- Key Laboratory of Sustainable Forest Ecosystem Management-Ministry of Education, College of Forestry, Northeast Forestry University, Harbin, China
Introduction: Forests play a pivotal role within the global carbon cycle. However, how to enhance carbon storage in existing forests remains unclear.
Methods: In this study, we conduct a comprehensive analysis of data from 2,948 forest sites across China. Utilizing structural equation modeling (SEM), we investigate the intricate relationship between climate, tree species diversity, stand structure, function traits, initial biomass carbon stocks (BCi), soil organic carbon stocks (Soil C content), and carbon accumulation in biomass (ΔBC) and soil organic carbon stocks (ΔSOC) within both natural forests (NF, n = 1,910) and planted forests (PF, n = 1,038).
Results: Our findings underscore the critical influence of tree species diversity and stand structure drivers of both direct and indirect carbon accumulation, with distinct drivers emerging based on the carbon pools in NF and PF. Specifically, increasing tree species diversity from its minimum to maximum value through management-results in a 14.798 tC/ha reduction in BC and 0.686 tC/ha in SOC in NF. Conversely, amplifies BC and SOC in PF by 0.338 tC/ha and 0.065 tC/ha, respectively. Enhancing stand structure-such as stand density-results in a 231.727 tC/ha reduction in BC and SOC in NF, and a 10.668 tC/ha in BC and 64.008 tC/ha increment in SOC in PF.
Discussion: Overall, our results indicate that higher tree species diversity, stand density and age-group limits further carbon accumulation in BC and SOC in current NF. In contrast, low tree species diversity and stand density limits the development of carbon storage potential in planted forests. To enhance carbon sequestration capacity, China should change its current policy of completely banning logging of natural forests. Natural forests need thinning and reduced tree species diversity, while planted forests should increase tree species diversity and stand density.
1 Introduction
Enhancing forest carbon stocks through effective management has emerged as a pressing imperative, given that forests constitute the cornerstone of terrestrial carbon sinks (Fang et al., 2001; Cai et al., 2022), accounting for ~80% of carbon in aboveground biomass and 40% in belowground biomass within terrestrial ecosystems, respectively (Carvalhais et al., 2014; Yu et al., 2020). They play a pivotal role in responding to climate change and striving toward the objective of carbon neutrality. China holds the top position globally in the establishment of planted forests (PFs) (Yu et al., 2019). Since the late 1980s, China has been successfully conducting national afforestation and reforestation programs. This effort has culminated in a total afforested and reforested area of 79.54 million hectares by fostering rapid forest expansion in 2019 (State Forestry Grassland Administration of China, 2021). Remarkably, this expansion has contributed to nearly 44% of the nation's terrestrial carbon sink (Li et al., 2016; Yu Z. et al., 2022). Nonetheless, assessments reveal a crucial consideration: Despite the government's ongoing commitment to expanding its planted areas (with a projected forest cover increase to 26% by 2050) (Ouyang et al., 2016; Lu et al., 2018), the available land suitable for such expansion is limited to ~40 million hectares (Zhang Y. et al., 2022), where only 12% of the afforestation land is of good quality (State Forestry Grassland Administration of China, 2021). Given these finite forest land resources, there exists a constraint on the capacity to enhance carbon storage solely through expanding forested regions. Furthermore, the vast planted forests predominantly consist of fast-growing, short-rotation species such as poplar, larch, and China fir (Yu et al., 2019). The aboveground biomass carbon storage within planted forests only constitutes 19.86% of the total forested area, indicating significant room for enhancement (State Forestry Grassland Administration of China, 2021). Thus, elevating the standards of forest management and adopting strategies for the preservation and efficient utilization of forest resources via scientific and technological innovation will emerge as a pivotal avenue for augmenting China's carbon storage in the forthcoming years.
Since the Protocol (1997) put forward the concept of carbon sink, the relative roles of tree species diversity, stand structure, and function traits in determining carbon stocks are still widely debated (Intergovernmental Panel on Climate Change, IPCC) (Yu G. R. et al., 2022). A large number of conclusions have been mostly built on the foundation of forest aboveground biomass carbon stock experiments and a variety of local-scale ecological mechanisms (e.g., niche complementarity, selection or mass ratio, and competitive exclusion effects) (Ali et al., 2019). When it comes to evaluating tree species diversity, stand structure, and function traits in carbon stocks, the results are mixed. Several studies have shown a high correlation between tree species diversity and carbon stocks (Liu et al., 2018; Shu et al., 2019; Augusto and Boča, 2022; Gamon, 2023; Schuldt et al., 2023; Yu et al., 2023), while others have shown that the relationship between tree species diversity and carbon stocks is weak (Gao et al., 2023), and even the complex stand structure reduces the carbon stocks function of forests (Carol Adair et al., 2018). These diverse outcomes suggest the existence of multiple mechanisms for carbon stocks, such as tree species diversity and stand structure in different carbon pools, which are usually not taken seriously.
Indeed, a major challenge impeding efforts to reconcile these conflicting patterns is that the majority of conclusions have focused on independently considering carbon stored in aboveground biomass (Keith et al., 2021; Schuldt et al., 2023), without acknowledging the fact that carbon stock in different carbon pools varies widely in the intensity of their responses to various variables. In reality, different carbon pool responses to tree species diversity, stand structure, and function traits are different. For instance, tree species diversity decreases carbon stock in forest aboveground and belowground biomass carbon (Carol Adair et al., 2018) and increases soil organic carbon (Chen X. et al., 2023). In addition, most of the studies on carbon focus on carbon stocks that are driven by tree biomass (Hardiman et al., 2011; Ge et al., 2021; Gao et al., 2023; Xu et al., 2023) rather than by carbon accumulation. However, in terms of realizing the goal of carbon neutrality, we pay more attention to the accumulation or carbon sequestration capacity of forests.
In addition, considering forest origin is critical for explaining the different results found across studies. In particular, the role of natural forests (NFs) has been overlooked (Cao et al., 2011), despite the fact that they prevail in China (accounting for 79.14% of the aboveground biomass carbon stocks total forest area in China) (State Forestry Grassland Administration of China, 2021). However, the current research focused more on carbon stocks (as stockage) in natural forests (Lin et al., 2016; Yuan et al., 2018; Ali et al., 2019; Li et al., 2019) and more on carbon flows (carbon sequestration) brought about by the expansion of planted forests, afforestation, and reforestation areas (Lu et al., 2018; Zhang D. N. et al., 2021; Yu Z. et al., 2022). Compared to planted forests, natural forests also contain more species diversity and show more habitat heterogeneity (Gao et al., 2023). Higher tree species diversity and structure complexity in multilayer natural forests may increase microhabitat complexity and niche diversity and promote resource utilization, enhancing forest carbon sequestration capacity (Yu et al., 2019). Higher tree species diversity and structure complexity may also lead to higher respiration and consumption. Conversely, planted forests with highly homogeneous stand structures may intensify competition. Although certain studies indicate greater wood production efficiency in planted forests (Brown et al., 2020; Gao et al., 2023), whether the mechanisms of carbon accumulation differ between natural forests and planted forests remains unclear.
Addressing these concerns is crucial, as changes in carbon stocks, such as gains and losses, are primarily driven by resource utilization and respiratory consumption. For example, increases in biomass carbon stocks mainly drive stand growth (Yang et al., 2023), while losses in biomass carbon stocks mainly drive tree death, coarse woody debris, roots, and litter decomposition (Harmon et al., 2020). Conversely, increases in soil organic carbon stocks mainly drive coarse woody debris, roots, and litter decomposition, while losses in soil carbon stocks mainly drive the decomposition and consumption of soil organic carbon (Nottingham et al., 2020). Carbon sink functionality in forest management may need to be balanced between biomass carbon and soil organic carbon. Forest soils also play a crucial role in responding to climate change and striving toward the objective of carbon neutrality, storing at least three times more carbon than what is found in biomass carbon (Tang et al., 2018; Chen X. et al., 2023). As a result, tree species diversity and variations in stand structure are associated with habitat heterogeneity (Yu et al., 2019). These factors may contribute to increased variability in light and moisture, thereby promoting resource utilization as well as the decomposition and transformation of coarse woody debris, roots, and litter. Furthermore, if these driving mechanisms originate from forests of different origins, such as natural forests and planted forests, the outcomes could be entirely contradictory. High species diversity and complex stand structure may enhance coarse woody debris, roots, and litter decomposition by increasing microbial diversity. These unclear mechanisms make forest management difficult, especially the improvement of carbon storage.
In this study, we assessed the contribution of different origins of forests to carbon accumulation in different carbon pools (BC and SOC) across China. We present three hypotheses: (i) Tree species diversity, stand structure, and function traits directly or indirectly influence carbon accumulation and the magnitude of positive or negative depends on the carbon pool (Figure 1); (ii) The role of tree species diversity, stand structure, and function traits is predominantly contingent on the initial carbon storage levels. Higher initial carbon stocks tend to result in these factors that may limit carbon accumulation. Conversely, tree species diversity, stand structure, and functional traits are more likely to promote carbon accumulation when initial carbon storage levels are low; (iii) Tree species diversity, stand structure, and functional traits negatively correlated with carbon accumulation in natural forests, due to high initial carbon stock and stand age. Conversely, in planted forests, these factors enhance carbon accumulation due to their lower initial carbon stocks.
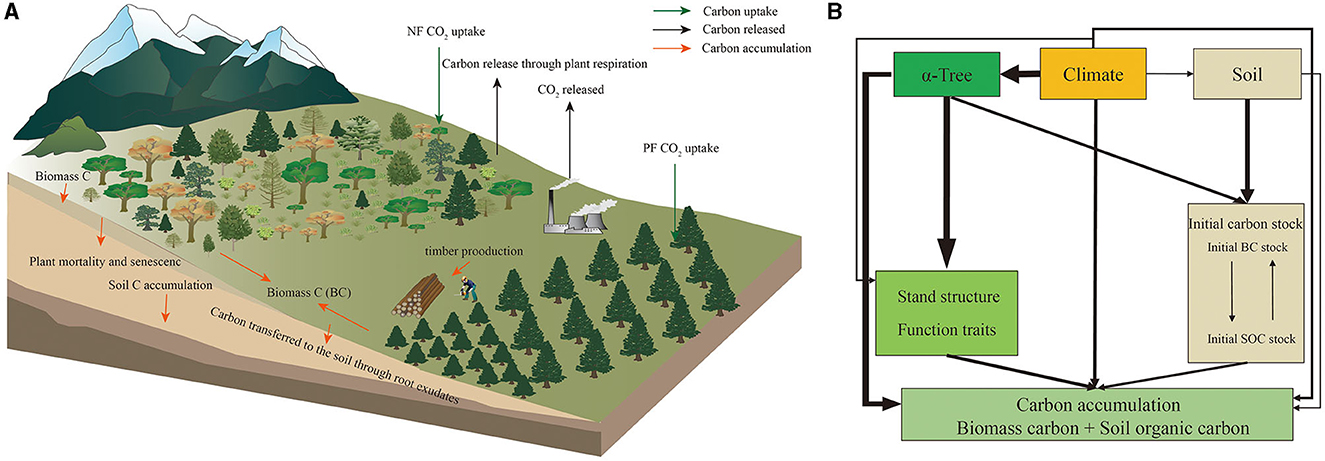
Figure 1. Conceptual model and main carbon accumulation on biomass and soil carbon in natural forests (NFs) and planted forests (PFs). (A) Carbon cycle diagram in forest ecosystems; (B) conceptual model.
