- 1Facultad de Ciencias Biológicas, Universidad Central del Ecuador, Quito, Ecuador
- 2Biodiversity and Landscape Research Unit, Gembloux Agro-Bio Tech, University of Liege, Gembloux, Belgium
- 3Faculty of Land and Food Systems, University of British Columbia, Vancouver, BC, Canada
Land use changes cause soil degradation and loss of biodiversity, thereby affecting ecological processes and soil-associated ecosystem services. However, land use change impacts on soil health have received little attention in the highland landscapes of the tropics. In this research, using the soil health framework, we assessed the impact of native forest conversion to anthropic systems (planted forests, pastures, and monocultures) on two ecosystem services: biodiversity conservation and soil fertility in the highlands of northern Ecuador. The biological dimension of our assessment focused on the diversity, abundance, and biomass of soil macroinvertebrate communities as proxies to soil functions, whereas soil chemical parameters were used to describe the soil fertility. The soil invertebrate communities and soil chemical parameters were studied in topsoil samples using 25 × 25 × 10 cm monoliths, obtained from 10 sampling sites randomly selected in each land use category. We hypothesized that native forests would present more diverse and even soil macroinvertebrate communities, and together with their soil chemical properties would indicate better soil quality than anthropic environments. Our results showed that the structure and composition of the edaphic macroinvertebrate communities significantly differed among the studied land use categories. As predicted, native forests presented greater values for richness, evenness and diversity of soil biota than did the other categories, demonstrating a significant loss of taxonomic biodiversity at order and genus levels. We also found a significant reduction of trophic diversity in native forests converted to anthropic environments. More trophic groups with greater abundances were found in native forests, where predators and detritivores stood out as dominant groups, indicating the good quality of the soil. The results from the soil chemical parameters also confirmed the distinction in soil health between native forests and anthropic environments. Our results highlight the risk associated with current trends of native forest loss and conversion to anthropic systems in high mountain ecosystems in the tropics, illustrating how these alterations could cause biodiversity loss and degradation of the chemical attributes of soil health. The findings of this research could contribute to the conservation and sustainable management of mountain agricultural landscapes in the study region.
Introduction
Considerable evidence demonstrates that the world's ecosystems are affected as a result of human activities Millennium Ecosystem Assessment, 2005; Díaz et al., 2006, with land use change being one of the most important factors that transforms terrestrial ecosystems (Foley et al., 2005; Winkler et al., 2021). Conversion of native forests to anthropic environments, such as agricultural land for food production or to planted forest for timber extraction, has generated alterations in vegetation cover and biodiversity for various biomes and continents, negatively impacting soils and their derived ecosystem services (Sylvain and Wall, 2011; Nielsen et al., 2015; Veldkamp et al., 2020; Zarafshar et al., 2020). Soil degradation attributed to land use conversion is a major problem in the tropics, affecting the overall resilience of the socio-ecological systems (Delelegn et al., 2017; De Valença et al., 2017).
Tropical mountain landscapes, such as the Ecuadorian Andes, are characterized by complex topography, severe intensity in weather conditions, and poor management practices (Farley, 2007). The long history of land use transformation in these landscapes, mostly shaped by agricultural and livestock activities, has caused severe soil degradation (Lema, 2016; Guarderas et al., 2022), affecting agricultural yield productivity, food security, and the overall delivery of vital ecosystem services (Suquilanda, 2008). In addition, today native montane forests occur only as remnant patches, and these fragile ecosystems are still highly threatened by land use changes (Gaglio et al., 2017; Guarderas et al., 2022). Thus, understanding the magnitude and trend of changes in soil quality due to land use conversion is pivotal to promote sustainable and productive landscapes in the Ecuadorian Andes (Nielsen et al., 2015).
Land use conversion can produce significant disturbances in the soil environment (Lukina et al., 2011; Sylvain and Wall, 2011; Comerford et al., 2013). Soil moisture, structure, aeration, pH, nutrient status, microbial biomass, enzymatic activities, and the structure of edaphic communities are largely altered due to land use changes and different soil management practices. Therefore, soil health could be severely affected by land use changes (Delelegn et al., 2017; Mann et al., 2019).
“Soil health, also referred to as soil quality, is defined as the continued capacity of soil to function as a vital living ecosystem that sustains plants, animals, and humans” (Natural Resources Conservation Service, 2022). According to Bünemann et al. (2018), the functions of living soil could be defined as the bundles of soil processes that underpin the delivery of ecosystem services. This integrated view transcends the productivity-oriented function of soils to wider frameworks that include the maintenance of environmental quality and biodiversity conservation. In addition, soil health is focused on the dynamic soil properties that can be strongly influenced by management and are mainly monitored in topsoils (0–25 cm) (Karlen et al., 2003). Soil health under different land uses can be assessed using indicators that measure the properties of soil or plants that provide clues about how well the soil can function. These indicators should interact synergistically and could encompass the physical, chemical, and biological attributes of soils (Karlen et al., 2003; Delelegn et al., 2017). However, quantifying soil health is still dominated by chemical indicators, despite the growing appreciation of the importance of soil biodiversity (Lehmann et al., 2020).
The biological attributes of soil health are those associated with the soil biota, in other words, its biodiversity, food web structure, activity, and the range of functions it performs (Bünemann et al., 2018). However, the remarkable biodiversity harbored in soils has been poorly described, and even when taxonomic information are available, less is known about the functional roles of the great majority of these organisms within the ecosystems they occur (Eisenhauer et al., 2017).
The use of edaphic macrofauna as a soil indicator is considered an advantage because they are the first to manifest the changes that involve environmental disturbances (Cabrera, 2014). Soil diversity and macrofaunal biota play an important ecological role in the functioning of the soil environment and the belowground part of terrestrial ecosystems. They regulate nutrient cycles, organic matter, mineralization, modification of the soil structure, and water regime (Food Agriculture Organization of the United Nations, 2015). In addition, soil fauna is considered an appropriate bioindicator to measure soil quality due to its sedentary characteristics, permanence throughout the year, ease of measurement, and high sensitivity and rapid response to environmental stress. Monitoring soil macrofaunal communities, in combination with chemical and other biological soil properties, could improve the assessment of soil health in a more integrative manner (Cabrera, 2014; Lehmann et al., 2020).
Although the study of soil ecology is currently growing in the scientific literature (Brown et al., 2001; Decaëns et al., 2006; Nielsen et al., 2015; Eisenhauer et al., 2017), we need better insight into soil chemical parameters and biodiversity to determine the impacts on soil health in response to the altered environments (Food Agriculture Organization, 2015). This need is highlighted in tropical mountain landscapes where few studies have been conducted (Lema, 2016).
Therefore, using the soil health framework, our study was aimed at assessing the effect of land use change on soil fertility and soil biodiversity conservation in the highlands of northern Ecuador. We studied soils under native forests, as a reference system, to compare the biological and chemical attributes of soil with anthropic environments representative of the study area. These land use types include planted forests to agricultural land (characterized mainly by pastures and maize monocultures). Specifically, our objectives were as follows: (1) to contrast the structure and composition (diversity, abundance, and biomass) of the edaphic macroinvertebrate communities at order and genus levels, as well as by trophic groups, among land use types and (2) to compare their soil chemical properties. In addition, we aim (3) to assess how the soil chemical parameters could affect soil macroinvertebrate communities among land use types, by applying different multivariate analyses. We hypothesized that native forests would present more diverse and more even soil communities, and their soil chemical properties would indicate better soil fertility attributes than soils under anthropic environments.
Materials and methods
Study area
The study area includes an agricultural landscape located in the mountainous area of northern Ecuador, which is located in La Esperanza parish of Pedro Moncayo county (longitude −78.25716, latitude 0.08222), covering an area of 13 km2. This region is classified as pluvial and cold temperate according to the Ministry of Environment of Ecuador (Food Agriculture Organization, 2015). It has an annual mean temperature of 14°C (± 1.3 SD) (GAD Municipal Pedro Moncayo, 2015). Seasonal variation of temperature is reduced, while precipitation is bimodal, with two wet seasons from February to May and September to November with monthly average precipitation of 70 mm (± 20.1 SD). The dry season presents an average precipitation of 25 mm (± 14.3 SD) (Cáceres-Arteaga et al., 2018). The study sites exhibit an altitudinal range that varies between 3,000 and 3,600 m.a.s.l. and the landscape is dominated by managed ecosystems, where 33.13% is represented by agricultural land, 23.28% by pastures, 21.92% by planted forests with exotic species, and 21.67% by native forests (Supplementary Table 1) (GAD Municipal Pedro Moncayo, 2015; Ministerio del Ambiente del Ecuador, 2016). Agriculture activities in this region consist of small-scale low input production systems, with the poor management practices.
Sampling design
We used a variation of the methodology of Fertility and Tropical Soil Biology (Baillie et al., 1990; De Valença et al., 2017) to investigate the effect of land use on the diversity of soil invertebrates and the physicochemical parameters of soil quality among four land use systems: (1) native forest, (2) planted forest, (3) pasture, and (4) monoculture (Ministerio del Ambiente del Ecuador, 2013; GAD Municipal Pedro Moncayo, 2015) (Supplementary Table 1). The natural forest was used as a reference system.
The study region presents a spatially explicit pattern of soil types that includes Entisols, Mollisols, and Inceptisols (Ministerio de Agricultura y Ganadería, 2017). To control for this variable that could affect the results, the sampling design focused on the Inceptisol soil type only, which represents more than 60% of soils in the study area. This soil order is generally fertile, young, less weathered and varies in depth from 1 to 2 m. Inceptisols are considered high-activity clay soils (Veldkamp et al., 2020). The soil texture of the study sites corresponds to loam.
Ten sampling sites were randomly selected in each land use category, with a distance of at least 50 m from each other. Within each site, five monoliths (25 × 25 × 10 cm) were obtained with a separation of 10 meters along a 2 × 50 m transect. Prior to the excavation of the monolith, the leaf litter and the existing vegetation cover above the soil layer were discarded. To control the possible effects of elevation across land use types, we established replicates along the altitudinal range (Figure 1). However, due to the historical patterns of land use transition in our study area, we could not find replicates for native forest at lower elevation. We conducted two field sampling trips to cover the rainy season, one in November 2018 when we conducted 4 transects of each land use category, and the other sampling trip was carried out in February 2019, covering 6 transects of each land use category.
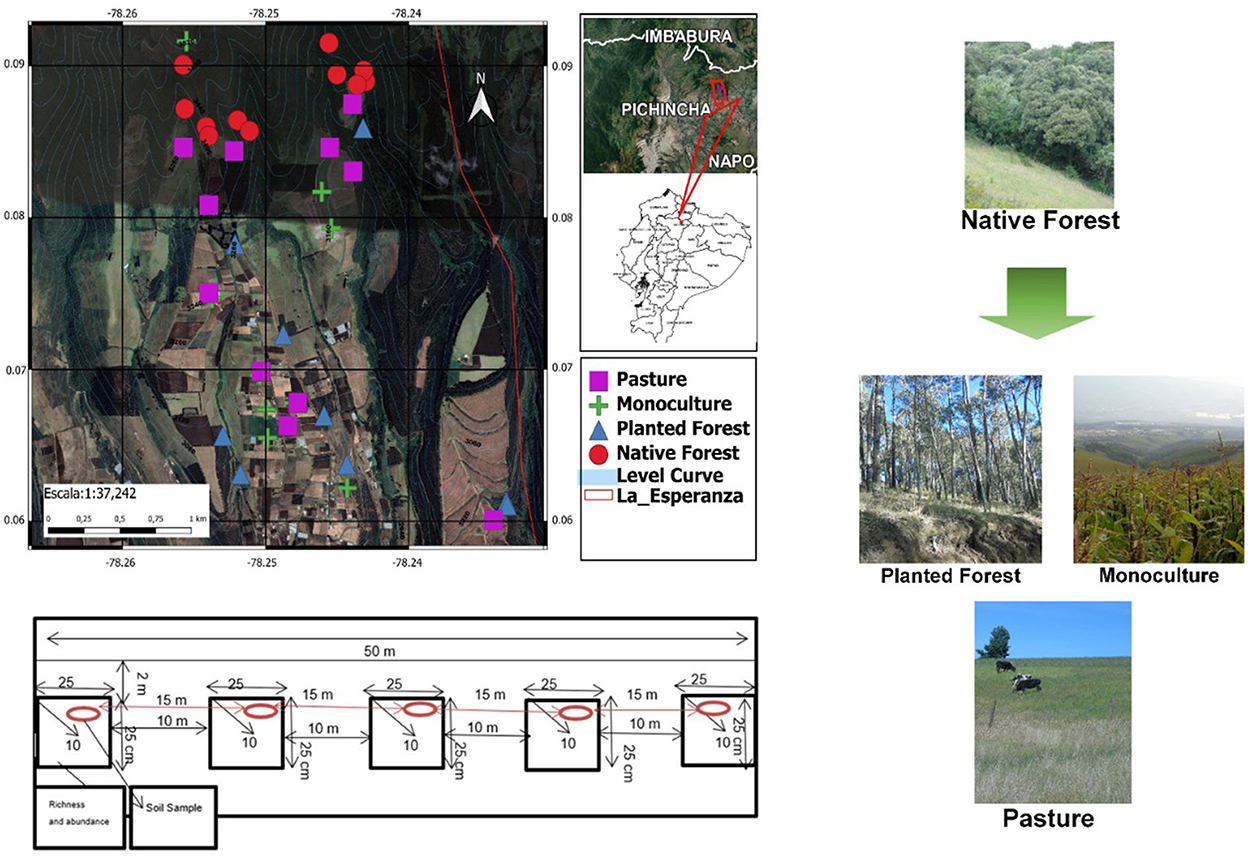
Figure 1. Study site location, representation of land use categories and diagram of the soil sampling scheme.
