- 1Jiangxi Provincial Key Laboratory of Silviculture, College of Forestry, Jiangxi Agricultural University, Nanchang, China
- 2Department of Biosciences, Rice University, Houston, TX, United States
Plant invasions affect biodiversity and seriously endanger the stability of ecosystems. Invasive plants show strong adaptability and growth advantages but are influenced by various factors. Soil bacteria and fungi are critical to plant growth and are important factors affecting plant invasions. Plant invasions also affect soil N2O emissions, but the effects of invasive plants from different population origins on N2O emissions and their microbial mechanisms are not clear. In this experiment, we grew Triadica sebifera from native (China) and invasive (USA) populations with or without bacterial (streptomycin) and/or fungal (iprodione) inhibitors in a factorial experiment in which we measured plant growth and soil N2O emissions of T. sebifera. Plants from invasive populations had higher leaf masses than those from native populations when soil bacteria were not inhibited (with or without fungal inhibition) which might reflect that they are more dependent on soil bacteria. Cumulative N2O emissions were higher for soils with invasive T. sebifera than those with a plant from a native population. Bacterial inhibitor application reduced cumulative N2O emissions but reductions were larger with application of the fungal inhibitor either alone or in combination with the bacterial inhibitor. This suggests that fungi play a strong role in plant performance and soil N2O emissions. Therefore, it is important to further understand the effects of soil microorganisms on the growth of T. sebifera and soil N2O emissions to provide a more comprehensive scientific basis for understanding the causes and consequences of plant invasions.
1. Introduction
With rapid industrialization, the concentration of greenhouse gases in the atmosphere has increased significantly, and global climate change is becoming increasingly problematic. The Paris Agreement proposed the goal of keeping the global average temperature less than 2°C above pre-industrial warming by 2,100. However, it is difficult to control warming below 1.5°C at present (Rogelj et al., 2016), and it is necessary to reduce total GHG emissions. Nitrous oxide (N2O) is an important greenhouse gas (Walling and Vaneeckhaute, 2020) that persists in the atmosphere for 121 years with a warming potential 265 times higher than that of CO2 on a 100-year scale (IPCC, 2014). N2O is also extremely damaging to the ozone layer (Ravishankara et al., 2009). While a variety of CO2 reduction measures are emerging, non-CO2 greenhouse gas reduction such as N2O should also receive attention.
Nitrous oxide is produced by complex nitrification and denitrification processes carried out by various microbial communities, including bacteria, fungi and archaea (Zhong et al., 2022). Soil N2O emissions are largely derived from these two processes (Hu et al., 2015), accounting for 70% of total global emissions (Fowler et al., 2013). Bacteria have been the main contributors to N2O release from soils (Johnson et al., 1996). However, in desert and semi-arid grassland soils, fungi are the main contributors (>70%) to N2O release (Marusenko et al., 2013). In order to reduce N2O emissions from agroforestry systems, studies have been conducted on the effects of bacterial and fungal inhibitors on N2O emissions. Herold et al. (2012) studied the effect of tilled soil on fungal and bacterial denitrification and biomass by adding cycloheximide (fungal inhibitor) and streptomycin (bacterial inhibitor). Castaldi and Smith (1998) found that the addition of peptone to forest soils significantly stimulated the release of N2O, while low concentrations of cycloheximide rapidly reduced N2O release, indicating that fungi are the main contributors to N2O release in forest soils. Biological inhibitors can be used to inhibit the activity of bacteria and fungi involved in nitrification and denitrification processes, and further studies are needed to investigate the effect of biological inhibitors on soil N2O emissions.
In the context of global climate change, plant invasion can affect biodiversity and jeopardize the stability of forest ecosystems (Bradley et al., 2010; Ehrenfeld, 2010). Soil microbes are an important component of forest ecosystems (Fritze et al., 1994), and bacteria and fungi are major components of soil microbial communities (Rousk et al., 2009). Soil biology is an important factor affecting plant performance and invasion potential (Reinhart and Callaway, 2006). Soil microorganisms (e.g., mycorrhizal fungi, rhizobia, etc.) can efficiently assist invasive plants to utilize nutrients such as nitrogen (Huang et al., 2016) and phosphorus (Zhang et al., 2013) to facilitate plant invasion. Invasive plants show strong adaptability and growth advantages but are influenced by various factors (Zou et al., 2006; Ehrenfeld, 2010; De Marco et al., 2022). For example, nitrogen deposition enhances the biomass and leaf area of invasive Triadica sebifera (Deng et al., 2019b) and increases the invasive potential of Mikania micrantha and Chromolaena odorata (Zhang et al., 2016). Higher temperatures, increased CO2, and nitrogen deposition can exacerbate plant invasion, and conversely, plant invasion can affect greenhouse gas emissions (Lei et al., 2010). Although there have been numerous studies on the impact of invasive exotic plants on ecosystem carbon and nitrogen cycles (Liao et al., 2007, 2008) knowledge of the mechanisms of N2O emissions is limited.
The growth and activity of soil microorganisms can be stimulated due to greater root biomass of invasive plants, production of more secretions, easier access to soil microorganisms, and increased rate of N uptake (Zou et al., 2006). Nijjer et al. (2008) found that exotic tree species benefited more from soil arbuscular mycorrhizal compared to native species in a pot experiment. This suggests that soil organisms play an important role in the invasion of alien species. There is also variation among populations of exotic plants in their interactions with soil organisms (Yang et al., 2015). For instance, T. sebifera plants from invasive populations have stronger soil microbial utilization capacity compared to those from native populations, confirming the role of soil microbes in promoting the growth of invasive T. sebifera (Zhang et al., 2012). However, the effects of invasive plants with different populations origins on N2O emissions and their microbial mechanisms are not clear.
Triadica sebifera (Euphorbiaceae; synonym Sapium sebiferum) is an economic tree endemic to China with a long history of cultivation and is an important industrial oilseed tree in China, combining economic and ornamental values. It was introduced to the United States as an oil species and ornamental tree in the late 18th century and has become invasive and impacted ecosystems in the southeastern United States in part because of increased competitive ability of invasive populations (Huang et al., 2012; Zhang et al., 2012, 2013, 2020). The effects of biotic and abiotic factors on plants of different species origins are complex (Reinhart and Callaway, 2006; Bradley et al., 2010). Invasive T. sebifera has higher plant height, stem and total biomass, higher nitrogen uptake rate and relative growth rate than native T. sebifera (Zou et al., 2007; Zhang et al., 2013; Deng et al., 2017), and lower root to shoot ratio (Zhang et al., 2017). Zou et al. (2006) found soil CO2 and N2O emissions were higher for soils associated with T. sebifera from invasive vs. native populations. But, the contributions of soil fungi and bacteria to growth of T. sebifera plants from different population origins and associated soil N2O emissions are unclear.
In this experiment, we grew T. sebifera from native (China) and invasive (USA) populations with or without bacterial (streptomycin) and/or fungal (iprodione) inhibitors in a factorial experiment in which we measured plant growth and soil N2O emissions of T. sebifera. We hypothesized that: (1) T. sebifera plants from invasive populations will be impacted more negatively by suppression of soil microbes than ones from native populations. (2) Suppression of soil microbes will have a larger impact on soil N2O emissions with T. sebifera plants from invasive populations.
