forest, split into groups according to panel (A) arboreality, (B) IUCN threat status, (C) body size and (D) taxonomic group. Richness data were extracted from rarefied species accumulation curves at a common sample size, together with 84% confidence intervals.
Rarefaction analyses based on comparisons of data from terrestrial-only versus terrestrial-and-canopy camera-traps showed that the inclusion of canopy sampling significantly improved inventories in both forest types (Figure 4). Canopy camera-traps added 12 unique species in unlogged forest, 15 unique species in logged forest, and 16 species overall, or 18 species if data from the experimental second canopy camera-traps is included (Figure 4 and Supplementary Table 2). This difference was greatest in logged forest, driven mainly by the lower number of species detected by terrestrial-only camera-traps in this forest type. However, the addition of a second camera-trap elsewhere in the canopy did not significantly improve inventories (Figure 4 and Supplementary Figure 3).
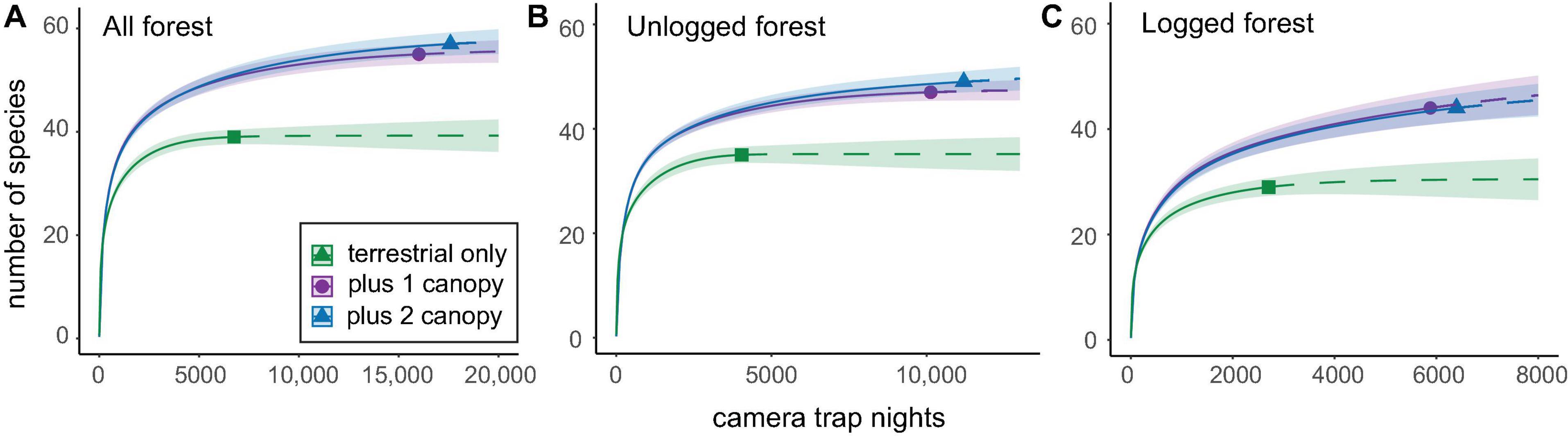
Figure 4. Species accumulation curves comparing species numbers obtained from 19 camera-traps set solely at terrestrial-level, with those obtained by pooling data from terrestrial- plus one canopy camera-trap, and terrestrial- plus two canopy camera-traps. Inventories are shown for panel (A) both forest types combined, (B) unlogged forest, and (C) logged forest. Confidence intervals were set at 84% in line with Figure 2 (MacGregor-Fors and Payton, 2013) and are represented by shaded areas under the curves. Analyses here represent data from the entire sampling period, totaling 6,661 CTN for terrestrial camera-traps, 9,156 CTN for single canopy camera-traps, and 1,409 CTN for experimental second canopy camera-traps. Analyses were repeated using a standardised subset of 1,409 CTN (the minimum trapping effort arising from the second canopy camera-traps), and these are presented in Supplementary Figure 3 for comparison.
Variation in Mammal Community Between Habitats and Strata
The NMDS ordination utilised information from 79 camera-trap locations that photographed mammals, and was statistically robust (stress = 0.120). Canopy and terrestrial camera-traps formed distinct groupings in ordination space, highlighting significant differences in community structure between these strata (PERMANOVA, R2 = 0.215, p < 0.001) (Figure 5). Terrestrial camera-trap locations were more similar to each other than canopy camera-trap locations, irrespective of forest type. Differences between the communities of unlogged and logged forest were much subtler (R2 = 0.027, p < 0.015). Key species identified by both envfit and Pearson’s coefficient tests to be driving community differences between strata were small-toothed palm civet Arctogalidia trivirgata and Prevost’s squirrel Callosciurus prevostii, indicative of the canopy, and Malay porcupine Thecurus crassispinis, tufted ground squirrel Rheithrosciurus macrotis, pig-tailed macaque Macaca nemestrina, red muntjac Muntiacus muntjak, Bornean yellow muntjac Muntiacus atherodes, greater mousedeer Tragulus napu, bearded pig Sus barbatus, sun bear Helarctos malayanus, Malay civet Viverra tangalunga, banded civet H. derbyanus and leopard cat Prionailurus bengalensis, indicative of ground level (Figure 5 and Supplementary Table 3).
