- 1Interdisciplinary Stem Cell Institute, University of Miami Miller School of Medicine, Miami, FL, United States
- 2Katz Family Division of Nephrology and Hypertension, University of Miami Miller School of Medicine, Miami, FL, United States
Chronic diseases and degenerative conditions are strongly linked with the geriatric syndrome of frailty and account for a disproportionate percentage of the health care budget. Frailty increases the risk of falls, hospitalization, institutionalization, disability, and death. By definition, frailty syndrome is characterized by declines in lean body mass, strength, endurance, balance, gait speed, activity and energy levels, and organ physiologic reserve. Collectively, these changes lead to the loss of homeostasis and capability to withstand stressors and resulting vulnerabilities. There is a strong link between frailty, inflammation, and the impaired ability to repair tissue injury due to decreases in endogenous stem cell production. Although exercise and nutritional supplementation provide benefit to frail patients, there are currently no specific therapies for frailty. Bone marrow-derived allogeneic mesenchymal stem cells (MSCs) provide therapeutic benefits in heart failure patients irrespective of age. MSCs contribute to cellular repair and tissue regeneration through their multilineage differentiation capacity, immunomodulatory, and anti-inflammatory effects, homing and migratory capacity to injury sites, and stimulatory effect on endogenous tissue progenitors. The advantages of using MSCs as a therapeutic strategy include standardization of isolation and culture expansion techniques and safety in allogeneic transplantation. Based on this evidence, we performed a randomized, double-blinded, dose-finding study in elderly, frail individuals and showed that intravenously delivered allogeneic MSCs are safe and produce significant improvements in physical performance measures and inflammatory biomarkers. We thus propose that frailty can be treated and the link between frailty and chronic inflammation offers a potential therapeutic target, addressable by cell therapy.
Definition and Epidemiology of Frailty
Frailty has been clinically defined as “a state of increased vulnerability resulting from aging-associated decline in reserve and function across multiple organ systems such that the ability to cope with everyday or acute stressors is compromised” (1). Central to this geriatric medical syndrome is the notion that it has multiple causes and contributors that lead to the characteristic decreases in strength, endurance, activity, energy levels, and physiologic function, which increase the susceptibility to dependency and death (2, 3). Of note, although frailty is not characterized as a disability, it does increase the risk of disability in affected individuals (3–5). Moreover, there is a close link between a patient's health and frailty (6, 7). These patients tend to show a greater risk of frailty when there are other comorbidities affecting their physical and psychological well-being, such as cardiovascular disease, diabetes, high blood pressure, cancer, or cognitive impairment (8). The main clinical presentations of frailty are falls, which are a result of impaired balance, gait, and awareness, fluctuating disability with independent and dependent days, and other non-specific signs and symptoms, such as unexplained weight loss, infections, and extreme fatigue.
Several instruments have been developed to assess frailty. These can be categorized as the unidimensional or phenotypic model, based on the physical or biological dimension, and multidimensional or cumulative deficit models, centered on the links between the physical, psychological, and social realms, all of which have been well validated (1, 8). The unidimensional or phenotypic model was first operationally defined by Fried et al. (9) and was used to develop the Cardiovascular Health Study (CHS) Index, commonly referred to as the “Physical Frailty Phenotype” (10). Using this model, frailty is defined as having three out of five phenotypic criteria indicating “compromised energetics”: weak grip strength, low energy levels or self-reported exhaustion, slow gait speed, low physical activity (low energy expenditure), and/or unintentional weight loss (9). On the other hand, the Canadian Study of Health and Aging (CSHA) frailty index was developed using the cumulative deficit model (11, 12). The CSHA frailty index measures several age-associated health deficits (13), and is computed by counting the number of health deficits and dividing this number by the total number of health questions tested (12), with a score of 1 being the maximum index and indicating the poorest prognosis. Indeed, an index >0.7 is associated with a high risk of mortality (14). This index has been simplified for use in the outpatient clinic setting as the CSHA “clinical frailty scale” (15). This 7-point rapid screening tool, consisting of 7 variables ranging from fit to complete functional dependence, highly correlates with the frailty index. The maximum in this scale is a score of 7, indicating “severe frailty” (16).
A number of other instruments to assess frailty have been developed and validated, including the “FRAIL (Fatigue, Resistance, Ambulation, Illnesses, Loss of weight)” frailty scale by the International Academy of Nutrition and Aging (17), the “Study of Osteoporotic Fractures (SOF)” frailty scale (18), the “Frailty Instrument for Primary Care of the Survey of Health, the Aging and Retirement in Europe (SHARE-FI)” scale (19), and the “Groningen Frailty Indicator” (20). The FRAIL and SOF, for example, predict new disability at 3 and 9 years of follow up and the FRAIL predicts 9-year mortality in an African American population (21). A multidimensional instrument based on a “structural questionnaire” is the “Tilburg Frailty Indicator” (TFI) (22). This instrument is made up of 10 questions on determinants of frailty that include demographics and other lifestyle questions, as well as, 15 frailty elements arranged according to physical, psychological, and social aspects. The total score of the TFI ranges from 0 to 15, with frailty ascertained if the total score is 5 or greater.
In view of the aging population worldwide, there is a growing medical and scientific interest in the accurate diagnosis and treatment of frailty. Despite multinational efforts to reach an agreement on the definition of frailty and how to assess it with a simple and easily accessible tool, no consensus has been reached, as evidenced by the various definitions and multiple assessment tools being currently used in the literature. However, an agreement has been reached broadly defining frailty as a clinical syndrome characterized by increased vulnerability to stressors that leads to functional impairments and adverse health outcomes (2). This definition is considered to be useful in primary care assessments. Moreover, these functional impairments and health outcomes may be preventable or treatable by pharmacologic or non-pharmacologic interventions.
With regards to the prevalence of frailty in community dwelling individuals over the age of 65 in the United States, it was estimated at 7–12% using the frailty criteria validated in the CHS (10). Moreover, frailty prevalence increased with age from 3.9 to 25% in the 65–74 and over 85 age groups, respectively. Frailty prevalence was also found to be greater in women than men (8 vs. 5%). Ethnic differences in frailty prevalence were noted, with black Americans more likely to be frail than white Americans (13 vs. 6%) and Mexican Americans similar to Caucasians 7.8% (23), based on the Hispanic Established Populations Epidemiologic Studies of the Elderly. Compared to frailty, pre-frailty has a much greater prevalence, ranging between 35 and 50% in adults aged 65 or older. Pre-frailty is considered to be present in patients exhibiting one or two of the phenotypic criteria described in CHS and is reportedly more common in women than in men, just like frailty (9). There is also an association between pre-frailty and lower educational level and socio-economic status (24, 25). Despite the higher accumulation of deficits in women than in men of the same age, men exhibit a higher risk of mortality even though this accumulation is associated with mortality in both genders (26–28). Importantly, comorbidities, especially cardiovascular, pulmonary, musculoskeletal, neurologic, and psychiatric, are more prevalent in pre-frail compared to non-frail persons (24, 25, 29).
Frailty and Cardiovascular Performance
The prevalence of cardiovascular disease (CVD) increases substantially in individuals 65 years of age and over, and especially in individuals aged 80 and over (30). Not surprisingly, increased CVD prevalence is linked with increased prevalence and incidence of frailty, as shown in a meta-analysis of 54,250 elderly patients without frailty at baseline (31).
