- 1Facultad del Medio Ambiente y Recursos Naturales, Universidad Distrital Francisco José de Caldas, Bogotá, Colombia
- 2Programa Ciencias Básicas de la Biodiversidad, Instituto de Investigación de Recursos Biológicos Alexander von Humboldt, Bogotá, Colombia
- 3Departamento de Ciencias Biológicas, Facultad de Ciencias Naturales, Universidad Icesi, Cali, Colombia
Previous studies have shown that environmental conditions and plant attributes determine biomass stocks and productivity across multiple tropical forests. However, it is less clear how these factors act at local scales. We evaluated how the spatial variation of soil resource availability (soil nutrient and water content) and plant functional traits determine species biomass stocks and productivity in a Colombian tropical dry forest, based on spatially explicit soil sampling and an intensive plant trait characterization of 89 species in three 1-ha permanent plots with similar climate and floristic composition. Within each plot, we measured nine soil variables and ten functional traits and quantified forest biomass stocks and productivity for 10,161 individual trees in a period of 3 years. The soil resources where species were located and their functional traits had coordinated effects on the spatial distribution of forest biomass stocks across the plots. The highest biomass stocks were concentrated on nutrient-rich soils with low water availability and were dominated by conservative species with dense tissues and low hydraulic failure risk, probably because they are able to better cope with water limitation. Most of the remaining forest biomass stocks were found in nutrient-poor soils with high water availability and were dominated by acquisitive species. Sites with nutrient-rich soils and low water availability increased biomass survival but also mortality; however, the presence of conservative species in these sites also increased biomass survival, decreased mortality, and led to biomass accumulation, probably because their strong and hydraulically secure tissues are able to deal with water limitation for nutrient absorption during dry seasons. Interestingly, soil resources and functional traits had no effects on biomass recruitment. We conclude that strong coordinated effects of soil resources and functional traits determine local biomass processes of tropical dry forests with a central role of conservative trait species types, whereby these species promote community assembly and functioning but are also vulnerable to potential changes in water availability. Thus, conservation and restoration actions should pay special attention to soil and plant functional trait trade-offs to improve management practices in these threatened forests.
1. Introduction
The functioning of tropical ecosystems and their ecological processes result from multiple interactions between biotic and abiotic factors (Poorter et al., 2017). One of the most important consequences of these interactions is the central role of biomass net primary productivity (from now on biomass productivity) on tropical forest carbon cycling (Malhi, 2012; Lohbeck et al., 2015). Whereby, to understand the mechanisms influencing processes related to biomass productivity is crucial to predict future scenarios of climate change, but also how they interact and operate in different ways across local and regional scales (Wennekes et al., 2012; Chave, 2013).
At regional scales, there is ample evidence showing that environmental conditions are the main drivers of biomass stocks and biomass productivity in tropical forests. In general, studies have shown that water availability is one of the main drivers of biomass (e.g., rainfall, Toledo et al., 2012; Poorter et al., 2017), given that it assures high rates of nutrient assimilation to support growth. Soil conditions have also been found to play important roles in forests biomass processes (Quesada et al., 2012) because high nutrient availability is usually related to the high biomass productivity of forest species (van der Sande et al., 2017a,b). In a similar way, plant functional traits have also been shown to be important drivers of biomass stocks and biomass productivity in tropical ecosystems because the way species are functionally shaped determines their ability to use water and nutrients (Finegan et al., 2015; Poorter et al., 2017; Phillips et al., 2019). For example, species characterized by conservative traits (e.g., low specific leaf area and water conduction capacity, thicker fiber cell walls and high wood, leaf and conduits density) are expected to dominate nutrient-poor soils, where they usually have higher survival rates and biomass stocks because their denser tissues make them more resistant to soil constraints (Reich, 2014; Finegan et al., 2015; Jager et al., 2015; Poorter et al., 2017). In contrast, species having acquisitive traits (e.g., high specific leaf area and water conduction capacity, thinner fiber cell walls and low wood, leaf and conduits density) are expected to dominate in fertile soils where no restrictions lead them to increase growth and have positive net biomass due to fast turnover rates typical of this side of the functional trait spectrum (Reich, 2014; Finegan et al., 2015; Jager et al., 2015: Poorter et al., 2017). Thus, it is important to understand how environmental conditions and traits interact in driving biomass stocks and biomass productivity to predict the effects of future environmental changes on forest functioning.
There is evidence showing that environmental conditions and plant traits are the most important drivers of biomass productivity across multiple tropical forests. However, there is a general agreement that driver effects can change at the local level, although few studies have shown this. This study aims to fill that gap and contribute to a better understanding of how local effects of soil resources and functional traits define plant coexistence and important ecosystem processes such as net primary productivity in tropical dry forests. These ecosystems are especially interesting due to water limitation exhibited during the dry season, which has critical impacts on plant species under potential environmental changes. Additionally, tropical dry forests are threatened due to the impacts of anthropic activities and current efforts of conservation and restoration need scientific information that is currently scarcely available for a successful implementation of their strategies (e.g., active restoration with climate-smart species).
Furthermore, drivers of biomass stocks and biomass productivity, measured based on demographic changes of species, can vary between wet and dry forests of the same region due to differences in environmental limitations (Lohbeck et al., 2013; van der Sande et al., 2016). For example, contrary to general expectations for a dry tropical forest in Brazil, soil fertility had a negative effect on biomass productivity and species with conservative traits increased biomass stocks and biomass productivity (Prado-Junior et al., 2016). Moreover, at finer scales, it is possible that in an ecosystem with similar environmental conditions, drivers of biomass stocks and biomass productivity may operate differently because of particular spatial constraints such as local topography, which determine soil resources availability (Roy and Singh, 1994; Roy, 1996; Pulla et al., 2016; Li et al., 2017) and spatial distribution of functional strategies (Méndez-Alonzo et al., 2013; Méndez-Toribio et al., 2017). For example, in a Bolivian tropical moist forest, functional traits did not influence biomass productivity and soil structure, and water availability had no effect on mortality despite its effects on biomass growth and recruitment. This may be due to functional traits having more important effects at regional than local scales due to the wide variation of environmental conditions in different ecosystems, which may filter specific traits with higher impacts on biomass productivity (van der Sande et al., 2017a). Additionally, in a Guyanese tropical moist forest with nutrient-poor soils, where conservative traits were expected to dominate due to soil nutrient limitation, soil P availability increased biomass stocks and biomass productivity, while acquisitive traits increased these processes due to higher nutrient absorption improving biomass gain (van der Sande et al., 2017b). These findings highlight the complexity of mechanisms that underlie biomass stock and biomass productivity in tropical forests and reveal the need for finer scaling to corroborate hypotheses that have been tested at larger scales.
Here, we performed an intensive field survey of spatially explicit soil resource availability (soil water content and nutrients) for three 1-ha permanent plots in a tropical dry forest (TDF), and we sampled functional traits of 89 TDF tree species to evaluate the effects of spatial variation in soil resources and functional traits on biomass stocks defined in this study as the standing biomass, and biomass productivity, treated here as biomass changes in time due to survival, recruitment, and mortality. Specifically, we assessed two main questions: (a) How do soil resource availability and functional traits, separately and in combination, determine species biomass stocks at the local scale in a dry forest? and (b) How do soil resource availability and functional traits drive species biomass productivity in a dry forest?
We expected that both soil resource availability and functional traits would determine biomass stocks and biomass productivity due to the pervasive effect of resource gradients on species selection and the overcoming of resource limitations of species via functional traits. However, the strength of coordination between soil resource availability and functional traits may result in contrasting soil resources—functional trait trade-offs at the local scale. Specifically, on poor soils, with low nutrient availability and water constraints, we expected plants with conservative traits (e.g., dense tissues with small and dense xylem conduits) to better cope with soil constraints, resulting in high biomass stocks and positive biomass productivity (high survival and recruitment and low mortality). In contrast, species occurring in rich soils should exhibit acquisitive traits (e.g., soft tissues with large and sparse xylem conduits) to take advantage of available resources, resulting in high biomass stocks and positive biomass productivity (high survival and recruitment and low mortality). Outside of this soil-trait coordination, species should have lower biomass stocks and productivity because their functional design is not efficient to hold up high rates of survival and recruitment and low mortality.