2. Materials and methods
2.1. Seed and soil collection
In November 2018, we collected seeds from three populations of T. sebifera in both China (where it is native) and the United States (where it is introduced and now invasive) (Table 1). In April 2019, we collected T. sebifera non-rhizosphere soil (depth 0–20 cm after removing surface litter) from under three trees (>300 m apart) in Nanchang Jiangxi, China. We removed visible stones, plant root fragments and other intrusive materials from the soil, sieved the soil with a 2 cm sieve and mixed it thoroughly (Yang et al., 2013; Deng et al., 2019b).
2.2. Experimental design
This study site is located at Jiangxi Agricultural University, Nanchang, China (115°50′10″ E, 28°45′53″ N). It has a subtropical humid monsoon climate. The average annual temperature is 18°C, the maximum temperature in midsummer can reach above 40°C, and the minimum temperature in winter can reach below −10°C; the average annual rainfall is 1,600–1,700 mm, the rainfall is uneven in the year, the rainfall is concentrated in April-June, the soil type is typical red soil.
We conducted a factorial experiment in pots in a greenhouse. The experimental factors were bacterial inhibitor (control vs. streptomycin), fungal inhibitor (control vs. iprodione) and plant population origin (native vs. invasive). We applied 5ml of streptomycin solution (3 g kg–1) to bacterial inhibitor pots and 5ml of iprodione solution (1 g kg–1) to fungal inhibitor pots (Nijjer et al., 2008; Fang et al., 2021). We applied 5 ml of deionized water to control soils. We included three T. sebifera populations for each range. In total, there were 72 pots (two bacterial inhibitor × two fungal inhibitor × two origins × three populations × three replications).
Soil characteristics were: organic carbon 14.41 ± 2.19 g kg–1, total N 1.33 g kg–1, NH4+-N 1.12 mg kg–1, NO3–-N 1.24 ± 0.42 mg kg–1, total phosphorus 0.32 ± 0.03 g kg–1 and soil pH 5.26 ± 0.01 (Fang et al., 2021). In April 2019, we planted seeds into trays filled with potting soil. After seed germination, we selected similar-sized seedlings and individually transplanted them into 1.5 L plastic pots, which were filled with 1.5 kg of the treated T. sebifera non-rhizosphere soil. Each pot had a ring for mounting a gas collection chamber.
We measured N2O fluxes by gas chromatography of air samples collected in static opaque chambers. We placed the plexiglass, foil-wrapped chamber (60 cm tall, 18 cm diameter) into the water filled ring (between 2:30 and 5:30 p.m.). We collected 40 ml of gas with a plastic 60 ml syringe 0, 10, 20, and 30 min after chamber installation which we brought back to the laboratory for analysis. We took gas samples from July to October in 2019, at days 1, 6, 9, 12, 18, 28, 35, 38, 41, 48, 56, 65, and 80, starting 1 week after transplantation. Soil temperature (Supplementary Figures 1A, B), and moisture (5 cm depth) (Supplementary Figures 2A, B) were monitored simultaneously when N2O emission rates (Supplementary Figures 3A, B) were measured.
We measured plant height and leaf number then harvested leaves, stems, and roots separately. We dried them at 60°C for 72 h then weighed them and calculated root to shoot ratio and total mass.
2.3. Soil N2O emission determination
We estimated soil N2O emission rates as:
where F indicates soil N2O emission rate (μg m–2h–1),P stands for standard atmospheric pressure (Pa) (which should be adjusted if partial pressure of water vapor of chamber air taken into consideration) (Carter and Gregorich, 2007; Deng et al., 2019a), V refers to the volume of chamber headspace (m3), △c/△t means the rate of N2O (ppb) concentration change with time based on linear regressions (Domeignoz-Horta et al., 2018; Pärn et al., 2018), R stands for universal gas constant (m3 mol–1 k–1), T is the absolute air temperature (k), M means the molecular mass N2O (g mol–1), and S indicates the collar area (m2).
We estimated cumulative soil N2O emissions as:
where E indicates cumulative soil N2O emissions (μg m–2) (Deng et al., 2019a; He et al., 2019), F indicates soil N2O emission rate (μg m–2h–1), i means the ith measurement, (ti+1-ti) refers to the time span (days) between two measurements, and n means the total number of the measurements.
2.4. Date analyses
We used ANOVAs to test the fixed effects of bacterial inhibitor, fungal inhibitor, T. sebifera origin and the their interactions on plant height, root mass, stem mass, leaf mass, total mass, root to shoot ratio, soil N2O emission rate and cumulative N2O emissions. We included population nested within origin as a random effect. We used least significant difference (LSD) tests to distinguish among means for significant factors with more than two levels (α = 0.05). We performed all statistical analyses using JMP 9.0 Software (Gary, NC, USA).
3. Results
3.1. Soil temperature and soil moisture
Soil temperatures and moisture were similar among treatments for both invasive and native T. sebifera population pots (Supplementary Figures 1, 2). Soil temperature and moisture reached their minimum and maximums, respectively, in October at the end of the experiment (Supplementary Figures 1, 2).
3.2. Plant growth
Plant height depended on the interaction of bacterial inhibitor with fungal inhibitor and with population origin (Figure 1 and Table 2). Plants from invasive populations in control soils were taller than those from native populations with bacterial inhibitor (Figure 1A). Plants were shorter with bacterial inhibitor than in control soils but plants were even shorter with fungal inhibitor applied alone or with both the fungal and bacterial inhibitor (Figure 1C). A similar pattern was found for root, stem and total mass (Figures 1E, F and Table 2).