We explored these scenarios with a distinctive dataset encompassing forest communities in both natural forests (NFs) and planted forests (PFs) in China. First, we evaluate the direct and indirect effects of climate, tree species diversity, stand structure, and function traits on changes in biomass carbon (ΔBC) and soil organic carbon (ΔSOC) stocks. Second, we assess whether higher initial BC and SOC limit carbon accumulation, and whether tree species diversity, stand structure, and functional characteristics limit carbon accumulation in the context of high initial carbon stocks. More specifically, as NFs and PFs belong to two different types of forest origins, NFs show more species diversity, structure complexity, and higher initial carbon stock. Carbon accumulation in NFs and PFs may show different responses to tree species diversity, stand structure, and function traits. NFs and PFs may require different forest management strategies to achieve the goal of carbon gain. Thus, we examine these questions by comparing the pattern observed for NFs and PFs, respectively. Finally, to provide guidance for effective forest management and balance between BC and SOC, we calculate the net gain or loss of total carbon stocks within the observed span of a specific variable. We have given different management suggestions for forests of different carbon pools and different origins in different regions of China.
2 Materials and methods
2.1 Study area and field data collection
For this study, we employed data that are publicly accessible from the National Earth Science Data Center, National Science & Technology Infrastructure of China (NESSDC, http://www.geodata.cn), spanning a substantial portion of China's forested terrain on a multi-biome scale. All plots were established and monitored between 2013 and 2017 following consistent ground sampling guidelines outlined in accordance with LY/T3128-2019 (The Regulations for Classification, Survey, and Mapping of the Forest Vegetation, Forestry Industry Standard of the People's Republic of China). The dataset underwent filtering based on several criteria: (i) exclusion of plots with missing data for any measured values; (ii) exclusion of sample plots that were imported and extended beyond land boundaries (Wang T. et al., 2023). Each field plot underwent additional filtering based on two criteria: (1) It needed to be situated in a consistently undisturbed site, and (2) it had to accurately represent the respective segment of vegetation formation or sub-formation (Wang T. et al., 2023). Overall, plots that met these criteria were distributed across the temperate region (TR, n = 1,606), subtropical region (SR, n = 946), Alpine region (AR, n = 259), and tropical region (TR2, n = 137) (Figure 2; Supplementary Figure 1).
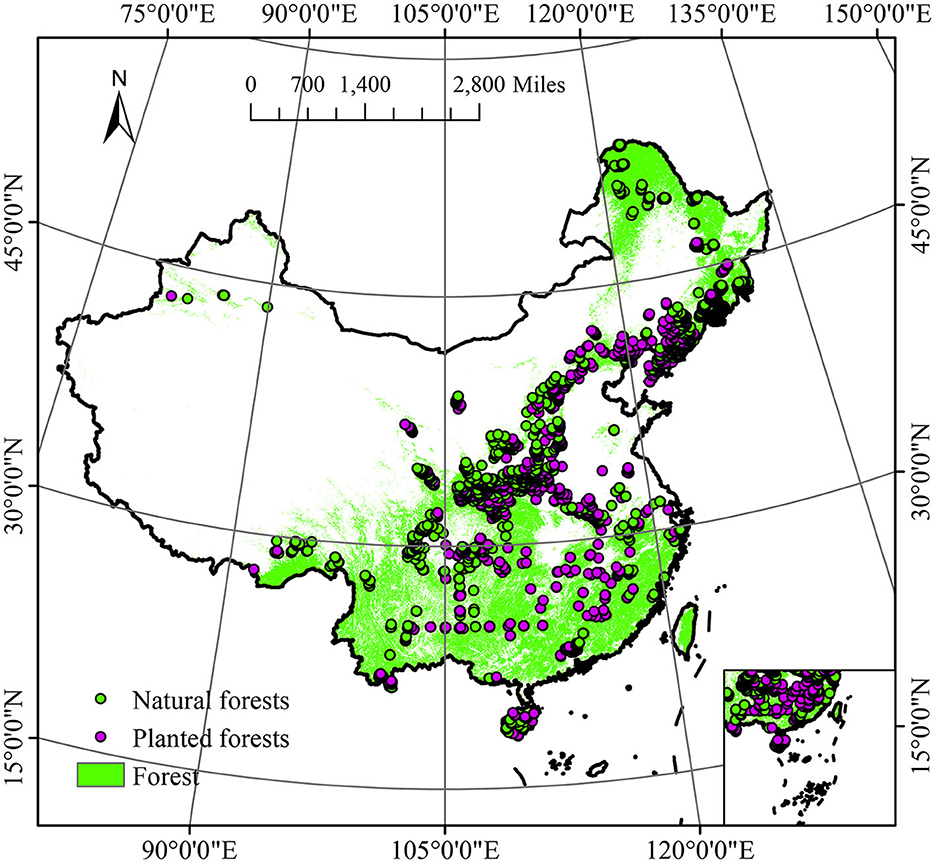
Figure 2. Study area and sample plot in forest cover area in 2013. Land cover according to Yang and Huang (2021).
In this dataset, natural forest (n = 1,910) was defined as forests resulting from natural seeding, artificial promotion of natural regeneration, or natural germination; and planted forest (n = 1,038) was defined as forests formed through various seeding methods, such as planting seedlings, direct seeding, or airplane seeding (Figure 2; Supplementary Figure 2). Surveys of vegetation were carried out during the periods of peak vegetation cover from July to October. In 2,948 plots measuring 0.067 ha2, all tree species with a diameter at breast height (DBH) ≥ 5 cm were numbered, tagged, and measured for DBH, height, and canopy width. For trees with a DBH < 5 cm and H > 2 m, the tree species name, DBH, and height were investigated. For trees with H < 2 m, the main species names, number of trees, average height, and average ground diameter were recorded. In addition, survey plots for understory woody vegetation (2 × 2 m) and herbaceous plants (1 × 1 m) were randomly placed within each permanent plot for species identification and to measure the average height and coverage of both woody and herbaceous plants.
2.2 Changes in biomass and soil organic carbon stocks
Initial aboveground and belowground biomass carbon stocks for 2013 and change in forest aboveground biomass carbon stocks (ΔAGBC) and belowground biomass carbon stocks (ΔBGBC) data for 2013–2017 were obtained from the National Tibetan Plateau Data Center (NTPDC,https://data.tpdc.ac.cn/) with a spatial resolution of 1 × 1 km (Chen Y. et al., 2023). Given the correlation of ΔAGBC and ΔBGBC, we sum AGBC and BGBC as initial biomass carbon stocks (hereafter BCi) and sum ΔAGBC and ΔBGBC as biomass carbon accumulation (hereafter ΔBC), (Supplementary Figure 3). Initial soil carbon stocks (hereafter Soil C content) for 2013 and change in organic carbon stocks (hereafter ΔSOC) data for 2013–2017 were obtained from the NESSDC (http://www.geodata.cn) with a spatial resolution of 1 × 1 km (Zhao et al., 2021; Xie et al., 2022) (Supplementary Figure 3). Additionally, we obtained the mean annual precipitation (MAP), mean annual temperature (MAT), and mean annual potential evapotranspiration (PET) from the NESSDC (http://www.geodata.cn) at a resolution of 1 × 1 km for the period of 2000–2020. Due to the high correlations among climate variables (MAT, MAP, and PET) (r = 0.782–0.945) (Supplementary Figure 4), we conducted principal component analysis (PCA) (Chu et al., 2019; Chen et al., 2021) and used the score of the first axis to represent climate (explaining 90.0% of the variation) (Supplementary Figure 5).
2.3 Quantization of tree species diversity, stand structure, and functional traits
In this study, the diversity of tree species (referred to as α-Tree) was assessed based on the number of tree species present within each sample plot (Wang T. et al., 2023). We defined five stand structure variables, including age group (hereafter Age, classification criteria according to Supplementary Tables 1, 2 and Supplementary Figure 6 and number of sample plots for each age group see Supplementary Table 3), stem abundance (hereafter SA, represents stand density, individual ha−1), crown density (hereafter CD), mean DBH (hereafter m-DBH, Penone et al., 2019), and tree diameter size variation (hereafter CV-DBH, computed as the coefficient of variation of DBH within sample plot, Chu et al., 2019; Wang T. et al., 2023). We employed two primary functional traits to characterize functional diversity: the stand structural complexity index (hereafter FDSSCI, defined by the extent of heterogeneity in leaf area and biomass, scaling by sample plot, and SSCI data are sourced from Ehbrecht et al., 2021; available at https://zenodo.org/records/4295910), canopy height (hereafter FDCH, scaling by sample plot, and CH data are obtained from Liu et al., 2022; available at https://3decology.org/dataset-software/). Specifically, the α-Tree is linked to changes in the composition and availability of resources (light, water, and soil nutrients) and an enhancement in horizontal and vertical space diversity (Penone et al., 2019; Wang T. et al., 2023). Therefore, the α-Tree is anticipated to increase niche diversity and resource-use efficiency. Tree species diversity also exerted more pronounced effects on the functioning of forests with soils possessing greater nutrient and water-holding capacities (Ratcliffe et al., 2017). Substantial differences in light transmittance can arise among various tree species, and there are also variations in water uptake by roots (Barbier et al., 2008; Wang T. et al., 2023). The photosynthetic carbon sequestration capacity and environmental adaptability of different tree species are also different, resulting in differences in carbon allocation between the aboveground and belowground parts, which affects the soil carbon sequestration function (Oostra et al., 2006). Roots of different tree species secrete specific compounds that affect soil microbial community diversity, soil quality, and microclimate within the soil, thereby affecting soil carbon sequestration (Bárta et al., 2017; Florio et al., 2021). The stand structure SA is closely related to forest aboveground biomass, associated with canopy density, and has the potential to reduce by canopy packing (Chiang et al., 2016), thereby impacting competitive intensity and resource utilization (Zhang Y. et al., 2021). The CD is closely related to the transmission of light and interception of water, consequently affecting resource availability and distribution, and resource-use efficiency (Curtis and Gough, 2018). The m-DBH affects both the chemical and structural properties of the soil (Dupouey et al., 2002; Wang T. et al., 2023). The CV-DBH, usually defined as stand structure complexity (Ali et al., 2016, 2019) and expected to boost niche diversity, can also impact the variation of stand microclimate (Wang T. et al., 2023). The function traits FDSSCI seeks to measure the distribution of canopies in three-dimensional space. This quantification represents spatial patterns of the canopy in three-dimensional space (Ehbrecht et al., 2021), closely tied to variations in the availability of resources (Barbier et al., 2008). The FDCH is a crucial factor in estimating forest biomass and has the potential to enhance niche diversity (Liu et al., 2022; Wang T. et al., 2023).