2. Materials and methods
2.1. Study site and plots
This study was carried out in a tropical dry forest (TDF) located in two private nature reserves in the Magdalena valley in Colombia (5°5″ N, 74°48′ W) which have been preserved for nearly 60 years (González-M et al., 2019; Supplementary Figure 1). The reserves are located in the same area and share the same environmental conditions, with an annual mean temperature of 27.6°C, total annual precipitation of 1,391 mm, and two regular annual dry seasons of two or more months with less than 100 mm per month (González-M et al., 2018). We used three 1-ha TDF permanent plots established between 2013 and 2015. All plots were located on soils of sedimentary origin composed of sandstones, claystone, and conglomerates (Gómez et al., 2015) and their topography varied from flat to hilly with slopes between 0 and 45°. Each plot was divided into 10 × 10 m subplots to facilitate sampling and all individuals with DBH (diameter at breast height measured at 1.3 m) ≥2.5 cm were marked and total height (m) and the x-y position within the plot (m) were measured.
2.2. Soil resources
At each plot, soil volumetric water content was measured at predawn (VWCpd) and middle day (VWCmd) in all 121 intersection points of the 10 × 10 m subplots during the same day in the 2019 dry season with a time domain reflectometer (TDR) Fieldscout 350. At each point, we took three volumetric water content records with the TDR and used their average for analyses. The daily change of volumetric water content (ΔVWC) was estimated as the difference between the average of VWCmd and VWCpd. Negative values indicated water deficit and positive values water surplus.
To determine soil nutrient content, 45 soil samples per plot were taken at 20 cm deep and were located around each 1-ha plot in the intersection points of 10 × 10 m (Supplementary Table 1). Each soil sample was oven-dried for 24 h at 40°C and sieved with a 2-mm sieve to remove roots. To determine cation exchange capacity (CEC, cmolc kg–1) and exchangeable bases [Calcium (Ca), Magnesium (Mg), Potassium (K), Sodium (Na), cmolc kg–1], we used the 1NpH7 acetate ammonium method (Lavkulich, 1981). Organic carbon (OC,%) was obtained by the chemical oxidation method of Walkley and Black (1934). All laboratory analyzes were conducted at the Soil Laboratory of the Faculty of Environment and Natural Resources at Universidad Distrital Francisco José de Caldas.
As both types of soil sampling, water, and nutrients, had different sample sizes due to planning and cost constraints, we performed an imputation and interpolation process to fit the soil source at 121 samples (see section Data analysis and Supplementary Table 1).
2.3. Functional traits
We wanted to evaluate the effects of functional traits on biomass stocks and productivity, as well as their relationship with soil resources since plant functional strategies respond to environmental constraints (e.g., nutrients and water availability) and impact ecosystem properties such as biomass productivity (Violle et al., 2007). We, therefore, measured leaf and stem traits of all tree species in three 1-ha permanent plots (89 species, excluding cacti and lianas), related to their acquisition strategy in addition to their response to variations in soil water and nutrient resources. Leaf traits included leaf area (LA, mm2), leaf dry matter content (LDMC, mg g–1), and specific leaf area (SLA, mm2 mg–1) and were selected because they indicate drought and nutrient stress, photosynthetic capacity, and the species investment in tissues (Lavorel and Garnier, 2002; Wright et al., 2004; Díaz et al., 2016). We also measured stem traits related to tree growth rate and mechanical resistance (Chave et al., 2009) including wood density (WD, g cm–3) and wall thickness of wood fibers (FWT, μm), an anatomical property of wood related to its resistance. Stem traits related to hydraulic conductivity, water storage, and cavitation resistance (Méndez-Alonzo et al., 2012; Díaz et al., 2016) included water content at maximum capacity (WCmax, kg kg–1) as an indicator of stem water storage capacity, xylem potential hydraulic conductivity (Kp, kg m–1 s–1 MPa–1) as an empirical measurement of the xylem capacity to transport water from roots to leaves using the hydraulically weighted vessel diameter and the Hagen-Poiseuille formula (Méndez-Alonzo et al., 2012). We also measured the pit aperture area of the vessels of wood (PA, μm2), which allows water flow within vessels and is related to cavitation risk, vessel density (VD, vessels mm–2) or the number of vessels per cross-sectional area of wood as an indicator of stem resistance to cavitation and vessel area (VA, μm2) or average area of vessels over a cross-section of wood related to water conduction capacity and embolism risk. For leaf traits, we sampled five leaves per individual for 3–12 individuals of each 89 species, and for stem traits, we collected branches of 10–15 cm in length and more ∼2 cm in diameter per sampled individual in the same number of species (Cornelissen et al., 2003; Pérez-Harguindeguy et al., 2013).
2.4. Biomass stocks and productivity
After plots were established (2013–2015), two censuses were performed between 2017 and 2019 to collect information on biomass dynamics of survivors, recruits, and dead trees for the three 1-ha plots. We measured a total of 10,161 individual trees of 89 species in a period of 3 years and used this information to analyze forest biomass stocks and productivity at a species level. The sum of standing biomass of all individuals of each species at plot establishment was considered as the biomass stocks (t ha–1) and was calculated with the type I and II allometric models of tropical dry forests (Alvarez et al., 2012). The biomass productivity of each species was evaluated using four individual parameters: biomass gained by surviving trees or biomass survival (BGS, t ha–1 yr–1), biomass gained by recruited trees or biomass recruitment (BGR, t ha–1 yr–1), biomass lost by tree mortality or biomass mortality (BLM, t ha–1 yr–1) and net biomass changes (NBC, t ha–1 yr–1), which represents the net annual increase or decrease of biomass stocks per species. BGS was obtained from the difference between the biomass of all surviving trees of the same species registered in the last censuses and the initial biomass of the same trees at the time of the establishment of the plots, divided by the annual difference between censuses; BGR was estimated as the biomass of all new trees of the same species registered in each census extracting the biomass calculated for the same individuals with a 2.5 cm of DBH to avoid overestimation (Talbot et al., 2014), and dividing by the annual difference between census; BLM as the difference between the biomass of all dead trees of the same species obtained of the last census when the tree was still alive and the biomass calculated for the same tree with 2.5 cm of DBH (Talbot et al., 2014), divided by the annual difference between census; and NBC as the annual change of species biomass obtained of the sum between biomass survival (BGS) and biomass recruitment (BGR), minus biomass mortality (BLM) of the same species.
2.5. Data analyses
To evaluate the effect of soil resources and functional traits on biomass stocks for each species, we first carried out an imputation process with the 45 sampled points of soil variables (Stekhoven and Bühlmann, 2012) to obtain a total of 121 sampling points per plot in all intersection points of the 10 × 10 m subplots (Supplementary Table 1). We then performed an interpolation process (Pebesma, 2004; Hiemstra et al., 2009) for all the variables measured in the soil on the 1-ha surface of the three plots using the ordinary kriging method (OK) due to its best performance (Supplementary Table 2 and Supplementary Figure 2). From the surfaces created for each soil variable, we obtained the soil values for all tree individuals present in each plot. We then performed multiple principal component analyses (PCA) to obtain two variation axes of soil resources and functional traits (Revelle, 2020) and we used these PCA axes to calculate bivariate kernel probability densities (BKPD) for each species scaled by its biomass stocks (Carmona et al., 2016). The results of the BKPD on soil resources were considered as the soil resources space and the results of the BKPD and on functional traits as the functional traits space. To test to which extent these variations were coordinated, we conducted a pairwise correlation of Pearson between BKPD of biomass stocks for soil resources and functional traits.
Finally, to test how biomass productivity was influenced by soil resources, functional traits, and biomass stocks of species, we related survival, recruitment, mortality, and net biomass changes (BGS, BGR, BLM, and NBC) to BKPD of biomass stocks on soil resources, BKPD of biomass stocks on functional traits and the interaction between these using generalized linear models (GLM) of the Gamma family and the link function “log” with the “stats” package (R Core Team, 2022). We performed all analyses in R version 4.0.0 (R Core Team, 2022).