The aging cardiovascular system has some very specific phenotypic alterations (9, 30, 32). These include aortic stiffness due to increased collagen and decreased elastin, endothelial dysfunction, left ventricular hypertrophy, and a diminution in exercise induced increase in ejection fraction. These characteristic abnormalities are hypothesized to contribute to specific symptoms of the frailty syndrome and to increase the morbidity and mortality from CVD in elderly individuals (7, 10, 30). Several studies document the increased risk for mortality in frail elderly patients with cardiovascular events such as non-ST-segment elevation myocardial infarction (NSTEMI) (3, 7). Frail individuals have increased disease burden and therefore more prolonged recuperation vs. a non-frail subject (2, 8, 10). There are additional associations between frailty and other cardiovascular diagnoses including angina, myocardial infarction, hypertension, heart failure with reduced ejection (HFrEF), heart failure with preserved ejection fraction (HFpEF), and stroke (10, 30). Gait speed is one symptom of frailty that is linked with increased cardiovascular events and mortality, specifically in ST elevation myocardial infarction patients (33, 34). Importantly, frailty presents a major challenge to the ability of CVD patients to undergo surgery and other medical interventions successfully, thus affecting outcomes (30).
Role of Inflammation in Aging and Frailty
“Inflammaging” is a term that has been used to depict the particular molecular and cellular inter-related events that promote the process of aging (35). With aging, there is a continuous accumulation of damaged macromolecules and cells, generation of toxic metabolites and microbial byproducts, and development of cellular senescence and immunosenescence (36, 37). Not only does inflammaging accelerate the aging process, it is linked with and accelerates the diseases associated with aging, including cardiovascular diseases, cognitive, and neurologic impairments, cancer, and degenerative joint disease. Importantly, the increased susceptibility to disease and death is a result of these molecular inflammation-related changes in physiological systems. As such, measuring the molecules or biomarkers that mediate inflammation has become a useful tool to assess the aging process (37). For instance, there is evidence that circulating levels of pro-inflammatory cytokines increase during aging. High levels of TNF-α, interleukin-6 (IL-6), and C-reactive protein (CRP), even in elderly populations considered healthy, are independent predictors of mortality (38). This same inflammatory response underlies the tissue damage linked to various age-related chronic diseases (39). Indeed, a multitude of studies have now reliably demonstrated that chronically high levels of pro-inflammatory biomarkers do predict risk of morbidity and mortality in the elderly population (37).
Frailty involves aging-related decreases in organ physiologic reserve, leading to impaired ability to withstand stressors and resulting in increased vulnerability to disease. Frail patients manifest disturbances in the hematologic and inflammatory systems, which seem to be at the core of this geriatric syndrome (37, 40). For instance, frail patients have elevated levels of fibrinogen, IL-6, factor VIII, D-dimer, and CRP compared to non-frail patients (32, 41). Studies also report reduced hemoglobin, high leukocytes, elevated TNF-α, and low vitamin D as biomarkers of frailty. Importantly, the inflammatory cytokine IL-6 strongly correlates with the frailty phenotype and with unfavorable health outcomes (32, 42–44). Of note, among frail subjects, women exhibit higher concentrations of inflammatory and coagulation factors than men (41).
CRP is an example of one biomarker that has a higher concentration in women experiencing symptoms of frailty. Differential white blood cell counts, on the other hand, similarly predict frailty risk in men and women. Although there is still insufficient data to show which markers specifically affect men or women, dysregulated inflammation is a considerable key physiological marker in correlation with the frailty syndrome in both genders. It is of interest to note that the strong correlation between frailty and inflammatory and hematologic biomarkers is remarkably similar to the strong correlation between CVD and these same biomarkers, supporting the notion that frailty and CVD are clinically interrelated (37). Importantly, frail individuals also have characteristic declines in cardiovascular reserve, as described above, which may in turn contribute to the symptoms of the syndrome.
Substantial evidence has shown that chronic inflammation underlies the syndrome of aging frailty, leading to impairments in mobility and gait, sarcopenia, osteopenia, and decreased strength. High levels of circulating IL-6 correlate with the development of mobility disability (45) and high levels of IL-6 and TNF-α, either alone or together, are linked with decreased muscle mass and strength, increasing the susceptibility to sarcopenia (46, 47). Moreover, high levels of IL-6 and CRP are independently associated with decreases in physical performance and strength in the elderly (48, 49). The Women's Health and Aging Study (WHAS) demonstrated that elevated IL-6 levels in older women were associated with a greater decline in the ability to walk and a greater risk of acquiring physical disabilities (50). The MacArthur Studies of Successful Aging also found an association between decreased walking speed and grip strength and elevated levels of IL-6 and CRP (51). With regards to all-cause mortality predictors, elevated systemic IL-6 levels correlate strongly with various causes of death, as well as, with mortality in the near future (52, 53). A strong correlation is also present between CRP and early mortality and TNF-α and mortality among the elderly (54, 55). Since these inflammatory biomarkers are not specifically indicative of a particular disease or cause of mortality, these increases in systemic inflammation are thought to reflect a fundamental aspect of the aging process (37).
Chronic inflammation also causes remodeling of the immune system, which diminishes immune responses and contributes to increased mortality in subjects over 60 years of age with frailty (32, 42–44). Immunologic remodeling, in turn, is an important pathophysiologic contributor to frailty in older humans (56, 57). This functional impairment in cell-mediated and humoral immunity in frailty is well documented (58–60) and leads to an increased vulnerability to infectious diseases (40). However, the level of inflammation that affects individuals with frailty is “low-grade,” for example, TNF-α levels range between 1.5 and 1.68 pg/ml (61). In this regard, it has been proposed that the chronicity of the inflammation causes more harm than the absolute level at any given time (62). This elevation in systemic TNF-α levels increases intracellular TNF-α in B cells, which causes a shift in B cell subsets producing an increased percentage of exhausted B cells and decreased switch memory B cells, thereby impairing B cell function (63, 64). In aging and frailty, T cell activity is also impaired, and can be assessed by a decrease in the ratio of CD4:CD8 cells (65). Together, these processes promote an immune cell refractory state where both T and B cell responses to de novo antigens and vaccines are diminished (66).
As there is no cure for aging or frailty, the therapeutic strategy is on developing approaches to lessen or at least regulate the effects of chronic inflammation on aging, with the goal to promote a healthier aging process. It is believed that frailty can ultimately be prevented or attenuated, and the link between frailty and inflammation offers a potential therapeutic target.
Endogenous Stem Cells in Frailty
An individual's endogenous stem cell production and function decreases with age and this decrease likely contributes to reduced ability to regenerate and repair organs and tissues (67–69). For instance, there is evidence that as mesenchymal stem cells (MSCs) undergo senescence, their multilineage differentiation and homing capacity and immunomodulatory and wound healing properties gradually disappear (69, 70). These aging-related declines may be due to intrinsic stem cell aging, for example there is evidence that aging induces a “quiescence-to-senescence switch” (71) in stem cells, and aging-related changes in extracellular matrix components and the stem cell niches in tissues (68, 72, 73). Collectively, these aging-related changes reduce stem cell self-renewal, maintenance and regenerative potential. With regard to frailty, altered and dysfunctional stem cell niches have been implicated in frailty syndrome (74, 75). As such, it has been proposed that a regenerative medicine therapeutic approach has the potential to improve or reverse the signs and symptoms of frailty (32, 70), as further discussed below.
Mesenchymal Stem Cells as a Therapeutic Strategy for Frailty
Medical advances and a more health aware society have contributed to a longer living population. However, as the population ages, the growing number of frail elderly patients will continue to increase the demand for healthcare services. Therefore, novel medical therapies for frailty are under investigation to address this unmet need amongst the elderly population. Although certain diets, especially the Mediterranean diet (76, 77), nutritional supplements (78), hormonal supplements (79), and exercise regimes (80, 81) have been shown independently or in combination (82) to improve the signs and symptoms of frailty (8), there is currently no specific medical therapy available to prevent or treat the frailty syndrome.
There are specific features of the frailty syndrome that support a potential role of MSCs to ameliorate or improve frailty. MSCs are drawn to sites of injury, where they act to reduce inflammation and promote cellular repair (83). Notably, MSCs improve cardiovascular outcomes in patients with acute myocardial infarction (84), as well as, ischemic (85) and non-ischemic cardiomyopathy (86), reduce TNF-α and CRP levels, and are safe in patients irrespective of age (83, 87). The strong association between frailty and CVD and the growing database documenting safety and potential favorable effects of cell-based therapy in CVD provide justification for the assessment of potential benefits of cell therapy in subjects with frailty (88, 89; Table 1, Figure 1).
