- 1Institute of Ecology and Earth Sciences, University of Tartu, Tartu, Estonia
- 2Sustainable Development Programme, Stockholm Environment Institute Tallinn Centre, Tallinn, Estonia
- 3Estonian Environment Agency, Tartu, Estonia
- 4Estonian Environmental Research Centre, Tallinn, Estonia
- 5Estonian Research Council, Tartu, Estonia
Litter decomposition is a key process that drives carbon and nutrient cycles in forest soils. The decomposition of five different substrate types was analyzed in hemiboreal coniferous forests, focusing on the mass loss and nutrient (N, P, and K) release of fine roots (FR) and needle litter in relation to the initial substrate and soil chemistry. A litterbag incubation experiment with site-specific FR and needle litter and three standard substrates (green and rooibos tea, α-cellulose) was carried out in four Norway spruce and four Scots pine-dominated stands in Estonia. Substrate type was the primary driver of mass loss and the decay rate of different substrates did not depend on the dominant tree species of the studied stands. Alpha-cellulose lost 98 ± 1% of the mass in 2-years, while the FR mass loss was on average 23 ± 2% after 3-years of decomposition. The FR decomposition rate could be predicted using a corresponding model of green tea, although the rate of FR decomposition is approximately five times lower than the rate of green tea in the first 3-years. The annual decomposition rate of the needle litter is rather constant in hemiboreal coniferous forests in the first 3 years. The initial substrate of fine roots or needle litter and soil chemistry jointly had a significant effect on mass loss in the later stage of decomposition. The critical N concentration for N release was lower for pine FR and needle litter (0.9–1.3% and 0.7–1.1%) compared to spruce (1.2–1.6% and 1.5–1.9%, respectively). The release rate of K depended on the initial K of substrate, while the release of N and P was significantly related to the initial C:N and N:P ratios, respectively. The results show the central role of soil and substrate initial chemistry in the decomposition of fine roots and needle litter across hemiboreal forests, especially at later stage (after 2 years) of decomposition. The slower decomposition and higher retention of N in the fine roots relative to needle litter suggests that fine roots have a substantial role in the carbon and nitrogen accumulation in boreal and hemiboreal forest ecosystems.
Introduction
Decomposition is a crucial process in forest soil regulating long-term storage of carbon and nutrient availability and determining soil fertility (Aerts, 1997; Berg and McClaugherty, 2008). More than 50% of the net primary production is returned to the soil in the form of aboveground and belowground litter (Wardle et al., 2004). The decomposition of plant litter is controlled by three main drivers – climate, litter quality and microbial communities, and can be separated into at least two stages (Berg and McClaugherty, 2008). The early stage decomposition process (mass loss approximately up to 40%) is characterized by the leaching of water-soluble compounds and the decomposition of solubles, cellulose and hemicellulose (Couteaux et al., 1995; Heim and Frey, 2004). The later stages of decomposition (approximately 40–100% mass loss) comprise the degradation of more recalcitrant compounds (Berg and McClaugherty, 2008). In general, litter chemical composition and decomposers dominate the decomposition process on regional or local scales, while climate is assumed to determine decomposition globally (Berg, 2000; Bradford et al., 2016). Litter quality, which is mainly determined by the relative content of recalcitrant organic compounds (e.g., lignin or phenols) and macronutrients, is of particular importance in controlling the mass loss and dynamics of litter nutrients during decay under similar climatic conditions (Prescott et al., 2000; Berg and McClaugherty, 2008). Several studies have shown that high initial nitrogen (N), phosphorus (P), and potassium (K) concentrations in litter regulate the early stages of decomposition by stimulating the decay of celluloses and solubles (Berg and Staaf, 1980; Berg and Matzner, 1997; Chen et al., 2002; Cusack et al., 2009; Sardans and Penuelas, 2021). However, high N inhibits the decay in the later stages by suppressing the activity of lignolytic enzymes (Carreiro et al., 2000) and shifting the decomposer-community composition (Couteaux et al., 1995). Considering the decomposition of soil organic matter, two opposing N cycling processes can be distinguished: immobilization as N accumulates in the substrate and mineralisation as N is released from the substrate. The concentration at which decomposing substrates switch from net accumulation to net release occurs is called the “critical N concentration” (Ågren and Bosatta, 1996). The critical N concentration depends on the initial N concentration of the litter, N availability in the soil, and the temperature and moisture conditions. In addition to climate (temperature, precipitation), local site conditions (fertility, litter and soil chemistry, decomposer community) also influence litter decomposition through the combined effect of nutrient availability and the soil moisture regime (Chapin et al., 2002). The main chemical soil properties include pH, organic carbon content and nutrients, of which soil nitrogen and phosphorus have been reported to be regulating and limiting factors (Coleman et al., 1999; DeLuca and Boisvenue, 2012).
The litterbag method, in which site-specific litter or standard litter material is retained for a certain period, is the most commonly used approach to determine litter decomposition in all ecosystems (Berg et al., 1993; Johansson, 1994). An exponential decay model (Lõhmus and Ivask, 1995) has been used for describing decomposition dynamics of different substrates. Site-specific litter is widely used to determine the specific decomposition rate of local litter since the decomposer communities are often adapted to site-specific litter. However, the use of cellulose or commercially available teabags allows us to compare driving factors of decomposition at the local, regional and global scales (Keuskamp et al., 2013) by excluding the site-specific litter quality effect but maintaining the role of local decomposers and abiotic factors. Plants typically contain up to 60% cellulose (Kögel-Knabner, 2002); thus, the decomposition of cellulose is a key activity of soil bacteria and is vital to the energy flow through soils and the cycling of N, P and other nutrients, since immobilization generally accompanies cellulose decomposition (Killham and Prosser, 2015). The decomposition rate of cellulose has been proposed as a general index of decomposer potential (Brække and Finér, 1990) which refers to diversity of soil microorganisms and or the activity of extracellular enzymes (Kurka, 2001). Therefore, cellulose can be used for assessing the relative decomposition potential of the soil, but not the absolute decomposition activity (Howard, 1988; Kurka et al., 2001). Similarly, the tea bag method, introduced by Keuskamp et al. (2013), is a standardized cost and time-efficient method that provides proxy data on decomposition rates and litter stabilization. According to the method, two types of tea material are used: relatively labile and rapidly decomposing green tea with a high cellulose content, and rooibos tea, which has a high lignin content and therefore expresses relatively low decomposition rates (Djukic et al., 2018). Similar to cellulose decomposition studies, the teabag method does not provide a quantitative measure of local carbon losses and decomposition rates because the tea is not equivalent to the site-specific litter. However, decomposition of standard materials highlights the influence of the different soil conditions or climatic factors at sites or biome level. Little is known, whether the decomposition rate of standard substrates could be used in modeling of native litter decay rate.
In this study, we examined the decomposition of five different site-specific and standard substrate types (fine roots and needle litter of Scots pine and Norway spruce, green tea, rooibos tea, α cellulose) across eight hemiboreal coniferous forests–four pine- and four spruce-dominated stands with different productivity using litterbag experiments for 3 years. We analyzed the effect of soil chemistry, soil moisture, soil temperature, precipitation, site productivity, and initial litter chemistry on decomposition. We aimed to: (1) compare decomposition dynamics of standard and site-specific substrates to determine the predictive power of standard substrates in the hemiboreal zone (2) analyze the dynamics of macronutrients (N, P, and K) of the incubated fine roots and needle litter; and (3) determine the range of critical N concentrations for fine roots and needle litter at which the net release of N begins in hemiboreal coniferous forests. We hypothesized that: (1) the substrate type and its initial chemistry, in particular the N content, is driving the mass loss and the rate of nutrient release is positively related to the initial nutrient concentration in the substrate; and (2) decomposition of standard substrates reflects the differences between forest sites and dominating conifer species.
Materials and Methods
Site Description
The study was carried out at four Scots pine (Pinus sylvestris L.) and four Norway spruce (Picea abies (L.) Karst) dominated stands in Estonia from 2012 to 2015 (fine roots, needle litter, α-cellulose; hereafter referred to as the main study period) and from 2016 to 2019 (green and rooibos tea) (Table 1). Studied stands covered a range of typical hemiboreal forest sites from dry, nutrient-poor and low-herb coniferous stands to mesic-moist and nutrient-rich coniferous forests consisting also some deciduous trees and a large number of herbs and shrubs (EEA, 2006). Approximately 81% of the forests in Estonia grow on mineral soils (Valgepea et al., 2017), and the current study sites represent approximately 40% of these forest site types. All of the stands are classified as upland mineral soils, except for the drained Polytrichum stand, which is growing on lowland mineral soil. The pine stands were rich in understory and dominated by perennial shrubs, while annual grasses were primarily found in the spruce stands. No remarkable herbaceous understory was present in the drained Polytrichum stand. However, the ground cover was dominated by moss (Pleurozium schreberi). Most of the stands belong to the International Co-operative Program on Assessment and Monitoring of Air Pollution Effects on Forests (ICP Forests) and the International Co-operative Program on Integrated Monitoring of Air Pollution Effects on Ecosystems (ICP IM) long-term forest monitoring network, which were established in the 1990s.
