- 1Wetland Wildlife Research Group, Minnesota Department of Natural Resources, Bemidji, MN, United States
- 2United States Department of Agriculture, Forest Service, Northern Research Station, St. Paul, MN, United States
- 3Biometrics Research Group, Minnesota Department of Natural Resources, Forest Lake, MN, United States
Wood duck females often nest in tree cavities located in large-diameter deciduous trees. Temporal changes in forest age and composition during recent decades may have influenced the abundance of stems with suitable nesting cavities in the forested portion of Northern MN, United States. Thus, we ascertained whether temporal changes in the abundance of such stems occurred in this area during six Forest Inventory and Analysis (FIA) evaluation cycles (i.e., 1977 to 2015–2019). We used FIA data and independently estimated proportions of stems with suitable cavities in different tree-species, health-status, and diameter classes to estimate change in the abundance of such stems at three spatial scales. These spatial scales increased in areal extent from a study area to three ecological subsections to the Laurentian Mixed Forest ecological province. We used a Bayesian analytical approach to accommodate changes in FIA protocols among cycles. Both the abundance of stems with suitable cavities and an attribute associated with cavity occurrence changed during the analysis period, but findings were not entirely consistent among spatial scales. The estimated abundance of both suitable stems overall and those of late-successional tree species generally increased at all scales during the analysis period. Since 1990, increases in the abundance of health-impacted stems that were suitable for nesting occurred at all spatial scales. Our estimated densities of suitable stems during the 2005–2009 (1.20 [85% CrI: 1.04–1.39] suitable stems / ha) and 2015–2019 cycles (1.41 [85% CrI: 1.21–1.63] suitable stems / ha) were substantially greater than the <0.25 and 0.25–0.49 suitable stems / ha another study predicted would occur in Northern Minnesota during 2008 and 2018, respectively. Our results can inform forest management decisions and research directions for follow-up studies of nesting wood ducks.
Introduction
Wood ducks (Aix sponsa) nest throughout much of temperate Eastern and parts of Western North United States (Hepp and Bellrose, 2013), usually in the cavities of large-diameter deciduous trees (Bellrose and Holm, 1994). Tree cavities are not thought to be limiting to the breeding populations of cavity-nesting ducks in the Upper Midwest (Denton et al., 2012a), but the formation of these structures can be a slow process (Bellrose and Holm, 1994). The selection of nest sites is attributable to habitat-related cues (Berg and Eadie, 2020). Distance from the nest tree to water has been suggested as a selection factor (e.g., Nagel, 1969), but the distance from the nest tree to the first wetlands used by some hens with broods [e.g., up to 3.9 km in Minnesota (Ball, 1973) and 11.7 km in Texas (Ridlehuber, 1980)] indicates that this factor is not of primary importance. In contrast, the variables tree species, diameter at breast height (DBH) of stems, cavity height above ground level, and the dimensions of cavity entrances and interiors are thought to be particularly influential for nest-site selection (e.g., Boyer, 1974; Haramis, 1975; Bellrose and Holm, 1994; Zwicker, 1999). The minimum and maximum dimensional bounds and other characteristics of cavities and associated stems used by nesting wood ducks have been measured and characterized at many sites across their breeding range but there were some differences in dimensions reported by different studies (summarized in Soulliere, 1990; Bellrose and Holm, 1994; see section “Materials and methods” for the minimum and maximum values we used).
The availability of stems containing cavities suitable for nesting wood ducks has been estimated using published bounds values and attributes in follow-up studies. Specifically, the proportions of stems in each tree-species- and DBH-class that had suitable nesting cavities were estimated in these investigations (Denton et al., 2012a; Zlonis et al., 2020). Tree health status (3 classes: dead, live–health impacted, live–healthy) was an important influence on the probability of suitable-cavity occurrence (hereafter, P occurrence), with dead stems having the greatest probability, closely followed by live–health impacted stems (i.e., those with defects that suggest a decline in health), and finally, live–healthy stems (Zlonis et al., 2020).
Surveys specifically designed to locate and measure cavities are needed to develop reliable models of P occurrence because these structures may not be easily detectable (Fan et al., 2003), may be in a clustered spatial distribution (Gilmer et al., 1978), and may have internal dimensions or structures that prohibit use despite seemingly suitable entrance dimensions from the vantage point of ground-based personnel (sensu Nelson and Roy, 2012). Cavity surveys usually are conducted at a local scale and not systematically repeated over time because of the intense effort required to collect sufficient data. However, the informed management of wildlife species that use cavities requires a better understanding of the dynamics of the abundance of these structures at spatiotemporal scales greater than that provided by short-term local studies.
Although the USDA Forest Service Forest Inventory and Analysis (FIA) program has reliably measured, classified, and estimated many forest attributes in plots established throughout the United States since the 1930s (Smith, 2002), it has not directly counted tree cavities in the North central United States except during the 1990s (Nelson and Roy, 2012). Information about wood duck nesting-substrate and FIA data has been used to predict future cavity availability, however. Based on projections of future forest conditions (U.S. Department of Agriculture Forest Service, 1982), Soulliere (1990) predicted that the population of stems with suitable cavities in the Eastern United States would increase between 1952 and 2020 but start to decline after 2020. Denton et al. (2012b) used empirical data from local cavity surveys conducted on public lands and FIA data collected at a relatively broad spatiotemporal scale to simulate future changes in the density of stems with structure suitable for several cavity-nesting duck species across the Midwestern United States (ostensibly including public and private lands) during 2008, 2018, and 2028, but did not provide variance estimates associated with their predictions. Such projections of wood duck nesting substrate should be examined with empirical data collected during a long time period (Nielsen et al., 2007).
The validity of Denton’s predictions for 2008 and 2018 now can be verified with empirical data. However, five considerations should be addressed as part of any analyses that can confirm or reject these predictions. First, the most appropriate spatial scales at which to make inferences about changes in the abundance of stems with suitable cavities should be identified. Intuitively, empirical cavity data should be spatially extrapolated only to areas with similar forest types and tree-species compositions yet represent spatial extents large enough to address wood duck habitat-monitoring needs. Second, there should be little meaningful difference between the manners in which FIA and cavity-survey data were collected. Similar field methodologies should generate data that is more likely to produce consistent abundance estimates of stems with suitable cavities. Third, any changes in FIA field methodology and sampling scheme over time could complicate efforts to accurately estimate the abundance of stems with suitable cavities. Therefore, it may be necessary to develop both a crosswalk to identify the most similar FIA damage, disease, and decay codes used during different evaluation cycles and an analytical approach that can accommodate changes in FIA methodology. Fourth, some data will be sparse, perhaps because some tree species are uncommon in the geographic area of interest or have relatively few cavities. Excluding data from such species in analyses likely would contribute to the underestimation of abundance of stems with suitable cavities, so it may be preferable to aggregate data from such species into an “other” species class. Fifth, the analytical method also should be able to accommodate the change in FIA sampling from periodic to annual evaluation cycles so that the temporal change in abundance of suitable stems and associated uncertainty can be accurately estimated.
We attempted to address these issues in an analysis that used both empirical cavity data collected on non-FIA plots during 2016–2018 and FIA tree data collected over decades to ascertain whether any temporal change occurred in the population of tree stems with suitable nesting cavities for wood ducks (hereafter, suitable stems) in the forested portion of Northern Minnesota. Our specific objectives were to (1) ascertain whether temporal changes in the abundance of suitable stems occurred in a study area during six FIA evaluation cycles that spanned 1977 to 2015–2019, (2) extrapolate these results from the spatial scale of the study area to two larger spatial strata with similar forest characteristics (i.e., three ecological subsections that intersected the study area and an ecological province that contained the study area plus the three subsections) within Minnesota during each of the aforementioned cycles, (3) examine temporal characteristics in cavity-stem abundance on private and public lands at each of these scales, (4) compare our results with predictions of cavity-stem density from previous research (i.e., Soulliere, 1990; Denton et al., 2012b), and (5) examine the plausibility of estimates of cavity-stem abundance and the associated uncertainty generated using our analytical approach.
Empirically based estimates of the abundance and density of stems with cavities suitable for nesting wood ducks during a long time period can both provide insight regarding how cavity availability may change with forest succession and inform contemporary forest management in North Central states. Further, our quantitative approach can be used to estimate the temporal change in the abundance of suitable stems in other portions of the wood duck breeding range or other species that use forest attributes that can be associated with FIA metrics. Any substantial differences between the simulated density of stems with suitable cavities Denton et al. (2012b) and our empirically based estimates will provide information that can be used to recalibrate the simulation model used by Denton so that future forest conditions can be more accurately predicted. Our approach provides further refinement in the estimation of wood duck nesting structure availability, and unlike previous work, reports the uncertainty associated with the estimated abundance and density of suitable stems. Researchers can use information we report (e.g., the extent to which results of local surveys should be extrapolated to greater spatial scales, likely sample size needs, specific FIA inventories that collected data in a manner that is inconsistent with project needs, potential sources of bias) during the design of their studies.
Materials and methods
Study site
We conducted cavity surveys in a study area of 254,051 ha (land and water combined) located in the Northern and Eastern portions of Cass County in North central MN, United States (Figure 1A). This area occurs within the Chippewa Plains, St Louis Moraine, and Pine Moraine-Outwash Plain (2,782,822 ha, Figure 1B) ecological subsections and the Laurentian Mixed Forest ecological province (9,375,320 ha, Figure 1C; Cleland et al., 2007). These subsections are contained within the ecological province of interest. The estimated area (ha) of land in public and private ownership at each spatial scale and during each inventory are presented in Table 1. We selected this study area because (1) it was generally representative of the greater ecological province in which it occurs, thus allowing results to be extrapolated to that spatial scale, (2) the airborne Lidar and remote-sensing data collected at this site allowed us to associate those data to stands with suitable cavities (Zlonis et al., 2022), and (3) of the ecological value to wood ducks (S. Cordts, Minnesota Department of Natural Resources, personal communication).
