- 1Institute for Atmospheric and Earth System Research/Physics, Faculty of Science, University of Helsinki, Helsinki, Finland
- 2Atmospheric Composition Research, Finnish Meteorological Institute, Helsinki, Finland
- 3School of Civil and Environmental Engineering, Harbin Institute of Technology, Shenzhen, China
Biogenic volatile organic compounds (BVOCs) are known to strongly influence the global climate by affecting various atmospheric constituents such as oxidants and aerosols. Among the several BVOCs that are emitted continuously into the atmosphere, studies have shown that up to 96% of the emissions have been missed out by current analytical techniques. In this study, we used a Vocus proton-transfer-reaction time-of-flight mass spectrometer (Vocus) to characterize and quantify emissions from a branch of a downy birch tree at a boreal forest site in Hyytiälä, Finland in August 2019. During the measurement period, we were able to observe real-time emissions of hydrocarbons with up to 20 carbon atoms and oxygenated compounds (OVOCs) with up to 4 oxygen atoms. OVOCs accounted for around 90% of the total observed emissions with the largest contribution from C8H8O3 (0.37 μgg–1h–1; ∼60% of total). For the first time, emissions of diterpenes (C20H32, C20H36, and C20H38) were observed from downy birch tree, although in minor quantities (0.1% of total emissions). During this late growing season, C10H16 and C10H14 contributed ∼7% in total emissions, while the sum of C5H8, C15H22, and C15H24 contributed around ∼3%. The branch experienced abiotic stress during the measurement period, which might explain the unusually high emissions of C8H8O3. Standardized emission potentials are reported for all compounds using two Guenther algorithms. While emissions of most compounds fit well with either of the two algorithms, emissions of certain compounds like C8H8O3 could not be explained by either suggesting the influence of other factors besides temperature and light. Vocus PTR-TOF-MS can help identify a diverse range of molecules even if emitted in minute quantities. The BVOCs detected from birch emissions may be important in the formation of secondary organic aerosols but their implications in the atmosphere need to be verified with further studies.
Introduction
Volatile organic compounds (VOCs) are emitted into the atmosphere from various biogenic and anthropogenic sources. The global annual biogenic VOC (BVOC) emissions are calculated to be ca. 1,000 Tg C, while the global anthropogenic estimation is suggested to be ten times lower (Simon et al., 2001). A diverse array of compounds has been identified to be emitted from vegetation. The major chemical classes can be classified into terpenoids (isoprene, monoterpenoids, and sesquiterpenoids), green leaf volatiles (GLVs; C6-aldehydes, -alcohols and their acetates), aromatic compounds such as benzenoids, and short-chained BVOCs (methanol, acetone, acetic acid, acetaldehyde, etc.). To avoid confusion, in this manuscript the word terpenes will refer to only the basic hydrocarbons, while the word terpenoids will refer to the whole group that includes both basic terpenes and a modified class of terpenes (oxygenated terpenes). These compounds have been recognized to have prominent effects on the atmosphere, with their lifetimes varying from seconds to days (Roger Atkinson and Arey, 2003). In the atmosphere, BVOCs can be oxidized by the hydroxyl radical (OH), nitrate radical (NO3) and tropospheric ozone (Li et al., 2018; Šimpraga et al., 2019). These oxidation processes lead to the production of various compounds that are less volatile, including Highly Oxygenated Molecules (HOMs) that are products formed in the atmosphere and contain multiple functional groups (Kirkby et al., 2016; Bianchi et al., 2019). The reaction products (e.g., HOMs) are known to contribute to new particle formation (NPF) and secondary organic aerosols (SOA) (Bianchi et al., 2019). SOA can help cool the Earth by scattering solar radiation thereby altering the net radiation balance and also act as cloud condensation nuclei (Yli-Juuti et al., 2021).
Besides their impact on the atmosphere, BVOCs also have important biological and ecological functions. They act as tools for communication within the plant community as well as between plants and insects. They also help to relieve plants from stress caused by biotic and abiotic factors. The quantity and quality of BVOCs emitted can describe the state of the vegetation (stressed and non-stressed). Factors like temperature, light intensity, carbon dioxide, ozone, nutrients, and other factors like insect infestation can shift the BVOC emission dynamics (Hartikainen et al., 2009; Loreto and Schnitzler, 2010; Ormeño and Fernandez, 2012). As a result of these biotic and abiotic stresses, constitutive BVOC emissions are either enhanced or inhibited (Toome et al., 2010; Kleist et al., 2012; Jiang et al., 2016; Guidolotti et al., 2019; Yu et al., 2021). However, various stress elements, especially herbivory damage or pathogen infestation, can lead to stimulation of biochemical pathways that can induce a new blend of BVOCs (Blande et al., 2007; Aartsma et al., 2017; Guidolotti et al., 2019). For example, GLVs are a class of compounds that are emitted almost immediately after any stress that cause membrane damage to plants (Šimpraga et al., 2019).
While more than 1700 BVOCs have been recognized to originate from plant emissions (Faiola and Taipale, 2020), there remains a large chunk that is yet to be characterized. Recent studies concerning total OH reactivity especially in the boreal region have revealed the huge fraction of reactivity that remained unexplained. The following studies present a fact that using the current measurement techniques several reactive species emitted directly from trees are overlooked particularly during periods of stress. In a study held in Hyytiälä, Finland in 2008, on average, 50% of measured total OH reactivity could not be explained (Sinha et al., 2010). Total OH reactivity measurements from direct emissions of Norway spruce revealed 84% to be missing during late summer and autumn, especially during periods of heat-stress (Nölscher et al., 2013). In a study by Praplan et al. (2020), missing fraction of OH reactivity from birch emissions remained as high as 96% constantly.
While some trees in the boreal zone like Norway spruce and Scots pine have been studied extensively for their BVOC emissions, studies involving birch species mostly revolve around terpenoids namely monoterpenoids and sesquiterpenoids (Hakola et al., 1998, 2001; Vuorinen et al., 2005; Haapanala et al., 2009). However, diverse VOCs have been observed from headspace of birch branches (Zhang et al., 1999), buds (Isidorov et al., 2019), and also in essential oils (Demirci et al., 2000). Recently, Hellén et al. (2021) observed compounds ranging from GLVs to oxygenated sesquiterpenes from the emissions of downy birch. The study was conducted in two different years starting from the budding stage to the late growing season. A high amount of sesquiterpenes and oxygenated sesquiterpenes were emitted during the growing season with considerable GLV emissions observed in times of stress. This is the only study to observe a diverse range of BVOCs to be emitted from birch over a long period.
Proton-transfer-reaction mass spectrometry (PTR-MS) provides high sensitivity and time resolution to observe the drastic changes from plant emissions of BVOCs. However, the sensitivities of traditional quadrupole PTR-MS (Blake et al., 2009) are not enough to measure compounds of high molecular weight such as diterpenes and oxygenated sesquiterpenes. In this study, we used a Vocus proton-transfer-reaction time-of-flight mass spectrometer (Vocus PTR-TOF-MS), which is an improvement over conventional PTR instruments with enhanced capability to detect a broader spectrum of VOCs (Krechmer et al., 2018; Riva et al., 2019; Li et al., 2020b). The Vocus PTR-TOF-MS was deployed in August 2019 during the late growing season in a boreal forest to measure emissions from downy birch. We report a comprehensive list of compounds, varying from hydrocarbons to oxygenated molecules with the number of oxygen atoms up to 4. This would be the first time that a wide range of different molecules have been detected in real time from direct birch emissions, highlighting the importance of birch in the boreal ecosystem.
Materials and methods
Measurement site
Biogenic volatile organic compound (BVOC) emissions from birch were measured at the SMEAR II station (Station for Measuring Forest Ecosystem-Atmosphere Relations) in Hyytiälä located in southern Finland (61°50’ N 24°17’ E, 181 m a.s.l.) (Hari and Kulmala, 2005). The site is mainly a forest with a few houses and fields in the surroundings. The forest is dominated by conifers, mainly Scots pine but also Norway spruce. The forest also has a minor population of birch and aspen. Emissions were observed from a young downy birch (Betula pubescens) tree which was roughly 4 m tall. Measurements were recorded for a period of 10 days, from 14 to 23 August in 2019, during Finnish late summer. It is expected that senescence of leaves started during this period (Hellén et al., 2021). All results mentioned in the study are in universal time coordinated (UTC) time standard.
Experimental setup
The sampling technique in this study was similar to that used by Hellén et al. (2021). The downy birch branches were placed inside a 6 L cylindrical Teflon (FEP) film branch chamber and the emission rates were measured using a dynamic flow through technique. The chamber was equipped with inlet and outlet ports and the temperature and relative humidity in the chamber were recorded with a thermistor (Philips KTY 80/110, Royal Philips Electronics, Amsterdam, Netherlands). The photosynthetically active radiation (PAR) was recorded on top of the enclosure using a quantum sensor (LI-190SZ, LI-COR, Biosciences, Lincoln, NE, USA).
Zero air produced by a zero air generator (HPZA-7000, Parker Hannifin Corporation) was pumped into the chamber at a rate of 7 L min–1 through FEP tubing. The zero air going into the chamber was humidified with ultrapure water to avoid dry conditions in the chamber. From the chamber outlet port, air was directed to the entrance of the Vocus PTR-TOF-MS at a rate of 2.5 L min–1 through a 3 m long FEP tubing. The main sampling line was heated to reduce wall losses in the tubing. While we assume wall losses in the sampling line and the chamber to be negligible due to the high flow rate, there could have been losses of compounds with high molecular weight causing underestimation in the emission rates.
Biogenic volatile organic compounds emission analysis
A Vocus PTR-TOF-MS (Tofwerk AG/Aerodyne Research Inc.) was deployed to quantify BVOC emissions from the birch tree. Compared to conventional PTR instruments (Blake et al., 2009), the Vocus PTR-TOF-MS, referred to thereafter as Vocus, is equipped with a new chemical ionization source with a low-pressure reagent ion source and focusing ion-molecule reactor, thus improving the detection efficiency of product ions (Krechmer et al., 2018; Li et al., 2020a). In this study, the ionization source of the Vocus was operated at 1.4 mbar. At this pressure, around 0.1 Lmin–1 of the total sample flow was directed into the Vocus, while the rest was used by other instruments. Krechmer et al. (2018) has given a detailed account on the dimensions and working of Vocus.
The instrument was automatically calibrated every 3 h using a calibration gas tank (Apel Riemer Environmental Inc.) containing 16 different compounds. The calibration gas tank included standards with the molecular formulas CxHy, CxHyOz, and CxHyNz. All compounds present in the calibration standard are listed in Supplementary Table 1. Isoprene and monoterpenes were quantified based on the calibration of their authentic standards. Other BVOCs with the formula of CxHy and CxHyOz were quantified using the average sensitivity of the standards C10H16 and C4H6O, respectively.
The data were recorded with a time resolution of 5s using TofDaq Recorder provided by Tofwerk AG. The data were processed using the software package Tofware developed by Tofwerk AG, that enables mass calibration, baseline subtraction and molecular formula assignment to the identified ions. The application runs in the Igor Pro environment (Wavemetrics, Lake Oswego, OR, USA). Further analyses of the data were done in Excel and Python. The ambient weather data of Hyytiälä was taken from FMI open weather data (URL: https://en.ilmatieteenlaitos.fi/download-observations; accessed on 22-04-2021).