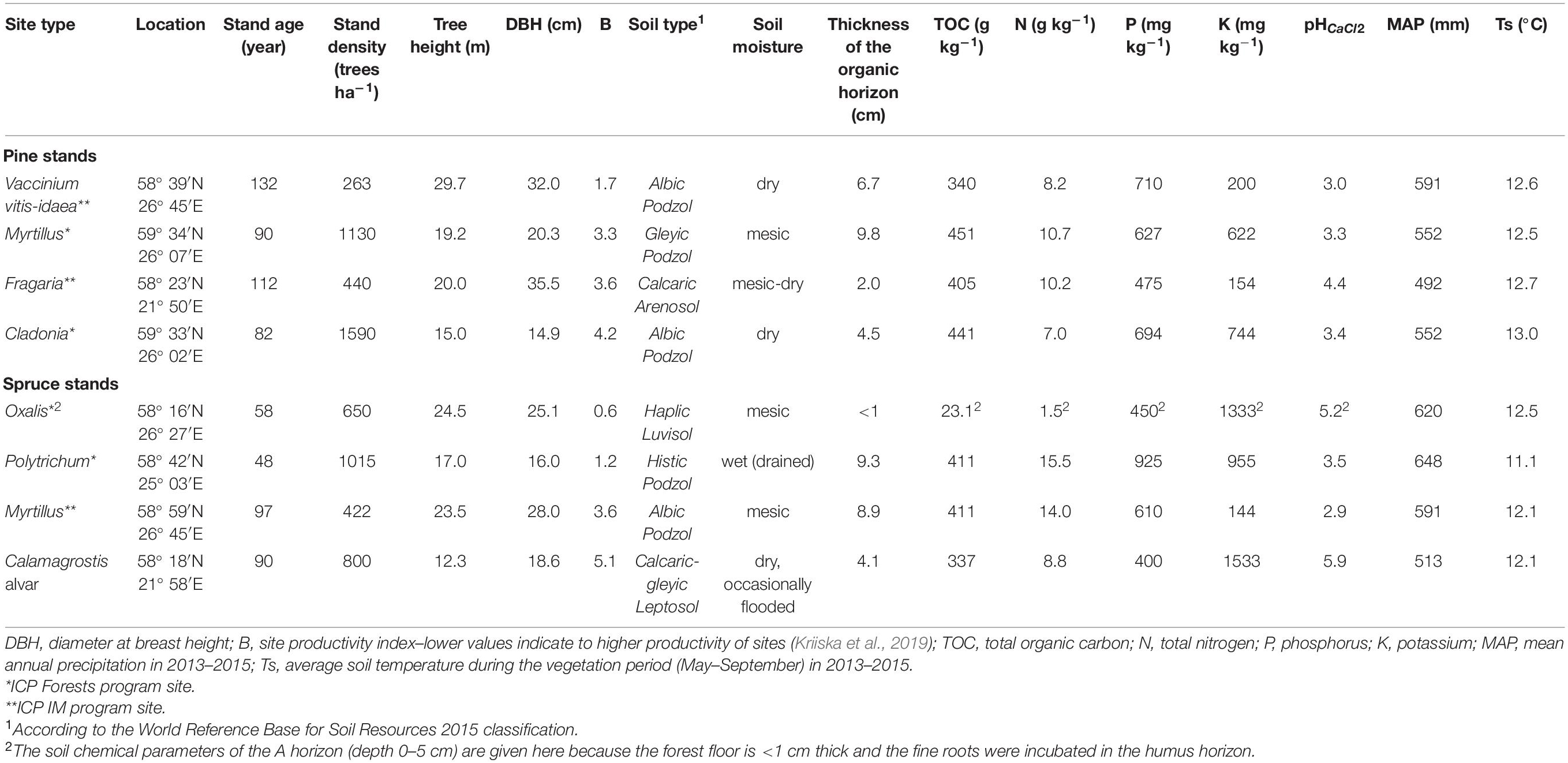
Table 1. Site characteristics and the chemical parameters of the soil organic layer (TOC, N, P, K, and pH) of the studied Scots pine and Norway spruce stands (Kriiska et al., 2019).
The average air temperature of the stands was 18.0°C in July and −4.0°C in January during the main study period. Mean annual precipitation (MAP) varied from 410 mm (Fragaria pine, Calamagrostis spruce) to 788 mm (Polytrichum spruce). Mean annual temperature (MAT) and MAP for all study years (2013–2019) and sites were obtained from the nearest weather stations1 (Supplementary Table 1). A more detailed description, including a map of the study sites, can be found in Kriiska et al. (2019).
Decomposition of Needle Litter, Fine Roots, α-Cellulose, Green Tea, and Rooibos Tea
The decomposition study was carried out using the in situ litterbag method. Incubated needles included site-specific pine or spruce needle litter collected throughout the previous year from each study site according to the ICP Forests and ICP IM manuals (methodology available at the ICP Forests website: http://icp-forests.net/). Site-specific fine roots (<2 mm in diameter) of dominating tree species were collected with soil cores in August and September 2012. Before the start of incubation, all litter types were oven-dried at 65°C for 48 h and the exact initial weight was recorded. For each sample, approximately 0.5 g of fine roots, 1 g of needle litter and 1 g of α-cellulose (1 mm thick, 30 × 50 mm wide sheets) was put in nylon bags. The mesh size was 1 mm for the α-cellulose and needle litter and 0.1 mm for fine roots. In total, 40 litter bags of each litter type were buried for each of the eight sites. Needle litter bags were placed on the soil surface and lightly covered with surrounding litter, while the α-cellulose and fine root bags were inserted at a depth of 5 cm into the soil organic horizon or in the humus horizon (in case of the Oxalis site) during October and November 2012. Five to seven samples of each litter type were collected at all sites after a field incubation period of 1 month (except needle litter), 7–8 months, 1, 2, and 3 years. A standardized protocol (Djukic et al., 2018) was applied for the decomposition of green and rooibos tea. Tea bags were buried in June or July 2016 in the top 5 cm of the soil and retrieved after 3, 12, 24, and 36 months. Soil and ingrown material (roots, mosses etc.) were removed from all of the retrieved litterbags, the decomposing material was dried to a constant weight at 65°C for a minimum of 48 h and the remaining mass was weighed to the nearest 0.1 mg. Mass loss was calculated for each litter sample as the relative difference between the initial and retrieved dry mass. Comparison of mass loss and decomposition rate of tea bags and other litter types were performed only on annual basis to exclude the effect of different installation time.
Chemical Analyses
Composite samples were used for further chemical analyses. Fine root and needle litter samples were analyzed for total nitrogen (modified Kjeldahl method; ISO 11261), total phosphorus [inductively coupled plasma atomic emission spectrometry (ICP-OES)], total organic carbon (IR-spectrometry) and potassium (flame-emission spectrometry) at the Estonian Environmental Research Centre laboratory, which holds a certificate for chemical analysis for ICP Programs. The concentrations of cellulose and lignin were determined using the acid detergent lignin method. Soil chemical parameters were determined during the BioSoil project in 2006–2008 following the ICP Forests and ICP IM manuals.
Statistical Analyses
Statistical analyses were carried out using the SigmaPlot 12.5 (Systat Software Inc., 2011) and STATISTICA 7.1 software (StatSoft, Inc., 2005); level of significance was set at p < 0.05. The normality of variable distribution was verified using the Kolmogorov–Smirnov test. One-way analysis of variance (ANOVA), followed by Tukey’s HSD was performed to test the differences in mass loss between different litter types and stands. The t-test for independent samples by groups was applied to determine differences between the initial chemistry of the incubated fine root and needle litter and differences in the initial chemical composition of the incubated pine and spruce litter. Means are presented with standard errors (SE).
Redundancy analysis (RDA) (CANOCO program et al., 2002) was applied to examine the relationships between the mass loss over time across all litter types (response variables) and the site productivity and chemical parameters of the soil organic layer (pHCaCl2, N, P, K, TOC, C:N ratio, N:P ratio; explanatory variables), the sites were included as dummy variables. First, we tested with manual selection the explanatory variables (Monte Carlo permutation test, p < 0.05). Then RDA with forward selection was used to test the significance of decomposition time, sites, stand productivity, soil K on mass loss of all five substrate types over a 3-year study period. GLM analysis was applied to test the effect of mean soil temperature and sum of precipitation of different years as continuous predictors of fine root and needle litter decomposition, the sampling year, stand and substrate type were considered as categorical factors. Analysis of covariance (Fixed Effect Test, Type III) was used to determine the relationships between the mass loss of site-specific litter (i.e., fine root and needle litter) and the combined effect of initial litter and the soil chemical parameters, using tree species and litter type as the grouping variables. Stepwise multiple linear regression analysis was carried out with each first-, second-, and third-year mass loss of each litter type separately to determine the effects of site productivity, environmental parameters (MAP, soil moisture and temperature during the vegetation period) and soil chemical parameters. T-test for dependent samples was applied for pairwise comparison of MAT and MAP of first, second, and third year as study periods November 2012/October 2013 vs. July 2016/June 2017, November 2013/October 2014 vs. July 2017/June 2018, and November 2014/October 2015 vs. July 2018/June 2019, respectively.
Site productivity indices (B) were obtained from Kriiska et al. (2019); B expresses the estimate of forest productivity considering the rate of height growth of dominating trees at different age.
When decomposition followed the exponential decay model (1), then the decomposition rate (k) was calculated from remaining dry mass using the exponential decay model (Olson, 1963 modified by Lõhmus and Ivask, 1995):
where Mt is the mass (g) at time t, M0 is the initial mass of incubated litter (g), k is the decomposition rate, and t is the elapsed time. For fine roots (Lõhmus and Ivask, 1995) and tea substrates, k decreases with time:
where a and b are coefficients, and t is time (years in our study). The litter type and dominating tree species effect on annual k was tested by factorial ANOVA separately for each year. Nutrient release rates were calculated from the percentage of nutrient mass remaining at time t (Lin et al., 2011):
where Rt is the nutrient release rate (% year–1) at time t, Nt is the percentage of nutrient remaining (as percent of original mass) at time t, and t is the elapsed time (year); data are in Supplementary Table 2. When Rt < 0, denotes nutrient immobilization into substrate. Rt of nitrogen was calculated at the end of the experiment, i.e., from the litter retrieved after 3 years of decomposition. Rt of phosphorus and potassium were calculated from the litter retrieved after 1.7 years of decomposition. Release-accumulation dynamics of P in needle litter were calculated for six stands out of eight, because the data for Fragaria pine and Calamagrostis spruce forests were incomplete. Pearson correlation analysis was used to evaluate the relationship between the nutrient release and the initial fine root and needle litter quality.