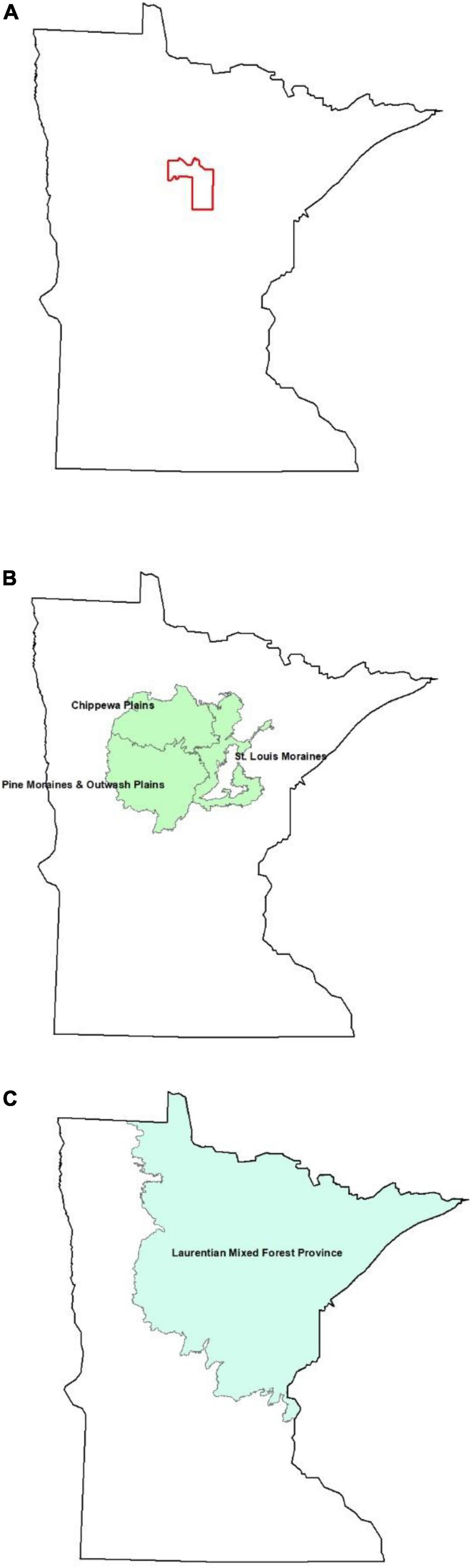
Figure 1. Estimates of the abundance of tree stems in three forest successional classes that were suitable for nesting wood ducks in (A) the ecological province that contained the study area, (B) three ecological subsections that intersected the study area, and (C) a North central MN, United States study area.
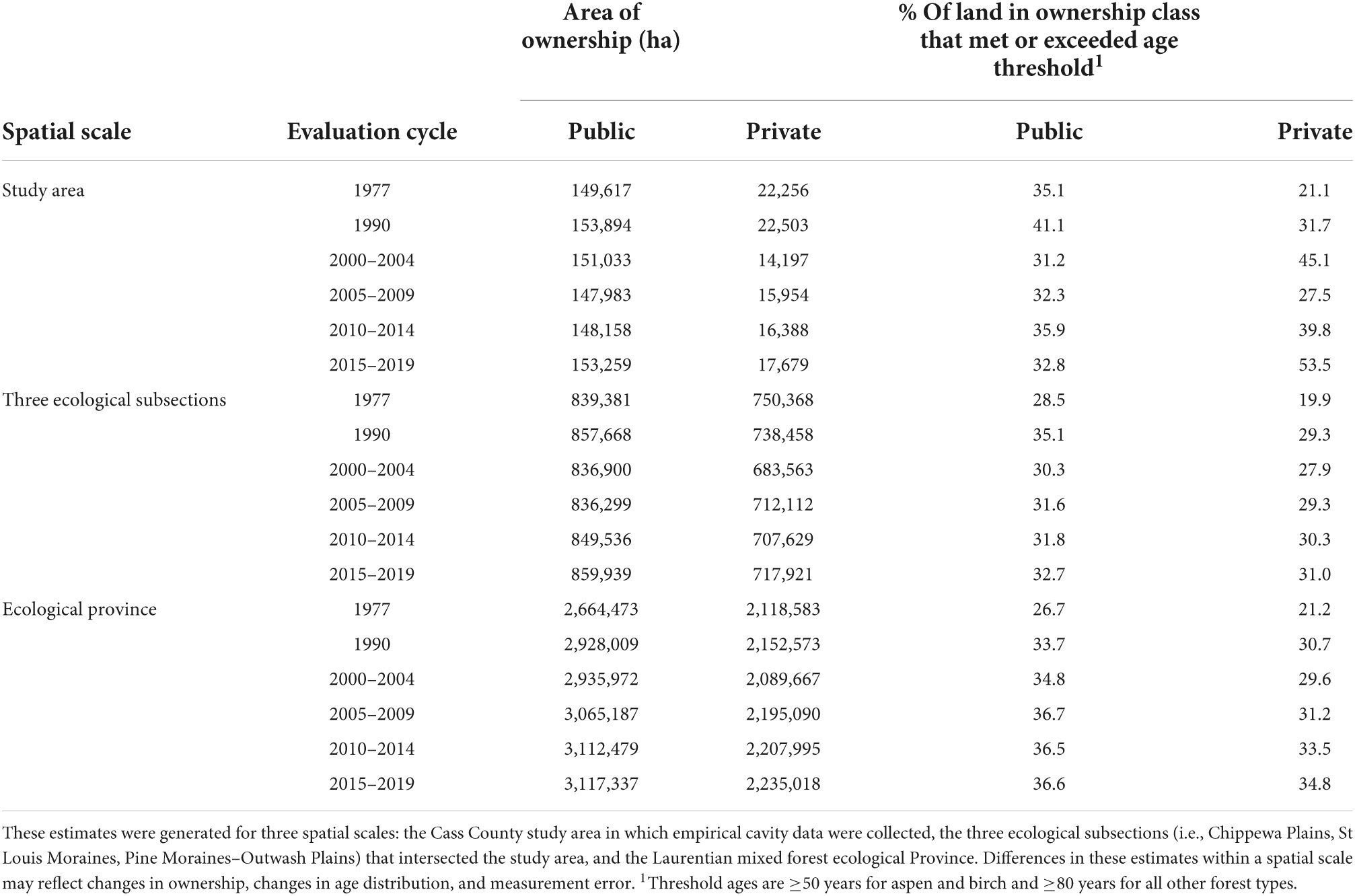
Table 1. The estimated area (ha) of land in public and private ownership overall and percentage of land in these ownership classes that meet or exceed the age threshold in which cavities suitable for nesting wood ducks are likely to occur in the forested portion of Minnesota during six forest inventory and analysis evaluation cycles.
Cavity survey
Minnesota Department of Natural Resources (MNDNR) personnel designed a survey to identify cavities suitable for nesting wood ducks (hereafter, Cavity Survey). Data from this survey were used to model and estimate the proportion of tree stems with cavities suitable for nesting wood ducks (Zlonis et al., 2020). We incorporated FIA field protocols (U.S. Department of Agriculture Forest Service, 2014) to the extent that was practical to ensure that the tree variables measured in the Cavity Survey were similar to FIA data (e.g., assignment of tree species, measurement of stem DBH). However, it was necessary to change some FIA protocols to increase the efficiency of the Cavity Survey and representativeness of the cavity data collected (e.g., altering the sample plot size and placement, reducing the number and complexity of variables measured under the FIA approach; see Zlonis et al. (2020) for a thorough description of methodology). Further, FIA has plots located on lands in public and private ownership and in all forest types and ages, but we conducted Cavity Surveys only on public lands (i.e., USDA Forest Service, State of Minnesota, Cass County) in our study area because most of timberland at this site was in this ownership class (U.S. Department of Agriculture Forest Service, 2022), there was greater ease of obtaining access to these properties by field personnel, and the forest databases of these properties contained information about forest types and stand ages that could be used to identify stands that had some likelihood of containing stems with suitable cavities. We also selected survey stands of only the ages and in the forest types in which there was some likelihood of suitable cavities occurring [i.e., aspen and birch stands ≥50 years old, and lowland deciduous, oak, upland pine, and northern hardwood stands ≥80 years old (see Gilmer et al., 1978)], and excluded stands of forest types or ages that had a low likelihood of containing suitable stems.
We randomly selected 300 forest stands (60 stands of each of the 5 targeted types) to be surveyed, then randomly selected locations for 1–2 sample plots within these stands. We stipulated that plot centers must be both ≥50 m apart and ≥30 m from the nearest stand boundary to increase the likelihood that the interior diversity of vegetation structure of each forest type was adequately represented. We then randomly selected n = 563 plots but could survey only 323 plots because of logistical reasons (e.g., flooding, time constraints) and the removal of 19 plots from the sample because of nearby heritage sites that restricted activities or scheduled timber harvests that would remove trees before surveys could be performed.
We navigated to the selected plot centers using a Garmin Montana Global Positioning System (GPS) receiver and established 20-m radius circular plots (0.126 ha) around those points. In a few situations, we adjusted the location of plots to where the forest structure was more representative of interior stand conditions (e.g., plots located near ecotones not indicated on available geospatial-data layers were moved a short distance into the stand interior, see Zlonis et al., 2020). We obtained location data for each plot center using Geneq Sx Blue II (0.9–1.8 m accuracy under closed forest canopy when obtaining 1 reading / 5 s for approximately 15 min) or Geneq Sx Blue II + GNSS (0.5–0.9 m accuracy under closed forest canopy when obtaining 1 reading / 5 s for approximately 15 min) GPS units.
We used U.S. Department of Agriculture Forest Service (2014) protocols to classify stand structure (5 classes: single story, two-storied, multi-storied, mosaic, unknown or not assessable) and classify and measure several tree variables for stems that were not leaning >45° and tall enough for the DBH to be measured (i.e., ≥1.37 m), including species and DBH (0.1 cm increments) for those stems large enough to accommodate cavities used by nesting wood ducks [i.e., ≥22.0 cm DBH (Haramis, 1975)]. We also recorded the health status of each stem [7 classes: (1) healthy live trees with no defects that threaten its long-term health, (2) live trees with defects that suggest a decline in health (e.g., dead limbs, decay on the bole, the presence of fungi), (3) recently dead trees with bark, limbs, and twigs largely intact, (4) dead trees that have lost some limbs and almost all the twigs, (5) dead trees that have lost most limbs and all twigs, (6) dead trees with broken tops and bole wood that is hard, and (7) dead trees with broken tops and bole wood that is soft (Thomas et al., 1979)]. We combined all stages of dead stems into a single class because these data were sparse. Thus, each stem was assigned to one of three health-status classes: live-healthy (1), live-health impacted (2), and dead (3–7).
