- 1Department of Animal Resources Technology, Gyeongnam National University of Science and Technology, Jinju, South Korea
- 2Department of Poultry Science, University of Arkansas, Fayetteville, AR, United States
- 3Cell and Molecular Biology Program, University of Arkansas, Fayetteville, AR, United States
- 4Department of Animal Science and Technology, Sunchon National University, Suncheon, South Korea
- 5Department of Biological Sciences, Silla University, Busan, South Korea
The intestinal microbial communities play critical roles in various aspects of body function of the host. Prebiotics, such as dietary fiber, can affect health of the host by altering the composition of intestinal microbiota. Although brown seaweed Laminaria japonica is rich in dietary fiber, studies on its prebiotic potential are quite rare. In this study, basal diet (control), basal diet supplemented with dried L. japonica (DLJ), heat-treated dried L. japonica (HLJ), or heated dried L. japonica with added fructooligosaccharide (FHLJ) was fed to rats for 16 weeks. Serum concentrations of IgG, triglyceride, and cholesterol were measured. In addition, the intestinal microbiota composition was analyzed by high-throughput sequencing of 16S rRNA gene. As compared to the control group, DLJ, HLJ, and FHLJ groups showed significantly higher serum IgG concentration, but had lower weight gain and serum triglyceride concentration. Moreover, DLJ, HLJ, and FHLJ groups showed lower Fimicutes to Bacteroidetes ratio when compared with the control group. As compared with the control group, obesity-associated bacterial genera (Allobaculum, Turicibacter, Coprobacillus, Mollicute, and Oscilibacter), and the genera with pathogenic potentials (Mollicute, Bacteroides, Clostridium, Escherichia, and Prevotella) decreased while leanness-associated genera (Alistipes, Bacteroides, and Prevotella), and lactic acid bacterial genera (Subdoligranulum, Streptococcus, Lactobacillus, Enterococcus, and Bifidobacterium) increased in all treatment groups. On the contrary, butyric acid producing genera including Subdoligranulum, Roseburia, Eubacterium, Butyrivibrio, and Anaerotruncus increased significantly only in FHLJ group. The overall results support multiple prebiotic effects of seaweed L. japonica on rats as determined by body weight reduction, enhanced immune response, and desirable changes in intestinal microbiota composition, suggesting the great potential of L. japonica as an effective prebiotic for promotion of host metabolism and reduction of obesity in humans.
Introduction
Unlike cellulose contained in vegetables, carbohydrates in seaweeds have multiple beneficial effects to human bodies, including improving intestinal activities with their dietary fibers, discharging heavy metals in foods, and reducing blood lipid concentrations (1, 2). Brown seaweed Laminaria japonica contains water-soluble dietary fibers (32.8%) that are highly bioactive in human bodies, and non-water-soluble dietary fibers (17.9%). The total dietary fiber contents in L. japonica is therefore 50.7%, which is the highest among all plants and seaweeds (3). The intake of L. japonica that has such high dietary fiber contents can inhibit the growth of pathogenic bacteria and promote the growth of beneficial bacteria, suggesting a great prebiotic potential of L. japonica (4).
Prebiotics promote the growth of beneficial bacteria, such as lactic acid bacteria, and inhibit the growth of bacteria with pathogenic potentials in the large intestine. In particular, fructooligosaccharide (FOS) is a well-characterized prebiotics, which was shown to promote selective growth of bifidobacteria in the large intestine (5).
Bacteria in the gut microbiota play crucial roles in the overall body function of the host, including host metabolism. It was previously reported that the gut microbiota of the patients with metabolic syndromes such as obesity, arteriosclerosis, or type 2 diabetes are different from those of healthy individuals (6). In addition to the host metabolism, the gut microbiota are also functionally linked to the immune responses and immune systems of the host (7, 8). Multiple factors, including diet, age, and antibiotics, have been demonstrated to influence the maintenance and shaping of the gut microbiota, thereby health status of the host (9–11).
Our previous study conducted using rats reported an increase in beneficial intestinal microbes following the intake of seaweeds Undaria pinnatifida and L. japonica (4). We were interested in further exploring the prebiotic potential of L. japonica. Therefore, this study was conducted to investigate the effects of L. japonica on rats when supplemented in diet as dried (DLJ), heat-treated (HLJ), or heat-treated form with added FOS (FHLJ) on body weight, serum concentration of IgG, triglycerides, and cholesterol, and intestinal microbiota composition.
Materials and Methods
Animal Experiment
Dried L. japonica powder was purchased from Haeormbio Co., Ltd. (Busan, Korea). The heat-treated L. japonica was prepared by heating dried L. japonica powder for 30 min at 100°C. The FOS used in the experiment was also purchased (OGMayTech Co., Ltd., Seoul, Korea).
Forty eight 6-week-old male Sprague-Dawley rats were purchased from Samtako (Osan, Korea). The rats were subjected to a basal diet (Control), the basal diet mixed with 10% dried L. japonica powder (DLJ), the basal diet mixed with 10% heat-treated L. japonica (HLJ), or the basal diet mixed with 10% heat-treated L. japonica and 0.6% FOS (FHLJ). The compositions of the diets for the control and treatment groups are shown in Table S1 in Supplementary Material. A total of 12 rats were used in each treatment group, which were further divided into 4 repetitions of 3 rats per cage. After being purchased, the rats underwent a 1-week acclimatization period before being subjected to the aforementioned diets for 16 weeks. The weight and feed intake of each rat were measured once weekly throughout the experimental period using all animals (12 rats/group). The animal housing was maintained at the temperature of 22 ± 3°C with relative humidity of 65 ± 5%, and light and dark cycles of 12 h. The feed and drinking water were provided ad libitum. At the end of the experiment, the animals were sacrificed, and the ceca and blood were collected from each rat. Cecal samples (four rats per group) were processed and analyzed individually as shown below for microbiome analysis. Serum samples were separated from the blood (four rats per group) to measure the concentrations of serum IgG, cholesterol, and triglyceride as previously described (4). All animal handling procedures were approved by the Institutional Animal Care and Use Committees at Gyeongnam National University of Science and Technology.