Diversity of soil macroinvertebrates
In each monolith (Figure 1), all visible organisms (>2 mm) of soil invertebrates were collected. Then, all the specimens were divided into arthropods and worms according to their type, weighed (balance accuracy 0.001 g), and transported to the laboratory for counting and identification. Then, the arthropod specimens were stored in a 50-ml plastic jar with 70% alcohol, while worms were preserved using 4% formalin. The collection of specimens is part of the research permit No. 007–2018-RC-AD-FLO-FAU-DPAP-MA, granted by the Environmental Authority of Ecuador.
The collected specimens were sorted and taxonomically identified up to the genus level, using the specialized keys (Merrit, 1996; Kitching, 2000; McGavin, 2000; Triplehorn et al., 2005; Leibensperger, 2016). The abundance and biomass of soil macrofauna were averaged among the 5 samples obtained along each transect and reported at the taxonomical level of order and genus, while the biodiversity measures were summed up among the subsamples within each transect (De Valença et al., 2017). In addition, each specimen was classified in its corresponding trophic groups (predators, herbivores, omnivores, detritivores, and parasites/hematophagous), as proposed by McGavin (2000), Cabrera (2014), and Soliveres et al. (2016) (Supplementary Tables 2, 3).
Soil chemical parameters
For the study of soil chemical parameters, surface soil samples (0–10 cm) were collected with a borehole every 10 meters along each transect. The soil sampling was carried out after removing the leaf litter and the surface vegetation layer. Then, the samples within the same transect were thoroughly mixed to obtain 40 composite samples, representing all the sites from the different land use types. From each composite sample, 500 cm3 was extracted, placed in plastic bags and taken to the Soils Laboratory of the Central University of Ecuador for further analysis. In the laboratory, the following chemical parameters were obtained: organic carbon, nutrients (Ca, P, K, N), and pH. The pH was measured with a potentiometer in aqueous solution, ratio 1:2.5. Organic carbon was obtained by Walkley–Black wet combustion, while %N was estimated by the Kjeldahl method and P by the colorimetrically-modified Olsen method with a photocolorimeter. Nutrients (K, Ca) were measured by atomic absorption spectrophotometry, using the PerkinElmer Analyst, and the extraction solution was sodium bicarbonate (NaHCO3) 0.5 N at a pH of 8.5 (Dominati et al., 2010; Lukina et al., 2011).
Statistical analysis
The estimated richness of edaphic macroinvertebrates at the genus level was measured with the nonparametric estimators Chao 1, Bootstrap, and Jacknife 1 (Jiménez-Valverde and Hortal, 2003; Villarreal et al., 2006) using EstimateS, version 9.1 (Colwell, 2013). According to Magurran (2004), the agreement of results obtained from different estimators that use conservative to flexible approaches demonstrates a robust sampling effort. Sampling completeness was considered as the percentage of observed relative to the estimated richness (Colwell and Coddington, 1994).
We used the Hill numbers approach, also known as true diversity, as a framework for a reliable estimation of alpha diversity of soil macrofaunal communities at the order and genus level (Chao et al., 2014). This approach provides generality and flexibility in controlling the effects of common and rare taxa in biodiversity quantification. We estimated the three orders of diversity within this approach: q = 0, q = 1, and q = 2, which are equivalent to species richness, Shannon diversity, and Simpson diversity, respectively (Jost, 2006; Moreno, 2011).
To test whether the alpha diversity measures and soil chemical properties differ between land use categories, we conducted analyses of variance after verifying the parametric assumptions using the Levene and Shapiro–Wilk tests. When the assumptions were not met, Kruskal–Wallis tests were carried out. If significant differences were detected in the analyses of variance, post hoc tests were conducted using Tukey's method. To test whether the abundance, biomass, and richness of the most represented trophic groups presented divergences among land use categories, we applied Kruskal—Wallis tests and the post hoc Wilcoxon rank sum test with continuity correction for nonparametric comparisons. All analyses of variance were conducted using JASP 0.16.3 software (JASP Team, 2020).
The structure of the edaphic macroinvertebrate communities was described by rank-abundance curves at the genus level, expressed in logarithm base 10 for each land use category and plotted with GraphPad Prism 8.0.1 (GraphPad Software, 1995). These plots highlight the differences in evenness among assemblages. Steep curves signify the assemblages with a higher dominance, while shallower slopes imply the higher evenness of the community (Whittaker, 1972; Whittaker et al., 2001). As reported by Magurran (2004), ordination analysis is a very simple and intuitive meaningful method of representing the differences among the samples and communities, which describes beta diversity in terms of compositional change. Therefore, ordination methods were conducted for the beta diversity comparison of the edaphic macrofauna communities across land use categories. First, abundance data were transformed using the “total” option in the decostand function within the R-vegan library, and then, the Bray–Curtis dissimilarity index was estimated (Villarreal et al., 2006). This index varies between 0 and 1, where 0 represents identical communities and 1 depicts communities that do not share any genus. The resulting dissimilarity matrix was used to perform the principal coordinates analysis (PCoA), which were plotted for sites and genera. Finally, to determine whether there were significant differences between the edaphic macrofaunal communities, multivariate analysis of variance with 999 permutations was carried out using the adonis function, with land use as the explanatory variable. Likewise, the soil chemical parameters were analyzed through the principal component analysis (PCA) to describe the general patterns of the soil environment across land uses (De Valença et al., 2017).
To understand whether the edaphic macroinvertebrate communities could be explained by the soil chemical parameters recorded across land uses, we applied canonical correspondence analysis (CCA) and redundancy analysis (RDA). For the CCA, the relative abundance of soil macroinvertebrate fauna, at genus level, was used as the biological matrix while the continuous parameters of soil quality accounted for the environmental matrix. For the RDA, the diversity metrics based on the Hill numbers (richness, Shannon, and Simpson) made up the biological matrix and, similar to the CCA, the explanatory environmental dataset corresponded to the chemical parameters of the soil.
For both the CCA and the RDA, the variables with the most significant influence on the edaphic macroinvertebrate community were chosen using a stepwise model based on the “ordistep” function. In addition, the variance inflation factors (VIFs) were estimated for each of the soil chemical variables, when showing VIF values <10 the noncollinearity in the matrix of environmental variables was demonstrated and maintained for the final analysis. The variables selected in this way were included in the final model to test whether the variation in the matrix of biological variables is explained more by the soil chemical variables than expected by chance. All multivariate analyses were performed with vegan and were plotted with ggplot packages in R (Hammer et al., 2001; Oksanen et al., 2019).
Results
Community composition of soil macroinvertebrates by land use categories
In total, 1,776 individuals of edaphic macrofauna were obtained, representing 50 genera, 42 families, and 21 orders (Table 1). From these, the most represented orders were Coleoptera (beetles, 31%), followed by Haplotaxida (earthworms, 29%), and Diplura (two-pronged bristletails, 10%). Other less abundant groups included the following: Isopoda (woodlice), Aranae (spiders), Lithobiomorpha (stone centipedes), Diptera (flies), and Scolopendromorpha (centipedes), which represented <10% of the total abundance of soil macrofauna registered (Table 1).
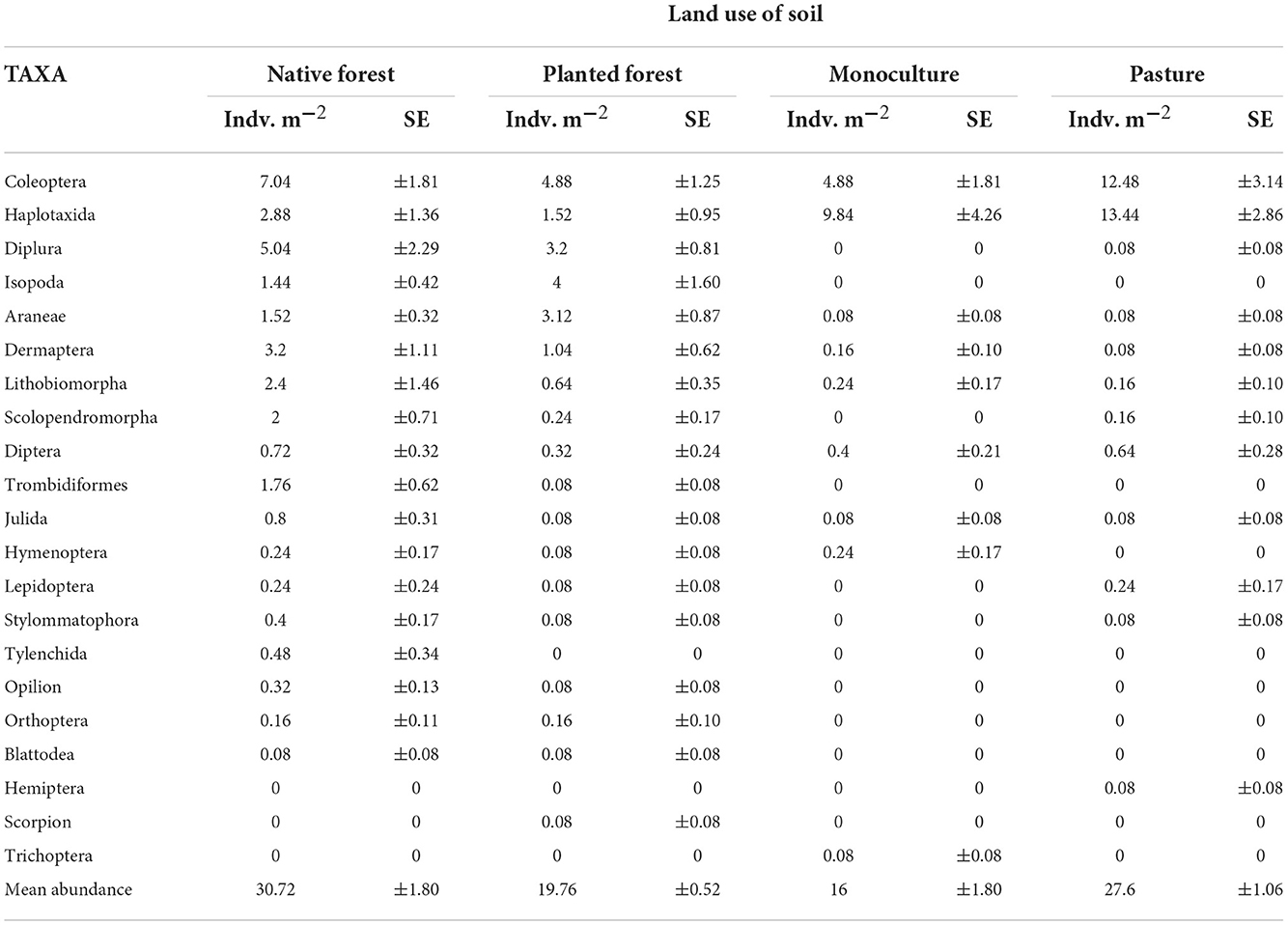
Table 1. Abundance (mean ± standard error) of soil macroinvertebrates at order level across different land use categories.
Compared to the reference system, diversity of soil macroinvertebrate communities at the level of order was notably reduced in pastures and monocultures (Table 1). From the 18 different orders found in native forests, only 8 were registered in monocultures and 12 in pastures. Planted forest shared the most orders observed in the reference system (Table 1).