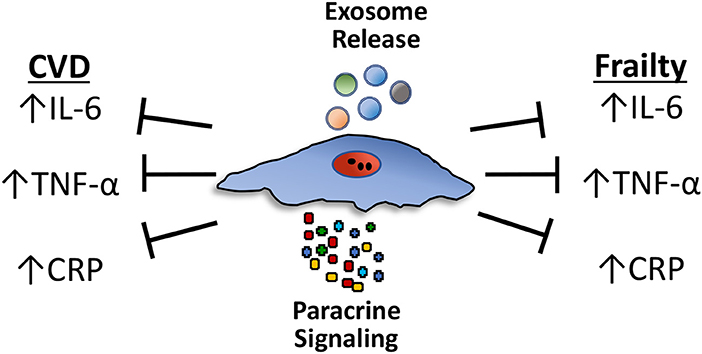
Figure 1. Circulating levels of proinflammatory cytokines, particularly TNF-α, interleukin-6 (IL-6), and C-reactive protein (CRP), increase during aging and are independent predictors of mortality in frail patients. These same pro-inflammatory cytokines are elevated and underlie the tissue damage linked to various age-related chronic diseases, particularly cardiovascular diseases (CVD). Mesenchymal stem cells (MSCs) reduce the expression of proinflammatory cytokines, including TNF-α, IL-6, and CRP, in both CVD and frailty syndrome through paracrine effects or via exosomes. Paracrine effects involve the secretion of a multitude of individual growth factors and cytokines. Exosomes are small extracellular vesicles that contain proteins, peptides and microRNAs (miRNAs).
Anti-inflammatory and Immunomodulatory Effects of Mesenchymal Stem Cells
MSCs can evade and modulate the host's immune system to prolong their therapeutic effects without being detected and eliminated. The absence of major histocompatibility complex (MHC)/human leukocyte antigen (HLA) class II and associated costimulatory molecules and low levels of MHC/HLA class I molecules expressed by MSCs (88, 89) enables them to evade detection by the host immune system. This absence of class II molecules provides the basis for allogeneic MSC therapy, although allogeneic MSCs may eventually induce an immune reaction due to their mismatched MHC-1 molecules, which can be recognized by the host CD8+ T-cells (90).
MSCs influence the host immune system in numerous ways. They reduce both B- and T-lymphocyte proliferation in a paracrine manner (secretion of factors) and by direct cell-cell contact (91, 92). MSCs reduce the expression of proinflammatory cytokines, including, TNF-α, interleukin (IL)-1β, IL-6, and CRP [see (93–95) for review; Figure 1]. The paracrine effects of MSCs are produced in response to either secretion of a wide array of individual factors, such as growth factors and cytokines, or via exosomes, small extracellular vesicles that contain proteins, peptides and microRNAs (miRNAs).
Factors secreted by MSCs include transforming growth factor (TGF)-β, hepatocyte growth factor (HGF) and interleukins, among many others [see (93) for review]. Many of these factors interact to produce an immunomodulatory effect (96). Furthermore, the effect of a specific factor may be modulated by the microenvironment (93). Perhaps the most well studied factor secreted by MSCs is TGF-ß. MSCs produce TGF-ß in response to IL-4 receptor mediated activation of the STAT6 pathway (97). TGF-ß inhibits the proliferation of CD4+ and CD8+ T-cells and the secretion of T helper1 (Th1) cells while increasing T-regulatory cells (Treg). Another factor secreted by MSCs, IL-10, is an anti-inflammatory and immunoregulatory cytokine also expressed by a variety of immune cells. MSC expression of IL-10 requires direct interaction with T-cells (98). IL-10 inhibits the ability of macrophages to produce pro-inflammatory cytokines. However, recent evidence suggests that increased production of IL-10 by cardiac macrophages promotes diastolic dysfunction (99). This result emphasizes that the effect of a specific factor, whether secreted by MSCs or another cell type, is dependent on context and it is the combination and interaction of secreted (and resident) factors that enable MSCs to modulate the host immune system.
MSCs also affect the immune system through their release of exosomes. Exosomes are 40–100 nm extracellular vesicles that can be isolated from MSC-conditioned media. Ex vivo studies demonstrate that MSC-derived exosomes reduce secretion of pro-inflammatory cytokines (IL-1ß, TNF-α) and increase production of TGF-ß by PBMCs, but don't affect PBMC proliferation (100). Administration of MSCs (101, 102) or MSC-derived exosomes (103) reduces the immune response in two mouse models of autoimmune disease, Type 1 diabetes mellitus and uveoretinitis. These results and those from many other studies, suggest that MSC-derived exosomes represent an alternative to stem cell therapy.
Exosomes are secreted by many cell types, including cells of the immune system. A recent study by Ipson et al. isolated exosomes from 7 frail and 7 robust individuals who suffered from similar chronic diseases. Eight exosome-derived miRNAs were identified that were differentially expressed in these two populations and are found at higher levels in frail individuals (104). While the sample size was small, this result suggests that exosome miRNA profiles may represent biomarkers for frailty. Perhaps isolating and administering MSC-derived exosomes containing miRNAs that can counteract these “frailty-specific” miRNAs, will provide a therapeutic option for treating frailty.
Not only do MSCs affect the host's immune system, but the host immune system also modulates the activity of MSCs (105–107). Exposure to interferon (IFN)-γ generally enhances the immunosuppressive action of MSCs while simultaneously increasing their HLA class I and II cell surface marker expression (94). However, low concentrations of both IFN-γ and TNF-α, can cause MSCs to become pro-inflammatory (94, 95). Furthermore, host-derived pro-inflammatory cytokines can impair the capability of MSCs to differentiate into bone, fat, and cartilage lineages [see (94)]. However, even after MSCs have differentiated into chondrocytes, they can exert immunosuppressive effects (108, 109).
MSCs are not all equal. Recent studies suggest that the tissue from which an MSC originates influences its immunomodulatory properties. Kim et al. compared the immunosuppressive properties of MSCs isolated from periodontal ligament, umbilical cord, and adipose tissue and determined that while they all similarly inhibited the proliferation and activation of PBMCs, UC-MSCs and to a lesser extent Ad-MSCs, secreted higher levels of immunosuppressive cytokines in response to IFN-γ (110). Furthermore, MSCs obtained from aged individuals possess reduced immunomodulatory properties compared to those from the young (111, 112). The tissue microenvironment into which MSCs migrate/are injected into also influences their immunosuppressive properties. MSCs located within an inflammatory microenvironment can suppress cytotoxic T cells (96, 113), induce T regulatory cells (114, 115), and stimulate macrophage polarization (116) (transition from an M1 to an M2 phenotype) thereby promoting an anti-inflammatory milieu. Furthermore, a recent study demonstrated that MSCs exert antibacterial effects (117) indicating that MSCs possess an immune function independent of the host's immune system.
Results of Phase I and II Clinical Trials of MSCs for Frailty
Currently, there is no specifically approved treatment for frail patients and therefore no established standard of care. The ultimate goal of a therapeutic strategy for frailty is to lengthen the healthy lifespan and restore or maintain cognitive and physical functionality of patients. We conducted a phase I and a phase II clinical trial, CRATUS (NCT02065245), investigating the safety (primary outcome) and efficacy (secondary outcome) of an intravenous infusion of allogeneic bone marrow-derived MSCs as a novel therapy for treating patients experiencing mild to moderate frailty (56, 57, 75). Efficacy outcomes included physical performance, quality of life, and systemic inflammation.