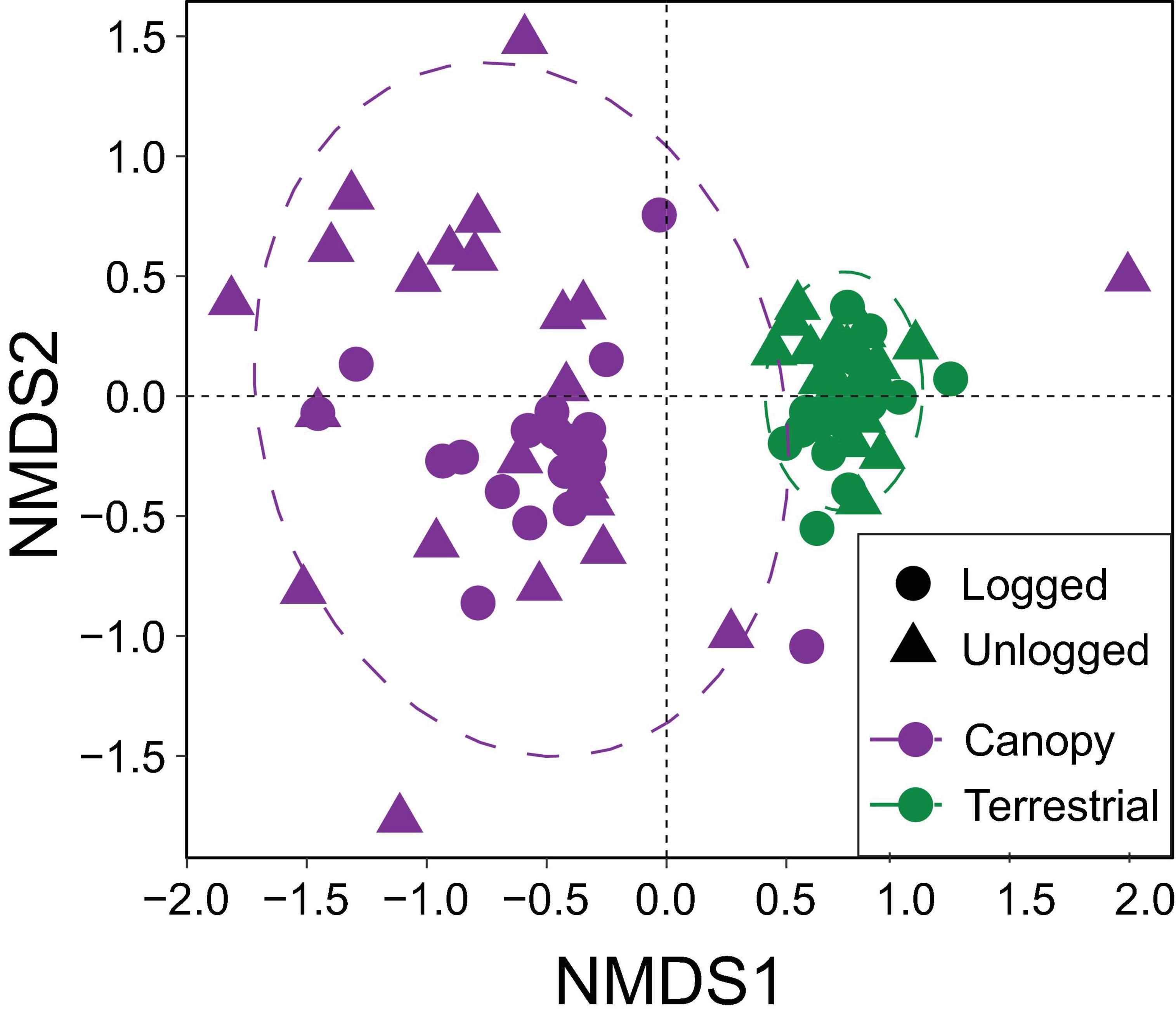
Figure 5. Non-metric multidimensional scaling (NMDS) ordination showing variation in mammal community structure detected by camera-traps set in the canopy versus terrestrial strata of unlogged and logged forest.
Cost
Canopy camera-trapping was more expensive than terrestrial camera-trapping due to the following additional costs that sampling at height brings: (i) hire or purchase of climbing equipment, (ii) canopy-access training; (iii) increased salary costs for trained climbers, (iv) more personnel needed to carry climbing equipment; and (v) increased time needed to rig trees and access the canopy (Table 2). For both terrestrial and canopy camera-trap locations, setup took substantially longer than maintenance or collection due to the extra time necessary to cut trails, identify suitable locations and position camera-traps. Total costs will vary depending on study design, field conditions and resources already available.
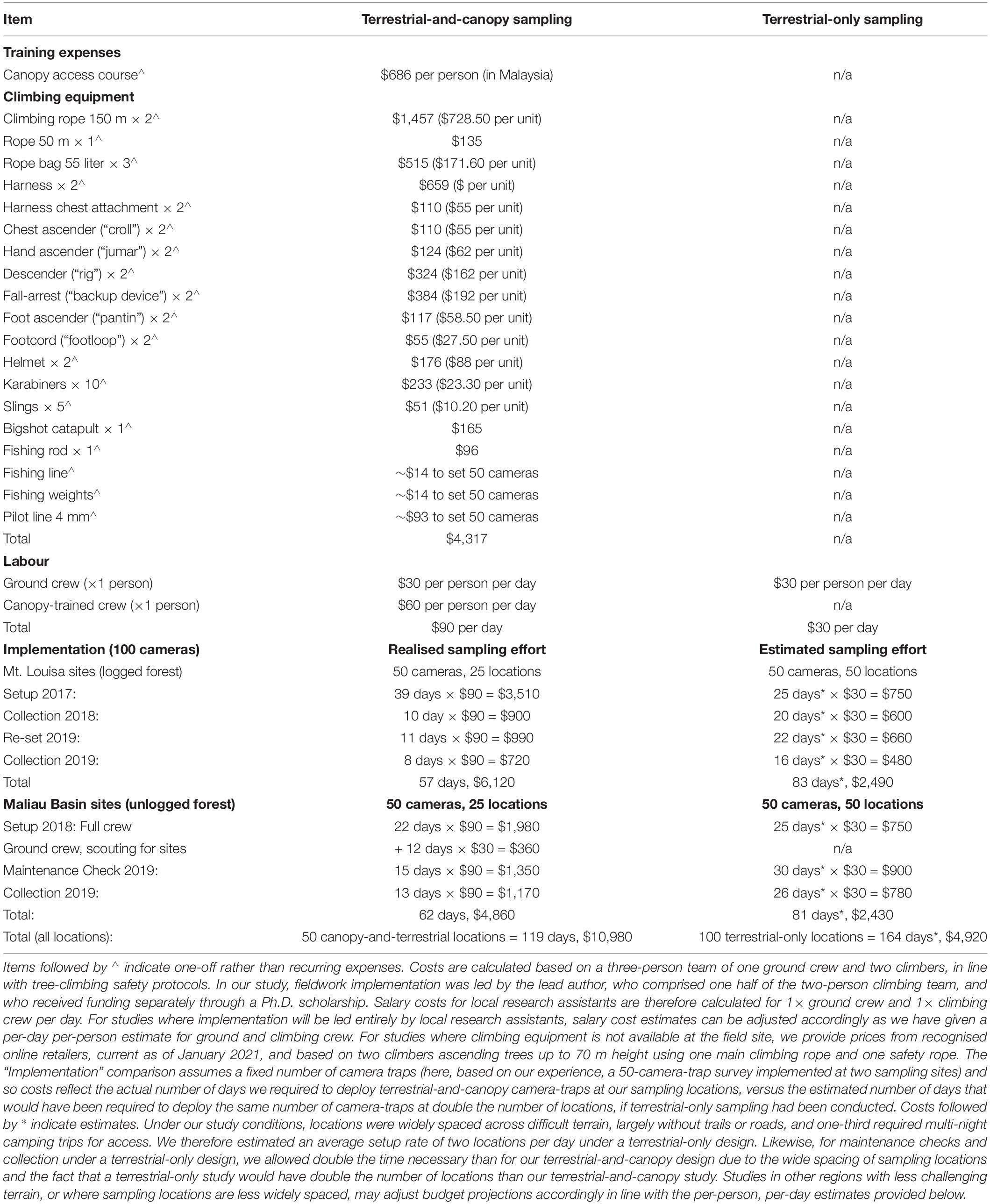
Table 2. Costs (US dollars) of terrestrial-only versus terrestrial-and-canopy camera-trapping, based on our experience in Sabah, where the cost of equipment hire was incorporated into the increased daily salary for climbing-trained staff.
