Impact of forest fire on radial growth of tree rings and their element concentrations of Pinus sylvestris and Larix gmelinii in northern China
- 1College of Forestry, Fujian Agriculture and Forestry University, Fuzhou, China
- 2Southern Swedish Forest Research Centre, Swedish University of Agricultural Sciences, Lomma, Sweden
- 3Department of Forest Sciences, University of Helsinki, Helsinki, Finland
Aims: Through analyzing the responses of the radial growth and element concentrations (B, Mg, Al, K, Ca, Mn, Fe, Zn, Na, P, Ni, and Cu) of tree rings of two dominant tree species to forest fires, we aimed to investigate the relationship between tree rings and the fires.
Methods: We sampled wood cores of Pinus sylvestris and Larix gmelinii in the northern forest region of China, where forest fires happened in 1990 and 2008. The ring-width growth of P. sylvestris and L. gmelinii from 1986 to 1995 and 2004 to 2013 in two sites of Tahe County were measured. Element concentrations in tree rings were determined using inductively coupled plasma mass spectrometry (ICP-MS).
Results: Our results showed that tree-ring radial growth was largely reduced after the fire, together with the increase in concentrations of B, Al, Mn, and Fe but the decrease in some samples in K. Strong correlations were observed between tree-ring growth and concentrations of Mg and Mn of P. sylvestris and Znof L. gmelinii.
Discussion: The results provide evidence that variations in tree-ring growth and element concentrations, particularly concentrations of B, Al, Mn, and Fe, are potentially useful to monitor forest fires, which add new insights into the study of forest fire history.
Introduction
As a major disturbance to the forest ecosystem, forest fires have significant effects on forest resources and climate change (Flannigan et al., 2009; Heydari et al., 2017). The rising global temperature and intensifying human activities increase the frequency and intensity of forest fires, resulting in greater economic and ecological losses (Liu et al., 2012; Carvalho et al., 2016). The use of tree-ring elemental compositions for environmental pollution monitoring holds great promise. For example, heavy metals in trees have been used to monitor the frequency and intensity of environmental pollution (Parzych and Jonczak, 2014; Turkyilmaz et al., 2018). Since Lepp (1975) first introduced the concept dendrochemistry, progress has been made in the study of pollution history (Mihaljevič et al., 2015; Odabasi et al., 2016; Xu et al., 2017; Muñoz et al., 2019). For instance, a decline in the number of ships in São Paulo, Brazil results in lower Cd, Cu, and Pb levels in tree rings (Locosselli et al., 2018). The Pb of black spruce tree rings was used to reconstruct the history of Pb pollution in Canada (Richer-Laflèche et al., 2008). Variations in Zn and Cd concentrations in tree rings of white poplar and ailanthus in Xi’an, China, are correlated with environmental changes (Liu et al., 2018). The Cu content of pine tree rings near a copper smelter in Zambia is higher (Mihaljevič et al., 2018).
Forest fires generate a large number of pollutants and the chemical elements that bind to them. During the fire, several elements, including B, Mg, Al, K, Ca, Mn, Fe, Zn, Na, P, Ni, and Cu, are released and deposited in the soil. These elements, which are absorbed by the roots of trees and accumulated in their woody tissues, are essential macro and trace nutrients for tree growth. Potentially, tree-ring element concentrations could be used to study forest fire history. It is essential for the evaluation and management of forest fires to dissect mechanisms of fire occurrence, which is made possible through the study of fire history. Currently numerous studies based on historical data or fire scars shed light on fire history (Lazarina et al., 2017; Mundo et al., 2017; Bobek et al., 2019; Krisnawati et al., 2021; Xu et al., 2021). However, historical data are only available for a few 100 years, and the formation of fire scars depends primarily on fire intensity (Van Horne and Fulé, 2006; McEwan et al., 2007). Under intense fires, a high mortality rate makes it difficult to observe fire scars, whereas they don’t form under low intensity. Thus, studies of the physiological characteristics of tree rings may provide additional insight into the reconstruction of fire history.
We aimed to examine the effects of forest fires on the radial growth and element concentration of tree rings of two conifer species. Coniferous trees have straight trunks, easily distinguishable tree rings, strong CO2 and SO2 adsorption capacities and pronounced environmental responses (Rucandio et al., 2011; Parzych and Jonczak, 2014). In this study, two dominant conifer species, Pinus sylvestris and Larix gmelinii were used to perform dendrochemistry in regions with a high incidence of forest fires. Using inductively coupled plasma mass spectrometry (ICP-MS), the concentrations of 12 elements (B, Mg, Al, K, Ca, Mn, Fe, Zn, Na, P, Ni, and Cu) before and after the fire were determined. Furthermore, we measured the ring width during the studied years. We hypothesized that forest fires would decrease radial growth, while increasing element concentrations in tree rings.
Materials and methods
Study area and sampling
This study was conducted in Tahe County in the Greater Khingan range in Heilongjiang Province (Figure 1). Tahe County is among the most important forest regions in China, where the terrain and vegetation conditions make the area prone to forest fires (Chang et al., 2007; Liu et al., 2012). There are two tree species, Pinus sylvestris and Larix gmelinii dominating the forest of the area. Through evolution, these two species have formed strong adaptability to forest fires and other environmental stresses. They have crowded needles making the understory vegetation high in water content, and a lot of ash that is an inflammable substance negatively influencing the flammability. Thus, it is not easy to burn. Besides, these species have straight trunk, good natural pruning ability, thick bark, and well-closed canopy. The discontinuous distribution of combustibles in the forest floor makes it difficult to form a crown fire. These features result in enhanced resistance of P. sylvestris and L. gmelinii to forest fires.
There were two sampling sites: Tahe 7.5 (7.5 km from Tahe County) and Tahe 18 (18 km from Tahe County), in which forest fires occurred in 1990 and 2008, respectively. Both fires were caused by lightning strikes, which had the burned area of about 670 hm2 and destroyed around 550 hm2 woodland. The fires were fast-moving surface fires with some tree crown fires. In this area, a large amount of humus has been deposited during the long-term vegetation succession. Because of plant ash, the soil color in the fire region is darker. Sampling was conducted in the same slope direction in the burned and unburned area. We relied on the main diffusion and settlement trajectory of flue gas particles that were mostly affected by wind direction (in the meteorological data) at the time when the fire happened to determine the unburned area. The unburned area was 1 km away from the burned area. Obvious fire marks in numerous trunks were found in the burned area, but not in the unburned area. Wood cores of P. sylvestris and L. gmelinii were collected in July 2021. Each species had seven trees in the burned area and seven in the unburned area. All trees were between 35 and 45 years old. We sampled seven trees in an area of 100 m2, and each tree was 5–10 m away from others. Punchers of 5 mm in diameter were used to collect core samples at 1.3 m from the ground. Each tree had four core samples from four directions.
Ring width analysis
The collected cores were dried at room temperature and were fixed using white latex. The core surface was polished with sandpaper until the boundary could be clearly seen under the microscope. Tree-ring analyzer (LINTAB 6.0, Heidelberg, Germany) with 0.001 mm precision was used to measure the ring width. COFECHA software was used to check the quality of cross-dating and to determine a calendar year of each ring (Holmes, 1983). We performed ARSTAN program to de-trend and standardize the ring width data after cross-dating (Cook, 1986). We focused on periods from 1986 to 1995 and 2004 to 2013.
