- 1Lyon Arboretum and School of Life Sciences, University of Hawai’i at Mānoa, Honolulu, HI, United States
- 2Department of Environmental Systems Science, ETH Zürich, Zurich, Switzerland
- 3Faculty of Forest Sciences and Forest Ecology, University of Göttingen, Göttingen, Germany
- 4Department of Environmental Studies, University of California, Santa Cruz, Santa Cruz, CA, United States
- 5Department of Ecology and Evolutionary Biology, University of California, Santa Cruz, Santa Cruz, CA, United States
- 6Las Cruces Biological Station, Organization for Tropical Studies, San Vito, Costa Rica
- 7Charles Darwin Foundation, Puerto Ayora, Ecuador
Choosing appropriate forest restoration interventions is challenging. Natural regeneration can rapidly facilitate forest recovery in many situations. However, barriers such as dispersal limitation and competition with non-native species can require assisted restoration approaches to facilitate plant community recovery. We used a study that has directly compared the outcomes of tropical wet forest restoration interventions across 11 replicate sites in southern Costa Rica. Within this framework, we examined the functional recovery trajectories of recruiting tree sapling communities across a gradient of restoration interventions including low (natural regeneration), intermediate (applied nucleation), and high (plantation) initial resource-investment, which we compared to remnant reference forest. We collated leaf and stem functional traits for tree species that comprised the bulk of recruiting saplings, then determined how community-weighted trait means and functional diversity metrics changed over a decade across treatments. Results show that assisted restoration approaches (applied nucleation, plantation) sped the development of more functionally diverse tree communities, more than tripling the functional richness (FRic) of recruiting communities when compared to natural regeneration. However, functional dispersion (i.e., the trait range of dominant species) was equivalent across interventions, and between 28 and 44% lower than remnant forest, indicating that increases in FRic under assisted restoration were driven by species recruiting in low abundances (<10 individuals across treatments). Recruits in assisted restoration treatments also had 10–15% tougher, less-palatable leaves, and leaves were even tougher in reference forest, which could be driven by increasing herbivory pressure along the gradient of interventions. Results show that tracking simple metrics such as species richness can mask a more mechanistic understanding of ecosystem recovery that is elucidated by taking a functional trait-driven approach toward evaluating outcomes. For example, our work identified a paucity of dense-wooded species recruiting across restoration interventions, wood density was 11–13% lower in restoration treatments than reference forests, underscoring such species as prime targets for enrichment planting. Overall, findings suggest that assisted restoration can catalyze the functional recovery of naturally recruiting tree communities in landscapes that are slow to recover naturally and highlight the importance of evaluating how different components of functional diversity shift over time to fully understand restoration outcomes.
Introduction
One of the core goals of the United Nations Decade on Ecosystem Restoration1 is to ensure that restoration science is better integrated with practice. Whereas it is imperative that the wealth of existing information on ecosystem recovery is better integrated into restoration practice, we also need more experiments that directly evaluate the efficacy of different restoration approaches and ecosystem recovery indicators at multiple sites (Cooke et al., 2019). Evaluating how and when to apply restoration approaches is especially important in the world’s forest-classified biomes as forest cover continues to decline globally, especially in tropical regions (FAO and UNEP, 2020). Additionally, a great deal of uncertainty remains regarding which restoration intervention to apply as the outcomes of natural and assisted forest restoration approaches (sensu Chazdon et al., 2021) can be highly variable and context dependent (Norden et al., 2015; Holl et al., 2017).
Natural regeneration (i.e., natural recovery after the main barriers to regeneration are removed) has tremendous capacity to restore the world’s tropical forests (Crouzeilles et al., 2017; Cook-Patton et al., 2020; Poorter et al., 2021), and targeted tree planting (i.e., assisted recovery) can catalyze tropical forest recovery in cases where succession is slow or arrested (Lamb, 2005; Holl and Aide, 2011; Philipson et al., 2020). However, there is a paucity of paired comparisons between natural and assisted recovery techniques in the same system, and most studies to date have been biased because more resource-intensive management interventions are often needed, and therefore implemented, at the most degraded sites (Reid et al., 2018). Assisted restoration methods span a gradient of management intensity, and techniques such as applied nucleation (i.e., planting trees in clusters leaving space for natural recovery) fall on the intermediate level of the resource-investment spectrum (Corbin and Holl, 2012). To objectively evaluate how restoration interventions influence the recovery rates of forest ecosystem processes, natural and assisted approaches must not only be evaluated side by side, but also across gradients of local- and landscape-scale degradation (Shoo and Catterall, 2013; Cook-Patton et al., 2021).
A variety of factors operating on local to regional scales influence tropical forest revegetation rates (Arroyo-Rodríguez et al., 2017). These include the intensity of prior land use (e.g., Meli et al., 2017), the dominance of invasive species (Ostertag et al., 2009; Holl, 2012), and plant dispersal and establishment processes at a given site (Holl, 1999; de la Peña-Domene et al., 2017; Werden et al., 2020a). In cases where natural recovery is slow, rapidly increasing canopy cover by planting trees can shade out invasive forage grasses, attract dispersers, and ameliorate microclimatic conditions for seedling establishment (Lamb, 2005; Holl, 2012). Moreover, a recent global meta-analysis demonstrates that native tree plantations can speed the recovery of plant species richness when compared to natural recovery (Wang et al., 2021), and intermediate levels of assisted recovery, such as applied nucleation, can recover tree species richness and composition to levels observed when using more resource-intensive plantation approaches (Holl et al., 2017, 2020). However, patterns of plant diversity and structure recovery only tell part of the story. By applying a functional trait-based framework we can more fully tease apart how gradients of restoration interventions influence the recovery of plant communities (Ostertag et al., 2015; Brancalion and Holl, 2016; Werden et al., 2018).
Plant functional traits can elucidate mechanisms underlying restoration outcomes (Ostertag et al., 2015; Chua and Potts, 2018; Li et al., 2018; Werden et al., 2020b). A plant functional trait is any morphological, physiological or phenological feature measurable at the individual level (Violle et al., 2007). These traits indicate how plants use and acquire resources, often reflect a trade-off between growth and mortality (Lohbeck et al., 2013), and encompass a spectrum of life history strategies from acquisitive to conservative species (Reich, 2014). Generally, species with an acquisitive strategy have high assimilation rates and fast growth, large, thin leaves with a high specific leaf area (SLA), low leaf dry matter content (LDMC) and leaf toughness as well as a low wood density. By contrast, conservative species concentrate their resource investments mainly into construction and longevity, enabling them to better tolerate biotic and abiotic stresses, which is reflected in low SLA and high wood density, LDMC, and leaf toughness (Wright et al., 2004; Chave et al., 2009; Lohbeck et al., 2013; Reich, 2014; Díaz et al., 2016).
In tropical forests, acquisitive tree species typically dominate in environments with high water and light availability, while conservative species are better adapted to drier environments and can tolerate shade (Wright et al., 2010; Lohbeck et al., 2015). Consequently, species with acquisitive strategies and fast growth generally dominate in the early stages of tropical wet forest succession, and as light availability decreases in the understory, more conservative functional strategies become dominant (Lohbeck et al., 2013). Environmental filters select for species with functional traits that promote high performance under local conditions (Diaz et al., 2007). Thus, the dominant trait value of a community, or the community-weighted mean (CWM), can explain the general response of plant communities to gradients of environmental conditions (e.g., those influenced by restoration interventions) and allow trajectories of community assembly and function to be examined in detail (Becknell and Powers, 2014; Lohbeck et al., 2015; Buzzard et al., 2016; Muscarella et al., 2017). Additionally, distance-based functional diversity (FD) metrics can be used to aggregate the variation of plant functional traits in a community and further tease apart overall community functional composition (Villéger et al., 2008). In contrast to traditional species diversity metrics, FD can provide a more precise understanding of how diversity links to ecosystem processes, resilience, and services (Dğíaz and Cabido, 2001; Diaz et al., 2007). For example, restoration interventions that promote the recovery of community-level FD typically improve both ecosystem resilience and services (Montoya et al., 2012).
Few studies have compared how natural vs. assisted restoration approaches influence the recovery of plant functional diversity over time, and how these dynamics can diverge across a gradient of restoration interventions at multiple sites is not well resolved. We used a study replicated at 11 sites to compare patterns of plant functional recovery among different wet forest restoration interventions over more than a decade across a network of replicate sites in southern Costa Rica. Within this framework, we evaluated this core question: How do the functional recovery trajectories of recruiting tree sapling communities vary across restoration interventions with low (natural regeneration), intermediate (applied nucleation), and high (plantation) initial resource-investment? We expected that assisted restoration treatments (applied nucleation, plantation) would promote the development of more functionally diverse plant communities that converge more rapidly toward the composition of remnant reference forests than the natural regeneration treatment (i.e., natural recovery). This prediction was based on observations that assisted interventions at these sites promoted the recruitment of more diverse plant communities (Holl et al., 2017, 2020). Moreover, we expected that CWMs in all treatments would shift toward more conservative values (e.g., communities with denser wood, lower SLA, and higher leaf toughness) over time, and that such shifts would be more rapid in the assisted restoration treatments, as microclimatic conditions in more developed tropical wet forests favor species with more conservative resource-use strategies (Lohbeck et al., 2013). Finally, we expected that plant communities in the applied nucleation treatment would incorporate a broader range of resource-use trait values than the plantation treatment (i.e., trait values in the applied nucleation treatment would fall along a wider gradient in the acquisitive to conservative continuum) because applied nucleation is inherently a mixture of both natural and assisted recovery approaches (Zahawi et al., 2013).