Field crew members then used 8 × 40, 10 × 40, or 10 × 50 binoculars to conduct a preliminary search of each tree in the plot to visually detect cavities that potentially were suitable for nesting by wood ducks. During the initial search, personnel ascertained whether the entrance dimensions likely were sufficient to permit a wood duck to pass through (i.e., 6 cm × 6 cm; Zwicker, 1999) and the bottom of cavity entrance was high enough to be used by nesting wood ducks [i.e., ≥0.6 m above ground level (Strom, 1969)]. When a potentially suitable cavity was encountered, we measured cavity height with either a 15.2 m measuring ruler (± 0.1 m), rangefinder (± 0.1 m), or clinometer (± 0.5 m). We then used a remote camera attached via a stiff, braided wire to the measuring ruler (sensu Waldstein, 2012) to perform a thorough examination of the entrance and interior of the cavity. We first determined whether cavity entrance dimensions were suitable by attempting to pass a cardboard cut-out of the minimum usable dimensions through the cavity opening. The cut-out was placed on the wire connecting the camera to the measuring ruler. We then examined cavity interiors with the camera to ascertain whether the following conditions had been met: horizontal depth appeared large enough for hens to move from the entrance to the interior of the cavity (approximately 10 cm from inner edge of the entrance opening toward the back of the cavity), vertical depth (from the bottom of the cavity to the bottom of the entrance) was ≥10.2 to 4.5 m; Bellrose and Holm, 1994 p. 176) and not hollow to the ground (Robb, 1986), potential nesting platforms in cavity interiors that were ≥14 cm × 15 cm (Boyer, 1974; Haramis, 1975; Denton et al., 2012a), and the cavity did not contain standing water or excess debris (Sousa and Farmer, 1984).
Field personnel used this information to classify the suitability of each examined cavity for wood duck nesting (4 levels: suitable, marginal, unsuitable, unknown). We considered a cavity to be suitable if all these conditions were met. A cavity was classified as marginal if it was unclear whether all dimensional requirements were met (i.e., ≥1 dimensional measurement appeared to be close to some minimum or maximum value). We typically classified cavities as unknown if personnel were unable to completely observe the cavity, either because of cavity height or some structural attribute did not permit observation with the camera. We also considered a cavity to be unsuitable if any dimensional measurement was not met or if there was standing water or excess debris in the cavity. Our assessment of the suitability of interior characteristics required some subjective image interpretation because direct measurements could not be made with our camera system.
Forest Inventory and Analysis
Minnesota FIA published reports have been available since 1936 and electronic field data are publicly available for the 1977 and 1990 periodic inventories and all annual inventories beginning in 1999. FIA sample designs and plot designs have changed considerably over time, but measurement protocols have remained relatively consistent during recent decades. However, four changes relevant to our analysis occurred between the 1977 and 2015–2019 cycles. First, all accessible plots were measured within a period of about 1 year during the 1977 and 1990 periodic inventories, but during annual inventories (1999–present) only about 20% of sample plots were measured each year, resulting in 5-year evaluation cycles to measure a full sample of plots (e.g., 1999–2003). Second, the 1990 evaluation used a model-assisted, two-phase design that includes data from a subsample of undisturbed plots that were modeled from 1977 data (i.e., not remeasured during the 1990 cycle). Third, definitions of standing dead trees used in the 1977 and 1990 periodic inventories and annual inventories (since 1999) changed. All inventories tallied mortality trees ≥12.7 cm. However, the 1977 inventory tallied mortality for both standing and down trees, but only if mortality occurred within the previous three years. During the 1990 inventory, such stems were tallied if either they were (1) dead in the 1977 inventory and were “…still standing or if they had been cut,” (2) alive in the 1977 inventory but dead during the 1990 inventory, or (3) were new trees not measured in 1977 (ingrowth, ongrowth) and had died within 3 years. Subsequent FIA annual inventories included only standing dead trees (i.e., down dead trees were excluded) and did not constrain mortality to having occurred within the previous 3 years. Beginning during the 1999 cycle, dead stems were tallied as a standing dead tree if the DBH was ≥12.7 cm, the unbroken length of the bole was ≥1.37 m, and the stem leaned <45°. Fourth, the coding of tree damage, disease, and decay (hereafter, damage codes) has changed several times from 1977 until present. Importantly, no tree damage codes were recorded during the 1999 annual inventory cycle.
Because we wanted to better understand how the abundance of suitable stems changed during as long a time period as possible, it was necessary to include data from both periodic and annual inventories in analyses. We took two steps to address these changes in FIA methodology that occurred both between and within these two types of inventories and to ensure that comparable data were queried from FIA and Cavity Survey databases. First, we did not include 1999 FIA data in our analysis because no tree-damage data were recorded during that evaluation. Rather, we used data from 1977 and 1990 periodic-evaluation cycles, as well as post-1999 annual-inventory data aggregated across 5-year periods (i.e., 2000–2004, 2005–2009, 2010–2014, 2015–2019). Second, we developed a health-status crosswalk to ensure that the classification of stem health was consistent both among FIA evaluation cycles and between FIA and the Cavity Survey. When it was not possible to completely reconcile differences among FIA evaluation cycles and between FIA and the Cavity Survey, we explicitly stated the assumptions that were necessary to proceed with our analyses.
Health-status crosswalk
We identified FIA damage code definitions that were similar among evaluation cycles and most closely corresponded to those used in the Cavity Survey for live-healthy, live-health impacted, and dead stems in the development of the health-status crosswalk. Although FIA damage codes and definitions have changed multiple times, an underlying premise across all these inventories is that damage or pathogen activities were recorded only when serious enough to reduce the quality or vigor of a tree (U.S. Department of Agriculture Forest Service, 1986). The FIA tree attributes most closely corresponding to the MNDNR live-health impacted definition (i.e., live stems with defects that suggest a decline in health) include AGENTCD (used during the 1977 and 1990 evaluation cycles), DAMAGE_AGENT1_NCRS (used during 2000–2012), and DAMAGE_AGENT_CD1 (used during 2013–2019). Stems classified as live-healthy in the Cavity Survey likely would not have been assigned a damage code in FIA. The manner in which stems that would have been classified as dead in the Cavity Survey has changed among FIA evaluation cycles, as described in the Forest Inventory and Analysis (FIA) subsection.
Forest Inventory and Analysis database query development
We queried the FIA database (FIADB) to extract data that closely corresponded to the characteristics of stems sampled in the Cavity Survey that were associated with P occurrence; (i.e., tree species, DBH, health status; Zlonis et al., 2020). We limited these data to stems ≥22.0 cm DBH located on sites classified as timberland by FIA [i.e., forest land that is producing or is capable of producing crops of industrial wood and not withdrawn from timber utilization by statute or administrative regulation, capable of producing >0.57 cubic meters per hectare per year of industrial wood in natural stands. Timberland includes currently inaccessible and inoperable areas (Bechtold and Patterson, 2005)]. Timberland comprises approximately 90% of all forest land in Minnesota. Forest land is land ≥10% canopy cover by live tally tree species of any size or has had ≥10% canopy cover of live tally tree species in the past, based on the presence of stumps, snags, or other evidence. Forest land includes transition zones, such as areas between heavily forested and non-forested lands that meet the minimal tree cover and forest areas adjacent to urban and built-up lands. The minimum area for classification of forest land is 0.4 ha and 36.6 m wide measured stem-to-stem from the outer-most edge. Unimproved roads and trails, streams, and clearings in forest areas are classified as forest if <36.6 m wide. To correspond with the Cavity Survey, we excluded FIADB forested plots classified as Reserved (e.g., Designated Wilderness) or “Other” (unproductive); i.e., we included only timberland, which was consistent across all Minnesota FIA inventories between 1977 and present. Timberland composed about 95% of all forest land at both the Cass County study area and three ecological subsections spatial scales, and 89% of that in the ecological province.
We aggregated the extracted tree-stem data by species, land ownership (private vs. public), geographic area (i.e., study area, three ecological subsections that intersected the study area, and the ecological province that contained the study area as well as the three subsections) and evaluation cycles (i.e., 1977, 1990, 2000–2004, 2005–2009, 2010–2014, 2015–2019). We also used the health-status crosswalk to aggregate stems into the three health-status classes used in the Cavity Survey.
Assumptions
1. The proportions of live-healthy, live-health impacted, and dead stems of each of the 8 individual tree species and the less common species aggregated into “other hardwoods” and “softwoods” classes that were >22.0 cm DBH and in older stands on public lands generated from the Cavity Survey were not significantly different than that observed in the corresponding species-health status-DBH bins of FIA data for the same time period, land ownerships, and geographic areas examined (i.e., study area, three ecological subsections, ecological province). However, class proportions could vary spatially (e.g., county-by-county) and temporally (e.g., year-by-year) because of the heterogeneous frequency of damage and death events.
2. The proportions (and associated distributions) of stems with suitable cavities in each species- health status-DBH bin measured in the Cavity Survey were representative of the geographic areas, ownership type (i.e., public, private), and time periods to which data were extrapolated.
3. FIA non-damaged trees, damaged trees, and dead trees were representative of the Cavity Survey’s live-healthy, live-health impacted, and dead classes, respectively, across all versions of FIA damage and dead tree definitions. For example, the different FIA damage codes assigned to stems [i.e., AGENTCD (1977, 1990), DAMAGE_AGENT1_NCRS (2000–2012), and DAMAGE_AGENT_CD1 (2013–2019)] had the same threshold for contribution to the live-health impacted class.
4. The stand-type classification system used by FIA is consistent with that used in the Cavity Survey (i.e., aspen-birch, lowland hardwood, upland pine, northern hardwood, oak, other). Note: plots in the “other deciduous” and “coniferous” classes were not included in the Cavity Survey but were examined by FIA and probably contained some tree species of interest.
5. Stand age was measured in the same manner during FIA, USFS, State, and Cass County surveys.
6. The areal extent of land in private and public ownership remained constant during the period of analysis.
7. The minimum DBH (22.0 cm) of a normally proportioned stem (i.e.., no unusual swelling), range of minimum and maximum dimensions of cavities, and other cavity characteristics in which the scientific literature indicates that wood ducks nest is applicable to our geographic area of inference (see Denton et al., 2012b).
8. Individual stems on public lands have the same probability of forming a suitable nesting cavity as an individual stem of the same species-DBH-health status class on private land (see Denton et al., 2012b).