DNA Extraction and PCR
The genomic DNA of intestinal contents was extracted using ZR Fecal DNA Mini Prep™ kit (Zymo Research, USA). The V1–V3 region of 16S rRNA gene was amplified using V1-9F (5′-CCTATCCCCTGTGTGCCTTGGCAGTCTCAGACGAGTTTGATCMTGGCTCAG-3′) and V3-541R (5′-CCATCTCATCCCTGCGTGTCTCCGACTCAG-barcode-ACWTTACCGCGGCTGCTGG-3′) as previously described (4). The amplified products were purified using QIAquick PCR purification kit (Qiagen, Valencia, CA, USA) which was followed by quantification using PicoGreen dsDNA quantitation assay kit (Invitrogen, Carlsbad, CA, USA). The amplicons of individual samples were combined together at equimolar concentration, which was then sequenced using a Roche/454 FLX system (ChunLab, Seoul, Korea).
Data Analysis
The pyrosequencing sequences of 16S rRNA gene were processed using Java-based multi-step bioinformatics pipeline. Unidirectional sequencing reads were identified with the help of unique barcodes in each individual read. Low-quality sequences (≤300 bp) were removed, and the trimmed reads were clustered at 97% sequence similarity level to pick operational taxonomic units [OTUs; (12)]. For taxonomic identification, representative sequences were selected from the OTUs, and their taxonomy were assigned based on the top five BLASTN hits in the EzTaxon-e database (13). The sequences that did not match in BLASTN searches in the EzTaxon-e database were classified as non-target sequences and were excluded from further analysis. The similarity between the query and candidate species was calculated using the Myers and Miller method (14). The cladogram was calculated using the TBC clustering algorithm (12). The read numbers in each sample were normalized by random subsampling. Overall analysis for phylogenetic differences was performed using the CL community program provided by ChunLab (Seoul, Korea). Statistical significance in relative abundance among different groups was calculated through variance analysis using General Linear Model procedure of the SAS (Ver. 9.1) and means comparison using Duncan’s multiple range tests at 5% significance level.
Results
Body Weight Gain and Feed Intake
The total weight gain was significantly lower in all three treatment groups (DLJ, HLJ, and FHLJ) when compared with the control group; however, no significant differences was observed among treatment groups as shown in Figure 1A. There was no significant difference in the total feed intake among all groups (Figure 1B), suggesting that the weight loss in treatment groups was not due to the reduced feed intake, but due to the consumption of L. japonica-supplemented diets. However, we cannot exclude the possibility that the less amount of energy and nutrient contents in the diets for the treatment groups (DLJ, HLJ, and FHLJ) when compared with the control group (Table S1 in Supplementary Material) could have contributed partially to the weight loss.
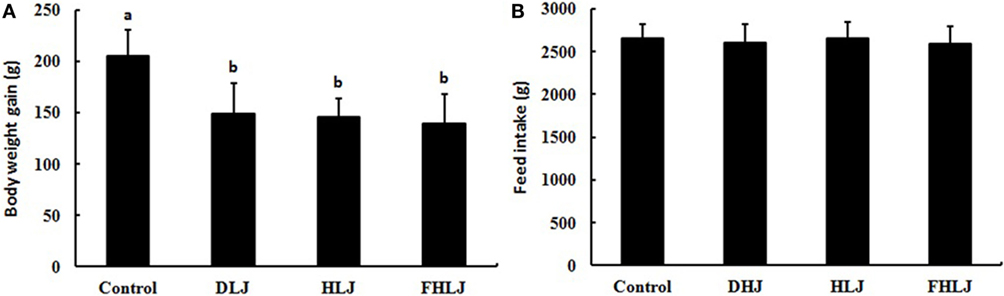
Figure 1. Effects of the consumption of the diets supplemented with Laminaria japonica and fructooligosaccharide (FOS) mixture for 16 weeks on (A) body weight gain and (B) feed intake of rats (n = 12 per group). Control: basal diet group, DLJ: basal diet + 10% dried L. japonica, HLJ: basal diet + 10% dried L. japonica heat-treated at 100°C for 30 min, FHLJ: HLJ + 0.6% FOS. Values are mean ± SD. No common superscripts on the bars indicate significant difference (p < 0.001).
Serum IgG and Triglyceride Concentration
As compared to the control group, serum IgG concentration was greater in both HLJ and FHLJ groups (Figure 2A). The serum concentration of high-density lipoprotein cholesterol and low-density lipoprotein cholesterol were not different across all treatment groups (data not shown). However, as compared to the control group, serum triglyceride concentration was lower significantly in all treatment groups (Figure 2B). Among the treatment groups, the HLJ group showed the lowest serum triglyceride concentration, although the differences were not significant. Thus, the supply of L. japonica, heat-treated L. japonica, and heat-treated L. japonica with added FOS increased serum IgG concentrations and reduced serum triglyceride concentrations in the experimental animals used in this study.
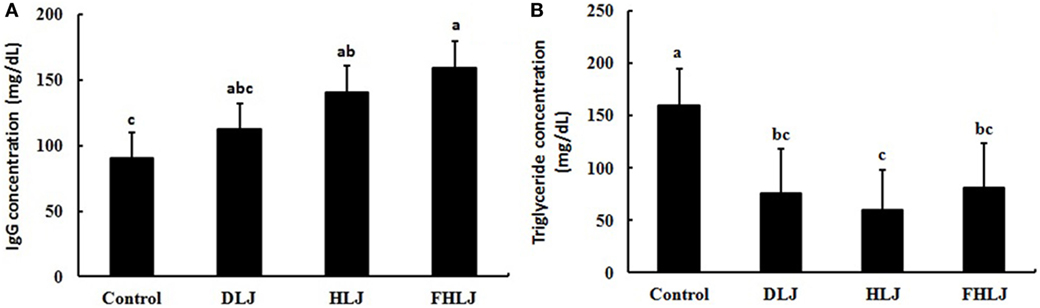
Figure 2. Effects of the consumption of the diets supplemented with Laminaria japonica and fructooligosaccharide (FOS) mixture for 16 weeks on (A) IgG and (B) triglyceride concentrations in the serum of rats (n = 4 per group). Control: basal diet group, DLJ: basal diet + 10% dried L. japonica, HLJ: basal diet + 10% dried L. japonica heat-treated at 100˚C for 30 min, FHLJ: HLJ + 0.6% FOS. Values are mean ± SD. No common superscripts on the bars indicate significant difference in IgG concentration at p < 0.05 and in triglyceride concentration at p < 0.01.