Determination of element concentrations
Core samples were separated and softened in ultrapure water, followed by cleaning using ultrasound (18.2 MΩ, 1 h) and 70% ethanol. Each ring was peeled using a stainless-steel blade under the microscope, and rings from the same calendar year were packaged to the same bag. All samples were dried at 60°C for 48 h, and were ground after cooling and kept in a drying vessel until further use. We weighed 0.05 g of each sample that was immersed in 2 mL HNO3 and 2 mL H2O2 in PTFE for 5 min. After digestion, the solution was diluted with 5% HNO3 to a final volume of 40 ml, and then was filtered through 0.45 μm syringe filters. Concentrations of 12 elements were measured by ICP-MS (Agilent 7900, Santa Clara, CA, USA) and calculated using the following equation:
where C is the concentration of an element (μg g–1), P is the concentration of an element in the solution (μg l–1), V is the volume of the solution (0.04 l), and M is the weight of each sample (0.05 g).
Statistical analysis
The normality of the data was assessed using lm function in R 4.1.0 (R Core Team, 2022). For each tree species, the ring width and element concentrations before and after the fire were compared by two-tailed unpaired t-test in SPSS 25.0 (IBM Corporation). Pearson correlation coefficients between the ring width and element concentrations, and between element concentrations were computed using the rcorr function in the package Hmisc in R (Harrell, 2020).
Results
Radial growth of tree rings
Variations in tree-ring radial growth of P. sylvestris and L. gmelinii were observed, with ring widths ranging from 3.9 to 14.2 mm (Figure 2A). Tree ring radial growth trends in the burned area were comparable to those in the unburned area. The width of the ring increased from 1986 to 1989 in Tahe 7.5 and from 2004 to 2007 in Tahe 18, but decreased in 1990 and 2008 when a fire happened. After the fire, P. sylvestris radial growth quickly returned to pre-fire levels, while L. gmelinii radial growth continued to decline for several years. To compare the radial growth before and after the fire, we focused on two time periods: before the fire (1986–1989, 2004–2007) and after the fire (1990–1992, 2008–2010). Forest fires impeded the tree-ring radial growth of P. sylvestri and L. gmelinii, particularly in Tahe 18. In the burned area, the average tree-ring width of two species was over 10 mm prior to the fire, but decreased to approximately 7 mm after the fire (Figure 2B).
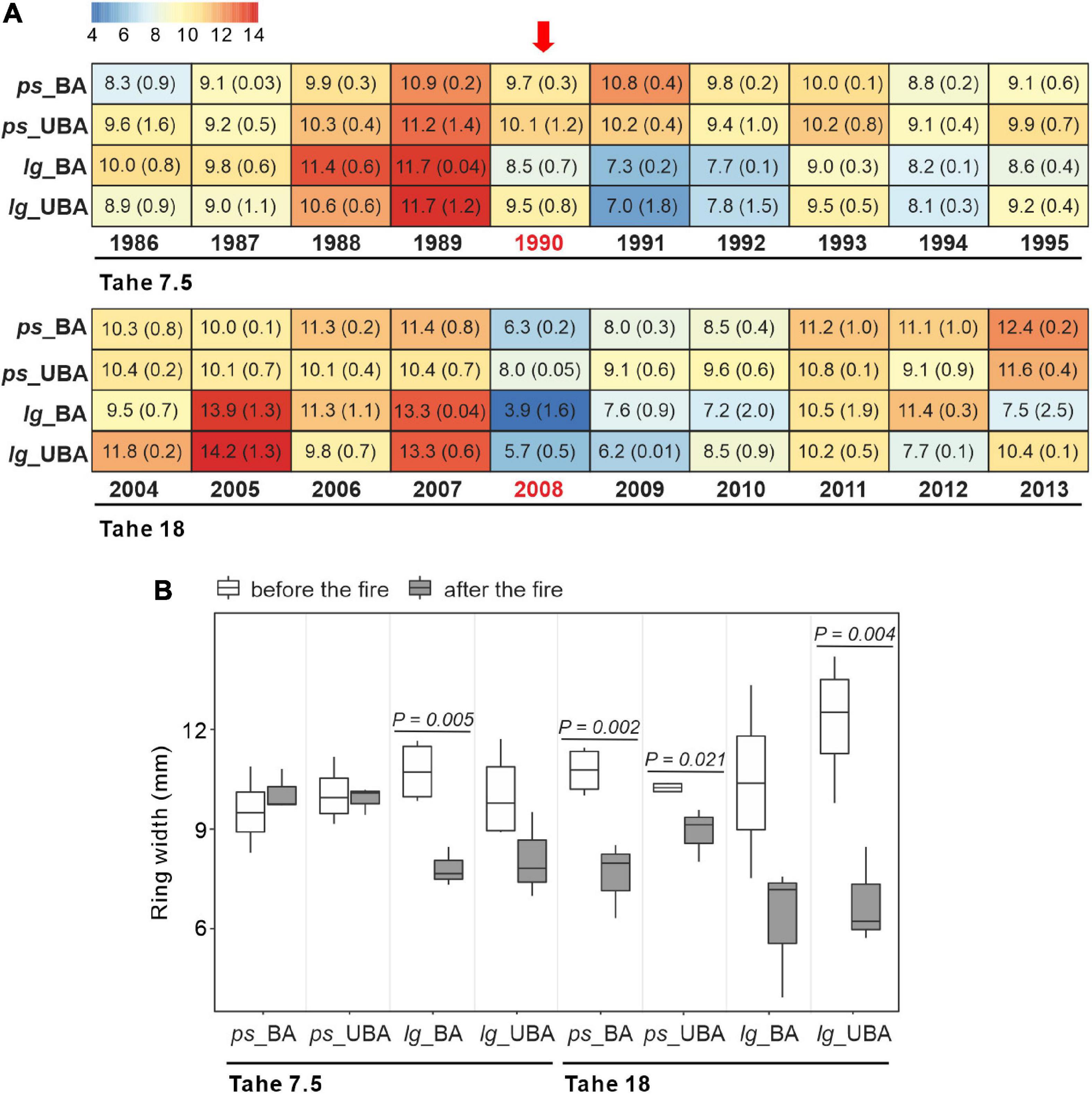
Figure 2. Radial growth of tree rings. (A) Heatmaps of ring width [mean (standard errors)] in Tahe 7.5 (1986–1995) and Tahe 18 (2004–2013) in the burned area (BA) and unburned area (UBA), where ps and lg stands for Pinus sylvestris and Larix gmelinii, respectively. (B) Boxplots of ring width before (1986–1989 in Tahe 7.5, 2004–2007 in Tahe 18) and after (1990–1992 in Tahe 7.5, 2008–2010 in Tahe 18) the fire. Statistical analysis was assessed with two-tailed unpaired t-test.