Regarding abundance, the majority of the more-represented orders of soil macroinvertebrates exhibited higher values in the soils from native forests, ranging from 2 to 40 times higher than the abundance found in the anthropic environments (Table 1). Some noticeable exceptions were the abundance of Haplotaxida (earthworms) and Coleoptera (beetles), which were two to three times higher in pastures (13.44 ind/m2) and monocultures (9.84 ind/m2) than in the other land use categories (Table 1).
According to the shape of the rank-abundance curves, the communities at the level of genus found in native and planted forests were richer and presented shallower slopes and then were more even than the pasture and monoculture communities. Soil macrofaunal communities in pastures and monocultures were dominated by few taxa (Figure 2). Another notable difference between the communities at this taxonomic level was their composition; only 8 genera were shared between all land use types. In pastures and monocultures, the most dominant genera were Eisenia sp. Malm, 1877, Lumbricus sp. Linnaeus, 1758, Naupactus sp. Dejean, 1821, and Heterogomphus sp. Guérin-Méneville, 1851, which are classified under the orders of Coleoptera and Haplotaxida, described above. In native forests, the most represented genera were Holojapyx sp. Silvestri, 1910, Forficula sp., and Naupactus sp.; while in the planted forests, Armadillidium sp. Latreille, 1804, Holojapyx sp., and Fufus sp. dominated the soil macrofauna community (Figure 2).
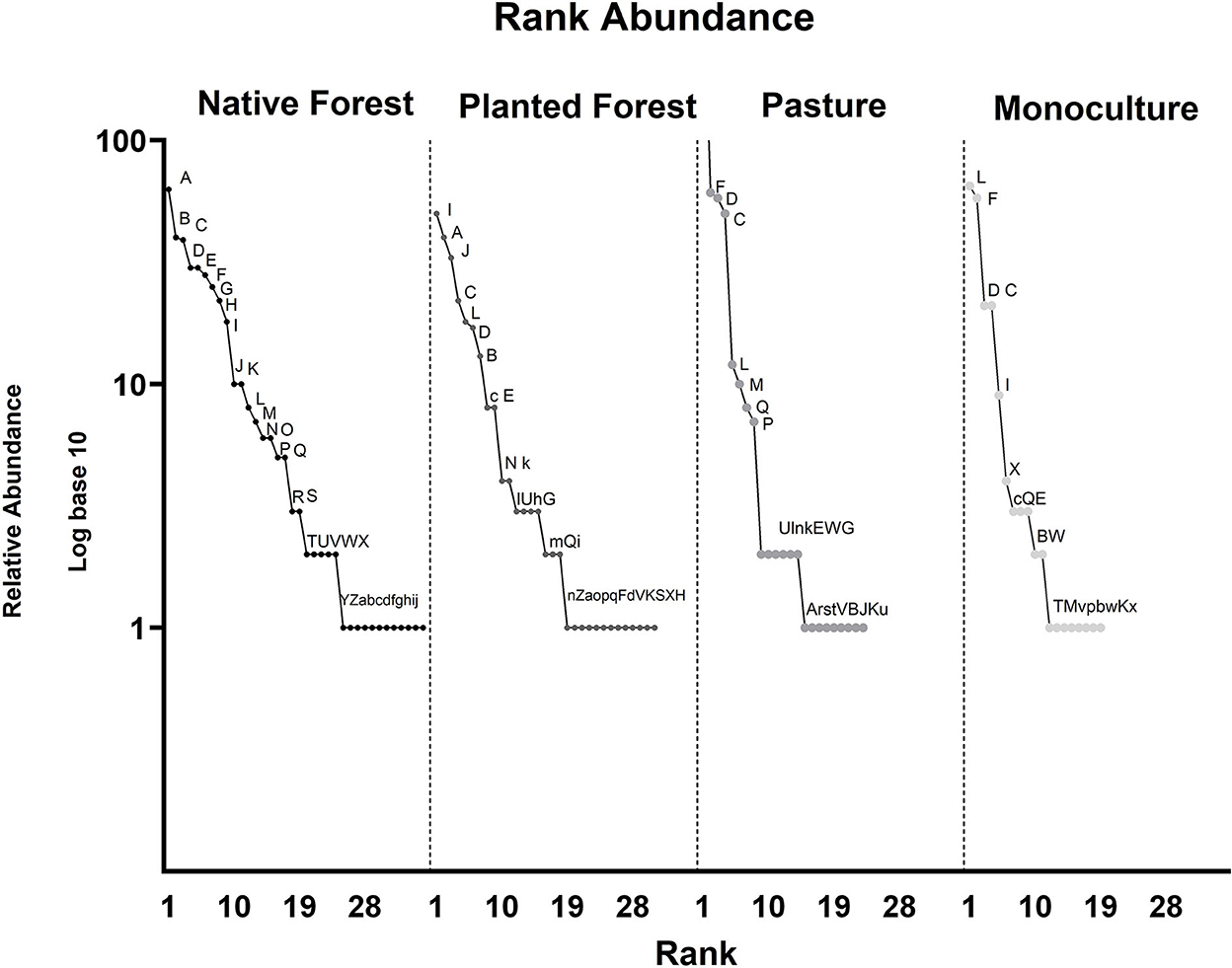
Figure 2. Rank-abundance curve in logarithm10 of edaphic macrofauna at genus level across land use categories. (A) Holojapyx sp., (B) Forficula sp., (C) Naupactus sp., (D) Heterogomphus sp., (E) Lithobius sp., (F) Eisenia sp., (G) Scolopendra sp., (H) Trombicula sp., (I) Armadillidium sp., (J) Fufius sp., (K) Julus sp., (L) Lumbricus sp., (M) Aspidolea sp., (N) Cheiracanthium sp., (O) Meloidogyne sp., (P) Arachnocampa sp., (Q) Eleodes sp., (R) Naesiotus sp., (S) Phalangium sp., (T) Aphididae sp., (U) Cyrtotrachelus sp., (V) Euconulus sp., (W) Passalus sp., (X) Stomoxys sp., (Y) Tegenaria sp., (Z) Allonemobius sp., latt asp sp., (b) Ctenus sp., (c) Dermestes sp., (d) Ensifera sp., (e) Gasterophilus sp., (f) Gonipterus sp., (g) Leiobunum sp., (h) Loxosceles sp., (i) Sitophilus sp., (j) Synoeca sp., (k) Enicmus sp., (l) Agriotes sp., (m) Calliphora sp., (n) Agrotis sp., (o) Chactas sp., (p) Cimex sp., (q) Drosophila sp., (r) Aphrastus sp., (s) Centrotus sp., (t) Cydia sp., (u) Melyris sp., (v) Cecidomyia sp., (w) Hydropsyche sp., and (x) Trypoxylon sp.
Diversity of soil macroinvertebrates across land use categories
The nonparametric richness estimators at genus level (Chao 1, Jacknife, and Bootstrap) used in the present study revealed a high degree of completeness of the sampling effort for all land use categories (Supplementary Figure 1). Although the asymptote was not reached, the level of completeness exceeded 67%, being the sampling in the native forest (more than 76% for all nonparametric estimators) the one that obtained the best results to estimate richness at genus level.
Edaphic diversity metrics at genus level (richness, Shannon, and Simpson) showed statistical differences across land use categories (Figure 3). Mean richness ranged from 8 (± 5 SD) in monocultures to 22 (± 6 SD) genera on average in native forests. The highest richness values were observed in native forests, followed by the planted forests, which were statistically different from the other categories [ANOVA F(3, 36) = 9.047, p = 0.001; Figure 3]. The same statistical pattern was observed for the Hill numbers: Shannon (q = 1) [Kruskal–Wallis H(3) = 20.671, p < 0.001] and Simpson (q = 2) [Kruskal–Wallis H(3) = 20.672, p < 0.0] 01; (Figure 3).
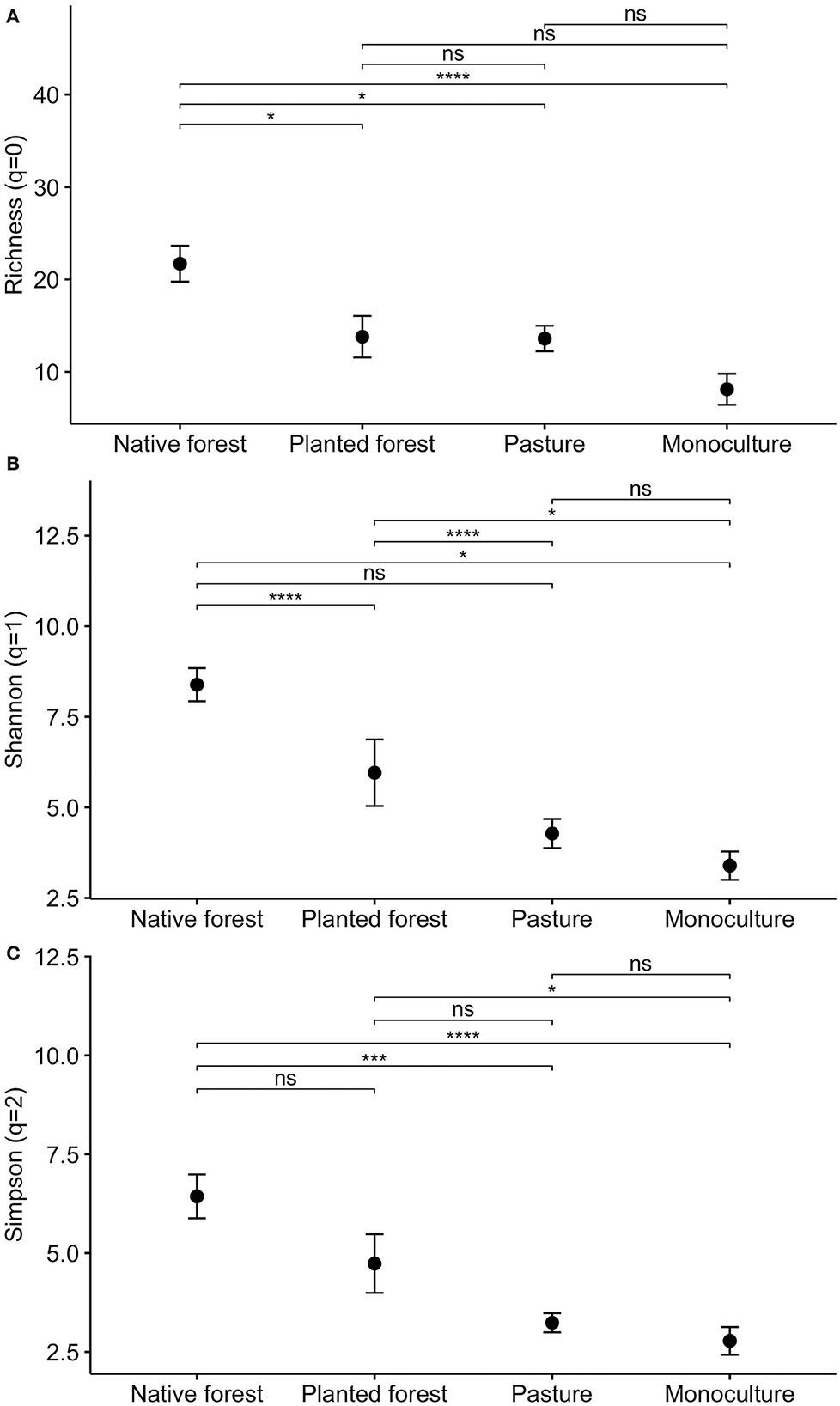
Figure 3. Average of Hill numbers: richness (A), Shannon (B), and Simpson (C) of soil macroinvertebrates at genus level across the land use categories. The length of the error bars is a 95% confidence interval for the mean. Asterisks illustrate the power of the levels of significance of the statistical tests: *p ≤ 0.05; **p ≤ 0.01; ***p ≤ 0.001; ****p ≤ 0.0001 and ns represents p > 0.05.
The macrofauna assemblages (beta diversity) differed significantly across the land use categories (PERMANOVA, pseudo-F = 5.0721, p = 0.001; Figure 4), demonstrating a clear pattern between forested and non-forested sites. This pattern was illustrated by the 2-dimensional ordination plot (PCoA; Figure 4).