Results
Initial Chemistry of the Incubated Substrates
Although the average concentrations of N, P, and K were higher in the fine roots, the initial chemistry did not differ significantly between the incubated fine root and needle litter (Table 2). The most productive stand, the Oxalis spruce had the highest concentration of N and K in the needles and the highest K concentration in the fine roots, as well as the lowest C:N ratio both in the needles and fine roots across the studied stands. The lowest concentration of N in the needles was found in the driest and low fertility Cladonia pine stand. The lowest level of P and cellulose was found in the fine roots of the calcareous and the least productive Calamagrostis spruce site.
Nitrogen concentration (R2 = 0.58, p = 0.03) and the C:N ratio (R2 = 0.54, p = 0.04) of the fine roots revealed a negative and positive correlation with the site productivity index (B; lower B value indicates higher site productivity), respectively, while the initial needle litter chemistry was not correlated with B.
The average content of TOC and the C:N ratio was significantly higher and the concentration of K was significantly lower in the pine needles compared to the spruce needles, while there were no significant differences in the chemical composition of the incubated fine roots between tree species.
Mass Loss and Its Dependence on the Soil and Substrate Chemistry
The mass loss of all substrates was significantly dependent on substrate type (<0.001; n = 5) and time (<0.001; n = 3). The effect of annual variation of the stand specific mean soil temperature was not significant over the whole study period. There was no difference in MAT and MAP between first, second, and third year pairs of two study periods, 2012/2013 vs. 2016/2017, 2013/2014 vs. 2017/2018, and 2014/2015 vs. 2018/2019, respectively (Supplementary Table 2).
The increase in mean mass loss in time for all substrates was described by power functions (Figures 1A,B). The highest mean (±SE) mass loss was recorded for the α-cellulose that had nearly completely decomposed by the end of the second year (98 ± 1%) (Table 3), followed by the green tea (76 ± 1%) and the needle litter (51 ± 3%). Although the mass loss of fine roots was higher compared to the α-cellulose 1 month after the start of incubation (8 ± 2% and 2 ± 1%, respectively), the mass loss of fine roots remained the lowest among the litter types throughout the study period, reaching 23 ± 2% by the end of the third year. The needle litter lost approximately three times more (66 ± 2%) of its original mass than the fine roots by the end of the study.
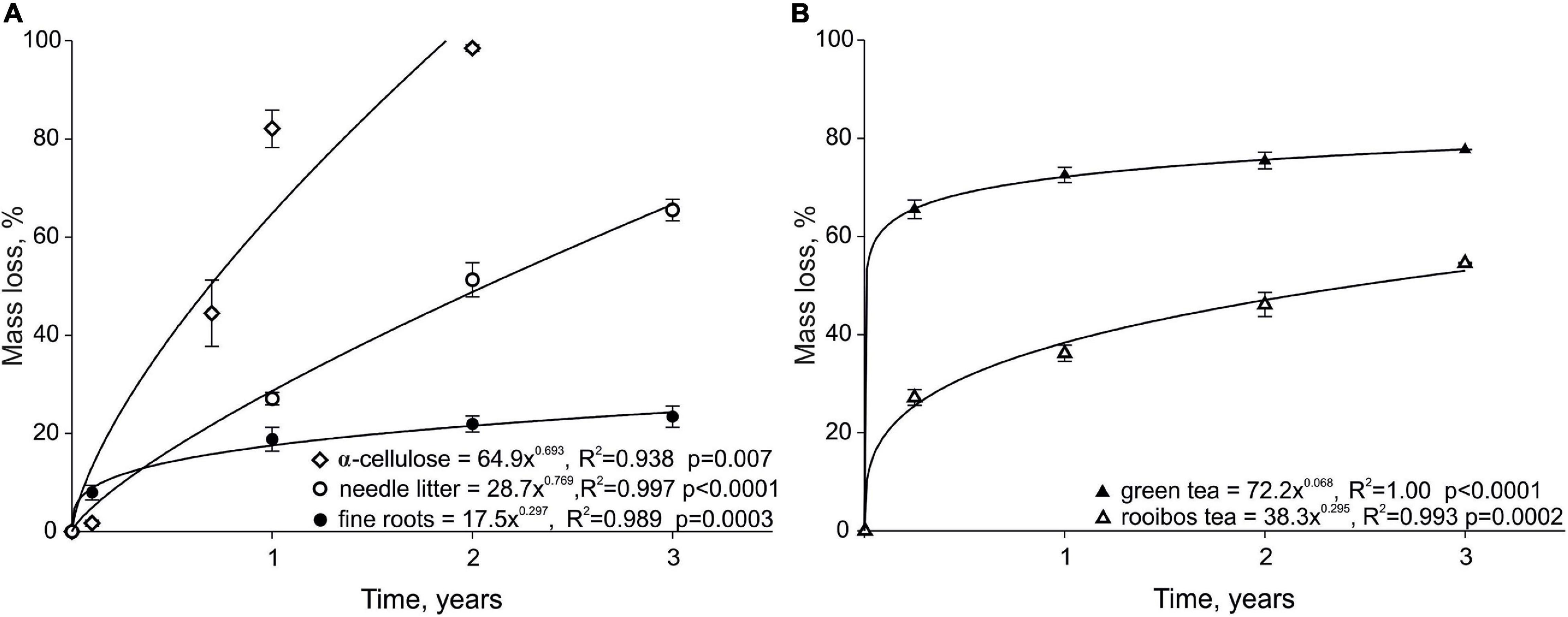
Figure 1. Mass loss (%) of α-cellulose, needle litter, fine roots studied from 2012 to 2015 (A), green tea and rooibos tea (B) studied from 2016 to 2019. Error bars represent the standard error of means.
The mass loss of fine roots and needle litter varied significantly between the different study sites after 1 year of decomposition; however, the differences in the mass loss between the stands diminished by the second year and almost disappeared by the end of the third year (Table 3). There were no significant differences in the mass loss of the standard substrates (α-cellulose, green and rooibos tea) between the study sites, except for the first year mass loss of the rooibos tea, which was significantly lower in the Oxalis spruce stand compared to the Vaccinium vitis-idaea pine stand. Although the mean value of the mass loss of all the substrate types was constantly higher in the pine stands, there was no significant difference in the substrate mass loss between the pine and spruce stands.
Results from RDA analysis with manual selection showed that the decomposition time, sites and stand productivity (B), and the average K content in the soil organic layer explained approximately 76% of the variation in mass loss of all substrates over 3 years of the study period (p < 0.05; Supplementary Figure 1). Among the latter, the decomposition time explained about 52% of variation in mass loss and correlated best with the first axis and the mass loss of standard substrates and needle litter, while mass loss of fine roots correlated better with the second axis (14%) being related to stand productivity (B) and the average K content in the soil organic layer. Multiple regression analysis with environmental parameters such as soil moisture, soil temperature and precipitation did not reveal any significant relationships with substrate mass loss.
According to the regression analysis carried out for each substrate type separately, the 1-year mass loss of the α-cellulose depended on the site productivity (p = 0.03, R2 = 0.58), with the mass loss being higher in the more fertile and productive sites (Supplementary Figure 2); however, the effect disappeared by the end of the second year. The 1-year mass loss of the green tea showed a negative relationship to the N:P ratio of the soil organic layer (p < 0.01, R2 = 0.76). The 2- and 3-year mass loss of the needle litter (p = 0.02, R2 = 0.67 and p = 0.01, R2 = 0.74, respectively) and the 2-year mass loss of green tea (p = 0.01, R2 = 0.68) were related negatively to the soil K content (Supplementary Figure 3).
Correlation analysis with initial substrate chemistry revealed that the 1-year mass loss of Scots pine fine roots (p = 0.01, R2 = 0.98) and needle litter (p = 0.04, R2 = 0.93) was significantly and positively related to the initial N content; however, the relationships were not significant for the incubated spruce litter. The 3-year mass loss of pine needles also expressed a positive dependence on the initial N content, though the relationship weakened (p = 0.047, R2 = 0.91). Additionally, the 1-year mass loss of pine needles showed a strong positive correlation with the initial P and K concentration (p < 0.01, R2 = 0.99 for both nutrients) and 1-year mass loss of pine fine roots expressed a positive relationship (p = 0.03, R2 = 0.94) with the initial litter K.
Since the mass loss of the site-specific substrates, i.e., fine roots and needle litter, did not reveal substantial relationships with the soil organic layer chemistry, a covariance analysis was applied to also take into account the differences in the initial substrate quality and its combined effects with soil properties. According to the covariance analysis, the 2-year mass loss depended significantly on the substrate type (Table 4), while the 3-year mass loss was significantly related to the N, P, and K concentration of the initial substrate and to the TOC, P, and K content of the soil organic layer, substrate type and interaction of the substrate type and tree species.