Element concentrations in tree rings
Concentrations of 12 elements in tree rings fluctuated over the study period, particularly B, Mg, Al, K, Ca, Mn, Fe, and Zn concentrations (Figure 3 and Supplementary Figure 1). Therefore, the analyses focused on these eight elements. The changing trends displayed a similar response regardless of sampling sites (Tahe 7.5 and Tahe 18) or studied areas (the burned and unburned area). Element concentrations were highly sensitive to fire effects during the first 3 years after the fire (1990–1992, 2008–2010). During these years, the highest concentrations of B, Al, K, and Mn were found in the burned area, whereas the concentration of Mg in P. sylvestri in Tahe 18 decreased. In the unburned area, the concentrations of Ca, Fe, and Zn raised while the concentration of K dropped to its lowest level. Similar results were observed concerning the comparison between two time periods (Figure 4), forest fires may increase or decrease element concentrations in specific regions and tree species.
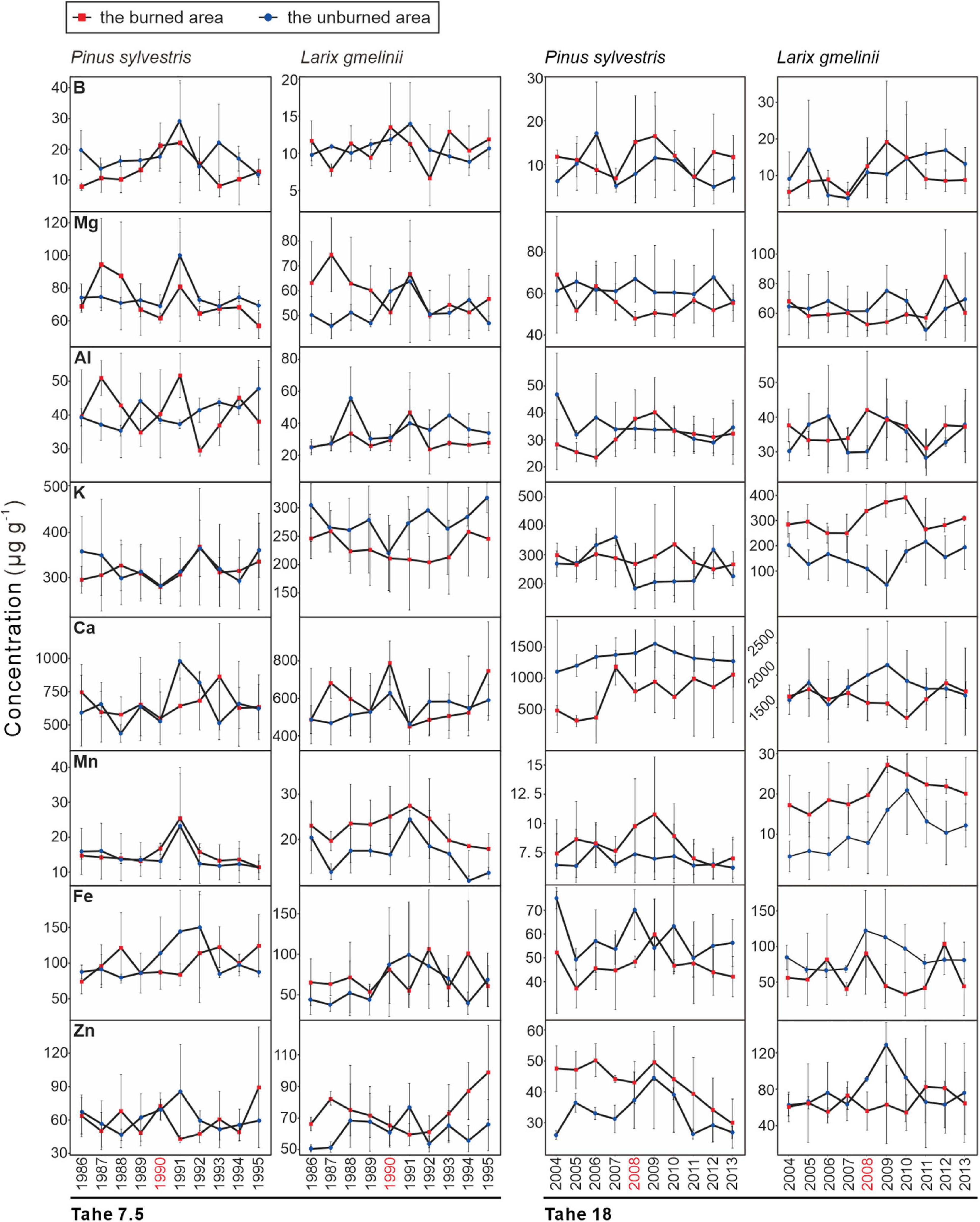
Figure 3. Concentrations of elements in Tahe 7.5 (1986–1995) and Tahe 18 (2004–2013) in the burned area and unburned area.
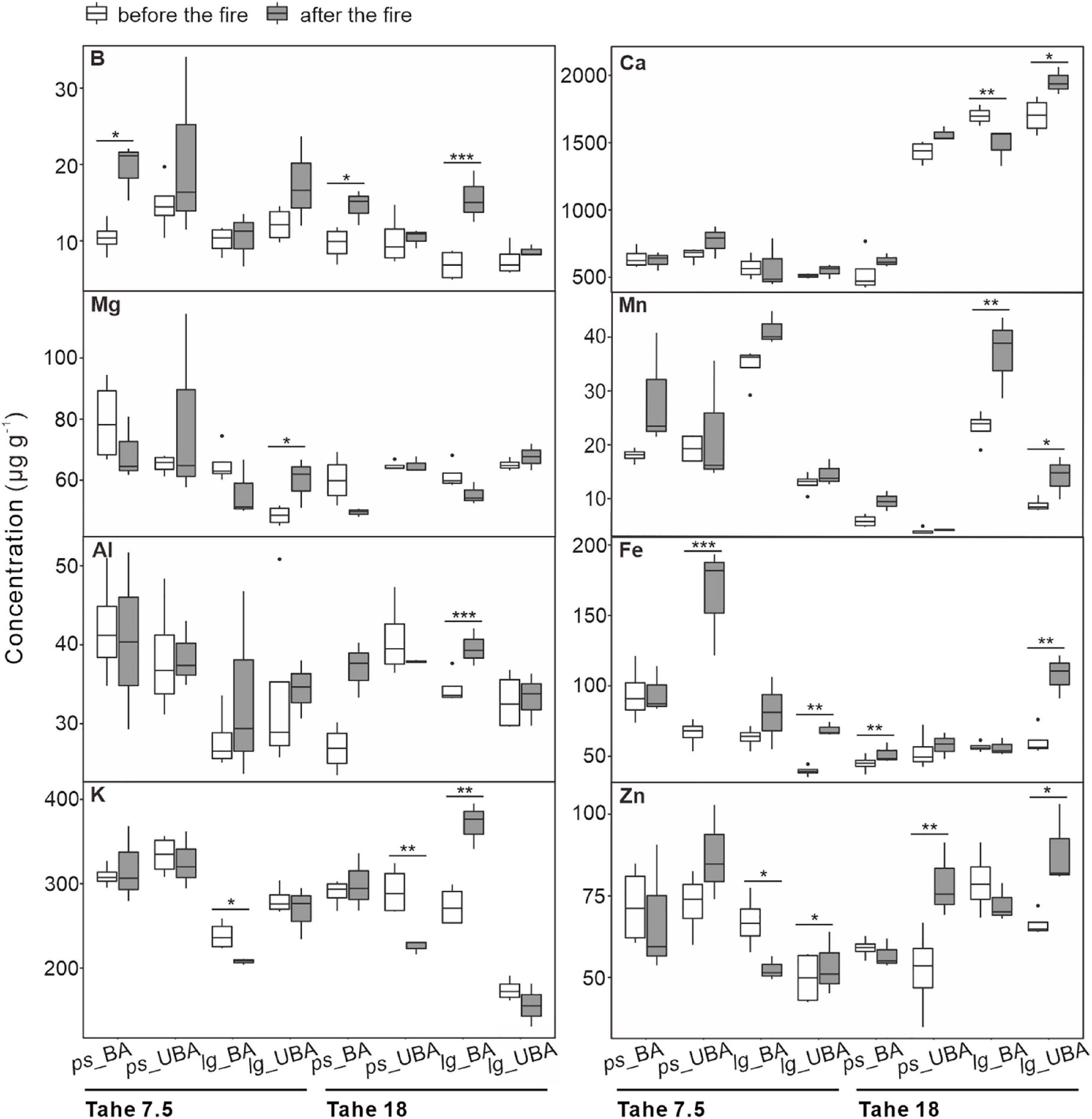
Figure 4. Boxplots of concentrations of eight elements in Tahe 7.5 and Tahe 18 before (1986–1989 in Tahe 7.5, 2004–2007 in Tahe 18) and after (1990–1992 in Tahe 7.5, 2008–2010 in Tahe 18) the fire. Statistical analysis was assessed with two-tailed unpaired t-test (*P < 0.05, **P < 0.01, ***P < 0.001).