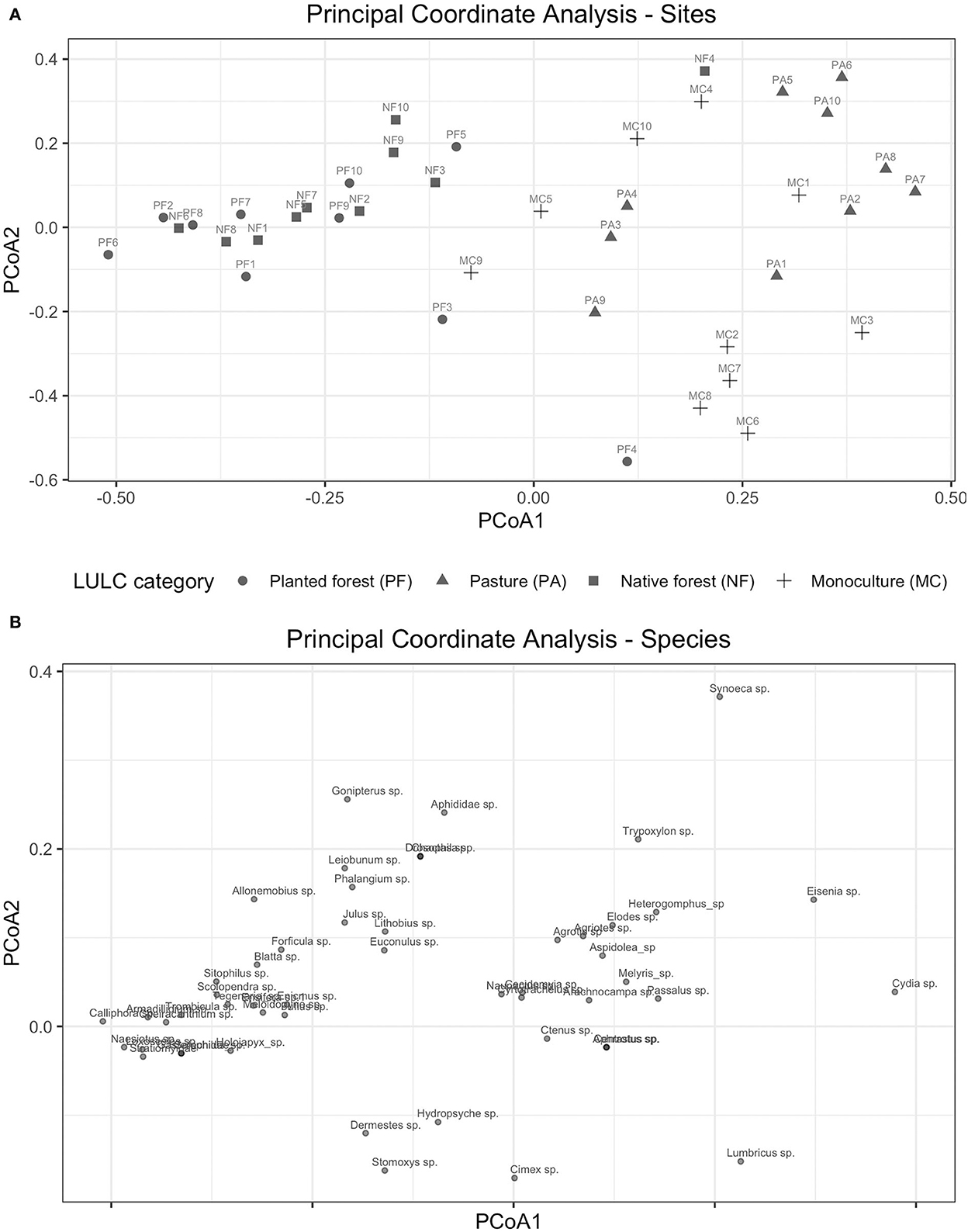
Figure 4. Beta diversity analysis of soil macroinvertebrates at genus level by land use category. (A) Principal coordinate analysis (PCoA) representing sites. (B) Principal coordinate analysis (PCoA) representing Bray–Curtis dissimilarity matrix at genus level.
The community composition patterns observed in the rank-abundance curves between land use categories were similar to the results obtained in the PCoA (Figures 4A,B). For instance, native and planted forest assemblages of soil macrofauna were represented by a large number of taxonomic groups, which were observed closer together in the PCoA ordination space. Monoculture and pasture sites were better characterized by less taxonomic groups, highly distinctive by the abundance of earthworms (Eisenia sp. and Lumbricus sp.) (Figures 4A,B). The first axis of the PCoA depicts the variability in soil fauna assemblages across land use categories (Figures 4A,B). Native and planted forests are clustered together in one side of the First PC, whereas monocultures and pastures are grouped on the other side of this axis.
Trophic group patterns of soil macroinvertebrates
Detritivores, predators, and herbivores were the most represented trophic groups in the edaphic macrofaunal communities (Figure 5A). However, there were differences in relative abundance, biomass, and richness between the land use categories. In general, relative abundance and richness by trophic group presented similar patterns between native and planted forests (Figures 5A,C). However, the most abundant trophic group in native forests were the predators, exhibiting significantly higher values than pastures and monocultures [H(3) = 26.218, p < 0.01; Figure 5A]. On the other hand, the edaphic macrofaunal communities in pastures and monocultures were similar to each other and dominated by detritivores (Figure 5A), but for this trophic group, the mean abundance in pastures was significantly different from native and planted forests [H(3) = 9.740, p < 0.05; Figure 5A]. Moreover, herbivore abundance did not show significant differences among the land uses.
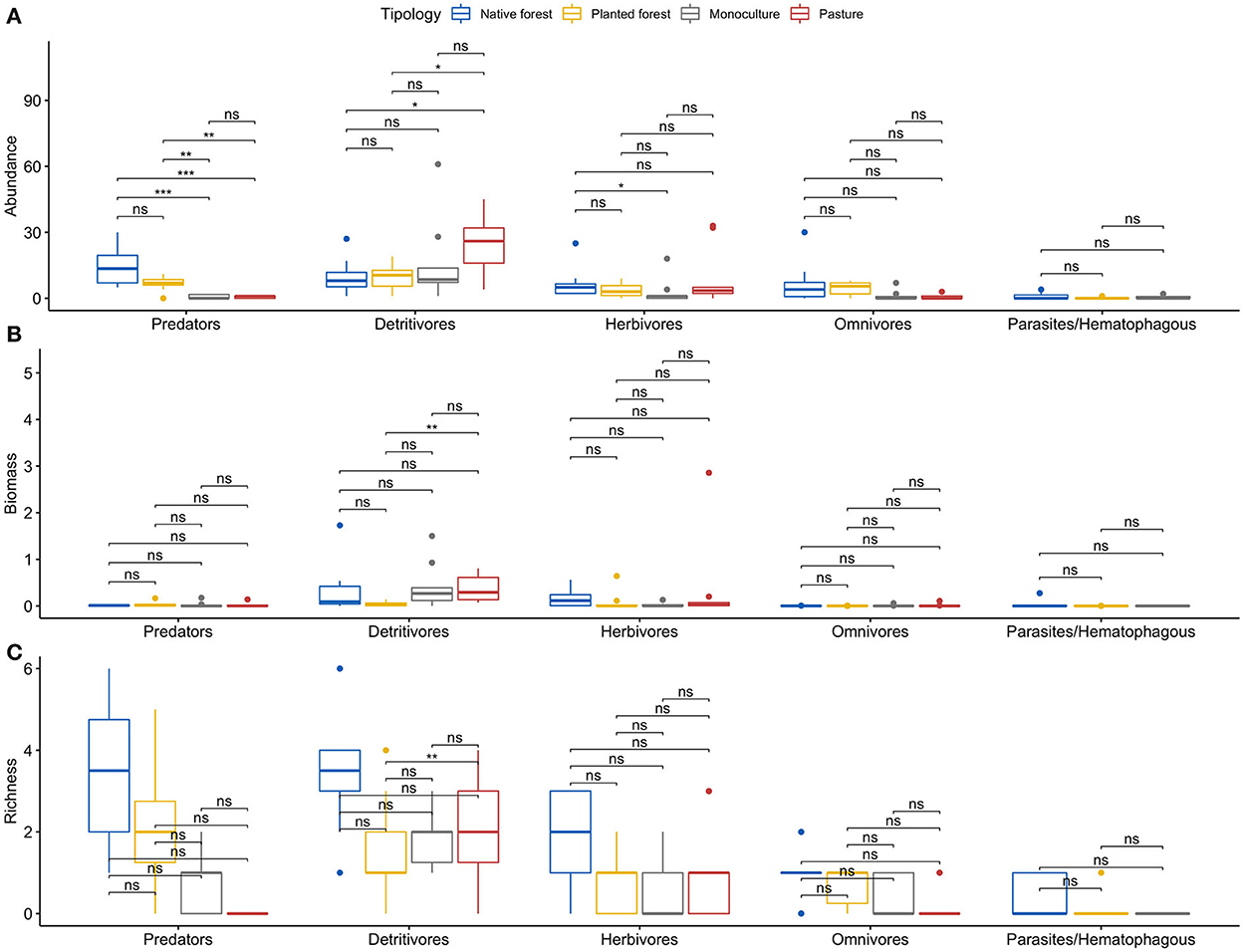
Figure 5. Multiple boxplots for (A) abundance, (B) biomass, and (C) richness for trophic groups of soil macroinvertebrates by land use category. Asterisks illustrate the power of the levels of significance of the statistical tests: *p ≤ 0.05; **p ≤ 0.01; ***p ≤ 0.001 and ns represents p > 0.05.
Different patterns from those described for relative abundance were observed when analyzing the biomass of trophic groups (Figure 5B), showing a marked dominance by detritivores, followed by an important component of herbivores in all systems, particularly in monocultures and pastures (Figure 5B). However, only detritivores in planted forests showed significantly lower values than the other land uses [H(3) = 12.068, p < 0.01] (Figure 5B).
Finally, richness exhibited different results across the land use categories (Figure 5C). A significantly greater number of genera within the predators [H(3) = 26.218, p < 0.001] was found in native and planted forests. Detritivore richness was significantly lower in planted forests and monocultures than in native forests [H(3) = 10.546, p < 0.05; Figure 5C].
Soil chemical parameters
Soil chemical properties showed significant differences across the land use categories. In general, organic carbon, pH, and the content of nutrients (N, Ca, and K) were significantly higher in the native forest compared to anthropic environments, although the average values of pH and P did not show the differences between pastures and native forests (Table 2). In contrast, P was the only parameter that was significantly higher in monocultures. All the soil chemical parameters studied were similar in planted forests and monocultures (Table 2).
Principal component analysis of the soil chemical properties showed clear groupings based on the land use categories (Figure 6). Most of the variation explained by the soil chemical parameters was represented in the first principal component axis (PC1, 58.58%), and a clear pattern of clustering of sites within each land use category was observed on this axis. Native forests clustered in the right side of the biplot, where high values of C, N, and Ca were also found. Pastures were represented in the central region of the plot. In contrast, monocultures and planted forests depicted a grouping pattern in the left side of the biplot, coinciding with the strikingly high values of P in their soils. The biplot of the PCoA demonstrated a positive correlation between organic carbon, Ca, and N, while a strong negative correlation was observed among these variables with P. These association patterns of soil structure variables were clearly related to PC1. Complementarily, an important part of the variability of the multivariate dataset was explained by PC2 (16.88%), which captured the variation of pH, C:N ratio, and micronutrients (especially K) (Figure 6) among sites.
Relationship between the soil macroinvertebrate community and soil fertility
The canonical correspondence analysis demonstrated that the inertia that was successfully constrained by the explanatory variables was 28%. From this percentage, constrained inertia associated with CCA1 was 53% and CCA2 was 28%. Both axes significantly explained (ANOVA p < 0.002) the variation in relative abundance of the soil macroinvertebrates at genus level. Therefore, there is a good representation of the relationship between biological variables and soil chemical parameters. According to the results of the ANOVA of the CCA, the main soil chemical parameters that influenced the structure of the edaphic macroinvertebrate community were organic carbon (p < 0.001), followed by pH, and K (p < 0.01). In addition, this analysis also identified that C:N and P significantly (p < 0.05) explained some of the variations in the matrix of the relative abundance of soil macrofauna analyzed (Figure 7, Supplementary Table 4).