Calculation of emission rates
The emission rate of BVOCs is defined as the mass of compounds emitted per gram from dried leaves and can be calculated using equation 1 (Hakola et al., 2001).
where E is the emission rate of BVOCs (μg g–1 h–1), C is the concentration of VOCs in the outgoing air (μg m–3), F is the flow rate of air through the enclosure (m3 h–1). The dry weight of the leaves is mDW (g). To determine dry weight, the leaves were cut and dried at 60°C for at least 24 h and then weighed.
Calculation of emission potentials
Biogenic volatile organic compound (BVOC) emissions are controlled by various factors such as leaf temperature, stomatal conductance, humidity, light, and others. The temperature-controlled emission of BVOCs from storage structures (pool emissions) and direct emissions (de novo emissions) of synthesized compounds that are dependent on both leaf temperature and light intensity have been used to effectively model BVOC emission in other studies (Tarvainen et al., 2005). In this study, emission potentials using both temperature (T) algorithm and temperature-light (TL) algorithm were calculated as proposed by Guenther et al. (1993).
For pool emissions, the emission rate is parameterised using the following equation:
where, E is the emission rate at temperature T (K). By performing a linear fit to the log of measured emissions and T-TS, we can get β (K–1), the slope () that describes the sensitivity of temperature on emissions, while exponential of y-intercept gives ET (μg g–1 h–1) which is the emission potential at standard temperature (TS = 303.15 K).
For the direct emission of compounds, the emission rate is parameterised using the formula.
where, ETL is the emission potential at standard temperature and light (303.15 K, 1,000 μmol m–2 s–1) obtained from the slope of linear fit between CT*CL and measured emission rate. CT and CL are temperature and light correction factors, respectively. These are formulated as,
where T (K) and TS are chamber temperature and standard temperature, respectively. L is the photosynthetically active radiation (PAR, μmol m–2 s–1), R is the universal gas constant and TM,CT1,CT2,CL1and α are empirical constants as given in Guenther et al. (1993).
Results
Molecular composition of biogenic volatile organic compound emissions
The BVOC emissions from birch were measured by Vocus for a period of 9 days. While several studies have deployed Vocus to study ambient VOCs, this would be the first one to quantify direct BVOC emissions from trees using Vocus. Figure 1 shows the mass defect plot of the identified BVOCs from birch emissions, colored according to their elemental composition. In addition to isoprene (C5H10) and monoterpenes (C10H14, C10H16), direct emissions of sesquiterpenes (C15H22, C15H24) and diterpenes (C20H32, C20H36, and C20H38) were also clearly observed. Overall, most of the compounds were identified as oxygenated VOCs.
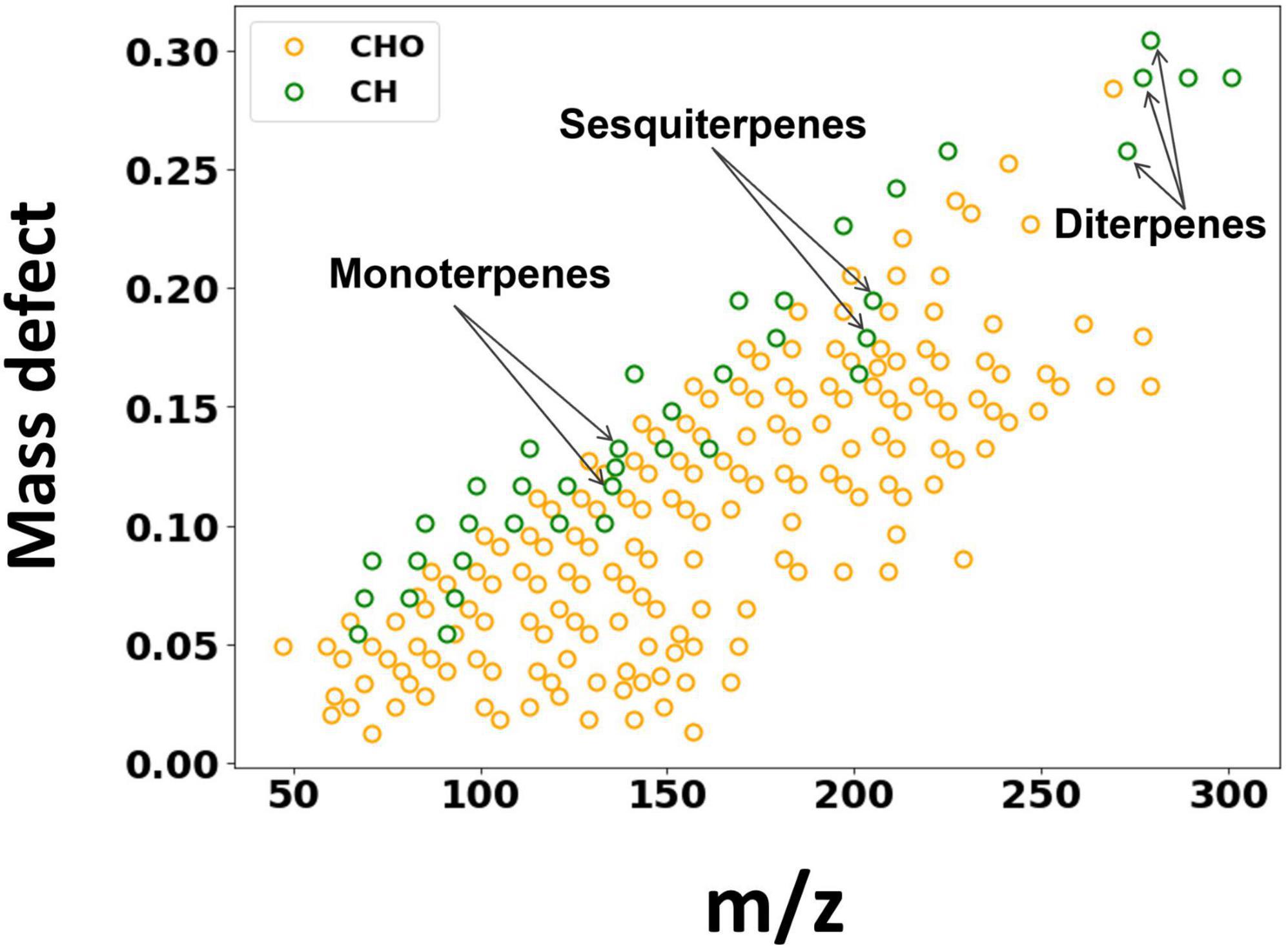
Figure 1. Mass defect plot of the ions identified by the Vocus. The x axis is the mass-to-charge ratio and the y axis shows the mass defect, which is the deviation of the exact mass from the nominal mass. Data points are colored by their elemental composition.
Among all the identified molecular formula, 49 of them were further investigated in this study (Supplementary Table 2). The oxygenated compounds are classified into three classes (OVOC class 1, OVOC class 2, and OVOC class 3) based on the number of oxygen atoms. Class 1 consists of compounds with 1 oxygen atom, class 2 consists of compounds with 2 oxygen atoms and class 3 contains compounds with 3 and 4 oxygen atoms. These compounds were selected according to the following guidelines to avoid the possibility of contamination or fragmentation: (1) they have a clear diurnal pattern that are similar to the more frequently measured BVOCs such as isoprene and monoterpenes, and (2) In PTR-MS, different isomers can fragment into smaller molecules based on the physical and chemical properties. It is difficult to separate real emission from fragmentation, and all compounds included in this list have been demonstrated to be present in plant emissions previously. Moreover, data from 19 August to 21 August were excluded for calculation of emission potentials due to the relatively higher background levels than the other days. The measurements are also attributed to uncertainties from the following sources: (1) Background signal could not be corrected due to contamination of zero air of Vocus. While this may not be an issue for highly emitted compounds, there were a couple of days that clearly affected the compounds emitted at a rate close to the detection limit of the instrument. (2) The drop in relative humidity in the chamber may have influenced the emissions of BVOCs. (3) The calibration standard does not contain all gases and hence this would contribute to the uncertainty as all gases respond differently in the PTR system. (4) While inlet lines and chamber have been tested for the sampling of terpenes (Helin et al., 2020), losses can still exist for oxygenated compounds.
Environmental parameters in the atmosphere and chamber
The branch experienced a certain level of stress inside the chamber. The ambient conditions remained relatively stable with temperature ranging between 6 and 21°C, while chamber temperature varied drastically. The lowest temperature was around 7°C and it rose to 41°C during the measurement period. The temperature inside the chamber was much higher than ambient when PAR was higher than 500 μmol m–2 s–1 during the day. Otherwise, the average temperature in the chamber did not differ from ambient by more than 2°C. High PAR was observed on 16 August although similar PAR was observed for a longer duration on 20 August which could have caused the chamber temperature to be slightly higher on 20 August.
Besides that, the branch also experienced dry conditions relative to the surroundings, for a period of 3 days (Figure 2) as relative humidity in the chamber decreased from 62–84% to 34–84% on 16 August. The relative humidity inside the chamber returned to higher RH on 19 August. The subsequent increase in RH was a consequence of refilling the humidifier. The relative humidity inside the chamber was similar to the ambient conditions differing only by 5% when ignoring the dry period. Generally, the branch experienced considerable stress due to the dry and warm conditions. While the stress due to relative humidity cannot be explained from the emissions, temperature stress has clearly induced higher BVOC emissions which will be discussed later.