Correlation studies
The autocorrelation results revealed that several tree elements migrated annually (Table 1 and Supplementary Table 1). A larger number of elements exhibited 1 and 2 years migrations in the burned area than in the unburned area. Among them, the 1 and 2 years migrations had the greatest effect on Fe and Zn in L. gmelinii. The results implied that element migration was more active in the burned area, which may contribute to the rise in element concentrations.
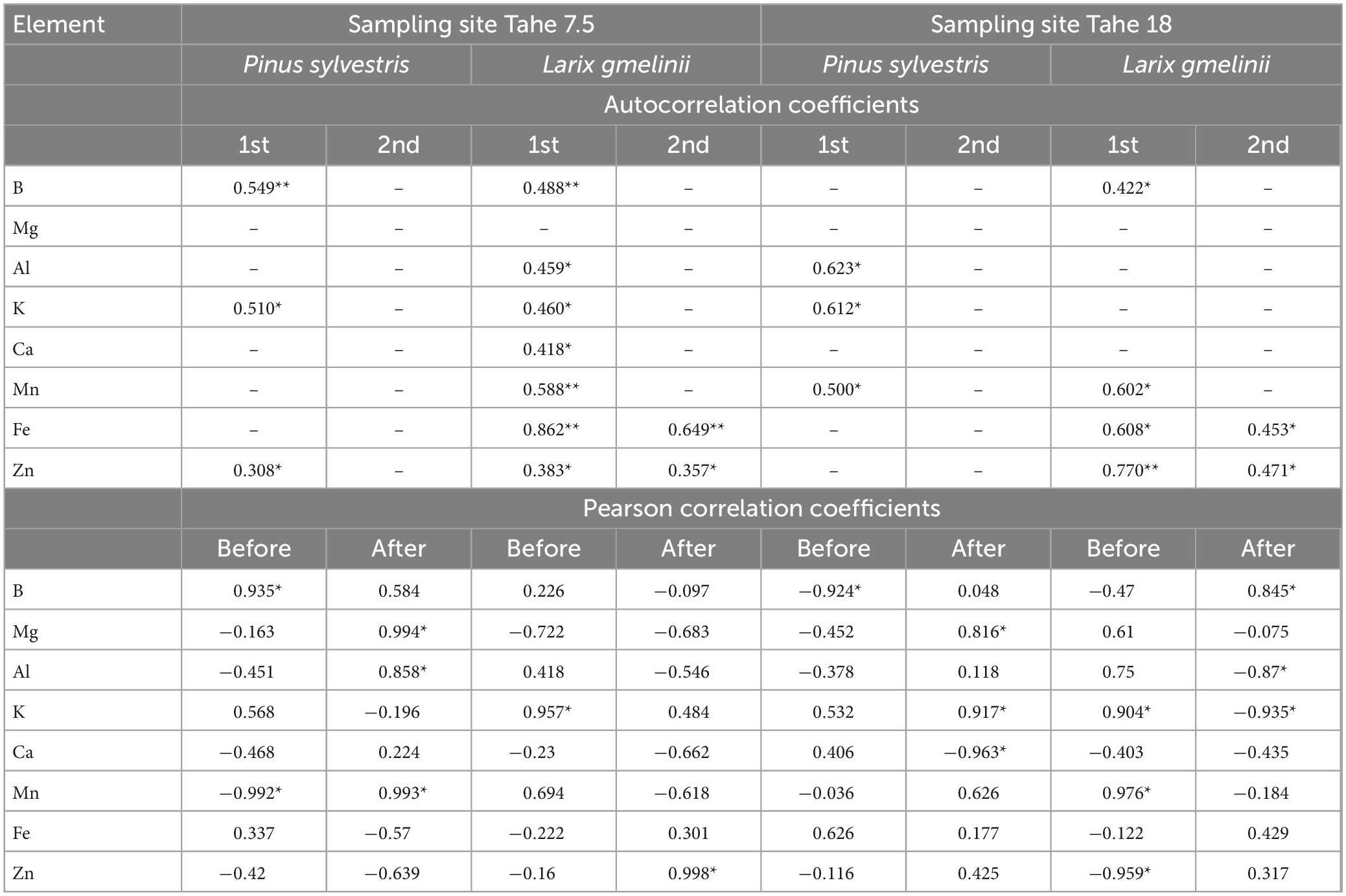
Table 1. Autocorrelation coefficients (with significant differences) of element concentrations and Pearson correlation coefficients (positive correlations, blue colored; negative correlations, orange colored) for tree-ring width and element concentrations in Pinus sylvestris and Larix gmelinii in the burned area of Tahe 7.5 and Tahe 18 (*P < 0.05, **P < 0.01).
Forest fires may enhance the relationship between pairs of elements, according to the interaction between elements (Figure 5 and Supplementary Figure 2). Notably, correlations between B and Zn in P. sylvestris in Tahe 7.5, which were negative prior to the fire, became positive after the fire. The results suggested that forest fires may play a role in the elemental proportions in tree rings. Similar correlations between the growth and element concentrations in tree rings were observed. In both the burned and unburned area, several negative correlations between ring-width growth and element concentrations of P. sylvestris became positive as a result of the fire (Table 1 and Supplementary Table 1). L. gmelinii exhibited the exact opposite results. Besides, different correlations were discovered between the burned and unburned area, indicating that the fire and species may influence the relationship between growth and element concentrations in tree rings. These findings clarify the potential interactions between tree-ring growth and element concentrations, as well as interactions between different elements.