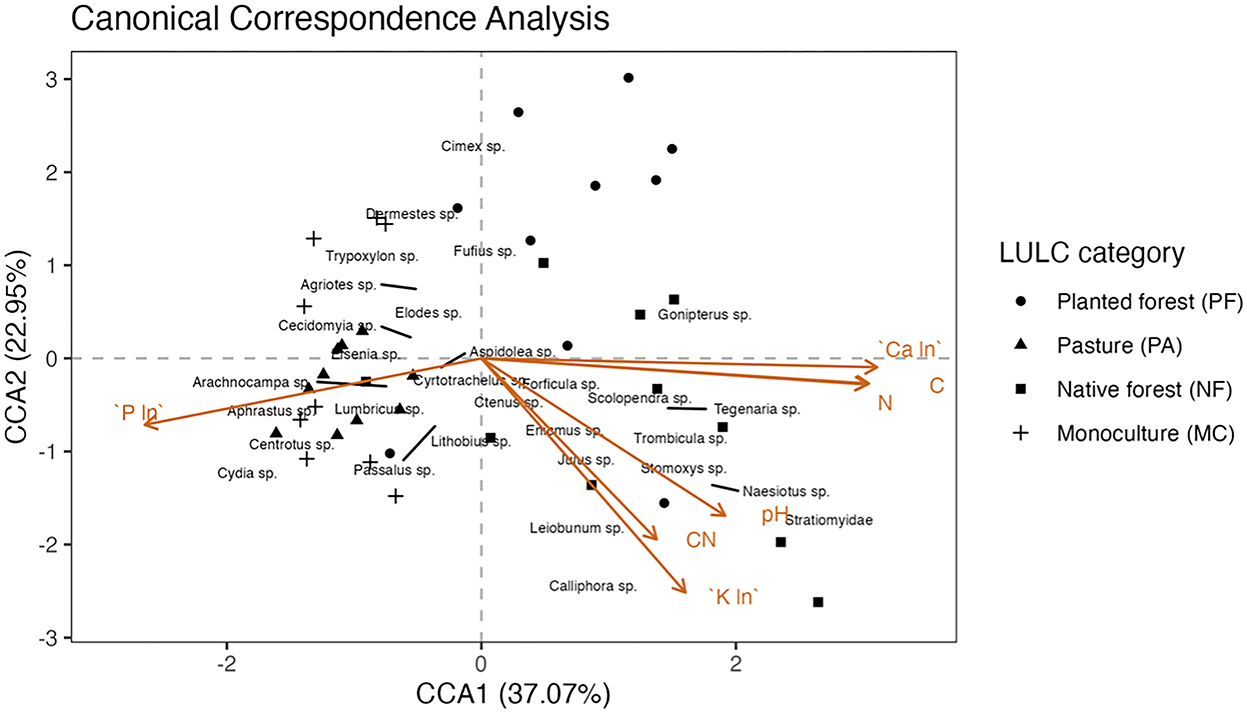
Figure 7. Canonical correspondence analysis of the soil chemical parameters and the relative abundance of the edaphic macroinvertebrate community. Significant parameters: ***Org carbon, **pH, K(ln), *C:N, P(ln). Asterisks illustrate the power of the levels of significance of the statistical test: *p ≤ 0.05; **p ≤ 0.01; ***p ≤ 0.001.
The redundancy analysis demonstrated that soil chemical parameters significantly explained 27% (ANOVA p < 0.05) of the variation in diversity metrics of the soil macrofauna community. The inertia associated with RDA1 was 25.606 and RDA2 was 1.313. The main chemical parameters that influenced the diversity of the edaphic macroinvertebrate community were organic carbon (p < 0.01) and pH (p < 0.05) (Figure 8, Supplementary Table 5).
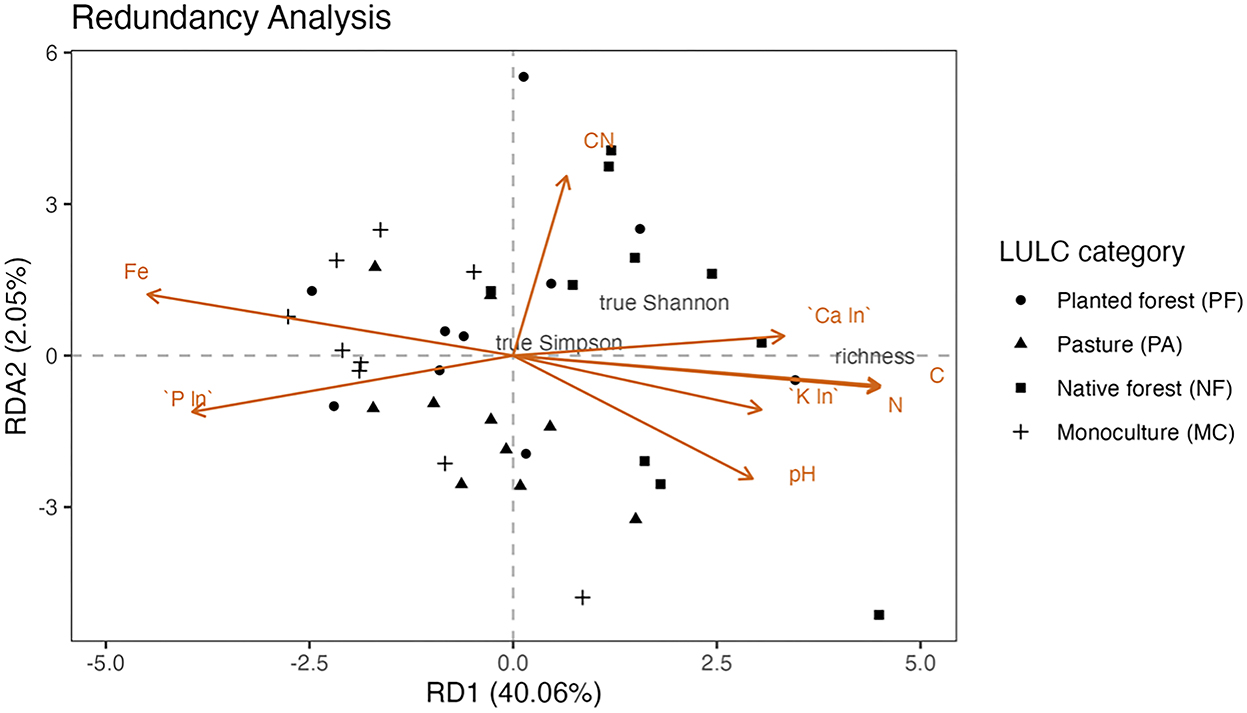
Figure 8. Redundancy analysis of the soil chemical parameters and diversity of edaphic macroinvertebrate community. Significant parameters: **Org carbon, *pH. Asterisks illustrate the power of the levels of significance of the statistical test: *p ≤ 0.05; **p ≤ 0.01.
Discussion
Our findings suggest that the conversion of native forests to anthropic environments, especially to agricultural land (pastures or crops), causes biodiversity loss and degrades soil fertility. These results are in line with other studies conducted in tropical mountain systems (Delelegn et al., 2017; De Valença et al., 2017; Zhang et al., 2017; Perez et al.1). Biodiversity loss was evident when structure and community composition of soil macrofauna were compared among the land use categories. As we expected, native forests showed the significantly higher values of alpha diversity metrics than pastures and monocultures. However, our reference system presented soil macrovinvertebrate assemblages as diverse as those found in planted forests, a result that was not expected. Moreover, our study demonstrated taxonomic diversity depletion at genus and order levels as a result of native forest conversion to agricultural land. The length of the abundance range curves of soil macroinvertebrates registered in native and planted forests almost doubled those found in pastures and monocultures. Strikingly, from the 18 distinct orders of soil macroinvertebrates found in native forests, only half were found in pastures and even less in monocultures. Additionally, our findings from the PCoA showed the differences in soil community composition across the land use categories. Native and planted forests depicted similar communities, which differed from those found in pastures and monocultures, a result also reflected in the permutation analysis of variance using distance matrix, where the aforementioned finding was statistically verified.
Differences in the structure and community composition of soil macrofauna among the land uses demonstrated in our study would likely be related to the degree of human disturbance in managed ecosystems (Lukina et al., 2011; Sylvain and Wall, 2011). A change in vegetation structure, such as the total or partial removal of its biomass due to land use conversion, can produce significant disturbances in the physical, chemical, and biological properties of soil (Ruiz et al., 2010; De Valença et al., 2017; Solórzano Flores, 2020). In turn, changes in the soil ecology could alter habitat conditions and resource availability for different groups of soil dwellers, who are sensitive to the changes in their environment (Rousseau et al., 2013). For example, the similarities observed between native and planted forests' soil fauna could be explained by the low level of physical disturbance in these land use types. Generally in the highlands of northern Ecuador, exotic species (Eucalyptus sp. and Pinus sp.) were planted mainly to restore deforested and/or degraded landscapes—and to a lesser extent for commercial purposes (Farley, 2007). Therefore, after their establishment, vegetation cover and the associated litter production do not substantially change in planted forests, a condition that is shared with native forests. Studies have shown that vegetation cover and litter production are the main factors that control the composition and distribution of soil fauna (Guangbin and Xiaodong, 2007; Zhou et al., 2022). In forested soils, these factors may have provided habitats to evade natural enemies and more resources to thrive (Shrewsbury and Raupp, 2006; Guangbin and Xiaodong, 2007).
Moreover, in this study, the less-disturbed systems—which correspond to native and planted forests exhibited high overall taxonomic diversity in addition to richness of predators, represented by centipedes (Lithobiomorpha, Scolopendromorph) and spiders (Aranea). Previous work in tropical systems (Halffter and Arellano, 2002; Cabrera and López, 2018) has demonstrated that soils protected from surface disturbance, such as forest and fallows, favor the development of taxonomically and functionally diverse macroinvertebrate communities (De Valença et al., 2017). These forested ecosystems are characterized by low soil disturbance, representative root biomass and soil plant cover, in addition to abundant leaf litter—providing complex topsoils where balanced food webs and abundant predators can thrive (Manhães et al., 2013; De Valença et al., 2017).
Our results from pastures and monocultures demonstrate a simplification pattern in soil community composition. High abundance and biomass of one trophic group (detritivores), mainly represented by some beetles (Coleoptera) and earthworms (Haplotaxida), depict soils under agricultural land in our study region. Intensively managed agricultural land, characterized by tillage, the application of pesticides and fertilizers, soil compaction, and the harvest of plant biomass have caused simplification of edaphic communities (Menta, 2012; Thiele-Bruhn et al., 2012). In these disturbed systems, species capable of withstanding stress predominate and rare taxa decrease or disappear (Brown et al., 2001; Decaëns et al., 2006). This result could be explained by the ability of some soil species (especially earthworms) to tolerate the disturbance associated with land preparation, planting, and even contamination caused by pesticides (Halffter and Arellano, 2002; Araneda, 2016; De Valença et al., 2017; Eisenhauer et al., 2017). Another complementary explanation of the high representation of detritivores in agricultural land could be related to higher inputs of manure and higher biomass of plant roots available to decomposers of soil organic matter (Ernst and Emmerling, 2009).
Another interesting result, related to the biological attributes of soils, was the lack of ants or termites in all topsoils studied across the land use categories in the highlands of northern Ecuador. These arthropods are the most commonly cited examples of soil engineers across all biomes (Lavelle et al., 2014). This result could be related to altitudinal limitations of these groups in our study sites (Brussaard et al., 2013), although this cannot yet be proven by our data.
Our findings related to soil chemical parameters of the topsoil layer (0–10 cm) demonstrated a strong degradation of soil fertility as a result of native forest conversion to anthropic environments: a result consistent with the findings from Lemenih et al. (2005). As expected, we found significantly higher values in many chemical properties that characterized healthy soils (total C, total N, and nutrient availability) in native forests compared to anthropic environments. Only phosphorus was found in less quantity in native forests, while in monocultures and planted forests, this nutrient had a significant greater value. This result coincides with the study of Escobar et al. (2017) that states that finding P in high quantities is expected in the soil of monoculture systems. Agricultural systems with a large amount of phosphorus added by mineral fertilization tend to accumulate this nutrient as a function of the time that the soil has been used for cultivation (Delelegn et al., 2017). Furthermore, the lower values of soil chemical parameters observed under the agricultural sites would likely be explained by a lack of soil cover, high levels of tillage disturbance, an accelerated rate of soil organic matter decomposition, and increased erosion (Delelegn et al., 2017).