Analysis of Intestinal Microbiota
Using high-throughput pyrosequencing of 16S rRNA gene, the composition of intestinal microbiota was analyzed using four replicates per group. At phylum level, Firmicutes and Bacteroidetes were found as dominant phyla since they altogether accounted for at least 95% of total sequence reads in all groups (Figure 3A). The decrease in Firmicutes and increase in Bacteroidetes were observed in all treatment groups (DLJ, HLJ, and FHLJ) when compared with the control group (Figure 3A). Comparing the proportions of Firmicutes and Bacteroidetes by normalizing the combined reads of both phyla to 100%, Firmicutes was 80.0% in the control, 37.3% in DLJ, 47.7% in HLJ, and 54.9% in FHLJ group (p < 0.01). On the contrary, Bacteroidetes was 20.0% in the control, 62.7% in DLJ, 52.3% in HLJ, and 45.1% in FHLJ group (p < 0.01) (Figure 3B). Among the treatment groups, difference between Firmicutes and Bacteroidetes was greatest in DLJ group.
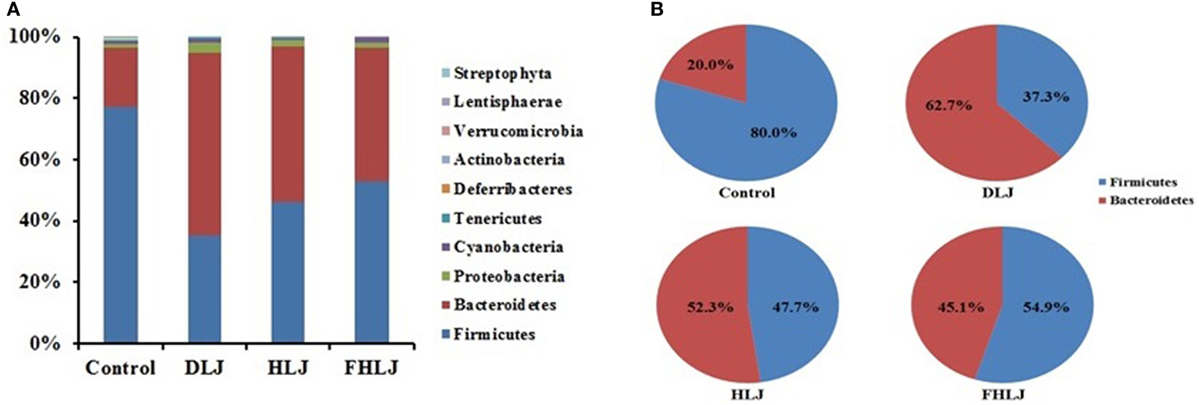
Figure 3. The relative abundance of different phyla in the cecal microbiota of rats (n = 4 per group). (A) The bar graph shows the relative abundance of 10 phyla, and (B) the pie graph shows only two dominant phyla, Firmicutes and Bacteriodetes, highlighting the changes in the ratio of the two phyla in DLJ, HLJ, and FHLJ in comparison to the control. Control: basal diet group, DLJ: basal diet + 10% dried L. japonica, HLJ: basal diet + 10% dried L. japonica heat-treated at 100°C for 30 min, FHLJ: HLJ + 0.6% fructooligosaccharide.
According to the functional characteristics of different genera, the microbiome data were further analyzed to determine the relative abundance of the following five functional bacterial groups at genus level: these functional groups included (i) the obesity-associated genera (11, 15), (ii) the leanness-associated genera (16, 17), (iii) the genera with pathogenic potentials (18–20), (iv) the genera belonging to lactic acid bacterial (LAB) (21, 22), and (v) the butyric acid producing genera (21, 23) (Figure 4). The relative abundance levels of these functional groups were indicated with the combined sequence read numbers of the OTUs corresponding to the genera that belong to each functional group (Figure 4). We reasoned that changes in the abundance of these functional groups might give better insights for correlating the changes in microbiota and other measurements, particularly body weight and serum triglyceride concentration when compared with the abundance changes in conventional taxonomic groups.