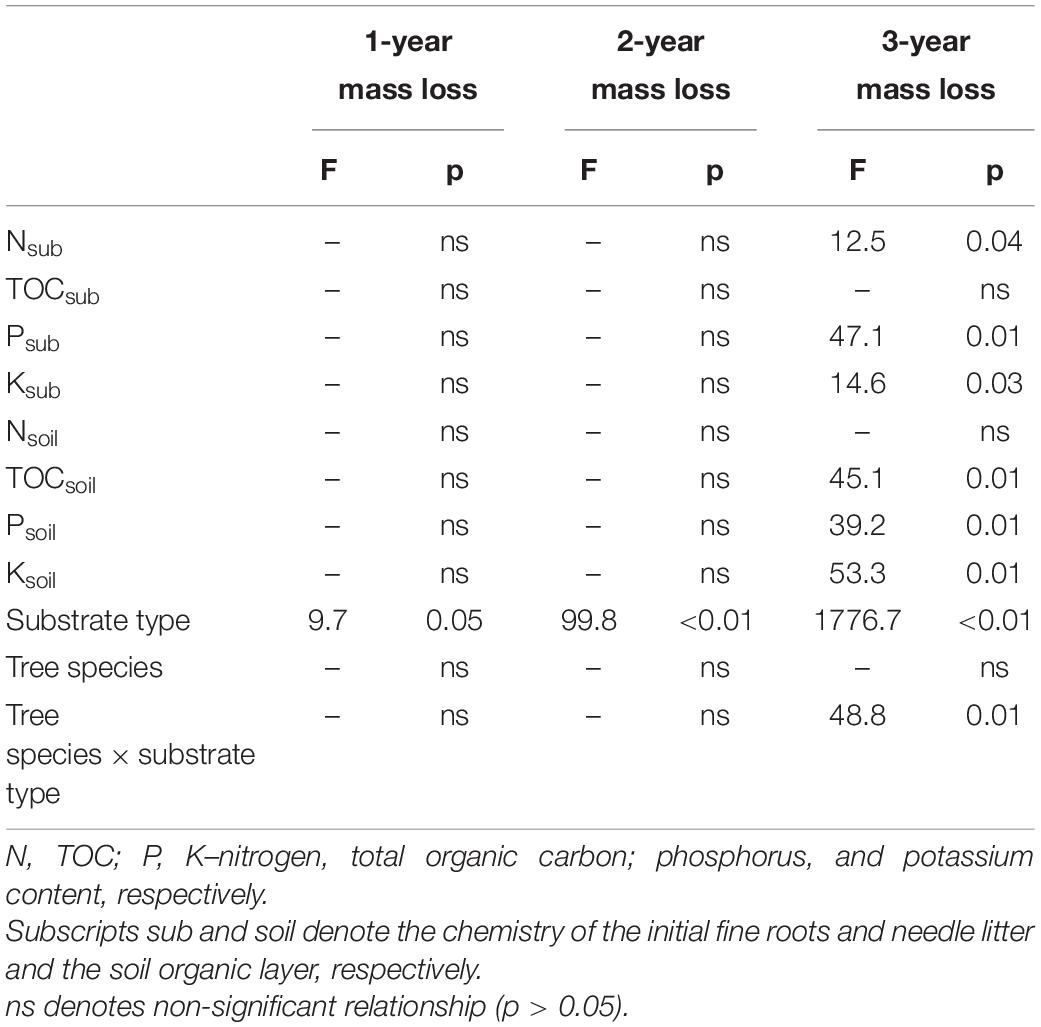
Table 4. Analysis of covariance of the pooled fine roots and needle litter mass loss (N = 15) after 1, 2, and 3 years, initial substrate and soil chemistry.
Decomposition Rates of the Fine Root, Needle Litter and Green and Rooibos Tea
A pattern of high decomposition rates of the fine roots during the first year followed by the stabilization of the k values in the second and third year was observed in the overall 3-year decomposition study period (Supplementary Figure 4). The modeled 1-year k rates of fine roots ranged from 0.13 to 0.31 (Table 5), being highest in the Vaccinium vitis-idaea pine stand where the initial lignin:cellulose ratio was the lowest (Table 2). The fine roots of the Myrtillus pine stand expressed the lowest k values throughout the study period. The difference between the first and third year k values of fine roots was approximately two-fold in all of the studied stands except for the Oxalis spruce stand where the difference was 1.3 fold. The average fine root decomposition rates did not differ significantly between the Scots pine and Norway spruce stands in any of the study years.
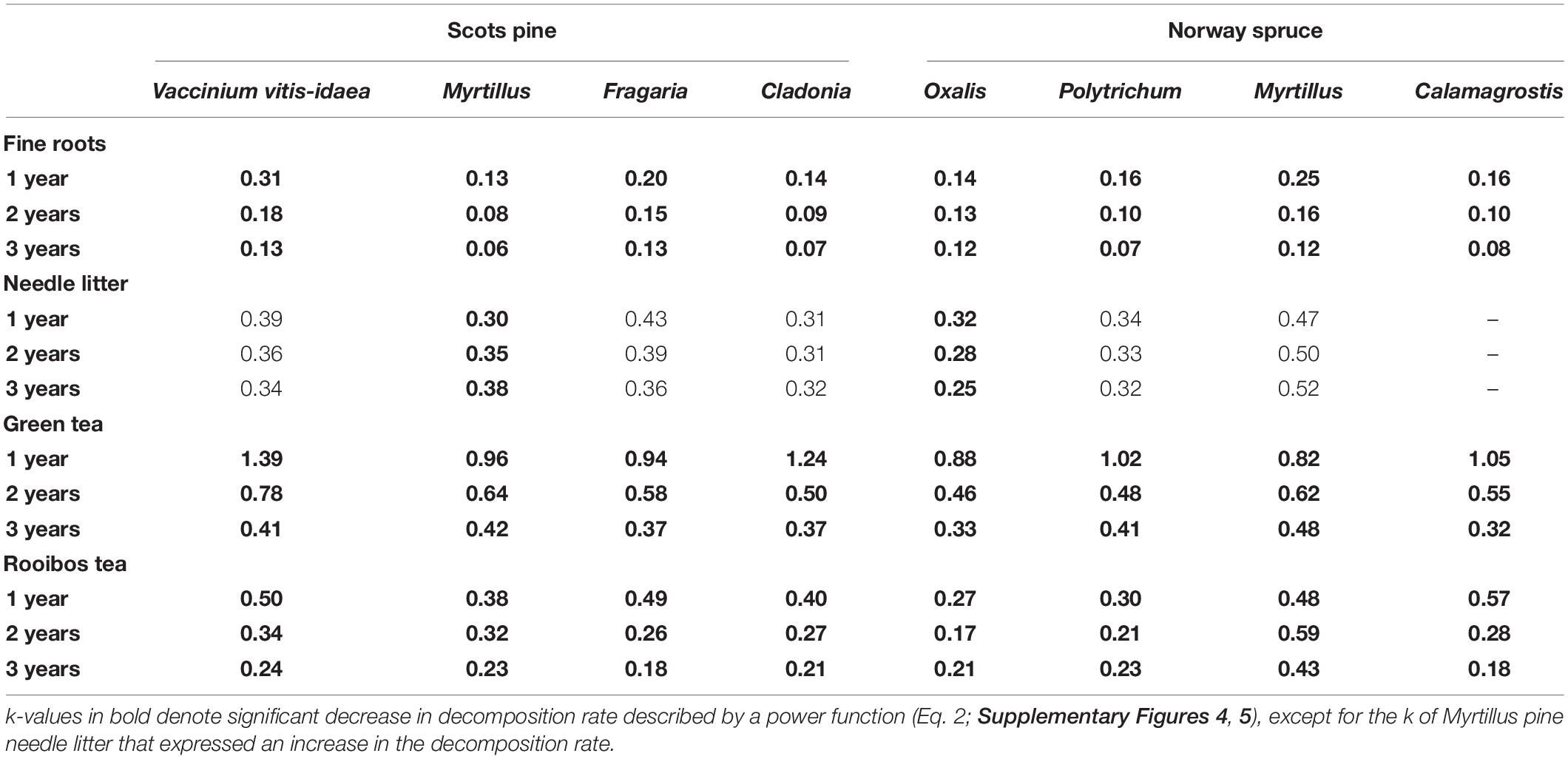
Table 5. The modeled decomposition rates (k; Eq. 1) of the fine roots, needle litter and green and rooibos tea at time 1, 2, and 3 years after start of the incubation.
The k function of needle litter was not significantly time-dependent, except for the Oxalis spruce and Myrtillus pine stands; in the latter however, k increased with time (Table 5).
The decomposition rate of green tea was on average three-fold higher compared to rooibos tea during the first 3 months (Supplementary Figure 5). The k values decreased in time for both tea substrates (p < 0.05; Table 5), however, the k of green tea remained significantly higher compared to rooibos tea in the first, second, and third year (p < 0.05). The range of k variation of tea substrates was higher in spruce stands compared to pine forests in all years, however, neither dominating tree species nor forest site type were significant.
The annual k values of fine roots, green and rooibos tea remained different (p < 0.001) along 3-year study period. The decomposition rate of fine roots and both tea substrates correlated positively (p < 0.01, r = 0.62–0.72), the strongest relationship was found between the decomposition rates of green tea and fine roots during 3-year study period (p < 0.001, r = 0.72).
Nutrient Dynamics of the Decomposing Fine Roots and Needle Litter
Nitrogen concentration increased significantly in the retrieved fine roots (p < 0.01, N = 56) and in the needle litter (p < 0.01, N = 42) during the 3-year decay period. Simultaneously, the C:N ratio of the decomposing needles (p < 0.01, N = 38) and fine roots (p = 0.02, N = 56) decreased over time.