In addition, we found that pH was highest in native forest soils. This result is in line with the studies by Urrego (1996) and Veldkamp et al. (2020), where topsoils under natural forests exhibited a higher pH than other land use categories. Moreover, our findings are consistent with the study conducted by Heitkamp et al. (2014) in the Central Andes. They demonstrated that the decrease in vegetation cover, as a result of forest conversion to rangeland systems, enhanced soil weathering and leaching rates, which in turn cause soil acidification. Distinct natural and anthropic processes lead to decreased soil pH (Goulding, 2016). However, in the agricultural land of our study region, a combination of these factors may explain the observed lower pH values. The opening of the system (more water percolating in the soil profile), disrupted biological cycles (lower return of Ca in the topsoil through litterfall), and nitrogen fertilization, containing acidifying products, might be the major driving factors for the acidification pattern detected in monocultures (Hao et al., 2020).
Our results highlighted a wide variation in the fertility parameters of pasture sites. Half of them had patterns similar to those observed in native forests, and although the rest of the sites resembled the conditions present in monocultures, the mean values showed significant differences for some soil chemical properties evaluated (total C, total N, and soil organic matter) compared to monocultures. These differences could be attributed to the history of land use, where soils recently converted to pastureland would present better conditions of soil fertility. Other contributing factors would be related to the differences in grass root biomass, levels of disturbance by tillage, and the addition of nutrients from organic wastes of livestock in the system. Mann et al. (2019) demonstrated that grass and mixed perennial-annual cropped fields presented higher levels in physicochemical and biological parameters of soil quality than more intensely managed fields, such as undiversified grain and vegetable crops.
We did not observe differences in soil chemical properties between plantations with exotic tree species (such as Eucalyptus spp.) and the degraded monoculture sites. These two land uses presented significant lower values in most of the chemical soil properties (organic carbon, N, K, Ca, and pH) than native forest, suggesting a strong degradation on soil fertility. Regarding the effect of native forest conversion to plantations with exotic tree species (such as Eucalyptus spp.) on soil properties, different investigations present contrasting findings. Some studies suggest a positive impact in degraded and treeless lands by increasing litter input on the soil surface (Yitaferu et al., 2013). On the other hand, many other studies demonstrated the high demand for soil nutrients of Eucalyptus plantations as a result of the combined effect of fast growth and the inability to fix nitrogen (Zegeye et al., 2011), causing detrimental effects on soil properties (Liang et al., 2016; Coca-Salazar et al., 2021). Our results are consistent with the latter findings. This would imply that reforestation efforts using exotic plants do not contribute to improving soil fertility (Liang et al., 2016; Veldkamp et al., 2020).
The divergent results of topsoil macrofauna diversity and soil chemical properties among land uses are remarkable. However, the effect of land use change on the soil biota and the soil chemical properties showed some differences. Although native forests stood out for presenting a greater diversity of soil biota and significantly higher values for chemical quality variables than the other land use typologies studied, the edaphic communities in the reference sites presented similarities with those found in planted forests. It appears that taxonomic diversity and composition in the soils studied are determined by a combination of factors, where acidification, soil nutrients, vegetation cover, and litter production seem to be relevant. Although, as vegetation cover and litter production were not measured directly, it is necessary to incorporate these variables to better understand the driving factors that influence the dynamics of edaphic communities in response to the altered environments of Andean landscapes.
Our results from the CCA and the RDA showed that a significant amount of variation in the community composition and diversity of soil macroinvertebrates was explained by soil chemical parameters. These results demonstrated a connection between biological attributes and chemical properties of topsoils, which likely affect soil health (De Valença et al., 2017). On one side, the CCA showed that relative abundances of different genera of soil macrofauna were significantly affected by pH, organic matter, C:N, and phosphorus. We observed distinct soil assemblages associated with high levels of pH and organic matter, attributing that the characterized native forests. In contrast, high levels of phosphorus were associated with communities characterized by earthworms mostly found in monocultures and pastures, which was in line with the findings of other Andean systems (De Valença et al., 2017). Furthermore, RDA demonstrated that all diversity metrics (richness, Shannon, and Simpson) of soil macrofauna were significantly explained by pH and C. The first axis of both multivariate analyses demonstrated a clear distinction between forested and agricultural sites and represented pH and nutrient variations, whereas the second axis was mostly defined by C:N, which was not explained by land use, suggesting a variation present in all land use categories.
Our findings are consistent with many studies that have reported that soil abiotic conditions interact with soil biodiversity (Lauber et al., 2008; Bardgett and Van Der Putten, 2014; Tibbett et al., 2020). In this study, the orders Lithobiomorpha and Scolopendromorpha, which belong to the class Chilopoda, have higher mean abundances in the native forest than in the other typologies. This typology in turn has high values of organic matter, organic C and N. Therefore, it can be inferred that the presence of chilopods demonstrates the good quality of the soil in the native forest. In contrast, we found a higher abundance and biomass of earthworms associated with more acidic soils (pastures and monocultures), and this result differs from the well-documented reduction of earthworm abundance with a decrease in soil pH (Urrego, 1996; Veldkamp et al., 2020). However, this contrasting result, especially in pastures, could be explained by the species composition among earthworms. Generally, topsoil communities are dominated by epigeic earthworms, who feed on plant litter and are less affected by pH (Duddigan et al., 2021). Since the taxonomic resolution of our research was at the genus level, further identification at the species level could better elucidate this issue. Moreover, we found that high values of phosphorus concentration on soils (characteristic of agricultural land) were negatively correlated with pH. According to Le Bayon and Milleret (2009), earthworm abundance and composition could directly or indirectly influence C, N, and P dynamics. Earthworms are keystone soil organisms in regulating nutrient cycling by their feeding and burrowing activities, as well as by their metabolism and metabolic wastes. But these possible interactions need further study. The higher soil fertile conditions in pastures may be mediated by the presence of earthworms.
Likewise, land use change could affect soil chemical and biological interactions in different ways, leading to cascading effects on other elements of the system. For instance, belowground communities are tightly linked to aboveground communities through multiple trophic interactions, at different scales and across the whole range of ecological processes that ultimately govern ecosystem functioning (Eisenhauer et al., 2017). Veldkamp et al. (2020) suggest that deforestation leads to drastic changes in inputs of litter organic residues, which may modify the soil microclimate, its biological activity, and decomposition rates. Differences in microclimates between native forests and other land uses were recorded by Guamán Trávez and Guarderas (In press), which may affect ecological processes taking place in topsoils (Gehlhausen et al., 2000; Montejo-Kovacevich et al., 2022). However, further integrated approaches that incorporate climate variations and other environmental parameters are needed to understand the cascading relationship among biodiversity, ecological functions, and the provision of soil ecosystem services (Leibensperger, 2016). Notwithstanding, we argue that shifts in soil community structure and biodiversity loss, generated by anthropogenic land use, may be mostly related to the changes of habitat structure (e.g., tree density, canopy cover), microclimatic conditions (e.g., temperature, humidity, light intensity, and incidence), and the disruption of ecological interactions among species (Gehlhausen et al., 2000; Košulič et al., 2016).
In our study, we controlled for the soil taxonomy (Inceptisols) and soil texture (mostly loams); therefore, we envisioned that our findings would mostly uncover the effects of the land use changes on soil quality parameters. However, the proportion of variance that is not explained by our study could be attributed to the differences in land use history and variability of other topographic variables such as slope or aspect across our study sites (Lavelle et al., 2014). Although our sampling design incorporated altitudinal variation throughout the study area, replicates of native forests were not found at lower elevations due to the land use history patterns. However, the similarities between native forest and planted forest, especially in the diversity and composition of soil macrofaunal communities, suggest that an altitudinal range of hundreds of meters is not a determining factor in high mountain areas above 3,000 m.a.s.l.
Our findings, consistent with other studies (Lavelle et al., 2014), also propose the irreplaceable value of native forests as biodiversity reservoirs. This study contributes to expanding the soil biodiversity knowledge in mountain landscapes of Ecuador, especially in remnant forests where few studies have been undertaken. However, our analysis focused on the taxonomic diversity of soil macrofauna at order and genus levels; therefore, more effort is required to elucidate the belowground species biodiversity, which has not yet been fully described (Eisenhauer et al., 2018; Phillips et al., 2019).
Landscape management implications
The findings of this research could contribute to the conservation and sustainable management of mountain agricultural landscapes in the study region. This should involve the management for restoring ecological processes and ecosystem services at the plot and at the landscape scale, by integrating plants and soil biota characteristic of native forests, as well as diminishing soil disturbance practices in agricultural systems (The Intergovernmental Science-Policy Platform on Biodiversity and Ecosystem Services, 2018).
For instance, there are clear indications that managing agroecosystem diversity within farms by higher amendments, promoting soil organic matter and beneficial soil fauna, assuring the maintenance of crop productivity and support for vital ecosystem services will improve the soil quality (Letourneau et al., 2011). However, an important guiding principle of landscape restoration implies the definition of conservation and ecosystem service' outcomes (Reed et al., 2013). For example, our results demonstrated that planted forests appear to support similar assemblages of soil macrofauna as native forests, but limited soil fertility. Thus, a restoration initiative framed in the soil health framework would imply improving both biodiversity conservation and soil fertility in degraded agricultural land. Therefore, it should emphasize the use of native plants and soil fauna occurring in the region landscape.
Another complementary approach involves spatial arrangement and connectivity at the landscape scale (Lavelle et al., 2014; Landis, 2016). Studies have suggested that the ecological quality of a homogenous matrix in an agricultural landscape could be highly enhanced by the addition of seminatural elements (Lavelle et al., 2014). For instance, setting aside relatively undisturbed natural systems such as native forests and connecting them with agricultural land through corridors may provide the sources of soil biodiversity, which could recolonize depleted soils under agricultural management (e.g., soil vegetation and macrofauna) (Nieminen et al., 2011). In addition, the spatial arrangement of pastures, which demonstrated better soil quality, alongside monoculture plots can support the recovery of soil macrofauna populations.
As described by Lehmann et al. (2020), the terminology, concept, and operationalization of soil health are still evolving to address the concerns over biodiversity, water quality, climate, recreation, and human and planetary health beyond humans. Likewise, appropriate soil health indicators should involve biological, chemical, and physical properties to measure soil multifunctionality and should be integrated into informative soil health indices, which are still under development. We are aware that our research only evaluated two dimensions of the soil health approach: soil fertility and biodiversity conservation. However, we envision that the inclusion of soil invertebrates, as biodiversity indicators, in combination with chemical attributes could contribute to expand the use of the soil health approach in soil assessment and management to attempt longer term sustainability challenges related to multiple ecosystem services.
Conclusion
This study shows clear differences in soil chemical properties and in the structure and composition of edaphic macrofaunal communities between anthropic land use categories and the reference natural system. Biodiversity loss was evident when structure and community composition of soil macrofauna were compared amongst land use categories. As we expected, native forests showed significantly higher values of alpha diversity metrics than pastures and monocultures. However, our reference system presented soil macrofaunal assemblages as diverse as those found in planted forests, a result that was not expected. Then, the biodiversity dimension of our assessment demonstrated a clear pattern between forested and non-forested sites.
Our findings related to soil chemical parameters of the topsoil layer (0-10 cm) demonstrated a strong degradation of soil fertility as a result of native forest conversion to anthropic environments. As expected, we found significantly higher values in many chemical properties that characterized healthy soils (total C, total N, nutrient availability) in native forests compared to anthropic environments. However, for this dimension we did not detect the patterns of forested and non-forested sites found for soil invertebrate diversity.
A significant amount of variation of the community composition and diversity of soil macroinvertebrates was explained by soil chemical parameters. These results demonstrated a connection between biological attributes and chemical properties of topsoils, which likely affect soil health. However, because soil invertebrate communities did not change between native and planted forest, even when chemical parameters of soil fertility were significantly different between them, suggests that other driving factors should be playing a more important role than soil chemical parameters in the establishment of the invertebrate communities in these highland landscapes.
Data availability statement
The original contributions presented in the study are included in the article/Supplementary material, further inquiries can be directed to the corresponding author/s.
Author contributions
Conceptualization, methodology, investigation, and writing—original draft preparation: PG and KT. Formal analysis: KT, PG, and MD. Writing—review and editing: PG, KT, FB, J-TC, and MD. Funding acquisition: PG and MD. All authors have read and agreed to the published version of the manuscript.
Funding
This research was funded by the Research Department and the Faculty of Biological Sciences at Universidad Central del Ecuador (grant N. DOCT-DI-19-05), along with financial support from the Académie de Recherche et d'Enseignement Superior of Belgium (ARES) to PG.
