- 1Department of Forestry and Management of Environment and Natural Resources, Democritus University of Thrace, Orestiada, Greece
- 2INRAE, BEF, Nancy, France
- 3Forest Research Institute, Hellenic Agricultural Organization Dimitra, Thessaloniki, Greece
- 4Université de Lorraine, AgroParisTech, INRAE, Nancy, France
- 5Laboratory of Environmental Fuels and Hydrocarbons, Center for Research and Technology Hellas, Thessaloniki, Greece
Robinia pseudoacacia L. (black locust) has been extensively used for restoring degraded lands, following anthropogenic interventions like coal mining. Here we have addressed the contribution of black locust restoration plantations, established on overburden post-mining material, to carbon storage and to soil nitrogen enrichment at the largest lignite center in Greece. Carbon stocks and fluxes in all pools of the ecosystem, as well as the foliar nitrogen resorption efficiency and soil N stocks were quantified and the effect of plantations’ age was tested. The young age of the plantations (4–24 years) resulted in a relatively low total ecosystem C stock (56.7 t ha−1), which was partitioned among the different pools in the following order: above-ground biomass (50%) > black locust-derived SOC (24%) > coarse roots (14%) > deadwood (6%) > forest floor (5%) > fine roots (less than 1%). Litterfall started early in the growing season and together with fine roots that had a turnover rate of 0.62 yr−1, fueled soil organic carbon. SOC accrual, referring to the accumulation of SOC derived by black locust, declined with age. However, further SOC accumulation is expected, based on the potential SOC storage capacity of soil at the area. C stocks in above- and below-ground biomass increased linearly with age. The same response was observed for soil N stock and NRE, indicating that despite the N2-fixing capacity of black locust, there was still a poor pedospheric N supply and a need for efficient N cycling. Overall, the studied restoration plantations have a considerable contribution to C and N accumulation at the degraded post-mining sites. These positive effects are expected to further increase at least until the plantations reach maturity.
1. Introduction
Anthropogenic CO2 emissions have been drastically increasing in the last several decades and are linked to global climate change (Malhi et al., 2021; Friedlingstein et al., 2022). Among other human activities, high CO2 emissions are due to the intensive and long-term utilization of coal and lignite resources. Open-cast lignite mines have been operating for decades to support the energy needs of several European countries, including Germany, Greece and others (Alves Dias et al., 2018; Deshaies, 2020; Ziouzios et al., 2021). These drastic interventions led to negative environmental impacts over large areas (Papadopoulos et al., 2015; Wang et al., 2016, 2022), and specifically to degradation of soil organic matter and total soil nitrogen (Wang et al., 2021).
The need for climate neutrality by 2050 is a goal highlighted by the European Green Deal and other initiatives in order to implement the Paris Agreement in regard to greenhouse emissions. To this end, forests can play a key role by sequestering almost 900 Pg C worldwide with an annual carbon uptake of 1.1 Pg C (Sabatini et al., 2019). Particularly forest plantations are promoted by the Common Agricultural Policy in Europe to restore reclaimed mine soils, which consist of the overburden material, like in the case of opencast lignite mines (Pavloudakis et al., 2020). These plantations support pedogenesis, soil nutrient enrichment and carbon sequestration in biomass and in the soils (Amichev et al., 2008; Chatterjee et al., 2009), the latter being the greatest carbon reservoir globally (FAO and ITPS, 2015). The recovery of element cycling in the plant – soil system is often linked to stand age (Sun et al., 2016; Deng et al., 2019). Age may also influence soil C and N sequestration, although both increasing and decreasing soil C and N contents with age were reported (Akala and Lal, 2001; Lorenz and Lal, 2007). Additionally, trees and species’ identity affect soil properties and nutrients, as well as soil C and N storage capacity, through litterfall and decomposing litter (Frouz et al., 2013). Thus, species’ selection is an important driver for nutrient provision and global climate regulation (Fossey et al., 2020) and it may be crucial for the successful restoration of the post-mining areas. N2-fixing legumes can greatly improve soil N availability and C accumulation (Paul et al., 2002; Liu et al., 2020), due to the control of C sequestration by soil N (Luo et al., 2004; van Groenigen et al., 2017; Zhang et al., 2018).
Robinia pseudoacacia L. or black locust is a forest tree often used for purposes of reclamation. It is a deciduous, broadleaf, light-demanding tree that is native to North America, has been introduced to Europe at the beginning of the 17th century (Peabody, 1982), and became naturalized in several Mediterranean and temperate regions (Vítková et al., 2017; Nicolescu et al., 2020). Black locust is fast-growing and drought-tolerant (Vítková et al., 2017; Carl et al., 2018) and it spreads rapidly even in infertile, poor and dry soils by root-suckers (Cierjacks et al., 2013). It is a legume with a high symbiotic N-fixation capacity. The symbiosis between the species’ roots and N-fixing rhizobia interacts also with arbuscular mycorrhizal fungi that grow around roots and enhance the ability of black locust to withstand environmental stresses such as drought or low nutrient availability (Liu et al., 2020). Therefore, black locust can greatly improve soil quality by enhancing soil C and N sequestration, soil structure, and generally the biological properties of the soil (Deng et al., 2014; Kanzler et al., 2015; Zhang et al., 2018; Deng et al., 2019). Another way for black locust to withstand infertile soil conditions is the adjustment of its nitrogen resorption efficiency (NRE). NRE is the resorption of nitrogen from senescing organs, before litterfall, and is a crucial mechanism for survival under soil nutrient limitations (Deng et al., 2019). Both positive and negative effects of stand age on NRE are reported (Sun et al., 2016; Qiu et al., 2017), depending on whether soil N availability or internal plant N regulation are needed for supporting tree growth.
Black locust is nowadays considered an invasive species (Vítková et al., 2017). Still, the advantageous responses of the species under hostile conditions led to its extensive planting for restoration of degraded areas due to anthropogenic activities, such as intensive agriculture (Deng et al., 2014; Kou et al., 2016) and mining (Annighöfer et al., 2012). Post-mining regions in several countries, such as China (Loess Plateau; Qiu et al., 2010), Germany (Lusatia; Quinkenstein et al., 2011) and Greece (Lignite complex of NW Greece; Papadopoulos et al., 2015; Spyroglou et al., 2021) have been rehabilitated by establishing black locust plantations.
The role that monoculture black locust plantations can play for climate change mitigation (Bukoski et al., 2022) and soil nutrient enrichment (Woś et al., 2020) is still not fully understood. Studies assessing the stocks and fluxes in all different reservoirs of an ecosystem are scarce. To this end, we aimed at a detailed assessment of the carbon and nitrogen stocks and fluxes of the black locust restoration plantations at the former open-cast lignite mines at the largest lignite center in NW-Greece, which is missing to date. Our specific goals were to (a) determine the carbon stored in above-ground biomass, coarse and fine roots, litter (litterfall and forest floor), deadwood and soil organic carbon in the study area, (b) assess the soil nitrogen stocks and nitrogen resorption efficiency at the black locust plantations, and (c) examine how these carbon and nitrogen pools change with the age, in order to predict the long-term potential of these restoration plantations for enhanced carbon sequestration and improved nitrogen supply in the reclaimed mine soils.
2. Materials and methods
2.1. Study area
This study was carried out at the lignite mining complex of the Public Power Corporation S.A. at NW Greece, at the sites of Kyrio and Notio Pedio (40.56 to 40.61 N and 21.62 to 21.69 E; area: 11,113 ha) and Amyntaio (40.39 to 40.51 N and 21.7 to 21.89 E; area: 4,307 ha). There, a post-mining area of 2,570 ha is planted, mainly with black locust; 1,782 ha in Kyrio and Notio Pedio and 788 ha in Amyntaio. The plantations were established with the aim to restore the landscape after mining, withhold erosion and improve the carbon footprint of the area (Pavloudakis et al., 2020; Spyroglou et al., 2021). Black locust accounts for more than 95% of the planted area, with weaver’s broom (Spartium junceum L.) and Arizona cypress (Cupressus arizonica Greene) accounting for 2.45 and 1.4%, respectively. Other planted species include oaks, maples, pines, and different deciduous broadleaf species. The elevation of the plantations ranges from 530 to 950 m.a.s.l. In addition to forest plantations, other land uses include grasslands, agricultural fields, and barren sites for solar parks and recycling facilities. On average, the study sites have a mean temperature of 12.95°C and a mean annual precipitation of 535.91 mm.
The plantations are established on waste heaps consisting of open-cast mining overburden material resulted from lignite excavation at the complex of NW-Greece. The creation of these waste heaps is a continuous process, given that lignite excavation still takes place at the complex. Thus, depositions of variable terrain (moderate to steep slopes, plains and terraces) and variable depth (from 3 to 30 m) are created. These depositions have a texture of sandy clay loam (on average 48.4% sand, 28.5% silt, 23.1% clay) and alkaline pH (mean 7.9; ranging from 7.7 to 8.3). Calcium carbonate content is relatively high (mean 27.2%; ranging from 1.2 to 65.0), probably due to the newly dumped marls that these mine spoils contain (Papadopoulos et al., 2015).
2.2. Sampling
Black locust planting at the lignite complex of NW-Greece is a dynamic and ongoing process, as long as post-mining depositions are created, meaning that plantation age varies across the reclamation area. A network of 214 sampling plots of 100 m2 area were systematically setup to estimate the aboveground biomass distribution and deadwood stocks across the study area (Spyroglou et al., 2021). Their tree age ranged from 4 to 24 years old. The mean diameter at breast height (DBH) of the plantations is 7.9 cm, ranging from 1.4 to 22.3 cm. More details on the descriptive traits of the 214 sampling plots are given by Spyroglou et al. (2021).
Out of the 214 plots, we selected 18 sampling plots to estimate the litterfall C flux, soil organic carbon (SOC) and soil N stock, C stock in forest floor and foliar NRE (Figure 1). The plots were selected based on a combination of the following criteria: (1) the spatial distribution of the plots, in order to cover most black locust plantations across the lignite center, (2) the mean age of the trees, in order to cover the entire age range of the plantations, (3) the accessibility, as plots located on deposition edges with extreme slopes would not facilitate repeated sampling campaigns and were avoided.
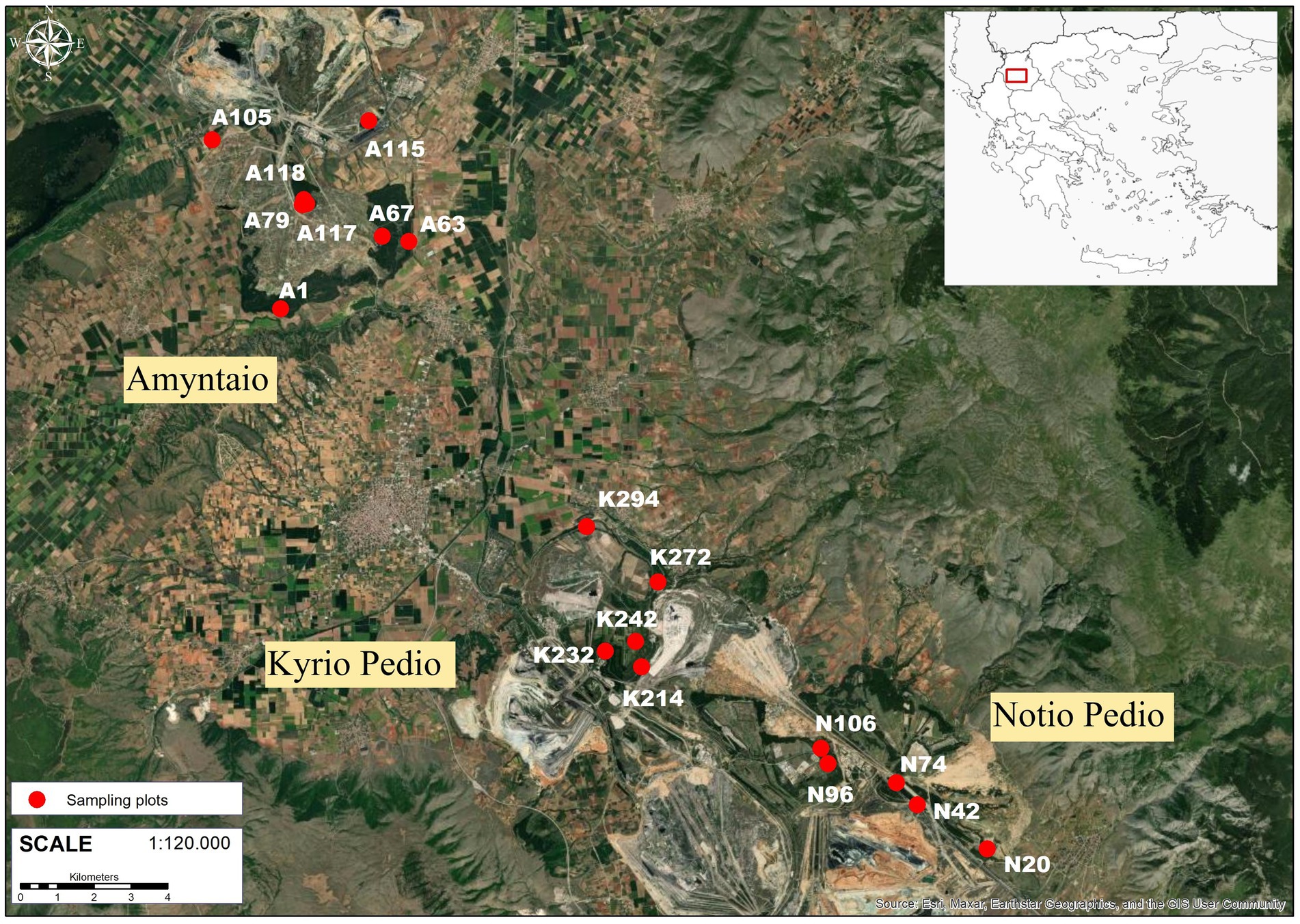
Figure 1. Location of the study area (Lignite Center of the Public Power Corporation) in NW-Greece and of the 18 sampling plots used for litterfall, forest floor and soil sampling across the study area. The map in figure 1 was created using ArcGIS® software by Esri. ArcGI® and ArcMapTM are the intellectual property of Esri and are used herein under license. Copyright © Esri. All rights reserved. For more information about Esri® software, please visit https://www.esri.com
The biometric traits of the trees in the 18 sampling plots are presented in Table 1. On average, diameter at breast height (DBH) and height (H) fluctuated from 4.7 cm to 12.7 cm and from 6.2 m to 16.9 m, respectively. The variation in these traits is due to the different age of the trees. Therefore, other biometric traits, such as tree density (N) and basal area (G) also vary among the 18 sampling plots (Table 1).