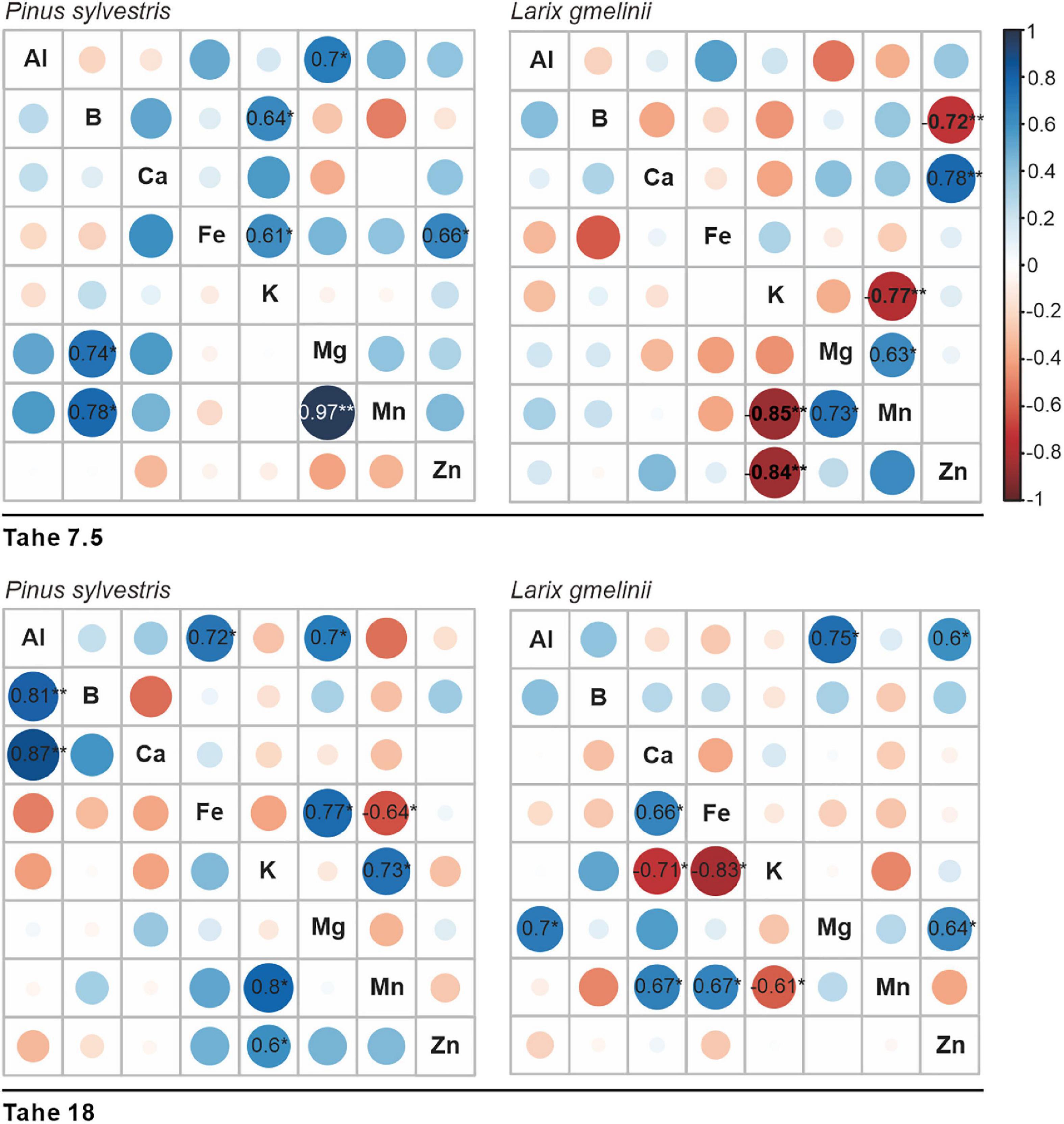
Figure 5. Correlation matrix plot between element concentrations in Tahe 7.5 and Tahe 18 in the burned area before (above the diagonal) and after (under the diagonal) the fire. Pearson correlation coefficients with significance levels were listed (*P < 0.05, **P < 0.01).
Discussion
We found that the radial growth of P. sylvestris and L. gmelinii tree rings decreased after the fire, particularly in Tahe 18. The suppression of growth may have been caused by the increasing temperature and drought stress (McLaughlin and Percy, 1999; Voelker, 2011), which were associated with the fires. Typically, forest fires are accompanied by the production of a large amount of flue gas. The particles and pollutants in the flue gas cause plants to close their stomata. Stomatal closure cuts down photosynthesis efficiency by decreasing the absorption of CO2 (Zhao et al., 2008; Malik et al., 2012), and consequently inhibits the growth of tree rings. Notable is the correlation between the increase in element concentrations due to air pollution and the reduction in ring width (Dmuchowski and Bytnerowicz, 2009). The fire may promote the absorption of certain elements, whose increased concentration will inhibit root development and auxin biosynthesis, thereby inhibiting plant growth. Since radial growth contains large-scale environmental information that is influenced by the forest ecosystem and atmospheric environment (Abiyu et al., 2018; Gaspard et al., 2018; Bosela et al., 2019), it has been considered as an indicator of environmental changes.
The fire caused variations in the element concentrations in tree rings of P. sylvestris and L. gmelinii. Following a wildfire, trees improve the utilization efficiency of water and nutrient elements from the soil (Pearson et al., 2005). Torres-Alvarado et al. (2011) noted that volcanic eruptions increased the amount of metal elements e.g., Al in tree rings. They pointed out that volcanic eruptions may melt glaciers at the mountain’s peak that contain pollutants that are absorbed into the xylem by the roots. Ballikaya et al. (2022) found that forest fires have a significant impact on the accumulation of elements in tree rings, which is primarily due to the metabolism of substances between atmosphere, soil, and trees. Using synchrotron radiation X-ray fluorescence analysis, researchers identified 20 elements in the particulates released by forest fires in Siberia, with relative high concentrations of B, K, Ca, Fe, and Mn (Golobokova et al., 2020; Ukraintsev et al., 2020). After the fire, soil nutrients may dominate the absorption of nutrients by plants during the growth process (Alcañiz et al., 2016). Due to the burning of the surface humus layer, which increases surface runoff, the damaged forest canopy reduces rainfall absorption, resulting in an increase in soil moisture (Johnson et al., 2007). As the growing season progresses into summer, the Greater Khingan range experiences an increase in precipitation, and surface runoff from the burned area rises. Numerous elements in the ash are distributed to the forest ecosystem via leaching, before entering the soil and being utilized by plants (Lagerström et al., 2009), which could increase the element content. These findings were consistent with those of other research. For instance, after the fire, the Mn concentration in the soil was more than five times that of soil without fire (Costa et al., 2014; Campos et al., 2016), and the Ni and Cu concentrations in the ash increased significantly (Bogacz et al., 2011). Moreover, the post-fire environment promotes the renewal rate of vegetation, and some elements in the soil are absorbed and utilized by plants and fixed in plants, leading to increases in certain element contents in tree rings (Badia and Marti, 2008).