In the context of our study, we estimated the implementation costs of canopy camera-trapping to be approximately double those of terrestrial-only sampling (approximately $5,000–$6,000 for terrestrial-and-canopy sampling versus approximately $2,500 for terrestrial-only sampling, per sampling site) (Table 2). However, we calculated that a terrestrial-only study under our sampling conditions would have taken longer to implement (an estimated 164 days vs. the 119 days for paired canopy-and-terrestrial locations, see Table 2). This is because terrestrial and canopy camera-traps are specifically targeting different elements of the mammal community (i.e., ground-dwelling vs. canopy-dwelling species), and thus are deliberately deployed very close to each other in order to fully sample as much of the mammal community as possible at each location. In contrast, terrestrial camera-traps target only ground-dwelling species, and are widely spaced in order to satisfy assumptions of independence. At our field site, two staff members had already been trained in canopy access techniques and climbing equipment was available, with the cost of equipment hire built into the increased daily salary of the climbing crew. Our additional one-off expenses therefore totalled $686 for canopy access training for one researcher (JKH, undertaken in Malaysia). For studies where equipment is not already available, we provide prices from recognised online retailers, current as of January 2021, and based on two climbers ascending trees up to 70 m height using one main climbing rope and one safety rope, at an approximate additional cost of $4,317.
Discussion
We describe the first canopy-based camera-trap inventory of arboreal mammals in Southeast Asia, and provide the first comparison of camera-trapping between terrestrial and canopy strata in this region. Our results reveal that arboreal mammals form a diverse community (Figure 2), comprising mainly species rarely or never detected at ground level (Figure 4 and Supplementary Table 2). This corroborates earlier findings on arboreal mammal communities from Peru (Gregory et al., 2017; Whitworth et al., 2019a), Brazil (Malcolm, 1991), Rwanda (Moore et al., 2020) and Sri Lanka (Jayasekara et al., 2007), and emphasises the importance of effective monitoring protocols for arboreal species that are easily overlooked by traditional terrestrial-based sampling.
Contribution of Canopy Cameras to Mammal Inventories
Our results clearly indicate that a sizeable proportion of the mammal community is routinely missed if sampling is only conducted at ground-level. Species accumulation curves showed canopy camera-traps significantly increased the overall mammal inventory compared to using only terrestrial camera-traps, and this pattern held across unlogged and logged forest (Figure 4). Across all locations, canopy camera-traps recorded 18 species that were not detected at ground level, adding 32% more species to inventories than would have been recorded using terrestrial camera-traps alone (Figure 4 and Supplementary Table 2). The mammal fauna detected on terrestrial camera-traps was more consistent across sampling locations than that documented by canopy camera-traps (Figure 5), reflecting the greater patchiness in detections and slower accumulation of arboreal species (Supplementary Figure 2). Differences between unlogged and logged forest were much weaker than those between terrestrial and canopy strata, and were largely driven by the greater number of species detected by terrestrial camera-traps in unlogged forest (Figure 2).
Despite high detections in both strata, twenty-one species known to be present and detectable in the landscape were not recorded (Supplementary Table 2): nine terrestrial, six arboreal and six semi-arboreal (i.e., potentially detectable in either strata) taxa. This finding is common to all survey methods, including camera-trapping in the canopy (Whitworth et al., 2016; Bowler et al., 2017; Moore et al., 2020) and terrestrial (e.g., Evans et al., 2016; Wearn et al., 2017) strata. Indeed, comparisons of the efficacy of visual surveys versus canopy camera-traps found both methods failed to detect some arboreal species that were picked up by the other (Whitworth et al., 2016; Bowler et al., 2017; Moore et al., 2020), and a similar pattern is reported for terrestrial versus canopy camera-traps elsewhere (Whitworth et al., 2019a; Moore et al., 2020). Possible reasons to explain this discrepancy in our study include low density and/or patchy distributions (e.g., bay cat Catopuma badia), preference for specific habitat conditions not represented in the survey (e.g., riverine areas for flat-headed cat Prionailurus planiceps), or population fluctuations linked to resource availability (e.g., rodents, Nakagawa et al., 2007).
Canopy camera-traps are most effective at detecting small- and medium-bodied species, gliding mammals and primates (Figure 3), reflecting the dominant arboreal taxa present in Borneo (Payne and Francis, 2007). Previous canopy camera-trap studies in the Neotropics have focussed on medium- and large-bodied mammals (Cassano et al., 2012; Whitworth et al., 2016; Bowler et al., 2017), as have most terrestrial camera-trap surveys, since small-bodied species are often fast-moving or obscured by vegetation, making identification difficult (Jayasekara et al., 2007; Glen et al., 2013). It is therefore encouraging to find that smaller mammals, including the highly diverse gliding mammals, can be readily identified from canopy camera-trap images, especially given the prevalence of these groups in Bornean rainforests (Thorington et al., 2012). Both canopy and terrestrial camera-traps recorded relatively high detections of non-gliding rodents (Figure 3), with little overlap in the identity of these species present in each strata (Supplementary Table 2), demonstrating the ability of canopy camera-traps to boost species inventories of often poorly-sampled taxa. Of the larger-bodied species, primates were well-sampled by canopy camera-traps, recording six of eight species known to be present, compared to only three species recorded by terrestrial camera-traps (Supplementary Table 2). Consistently higher detection frequencies in the canopy for all recorded primates (Supplementary Table 3) indicates that canopy camera-trapping may prove useful for studying the activity and behaviour of this group, which can be sensitive to human presence, in a manner that is minimally invasive and able to be deployed for long time periods across large spatial scales (see as example Whitworth et al., 2019b).