Materials and methods
Study site
From 2004 to 2006, a large-scale research project was established by Zahawi et al. (2013) to study the restoration and regeneration of the area around the Las Cruces Biological Station (8°47’ 7″ N, 82°57’ 32″ W) in Coto Brus County, Costa Rica. The forests in this region are at the boundary between Tropical Premontane Wet and Rain Forest zones (Holdridge et al., 1971) with a mean annual rainfall of 3,500–4,000 mm, a dry season from December to March, and a mean annual temperature of ∼21°C. The experimental sites are located at 1,100–1,430 m elevation. Sites are located mostly on strong to steep slopes (15–30%), and have similar edaphic characteristics with soils of volcanic origin that have similar bulk densities, pH of ∼5.5, and low phosphorus concentrations (Holl et al., 2011). The landscape of the study region is typical of most in Central America and is characterized by a mosaic of agricultural fields and pasture interspersed with small remnant forest patches, with highly fragmented forest cover of <30% (Zahawi et al., 2015). Before the restoration plots were established, all sites were burned at least once and were used for a mixture of mostly cattle grazing and coffee farming for at least 18 years (Holl et al., 2011; see Supplementary Table 1 for site characteristics and initial conditions and Supplementary Figure 1 for a map of the sites).
Experimental design and sapling surveys
The experiment was set up as a randomized complete block design, and we present data from 11 replicate sites distributed over an area of 100 km2 to capture the influences of landscape-level processes on restoration outcomes. At each site, three 0.25 ha (50 × 50 m) plots were established at the start of the experiment (2004-2006). Each plot received one of the following restoration treatments: (i) planted throughout with mixed-species plantations (plantation, 313 trees per plot), (ii) planted with six tree islands of three sizes (applied nucleation, 86 trees per plot), and (iii) no restoration planting intervention applied (natural regeneration). In the applied nucleation and plantation plots, two native [Terminalia amazonia (J.F. Gmel.) Exell (Combretaceae) and Vochysia guatemalensis Donn. Sm. (Vochysiaceae)] and two naturalized tree species [Erythrina poeppigiana (Walp.) Skeels and Inga edulis Mart; both Fabaceae], were planted at a uniform planting distance (2.8 m; Holl et al., 2011). Terminalia amazonia is the one planted species that has been observed in the reference forests. By the time of this study the islands had converged, creating continuous canopy cover over the applied nucleation plot at most sites, making it difficult to tease apart the effect of the island sizes on recruitment. Therefore, we focused our analysis on the treatment-level effect of the applied nucleation treatment.
We surveyed tree saplings (≥1 m tall and ≥1 cm and <5 cm diameter-at-breast height; DBH) in all restoration plots annually in June and July of each year from 2007 to 2019. We also surveyed tree seedlings (≥20 cm tall and < 1 cm DBH; see Kulikowski et al., 2022b). We present findings for saplings as leaf traits were measured on saplings and saplings have the highest probability of becoming adults, therefore contributing more to overall ecosystem function. However, we note that the patterns we observed were consistent for both seedling and saplings. At five sites, surveys in adjacent remnant forests (reference) that have been subjected to minimal anthropogenic disturbances in the past were initiated in 2014. We used 2010 as the first year in our analysis as the number of recruits was low the first 3 years since restoration. All tree saplings were measured in forty 2 × 4 m quadrats in each plot (320 m2 per plot) placed along belt transects. In the natural regeneration, plantation, and reference forest treatments, four belt transects 10 m long were randomly located in each of the four quadrants of the plot. In the applied nucleation treatment, the sampling quadrats were distributed along six belt transects installed from within the interior of the planted area to outside the planted area (320 m2 per plot; Supplementary Figure 2). We standardized for different sampling intensities (i.e., total area sampled in individual belt transect) in the applied nucleation and other treatments when computing species abundances per hectare. Each tree sapling was permanently tagged, identified to species, and the DBH of its largest stem recorded.
Functional traits of the sapling community
To robustly calculate community-weighted trait means, we identified 55 tree species that represent an average of at least 70% of all recruiting sapling individuals observed from 2010 to 2019 across all four treatments (Supplementary Table 2). These species represent an average of 86.5% (±2.1 SE), 87.2% (±1.5), 88.5% (±1.1), and 73.4% (±1.8) of all sapling recruits observed in the natural regeneration, applied nucleation, plantation, and reference forest plots, respectively. We reached slightly below the 80% recommend threshold for species coverage (Pakeman and Quested, 2007) in the five reference forests, which differ strongly in their species composition. Because the reference forests differed from the restoration treatments for almost all metrics this limitation did not likely affect the interpretation of our results. We included all species that recruited from seeds dispersed from outside the plots, and the few recruits from species planted in the applied nucleation and plantation plots (21 Erythrina poeppigiana, 13 Inga edulis, 5 Terminalia amazonia). Including or removing the planted species from analyses did not influence the results.
The majority of leaf trait data were collected from saplings of the 55 focal tree species from the understory of forests in the Las Cruces Biological Station, which has a similar climate to the experimental restoration sites. We ensured that there was sufficient distance between sampled individuals of the same species (>30 m), to allow for intraspecific variation in trait characteristics due to differing abiotic conditions. We followed standard methods (Pérez-Harguindeguy et al., 2013) to measure leaf lamina area (leaf area; cm2), thickness (mm), toughness (g-force to punch), petiole length (mm); as well as fresh and oven dry weight (g; dried for ∼72 h at 60°C) on four leaves of three individuals per species. We measured leaf traits on fully expanded mature leaves with no damage. Specific leaf area (SLA; mm2 g–1) and leaf dry matter content (LDMC; mg g–1) were calculated from these measurements. Tree cores were collected on adult trees, as we were unable to destructively harvest sapling wood from the forest reserve, from at least three individuals (up to five) per species with a DBH of 10–30 cm to calculate stem specific gravity (wood density; g cm–3). We calculated wood density using the volume (measured with the water displacement method) and the oven dry weight (dried for ∼72 h at 100°C) obtained from each core. For 16 rare species present only in the reference forests, leaf thickness and wood density data were obtained from publicly available TRY data (Kattge et al., 2020). For these 16 species, genus-level averages from TRY were used in ten cases for leaf thickness, and in seven cases for wood density.
Community-weighted trait means and functional diversity indices
For all analyses we used the treatment plot as the sampling unit (n = 11 for restoration treatments; n = 5 for reference forests). For each treatment plot at each site, we calculated CWMs of sapling traits for each year (2010–2019). Based on the mass ratio hypothesis (Grime, 1998), the CWM of functional traits represents the average or dominant trait value of a community, weighted by species abundances. CWMs allow for the detection of trends and shifts in overall plant trait strategies in response to different restoration treatments, abiotic gradients (e.g., wet to dry ecosystems) and successional stages (Lohbeck et al., 2013; Becknell and Powers, 2014; Buzzard et al., 2016; Muscarella et al., 2017). CWMs were calculated as follows:
Where, S is the total number of species, wi is the relative abundance of the ith species, and xi is the respective trait value. Results were consistent when weighting CWMs by basal area so only abundance weighted results are reported.
We also calculated four distance-based functional diversity (FD) indices (Laliberté and Legendre, 2010; package FD) of the recruiting sapling community. First, we scaled and centered all trait values (-1 to 1) to attain comparable results. Then we calculated the following FD indices including the seven traits annually for each treatment plot from 2010 to 2019, weighted by species abundance per hectare when necessary: Functional richness (FRic) is the amount of functional space filled by the community (Villéger et al., 2008) and can also be interpreted as the amount of biological niche space occupied by a community (Mason et al., 2005). Functional evenness (FEve) describes how evenly trait values are distributed within community trait space (Mason et al., 2005). Functional divergence (FDiv) describes the degree to which species trait values differ from the community trait mean (Villéger et al., 2008). In contrast to FRic, functional dispersion (FDis) describes the dominant range of traits within community trait space; thus rare species with extreme trait values do not influence results (Laliberté and Legendre, 2010). All FD indices range from 0 (lowest) to 1 (highest). Treatment-level summaries of functional diversity metrics, species richness, and CWM traits can be found in Supplementary Table 3.