The phase I trial was a non-randomized, dose-escalation study in which 15 patients diagnosed with frailty, based on the CSHA clinical frailty scale, received allogeneic MSCs by intravenous infusion at doses of 20, 100, or 200 million MSCs (5 patients per group). All of the doses were administered as an 80 mL infusion at a speed of 2 mL/min, for a total infusion time of 40 min. Incidence of any treatment-emergent serious adverse events (TE-SAEs) at 1 month post-infusion was the primary outcome. Physical function measurements and circulating inflammatory biomarkers, measured at 3 and 6 months post-infusion, were the secondary outcomes. No TE-SAEs were reported with any of the doses at 1-month post-infusion and no clinically significant donor-specific immune reactions occurred during the first 6 months post-infusion. The six-min walk distance significantly increased at 3 and 6 months and circulating TNF-α levels significantly decreased at 6 months in all treatment groups. The best improvement in all efficacy outcomes was observed with the 100-million dose, except in the case of TNF-α, which showed a significant improvement with both the 100- and 200-million doses. The physical component of the SF-36 quality of life assessment also showed significant improvements in the 100-million dose group at all time points relative to baseline. This study indicated that allogeneic infusion of MSCs is safe and immunologically tolerated in aging frailty patients.
The phase II trial was a randomized, double-blinded, dose-finding study of intravenous allogeneic MSCs at doses of 100- or 200-million compared to placebo in 30 frailty patients (mean age 75.5 ± 7.3) (57). The primary outcome was safety, namely incidence of TE-SAEs at 1-month post-infusion. The secondary outcomes were physical performance measures, patient-reported quality outcomes, and immune markers of frailty, measured at 6 months post-infusion. There were no therapy-related TE-SAEs reported at 1-month post-infusion. Physical performance improved to a greater extent in the 100-million dose group and measures of immunologic parameters improved in both the 100-million and 200-million dose groups. The 6-min walk test, short physical performance exam, and forced expiratory volume in 1 s improved significantly in the 100-million dose group but not in the 200-million dose or placebo groups. Moreover, there was improvement noted in the female sexual quality of life questionnaire and decreases in serum TNF-α levels in the 100-million dose group. B cell intracellular TNF-α improved significantly in both the 100-million and 200-million dose groups compared to placebo. Early and late activated T-cells were decreased as well by MSC infusion compared to placebo. Although there were no safety concerns with the 200-million dose, there was no added benefit observed with this higher dose compared to the 100-million dose. In summary, intravenous allogeneic MSCs were found to be safe in individuals with aging frailty and produced significant benefits in measures of physical performance as well inflammatory biomarkers, which are important therapeutic outcomes in the frailty syndrome.
Allogeneic therapy was used in these studies because it offers “off-the-shelf” availability and a consistency to the cell product (118). These properties are extremely important as autologous cells may have deficiencies in function due to the underlying disease process, co-morbidities, lifestyle, concomitant medications, and/or patient age (119–121). Despite reports that allogeneic MSCs may be immunologically cleared more rapidly than autologous cells after differentiation (122), due to immunogenicity, this immunologic clearance might be beneficial in reducing any long-term risks of cell engraftment (123). Given the excellent safety profile and promising therapeutic efficacy demonstrated in these early phase trials (Table 1), studies with repeat dosing and longer follow up time (CRATUS; NCT02065245), as well as, a larger phase IIb (NCT03169231) clinical trial are ongoing to establish the efficacy of MSCs in the frailty syndrome.
Author Contributions
IS drafted and edited the manuscript. WB wrote a section, edited the manuscript for scientific content, and made the figure. JH edited the manuscript for scientific content.
Funding
JH and IS are supported by the National Institute of Health (NIH) grants, UM1 HL113460, 1R01 HL134558-01, 1R01 HL137355-01, as well as by the Starr and Soffer Family Foundations.
Conflict of Interest Statement
JH reported having a patent for cardiac cell-based therapy. He holds equity in Vestion Inc. and maintains a professional relationship with Vestion Inc. as a consultant and member of the Board of Directors and Scientific Advisory Board. He is the Chief Scientific Officer, a compensated consultant and advisory board member for Longeveron, and holds equity in Longeveron. He is also the co-inventor of intellectual property licensed to Longeveron. Longeveron LLC and Vestion Inc. did not participate in funding this work.
The remaining authors declare that the research was conducted in the absence of any commercial or financial relationships that could be construed as a potential conflict of interest.
References
1. Chen X, Mao G, Leng SX. Frailty syndrome: an overview. Clin Int Aging (2014) 9:433–41. doi: 10.2147/CIA.S45300
2. Morley JE, Vellas B, van Kan GA, Anker SD, Bauer JM, Bernabei R, et al. Frailty consensus: a call to action. J Am Med Dir Assoc. (2013) 14:392–7. doi: 10.1016/j.jamda.2013.03.022
3. Ekerstad N, Swahn E, Janzon M, Alfredsson J, Lofmark R, Lindenberger M, et al. Frailty is independently associated with 1-year mortality for elderly patients with non-ST-segment elevation myocardial infarction. Eur J Prev Cardiol. (2014) 21:1216–24. doi: 10.1177/2047487313490257
4. Ebrahimi Z, Wilhelmson K, Eklund K, Moore CD, Jakobsson A. Health despite frailty: exploring influences on frail older adults' experiences of health. Geriatr Nurs. (2013) 34:289–94. doi: 10.1016/j.gerinurse.2013.04.008
5. Koller K, Rockwood K. Frailty in older adults: implications for end-of-life care. Cleve Clin J Med. (2013) 80:168–74. doi: 10.3949/ccjm.80a.12100
6. Jylha M, Guralnik JM, Balfour J, Fried LP. Walking difficulty, walking speed, and age as predictors of self-rated health: the women's health and aging study. J Gerontol A Biol Sci Med Sci. (2001) 56:M609–17. doi: 10.1093/gerona/56.10.M609
7. Newman AB, Gottdiener JS, McBurnie MA, Hirsch CH, Kop WJ, Tracy R, et al. Associations of subclinical cardiovascular disease with frailty. J Gerontol A Biol Sci Med Sci. (2001) 56:M158–66. doi: 10.1093/gerona/56.3.M158
8. Sacha J, Sacha M, Sobon J, Borysiuk Z, Feusette P. Is it time to begin a public campaign concerning frailty and pre-frailty? a review article. Front Physiol. (2017) 8:484. doi: 10.3389/fphys.2017.00484
9. Fried LP, Tangen CM, Walston J, Newman AB, Hirsch C, Gottdiener J, et al. Frailty in older adults: evidence for a phenotype. J Gerontol A Biol Sci Med Sci. (2001) 56:M146–56. doi: 10.1093/gerona/56.3.M146
10. Fried LP, Kronmal RA, Newman AB, Bild DE, Mittelmark MB, Polak JF, et al. Risk factors for 5-year mortality in older adults: the Cardiovascular Health Study. JAMA (1998) 279:585–92.