The accumulation of elements in the xylem of trees is mainly influenced by physiological characteristics of trees, such as the storage and transport of elements, and environmental factors (Kalugina et al., 2017; Zakrzewska and Klimek, 2018). Trees can absorb numerous elements from the environment into their wood tissues through their roots, leaves and bark (Solgi et al., 2020). After entering the xylem, elements may migrate horizontally between tree rings, moving from the physiologically active region to the inactive region (Battipaglia et al., 2010). The gradual increase in Zn concentration from heartwood to sapwood in tree rings, is likely due to Zn’s horizontal migration (Amais et al., 2021; Nechita et al., 2021). The forest fire’s flue gas contains a high concentration of Zn, which can be absorbed by plants and accumulate in tree rings. Elements e.g., K can be transferred via the xylem or phloem, or even across boundaries (Yamaji and Ma, 2019). When the content of K in the environment increases, it will stress the transboundary movement of K in tree rings (Takahashi et al., 2020; Bardule et al., 2021). Some elements, e.g., B and Ca, are fixed or have limited mobility in the phloem (Stanfield et al., 2019). Besides, the movement of elements in tree rings varies among tree species, which is related to the xylem structure or xylem sap in trees (Smith and Shortle, 2001). Fe and Zn have poor migration abilities in tree rings of Picea mariana and Pinus massoniana, whereas Zn can migrate across the rings in Pinus tabuliformis (Prohaska et al., 1998; Liu et al., 2009). Thus, characterizing the migration of elements in tree rings may contribute to forest fire monitoring.
In this study, forest fires inhibited the growth of ring width, and altered the element content in tree rings. In the burned area the concentrations of B, Al, and Mn showed an increase after the fire, and Fe and Zn in the unburned area. The fire intensity at the two sampling sites was relatively high, so the effects on the content of some elements in tree rings of P. sylvestris and L. gmelinii were similar. This may be resulted from the sensitivity of the soil environment and the bark of trees to forest fires (Hannam et al., 2019). Fires of high intensity that have a negative impact on the availability of nutrient elements can raise the ground surface temperature in forests, stimulate microbial activity, and consequently promote nutrient absorption through the roots. The responses may lead to a rise in element concentrations within tree rings (Goodale and Aber, 2001). Our research indicates that tree-ring elements, particularly B, Al, Mn, and Fe concentrations, have the potential to be used as indicators of forest fire occurrence and are promising for reconstructing fire history.
Data availability statement
The original contributions presented in this study are included in the article/Supplementary material, further inquiries can be directed to the corresponding author.
Author contributions
MW, XG, and FG contributed to conception and design of the study. MW, XG, YM, and WZ performed the experiments. ML and MW analyzed the data and wrote the first draft of the manuscript. MT revised and edited the manuscript. All authors contributed to manuscript revision, read, and approved the submitted version.
Funding
This study was supported by the National Natural Science Foundation of China (Grant Number: 32171807) to FG.
Conflict of interest
The authors declare that the research was conducted in the absence of any commercial or financial relationships that could be construed as a potential conflict of interest.
Publisher’s note
All claims expressed in this article are solely those of the authors and do not necessarily represent those of their affiliated organizations, or those of the publisher, the editors and the reviewers. Any product that may be evaluated in this article, or claim that may be made by its manufacturer, is not guaranteed or endorsed by the publisher.
Supplementary material
The Supplementary Material for this article can be found online at: https://www.frontiersin.org/articles/10.3389/ffgc.2023.1136039/full#supplementary-material
References
Abiyu, A., Mokria, M., Gebrekirstos, A., and Braeuning, A. (2018). Tree-ring record in Ethiopian church forests reveals successive generation differences in growth rates and disturbance events. For. Ecol. Manag. 409, 835–844. doi: 10.1016/j.foreco.2017.12.015
Alcañiz, M., Outeiro, L., Francos, M., Farguell, J., and Úbeda, X. (2016). Long-term dynamics of soil chemical properties after a prescribed fire in a Mediterranean forest (Montgrí Massif, Catalonia, Spain). Sci. Total Environ. 572, 1329–1335. doi: 10.1016/j.scitotenv.2016.01.115
Amais, R. S., Moreau, P. S., Francischini, D. S., Magnusson, R., Locosselli, G. M., Godoy-Veiga, M., et al. (2021). Trace elements distribution in tropical tree rings through high-resolution imaging using LA-ICP-MS analysis. J. Trace Elem. Med. Biol. 68:126872. doi: 10.1016/j.jtemb.2021.126872
Badia, D., and Marti, C. (2008). Fire and rainfall energy effects on soil erosion and runoff generation in semi-arid forested lands. Arid. Land Res. Manag. 22, 93–108. doi: 10.1080/15324980801957721
Ballikaya, P., Marshall, J., and Cherubini, P. (2022). Can tree-ring chemistry be used to monitor atmospheric nanoparticle contamination over time? Atmos. Environ. 268:118781. doi: 10.1016/j.atmosenv.2021.118781
Bardule, A., Bārdulis, A., Polmanis, K., Krumsteds, L., Butlers, A., Stola, J., et al. (2021). Trends of Scots pine forest health and element flow changes in the ICP Forests monitoring sites in Latvia. Balt. For. 27:536. doi: 10.46490/BF536
Battipaglia, G., Marzaioli, F., Lubritto, C., Altieri, S., Strumia, S., Cherubini, P., et al. (2010). Traffic pollution affects tree-ring width and isotopic composition of Pinus pinea. Sci. Total Environ. 408, 586–593. doi: 10.1016/j.scitotenv.2009.09.036
Bobek, P., Svobodová-Svitavská, H., Pokorný, P., Šamonil, P., Kuneš, P., Kozáková, R., et al. (2019). Divergent fire history trajectories in Central European temperate forests revealed a pronounced influence of broadleaved trees on fire dynamics. Quat. Sci. Rev. 222:105865. doi: 10.1016/j.quascirev.2019.105865
Bogacz, A., Wozniczka, P., and Labaz, B. (2011). Concentration and pools of heavy metals in organic soils in post-fire areas used as forests and meadows. J. Elem. 16, 515–524. doi: 10.5601/jelem.2011.16.4.01
Bosela, M., Kulla, L., Roessiger, J., Šebeň, V., Dobor, L., Büntgen, U., et al. (2019). Long-term effects of environmental change and species diversity on tree radial growth in a mixed European forest. For. Ecol. Manag. 446, 293–303. doi: 10.1016/j.foreco.2019.05.033
Campos, I., Abrantes, N., Keizer, J. J., Vale, C., and Pereira, P. (2016). Major and trace elements in soils and ashes of eucalypt and pine forest plantations in Portugal following a wildfire. Sci. Total Environ. 572, 1363–1376. doi: 10.1016/j.scitotenv.2016.01.190
Carvalho, Jr J, Amaral, S., Costa, M., Neto, T. S., Veras, C., Costa, F., et al. (2016). CO2 and CO emission rates from three forest fire controlled experiments in Western Amazonia. Atmos. Environ. 135, 73–83.
Chang, Y., He, H. S., Bishop, I., Hu, Y., Bu, R., Xu, C., et al. (2007). Long-term forest landscape responses to fire exclusion in the Great Xing’an Mountains. China. Int. J. Wildland Fire 16, 34–44. doi: 10.1071/WF05093
Cook, E. R. (1986). Tree-ring chronologies of western North America: California, Eastern Oregon and northern Great Basin. Tucson: University of Arizona.