Statistical analysis
We used rarefaction to assess our sampling coverage by calculating Shannon diversity (Hill order q = 1) and plotting sample size-based species accumulation curves and confidence intervals (α = 0.05) for tree saplings observed in 2019 in each treatment (iNEXT package). We then visualized how trait CWMs, mean species richness, and FD metrics of the recruiting sapling community changed over time by plotting a time series for each metric separated by treatment. For the final survey year (2019), more than a decade (11–13 years) after the experiment was initiated, we built linear mixed models to determine how each treatment and reference forest (treatmenti in Equation 2) differed in terms of each trait CWM, mean species richness, and FD values (yij in Equation 2). We compared between communities present in treatments in 2019 as we were most interested in the end point of the recovery trajectory and differences between treatments were relatively stable at that point. Site (n = 11) was included as a blocking factor (random effect; b1 in Equation 2):
In this model, i indexes observations, j indexes species; b1 are the normally distributed random intercepts for sites; and ∈ are the normally distributed subject residuals for trait CWMs, Poisson distributed residuals for species richness, and beta distributed residuals for FD indices bounded between 0,1 (lme4 package for Gaussian and Poisson regression; glmmTMB for beta regression). We used a zero-inflated beta regression when modeling FDis as there were three natural regeneration plots with only one species of recruit observed, resulting in a FDis of zero.
When necessary, we log transformed certain response variables (leaf area, leaf thickness, petiole length, SLA) to meet model residual normality assumptions. To quantify the percentage of variance explained by the treatments and random effects we calculated marginal (R2m; treatment effects) and conditional (R2c; treatment and site effects) pseudo-R2 values for each linear model (Nakagawa and Schielzeth, 2013; MuMIn package). We tested for treatment effects with one-way ANOVAs (type II) for trait CWMs, and likelihood-ratio χ2 tests for functional and species diversity (car package). Estimated marginal means (EMMs) of the seven CWMs, four FD metrics, and sapling species richness were calculated at the treatment level, and Tukey’s HSD post-hoc tests with adjusted p-values (α = 0.05) were used to detect differences between treatment-level means (emmeans package). For the CWM wood density model, one natural regeneration plot was determined to be an outlier using Cook’s Distance, even after transformation, and was removed to obtain normally distributed residuals. This outlier occurred because only one species with a relatively high mean high wood density (Miconia schlimiil; mean = 0.55) was observed at this site, and most natural regeneration sites typically had a mix of species with less dense wood. Removing this outlier did not affect the outcomes of pairwise comparisons between treatments for CWM wood density. Finally, we visualized the trajectories of species composition and the CWM trait values for each treatment from 2010 to 2019 (reference forest 2014–2019) using non-metric multidimensional scaling (NMDS; ecotraj package; De Cáceres et al., 2019) performed on a Bray-Curtis dissimilarity matrix for species abundance, and a Euclidean distance matrix for CWM values (vegan package) sensu Catano et al. (2022). We used R version 4.0.4 for all analyses (R Development Core Team, 2021).
Results
Species composition and community-weighted mean functional traits
For the 55 focal species from 28 families, we censused a total of 3,603 saplings (natural regeneration: 530, applied nucleation: 1,771, plantation: 946, reference: 356; total number of saplings not standardized by sampling area) from 2010 to 2019 (reference censused from 2014 to 2019). Sample-based rarefaction curves demonstrated that the sampling intensity was sufficient to characterize species diversity within the treatments, and that Shannon diversity by 2019 was highest in the reference, intermediate in both assisted restoration treatments (applied nucleation and plantation), and lowest in the natural regeneration treatment (Supplementary Figure 3). CWMs of traits of saplings recruiting in the restoration treatments generally became less variable over time, and the values of certain traits (LDMC, wood density, leaf toughness) converged toward those of the reference over time in the assisted restoration treatments (Figure 1). CWMs of leaf area, leaf toughness, and wood density differed among treatments, but LDMC, leaf thickness, petiole length, and SLA did not (Table 1). Leaf area of the sapling communities did not differ between the restoration treatments (F = 4.84, p = 0.009). However, leaves of the communities in the reference had double the area of those in the natural regeneration treatment on average (t = -3.24, p = 0.016). Leaf toughness was lowest in natural regeneration plots, intermediate in assisted restoration plots, and highest in the reference plots (Table 1). Sapling leaves in the applied nucleation and plantation plots were 10.1 and 15.2% tougher, respectively, than in the natural regeneration treatment. Sapling community wood density was equivalent across all restoration treatments, and significantly lower than reference forest values (11.3–13.2% lower).
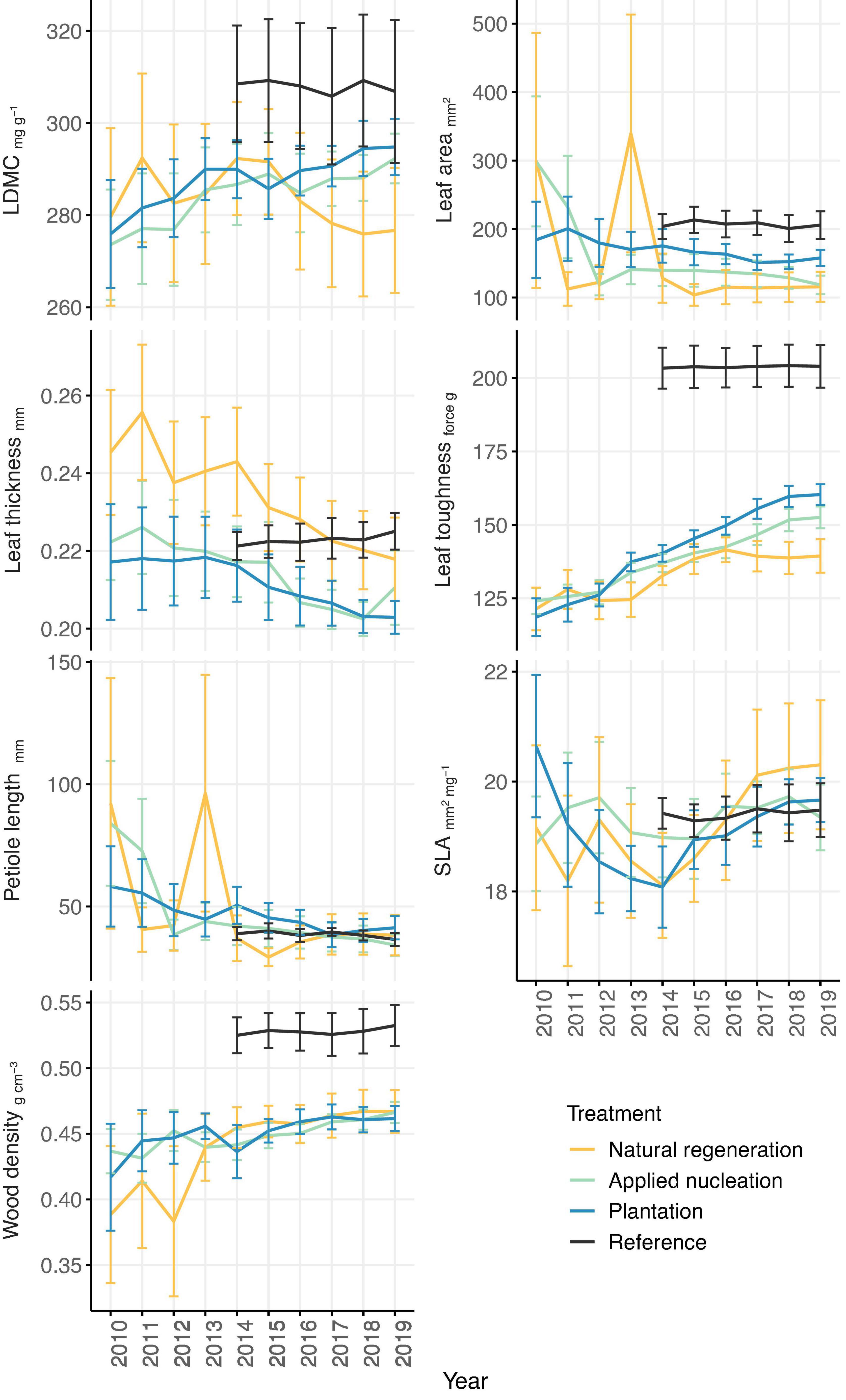
Figure 1. Annual treatment-level means (± SE) of tree sapling functional trait community-weighted means (CWMs) from 2010 to 2019 (2014–2019 in reference forest) in each of the treatments. Sample size: n = 11 sites in applied nucleation, natural regeneration, and plantation; n = 5 sites in reference; for wood density, n = 10 sites in natural regeneration as one outlier was removed.
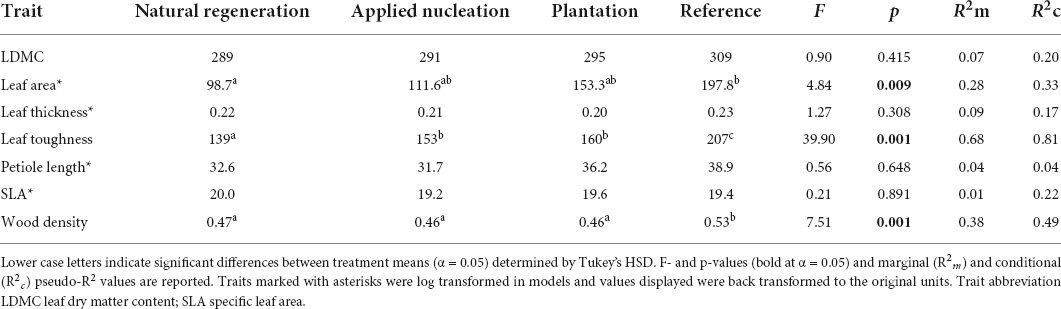
Table 1. Estimated marginal means (EMMs) for tree sapling functional trait community-weighted means (CWMs) in each treatment in 2019, estimated with linear mixed models.