11. Rockwood K, Mitnitski A. Frailty in relation to the accumulation of deficits. J Gerontol A Biol Sci Med Sci. (2007) 62:722–7. doi: 10.1093/gerona/62.7.722
12. Clegg A, Young J, Iliffe S, Rikkert MO, Rockwood K. Frailty in elderly people. Lancet (2013) 381:752–62. doi: 10.1016/S0140-6736(12)62167-9
13. Rockwood K, Blodgett JM, Theou O, Sun MH, Feridooni HA, Mitnitski A, et al. A Frailty index based on deficit accumulation quantifies mortality risk in humans and in mice. Sci Rep. (2017) 7:43068. doi: 10.1038/srep43068
14. Rockwood K, Mitnitski A. Limits to deficit accumulation in elderly people. Mech Ageing Dev. (2006) 127:494–6. doi: 10.1016/j.mad.2006.01.002
15. Ritt M, Ritt JI, Sieber CC, Gassmann KG. Comparing the predictive accuracy of frailty, comorbidity, and disability for mortality: a 1-year follow-up in patients hospitalized in geriatric wards. Clin Interv Aging (2017) 12:293–304. doi: 10.2147/CIA.S124342
16. Rockwood K, Song X, MacKnight C, Bergman H, Hogan DB, McDowell I, et al. A global clinical measure of fitness and frailty in elderly people. CMAJ (2005) 173:489–95. doi: 10.1503/cmaj.050051
17. Morley JE, Malmstrom TK, Miller DK. A simple frailty questionnaire (FRAIL) predicts outcomes in middle aged african americans. J Nutr Health Aging (2012) 16:601–8. doi: 10.1007/s12603-012-0084-2
18. Ensrud KE, Ewing SK, Cawthon PM, Fink HA, Taylor BC, Cauley JA, et al. A Comparison of frailty indexes for the prediction of falls, disability, fractures and mortality in older men. J Am Geriatr Soc. (2009) 57:492–8. doi: 10.1111/j.1532-5415.2009.02137.x
19. Romero-Ortuno R, Walsh CD, Lawlor BA, Kenny RA. A Frailty Instrument for primary care: findings from the Survey of Health, Ageing and Retirement in Europe (SHARE). BMC Geriatrics (2010) 10:57. doi: 10.1186/1471-2318-10-57
20. Drubbel I, Bleijenberg N, Kranenburg G, Eijkemans RJC, Schuurmans MJ, de Wit NJ, et al. Identifying frailty: do the frailty index and groningen frailty indicator cover different clinical perspectives? A cross-sectional study. BMC Family Pract. (2013) 14:64. doi: 10.1186/1471-2296-14-64
21. Malmstrom TK, Miller DK, Morley JE. A comparison of four frailty models. J Am Geriatr Soc. (2014) 62:721–6. doi: 10.1111/jgs.12735
22. Gobbens RJ, van Assen MA, Luijkx KG, Wijnen-Sponselee MT, Schols JM. The Tilburg frailty indicator: psychometric properties. J Am Med Direct Assoc. (2010) 11:344–55. doi: 10.1016/j.jamda.2009.11.003
23. Ottenbacher KJ, Graham JE, Snih SA, Raji M, Samper-Ternent R, Ostir GV, et al. Becoming frail: findings from the hispanic established populations epidemiologic study of the elderly. Am J Public Health (2009) 99:673–9. doi: 10.2105/AJPH.2008.143958
24. Raji MA, Al Snih S, Ostir GV, Markides KS, Ottenbacher KJ. Cognitive status and future risk of frailty in older Mexican Americans. J Gerontol A Biol Sci Med Sci. (2010) 65:1228–34. doi: 10.1093/gerona/glq121
25. Danon-Hersch N, Rodondi N, Spagnoli J, Santos-Eggimann B. Prefrailty and chronic morbidity in the youngest old: an insight from the Lausanne cohort Lc65+. J Am Geriatr Soc. (2012) 60:1687–94. doi: 10.1111/j.1532-5415.2012.04113.x
26. Mitnitski AB, Mogilner AJ, MacKnight C, Rockwood K. The mortality rate as a function of accumulated deficits in a frailty index. Mech Ageing Dev. (2002) 123:1457–60. doi: 10.1016/S0047-6374(02)00082-9
27. Mitnitski AB, Mogilner AJ, MacKnight C, Rockwood K. The accumulation of deficits with age and possible invariants of aging. Sci. World J. (2002) 2:1816–22. doi: 10.1100/tsw.2002.861
28. Puts MT, Lips P, Deeg DJ. Sex differences in the risk of frailty for mortality independent of disability and chronic diseases. J Am Geriatr Soc. (2005) 53:40–7. doi: 10.1111/j.1532-5415.2005.53008.x
29. Fernandez-Garrido J, Ruiz-Ros V, Buigues C, Navarro-Martinez R, Cauli O. Clinical features of prefrail older individuals and emerging peripheral biomarkers: a systematic review. Arch Gerontol Geriatr. (2014) 59:7–17. doi: 10.1016/j.archger.2014.02.008
30. Paneni F, Diaz Canestro C, Libby P, Luscher TF, Camici GG. The aging cardiovascular system: understanding it at the cellular and clinical levels. J Am Coll Cardiol. (2017) 69:1952–67. doi: 10.1016/j.jacc.2017.01.064
31. Afilalo J, Karunananthan S, Eisenberg MJ, Alexander KP, Bergman H. Role of frailty in patients with cardiovascular disease. Am J Cardiol. (2009) 103:1616–21. doi: 10.1016/j.amjcard.2009.01.375
32. Kanapuru B, Ershler WB. Inflammation, coagulation, and the pathway to frailty. Am J Med. (2009) 122:605–13. doi: 10.1016/j.amjmed.2009.01.030
33. Bouillon K, Batty GD, Hamer M, Sabia S, Shipley MJ, Britton A, et al. Cardiovascular disease risk scores in identifying future frailty: the Whitehall II prospective cohort study. Heart (2013) 99:737–42. doi: 10.1136/heartjnl-2012-302922
34. Matsuzawa Y, Konishi M, Akiyama E, Suzuki H, Nakayama N, Kiyokuni M, et al. Association between gait speed as a measure of frailty and risk of cardiovascular events after myocardial infarction. J Am Coll Cardiol. (2013) 61:1964–72. doi: 10.1016/j.jacc.2013.02.020
35. Franceschi C, Bonafe M, Valensin S, Olivieri F, De Luca M, Ottaviani E, et al. Inflamm-aging. An evolutionary perspective on immunosenescence. Ann N Y Acad Sci. (2000) 908:244–54. doi: 10.1111/j.1749-6632.2000.tb06651.x
36. Franceschi C, Campisi J. Chronic inflammation (inflammaging) and its potential contribution to age-associated diseases. J Gerontol A Biol Sci Med Sci. (2014) 69(Suppl. 1):S4–9. doi: 10.1093/gerona/glu057
37. Gonzalez R, Woynarowski D, Geffner L. Stem cells targeting inflammation as potential anti-aging strategies and therapies. Cell Tissue Transpl Ther. (2015) 7:1–8. doi: 10.4137/CTTT.S19477
38. Bruunsgaard H, Pedersen BK. Age-related inflammatory cytokines and disease. Immunol Allergy Clin North Am. (2003) 23:15–39. doi: 10.1016/S0889-8561(02)00056-5
39. Licastro F, Candore G, Lio D, Porcellini E, Colonna-Romano G, Franceschi C, et al. Innate immunity and inflammation in ageing: a key for understanding age-related diseases. Immun Ageing (2005) 2:8. doi: 10.1186/1742-4933-2-8
40. Mitnitski A, Collerton J, Martin-Ruiz C, Jagger C, von Zglinicki T, Rockwood K, et al. Age-related frailty and its association with biological markers of ageing. BMC Med. (2015) 13:161. doi: 10.1186/s12916-015-0400-x
41. Gale CR, Baylis D, Cooper C, Sayer AA. Inflammatory markers and incident frailty in men and women: the English Longitudinal Study of Ageing. Age (2013) 35:2493–501. doi: 10.1007/s11357-013-9528-9
42. Ershler WB, Keller ET. Age-associated increased interleukin-6 gene expression, late-life diseases, and frailty. Annu Rev Med. (2000) 51:245–70. doi: 10.1146/annurev.med.51.1.245
43. de Gonzalo-Calvo D, Neitzert K, Fernandez M, Vega-Naredo I, Caballero B, Garcia-Macia M, et al. Differential inflammatory responses in aging and disease: TNF-alpha and IL-6 as possible biomarkers. Free Radic Biol Med. (2010) 49:733–7. doi: 10.1016/j.freeradbiomed.2010.05.019
44. Bruunsgaard H, Andersen-Ranberg K, Hjelmborg J, Pedersen BK, Jeune B. Elevated levels of tumor necrosis factor alpha and mortality in centenarians. Am J Med. (2003) 115:278–83. doi: 10.1016/S0002-9343(03)00329-2
45. Ferrucci L, Harris TB, Guralnik JM, Tracy RP, Corti MC, Cohen HJ, et al. Serum IL-6 level and the development of disability in older persons. J Am Geriatr Soc. (1999) 47:639–46.