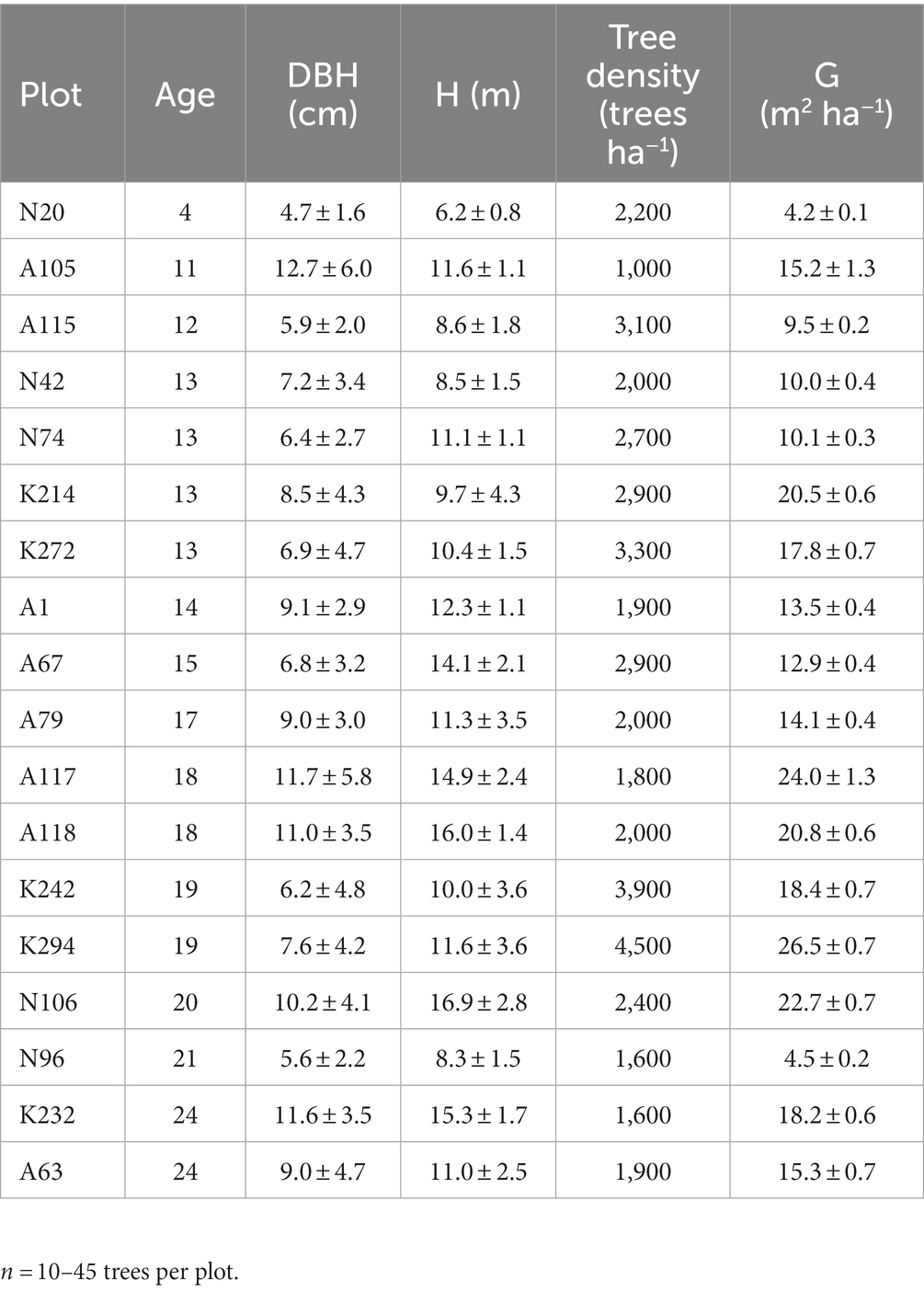
Table 1. Mean biometric traits (± standard deviation) of the 18 sampling plots used for the assessment of litterfall C flux, soil organic carbon (SOC) and the C stock in forest floor at the study post-mining area in NW Greece (DBH = Diameter at breast height, H = tree height, G = mean basal area of the trees).
2.3. Estimation of C sequestration in different carbon pools
2.3.1. Aboveground biomass
The above-ground biomass of the studied plantations was determined based on their DBH, by using an allometric equation established by Spyroglou et al. (2021) for the study area. Based on the DBH distribution of the black locust trees, five DBH classes were identified: 0–5, 5–10, 10–15, 15–20, and >20 cm. Given that the carbon content in tree wood is rather stable (Wang et al., 2015; Ma et al., 2019; Bārdule et al., 2021; Martin et al., 2021), five samples from stem disks at breast height (1.3 m) representative of the five DBH classes of the plantations were selected for elemental analysis to determine their carbon content.
2.3.2. Deadwood
To estimate the carbon stocks of lying and standing deadwood in each of the 214 plots, the biomass of each type of deadwood, which was estimated in the study area by Spyroglou et al. (2021), was multiplied by the generalized mean deadwood carbon fractions reported by Martin et al. (2021). For lying deadwood C stock, the deadwood carbon fractions of 47.53, 47.55, 47.98, 48.68, and 48.61% were used for the decay classes 1, 2, 3, 4, and 5, respectively and then the carbon stocks of all five decay classes were summed up. For standing deadwood C stock, a carbon content of 48.22% was used.
2.3.3. Coarse roots
Five black locust individuals, each one belonging to one of the five DBH classes, were selected for root excavation. From September until October 2020, the selected trees were harvested. All roots were excavated with a power washer, followed until their diameter reached approximately 2.0 mm and collected to estimate coarse roots C sequestration (Quinkenstein et al., 2012; Sohrabi et al., 2016). Given that excavation of the entire root system of the trees with the use of water is a rather laborious and time-demanding process, it was not possible to repeat it for a larger number of trees. The fresh weight of the roots was determined in the field and then roots were taken to the lab and divided into three diameter categories (Olajuyigbe et al., 2011): small roots (2–10 mm), medium roots (10–50 mm), and large roots (>50 mm). The stumps of the trees were classified as large roots. From the root system of each harvested tree, one sample per size category was selected, its fresh weight was determined and then it was oven-dried for at least 48 h at 80°C until weight stabilization. Based on the fresh-to-dry weight ratio of these samples, the total dry weight of each root was determined. Finally, an allometric model was established (Eq. 1; R2 = 0.831, p ≤ 0.05, Supplemental Figure 1) to calculate the below-ground biomass (BGB in kg; referring only to coarse roots with d > 2 mm) based on the mean DBH (cm) of all 214 sampling plots.
2.3.4. Fine root standing biomass and production
For the determination of fine root (≤2 mm) standing biomass (FRB), 12 soil samples were taken around the perimeter of each of the five felled trees with a soil auger (Split tube sampler; Eijkelkamp, Giesbeek, The Netherlands; 10 cm diameter, 60 cm length). These samples were collected at 1 m intervals and up to 3 m away from the stump of the harvested tree in a crosswise manner (North, South, East, West) as indicated in Supplemental Figure 2. Then, each sample was divided into 0–10 cm and 10–30 cm soil depth sub-samples and placed in small, numbered plastic bags. For their preservation, the samples were placed in a cool box until transport to the laboratory. There, the soil was removed, and the fine roots were carefully separated. The samples were oven-dried at 80°C for at least 48 h until weight stabilization, which was determined with an analytical balance (Gibertini E42S-B, Cottrill & Co, Birmingham, UK). The mean fine root standing biomass per soil area was calculated taking into account the area of the soil auger.
The fine root annual production and turnover were assessed with the use of in-growth cores. 48 in-growth cores were placed around four trees of different DBH, in four of the sampling plots where the littertraps were established (A79, K232, N106, N74). Their establishment took place in March 2021, before the initiation of the growing period, using the same design as for estimating fine root standing biomass (Supplemental Figure 2). The soil was collected from the 12 spots around each selected tree using the soil auger (Split tube sampler; Eijkelkamp, Giesbeek, The Netherlands; 10 cm diameter, 60 cm length) and a sieve to remove any standing fine roots and stones. Then, the in-growth cores were placed at the points where the soil was extracted and filled with the sieved soil. The top of the in-growth cores was sealed, and they were covered with soil to be protected from disturbance factors such as animals. Sticks were used to mark their position. After the end of the growing season, in December 2021, the in-growth cores were removed, and transported to the laboratory to estimate the fine root production (FRP; t ha−1 yr−1) and the fine root turnover (yr−1) in a given time period. The samples obtained from each in-growth core were divided into 0–10 cm and 10–30 cm soil depth sub-samples. The fine roots developed within the in-growth cores were separated and carefully washed and their dry weight was determined after oven-drying (at 80°C for at least 48 h until a steady dry weight was reached) to estimate FRP. According to Gill and Jackson (2000), fine root turnover (FRT) was calculated as:
2.3.5. Litterfall
Canopy density varied within each sampling plot. Based on measurements with an Li2200 Canopy Analyser (Licor Biosciences, Nebraska, USA), leaf area index was on average 2.91 under closed canopy and 2.12 in canopy gaps. To consider this variability, we have established one litter trap under closed canopy and one in a canopy gap at each plot. Thus, 36 litter traps were used in total.
Sampling of litterfall was carried out bimonthly for 1 year (thus 6 samplings). The plant material collected from the litter traps was placed in numbered plastic bags and taken to the laboratory for separation into five classes: (1) R. pseudoacacia Leaves (RL), (2) R. pseudoacacia Branches (RB), (3) R. pseudoacacia Fruits (RF), (4) Leaves from other species (LOS) and (5) Fruits from other species (FOS). The samples were oven-dried for at least 48 h at 80°C and weighted until a steady dry weight was reached.
2.3.6. Forest floor
Forest floor was collected with a rectangular metal sampler (total area of 700 cm2) and it included the biomass of annual herbaceous vegetation (grasses). Sampling was conducted on a bimonthly basis to consider the seasonal contribution of these grasses to forest floor biomass. Samples were collected randomly from different spots of the forest floor, close to each litter trap and transported to the laboratory in numbered plastic bags. In order to separate the forest floor from soil and other particles, the samples were first oven-dried at 80°C for 48 h and then sieved with 6 mm and 1 mm sieves, successively. The sieved samples were again oven-dried at 80°C for 48 h, till weight stabilization and their dry weight was determined. Finally, the mean annual biomass of the forest floor was calculated.