Data analysis
We used the R2jags package (Su and Yajima, 2020) in the R Programming Language (R Core Team, 2020) to construct a Bayesian estimator of trees >22.0-cm DBH that potentially contained at least one cavity suitable for nesting wood ducks in Northern Minnesota. Our primary reason for using a Bayesian approach was to incorporate estimates of sampling uncertainty associated with both our P occurrence estimates and FIA estimates. Estimating overall uncertainty was complicated in this case because the logistic cavity model was applied to each FIA estimate and FIA estimates were generated from a sampling design, but we only had estimates of total trees and sampling error by summary group (vs. plot-level counts). We explored treating estimates of total cavities as the product of two independent random variables (i.e., the probability of a tree having a suitable cavity x the number of trees in that subgroup) and using the Delta method (Goodman, 1960; Seber, 2002, pp. 7–9) to compute an approximate variance. However, the assumption of independence likely was not entirely valid when summing variances across groups, which could have resulted in variance estimates that were negatively biased. Thus, we chose to use a Bayesian approach to compute the variance of our cavity-stem estimates because this approach could provide () that was closer to the truth in this complex estimation problem. We acknowledge our Bayesian approach might result in positively biased variance estimates because we could not account for the FIA sampling design and the likely covariance among FIA estimates (subgroupings). We believed this was better than presenting variance estimates that were too conservative with respect to estimating var (Y), which when making comparisons would lead us to conclude that important changes in abundance had occurred when actually differences might have represented sampling error.
We conducted our analysis at three spatial scales [i.e., study area, three ecological subsections that intersected the study area, the Laurentian Mixed Forest (ecological province 212)] over six FIA analysis periods (1977, 1990, 2000–2004, 2005–2009, 2010–2014, and 2015–2019). There appeared to be sufficient data to estimate the abundance of suitable stems for the individual tree species American basswood (Tilia americana), bigtooth aspen (Populus grandidentata), Northern red oak (Quercus rubra), paper birch (Betula papyrifera), quaking aspen (Populus tremuloides), red maple (Acer rubrum), sugar maple (Acer saccharum), and yellow birch (Betula alleghaniensis). It was necessary to aggregate the 22 less common tree species into “other hardwoods” and “softwoods” classes, which comprised 12 and 10% of the stems measured in the study area (Zlonis et al., 2020), respectively, so that the abundance of these two groups also could be estimated. We also aggregated tallies of the eight most common tree species into late- (i.e., American basswood, Northern red oak, sugar maple), mid- (i.e., red maple, yellow birch), and early-successional species (i.e., bigtooth aspen, paper birch, quaking aspen) for an additional analysis that facilitated an improved understanding of how cavity abundance may change with successional stages of forests in Minnesota.
We started by fitting a Bayesian version of the logistic cavity model described by Zlonis et al. (2020), which was used to estimate the mean probability E(p) of a tree >22.0-cm DBH containing ≥1 cavity suitable for nesting wood ducks given covariates for species, health status (i.e., live healthy, live-health Impacted, dead), and DBH (>=22.0 cm). We expanded the species groupings to include “other hardwoods” and “softwoods” in addition to eight common tree species; otherwise the model was similar to that of Zlonis et al. (2020). We used flat priors for the logistic-cavity model but restricted starting values to being drawn from a multivariate-normal distribution using estimated regression coefficients () and their variance-covariance matrix (Σ) from a frequentist version of the same model. We used flat priors for two reasons. First, we did not have prior information or beliefs regarding the parameter of interest or its statistical distribution. Second, we wanted to emulate our frequentist approach to estimating the abundance of suitable stems, so used a Bayesian approach to improve our estimate of the var(), which had multiple sources of uncertainty. Using flat priors is equivalent to fitting our cavity model using a frequentist approach (glm fnc).
We then used the resulting regression equation to estimate E(p) for each observation in each FIA dataset (from three spatial scales) based on species, health status, and mid-point of DBH bins (5-cm bins starting at 22.0 cm, with the largest bin having a midpoint of 81 cm). Each observation reflected FIA estimates of total trees by species, health status, DBH bin (≥22.0 cm), FIA evaluation cycles, and ownership class (public vs. private timberland). We treated FIA estimates of total trees in each subgrouping as independent random variables γ having negative binomial distributions with p and r hyper-parameters defined using the following equations:
where μ = FIA estimate of tree abundance in each subgrouping, σ2 = var(FIA estimate), r = dispersion parameter, and p = probability of success in a Bernoulli trial.
We then defined estimated cavities E(Cav) for each subgrouping as the product of E(P) and γ. We used three Monte Carlo chains with 5,000 iterations each, a burn-in of 800, and thinning rate = 3 to construct the posterior distributions for E(p) and E(Cav). We evaluated convergence using the Gelman–Rubin statistic and traceplots. We also compared and Σ from our Bayesian and frequentist models as a quality-control measure. Likewise, we compared point estimates of total cavity trees from the Bayesian model to point estimates derived using our frequentist model applied to FIA estimates of total trees. Finally, we computed mean values and 85% credible intervals (CrIs) based on the posterior distributions of E(Cav) for each spatial scale and various subgroupings of species, health status, FIA evaluation cycle, and ownership type.
Conversion of abundance estimates to density estimates
The density of suitable stems is a more meaningful metric than abundance when comparing available nesting substrate among geographic areas of different spatial extent. Therefore, we converted estimates of the latter to densities of suitable stems [i.e., estimated number of suitable stems / estimated land area (ha) classified as timberland by FIA] for geographic areas of interest during each evaluation cycle (Table 1). We chose to estimate the area of timberland using FIA EVALIDator (U.S. Department of Agriculture Forest Service, 2022) rather than GIS because the associated metric stem abundance was estimated using that tool.
The uncertainty associated with the estimated area of timberland using EVALIDator in conjunction with that associated with abundance estimates probably was greater than the uncertainty associated with the latter parameter. Thus, temporal changes in density may not be as detectable as those of abundance. Therefore, we focused on estimates of abundance when examining temporal changes in the availability of cavity stems within particular geographic areas and those of density when comparing our results with other studies.
Plausibility of results
Because we used a new analytical approach to estimate the abundance of stems with suitable cavities and FIA sampling methodology changed somewhat during the analysis period, we examined results for evidence of spurious estimates and general plausibility (e.g., whether temporal changes in estimated cavity abundance were as expected under a scenario of forest succession). We also identified potential sources of biases, and when possible, the likely direction and extent of these biases.
Generating plausible results under our approach relied in part on making realistic assumptions. Unfortunately, it is not possible to test many of the assumptions that were necessary for our analysis. For example, it was impossible to determine whether proportions of stems with suitable cavities in each species-health status-DBH bin measured in the Cavity Survey were representative of the geographic areas, ownership types, and FIA cycles under consideration because the former survey was not conducted during earlier periods. However, it is possible to examine support for Assumption 1 using estimates of the mean percentage of public timberland in these potentially suitable age classes and Assumption 6 using estimates of the land area in public and private ownership at each spatial scale of interest during each FIA inventory (Table 1).
Results
Temporal changes in the abundance and density of stems suitable for nesting wood ducks
The estimated abundance (Figures 2A–C) and density (Table 2) of suitable stems of all species aggregated generally increased at the three spatial scales examined during the period of analysis, with the lowest estimated density occurring at the ecological-province scale and greatest at the study-area scale during corresponding time periods. Density estimates ranged from 0.90 suitable stems/ha at the ecological-province scale during the 1977 inventory to 1.90 stems / ha at the study-area scale during the 2015–2019 evaluation cycle.
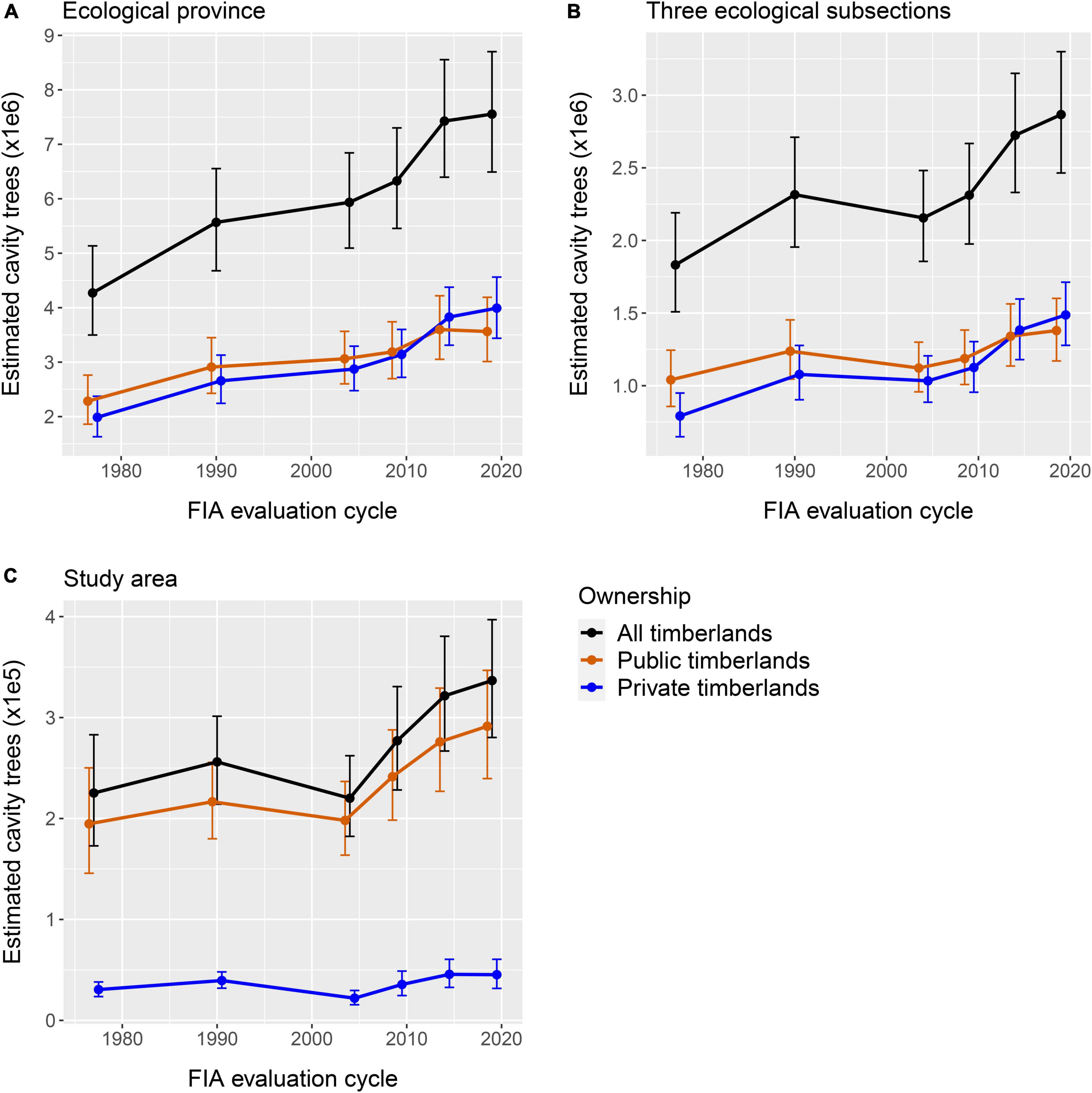
Figure 2. (A–C) The three spatial scales in MN, United States in which the abundance of stems with cavities suitable for nesting wood ducks was estimated using tree-cavity data from and Forest Inventory and Analysis data. (A) Is the study area in which cavity data were collected, (B) is the three ecological subsections the intersect with the study area, and (C) is the ecological province in which the study area occurred.