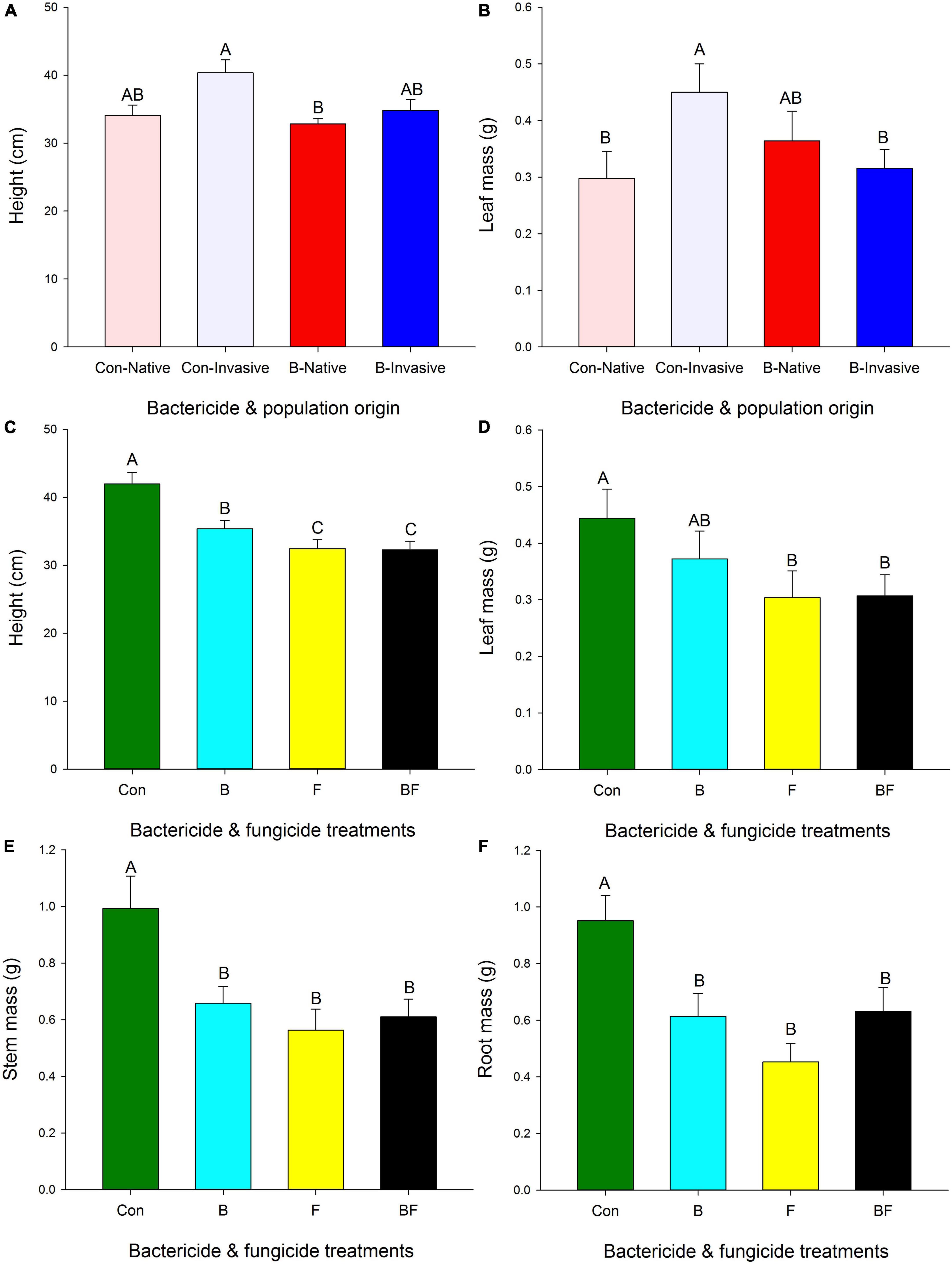
Figure 1. Effect of origin, bacterial and fungal inhibitor on height (A), leaf mass (B), height (C), leaf mass (D), stem mass (E), root mass (F) of Triadica sebifera (mean ± se). Con, control; B, bacteria inhibitor; F, fungal inhibitor; BF, bacteria inhibitor and fungal inhibitor. Means with the same letters did not differ in post-hoc tests (p < 0.05).
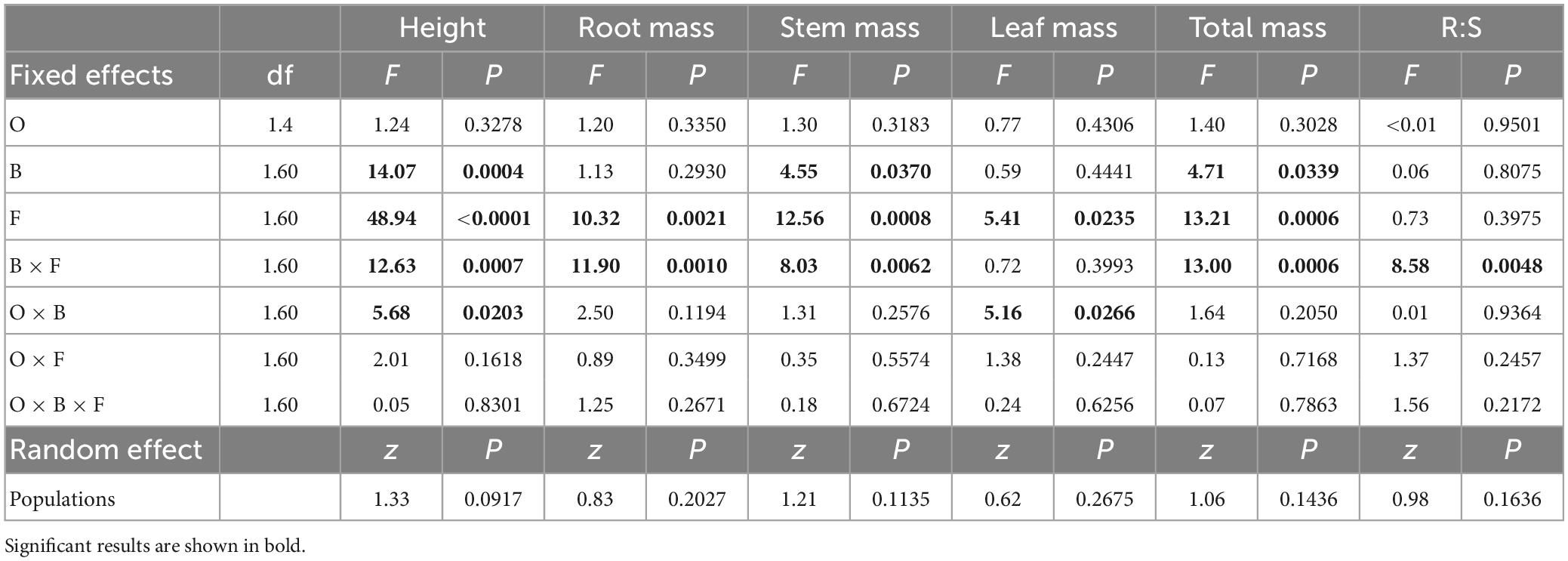
Table 2. The dependence of growth on Triadica sebifera with different populations, origin (O), bacteria inhibitor (B), fungal inhibitor (F), and their interaction in ANOVAs.
Leaf mass was lower with fungal inhibitor (Figure 1D and Table 2). The interaction between the bacterial inhibitor and T. sebifera population origin had a significant effect on the leaf mass of T. sebifera (Table 2). Under the control, the leaf mass of T. sebifera was significantly higher than that of the native T. sebifera, while the leaf mass of T. sebifera of the invasive population origin was significantly lower after the application of bacterial inhibitors (Figure 1B). Root:shoot depended on the interaction of bacterial and fungal inhibitors with lower root:shoot with either inhibitor applied alone (B = 0.60, F = 0.52) than when neither (0.66) or both (0.69) were applied (Table 2). Population did not significantly affect any variable (Table 2).
3.3. Soil N2O emission rate and cumulative N2O emission
Both N2O emission rate (+13.73%) and cumulative N2O emissions were higher with soils associated with plants from invasive vs. native populations (Figure 2A, Table 3, and Supplementary Figure 3A). The strength of this effect on soil N2O emissions was stronger later in the experiment (Figure 3A and Table 3). Application of bacterial inhibitors, fungal inhibitors and both had significant negative effects on N2O emission rates compared to untreated control soils (decreased by −22.23, −43.81, and −46.04%, respectively) (Supplementary Figure 3B and Table 3). These effects were stronger at times of higher N2O emissions (Figure 3B). Cumulative N2O emissions showed a similar dependence on inhibitors with smaller reductions with bacterial inhibitor alone than with the application of fungal inhibitor alone or with the bacterial inhibitor (Figure 2B and Table 3).