2.3.7. Soil sample collection
Three samples per plot were obtained from 0 to 90 cm soil depth (divided to 0–30, 30–60, 60–90 cm sub-samples) for the determination of bulk SOC and black locust derived-SOC. Four samples per plot were collected for the assessment of soil bulk density and soil N stock at 0–30 cm depth. All soil samples were left to dry at room temperature and were ground to fine powder.
2.3.8. Sample preparation, SOC and elemental analysis
All collected dried samples were ground to fine powder by using first a mass mill (PX-MFC 90 D, Kinematica AG, Malters, Switzerland), then 200 μm sieves and finally a ball mill (Biospec Mini-Beadbeater 16, Biospec products, Bartlesville, Oklahoma, USA). Aliquots of the samples (0.9 to 1.2 mg) were weighted with an analytical microbalance (M2P microbalance, Sartorius, Zaprešić, Croatia; precision: 0.001 mg) and put in tin capsules for solids (SA76980702, IVA Analysentechnik GmbH & Co. KG, Meerbusch, Germany). The samples were analysed for the determination of carbon and nitrogen contents with an elemental analyser (vario ISOTOPE cube) coupled to a continuous flow isotope ratio mass spectrometer (Isoprime100, IRMS), at the exception of soil samples which contained carbonates. SOC concentration was determined with the method of Walkley and Black (1934) by using a 1.3 correction factor, according to FAO (2019).
The C concentration of the aboveground biomass, belowground biomass (referring to coarse roots with d > 2 mm), fine roots, forest floor, lying deadwood and litterfall was also calculated based on the general assumption that 50% of their biomass consists of carbon, according to IPCC guidelines (IPCC, 2006) to estimate the deviation between the two approaches.
2.4. Calculation of soil organic carbon stock, black locust derived-SOC stock, and soil N stocks
The SOC and soil N stock were determined as following:
Where:
conc is the concentration expressed as kg C/N kg−1 soil and thickness refers to the thickness of the sampled soil layer (m).
BDf (kg m−3) is the bulk density of fine soil. It was used to calculate the density in a given soil volume due to the absence of large diameter soil particles such as stones.
To determine the SOC derived from black locust plantations, we had to eliminate the contribution of lignite residues (Papadopoulos et al., 2015). For this purpose, we assumed that reclaimed soil has been uniformized during post-mining operation and that the deepest horizon (60-90 cm) is representative of the initial soil due to limited impact of black locust at this high depth. The SOC derived from black locust was then computed by subtraction of the SOC concentration of deep soil (60–90 cm) from the SOC concentration of topsoil (0–30 cm), according to Frouz and Vindušková (2018) – see Eq. (6).
where SOC = SOC concentration
The plots where no correction of SOC was applied showed higher SOC concentration in deep soil compared to topsoil, which likely indicates multiple post-mining depositions. These plots could not be used to investigate the impact of black locust on SOC stock building. Thus, soil samples from 12 sampling plots were finally used for corrected SOC estimation. To determine the soil annual accumulation (or accrual) of SOC derived from black locust, the black locust-SOC stocks were divided by the age of the plantation of each plot.
2.5. Nitrogen resorption efficiency
Four dominant, non-neighboring trees were selected in each of the 18 sampling plots and were marked. Their DBH and height were measured. In June 2021, five green, fully-expanded, healthy leaves were collected from each tree. The leaves were selected from the middle of the crown’s height and were S-SW oriented in order to be fully exposed to sun (Hoch et al., 2003; Wang et al., 2020). In November 2021, five recently senescent leaves were selected from the forest floor under each selected tree. Both green and senescent leaves, were scanned for the determination of their leaf area (mm2). Their fresh weight and dry weight (after oven-drying at 65°C until constant weight) were also recorded.
NRE (%) was calculated as following, according to Vergutz et al. (2012) and Enta et al. (2020):
Due to mass loss of senescent leaves, which may lead to an underestimation of NRE (van Heerwaarden et al., 2003; Enta et al., 2020), the mass loss correction factor of 0.784 for deciduous broadleaves was used (Vergutz et al., 2012; Enta et al., 2020).
2.6. Data analysis
Statistical analysis was performed using OriginPro version 2022b (OriginLab Corporation, Northampton, MA, USA). One-way repeated measures Anova (p ≤ 0.05) was applied to identify significant differences between the sampling months for litterfall. A Tukey post-hoc test was used to separate homogenous groups. Linear and non-linear regression analysis was performed to test the effect of age on different carbon stocks and fluxes. In all cases linear regression produced the strongest models. Non-linear regression was also used to establish the allometric model predicting below-ground (coarse roots) biomass from DBH. All regression analyses were run at p ≤ 0.05.
3. Results
In total the black locust plantation ecosystem, which is established on the open cast mining depositions, has sequestered to date 56.71 tC ha−1. The portitioning of ecosystem C stocks among the different ecosystem pools is given in Figure 2A. Above-ground biomass is the greatest carbon pool, (50% of the stored C), followed by black locust-derived soil organic carbon (24%), coarse roots (14%), deadwood (6%), forest floor (5%) and finally fine roots, which contain less than 1% of the C sequestered in the recently developed soil–plant ecosystem (Figure 2A). The main ecosystem C fluxes (litterfall and fine root production) are presented in Figure 2B. The flux of litterfall contributes about 1.41 t C ha−1 annually, while the carbon flux provided by fine roots production is almost 10-fold lower (Figure 2B). The annual turnover rate of C in fine roots was estimated at 0.62 ± 0.03 years.
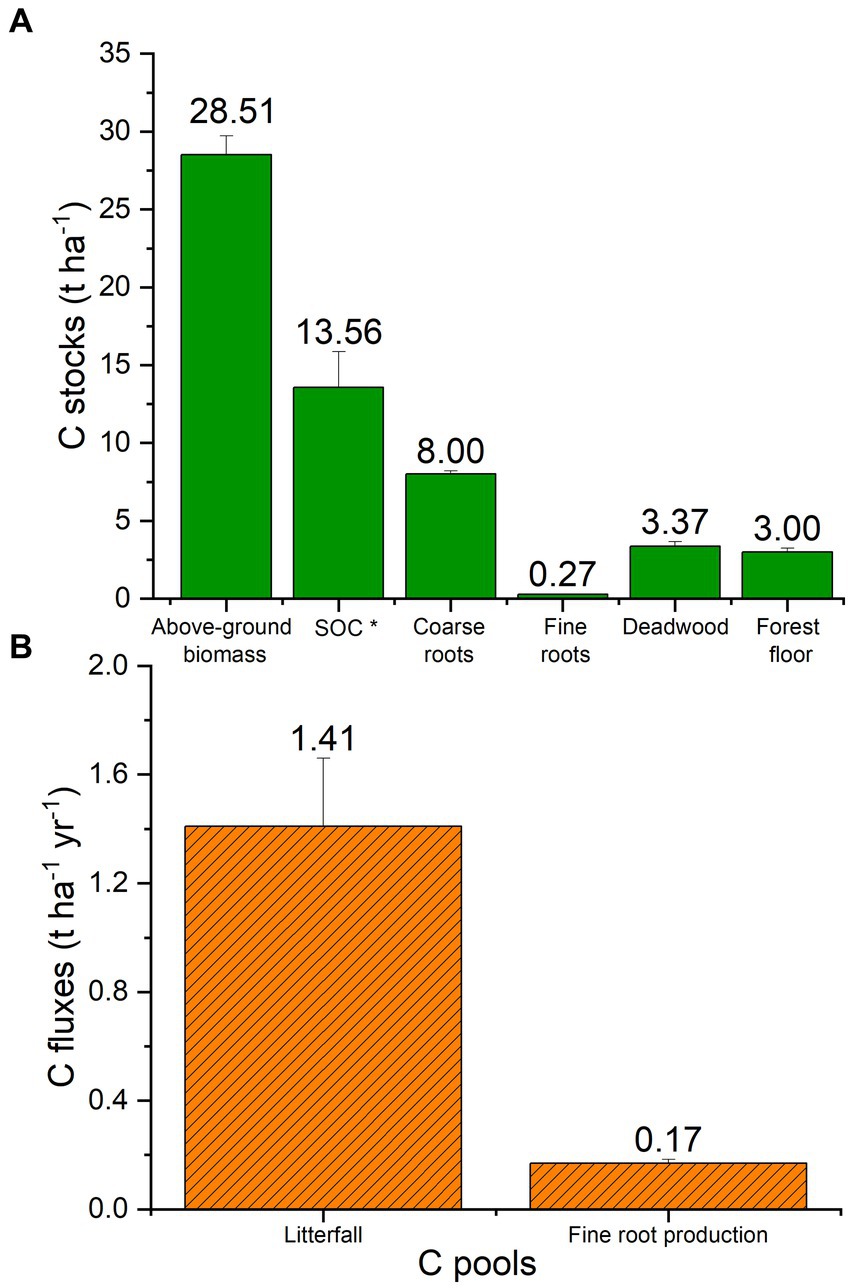
Figure 2. Carbon stocks (t ha−1) and fluxes (t ha−1 yr−1) of all pools of the black locust restoration plantations. Columns represent mean values (n = 214 for above-ground biomass, coarse roots and deadwood, n = 36 for forest floor and litterfall, n = 12 for SOC *; black locust-derived SOC), n = 117 for fine roots stock and n = 96 for fine root production. The error bars depict the SE of the means.
The determination of precise C contents for each ecosystem pool by means of elemental analysis instead of using the default IPCC values (50% C content) resulted in a slightly lower carbon stock in above-ground biomass and lying deadwood, (Supplemental Table 1). The difference was greater for litterfall, fine roots, and forest floor. Elemental analysis vs. IPCC default values produced C stocks that differed by 10.7, 20.6 and 106.3%, for litterfall, fine roots, and forest floor, respectively.
The flux of carbon in litterfall varied seasonally, as indicated in Figure 3A. It was the lowest from January to April, followed by a significant increase from May to August. Leaf senescence and, consequently, litterfall C peaked in September – October and declined, but was still quite high, in the last 2 months of the year. The litterfall composition comprising leaves, branches, fruits of black locust, as well as leaves of other species. Clearly differed in the two halves of the year (Figure 3B). From January to June, litterfall consisted mostly of fruits (66–78%), while from July to December fallen leaves of black locust comprised 75–94% of litterfall.
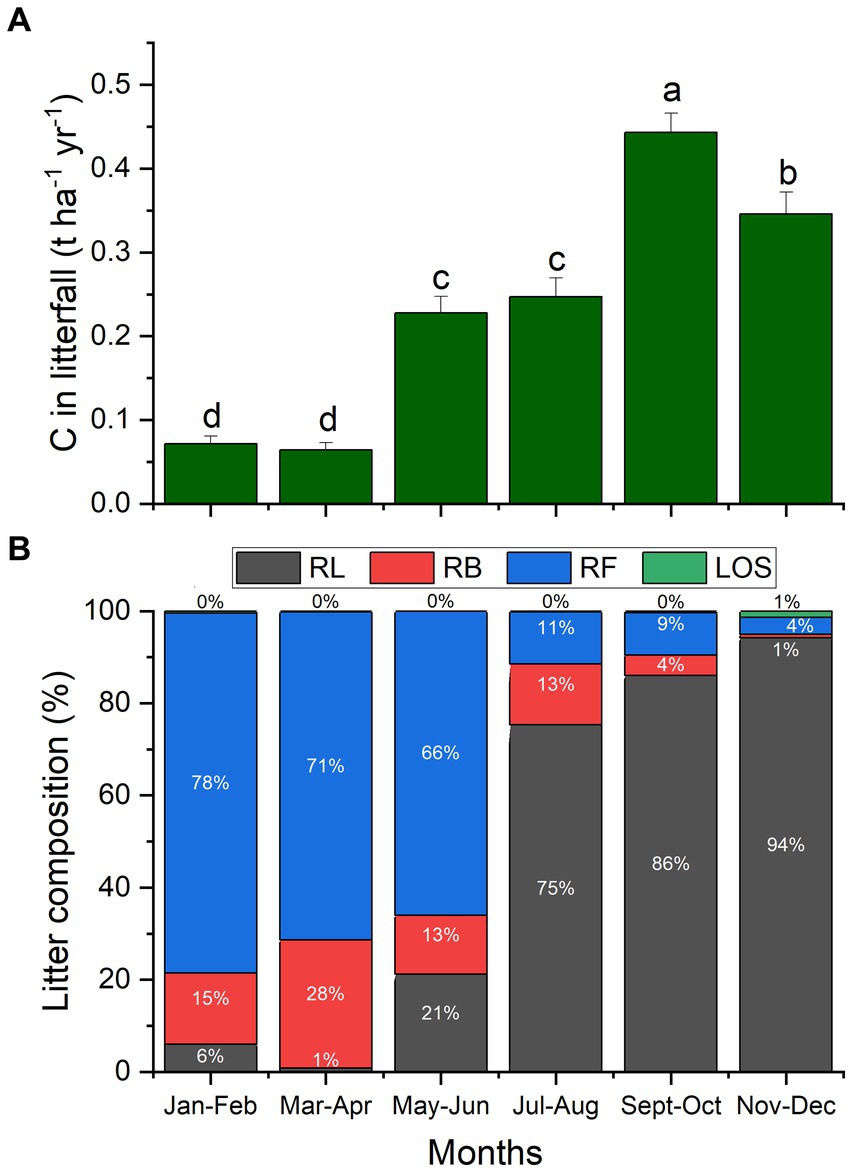
Figure 3. Seasonal variation of C in the litterfall flux (A) and of litterfall composition (B), comprising of black locust leaves (RL), branches (RB), fruits (RF) and leaves of other species (LOS). Columns indicate means (n = 36) per sampling which was conducted on a bimonthly basis, and error bars depict the standard errors of the means. Different letters show significant differences of C in litterfall between months (p ≤ 0.05).