3. Results
3.1. Influence of soil resources and functional traits on species biomass stocks
Biomass stocks showed a wide variation for BKPD along the soil resources and functional traits spaces which are represented by the first two PCA axes (Figure 1). The first PCA axis of the soil resources space (Figure 1A, 35.0% of the explained variance) indicated differences in nutrient availability from low (left side of the PC1 axis) to high (right side of the PC1 axis) cation exchange capacity and base cation availability (Mg, Ca). The second PCA axis (28.42% of the explained variance) showed differences in soil volumetric water content and nutrients. Similarly, negative values in the PC2 axis were related to low daily changes in the volumetric water content and high Na, while high values in this axis were associated with high K and OC content, and high soil volumetric water content at predawn and middle day (VWCpd and VWCmd, respectively). The highest species biomass stocks (hotspots in the BKPD20%; Figure 1A) were associated with low soil volumetric water content but high soil nutrient availability. However, the 50% threshold probability density of biomass stocks (BKPD20–50%) showed two opposing concentration points, one in high nutrients and low water content, and the other in high water and low nutrient content (cold spots in the BKPD20–50%; Figure 1A).
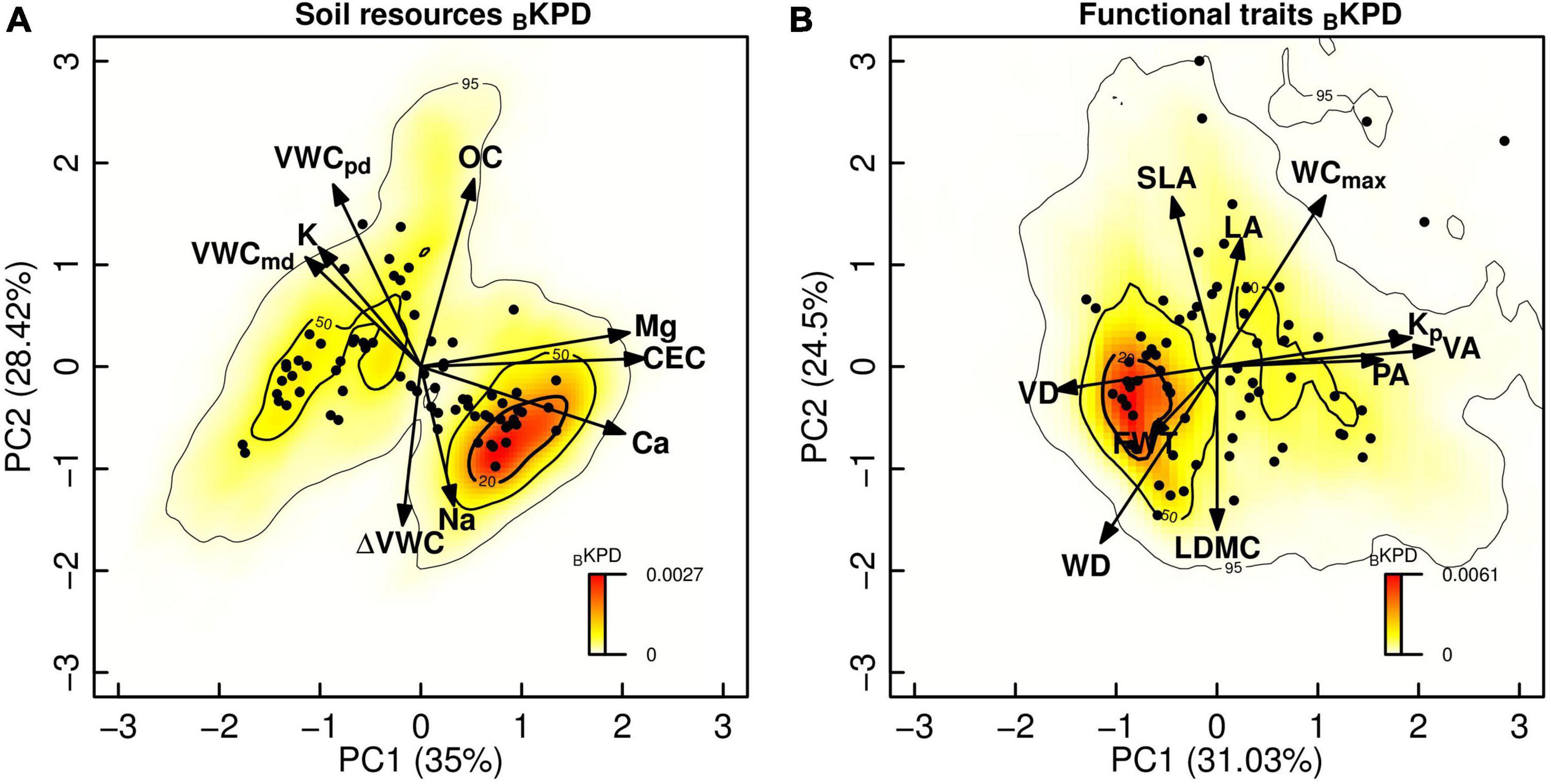
Figure 1. Bivariate kernel probability densities of the biomass stocks of TDF tree species (BKPD) showing the spatial variation of soil conditions (A) and functional trait combinations (B). Soil variables: predawn soil volumetric water content (VWCpd,%), midday soil volumetric water content (VWCmd,%), daily soil volumetric water content change (ΔVWC,%), cation exchange capacity (CEC, cmolc kg–1), exchangeable bases [Calcium (Ca), Magnesium (Mg), Potassium (K), Sodium (Na), cmolc kg–1] and organic carbon (OC,%). Functional traits: leaf area (LA, mm2), leaf dry matter content (LDMC, mg g–1), specific leaf area (SLA, mm2 mg–1), wood density (WD, g cm–3), fiber wall thickness (FWT, μm), water content at maximum capacity (WCmax, kg kg–1), and xylem potential hydraulic conductivity (Kp, kg m–1 s–1 MPa–1), pit aperture area (PA, μm2), vessel area (VA, μm2), vessel density (VD, vessels mm–2). Thresholds of 20, 50, and 95% indicate the probability density accounted for one species across the soil resources space (A) and functional trait space (B) scaled by its biomass stock. The species biomass dominance is shown from hot (22.1 t ha–1) to cold spots (0.0001 t ha–1).
Regarding the functional traits space (Figure 1B), in the first PCA axis (31.03% of explained variance), differences in water conduction between species were evident, with values on the left of the PC1 axis indicating a high density of narrow vessels, and values on the right indicating high hydraulic potential conductivity (Kp) and large vessel and pit areas. The second PCA axis (24.5% of explained variance) showed differences in tissue investment, where negative values of the PC2 axis were associated with high wood density (WD), high leaf dry matter content (LDMC), and thicker fiber walls (TFW), and positive values with species with large and thin leaves (high LA and SLA, respectively), and high xylem water content (WCmax). The highest species biomass stocks were associated with dense tissues and low water conduction capacity (hotspots in the BKPD20%; Figure 1B). Like soil resources, the 50% threshold probability density of biomass stocks in the functional traits space (BKPD20–50%) showed two opposing concentration points. On one side we found species with dense tissues and low water conduction capacity, while on the other we found species with soft tissues and high-water conduction capacity (cold spots in the BKPD20–50%; Figure 1B).
Lastly, we found a significant positive correlation between BKPD of biomass stocks for soil resources and functional traits spaces (ρ = 0.9, P < 0.001; Figure 2), whereby biomass of dominant species was associated with dense tissues, and low water conduction capacity, and were found on soils with low water content and high nutrients (hot spots in the BKPD20%; Figure 2). Species dominance decreased toward soft plant tissues with a high capacity of water conduction and soils with high water content and low nutrients (cold spots in the BKPD20–50%; Figure 2). Additionally, this correlation showed that some combinations of functional traits and soil resources did not occur and therefore did not contribute to the biomass stocks of this forest. For example, there were no species with dense tissues and low water capacity conduction on soils with high water and low nutrient content (upper-left part of Figure 2) or species with soft tissues and high-water conduction capacity on soils with low water content and high nutrients (lower-right part of Figure 2). These results were consistent when we evaluated the BKPD at the individual plot level (Supplementary Figure 3).
