- 1The New Zealand Institute for Plant and Food Research Ltd., Palmerston North, New Zealand
- 2The New Zealand Institute for Plant and Food Research Ltd., Hamilton, New Zealand
- 3AgResearch Ltd., The Hopkirk Research Institute, Palmerston North, New Zealand
Aim: To evaluate blackcurrant anthocyanin-rich extract (BAE) consumption on time- and dose-dependent plasma anthocyanin bioavailability and conduct a pilot study to explore the potential effect of BAE in promoting recovery from exercise-induced oxidative stress, and maintenance of circulating neutrophil function.
Methods: Time- and dose-dependent blackcurrant anthocyanin bioavailability was assessed using LC-MS in 12 participants over 6 h after the ingestion of a placebo or BAE containing 0.8, 1.6, or 3.2 mg/kg total anthocyanins. In a separate pilot intervention exercise trial, 32 participants consumed either a placebo or 0.8, 1.6, or 3.2 mg/kg BAE (8 individuals per group), and then 1 h later performed a 30 min row at 70% VO2max. Blood was collected during the trial for oxidative, antioxidant, inflammatory, and circulating neutrophil status.
Results: Consumption of BAE caused a time- and dose-dependent increase in plasma anthocyanins, peaking at 2 h after ingestion of 3.2 mg/kg BAE (217 ± 69 nM). BAE consumed 1 h prior to a 30 min row had no effect on plasma antioxidant status but hastened the recovery from exercise-induced oxidative stress: By 2 h recovery, consumption of 1.6 mg/kg BAE prior to exercise caused a significant (P < 0.05) 34 and 32% decrease in post-exercise plasma oxidative capacity and protein carbonyl levels, respectively, compared to placebo. BAE consumption prior to exercise dose-dependently attenuated a small, yet significant (P < 0.01) transient 13 ± 2% decline in circulating neutrophils observed in the placebo group immediately post-exercise. Furthermore, the timed consumption of either 1.6 or 3.2 mg/kg BAE attenuated a 17 ± 2.4% (P < 0.05) decline in neutrophil phagocytic capability of opsonised FITC-Escherichia coli observed 6 h post-exercise in the placebo group. Similarly, a dose-dependent increase in neutrophil surface expression of complement receptor-3 complex (CR3, critical for effective phagocytosis of opsonised microbes), was observed 6 h post-exercise in both 1.6 and 3.2 mg/kg BAE intervention groups.
Conclusions: Consumption of BAE (>1.6 mg/kg) 1 h prior to exercise facilitated recovery from exercise-induced oxidative stress and preserved circulating neutrophil function. This study provides data to underpin a larger study designed to evaluate the efficacy of timed BAE consumption on post-exercise recovery and innate immunity.
Introduction
Whether an athlete is undertaking an intense training program for a sporting event, or is a recreational exerciser just trying to keep fit and healthy, there is a fine balance between achieving the maximum health benefits of exercise and the accumulation of tissue damaging oxidative stress. Exercise-induced reactive oxygen (ROS) and nitrogen species (RNS) are unavoidable by-products of increased oxidative metabolism. Although initially thought to be detrimental to health (1), ROS/RNS have recently been shown to play a pivotal role in the body's adaptive response to exercise (2, 3) as blocking ROS/RNS by high doses of dietary antioxidants eliminates the health promoting effects of exercise (4, 5). ROS/RNS released during exercise serve as signaling molecules and act at various cellular transcription factors (by direct oxidation or through reversible interactions with upstream redox-sensitive proteins) to regulate numerous cellular innate defense systems, including mitochondrial adaptation to enhance antioxidant capacity (6), and modulation of immune status (7). Moreover, whilst regular exercise boosts the body's natural defenses overall (8–10), studies have shown a transient drop in circulating leukocyte number and/or function following exercise. This is driven by an oxidative imbalance that is dependent upon the exercise intensity and duration as well as the fitness of the individuals, which can create an “open window” for opportunistic infections (10–12). Circulating neutrophils are the first line of defense against microbial infection, and are instrumental in coordinating acute inflammatory, tissue repair and appropriate adaptive immune responses (13). Therefore, it is important that the number and functionality of circulating neutrophils are maintained during exercise and recovery to preserve immune defenses. Under most exercise conditions, oxidative balance is maintained within physiological limits minimizing the potential for oxidative damage and increased susceptibility to infection (2, 10). However, prolonged intense exercise in the absence of sufficient recovery time can result in oxidative stress leading to immune fatigue, delayed tissue repair/adaptation, enhanced susceptibility to infection, and ultimately immune suppression and loss of performance (11). Foods and/or dietary supplements that support innate immunity (including maximizing cellular antioxidant capacity) may serve to reduce the possible detrimental effects of exercise training and promote exercise-induced physiological adaptation.