Costa, M. R., Calvão, A. R., and Aranha, J. (2014). Linking wildfire effects on soil and water chemistry of the Marão River watershed, Portugal, and biomass changes detected from Landsat imagery. Appl. Geochem. 44, 93–102. doi: 10.1016/j.apgeochem.2013.09.009
Dmuchowski, W., and Bytnerowicz, A. (2009). Long-term (1992–2004) record of lead, cadmium, and zinc air contamination in Warsaw, Poland: Determination by chemical analysis of moss bags and leaves of Crimean linden. Environ. Pollut. 157, 3413–3421. doi: 10.1016/j.envpol.2009.06.019
Flannigan, M. D., Krawchuk, M. A., de Groot, W. J., Wotton, B. M., and Gowman, L. M. (2009). Implications of changing climate for global wildland fire. Int. J. Wildland Fire 18, 483–507. doi: 10.1071/WF08187
Gaspard, D. T., Venegas-González, A., Beeckman, H., Randriamalala, J. R., Tomazello-Filho, M., De Ridder, M., et al. (2018). Tree ring responses to climate variability of xerophytic thickets from South Soalara, Madagascar. Dendrochronologia 49, 57–67. doi: 10.1016/j.dendro.2018.02.003
Golobokova, L., Khodzher, T., Khuriganova, O., Marinayte, I., Onishchuk, N., Rusanova, P., et al. (2020). Variability of chemical properties of the atmospheric aerosol above Lake Baikal during large wildfires in Siberia. Atmosphere 11:1230. doi: 10.3390/atmos11111230
Goodale, C. L., and Aber, J. D. (2001). The long-term effects of land-use history on nitrogen cycling in northern hardwood forests. Ecol. Appl. 11, 253–267. doi: 10.1890/1051-0761(2001)011[0253:TLTEOL]2.0.CO;2
Hannam, K., Fleming, R., Venier, L., and Hazlett, P. (2019). Can bioenergy ash applications emulate the effects of wildfire on upland forest soil chemical properties? Soil Sci. Soc. Am. J. 83, S201–S217. doi: 10.2136/sssaj2018.10.0380
Harrell, F. E. Jr. (2020). Hmisc: Harrell Miscellaneous. R package version 4.4-2 (Harrell, F. E., Jr., with contributions from Charles Dupont and many others). Available online at: https://dirk.eddelbuettel.com/cranberries/2020/08/10/ (accessed August 13, 2022).
Heydari, M., Rostamy, A., Najafi, F., and Dey, D. (2017). Effect of fire severity on physical and biochemical soil properties in Zagros oak (Quercus brantii Lindl.) forests in Iran. J. For. Res. 28, 95–104. doi: 10.1007/s11676-016-0299-x
Holmes, R. L. (1983). Computer-assisted quality control in tree-ring dating and measurement. Tree Ring Bull. 43, 51–67. doi: 10.1016/j.dib.2018.08.019
Johnson, D., Murphy, J. D., Walker, R. F., Glass, D. W., and Miller, W. W. (2007). Wildfire effects on forest carbon and nutrient budgets. Ecol. Eng. 31, 183–192.
Kalugina, O. V., Mikhailova, T. A., and Shergina, O. V. (2017). Pinus sylvestris as a bio-indicator of territory pollution from aluminum smelter emissions. Environ. Sci. Pollut. Res. 24, 10279–10291. doi: 10.1007/s11356-017-8674-5
Krisnawati, H., Adinugroho, W. C., Imanuddin, R., Weston, C. J., and Volkova, L. (2021). Carbon balance of tropical peat forests at different fire history and implications for carbon emissions. Sci. Total Environ. 779:146365. doi: 10.1016/j.scitotenv.2021.146365
Lagerström, A., Esberg, C., Wardle, D. A., and Giesler, R. (2009). Soil phosphorus and microbial response to a long-term wildfire chronosequence in northern Sweden. Biogeochemistry 95, 199–213. doi: 10.1371/journal.pone.0129892
Lazarina, M., Sgardelis, S. P., Tscheulin, T., Devalez, J., Mizerakis, V., Kallimanis, A. S., et al. (2017). The effect of fire history in shaping diversity patterns of flower-visiting insects in post-fire Mediterranean pine forests. Biodivers. Conserv. 26, 115–131.
Lepp, N. (1975). The potential of tree-ring analysis for monitoring heavy metal pollution patterns. Environ. Pollut. 9, 49–61.
Liu, Y., Ta, W., Bao, T., Yang, Z., Song, H., Liu, N., et al. (2009). Trace elements in tree rings and their environmental effects: A case study in Xi’an City. Sci. China Earth Sci. 52, 504–510. doi: 10.1007/s11430-009-0048-5
Liu, Y., Ta, W., Cherubini, P., Liu, R., Wang, Y., and Sun, C. (2018). Elements content in tree rings from Xi’an, China and environmental variations in the past 30 years. Sci. Total Environ. 619, 120–126. doi: 10.1016/j.scitotenv.2017.11.075
Liu, Z., Yang, J., Chang, Y., Weisberg, P. J., and He, H. S. (2012). Spatial patterns and drivers of fire occurrence and its future trend under climate change in a boreal forest of Northeast China. Glob. Chang. Biol. 18, 2041–2056. doi: 10.1111/j.1365-2486.2012.02649.x
Locosselli, G. M., Chacón-Madrid, K., Arruda, M. A. Z., de Camargo, E. P., Moreira, T. C. L., de André, C. D. S., et al. (2018). Tree rings reveal the reduction of Cd, Cu, Ni and Pb pollution in the central region of São Paulo, Brazil. Environ. Pollut. 242, 320–328. doi: 10.1016/j.envpol.2018.06.098
Malik, I., Danek, M., Marchwińska-Wyrwał, E., Danek, T., Wistuba, M., and Krąpiec, M. (2012). Scots Pine (Pinus sylvestris L.) growth suppression and adverse effects on human health due to air pollution in the Upper Silesian Industrial District (USID), Southern Poland. Water Air Soil Pollut. 223, 3345–3364. doi: 10.1007/s11270-012-1114-8
McEwan, R. W., Hutchinson, T. F., Ford, R. D., and McCarthy, B. C. (2007). An experimental evaluation of fire history reconstruction using dendrochronology in white oak (Quercus alba). Can. J. For. Res. 37, 806–816. doi: 10.1139/X06-294
McLaughlin, S., and Percy, K. (1999). Forest health in North America: Some perspectives on actual and potential roles of climate and air pollution. Water Air Soil Pollut. 116, 151–197. doi: 10.1023/A:1005215112743
Mihaljevič, M., Ettler, V., Vaněk, A., Penížek, V., Svoboda, M., Kříbek, B., et al. (2015). Trace elements and the lead isotopic record in marula (Sclerocarya birrea) tree rings and soils near the Tsumeb smelter, Namibia. Water Air Soil Pollut. 226, 1–11.