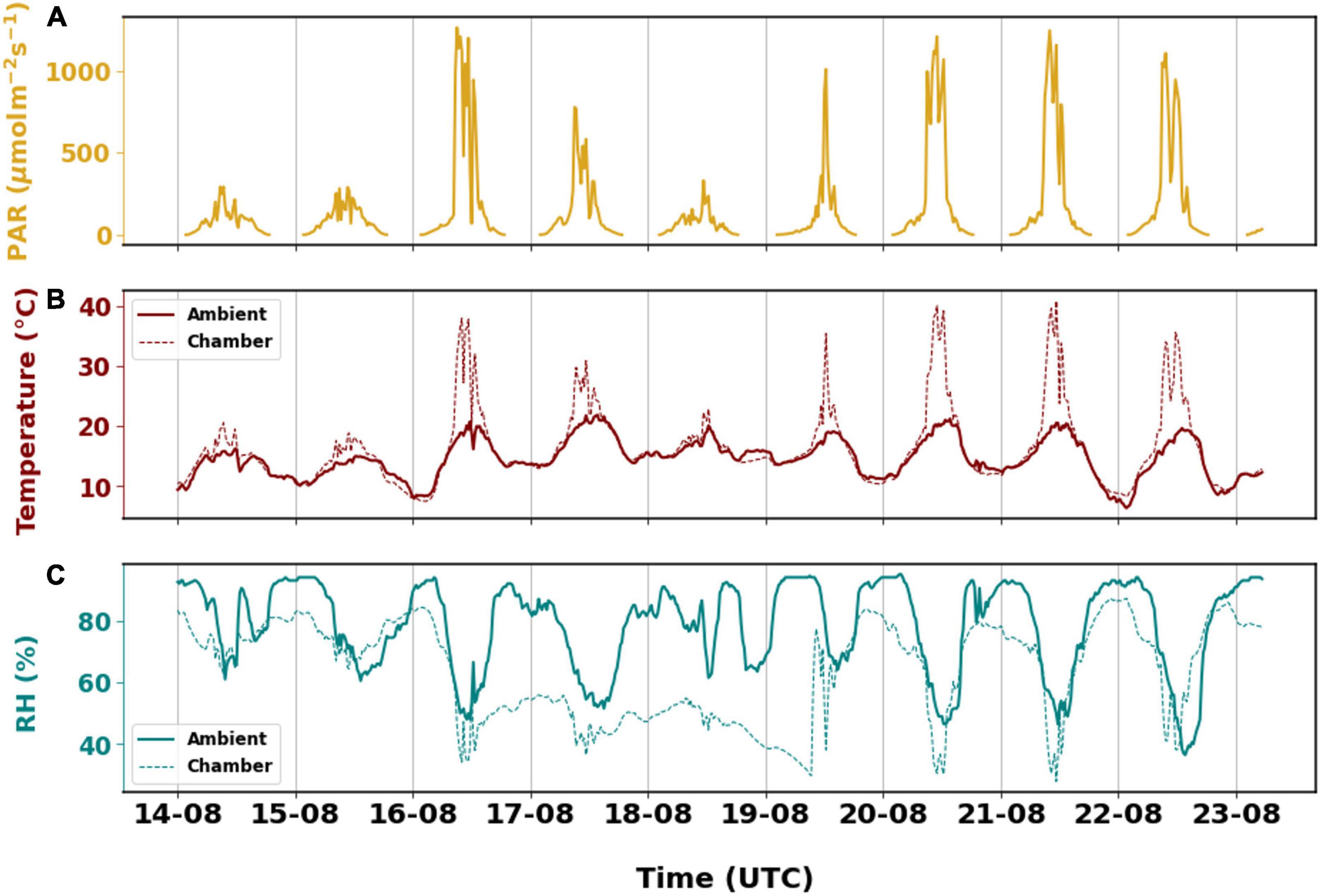
Figure 2. The different environmental parameters that are observed from August 14th, 2019 to August 23rd, 2019. (A) Photosynthetic active radiation recorded on top of the branch enclosure. Observations of (B) temperature and (C) relative humidity inside the chamber and in ambient environment. In panels (B,C), the solid lines are ambient observations downloaded from FMI open weather data. The dashed lines are measurements from the sampling enclosure.
Overview of biogenic volatile organic compound emissions
Figures 3A,C show the average diurnal pattern of BVOC emissions from the measured downy birch. BVOC emissions peaked at 10:00. However, PAR was high an hour before while temperature was seen to be high at both 9:00 and 11:00 (Figure 3B). The branch seemed to have responded an hour later at 10:00 with higher BVOC emissions as a consequence of high PAR and temperature. An increase of 20°C in chamber temperature was observed from 8:00 to 9:00. Consequently a 90% increase in total emission rate also occurred.
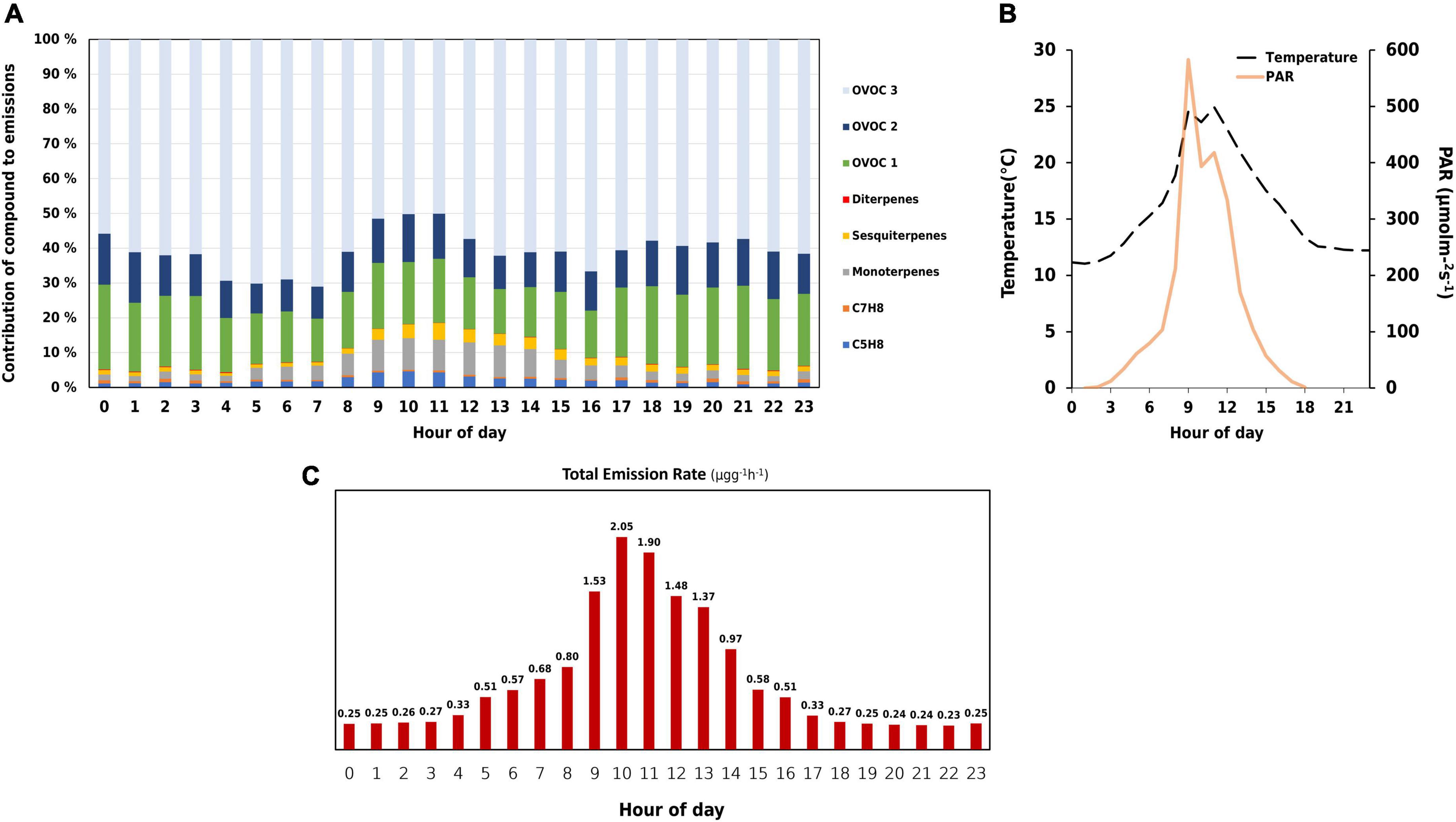
Figure 3. Plots describing hourly variations of (A) emission contribution of different biogenic volatile organic compounds (BVOCs). Monoterpenes include C10H14 and C10H16, sesquiterpenes include C15H22 and C15H24, and diterpenes include C20H32, C20H36, and C20H38. Oxygenated compounds (OVOCs) have been split into three classes based on the oxygen number. OVOC class 1 consists of 1 oxygen, OVOC class 2 consists of compounds with 2, while OVOC class 3 contains compounds with 3 or more. (B) Chamber temperature (°C) and PAR (μmol m– 2 s– 1), and (C) total emission rate (μgg– 1h– 1).
From the daily variations, almost every compound (or) class reached its maximum on 20 August, which could be attributed to the high PAR and considerably high temperature (Table 1). In this study, oxygenated compounds were seen to contribute significantly to the birch emissions. OVOC class 3 emissions increased gradually with a dip on 19 August. OVOC class 1 and 2 peaked on days when the mean temperature was at least 3°C higher than the previous day. However, a burst in their emissions was seen on 19 August which could be a result of the sudden increase in humidity in the chamber around noon. Emissions from OVOC class 3 were highest on 22 August. Due to low temperature and PAR during the first two days of the campaign, emissions were low too.
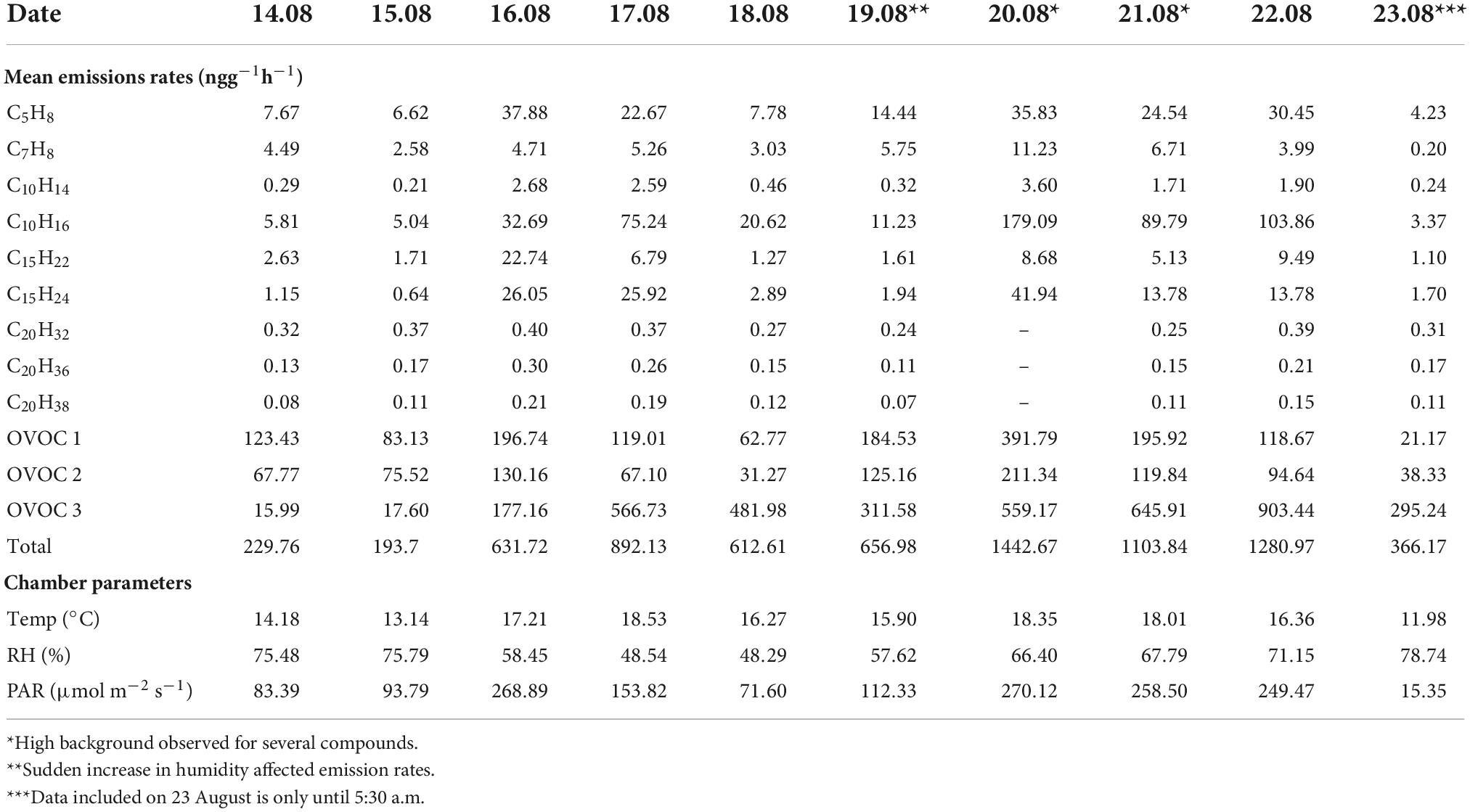
Table 1. Daily mean emission rates (ngg–1h–1) of different compounds and mean of observed chamber parameters. The emission rates of oxygenated compounds (OVOC) classes are sum of the mean of individual compounds in the respective classes.