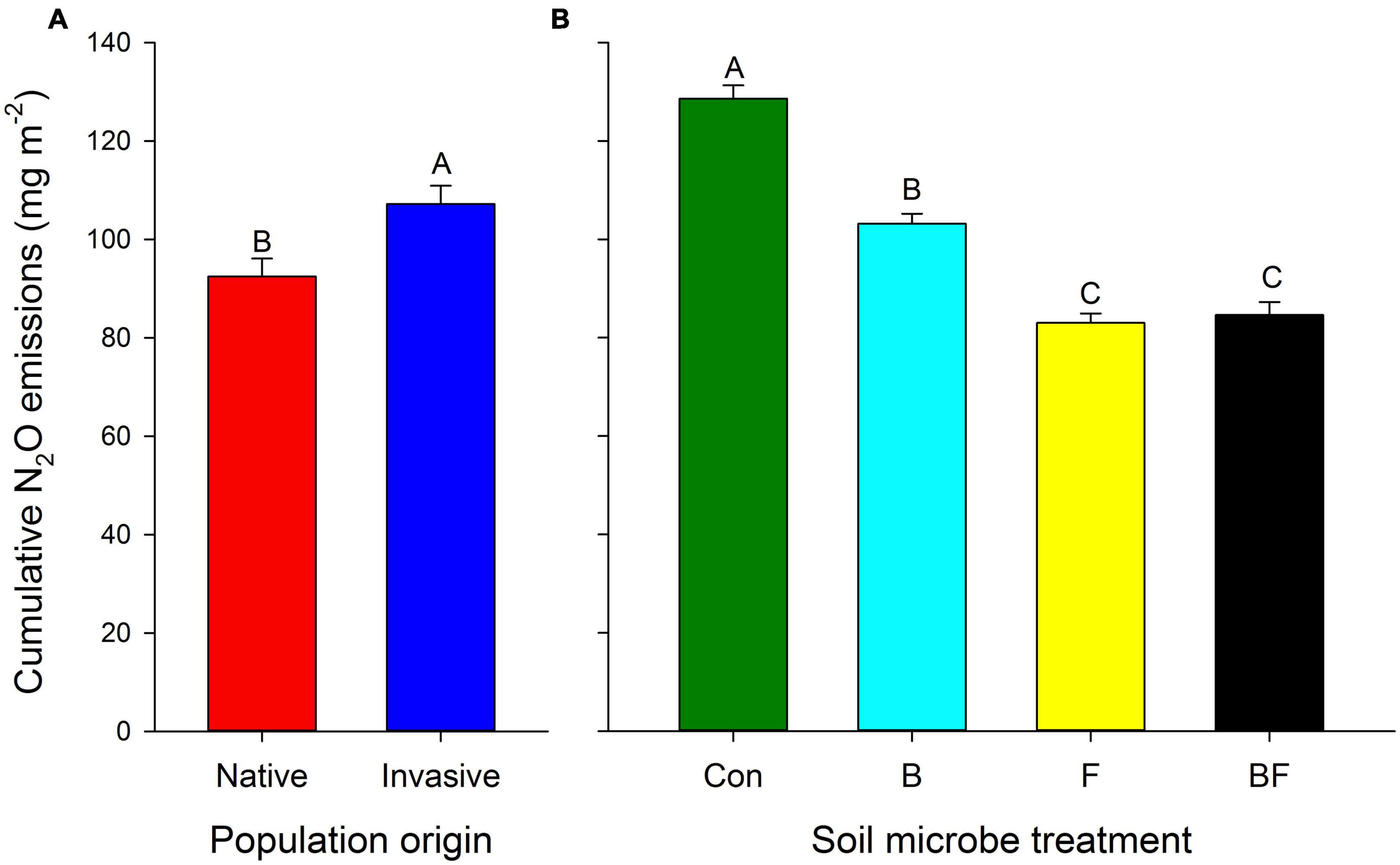
Figure 2. Cumulative N2O emissions (mean ± se) (A) population origin treatments, and (B) under control (Con), bacterial inhibitor (B), fungal inhibitor (F) and combination (BF) treatments. Different capital letters indicate significant differences (p < 0.05).
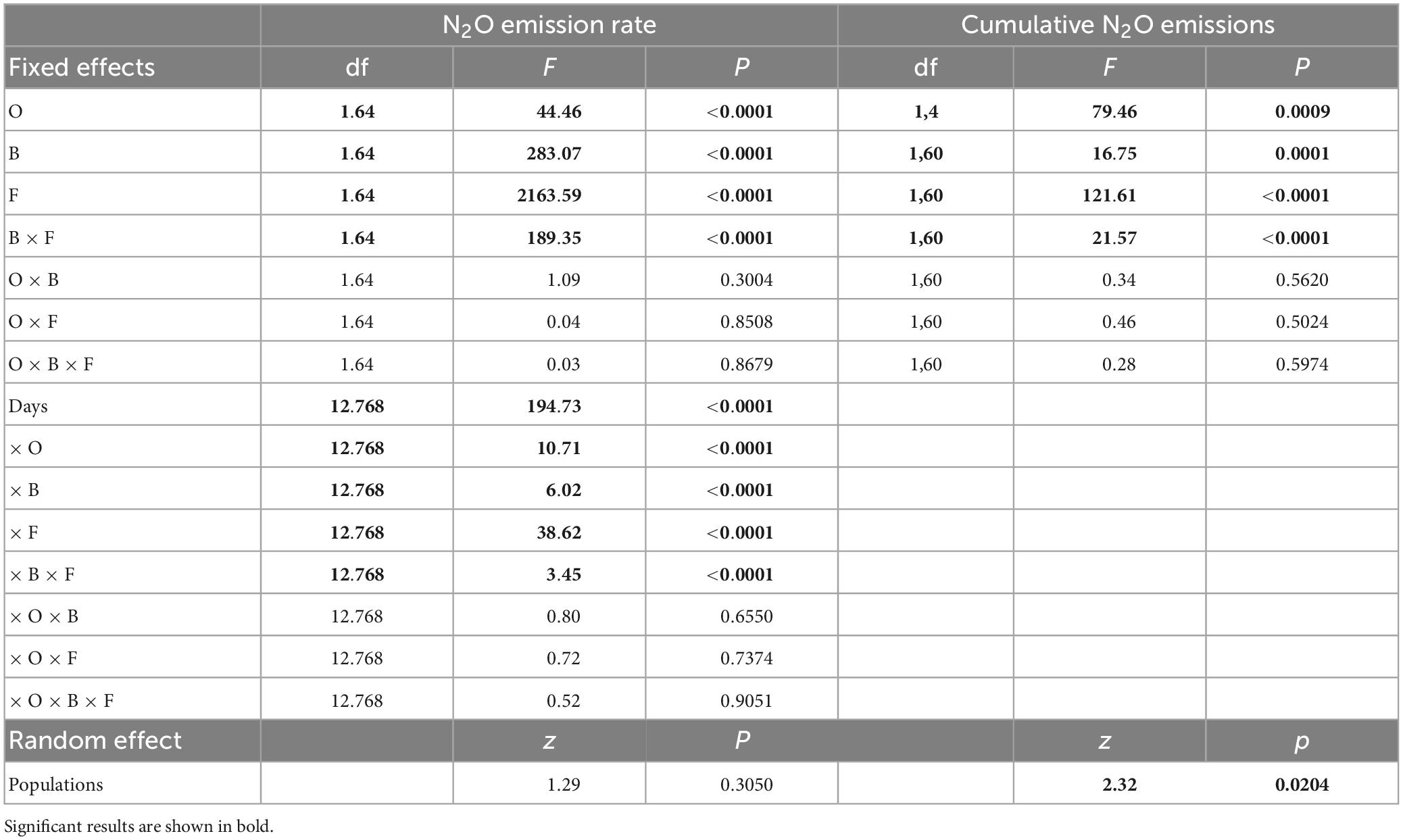
Table 3. The dependence of N2O emission on Triadica sebifera with different populations, origin (O), bacteria inhibitor (B), fungal inhibitor (F), and their inhibitor as a fixed factor and measurement time (day) as a random factor in ANOVAs.