2.4 Statistical analysis
We added a linear mixed-effects model to the piecewise structural equation model (SEM) (Jin et al., 2022) to evaluate the direct and the indirect effects of climate, tree species diversity, stand structure, and function traits on carbon accumulation (ΔBC and ΔSOC), considering climate gradients as random effects. We eliminated collinearity among climate factors and the diversity of tree species, stand structure, and function traits through separate analyses (Supplementary Figures 7, 8) and excluded variables highly correlated with others (Pearson's r > 0.60) by conducting a principal component analysis (PCA) (Supplementary Figure 5) (Chu et al., 2019; Wang T. et al., 2023). Hence, one climate variable (MAP), tree species diversity, five stand structure features (Age, SA, CD, m-DBH, and CV-DBH), and two functional diversity factors (FDSSCI and FDCH) were included in the SEM. We also used the corresponding initial BC and SOC stocks (BCi and Soil C content) for assessing changes in biomass and soil carbon stocks. To ensure comparability of model coefficients, we standardized both the response variables and predictors using the z-score (with an overall mean of 0 and a standard deviation of 1) prior to analysis (Penone et al., 2019; Chen et al., 2021; Zhang R. et al., 2022). Model evaluation by the criteria: (1) pathway significance (p < 0.05) and satisfactory fit (P > 0.05) and (2) the goodness of fit of the model [the chi-square test (χ2) (P > 0.05) and 0 ≤ Fisher′sC/df ≤ 2]. Finally, We removed paths with non-significant associations (P > 0.05) in SEMs that exhibited satisfactory model fit and then re-evaluated the model fit (Chu et al., 2019; Wang H. et al., 2023). Similar to a previous study (Carol Adair et al., 2018), we computed the gain or loss in biomass carbon and soil organic carbon stocks (via direct and indirect effects) by multiplying the unstandardized effect size by the range of that variable (Supplementary Table 4) (Chen X. et al., 2023).
The analyses of this study were conducted in the RStudio 4.2.3 environment (R Core Team, 2023). The SEM analysis was performed using the “piecewiseSEM 2.1.0” package (Lefcheck, 2016), and LMM analysis was implemented using the “nlme 3.1-162” (Pinheiro et al., 2021). All of these results were visualized with the “ggplot2” package (Wickham, 2016) in the RStudio 4.2.3.
3 Results
3.1 Change in biomass carbon stocks (ΔBC)
The structural equation model (SEM) for changes in forest biomass carbon stocks in both natural forests (ΔBCNF) and planted forests (ΔBCPF) exhibited a strong fit with the data (ΔBCNF stock: P = 0.68, Fisher's C/df < 2; ΔBCPF stock: P = 0.38, Fisher's C/df < 2) (Figures 3A, C). Both ΔBCNF and ΔBCPF stocks were influenced by tree species diversity and stand structure, although climate and carbon background conditions (BCi and Soil C content) had distinct effects on ΔBCNF and ΔBCPF. Specifically, tree species diversity (α-Tree) exhibited a direct and negative correlation with ΔBCNF, with a total effect size of −0.036 (Figures 3A, B; Supplementary Figure 9). Conversely, α-Tree had an indirect positive correlation with ΔBCPF, with a total effect size of 0.012 (Figures 3C, D; Supplementary Figure 9). The Age and stem abundance (SA) were positively correlated with ΔBCPF, with total effect sizes of −0.015 and −0.043, while negatively correlated with ΔBCNF, with total effect sizes of 0.015 and 0.004. The mean DBH (m-DBH), crown density (CD), and functional traits of stand structure complexity index (FDSSCI) had an indirect impact on ΔBCPF but showed no correlation with ΔBCNF. In both NFs and PFs, the initial biomass carbon (BCi) was directly negatively correlated with ΔBC, with total effect sizes of −0.142 in NFs and −0.095 in PFs (Figure 3; Supplementary Figure 9). Based on the SEM results, increasing α-Tree from its minimum to maximum value (Supplementary Table 4) led to a decrease of −13.034 tC/ha in ΔBCNF and an increase of 1.443 tC/ha in ΔBCPF, increasing SA from its minimum to maximum value led to a decrease of −231.727 tC/ha in ΔBCNF, and an increase of 10.668 tC/ha in ΔBCPF, increasing BCi from its minimum to maximum value led to a decrease of −13.889 tC/ha in ΔBCNF and an increase of −6.067 tC/ha in ΔBCPF (Table 1).
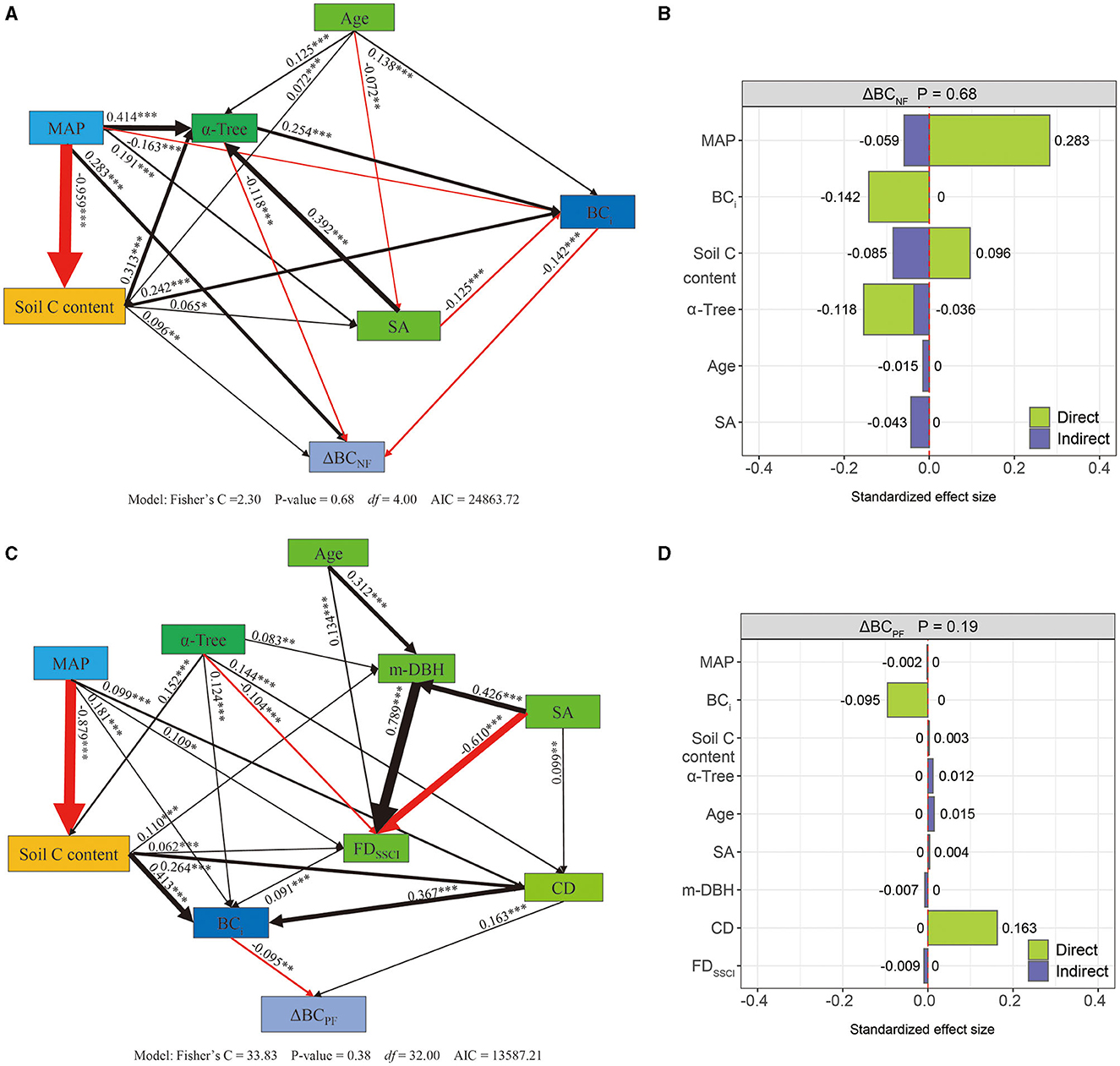
Figure 3. Structural equation model showing the effects of climatic, tree species diversity, and stand structure on forest biomass carbon accumulation in natural forests (ΔBCNF, n = 1,910, A, B) and planted forests (ΔBCPF, n = 1,038, C, D). (A, C) Path diagrams of factors influencing changes in ΔBC—the black and red arrows represent significant positive and negative pathways, respectively. The path coefficients are denoted by bold numbers, and the width of the arrows reflects the strength of the relationship; the significance levels are indicated as follows: p < 0.001 (***), p < 0.01 (**), and p < 0.05 (*). (a2, b2) Summed direct and indirect effects. (B, D) Standardized effect size of variables influencing changes in ΔBC—the red dotted line is the reference line, indicating that the effect is 0.
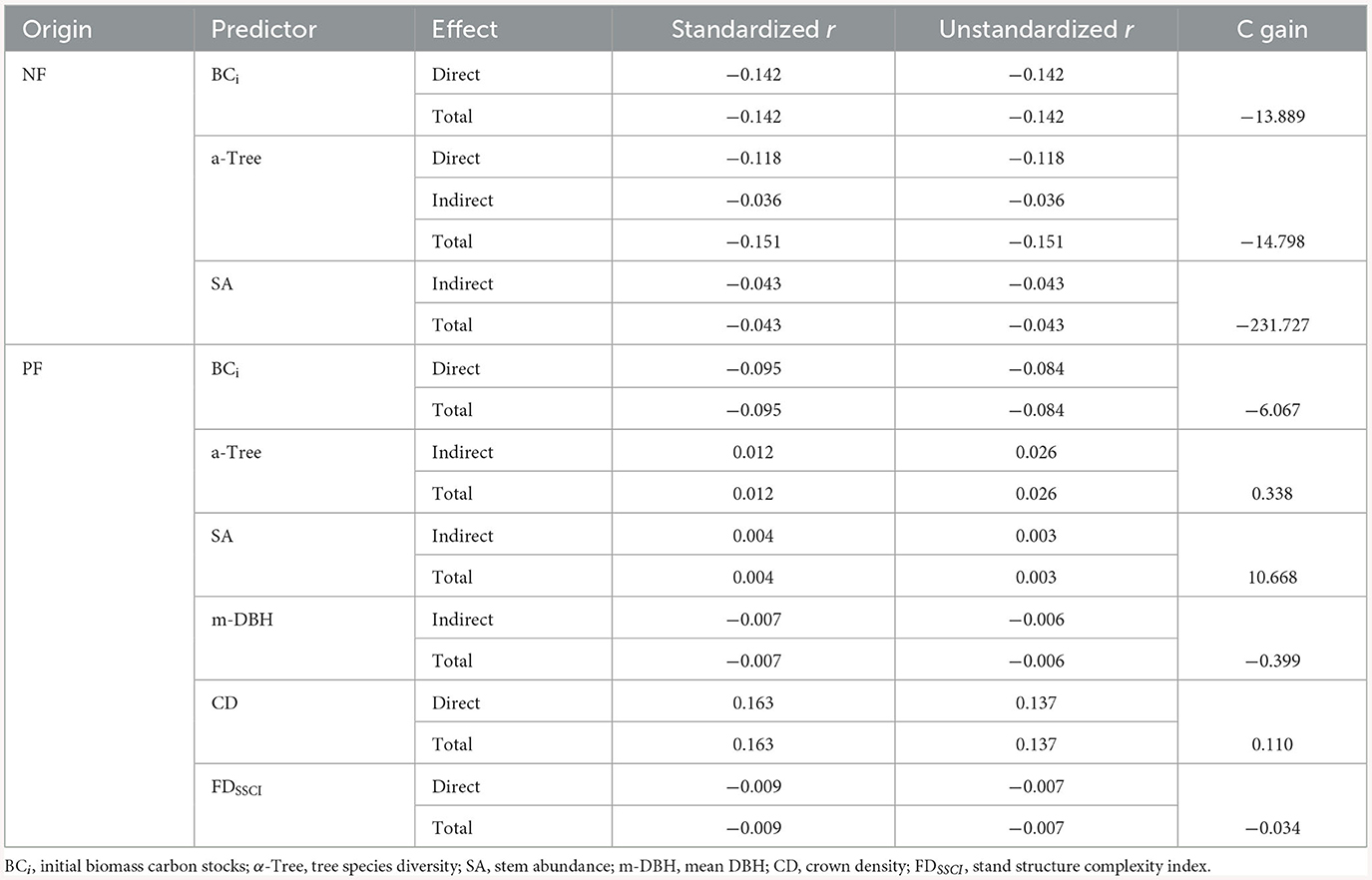
Table 1. Standardized and unstandardized effect sizes and the changes of forest aboveground biomass carbon stock (t C/ha) in natural (ΔBCNF, n = 1,910) and planted forests (ΔBCPF, n = 1,910).