46. Visser M, Pahor M, Taaffe DR, Goodpaster BH, Simonsick EM, Newman AB, et al. Relationship of interleukin-6 and tumor necrosis factor-alpha with muscle mass and muscle strength in elderly men and women: the Health ABC Study. J Gerontol A Biol Sci Med Sci. (2002) 57:M326–32. doi: 10.1093/gerona/57.5.M326
47. Schaap LA, Pluijm SM, Deeg DJ, Harris TB, Kritchevsky SB, Newman AB, et al. Higher inflammatory marker levels in older persons: associations with 5-year change in muscle mass and muscle strength. J Gerontol A Biol Sci Med Sci. (2009) 64:1183–9. doi: 10.1093/gerona/glp097
48. Cesari M, Penninx BW, Pahor M, Lauretani F, Corsi AM, Rhys Williams G, et al. Inflammatory markers and physical performance in older persons: the InCHIANTI study. J Gerontol A Biol Sci Med Sci. (2004) 59:242–8. doi: 10.1093/gerona/59.3.M242
49. Barbieri M, Ferrucci L, Ragno E, Corsi A, Bandinelli S, Bonafe M, et al. Chronic inflammation and the effect of IGF-I on muscle strength and power in older persons. Am J Physiol Endocrinol Metab. (2003) 284:E481–7. doi: 10.1152/ajpendo.00319.2002
50. Ferrucci L, Penninx BW, Volpato S, Harris TB, Bandeen-Roche K, Balfour J, et al. Change in muscle strength explains accelerated decline of physical function in older women with high interleukin-6 serum levels. J Am Geriatr Soc. (2002) 50:1947–54. doi: 10.1046/j.1532-5415.2002.50605.x
51. Taaffe DR, Harris TB, Ferrucci L, Rowe J, Seeman TE. Cross-sectional and prospective relationships of interleukin-6 and C-reactive protein with physical performance in elderly persons: MacArthur studies of successful aging. J Gerontol A Biol Sci Med Sci. (2000) 55:M709–15. doi: 10.1093/gerona/55.12.M709
52. Newman AB, Sachs MC, Arnold AM, Fried LP, Kronmal R, Cushman M, et al. Total and cause-specific mortality in the cardiovascular health study. J Gerontol A Biol Sci Med Sci. (2009) 64:1251–61. doi: 10.1093/gerona/glp127
53. Walston JD, Matteini AM, Nievergelt C, Lange LA, Fallin DM, Barzilai N, et al. Inflammation and stress-related candidate genes, plasma interleukin-6 levels, and longevity in older adults. Exp Gerontol. (2009) 44:350–5. doi: 10.1016/j.exger.2009.02.004
54. Jenny NS, Yanez ND, Psaty BM, Kuller LH, Hirsch CH, Tracy RP. Inflammation biomarkers and near-term death in older men. Am J Epidemiol. (2007) 165:684–95. doi: 10.1093/aje/kwk057
55. Harris TB, Ferrucci L, Tracy RP, Corti MC, Wacholder S, Ettinger WH Jr, et al. Associations of elevated interleukin-6 and C-reactive protein levels with mortality in the elderly. Am J Med. (1999) 106:506–12.
56. Golpanian S, DiFede DL, Khan A, Schulman IH, Landin AM, Tompkins BA, et al. Allogeneic human mesenchymal stem cell infusions for aging frailty. J Gerontol Ser A Biolog Sci Med Sci. (2017) 72:1505–12. doi: 10.1093/gerona/glx056
57. Tompkins BA, DiFede DL, Khan A, Landin AM, Schulman IH, Pujol MV, et al. Allogeneic mesenchymal stem cells ameliorate aging frailty: a phase II randomized, double-blinded, placebo controlled clinical trial. J Gerontol Ser A Biol Sci Med Sci. (2017) 72:1513–22. doi: 10.1093/gerona/glx137
58. Ghatreh-Samani M, Esmaeili N, Soleimani M, Asadi-Samani M, Ghatreh-Samani K, Shirzad H. Oxidative stress and age-related changes in T cells: is thalassemia a model of accelerated immune system aging? Cent Eur J Immunol. (2016) 41:116–24. doi: 10.5114/ceji.2015.56973
59. Pinti M, Appay V, Campisi J, Frasca D, Fulop T, Sauce D, et al. Aging of the immune system: focus on inflammation and vaccination. Eur J Immunol. (2016) 46:2286–301. doi: 10.1002/eji.201546178
60. Qin L, Jing X, Qiu Z, Cao W, Jiao Y, Routy JP, et al. Aging of immune system: Immune signature from peripheral blood lymphocyte subsets in 1068 healthy adults. Aging (2016) 8:848–59. doi: 10.18632/aging.100894
61. Hubbard RE, O'Mahony MS, Savva GM, Calver BL, Woodhouse KW. Inflammation and frailty measures in older people. J Cell Mol Med. (2009) 13:3103–9. doi: 10.1111/j.1582-4934.2009.00733.x
62. De Martinis M, Franceschi C, Monti D, Ginaldi L. Inflammation markers predicting frailty and mortality in the elderly. Exp Mol Pathol. (2006) 80:219–27. doi: 10.1016/j.yexmp.2005.11.004
63. Frasca D, Blomberg BB. Inflammaging decreases adaptive and innate immune responses in mice and humans. Biogerontology (2016) 17:7–19. doi: 10.1007/s10522-015-9578-8
64. Frasca D, Diaz A, Romero M, Landin AM, Blomberg BB. Age effects on B cells and humoral immunity in humans. Ageing Res Rev. (2011) 10:330–5. doi: 10.1016/j.arr.2010.08.004
65. Strindhall J, Skog M, Ernerudh J, Bengner M, Lofgren S, Matussek A, et al. The inverted CD4/CD8 ratio and associated parameters in 66-year-old individuals: the Swedish HEXA immune study. Age (2013) 35:985–91. doi: 10.1007/s11357-012-9400-3
66. Frasca D, Diaz A, Romero M, Landin AM, Phillips M, Lechner SC, et al. Intrinsic defects in B cell response to seasonal influenza vaccination in elderly humans. Vaccine (2010) 28:8077–84. doi: 10.1016/j.vaccine.2010.10.023
67. Zhuo Y, Li SH, Chen MS, Wu J, Kinkaid HY, Fazel S, et al. Aging impairs the angiogenic response to ischemic injury and the activity of implanted cells: combined consequences for cell therapy in older recipients. J Thorac Cardiovasc Surg. (2010) 139:1286–94.e1–2. doi: 10.1016/j.jtcvs.2009.08.052
68. Jones DL, Rando TA. Emerging models and paradigms for stem cell ageing. Nat Cell Biol. (2011) 13:506–12. doi: 10.1038/ncb0511-506
69. Yu KR, Kang KS. Aging-related genes in mesenchymal stem cells: a mini-review. Gerontology (2013) 59:557–63. doi: 10.1159/000353857
70. Raggi C, Berardi AC. Mesenchymal stem cells, aging and regenerative medicine. Muscles Ligaments Tendons J. (2012) 2:239–42.