Acknowledgments
The authors would like to thank the Research Department and the Faculty of Biological Sciences at Universidad Central del Ecuador for their financial support of this research. The authors are also grateful for the hard physical work of a group of students, who helped during data collection in the field and sorting samples in the lab. We also express our gratitude to the representatives of the community of Guaraquí Alto and the local authorities of the parish of La Esperanza for their logistical support and help in contacting farmers and landowners that allowed us to access the different study sites. Reviewers provided useful comments on a previous version of the manuscript.
Conflict of interest
The authors declare that the research was conducted in the absence of any commercial or financial relationships that could be construed as a potential conflict of interest.
Publisher's note
All claims expressed in this article are solely those of the authors and do not necessarily represent those of their affiliated organizations, or those of the publisher, the editors and the reviewers. Any product that may be evaluated in this article, or claim that may be made by its manufacturer, is not guaranteed or endorsed by the publisher.
Supplementary material
The Supplementary Material for this article can be found online at: https://www.frontiersin.org/articles/10.3389/ffgc.2022.959799/full#supplementary-material
Footnotes
1. ^Perez, A., Acosta-Lopez, C., Buitrón, S., Guarderas, P. (2022). ‘Land use changes alters the diversity and composition of soil bacteria in an Andean landscape of northern Ecuador', Microbiology Society. (submitted).
References
Araneda, A. (2016). Uso de la lombriz de tierra como organismo indicador del impacto de pesticidas en el agrosistema (Concepción: Repositorio Dspace).
Baillie, I. C., Anderson, J. M., and Ingram, J. S. I. (1990). Tropical soil biology and fertility: a handbook of methods. J. Ecol. 78, 547. doi: 10.2307/2261129
Bardgett, R. D., and Van Der Putten, W. H. (2014). Belowground biodiversity and ecosystem functioning. Nature. 515, 505–511. doi: 10.1038/nature13855
Brown, G. G., Fragoso, C., Barois, I., Rojas, P., Patrón, J. C., Bueno, J., Moreno, A. G., Lavelle, P., Ordaz, V., and y Rodríguez, C. (2001). Diversidad y rol funcional de la macrofauna edáfica en los ecosistemas tropicales mexicanos, ACTA ZOOLÓGICA MEXICANA (N.S.), pp. 79–110. doi: 10.21829/azm.2001.8401847
Brussaard, L., Aanen, D. K., Briones, M. J. I., Decaëns, T., Deyn, G. B., De, Fayle, T. M., et al. (2013). “Biogeography and phylogenetic community structure,” in Soil Ecology and Ecosystem Services, eds D. H. Wall, R. Bardgett, V. Behan-Pelletier, J. E. Herrick, T. H. Jones, K. Ritz, D. R. Strong, and W. H. van der Putten (Oxford: Oxford Academic). doi: 10.1093/acprof:oso/9780199575923.003.0018, (accessed September 20, 2022).
Bünemann, E. K., Bongiorno, G., Bai, Z., Creamer, R. E., De Deyn, G., de Goede, R., et al. (2018). Soil quality – a critical review. Soil Biol. Biochem. 120, 105–125. doi: 10.1016/j.soilbio.2018.01.030
Cabrera, G. (2014). Manual práctico sobre la macrofauna edáfica como indicador biológico de la calidad del suelo, según resultados en Cuba., ed. The Rofford Cuba. Habana: Fundación Rufford.
Cabrera, G., and López, I. (2018). Ecological characterization of soil macrofauna in two evergreen forest sites at el salón, sierra del rosario, Cuba. Bosque. 39, 363–373. doi: 10.4067/S0717-92002018000300363
Cáceres-Arteaga, N., Ayala-Campaña, O., Rosero-Vaca, D. D., and Lane, K. (2018). ¿Que nos depara el futuro? Análisis climático histórico y proyección de escenarios climáticos futuros para el cantón andino de Pedro Moncayo, Ecuador. Rev. Geográfica América Cent. 3, 297–318. doi: 10.15359/rgac.61-3.15
Chao, A., Chiu, C. H., and Jost, L. (2014). Unifying species diversity, phylogenetic diversity, functional diversity, and related similarity and differentiation measures through hill numbers. Annu. Rev. Ecol. Evol. Syst. 45, 297–324. doi: 10.1146/annurev-ecolsys-120213-091540
Coca-Salazar, A., Cornelis, J. T., and Carnol, M. (2021). Soil properties and microbial processes in response to land-use change in agricultural highlands of the Central Andes. Eur. J. Soil Sci. 72, 2292–2307. doi: 10.1111/ejss.13110
Colwell, R. (2013). EstimateS: biodiversity estimation. Available at: http://viceroy.eeb.uconn.edu/estimates/ (accessed May 10, 2019).
Colwell, R. K., and Coddington, J. A. (1994). Estimating terrestrial biodiversity through extrapolation. Philosophical Transactions of the Royal Society of London B—Biological Sciences, 345, 101–118. https://doi.org/10.1098/rstb.1994.0091
Comerford, N. B., Franzluebbers, A. J., Stromberger, M. E., Morris, L., Markewitz, D., and Moore, R. (2013). Assessment and evaluation of soil ecosystem services. Soil Horizons. 54, 1–14. doi: 10.2136/sh12-10-0028
De Valença, A. W., Vanek, S. J., Meza, K., Ccanto, R., Olivera, E., Scurrah, M., et al. (2017). Land use as a driver of soil fertility and biodiversity across an agricultural landscape in the Central Peruvian Andes. Ecol. Appl. 27, 1138–1154. doi: 10.1002/eap.1508
Decaëns, T., Jiménez, J. J., Gioia, C., Measey, G. J., and Lavelle, P. (2006). The values of soil animals for conservation biology. Eur. J. Soil Biol. 42, S23–S38. doi: 10.1016/j.ejsobi.2006.07.001
Delelegn, Y. T., Purahong, W., Blazevic, A., Yitaferu, B., Wubet, T., Göransson, H., et al. (2017). Changes in land use alter soil quality and aggregate stability in the highlands of northern Ethiopia. Sci. Rep. 7, 1–12. doi: 10.1038/s41598-017-14128-y
Díaz, S., Fargione, J., Chapin, F., and Tilman, D. (2006). Biodiversity loss threatens human well-being. PLoS Biol. 4, e277. doi: 10.1371/journal.pbio.0040277
Dominati, E., Mackay, A., and Patterson, M. (2010). Modelling the provision of ecosystem services from soil natural capital. World Congr. Soil Sci. 32–35. Available online at: https://www.iuss.org/19th%20WCSS/Symposium/pdf/1841.pdf
Duddigan, S., Fraser, T., Green, I., Diaz, A., Sizmur, T., and Tibbett, M. (2021). Plant, soil and faunal responses to a contrived pH gradient. Plant Soil 462, 505–524. doi: 10.1007/s11104-021-04879-z
Eisenhauer, N., Antunes, P. M., Bennett, A. E., Birkhofer, K., Bissett, A., Bowker, M. A., et al. (2017). Priorities for research in soil ecology. Pedobiologia (Jena). 63, 1–7. doi: 10.1016/j.pedobi.2017.05.003
Eisenhauer, N., Antunes, P. M., Bennett, A. E., Birkhofer, K., Bowker, M. A., Caruso, T., et al. (2018). Europe PMC Funders Group Priorities for research in soil ecology. 63, 1–7.
Ernst, G., and Emmerling, C. (2009). Impact of five different tillage systems on soil organic carbon content and the density, biomass, and community composition of earthworms after a ten year period. Eur. J. Soil Biol. 45, 247–251. doi: 10.1016/j.ejsobi.2009.02.002
Escobar, A., Filella, J., González, V., and Noel, A. (2017). Estudio comparativo macrofauna del suelo en sistema agroforestal, potrero tradicional y bosque latifoliado en microcuenca del trópico seco, Tomabú, Nicaragua. Rev. Científica FAREM-Estelí. 22, 39–49. doi: 10.5377/farem.v0i22.4520
Farley, K. A. (2007). Grasslands to tree plantations: forest transition in the Andes of Ecuador. Ann. Assoc. Am. Geogr. 97, 755–771. doi: 10.1111/j.1467-8306.2007.00581.x
Foley, J. A., DeFries, R., Asner, G. P., Barford, C., Bonan, G., Carpenter, S. R., et al. (2005). Global consequences of land use. Science. 309, 570–574. doi: 10.1126/science.1111772
Food and Agriculture Organization (2015). “Intergovernmental technical panel on soils status of the world's soil resources”, in Food and Agriculture Organization of the United Nations and Intergovernmental Technical Panel on Soils (Italia).
Food and Agriculture Organization of the United Nations. (2015). Suelos y Biodiversidad. 1 ed. Italia: Roma,
GAD Municipal Pedro Moncayo. (2015). GAD Municipal Pedro Moncayo. Available online at: http://sitp.pichincha.gob.ec/repositorio/diseno_paginas/archivos/PDOTLAESPERANZA2015.pdf (accessed March 28, 2019).
Gaglio, M., Aschonitis, V. G., Mancuso, M. M., Puig, J. P. R., Moscoso, F., Castaldelli, G., et al. (2017). Changes in land use and ecosystem services in tropical forest areas: a case study in Andes mountains of Ecuador. Int. J. Biodivers. Sci. Ecosyst. Serv. Manag. 13, 264–279. doi: 10.1080/21513732.2017.1345980
Gehlhausen, S. M., Schwartz, M. W., and Augspurger, C. K. (2000). Vegetation and microclimatic edge effects in two mixed-mesophytic forest fragments
Goulding, K. W. T. (2016). Soil acidification and the importance of liming agricultural soils with particular reference to the United Kingdom. Soil Use Manag. 32, 390–399. doi: 10.1111/sum.12270
Guamán Trávez, K., and Guarderas, P. (In press). Land use affects the local climate of a tropical mountain landscape in Northern Ecuador. Mt. Re. Dev.
Guangbin, Y., and Xiaodong, Y. (2007). Characteristics of litter and soil arthropod communities at different suc-cessional stages of tropical forests. Biodivers. Sci. 15, 188. doi: 10.1360/biodiv.060292
Guarderas, P., Smith, F., and Dufrene, M. (2022). Land use and land cover change in a tropical mountain landscape of northern Ecuador: altitudinal patterns and driving forces. PLoS ONE. 17, 1–26. doi: 10.1371/journal.pone.0260191
Halffter, G., and Arellano, L. (2002). Response of dung beetle diversity to human-induced changes in a tropical landscape. Biotropica. 34, 144–154. doi: 10.1111/j.1744-7429.2002.tb00250.x
Hammer, Ø., Harper, D., and Ryan, P. (2001). Past: Paleontological Statistics Software Package for Education and Data Analysis. Oslo: Palaeontological Association.
Hao, T., Zhu, Q., Zeng, M., Shen, J., Shi, X., Liu, X., et al. (2020). Impacts of nitrogen fertilizer type and application rate on soil acidification rate under a wheat-maize double cropping system. J. Environ. Manage. 270, 110888. doi: 10.1016/j.jenvman.2020.110888
Heitkamp, F., Sylvester, S. P., Kessler, M., Sylvester, M. D. P. V., and Jungkunst, H. F. (2014). Inaccessible Andean sites reveal human-induced weathering in grazed soils. Prog. Phys. Geogr. 38, 576–601. doi: 10.1177/0309133314544918
JASP Team. (2020). Jasp Version 0.11.1. Comput. Softw. Available online at: https://jasp-stats.org/faq/how-do-i-cite-jasp/ (accessed May 10, 2019).