3.2 Change in soil organic carbon stocks (ΔSOC)
The structural equation model (SEM) for changes in forest soil organic carbon stocks in both natural forests (ΔSOCNF) and planted forests (ΔSOCPF) also demonstrated a strong fit with the data (ΔSOCNF stock: P = 0.19, Fisher's C/df < 2; ΔSOCPF stock: P = 0.63, Fisher's C/df < 2) (Figures 4A, C). The α-Tree indirectly exhibited a negative correlation with ΔSOCNF, with a total effect size of −0.004 (Figures 4A, B; Supplementary Figure 10). Conversely, the α-Tree had an indirect positive correlation with ΔSOCPF, with a direct effect size of 0.001 (Figures 4C, D; Supplementary Figure 10). The Age and SA indirectly exhibited a negative correlation with ΔSOCNF, with a total effect size of −0.032 and −0.022 (Figure 4B; Supplementary Figure 10). Conversely, the Age and SA have an indirect positive correlation with ΔSOCPF, with a direct effect size of 0.005 and 0.015 (Figure 4D; Supplementary Figure 10). In comparison with ΔBCNF, the tree diameter size variation (CV-DBH) and m-DBH negatively correlated with ΔSOCNF but not positively correlated with ΔBC, with total effect sizes of −0.001 and −0.091, respectively. In comparison with ΔBCPF, the m-DBH and FDSSCI show a positive correlation with ΔSOCPF (effect size is 0.009 and 0.012), while the effect size in ΔBCPF is −0.007 and −0.009. Overall, in both NFs and PFs, the initial biomass carbon (BCi) was directly positively correlated with ΔSOC, with total effect sizes of 0.087 in NFs and 0.130 in PFs (Figure 4; Supplementary Figure 10). Soil C content shows a negative correlation with ΔSOC but a positive correlation with ΔBC. Based on the SEM results, increasing α-Tree from its minimum to maximum value (Supplementary Table 4) resulted in a decrease of −0.686 tC/ha in ΔSOCNF and an increase of 0.065 tC/ha in ΔSOCPF (Table 2), increasing SA from its minimum to maximum value led to decreases of −231.727 tC/ha in ΔSOCNF and 64.008 tC/ha in ΔSOCPF stocks, increasing BCi from its minimum to maximum value led to decreases of 8.607 tC/ha in ΔSOCNF and 11.485 tC/ha in ΔSOCPF stocks.
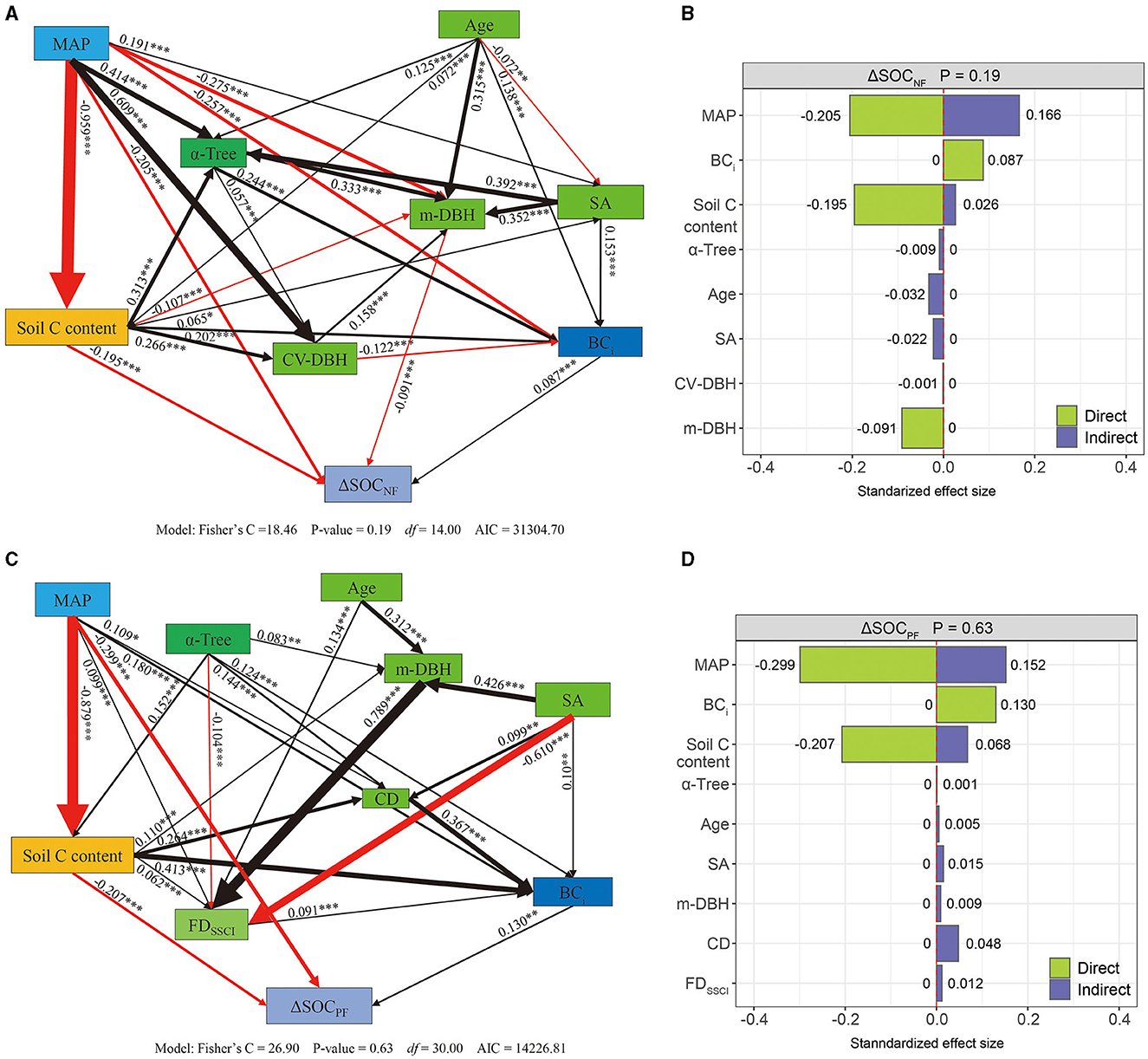
Figure 4. Structural equation model showing the effects of climatic, tree species diversity, and stand structure on forest soil organic carbon accumulation in natural (ΔSOCNF, n = 1,910, A, B) and planted forests (ΔSOCPF, n = 1,038, C, D). (C, D) Path diagrams of factors influencing changes in ΔSOC—the red and black arrows represent significant positive and negative pathways, respectively. The path coefficients are denoted by bold numbers, and the width of the arrows reflects the strength of the relationship, where positive values are represented in black font and negative values are indicated in red font, the significance levels are indicated as follows: p < 0.001 (***), p < 0.01 (**), p < 0.05 (*). (B, D) Standardized effect size of factors influencing changes in ΔSOC—the red dotted line is the reference line, indicating that the effect is 0.
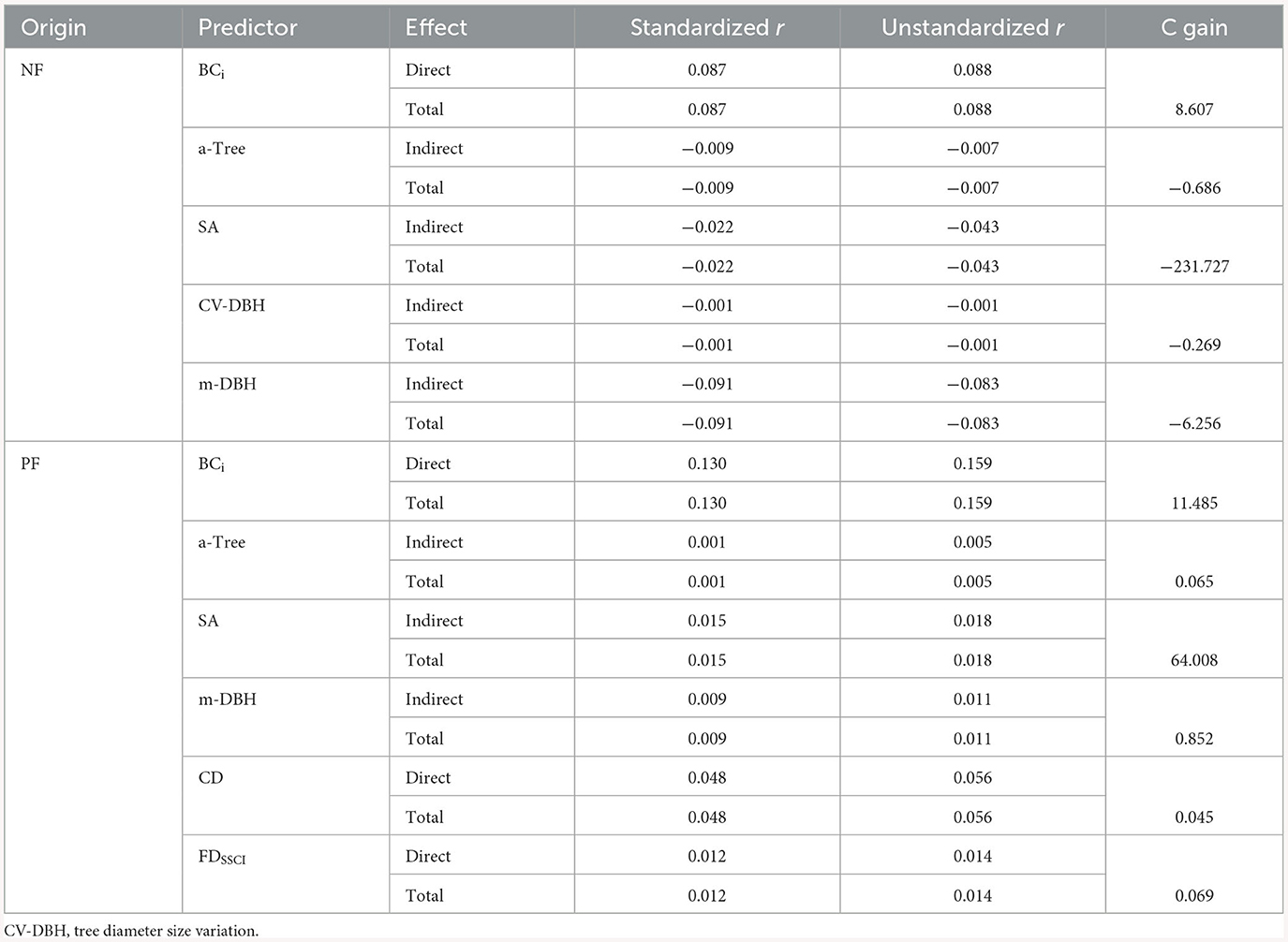
Table 2. Standardized and unstandardized effect sizes and the changes of forest soil organic carbon (t C/ha) in natural (ΔSOCNF, n = 1,910) and planted forests (ΔSOCPF, n = 1,038).