Figure 4 shows the change of the three greatest carbon stocks (above-ground biomass, below-ground biomass, consisting of coarse roots with d > 2 mm, black locust-derived SOC) of the studied black locust plantations with age. Carbon stocks in both above- and below-ground biomass presented significant positive relationships with age (Figures 4A,B), indicating that carbon storage in these pools increases during the first 24 years after plantations’ establishment. On the contrary, annual SOC accrual declines with plantations’ age, as shown by the negative linear relationship among the two parameters (Figure 4C).
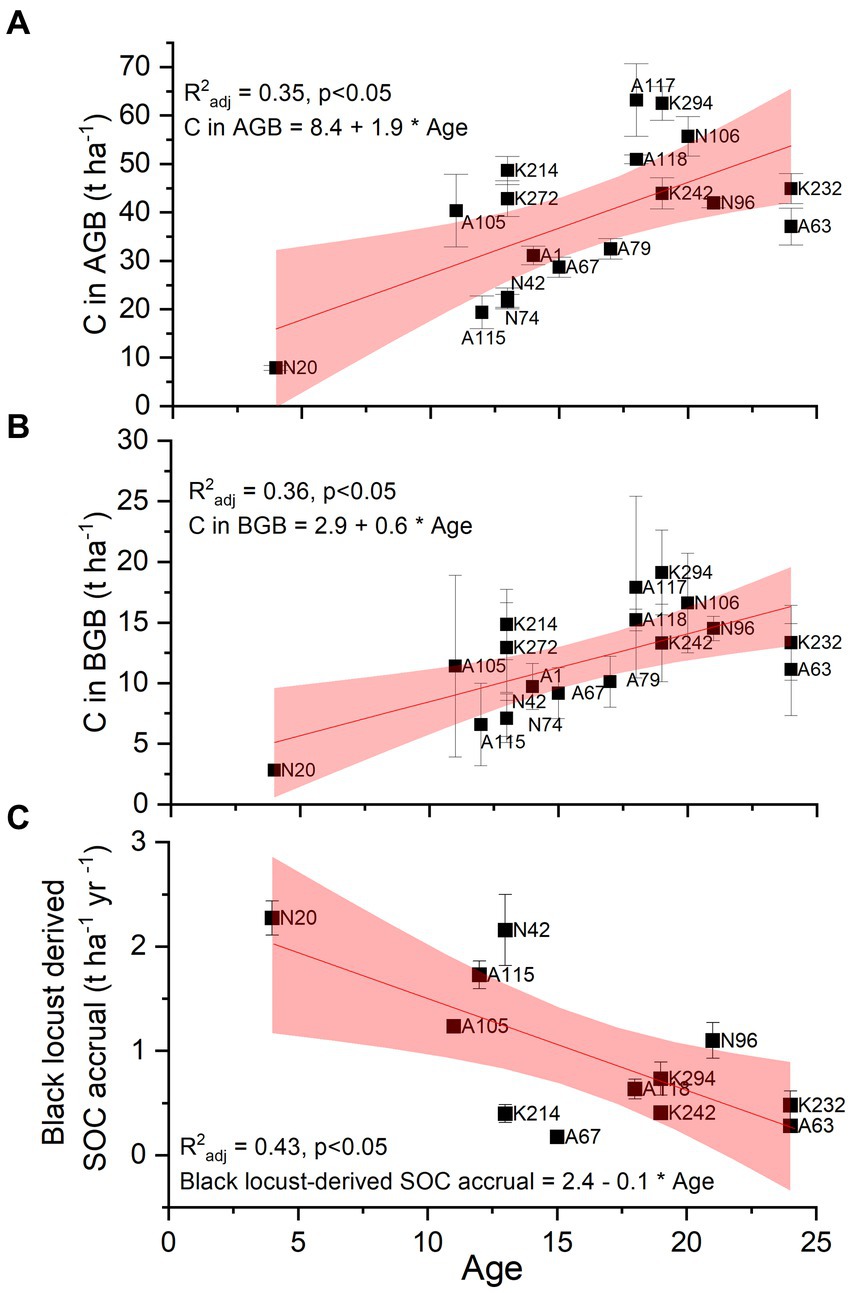
Figure 4. Relationship between plantation age and carbon stocks in above-ground biomass (AGB; plot A), below-ground biomass (BGB referring only to coarse roots – d > 2 mm; plot B) and annual SOC accrual derived by black locust (plot C) at p ≤ 0.05. n = 10–45 trees per plot for C in above- and below-ground biomass, n = 4 samples per plot for annual black locust-derived C accrual. Squares depict mean values ± SE. The labels depict the different sampling plots.
Figure 5A illustrates how the age of the black locust plantations affected their ability to minimize the foliar nitrogen losses during senescence by means of nitrogen resorption efficiency. Additionally, the enriching effect of the black locust plantations’ age on soil N stock is presented in Figure 5B. It was found that both parameters increased as plantations grow older, as supported by the positive linear relationships between NRE and age (Figure 5A; R2adj: 0.31, p ≤ 0.05), and soil N stock and age (Figure 5B; R2adj: 0.31, p ≤ 0.05).
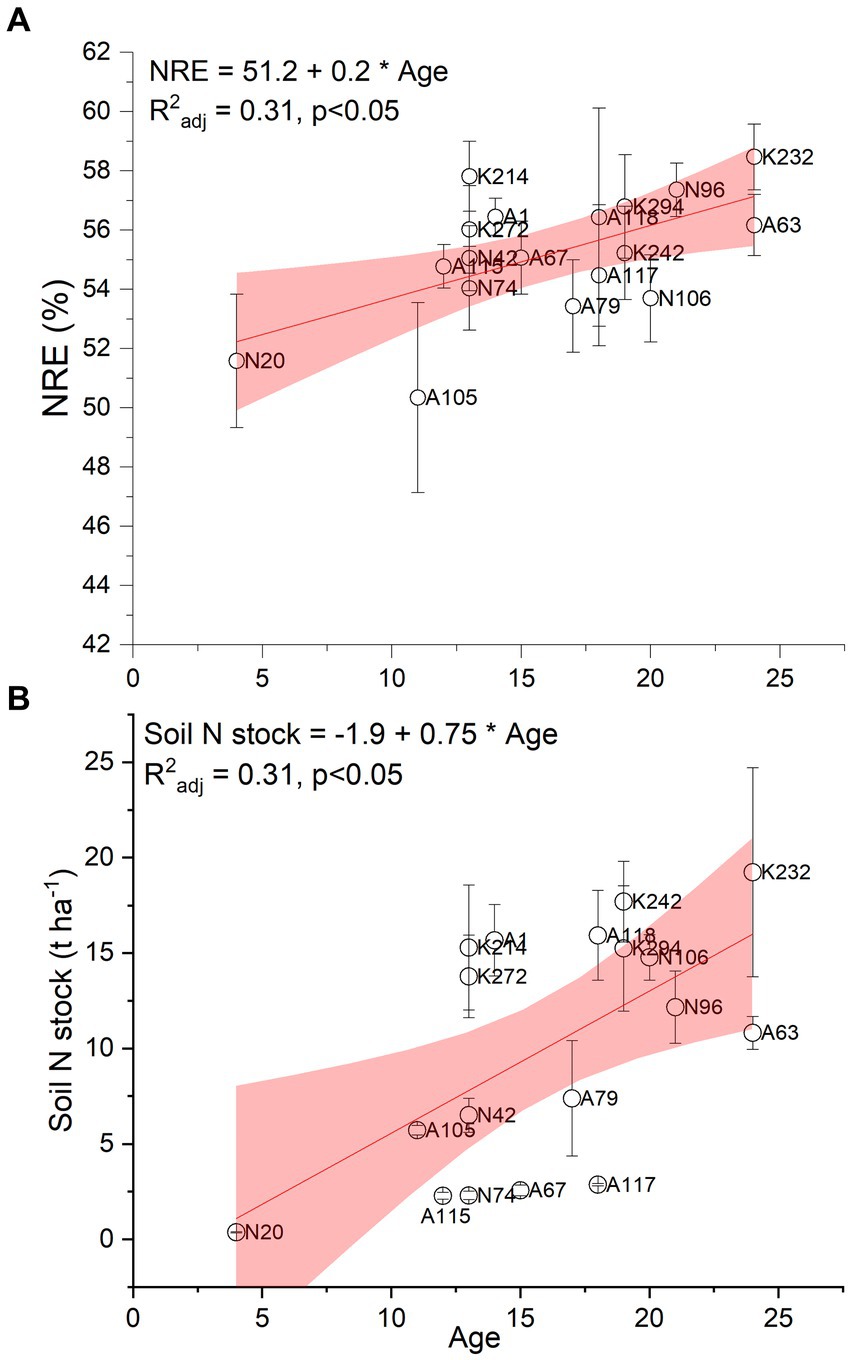
Figure 5. Relationship between plantation age and foliar nitrogen resorption efficiency (NRE; plot A) and soil N stock (plot B) at p ≤ 0.05. n = 4 trees per plot for NRE, n = 4 soil samples per plot for soil N stock. The circles depict means ± SE. The labels depict the different sampling plots.
The fact that the R2 coefficients of the regression models presented in Figures 4, 5 were moderate, is at least partially attributed to the particular heterogeneity of the post-mining landscape and, consequently, of the plots, as indicated in Supplemental Tables 2, 3 and 4.
4. Discussion
Βlack locust restoration plantations may support the reclamation of degraded landscapes and enhance ecosystem services, such as carbon sequestration, and nitrogen cycling (Nicolescu et al., 2018) which are priorities for resilience to climate extremes and for climate change mitigation. Here, we quantified the contribution of black locust plantations, established over the last decades at the largest lignite center in Greece, to soil nitrogen enrichment and carbon storage, which was missing to date. We also studied how these ecosystem services are affected by plantation age, which was the most important driver of C and N stocks in other black locust ecosystems (Xiang et al., 2021).
4.1. Carbon stocks and fluxes induced by the establishment of black locust plantations on the post-mining deposits
Varying levels of carbon stocks either per pool or for the entire ecosystem are reported in literature for black locust restoration plantations. During the study period, the mean age of the plantations was 16 years (Table 1; Supplemental Table 2). At this stage, a total of 56.7 t ha−1 C were stored in above- and below-ground biomass, forest floor and soil, in line with the C-caring capacity of black locust sites with comparable age and climatic conditions (Li et al., 2015). Estimations of carbon storage in above-ground biomass (AGB) of black locust plantations range from 8.9 t ha−1 (Sohrabi et al., 2016) to 103.8 t ha−1 (Zhang et al., 2010). Moreover, the AGB carbon in forest monoculture plantations globally is on average 47 t ha−1 for a mean age of 21 years (Bukoski et al., 2022). The fact that C in AGB is considerably lower at the studied area (28.1 t ha−1), reflects the negative effect of the heavily degraded environment where the black locust restoration plantations grow. Carbon in below-ground biomass (BGB) accounted for approximately 29% of the C stock in AGB, indicating that the ratio proposed by IPCC for this vegetation type (46%; IPCC, 2006) overestimates the contribution of BGB to carbon stocks. Our results are within the range of other black locust studies, fluctuating from 20 (Zhang et al., 2010; Sohrabi et al., 2016) to 34% (Quinkenstein et al., 2012). Thus, our estimation of BGB by the methodology employed was sound. In the same context, the carbon stored belowground (8.27 t ha−1; Figure 2) was similar to that found in literature for black locust plantations of the same age (Li et al., 2015).