CxHy compounds
Figure 4 shows the different CxHy compounds that were observed during the measurement period. Monoterpenes (C10H14 and C10H16) had highest emissions among non-oxygenated compounds (∼7% overall). Emissions of C10H16 were greatest on 20 August although similar chamber temperatures were observed on 16 August too. During the measurement period, C15H22 emissions were the highest on 16 August, while highest emissions of C15H24 were observed on 20 August. We observed emissions of isoprene to be emitted similarly (∼0.02 μg g–1h–1) to sesquiterpenes in the late growing period. Emissions of isoprene correlated well with PAR (r = 0.90) in our study.
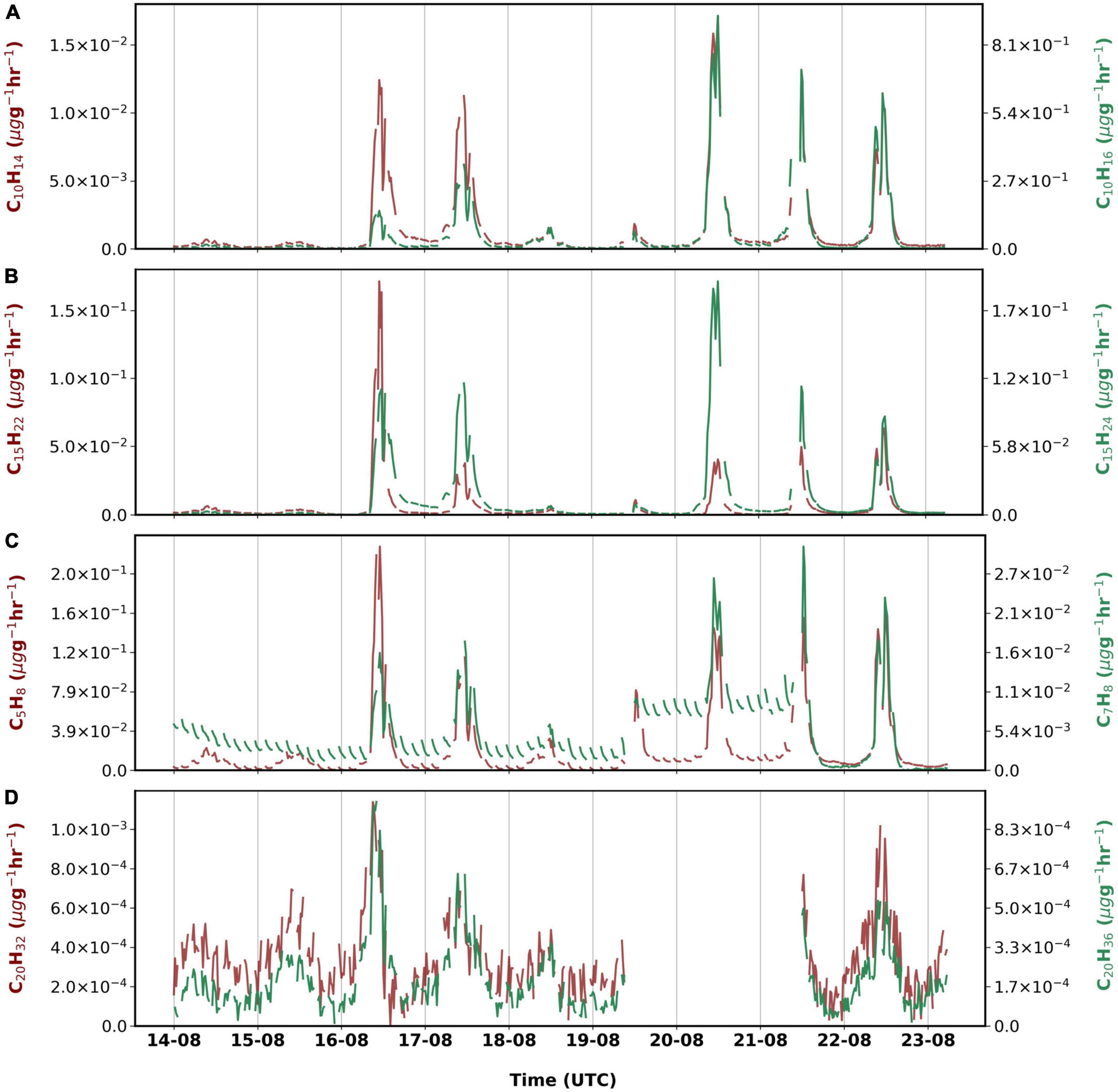
Figure 4. Emissions rates of (A) monoterpenes, (B) sesquiterpenes, (C) isoprene and toluene and (D) diterpenes measured from 14 August 2019 to 23 August 2019.
For the first time, emissions of different diterpenes (C20H32, C20H36, and C20H38) from birch were observed using the Vocus. The sum of their emissions accounts for less than a percent of the total observed, which could be due to the time of the measurements during the late growing season.
Oxygenated compounds classes 1 and 2 emissions
A wide array of oxygenated compounds was observed from birch emissions using the Vocus. In OVOC class 1, 65% was seen from C3H6O, while C4H6O, C6H10O, and C9H18O amounted to 17%. In OVOC class 2, emissions were mostly from C2H4O2 (∼76%) and around 17% from C3H6O2, C7H6O2, and C8H14O2. Several compounds in class 1 and 2 emitted a lot more on 16 and 20 August which was a result of the high temperature observed during the daytime. Emissions of the observed oxygenated compounds have rarely been reported.
The compounds C2H4O2 and C3H6O were emitted in a similar pattern (r = 0.92) and contributed approximately 9 and 11% to the total observed emissions, respectively. C2H4O2 and C3H6O are tentatively identified as acetic acid and methyl acetate. In the overall OVOC class, all other 37 compounds belong to minor components and have a total share of about only 10%. We also observed emissions of C10H18O, C10H160, and C10H20O which could be oxygenated monoterpenes. However, their sum emissions contributed up to only 0.5% to the total observed emissions. All three positively responded to the spikes in temperature with significant emissions observed on 16 August and 21 August. We also observed several oxygenated sesquiterpenes (C15H22O, C15H24O, C15H24O2, C15H22O2, and C15H26O) with C15H22O contributing the highest (0.3%) to the total emissions. While C15H26O had only 0.1% share in the total, the compound was emitted at a higher rate than the other four on 20 August.
C4H6O, C5H6O, and C5H10O could be in plant isoprene oxidation products (methyl acrolein (MACR)/methyl vinyl ketone (MVK) and 3-methyl furan (3-MF) respectively) and 2-methyl-3-buten-2-ol (MBO). All these three masses were found to be emitted in minor quantities, lower than isoprene. In our study we observed significant emissions of C4H6O (0.01 μg g–1h–1), but much lower emissions of C5H6O (0.0017 μg g–1h–1) and C5H10O (0.0019 μg g–1h–1). Relatively higher emissions of all these compounds were observed during high temperature and light events such as on 16 August and 22 August.
Emissions of other BVOCs such as C6H10O, C6H12O, C8H14O2, and C9H18O were an order of magnitude more on 16 August and 22 August in comparison to other days. The branch in our study experienced dry periods (RH < 40%) from August 16 to August 19 and high temperatures (> 30°C) between 9:00 a.m. and 14:00 p.m. on 16, 19 20, and 21 August (Figure 2.). The increased emissions of compounds observed during this period may have been a consequence of stress (Figure 3).
Oxygenated compound class 3 emissions
The compound with molecular formula C8H8O3 was emitted the most during our measurement period, contributing to around 60% in total emissions and 99% of the OVOC 3 group. Diurnal variation of the C8H8O3 emissions followed the temperature, but daily mean emissions showed a delay in response to temperature (Table 1 and Figure 5). For example, daily mean temperature was > 18oC on 20 and 21 August, while on 22 August, when the emissions of C8H8O3 were highest, the mean temperature was only 16°C (Figure 5). Likewise, temperatures rose to around 40°C on August 16, but emissions of C8H8O3 were higher on 17 August. The correlation between temperature and C8H8O3 was better when temperature was shifted forward by 1 day.
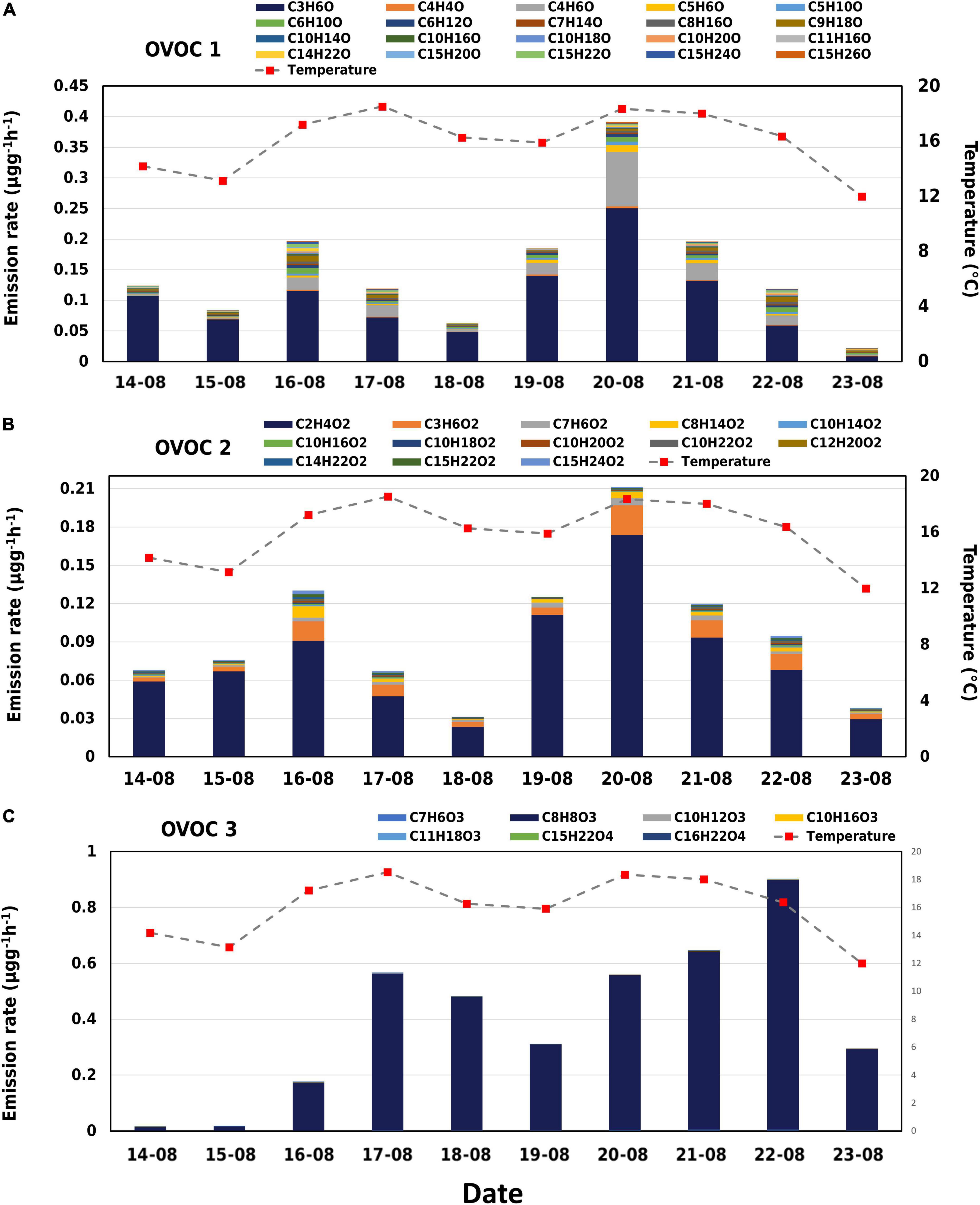
Figure 5. Emissions of the oxygenated compounds split into different classes measured from 14 August 2019 to 23 August 2019. (A) OVOC class 1 consists of compounds with 1 oxygen atom. (B) OVOC class 2 consists of compounds with 2 oxygen atoms. (C) OVOC class 3 consists of compounds with 3 or more oxygen atoms.