Functional diversity and species richness
FRic, and species richness means in the assisted restoration treatments became more similar to values in reference plots over time, while FRic remained relatively constant in the natural regeneration plots. Interestingly, FEve values in the assisted restoration treatments diverged from the reference over time (Figure 2). At the end of the study, FDis, FEve, FRic, and species richness values differed among treatments in various ways, but no differences in FDiv were detected among treatments (Table 2). However, sapling FRic increased alongside increasing species richness in all restoration treatments, however, and by 2019 sapling species richness was more than double, and FRic more than triple, in both assisted regeneration treatments compared to natural regeneration. Moreover, the sapling community in the assisted restoration treatments had recovered to approximately half the FRic observed in the reference plots, whereas the natural regeneration treatment had an FRic value only 20% of the reference. By contrast, FDis was equivalent across the restoration treatments and between 28 and 44% lower in all restoration treatments than the reference (χ2 = 41.38, p ≤ 0.001). Finally, FEve did not differ between the assisted restoration treatments (t = -1.42, p = 0.497) but was significantly lower in the applied nucleation treatment than both the natural regeneration treatment (t = -3.21, p = 0.016) and the reference (t = -3.25, p = 0.014).
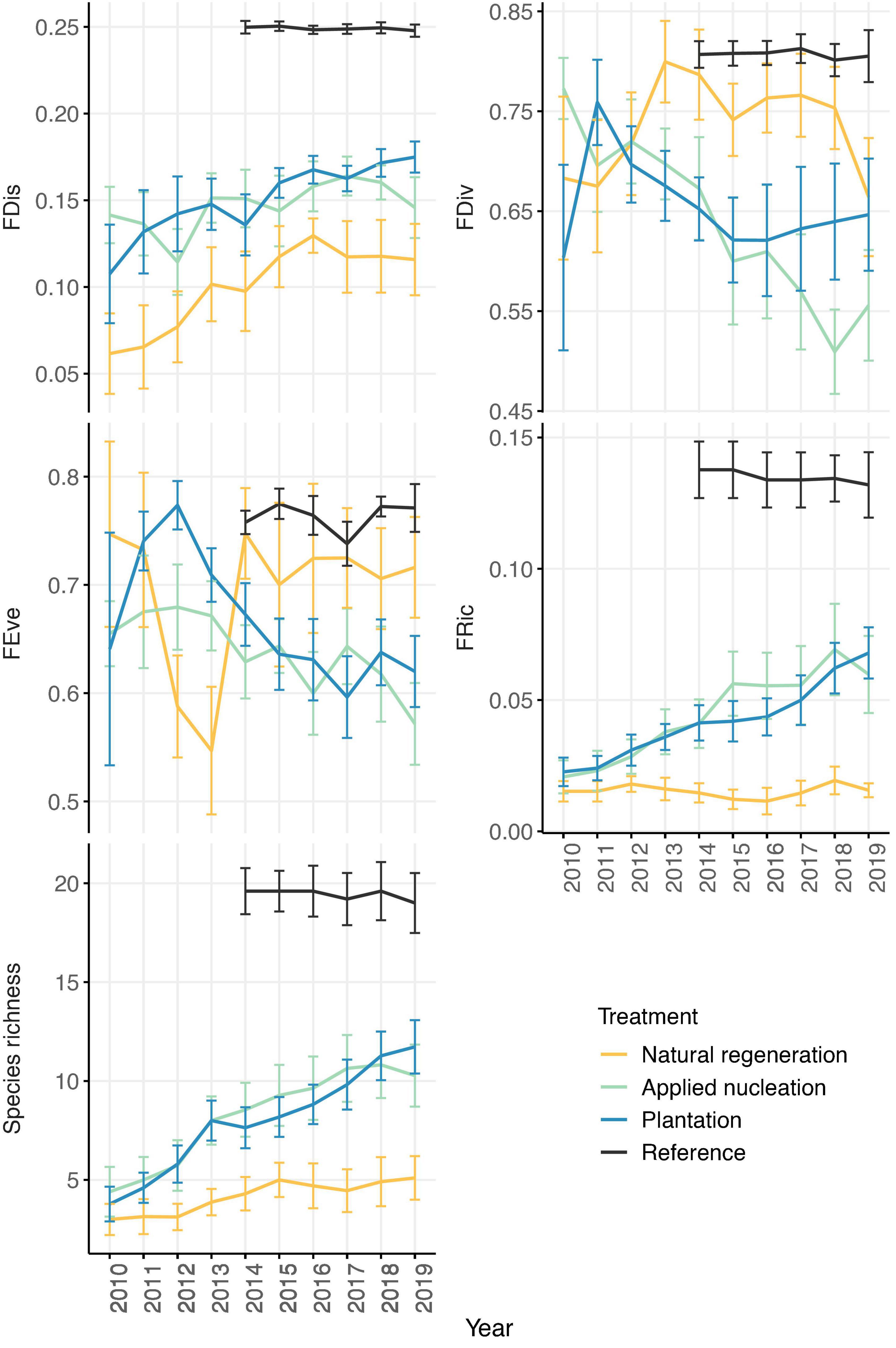
Figure 2. Annual treatment-level means (± SE) of tree sapling functional richness (FRic), divergence (FDiv), dispersion (FDis), evenness (FEve), and mean species richness values from 2010 to 2019 (2014–2019 in reference forest) in each treatment. Sample size: n = 11 sites in natural regeneration, applied nucleation, plantation; n = 5 sites in reference.
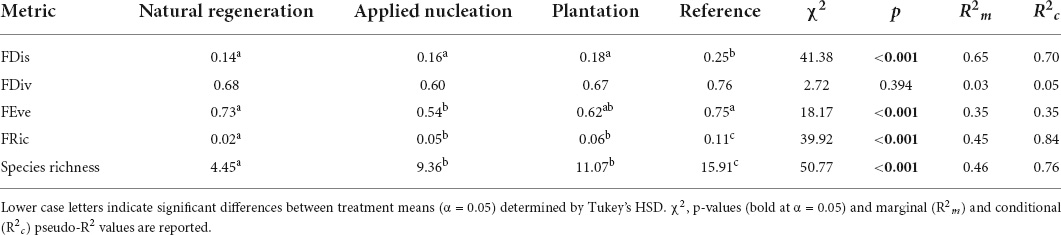
Table 2. Estimated marginal means (EMMs) for tree sapling functional richness (FRic), divergence (FDiv), dispersion (FDis), and evenness (FEve), and mean species richness values in each treatment in 2019, estimated with linear mixed models.
Trajectories of species and trait composition
Trajectory analysis indicated that focal species community composition shifted toward the reference forest from 2010 to 2019 in all restoration treatments (Figure 3). By 2019, species composition similarity to the reference forest was greatest in the plantation plots, intermediate in the applied nucleation plots, and lowest in the natural regeneration plots (Figure 3A; NMDS stress = 0.01). The same general patterns were observed for CWM trait composition (Figure 3B; NMDS stress = 0.04), and overall trait composition was most similar to the reference forest in plantation plots by 2019. Sapling species and trait composition in the reference forest remained relatively constant year to year.