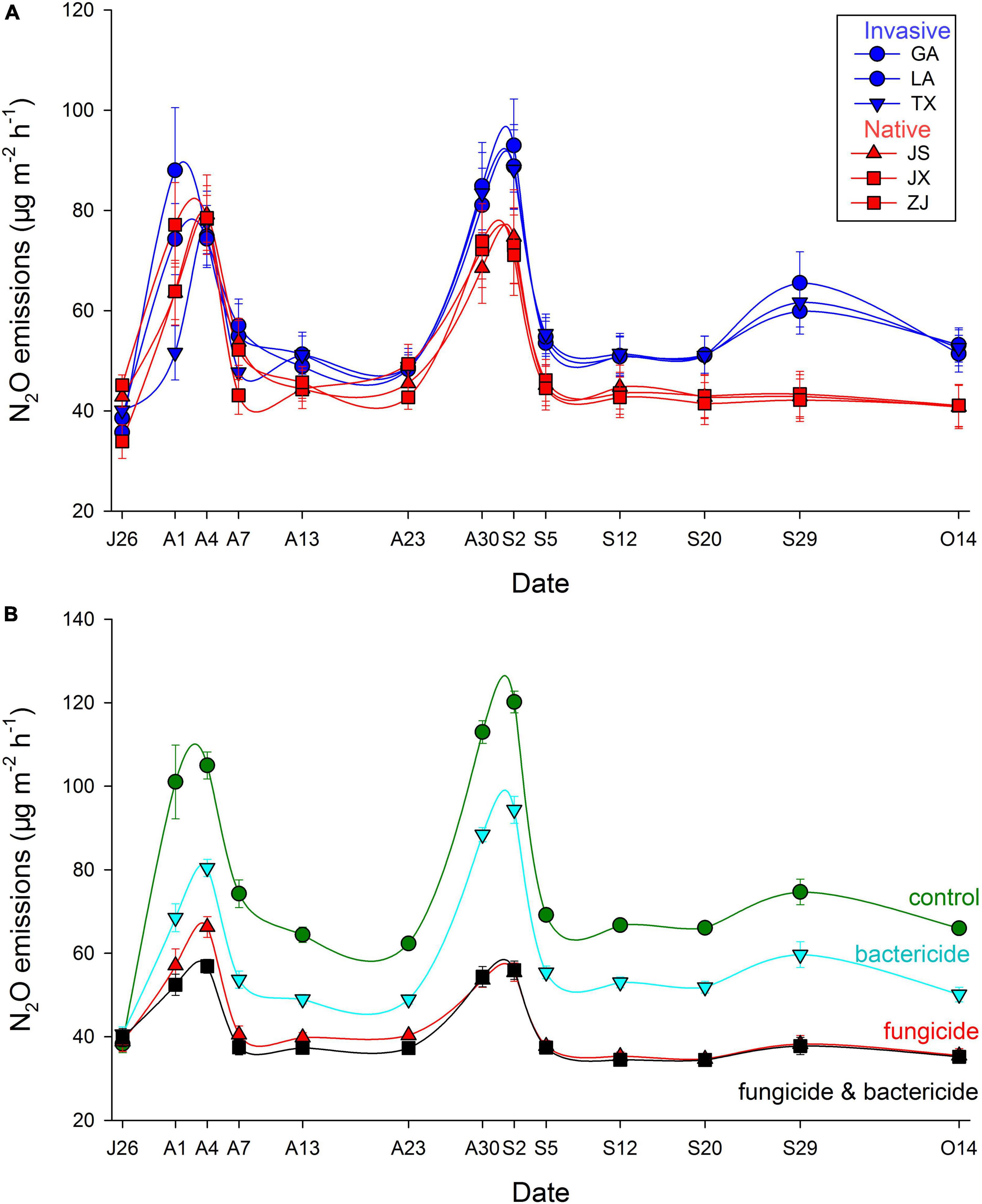
Figure 3. Dynamics of soil N2O emission of different origins in the (A) native (China) and (B) invasive sites (USA) with bacterial and fungal inhibitor (mean ± se).
4. Discussion
4.1. Effect of population origin and inhibitors on growth of T. sebifera
The bacterial inhibitor had a larger negative impact on the height and leaf mass of T. sebifera plants from invasive populations more than ones from native populations (Figures 1A, B). Other studies have found that the interactions of T. sebifera from invasive populations with soil microbes differ from those of plants from native populations (e.g., Zou et al., 2007; Yang et al., 2015; Shad et al., 2022). For instance, soil microorganisms can promote the rapid mineralization and uptake of soil organic N by T. sebifera, and it has been found that invasive T. sebifera promotes soil N mineralization more than native T. sebifera (Zhang et al., 2012, 2017). Increased rates of N uptake by invasive T. sebifera will likely also stimulate the growth and activity of soil microorganisms (Zou et al., 2006, 2007; Zhang et al., 2013). Soil microbial communities play a critical role in the successful invasion of plants (Reinhart and Callaway, 2006; Callaway et al., 2011; Zhang et al., 2012) so this finding that invasive and native populations differ in their responses to inhibition of soil bacteria suggests that there may be important differences in the interactions of T. sebifera with soil bacteria in the invasive vs. native ranges.
The bacterial and fungal inhibitors had widespread effects on T. sebifera performance that did not depend on plant population origin. Across a number of response variables, the bacterial inhibitor decreased T. sebifera performance but these decreases were generally much smaller than the negative impacts of application of the fungal inhibitor alone or in combination with the bacterial inhibitor. Soil beneficial microorganisms (e.g., mycorrhizal fungi, rhizobia, etc.) can promote plant invasions by increasing uptake of nitrogen, water and phosphorus from the soil (Reinhart and Callaway, 2006; Callaway et al., 2011; Huang et al., 2016). Because T. sebifera receives significant benefits from mycorrhizal associations, it is likely suppression of mycorrhizae contributed to the large negative effect of the fungal inhibitor on plant performance (Nijjer et al., 2007, 2008; Yang et al., 2015; Deng et al., 2017). The relatively lower impact of suppression of soil bacteria on T. sebifera performance may reflect the fact that it does not have a close symbiotic relationship with bacteria such as hosting nitrogen fixing bacteria (Pile et al., 2017). However, a larger impact of bacterial inhibition vs. fungal inhibition might occur for a plant that hosts rhizobia but is not mycorrhizal.