Conversely, terrestrial camera-traps were more effective at detecting medium- and larger-bodied species, particularly felids, civets, and mustelids. Although many of these species are classified as semi-arboreal and therefore potentially detectable by cameras in either strata, we found they were more often detected on terrestrial camera-traps in our study area, indicating that they may be more habitually terrestrial than arboreal. However, this may also be a result of lower detection probability in the canopy, linked to the overall greater three-dimensional area of this strata and the resultant slower accumulation of species. It is also possible that larger-bodied semi-arboreal species tend to utilise the lower canopy strata, or habitat features such as vine tangles (e.g., masked palm civet Paguma larvata, Chutipong et al., 2015) or tree holes (e.g., pangolin Manis javanica, Lim and Ng, 2008), whereas most of our canopy camera-traps were set in the mid- to upper-canopy facing large branches or trunks. These differences between presumed and detected arboreality serve to highlight that there is much we do not yet know about vertical space use by rainforest mammals.
Lower detections of threatened species by canopy camera-traps reflect the fact that fewer arboreal species in our sampling locations are categorised as threatened compared to terrestrial species (Supplementary Table 2): particularly among rodents. However, canopy camera-traps at unlogged forest locations detected significantly higher numbers of threatened arboreal mammals than those in logged forest. In contrast, there was no significant difference in numbers of threatened terrestrial species between logged and unlogged forest. This may have implications for survey design where the aim is to monitor threatened species.
Species-Specific Insights
For most of the arboreal species we recorded, little is known about their ecology, geographic distribution and tolerance to habitat modification (Meijaard et al., 2005; Payne and Francis, 2007). For example, our record of an arboreal squirrel not fitting any known description for Bornean species (Callosciurus sp., Supplementary Table 2 and Supplementary Figure 4), as well as our putative record of smoky flying squirrel (Pteromyscus pulverulentus), for which no confirmed photographic image currently exists (Thorington et al., 2012; Clayton, 2016), suggests that much remains to be discovered about Borneo’s little-known arboreal small mammals. Canopy camera-traps also documented interesting behavioural insights such as confirmed use of the canopy by plain treeshrews Tupaia longipes, a species previously considered strictly terrestrial (Payne and Francis, 2007); probable branch scent-marking in tufted ground squirrels R. macrotis, a threatened and poorly-known Borneo endemic; prevalence of “branch-walking” rather than brachiating locomotion in Bornean gibbons Hylobates funereus, especially in unlogged forest locations; and canopy mating behaviour of the strictly arboreal small-toothed palm civet A. trivirgata (Supplementary Figure 4).
Effects of Logging on Rainforest Mammals
Overall, our results suggest that the arboreal mammal community of logged forest is comparable in terms of species identity and diversity to that of unlogged forest (Figure 2 and Supplementary Table 2). This is encouraging from the perspective of species conservation, and corroborates similar findings from terrestrial mammal research (e.g., Brodie et al., 2014; Jati et al., 2018). In contrast, we recorded lower terrestrial species diversity in logged versus unlogged forest (Figure 2), indicating that logged forest inventories are not artificially inflated by arboreal species more frequently descending to ground level in this forest type, as sometimes proposed in the literature (e.g., Lambert et al., 2005; Berry et al., 2010). However, these findings should be viewed in the context of the relative habitat quality of our logged forest locations, which underwent selective logging 10 years prior to sampling and have been regenerating since that time. It is possible that there is a threshold of disturbance beyond which most arboreal species cannot persist (Deere et al., 2020), and that this threshold had not been met in our study system. It is also critical to note that our logged forest sampling area is subject to hunting levels which are very low in the regional context (Wearn et al., 2017). More commonly, logging is associated with a substantial increase in the hunting of medium and large mammals, due to the increased access given to the forest (Brodie et al., 2014).
Results from other locations are inconclusive, with canopy camera-trapping in the Neotropics showing medium- and large-bodied arboreal species to be more susceptible to habitat disturbance than their terrestrial counterparts (Cassano et al., 2012; Whitworth et al., 2019a), whereas live-trapping studies of small mammals documented varying responses to logging (e.g., Pardini, 2004; Wells et al., 2007; Laurance et al., 2008). In our study, it is also notable that while arboreal species diversity was similar between forest types, the frequency of capture events for some arboreal primates and gliding mammals was substantially lower in logged forest, whereas that of more common species (e.g., Prevost’s squirrel C. prevosti, pig-tailed macaque M. nemestrina) increased markedly in logged forest (Supplementary Table 3). The causes of these differences warrant further examination. However, they serve to highlight the fact that to fully understand the impacts of logging, we should not rely solely on species diversity. Changes in the relative abundance of mammals can impact ecosystem functioning, with declines in key species negatively affecting forest regeneration (Chazdon et al., 2009). What seems clear from the limited but increasing number of canopy-based studies is that habitat management strategies derived from terrestrial-only sampling risk overlooking the effect of logging on arboreal mammals, and thereby underestimating its true impact on rainforest ecosystems.