4 Discussion
In contrast to conventional experiments that solely take into account independent carbon pools or stocks, we drive that biomass carbon and soil organic carbon accumulation provides large-scale evidence that tree species diversity, stand structure, and function traits in diffident carbon accumulation play different roles. More importantly, these direct or indirect, positive or negative effects were different in natural forests and planted forests, which enhanced our capacity to predict the outcomes of changes in carbon stocks via management across a large scale. Overall, this study highlights the diffident management need to be implanted in different carbon pools in natural forests and planted forests for carbon stock gain and carbon neutrality.
4.1 Tree species diversity and stand structure play multiple roles in carbon accumulation across diffident carbon pool
Various local-scale ecological mechanisms, including niche complementarity, selection, mass ratio, and the effects of competitive exclusion, have been suggested to clarify the connection between biodiversity and carbon stocks (Poorter et al., 2015; Ali et al., 2016, 2019). Our findings align with these conclusions, highlighting that tree species diversity and stand structure play significant roles in carbon storage. These results can be extrapolated to a broader context, encompassing all of China, where tree species diversity and stand structure are positively correlated with initial biomass carbon stocks (BCi) (Chen et al., 2018). Nevertheless, our research indicates that tree species diversity and stand structure may diminish the carbon accumulation of biomass carbon in natural forests (ΔBCNF). Consistent with our hypothesis, initial BCi displays a negative correlation with carbon accumulation in BC and a positive correlation with soil organic carbon (SOC). This leads us to postulate that the adverse relationship between tree species diversity, stand structure, and BC accumulation is intertwined with China's unique forest management policy. Since 1998, China has been implementing comprehensive static logging practices in its natural forests, fostering forest restoration and ecological succession (Jing et al., 2021). However, in light of the predominant tree species in China's natural forests, many forests have now reached middle age (China State Forestry and Grassland Administration, 2019; Xia et al., 2023). Data show that the proportion of middle-aged natural forests is 36% (Supplementary Table 3). The net carbon gain in the forest rises swiftly during young age, reaches its peak in middle age, and subsequently declines in old age (Ryan et al., 2004; Fonseca et al., 2011; Goulden et al., 2011; Shang et al., 2023). As stand age increases, stand growth slows down (Tang et al., 2014) and BC accumulation levels remain stagnant or even become a carbon source (Shang et al., 2023). Yue and Li (2021) concluded that the largest BC in China's natural forests is found in middle-aged forests, He et al. (2017) concluded that the BC sequestration rate of China might reach its maximum around 2020, and Shang et al. (2023) concluded that it would reach the maximum in 2025. Additionally, a portion of the BC is released into the atmosphere, while the rest enters the soil through coarse woody debris, roots, and litter, eventually becoming sequestered in the soil organic layer (Zhu et al., 2017; Curtis and Gough, 2018; Basile-Doelsch et al., 2020; Fan et al., 2023). These conclusions are corroborated by our findings, which demonstrate that higher BCi positively correlates with SOC accumulation. In this process, higher tree species diversity and a more intricate stand structure facilitate the conversion of BC into SOC. Elevated stand density intensifies competition and mortality rates (Zhang et al., 2020) and increased stand density also increases tree diversity (Chu et al., 2019), thereby enhancing the likelihood of BC-to-SOC conversion. On the other hand, stand density is positively related to initial BC storage levels. High stand density often means high initial BC storage levels. Building upon these insights, we recommend reducing BCi and stand density through selective logging practices, sequestering this BC fraction in wood products. Simultaneously, we propose actively promoting understory regeneration to encourage more BC to serve as carbon sinks. This measure not only aids the biomass carbon gains of natural forests but also involves planting tree species in the advanced successional stage within the forest. Some top-tier tree species, such as Pinus koraiensis and genetically improved tree species, exhibit a longer biomass carbon storage duration.
Forest soils play a vital role in capturing atmospheric carbon, accounting for 82.9% of carbon storage in China's terrestrial ecosystems, exceeding 16.5% of carbon stored in biomass (Tang et al., 2018). Recent research by Chen X. et al. (2023) suggests that tree species evenness increases forest soil carbon accrual in the mineral soil horizon, and tree species diversity has no significant effect on carbon accumulation in the organic horizon in natural forests. Our findings are different from this conclusion, highlighting that tree species diversity and stand structure are negatively related to soil carbon accumulation in the organic soil horizon in natural forests. In contrast, in our study, high tree species diversity and stand density demonstrated a positive correlation with initial SOC stocks. However, consistent with our hypothesis, initial SOC stocks were negatively correlated with SOC accumulation, as elevated SOC levels inhibited further carbon accumulation. Additionally, initial SOC storage exhibits a negative correlation with precipitation. High precipitation leading to increased tree species diversity (Chu et al., 2019) and stand structure complexity (Ehbrecht et al., 2021), intensifies microorganism-driven decomposition, further amplifying SOC decomposition (Averill et al., 2014; Chen et al., 2018; Tang et al., 2018; Li B. et al., 2023). This collective evidence demonstrates that climate indirectly influences the conversion of BC to SOC through the effect of increasing species diversity and stand structure complexity, ultimately bolstering SOC accumulation. Notably, this effect of the conversion of BC to SOC has overshadowed the respiration and consumption of SOC. With higher tree diversity and complex stand structures, microorganisms are more effective at the decomposition of coarse woody debris, roots, and litter (Frey et al., 2014; Tao et al., 2023). However, higher tree species diversity and structure complexity may also lead to higher respiration and consumption of SOC. Increasing stand density increases the conversion potential of BC to SOC. However, increased stand density also increases tree diversity (Chu et al., 2019), which results in enhanced respiration and consumption of SOC. Therefore, we recommend selective logging in natural forests for both biomass carbon and soil organic carbon accumulation. Furthermore, we recommend focusing more on soil organic carbon in the north and northwest China (Supplementary Figure 4), where the carbon sequestration in soil duration is longer due to the colder and drought climate (Muñoz et al., 2023). In addition, given the lower initial carbon stocks in north and northwest China (Supplementary Figure 3), this region has greater SOC sequestration potential. In contrast, in regions with abundant precipitation (Supplementary Figure 4) and BC sequestration potential in east and south China (Peng et al., 2023), it is advisable to focus more on biomass carbon accumulation. It must be noted that our study only considered soil surface organic carbon. The above results may become inconsistent for SOC in deeper soil layers. In addition, soil carbon is also stored in other ways, such as minerals. The mechanisms influencing deep SOC and other forms of carbon gains require further study.
4.2 The relationship between tree species diversity, stand structure, and carbon accumulation in both NFs and PFs
Examining the disparity in carbon accumulation capacity between natural and planted forests is essential for promoting sustainable forest management. In comparison with natural forests, we observed positive correlations in tree species diversity, stand structure, BC, and SOC accumulation in planted forests. This result is consistent with those of Huang et al. (2018), Augusto and Boča (2022), and Feng et al. (2022). Our results also demonstrate that stand age (Age) is positively correlated with BC and SOC accumulation in planted forests and negatively correlated with BC and SOC accumulation in natural forests. When comparing planted forests to natural forests, the dataset reveals that planted forests have a lower tree species diversity and stand density (Wang Y. et al., 2023). Moreover, a substantial portion−40.72%—of China's planted forests is comprised of young-aged forests (Tang et al., 2018; Li X. et al., 2023). Finally, planted forests show a lower initial BC stock, the aboveground BC storage only constitutes 19.86% of the total forested area in China (State Forestry Grassland Administration of China, 2021). This stage is dominated by carbon gain rather than carbon loss (Cheng et al., 2015), the roles of tree species diversity are more pronounced in promoting BC accumulation than facilitating the conversion of BC into SOC. Lower tree species diversity and homogeneous stand structure also may tend to decelerate the conversion of BC into SOC and SOC loss. Therefore, we speculate that the roles of tree species diversity on carbon accumulation are closely correlated with the level of initial BC and SOC stock. In addition, our research highlights that an increase in stand density leads to significant initial BC and SOC accumulation enhancement in PF. Increasing stand density notably intensifies interspecific competition and raises the potential for biomass BC to transform into SOC. However, compared with NFs, PFs have lower stand density and initial carbon storage levels and are mostly in the young-aged stage, which is the accumulation stage of BC (Peichl and Arain, 2006; Cheng et al., 2015). Increased stand density also increases BC accumulation. Therefore, we infer that the role of stand density in carbon accumulation depends primarily on the initial carbon storage level. When carbon storage levels are initially low, species diversity and stand structure play more positive roles. Overall, PFs should increase tree species diversity and stand density for carbon stock gain. Furthermore, our results indicate that initial BC and SOC levels are related to stand age, similarly in NFs and PFs. It shows that stand age is an important stand structure indicator in the process of forest management (Ouyang et al., 2019). To achieve the goals of carbon gains and carbon neutrality, different stand ages may require different forest management strategies, which requires further research. Finally, in comparison with NFs, tree species diversity is more significantly influenced by afforestation and less affected by climate. As a result, our model does not consider the influence of climate on tree species diversity in PFs.