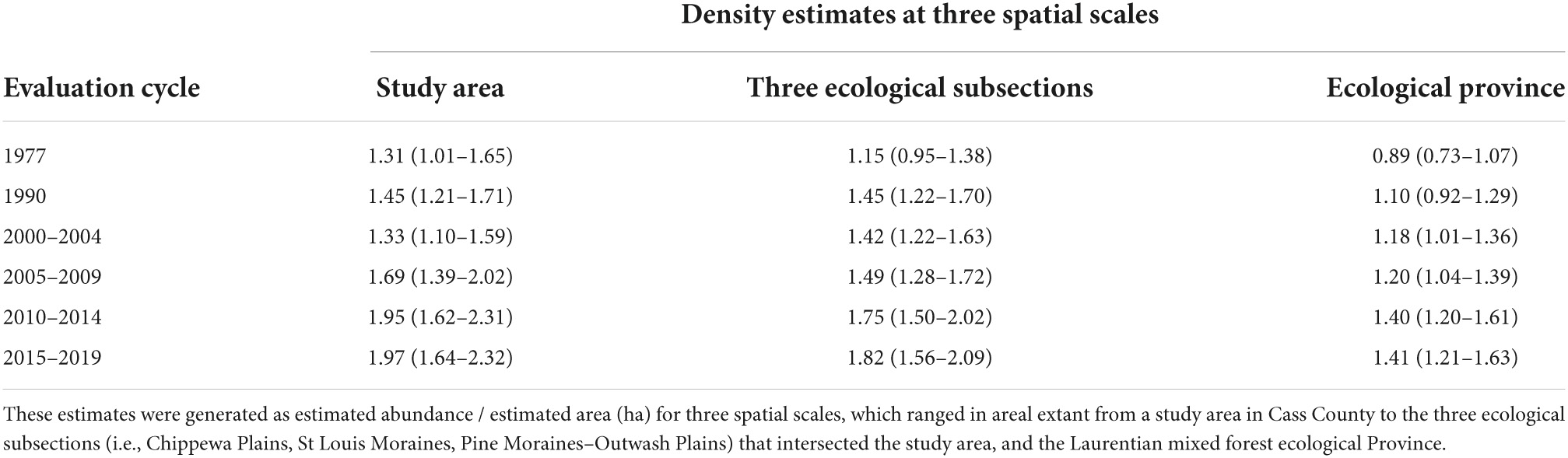
Table 2. Estimates of the density (85% Credible Intervals) of tree stems with cavities suitable for nesting wood ducks in the forested portion of Minnesota during six forest inventory and analysis evaluation cycles.
Interestingly, there were differences among the spatial scales regarding the manner in which the abundance and density of suitable stems increased during the analysis period. Specifically, there were 50.8, 57.5, and 72.3% increases in the point estimates of suitable stem densities at the study-area, three-ecological-subsection, and ecological-province scales, respectively, between 1977 and 2015–2019. Further, the abundance of suitable stems at the ecological-province scale increased in a consistent manner between the 1977 and 2010–2014, and then was approximately stable between 2010–2014 and 2015–2019. Abundance of suitable stems generally increased at the three-ecological-subsection and study-area scales during the period of analysis, but there were anomalous peaks during the 1990 cycle, followed by decreases during the 2000–2004 cycle, then increases during the remainder of the analysis period (Figures 2A–C).
The temporal changes of estimated abundance of suitable cavities on public and private lands were similar at the two larger spatial scales during the period of analysis. In contrast, cavity-stem abundance at the study area scale was relatively stable over time on private land, but generally increased on public land beginning during the 2000–2004 cycle (Figures 2A–C).
Abundance estimates for individual trees species and successional groups
Our results indicate that temporal variation occurred in the abundance of stems of relatively common species (i.e., American basswood, sugar maple, Northern red oak, red maple, yellow birch, quaking aspen, bigtooth aspen, paper birch), as well as the 22 less common tree species aggregated into “other hardwoods” and “softwoods” classes, that had cavities suitable for nesting wood ducks (Supplementary Table 1). Important similarities and differences in the temporal changes in estimated abundance of suitable stems of these common species at the spatial scales examined are as follows.
The abundance of suitable American basswood, sugar maple, and Northern red oak stems generally increased at all scales examined during the period of analysis. However, suitable Northern red oak stems decreased slightly in abundance at the two larger spatial scales after the peak occurring during the 2000–2014 evaluation cycle. Suitable red maple and yellow birch stems increased in abundance at the two larger spatial scales between the 1977 and 2015–2019 cycle. At the study area scale, however, red maple peaked in abundance during the 2010–2014 cycle and then declined substantially. Yellow birch peaked in abundance during the 1990 inventory, had an estimate of zero suitable stems during the 2000–2004 cycle, and was approximately stable during the 2005–2009 to 2010–2019 cycle. The highest abundance of suitable quaking aspen stems at the two larger spatial scales occurred during the 1990 inventory, then decreased and was approximately stable between the 2000–2004 and 2015–2019 cycles. Suitable stems of this species generally decreased at the study area scale between the 1977 and 2000–2004 cycles, then increased slightly until the end of the analysis period. The abundance of suitable bigtooth aspen stems generally increased at the two larger spatial scales during the analysis period. However, the abundance of this species at the study area scale was approximately stable between the 1977 and 2000–2004 cycles, peaked during the 2005–2009 cycle, decreased during the 2010–2014 cycle, and then increased. Suitable paper birch stems increased in abundance at the two larger scales from the 1977 inventory until the 2000–2004 cycle, and then was approximately stable until the end of the analysis period. At study area scale, suitable paper birch stems increased in abundance from 1977 until the peak during the 2000–2004 cycle, and then was approximately stable until the end of the analysis period.
There also was temporal variation in the abundance of suitable stems in each successional class, but these changes sometimes were not consistent among spatial scales (Figures 3A–C). The estimated abundance of suitable stems of late-successional species (i.e., American basswood, Northern red oak, sugar maple) increased from 1977 through 2015–2019 at all spatial scales examined but appeared to stabilize at the ecological-province scale after 2010–2014. The abundance of suitable mid-successional species (i.e., red maple, yellow birch) stems increased throughout the analysis period at the two larger spatial scales, but at the study-area scale increased until 2010–2014 and then decreased slightly. The abundance of suitable early successional species (i.e., bigtooth aspen, paper birch, quaking aspen) stems peaked during 1990 at the two larger spatial scales and during 1977 at the study-area scale. Estimates of suitable early successional stems decreased slightly after the 1990 peak and then were somewhat stable at the two larger spatial scales, but decreased relatively markedly from the 1977 peak until 2000–2004 and then increased at the study-area scale.
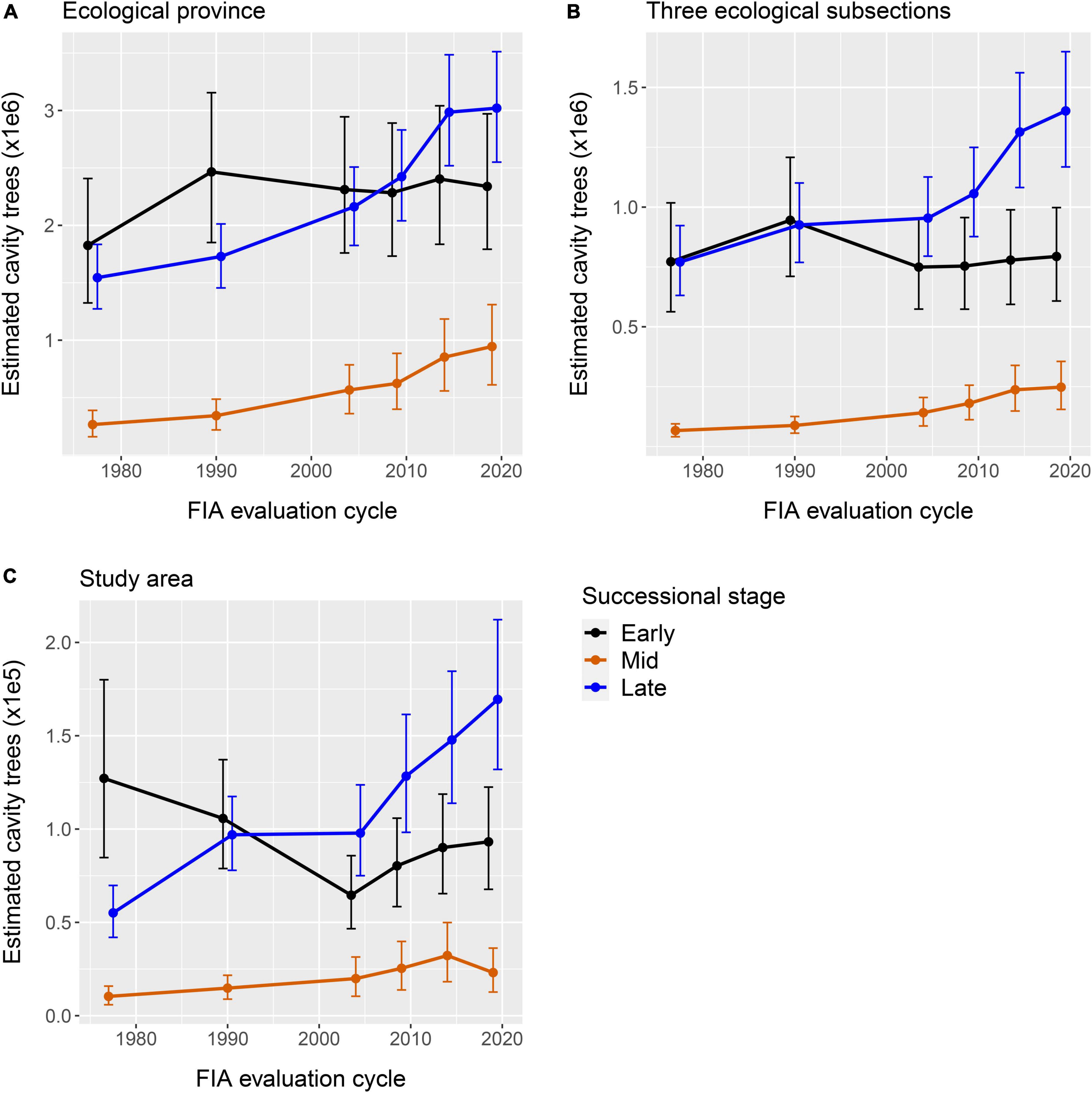
Figure 3. Estimates (85% CrIs) of the abundance of tree stems (all species aggregated) which were suitable for nesting wood ducks in (A) a North Central MN, United States study area, (B) three ecological subsections that intersected the study area, and (C) the ecological province that contained the study area.