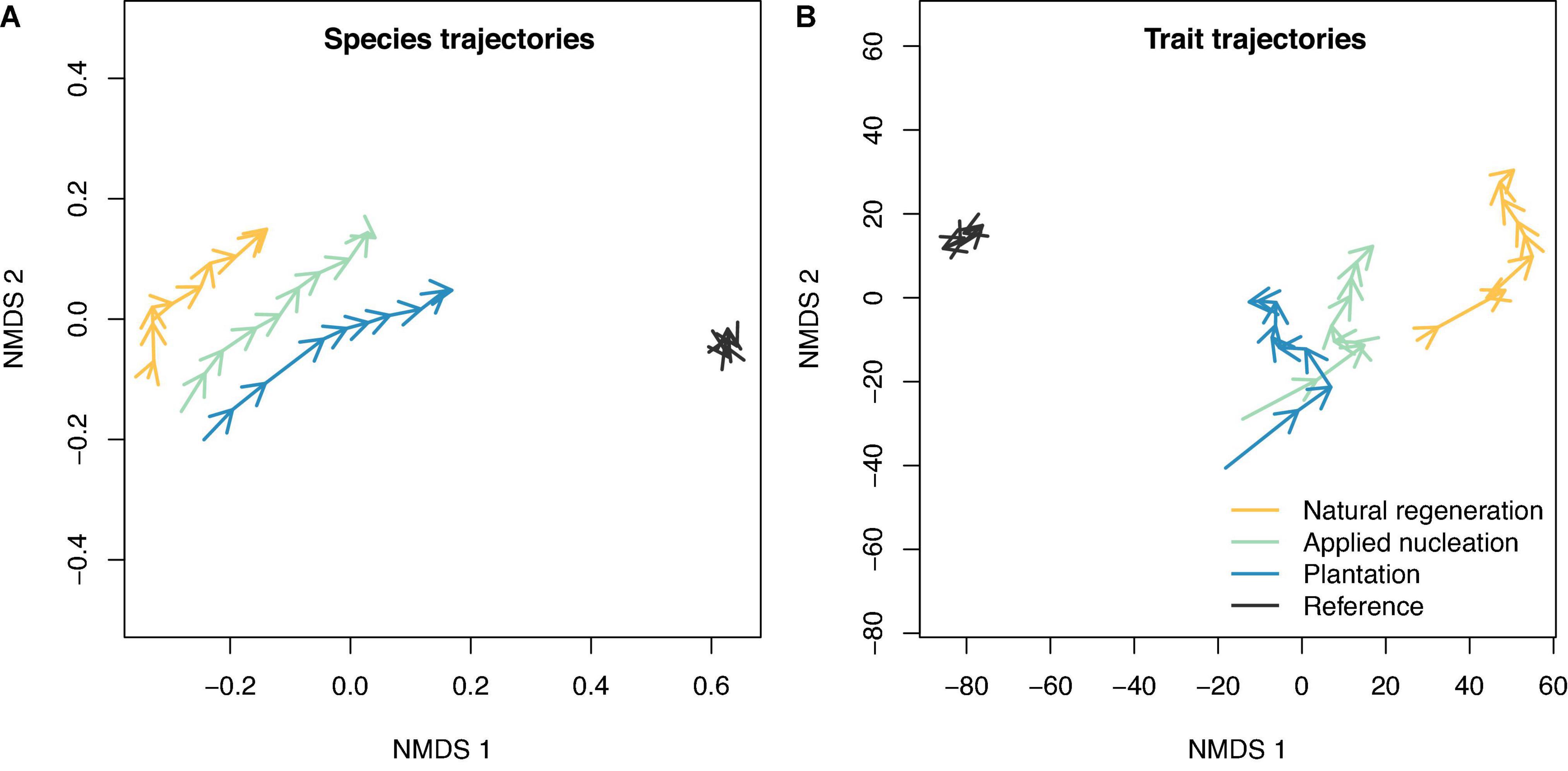
Figure 3. Community trajectories of non-metric multidimensional scaling (NMDS) fits based on a Bray-Curtis dissimilarity matrix for (A) sapling species composition, and a Euclidean distance matrix for (B) sapling community-weighted mean traits for the restoration treatments and reference forest. The tips of arrows indicate species or trait composition at the end of a year.
Discussion
Results show that assisted forest restoration interventions (i.e., applied nucleation, plantation) sped the recuperation of tree species and FRic when compared to natural regeneration. Assisted restoration interventions also shifted CWMs of specific traits toward remnant forest faster than did natural regeneration, indicating that assisted restoration interventions can facilitate the recovery of specific aspects of plant community function in this system. However, plant functional recovery in assisted restoration treatments was strongly influenced by the establishment of species observed in relatively low abundances, highlighting that arrival and establishment barriers still strongly influence vegetation recovery trajectories even after more than a decade of recovery.
Assisted interventions drive plant functional recovery
Trajectories of plant functional recovery can vary greatly in naturally regenerating tropical forests (Muscarella et al., 2017), and gradients of restoration interventions can influence the speed and direction of these trajectories (Li et al., 2018). Previous work at our sites has shown that assisted restoration interventions can speed the recovery of tree recruit species richness (Holl et al., 2017), by increasing canopy cover, which in turn attracts the dispersal of more diverse seed communities to assisted restoration plots and shade out pasture grasses that reduce seedling establishment (Holl et al., 2020). We expand on this work to elucidate how this gradient of restoration interventions have influenced the recovery of plant functional diversity over time.
Whereas, tree recruit species richness shifted toward the reference forest in all restoration treatments, FRic values in the natural regeneration treatment remained low and did not shift over time (Figure 2). Moreover, at the end of the study, tree sapling FRic in the assisted restoration plots was triple that of the natural regeneration plots on average (Table 2). Taken together, this indicates that the functional recovery of recruiting sapling communities has not only been much more rapid in the assisted restoration treatments, but also that tree communities in the natural regeneration treatment remain functionally narrow despite becoming more species rich over time. These results are similar to an experiment in tropical moist forest in Mexico, where active restoration interventions led to increases in recruit phylogenetic and functional diversity relative to natural regeneration plots over time (Li et al., 2018). However, the threefold higher FRic we observed in our assisted vs. natural recovery restoration plots was much more dramatic.
Higher FRic observed in the assisted restoration treatments was likely influenced by observed dispersal of more seeds of more species to these treatments (Werden et al., 2021), and by the presence of more suitable safe-sites for tree establishment and persistence (e.g., Norden et al., 2009). In particular the higher canopy cover, which has been observed to increase seed-to-seedling transition rates for specific species across our sites (Werden et al., 2020a). Importantly, while FRic differed considerably between assisted and natural restoration interventions at the end of the study, functional dispersion (FDis) did not (Table 2). Because FDis acts to remove the influence of species with low abundances on FRic (Laliberté and Legendre, 2010), this highlights that high values of FDis observed in the assisted restoration treatments were driven by the establishment of tree recruits of species observed in relatively low abundances. Therefore, although assisted restoration interventions are driving the regeneration of tree communities that occupy a broader functional space, communities in the assisted restoration treatments are currently far from being functionally equivalent to remnant forest communities, where higher FDis values indicate a more functionally dissimilar sapling community that is likely more resilient to disturbance (Laliberté and Legendre, 2010). Additionally, while functional evenness (FEve) did not differ across the assisted restoration treatments (Table 2), FEve in the natural restoration treatment increased over time and became more similar to the reference forest than did the assisted restoration treatments (Figure 2). This aligns with the observed dynamics of FRic and FDis, as species present in low abundances in the assisted restoration treatments are leading to an uneven distribution across trait space; whereas function in the natural regeneration treatment is likely even due to limited trait ranges, reference forest communities have high FEve due to more equitable distributions of species (Mason et al., 2005).
Restoration interventions influence trait recovery trajectories
Wood density values did not shift toward reference forests in any restoration treatment (Table 1), indicating that dense-wooded species with the potential to accumulate and store large amounts of carbon over time have not yet reached the sapling stage in the restoration treatments. While trees planted in the assisted restoration treatments have rapidly accumulated biomass, tree recruit biomass did not differ across the restoration treatments after a decade (Holl and Zahawi, 2014). Thus, trees recruiting into restoration treatments may not be making a large contribution to aboveground biomass, though these dynamics could have changed over time.
Sapling communities in both assisted restoration treatments had increased leaf toughness values that were shifting toward those observed in the reference forest (Table 1). Herbivory is a leading cause of tropical tree seedling mortality (Clark and Clark, 1985) and tougher leaves are less palatable to herbivores (Agosta et al., 2017). Kulikowski et al. (2022a) found that herbivory-driven mortality of later-successional seedlings was higher in reference forest than plantation plots, indicating that herbivory pressure, from insect folivores (i.e., caterpillars, orthopteroids, and leaf beetles; (Kulikowski et al., 2022a), is higher in reference forest. Therefore, the increasing presence and/or abundance of species with tougher, less-palatable leaves from the natural regeneration to the assisted restoration treatments, coupled with a further increase in communities dominated by species with tough leaves in the reference forest, could be a response to increasing herbivory pressure along this gradient. Moreover, this result demonstrates a shift from communities dominated by acquisitive (low toughness) to conservative (high toughness) values of this trait, indicating that, at least for certain traits, assisted restoration interventions are facilitating shifts in community-level trait values along the expected successional trajectories for tropical wet forest (i.e., acquisitive to conservative trajectory; Lohbeck et al., 2013).
We also observed a trend of increasing community-level leaf area across the gradient of restoration interventions, whereby communities in the natural regeneration treatment had the smallest leaves (low area), assisted restoration treatments had intermediate-sized leaves, and remnant forests the largest leaves (Table 1). As we measured leaf area on saplings in nearby remnant forest, this observed increase in community mean leaf area was not influenced by species-level differences in leaf area across treatments due to variation in understory light availability (i.e., lower canopy cover and thus higher light availability in the natural regeneration plots; Holl et al., 2020). Constructing larger leaves is an acquisitive resource-use strategy assumed to be typical of species in early successional environments (Westoby et al., 2002). Accordingly, the expectation is that leaf area should decrease as tropical wet forest canopy develops. However, a study in Mexico observed no changes in leaf area during tropical wet forest succession, and showed a decrease in leaf area during tropical dry forest succession (Lohbeck et al., 2013). Although our study region has high mean annual precipitation, water stress can be high at certain times of the year (San-José et al., 2021). Water stress can likely reach higher levels in the restoration treatments than the reference forest and could be especially high in the natural regeneration treatment during the 2–3 month dry season. Therefore, communities that recruit and survive in the restoration treatments could be dominated by tree species with smaller leaves, as this strategy can help minimize water loss during dry spells (Poorter et al., 2009), especially in the low canopy cover natural regeneration treatment. A second explanation could be that lower light environments in the assisted restoration treatments and reference forest favor the recruitment of tree communities with larger leaves that can capture more light. Indeed, a study in this region noted that seedlings planted under different light conditions typically construct larger leaves when planted in the shade (Loik and Holl, 1999), though further investigation is necessary to describe patterns of intraspecific variation in leaf size across the treatments.