Obtaining large-scale vegetation functional traits can be challenging, such as nitrogen content per leaf dry mass, phosphorus content per leaf dry mass, and specific leaf area. In this study, we focused on two functional traits: the Stand Structure Complexity Index (FDSSCI) and Canopy Height (FDCH). FDSSCI represents the heterogeneity of the three-dimensional spatial distribution of leaf area and leaf biomass, scaling by sample plot (Ehbrecht et al., 2021; Ray et al., 2023), while FDCH serves as the potential maximum height, scaling by sample plot (Liu et al., 2022). We found that functional traits of FDSSCI negatively influenced BC accumulation and positively influenced SOC accumulation in PFs, whereas there was no significant effect in NFs. The relationship between function traits and carbon accumulation could be associated with “canopy packing” (Ren et al., 2021), a strategy that maximizes the utilization of space (Chiang et al., 2016). High canopy packing promotes initial biomass carbon storage. Our results also demonstrate that canopy density (CD) showed a positive correlation with BC and SOC accumulation in PFs, whereas there was no significant effect in NFs. Increased canopy density (CD) also leads to a higher canopy packing (Xu et al., 2018), consequently leading to higher initial BC storage. The initial BC storage promotes the accumulation of SOC but limits the accumulation of BC. Compared with functional traits, canopy density not only promotes initial BC but also directly promotes BC accumulation when initial carbon stock levels are low in PFs. Therefore, we speculate that the roles of canopy density on carbon accumulation are closely correlated with the level of initial BC and SOC stock. In conclusion, our findings suggest that higher tree species diversity, stand density, and canopy density equally stimulate the accumulation of both BC and SOC in PFs. Our results also show that the average diameter (m-DBH) had a negative correlation with BC accumulation and a positive correlation with SOC accumulation in PFs. On the one hand, the average diameter of the tree reflects the stand age (Dupouey et al., 2002), which is related to the initial carbon storage level (Stephenson et al., 2014; Meakem et al., 2018; Piponiot et al., 2022). Generally speaking, the larger the average diameter of a tree, the higher the initial biomass carbon storage level (Hauck et al., 2023). Consistent with our hypothesis, these results demonstrate our hypothesis that the role of tree species diversity and stand structure variables in the carbon accumulation process mainly depends on the initial carbon storage level. These findings suggest that, when managing existing forests to achieve carbon-increasing goals, careful consideration of the forest's initial carbon storage level is necessary. Previous research has indicated that a forest with initially high carbon storage may not exhibit a high carbon sink potential (Baker et al., 2019; Peng et al., 2023). Our research further demonstrates that in cases of high initial carbon storage, tree species diversity and stand structural characteristics, such as stand density and canopy density, may also have a negative impact, further diminishing the forest's carbon sink potential. However, a comprehensive exploration of the maximum carbon sink potential in established forest lands still requires further study. Conversely, when carbon storage levels are initially low, we can enhance the forest's carbon sink capacity by increasing tree species diversity and stand density.
5 Conclusion
Our findings illustrate the impact of tree species diversity, stand structure, and functional traits on carbon accumulation across various carbon pools in forests of different origin types on a national spatial scale. These very important findings underscore that various carbon pools respond differently to distinct tree species diversity, stand structure, and function traits in natural forests and planted forests. Consistent with our hypothesis, increasing tree species diversity is beneficial for the accumulation of biomass carbon and soil organic carbon in planted forests. However, the higher tree species diversity may limit the accumulation of biomass carbon and soil organic carbon in natural forests in the context of high initial carbon stocks. Furthermore, our results indicate that high stand density may limit further carbon accumulation in biomass carbon and soil organic carbon in natural forests in the context of high initial carbon stocks. In contrast, low stand density may limit the development of carbon storage potential in planted forests in China. These differentiated results raise our awareness that different management strategies also need to be implemented in natural forests and planted forests. To enhance carbon sequestration capacity, China should change its current policy of completely banning logging of natural forests. Natural forests need thinning and reduced tree species diversity, while planted forests should increase tree species diversity and stand density. In the northeast and southwest forest regions of China, the primary consideration should revolve around SOC in NFs. Conversely, in the south and east regions of China, the primary focus should be on BC in PFs.
Data availability statement
The original contributions presented in the study are included in the article/Supplementary material, further inquiries can be directed to the corresponding author.
Author contributions
TW: Data curation, Formal analysis, Writing—original draft. LD: Conceptualization, Writing—review & editing. ZL: Funding acquisition, Writing—review & editing.
Funding
The author(s) declare financial support was received for the research, authorship, and/or publication of this article. This work was supported by the Fundamental Research Funds for the Central Universities of China [Grant Number: 2572021DT07], Northeast Forestry University 2022 doctoral candidate independent innovation fund project program [Grant Number: 2572022AW08], and National Key R&D Program of China [Grant Number: 2022YFD2200502].
Acknowledgments
The authors acknowledge the National Earth System Science Data Center, the National Science & Technology Infrastructure of China (NESSDC, http://www.geodata.cn), and the National Tibetan Plateau Data Center (NTPDC, https://data.tpdc.ac.cn/) for providing data support.
Conflict of interest
The authors declare that the research was conducted in the absence of any commercial or financial relationships that could be construed as a potential conflict of interest.
Publisher's note
All claims expressed in this article are solely those of the authors and do not necessarily represent those of their affiliated organizations, or those of the publisher, the editors and the reviewers. Any product that may be evaluated in this article, or claim that may be made by its manufacturer, is not guaranteed or endorsed by the publisher.
Supplementary material
The Supplementary Material for this article can be found online at: https://www.frontiersin.org/articles/10.3389/ffgc.2023.1333868/full#supplementary-material
References
Ali, A., Lin, S. L., He, J. K., Kong, F. M., Yu, J. H., and Jiang, H. S. (2019). Climate and soils determine aboveground biomass indirectly via species diversity and stand structural complexity in tropical forests. For. Ecol. Manage. 432, 823–831. doi: 10.1016/j.foreco.2018.10.024
Ali, A., Yan, E. R., Chen, H. Y., Chang, S. X., Zhao, Y. T., Yang, X. D., et al. (2016). Stand structural diversity rather than species diversity enhances aboveground carbon storage in secondary subtropical forests in Eastern China. Biogeosciences 13, 4627–4635. doi: 10.5194/bg-13-4627-2016
Augusto, L., and Boča, A. (2022). Tree functional traits, forest biomass, and tree species diversity interact with site properties to drive forest soil carbon. Nat. Commun. 13, 1097. doi: 10.1038/s41467-022-28748-0
Averill, C., Turner, B. L., and Finzi, A. C. (2014). Mycorrhiza-mediated competition between plants and decomposers drives soil carbon storage. Nature 505, 543–545. doi: 10.1038/nature12901
Baker, J. S., Wade, C. M., Sohngen, B. L., Ohrel, S., and Fawcett, A. A. (2019). Potential complementarity between forest carbon sequestration incentives and biomass energy expansion. Energy Policy 126, 391–401. doi: 10.1016/j.enpol.2018.10.009
Barbier, S., Gosselin, F., and Balandier, P. (2008). Influence of tree species on understory vegetation diversity and mechanisms involved—a critical review for temperate and boreal forests. For. Ecol. Manage. 254, 1–15. doi: 10.1016/j.foreco.2007.09.038
Bárta, J., Tahovská, K., Šantručková, H., and Oulehle, F. (2017). Microbial communities with distinct denitrification potential in spruce and beech soils differing in nitrate leaching. Sci. Rep. 7, 9738. doi: 10.1038/s41598-017-08554-1
Basile-Doelsch, I., Balesdent, J., and Pellerin, S. (2020). Reviews and syntheses: the mechanisms underlying carbon storage in soil. Biogeosciences 17, 5223–5242. doi: 10.5194/bg-17-5223-2020
Brown, H. C., Berninger, F. A., Larjavaara, M., and Appiah, M. (2020). Above-ground carbon stocks and timber value of old timber plantations, secondary and primary forests in southern Ghana. For. Ecol. Manage. 472:118236. doi: 10.1016/j.foreco.2020.118236
Cai, W. X., He, N. P., Li, M. X., Xu, L., Wang, L. Z., Zhu, J. H., et al. (2022). Carbon sequestration of Chinese forests from 2010 to 2060: spatiotemporal dynamics and its regulatory strategies. Sci. Bull. 67, 836–843. doi: 10.1016/j.scib.2021.12.012
Cao, S. R., Li, C., Shankman, D., Wang, C. M., Wang, X. B., and Zhang, H. (2011). Excessive reliance on afforestation in china's arid and semi-arid regions: lessons in ecological restoration. Earth Sci. Rev. 104, 240–245. doi: 10.1016/j.earscirev.2010.11.002
Carol Adair, E., Hooper, D. U., Paquette, A., and Hungate, B. A. (2018). Ecosystem context illuminates conflicting roles of plant diversity in carbon storage. Ecol. Lett. 21, 1604–1619. doi: 10.1111/ele.13145
Carvalhais, N., Forkel, M., Khomik, M., Bellarby, J., Jung, M., Migliavacca, M., et al. (2014). Global covariation of carbon turnover times with climate in terrestrial ecosystems. Nature 514, 213–217. doi: 10.1038/nature13731
Chen, L., Jiang, L., Jing, X., Wang, J., Shi, Y., Chu, H., et al. (2021). Above and belowground biodiversity jointly drive ecosystem stability in natural alpine grasslands on the Tibetan Plateau. Glob. Ecol. Biogeogr. 30, 1418–1429. doi: 10.1111/geb.13307
Chen, S., Wang, W., Xu, W., Wang, Y., Wan, H., Chen, D., et al. (2018). Plant diversity enhances productivity and soil carbon storage. Proc. Nat. Acad. Sci. 115, 4027–4032. doi: 10.1073/pnas.1700298114
Chen, X., Taylor, A. R., Reich, P. B., Hisano, M., Chen, H. Y. H., and Chang, S. X. (2023). Tree diversity increases decadal forest soil carbon and nitrogen accrual. Nature 618, 94–101. doi: 10.1038/s41586-023-05941-9
Chen, Y., Feng, X., and Fu, B. (2023). Above- and belowground forest biomass carbon pool in China during 2002~2021. National Tibetan Plateau Data Center. doi: 10.5194/essd-15-897-2023
Cheng, J., Lee, X., Theng, B. K., Zhang, L., Fang, B., and Li, F. (2015). Biomass accumulation and carbon sequestration in an age-sequence of Zanthoxylum bungeanum plantations under the Grain for Green Program in karst regions, Guizhou province. Agric. Forest Meteorol. 203, 88–95. doi: 10.1016/j.agrformet.2015.01.004
Chiang, J. M., Spasojevic, M. J., Muller-Landau, H. C., Sun, I. F., Lin, Y., Su, S. H., et al. (2016). Functional composition drives ecosystem function through multiple mechanisms in a broadleaved subtropical forest. Oecologia 182, 829–840. doi: 10.1007/s00442-016-3717-z
China State Forestry and Grassland Administration (2019). China Forest Resources Report (2014-2018). Beijing: China Forestry Press.