Health status
The estimated abundance of suitable stems of all health-status classes exhibited temporal changes, but that of live-health impacted stems was greater than those of the two other health-status classes at all spatial scales and during corresponding evaluation cycles (Supplementary Table 2). Similarly, the estimated abundance of suitable dead stems was greater than that of live-healthy stems except at the two largest spatial scales during the 1977 cycle.
Generally, the abundance estimates of suitable stems in the live-health impacted class temporally increased at all spatial scales, with lowest estimates occurring within the ecological-province spatial scale during the 1990 cycle. At the two smaller scales, the lowest estimates of suitable live-health impacted occurred during the 2000–2004 cycle. The estimated abundance of suitable live-healthy stems increased at all spatial scales examined between the 1977 and 1990 cycles, but then became somewhat stable during the remainder of the analysis period. The estimated abundance of dead stems with suitable cavities was approximately stable from the 1990 cycle onward at all spatial scales.
Plausibility of results
Most of our results appear plausible, with the width of 85% CrIs decreasing as both the spatial scale of the area examined and number of FIA plots increased (Supplementary Tables 1, 2). However, a few estimates of the abundance of suitable stems, as well as the characteristics of temporal change of some estimates, appear anomalous (e.g., abundance estimates during one evaluation cycle that were markedly different than those of immediately preceding and following cycles). Many questionable abundance estimates are associated with less common species, the smallest spatial scale examined, and the abundance of dead and live-health impacted stems during the 1977 and 1990 FIA inventories. Specific examples of questionable results are (1) the estimated abundance of zero suitable yellow birch stems at the study-area scale during 2000–2004 is unexplainably low, given that the estimates for such stems were >3000 during 1990 and 2005–2009, (2) the estimated abundance of Northern red oak at the study-area scale during 2000–2004 cycle was substantially lower than those from preceding and following cycles, (3) the estimated abundances of American basswood at the three-ecological-subsection scale during 1990 and bigtooth aspen at the study-area scale during 2005–2009 were higher than estimates from preceding and following cycles, (4) substantial decreases in the abundance of live-health impacted stems following the 1977 inventory at the two largest scales examined and after the 1990 inventory at the study-area scale, and (5) the abundance estimates of suitable dead stems at the two largest spatial scales were exceptionally low during 1977.
Discussion
Temporal changes in the abundance and density of stems suitable for nesting wood ducks
Our quantitative approach used data from a local survey of tree cavities and FIA to generate plausible estimates of the abundance of stems suitable for nesting wood ducks, as well as the associated 85% CrIs, at both this and greater spatial scales (i.e., the three ecological subsections and ecological province containing the study site) and to different time periods. This extrapolation provided insight regarding the temporal dynamics of suitable stem abundance in the forested portion of Northern Minnesota. For example, our results suggest that the abundance of stems with suitable cavities increased at the three spatial scales examined between the 1977 and 2015–2019 FIA. We attribute these increases in part to the advancing of forest succession in Northern Minnesota (see Kilgore and Ek, 2013), as evidenced by the general increase in abundance of suitable stems of mid- and late successional tree species, a decrease in the abundance of suitable stems of early successional species, and an increase in the estimated abundance of suitable live-health impacted stems (see Hale et al., 1999).
Two characteristics of the temporal variation in abundance and density of suitable stems are particularly relevant to the understanding of how these forest attributes might change in different situations. First, the greatest density of suitable stems occurred at the study-area and the lowest at the ecological-province scales during corresponding evaluation cycles, but the greatest percentage of increase occurred at the ecological-province scale and lowest at the study-area scale. The apparently greater population growth rate of suitable stems at the spatial scale with lowest density during the 1977 inventory suggests that cavity density can increase from the past conditions that were associated with low density. However, the lower rate of increase of suitable-stem density in the spatial scale with the greatest initial density suggests that there may be some maximum density of suitable stems that can occur in the forested portion of Northern Minnesota. Second, the estimated abundance of suitable stems on public and private lands increased in a similar manner at the two largest spatial scales examined, but temporally diverged at the study-area scale beginning during the 2000–2004 cycle; i.e., the abundance of suitable stems on public lands increased but was approximately stable on private lands during the same period. Although results for private land at the study-area scale should be viewed with caution because of small sample sizes (see below), it also may be that ownership-based differences in cavity density were influenced by associated differences in site productivity and land management practices. In general, managed landscapes have fewer cavities than do the unmanaged (e.g., Andersson et al., 2018), but sample size limitations did not permit an examination of how management differences among each public land administrator (USDA Forest Service, State, County) and private landowners influenced cavity density in our study area.
Abundance estimates for individual trees-species, successional, and health-status groups
As would be expected in a geographic area in which the areal extent of old forests increased (Peters et al., 2021), there generally were increases in the abundance of suitable stems of late successional tree species (i.e., American basswood, Northern red oak, sugar maple), both individually (Supplementary Table 1) and as a successional group, during the period of analysis (Figures 3A–C). A similar increase occurred with the mid-successional species (i.e., red maple, yellow birch) group, but there were species-specific differences in the temporal change in abundance. It is predictable that the greatest abundance of suitable early successional stems occurred during the earlier evaluation cycles, when the forests in Minnesota ostensibly were younger. The persistence of a relatively moderate abundance of these stems until the end of the analysis period, along with an increase in the areal extent of old (i.e., ≥90 years) aspen and birch stands in Minnesota between 2003 to 2018 (Peters et al., 2021), initially appear encouraging from the perspective of wood duck population management because quaking aspen (Gilmer et al., 1978) and bigtooth aspen (Nagel, 1969) were their most frequently used species of nest tree at two study sites in Northern Minnesota during the 1960s. It should be noted that a variety of tree species also have been used as nesting substrate (Bellrose and Holm, 1994), some of which commonly occur in types of old forest (i.e., lowland hardwoods, Northern hardwood, oak ≥120 years) that increased in areal extent within Minnesota during 2003 to 2018 (Peters et al., 2021). Unfortunately, it is not known which species are selected, avoided, or used in proportion to availability as nesting substrate in many geographic areas.
Characteristics of the temporal changes in abundance of suitable stems in each health-status group provide some insight regarding how cavity availability varied at each spatial scale during the analysis period. More specifically, the abundance of suitable live-health impacted stems was greater than those of either dead and live-healthy classes during all evaluation cycles, the former continued to increase at the two largest spatial scales examined, and the latter two have remained relatively stable at most spatial scales since the 1990 inventory. We suggest that the increasing difference in abundance between suitable live-health impacted and dead stems during the analysis period is attributable to the former persisting longer and accumulating over time as forests in Minnesota increased in age.
Comparison of our abundance and density estimates with independent predictions
Our finding that the abundance of suitable cavity stems in Northern Minnesota increased between 1977 and 2015–2019 is largely consistent with the prediction that an increase would occur in the Eastern United States during an overlapping period (i.e., 1952 to 2020; Soulliere, 1990). Despite not including the effects of timber harvesting in their simulations of future conditions, the density of suitable stems that Denton et al. (2012b) predicted would occur in Northern Minnesota during 2008 and 2018 (from <0.25 to 0.25–0.49 suitable cavity trees / ha in different ecoregions and during different years) still were substantially less than our range of estimates during overlapping time periods (i.e., 1.21 to 1.63 and 1.41 to 1.90 stems / ha during 2005–2009 and 2015–2019, respectively; Table 2). Further, our density estimates increased at all spatial scales between 2005–2009 and 2015–2019 but increased more slowly between the 2010–2014 and 2015–2019 cycles whereas Denton et al. (2012b) predicted the density of suitable stems would be approximately stable in much of Northern Minnesota during 2008 and 2018. Interestingly, Soulliere (1990) predicted that a decline in cavity stems would begin in the Eastern United States after 2020.
We predominantly attribute differences in density estimates we generated to the predictions of Denton et al. (2012b) to two of their approaches. First, tallies of dead stems and the influence of stem health-status on P occurrence were not included in the simulation of Denton et al. (2012b). Health status is an important predictor of P occurrence (Zlonis et al., 2020) and a substantial proportion of suitable stems in the forested portion of Minnesota were classified as live-health impacted and dead (Supplementary Table 2). Second, Denton et al. (2012b) used some occurrence values that differed substantially from corresponding species- and DBH-classes in our study area. For example, the occurrence values for DBH classes ≥30-cm of a common and important cavity species in North central Minnesota, quaking aspen (i.e., 0.018 to 0.129; Zlonis et al., 2020), were much greater than the estimate of 0.002 for all DBH classes ≥28 cm of this species in central Wisconsin (Denton et al., 2012b). Thus, applying this occurrence value from central Wisconsin to North central Minnesota likely contributed to an under-predicted density of suitable stems in the latter location.
Several other differences between our approach and that used by Denton et al. (2012b) may have contributed to our somewhat disparate results, including (1) our use of data from only FIA plots classified as timberland in analyses, whereas Denton et al. (2012b) used data from forest land plots; (2) our use of slightly different values of the minimum and maximum cavity dimensions and heights to assess suitability (Zlonis et al., 2020) than did Denton et al. (2012b); (3) our tallying of stems ≥22.0 cm DBH while Denton et al. (2012b) included those ≥28.0 cm DBH in their survey; and (4) Denton et al. (2012b) restricted their analysis to plots within 0.5 km of wetland types frequented by cavity-nesting ducks, whereas we had no such restrictions because wood duck broods have moved substantially greater distances between nest trees and water (e.g., Ball, 1973; Ryan et al., 1998). Most of these differences are minor and do not fully explain the substantial differences in the point estimates of the density of suitable cavities generated by the two studies. For example, restricting our analyses to only timberland (i.e., excludes reserved forest and “other” unproductive lands) excluded only about 11% of forest land (MDN, unpublished data); there were relatively small occurrence values for stems 22.0–28.0 cm DBH (Zlonis et al., 2020); and a relatively high proportion of the landscape of Northern Minnesota appeared to be suitable nesting habitat in close proximity to water (Denton et al., 2012b, p. 427). Some of the unsuitable nesting habitat in that area overlapped with the locations of lakes, bogs, and extensive conifer habitats.