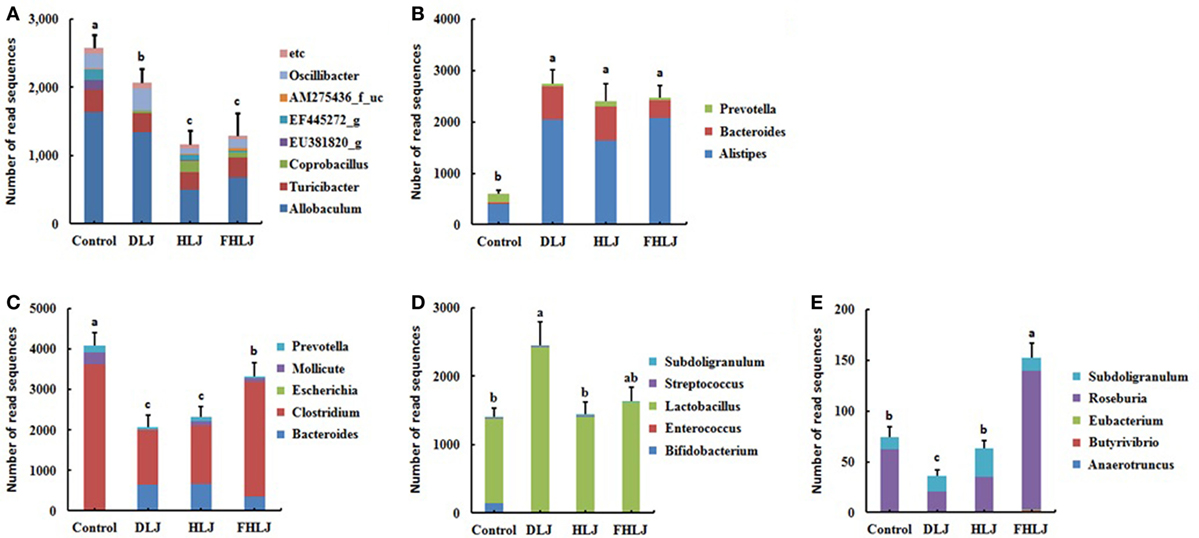
Figure 4. The relative abundance of the functional bacterial groups in the cecal microbiota of rats (n = 4 per group). (A) Obesity-associated genera, (B) leanness-associated genera, (C) genera with pathogenic potentials, (D) genera that belong to lactic acid bacteria, and (E) butyric acid producing genera. Control: basal diet group, DLJ: basal diet + 10% dried Laminaria japonica, HLJ: basal diet + 10% dried L. japonica heat-treated at 100˚C for 30 min, FHLJ: HLJ + 0.6% fructooligosaccharide. Values are mean ± SD of the numbers of sequence reads of all OTUs belonging to each functional group. No common superscripts on the bars indicate significant difference in the relative abundance of (A) obesity-related genera, (D) genera that belong to lactic acid bacteria, and (E) butyric acid producing genera at p < 0.05, and (B) leanness-associated genera and (C) genera with pathogenic potentials at p < 0.01.
The obesity-associated genera including Allobaculum, Turicibacter, and Oscillibater were found in all groups, and they were significantly decreased in all treatment groups (DLJ, HLJ, and FHLJ) when compared with the control group (Figure 4A). Interestingly, even among the treatment groups, the level of the obesity-associated genera was significantly lower in HLJ and FHLJ as compared to DLJ (Figure 4A). Among the leanness-associated genera including Alistipes, Bacteroides, and Prevotella, Alistipes was dominant in all groups (Figure 4B). As compared to the control group, all treatment groups showed significant increase in the abundance of these genera. Particularly, Alistipes increased at least four-folds and Bacteroides increased at least 10 times in all treatment groups when compared with the control group (Figure 4B). The genera with pathogenic potentials, which includes Bacteroides, Clostridium, Escherichia, Mollicute, and Prevotella, were found in all groups except HLJ in which Escherichia was absent (Figure 4C). Within this functional group, Clostridium was the dominant genus that accounted for 60–87% sequence reads in all groups. The relative abundance of the genera with pathogenic potentials was significantly lower in all treatment groups (DLJ, HLJ, and FHLJ) when compared with the control group. The abundance of this functional group was even lower significantly in DLJ and HLJ groups when compared with FHLJ group (p < 0.01).
When it comes to the genera that belong to LAB, Bifidobacterium, Enterococcus, Lactobacillus, Streptococcus, and Subdoligranulum were found in all groups, except that Enterococcus was not found in DLJ and FHLJ groups (Figure 4D). Among LAB, Lactobacillus was the dominant genus in all groups, which was 87% in the control group, and at least 97% in all treatment groups (DLJ, HLJ, and FHLJ). This functional group increased significantly in DLJ group (p < 0.05), while the increase was not significant in HLJ and FHLJ when compared with the control group.
For the butyric acid producing genera, there were dramatic differences across different treatment groups. Anaerotruncus, Roseburia, and Subdoligranulum were found in the control group. In the treatment groups, Roseburia and Subdoligranulum were found in all treatment groups, while Butyrivibrio and Eubacterium were additionally found in FHLJ group (Figure 4E). As compared to the control group, total butyric acid producing genera were significantly higher in FHLJ group (p < 0.01).
To gain more insights on the role of microbiota in mediating the effects of the seaweed supplementation on body weights (Figure 1A), serum IgG concentrations (Figure 2A), and serum triglyceride concentrations (Figure 2B), we further examined the microbiome data to understand the dynamic changes in the microbiota at species level. We found the three species, such as FJ880918_s, Alistipes_us, and Bacteroides eggerthii, exhibited most dramatic changes in response to the treatments.
Species FJ880918_s that belong to genus Allobaculum, which was one of the obesity-associated genera shown in Figure 4A, decreased significantly (p < 0.01) in the DLJ, HLJ, and FHLJ groups (by 27.5, 8.9, and 17.2%, respectively) when compared with the control group. This species FJ880918_s showed the highest decrease as a single species by the treatments (Figure 5A). The species Alistipes_us belongs to genus Alistipes, which was a dominant member of the leanness-associated genera as shown in Figure 4B. The relative abundance of Alistipes_us was at least 93% within the genus Alistipes for all groups, and was 5.37-, 4.23-, and 5.48-fold greater in DLJ, HLJ, and FHLJ groups, respectively, showing significant increase, when compared with the control group (p < 0.05; Figure 5B). The species Bacteroides eggerthii increased in DLJ, HLJ, and FHLJ groups significantly (by 74.6-, 88.6-, and 42.8-folds, respectively) when compared with the control group (p < 0.01; Figure 5C).