Jiménez-Valverde, A., and Hortal, J. (2003). Las curvas de acumulación de especies y la necesidad de evaluar la calidad de los inventarios biológicos. Rev. Ibérica Aracnol. 8, 151–116. Available online at: https://jhortal.com/pubs/2003-Jimenez-Valverde&Hortal_Rev_Ib_Aracnol.pdf
Karlen, D. L., Ditzler, C. A., and Andrews, S. S. (2003). Soil quality: why and how? Geoderma. 114, 145–156. doi: 10.1016/S0016-7061(03)00039-9
Kitching, R. (2000). Food webs and container habitats: the natural history and ecology of phytotelmata. J. Ecol. 89, 461. doi: 10.1046/j.0022-0477.2001.00593-4.x
Košulič, O., Michalko, R., and Hula, V. (2016). Impact of canopy openness on spider communities: implications for conservation management of formerly coppiced oak forests. PLoS ONE. 11, 1–18. doi: 10.1371/journal.pone.0148585
Landis, D. A. (2016). Designing agricultural landscapes for biodiversity-based ecosystem services. Basic Appl. Ecol. 18, 1–12. doi: 10.1016/j.baae.2016.07.005
Lauber, C. L., Strickland, M. S., Bradford, M. A., and Fierer, N. (2008). The influence of soil properties on the structure of bacterial and fungal communities across land-use types. Soil Biol. Biochem. 40, 2407–2415. doi: 10.1016/j.soilbio.2008.05.021
Lavelle, P., Rodríguez, N., Arguello, O., Bernal, J., Botero, C., Chaparro, P., et al. (2014). Soil ecosystem services and land use in the rapidly changing orinoco river basin of colombia. Agric. Ecosyst. Environ. 185, 106–117. doi: 10.1016/j.agee.2013.12.020
Le Bayon, R.-C., and Milleret, R. (2009). Effects of earthworms on phosphorus dynamics – a review. Dyn. Soil, Dyn. Plant. Glob. Sci. Books. 3, 21–27. Available online at: http://www.globalsciencebooks.info/Online/GSBOnline/images/0906/DSDP_3(SI2)/DSDP_3(SI2)21-27o.pdf
Lehmann, J., Bossio, D. A., Kögel-Knabner, I., and Rilling, M. C. (2020). The concept and future prospects of soil health. Nat. Rev. Earth. Environ. 1, 544–553. doi: 10.1038/s43017-020-0080-8
Leibensperger, L. B. (2016). Herbert Walter Levi (1921–2014) and Lorna Levi (1928–2014). Breviora. 551, 1–37. doi: 10.3099/mcz28.1
Lema, N. (2016). Determinación de la macrofauna edáfica en distintos usos de suelos en tres agroecosistemas de la comunidad de Naubug. Ecuador: Riobamba: Escuela Superior Politécnica de Chimborazo.
Lemenih, M., Karltun, E., and Olsson, M. (2005). Assessing soil chemical and physical property responses to deforestation and subsequent cultivation in smallholders farming system in Ethiopia. Agric. Ecosyst. Environ. 105, 373–386. doi: 10.1016/j.agee.2004.01.046
Letourneau, D. K., Armbrecht, I., Rivera, B. S., Lerma, J., Carmona, E. J., Daza, M. C., et al. (2011). Does plant diversity benefit agroecosystems? A synthetic review. Ecol. Appl. 21, 9–21. doi: 10.1890/09-2026.1
Liang, J., Reynolds, T., Wassie, A., Collins, C., and Wubalem, A. (2016). Effects of exotic Eucalyptus spp. plantations on soil properties in and around sacred natural sites in the northern Ethiopian Highlands. AIMS Agric. Food. 1, 175–193. doi: 10.3934/agrfood.2016.2.175
Lukina, N. V., Orlova, M. A., and Isaeva, L. G. (2011). Forest soil fertility: the base of relationships between soil and vegetation. Contemp. Probl. Ecol. 4, 725–733. doi: 10.1134/S1995425511070046
Manhães, C. M. C., Gama-Rodrigues, E. F., Silva Moço, M. K., and Gama-Rodrigues, A. C. (2013). Meso- and macrofauna in the soil and litter of leguminous trees in a degraded pasture in Brazil. Agrofor. Syst. 87, 993–1004. doi: 10.1007/s10457-013-9614-0
Mann, C., Lynch, D., Fillmore, S., and Mills, A. (2019). Relationships between field management, soil health, and microbial community composition. Appl. Soil Ecol. 144, 12–21. doi: 10.1016/j.apsoil.2019.06.012
McGavin, G. (2000). Manual de Identificacion de Insectos, Arañas y Otros Artropodos Terrestres. Barcelona: Omega S. A.
Menta, C. (2012). Soil fauna diversity - function, soil degradation, biological indices, soil restoration. Biodivers. Conserv. Util. a Divers. World. doi: 10.5772/51091
Millennium Ecosystem Assessment. (2005). Ecosystems and Human Well-Being : Synthesis. Washington, DC: Island Press
Ministerio de Agricultura y Ganadería. (2017). Mapa de órdenes de suelos del Ecuador. Quito: SIGTIERRAS (Sistema Nac. Inf. y Gestión Tierras Rural. e Infraestruct. Tecnológica). 15.
Ministerio del Ambiente del Ecuador. (2013). “Sistema de Clasificación de los Ecosistemas del Ecuador Continental”, in Subsecretaría de Patrimonio Natural (Ecuador).
Ministerio del Ambiente del Ecuador. (2016). Análisis de la deforestación en el Ecuador Continental 1990 - 2014. Quito: Ecuador
Montejo-Kovacevich, G., Marsh, C. J., Smith, S. H., Peres, C. A., and Edwards, D. P. (2022). Riparian reserves protect butterfly communities in selectively logged tropical forest. J. Appl. Ecol. 59, 1524–1535. doi: 10.1111/1365-2664.14162
Natural Resources Conservation Service. (2022). Soil health. Washington, DC: U.S. DEPARTMENT OF AGRICULTURE.
Nielsen, U. N., Wall, D. H., and Six, J. (2015). Soil biodiversity and the environment. Annu. Rev. Environ. Resour. 40, 63–90. doi: 10.1146/annurev-environ-102014-021257
Nieminen, M., Ketoja, E., Mikola, J., Terhivuo, J., Sirén, T., and Nuutinen, V. (2011). Local land use effects and regional environmental limits on earthworm communities in finnish arable landscapes. Ecol. Appl. 21, 3162–3177. doi: 10.1890/10-1801.1
Oksanen, J., Blanchet, F. G., Friendly, M., Kindt, R., Legendre, P., McGlinn, D., et al. (2019). Vegan: Community Ecology Package. Oulu: Benjamin Cummings.
Phillips, H. R. P., Guerra, C. A., Bartz, M. L. C., Briones, M. J. I., Brown, G., Crowther, T. W., et al. (2019). Global distribution of earthworm diversity. Science (80-.). 366, 480–485. doi: 10.1126/science.aax4851
Reed, M. S., Hubacek, K., Bonn, A., Burt, T. P., Holden, J., Stringer, L. C., et al. (2013). Anticipating and managing future trade-offs and complementarities between ecosystem services. Ecol. Soc. 18, 1–21. doi: 10.5751/ES-04924-180105
Rousseau, L., Fonte, S. J., Téllez, O., Van Der Hoek, R., and Lavelle, P. (2013). Soil macrofauna as indicators of soil quality and land use impacts in smallholder agroecosystems of western Nicaragua. Ecol. Indic. 27, 71–82. doi: 10.1016/j.ecolind.2012.11.020
Ruiz, D., Bueno-Villegas, J., and Feijoo-Martínez, A. (2010). Uso de la tierra y diversidades alfa, beta y gamma de diplópodos en la cuenca del río Otún, Colombia. Univ. Sci. 15, 59–67. doi: 10.11144/javeriana.sc15-1.luaa
Shrewsbury, P. M., and Raupp, M. J. (2006). Do top-down or bottom-up forces determine Stephanitis pyrioides abundance in urban landscapes? Ecol. Appl. 16, 262–272. doi: 10.1890/04-1347
Soliveres, S., Van Der Plas, F., Manning, P., Prati, D., Gossner, M. M., Renner, S. C., et al. (2016). Biodiversity at multiple trophic levels is needed for ecosystem multifunctionality. Nature 536, 456–459. doi: 10.1038/nature19092
Solórzano Flores, A. (2020). Comparación de la diversidad vegetal y calidad orgánica del suelo entre un remanente de bosque nativo y vegetación introducida, Parroquia La Esperanza, Cantón Pedro Moncayo. Pichincha: Ecuador.
Suquilanda, M. B. (2008). El deterioro de los suelos en el Ecuador y la producción agricola. Ecuador: Universidad Central del Ecuador.
Sylvain, Z. A., and Wall, D. H. (2011). Linking soil biodiversity and vegetation: implications for a changing planet. Am. J. Bot. 98, 517–527. doi: 10.3732/ajb.1000305
The Intergovernmental Science-Policy Platform on Biodiversity and Ecosystem Services. (2018). The assessment report on land degradation and restoration. 1–44
Thiele-Bruhn, S., Bloem, J., de Vries, F. T., Kalbitz, K., and Wagg, C. (2012). Linking soil biodiversity and agricultural soil management. Curr. Opin. Environ. Sustain. 4, 523–528. doi: 10.1016/j.cosust.2012.06.004
Tibbett, M., Fraser, T. D., and Duddigan, S. (2020). Identifying potential threats to soil biodiversity. PeerJ 8, e9271. doi: 10.7717/peerj.9271
Triplehorn, C., Johnson, N., and Borror, D. (2005). Borror and DeLong's Introduction to the Study of Insects. Minnesota: Thompson Brooks.
Urrego, B. (1996). La reforestación con coniferas y sus efectos sobre la acidificación, podsolización y perdida de fertilidad de los suelos. Smurfit Cartón de Colombia Colombia.
Veldkamp, E., Schmidt, M., Powers, J. S., and Corre, M. D. (2020). Deforestation and reforestation impacts on soils in the tropics. Nat. Rev. Earth Environ. 1, 590–605. doi: 10.1038/s43017-020-0091-5
Villarreal, H., Álvarez, M., Córdoba, S., Escobar, F., Fagua, F., Gast, F., et al. (2006). Manual de métodos para el desarrollo de inventarios de biodiversidad. 2nd ed. Colombia: Instituto de Investigación de Recursos Biológicos Alexander von Humboldt.
Whittaker, R. (1972). Evolution and measurement of species diversity. Taxon. 21, 213–251. doi: 10.2307/1218190
Whittaker, R., Willis, J., and Field, R. (2001). Scale and species richness: towards a general, hierarchical theory of species diversity. J. Biogeogr. 28, 453–470. doi: 10.1046/j.1365-2699.2001.00563.x
Winkler, K., Fuchs, R., Rounsevell, M., and Herold, M. (2021). Global land use changes are four times greater than previously estimated. Nat. Commun. 12, 1–10. doi: 10.1038/s41467-021-22702-2
Yitaferu, B., Abewa, A., and Amare, T. (2013). Expansion of eucalyptus woodlots in the fertile soils of the highlands of ethiopia: could it be a treat on future cropland use? J. Agric. Sci. 5, 1–12. doi: 10.5539/jas.v5n8p97
Zarafshar, M., Bazot, S., Matinizadeh, M., Bordbar, S. K., Rousta, M. J., Kooch, Y., et al. (2020). Do tree plantations or cultivated fields have the same ability to maintain soil quality as natural forests? Appl. Soil Ecol. 151, 103536. doi: 10.1016/j.apsoil.2020.103536
Zegeye, H., Teketay, D., and Kelbessa, E. (2011). Diversity and regeneration status of woody species in Tara Gedam and Abebaye forests, northwestern Ethiopia. J. For. Res. 22, 315–328. doi: 10.1007/s11676-011-0176-6
Zhang, X., Zhao, G., Zhang, X., Li, X., Yu, Z., Liu, Y., et al. (2017). Ground beetle (coleoptera: carabidae) diversity and body-size variation in four land use types in a mountainous area near Beijing, China. Coleopt. Bull. 71, 402–412. doi: 10.1649/0010-065X-71.2.402
Keywords: soil diversity, soil health, native forest conversion, land use change, soil macroinvertebrates, soil ecosystem services, tropical mountain systems
Citation: Guarderas P, Trávez K, Boeraeve F, Cornelis J-T and Dufrêne M (2022) Native forest conversion alters soil macroinvertebrate diversity and soil quality in tropical mountain landscapes of northern Ecuador. Front. For. Glob. Change 5:959799. doi: 10.3389/ffgc.2022.959799
Received: 02 June 2022; Accepted: 08 November 2022;
Published: 02 December 2022.
Edited by:
Shri Kant Tripathi, Mizoram University, IndiaReviewed by:
Carlos Ivan Espinosa, Universidad Técnica Particular de Loja, EcuadorAugustine Ovie Edegbene, Federal University of Health Sciences Otukpo, Nigeria
Daniela Cortes, Senckenberg Biodiversity and Climate Research Centre, Germany
Copyright © 2022 Guarderas, Trávez, Boeraeve, Cornelis and Dufrêne. This is an open-access article distributed under the terms of the Creative Commons Attribution License (CC BY). The use, distribution or reproduction in other forums is permitted, provided the original author(s) and the copyright owner(s) are credited and that the original publication in this journal is cited, in accordance with accepted academic practice. No use, distribution or reproduction is permitted which does not comply with these terms.
*Correspondence: Paulina Guarderas, YXBndWFyZGVyYXNAdWNlLmVkdS5lYw==
†These authors have contributed equally to this work and share first authorship