71. Sousa-Victor P, Gutarra S, Garcia-Prat L, Rodriguez-Ubreva J, Ortet L, Ruiz-Bonilla V, et al. Geriatric muscle stem cells switch reversible quiescence into senescence. Nature (2014) 506:316–21. doi: 10.1038/nature13013
72. Geissler S, Textor M, Schmidt-Bleek K, Klein O, Thiele M, Ellinghaus A, et al. In serum veritas-in serum sanitas? Cell non-autonomous aging compromises differentiation and survival of mesenchymal stromal cells via the oxidative stress pathway. Cell Death Dis. (2013) 4:e970. doi: 10.1038/cddis.2013.501
73. Ballard VL. Stem cells for heart failure in the aging heart. Heart Fail Rev. (2010) 15:447–56. doi: 10.1007/s10741-010-9160-z
74. Lopez-Otin C, Blasco MA, Partridge L, Serrano M, Kroemer G. The hallmarks of aging. Cell (2013) 153:1194–217. doi: 10.1016/j.cell.2013.05.039
75. Golpanian S, DiFede DL, Pujol MV, Lowery MH, Levis-Dusseau S, Goldstein BJ, et al. Rationale and design of the allogeneiC human mesenchymal stem cells (hMSC) in patients with aging fRAilTy via intravenoUS delivery (CRATUS) study: a phase I/II, randomized, blinded and placebo controlled trial to evaluate the safety and potential efficacy of allogeneic human mesenchymal stem cell infusion in patients with aging frailty. Oncotarget (2016) 7:11899–912. doi: 10.18632/oncotarget.7727
76. Voelker R. The mediterranean diet's fight against frailty. JAMA (2018) 319:1971–2. doi: 10.1001/jama.2018.3653
77. Kojima G, Avgerinou C, Iliffe S, Walters K. Adherence to mediterranean diet reduces incident frailty risk: systematic review and meta-analysis. J Am Geriatr Soc. (2018) 66:783-8. doi: 10.1111/jgs.15251
78. Kim CO, Lee KR. Preventive effect of protein-energy supplementation on the functional decline of frail older adults with low socioeconomic status: a community-based randomized controlled study. J Gerontol A Biol Sci Med Sci. (2013) 68:309–16. doi: 10.1093/gerona/gls167
79. O'Connell MD, Wu FC. Androgen effects on skeletal muscle: implications for the development and management of frailty. Asian J Androl. (2014) 16:203–12. doi: 10.4103/1008-682X.122581
80. Cadore EL, Moneo AB, Mensat MM, Munoz AR, Casas-Herrero A, Rodriguez-Manas L, et al. Positive effects of resistance training in frail elderly patients with dementia after long-term physical restraint. Age (2014) 36:801–11. doi: 10.1007/s11357-013-9599-7
81. Cadore EL, Rodriguez-Manas L, Sinclair A, Izquierdo M. Effects of different exercise interventions on risk of falls, gait ability, and balance in physically frail older adults: a systematic review. Rejuvenation Res. (2013) 16:105–14. doi: 10.1089/rej.2012.1397
82. Artaza-Artabe I, Saez-Lopez P, Sanchez-Hernandez N, Fernandez-Gutierrez N, Malafarina V. The relationship between nutrition and frailty: effects of protein intake, nutritional supplementation, vitamin D and exercise on muscle metabolism in the elderly. A systematic review. Maturitas (2016) 93:89–99. doi: 10.1016/j.maturitas.2016.04.009
83. Bagno L, Hatzistergos KE, Balkan W, Hare JM. Mesenchymal stem cell-based therapy for cardiovascular disease: progress and challenges. Mol Ther. (2018) 26:1610–23. doi: 10.1016/j.ymthe.2018.05.009
84. Hare JM, Traverse JH, Henry TD, Dib N, Strumpf RK, Schulman SP, et al. A randomized, double-blind, placebo-controlled, dose-escalation study of intravenous adult human mesenchymal stem cells (prochymal) after acute myocardial infarction. J Am Coll Cardiol. (2009) 54:2277–86. doi: 10.1016/j.jacc.2009.06.055
85. Hare JM, Fishman JE, Gerstenblith G, Difede Velazquez DL, Zambrano JP, Suncion VY, et al. Comparison of allogeneic vs autologous bone marrow-derived mesenchymal stem cells delivered by transendocardial injection in patients with ischemic cardiomyopathy: the POSEIDON randomized trial. JAMA (2012) 308:2369–79. doi: 10.1001/jama.2012.25321
86. Hare JM, DiFede DL, Rieger AC, Florea V, Landin AM, El-Khorazaty J, et al. Randomized comparison of allogeneic versus autologous mesenchymal stem cells for nonischemic dilated cardiomyopathy: POSEIDON-DCM trial. J Am Coll Cardiol. (2017) 69:526–37. doi: 10.1016/j.jacc.2016.11.009
87. Golpanian S, El-Khorazaty J, Mendizabal A, DiFede DL, Suncion VY, Karantalis V, et al. Effect of aging on human mesenchymal stem cell therapy in ischemic cardiomyopathy patients. J Am Coll Cardiol. (2015) 65:125–32. doi: 10.1016/j.jacc.2014.10.040.
88. Jacobs SA, Roobrouck VD, Verfaillie CM, Van Gool SW. Immunological characteristics of human mesenchymal stem cells and multipotent adult progenitor cells. Immunol Cell Biol. (2013) 91:32–9. doi: 10.1038/icb.2012.64
89. Ryan JM, Barry FP, Murphy JM, Mahon BP. Mesenchymal stem cells avoid allogeneic rejection. J Inflamm. (2005) 2:8. doi: 10.1186/1476-9255-2-8
90. Berglund AK, Fortier LA, Antczak DF, Schnabel LV. Immunoprivileged no more: measuring the immunogenicity of allogeneic adult mesenchymal stem cells. Stem Cell Res Ther. (2017) 8:288. doi: 10.1186/s13287-017-0742-8
91. Munoz-Fernandez R, Prados A, Leno-Duran E, Blazquez A, Garcia-Fernandez JR, Ortiz-Ferron G, et al. Human decidual stromal cells secrete C-X-C motif chemokine 13, express B cell-activating factor and rescue B lymphocytes from apoptosis: distinctive characteristics of follicular dendritic cells. Hum Reprod. (2012) 27:2775–84. doi: 10.1093/humrep/des198
92. Castro-Manrreza ME, Mayani H, Monroy-Garcia A, Flores-Figueroa E, Chavez-Rueda K, Legorreta-Haquet V, et al. Human mesenchymal stromal cells from adult and neonatal sources: a comparative in vitro analysis of their immunosuppressive properties against T cells. Stem Cells Dev. (2014) 23:1217–32. doi: 10.1089/scd.2013.0363
93. Fontaine MJ, Shih H, Schafer R, Pittenger MF. Unraveling the mesenchymal stromal cells' paracrine immunomodulatory effects. Transfus Med Rev. (2016) 30:37–43. doi: 10.1016/j.tmrv.2015.11.004
94. Najar M, Krayem M, Merimi M, Burny A, Meuleman N, Bron D, et al. Insights into inflammatory priming of mesenchymal stromal cells: functional biological impacts. Inflamm Res. (2018) 67:467–77. doi: 10.1007/s00011-018-1131-1
95. Shi Y, Wang Y, Li Q, Liu K, Hou J, Shao C, et al. Immunoregulatory mechanisms of mesenchymal stem and stromal cells in inflammatory diseases. Nat Rev Nephrol. (2018) 14:493–507. doi: 10.1038/s41581-018-0023-5
96. Di Nicola M, Carlo-Stella C, Magni M, Milanesi M, Longoni PD, Matteucci P, et al. Human bone marrow stromal cells suppress T-lymphocyte proliferation induced by cellular or nonspecific mitogenic stimuli. Blood (2002) 99:3838–43. doi: 10.1182/blood.V99.10.3838
97. Takeda K, Tanaka T, Shi W, Matsumoto M, Minami M, Kashiwamura S, et al. Essential role of Stat6 in IL-4 signalling. Nature (1996) 380:627–30. doi: 10.1038/380627a0
98. Nasef A, Chapel A, Christelle M, Bouchet S, Lopez M, Mathieu N, et al. Identification of IL-10 and TGF-beta transcripts involved in the inhibition of T-lymphocyte proliferation during cell contact with human mesenchymal stem cells. Gene Exp. (2007) 13:217–26. doi: 10.3727/000000006780666957