The release rate of N correlated significantly with the initial C:N ratio in the fine roots (Table 6), indicating higher N release from roots, where the initial relative N content is higher. P release from the fine roots and needle litter both correlated negatively to the initial litter N:P ratio, while the RtP of fine roots also correlated with the initial litter P content. The release rate of K revealed a strong positive correlation with the initial N and K concentration in the needle litter.
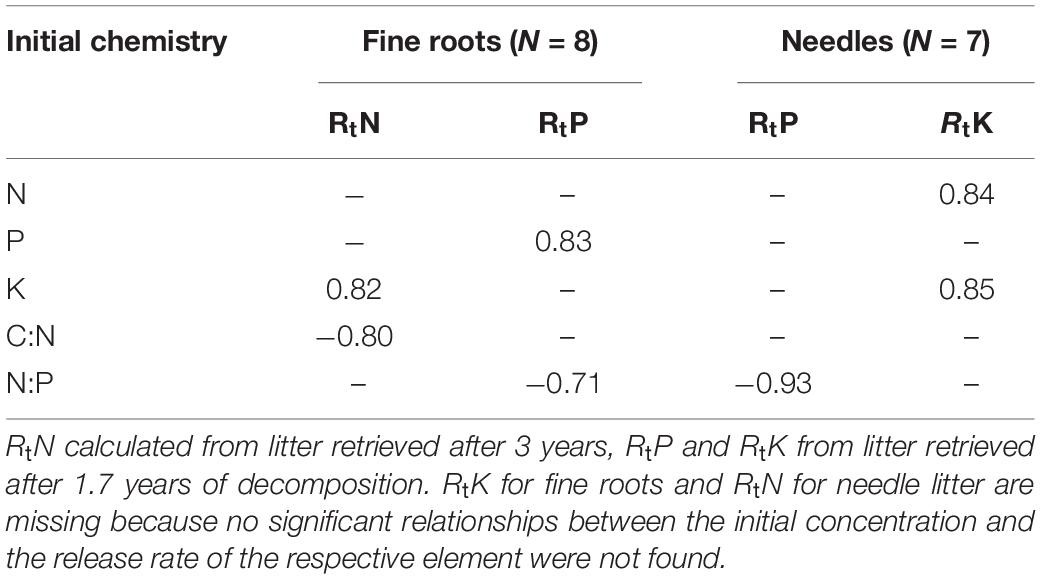
Table 6. Pearson correlations (p < 0.05) for the fine roots and needle litter initial chemistry, respectively, and nutrient release rates (Rt).
A loss of N could be observed in all the pine fine roots and in the fine roots of the Polytrichum spruce after the first month of incubation (Figure 2). Both, the needle litter and fine roots showed an increase in the absolute amount of nitrogen in the decomposing litter mass; however, a pattern of two rapid N immobilization peaks appeared in the retrieved fine roots at around 0.7 and 2 years after the start of the decomposition in most of the study sites. The decomposing fine roots immobilized up to 1.5 times (Myrtillus pine) and needle litter up to 1.1 times (Myrtillus spruce) of its initial N amount. The release of N after accumulation started in the critical N concentration range of 0.9–1.3% and 1.2–1.6% for the pine and spruce fine roots, respectively (Figure 2). For the needle litter, the critical level of N varied in the range of 0.7–1.1% and 1.5–1.9% for the pine and spruce, respectively. Irrespective of conifer species, fine roots retained relatively more N compared to the initial amount than the needles in all of the studied stands. The final N release was on average 11 ± 4% from fine roots and 34 ± 5% from needles at the end of the 3-year decomposition period.
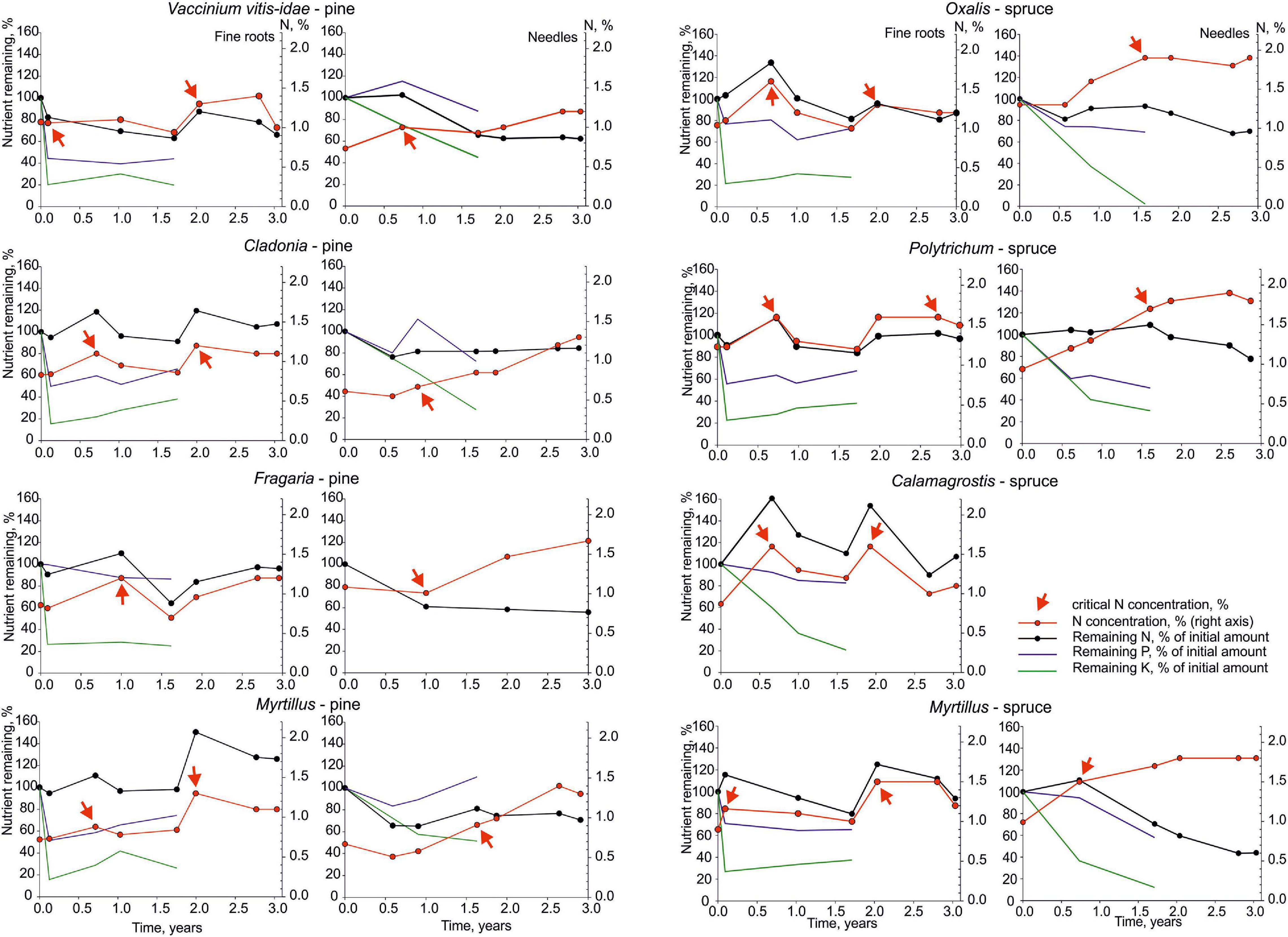
Figure 2. Changes in mass remaining (percentage of initial amount) of nitrogen (N; black line), phosphorus (P; blue line) and potassium (K; green line) in the incubated pine and spruce fine roots and needle litter (left axis). N concentration of the substrate is indicated with red line (right axis) and the critical N concentrations with red arrows.
There were no significant differences in the retention of P in the fine roots and needle litter after 1.7 years of incubation (70 ± 4% and 75 ± 8%, respectively, Figure 2). However, pine needles that also had low initial P concentrations (<400 mg kg–1, Table 2) displayed a net P accumulation, while the spruce needles that had high initial P concentration (>800 mg kg–1, Table 2) were constantly losing P from the beginning of the decomposition study.
Fine roots released K rapidly in the first month, losing approximately 80% of their initial amount of K. Although needle litter retained more K (51 ± 6%) than fine roots (33 ± 2%) 1 year after the start of the decomposition, the difference leveled out by the time of the retrieval (1.7 years of decomposition), when both litter types had 30% of the initial K remaining on average.
Discussion
Mass Loss and Decomposition Rate of Different Substrate Types and the Impact of Environmental Factors, Soil and Initial Substrate Chemistry
The substrate type and decomposition time are the main factors explaining the mass loss and the decomposition rate of all studied decomposing substrates. Alpha-cellulose was the most rapidly decaying litter type that nearly completely decomposed in 2 years indicating that cellulose utilizing soil microorganisms are not limiting decomposition in the studied stands. This is in good accordance with the results of López-Mondéjar et al. (2016) who showed cellulose hydrolysis to be a relatively common trait of microbes inhabiting forest litter and soil. The 3-month mass loss of green and rooibos tea was on average 66 ± 2% and 27 ± 2%, respectively in Estonia, while Djukic et al. (2018) found globally (across nine biomes) 3-month mass loss of green and rooibos tea 62 and 22%, respectively. Duddigan et al. (2020) analyzed chemical changes in both tea substrates during 90 days’ incubation, concluding that both green and rooibos tea are comparable with native litter decomposition. After 1 year of decomposition the mass loss of rooibos tea was approximately two-fold lower compared to green tea, on average 36 and 73% of their initial mass, respectively, which can be explained by higher fraction of water-soluble compounds in the green tea (Didion et al., 2016; Djukic et al., 2018). In the current study, the mass loss of the green tea was significantly related to the N:P ratio of the soil organic layer; however, the mass loss decreased with the relative increase of nitrogen. Green tea had a remarkably high initial N concentration, thus the surplus of N could have inhibited decay due to the suppression of lignolytic enzymes (Prescott, 2010). However, since green tea was low in lignin, the mass loss rate could have decreased due to the P limitation. The decomposition of labile, nutrient-rich organic matter is generally driven by fast-growing microbes with high metabolic activity (Fontaine et al., 2003) and such fast-growing microbes have particularly high demands for P because rapid cell division require large amounts of P-rich RNA (Elser et al., 2003).