4.2. Effect of population origin and inhibitors on soil N2O emissions of T. sebifera
Soil N2O emissions are mainly produced by microbially mediated nitrification and denitrification (Hu et al., 2015), and microbial community structure and abundance affect soil N2O emissions (Banerjee et al., 2016). Zhang et al. (2018) found that perennial invasive plants affect soil GHG emissions, and invasive sites increased soil N2O emissions compared to the native sites. Triadica sebifera plants from invasive populations had a higher N2O emission rate (Supplementary Figure 3A) and cumulative N2O emission (Figure 2A) than those from native populations, which is consistent with the findings of Zou et al. (2006) and Zhang et al. (2018). One likely explanation for this stimulation of N2O emissions is the higher root activity found for T. sebifera from invasive populations reviewed by Pile et al. (2017).
Fang et al. (2022) found that nitrification and denitrification rates and subsequent soil N2O emissions were inhibited when bacterial and fungal inhibitors were applied, and that streptomycin and iprodione applied alone or simultaneously had an inhibitory effect on N2O emission rates. This is consistent with our experimental results, where both bacterial and fungal inhibitors and their interactions had a significant inhibitory effect on cumulative N2O emission compared to the control (Figure 2B). Not only are bacteria the main contributors to N2O release from soil (Johnson et al., 1996), but fungi also play an important role. Fungal nitrification occurs mainly in acidic forest soils, and denitrification occurs mainly in forest soils, grassland soils, and semi-arid areas, where it plays a major role in N2O release (Huang and Long, 2014). Cumulative N2O emissions with fungal inhibitors were significantly lower than those from bacterial inhibitors (Figure 2B). It showed that both fungi and bacteria played an important role in soil N2O emissions, but fungi contributed more to promote T. sebifera soil N2O emissions. It is important to note, however, that there could be non-target effects of the bacterial and/or fungal inhibitors that we did not explicitly test in this experiment.
Climate change can lead to changes in soil temperature and humidity, which can affect the rate of nitrogen mineralization (Guntiñas et al., 2012), and it is important to include soil temperature and humidity when studying soil greenhouse gas emissions. It has been shown that CO2 emissions from different land uses are significantly and positively correlated with soil temperature, and that N2O emission fluxes from different land use types increase and then decrease with increasing soil moisture, reaching a maximum at 25% gravity water content (Sang et al., 2021). Although soil temperature and moisture were not confounded with our treatments here, the insights from this research area would be strengthened by having a variety of environmental treatments.
Exotic plant invasion and global climate change interact to shape greenhouse gas emissions with climate change promoting plant invasion and plant invasion affecting greenhouse gas emissions such as N2O by influencing ecosystem carbon and nitrogen cycles (Yuan et al., 2015). Plant invasion can have an impact on GHG emissions, such as increasing soil N2O emissions (Zou et al., 2006; Zhang et al., 2018). Increased nitrogen deposition, increased greenhouse gas emissions, and higher temperatures affect ecosystem stability and lead to increased plant invasiveness (Lei et al., 2010; Bradley et al., 2012; Deng et al., 2019b). Studies have been conducted to combine global climate change and plant invasion (Fennell et al., 2013; Huang et al., 2013; Sorte et al., 2013), but scientific studies on how non-CO2 greenhouse gases such as N2O interact with plant invasion are still lacking, and research in this area should continue to be strengthened to understand the causes and consequences of plant invasions, especially in the context of greenhouse gas emissions.
5. Conclusion
We found that soil microorganisms play an important role in the performance of T. sebifera with fungal inhibition causing large decreases in plant performance and bacterial inhibition causing smaller decreases in plant performance, especially for plants from native populations that were less sensitive to bacterial suppression. The application of bacterial and fungal inhibitors was effective in mitigating soil N2O emissions, with fungal inhibitors being more effective. Application of fungal inhibitors alone had lower cumulative N2O emission than bacterial inhibitors alone, indicating that fungi played an important role in contributing to soil N2O emissions. More long-term, comprehensive studies are needed to more fully understand the effects of this potential microbial mechanism on invasive plant growth and soil N2O emissions.
Data availability statement
The original contributions presented in this study are included in the article/Supplementary material, further inquiries can be directed to the corresponding author.
Author contributions
XL was responsible for writing this manuscript, analyzed and processed the data, and made figures and tables. LZ designed the experiment and reviewed and edited the manuscript. LL and HF helped with the experiment. NS, JB, XZ, YY, and HW reviewed and edited the manuscript. ES reviewed and edited the manuscript, and revised the tables and figures. All authors contributed to the article and agreed to the final version of the manuscript.
Funding
This study was supported by the National Natural Science Foundation of China (31770749) and Jiangxi “Double Thousand Plan” Science and Technology Innovation High-end Talent Project (jxsq2019201078).
Acknowledgments
We thank Prof. Jianqing Ding and Dr. Baoliang Tian of Henan University for providing seeds of different populations of Triadica sebifera.
Conflict of interest
The authors declare that the research was conducted in the absence of any commercial or financial relationships that could be construed as a potential conflict of interest.
Publisher’s note
All claims expressed in this article are solely those of the authors and do not necessarily represent those of their affiliated organizations, or those of the publisher, the editors and the reviewers. Any product that may be evaluated in this article, or claim that may be made by its manufacturer, is not guaranteed or endorsed by the publisher.