Implementation of an appropriate nutritional strategy is integral to boosting the adaptive effects of regular exercise (12, 14). Functional nutritional strategies show that consumption of carbohydrate and/or protein post-exercise not only replenishes glycogen stores, but also improves skeletal muscle strength and ergonomics (15, 16). Recently, the potential bioactive efficacy of dietary micronutrients, vitamins, minerals, and plant extracts within an exercise regime has been explored (12, 17, 18). The primary focus has been on tailoring micronutrient bioactivity to the needs of the athlete or recreational exerciser, such as speeding up recovery between exercise bouts or maintaining immune defenses to avoid illness. Dietary antioxidants, such as vitamin C and E, are often taken during exercise training to reduce exercise-related oxidative stress damage (19, 20). However, while these are an essential component of daily dietary intake, and have been shown to prevent protein oxidation during exercise, their suitability for the prevention of exercise-induced oxidative stress is questionable. Nutritional intervention with high strength antioxidants have been shown to prevent the adaptive action of ROS/RNS and should be avoided as a post-exercise recovery strategy (2). Select plant-derived polyphenolic compounds show a high endogenous antioxidant capacity (21), and numerous in vitro cell studies have demonstrated the inherent antioxidant properties of polyphenolic compounds, including anthocyanins (22, 23). However, nutritional intervention studies with dietary polyphenolic-rich foods/supplements have found that the attenuation of exercise-induced oxidative stress, exercise recovery and adaptation events was independent of their direct antioxidant properties (17, 24, 25). Furthermore, daily consumption of foods, drinks and/or supplements rich in polyphenolic compounds have been shown to support innate immune defenses and ameliorate metabolic-related (as well as delay the onset of age-related) chronic inflammatory illnesses (26, 27). Nutritional intervention has also been successfully employed to reduce post-exercise susceptibility to infection in athletes undertaking demanding training schedules (2). However, the efficacy of timed polyphenolic rich supplementation to support sports training adaptation and performance benefits is unclear.
Blackcurrants (Ribes nigrum) possess a high inherent antioxidant capacity compared to most other fruits, primarily due to their high anthocyanin and vitamin C content (28, 29). Recent studies, however, show that the inherent chemical antioxidant properties of fruits is not necessarily a good indicator of their actual health-benefiting properties. Blackcurrant anthocyanins, for example, have also been shown to exhibit health properties independent of their antioxidant capability using both in vitro cell studies (30–32) and human nutrition studies, whereby consumption of blackcurrant anthocyanin compounds have been reported to support innate immunity (33–35), improve eye blood flow (36) and augment the adaptive events of exercise (37, 38). In this study, we assessed the dose and temporal bioavailability of anthocyanins after consumption of a New Zealand blackcurrant anthocyanin-rich extract (BAE) and apply this knowledge in a pilot trial designed to examine the dose-dependent effect of pre-exercise BAE consumption on recovery from exercise-induced oxidative stress and the maintenance of circulating neutrophil function.