Carbon sequestration in deadwood represents 6% of the total C stocks in the studied black locust ecosystem (approximately 3.4 t ha−1), as similarly reported by Lee et al. (2018). This is slightly lower that the global estimate for C in deadwood, which accounts on average for 8% of the total C pool in forests (Pan et al., 2011; Martin et al., 2021). This difference may be attributed to the fact that deadwood C stock is found in recently decomposing lying deadwood (decay classes 1 to 3 correspond to 96% of deadwood). On the contrary, decay class 5 (fully decomposed deadwood) is still totally missing (Supplemental Figure 3), as deadwood production and decomposition has recently started in these young plantations. However, deadwood decomposition and its contribution to soil organic carbon and to the plantations’ total C storage is expected to increase with age, as observed in older restoration plantations (Lukić et al., 2015).
The accumulation of carbon in forest floor was 3.0 t ha−1 C (Figure 3) falling within the wide range reported for black locust plantations in literature (0.42 to 14.8 t C ha−1; Li et al., 2015, Wang et al., 2015; Sohrabi et al., 2016; Kanzler et al., 2021). However, few studies included the herbaceous annual vegetation in the estimation of the forest floor C stock in plantations of similar age and their outcomes were variable (Li and Liu, 2014; Zhang et al., 2019), perhaps due to differences in herbaceous species’ composition and their respective C contents. It is worth mentioning that the forest floor C stock of the studied black locust is higher than that of several other European broadleaf forest trees, like Lime, Ash, Maple and different oak species, with the exception of European beech (Vesterdal et al., 2008; Gartzía-Bengoetxea et al., 2009; Gasparini and Di Cosmo, 2015; Jonard et al., 2017).
The rate of litterfall C flux in the studied plantations (1.4 t C ha−1 yr−1) was comparable to other findings for black locust (González et al., 2020). Still, this C flux is in the lower quartile of annual litterfall in forest ecosystems worldwide (0.6–11 t C ha−1 yr−1; Shen et al., 2019). Apart from the annual litterfall, it is vital to identify the initiation and seasonal fluctuation of litterfall for a reliable estimation of its contribution to the carbon (and nitrogen) cycle and for providing input to vegetation models, which currently assume that litterfall flux is evenly distributed during the year (Zhang et al., 2014). We found that litterfall increased already during the growing season (May to August; Figure 3A), as similarly reported by Andivia et al. (2018), and it partly coincided with the xerothermic period of the study site (Tziaferidis et al., 2022). Several studies have highlighted this sensitivity of litterfall dynamics to warmer and drier conditions (e.g., Aryal et al., 2015; Bou et al., 2015; Liu et al., 2015), which is probably aiming to limit the plants’ drought stress (Reich, 1995). However, phenology had the strongest effect on litterfall, which peaked in September – October when leaf senescence occurs in black locust (Mantovani et al., 2014), and continued at high rates till the end of the year. On an annual average, leaves were the major component of litterfall (Figure 3B), in line with Zhang et al. (2014), but on a seasonal basis leaves dominated only in the second half of the year, when litterfall peaked.
Together with deadwood and litter, fine roots (diameter ≤ 2 mm) comprise a major carbon pathway in forests. Almost 75% of a forest ecosystem’s total net primary productivity is used for fine root production due to its short lifespan and high turnover rate (Gill and Jackson, 2000). Although they constitute a minor carbon stock due to their small contribution to the total root biomass (Vogt et al., 1995), fine roots fuel the soil organic carbon and promote carbon cycling (Gill and Jackson, 2000; Helmisaari et al., 2002). We estimated a low C flux in fine root annual production (0.17 t C ha−1 yr−1; Supplemental Table 1), compared to other black locust restoration plantations of approximately the same age (Guo et al., 2022) and temperate forest ecosystems (Gill and Jackson, 2000; Brunner and Godbold, 2007; Finér et al., 2011). On the contrary, the fine root turnover rate of the studied black locust (0.62 yr−1) was higher compared to others (Guo et al., 2022), indicating that fine roots at the lignite complex of NW-Greece form a smaller but faster regenerated carbon flux.
Decomposition of all the above-mentioned compartments have added carbon input to the forest floor and have fueled soil organic carbon. In this context, we have computed the SOC accumulation of the post-mining depositions, resulted by the establishment of black locust, as it is considered a useful indicator of soil quality and ecosystem recovery (Macdonald et al., 2015). We estimated that black locust contributed to SOC by 13.6 t ha−1 in 0–30 cm depth, which is similar or somewhat higher that the SOC estimates in black locust restoration sites in other countries (Yüksek, 2012; Li and Liu, 2014). This black locust-derived SOC addition definitely has a positive effect on the fertility of the overburden material (Kanzler et al., 2021) and it improves the carbon footprint of the post-mining reclamation sites.
4.2. The effect of plantation age on carbon storage
Depending on the geological bedrock, the reclaimed mine soils can be highly diverse in pH and texture, ranging from acidic to alkaline pH, and from sand to clay (Bradshaw, 1997; Yuan et al., 2017). The depositions created by the overburden material of the open-cast lignite mines have built a fragmented plantation area (Spyroglou et al., 2021), which was reflected in the variation among the plots in all monitored C stocks and fluxes (Supplemental Tables 2 and 4). Nevertheless, age had a clear effect on carbon sequestration in both above- and below-ground biomass. The potential of black locust to store carbon in AGB increased steadily with age (Figure 4A). This is resulted by the increase in above-ground biomass with age, previously indicated by Tziaferidis et al. (2022) for the same sites. A similar positive age effect is reported for R.pseudoacacia restoration plantations of the same age range (5–25 years; Zhang et al., 2010; Wang et al., 2015), as well as for younger plantations (Böhm et al., 2011). Approximately 2.44 t ha−1 yr−1 C are stored annually in the above-ground biomass of the studied black locust plantations (Table 2), consistent to the findings in 14-years-old black locust (Quinkenstein et al., 2011). Still, it is important to note that all above-mentioned studies refer to young plantations (<25 years old) that have not been subjected to any forest management practices yet. Black locust reaches maturity at the age of 25–30 years, while growth and ABG production is levelling off after that age, as observed at other degraded sites (Wang et al., 2015; Guo et al., 2022) and at our study site (Manolopoulos et al., 2022). Based on these, we assumed an average age for stand maturity at 27.5 years and we estimated that the current total above-ground biomass of the plantations (28.5 t ha−1; Figure 2) at a mean age of 16 years, represents less than 40% of the total AGB at maturity, which is expected to reach at least 80.0 t ha−1 if the linear increase with time (Figure 4A) continues until that age. However, this trend in above-ground carbon storage may saturate as the plantations grow older. For example, Norbert et al. (2020) projected that the standing biomass of black locust forests declines after the age of 60 and total carbon accumulation decreases with years. In parallel to above-ground C stock, below-ground carbon storage also increased linearly with age (Figure 4B), as similarly observed by Li et al. (2013) in R.pseudoacacia restoration plantations. In our study, the annual total root C storage (in coarse and fine roots) was estimated at 0.76 t ha−1 yr−1 (Table 2) which is lower than in younger black locust plantations on degraded soils (Quinkenstein et al., 2012). This difference could indicate that the infertile post-mining sites in NW-Greece may have been more challenging for the growth of black locust, but it may change with age. Contrary to the observed patterns for coarse roots and to other findings (Guo et al., 2022), we could detect no relationship of fine root production and annual turnover with tree age (data not shown).
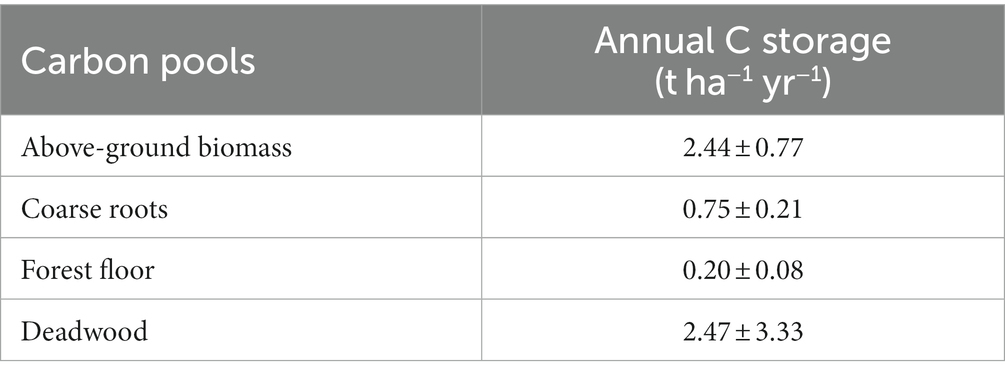
Table 2. Mean annual carbon storage rates (± standard deviation) in above-ground biomass, coarse roots, forest floor and deadwood (lying and standing) at the study post-mining area in NW Greece (n = 18 plots for all C stocks apart from deadwood, where n = 15 as 3 plots had no deadwood).
We estimated a mean black locust derived-SOC accrual of 1.0 t ha−1 yr−1, which falls within the range reported for black locust plantations (0.7–1.3 t ha−1 yr−1; Chang et al., 2011; Kanzler et al., 2021). Considerably higher SOC accumulation rates in post-mining rehabilitation sites, may be due to overestimations driven by accounting remaining lignite traces as plantation derived SOC (Quinkenstein et al., 2011; Frouz and Vindušková, 2018). The low initial C stocks in the studied plantations resulted in high SOC accumulation rates (Minasny et al., 2017). Similarly, Georgiou et al. (2022) observed enhanced SOC sequestration rate in soils being quite low from the maximum capacity of their mineral phases, considering that most of soil organic matter is associated to mineral phases (Cotrufo et al., 2019). Black locust-derived C accumulation rate declined linearly with plantation age (Figure 4C), as mineral phases became progressively loaded with organic matter. We can assume that the reclaimed soil deposit will progressively develop the characteristics of the soil in the surrounding area (similar parent material, similar local climate). As SOC at 0–30 cm depth of natural soils close to the study site ranges between 22.9 and 58.6 t ha−1 (Triantakonstantis and Detsikas, 2021), and currently SOC derived from the vegetation is estimated at 13.6 t ha−1 (Figure 2), there is a great potential for additional SOC accumulation, which may lead to a 2 to 4-fold increase in SOC in the upper 30 cm in the near future. Considerable SOC storage also takes place at greater depths (Jobbágy and Jackson, 2000), indicating that the total black locust-induced SOC sequestration will increase beyond the estimations of this study. These findings demonstrate that the studied plantations have a large potential for further contribution to C sequestration (Li et al., 2013, 2015; Lukić et al., 2015; Yuan et al., 2017), improvement of the properties of the mining overburden material and rehabilitation of marginal lands. On the other hand, it should be realized that the surface exposure of the residual lignite C, previously preserved in fossil in an inert form and currently contained in the post-mining overburden material, will induce the slow production of CO2 (Rumpel and Kögel-Knabner, 2002). This may explain why in some cases deep soil (60–90 cm) had higher SOC concentration than topsoil (0–30 cm; Supplemental Figure 4).