During the first year of decomposition, the mass loss of site-specific needle litter and fine roots was significantly lower compared to standard substrates. The mass loss of needles during the first year varied from 25 to 35%, which falls in the mass loss range of 11–44% reported for pine and spruce needles in boreal coniferous forests (Berg and Staaf, 1980; Johansson, 1994; Kurka, 2001; Prescott et al., 2004). The average mass loss of needle litter reached the same level as rooibos tea after 2 years of decomposition. However, the needle litter decomposition exceeded annual mass loss of the rooibos tea about 10% after the third year of decomposition, while the mass loss of rooibos tea slowed down and stabilized at a mass loss decrease rate of 9–10% per year after the first year of decomposition.
The mass loss of fine roots was the lowest across the studied substrate types throughout the study period, except for the first month since incubation when α-cellulose expressed lower mass loss. Our findings are in agreement with results found in the literature showing that roots decompose up to 10 times more slowly than leaf litter due to their higher concentrations of lignin and suberin (Silver and Miya, 2001; Freschet et al., 2013; Kyaschenko et al., 2019). An earlier study conducted in Estonia by Lõhmus and Ivask (1995) reported that the fine roots of Norway spruce lost 21–33% of their dry weight after 1 year of incubation, which is higher than the average mass loss of spruce fine roots (17 ± 2%) in the current study. However, the average site productivity of the stands in Lõhmus and Ivask (1995) was higher compared to the stands in the current study. This is in good agreement with our results that fine roots decompose faster in stands with higher productivity.
Our study revealed that the decay rate k of two native litter and two tea types as standard substrates ranged from 0.19 ± 0.02 to 1.04 ± 0.07 in the first year, from 0.12 ± 0.01 to 0.58 ± 0.04 in the second year and from 0.10 ± 0.01 to 0.39 ± 0.02 in the third year being lowest for fine roots and highest for green tea in the hemiboreal coniferous forests throughout the whole study period. According to exponential decay model (Eq. 1; Lõhmus and Ivask, 1995) the k dynamics (Eq. 2) of green tea can be used to predict the rate of fine root decomposition, which was approximately five times lower during the studied 3-year period. After an initial short and fast decomposition phase within the first year, both substrates reached the plateau with a very low decomposition rate. Duddigan et al. (2020) suggests that microbial community cannot exploit part of the remaining carbohydrates in green tea that can be stabilized during decomposition rendering them resistant to decay, or waxes and lipids containing fraction of alkyl C that require specific enzymes to decompose them (Baumann et al., 2009).
The decay rate of all substrates varied across studied forest sites, especially in the early stage of decomposition. However, we failed to prove our second hypothesis that the decomposition rate of standard substrates depends on forest site or dominating conifer species. Since the second year after the installation, the decomposition rate of both green tea and fine roots was highest in Vaccinium vitis-idaea pine forest coinciding with highest stand productivity among pine dominating stands, high initial N contents of both substrates and the lowest lignin:cellulose ratio of fine roots. The differences in the fine root decomposition rate can be related to the different initial concentrations of lignin in the fine roots and differences in root colonizing ectomycorrhizal communities. In the overview by Zhang and Wang (2015), the initial lignin content was reported to be the most important predictor of fine root decomposition.
In the current study, the annual decomposition rate changed in time for all substrates, except needle litter, which decomposition rate remained almost constant for 3 years and no deceleration was achieved. In accordance with several studies stating that foliar litter degradation in the early stage is enhanced by higher concentrations of N (e.g., Berg and Staaf, 1980; Berg and Matzner, 1997; Berg, 2014), the 1-year mass loss of Scots pine needles correlated positively with the initial N content of litter, the positive trend for the spruce needles was non-significant.
The concentration of N along with other nutrients such as P and K in the incubated substrate, and soil organic carbon, soil P and soil K concentrations expressed a significant impact on the later stage decomposition, i.e., on the 3-year mass loss of fine root and needle litter. The positive effect of macronutrients (N, P) on the decomposition of plant litter has been presented in a wide range of studies (e.g., Aerts, 1997; Berg, 2000; Błońska et al., 2021). K in conifer needle litter has been shown to have a positive impact on mass loss both in the early (Prescott et al., 2004) and late stages (Berg et al., 2000) of decomposition. In our study, the higher initial K of the incubated fine roots and needle litter significantly increased the mass loss in the first year, while higher K concentrations in the soil organic layer expressed a negative effect on the mass loss of needle litter and green tea (Supplementary Figure 3). Ochoa-Hueso et al. (2019) found that K addition significantly affected the ability of microbes to degrade organic matter, suggesting the potential key role for soil K in controlling litter decomposition. In our study, soil K correlated with soil pH which in turn strongly influences the biomass, composition (fungi:bacteria ratio) and activity of soil microorganisms (e.g., Högberg et al., 2007; Matthies et al., 2007).
According to the concept of thresholds (Prescott, 2010), single factors, i.e., certain critical levels of temperature, moisture, lignin and litter nutrient concentrations control the rate of decomposition. Our study sites were likely to represent the range of environmental and soil nutrient conditions in hemiboreal coniferous forests, however no single variable was found to determine the mass loss of each studied substrate type. However, in the later stage when nutrients started to deplete, the concentrations of litter macronutrients (N, P, and K) and soil TOC, P, and K had a significant impact on the mass loss of the site-specific substrates. This suggests that analyzing single factors may not be sufficient, instead the combined effect of environmental parameters, litter quality and soil chemistry might reveal the prevailing factors that drive the rate of decomposition. The latter assumption is also supported by Prescott (2010) and Zhang et al. (2008) who argue that single factors, e.g., lignin and nutrient concentrations are useful for predicting mass loss rate, however they do not determine the decay rate of litter on their own.
Nutrient Dynamics and Net Release During Decomposition
The release pattern of N, P, and K in the decomposing fine roots and needle litter differed considerably with the element. Our results confirmed the existence of a critical N concentration for net N-release from decomposing substrate (Ågren and Bosatta, 1996). Well-observable dynamics of N release-immobilization occurred in the incubated fine roots but the pattern was of smaller magnitude and less clear in the needle litter, even though the initial N concentrations were similar in both substrate types. Our results show lower critical N values for the fine roots and needle litter of Scots pine (0.7–1.3% N) compared to Norway spruce (1.2–1.9% N); however, this is in good accordance with the critical N concept by Ågren and Bosatta (1996), who predicted the N release to start in the range from 1.4 to 1.7% N.
Nitrogen accumulation in the decomposing fine roots displayed in most of the stands a two-peaked pattern as described by Melillo et al. (1984). The initial leaching of the soluble fine root components is followed by rapid microbial growth during which nitrogen-enriched compounds are formed and N is immobilized in the detritus-microbe complex. The first stage of net N accumulation occurred at around 0.7 years (8 months) which is in agreement with other studies reporting peak N immobilization in decomposing fine roots between 6 and 10 months after incubation (Lõhmus and Ivask, 1995; Chen et al., 2002). Due to the recondensation of the lignin degradation products with nitrogenous compounds in the later stage of decomposition, additional nitrogen-rich recalcitrant compounds were produced, causing the effect of the second-phase immobilization. The later stage N accumulation peak of fine roots appeared at around 2 years after the start of the incubation, which falls in the time range of 1.4 and 2.3 years reported for the Norway spruce fine and finest roots (<1 mm), respectively, in the decomposition study carried out in Estonia by Lõhmus and Ivask (1995).
The percentage of remaining N at the end of the 3-year study was higher in the fine roots compared to the needle litter at every study site, indicating to a longer retention of N in the fine roots. The delay of N release in fine roots as compared to foliar decomposition is in line with several studies (Fujii and Takeda, 2010; Luo et al., 2017), emphasizing that fine roots have a significant role in nitrogen retention in forest ecosystems.
The pattern of P release is more variable, depending on litter type and site, both initial loss and gain of P have been widely reported (Moore et al., 2006). A fast loss of P was observed both in the fine root and needle litter with high initial P content, which is consistent with previous studies (Johansson, 1993) that reported initial P leaching from P-rich litters. In the needle litter however, a remarkable net gain of phosphorus occurred in the P-poor pine needles, while spruce needles with high initial P content expressed a gradual net loss of phosphorus. The net uptake of P in decomposing pine needles has been demonstrated by Berg and Cortina (1995), confirming that if carbon is relatively available, the immobilization of a limiting nutrient is common during litter decomposition (Prescott, 2005).
Potassium is not a structural component of plant litter or bound into any known organic compounds (Aber and Melillo, 2001), thus it was most rapidly lost from the incubated fine root and needle litter, as found in many studies (e.g., Berg and Cortina, 1995; Lin et al., 2011). After about 1 month of decomposition, approximately 80% of K was lost from the fine roots, irrespective of the initial concentration. Needle litter, however, expressed a slower K release, probably because the sampling interval was less frequent compared to the fine roots, especially at the beginning of the incubation.