Methodological Considerations
Species accumulated more slowly in canopy inventories compared to those on the ground, as reported by other studies (Gregory et al., 2014; Whitworth et al., 2016, 2019a). While terrestrial communities in both forest types reached an asymptote at a sampling period of 3,000–5,000 CTN (Figure 2), this increased to an estimated 12,000–13,000 CTN for arboreal communities, or up to 57,000 CTN when data from forest types were combined (Supplementary Figure 2). Mammals utilising the terrestrial space are limited mostly to a horizontal distribution at ground level unless they exhibit arboreal tendencies. In the canopy space, however, mammals can be distributed both horizontally across branches and vertically across interlinked canopy strata (Nakamura et al., 2017; Hanya et al., 2020), which in Borneo can incorporate heights of up to 100 m in emergent trees (Shenkin et al., 2019). Mammal density in the canopy space is therefore much lower (i.e., animals per cubic kilometre). The passive infrared sensors on most camera-traps are designed to maximise the horizontal width of the detection zone (which may span 10+ m), but not the vertical height (typically spanning 1–2 m) (Wearn and Glover-Kapfer, 2017). In addition, while terrestrial camera-traps sample within their detection zone relatively evenly up to their maximum trigger distance, canopy camera-traps facing a focal branch or trunk are effectively sampling only the width and length of that feature, with the rest of the surrounding sampling area comprising empty space. It is therefore intuitive that species will accumulate more slowly in canopy inventories, and this should be factored into study design.
It has become increasingly common in terrestrial camera-trapping studies to deploy multiple camera-traps per location to boost detection probabilities when they are low (Pease et al., 2016; O’Connor et al., 2017; Wong et al., 2019). There has been little research into whether this method is useful in canopy settings, although one study in Brazil deploying arrays of 2–8 canopy camera-traps found detection probabilities of primates increased with the number of camera-traps per sampling location (Kaizer, 2019). Our trials deploying a second canopy camera-trap did not yield much improvement to new species detections (Figure 4 and Supplementary Figure 3); however, they were limited to a small subset of trees and a shorter sampling period. It is worth noting that the gains in unique species detections from additional canopy camera-traps came from unlogged forest locations, which makes sense in the context of the greater height and structural complexity – and therefore larger potential sampling area – of unlogged forest canopies (Deere et al., 2020). Canopy camera-trapping as a sampling method is in its infancy, and forest canopies present a much more complex sampling space than the forest floor. While our results do not show strong support for prioritising additional canopy camera-traps per sampling location, we do not rule this out as an avenue for future research into maximising arboreal species detections, depending on study aims and resources available.
Costs
Research budgets are almost always a limiting factor in study design. Canopy camera-trapping is reported to be cost-effective when compared with alternative methods such as line transects (Whitworth et al., 2016; Kaizer, 2019). However, for studies where camera-trapping alone is the preferred sampling method, we could find no information evaluating the extra expenses incurred by deploying camera-traps in the canopy. We found canopy-and-terrestrial camera-trapping to be approximately twice as expensive as equivalent sampling effort at ground-level only (Table 2), although exact costs will vary according to study design and field conditions. At our field sites, the greatest cost arose from the additional personnel needed to carry climbing equipment and access the canopy. This was due in part to our study design, with camera-trap locations widely spaced across difficult, off-trail terrain and therefore requiring substantial time to access regardless of the forest type, or the strata in which our camera-traps were set. However, we note that for studies with easier access to sampling locations, the cost of canopy camera-trapping is likely to be proportionately higher in comparison to that of terrestrial-only sampling because once a location has been accessed, the greatest time cost of canopy camera-trapping arises from the process of canopy access itself. Selecting a suitable tree, rigging it with climbing ropes, ascending to the canopy and positioning a camera-trap took between 1.5 and 6 h per tree (although this will vary with field conditions and, to some extent, experience). In contrast, once a location has been accessed, a terrestrial camera-trap can generally be set within 0.5–1 h.
For these reasons, costs must be considered in the context of individual study aims and budget, and weighed against the value of the additional data likely to be obtained by canopy camera-traps (Figure 4 and Supplementary Table 2). For researchers wishing to implement canopy camera-trapping, we recommend the following ways of reducing costs: (1) deploy camera-traps over one, longer period rather than two separate shorter periods, thus reducing the number of times locations need to be accessed to setup, check and collect camera-traps; (2) scout for suitable focal trees first with ground crew only and minimal equipment, marking positions and preparing access without the burden of heavy bags; (3) leave pilot line (thin, inexpensive rope running from canopy to ground) in place in focal trees so that climbing ropes can then be easily re-installed upon subsequent visits, avoiding the lengthy rigging process; (4) consider study longevity. Investment in climbing equipment and training is more cost-effective for multi-season studies as both last for several years; and (5) where possible, source equipment and training locally as this is often less expensive, reduces transport costs, and has the additional benefit of enabling familiarisation with location-specific climbing conditions (humidity, terrain, insects, etc.).
Recommendations
Based on our findings, we recommend that studies aiming to inventory the entire, non-flying mammal community of a given area include canopy camera-traps alongside paired units at ground-level (although detection of very small-bodied species and bats may require additional live-trapping). Canopy camera-traps have shown comparative efficiency to ground-based visual surveys for inventorying arboreal mammal communities (Bowler et al., 2017; Moore et al., 2020). We provide evidence corroborating the ability of canopy camera-traps to reliably detect strictly arboreal and semi-arboreal species, and suggest that studies focussing on primarily arboreal species may consider deploying canopy camera-traps as a stand-alone method. However, consideration should be given to specific study aims, target species and resources available, as both camera-traps and visual surveys may miss a small proportion of the mammal community (see for example Whitworth et al., 2016). On the other hand, for studies concentrating on terrestrial and larger-bodied semi-arboreal species, it may be more cost-effective to deploy camera-traps only at ground-level. Behavioural studies of larger-bodied semi-arboreal species known to use both strata (e.g., orangutan Pongo pygmaeus, binturong Arctictis binturong) may benefit from camera-trapping at both levels as species likely utilise each stratum differently (Ancrenaz et al., 2014; Chutipong et al., 2015; Whitworth et al., 2019b).
Conclusion
We provide compelling evidence that the inclusion of canopy camera-trapping significantly increases overall rainforest mammal inventories. However, this survey method can incur substantial additional costs, which should be factored into project planning alongside consideration of study aims and design. There is great scope for further study in this field, including increasing knowledge of arboreal species’ geographic distributions, activity and vertical niche partitioning, tolerance to human-induced habitat disturbance, and optimal sampling methods for the canopy strata. Canopy camera-trapping also provides the potential for behavioural studies of species that are not easily observable from ground level. Our results add to the small but growing body of evidence that by overlooking arboreal communities, we are missing crucial insights into the true diversity and functional significance of rainforest mammals. Tropical rainforests are complex habitats covering vast horizontal and vertical areas, and with a high proportion of species utilising the canopy strata. In order to fully understand these ecosystems, and by extension effectively conserve them, it is vital that future research includes canopy-based sampling.