4.3. Black locust responses that enhance soil N enrichment
In addition to the general improvement of soil properties by R.pseudoacacia plantations (e.g., Qiu et al., 2010), black locust may contribute to increased nitrogen supply to soil (Yuan et al., 2017; Woś et al., 2020), which can even duplicate soil N stocks (Li et al., 2013; Papaioannou et al., 2016). In our study, soil N stock was on average 10 t ha−1 and it elevated steadily with increasing plantation age (Figure 5B). On average, annual soil N sequestration was 0.60 t ha−1 yr−1, being substantially higher than that of other black locust plantations (Yang et al., 2017). Most often, soil N enrichment in forest plantations is attributed to the release of N from decaying plant material. In our study we did not assess the decomposition rates of litter (forest floor and litterfall) but the fast turnover rate of fine roots (almost twice per year) probably facilitated the input of nutrients to the soil. On the other hand, the contribution of decaying deadwood to soil N could not have been high as the decay degree of lying deadwood in the studied plantations was still low. Decay classes 1 to 3 accounted for 96% of the total deadwood monitored, while highly decomposed deadwood (decay class 5) was missing (Supplementary Figure 2) and there was a weak correlation between lying deadwood biomass and soil N stock (R2adj = 0.17, p ≤ 0.05; data not shown). Given the young mean age of the plantations (16 years old), it is expected that the decay degree and the amount of deadwood will increase with age and contribute more to the enrichment in soil nutrients. Still, based on the dinitrogen (N2)-fixation ability of black locust through the symbiosis with rhizobia (Liu et al., 2020), it is plausible that the high annual soil N sequestration at the study site was mostly of atmospheric origin. Indeed, Marron et al. (2018) estimated that more than 75% of N in black locust trees is obtained by symbiotic N.
We have combined soil N stocks with the assessment of nitrogen resorption efficiency and the fluctuation of these parameters with age (Figure 5) in order to better characterize N cycling of the N-depleted post-mining environment. Nitrogen resorption efficiency during leaf senescence is a vital mechanism for retention and economy of nitrogen, as on average almost half of foliar N is resorbed through this function and is stored to roots and wood to support new growth in the next spring (e.g., Singh, 2014). However, deciduous forest trees, and particularly legumes like black locust, depend less on soil N supply and are generally characterized by lower NRE levels, compared to other species (Stewart et al., 2008; Yuan and Chen, 2009; Singh, 2014, 2015; Surmen et al., 2014). On the contrary, the mean NRE of the studied black locust plantations was 55.2%, which is higher than previously reported for black locust (35%, Enta et al., 2020; 52%, Deng et al., 2019). Such a difference could be partly due to the warm environment at the study area, which generally increases NRE in black locust (Sun et al., 2016), but it also reflects the N-poor environment of the post-mining depositions and the high needs of black locust for N supply. This explains the gradual increase of NRE with age (Figure 5A) to support the N needs for the young trees’ growth and it is expected to continue until the plantations reach maturity at the age of 25–30 years and then level-off (Deng et al., 2019).
The parallel increase of NRE and soil N stock with age (Figures 5A,B), indicates that despite the increasing soil N, the need for internal N control by means of NRE is still pronounced in these young black locust plantations, and it cannot be fully met by symbiotically fixed nitrogen. This response is in line with studies indicating that the simultaneous increase in NRE and soil N continues in black locust restoration plantations until the age of 25 and may decrease later on, when soil N becomes sufficient (Deng et al., 2019; Xu et al., 2020). Therefore, the coordination of increasing NRE and efficient N-fixation by the studied black locust (unpublished data) will cover the N needs of these restoration plantations and increase the fertility of the mining overburden material for at least another decade, given their current mean age.
5. Conclusion
The black locust restoration plantations at the lignite center of NW-Greece contribute to the improvement of the carbon footprint of this post-mining area by having stored to date 57 t C ha−1 in the ecosystem and by adding every year 1.5 t C ha−1 through litterfall and fine root production, 3.2 t C ha−1 through above- and below-ground biomass increment and 1.0 t ha−1 through black locust-derived SOC. Given that these plantations are still young (4–24 years old), total ecosystem C sequestration will increase with age until all plantations reach maturity. The plantations will progressively also enable the development of soil characteristics at the reclaimed soil depositions, based on the considerable black locust-derived SOC accrual and the N storage in the depositions, which currently adds annually 0.6 t N ha−1 and is increasing with age. Therefore, reclamation of open-cast mines with black locust contributes not only to landscape restoration, but also to the ecosystem services of carbon sequestration – climate regulation and N cycling. Future research should focus on the proper forest management of these plantations and on their long-term responses to climate change.
Data availability statement
The original contributions presented in the study are included in the article/Supplementary material, further inquiries can be directed to the corresponding author.
Author contributions
MF, GS, and KR conceived and designed the study. KR was responsible for funding acquisition and project administration. GX, GS, NA, and GT performed field and laboratory measurements. GX, DD, GS, and MF organized the database. GX and MF performed the statistical analyses. GX, DD, and MF were involved in original draft preparation. All authors contributed to the article and approved the submitted version.
Funding
The study was funded by the COFORMIT project “Contribution of the tree planted land of West Macedonia lignite center to protection of environment and to mitigation of climate change” (T1EDK-02521), which was financially supported by the Single RTDI state Aid Action Research - Create - Innovate funded by the Operational Program Competitiveness, Entrepreneurship and Innovation 2014-2020 (EPAnEK). The authors would like to thank SILVATECH (Silvatech, INRAE, 2018. Structural and functional analysis of tree and wood Facility, doi:10.15454/1.5572400113627854E12) from UMR 1434 SILVA, 1136 IAM, 1138 BEF, and 4370 EA LERMAB research center INRAE Nancy-Lorraine for its contribution to elemental and isotopic analysis. SILVATECH facility is supported by the French National Research Agency through the Laboratory of Excellence ARBRE (ANR-11-LABX-0002-01) and the French National Infrastructure IN-SYLVA.
Acknowledgments
We kindly acknowledge the Hellenic Public Power Corporation (HPPC) and, in particular Tryfon Barbas, Melina Andreadi, Marina Tentsoglidou, Aris Azas, Christos Papadopoulos, and Agni Patra for their support with personnel and equipment during the field campaigns and for providing all necessary information for the completion of this work. We are also thankful to Stamatios-Rafail Tzaferidis, Dimitris Manolopoulos and Florian Wilms for their assistance in field work and sampling.
Conflict of interest
The authors declare that the research was conducted in the absence of any commercial or financial relationships that could be construed as a potential conflict of interest.
Publisher’s note
All claims expressed in this article are solely those of the authors and do not necessarily represent those of their affiliated organizations, or those of the publisher, the editors and the reviewers. Any product that may be evaluated in this article, or claim that may be made by its manufacturer, is not guaranteed or endorsed by the publisher.
Supplementary material
The Supplementary material for this article can be found online at: https://www.frontiersin.org/articles/10.3389/ffgc.2023.1190026/full#supplementary-material
References
Akala, V. A., and Lal, R. (2001). Soil organic carbon pools and sequestration rates in reclaimed Minesoils in Ohio. J. Environ. Qual. 30, 2098–2104. doi: 10.2134/jeq2001.2098
Alves Dias, P., Kanellopoulos, K., Medarac, H., Kapetaki, Z., Miranda-Barbosa, E., Shortall, R., et al. (2018). EU coal regions: opportunities and challenges ahead; EUR 29292 EN ; Publications Office of the European Union: Luxembourg.
Amichev, B. Y., Burger, J. A., and Rodrigue, J. A. (2008). Carbon sequestration by forests and soils on mined land in the Midwestern and Appalachian coalfields of the U.S. For. Ecol. Manag. 256, 1949–1959. doi: 10.1016/j.foreco.2008.07.020
Andivia, E., Bou, J., Fernández, M., Caritat, A., Alejano, R., Vilar, L., et al. (2018). Assessing the relative role of climate on litterfall in Mediterranean cork oak forests. iForest 11, 786–793. doi: 10.3832/ifor2825-011
Annighöfer, P., Mölder, I., Zerbe, S., Kawaletz, H., Terwei, A., and Ammer, C. (2012). Biomass functions for the two alien tree species Prunus serotina Ehrh. And Robinia pseudoacacia L. in floodplain forests of northern Italy. Eur. J. For. Res. 131, 1619–1635. doi: 10.1007/s10342-012-0629-2
Aryal, D. R., De Jong, B. H. J., Ochoa-Gaona, S., Mendoza-Vega, J., and Esparza-Olguin, L. (2015). Successional and seasonal variation in litterfall and associated nutrient transfer in semi-evergreen tropical forests of SE Mexico. Nutr. Cycl. Agroecosyst. 103, 45–60. doi: 10.1007/s10705-015-9719-0
Bārdule, A., Liepiņš, J., Liepiņš, K., Stola, J., Butlers, A., and Lazdiņš, A. (2021). Variation in carbon content among the major tree species in Hemiboreal forests in Latvia. Forests 12:1292. doi: 10.3390/f12091292
Böhm, C., Quinkenstein, A., and Freese, D. (2011). Yield prediction of young black locust (Robinia pseudoacacia L.) plantations for woody biomass production using allometric relations. Ann. For. Res. 54, 215–227. doi: 10.15287/afr.2011.91
Bou, J., Caritat, A., and Vilar, L. (2015). Litterfall and growth dynamics relationship with the meteorological variability in three forests in the Montseny natural park. Folia For. Pol. Ser. A For. 57, 145–159. doi: 10.1515/ffp-2015-0015
Bradshaw, A. (1997). Restoration of mined lands—using natural processes. Ecol. Eng. 8, 255–269. doi: 10.1016/S0925-8574(97)00022-0
Brunner, I., and Godbold, D. L. (2007). Tree roots in a changing world. J. For. Res. 12, 78–82. doi: 10.1007/s10310-006-0261-4
Bukoski, J. J., Cook-Patton, S. C., Melikov, C., Ban, H., Chen, J. L., Goldman, E. D., et al. (2022). Rates and drivers of aboveground carbon accumulation in global monoculture plantation forests. Nat. Commun. 13:4206. doi: 10.1038/s41467-022-31380-7
Carl, C., Biber, P., Veste, M., Landgraf, D., and Pretzsch, H. (2018). Key drivers of competition and growth partitioning among Robinia pseudoacacia L. trees. For. Ecol. Manag. 430, 86–93. doi: 10.1016/j.foreco.2018.08.002
Chang, R., Fu, B., Liu, G., and Liu, S. (2011). Soil carbon sequestration potential for “grain for green” project in loess plateau. China. Environ. Manag. 48, 1158–1172. doi: 10.1007/s00267-011-9682-8
Chatterjee, A., Lal, R., Shrestha, R. K., and Ussiri, D. A. N. (2009). Soil carbon pools of reclaimed minesoils under grass and forest landuses: soil carbon pools of reclaimed mine soils. Land Degrad. Dev. 20, 300–307. doi: 10.1002/ldr.916
Cierjacks, A., Kowarik, I., Joshi, J., Hempel, S., Ristow, M., von der Lippe, M., et al. (2013). Biological Flora of the British Isles: Robinia pseudoacacia. J. Ecol. 101, 1623–1640. doi: 10.1111/1365-2745.12162
Cotrufo, M. F., Ranalli, M. G., Haddix, M. L., Six, J., and Lugato, E. (2019). Soil carbon storage informed by particulate and mineral-associated organic matter. Nat. Geosci. 12, 989–994. doi: 10.1038/s41561-019-0484-6
Deng, L., Liu, G., and Shangguan, Z. (2014). Land-use conversion and changing soil carbon stocks in China’s ‘grain-for-green’ program: a synthesis. Glob. Chang. Biol. 20, 3544–3556. doi: 10.1111/gcb.12508
Deng, J., Wang, S., Ren, C., Zhang, W., Zhao, F., Li, X., et al. (2019). Nitrogen and phosphorus resorption in relation to nutrition limitation along the chronosequence of Black locust (Robinia pseudoacacia L.) plantation. Forests 10:261. doi: 10.3390/f10030261
Deshaies, M. (2020). Metamorphosis of mining landscapes in the lower Lusatian lignite Basin (Germany): New uses and new image of a mining region. craup.
Enta, A., Hayashi, M., Lopez Caceres, M. L., Fujiyoshi, L., Yamanaka, T., Oikawa, A., et al. (2020). Nitrogen resorption and fractionation during leaf senescence in typical tree species in Japan. J. For. Res. 31, 2053–2062. doi: 10.1007/s11676-019-01055-z
FAO (2019). Standard operating procedure for soil organic carbon Walkley-Black method Titration and colorimetric method. Available at: https://www.fao.org/3/ca7471en/ca7471en.pdf [Accessed February 11, 2023].