Chu, C. J., Lutz, J. A., Král, K., Vrška, T., Xue, Y., Myers, J. A., et al. (2019). Direct and indirect effects of climate on richness drive the latitudinal diversity gradient in forest trees. Ecol. Lett. 22, 245–255. doi: 10.1111/ele.13175
Curtis, P. S., and Gough, C. M. (2018). Forest aging, disturbance and the carbon cycle. New Phytol. 219, 1188–1193. doi: 10.1111/nph.15227
Dupouey, J. L., Dambrine, E., Laffite, J. D., and Moares, C. (2002). Irreversible impact of past land use on forest soils and biodiversity. Ecology 83, 2978–2984. doi: 10.1890/0012-9658(2002)083[2978:IIOPLU]2.0.CO;2
Ehbrecht, M., Seidel, D., Annighöfer, P., Kreft, H., Köhler, M., Zemp, D. C., et al. (2021). Global patterns and climatic controls of forest structural complexity. Nat. Commun. 12, 519. doi: 10.1038/s41467-020-20767-z
Fan, X., Bai, E., Zhang, J., Wang, X., Yuan, W., and Piao, S. (2023). The carbon transfer from plant to soil is more efficient in less productive ecosystems. Global Biogeochem. Cycles 37:e2023GB007727. doi: 10.1029/2023GB007727
Fang, J. Y., Chen, A. P., Peng, C. H., Zhao, S. Q., and Ci, L. J. (2001). Changes in forest biomass carbon storage in China between 1949 and 1998. Science 292, 2320–2322. doi: 10.1126/science.1058629
Feng, Y., Schmid, B., Loreau, M., Forrester, D. I., Fei, S., Zhu, J., et al. (2022). Multispecies forest plantations outyield monocultures across a broad range of conditions. Science 376, 865–868. doi: 10.1126/science.abm6363
Florio, A., Marechal, M., Legout, A., Des Chatelliers, C. C., Gervaix, J., Didier, S., et al. (2021). Influence of biological nitrification inhibition by forest tree species on soil denitrifiers and N2O emissions. Soil Biol. Biochem. 155:108164. doi: 10.1016/j.soilbio.2021.108164
Fonseca, W., Benayas, J. M. R., and Alice, F. E. (2011). Carbon accumulation in the biomass and soil of different aged secondary forests in the humid tropics of Costa Rica. For. Ecol. Manage. 262, 1400–1408. doi: 10.1016/j.foreco.2011.06.036
Frey, S. D., Ollinger, S., Nadelhoffer, K. E., Bowden, R., Brzostek, E., Burton, A., et al. (2014). Chronic nitrogen additions suppress decomposition and sequester soil carbon in temperate forests. Biogeochemistry 121, 305–316. doi: 10.1007/s10533-014-0004-0
Gamon, J. A. (2023). Revisiting the carbon–biodiversity connection. Glob. Chang. Biol. 29, 5117–5119. doi: 10.1111/gcb.16829
Gao, J., Ji, Y., and Zhang, X. (2023). Net primary productivity exhibits a stronger climatic response in planted versus natural forests. For. Ecol. Manage. 529:120722. doi: 10.1016/j.foreco.2022.120722
Ge, W., Deng, L., Wang, F., and Han, J. (2021). Quantifying the contributions of human activities and climate change to vegetation net primary productivity dynamics in China from 2001 to 2016. Sci. Total Environ. 773:145648. doi: 10.1016/j.scitotenv.2021.145648
Goulden, M. L., McMillan, A. M. S., Winston, G. C., Rocha, A. V., Manies, K. L., Harden, J. W., et al. (2011). Patterns of NPP, GPP, respiration, and NEP during boreal forest succession. Glob. Chang. Biol. 17, 855–871. doi: 10.1111/j.1365-2486.2010.02274.x
Hardiman, B. S., Bohrer, G., Gough, C. M., Vogel, C. S., and Curtis, P. S. (2011). The role of canopy structural complexity in wood net primary production of a maturing northern deciduous forest. Ecology 92, 1818–1827. doi: 10.1890/10-2192.1
Harmon, M. E., Fasth, B. G., Yatskov, M., Kastendick, D., Rock, J., and Woodall, C. W. (2020). Release of coarse woody detritus-related carbon: a synthesis across forest biomes. Carbon Balance Manag. 15, 1–21. doi: 10.1186/s13021-019-0136-6
Hauck, M., Csapek, G., and Dulamsuren, C. (2023). The significance of large old trees and tree cavities for forest carbon estimates. For. Ecol. Manage. 546:121319. doi: 10.1016/j.foreco.2023.121319
He, N., Wen, D., Zhu, J., Tang, X., Xu, L., Zhang, L., et al. (2017). Vegetation carbon sequestration in Chinese forests from 2010 to 2050. Glob. Chang. Biol. 23, 1575–1584. doi: 10.1111/gcb.13479
Huang, Y., Chen, Y., Castro-Izaguirre, N., Baruffol, M., Brezzi, M., Lang, A., et al. (2018). Impacts of species richness on productivity in a large-scale subtropical forest experiment. Science 362, 80–83. doi: 10.1126/science.aat6405
Jin, Y., Liu, C., Qian, S. S., Luo, Y., Zhou, R., Tang, J., et al. (2022). Large-scale patterns of understory biomass and its allocation across China's forests. Sci. Total Environ. 804:150169. doi: 10.1016/j.scitotenv.2021.150169
Jing, L., Wenmei, L., Huajin, H., and Jiawei, W. (2021). Will the policy of completely stopping commercial logging of natural forests affect the livelihood capital of forestry farmers? Forestry Econ. 43, 16.
Keith, H., Vardon, M., Obst, C., Young, V., Houghton, R. A., and Mackey, B. (2021). Evaluating nature-based solutions for climate mitigation and conservation requires comprehensive carbon accounting. Sci. Total Environ. 769:144341. doi: 10.1016/j.scitotenv.2020.144341
Lefcheck, J. S. (2016). piecewiseSEM: piecewise structural equation modelling in r for ecology, evolution, and systematics. Methods Ecol. Evol. 7, 573–579. doi: 10.1111/2041-210X.12512
Li, B., Gao, G., Luo, Y., Xu, M., Liu, G., and Fu, B. (2023). Carbon stock and sequestration of planted and natural forests along climate gradient in water-limited area: a synthesis in the China's Loess plateau. Agric. Forest Meteorol. 333:109419. doi: 10.1016/j.agrformet.2023.109419
Li, P., Zhu, J., Hu, H., Guo, Z., Pan, Y., Birdsey, R., et al. (2016). The relative contributions of forest growth and areal expansion to forest biomass carbon. Biogeosciences 13, 375–388. doi: 10.5194/bg-13-375-2016
Li, X., Aguila, L. C. R., Wu, D., Lie, Z., Xu, W., Tang, X., et al. (2023). Carbon sequestration and storage capacity of Chinese fir at different stand– ages. Sci. Total Environ. 904, 166962. doi: 10.1016/j.scitotenv.2023.166962
Li, Y., Bao, W., Bongers, F., Chen, B., Chen, G., Guo, K., et al. (2019). Drivers of tree carbon storage in subtropical forests. Sci. Total Environ. 654, 684–693. doi: 10.1016/j.scitotenv.2018.11.024
Lin, D., Anderson-Teixeira, K. J., Lai, J., Mi, X., Ren, H., and Ma, K. (2016). Traits of dominant tree species predict local scale variation in forest aboveground and topsoil carbon stocks. Plant Soil 409, 435–446. doi: 10.1007/s11104-016-2976-0
Liu, X., Trogisch, S., He, J. S., Niklaus, P. A., Bruelheide, H., Tang, Z., et al. (2018). Tree species richness increases ecosystem carbon storage in subtropical forests. Proc. R. Soc. B 285:20181240. doi: 10.1098/rspb.2018.1240
Liu, X. Q., Su, Y. J., Hu, T. Y., Yang, Q. L., Liu, B. B., Deng, Y. F., et al. (2022). Neural network guided interpolation for mapping canopy height of China's forests by integrating GEDI and ICESat-2 data. Remote Sens. Environ. 269:112844. doi: 10.1016/j.rse.2021.112844
Lu, F., Hu, H. F., Sun, W. J., Zhu, J. J., Liu, G. B., Zhou, W. M., et al. (2018). Effects of national ecological restoration projects on carbon sequestration in China from 2001 to 2010. Proc. Natl. Acad. Sci. USA. 115, 4039–4044. doi: 10.1073/pnas.1700294115
Meakem, V., Tepley, A. J., Gonzalez-Akre, E. B., Herrmann, V., Muller-Landau, H. C., Wright, S. J., et al. (2018). Role of tree size in moist tropical forest carbon cycling and water deficit responses. New Phytol. 219, 947–958. doi: 10.1111/nph.14633
Muñoz, E., Chanca, I., and Sierra, C. A. (2023). Increased atmospheric CO2 and the transit time of carbon in terrestrial ecosystems. Glob. Chang. Biol. 29, 6441–6452. doi: 10.1111/gcb.16961
Nottingham, A. T., Meir, P., Velasquez, E., and Turner, B. L. (2020). Soil carbon loss by experimental warming in a tropical forest. Nature 584, 234–237. doi: 10.1038/s41586-020-2566-4
Oostra, S., Majdi, H., and Olsson, M. (2006). Impact of tree species on soil carbon stocks and soil acidity in southern Sweden. Scand. J. For. Res. 21, 364–371. doi: 10.1080/02827580600950172
Ouyang, S., Xiang, W., Wang, X., Xiao, W., Chen, L., Li, S., et al. (2019). Effects of stand age, richness and density on productivity in subtropical forests in China. J. Ecol. 107, 2266–2277. doi: 10.1111/1365-2745.13194
Ouyang, Z. Y., Zheng, H., Xiao, Y., Polasky, S., Liu, J. G., Xu, W. H., et al. (2016). Improvements in ecosystem services from investments in natural capital. Science 352, 1455–1459. doi: 10.1126/science.aaf2295
Peichl, M., and Arain, M. A. (2006). Above-and belowground ecosystem biomass and carbon pools in an age-sequence of temperate pine plantation forests. Agric. For. Meteorol. 140, 51–63. doi: 10.1016/j.agrformet.2006.08.004
Peng, B., Zhou, Z., Cai, W., Li, M., Xu, L., and He, N. (2023). Maximum potential of vegetation carbon sink in Chinese forests. Sci. Total Environ. 905:167325. doi: 10.1016/j.scitotenv.2023.167325
Penone, C., Allan, E., Soliveres, S., Felipe-Lucia, M. R., Gossner, M. M., Seibold, S., et al. (2019). Specialisation and diversity of multiple trophic groups are promoted by different forest features. Ecol. Lett. 22, 170–180. doi: 10.1111/ele.13182
Pinheiro, J., Bates, D., DebRoy, S., Sarkar, D., and R Core Team (2021). nlme: Linear and nonlinear mixed effects models. R package version 3.1-152. Available online at: https://CRAN.R-project.org/package=nlme
Piponiot, C., Anderson-Teixeira, K. J., Davies, S. J., Allen, D., Bourg, N. A., Burslem, D. F., et al. (2022). Distribution of biomass dynamics in relation to tree size in forests across the world. New Phytol. 234, 1664–1677. doi: 10.1111/nph.17995
Poorter, L., van der Sande, M. T., Thompson, J., Arets, E. J., Alarcón, A., Álvarez-Sánchez, J., et al. (2015). Diversity enhances carbon storage in tropical forests. Glob. Ecol. Biogeogr. 24, 1314–1328. doi: 10.1111/geb.12364
Protocol, K. (1997). United Nations framework convention on climate change. Kyoto Protocol, Kyoto. 19, 1–21.