Data Availability Statement
The raw data supporting the conclusions of this article has been made available by the authors (https://zenodo.org/record/5018280#.YNM-pehKiUk).
Ethics Statement
The animal study was reviewed and approved by the School of Anthropology and Conservation Research Ethics Committee, University of Kent.
Author Contributions
JH, MS, ND, and AM conceived and designed the study. JH conducted the fieldwork and performed the statistical analysis under guidance from MS, ND, and OW. JH, ND, and MS wrote the manuscript. All authors contributed to the manuscript revision and read and approved the submitted version.
Funding
This study was funded by the UK Natural Environment Research Council (NERC) via the Human Modified Tropical Forests Programme (NE/K016407/1; https://lombok.nerc-hmtf.info/). JH was supported by a Ph.D. scholarship funded by NERC through the EnvEast DTP scholarship (Grant Number NE/L002582/1).
Conflict of Interest
The authors declare that the research was conducted in the absence of any commercial or financial relationships that could be construed as a potential conflict of interest.
Acknowledgments
We thank the Sabah Biodiversity Council, Sabah Forest Department, Yayasan Sabah, and Benta Wawasan Sdn. Bhd. for research permissions [access license number JKM/MBS.1000-2/2 JLD.5 (53)]. Henry Bernard advised on in-country logistics, and Mohd. Sabri bin Bationg, Almius Jupri, Fredino John, Arnold James, Masliadi bin Asri, Masdi bin Asri, Sabidi Mohd. Rizan, Anis-Ampat Siliwong, and David Paul were instrumental to the camera-trapping campaign.
Supplementary Material
The Supplementary Material for this article can be found online at: https://www.frontiersin.org/articles/10.3389/ffgc.2021.673071/full#supplementary-material
References
Ancrenaz, M., Sollmann, R., Meijaard, E., Hearn, A. J., Ross, J., Samejima, H., et al. (2014). Coming down from the trees: is terrestrial activity in Bornean orangutans natural or disturbance driven? Sci. Rep. 4:4024. doi: 10.1038/srep04024
Barlow, J., Franca, F., Gardner, T. A., Hicks, C. C., Lennox, G. D., Berenguer, E., et al. (2018). The future of hyperdiverse tropical ecosystems. Nature 559, 517–526. doi: 10.1038/s41586-086-0301-1
Berry, N. J., Phillips, O. L., Lewis, S. L., Hill, J. K., Edwards, D. P., Tawatao, N. B., et al. (2010). The high value of logged tropical forests: lessons from northern Borneo. Biodivers. Conserv. 19, 985–997. doi: 10.1007/s10531-010-9779-z
Bowler, M. T., Tobler, M. W., Endress, B. A., Gilmore, M. P., and Anderson, M. J. (2017). Estimating mammalian species richness and occupancy in tropical forest canopies with arboreal camera-traps. Remote Sensing Ecol. Conserv. 3, 146–157. doi: 10.1002/rse2.35
Brodie, J. F., Giordano, A. J., Zipkin, E. F., Bernard, H., Mohd-Azlan, J., and Ambu, L. (2014). Correlation and persistence of hunting and logging impacts on tropical rainforest mammals. Conserv. Biol. 29, 110–121. doi: 10.1111/cobi.12389
Caravaggi, A., Burton, A. C., Clark, D. A., Fisher, J. T., Grass, A., Green, S., et al. (2020). A review of factors to consider when using camera traps to study animal behaviour to inform wildlife ecology and conservation. Conserv. Sci. Pract. 2:e239. doi: 10.1111/csp2.239
Cassano, C. R., Barlow, J., and Pardini, R. (2012). Large mammals in an agroforestry mosaic in the Brazilian Atlantic Forest. Biotropica 44, 818–825. doi: 10.1111/j.1744.7429.2012.00870.x
Chazdon, R. L., Peres, C. A., Dent, D., Sheil, D., Lugo, A. E., Lamb, D., et al. (2009). The potential for species conservation in tropical secondary forests. Conserv. Biol. 23, 1406–1417. doi: 10.1111/j.1523-1739.2009.01338.x
Chutipong, W., Steinmetz, R., Savini, T., and Gale, G. A. (2015). Sleeping site selection in two Asian viverrids: effects of predation risk, resource access and habitat characteristics. Raffles Bull. Zool. 63, 516–528.
Clayton, E. (2016). Pteromyscus pulverulentus. IUCN Red List Threat. Species 2016:e.T18703A22245307.
De Bondi, N., White, J. G., Stevens, M., and Cooke, R. (2010). A comparison of the effectiveness of camera trapping and live trapping for sampling terrestrial small-mammal communities. Wildlife Res. 37, 456–466. doi: 10.1071/WR10046
Deere, N. J., Guillera-Arroita, G., Swinfield, T., Milodowski, D. T., Coomes, D. A., Bernard, H., et al. (2020). Maximizing the value of forest restoration for tropical mammals by detecting three-dimensional habitat associations. PNAS 117, 26254–26262. doi: 10.1073/pnas.2001823117
Dudley, D., and De Vries, P. (1990). Tropical rain forest structure and the geographical distribution of gliding vertebrates. Biotropica 22, 432–434. doi: 10.2307/2388564
Evans, M. N., Vickers, S. H., and Abu-Bakar, M. S. (2016). Small carnivores of the Lower Kinabatangan Wildlife Sanctuary, Sabah, Borneo, including a new locality for the Otter Civet Cynogale bennettii. Small Carnivore Specialist Group 54, 26–38.