Implications for forest restoration
Our results show that assisted restoration interventions catalyzed recovery of FRic when compared to natural regeneration in this degraded system, with the caveat that shifts in FRic were driven by the recruitment of tree species present in relatively low abundances at the end of the study. A pan-tropical analysis of natural forest recovery estimated that it generally takes at least two decades for tropical forests to recover average wood density observed in remnant forests, and up to four decades to recover natural proportions of nitrogen-fixing trees (Poorter et al., 2021). While all treatments still differed considerably from the reference forest after a decade of recovery, FRic in the assisted restoration treatments recovered more rapidly than the natural regeneration treatment (Figure 2). Thus, while functional recovery can be slow in tropical systems, our results highlight that planting trees can speed the recovery of plant functional composition by creating canopy cover that attracts seed dispersers to restored areas (Reid et al., 2014). Which species recruit in a given area and how quickly they do so is also strongly affected by the presence of mother trees within 100 m of restoration plots (Zahawi et al., 2021). As such, it is important to ensure that there are sufficient adjacent seed sources to restoration interventions, or planted trees with animal-dispersed seeds that attract dispersers (de la Peña-Domene et al., 2013), as this will ultimately impose a major filter on the initial functional composition of tree recruits. Additionally, other studies have noted the importance of including woody species that are slow to recruit in the initial floristic composition of plantings (Toledo-Aceves et al., 2021), such as shade-tolerant species with low SLA (Toledo-Aceves et al., 2022).
Detailing how gradients of restoration intervention influence functional recovery trajectories does not only refine frameworks facilitating the prediction of ecosystem restoration outcomes across a variety of contexts (Brudvig et al., 2017; Brudvig and Catano, 2021), but also can also help to standardize the comparison of recovery trajectories between sites/projects (Brancalion and Holl, 2016). To that end, our findings showed that simply quantifying the recovery of metrics such as species richness can mask a more mechanistic understanding of ecosystem recovery that is elucidated by taking a functional approach toward evaluating outcomes, and tracking long-term changes in forest structure (San-José et al., 2021). For example, our work identified a paucity of species with dense wood recruiting across our restoration interventions, and showed that mean wood density of recruiting communities did not differ across intervention, underscoring that species with dense wood are prime targets for enrichment planting (e.g., Mangueira et al., 2019). As such, using enrichment planting to fill in areas of trait space not encompassed by natural recruits could potentially further speed the functional recovery of tropical forests, in addition to other promising approaches such as designing enrichment plantings with the phylogenetic composition of overstory tree species in mind (Schweizer et al., 2013). Doing so would simultaneously address the common goals of restoring forest for both biodiversity and carbon sequestration (e.g., Poorter et al., 2021).
Conclusion
By examining the functional recovery of tree species across a gradient of restoration interventions, our analysis demonstrates that assisted restoration spurs the functional recovery of tree communities, when compared to slow recovery observed when employing natural regeneration in this system. Moreover, functional recovery was equivalent in the assisted restoration treatments, highlighting that applied nucleation could be a more cost-effective approach in this context, as it requires approximately a third of the investment of standard plantation forestry (Holl et al., 2011). Results also indicate that multi-trait analysis can provide a more complete picture of recovery trajectories than single community-weighted trait means, as leaf toughness was the only trait that separated the assisted restoration treatments from natural regeneration. Overall, our work contributes to a growing predictive framework focused on how and when to restore the world’s forests, which are increasingly impacted by anthropogenic disturbances and global change.
Data availability statement
All leaf and stem functional trait data collected for this study have been archived in TRY. All sapling recruitment data can be found in Dryad archive: https://datadryad.org/stash/dataset/doi:10.7291/D1H10M.
Author contributions
KH and RZ created and implemented the restoration experiment. LW and SZ designed and implemented the functional recovery study. SZ, CO, and LW collected functional trait data. KH, RZ, FO-B, JR, and LW conducted sapling surveys. SZ and LW conducted the statistical analyses. LW wrote the first draft of the manuscript. All authors contributed to the revisions.
Funding
This study was supported by the US National Science Foundation (NSF) grants (DEB 05-15577, DEB 09-18112, DEB 14-56520, and DEB 20-16623) to KH and RZ and a NSF PRFB (#1907160) to LW.
Acknowledgments
We thank J. Flores for helping locate saplings for trait measurements; A. Kulikowski, A. Rubio, J. M. Chaves-Fallas, S. Rosas, and several others for help with sapling surveys; Las Cruces Biological Station for logistic support; M. San-José for making the map of the plots; R. Cole and other landowners for use of their land. We also thank two reviewers and subject editor Arshad Ali who provided excellent feedback that helped to improve our manuscript.
Conflict of interest
The authors declare that the research was conducted in the absence of any commercial or financial relationships that could be construed as a potential conflict of interest.
Publisher’s note
All claims expressed in this article are solely those of the authors and do not necessarily represent those of their affiliated organizations, or those of the publisher, the editors and the reviewers. Any product that may be evaluated in this article, or claim that may be made by its manufacturer, is not guaranteed or endorsed by the publisher.
Supplementary material
The Supplementary Material for this article can be found online at: https://www.frontiersin.org/articles/10.3389/ffgc.2022.935011/full#supplementary-material
Footnotes
References
Agosta, S. J., Hulshof, C. M., and Staats, E. G. (2017). Organismal responses to habitat change: Herbivore performance, climate and leaf traits in regenerating tropical dry forests. J. Anim. Ecol. 86, 590–604. doi: 10.1111/1365-2656.12647
Arroyo-Rodríguez, V., Melo, F. P. L., Martínez-Ramos, M., Bongers, F., Chazdon, R. L., Meave, J. A., et al. (2017). Multiple successional pathways in human-modified tropical landscapes: New insights from forest succession, forest fragmentation and landscape ecology research: Multiple successional pathways. Biol. Rev. 92, 326–340. doi: 10.1111/brv.12231
Becknell, J. M., and Powers, J. S. (2014). Stand age and soils as drivers of plant functional traits and aboveground biomass in secondary tropical dry forest. Can. J. For. Res. 44, 604–613. doi: 10.1139/cjfr-2013-0331
Brancalion, P. H. S., and Holl, K. D. (2016). Functional composition trajectory: A resolution to the debate between Suganuma, Durigan, and Reid. Restor. Ecol. 24, 1–3. doi: 10.1111/rec.12312
Brudvig, L. A., and Catano, C. P. (2021). Prediction and uncertainty in restoration science. Restor. Ecol. e13380. doi: 10.1111/rec.13380
Brudvig, L. A., Barak, R. S., Bauer, J. T., Caughlin, T. T., Laughlin, D. C., Larios, L., et al. (2017). Interpreting variation to advance predictive restoration science. J. Appl. Ecol. 54, 1018–1027. doi: 10.1111/1365-2664.12938
Buzzard, V., Hulshof, C. M., Birt, T., Violle, C., and Enquist, B. J. (2016). Re-growing a tropical dry forest: Functional plant trait composition and community assembly during succession. Funct. Ecol. 30, 1006–1013. doi: 10.1111/1365-2435.12579
Catano, C. P., Bassett, T. J., Bauer, J. T., Grman, E., Groves, A. M., Zirbel, C. R., et al. (2022). Soil resources mediate the strength of species but not trait convergence across grassland restorations. J. Appl. Ecol. 59, 384–393. doi: 10.1111/1365-2664.13929
Chave, J., Coomes, D., Jansen, S., Lewis, S. L., Swenson, N. G., and Zanne, A. E. (2009). Towards a worldwide wood economics spectrum. Ecol. Lett. 12, 351–366. doi: 10.1111/j.1461-0248.2009.01285.x
Chazdon, R. L., Falk, D. A., Banin, L. F., Wagner, M., Wilson, S., Grabowski, R. C., et al. (2021). The intervention continuum in restoration ecology: Rethinking the active–passive dichotomy. Restor. Ecol. e13535. doi: 10.1111/rec.13535
Chua, S. C., and Potts, M. D. (2018). The role of plant functional traits in understanding forest recovery in wet tropical secondary forests. Sci. Total Environ. 642, 1252–1262. doi: 10.1016/j.scitotenv.2018.05.397
Clark, D. B., and Clark, D. A. (1985). Seedling dynamics of a tropical tree: Impacts of herbivory and meristem damage. Ecology 66, 1884–1892. doi: 10.2307/2937384
Cooke, S. J., Bennett, J. R., and Jones, H. P. (2019). We have a long way to go if we want to realize the promise of the “Decade on ecosystem restoration.”. Conserv. Sci. Pract. 1:e129. doi: 10.1111/csp2.129