Conclusion
The substrate type has the strongest impact on mass loss of all standard (α-cellulose, green and rooibos tea) and site-specific substrates (needle litter and fine roots) in hemiboreal pine and spruce forests. The dominant conifer species’ do not affect the decomposition rate of substrate or the proportional release of N from the litter. Our study revealed that the rate of the fine root decomposition can be predicted using the decomposition model for green tea, however, during the first 3 years the fine root decomposition rate is approximately five times lower than the rate of green tea. The annual decomposition rate of the needle litter is rather constant in the first 3 years and any of the decay rate models for standard substrates cannot be applied for describing the decomposition process of the needle litter in hemiboreal coniferous forests.
Despite the effect of faster litter mass loss with higher initial substrate N concentration and the additional explanatory effect of site productivity as well as soil K content on decomposition, there was no single environmental characteristic that would predict the mass loss of all the studied substrate types. However, the soil macronutrients (P, K) and TOC content became increasingly important with time, having a considerable influence in conjunction with initial litter composition on the third year decomposition of fine roots and needle litter.
We determined critical N concentrations of fine roots and needle litter at which level the net release of nitrogen begins in hemiboreal coniferous forests. The critical N concentration for N release was clearly lower for Scots pine fine roots and needle litter, (corresponding ranges were 0.9–1.3% and 0.7–1.1%) than for the Norway spruce (1.2–1.6% and 1.5–1.6%). Nitrogen release from litter or its accumulation into the decaying litter fluctuated over 3 years of decomposition, however, the integrated release-accumulation flux resulted with three times lower N release from fine roots than from needle litter. The slower decomposition and higher retention of N in the fine roots relative to needle litter suggests that fine roots have a substantial role in the carbon and nitrogen accumulation in boreal and hemiboreal forest ecosystems.
Data Availability Statement
The raw data supporting the conclusions of this article will be made available by the authors, without undue reservation.
Author Contributions
KK, JF, EA, and IO planned and designed the research. KK, JF, EA, NK, ÜN, and IO participated on preparation, installation and collection of soil, alpha-cellulose, and fine root and leaf litter samples. KK and IO performed the tea bag research. KL provided with alpha-cellulose. KK completed the final dataset, wrote the manuscript, with inputs from IO and helpful comments from the all authors. KK, KL, and IO performed data analyses. All authors contributed to the article and approved the submitted version.
Funding
This study was funded by the Environmental Investment Centre project No 6250, the Estonian Research Council grants IUT2-16 and PRG916, the CAR-ES Nordic Network, the EU via the European Regional Development Fund (Center of Excellence ENVIRON and EcolChange).
Conflict of Interest
The authors declare that the research was conducted in the absence of any commercial or financial relationships that could be construed as a potential conflict of interest.
Acknowledgments
We thank the staff of Estonian Environment Agency for the collection and processing of needle litter samples and for providing background information of the study sites. We are grateful to Aime Randveer and Igor Miilvee for assistance in the laboratory.
Supplementary Material
The Supplementary Material for this article can be found online at: https://www.frontiersin.org/articles/10.3389/ffgc.2021.686468/full#supplementary-material
Footnotes
References
Aber, J. D., and Melillo, J. M. (2001). Terrestrial Ecosystems, 2nd Edn. San Diego CA: Harcourt-Academic Press.
Aerts, R. (1997). Climate, leaf litter chemistry and leaf litter decomposition in terrestrial ecosystems: a triangular relationship. Oikos 79, 439–449. doi: 10.2307/3546886
Ågren, G. I., and Bosatta, E. (1996). Theoretical Ecosystem Ecology. Understanding Element Cycles. Cambridge: Cambridge University Press.
Baumann, K., Marschner, P., Smernik, R. J., and Baldock, J. A. (2009). Residue chemistry and microbial community structure during decomposition of eucalypt, wheat and vetch residues. Soil Biol. Biochem. 41, 1966–1975. doi: 10.1016/j.soilbio.2009.06.022
Berg, B. (2000). Litter decomposition and organic matter turnover in northern forest soils. For. Ecol. Manage. 133, 13–22. doi: 10.1016/s0378-1127(99)00294-7
Berg, B. (2014). Decomposition patterns for foliar litter – a theory for influencing factors. Soil. Biol. Biochem. 78, 222–232. doi: 10.1016/j.soilbio.2014.08.005
Berg, B., Berg, M. P., Bottner, P., Box, E., Breymeyer, A., Calco de Anta, R., et al. (1993). Litter mass loss in pine forests of Europe and Eastern United States: some relationships with climate and litter quality. Biogeochemistry 20, 127–153. doi: 10.1007/bf00000785
Berg, B., and Cortina, J. (1995). Nutrient dynamics in some leaf and needle litter types of different chemical composition in a Scots pine forest. Scand. J. For. Res. 10, 1–11. doi: 10.1080/02827589509382860
Berg, B., Johansson, M.-B., and Meentemeyer, V. (2000). Litter decomposition in a transect of Norway spruce forests: substrate quality and climate control. Can. J. For. Res. 30, 1136–1147. doi: 10.1139/cjfr-30-7-1136
Berg, B., and Matzner, E. (1997). Effect of N deposition on decomposition of plant litter and soil organic matter in forest systems. Environ. Rev. 5, 1–25. doi: 10.1139/a96-017
Berg, B., and McClaugherty, C. (2008). Plant Litter: Decomposition, Humus Formation, Carbon Sequestration. Berlin: Springer-Verlag.
Berg, B., and Staaf, H. (1980). Decomposition rate and chemical changes in decomposing needle litter of Scots pine. II. Influence chem. Composition. Ecol. Bull. 32, 373–390.
Błońska, E., Piaszczyk, W., Staszel, K., and Lasota, J. (2021). Enzymatic activity of soils and soil organic matter stabilization as an effect of components released from the decomposition of litter. Applied Soil Ecol. 157:103723. doi: 10.1016/j.apsoil.2020.103723
Bradford, M. A., Berg, B., Maynard, D. S., Wieder, W. R., and Wood, S. A. (2016). Understanding the dominant controls on litter decomposition. J. Ecol. 104, 229–238.
Brække, F. H., and Finér, L. (1990). Decomposition of cellulose in litter layer and surface peat of low-shrub pine bogs. Scand. J. For. Res. 5, 297–310. doi: 10.1080/02827589009382614
CANOCO program, ter Braak, C. J. F., and Šmilauer, P. (2002). CANOCO Reference Manual and CanoDraw for Windows User’s Guide: Software for Canonical Community Ordination (Version 4.5). Ithaca, NY: Microcomputer Power.
Carreiro, M. M., Sinsabaugh, R. L., Repert, D. A., and Parkhurst, D. F. (2000). Microbial enzyme shifts explain litter decay responses to simulated nitrogen deposition. Ecology 81, 2359–2365.
Chapin, F. S. III, Matson, P., and Mooney, H. A. (2002). Principles of Terrestrial Ecosystem Ecology. New York NY: Springer-Verlag.
Chen, H., Harmon, M. E., Sexton, J., and Fasth, B. (2002). Fine root decomposition and N dynamic in coniferous forests of the Pacific Northwest. USA. Can. J. For. Res. 32, 320–331. doi: 10.1139/X01-202
Coleman, D. C., Blair, J. M., Eliott, E. T., and Freckman, D. W. (1999). “Soil invertebrates,” in Standard Soil Methods for Long-Term Ecological Research, eds P. Sollins, G. P. Robertson, C. S. Bledsoe, and D. C. Coleman (New York NY: Oxford University Press), 349–377.
Couteaux, M. M., Bottner, P., and Berg, B. (1995). Litter decomposition, climate and litter quality. Trends Ecol. Evol. 10, 63–66. doi: 10.1016/S0169-5347(00)88978-8
Cusack, D. F., Chou, W. W., Yang, W. H., Harmon, M. E., Silver, W. L., and The Lidet Team. (2009). Controls on long-term root and leaf litter decomposition in neotropical forests. Glob. Chang. Biol. 15, 1339–1355. doi: 10.1111/j.1365-2486.2008.01781.x
DeLuca, T. H., and Boisvenue, C. (2012). Boreal forest soil carbon: distribution, function and modelling. Forestry 85, 161–184. doi: 10.1093/forestry/cps003
Didion, M., Repo, A., Liski, J., Forsius, M., Bierbaumer, M., and Djukic, I. (2016). Towards harmonizing leaf litter decomposition studies using standard tea bags—a field study and model application. Forests 7:167. doi: 10.3390/f7080167
Djukic, I., Kepfer Rojas, S., Schmidt, I. K., Larsen, K. S., Beier, C., Berg, B., et al. (2018). Early stage litter decomposition across biomes. Sci. Total Environ. 628–629, 1369–1394. doi: 10.1016/j.scitotenv.2018.01.012
Duddigan, S., Shaw, L. J., Alexander, P. D., and Collins, C. D. (2020). Chemical underpinning of the Tea Bag Index: An examination of the decomposition of tea leaves. Applied Envir. Soil Sci. 2020:6085180. doi: 10.1155/2020/6085180
EEA (2006). European Forest Types. Categories and Types for Sustainable Forest Management and Reporting. European Environment Agency, EEA Technical Report No. 9/2006. ISSN 1725-2237. Available online at: http://www.foresteurope.org/docs/other_meetings/2006/wfc/WFC_4_eea_technical_report_92006.pdf. (accessed May 10, 2021).