Another OVOC 3 compound, C7H6O3 was also observed here, and it correlated closely with C8H8O3 emissions (r = 0.96). Compounds consisting of even 4 oxygen atoms were also observed but their contributions were negligible.
Temperature and light dependence of biogenic volatile organic compound emissions
In this study, we tried to understand how temperature and light influences BVOC emissions using the algorithms given in section “Calculation of emission potentials.” Terpenoid emissions from trees originate as a result of evaporation from storage pool (pool emissions) such as resins and canals found in conifers (Grote and Niinemets, 2008; Ghirardo et al., 2010; Loreto and Schnitzler, 2010). However, they can also be emitted directly (de novo) as a result of photosynthesis in chloroplasts in deciduous trees like birch (Loreto et al., 1996; Ghirardo et al., 2010; Taipale et al., 2011). In reality it is not possible to give a clear distinction with regard to BVOC emissions between storage pools and de novo production (Grote and Niinemets, 2008). Other BVOCs besides terpenoids are emitted directly as a result of stress or in connection with plant metabolic activity (Šimpraga et al., 2019).
A graph illustrating the fit for C10H16 using the two algorithms has been shown as an example in the Supplementary Figure 1. The same was repeated for all compounds to obtain the different parameters using both algorithms. The T-algorithm explained the emission rates of monoterpenes and sesquiterpenes better than the TL-algorithm and yielded a higher R2. As shown in Table 2, the R2 of T-algorithm was slightly higher than that of TL-algorithm for C15H22 and C15H24. For C10H16, R2 was much higher using the T-algorithm than the TL- algorithm. For isoprene, the temperature and light algorithm better explained its emissions (R2 = 0.95; Table 2). The correlations of diterpenes using both algorithms were generally less (R2 < 0.7). Although we see a slightly better correlation using the TL-algorithm, conclusive results cannot be obtained from our field experiments. The emissions of C8H8O3 gave one of the lowest correlations with both algorithms (R2 < 0.4). Its emissions from downy birch tree may be induced by stress. In our study, C10H18O was better correlated using the TL-algorithm (R2 = 0.96) than just using temperature. The TL-algorithm clearly fit the emissions of several oxygenated compounds much better than the T-algorithm, producing R2 as high as 0.96.
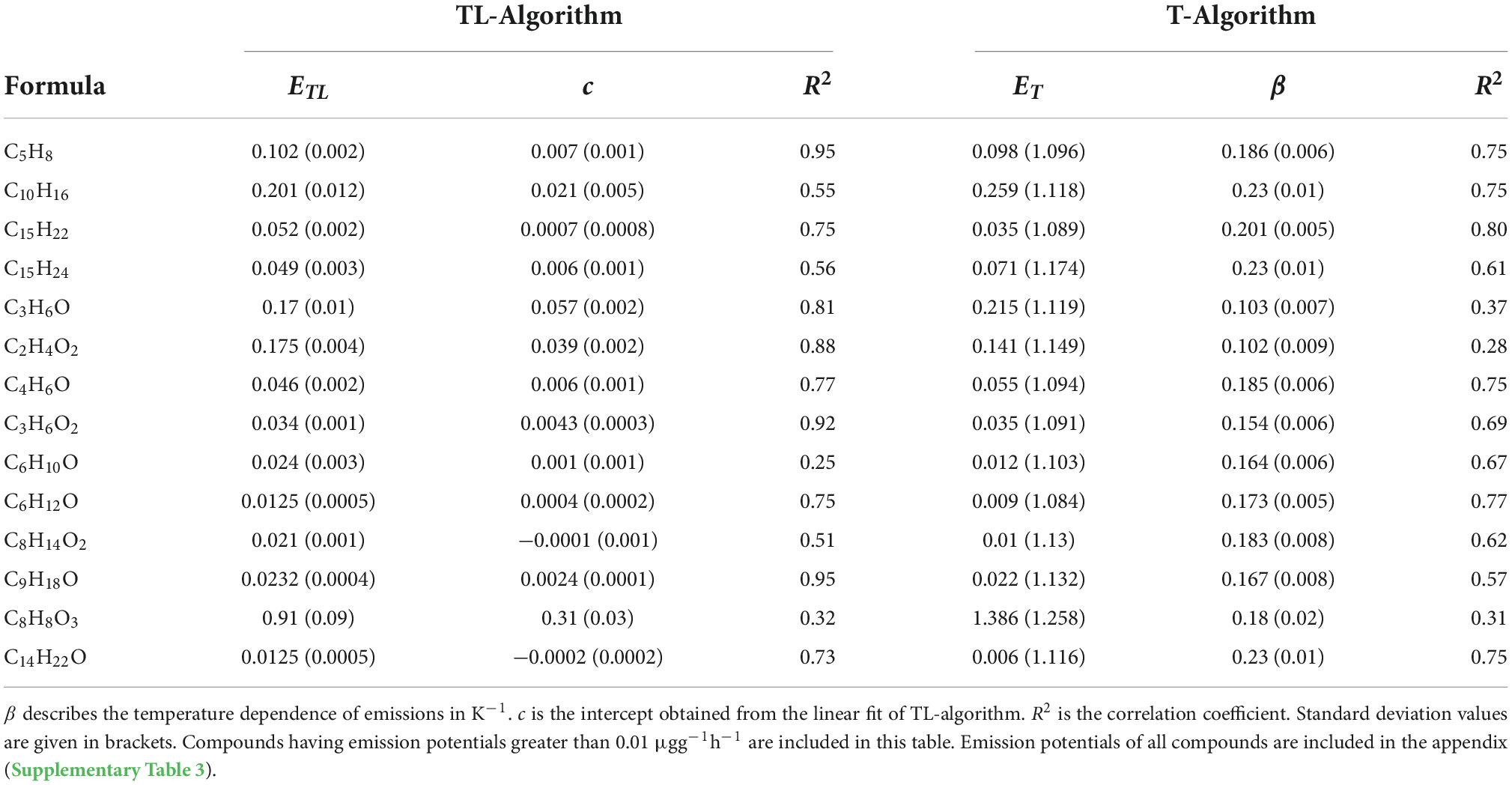
Table 2. Emission potentials of different compounds calculated using temperature (ET) and temperature-light algorithm (ETL) in μgg–1h–1.
The β values that describe the sensitivity of emissions on temperature calculated here are higher than usual (Table 2). The highest β value calculated in this study was 0.23 K–1 for C10H16, C15H24, and C14H22O respectively. Temperature dependency for both sesquiterpenes and monoterpenes were similar in our study. The diterpene C20H32 showed the least temperature dependency, with a β value of only 0.04 K–1, unlike the other two diterpenes.
Emission potentials
All emission potentials (Supplementary Table 3) were calculated at the temperature of 30°C for comparison with other studies. This is one of the first studies to calculate emission potentials for a vast range of oxygenated compounds and therefore is difficult to compare with other studies. Emission potentials of the different compounds observed vary over three orders of magnitude.
We report for the first-time emission potentials of diterpenes from birch. The values varied from 0.0002 to 0.0003 μg g–1 h–1 (TL-algorithm) and 0.001 to 0.0004 μg g–1h–1 (T-algorithm) depending on the compound (Supplementary Table 3). The emission potentials of all diterpenes calculated for birch in our study were found to be three orders lower than C10H16, which highlights low emissions of diterpenes even in high temperatures during late summer. The highest emissions potentials were observed for C8H8O3 (ET: 1.386 μg g–1h–1; ETL: 0.91 μg g–1h–1) followed by C10H16 (ET: 0.259 μg g–1h–1; ETL: 0.201 μg g–1h–1) using both T and TL algorithms.
Discussion
The emission spectra
The downy birch emissions in our study were dominated by oxygenated compounds, while monoterpenes (C10H14 and C10H16) dominated the emissions of non-oxygenated compounds. Higher emissions of monoterpenes in comparison to sesquiterpernes during the late growing season are in agreement with that reported in Hellén et al. (2021) and Zhang et al. (1999). Both studies also reported that sesquiterpenes and oxygenated sesquiterpenes dominated during the main growing season while monoterpenes were emitted in the largest proportions during late summer. In the study by Hellén et al. (2021), sesquiterpenes were emitted 2–15 times more than monoterpenes during the early and main growing seasons.
C7H8 which is speculated to be toluene, was measured to be around 1% for the whole period. A few other studies have reported toluene emissions from plants (Heiden et al., 1999; Holzinger et al., 2000; White et al., 2009; Misztal et al., 2015). Toluene is a compound that is widely present in the atmosphere and assumed to be emitted primarily from various anthropogenic sources (White et al., 2009). While a broad array of benzenoid compounds are produced in plants from different metabolic pathways, studies reporting natural emissions of toluene are less and hence their emissions into the atmosphere could be underestimated (Misztal et al., 2015).
Matsunaga et al. (2011) and Yáñez-Serrano et al. (2018) have reported emissions of diterpenes from shrubs and conifers, respectively. However, kaur-16-ene emissions observed in their studies were either comparable or exceeded monoterpene emissions. Helin et al. (2020) found six different diterpenes in the emission from Norway spruce needles while heated to > 40°C. In our study the emissions of diterpenes were almost three orders of magnitude lower than those of monoterpenes.
Compared with previous birch studies that have taken place in natural growing conditions, we have reported oxygenated molecules, with up to 4 oxygen atoms to be emitted from the downy birch leaves. Only one another study which was conducted in the headspace of birch buds has reported similar oxygenated compounds (Isidorov et al., 2019). The study identified 156 compounds, most of which had a minor contribution (< 1% of the chromatograph), besides a few belonging to oxygenated monoterpenes and oxygenated sesquiterpenes. In our study, 37 oxygenated molecules have a share of about only 10% in the OVOC group, while C8H8O3 contributed to 65% of the OVOC emissions. Zhang et al. (1999) also observed oxygenated compound emissions from downy birch although many of them were not identified. Klika et al. (2004) reported several oxygenated sesquiterpenes with up to three oxygen atoms present in the essential oil of mountain birch buds. While studies have given insights on the wide array of oxygenated compounds present in oils or emitted from buds, only Hellén et al. (2021) has observed leaf emissions of different oxygenated compounds from downy birch.