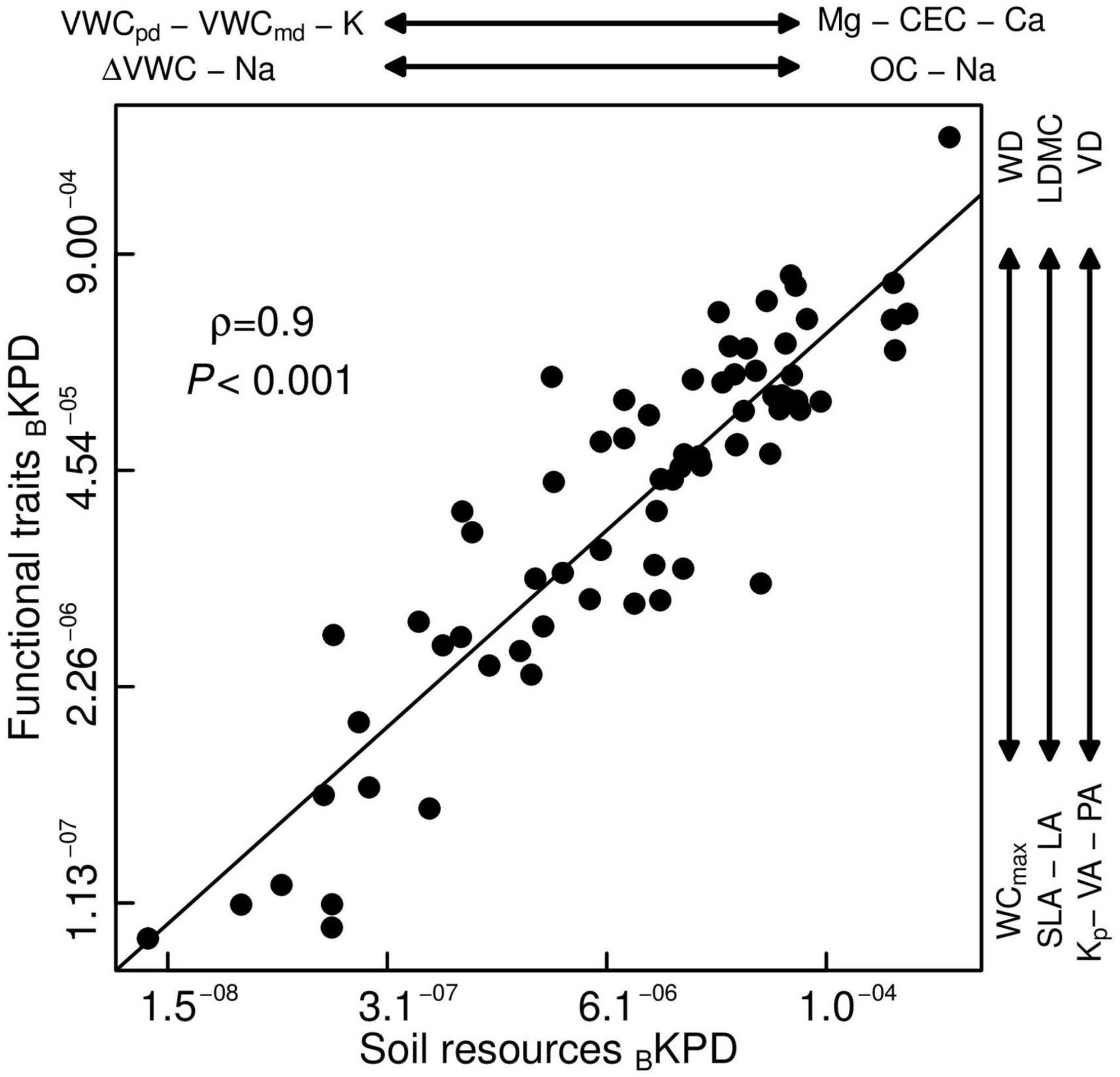
Figure 2. Pearson’s pairwise correlation of bivariate kernel probability densities of the TDF species biomass stocks (BKPD) between soil resources and functional trait spaces. Soil variables: predawn soil volumetric water content (VWCpd,%), midday soil volumetric water content (VWCmd,%), daily soil volumetric water content change (ΔVWC,%), cation exchange capacity (CEC, cmolc kg–1), exchangeable bases [Calcium (Ca), Magnesium (Mg), Potassium (K), Sodium (Na), cmolc kg–1] and organic carbon (OC,%). Functional traits: leaf area (LA, mm2), leaf dry matter content (LDMC, mg g–1), specific leaf area (SLA, mm2 mg–1), wood density (WD, g cm–3), fiber wall thickness (FWT, μm), water content at maximum capacity (WCmax, kg kg–1), and xylem potential hydraulic conductivity (Kp, kg m–1 s–1 MPa–1), pit aperture area (PA, μm2), vessel area (VA, μm2), vessel density (VD, vessels mm–2).
3.2. Effects of soil resources, functional traits, and biomass stocks on biomass productivity
The GLM indicated that BKPD of biomass stocks on soil resources and functional traits spaces had strong effects on biomass survival (BGs, R2 = 0.96), biomass mortality (BLM, R2 = 0.27), and net biomass changes (NBC, R2 = 0.54), but not on biomass recruitment (BGR, Figure 3 and Supplementary Table 3). Both soil resources and functional traits had positive effects on biomass survival, with soils having a greater effect (β = 5.71, Figure 3A) than functional traits (β = 4.32, Figure 3A). Thus, plants with high tissue density and low conduction capacity on rich-nutrient soils with low water content were the species with the highest biomass stocks which tended to promote biomass survival. In contrast, soil resources alone had positive effects on biomass mortality (β = 5.75, Figure 3C), indicating that sites with rich-nutrient soils and low water content, where species with the highest biomass stocks were located, were sites with also a high biomass loss. However, the negative effect of the interaction between soil resources and functional trait spaces (β = −12.31, Figure 3C) indicated that if plant species with high tissue density and low conduction capacity were present in these sites, biomass mortality drastically decreased. Interestingly, net biomass changes were only explained by the interaction between soil resources and functional trait spaces (β = 12.27, Figure 3D), which indicates that only sites with rich-nutrient soils and low water content, dominated by plant species with high tissue density and low conduction capacity with the highest biomass stocks, result in long-term biomass accumulation in this forest. Despite this, neither soil resources space, nor functional traits space, explained biomass recruitment (Figure 3B).
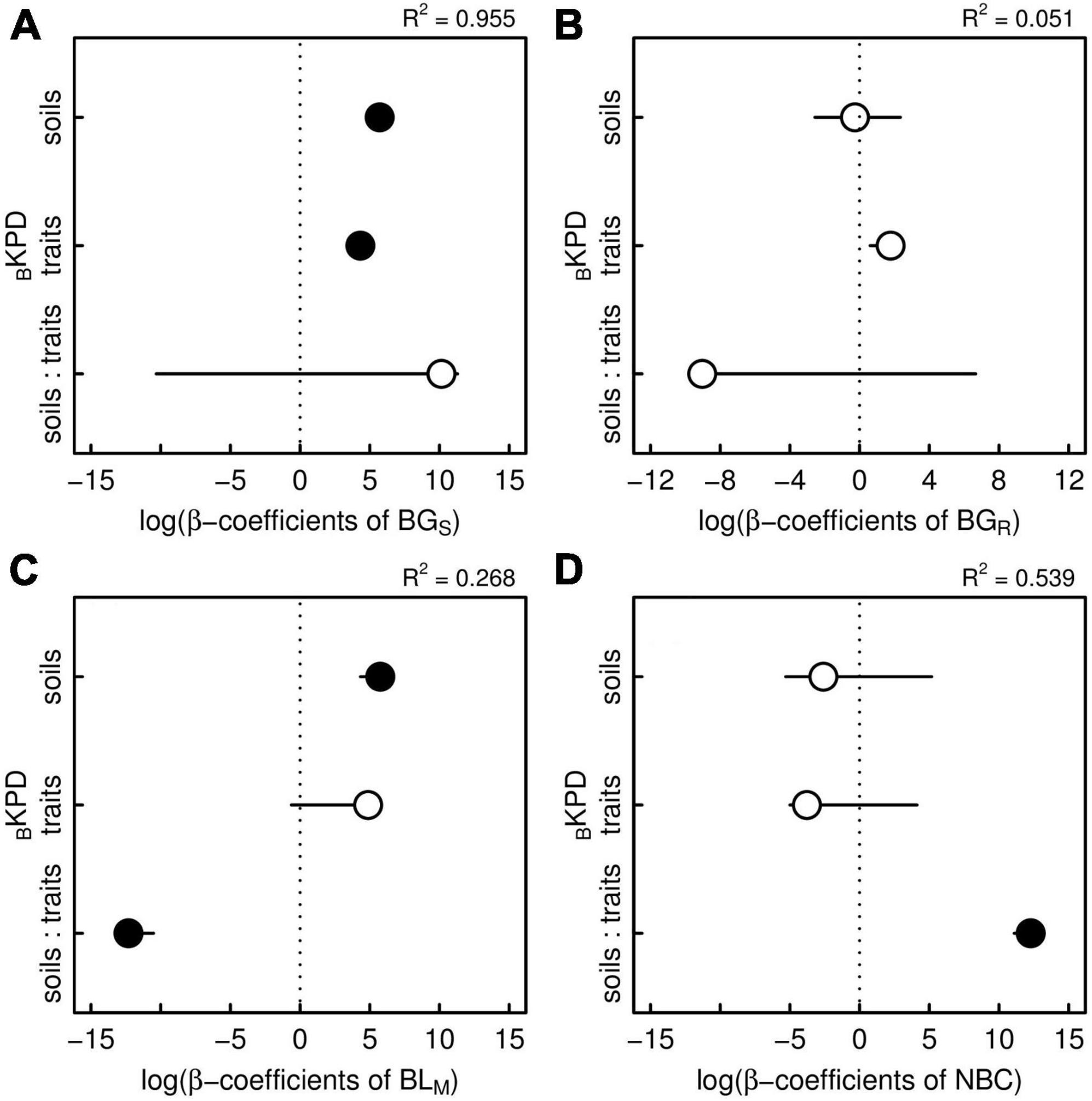
Figure 3. β-coefficients of the generalized linear models (GLM) showing the effects of bivariate kernel probability densities of species biomass stocks (BKPD) based on soil resources (soils), functional traits (traits), and their interaction (soils: traits) on each component of biomass productivity: (A) biomass gained by surviving trees (BGS), (B) biomass gained by recruited trees (BGR), (C) biomass lost by tree mortality (BLM) and (D) net biomass changes (NBC) in t ha–1 yr–1. Significant (closed points) and non-significant (open points) coefficients are shown under a significance level of 0.05.