Elser, J. J., Acharya, K., Kyle, M., Cotner, J., Makino, W., Markow, T., et al. (2003). Growth rate-stoichiometry couplings in diverse biota. Ecol. Lett. 6, 936–943. doi: 10.1046/j.1461-0248.2003.00518.x
Fontaine, S., Mariotti, A., and Abbadie, L. (2003). The priming effect of organic matter: a question of microbial competition? Soil Biol. Biochem. 35, 837–843. doi: 10.1016/S0038-0717(03)00123-8
Freschet, G. T., Cornwell, W. K., Wardle, D. A., Elumeeva, T. G., Liu, W., Jackson, B. G., et al. (2013). Linking litter decomposition of above- and below-ground organs to plant–soil feedbacks worldwide. J. Ecol. 101, 943–952. doi: 10.1111/1365-2745.12092
Fujii, S., and Takeda, H. (2010). Dominant effects of litter substrate quality on the difference between leaf and root decomposition process above-and belowground. Soil Biol. Biochem. 42, 2224–2230. doi: 10.1016/j.soilbio.2010.08.022
Heim, A., and Frey, B. (2004). Early stage litter decomposition rates for Swiss forests. Biogeochemistry 70, 299–313. doi: 10.1007/s10533-003-0844-5
Högberg, M. N., Högberg, P., and Myrold, D. D. (2007). Is microbial community composition in boreal forest soils determined by pH. C-to-N ratio, the trees, or all three? Oecologia 150, 590–601. doi: 10.1007/s00442-006-0562-5
Howard, P. J. A. (1988). “A critical evaluation of the cotton strip assay,” in Cotton Strip Assay: An Index of Decomposition in Soils, eds A. F. Harrison, P. M. Latter, and D. W. H. Walton (Great Britain: Institute of Terrestrial Ecology Symposium no. 24, Grange-over-Sands: Institute of Terrestrial Ecology), 34–42.
Johansson, M. (1993). Biomass, decomposition and nutrient release of Vaccinium myrtillus leaf litter in four forest stands. Scan. J. For. Res. 8, 466–479. doi: 10.1080/02827589309382793
Johansson, M. B. (1994). Decomposition rates of Scots pine needle litter related to site properties, litter quality, and climate. Can. J. For. Res. 24, 1771–1781. doi: 10.1139/x94-229
Keuskamp, J. A., Dingemans, B. J. J., Lehtinen, T., Sarneel, J. M., and Hefting, M. M. (2013). Tea bag index: a novel approach to collect uniform decomposition data across ecosystems. Methods Ecol. Evol. 4, 1070–1075. doi: 10.1111/2041-210X.12097
Killham, K., and Prosser, J. (2015). “The bacteria and archaea,” in Soil Microbiology, Ecology and Biochemistry, 4th Edn, ed. E. A. Paul (Cambridge MA: Academic Press), 41–76.
Kögel-Knabner, I. (2002). The macromolecular organic composition of plant and microbial residues as inputs to soil organic matter. Soil Biol. Biochem. 34, 139–162. doi: 10.1016/S0038-0717(01)00158-4
Kriiska, K., Frey, J., Asi, E., Kabral, N., Uri, V., Aosaar, J., et al. (2019). Variation in annual carbon fluxes affecting the SOC pool in hemiboreal coniferous forests in Estonia. For. Ecol. Management 433, 419–430. doi: 10.1016/j.foreco.2018.11.026
Kurka, A. M. (2001). The use of cellulose strips to study organic matter decomposition in boreal forested soils. Boreal Environ. Res. 6, 9–17.
Kurka, A. M., Starr, M., Karsisto, M., and Salkinoja-Salonen, M. (2001). Relationship between decomposition of cellulose strips and chemical properties of humus layer in natural boreal forests. Plant Soil 229, 137–146. doi: 10.1023/A:1004887819813
Kyaschenko, J., Ovaskainen, O., Ekblad, A., Hagenbo, A., Karltun, E., Clemmensen, K. E., et al. (2019). Soil fertility in boreal forest relates to root-driven nitrogen retention and carbon sequestration in the mor layer. New Phytol. 221, 1492–1502. doi: 10.1111/nph.15454
Lin, C., Yang, Y., Guo, J., Chen, G., and Xie, J. (2011). Fine root decomposition of evergreen broadleaved and coniferous tree species in mid-subtropical China: dynamics of dry mass, nutrient and organic fractions. Plant Soil 338, 311–327. doi: 10.1007/s11104-010-0547-3
Lõhmus, K., and Ivask, M. (1995). Decomposition and nutrient dynamics of fine roots of Norway spruce (Picea abies (L.) Karst.) at different sites. Plant Soil 16, 89–94. doi: 10.1007/BF00029317
López-Mondéjar, R., Zühlke, D., Becher, D., Riedel, K., and Baldrian, P. (2016). Cellulose and hemicellulose decomposition by forest soil bacteria proceeds by the action of structurally variable enzymatic systems. Sci. Rep. 6:25279. doi: 10.1038/srep25279
Luo, D., Cheng, R. M., Shi, Z. M., and Wang, W. X. (2017). Decomposition of leaves and fine roots in three subtropical plantations in china affected by litter substrate quality and soil microbial community. Forests 8:412. doi: 10.3390/f8110412
Matthies, C., Erhard, H. P., and Drake, H. L. (2007). Effects of pH on the comparative culturability of fungi and bacteria from acidic and less acidic forest soils. J. Basic Microbiol. 37, 335–343. doi: 10.1002/jobm.3620370506
Melillo, J. M., Naiman, R. J., Aber, J. D., and Linkins, A. E. (1984). Factors controlling mass loss and nitrogen dynamics of plant litter decaying in northern streams. Bull. Mar. Sci. 35, 341–356.
Moore, T. R., Trofymow, J. A., Prescott, C. E., Fyles, J., Titus, B. D., and Cidet Working Group. (2006). Patterns of carbon, nitrogen and phosphorus dynamics in decomposing foliar litter in Canadian forests. Ecosystems 9, 46–62. doi: 10.1007/s10021-004-0026-x
Ochoa-Hueso, R., Delgado-Baquerizo, M., An King, P. T., Benham, M., Arca, A., and Power, S. A. (2019). Ecosystem type and resource quality are more important than global change drivers in regulating early stages of litter decomposition. Soil Biol. Biochem. 129, 144–152. doi: 10.1016/j.soilbio.2018.11.009
Olson, J. S. (1963). Energy storage and the balance of producers and decomposers in ecological systems. Ecology 44, 322–331. doi: 10.2307/1932179
Prescott, C., Maynard, D. G., and Laiho, R. (2000). Humus in northern forests: friend or foe? For. Ecol. Manag. 133, 23–36. doi: 10.1016/S0378-1127(99)00295-9
Prescott, C. E. (2005). “Decomposition and mineralization of nutrients from litter and humus,” in Nutrient Acquisition by Plants, ed. H. BassiriRad (Berlin: Springer), 15–41.
Prescott, C. E. (2010). Litter decomposition: what controls it and how can we alter it to sequester more carbon in forest soils? Biogeochemistry 101, 133–149. doi: 10.1007/s10533-010-9439-0
Prescott, C. E., Vesterdal, L., Preston, C. M., and Simard, S. W. (2004). Influence of initial chemistry on decomposition of foliar litter in contrasting forest types in British Columbia. Can. J. For. Res. 34, 1714–1729. doi: 10.1139/X04-040
Sardans, J., and Penuelas, J. (2021). Potassium Control of Plant Functions: Ecological and Agricultural Implications. Plants 10:419. doi: 10.3390/plants10020419
Silver, W. L., and Miya, R. K. (2001). Global patterns in root decomposition: Comparisons of climate and litter quality effects. Oecologia 129, 407–419. doi: 10.1007/s004420100740
Valgepea, M., Sims, A., Raudsaar, M., and Timmusk, T. (2017). “Raied [fellings],” in Aastaraamat mets 2017 [Yearbook Forest 2016], eds M. Raudsaar, K. L. Siimon, and M. Valgepea (Tartu: Estonian Environment Agency), 293. (In Estonian).
Wardle, D. A., Bardgett, R. D., Klironomos, J. N., Setälä, H., Van Der Putten, W. H., and Wall, D. H. (2004). Ecological linkages between aboveground and belowground biota. Science 304, 1629–1633. doi: 10.1126/science.1094875
Zhang, D., Hui, D., Luo, Y., and Zhou, G. (2008). Rates of litter decomposition in terrestrial ecosystems: global patterns and controlling factors. J. Plant Ecol. 1, 85–93. doi: 10.1093/jpe/rtn002
Keywords: nutrient release, tea bag, cellulose, needle litter, fine root, decomposition, forest soil
Citation: Kriiska K, Lõhmus K, Frey J, Asi E, Kabral N, Napa Ü and Ostonen I (2021) The Dynamics of Mass Loss and Nutrient Release of Decomposing Fine Roots, Needle Litter and Standard Substrates in Hemiboreal Coniferous Forests. Front. For. Glob. Change 4:686468. doi: 10.3389/ffgc.2021.686468
Received: 26 March 2021; Accepted: 21 June 2021;
Published: 14 July 2021.
Edited by:
Nicolas Fanin, INRAE Nouvelle-Aquitaine Bordeaux, FranceReviewed by:
Mark Bakker, Ecole Nationale Supérieure des Sciences Agronomiques de Bordeaux Aquitaine, FranceMarkus Didion, Swiss Federal Institute for Forest, Snow and Landscape Research (WSL), Switzerland
Dunmei Lin, Chongqing University, China
Copyright © 2021 Kriiska, Lõhmus, Frey, Asi, Kabral, Napa and Ostonen. This is an open-access article distributed under the terms of the Creative Commons Attribution License (CC BY). The use, distribution or reproduction in other forums is permitted, provided the original author(s) and the copyright owner(s) are credited and that the original publication in this journal is cited, in accordance with accepted academic practice. No use, distribution or reproduction is permitted which does not comply with these terms.
*Correspondence: Ivika Ostonen, aXZpa2Eub3N0b25lbkB1dC5lZQ==