Signs of stress
The branch experienced heat and dry conditions. Signs of other infections such as insect herbivory were not visible at the time of measurement. C8H8O3 that was emitted in plenty could be methyl salicylate (MeSa), which is a BVOC emitted upon both biotic and abiotic stresses (Llusià et al., 2005; Filella et al., 2007; Vuorinen et al., 2007; Blande et al., 2010; Liu et al., 2018). Llusià et al. (2005) and Filella et al. (2007) found increasing MeSa emissions with increase in temperature. MeSa emissions from Norway spruce observed by Filella et al. (2007) were lower than those of monoterpenes. When silver birch was infested with aphids in a study by Blande et al. (2010), MeSa contributed up to 50% to the observed emissions, while Vuorinen et al. (2007) reported greater induction of terpenoids than MeSa when silver birch was infested with moths. There is evidence that MeSa may have an impact on monoterpene emissions. Liu et al. (2018) found that when silver birch was exposed to MeSa, monoterpene emissions decreased. Only exception was β-ocimene, which had positive correlation with MeSa concentrations. However, it is not possible to check such correlations with just Vocus data. Based on the studies by Hellén et al. (2021) high emissions of β-ocimene were measured from the downy birch branch in 2019 at the same time as C8H8O3 emissions were detected in this study. Diurnal variation of the C8H8O3 emissions followed the temperature (Figure 6), but daily mean emissions showed a delay in response to temperature (Table 1 and Figure 5). For example, daily mean temperature was > 18oC on 20 and 21 August, while on 22 August, when the emissions of C8H8O3 were highest, the mean temperature was only 16°C (Figure 5). Likewise, temperatures rose to around 40°C on August 16, but emissions of C8H8O3 were higher on 17 August. The correlation between temperature and C8H8O3 was better when temperature was shifted forward by 1 day. The emissions of C8H8O3 also gave one of the lowest correlations with both algorithms (R2 < 0.4). Therefore, implying their emissions from downy birch tree may be induced by stress. Bourtsoukidis et al. (2014) also reported that MeSa and linalool emissions from Norway spruce were poorly described by the T-algorithm (R2 < 0.2). In addition to temperature and other stress factors, late growing season with starting senescence of the leaves may have an impact on these emissions. The emission of C7H6O3 that correlated well with MeSa, could be salicylic acid, which is an important signaling molecule that induces heat tolerance in plants (Filella et al., 2007).
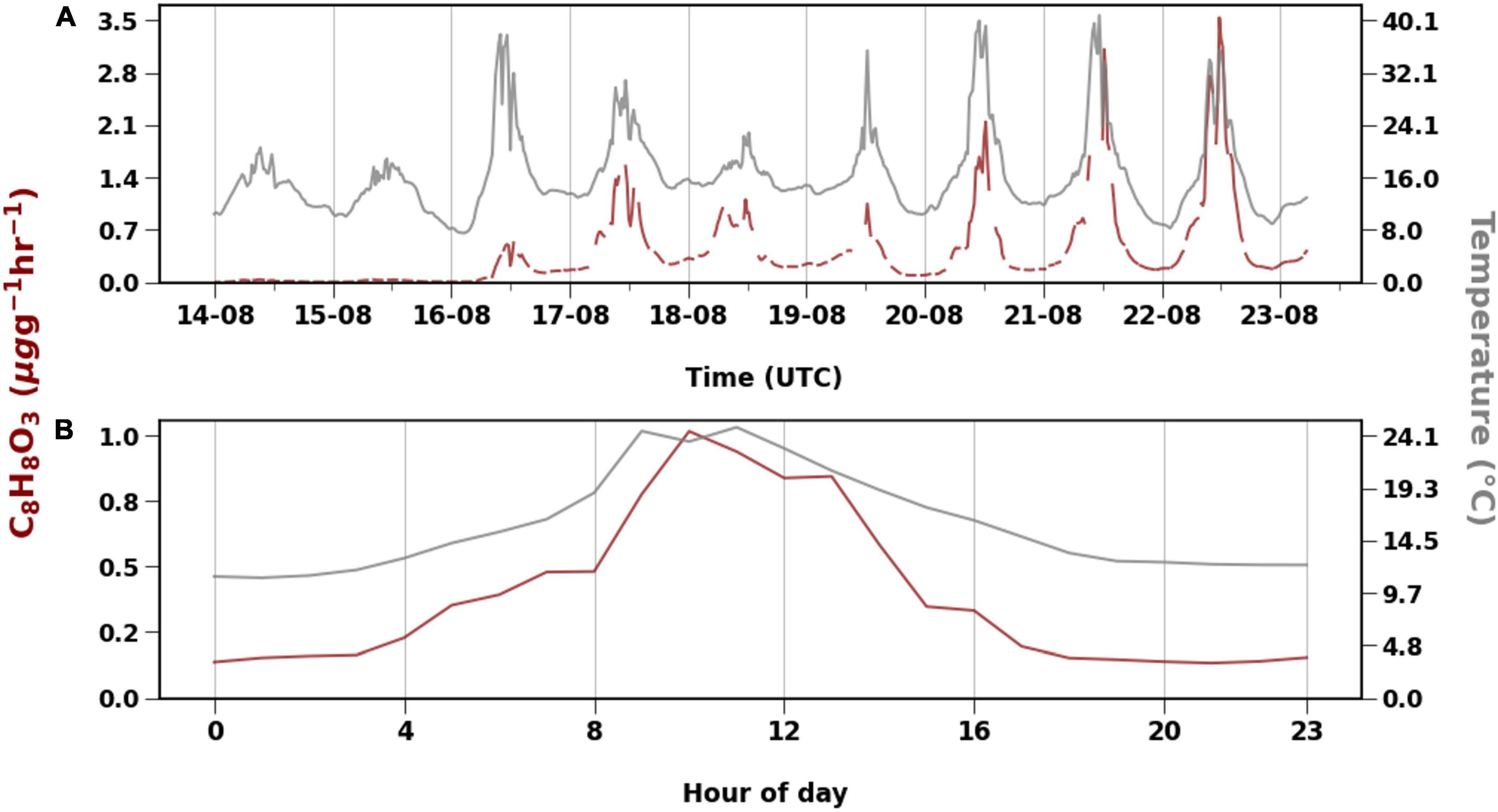
Figure 6. Emission of C8H8O3 with temperature (°C) for the whole measurement period (A) and diurnal variation (B).
Toluene can also be induced from plants in response to stress (Heiden et al., 1999; Guenther, 2013). Heiden et al. (1999) measured toluene emissions from non-stressed pine trees and it reached a maximum of approximately 13 ng g–1h–1, which is less than what was observed here (max ∼ 30 ng g–1h–1). Misztal et al. (2015) observed spikes in toluene emissions when the plant was subjected to rapid temperature bursts for short periods which normally occur with the type of setup used in our study.
Emissions of other BVOCs such as C6H10O, C6H12O, C8H14O2, and C9H18O increased by an order of magnitude on 16 August and 22 August in comparison to other days. Hellén et al. (2021) observed C4–C10 aldehydes and green leaf volatiles (GLVs) such as cis-3-hexenyl acetate and cis-3-hexen-1-ol from downy birch trees. In the same study, aldehydes were not measured in 2019 but their mean emission rate in 2017 was measured to be 0.023 μg g–1h–1. The emission rate of cis-3-hexenol measured in that study was 0.006 μg g–1h–1 during the late growing season in 2019. In our study, we observed lower emissions of C6H12O (0.0019 μg g–1h–1). GLVs are compounds that are normally released in large amounts from damaged membranes of the leaves (Holopainen, 2004). Jardine et al. (2015) observed bursts of C6 and C5 GLV emissions at high temperature (42°C) with a continuous increase even after stabilization of leaf temperature. It was hypothesized that high temperatures and low humidity levels may cause membrane damage and consequently increase GLV emissions (Kleist et al., 2012; Jardine et al., 2015). The branch in our study experienced dry periods (RH<40%) from August 16 to August 19 and high temperatures (> 30°C) between 9:00 a.m. and 14:00 p.m. on 16, 19 20 and 21 August (Figure 2.). The increased emissions of compounds were observed during this period which may have been a consequence of such stress (Figure 3).
C4H6O, C5H6O, and C5H10O could be in plant isoprene oxidation products [methyl acrolein (MACR)/methyl vinyl ketone (MVK) and 3-methyl furan (3-MF), respectively] and 2-methyl-3-buten-2-ol (MBO). Reports of direct emissions of isoprene oxidation products are less, although MBO has been reported in plenty. Jardine et al. (2012) confirmed in-plant isoprene oxidation and high emissions of MVK and MACR relative to isoprene during temperature and light stress. Jardine et al. (2013) conducted stress experiments on isoprene and non-isoprene emitting species (mango tree and soursop, respectively) to observe emissions of isoprene, MACR, MVK, 3-MF, and MBO. In the same study, while there was clear enhancement of isoprene oxidation products in response to high temperature and light from mango trees, this was not seen from non-isoprene emitting species. In our study we observed significant emissions of C4H6O (0.01 μg g–1h–1), but much lower emissions of C5H6O (0.0017 μg g–1h–1) and C5H10O (0.0019 μg g–1h–1) were detected. Meanwhile, C5H10O could be attributed to pentanal, a common aldehyde that can be constitutive but also induced (Hakola et al., 2017; Kanagendran et al., 2018). In this study, we found relatively higher emissions of all these compounds during high temperature and light events such as on 16 August and 22 August.
While previous emission studies of birch leaves have shown substantial amounts of monoterpenes released especially closer to autumn, emission of many of the detected oxygenated compounds has not been observed from downy birches before. In addition to differences in the emissions and stress situations, the reason for this may also be instrumental limitations. Basic gas chromatographic set ups traditionally used for the BVOC emission studies are not sensitive to MeSa or other compounds with 3 oxygen atoms and therefore even relatively high emissions may have remained undetected. To confirm significance of these emissions more measurements during different seasons and stress situations are needed.
Conclusion and outlook
A Vocus PTR-TOF-MS was deployed in a boreal forest to measure BVOC emissions of downy birch during late August 2019. With increased detection efficiency and higher mass resolution, the Vocus proved much more than capable to be used as a tool to observe direct biogenic emissions. In addition to common BVOCs, it was able to detect for example larger terpenoids (e.g., diterpenes) and huge set of oxygenated compounds which have not been traditionally measured.
Due to the late growing season, the emission rates from downy birch leaves were relatively low albeit diverse. Hydrocarbons with up to 20 carbon atoms, as well as oxygenated compounds with up to 4 oxygen atoms from birch emissions are reported in this study. Monoterpenes contributed the largest among non-oxygenated compounds, around 7% of total BVOC emissions. For oxygenated compounds, C8H8O3 contributed most and accounted for ∼57% of total birch emissions. It was tentatively identified as methyl salicylate. Methyl salicylate is known to be a stress induced compound. During the late growing season, the high temperatures could have stressed the branch in this study, causing MeSa emissions. Among oxygenated compounds, the total contribution besides C8H8O3, C3H6O, and C2H4O2 amounted only to 10%. Although in minor quantities we observed for the first-time emissions of rarely reported diterpenes from birch (C20H32: 0.0003 μg g–1h–1; C20H36: 0.0002 μg g–1h–1 C20H38: 0.0001 μg g–1h–1). Due to their low volatility and instrument limitations, only a few studies have observed diterpene emissions from vegetation.