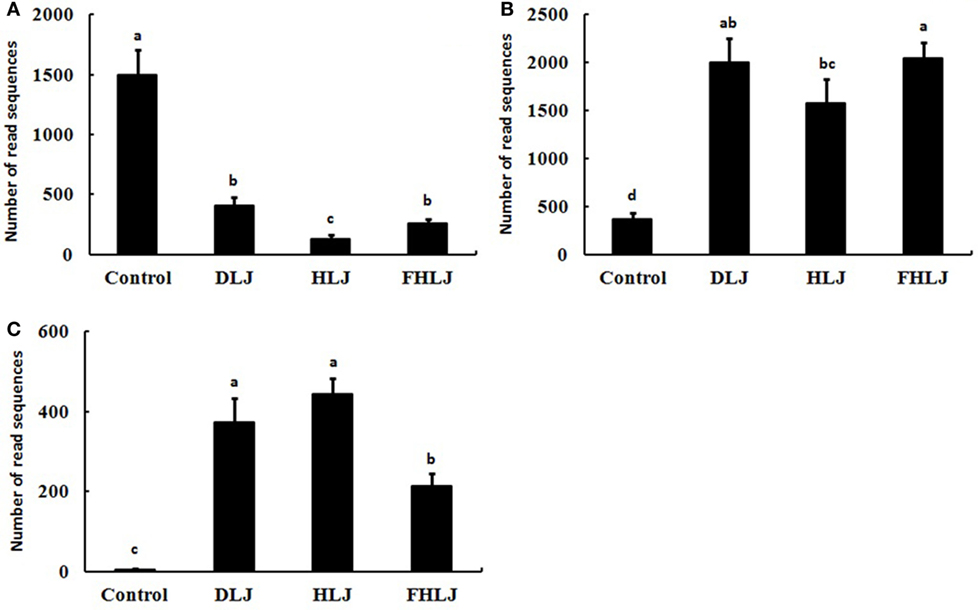
Figure 5. The relative abundance of the bacterial species of interest in the cecal microbiota of rats (n = 4 per group). Sequence read numbers of (A) FJ880918_s, (B) Alistipes_us, and (C) Bacteroides eggerthii. Control: basal diet group, DLJ: basal diet + 10% dried Laminaria japonica, HLJ: basal diet + 10% dried L. japonica heat-treated at 100°C for 30 min, FHLJ: HLJ + 0.6% fructooligosaccharide. Values are mean ± SD of the numbers of sequence reads of all OTUs belonging to each species. No common superscripts on the bars indicate significant difference in (B) Alistipes us at p < 0.05, and (A) FJ880918_s and (C) Bacteroides eggerthii at p < 0.01.
Discussion
Seaweeds are a source of abundant dietary fibers, and L. japonica contains the highest dietary fibers among all seaweeds and vegetables (3). Dietary fibers cannot be digested by the digestive enzymes in the stomach and the small intestine, and thus bypass to the large intestine where they are fermented by anaerobic microorganisms (24). Therefore, L. japonica has a great potential to be used for effective prevention and treatment of metabolic syndrome caused by high-calorie intakes. Although the efficacy of L. japonica has been reported in previous researches (4), limited information is available on the effect of this seaweed on the composition and structure of intestinal microbiota. Our previous study reported reduced weight gain, and proliferations of the leanness-associated bacterial genera as well as other beneficial intestinal bacteria in rats fed with a diet supplemented with U. pinnatifida and L. japonica (4). This study was aimed at investigating the dietary effects of L. japonica in different forms and variations (DLJ, HLJ, and FHLJ) on intestinal microbiota using rats as an animal model. It has been well established that fiber intake is inversely associated with body weight (25). Dietary fibers produce short-chain fatty acids (SCFAs) through fermentation by anaerobic microorganisms in the large intestine (26). Among the SCFAs, butyric acid is used as energy source by intestinal epithelial cells and the remaining SCFAs are absorbed into bloodstream to promote lipolysis by fat cells, control gut hormones, and inhibit fat accumulation by insulin (27–29). In this study, we demonstrated that the supplementation of L. japonica (DLJ and HLJ) and FOS-added Laminaria japonica (FHLJ) significantly reduced weight gain and serum triglyceride concentrations in rats without affecting feed intakes (Figures 1A and 2B).
Prebiotics have several beneficial effects, such as preventing allergic diseases, regulating immunity, and decreasing the risks of cancers (30–32). The intake of red seaweed Chondrus crispus increased blood IgA and IgG concentrations in weaning rats (33). We also reported increase in serum IgG concentrations in all treatment groups (DLJ, HDJ, and FHDJ), indicating that L. japonica can enhance immune responses in rats (Figure 2A).
In previous studies, when the intestinal microorganisms of an obese mouse was transplanted into a germ-free mouse, the fat accumulation in germ-free mouse remarkably increased, suggesting intestinal microbes are important players in modulation of obesity (11). Firmicutes and Bacteroidetes are two major phyla that can affect obesity. Increased level of Firmicutes was associated with obesity, while Bacteroidetes was shown to promotes leanness (34). In our study, Firmicutes and Bacteroidetes were dominant phyla in all groups, and Firmicutes decreased while Bacteroidetes increased when rats were fed with L. japonica-supplemented diets (Figure 3). The intake of L. japonica (DLJ and HLJ) and FOS-added L. japonica (FHLJ) reduced the obesity-associated genera. Allobaculum, Turicibacter, and Coprobacillus are the genera that belong to the class Erysipelotrichi which was found to increase in obese body types (15). EU381820_g, EF445272_g, and AM275436_f_uc are the genera that fall under the class Mollicutes. Mollicutes and Oscillibacter were shown to increase in obese mouse fed with high-fat diets, which then caused mild inflammations to affect the insulin pathway leading to fat accumulation (11, 35). L. japonica (DLJ and HLJ) and FOS-added L. japonica (FHLJ) reduced the relative abundance of obesity-associated genera in rat intestine (Figure 4A). FJ880918s that falls under the genus Allobaculum showed the highest decrease as a single species, suggesting the need for further investigation on this species in future researches (Figure 5A).