Finér, L., Ohashi, M., Noguchi, K., and Hirano, Y. (2011). Fine root production and turnover in forest ecosystems in relation to stand and environmental characteristics. For. Ecol. Manag. 262, 2008–2023. doi: 10.1016/j.foreco.2011.08.042
Fossey, M., Angers, D., Bustany, C., Cudennec, C., Durandm, P., Gascuel-Odoux, C., et al. (2020). A framework to consider soil ecosystem services in territorial planning. Front. Environ. Sci. 8:28. doi: 10.3389/fenvs.2020.00028
Friedlingstein, P., Jones, M. W., O'Sullivan, M., Andrew, R. M., Bakker, D. C. E., Hauck, J., et al. (2022). Global Carbon Budget 2021. Earth Syst. Sci. Data 14, 1917–2005. doi: 10.5194/essd-14-1917-2022
Frouz, J., Livečková, M., Albrechtová, J., Chroňáková, A., Cajthaml, T., Pižl, V., et al. (2013). Is the effect of trees on soil properties mediated by soil fauna? A case study from post-mining sites. For. Ecol. Manag. 309, 87–95. doi: 10.1016/j.foreco.2013.02.013
Frouz, J., and Vindušková, O. (2018). “Soil organic matter accumulation in Postmining sites: potential drivers and mechanisms” in Soil management and climate change. eds. M. Á. Muñoz and R. Zornoza (Cambridge, MA, USA: Academic Press), 103–120.
Gartzía-Bengoetxea, N., González-Arias, A., and Martínez de Arano, I. (2009). Effects of tree species and clear-cut forestry on forest-floor characteristics in adjacent temperate forests in northern Spain. Can. J. For. Res. 39, 1302–1312. doi: 10.1139/X09-053
Gasparini, P., and Di Cosmo, L. (2015). Forest carbon in Italian forests: stocks, inherent variability and predictability using NFI data. For. Ecol. Manag. 337, 186–195. doi: 10.1016/j.foreco.2014.11.012
Georgiou, K., Jackson, R. B., Vindušková, O., Abramoff, R. Z., Ahlström, A., Feng, W., et al. (2022). Global stocks and capacity of mineral-associated soil organic carbon. Nat. Commun. 13:3797. doi: 10.1038/s41467-022-31540-9
Gill, R. A., and Jackson, R. B. (2000). Global patterns of root turnover for terrestrial ecosystems. New Phytol. 147, 13–31. doi: 10.1046/j.1469-8137.2000.00681.x
González, I., Sixto, H., Rodríguez-Soalleiro, R., and Oliveira, N. (2020). Nutrient contribution of Litterfall in a short rotation plantation of pure or mixed plots of Populus alba L. and Robinia pseudoacacia L. Forests 11:1133. doi: 10.3390/f11111133
Guo, L., Pang, Y., Cao, B., Fan, Z., Mao, P., Li, Z., et al. (2022). Robinia pseudoacacia decline and fine root dynamics in a plantation chronosequence in the Yellow River Delta, China. For. Sci. 68, 425–433. doi: 10.1093/forsci/fxac020
Helmisaari, H.-S., Makkonen, K., Kellomäki, S., Valtonen, E., and Mälkönen, E. (2002). Below- and above-ground biomass, production and nitrogen use in scots pine stands in eastern Finland. For. Ecol. Manag. 165, 317–326. doi: 10.1016/S0378-1127(01)00648-X
Hoch, W. A., Singsaas, E. L., and McCown, B. H. (2003). Resorption protection. Anthocyanins facilitate nutrient recovery in autumn by shielding leaves from potentially damaging light levels. Plant Physiol. 133, 1296–1305. doi: 10.1104/pp.103.027631
IPCC (2006). “IPCC guidelines for national greenhouse gas inventories” in Prepared by the National Greenhouse gas Inventories Programme. eds. S. Eggleston, L. Buendia, K. Miwa, T. Ngara, and K. Tanabe (Japan: IGES).
Jobbágy, E. G., and Jackson, R. B. (2000). The vertical distribution of soil organic carbon and its relation to climate and vegetation. Ecol. Appl. 10, 423–436. doi: 10.1890/1051-0761(2000)010[0423:TVDOSO]2.0.CO;2
Jonard, M., Nicolas, M., Coomes, D. A., Caignet, I., Saenger, A., and Ponette, Q. (2017). Forest soils in France are sequestering substantial amounts of carbon. Sci. Total Environ. 574, 616–628. doi: 10.1016/j.scitotenv.2016.09.028
Kanzler, M., Böhm, C., and Freese, D. (2015). Impact of P fertilisation on the growth performance of black locust (Robinia pseudoacacia L.) in a lignite post-mining area in Germany. Ann. For. Res. 58:1. doi: 10.15287/afr.2015.303
Kanzler, M., Böhm, C., and Freese, D. (2021). The development of soil organic carbon under young black locust (Robinia pseudoacacia L.) trees at a post-mining landscape in eastern Germany. New For. 52, 47–68. doi: 10.1007/s11056-020-09779-1
Kou, M., Garcia-Fayos, P., Hu, S., and Jiao, J. (2016). The effect of Robinia pseudoacacia afforestation on soil and vegetation properties in the loess plateau (China): a chronosequence approach. For. Ecol. Manag. 375, 146–158. doi: 10.1016/j.foreco.2016.05.025
Lee, S., Yim, J., Son, Y., Son, Y., and Kim, R. (2018). Estimation of Forest carbon stocks for National Greenhouse gas Inventory Reporting in South Korea. Forests 9:625. doi: 10.3390/f9100625
Li, H., Li, J., He, Y., Li, S., Liang, Z., Peng, C., et al. (2013). Changes in carbon, nutrients and stoichiometric relations under different soil depths, plant tissues and ages in black locust plantations. Acta Physiol. Plant. 35, 2951–2964. doi: 10.1007/s11738-013-1326-6
Li, T., and Liu, G. (2014). Age-related changes of carbon accumulation and allocation in plants and soil of black locust forest on loess plateau in Ansai County, Shaanxi Province of China. Chin. Geogr. Sci. 24, 414–422. doi: 10.1007/s11769-014-0704-3
Li, T., Ren, B., Wang, D., and Liu, G. (2015). Spatial variation in the storages and age-related dynamics of forest carbon sequestration in different climate zones—evidence from black locust plantations on the loess plateau of China. PLoS One 10:e0121862. doi: 10.1371/journal.pone.0121862
Liu, Z., Hu, B., Bell, T. L., Flemetakis, E., and Rennenberg, H. (2020). Significance of mycorrhizal associations for the performance of N2-fixing Black locust (Robinia pseudoacacia L.). Soil Biol. Biochem. 145:107776. doi: 10.1016/j.soilbio.2020.107776
Liu, D., Ogaya, R., Barbeta, A., Yang, X., and Peñuelas, J. (2015). Contrasting impacts of continuous moderate drought and episodic severe droughts on the aboveground-biomass increment and litterfall of three coexisting Mediterranean woody species. Glob. Chang. Biol. 21, 4196–4209. doi: 10.1111/gcb.13029
Lorenz, K., and Lal, R. (2007). Stabilization of organic carbon in chemically separated pools in reclaimed coal mine soils in Ohio. Geoderma 141, 294–301. doi: 10.1016/j.geoderma.2007.06.008
Lukić, S., Pantić, D., Simić, S., Borota, D., Tubić, B., Djukić, M., et al. (2015). Effects of black locust and black pine on extremely degraded sites 60 years after afforestation - a case study of the Grdelica gorge (southeastern Serbia). iForest 9, 235–243. doi: 10.3832/ifor1512-008
Luo, Y., Su, B., Currie, W. S., Dukes, J. S., Finzi, A., Hartwig, U., et al. (2004). Progressive nitrogen limitation of ecosystem responses to rising atmospheric carbon dioxide. Bioscience 54:731. doi: 10.1641/0006-3568(2004)054[0731:PNLOER]2.0.CO;2
Ma, S., Eziz, A., Tian, D., Yan, Z., Cai, Q., Jiang, M., et al. (2019). Tree size and age induced stem carbon content variations cause an uncertainty in forest carbon stock estimation. Biogeosci. Discuss. doi: 10.5194/bg-2019-87
Macdonald, S. E., Landhäusser, S. M., Skousen, J., Franklin, J., Frouz, J., Hall, S., et al. (2015). Forest restoration following surface mining disturbance: challenges and solutions. New For. 46, 703–732. doi: 10.1007/s11056-015-9506-4
Malhi, G. S., Kaur, M., and Kaushik, P. (2021). Impact of climate change on agriculture and its mitigation strategies: a review. Sustainability 13:1318. doi: 10.3390/su13031318
Manolopoulos, D., Vasileioris, K., Milios, E., Kitikidou, K., Spyroglou, G., and Radoglou, K. (2022). Allometric models for estimating the height of Robinia pseudoacacia L. in restoration plantations. Land 11:471. doi: 10.3390/land11040471
Mantovani, D., Veste, M., and Freese, D. (2014). Black locust (Robinia pseudoacacia L.) ecophysiological and morphological adaptations to drought and their consequence on biomass production and water-use efficiency. N. Z. J. For. Sci. 44:29. doi: 10.1186/s40490-014-0029-0
Marron, N., Gana, C., Gérant, D., Maillard, P., Priault, P., and Epron, D. (2018). Estimating symbiotic N 2 fixation in Robinia pseudoacacia. J. Plant Nutr. Soil Sci. 181, 296–304. doi: 10.1002/jpln.201700503
Martin, A. R., Domke, G. M., Doraisami, M., and Thomas, S. C. (2021). Carbon fractions in the world’s dead wood. Nat. Commun. 12:889. doi: 10.1038/s41467-021-21149-9
Minasny, B., Malone, B. P., McBratney, A. B., Angers, D. A., Arrouays, D., Chambers, A., et al. (2017). Soil carbon 4 per mille. Geoderma 292, 59–86. doi: 10.1016/j.geoderma.2017.01.002
Nicolescu, V.-N., Hernea, C., Bakti, B., Keserű, Z., Antal, B., and Rédei, K. (2018). Black locust (Robinia pseudoacacia L.) as a multi-purpose tree species in Hungary and Romania: a review. J. For. Res. 29, 1449–1463. doi: 10.1007/s11676-018-0626-5
Nicolescu, V.-N., Rédei, K., Mason, W. L., Vor, T., Pöetzelsberger, E., Bastien, J. C., et al. (2020). Ecology, growth and management of black locust (Robinia pseudoacacia L.), a non-native species integrated into European forests. J. For. Res. 31, 1081–1101. doi: 10.1007/s11676-020-01116-8
Norbert, B., Anda, R., Lucian, L., Monica, M., Madalina, B., Valentina, C., et al. (2020). Carbon dynamics projection for the Quercus petraea and Robinia pseudoacacia species-case study. IOP Conf. Ser.: Earth Environ. Sci. 616:012007. doi: 10.1088/1755-1315/616/1/012007
Olajuyigbe, S. O., Tobin, B., Gardiner, P., and Nieuwenhuis, M. (2011). Stocks and decay dynamics of above- and belowground coarse woody debris in managed Sitka spruce forests in Ireland. For. Ecol. Manag. 262, 1109–1118. doi: 10.1016/j.foreco.2011.06.010
Pan, Y., Birdsey, R. A., Fang, J., Houghton, R., Kauppi, P. E., Kurz, W. A., et al. (2011). A large and persistent carbon sink in the World’s forests. Science 333, 988–993. doi: 10.1126/science.1201609
Papadopoulos, C., Gekaa, C., Pavloudakis, F., Roumpos, C., and Andreadou, S. (2015). Evaluation of the soil quality on the reclaimed lignite mine land in West Macedonia, Greece. Proc. Earth Planet. Sci. 15, 928–932. doi: 10.1016/j.proeps.2015.08.148
Papaioannou, A., Chatzistathis, T., Papaioannou, E., and Papadopoulos, G. (2016). Robinia pseudοacacia as a valuable invasive species for the restoration of degraded croplands. Catena 137, 310–317. doi: 10.1016/j.catena.2015.09.019
Paul, K. I., Polglase, P. J., Nyakuengama, J. G., and Khanna, P. K. (2002). Change in soil carbon following afforestation. For. Ecol. Manag. 168, 241–257. doi: 10.1016/S0378-1127(01)00740-X
Pavloudakis, F., Roumpos, C., Karlopoulos, E., and Koukouzas, N. (2020). Sustainable rehabilitation of surface coal mining areas: the case of Greek lignite mines. Energies 13:3995. doi: 10.3390/en13153995
Peabody, F.J. (1982). A 350-year-old American legume in Paris. Castanea, 47, 99–104. Available at: http://www.jstor.org/stable/4033219 (Accessed March 1, 202).