4. Discussion
We evaluated the effects and coordination of spatial variation in soil resources (nutrients and water) and combinations of plant functional traits on the local distribution of species biomass stocks and biomass productivity (changes in biomass with time). Our results suggest that (1) although soil resources and functional traits exhibited a wide variation at the local level, the highest biomass stocks were found in nutrient-rich soils with low water content, where species with conservative traits were mostly found (Figures 1, 2). Furthermore, most of the remaining biomass stocks were found on nutrient-poor soils with high water content occupied by species with acquisitive traits (Figures 1, 2); (2) both soil resources and functional traits had important controls on biomass productivity. Soil resources strongly determined survival and mortality, but functional traits also defined survival and shaped the response of species to soil conditions to decrease biomass mortality resulting in high biomass accumulation. Interestingly, soil resources and functional traits had no significant effects on biomass recruitment.
4.1. Soil resources and functional traits govern the local distribution of biomass stocks in a tropical dry forest
We had predicted that locally, poor soils (low nutrient availability and low water content) and conservative traits would be associated with higher biomass stocks due to their effects on low growth rates and high tissue investment. Our results support much of this prediction since most of the biomass stocks were on sites with low soil water content and were associated with species with more conservative traits (high WD, Lth, LDMC, and VD). However, these soils had higher, instead of lower, nutrient contents (Figures 1, 2).
At the regional scale, a higher accumulation of aboveground biomass in tropical forests has been associated with high water availability as it promotes plant growth and productivity (Toledo et al., 2011; Malhi, 2012; Poorter et al., 2017; Ali et al., 2019), but the effects of soil nutrients remain unclear as some authors have found either positive (Ali et al., 2019), negative (Toledo et al., 2011), or no significant effects (Poorter et al., 2017). For example, in several TDF around the world, higher biomass stocks were found in wetter sites (Becknell et al., 2012; Corona-Núñez et al., 2018), but the effect of soil fertility showed opposite directions (Prado-Junior et al., 2016; Maia et al., 2020) or was not significant (Corona-Núñez et al., 2018), suggesting this ecosystem is rather limited by drought (Prado-Junior et al., 2016). Nevertheless, our results suggest that at the local scale, biomass stocks increase in sites with low water and high nutrient content (Figure 1). This probably occurs because water scarcity promotes nutrient accumulation due to low leaching (Campo et al., 2000), resulting in higher fertility to support biomass production and accumulation during rainy seasons (Maia et al., 2020); but also water scarcity may impose limitations on nutrient absorption during dry seasons which are successfully supported by slow-growing species that invest higher energy in strong and dense tissues (e.g., leaf and stem) to avoid the effects of drought such as embolism (Markesteijn et al., 2011; Pineda-García et al., 2015). To this extent, it is likely that sites where the greatest biomass accumulates are limited by water scarcity rather than by nutrients.
Unlike soil variables, our results on functional traits coincide with other studies showing that in tropical forests, biomass stocks are highly and positively influenced by tree height, stem diameter, wood density, and certain leaf traits (Baker et al., 2004; Baraloto et al., 2011; Finegan et al., 2015; Poorter et al., 2017). Some studies in dry ecosystems have found similar results. For example, in the Chaco Forest of Argentina, Conti and Díaz (2013) found that total height and wood density were positively correlated with biomass accumulation and in a tropical dry forest in Brazil, Prado-Junior et al. (2016) found higher biomass stocks in stands where conservative species with high wood density and stem diameters dominated. Thus, our results also show that in TDF, higher biomass stocks are governed by conservative species with high WD and LDMC, and low SLA, because these species show a slow growth but higher investment in tissue construction (e.g., more mass storage per unit volume Chave et al., 2009) resulting in a higher biomass accumulation compared to acquisitive species with higher turnover rates, which have been considered as the best contributors to tropical forest productivity (Finegan et al., 2015; Prado-Junior et al., 2016; Poorter et al., 2017). However, we also found that species with acquisitive traits occurred on soils with high water content and low nutrients and concentrated a good part of the remaining biomass (Figures 1, 2). This indicates that although conservative species concentrate the highest amount of biomass stocks at the local scale, other species with more exploitative strategies can dominate if soil resources are available (e.g., high soil water content), contributing to the production and storage of dry forest biomass.
Previous studies have shown the combined effects of functional traits and environmental factors on tropical ecosystem productivity (Conti and Díaz, 2013; Finegan et al., 2015; Prado-Junior et al., 2016; Poorter et al., 2017). At the local scale and within the same forest type, we confirmed the coordinated effects of soil resources and functional traits on the accumulation of biomass (Figure 2). This suggests that in TDF these variables operate at the same time to assemble the community: sites with high nutrient and low water content should be occupied by conservative species with a higher biomass stock, while sites with low nutrients and high-water content are expected to be dominated by acquisitive species with a lower, but important accumulation of biomass. This may be explained by the staggered effect of soil resources which generate stress on plants that respond through their traits (Fonseca et al., 2000; Ordoñez et al., 2009; García-Palacios et al., 2012), affecting their performance and biomass accumulation (García-Palacios et al., 2013; Loiola et al., 2015). Soil resource conditions determine the dominance of species with different strategies (conservative in drier and acquisitive in wetter sites, Markesteijn et al., 2011; Méndez-Alonzo et al., 2013), but turnover rates and biomass stocks depend on these strategies (Finegan et al., 2015; Poorter et al., 2017) whereby conservative species have slow growth, but high biomass accumulation (Prado-Junior et al., 2016), as we found in this study. In this way, the scarcity of water, not of nutrients, forces species with conservative traits to persist in these places, accumulating higher biomass, as opposed to acquisitive species that are more successful in sites with higher water but lower soil nutrient contents and they contribute to a lower, but important biomass accumulation in TDF. Thus, our results suggest that at local scales, soil resource variation and functional traits have coordinated effects that govern the biomass stocks of dry forests.
4.2. Soil resources, functional traits, and biomass stocks determine survival, mortality, and net changes in biomass but not in recruitment
We had predicted that despite differences in environmental requirements and functional strategies, species with conservative and acquisitive traits would have positive biomass productivity (high survival and recruitment and low mortality). However, our results only partially support these predictions, as we found that rich soils with low water content followed by more conservative species had the highest controls on biomass survival, mortality, and net biomass changes but not on biomass recruitment.