R Core Team (2023). R: A Language and Environment for Statistical Computing. R Development Core Team. 3.1-164.
Ratcliffe, S., Wirth, C., Jucker, T., van Der Plas, F., Scherer-Lorenzen, M., Verheyen, K., et al. (2017). Biodiversity and ecosystem functioning relations in European forests depend on environmental context. Ecol. Lett. 20, 1414–1426. doi: 10.1111/ele.12849
Ray, T., Delory, B., Bruelheide, H., Eisenhauer, N., Ferlian, O., Quosh, J., et al. (2023). Tree diversity increases productivity through enhancing structural complexity across mycorrhizal types. Sci. Adv. 9, eadi2362. doi: 10.1126/sciadv.adi2362
Ren, S., Ali, A., Liu, H., Yuan, Z., Yang, Q., Shen, G., et al. (2021). Response of community diversity and productivity to canopy gap disturbance in subtropical forests. For. Ecol. Manage. 502:119740. doi: 10.1016/j.foreco.2021.119740
Ryan, M. G., Binkley, D., Fownes, J. H., Giardina, C. P., and Senock, R. S. (2004). An experimental test of the causes of forest growth decline with stand age. Ecol. Monogr. 74, 393–414. doi: 10.1890/03-4037
Schuldt, A., Liu, X., Buscot, F., Bruelheide, H., Erfmeier, A., He, J. S., et al. (2023). Carbon–biodiversity relationships in a highly diverse subtropical forest. Glob. Chang. Biol. 29, 5321–5333. doi: 10.1111/gcb.16697
Shang, R., Chen, J. M., Xu, M., Lin, X., Li, P., Yu, G., et al. (2023). China's current forest age structure will lead to weakened carbon sinks in the near future. Innovation 4:100515. doi: 10.1016/j.xinn.2023.100515
Shu, S. M., Zhu, W. Z., Wang, W. Z., Jia, M., Zhang, Y. Y., and Sheng, Z. L. (2019). Effects of tree size heterogeneity on carbon sink in old forests. For. Ecol. Manage. 432, 637–648. doi: 10.1016/j.foreco.2018.09.023
State Forestry Grassland Administration of China (2021). Enhance the Carbon Sink Potential of Forests and Grasses and Help Achieve the Goal of Carbon Peak Carbon Neutrality. Available online at: https://www.forestry.gov.cn/zlszz/4262/20211123/111212335990327.html (accessed November 15, 2021).
Stephenson, N. L., Das, A. J., Condit, R., Russo, S. E., Baker, P. J., Beckman, N. G., et al. (2014). Rate of tree carbon accumulation increases continuously with tree size. Nature 507, 90–93. doi: 10.1038/nature12914
Tang, J., Luyssaert, S., Richardson, A. D., Kutsch, W., and Janssens, I. A. (2014). Steeper declines in forest photosynthesis than respiration explain age-driven decreases in forest growth. Proc. Nat. Acad. Sci. 111, 8856–8860. doi: 10.1073/pnas.1320761111
Tang, X., Zhao, X., Bai, Y., Tang, Z., Wang, W., Zhao, Y., et al. (2018). Carbon pools in China's terrestrial ecosystems: new estimates based on an intensive field survey. Proc. Nat. Acad. Sci. 115, 4021–4026. doi: 10.1073/pnas.1700291115
Tao, F., Huang, Y., Hungate, B. A., Manzoni, S., Frey, S. D., Schmidt, M. W., et al. (2023). Microbial carbon use efficiency promotes global soil carbon storage. Nature 618, 981–985. doi: 10.1038/s41586-023-06042-3
Wang, H., Ding, Y., Zhang, Y., Wang, J., Freedman, Z. B., Liu, P., et al. (2023). Evenness of soil organic carbon chemical components changes with tree species richness, composition and functional diversity across forests in China. Glob. Chang. Biol. 29, 2852–2864. doi: 10.1111/gcb.16653
Wang, T., Dong, L., and Liu, Z. (2023). Climate strengthens the positive effects of stand structure on understory plant diversity in chinese temperate forests. Forests 11:2138. doi: 10.3390/f14112138
Wang, Y. -T., Tong, X. -J., Guan, C. -F., Shi, Q., Zhang, J. -S., Li, J., et al. (2023). Relationship between net primary productivity and stand age I typical plantation forests in China. Chin. J. Appl. Ecol. 34, 2017–2028. doi: 10.13287/j.1001-9332.202308.008
Wickham, H. (2016). ggplot2: Elegant Graphics For Data Analysis. Houston, TX: Springer. doi: 10.1007/978-3-319-24277-4
Xia, J., Xia, X., Chen, Y., Shen, R., Zhang, Z., Liang, B., et al. (2023). Reconstructing long-term forest age of China by combining forest inventories, satellite-based forest age and forest cover datasets. J. Geophys. Res. Biogeosci. 128:e2023JG007492. doi: 10.1029/2023JG007492
Xie, E., Zhang, X., Lu, F. Y., Peng, Y. X., Chan, J., and Zhao, Y. C. (2022). Integration of a process-based model into the digital soil mapping improves the space-time soil organic carbon modelling in intensively human-impacted area. Geoderma 409:115599. doi: 10.1016/j.geoderma.2021.115599
Xu, L., Shi, Y., Fang, H., Zhou, G., Xu, X., Zhou, Y., et al. (2018). Vegetation carbon stocks driven by canopy density and forest age in subtropical forest ecosystems. Sci. Total Environ. 631, 619–626. doi: 10.1016/j.scitotenv.2018.03.080
Xu, X., Liu, J., Jiao, F., Zhang, K., Ye, X., Gong, H., et al. (2023). Ecological engineering induced carbon sinks shifting from decreasing to increasing during 1981–2019 in China. Sci. Total Environ. 864:161037. doi: 10.1016/j.scitotenv.2022.161037
Yang, H., Ciais, P., Frappart, F., Li, X., Brandt, M., Fensholt, R., et al. (2023). Global increase in biomass carbon stock dominated by growth of northern young forests over past decade. Nat. Geosci. 16, 886–892. doi: 10.1038/s41561-023-01274-4
Yang, J., and Huang, X. (2021). The 30 m annual land cover dataset and its dynamics in China from 1990 to 2019. Earth Syst. Sci. Data 13, 3907–3925. doi: 10.5194/essd-13-3907-2021
Yu, G. R., Zhu, J. X., Xu, L., and He, N. P. (2022). Technological approaches to enhance ecosystem carbon sink in China: Nature-based solutions. Bull. Chin. Acad. Sci. 37, 490–501. doi: 10.16418/j.issn.1000-3045.20220121002
Yu, Z., Ciais, P., Piao, S. L., Houghton, R. A., Lu, C. Q., Tian, H. Q., et al. (2022). Forest expansion dominates China's land carbon sink since 1980. Nat. Commun. 13, 5374. doi: 10.1038/s41467-022-32961-2
Yu, Z., Liu, S. R., Wang, J. X., Wei, X. H., Schuler, J., Sun, P. S., et al. (2019). Natural forests exhibit higher carbon sequestration and lower water consumption than planted forests in China. Glob. Chang. Biol. 25, 68–77. doi: 10.1111/gcb.14484
Yu, Z., Wang, K., Li, J., Shangguan, Z., and Deng, L. (2023). Mixed plantations have more soil carbon sequestration benefits than pure plantations in China. For. Ecol. Manage. 529:120654. doi: 10.1016/j.foreco.2022.120654
Yu, Z., Zhou, G. Y., Liu, S. R., Sun, P. S., and Agathokleous, E. (2020). Impacts of forest management intensity on carbon accumulation of China's forest plantations. For. Ecol. Manage. 472:118252. doi: 10.1016/j.foreco.2020.118252
Yuan, Z., Ali, A., Wang, S., Gazol, A., Freckleton, R., Wang, X., et al. (2018). Abiotic and biotic determinants of coarse woody productivity in temperate mixed forests. Sci. Tot. Environ. 630, 422–431. doi: 10.1016/j.scitotenv.2018.02.125
Yue, J. W., and Li, H. Y. (2021). Difference analysis of carbon sequestration between natural and planted forests in China. J. Fujian Forest. Sci. Technol. 48, 1–7.
Zhang, D. N., Zuo, X. X., and Zang, C. F. (2021). Assessment of future potential carbon sequestration and water consumption in the construction area of the Three-North Shelterbelt Programme in China. Agric. For. Meteorol. 303:108377. doi: 10.1016/j.agrformet.2021.108377
Zhang, R., Tian, D., Wang, J., Pan, J., Zhu, J., Li, Y., et al. (2022). Dryness weakens the positive effects of plant and fungal β diversities on above-and belowground biomass. Glob. Chang. Biol. 28, 6629–6639. doi: 10.1111/gcb.16405
Zhang, X., Wang, Z., Chhin, S., Wang, H., Duan, A., and Zhang, J. (2020). Relative contributions of competition, stand structure, age, and climate factors to tree mortality of Chinese fir plantations: long-term spacing trials in southern China. For. Ecol. Manage. 465:118103. doi: 10.1016/j.foreco.2020.118103
Zhang, Y., Li, X. G., and Wen, Y. L. (2022). Forest carbon sequestration potential in China under the background of carbon emission peak and carbon neutralization. J. Beijing Forestry Univ. 44, 38–47. 10.12171/j.1000-1522.20210143
Zhang, Y., Liu, T., Guo, J., Tan, Z., Dong, W., and Wang, H. (2021). Changes in the understory diversity of secondary Pinus tabulaeformis forests are the result of stand density and soil properties. Glob. Ecol. Conserv. 28:e01628. doi: 10.1016/j.gecco.2021.e01628
Zhao, Y. C., Xie, E., Zhang, X., and Peng, Y. X. (2021). Global topsoil SOC stock from 1981 to 2018 estimated by combining process-based model and space-for-time digital soil mapping (VER 1.0) [Data set]. Zenodo.
Keywords: biomass carbon, soil organic carbon, carbon accumulation, natural forests, planted forests, China
Citation: Wang T, Dong L and Liu Z (2024) Factors driving carbon accumulation in forest biomass and soil organic carbon across natural forests and planted forests in China. Front. For. Glob. Change 6:1333868. doi: 10.3389/ffgc.2023.1333868
Received: 06 November 2023; Accepted: 08 December 2023;
Published: 08 January 2024.
Edited by:
Yashwant Singh Rawat, Federal Technical and Vocational Education and Training Institute (FTVETI), EthiopiaReviewed by:
Xiongqing Zhang, Chinese Academy of Forestry, ChinaLydie-Stella Koutika, CRDPI, Republic of Congo
Copyright © 2024 Wang, Dong and Liu. This is an open-access article distributed under the terms of the Creative Commons Attribution License (CC BY). The use, distribution or reproduction in other forums is permitted, provided the original author(s) and the copyright owner(s) are credited and that the original publication in this journal is cited, in accordance with accepted academic practice. No use, distribution or reproduction is permitted which does not comply with these terms.
*Correspondence: Zhaogang Liu, bHpnMTk3MDA2MDJAMTYzLmNvbQ==