Higher abundance of Prevotella and Bacteroides were reported in African children, who mainly eat typical vegetarian diet when compared with European children, who mainly eat high-fat high-calorie diet (16). Both genera contain microbes that can hydrolyze cellulose and xylan. Mice with higher intestinal abundance of Prevotella showed reduction in fat accumulation (36). Alistipese that belong to the phylum Bacteroidetes was also found to increase in rat with reduced weight gains (37). In this study, the increase in Alistipes_uc and Bacteroides eggerthii are reported in accordance with the increase in the genera Alistipes and Bacteroides (Figures 5B,C). Further research is necessary to investigate the interactions between these two species and the host. As compared to the control group, leanness-associated bacterial genera increased in the treatment group supplied with L. japonica (DLJ, HLJ, and FHLJ; Figure 4B). Intestinal pathogenic microbes affect immune responses and metabolic status in the hosts. Intestinal pathogenic microbes can also cause mild-grade inflammations. Mild-grade inflammations that appear as immune reactions affect metabolic signaling pathways to promote obesity (38, 39). High-fat diets increase intestinal pathogenic bacteria that induce the expression of cytokines, which are inflammatory substances, and the permeability of the intestine (40). Endotoxins secreted by pathogenic bacteria can cause inflammations due to the activity of macrophages (41). These inflammations increase the expression of TNF-α and NF-κB, and affect the secretion of metabolic hormones, such as insulin, adiponectin, leptin, and resistin, promoting obesity (38, 42). In our study, all treatment groups supplemented with L. japonica (DLJ, HLJ, and FHLJ) showed significant decrease in the genera with pathogenic potentials (Figure 4C).
Prebiotics promote the proliferation of intestinal LAB. LAB can inhibit the growth of pathogenic microbes by lowering pH and secreting bacteriocins, as well as can affect immune modulation by increasing concentrations of IgA and γ-interferon in blood (43). It was previously reported that Bifidobacterium and Lactobacillus in the intestinal microbiota and IgA and IgG increased in rats supplied with red seaweeds (33). Bifidobacterium and Lactobacillus were also shown to reduce blood cholesterol and lipid components (44). The LAB that have diverse physiological functions increased in all treatment groups supplied with L. japonica (DLJ, HLJ, and FHLJ), although the increase was significant only in DLJ when compared with the control group (Figure 4D).
Dietary fibers are fermented by anaerobic microorganisms in the large intestine to produce SCFAs. Butyric acid inhibits insulin’s fat accumulation signaling through SCFA receptor GPR43, relieves inflammations, and reduces liver fat, cholesterol, and triglycerides concentrations (29, 45). In addition, butyric acid generates glucagon-like peptide 1 to stimulate satiety (46). In our study, butyric acid producing bacteria increased only in FHLJ group (Figure 4E).
In summary, the supply of dried L. japonica (DLJ), heat-treated L. japonica (HLJ), and FOS-added heat-treated L. japonica (FHLJ) was found to reduce obesity by lowering weight gain and affect immune modulation by increasing serum concentration of IgG. In addition, they were found to reduce the ratio of Fimicutes to Bacteroidetes, and the genera with pathogenic potentials, while increasing leanness-associated bacterial genera and LAB. Overall, the effects of dried L. japonica, heat-treated L. japonica, and FOS-added heat-treated L. japonica on intestinal microbiota composition were not significantly different from each other. Therefore, the results of this animal study support the great potential of brown seaweed L. japonica per se (without heat treatment or FOS addition) as an effective functional food in humans with beneficial prebiotic effects.
It is important to note that the less amount of energy and nutrient contents in the diets for the treatment groups (DLJ, HLJ, and FHLJ) in comparison with the control group could have contributed partially to the modulation of cecal microbiomes. In the future studies, an improved experimental design employing appropriate inert filler in place of 10% L. japonica will be desirable to attribute the microbiome changes completely to the prebiotic effects of different forms of L. japonica.
Ethics Statement
All procedures were approved by the Institutional Animal Care and Use Committees at Gyeongnam National University of Science and Technology (No. 2015-10).
Author Contributions
J-YK drafted the manuscript and designed the experiments; YMK jointly led the study and revised the manuscript; I-SK, J-AK, and D-YY performed the experiments; BA revised the manuscript; S-SL, I-SC, and K-KC collected and analyzed the data. All the authors read and approved the final manuscript.
Conflict of Interest Statement
The authors declare that the research was conducted in the absence of any commercial or financial relationships that could be construed as a potential conflict of interest.
Funding
This research was supported by the Priority Research Centers Program through the National Research Foundation of Korea (NRF) funded by the Ministry of Education (2009-0093813).
Supplementary Material
The Supplementary Material for this article can be found online at https://www.frontiersin.org/articles/10.3389/fnut.2018.00023/full#supplementary-material.
References
1. Kim HJ, Kim SI, Han YS. Effects of sea tangle extract and sea tangle yogurt on constipation relief. Korean J Food Cookery Sci (2008) 24(1):59–67.
2. Hwang HJ, Kwak YS, Kim HS, Choi YH, Kim BW, Kwon HJ, et al. The effect of sea tangle supplementation and exercise training on blood glucose and lipid profile in rats. J Life Sci (2010) 2:805–10.
3. Do JR, Kim EM, Koo JG, Jo KS. Dietary fiber contents of marine algae and extraction condition of the fiber. J Korean Fish Soc (1997) 30:291–6.
4. Kim JY, Yu DY, Kim JA, Choi EY, Lee CY, Hong YH, et al. Effects of Undaria linnatifida and Laminaria japonica on rat’s intestinal microbiota and metabolite. J Nutr Food Sci (2016) 6:3. doi:10.4172/2155-9600.1000502
5. Gibson GR, Roberfroid MB. Dietary modulation of the human colonic microbiota: introducing the concept of prebiotics. J Nutr (1995) 125:1401–12.