Previous studies have shown that at regional scales, water availability is the main driver of biomass productivity in tropical forests (Toledo et al., 2011; Poorter et al., 2017), and soil nutrients have been only poorly or not associated with growth, recruitment, or mortality (Poorter et al., 2017). However, the influence of soil nutrient conditions depends on local biotic and abiotic interactions, as well as specific geological and historical characteristics (Prado-Junior et al., 2016; van der Sande et al., 2017b). Other studies have found that biomass stocks and acquisitive traits directly influence biomass productivity (Finegan et al., 2015; Poorter et al., 2017; van der Sande et al., 2017a). Here, we found that soils with low water and high nutrient contents, where species with the highest biomass stocks were located, had greater effects on biomass survival than species with conservative traits, but both had positive effects, and only where these two characteristics coincided, there was a long-term accumulation of biomass. This is because despite soils being fertile, conservative species were able to deal with water scarcity and resource absorption limitations during the dry season through a high energy investment in building strong tissues, which improves survival and results in a long-term net positive increase in biomass productivity (higher biomass survival than biomass mortality) (Poorter et al., 2008; Markesteijn and Poorter, 2009), compared to other possible strategies that were found in the studied TDF. These results support the findings of Prado-Junior et al. (2016) confirming that TDF biomass growth and accumulation is driven by species with conservative traits but also supports the “green soup or vegetation quantity” hypothesis (Finegan et al., 2015; Prado-Junior et al., 2016) since conservative species also accumulated the highest biomass stocks.
Sites with low water but high nutrient contents with higher biomass stocks influenced biomass mortality; however, the presence of conservative species strongly decreased biomass mortality because they are highly resistant to water scarcity during dry seasons exhibiting low mortality rates. Contrary to our results, previous studies did not find any relationship between environmental variables or functional traits and the mortality of tropical forest tree species (Prado-Junior et al., 2016; Poorter et al., 2017; van der Sande et al., 2017a), but other authors showed that the higher biomass stocks, the higher the mortality rate (Prado-Junior et al., 2016; Poorter et al., 2017) because sites with the highest biomass stocks are dominated by the largest trees, which are more prone to die (Johnson et al., 2016). Our results support these last findings as the biomass mortality increased in sites with high biomass stocks and low water availability, but although the pressure of water scarcity during the dry seasons may impact nutrient absorption and plant functioning (Markesteijn and Poorter, 2009; Markesteijn et al., 2011), conservative species are able to deal with this environmental constraint exhibiting a reduction in the biomass mortality of the community assembly.
Contrary to previous studies showing a negative effect of soil water scarcity on biomass recruitment (van der Sande et al., 2017a), we did not find a significant effect of soil resources and functional traits on biomass recruitment. This is unusual because resource availability is a limiting factor for seedling establishment (Muller-Landau et al., 2002), especially in tropical dry forests highly limited by water availability (Murphy and Lugo, 1986). Moreover, it has been shown that wood density and biomass stocks have negative effects on biomass recruitment (Finegan et al., 2015; Prado-Junior et al., 2016; Poorter et al., 2017; van der Sande et al., 2017a) because larger trees can limit light availability under the canopy or they can promote it through deciduousness (Prado-Junior et al., 2016). This suggests that although it has been shown that soil resources and certain functional traits impose limitations to the establishment of seedlings, it is possible that these processes operate at different spatial or time scales than the ones explored in this study, in addition to other mechanisms such as seed limitation (dispersal and predation, Inman-Narahari et al., 2013) or negative density dependence processes (Wiegand et al., 2009; Clark et al., 2018).
5. Conclusion
We demonstrated that in a single ecosystem with the same environmental conditions, soil resources and functional traits vary spatially at the local scale (1-ha), and both exert coordinated effects on biomass stocks, which accumulated on two extremes. The highest biomass stocks were located on nutrient-rich soils with low water availability dominated by conservative species, which are able to deal with water scarcity limitations due to a high energy investment to build resistant tissues that contribute to a higher long-term biomass accumulation. On the other hand, most of the remaining biomass stocks were located on nutrient-poor soils with high water availability dominated by acquisitive species, which perform well under these conditions and contribute to biomass accumulation despite their fast turnover rates. Moreover, despite nutrient-rich soils with low water content and conservative species being the most important predictors of biomass productivity, the former had the strongest effect on biomass survival and mortality, while the latter improved species response to reduce mortality and increase survival resulting in long-term biomass storage. However, none of the explored variables significantly affected biomass recruitment. This study contributes to a better understanding of how local effects of soil resources and functional traits define plant coexistence and functioning of tropical dry forests via coordinated effects. As conservative species showed the highest effects on biomass stocks and biomass productivity, they should receive special attention under potential climate change scenarios since they dominated soils with low water availability, which may be critical during the dry season. In this way, future conservation and restoration efforts of this highly threatened ecosystem should consider the interactions between soil resources and plant traits, as well as the combinations of functional strategies, to recover the structure of this ecosystem taking into account the importance of conservative species.
Data availability statement
The original contributions presented in this study are included in the article/Supplementary material, further inquiries can be directed to the corresponding author.
Author contributions
BC-R and RG-M: conceptualization, framework development, data analysis, and preparation of the manuscript. CP: collection and organization of the database, review, and editing. FG, RL-C, and VS: collection and organization of the database. All authors contributed to the article and approved the submitted version.
Funding
This study was partially supported by the Inter-American Development Bank Technical Cooperation # ATN/BD-15408-CO. The fieldwork of this study could not have been carried out without the field assistance of all researchers, students, and field assistants who participated in the establishment and censuses of the plots and the collection and processing of leaf and stem traits.
Acknowledgments
We are grateful to Humboldt Institute for handling resources to collect and process data. We are also thankful to the owners of the two private reserves (Cardonal and Jabirú), where we carried out all the fieldwork. We thank Miguel Cadena and Paula Meneses for their help with chemical analyses of soils, Ángela Parrado, Andres Avella, and two reviewers for all their comments that helped to improve the quality of this manuscript.
Conflict of interest
The authors declare that the research was conducted in the absence of any commercial or financial relationships that could be construed as a potential conflict of interest.
Publisher’s note
All claims expressed in this article are solely those of the authors and do not necessarily represent those of their affiliated organizations, or those of the publisher, the editors and the reviewers. Any product that may be evaluated in this article, or claim that may be made by its manufacturer, is not guaranteed or endorsed by the publisher.