Plausibility of results
Most of the estimates of abundance of stems that have suitable nesting cavities and associated uncertainty generated using our quantitative approach were plausible. However, a few of our results appeared to be anomalous. Below we describe the two most likely sources of biases that contributed to anomalous estimates, two protocols that may have contributed to minor biases, and the direction and extent of any biases when possible.
First, caution should be used when viewing results for spatial scales and tree species for which sample sizes were limited (e.g., yellow birch at the study-area scale). Only 4–11 plots were measured on privately owned timberlands during each FIA evaluation at the study-area scale because only 10% of timberland of stand ages in which suitable nesting cavities were likely to occur (i.e., ≥50 years for aspen and birch, ≥80 years for other forest types) was in this ownership class. In contrast, 45–55 plots on public ownerships that met this age and forest criteria were measured during each FIA evaluation at the study area scale, as were hundreds of FIA plots on older timberland in both public and private ownership at the two largest spatial scales during each evaluation. Thus, sample sizes of FIA plots appeared to be sufficient for analyses for both public and private timberland at the largest two spatial scales as well as for public lands at the study area spatial scale. Second, the estimated abundance of standing dead stems, and ultimately that of suitable stems, probably was negatively biased during the 1977 inventory because of the manner in which dead trees were tallied. Stems of that health-status class had relatively high occurrence values for seven tree species (Zlonis et al., 2020). The inclusion of dead, down stems probably was not completely offset by the omission of stems dead >3 years in FIA tallies. The estimated volume of live stems on timberland was positively biased by about 6% during the 1990 cycle (Miles et al., 2007) because a model-assisted, two-phase design applied to a subset of undisturbed plots. Thus, we suspect that the 1990 estimates of abundance for suitable stems was similarly biased.
Two field protocols of FIA may have contributed to minor undercounts of stems with suitable cavities. First, only stems that had <45° lean were tallied in FIA. We are not aware of any documentation of the influence of tree lean on cavity use by wood ducks. Second, only stems ≥1.37 m in height were tallied in FIA, but wood ducks may nest in cavities as low as 0.6 m in height (Strom, 1969). Admittedly, most cavities used by wood ducks have been substantially greater in height than 1.37 m (e.g., Bellrose and Holm, 1994). It is unlikely that the variables tree species and DBH were sources of bias because both were classified and measured, respectively, in the same manner both by the Cavity Survey and FIA. We are not aware of any variability in sample or plot designs or measurement protocols that may have occurred during the 2000–2019 evaluation cycles, except for the use of two tree damage attributes used during different portions of this period [see Randolph et al. (2021) for descriptions of changes in FIA tree damage codes].
Recommendations
Denton et al. (2012a,b) developed valuable procedures for predicting the location and spatial extent of wood duck nesting habitat at the landscape and regional spatial scales, but it may be necessary to alter several of their approaches to reduce the likelihood of generating underestimates of suitable stem abundance and density. We recommend three ways to improve these predictions. First, we suggest the exercise of caution regarding sample size needs and the spatial scales to which these data may be extrapolated. For example, we examined many attributes of >7800 stems over multiple field seasons in a 254,000-ha study area (Zlonis et al., 2020), but the 85% CrIs generated using these and FIA data from this site were somewhat greater than those associated with the two larger spatial areas examined. It is possible to increase the precision of abundance estimates by extrapolating occurrence values to FIA tallies collected over greater spatial areas, but biases could have been introduced if pertinent forest attributes varied substantially over the expanded area [e.g., high spatial variation of the effects of various tree damages (see Morin et al., 2016) on cavity formation across the geographic area of inference]. We recommend that occurrence values from local study sites not be extrapolated to a greater spatial extent than that of the ecological province in which such study sites occur, at least until further research identifies the spatial scales to which such estimates can be extrapolated reliably. Researchers considering increasing their geographic area of inference should be aware that the sampling regime of FIA plots may vary among adjoining states (i.e., frequency of plot visits, number of plots per-unit area; Scott et al., 2005). Second, researchers considering using FIA data from both the older periodic (generally prior to 1999) and more contemporary annual inventories (generally beginning during or after 1999) in a single analysis should be aware that differences exist in the sample and plot designs and measurement protocols of these two types of inventories. Third, future analyses and simulations should include the influence of stem health-status and the abundance of dead stems. If P occurrence data are sparse, it may be preferable to aggregate data within DBH classes of similar species rather than among DBH classes within individual species.
Despite the knowledge gained in numerous examinations of wood duck nesting habitat, there are several information gaps that should be addressed to improve the understanding of the nesting ecology of wood ducks and our ability to monitor the abundance of potential nest sites. It is particularly important to develop a better understanding of the influence of temporal and successional changes in forest attributes (e.g., abundance of suitable stems, tree-species composition, health status of stems) on the breeding population of this species. This includes determining whether wood ducks will select (e.g., Aebischer et al., 1993) nest trees of mid- or late successional tree species during the contemporary period more frequently than during periods when a younger forest dominated the landscape or that suitable stems of early successional species have remained sufficiently common and spatially distributed to receive frequent use. Similarly, there is need to determine whether stems of any specific health-status class are selected for nesting. The time of persistence for stems of each health class that have suitable cavities (sensu Edworthy et al., 2012) should be estimated in different ecoregions. There is a need to better understand how nest-site selection is influenced by the spatial proximity of suitable brood habitats, as well whether demographic vital rates (e.g., nest success, hen survival) are associated with this selection. It has been recommended that the interaction among survival, recruitment, immigration, and emigration be examined to better understand how influence the local populations of wood ducks, particularly in areas in which the recruitment of females from artificial nest boxes is insufficient to maintain the population segment that uses these structures (Hepp et al., 2020; Croft et al., 2022), but such an investigation should also examine whether these vital rates are influenced by the proportion of landscape comprised of suitable nesting habitat (i.e., mature deciduous forest). The nest webs (Martin et al., 2004) and influence of changes in tree and cavity characteristics on occupancy by different wildlife species (Edworthy et al., 2018) in different forest types of the North Central United States should be investigated. Researchers should ascertain whether the methodology FIA has used to tally stems with cavities in California, Oregon, and Washington is a tractable approach to estimating the abundance and temporal change of these structures and can be expanded to other regions. Providing such information could inform forest management decisions (e.g., identifying the most appropriate stems to reserve as leave trees in timber harvest units), improve current monitoring systems, and update existing wood duck habitat suitability indices (Sousa and Farmer, 1984). Further, our approach can be adapted to model habitat availability for other species that are associated with cavities or other forest attributes that can be associated with variables measured in FIA.
Data availability statement
The raw tree-cavity data and downloaded FIA data supporting the conclusions of this article will be made available by the authors, without undue reservation.
Author contributions
JB and EZ performed data collection. JG performed data analysis. MN queried the FIA database. All authors contributed to the conception and design of the study and wrote this manuscript.
Funding
This project was funded by the Wildlife Restoration (Pittman-Robertson) Program (Grant # F21AF02430-1) and the Minnesota Department of Natural Resources.
Acknowledgments
We thank S. Mortenson (Leech Lake D.R.M.), J. Watts (Cass County M.I.S.), S. Dunham, J. Rickers, and T. Tisler (U.S. Department of Agriculture Forest Service) for logistical support. We also thank C. Scharenbroich and R. Wright for technical assistance. B. Coleman, T. Duquette, C. McCarty, H. North, T. Roerick, and E. Spry provided assistance with field data collection. J. Lawrence for provided reviews of research proposals. B. Davis, J. Davis, J. Eadie, L. Elliott, S. Hillard, R. Kaminski, and four reviewers provided suggestions that improved this manuscript.
Conflict of interest
The authors declare that the research was conducted in the absence of any commercial or financial relationships that could be construed as a potential conflict of interest.
Publisher’s note
All claims expressed in this article are solely those of the authors and do not necessarily represent those of their affiliated organizations, or those of the publisher, the editors and the reviewers. Any product that may be evaluated in this article, or claim that may be made by its manufacturer, is not guaranteed or endorsed by the publisher.
Supplementary material
The Supplementary Material for this article can be found online at: https://www.frontiersin.org/articles/10.3389/ffgc.2022.967060/full#supplementary-material
References
Aebischer, N. J., Robertson, P. A., and Kenward, R. E. (1993). Compositional analysis of habitat use from animal radio-tracking data. Ecology 74, 1313–1325. doi: 10.2307/1940062
Andersson, J., Domingo Gómez, E., Michon, S., and Roberge, J. M. (2018). Tree cavity densities and characteristics in managed and unmanaged Swedish boreal forest. Scand. J. For. Res. 33, 233–244. doi: 10.1080/02827581.2017.1360389
Ball, I. J. Jr. (1973). Ecology of duck broods in a forested region of north-central Minnesota. Minneapolis, MN: University of Minnesota.
Bechtold, W. A., and Patterson, P. L. (2005). The enhanced forest inventory and analysis program–national sampling design and estimation procedures, Vol. 80. Asheville, NC: U.S. Department of Agriculture Forest Service, Southern Research Station.
Bellrose, F. C., and Holm, D. J. (1994). Ecology and management of the wood duck. Mechanicsburg, PA: Wildlife Management Institute and Stackpole.
Berg, E. C., and Eadie, J. M. (2020). An experimental test of information use by wood ducks (Aix sponsa): External habitat cues, not social visual cues, influence initial nest site selection. Behav. Ecol. Sociobiol. 74, 1–16. doi: 10.1007/s00265-020-02904-2
Boyer, R. L. (1974). A survey of wood duck nest sites and brood rearing habitat on the Shiawassee National Wildlife Refuge. Mount Pleasant, MI: Central Michigan University.
Cleland, D. T., Freeouf, J. A., Keys, J. E., Nowacki, G. J., Carpenter, C. A., and McNab, W. H. (2007). Ecological subregions: Sections and subsections for conterminous United States. General technical report WO-76D. Washington, DC: U.S. Department of Agriculture Forest Service [Map on CD-R0M]. doi: 10.2737/WO-GTR-76D
Croft, G. D., Kaminski, R. M., Wiggers, E. P., Gerard, P. D., and Yarrow, G. K. (2022). Box-nesting wood ducks and black-bellied whistling ducks in Coastal South Carolina. J. Southeast. Assoc. Fish Wildl. Agencies 9, 89–95.