6. Karlsson F, Tremaroli V, Nielsen J, Backhed F. Assessing the human gut microbiota in metabolic diseases. Diabetes (2013) 62:3341–9. doi:10.2337/db13-0844
7. Steimle A, Gronbach K, Beifuss B, Schafer A, Harmening R, Bender A, et al. Symbiotic gut commensal bacteria act as host cathepsin S activity regulators. J. Autoimmun (2016) 75:82–95. doi:10.1016/j.jaut2016.07.009
8. Kelly JR, Borre Y, O’ Brien C, Patterson E, El Aidy S, Deane J, et al. Transferring the blues: depression-associated gut microbiota induces neurobehavioural changes in the rat. J Psychiatr Res (2016) 82:109–18. doi:10.1016/j.jpsychires.2016.07.019
9. Jernberg C, Sullivan A, Edlund C, Jansson JK. Monitoring of antibiotic-induced alterations in the human intestinal microflora and detection of probiotic strains by use of terminal restriction fragment length polymorphism. Appl Environ Microbiol (2005) 71:501–56. doi:10.1128/AEM.71.1.501-506.2005
10. Mariat D, Firmess O, Levenez F, Guimaraes V, Sokol H, Dore J, et al. The Firmicutes/Bacteroidetes ratio of the human microbiota changes with age. BMC Microbiol (2009) 9:123. doi:10.1186/1471-2180-9-123
11. Turnbaugh PJ, Backhed F, Fulton L, Gordon JI. Diet-induced obesity is linked to marked but reversible alterations in the mouse distal gut microbiome. Cell Host Microbe (2008) 3:213–23. doi:10.1016/j.chom.2008.02.015
12. Lee JH, Yi H, Jeon YS, Won S, Chun J. TBC: a clustering algorithm based on prokaryotic taxonomy. J Microbiol (2012) 50:181–5. doi:10.1007/s12275-012-1214-6
13. Kim OS, Cho YJ, Lee K, Yoon SH, Kim M, Na H, et al. Introducing EzTaxon-e: a prokaryotic 16S rRNA gene sequence database with phylotypes that represent uncultured species. Int J Syst Evol Microbiol (2012) 62:716–21. doi:10.1099/ijs.0.038075-0
15. Greiner T, Backhed F. Effects of the gut microbiota on obesity and glucose homeostasis. Trends Endocrinol Metab (2011) 22:117–23. doi:10.1016/j.tem.2011.01.002
16. De Filippo C, Cavalieri D, Di Paola M, Ramazzotti M, Poullet JB, Massart S, et al. Impact of diet in shaping gut microbiota revealed by a comparative study in children from Europe and rural Africa. Proc Natl Acad Sci U S A (2010) 107:14691–6. doi:10.1073/pnas.1005963107
17. Song Y, Kononen E, Rautio M, Liu C, Bryk A, Eerola E, et al. Alistipes onderdonkii sp. nov. and Alistipes shahii sp. nov., of human origin. Int J Syst Evol Microbiol (2006) 56:1985–90. doi:10.1099/ijs.0.64318-0
18. Calhoun LN, Liyanage R, Lay JO Jr, Kwon YM. Proteomic analysis of Salmonella enterica serovar Enteritidis following propionate adaptation. BMC Microbiol (2010) 10:249. doi:10.1186/1471-2180-10-249
19. Wang H, Kong F, Jelfs P, James G, Gilbert GL. Simultaneous detection and identification of common cell culture contaminant and pathogenic mollicutes strains by reverse line blot hybridization. Appl Environ Microbiol (2004) 70:1483–6. doi:10.1128/AEM.70.3.1483-1486.2004
20. Falagas ME, Siakavellas E. Bacteroides, Prevotella, and Porphyromonas species: a review of antibiotic resistance and therapeutic options. Int J Antimicrob Agents (2000) 15:1–9. doi:10.1016/S0924-8579(99)00164-8
21. Louis P, Flint HJ. Diversity, metabolism and microbial ecology of butyrate-producing bacteria from the human large intestine. FEMS Microbiol Lett (2009) 294:1–8. doi:10.1111/j.1574-6968.2009.01514.x
22. Antharam VC, Li EC, Ishmael A, Sharma A, Mai V, Rand KH, et al. Intestinal dysbiosis and depletion of butyrogenic bacteria in Clostridium difficile infection and nosocomial diarrhea. J Clin Microbiol (2013) 51:2884–92. doi:10.1128/JCM.00845-13
23. De Vuyst L, Leroy F. Cross-feeding between bifidobacteria and butyrate-producing colon bacteria explains bifdobacterial competitiveness, butyrate production, and gas production. Int J Food Microbiol (2011) 149:73–80. doi:10.1016/j.ijfoodmicro.2011.03.003
24. Hadwiger LA, Fristensky B, Riggleman RC. In: Zikakis JP, editor. Chitosan, a natural regulator in plant-fungal pathogen interactions, increases crop yield. Chitin, Chitosan and Related Enzymes. New York: Academic Press (1984). p. 291–302.