We observed for this brief period that emissions of all non-oxygenated compounds besides isoprene were slightly better explained using the temperature algorithm. This study is one of the few to report emission potentials for a wide range of oxygenated compounds. For oxygenated compounds, emissions of many compounds better correlated using the TL-algorithm. However, for compounds like C8H8O3, both algorithms failed to explain its emissions suggesting that there are other factors besides temperature and light that may strongly control its emissions from downy birch. The emissions potentials calculated here are quite low in comparison to previous studies, which may be attributed to the late growing season.
This study, with the use of a new PTR-MS, clearly shows the diverse range of molecules that the downy birch can emit into the atmosphere. With downy birch being a prominent species in the boreal zone, these compounds can be vital in a forest environment like Hyytiälä where BVOCs have a major impact in events like new particle formation. The emissions of several compounds observed could become prominent in times of stress or during other seasons. The differences in behavior of these compounds and their importance in the environment deems it necessary to conduct more BVOC emissions studies especially in response to stress. While several studies based on stress induced compounds have been conducted, there is still a lack of understanding in their emission mechanism and identity and amount of emitted BVOCs. In a time where such stress episodes are predicted to occur more frequently it is crucial to investigate them as they can also have prominent effects on the atmospheric chemistry and physics.
Further studies to understand the emission profiles of these BVOCs across different seasons and in response to stress along with their influence in SOA formation and concentration of oxidants such as OH, O3, and NO3 are required to comprehend the importance of these compounds on regional and global scale.
Data availability statement
The data that support the findings of this study are available in http://doi.org/10.23728/fmi-b2share.d52b45c85f1e49729e033d15aa5fb8c8. The raw data measured by the Vocus PTR-TOF-MS and other sensors will be made available upon request.
Author contributions
ST, HL, and FB designed the experiment. ST and HL conducted the experiment and analyzed the data. AP and HH helped to design the study. AP acquired the environmental data. ST interpreted the results and wrote the manuscript. All authors discussed the results and contributed to the manuscript.
Funding
This research was funded by European Union (EU) H2020 program via the fundings European Research Council (ERC; project CHAPAs, 850614).
Acknowledgments
We thank the European Union (EU) H2020 program via the fundings European Research Council (ERC; project CHAPAs, 850614). We also thank SMEAR II station and their staff for providing infrastructure and support during the campaign. HL acknowledges support from Shenzhen Outstanding Scientific and Technological Innovation Talent Training Project (RCBS20210609103731062) and Guangdong Province Basic and Applied Basic Research Fund Project (2021A1515110887).
Conflict of interest
The authors declare that the research was conducted in the absence of any commercial or financial relationships that could be construed as a potential conflict of interest.
Publisher’s note
All claims expressed in this article are solely those of the authors and do not necessarily represent those of their affiliated organizations, or those of the publisher, the editors and the reviewers. Any product that may be evaluated in this article, or claim that may be made by its manufacturer, is not guaranteed or endorsed by the publisher.
Supplementary material
The Supplementary Material for this article can be found online at: https://www.frontiersin.org/articles/10.3389/ffgc.2022.1030348/full#supplementary-material
References
Aartsma, Y., Bianchi, F. J. J. A., Werf, W. V. D., Poelman, E. H., and Dicke, M. (2017). Herbivore-induced plant volatiles and tritrophic interactions across spatial scales. New Phytol. 216, 1054–1063. doi: 10.1111/NPH.14475
Bianchi, F., Kurtén, T., Riva, M., Mohr, C., Rissanen, M. P., Roldin, P., et al. (2019). Highly oxygenated organic molecules (HOM) from gas-phase autoxidation involving peroxy radicals: A key contributor to atmospheric aerosol. Chem. Rev. 119, 3472–3509. doi: 10.1021/ACS.CHEMREV.8B00395
Blake, R. S., Monks, P. S., and Ellis, A. M. (2009). Proton-transfer reaction mass spectrometry. Chem. Rev. 109, 861–896. doi: 10.1021/CR800364Q
Blande, J. D., Korjus, M., and Holopainen, J. K. (2010). Foliar methyl salicylate emissions indicate prolonged aphid infestation on silver birch and black alder. Tree Physiol. 30, 404–416. doi: 10.1093/treephys/tpp124
Blande, J. D., Tiiva, P., Oksanen, E., and Holopainen, J. K. (2007). Emission of herbivore-induced volatile terpenoids from two hybrid aspen (Populus tremula×tremuloides) clones under ambient and elevated ozone concentrations in the field. Glob. Change Biol. 13, 2538–2550. doi: 10.1111/J.1365-2486.2007.01453.X
Bourtsoukidis, E., Bonn, B., and Noe, S. (2014). On-line field measurements of BVOC emissions from Norway spruce (Picea abies) at the hemiboreal SMEAR-Estonia site under autumn conditions. Boreal Environ. Res. 19, 153–167.
Demirci, F., Demirci, B., Baser, K. H. C., and Guven, K. (2000). The composition and antifungal bioassay of the essential oils of different Betula species growing in Turkey. Chem. Nat. Compd. 36, 159–165. doi: 10.1007/BF02236421
Faiola, C., and Taipale, D. (2020). Impact of insect herbivory on plant stress volatile emissions from trees: A synthesis of quantitative measurements and recommendations for future research. Atmos. Environ. X 5:100060. doi: 10.1016/j.aeaoa.2019.100060
Filella, I., Wilkinson, M. J., Llusià, J., Hewitt, C. N., and Peñ uelas, J. (2007). Volatile organic compounds emissions in Norway spruce (Picea abies) in response to temperature changes. Physiol. Plant. 130, 58–66. doi: 10.1111/j.1399-3054.2007.00881.x
Ghirardo, A., Koch, K., Taipale, R., Zimmer, I., Schnitzler, J. P., and Rinne, J. (2010). Determination of de novo and pool emissions of terpenes from four common boreal/alpine trees by 13CO2 labelling and PTR-MS analysis. Plant Cell Environ. 33, 781–792. doi: 10.1111/j.1365-3040.2009.02104.x
Grote, R., and Niinemets, Ü (2008). Modeling volatile isoprenoid emissions - A story with split ends. Plant Biol. 10, 8–28. doi: 10.1055/s-2007-964975
Guenther, A. (2013). Biological and chemical diversity of biogenic volatile organic emissions into the atmosphere. ISRN Atmos. Sci. 2013, 1–27. doi: 10.1155/2013/786290
Guenther, A. B., Zimmerman, P. R., Harley, P. C., Monson, R. K., and Fall, R. (1993). Isoprene and monoterpene emission rate variability: Model evaluations and sensitivity analyses. J. Geophys. Res. 98, 12609–12617. doi: 10.1029/93jd00527
Guidolotti, G., Pallozzi, E., Gavrichkova, O., Scartazza, A., Mattioni, M., Loreto, F., et al. (2019). Emission of constitutive isoprene, induced monoterpenes, and other volatiles under high temperatures in Eucalyptus camaldulensis: A 13C labelling study. Plant Cell and Environ. 42, 1929–1938. doi: 10.1111/PCE.13521
Haapanala, S., Ekberg, A., Hakola, H., Tarvainen, V., Rinne, J., Hellé, H., et al. (2009). Mountain birch–Potentially large source of sesquiterpenes into high latitude atmosphere. Biogeosciences 6, 2709–2718. doi: 10.5194/bg-6-2709-2009
Hakola, H., Laurila, T., Lindfors, V., Hellén, H., Gaman, A., and Rinne, J. (2001). Variation of the VOC emission rates of birch species during the growing season. Boreal Environ. Res. 6, 237–249.
Hakola, H., Rinne, J., and Laurila, T. (1998). The hydrocarbon emission rates of tea-leafed willow (Salix phylicifolia), silver birch (Betula pendula) and European aspen (Populus tremula). Atmos. Environ. 32, 1825–1833. doi: 10.1016/S1352-2310(97)00482-2
Hakola, H., Tarvainen, V., Praplan, A. P., Jaars, K., Hemmilä, M., Kulmala, M., et al. (2017). Terpenoid and carbonyl emissions from Norway spruce in Finland during the growing season. Atmos. Chem. Phys. 17, 3357–3370. doi: 10.5194/acp-17-3357-2017
Hari, P., and Kulmala, M. (2005). Station for measuring ecosystem-atmosphere relations (SMEAR II). Boreal Environ. Res. 10, 315–322.