Supplementary material
The Supplementary Material for this article can be found online at: https://www.frontiersin.org/articles/10.3389/ffgc.2023.1028359/full#supplementary-material
References
Ali, A., Lin, S., He, J., Kong, F., Yu, J., Jiang, H., et al. (2019). Climate and soils determine aboveground biomass indirectly via species diversity and stand structural complexity in tropical forests. For. Ecol. Manag. 432, 823–831. doi: 10.1016/j.foreco.2018.10.024
Alvarez, E., Duque, A., Saldarriaga, J., Cabrera, K., de las Salas, G., del Valle, I., et al. (2012). Tree above-ground biomass allometries for carbon stocks estimation in the natural forests of Colombia. For. Ecol. Manag. 267, 297–308. doi: 10.1016/j.foreco.2011.12.013
Baker, T. R., Phillips, O. L., Malhi, Y., Almeida, S., Arroyo, L., Dl Fiore, A., et al. (2004). Variation in wood density determines spatial patterns in Amazonian forest biomass. Glob. Change Biol. 10, 545–562. doi: 10.1111/j.1365-2486.2004.00751.x
Baraloto, C., Rabaud, S., Moltos, Q., Blanc, L., Fortunel, C., Hérault, B., et al. (2011). Disentangling stand and environmental correlates of aboveground biomass in Amazonian forests. Glob. Change Biol. 17, 2677–2688. doi: 10.1111/j.1365-2486.2011.02432.x
Becknell, J. M., Kissing Kucek, L., and Powers, J. S. (2012). Aboveground biomass in mature and secondary seasonally dry tropical forests: A literature review and global synthesis. For. Ecol. Manag. 276, 88–95. doi: 10.1016/j.foreco.2012.03.033
Campo, J., Maass, J. M., Jaramillo, V. J., and Martinez Yrizar, A. (2000). Calcium, potassium, and magnesium cycling in a Mexican tropical dry forest ecosystem. Biogeochemistry. 49, 21–36. doi: 10.1023/A
Carmona, C. P., de Bello, F., Mason, N., and Lepš, J. (2016). Traits without borders: integrating functional diversity across scales. Trends Ecol. Evol. 31, 382–394. doi: 10.1016/j.tree.2016.02.003
Chave, J. (2013). The problem of pattern and scale in ecology: What have we learned in 20 years? Ecol. Lett. 16, 4–16. doi: 10.1111/ele.12048
Chave, J., Coomes, D., Jansen, S., Lewis, S. L., Swenson, N. G., and Zanne, A. E. (2009). Towards a worldwide wood economics spectrum. Ecol. Lett. 12, 351–366. doi: 10.1111/j.1461-0248.2009.01285.x
Clark, A. T., Detto, M.,. Muller Landau, H. C., Schnitzer, S. A., Wright, S. J., Condit, R., et al. (2018). Functional traits of tropical trees and lianas explain spatial structure across multiple scales. J. Ecol. 106, 795–806. doi: 10.1111/1365-2745.12804
Conti, G., and Díaz, S. (2013). Plant functional diversity and carbon storage–an empirical test in semi-arid forest ecosystems. J. Ecol. 101, 18–28. doi: 10.2307/23354662
Cornelissen, J. H. C., Lavorel, E., Garnier, S., Díaz, N., Buchmann, D. E., Gurvich, P. B., et al. (2003). A handbook of protocols for standardized and easy measurement of plant functional traits worldwide. Aust. J. Bot. 51, 335–380. doi: 10.1071/BT02124
Corona-Núñez, R. O., Campo, J., and Williams, M. (2018). Aboveground carbon storage in tropical dry forest plots in Oaxaca, Mexico. For. Ecol. Manag. 409, 202–214. doi: 10.1016/j.foreco.2017.11.014
Díaz, S., Kattge, J., Cornelissen, J., Wright, I., Lavorel, S., Dray, S., et al. (2016). The global spectrum of plant form and function. Nature 529, 167–171. doi: 10.1038/nature16489
Finegan, B., Pena-Claros, M., de Oliveira, A., Ascarrunz, N., Bret-Harte, M., Carreno-Rocabado, G., et al. (2015). Does functional trait diversity predict above-ground biomass and productivity of tropical forests? Testing three alternative hypotheses. J. Ecol. 103, 191–201. doi: 10.1111/1365-2745.12346
Fonseca, C. R., Overton, J. M., Collins, B., and Westoby, M. (2000). Shifts in trait-combinations along rainfall and phosphorus gradients. J. Ecol. 88, 964–977. doi: 10.1046/j.1365-2745.2000.00506.x
García-Palacios, P., Maestre, F. T., Bardgett, R. D., and Kroon, H d (2012). Plant responses to soil heterogeneity and global environmental change. J. Ecol. 100, 1303–1314. doi: 10.1111/j.1365-2745.2012.02014.x
García-Palacios, P., Maestre, F. T., and Milla, R. (2013). Community-aggregated plant traits interact with soil nutrient heterogeneity to determine ecosystem functioning. Plant Soil 364, 119–129. doi: 10.1007/s11104-012-1349-6
Gómez, J., Montes, N. E., Nivia, A., and Diederix, H. (2015). Mapa geológico de Colombia 2015. Escala 1:1 000 000, Servicio geológico colombiano. Available online at: https://www2.sgc.gov.co/MGC/Paginas/mgc_1M2015.aspx (Accessed November 1, 2020).
González-M, R., García, H., Isaacs, P., Cuadros, H., López-Camacho, R., and Rodríguez, N. (2018). Disentangling the environmental heterogeneity, floristic distinctiveness and current threats of tropical dry forests in Colombia. Environ. Res. Lett. 13:045007.
González-M, R., Norden, N., Posada, J., Pizano, C., García, H., Idárraga-Piedrahita, A., et al. (2019). Climate severity and land-cover transformation determine plant community attributes in Colombian dry forests. Biotropica 51, 826–837. doi: 10.1111/btp.12715
Hiemstra, P. H., Pebesma, E., Twenhöfel, C., and Heuvelink, G. (2009). Real-time automatic interpolation of ambient gamma dose rates from the Dutch radioactivity monitoring network. Comput. Geosci. 35, 1711–1721. doi: 10.1016/j.cageo.2008.10.011
Inman-Narahari, F., Ostertag, R., Susan, C., Christian, P. G., and Nelson-Kaula, K. (2013). Seedling recruitment factors in low-diversity Hawaiian wet forest: Towards global comparisons among tropical forests. Ecosphere 4:24. doi: 10.1890/ES12-00164.1
Jager, M. M., Richardson, S. J., Bellingham, P. J., Clearwater, M. J., and Laughlin, D. C. (2015). Soil fertility induces coordinated responses of multiple independent functional traits. J. Ecol. 103, 374–385. doi: 10.1111/1365-2745.12366
Johnson, M. O., Galbraith, D., Gloor, M., De Deurwaerder, H., Guimberteau, M., Rammig, A., et al. (2016). Variation in stem mortality rates determines patterns of above-ground biomass in Amazonian forests: Implications for dynamic global vegetation models. Glob. Change Biol. 22, 3996–4013. doi: 10.1111/gcb.13315
Lavkulich, L. M. (1981). Methods manual: pedology laboratory. Vancouver, BC: University of British Columbia.
Lavorel, S., and Garnier, E. (2002). Predicting changes in community composition and ecosystem functioning from plant traits: Revisiting the holy grail. Funct. Ecol. 16, 545–556.
Li, X., Chang, S. X., Liu, J., Zheng, Z., and Wang, X. (2017). Topography-soil relationships in a hilly evergreen broadleaf forest in subtropical China. J. Soils Sediments 17, 1101–1115. doi: 10.1007/s11368-016-1573-4
Lohbeck, M., Poorter, L., Lebrija-Trejos, E., Martínez-Ramos, M., Meave, J., Paz, H., et al. (2013). Successional changes in functional composition contrast for dry and wet tropical forest. Ecology 94, 1211–1216. doi: 10.1890/12-1850.1
Lohbeck, M., Poorter, L., Martínez-Ramos, M., and Bongers, F. (2015). Biomass is the main driver of changes in ecosystem process rates during tropical forest succession. Ecology 96, 1242–1252. doi: 10.1890/14-0472.1
Loiola, P. P., Scherer-Lorenzen, M., and Batalha, M. A. (2015). The role of environmental filters and functional traits in predicting the root biomass and productivity in savannas and tropical seasonal forests. For. Ecol. Manag. 342, 49–55. doi: 10.1016/j.foreco.2015.01.014
Maia, V. A., de Souza, C., de Aguiar-Campos, N., Alencar Fagundes, N., and Miranda Santos, A. (2020). Interactions between climate and soil shape tree community assembly and above-ground woody biomass of tropical dry forests. For. Ecol. Manage. 474:118348. doi: 10.1016/j.foreco.2020.118348
Malhi, Y. (2012). The productivity, metabolism, and carbon cycle of tropical forest vegetation. J. Ecol. 100, 65–75. doi: 10.1111/j.1365-2745.2011.01916.x
Markesteijn, L., and Poorter, L. (2009). Seedling root morphology and biomass allocation of 62 tropical tree species in relation to drought- and shade-tolerance. J. Ecol. 97, 311–325. doi: 10.1111/j.1365-2745.2008.01466.x
Markesteijn, L., Poorter, L., Bongers, F., Paz, H., and Sack, L. (2011). Hydraulics and life history of tropical dry forest tree species: Coordination of species’ drought and shade tolerance. New Phytol. 191, 480–495. doi: 10.1111/j.1469-8137.2011.03708.x
Méndez-Alonzo, R., Paz, H., Cruz Zuluaga, R., Rosell, J., and Olson, M. (2012). Coordinated evolution of leaf and stem economics in tropical dry forest trees. Ecology 93, 2397–2406. doi: 10.2307/41739311
Méndez-Alonzo, R., Pineda-García, F., Paz, H., Rosell, J., and Olson, M. (2013). Leaf phenology is associated with soil water availability and xylem traits in a tropical dry forest. Trees 27, 745–754. doi: 10.1007/s00468-012-0829-x
Méndez-Toribio, M., Ibarra-Manríquez, G., Navarrete-Segueda, A., and Paz, H. (2017). Topographic position, but not slope aspect, drives the dominance of functional strategies of tropical dry forest trees. Environ. Res. Lett. 12: 085002.