Qiu, L., Hu, H., Lin, Y., Ge, L., Wang, K., He, Z., et al. (2017). Nutrient resorption effciency and C:N:P stoichiometry of Eucalyptus urophylla x E. grandis of different ages in a sandy coastal plain area. Chin. J. Appl. Environ. Biol. 23, 739–744. doi: 10.3724/SP.J.1145.2016.1102
Qiu, L., Zhang, X., Cheng, J., and Yin, X. (2010). Effects of black locust (Robinia pseudoacacia) on soil properties in the loessial gully region of the loess plateau, China. Plant Soil 332, 207–217. doi: 10.1007/s11104-010-0286-5
Quinkenstein, A., Böhm, C., and Matos, E.da S., Freese, D., and Hüttl, R.F. (2011). “Assessing the carbon sequestration in short rotation coppices of Robinia pseudoacacia L. on marginal sites in Northeast Germany”, in Carbon sequestration potential of agroforestry systems advances in agroforestry., eds. B.M. Kumar and P.K.R. Nair (Dordrecht: Springer Netherlands), 201–216.
Quinkenstein, A., Pape, D., Freese, D., Schneider, B. U., and Hüttl, R. F. (2012). Biomass, carbon and nitrogen distribution in living Woody Plant parts of Robinia pseudoacacia L. growing on reclamation sites in the mining region of lower Lusatia (Northeast Germany). Int. J. For. Res. 2012, 1–10. doi: 10.1155/2012/891798
Reich, P. B. (1995). Phenology of tropical forests: patterns, causes and consequences. Can. J. Bot. 73, 164–174. doi: 10.1139/b95-020
Rumpel, C., and Kögel-Knabner, I. (2002). The role of lignite in the carbon cycle of lignite-containing mine soils: evidence from carbon mineralisation and humic acid extractions. Org. Geochem. 33, 393–399. doi: 10.1016/S0146-6380(01)00169-3
Sabatini, F. M., de Andrade, R. B., Paillet, Y., Ódor, P., Bouget, C., Campagnaro, T., et al. (2019). Trade-offs between carbon stocks and biodiversity in European temperate forests. Glob. Chang. Biol. 25, 536–548. doi: 10.1111/gcb.14503
Shen, G., Chen, D., Wu, Y., Liu, L., and Liu, C. (2019). Spatial patterns and estimates of global forest litterfall. Ecosphere 10:e02587. doi: 10.1002/ecs2.2587
Singh, A. (2014). Nitrogen and phosphorus resorption efficiency in some, native tropical trees planted on a mine spoil in Singrauli coalfields, India. Int. J. Environ. Bioenerg. 9, 161–170.
Singh, A. (2015). Nitrogen and phosphorus resorption efficiency in some leguminous and non-leguminous tropical tree species planted on coal mine spoil in a tropical dry environment. Ambit. J. Educ. Res. Rev. 1, 1–7.
Sohrabi, H., Bakhtiarvand-Bakhtiari, S., and Ahmadi, K. (2016). Above- and below-ground biomass and carbon stocks of different tree plantations in Central Iran. J. Arid. Land 8, 138–145. doi: 10.1007/s40333-015-0087-z
Spyroglou, G., Fotelli, M., Nanos, N., and Radoglou, K. (2021). Assessing black locust biomass accumulation in restoration plantations. Forests 12:1477. doi: 10.3390/f12111477
Stewart, J. R., Kennedy, G. J., Landes, R. D., and Dawson, J. O. (2008). Foliar-nitrogen and phosphorus resorption patterns differ among nitrogen-fixing and nonfixing temperate-deciduous trees and shrubs. Int. J. Plant Sci. 169, 495–502. doi: 10.1086/528749
Sun, Z., Liu, L., Peng, S., Peñuelas, J., Zeng, H., and Piao, S. (2016). Age-related modulation of the nitrogen resorption efficiency response to growth requirements and soil nitrogen availability in a temperate pine plantation. Ecosystems 19, 698–709. doi: 10.1007/s10021-016-9962-5
Surmen, B., Güray Kutbay, H., Duygu Kilic, D., and Sürmen, M. (2014). Foliar resorption in nitrogen-fixing and non-fixing species in a swamp forest in northern Turkey. Rev. Ecol. 69, 318–327. doi: 10.3406/revec.2014.1754
Triantakonstantis, D., and Detsikas, S. (2021). Greek National map of soil organic carbon. EGU General Assembly 2021, EGU21–EGU13211. doi: 10.5194/egusphere-egu21-13211
Tziaferidis, S., Spyroglou, G., Fotelli, M., and Radoglou, K. (2022). Allometric models for the estimation of foliage area and biomass from stem metrics in black locust. iForest 15, 281–288. doi: 10.3832/ifor3939-015
van Groenigen, J. W., van Kessel, C., Hungate, B. A., Oenema, O., Powlson, D. S., and van Groenigen, K. J. (2017). Sequestering soil organic carbon: a nitrogen dilemma. Environ. Sci. Technol. 51, 4738–4739. doi: 10.1021/acs.est.7b01427
Van Heerwaarden, L. M., Toet, S., and Aerts, R. (2003). Current measures of nutrient resorption efficiency lead to a substantial underestimation of real resorption efficiency: facts and solutions. Oikos 101, 664–669. doi: 10.1034/j.1600-0706.2003.12351.x
Vergutz, L., Manzoni, S., Porporato, A., Novais, R. F., and Jackson, R. B. (2012). Global resorption efficiencies and concentrations of carbon and nutrients in leaves of terrestrial plants. Ecol. Monogr. 82, 205–220. doi: 10.1890/11-0416.1
Vesterdal, L., Schmidt, I. K., Callesen, I., Nilsson, L. O., and Gundersen, P. (2008). Carbon and nitrogen in forest floor and mineral soil under six common European tree species. For. Ecol. Manag. 255, 35–48. doi: 10.1016/j.foreco.2007.08.015
Vítková, M., Müllerová, J., Sádlo, J., Pergl, J., and Pyšek, P. (2017). Black locust (Robinia pseudoacacia) beloved and despised: a story of an invasive tree in Central Europe. For. Ecol. Manag. 384, 287–302. doi: 10.1016/j.foreco.2016.10.057
Vogt, K. A., Vogt, D. J., Palmiotto, P. A., Boon, P., O’Hara, J., and Asbjornsen, H. (1995). Review of root dynamics in forest ecosystems grouped by climate, climatic forest type and species. Plant Soil 187, 159–219. doi: 10.1007/BF00017088
Walkley, A., and Black, I. A. (1934). An examination of the Degtjareff method for determining soil organic matter, and a proposed modification of the chromic acid titration method. Soil Sci. 37, 29–38. doi: 10.1097/00010694-193401000-00003
Wang, S., Cao, Y., Pietrzykowski, M., Zhou, W., and Bai, Z. (2022). Research on the influence of vegetation restoration in loess open-pit coal mines of China: influencing factors and mechanism. Ecol. Eng. 177:106549. doi: 10.1016/j.ecoleng.2022.106549
Wang, J. J., Hu, C. X., Bai, J., and Gong, C. M. (2015). Carbon sequestration of mature black locust stands on the loess plateau, China. Plant Soil Environ. 61, 116–121. doi: 10.17221/931/2014-PSE
Wang, L., Li, Y., Duan, Y., Lian, J., Luo, Y., Wang, X., et al. (2020). Effects of nitrogen addition and reproductive effort on nutrient resorption of a sand-fixing shrub. Front. Plant Sci. 11:588865. doi: 10.3389/fpls.2020.588865
Wang, J., Wang, H., Cao, Y., Bai, Z., and Qin, Q. (2016). Effects of soil and topographic factors on vegetation restoration in opencast coal mine dumps located in a loess area. Sci. Rep. 6:22058. doi: 10.1038/srep22058
Wang, Z., Wang, G., Ren, T., Wang, H., Xu, Q., and Zhang, Q. (2021). Assessment of soil fertility degradation affected by mining disturbance and land use in a coalfield via machine learning. Ecol. Indic. 125:107608. doi: 10.1016/j.ecolind.2021.107608
Woś, B., Pająk, M., Krzaklewski, W., and Pietrzykowski, M. (2020). Verifying the utility of Black locust (Robinia pseudoacacia L.) in the reclamation of a lignite combustion waste disposal site in central European conditions. Forests 11:877. doi: 10.3390/f11080877
Xiang, Y., Liu, Y., Yue, X., Yao, B., Zhang, L., He, J., et al. (2021). Factors controlling soil organic carbon and total nitrogen stocks following afforestation with Robinia pseudoacacia on cropland across China. For. Ecol. Manag. 494:119274. doi: 10.1016/j.foreco.2021.119274
Xu, M.-P., Zhang, X.-Y., Li, W.-J., Ren, C.-J., Yang, G.-H., and Han, X.-H. (2020). Leaf nutrient resorption characteristics of Robinia pseudoacacia at different ages and their response to soil nutrient availability. Ying Yong Sheng Tai Xue Bao 31, 3357–3364. doi: 10.13287/j.1001-9332.202010.001
Yang, D., Pan, S., Ding, Y., and Tyree, M. T. (2017). Experimental evidence for negative turgor pressure in small leaf cells of Robinia pseudoacacia L versus large cells of Metasequoia glyptostroboides Hu et W.C. Cheng. 1. Evidence from pressure-volume curve analysis of dead tissue. Plant Cell Environ. 40, 351–363. doi: 10.1111/pce.12861
Yuan, Z., and Chen, H. Y. H. (2009). Global trends in senesced-leaf nitrogen and phosphorus. Glob. Ecol. Biogeogr. 18, 532–542. doi: 10.1111/j.1466-8238.2009.00474.x
Yuan, Y., Zhao, Z., Zhang, P., Chen, L., Hu, T., Niu, S., et al. (2017). Soil organic carbon and nitrogen pools in reclaimed mine soils under forest and cropland ecosystems in the loess plateau, China. Ecol. Eng. 102, 137–144. doi: 10.1016/j.ecoleng.2017.01.028
Yüksek, T. (2012). The restoration effects of black locust (Robinia pseudoacacia L) plantation on surface soil properties and carbon sequestration on lower hillslopes in the semi-humid region of Coruh Drainage Basin in Turkey. Catena 90, 18–25. doi: 10.1016/j.catena.2011.10.001
Zhang, J., Chen, G.-C., Xing, S., Sun, Q., Shan, Q., Zhou, J., et al. (2010). Carbon sequestration of black locust forests in the Yellow River Delta region, China. Int. J. Sustain. Dev. World Ecol. 17, 475–480. doi: 10.1080/13504509.2010.520855
Zhang, W., Liu, W., Xu, M., Deng, J., Han, X., Yang, G., et al. (2019). Response of forest growth to C:N:P stoichiometry in plants and soils during Robinia pseudoacacia afforestation on the loess plateau, China. Geoderma 337, 280–289. doi: 10.1016/j.geoderma.2018.09.042
Zhang, B., Luo, H., Lv, L., Wang, J., and Zhang, B. (2018). Analysis of ecological environment impact of coal exploitation and utilization. IOP Conf. Ser.: Earth Environ. Sci. 121:032002. doi: 10.1088/1755-1315/121/3/032002
Zhang, H., Yuan, W., Dong, W., and Liu, S. (2014). Seasonal patterns of litterfall in forest ecosystem worldwide. Ecol. Complex. 20, 240–247. doi: 10.1016/j.ecocom.2014.01.003
Keywords: black locust, above-ground biomass, coarse roots, fine roots, litter, SOC accrual, nitrogen resorption efficiency, soil N stock
Citation: Xanthopoulos G, Radoglou K, Derrien D, Spyroglou G, Angeli N, Tsioni G and Fotelli MN (2023) Carbon sequestration and soil nitrogen enrichment in Robinia pseudoacacia L. post-mining restoration plantations. Front. For. Glob. Change. 6:1190026. doi: 10.3389/ffgc.2023.1190026
Edited by:
Jianping Wu, Yunnan University, ChinaReviewed by:
Yang Cao, Northwest A&F University Herbarium, ChinaDirk Landgraf, University of Applied Sciences Erfurt, Germany
Copyright © 2023 Xanthopoulos, Radoglou, Derrien, Spyroglou, Angeli, Tsioni and Fotelli. This is an open-access article distributed under the terms of the Creative Commons Attribution License (CC BY). The use, distribution or reproduction in other forums is permitted, provided the original author(s) and the copyright owner(s) are credited and that the original publication in this journal is cited, in accordance with accepted academic practice. No use, distribution or reproduction is permitted which does not comply with these terms.
*Correspondence: Mariangela N. Fotelli, fotelli@elgo.gr