Cook-Patton, S. C., Leavitt, S. M., Gibbs, D., Harris, N. L., Lister, K., Anderson-Teixeira, K. J., et al. (2020). Mapping carbon accumulation potential from global natural forest regrowth. Nature 585, 545–550. doi: 10.1038/s41586-020-2686-x
Cook-Patton, S. C., Shoch, D., and Ellis, P. W. (2021). Dynamic global monitoring needed to use restoration of forest cover as a climate solution. Nat. Clim. Change 11, 366–368. doi: 10.1038/s41558-021-01022-9
Corbin, J. D., and Holl, K. D. (2012). Applied nucleation as a forest restoration strategy. For. Ecol. Manag. 265, 37–46. doi: 10.1016/j.foreco.2011.10.013
Crouzeilles, R., Ferreira, M. S., Chazdon, R. L., Lindenmayer, D. B., Sansevero, J. B. B., Monteiro, L., et al. (2017). Ecological restoration success is higher for natural regeneration than for active restoration in tropical forests. Sci. Adv. 3:e1701345. doi: 10.1126/sciadv.1701345
De Cáceres, M., Coll, L., Legendre, P., Allen, R. B., Wiser, S. K., Fortin, M.-J., et al. (2019). Trajectory analysis in community ecology. Ecol. Monogr. 89:e01350. doi: 10.1002/ecm.1350
de la Peña-Domene, M., Howe, H. F., Cruz-León, E., Jiménez-Rolland, R., Lozano-Huerta, C., and Martínez-Garza, C. (2017). Seed to seedling transitions in successional habitats across a tropical landscape. Oikos 126, 410–419. doi: 10.1111/oik.03394
de la Peña-Domene, M., Martínez-Garza, C., and Howe, H. F. (2013). Early recruitment dynamics in tropical restoration. Ecol. Applic. 23, 1124–1134. doi: 10.1890/12-1728.1
Dğíaz, S., and Cabido, M. (2001). Vive la différence: Plant functional diversity matters to ecosystem processes. Trends Ecol. Evol. 16, 646–655. doi: 10.1016/S0169-5347(01)02283-2
Díaz, S., Kattge, J., Cornelissen, J. H. C., Wright, I. J., Lavorel, S., Dray, S., et al. (2016). The global spectrum of plant form and function. Nature 529, 167–171. doi: 10.1038/nature16489
Diaz, S., Lavorel, S., de Bello, F., Quetier, F., Grigulis, K., and Robson, T. M. (2007). Incorporating plant functional diversity effects in ecosystem service assessments. Proc. Natl. Acad. Sci. U.S.A. 104, 20684–20689. doi: 10.1073/pnas.0704716104
Grime, J. P. (1998). Benefits of plant diversity to ecosystems: Immediate, filter and founder effects. J. Ecol. 86, 902–910. doi: 10.1046/j.1365-2745.1998.00306.x
Holdridge, L. R., Grenke, W. C., Hatheway, W. H., Liang, T., and Tosi, J. A. (1971). Forest Environments in Tropical life Zones. Oxford: Pergamon Press.
Holl, K. D. (1999). Factors limiting tropical rain forest regeneration in abandoned pasture: Seed rain, seed germination, microclimate, and soil. Biotropica 31, 229–242. doi: 10.1111/j.1744-7429.1999.tb00135.x
Holl, K. D. (2012). “Restoration of tropical forests,” in Restoration Ecology, eds J. van Andel and J. Aronson (Hoboken, NJ: John Wiley & Sons, Ltd), 103–114. doi: 10.1002/9781118223130.ch9
Holl, K. D., and Aide, T. M. (2011). When and where to actively restore ecosystems? For. Ecol. Manag. 261, 1558–1563. doi: 10.1016/j.foreco.2010.07.004
Holl, K. D., and Zahawi, R. A. (2014). Factors explaining variability in above-ground biomass accumulation in restored tropical forest. For. Ecol. Manag. 319, 36–43. doi: 10.1016/j.foreco.2014.01.024
Holl, K. D., Reid, J. L., Chaves-Fallas, J. M., Oviedo-Brenes, F., and Zahawi, R. A. (2017). Local tropical forest restoration strategies affect tree recruitment more strongly than does landscape forest cover. J. Appl. Ecol. 54, 1091–1099. doi: 10.1111/1365-2664.12814
Holl, K. D., Reid, J. L., Cole, R. J., Oviedo-Brenes, F., Rosales, J. A., and Zahawi, R. A. (2020). Applied nucleation facilitates tropical forest recovery: Lessons learned from a 15-year study. J. Appl. Ecol. 57, 2316–2328. doi: 10.1111/1365-2664.13684
Holl, K. D., Zahawi, R. A., Cole, R. J., Ostertag, R., and Cordell, S. (2011). Planting seedlings in tree Islands versus plantations as a large-scale tropical forest restoration strategy. Restor. Ecol. 19, 470–479. doi: 10.1111/j.1526-100X.2010.00674.x
Kattge, J., Bönisch, G., Díaz, S., Lavorel, S., Prentice, I. C., Leadley, P., et al. (2020). TRY plant trait database – enhanced coverage and open access. Glob. Change Biol. 26, 119–188. doi: 10.1111/gcb.14904
Kulikowski, A. J., Zahawi, R. A., and Holl, K. D. (2022a). Effects of insect herbivory on seedling mortality in restored and remnant tropical forest. Restor. Ecol. 30, 1–8. doi: 10.1111/rec.13467
Kulikowski, A. J., Zahawi, R. A., Werden, L. K., Zhu, K., and Holl, K. D. (2022b). Restoration interventions mediate tropical tree recruitment dynamics over time. Philos. Trans. R. Soc. B Biol. Sci.
Laliberté, E., and Legendre, P. (2010). A distance-based framework for measuring functional diversity from multiple traits. Ecology 91, 299–305. doi: 10.1890/08-2244.1
Lamb, D. (2005). Restoration of degraded tropical forest landscapes. Science 310, 1628–1632. doi: 10.1126/science.1111773
Li, L., Cadotte, M. W., Martínez-Garza, C., de la Peña-Domene, M., and Du, G. (2018). Planting accelerates restoration of tropical forest but assembly mechanisms appear insensitive to initial composition. J. Appl. Ecol. 55, 986–996. doi: 10.1111/1365-2664.12976
Lohbeck, M., Lebrija-Trejos, E., Martínez-Ramos, M., Meave, J. A., Poorter, L., and Bongers, F. (2015). Functional trait strategies of trees in dry and wet tropical forests are similar but differ in their consequences for succession. PLoS One 10:e0123741. doi: 10.1371/journal.pone.0123741
Lohbeck, M., Poorter, L., Lebrija-Trejos, E., Martínez-Ramos, M., Meave, J. A., Paz, H., et al. (2013). Successional changes in functional composition contrast for dry and wet tropical forest. Ecology 94, 1211–1216. doi: 10.1890/12-1850.1
Loik, M. E., and Holl, K. D. (1999). Photosynthetic responses to light for rainforest seedlings planted in abandoned pasture, Costa Rica. Restor. Ecol. 7, 382–391. doi: 10.1046/j.1526-100X.1999.72033.x
Mangueira, J. R. S. A., Holl, K. D., and Rodrigues, R. R. (2019). Enrichment planting to restore degraded tropical forest fragments in Brazil. Ecosyst. People 15, 3–10. doi: 10.1080/21513732.2018.1529707
Mason, N. W. H., Mouillot, D., Lee, W. G., and Wilson, J. B. (2005). Functional richness, functional evenness and functional divergence: The primary components of functional diversity. Oikos 111, 112–118. doi: 10.1111/j.0030-1299.2005.13886.x
Meli, P., Holl, K. D., Rey Benayas, J. M., Jones, H. P., Jones, P. C., Montoya, D., et al. (2017). A global review of past land use, climate, and active vs. passive restoration effects on forest recovery. PLoS One 12:e0171368. doi: 10.1371/journal.pone.0171368
Montoya, D., Rogers, L., and Memmott, J. (2012). Emerging perspectives in the restoration of biodiversity-based ecosystem services. Trends Ecol. Evol. 27, 666–672. doi: 10.1016/j.tree.2012.07.004
Muscarella, R., Lohbeck, M., Martínez-Ramos, M., Poorter, L., Rodríguez-Velázquez, J. E., van Breugel, M., et al. (2017). Demographic drivers of functional composition dynamics. Ecology 98, 2743–2750. doi: 10.1002/ecy.1990
Nakagawa, S., and Schielzeth, H. (2013). A general and simple method for obtaining R 2 from generalized linear mixed-effects models. Methods Ecol. Evol. 4, 133–142. doi: 10.1111/j.2041-210x.2012.00261.x
Norden, N., Angarita, H. A., Bongers, F., Martínez-Ramos, M., Granzow-de la Cerda, I., van Breugel, M., et al. (2015). Successional dynamics in Neotropical forests are as uncertain as they are predictable. Proc. Natl. Acad. Sci. U.S.A. 112, 8013–8018. doi: 10.1073/pnas.1500403112
Norden, N., Chave, J., Belbenoit, P., Caubère, A., Châtelet, P., Forget, P.-M., et al. (2009). Interspecific variation in seedling responses to seed limitation and habitat conditions for 14 Neotropical woody species. J. Ecol. 97, 186–197. doi: 10.1111/j.1365-2745.2008.01444.x
Ostertag, R., Cordell, S., Michaud, J., Cole, T. C., Schulten, J. R., Publico, K. M., et al. (2009). Ecosystem and restoration consequences of invasive woody species removal in hawaiian lowland wet forest. Ecosystems 12, 503–515. doi: 10.1007/s10021-009-9239-3
Ostertag, R., Warman, L., Cordell, S., and Vitousek, P. M. (2015). Using plant functional traits to restore Hawaiian rainforest. J. Appl. Ecol. 52, 805–809. doi: 10.1111/1365-2664.12413
Pakeman, R. J., and Quested, H. M. (2007). Sampling plant functional traits: What proportion of the species need to be measured? Appl. Veget. Sci. 10, 91–96. doi: 10.1111/j.1654-109X.2007.tb00507.x
Pérez-Harguindeguy, N., Díaz, S., Garnier, E., Lavorel, S., Poorter, H., Jaureguiberry, P., et al. (2013). New handbook for standardised measurement of plant functional traits worldwide. Austr. J. Bot. 61, 167. doi: 10.1071/BT12225
Philipson, C. D., Cutler, M. E. J., Brodrick, P. G., Asner, G. P., Boyd, D. S., Moura Costa, P., et al. (2020). Active restoration accelerates the carbon recovery of human-modified tropical forests. Science 369, 838–841. doi: 10.1126/science.aay4490
Poorter, H., Niinemets, Ü, Poorter, L., Wright, I. J., and Villar, R. (2009). Causes and consequences of variation in leaf mass per area (LMA): A meta-analysis. N. Phytol. 182, 565–588. doi: 10.1111/j.1469-8137.2009.02830.x
Poorter, L., Craven, D., Jakovac, C. C., van der Sande, M. T., Amissah, L., Bongers, F., et al. (2021). Multidimensional tropical forest recovery. Science 374, 1370–1376. doi: 10.1126/science.abh3629
R Development Core Team (2021). R: A Language and Environment for Statistical Computing. Version 4.0.4. Vienna: R Foundation for Statistical Computing.