Muller-Landau, H. C., Wright, J., Calderon, O., Hubbell, S., and Foster, R. (2002). “Assessing recruitment limitation: concepts, methods, and case-studies from a tropical forest,” in Seed dispersal and frugivory: ecology, evolution, and conservation, eds D. J. Levey, W. R. Silva, and M. Galetti (Wallingford: CAB International), 35–53. doi: 10.1079/9780851995250.0035
Murphy, P. G., and Lugo, A. E. (1986). Ecology of tropical dry forest. Annu. Rev. Ecol. Syst. 17, 67–88.
Ordoñez, J. C., Van Bodegom, P., Witte, J. M., Wright, I., Reich, P., and Aerts, R. (2009). A global study of relationships between leaf traits, climate and soil measures of nutrient fertility. Glob. Ecol. Biogeogr. 18, 137–149. doi: 10.1111/j.1466-8238.2008.00441.x
Pebesma, E. J. (2004). Multivariable geostatistics in S: The gstat package. Comput. Geosci. 30, 683–691. doi: 10.1016/j.cageo.2004.03.012
Pérez-Harguindeguy, N., Díaz, S., Garnier, E., Lavorel, S., Poorter, H., Jaureguiberry, P., et al. (2013). New handbook for standardized measurement of plant functional traits worldwide. Aust. J. Bot. 61, 167–234. doi: 10.1071/BT12225
Phillips, O. L., Sullivan, M. J. P., Baker, T. R., Monteagudo Mendoza, A., and Vargas, P. (2019). Species matter: Wood density influences tropical forest biomass at multiple scales. Surv. Geophys. 40, 913–935. doi: 10.1007/s10712-019-09540-0
Pineda-García, F., Paz, H., Meinzer, F., and Angeles, G. (2015). Exploiting water versus tolerating drought: Water-use strategies of trees in a secondary successional tropical dry forest. Tree Physiol. 36, 208–217. doi: 10.1093/treephys/tpv124
Poorter, L., Van Der Sande, M., Arets, E., Ascarrunz, N., and Enquist, B. (2017). Biodiversity and climate determine the functioning of neotropical forests. Glob. Ecol. Biogeogr. 26, 1423–1434. doi: 10.1111/geb.12668
Poorter, L., Wright, S., Ackerly, D., Condit, R., Ibarra-Manríquez, G., Harms, K., et al. (2008). Are functional traits good predictors of demographic rates? Evidence from five neotropical forests. Ecology 89, 1908–1920. doi: 10.1890/07-0207.1
Prado-Junior, J. A., Schiavini, I., Vale, V., Arantes, C., Van Der Sande, M., Lohbeck, M., et al. (2016). Conservative species drive biomass productivity in tropical dry forests. J. Ecol. 104, 817–827. doi: 10.1111/1365-2745.12543
Pulla, S., Riotte, J., Suresh, H. S., Dattaraja, H. S., and Sukumar, R. (2016). Controls of soil spatial variability in a dry tropical forest. PLoS One 11:e0153212. doi: 10.1371/journal.pone.0153212
Quesada, C. A., Phillips, O. L., Schwarz, M., Czimczik, C. I., and Baker, T. R. (2012). Basin-wide variations in Amazon Forest structure and function are mediated by both soils and climate. Biogeosciences 9, 2203–2246. doi: 10.5194/bg-9-2203-2012
R Core Team (2022). R: a language and environment for statistical computing. Vienna: R Foundation for Statistical Computing.
Reich, P. B. (2014). The world-wide ‘fast–slow’ plant economics spectrum: A traits manifesto. J. Ecol. 102, 275–301. doi: 10.1111/1365-2745.12211
Revelle, W. (2020). psych: procedures for personality and psychological research. Evanston, IL: Northwestern University.
Roy, S. (1996). Spatial variation of soil physico-chemical properties influenced by spatial and temporal variation of litter in a dry tropical forest floor. Oecol. Montana 5, 21–26.
Roy, S., and Singh, J. S. (1994). Consequences of habitat heterogeneity for availability of nutrients in a dry tropical forest. J. Ecol. 82:503. doi: 10.2307/2261259
Stekhoven, D. J., and Bühlmann, P. (2012). Missforest-non-parametric missing value imputation for mixed-type data. Bioinformatics 28, 112–118. doi: 10.1093/bioinformatics/btr597
Talbot, J., Lewis, S., Lopez-Gonzalez, J., Brienen, R., Monteagudo, A., Baker, T., et al. (2014). Methods to estimate aboveground wood productivity from long-term forest inventory plots. For. Ecol. Manag. 320, 30–38. doi: 10.1016/j.foreco.2014.02.021
Toledo, M., Peña-Claros, M., Bongers, F., Alarcón, A., Balcázar, J., Chubiña, J., et al. (2012). Distribution patterns of tropical woody species in response to climatic and edaphic gradients. J. Ecol. 100, 253–263. doi: 10.1111/j.1365-2745.2011.01890.x
Toledo, M., Poorter, L., Peña-Claros, M., Alarcón, A., Balcázar, J., Leaño, C., et al. (2011). Climate is a stronger driver of tree and forest growth rates than soil and disturbance. J. Ecol. 99, 254–264. doi: 10.1111/j.1365-2745.2010.01741.x
van der Sande, M. T., Arets, E.J. M. M., Peña-Claros, M., de Avila, A. L., Roopsind, A., Mazzei, L., Ascarrunz, N., et al. (2016). Old-growth Neotropical forests are shifting in species and trait composition. Ecol. Monogr. 86, 228–243. doi: 10.1890/15-1815.1
van der Sande, M. T., Peña-Claros, M., Ascarrunz, N., Arets, E., Licona, J., Toledo, M., et al. (2017a). Abiotic and biotic drivers of biomass change in a neotropical forest. J. Ecol. 105, 1223–1234. doi: 10.1111/1365-2745.12756
van der Sande, M. T., Arets, E. J. M. M., Peña-Claros, M., Hoosbeek, M., Cáceres-Siani, Y., Van Der Hout, P., et al. (2017b). Soil fertility and species traits, but not diversity, drive productivity and biomass stocks in a Guyanese tropical rainforest. Funct. Ecol. 32, 461–474. doi: 10.1111/1365-2435.12968
Violle, C., Navas, M., Vile, D., Kazakou, E., Fortunel, C., and Hummel, I. (2007). Let the concept of trait be functional!. Oikos 116, 882–892. doi: 10.1111/j.0030-1299.2007.15559.x
Walkley, A., and Black, A. I. (1934). An examination of the Degtjareff method for determining soil organic matter, and a proposed modification of the chromic acid titration method. Soil Sci. 37, 29–38.
Wennekes, P. L., Rosindell, J., and Etienne, R. S. (2012). The neutral-niche debate: A philosophical perspective. Acta Biotheor. 60, 257–271. doi: 10.1007/s10441-012-9144-6
Wiegand, T., Martínez, I., and Huth, A. (2009). Recruitment in tropical tree species: Revealing complex spatial patterns. Am. Nat. 174, 106–140. doi: 10.1086/605368
Keywords: mortality, net biomass changes, recruitment, soil nutrients, soil water availability, survival
Citation: Caleño-Ruiz BL, Garzón F, López-Camacho R, Pizano C, Salinas V and González-M R (2023) Soil resources and functional trait trade-offs determine species biomass stocks and productivity in a tropical dry forest. Front. For. Glob. Change 6:1028359. doi: 10.3389/ffgc.2023.1028359
Received: 25 August 2022; Accepted: 08 February 2023;
Published: 02 March 2023.
Edited by:
Fernanda De Vasconcellos Barros, University of Exeter, United KingdomReviewed by:
Sylvanus Mensah, Université d’Abomey-Calavi, BeninPaula Meli, Universidad de Concepción, Chile
Copyright © 2023 Caleño-Ruiz, Garzón, López-Camacho, Pizano, Salinas and González-M. This is an open-access article distributed under the terms of the Creative Commons Attribution License (CC BY). The use, distribution or reproduction in other forums is permitted, provided the original author(s) and the copyright owner(s) are credited and that the original publication in this journal is cited, in accordance with accepted academic practice. No use, distribution or reproduction is permitted which does not comply with these terms.
*Correspondence: Blanca Luz Caleño-Ruiz, YmxjYWxlbm9yQGNvcnJlby51ZGlzdHJpdGFsLmVkdS5jbw==, YmxhbmNhLmNhbGVub0BnbWFpbC5jb20=