25. Slavin JL. Dietary fiber and body weight. Nutrition (2005) 21:411–8. doi:10.1016/j.nut.2004.08.018
26. Yang J, Martinez I, Walter J, Keshavarzian A, Rose DJ. In vitro characterization the impact of selected dietary fibers on fecal microbiota composition and short chain fatty acid production. Anaerobe (2013) 23:74–81. doi:10.1016/j.anaerobe.2013.06.012
27. Rumberger JM, Arch JR, Green A. Butyrate and other short-chain fatty acids increase the rate of lipolysis in 3T3-L1 adipocytes. PeerJ (2014) 7:e611. doi:10.7717/peerj.611
28. Lin HV, Frassetto A, Kowalik EJ Jr, Nawrocki AR, Lu MM, Kosinski JR, et al. Butyrate and propionate protect against diet-induced obesity and regulate gut hormones via free fatty acid receptor 3-independent mechanisms. PLoS One (2012) 7:e35240. doi:10.1371/journal.pone.0035240
29. Kimura I, Ozawa K, Inoue D, Imamura T, Kimura K, Maeda T, et al. The gut microbiota suppresses insulin-mediated fat accumulation via the short-chain fatty acid receptor GPR43. Nat Commun (2013) 4:1829. doi:10.1038/ncomms2852
30. Tuohy KM, Probert HM, Smejkal CW, Gibson GR. Using probiotics and prebiotics to improve gut health. Drug Discov Today (2003) 8:692–700. doi:10.1016/S1359-6446(03)02746-6
31. Spiller R. Review article: probiotics and prebiotics in irritable bowel syndrome. Aliment Pharmacol Ther (2008) 28:385–96. doi:10.1111/j.1365-2036.2008.03750.x
32. Davis CD, Milner JA. Gastrointestinal microflora, food components and colon cancer prevention. J Nutr Biochem (2009) 20:743–52. doi:10.1016/j.jnutbio.2009.06.001
33. Liu J, Kandasamy S, Zhang J, Kirby CW, Karakach T, Hafting J, et al. Prebiotic effects of diet supplemented with the cultivated red seaweed Chondrus crispus or with fructo-oligo-saccharide on host immunity, colonic microbiota and gut microbial metabolites. BMC Complement Altern Med (2015) 14:279. doi:10.1186/s12906-015-0802-5
34. Schwiertz A, Taras D, Schafer K, Beijer S, Bos NA, Donus C, et al. Microbiota and SCFA in lean and overweight healthy subjects. Obesity (2010) 18:190–5. doi:10.1038/oby.2009.167
35. Sinh P, Karimi A, Devendra K, Waldroup PW, Cho KK, Kwon YM. Influence of penicillin on microbial diversity of the cecal microbiota in broiler chickens. Poult Sci (2003) 92:272–6. doi:10.3382/ps.2012-02603
36. Neyrinck AM, Possemiers S, Druart C, Van de Wiele T, De Backer F, Cani PD, et al. Prebiotic effects of wheat arabinoxylan related to the increase in bifidobacteria, roseburia and Bacteroides/Prevotella in diet-induced obese mice. PLoS One (2011) 6:e20944. doi:10.1371/journal.pone.0020944
37. Kim JY, Choi EY, Hong YH, Song YO, Han JS, Lee SS, et al. Changes in Korean adult females’ intestinal microbiota resulting from kimchi intake. J Nutr Food Sci (2016) 6:486. doi:10.4172/2155-9600.1000486
38. Lam YY, Ha CW, Campbell CR, Mitchell AJ, Dinudom A, Oscarsson J, et al. Increased gut permeability and microbiota change associate with mesenteric fat inflammation and metabolic dysfunction in diet-induced obese mice. PLoS One (2012) 7:e34233. doi:10.1371/journal.pone.0034233
39. Blaut M, Klaus S. Intestinal microbiota and obesity. Handb Exp Pharmacol (2012) 209:251–73. doi:10.1007/978-3-642-24716-3_11
40. Kim KA, Gu W, Lee IA, Joh EH, Kim DH. High fat diet-induced gut microbiota exacerbates inflammation and obesity in mice via the TLR4 signaling pathway. PLoS One (2012) 7:e47713. doi:10.1371/journal.pone.0047713
41. Choi E, Jin JY, Lee JY, Choi JI, Choi IS, Kim SJ. Anti-inflammatory effects and the underlying mechanisms of action of daidzein in murine macrophages stimulated with Prevotella intermedia lipopolysaccharide. J Periodontal Res (2012) 47:204–11. doi:10.1111/j.1600-0765.2011.01422.x
42. Ding S, Chi MM, Scull BP, Rigby R, Schwerbrock NM, Magness S, et al. High-fat diet: bacteria interactions promote intestinal inflammation which precedes and correlates with obesity and insulin resistance in mouse. PLoS One (2010) 5:e12191. doi:10.1371/journal.pone.0012191
43. Settanni L, Corsetti A. Application of bacteriocins in vegetable food biopreservation. Int J Food Microbiol (2008) 121:123–38. doi:10.1016/j.ijfoodmicro.2007.09.001
44. Park YH, Kim JG, Shin YW, Kim HS, Kim YJ, Chun T, et al. Effects of Lactobacillus acidophilus 43121 and a mixture of Lactobacillus casei and Bifidobacterium longum on the serum cholesterol level and fecal sterol excretion in hypercholesterolemia-induced pigs. Biosci Biotechnol Biochem (2008) 72:595–600. doi:10.1271/bbb.70581
45. Jakobsdottir G, Xu J, Molin G, Ahrne S, Nyman M. High-fat diet reduces the formation of butyrate, but increases succinate, inflammation, liver fat and cholesterol in rats, while dietary fibre counteracts these effects. PLoS One (2013) 8:e80476. doi:10.1371/journal.pone.0080476
Keywords: brown seaweed, experimental model, intestinal microbiota, Laminaria japonica, pyrosequencing
Citation: Kim J-Y, Kwon YM, Kim I-S, Kim J-A, Yu D-Y, Adhikari B, Lee S-S, Choi I-S and Cho K-K (2018) Effects of the Brown Seaweed Laminaria japonica Supplementation on Serum Concentrations of IgG, Triglycerides, and Cholesterol, and Intestinal Microbiota Composition in Rats. Front. Nutr. 5:23. doi: 10.3389/fnut.2018.00023
Received: 11 September 2017; Accepted: 22 March 2018;
Published: 12 April 2018
Edited by:
Michael Gänzle, University of Alberta, CanadaCopyright: © 2018 Kim, Kwon, Kim, Kim, Yu, Adhikari, Lee, Choi and Cho. This is an open-access article distributed under the terms of the Creative Commons Attribution License (CC BY). The use, distribution or reproduction in other forums is permitted, provided the original author(s) and the copyright owner are credited and that the original publication in this journal is cited, in accordance with accepted academic practice. No use, distribution or reproduction is permitted which does not comply with these terms.
*Correspondence: Kwang-Keun Cho, Y2hvdHdvMkBnbnRlY2guYWMua3I=