Denton, J. C., Roy, C. L., Soulliere, G. J., and Potter, B. A. (2012a). Change in density of duck nest cavities at forests in the north central United States. J. Fish Wildl. Manage. 3, 76–88. doi: 10.3996/112011-JFWM-067
Denton, J. C., Roy, C. L., Soulliere, G. J., and Potter, B. A. (2012b). Current and projected abundance of potential nest sites for cavity-nesting ducks in hardwoods of the north central United States. J. Wildl. Manage. 76, 422–432. doi: 10.1002/jwmg.271
Edworthy, A. B., Trzcinski, M. K., Cockle, K. L., Wiebe, K. L., and Martin, K. (2018). Tree cavity occupancy by nesting vertebrates across cavity age. J. Wildl. Manage. 82, 639–648. doi: 10.1002/jwmg.21398
Edworthy, A. B., Wiebe, K. L., and Martin, K. (2012). Survival analysis of a critical resource for cavity-nesting communities: Patterns of tree cavity longevity. Ecol. Appl. 22, 1733–1742. doi: 10.1890/11-1594.1
Fan, Z., Shifley, S. R., Spetich, M. A., Thompson, F. R. III, and Larsen, D. R. (2003). Distribution of cavity trees in midwestern old-growth and second-growth forests. Can. J. For. Res. 33, 1481–1494. doi: 10.1139/x03-068
Gilmer, D. S., Ball, I. J., Cowardin, L. M., Mathisen, J. E., and Riechmann, J. H. (1978). Natural cavities used by wood ducks in north-central Minnesota. J. Wildl. Manage. 42, 288–298. doi: 10.2307/3800266
Goodman, L. A. (1960). On the exact variance of products. J. Am. Stat. Assoc. 55, 708–713. doi: 10.1080/01621459.1960.10483369
Hale, C. M., Pastor, J., and Rusterholz, K. A. (1999). Comparison of structural and compositional characteristics in old-growth and mature, managed hardwood forests of Minnesota, USA. Can. J. For. Res. 29, 1479–1489. doi: 10.1139/x99-076
Haramis, G. M. (1975). Wood duck (Aix sponsa) ecology and management within the green-timber impoundments at Montezuma National Wildlife Refuge. Ithaca, NY: Cornell University.
Hepp, G. R., and Bellrose, F. C. (2013). Wood duck (Aix sponsa), version 2.0. The birds of North America. Ithaca, NY: Cornell Lab of Ornithology. doi: 10.2173/bna.169
Hepp, G. R., Gitzen, R. A., and Kennamer, R. A. (2020). Relative importance of vital rates to population dynamics of wood ducks. J. Wildl. Manage. 84, 320–330. doi: 10.1002/jwmg.21792
Kilgore, M. A., and Ek, A. R. (2013). Minnesota forest age class distribution, 2011. Minn. For. Res. Notes 295, 1–3.
Martin, K., Aitken, K. E., and Wiebe, K. L. (2004). Nest sites and nest webs for cavity-nesting communities in interior British Columbia, Canada: Nest characteristics and niche partitioning. Condor 106, 5–19. doi: 10.1093/condor/106.1.5
Miles, P. D., Jacobson, K., Brand, G. J., Jepsen, E., Meneguzzo, D., Mielke, M. E., et al. (2007). Minnesota’s forests 1999–2003 (Part A). Resour. Bull. NRS-12A. Newtown Square, PA: U.S. Department of Agriculture Forest Service, Northern Research Station, 92. doi: 10.2737/NRS-RB-12
Morin, R. S., Pugh, S. A., and Steinman, J. (2016). Mapping the occurrence of tree damage in the forests of the northern United States. General Technical Report NRS-GTR-162. Newtown Square, PA: U.S. Department of Agriculture, Forest Service Northern Research Station, 1–19. doi: 10.2737/NRS-GTR-162
Nagel, R. E. (1969). Predation on eggs in simulated nests and tree cavity abundance in wood duck nesting habitat. Master’s thesis. Ames, IA: Iowa State University.
Nelson, M. D., and Roy, C. (2012). “Estimating tree cavity distributions from historical FIA data,” in Proceedings of the Moving From Status to Trends: Forest Inventory and Analysis (FIA) Symposium 2012, eds R. S. Morin and G. C. Liknes (Newtown Square, PA: U.S. Department of Agriculture Forest Service, Northern Research Station.[CD-ROM]), 199–203.
Nielsen, C. L. R., Gates, R. J., and Zwicker, E. H. (2007). Projected availability of natural cavities for wood ducks in southern Illinois. J. Wildl. Manage. 71, 875–883. doi: 10.2193/2006-134
Peters, E. B., Wilson, D. C., Edgar, C. B., and Ek, A. R. (2021). Old forest extent and changes in minnesota 2003-2018. Saint Paul, MN: Minnesota Forestry Research Notes, 311.
R Core Team (2020). R: A language and environment for statistical computing. R foundation for statistical computing. Available online at: https://www.R-project.org/ (accessed December 4, 2020).
Randolph, K. C., Dooley, K., Shaw, J. D., Morin, R. S., Asaro, C., and Palmer, M. M. (2021). Past and present individual-tree damage assessments of the US national forest inventory. Environ. Monit. Assess. 193, 1–18. doi: 10.1007/s10661-020-08796-z
Ridlehuber, K. T. (1980). Wood duck production and habitat use. Doctoral Dissertation. Texas: Texas A&M University.
Robb, J. R. (1986). The importance of nesting cavities and brood habitat to wood duck production. Master’s thesis. Columbus, OH: The Ohio State University.
Ryan, D. C., Kawula, R. J., and Gates, R. J. (1998). Breeding biology of wood ducks using natural cavities in southern Illinois. J. Wildl. Manage. 62, 112–123. doi: 10.2307/3802269
Scott, C. T., Bechtold, W. A., Reams, G. A., Smith, W. D., Westfall, J. A., Hansen, M. H., et al. (2005). “Sample-based estimators used by the forest inventory and analysis national information management system,” in The enhanced forest inventory and analysis program–national sampling design and estimation procedures, eds W. A. Bechtold and P. L. Patterson (Asheville, NC: U.S. Department of Agriculture Forest Service), 43–67.
Smith, W. B. (2002). Forest inventory and analysis: A national inventory and monitoring program. Environ. Pollut. 116, S233–S242. doi: 10.1016/S0269-7491(01)00255-X
Soulliere, G. J. (1990). “Review of wood duck nest-cavity characteristics,” in Proceedings of the 1988 North American Wood Duck Symposium (Columbia, IN: University of Missouri), 153–162.
Sousa, P., and Farmer, A. (1984). Habitat suitability index models: Wood duck. Bailey’s Crossroads, VA: U.S. Department of Interior Fish and Wildlife Service.
Strom, D. W. (1969). A determination and evaluation of what constitutes wood duck brood habitat in the Nelson-Trevino bottoms of the upper Mississippi refuge. Saint Paul, MN: U.S. Department of Interior Fish and Wildlife Service Division of Wildlife Refuges (Region 3) Final Report.
Su, Y., and Yajima, M. (2020). R2jags: Using R to run ‘JAGS’. R package version 0.6-1. Available online at: https://CRAN.R-project.org/package=R2jags (accessed December 4, 2020).
Thomas, J. W., Anderson, R. G., Maser, C., and Bull, E. L. (1979). Snags. Wildlife habitats in managed forests: The blue mountains of Oregon and Washington. Agric. Handbook 553, 60–77.
U.S. Department of Agriculture Forest Service (1982). An analysis of the timber situation of the United States 1952–2030. Rome: Forest Resources Report, 23.
U. S. Department of Agriculture Forest Service (1986). North Central Forest Experiment Station Forest Inventory and Analysis field manual: Missouri, 1986; Minnesota, 1986. St Paul, MN, 107.
U.S. Department of Agriculture Forest Service (2014). Forest Inventory and Analysis national core field guide, Volume 1: Field data collection procedures for Phase 2 plots. Version 6.1. Available online at: https://www.fia.fs.usda.gov/library/field-guides-methods-proc/docs/2014/Core%20FIA%guide_6_1.pdf> (accessed November 1, 2016)
U.S. Department of Agriculture Forest Service (2022). Forest Inventory and Analysis program, forest inventory EVALIDator web-application. Version 1.8.0.01. Available online at https://apps.fs.usda.gov/fiadb-api/evalidator (accessed July 11, 2022).
Waldstein, A. L. (2012). An inexpensive camera system for monitoring cavity nests. J. Field Ornithol. 83, 302–305. doi: 10.1111/j.1557-9263.2012.00379.x
Zlonis, E. J., Berdeen, J. B., and St-Louis, V. (2020). Forest inventory attributes predict the occurrence of cavities suitable for nesting by Wood Ducks (Aix sponsa). For. Ecol. Manage. 461:117951. doi: 10.1016/j.foreco.2020.117951
Zlonis, E. J., Deo, R., and Berdeen, J. B. (2022). LiDAR and multispectral imagery predict the occurrence of tree cavities suitable for a cavity-nesting duck. Remote Sens. Ecol. Conserv. 8, 191–207. doi: 10.1002/rse2.236
Keywords: Aix sponsa, FIA, Forest Inventory and Analysis, Minnesota, nesting, tree cavity, wood duck
Citation: Berdeen JB, Nelson MD, Zlonis EJ and Giudice JH (2022) Temporal change in abundance of potential nesting cavities for wood ducks (Aix sponsa) in Northern Minnesota, United States. Front. For. Glob. Change 5:967060. doi: 10.3389/ffgc.2022.967060
Received: 12 June 2022; Accepted: 12 August 2022;
Published: 21 September 2022.
Edited by:
John M. Eadie, University of California, Davis, United StatesReviewed by:
J. Brian Davis, Mississippi State University, United StatesMatt Dyson, Ducks Unlimited Canada, Canada
Gary Hepp, Auburn University, United States
Richard Kaminski, Clemson University, United States
Copyright © 2022 Berdeen, Nelson, Zlonis and Giudice. This is an open-access article distributed under the terms of the Creative Commons Attribution License (CC BY). The use, distribution or reproduction in other forums is permitted, provided the original author(s) and the copyright owner(s) are credited and that the original publication in this journal is cited, in accordance with accepted academic practice. No use, distribution or reproduction is permitted which does not comply with these terms.
*Correspondence: James B. Berdeen, amltLmJlcmRlZW5Ac3RhdGUubW4udXM=
†These authors have contributed equally to this work