Ewers, R. M., Didham, R. K., Fahrig, L., Ferraz, G., Hector, A., Holt, R. D., et al. (2011). A large-scale forest fragmentation experiment: the Stability of Altered Forest Ecosystems Project. Philosophical Trans. R. Soc. B 366, 3292–3302. doi: 10.1098/rstb.2011.0049
Gardner, C. J., Bicknell, J. E., Baldwin-Cantello, W., Struebig, M. J., and Davies, Z. G. (2019). Quantifying the impacts of defaunation on natural forest regeneration in a global meta-analysis. Nat. Commun. 10:4590. doi: 10.1038/s41467-019-12539-1
Glen, A. S., Cockburn, S., Nichols, M., Ekanayake, J., and Warburton, B. (2013). Optimising camera traps for monitoring small mammals. PLoS One 8:e67940. doi: 10.1371/journal.pone.0067940
Godoy-Guinao, J., Diaz, I. A., and Celis-Diez, J. L. (2018). Confirmation of arboreal habits in Dromiciops gliroides: a key role in chilean temperate rainforests. Ecosphere 9:e02424. doi: 10.1002/ecs2.2424
Gregory, T., Carrasco Rueda, F., Deichmann, J., Kolowski, J., and Alonso, A. (2014). Arboreal camera trapping: taking a proven method to new heights. Methods Ecol. Evol. 5, 443–451. doi: 10.1111/2041-210X.12177
Gregory, T., Carrasco-Rueda, F., Alonso, A., Kolowski, J., and Deichmann, J. L. (2017). Natural canopy bridges effectively mitigate tropical forest fragmentation for arboreal mammals. Sci. Rep. 7:3892. doi: 10.1038/s41598-017-04112-x
Hanya, G., Kanamori, T., Kuze, N., Wong, S. T., and Bernard, H. (2020). Habitat use by a primate community in a lowland dipterocarp forest in Danum Valley, Borneo. Am. J. Primatol. 84, e23157. doi: 10.1002/ajp.23157
Hongo, S., Dzefack, Z. C. B., Vernyuy, L. N., Sosuke, M., Nakashima, Y., Djieto-Lordon, C., et al. (2020). Use of multi-layer camera trapping to inventory mammals in rainforests in southeast Cameroon. Afr. Study Monogr. 60, 21–37. doi: 10.1007/978-94-007-2175-3_2
Hsieh, T. C., Ma, K. H., and Chao, A. (2016). iNEXT: an R package for rarefaction and extrapolation of species diversity (Hill numbers). Methods Ecol. Evol. 7, 1451–1456. doi: 10.1111/2041-210X.12613
International Union for Conservation of Nature (2021). Red List. Available online at: https://www.iucnredlist.org (accessed February 16 2021).
Jati, A. S., Samejima, H., Fujiki, S., Kurniawan, Y., Aoyagi, R., and Kitayama, K. (2018). Effects of logging on wildlife communities in certified tropical rainforests in East Kalimantan, Indonesia. Forest Ecol. Manag. 427, 124–134. doi: 10.1016/j.foreco.2018.05.054
Jayasekara, P., Weerasinghe, U. R., Wijesundara, S., and Takatsuki, S. (2007). Identifying diurnal and nocturnal frugivores in the terrestrial and arboreal layers of a tropical rain forest in Sri Lanka. Ecotropica 13, 7–15.
Kaizer, M. (2019). Non-Invasive Monitoring for Population Assessments of A Critically Endangered Neotropical Primate. [PhD thesis]. Salford: University of Salford.
Kays, R., and Allison, A. (2001). Arboreal tropical forest vertebrates : current knowledge and research trends. Plant Ecol. 153, 109–120. doi: 10.1007/978-94-017-3606-0_9
Lambert, T. D., Malcolm, J. A. Y. R., and Zimmerman, B. L. (2005). Variation in small mammal species richness by trap height and trap type in southeastern amazonia. J. Mammal. 86, 982–990. doi: 10.1644/1545-1542(2005)86[982:vismsr]2.0.co;2
Laughlin, M. M., Martin, J. G., Olson, E. R., College, N., and Avenue, E. (2020). Arboreal camera trapping reveals seasonal behaviors of Peromyscus spp. in Pinus strobus canopies. Am. Midland Nat. 183, 210–222.
Laurance, W. F., Croes, B. M., Guissouegou, N., Buij, R., Dethier, M., and Alonso, A. (2008). Impacts of roads, hunting, and habitat alteration on nocturnal mammals in African rainforests. Conserv. Biol. 22, 721–732. doi: 10.1111/j.1523-1739.2008.00917.x
Lim, N. T. L., and Ng, P. K. L. (2008). Home range, activity cycle and natal den usage of a female Sunda pangolin Manis javanica (Mammalia: Pholidota) in Singapore. Endanger. Species Res. 4, 233–240. doi: 10.3354/esr00032
Lowman, M., Devy, S., and Ganesh, T. (2013). Treetops at Risk: Challenges of Global Canopy Ecology and Conservation. New York, NY: Springer, 436.
MacGregor-Fors, I., and Payton, M. E. (2013). Contrasting diversity values: Statistical inferences based on overlapping confidence intervals. PLoS One 8:e0056794. doi: 10.131/journal.pone.0056794
Malcolm, J. R. (1991). Comparative abundances of neotropical small mammals by trap height. J. Mammal. 72, 188–192. doi: 10.2307/1381995
Malcolm, J. R. (1997). “Biomass and diversity of small mammals in Amazonian forest fragments,” in Tropical Forest Remnants: Ecology, Management, and Conservation of Fragmented Communities, eds R. O. Bierregaard and W. F. Laurance (Chicago: University of Chicago Press), 207–221.
Malcolm, J. R., and Ray, J. C. (2000). Influence of timber extraction routes on Central African small-mammal communities, forest structure and tree diversity. Conserv. Biol. 14, 1623–1638. doi: 10.1111/j.1523-1739.2000.99070.x
Meijaard, E., Sheil, D., Nasi, R., Augeri, D., Rosenbaum, B., Iskandar, D., et al. (2005). Life After Logging: Reconciling Wildlife Conservation and Production Forestry in Indonesian Borneo. Indonesia: CIFOR, 378.
Moffett, M. W. (2000). What’s “Up” ? A critical look at the basic terms of canopy biology. Biotropica 32, 569–596. doi: 10.1111/j.1744-7429.2000.tb00506.x
Moore, J. F., Pine, W. E., Mulindahabi, F., Niyigaba, P., Gatorano, G., Masozera, M. K., et al. (2020). Comparison of species richness and detection between line transects, ground camera traps, and arboreal camera traps. Animal Conserv. 23, 561–572. doi: 10.1111/acv.12569
Nakabayashi, M., Inoue, Y., Ahmah, A. H., and Izawa, M. (2019). Limited directed seed dispersal in the canopy as one of the determinants of the low hemi-epiphytic figs’ recruitment in Bornean rainforests. PLoS One 14:e0217590. doi: 10.1371/journal.pone.0217590
Nakagawa, M., Miguchi, H., Sato, K., Sakai, S., and Nakashizuka, T. (2007). Population dynamics of arboreal and terrestrial small mammals in a tropical rainforest, Sarawak, Malaysia. Raffles Bull. Zool. 55, 389–395.