Supplementary material
The Supplementary Material for this article can be found online at: https://www.frontiersin.org/articles/10.3389/ffgc.2023.1183336/full#supplementary-material
References
Banerjee, S., Helgason, B., Wang, L., Winsley, T., Ferrari, B. C., and Siciliano, S. D. (2016). Legacy effects of soil moisture on microbial community structure and N2O emissions. Soil Biol. Biochem. 95, 40–50. doi: 10.1016/j.soilbio.2015.12.004
Bradley, B. A., Blumenthal, D. M., Wilcove, D. S., and Ziska, L. H. (2010). Predicting plant invasions in an era of global change. Trends Ecol. Evol. 25, 310–318. doi: 10.1016/j.tree.2009.12.003
Bradley, B. A., Blumenthal, D. M., Early, R., Grosholz, E. D., Lawler, J. J., Mille, L. P., et al. (2012). Global change, global trade, and the next wave of plant invasions. Front. Ecol. Environ. 10:20–28. doi: 10.1890/110145
Callaway, R. M., Bedmar, E. J., Reinhart, K. O., Silvan, C. G., and Klironomos, J. (2011). Effects of soil biota from different ranges on Robinia invasion: Acquiring mutualists and escaping pathogens. Ecology 92, 1027–1035. doi: 10.1890/10-0089.1
Castaldi, S., and Smith, K. A. (1998). Effect of cycloheximide on N2O and NO3–production in a forest and an agricultural soil. Biol. Fertil. Soils 27, 27–34. doi: 10.1007/s003740050395
Carter, M. R., and Gregorich, E. G. (2007). Soil sampling and methods of analysis. Boca Raton, FL: CRC Press. doi: 10.1201/9781420005271
De Marco, A., Panico, S. C., Memoli, V., Santorufo, L., Zarrelli, A., Barile, R., et al. (2022). Differences in soil carbon and nitrogen pools between afforested pine forests and natural shrublands in a Mediterranean area. Appl. Soil Ecol. 170:104262. doi: 10.1016/j.apsoil.2021.104262
Deng, B. L., Liu, Q., Liu, X. S., Zheng, L. Y., Jiang, L. B., Guo, X. M., et al. (2017). Effects of enhanced UV-B radiation and nitrogen deposition on the growth of invasive plant Triadica sebifera. Chin. J. Plant Ecol. 41, 471–479. doi: 10.17521/cjpe.2016.0292
Deng, B., Liu, X., Zheng, L., Liu, Q., Guo, X., and Zhang, L. (2019b). Effects of nitrogen deposition and UV-B radiation on seedling performance of Chinese tallow tree (Triadica sebifera): A photosynthesis perspective. For. Ecol. Manage. 433, 453–458. doi: 10.1016/j.foreco.2018.11.038
Deng, B, Fang, H., Jiang, N., Feng, W., Luo, L., Wang, J., et al. (2019a). Biochar is comparable to dicyandiamide in the mitigation of nitrous oxide emissions from Camellia oleifera Abel. fields. Forests 1012:1076. doi: 10.3390/f10121076
Domeignoz-Horta, L. A., Philippot, L., Peyrard, C., Bru, D., Breuil, M. C., Bizouard, F., et al. (2018). Peaks of in situ N2O emissions are influenced by N2O-producing and reducing microbial communities across arable soils. Glob. Chang. Biol. 24, 360–370. doi: 10.1111/gcb.13853
Ehrenfeld, J. G. (2010). Ecosystem consequences of biological invasions. Annu. Rev. Ecol. Evol. Syst. 41, 59–80. doi: 10.1146/annurev-ecolsys-102209-144650
Fang, H. F., Feng, W. X., Luo, L. C., Gao, Y., Wang, B. H., Nasir, S., et al. (2021). Effects of soil microorganisms on chlorophyll fluorescence characteristics of invasive Triadica sebifera with nitrogen deposition. Acta Ecol. Sin. 41, 9377–9387. doi: 10.5846/stxb202004080828
Fang, H., Gao, Y., Zhang, Q., Ma, L., Wang, B., Shad, N., et al. (2022). Moso bamboo and Japanese cedar seedlings differently affected soil N2O emissions. J. Plant Ecol. 15, 277–285. doi: 10.1093/jpe/rtab091
Fennell, M., Murphy, J. E., Gallagher, T., and Osborne, B. (2013). Simulating the effects of climate change on the distribution of an invasive plant, using a high resolution, local scale, mechanistic approach: challenges and insights. Glob. Chang. Biol. 19, 1262–1274. doi: 10.1111/gcb.12102
Fowler, D., Coyle, M., Skiba, U., Sutton, M. A., Cape, J. N., Reis, S., et al. (2013). The global nitrogen cycle in the twenty-first century. Philos. T. R. Soc. B 368:20130164. doi: 10.1098/rstb.2013.0164
Fritze, H., Smolander, A., Levula, T., Kitunen, V., and Mälkönen, E. (1994). Wood-ash fertilization and fire treatments in a Scots pine forest stand: Effects on the organic layer, microbial biomass, and microbial activity. Biol. Fertil. Soils. 17, 57–63.
Guntiñas, M. E., Leirós, M. C., Trasar-Cepeda, C., and Gil-Sotres, F. (2012). Effects of moisture and temperature on net soil nitrogen mineralization: A laboratory study. Eur. J. Soil Biol. 48, 73–80. doi: 10.1016/j.ejsobi.2011.07.015
Herold, M. B., Baggs, E. M., and Daniell, T. J. (2012). Fungal and bacterial denitrification are differently affected by long-term pH amendment and cultivation of arable soil. Soil Boil. Biochem. 54, 25–35. doi: 10.1016/j.soilbio.2012.04.031
He, T., Yuan, J., Luo, J., Wang, W., Fan, J., Liu, D., et al. (2019). Organic fertilizers have divergent effects on soil N2O emissions. Biol. Fertil. Soils 55, 685–699. doi: 10.1007/s00374-019-01385-4
Huang, J., Xu, X., Wang, M., Nie, M., Qiu, S., Wang, Q., et al. (2016). Responses of soil nitrogen fixation to Spartina alterniflora invasion and nitrogen addition in a Chinese salt marsh. Sci. Rep. 6:20384. doi: 10.1038/srep20384
Huang, W., Carrillo, J., Ding, J., and Siemann, E. (2012). Interactive effects of herbivory and competition intensity determine invasive plant performance. Oecologia 170, 373–382. doi: 10.1007/s00442-012-2328-6
Huang, W., Wang, Y., and Ding, J. Q. (2013). A review of adaptive evolution of defense strategies in an invasive plant species, Chinese tallow (Triadica sebifera). Chin. J. Plant Ecol. 37, 889–900. doi: 10.3724/SP.J.1258.2013.00092
Huang, Y., and Long, X. E. (2014). Contribution of fungi to soil nitrous oxide emission and their research methods: A review. Chin. J. Appl. Ecol. 25, 1213–1220.
Hu, H. W., Chen, D., and He, J. Z. (2015). Microbial regulation of terrestrial nitrous oxide formation: Understanding the biological pathways for prediction of emission rates. FEMS Microbiol. Rev. 39, 729–749. doi: 10.1093/femsre/fuv021
IPCC (2014). Synthesis Report, Climate Change. Geneva, Switzerland: Contribution of working groups I, II, III to the fifth assessment report of the Intergovernmental Panel on Climate Change. Geneva: IPCC, 1–164.
Johnson, C. K., Vigil, M. F., Doxtader, K. G., and Beard, W. E. (1996). Measuring bacterial and fungal substrate-induced respiration in dry soils. Soil Boil. Biochem. 28, 427–432. doi: 10.1016/0038-0717(96)00004-1
Lei, Y., Xiao, H., and Feng, Y. (2010). Impacts of alien plant invasions on biodiversity and evolutionary responses of native species. Biodiv. Sci. 18:622. doi: 10.3724/SP.J.1003.2010.622
Liao, C., Luo, Y., Jiang, L., Zhou, X., Wu, X., Chen, J., et al. (2007). Invasion of Spartina alterniflora enhanced ecosystem carbon and nitrogen stocks in the Yangtze estuary, China. Ecosystems 10, 1351–1361. doi: 10.1007/s10021-007-9103-2
Liao, C., Peng, R., Luo, Y., Zhou, X., Wu, X., Chen, J., et al. (2008). Altered ecosystem carbon and nitrogen cycles by plant invasion: A meta-analysis. New Phytol. 177, 706–714. doi: 10.1111/j.1469-8137.2007.02290.x
Marusenko, Y., Huber, D. P., and Hall, S. J. (2013). Fungi mediate nitrous oxide production but not ammonia oxidation in aridland soils of the southwestern US. Soil Boil. Biochem. 63, 24–36. doi: 10.1016/j.soilbio.2013.03.018
Nijjer, S., Rogers, W. E., Lee, C. T. A., and Siemann, E. (2008). The effects of soil biota and fertilization on the success of Sapium sebiferum. Appl. Soil Ecol. 38, 1–11. doi: 10.1016/j.apsoil.2007.08.002
Nijjer, S., Rogers, W. E., and Siemann, E. (2007). Negative plant-soil feedbacks may limit persistence of an invasive tree due to rapid accumulation of soil pathogens. Proc. R. Soc. B: Biol. Sci. 274, 2621–2627.