Mihaljevič, M., Jarošíková, A., Ettler, V., Vaněk, A., Penížek, V., Kříbek, B., et al. (2018). Copper isotopic record in soils and tree rings near a copper smelter. Copperbelt, Zambia. Sci. Total Environ. 621, 9–17. doi: 10.1016/j.scitotenv.2017.11.114
Mundo, I. A., Villalba, R., Veblen, T. T., Kitzberger, T., Holz, A., Paritsis, J., et al. (2017). Fire history in southern Patagonia: Human and climate influences on fire activity in Nothofagus pumilio forests. Ecosphere 8:e01932. doi: 10.1002/ecs2.1932
Muñoz, A. A., Klock-Barría, K., Sheppard, P. R., Aguilera-Betti, I., Toledo-Guerrero, I., Christie, D. A., et al. (2019). Multidecadal environmental pollution in a mega-industrial area in central Chile registered by tree rings. Sci. Total Environ. 696:133915. doi: 10.1016/j.scitotenv.2019.133915
Nechita, C., Iordache, A. M., Lemr, K., Levanič, T., and Pluhacek, T. (2021). Evidence of declining trees resilience under long term heavy metal stress combined with climate change heating. J. Clean. Prod. 317:128428. doi: 10.1016/j.jclepro.2021.128428
Odabasi, M., Tolunay, D., Kara, M., Falay, E. O., Tuna, G., Altiok, H., et al. (2016). Investigation of spatial and historical variations of air pollution around an industrial region using trace and macro elements in tree components. Sci. Total Environ. 550, 1010–1021. doi: 10.1016/j.scitotenv.2016.01.197
Parzych, A., and Jonczak, J. (2014). Pine needles (Pinus sylvestris L.) as bioindicators in the assessment of urban environmental contamination with heavy metals. J. Ecol. Eng. 15, 29–38.
Pearson, C., Manning, S. W., Coleman, M., and Jarvis, K. (2005). Can tree-ring chemistry reveal absolute dates for past volcanic eruptions? J. Archaeol. Sci. 32, 1265–1274. doi: 10.1016/j.jas.2005.03.007
Prohaska, T., Stadlbauer, C., Wimmer, R., Stingeder, G., Latkoczy, C., Hoffmann, E., et al. (1998). Investigation of element variability in tree rings of young Norway spruce by laser-ablation-ICPMS. Sci. Total Environ. 219, 29–39. doi: 10.1016/S0048-9697(98)00224-1
R Core Team (2022). R: A language and environment for statistical computing. R Foundation for Statistical Computing, Vienna, Austria. Available online at: URL https://www.R-project.org/ (accessed August 13, 2022).
Richer-Laflèche, M., Bégin, C., and Rodrigue, R. (2008). Spatiotemporal reconstruction of lead contamination using tree rings and organic soil layers. Sci. Total Environ. 407, 233–241. doi: 10.1016/j.scitotenv.2008.09.044
Rucandio, M. I., Petit-Domínguez, M. D., Fidalgo-Hijano, C., and García-Giménez, R. (2011). Biomonitoring of chemical elements in an urban environment using arboreal and bush plant species. Environ. Sci. Pollut. R. 18, 51–63. doi: 10.1007/s11356-010-0350-y
Smith, K. T., and Shortle, W. C. (2001). Conservation of element concentration in xylem sap of red spruce. Trees 15, 148–153. doi: 10.1007/s004680000085
Solgi, E., Keramaty, M., and Solgi, M. (2020). Biomonitoring of airborne Cu, Pb, and Zn in an urban area employing a broad leaved and a conifer tree species. J. Geochem. Explor. 208:106400.
Stanfield, R. C., Schulte, P. J., Randolph, K. E., and Hacke, U. G. (2019). Computational models evaluating the impact of sieve plates and radial water exchange on phloem pressure gradients. Plant Cell Environ. 42, 466–479. doi: 10.1111/pce.13414
Takahashi, M., Feng, Z., Mikhailova, T. A., Kalugina, O. V., Shergina, O. V., Afanasieva, L. V., et al. (2020). Air pollution monitoring and tree and forest decline in East Asia: A review. Sci. Total Environ. 742:140288. doi: 10.1016/j.scitotenv.2020.140288
Torres-Alvarado, I. S., Smith, A. D., and Castillo-Román, J. (2011). Sr, Nd and Pb isotopic and geochemical constraints for the origin of magmas in Popocatepetl volcano (central Mexico) and their relationship with the adjacent volcanic fields. Int. Geol. Rev. 53, 84–115.
Turkyilmaz, A., Sevik, H., Isinkaralar, K., and Cetin, M. (2018). Using Acer platanoides annual rings to monitor the amount of heavy metals accumulated in air. Environ. Monit. Assess. 190:578. doi: 10.1007/s10661-018-6956-0
Ukraintsev, A. V., Plyusnin, A. M., and Zaikovskii, V. I. (2020). Morphology and chemical composition of dispersed particles in the snow cover of burnt forest areas in Western Transbaikalia (Russia). Appl. Geochem. 122:104723. doi: 10.1016/j.apgeochem.2020.104723
Van Horne, M. L., and Fulé, P. Z. (2006). Comparing methods of reconstructing fire history using fire scars in a southwestern United States ponderosa pine forest. Can. J. For. Res. 36, 855–867.
Voelker, S. L. (2011). “Age-dependent changes in environmental influences on tree growth and their implications for forest responses to climate change,” in Size-and age-related changes in tree structure and function, eds F. Meinzer, B. Lachenbruch, and T. Dawson (New York, NY: Springer), 455–479. doi: 10.1007/978-94-007-1242-3_17
Xu, J., Jing, B., Zhang, K., Cui, Y., Malkinson, D., Kopel, D., et al. (2017). Heavy metal contamination of soil and tree-ring in urban forest around highway in Shanghai, China. Hum. Ecol. Res. Assess. 23, 1745–1762. doi: 10.1080/10807039.2017.1340826
Xu, X., Li, F., Lin, Z., and Song, X. (2021). Holocene fire history in China: Responses to climate change and human activities. Sci. Total Environ. 753:142019. doi: 10.1016/j.scitotenv.2020.142019
Yamaji, N., and Ma, J. F. (2019). Bioimaging of multiple elements by high-resolution LA-ICP-MS reveals altered distribution of mineral elements in the nodes of rice mutants. Plant J. 99, 1254–1263. doi: 10.1111/tpj.14410
Zakrzewska, M., and Klimek, B. (2018). Trace element concentrations in tree leaves and lichen collected along a metal pollution gradient near Olkusz (Southern Poland). Bull. Environ. Contam. Toxicol. 100, 245–249. doi: 10.1007/s00128-017-2219-y
Keywords: conifer, fire history, forest fire, ring width, tree ring, wood cores
Citation: Wei M, Guo X, Ma Y, Tigabu M, Zheng W, Liu M and Guo F (2023) Impact of forest fire on radial growth of tree rings and their element concentrations of Pinus sylvestris and Larix gmelinii in northern China. Front. For. Glob. Change 6:1136039. doi: 10.3389/ffgc.2023.1136039
Received: 02 January 2023; Accepted: 24 February 2023;
Published: 09 March 2023.
Edited by:
Kajar Köster, University of Eastern Finland, FinlandReviewed by:
Paul Sheppard, University of Arizona, United StatesNinghua Zhu, Central South University of Forestry and Technology, China
Copyright © 2023 Wei, Guo, Ma, Tigabu, Zheng, Liu and Guo. This is an open-access article distributed under the terms of the Creative Commons Attribution License (CC BY). The use, distribution or reproduction in other forums is permitted, provided the original author(s) and the copyright owner(s) are credited and that the original publication in this journal is cited, in accordance with accepted academic practice. No use, distribution or reproduction is permitted which does not comply with these terms.
*Correspondence: Mengxia Liu, mengxia0liu@163.com
†These authors have contributed equally to this work and share first authorship