Nakamura, A., Kitching, R. L., Cao, M., Creedy, T. J., Fayle, T. M., Freiberg, M., et al. (2017). Forests and their canopies: achievements and horizons in canopy science. Trends Ecol. Evol. 32, 438–451. doi: 10.1016/j.tree.2017.02.020
O’Connor, K. M. O., Nathan, L. R., Liberati, M. R., Tingley, M. W., Vokoun, J. C., and Rittenhouse, T. A. G. (2017). Camera trap arrays improve detection probability of wildlife: investigating study design considerations using an empirical dataset. PLoS One 12:e0175684. doi: 10.1371/journal.pone.0175684
Otani, T. (2001). Measuring fig foraging frequency of the Yakushima macaque by using automatic cameras. Ecol. Res. 16, 49–54. doi: 10.1046/j.1440-1703.2001.00370.x
Pardini, R. (2004). Effects of forest fragmentation on small mammals in an Atlantic Forest landscape. Biodivers. Conserv. 13, 2567–2586. doi: 10.1023/b:bioc.0000048452.18878.2d
Payne, J., and Francis, C. M. (2007). Field Guide to the Mammals of Borneo. Kota Kinabalu: The Sabah Society, 332.
Pease, B. S., Nielsen, C. K., and Holzmueller, E. J. (2016). Single-camera trap survey designs miss detections: impacts on estimates of occupancy and community metrics. PLoS ONE 11:e0166689. doi: 10.1371/journal.pone.0166689
Reynolds, G., Payne, J., Sinin, W., Mosigil, G., and Walsh, R. P. D. (2011). Changes in forest land use and management in Sabah, Malaysian Borneo, 1990-2010, with a focus on the Danum Valley region. Philosophical Trans. R. Soc. B 366, 3168–3176. doi: 10.1098/rstb.2011.0154
Schipper, J. (2007). Camera-trap avoidance by Kinkajous Potos flavus: rethinking the “non-invasive” paradigm. Small Carnivore Conserv. 36, 38–41. doi: 10.1105/tpc.13.7.1567
Shenkin, A., Chandler, C. J., Boyd, D. S., Jackson, T., Disney, M., Majalap, N., et al. (2019). The world’s tallest tropical tree in three dimensions. Front. Global Change 2:32. doi: 10.3389/ffgc.2019.00032
Suzuki, K. K., and Ando, M. (2019). Early and efficient detection of an endangered flying squirrel by arboreal camera trapping. Mammalia 83, 372–378. doi: 10.1515/mammalia-2018-0055
Thorington, R. W. Jr., Koprowski, J. L., Steele, M. A., and Whatton, J. F. (2012). Squirrels of the World. Baltimore: The Johns Hopkins University Press, 459.
Wearn, O. R., and Glover-Kapfer, P. (2017). Camera-trapping for conservation: a guide to best-practices. WWF Conserv. Technol. Series 1, 2014–2019.
Wearn, O. R., and Glover-Kapfer, P. (2019). Snap happy: camera traps are an effective sampling tool when compared with alternative methods. R. Soc. Open Sci. 6:181748. doi: 10.1098/rsos.181748
Wearn, O. R., Rowcliffe, J. M., Carbone, C., Pfeifer, M., Bernard, H., and Ewers, R. M. (2017). Mammalian species abundance across a gradient of tropical land-use intensity: a hierarchical multi-species modelling approach. Biol. Conserv. 212, 162–171. doi: 10.1016/j.biocon.2017.05.007
Wells, K., Kalko, E. K. V., Lakim, M. B., and Pfeiffer, M. (2007). Effects of rain forest logging on species richness and assemblage composition of small mammals in Southeast Asia. J. Biogeogr. 34, 1087–1099. doi: 10.1111/j.1365-2699.2006.01677.x
Whitworth, A., Beirne, C., Huarcaya, R. P., Whittaker, L., Rojas, S. J. S., Tobler, M. W., et al. (2019a). Human disturbance impacts on rainforest mammals are most notable in the canopy, especially for larger−bodied species. Diversity Distrib. 25, 1166–1178. doi: 10.1111/ddi.12930
Whitworth, A., Braunholtz, L. D., Huarcaya, R. P., Macleod, R., and Beirne, C. (2016). Out on a limb: arboreal camera traps as an emerging methodology for inventorying elusive rainforest mammals. Tropical Conserv. Sci. 9, 675–698. doi: 10.1177/194008291600900208
Whitworth, A., Whittaker, L., Huarcaya, R. P., Flatt, E., Morales, M. L., Connor, D., et al. (2019b). Spider monkeys rule the roost: ateline sleeping sites influence rainforest heterogeneity. Animals 9, 1052. doi: 10.3390/ani9121052
Keywords: camera-trap, biodiversity monitoring, tropical rainforest, disturbance, Southeast Asia, mammal survey
Citation: Haysom JK, Deere NJ, Wearn OR, Mahyudin A, Jami J, Reynolds G and Struebig MJ (2021) Life in the Canopy: Using Camera-Traps to Inventory Arboreal Rainforest Mammals in Borneo. Front. For. Glob. Change 4:673071. doi: 10.3389/ffgc.2021.673071
Received: 26 February 2021; Accepted: 11 June 2021;
Published: 09 July 2021.
Edited by:
Akihiro Nakamura, Xishuangbanna Tropical Botanical Garden (CAS), ChinaReviewed by:
William J. McShea, Smithsonian Conservation Biology Institute (SI), United StatesLaura Braunholtz, Newcastle University, United Kingdom
Seth Wong, Leibniz Institute for Zoo and Wildlife Research, Germany
Copyright © 2021 Haysom, Deere, Wearn, Mahyudin, Jami, Reynolds and Struebig. This is an open-access article distributed under the terms of the Creative Commons Attribution License (CC BY). The use, distribution or reproduction in other forums is permitted, provided the original author(s) and the copyright owner(s) are credited and that the original publication in this journal is cited, in accordance with accepted academic practice. No use, distribution or reproduction is permitted which does not comply with these terms.
*Correspondence: Jessica Karen Haysom, jkh27@kent.ac.uk