Reich, P. B. (2014). The world-wide ‘fast-slow’ plant economics spectrum: A traits manifesto. J. Ecol. 102, 275–301. doi: 10.1111/1365-2745.12211
Reid, J. L., Fagan, M. E., and Zahawi, R. A. (2018). Positive site selection bias in meta-analyses comparing natural regeneration to active forest restoration. Sci. Adv. 4:eaas9143. doi: 10.1126/sciadv.aas9143
Reid, J. L., Mendenhall, C. D., Rosales, J. A., Zahawi, R. A., and Holl, K. D. (2014). Landscape context mediates avian habitat choice in tropical forest restoration. PLoS One 9:e90573. doi: 10.1371/journal.pone.0090573
San-José, M., Werden, L., Peterson, C. J., Oviedo-Brenes, F., and Zahawi, R. A. (2021). Large tree mortality leads to major aboveground biomass decline in a tropical forest reserve. Oecologia 197, 795–806. doi: 10.1007/s00442-021-05048-w
Schweizer, D., Gilbert, G. S., and Holl, K. D. (2013). Phylogenetic ecology applied to enrichment planting of tropical native tree species. For. Ecol. Manag. 297, 57–66. doi: 10.1016/j.foreco.2013.02.012
Shoo, L. P., and Catterall, C. P. (2013). Stimulating natural regeneration of tropical forest on degraded land: Approaches, outcomes, and information gaps: Stimulating natural regeneration on degraded land. Restor. Ecol. 21, 670–677. doi: 10.1111/rec.12048
Toledo-Aceves, T., Bonilla-Moheno, M., Sosa, V. J., López-Barrera, F., and Williams-Linera, G. (2022). Leaf functional traits predict shade tolerant tree performance in cloud forest restoration plantings. J. Appl. Ecol. doi: 10.1111/1365-2664.14128
Toledo-Aceves, T., Trujillo-Miranda, A. L., and López-Barrera, F. (2021). Tree regeneration in active and passive cloud forest restoration: Functional groups and timber species. For. Ecol. Manag. 489:119050. doi: 10.1016/j.foreco.2021.119050
Villéger, S., Mason, N. W. H., and Mouillot, D. (2008). New multidimensional functional diversity indices for a multifaceted framework in functional ecology. Ecology 89, 2290–2301. doi: 10.1890/07-1206.1
Violle, C., Navas, M.-L., Vile, D., Kazakou, E., Fortunel, C., Hummel, I., et al. (2007). Let the concept of trait be functional! Oikos 116, 882–892. doi: 10.1111/j.0030-1299.2007.15559.x
Wang, C., Zhang, W., Li, X., and Wu, J. (2021). A global meta-analysis of the impacts of tree plantations on biodiversity. Glob. Ecol. Biogeogr. 31:geb.13440. doi: 10.1111/geb.13440
Werden, L. K., Alvarado, J. P., Zarges, S., Calderón, M. E., Schilling, E. M., Gutiérrez, L. M., et al. (2018). Using soil amendments and plant functional traits to select native tropical dry forest species for the restoration of degraded Vertisols. J. Appl. Ecol. 55, 1019–1028. doi: 10.1111/1365-2664.12998
Werden, L. K., Calderón-Morales, E., Alvarado, J. P., Gutiérrez, L. M., Nedveck, D. A., and Powers, J. S. (2020a). Using large-scale tropical dry forest restoration to test successional theory. Ecol. Applic. 30, 1–17. doi: 10.1002/eap.2116
Werden, L. K., Holl, K. D., Rosales, J. A., Sylvester, J. M., and Zahawi, R. A. (2020b). Effects of dispersal- and niche-based factors on tree recruitment in tropical wet forest restoration. Ecol. Applic. 30:e02139. doi: 10.1002/eap.2139
Werden, L. K., Holl, K. D., Chaves-Fallas, J. M., Oviedo-Brenes, F., Rosales, J. A., and Zahawi, R. A. (2021). Degree of intervention affects interannual and within-plot heterogeneity of seed arrival in tropical forest restoration. J. Appl. Ecol. 58, 1693–1704. doi: 10.1111/1365-2664.13907
Westoby, M., Falster, D. S., Moles, A. T., Vesk, P. A., and Wright, I. J. (2002). Plant ecological strategies: Some leading dimensions of variation between species. Annu. Rev. Ecol. Syst. 33, 125–159. doi: 10.1146/annurev.ecolsys.33.010802.150452
Wright, I. J., Reich, P. B., Westoby, M., Ackerly, D. D., Baruch, Z., Bongers, F., et al. (2004). The worldwide leaf economics spectrum. Nature 428, 821–827. doi: 10.1038/nature02403
Wright, S. J., Kitajima, K., Kraft, N. J. B., Reich, P. B., Wright, I. J., Bunker, D. E., et al. (2010). Functional traits and the growth–mortality trade-off in tropical trees. Ecology 91:11.
Zahawi, R. A., Duran, G., and Kormann, U. (2015). Sixty-seven years of land-use change in southern Costa Rica. PLoS One 10:e0143554. doi: 10.1371/journal.pone.0143554
Zahawi, R. A., Holl, K. D., Cole, R. J., and Reid, J. L. (2013). Testing applied nucleation as a strategy to facilitate tropical forest recovery. J. Appl. Ecol. 50, 88–96. doi: 10.1111/1365-2664.12014
Keywords: applied nucleation, community assembly, Costa Rica, functional diversity, natural regeneration, tree plantation, sapling recruitment, tropical wet forest
Citation: Werden LK, Zarges S, Holl KD, Oliver CL, Oviedo-Brenes F, Rosales JA and Zahawi RA (2022) Assisted restoration interventions drive functional recovery of tropical wet forest tree communities. Front. For. Glob. Change 5:935011. doi: 10.3389/ffgc.2022.935011
Received: 03 May 2022; Accepted: 04 August 2022;
Published: 25 August 2022.
Edited by:
Arshad Ali, Hebei University, ChinaReviewed by:
Tarin Toledo-Aceves, Instituto de Ecología (INECOL), MexicoGreg R. Guerin, University of Adelaide, Australia
Copyright © 2022 Werden, Zarges, Holl, Oliver, Oviedo-Brenes, Rosales and Zahawi. This is an open-access article distributed under the terms of the Creative Commons Attribution License (CC BY). The use, distribution or reproduction in other forums is permitted, provided the original author(s) and the copyright owner(s) are credited and that the original publication in this journal is cited, in accordance with accepted academic practice. No use, distribution or reproduction is permitted which does not comply with these terms.
*Correspondence: Leland K. Werden, bHdlcmRlbkBnbWFpbC5jb20=
†These authors have contributed equally to this work