99. Hulsmans M, Sager HB, Roh JD, Valero-Munoz M, Houstis NE, Iwamoto Y, et al. Cardiac macrophages promote diastolic dysfunction. J Exp Med. (2018) 215:423–40. doi: 10.1084/jem.20171274
100. Chen W, Huang Y, Han J, Yu L, Li Y, Lu Z, et al. Immunomodulatory effects of mesenchymal stromal cells-derived exosome. Immunol Res. (2016) 64:831–40. doi: 10.1007/s12026-016-8798-6
101. Kota DJ, Wiggins LL, Yoon N, Lee RH. TSG-6 produced by hMSCs delays the onset of autoimmune diabetes by suppressing Th1 development and enhancing tolerogenicity. Diabetes (2013) 62:2048–58. doi: 10.2337/db12-0931
102. Ko JH, Lee HJ, Jeong HJ, Kim MK, Wee WR, Yoon SO, et al. Mesenchymal stem/stromal cells precondition lung monocytes/macrophages to produce tolerance against allo- and autoimmunity in the eye. Proc Natl Acad Sci USA. (2016) 113:158–63. doi: 10.1073/pnas.1522905113
103. Shigemoto-Kuroda T, Oh JY, Kim DK, Jeong HJ, Park SY, Lee HJ, et al. MSC-derived extracellular vesicles attenuate immune responses in two autoimmune murine models: type 1 diabetes and uveoretinitis. Stem Cell Rep. (2017) 8:1214–25. doi: 10.1016/j.stemcr.2017.04.008
104. Ipson BR, Fletcher MB, Espinoza SE, Fisher AL. Identifying exosome-derived MicroRNAs as candidate biomarkers of frailty. J Frailty Aging (2018) 7:100–3. doi: 10.14283/jfa.2017.45
105. Chen K, Wang D, Du WT, Han ZB, Ren H, Chi Y, et al. Human umbilical cord mesenchymal stem cells hUC-MSCs exert immunosuppressive activities through a PGE2-dependent mechanism. Clin Immunol. (2010) 135:448–58. doi: 10.1016/j.clim.2010.01.015
106. Krampera M, Cosmi L, Angeli R, Pasini A, Liotta F, Andreini A, et al. Role for interferon-gamma in the immunomodulatory activity of human bone marrow mesenchymal stem cells. Stem Cells (2006) 24:386–98. doi: 10.1634/stemcells.2005-0008
107. Bortolotti F, Ruozi G, Falcione A, Doimo S, Dal Ferro M, Lesizza P, et al. In vivo functional selection identifies cardiotrophin-1 as a cardiac engraftment factor for mesenchymal stromal cells. Circulation (2017) 136:1509–24. doi: 10.1161/CIRCULATIONAHA.117.029003
108. Du WJ, Reppel L, Leger L, Schenowitz C, Huselstein C, Bensoussan D, et al. Mesenchymal stem cells derived from human bone marrow and adipose tissue maintain their immunosuppressive properties after chondrogenic differentiation: role of HLA-G. Stem Cells Dev. (2016) 25:1454–69. doi: 10.1089/scd.2016.0022
109. Lee HJ, Kang KS, Kang SY, Kim HS, Park SJ, Lee SY, et al. Immunologic properties of differentiated and undifferentiated mesenchymal stem cells derived from umbilical cord blood. J Vet Sci. (2016) 17:289–97. doi: 10.4142/jvs.2016.17.3.289
110. Kim JH, Jo CH, Kim HR, Hwang YI. Comparison of immunological characteristics of mesenchymal stem cells from the periodontal ligament, umbilical cord, and adipose tissue. Stem Cells Int. (2018) 2018:8429042. doi: 10.1155/2018/8429042
111. Wu LW, Wang YL, Christensen JM, Khalifian S, Schneeberger S, Raimondi G, et al. Donor age negatively affects the immunoregulatory properties of both adipose and bone marrow derived mesenchymal stem cells. Trans Immunol. (2014) 30:122–7. doi: 10.1016/j.trim.2014.03.001
112. Kizilay Mancini O, Shum-Tim D, Stochaj U, Correa JA, Colmegna I. Age, atherosclerosis and type 2 diabetes reduce human mesenchymal stromal cell-mediated T-cell suppression. Stem Cell Res Ther. (2015) 6:140. doi: 10.1186/s13287-015-0127-9
113. Maggini J, Mirkin G, Bognanni I, Holmberg J, Piazzon IM, Nepomnaschy I, et al. Mouse bone marrow-derived mesenchymal stromal cells turn activated macrophages into a regulatory-like profile. PLoS ONE (2010) 5:e9252. doi: 10.1371/journal.pone.0009252
114. Li H, Wang L, Pang Y, Jiang Z, Liu Z, Xiao H, et al. In patients with chronic aplastic anemia, bone marrow-derived MSCs regulate the Treg/Th17 balance by influencing the Notch/RBP-J/FOXP3/RORgammat pathway. Sci Rep. (2017) 7:42488. doi: 10.1038/srep42488
115. Lee HJ, Kim SN, Jeon MS, Yi T, Song SU. ICOSL expression in human bone marrow-derived mesenchymal stem cells promotes induction of regulatory T cells. Sci Rep. (2017) 7:44486. doi: 10.1038/srep44486
116. Melief SM, Schrama E, Brugman MH, Tiemessen MM, Hoogduijn MJ, Fibbe WE, et al. Multipotent stromal cells induce human regulatory T cells through a novel pathway involving skewing of monocytes toward anti-inflammatory macrophages. Stem Cells (2013) 31:1980–91. doi: 10.1002/stem.1432
117. Alcayaga-Miranda F, Cuenca J, Khoury M. Antimicrobial activity of mesenchymal stem cells: current status and new perspectives of antimicrobial peptide-based therapies. Front Immunol. (2017) 8:339. doi: 10.3389/fimmu.2017.00339
118. Boyle AJ, McNiece IK, Hare JM. Mesenchymal stem cell therapy for cardiac repair. Methods Mol Biol. (2010) 660:65–84. doi: 10.1007/978-1-60761-705-1_5
119. Kissel CK, Lehmann R, Assmus B, Aicher A, Honold J, Fischer-Rasokat U, et al. Selective functional exhaustion of hematopoietic progenitor cells in the bone marrow of patients with postinfarction heart failure. J Am Coll Cardiol. (2007) 49:2341–9. doi: 10.1016/j.jacc.2007.01.095
120. Kovacic JC, Moreno P, Hachinski V, Nabel EG, Fuster V. Cellular senescence, vascular disease, and aging: part 1 of a 2-part review. Circulation (2011) 123:1650–60. doi: 10.1161/CIRCULATIONAHA.110.007021
121. Heiss C, Keymel S, Niesler U, Ziemann J, Kelm M, Kalka C. Impaired progenitor cell activity in age-related endothelial dysfunction. J Am Coll Cardiol. (2005) 45:1441–8. doi: 10.1016/j.jacc.2004.12.074
122. Huang XP, Sun Z, Miyagi Y, McDonald Kinkaid H, Zhang L, Weisel RD, et al. Differentiation of allogeneic mesenchymal stem cells induces immunogenicity and limits their long-term benefits for myocardial repair. Circulation (2010) 122:2419–29. doi: 10.1161/CIRCULATIONAHA.110.955971
Keywords: cell transplantation, regenerative medicine, inflammation, immunosenescence, geriatrics
Citation: Schulman IH, Balkan W and Hare JM (2018) Mesenchymal Stem Cell Therapy for Aging Frailty. Front. Nutr. 5:108. doi: 10.3389/fnut.2018.00108
Received: 17 August 2018; Accepted: 26 October 2018;
Published: 15 November 2018.
Edited by:
Akio Inui, Kagoshima University, JapanReviewed by:
Katsuyuki Oki, BioMimetics Sympathies Inc., JapanTakahito Nishikata, Konan University, Japan
Copyright © 2018 Schulman, Balkan and Hare. This is an open-access article distributed under the terms of the Creative Commons Attribution License (CC BY). The use, distribution or reproduction in other forums is permitted, provided the original author(s) and the copyright owner(s) are credited and that the original publication in this journal is cited, in accordance with accepted academic practice. No use, distribution or reproduction is permitted which does not comply with these terms.
*Correspondence: Ivonne Hernandez Schulman, aXNjaHVsbWFuQG1lZC5taWFtaS5lZHU=