Hartikainen, K., Nerg, A.-M., Kivimäenpää, M., Kontunen-soppela, S., Mäenpää, M., Oksanen, E., et al. (2009). Emissions of volatile organic compounds and leaf structural characteristics of European aspen (Populus tremula) grown under elevated ozone and temperature. Tree Physiol. 29, 1163–1173. doi: 10.1093/TREEPHYS/TPP033
Heiden, A. C., Kobel, K., Komenda, M., Koppmann, R., Shao, M., and Wildt, J. (1999). Toluene emissions from plants. Geophys. Res. Lett. 26, 1283–1286. doi: 10.1029/1999GL900220
Helin, A., Hakola, H., and Hellén, H. (2020). Optimisation of a thermal desorption-gas chromatography-mass spectrometry method for the analysis of monoterpenes, sesquiterpenes and diterpenes. Atmos. Meas. Tech. 13, 3543–3560. doi: 10.5194/AMT-13-3543-2020
Hellén, H., Praplan, A. P., Tykkä, T., Helin, A., Schallhart, S., Schiestl-Aalto, P. P., et al. (2021). Sesquiterpenes and oxygenated sesquiterpenes dominate the VOC (C5-C20) emissions of downy birches. Atmos. Chem. Phys. 21, 8045–8066. doi: 10.5194/acp-21-8045-2021
Holopainen, J. K. (2004). Multiple functions of inducible plant volatiles. Trends Plant Sci. 9, 529–533. doi: 10.1016/j.tplants.2004.09.006
Holzinger, R., Sandoval-Soto, L., Rottenberger, S., Crutzen, P. J., and Kesselmeier, J. (2000). Emissions of volatile organic compounds from Quercus ilex L. measured by Proton Transfer Reaction Mass Spectrometry under different environmental conditions. J. Geophys. Res. 105, 20573–20579. doi: 10.1029/2000JD900296
Isidorov, V. A., Stocki, M., and Vetchinikova, L. (2019). Inheritance of specific secondary volatile metabolites in buds of white birch Betula pendula and Betula pubescens hybrids. Trees Struct. Funct. 33, 1329–1344. doi: 10.1007/s00468-019-01861-2
Jardine, K. J., Chambers, J. Q., Holm, J., Jardine, A. B., Fontes, C. G., Zorzanelli, R. F., et al. (2015). Green leaf volatile emissions during high temperature and drought stress in a central Amazon rainforest. Plants 4, 678–690. doi: 10.3390/plants4030678
Jardine, K. J., Meyers, K., Abrell, L., Alves, E. G., Yanez Serrano, A. M., Kesselmeier, J., et al. (2013). Emissions of putative isoprene oxidation products from mango branches under abiotic stress. J. Exp. Bot. 64, 3697–3709. doi: 10.1093/jxb/ert202
Jardine, K. J., Monson, R. K., Abrell, L., Saleska, S. R., Arneth, A., Jardine, A., et al. (2012). Within-plant isoprene oxidation confirmed by direct emissions of oxidation products methyl vinyl ketone and methacrolein. Glob. Change Biol. 18, 973–984. doi: 10.1111/j.1365-2486.2011.02610.x
Jiang, Y., Ye, J., Veromann, L.-L., and Niinemets, Ü (2016). Scaling of photosynthesis and constitutive and induced volatile emissions with severity of leaf infection by rust fungus (Melampsora larici-populina) in Populus balsamifera var. suaveolens. Tree Physiol. 36, 856–872. doi: 10.1093/TREEPHYS/TPW035
Kanagendran, A., Pazouki, L., Li, S., Liu, B., Kännaste, A., and Niinemets, Ü (2018). Ozone-triggered surface uptake and stress volatile emissions in Nicotiana tabacum ‘Wisconsin’. J. Exp. Bot. 69, 681–697. doi: 10.1093/jxb/erx431
Kirkby, J., Duplissy, J., Sengupta, K., Frege, C., Gordon, H., Williamson, C., et al. (2016). Ion-induced nucleation of pure biogenic particles. Nature 533, 521–526. doi: 10.1038/NATURE17953
Kleist, E., Mentel, T. F., Andres, S., Bohne, A., Folkers, A., Kiendler-Scharr, A., et al. (2012). Irreversible impacts of heat on the emissions of monoterpenes, sesquiterpenes, phenolic BVOC and green leaf volatiles from several tree species. Biogeosciences 9, 5111–5123. doi: 10.5194/bg-9-5111-2012
Klika, K. D., Demirci, B., Salminen, J. P., Ovcharenko, V. V., Vuorela, S., Can Başer, K. H., et al. (2004). New, sesquiterpenoid-type bicyclic compounds from the buds of Betula pubescens–Ring-contracted products of β-caryophyllene? Eur. J. Org. Chem. 2004, 2627–2635. doi: 10.1002/ejoc.200300808
Krechmer, J., Lopez-Hilfiker, F., Koss, A., Hutterli, M., Stoermer, C., Deming, B., et al. (2018). Evaluation of a new reagent-ion source and focusing ion- molecule reactor for use in proton-transfer-reaction mass spectrometry. Anal. Chem. 90, 12011–12018. doi: 10.1021/acs.analchem.8b02641
Li, H., Riva, M., Rantala, P., Heikkinen, L., Daellenbach, K., Krechmer, J. E., et al. (2020a). Terpenes and their oxidation products in the French Landes forest: Insights from Vocus PTR-TOF measurements. Atmos. Chem. Phys. 20, 1941–1959. doi: 10.5194/acp-20-1941-2020
Li, H., Väliranta, M., Mäki, M., Kohl, L., Britta Sannel, A. K., Pumpanen, J., et al. (2020b). Overlooked organic vapor emissions from thawing Arctic permafrost. Environ. Res. Lett. 15, 104097–104097. doi: 10.1088/1748-9326/abb62d
Li, N., He, Q., Greenberg, J., Guenther, A., Li, J., Cao, J., et al. (2018). Impacts of biogenic and anthropogenic emissions on summertime ozone formation in the Guanzhong Basin, China. Atmos. Chem. Phys. 18, 7489–7507. doi: 10.5194/ACP-18-7489-2018
Liu, B., Kaurilind, E., Jiang, Y., and Niinemets, Ü (2018). Methyl salicylate differently affects benzenoid and terpenoid volatile emissions in Betula pendula. Tree Physiol. 38, 1513–1525. doi: 10.1093/TREEPHYS/TPY050
Llusià, J., Peñuelas, J., and Munné-Bosch, S. (2005). Sustained accumulation of methyl salicylate alters antioxidant protection and reduces tolerance of holm oak to heat stress. Physiol. Plant. 124, 353–361. doi: 10.1111/j.1399-3054.2005.00519.x
Loreto, F., and Schnitzler, J. P. (2010). Abiotic stresses and induced BVOCs. Trends Plant Sci. 15, 154–166. doi: 10.1016/j.tplants.2009.12.006
Loreto, F., Ciccioli, P., Cecinato, A., Brancaleoni, E., Frattoni, M., Fabozzi, C., et al. (1996). Evidence of the photosynthetic origin of monoterpenes emitted by Quercus ilex L. leaves by 13C labeling. Plant Physiol. 110, 1317–1322. doi: 10.1104/pp.110.4.1317
Matsunaga, S. N., Chatani, S., Nakatsuka, S., Kusumoto, D., Kubota, K., Utsumi, Y., et al. (2011). Volatile diterpene emission from dominant conifers in Japan. Biogeosci. Discuss. 8, 6681–6700. doi: 10.5194/bgd-8-6681-2011
Misztal, P. K., Hewitt, C. N., Wildt, J., Blande, J. D., Eller, A. S. D., Fares, S., et al. (2015). Atmospheric benzenoid emissions from plants rival those from fossil fuels. Sci. Rep. 5, 52425–52425. doi: 10.1038/srep12064
Nölscher, A. C., Bourtsoukidis, E., Bonn, B., Kesselmeier, J., Lelieveld, J., and Williams, J. (2013). Seasonal measurements of total OH reactivity emission rates from Norway spruce in 2011. Biogeosciences 10, 4241–4257. doi: 10.5194/BG-10-4241-2013
Ormeño, E., and Fernandez, C. (2012). Effect of soil nutrient on production and diversity of volatile terpenoids from Plants. Curr. Bioact. Compd. 8, 71–71. doi: 10.2174/157340712799828188
Praplan, A. P., Tykkä, T., Schallhart, S., Tarvainen, V., Bäck, J., and Hellén, H. (2020). OH reactivity from the emissions of different tree species: Investigating the missing reactivity in a boreal forest. Biogeosciences 17, 4681–4705. doi: 10.5194/BG-17-4681-2020
Riva, M., Rantala, P., Krechmer, E. J., Peräkylä, O., Zhang, Y., Heikkinen, L., et al. (2019). Evaluating the performance of five different chemical ionization techniques for detecting gaseous oxygenated organic species. Atmos. Meas. Tech. 12, 2403–2421. doi: 10.5194/amt-12-2403-2019
Roger Atkinson, A., and Arey, J. (2003). Atmospheric degradation of volatile organic compounds. Chem. Rev. 103, 4605–4638. doi: 10.1021/CR0206420
Simon, V., Luchetta, L., and Torres, L. (2001). Estimating the emission of volatile organic compounds (VOC) from the French forest ecosystem. Atmos. Environ. 35(Suppl. 1), S115–S126. doi: 10.1016/S1352-2310(00)00565-3
Šimpraga, M., Ghimire, R. P., Van Der Straeten, D., Blande, J. D., Kasurinen, A., Sorvari, J., et al. (2019). Unravelling the functions of biogenic volatiles in boreal and temperate forest ecosystems. Eur. J. For. Res. 138, 763–787. doi: 10.1007/s10342-019-01213-2
Sinha, V., Williams, J., Lelieveld, J., Ruuskanen, T. M., Kajos, M. K., Patokoski, J., et al. (2010). OH reactivity measurements within a boreal forest: Evidence for unknown reactive emissions. Environ. Sci. Technol. 44, 6614–6620. doi: 10.1021/ES101780B
Taipale, R., Kajos, M. K., Patokoski, J., Rantala, P., Ruuskanen, T. M., and Rinne, J. (2011). Role of de novo biosynthesis in ecosystem scale monoterpene emissions from a boreal Scots pine forest. Biogeosciences 8, 2247–2255. doi: 10.5194/bg-8-2247-2011
Tarvainen, V., Hakola, H., Hellén, H., Bäck, J., Hari, P., and Kulmala, M. (2005). Temperature and light dependence of the VOC emissions of Scots pine. Atmos. Chem. Phys. 5, 989–998. doi: 10.5194/acp-5-989-2005
Toome, M., Randjärv, P., Copolovici, L., Niinemets, Ü, Heinsoo, K., Luik, A., et al. (2010). Leaf rust induced volatile organic compounds signalling in willow during the infection. Planta 232, 235–243. doi: 10.1007/S00425-010-1169-Y
Vuorinen, T., Nerg, A. M., Vapaavuori, E., and Holopainen, J. K. (2005). Emission of volatile organic compounds from two silver birch (Betula pendula Roth) clones grown under ambient and elevated CO2 and different O3 concentrations. Atmos. Environ. 39, 1185–1197. doi: 10.1016/j.atmosenv.2004.09.077
Vuorinen, T., Nerg, A.-M., Syrjälä, L., Peltonen, P., and Holopainen, J. K. (2007). Epirrita autumnata induced VOC emission of silver birch differ from emission induced by leaf fungal pathogen. Arthropod. Plant Interact. 1, 159–165. doi: 10.1007/s11829-007-9013-4
White, M. L., Russo, R. S., Zhou, Y., Ambrose, J. L., Haase, K., Frinak, E. K., et al. (2009). Are biogenic emissions a significant source of summertime atmospheric toluene in the rural Northeastern United States? Atmos. Chem. Phys. 9, 81–92. doi: 10.5194/acp-9-81-2009
Yáñez-Serrano, A. M., Fasbender, L., Kreuzwieser, J., Dubbert, D., Haberstroh, S., Lobo-Do-Vale, R., et al. (2018). Volatile diterpene emission by two Mediterranean cistaceae shrubs. Sci. Rep. 8, 1–13. doi: 10.1038/s41598-018-25056-w
Yli-Juuti, T., Mielonen, T., Heikkinen, L., Arola, A., Ehn, M., Isokääntä, S., et al. (2021). Significance of the organic aerosol driven climate feedback in the boreal area. Nat. Commun. 12, 1–9. doi: 10.1038/s41467-021-25850-7
Yu, H., Holopainen, J. K., Kivimäenpää, M., Virtanen, A., and Blande, J. D. (2021). Potential of climate change and herbivory to affect the release and atmospheric reactions of BVOCs from boreal and subarctic forests. Molecules 26, 2283–2283. doi: 10.3390/MOLECULES26082283
Zhang, Q. H. E., Birgersson, G., Zhu, J., Löfstedt, C., Löfqvist, J., and Schlyter, F. (1999). Leaf volatiles from nonhost deciduous trees: Variation by tree species, season and temperature, and electrophysiological activity in Ips typographus. J. Chem. Ecol. 25, 1923–1943. doi: 10.1023/A:1020994119019
Keywords: BVOCs, Vocus PTR-TOF-MS, downy birch, diterpene, oxygenated VOC
Citation: Thomas SJ, Li H, Praplan AP, Hellén H and Bianchi F (2022) Complexity of downy birch emissions revealed by Vocus proton transfer reaction time-of-flight mass spectrometer. Front. For. Glob. Change 5:1030348. doi: 10.3389/ffgc.2022.1030348
Received: 28 August 2022; Accepted: 02 November 2022;
Published: 24 November 2022.
Edited by:
Jose D. Fuentes, The Pennsylvania State University, United StatesReviewed by:
Nora Zannoni, Max Planck Institute for Chemistry, GermanyPallavi Saxena, University of Delhi, India
Copyright © 2022 Thomas, Li, Praplan, Hellén and Bianchi. This is an open-access article distributed under the terms of the Creative Commons Attribution License (CC BY). The use, distribution or reproduction in other forums is permitted, provided the original author(s) and the copyright owner(s) are credited and that the original publication in this journal is cited, in accordance with accepted academic practice. No use, distribution or reproduction is permitted which does not comply with these terms.
*Correspondence: Steven Job Thomas, c3RldmVuLnRob21hc0BoZWxzaW5raS5maQ==; Haiyan Li, bGloYWl5YW4yMDIxQGhpdC5lZHUuY24=