Pärn, J., Verhoeven, J. T., Butterbach-Bahl, K., Dise, N. B., Ullah, S., Aasa, A., et al. (2018). Nitrogen-rich organic soils under warm well-drained conditions are global nitrous oxide emission hotspots. Nat. Commun. 9:1135. doi: 10.1038/s41467-018-03540-1
Pile, L. S., Wang, G. G., Stovall, J. P., Siemann, E., Wheeler, G. S., and Gabler, C. A. (2017). Mechanisms of Chinese tallow (Triadica sebifera) invasion and their management implications - A review. For. Ecol. Manage. 404, 1–13. doi: 10.1016/j.foreco.2017.08.023
Ravishankara, A. R., Daniel, J. S., and Portmann, R. W. (2009). Nitrous oxide (N2O): The dominant ozone-depleting substance emitted in the 21st century. Science 326, 123–125. doi: 10.1126/science.1176985
Reinhart, K. O., and Callaway, R. M. (2006). Soil biota and invasive plants. New Phytol. 170, 445–457. doi: 10.1111/j.1469-8137.2006.01715.x
Rogelj, J., Den Elzen, M., Höhne, N., Fransen, T., Fekete, H., and Winkler, H. (2016). Paris Agreement climate proposals need a boost to keep warming well below 2oC. Nature 534, 631–639. doi: 10.1038/nature18307
Rousk, J., Brookes, P. C., and Bååth, E. (2009). Contrasting soil pH effects on fungal and bacterial growth suggest functional redundancy in carbon mineralization. Appl. Environ. Microbiol. 75, 1589–1596. doi: 10.1128/AEM.02775-08
Sang, W. X., Yang, H. L., and Tang, J. W. (2021). Response of soil greenhouse gas emissions to temperature and moisture across different land-use types. J. East Chin. Norm. Univ (Nat Sci). 2021, 109–120.
Shad, N., Liu, Q., Fang, H., Wang, B., Gao, Y., Liu, X., et al. (2022). Soil sterilization and fertility impacts on urease and belowground mass specific phosphatase activity vary among Chinese tallow tree (Triadica sebifera) populations. Plant Ecol. 223, 397–406. doi: 10.1007/s11258-021-01215-4
Sorte, C. J., Ibáñez, I., Blumenthal, D. M., Molinari, N. A., Miller, L. P., Grosholz, E. D., et al. (2013). Poised to prosper? A cross-system comparison of climate change effects on native and non-native species performance. Ecol. lett. 16, 261–270. doi: 10.1111/ele.12017
Walling, E., and Vaneeckhaute, C. (2020). Greenhouse gas emissions from inorganic and organic fertilizer production and use: A review of emission factors and their variability. J. Environ. Manage. 276:111211. doi: 10.1016/j.jenvman.2020.111211
Yang, Q., Carrillo, J., Jin, H., Shang, L., Hovick, S. M., Nijjer, S., et al. (2013). Plant-soil biota interactions of an invasive species in its native and introduced ranges: Implications for invasion success. Soil Biol. Biochem. 65, 78–85. doi: 10.1016/j.soilbio.2013.05.004
Yang, Q., Li, B., and Siemann, E. (2015). The effects of fertilization on plant-soil interactions and salinity tolerance of invasive Triadica sebifera. Plant Soil 394, 99–107. doi: 10.1007/s11104-015-2520-7
Yuan, J., Ding, W., Liu, D., Kang, H., Freeman, C., Xiang, J., et al. (2015). Exotic Spartina alterniflora invasion alters ecosystem-atmosphere exchange of CH4 and N2O and carbon sequestration in a coastal salt marsh in China. Glob. Chang. Biol. 21, 1567–1580. doi: 10.1111/gcb.12797
Zhang, J., Siemann, E., Tian, B., Huang, W., and Ding, J. (2020). Differences in seed properties and germination between native and introduced populations of Triadica sebifera. J. Plant Ecol. 13, 70–77. doi: 10.1093/jpe/rtz048
Zhang, L., Chen, X., and Wen, D. (2016). Interactive effects of rising CO2 and elevated nitrogen and phosphorus on nitrogen allocation in invasive weeds Mikania micrantha and Chromolaena odorata. Biol. Invasions 18, 1391–1407. doi: 10.1007/s10530-016-1089-2
Zhang, L., Wang, H., Chen, N. N., and Zou, J. W. (2012). Effects of soil biotic communities on the seedling performance of native and invasive provenances of Triadica sebifera. J. Biosafety 21, 41–45.
Zhang, L., Wang, S., Liu, S., Liu, X., Zou, J., and Siemann, E. (2018). Perennial forb invasions alter greenhouse gas balance between ecosystem and atmosphere in an annual grassland in China. Sci. Total Environ. 642, 781–788. doi: 10.1016/j.scitotenv.2018.06.111
Zhang, L., Zhang, Y., Wang, H., Zou, J., and Siemann, E. (2013). Chinese tallow trees (Triadica sebifera) from the invasive range outperform those from the native range with an active soil community or phosphorus fertilization. PLoS One 8:e74233. doi: 10.1371/journal.pone.0074233
Zhang, L., Zou, J., and Siemann, E. (2017). Interactive effects of elevated CO2 and nitrogen deposition accelerate litter decomposition cycles of invasive tree (Triadica sebifera). For. Ecol. Manage. 385, 189–197. doi: 10.1016/j.foreco.2016.11.045
Zhong, L., Qing, J., Liu, M., Cai, X., Li, G., Chen, G., et al. (2022). Fungi and archaea control soil N2O production potential in Chinese grasslands rather than bacteria. Front. Microbiol. 13:1494. doi: 10.3389/fmicb.2022.844663
Zou, J., Rogers, W. E., DeWalt, S. J., and Siemann, E. (2006). The effect of Chinese tallow tree (Sapium sebiferum) ecotype on soil–plant system carbon and nitrogen processes. Oecologia 150, 272–281. doi: 10.1007/s00442-006-0512-2
Keywords: bacterial inhibitor, fungal inhibitor, soil microorganisms, plant growth, soil N2O emissions, invasive plant
Citation: Lai XQ, Luo LC, Fang HF, Zhang L, Shad N, Bai J, Li AX, Zhang X, Yu YD, Wang H and Siemann E (2023) Bacterial and fungal inhibitor interacted impacting growth of invasive Triadica sebifera and soil N2O emissions. Front. For. Glob. Change 6:1183336. doi: 10.3389/ffgc.2023.1183336
Received: 10 March 2023; Accepted: 05 May 2023;
Published: 24 May 2023.
Edited by:
Matthieu Chauvat, Université de Rouen, FranceReviewed by:
Qiang Yang, Lanzhou University, ChinaChristina Biasi, University of Eastern Finland, Finland
Copyright © 2023 Lai, Luo, Fang, Zhang, Shad, Bai, Li, Zhang, Yu, Wang and Siemann. This is an open-access article distributed under the terms of the Creative Commons Attribution License (CC BY). The use, distribution or reproduction in other forums is permitted, provided the original author(s) and the copyright owner(s) are credited and that the original publication in this journal is cited, in accordance with accepted academic practice. No use, distribution or reproduction is permitted which does not comply with these terms.
*Correspondence: Ling Zhang, bGluZ3poYW5nMDlAMTI2LmNvbQ==