Materials and Methods
Human Trial Design
Subject Selection
The human trials conducted in this study were approved by either the Northern (NTY/09/10/105, Hamilton) or Central (CEN/09/11/088, Wellington) Ethical Regional Committees of New Zealand. Healthy individuals between 20 and 60 years old were recruited from Hamilton and Palmerston North communities and within The New Zealand Institute for Plant and Food Research Ltd. All subjects provided written informed consent and refrained from (i) eating foods (including drinks) containing anthocyanins and berryfruits and (ii) taking antioxidant dietary supplements 24 h prior to the start of the trials. Participants recruited for the pilot intervention exercise trial, exercised daily, displayed similar physical characteristics and habitual activity scores (Table 1); assessed by Baêcke questionnaire (39). In addition, the 70% VO2max exercise intensity used in this pilot trial has successfully been shown by us to evoke a measureable increase in oxidative stress (33, 40): The 70% VO2max exercise intensity was achieved by participants rowing at a pace that raised their heart rate to the equivalent of their predicted 70% VO2max (41, 42) through the monitoring of their heart rate using a heart monitor (model AXN700 Polar Electro, Auckland, New Zealand) for the duration of the 30 min row. Subjects were excluded from the study if they had known fruit allergies, blood borne diseases (e.g., hepatitis), viral or bacterial illness, were under-going an immunization programme, taking mediation that affected blood properties (e.g., clotting), pregnant, or planning to get pregnant. Individuals participating in the exercise trial were also excluded if they were unable to perform the 30 min rowing exercise (e.g., current injury or recovering from injury received within the last 3 months) or did not meet the required fitness criteria.
Nutritional Intervention
The blackcurrant anthocyanin-rich extract (BAE) consisted of 34% anthocyanins and can be sourced from the New Zealand Blackcurrant Co-operative (www.nzblackcurrants.com/, New Zealand). The dietary extract was made from New Zealand blackcurrants and displayed a high inherent antioxidant capacity (6424; ORAC and 3810; FRAP μmol Trolox equivalents) and contained < 1 mg/100 g of vitamin C. Administered BAE (0.8, 1.6, or 3.2 mg/kg) amounts were calculated using participant's weight (kg) and total anthocyanins (mg) measured by LC-MS. A fruit sugar placebo (PLA) similar to that used in previous nutrition exercise intervention trials was selected.
Study Format (Figure 1)
(i) Plasma anthocyanin bioavailability trials. All trials were performed at the same time of day (morning ~8 am) and 2 h after eating a set breakfast consisting of One-Square MealTM (Queenstown, New Zealand) cereal bar and drink (water). The trial design for time-dependent plasma anthocyanin profiles was a non-blinded, single arm format recruiting 12 individuals (6 female and 6 males). Trial participants donated blood samples before consuming two opaque gelatine capsules containing BAE (3.2 mg/kg total anthocyanins). Further blood samples were taken at 0.5, 1, 2, and 6 h post ingestion. To examine the dose-dependent plasma bioavailability of blackcurrant anthocyanins, a non-blinded parallel study design was employed where 24 participants were randomly assigned into one of four arms (6 individuals per arm): placebo (PLA) or BAE (0.8, 1.6, or 3.2 mg/kg total anthocyanins). A blood sample was taken prior to participants consuming 1–2 opaque gelatine capsules and then 1 h later.
(ii) Pilot study. BAE intervention exercise recovery. A double-blind placebo-controlled parallel trial design consisted of four arms: PLA or BAE at 0.8, 1.6, or 3.2 mg/kg total anthocyanins. Thirty-two trial participants were randomly assigned to one of the four arms (8 individuals per arm) by an independent researcher and blinded from both trial participants and the trial coordinator. This trial was performed in the morning and all participants prepared for the trial as described above. At the start of the trial, a blood sample was taken and participants were fitted with a heart monitor and asked to consume 1-2 gelatin capsules with water. After 1 h, participant's heart rate was recorded and another blood sample collected, participants then performed a 30 min row (using a Concept 2 rower) at their predicted 70% VO2max (see methods). Upon completion, a blood sample was taken and the distance (meters) rowed and participants heart rates were recorded. Further blood samples were collected during post-exercise recovery at 2 and 6 h.
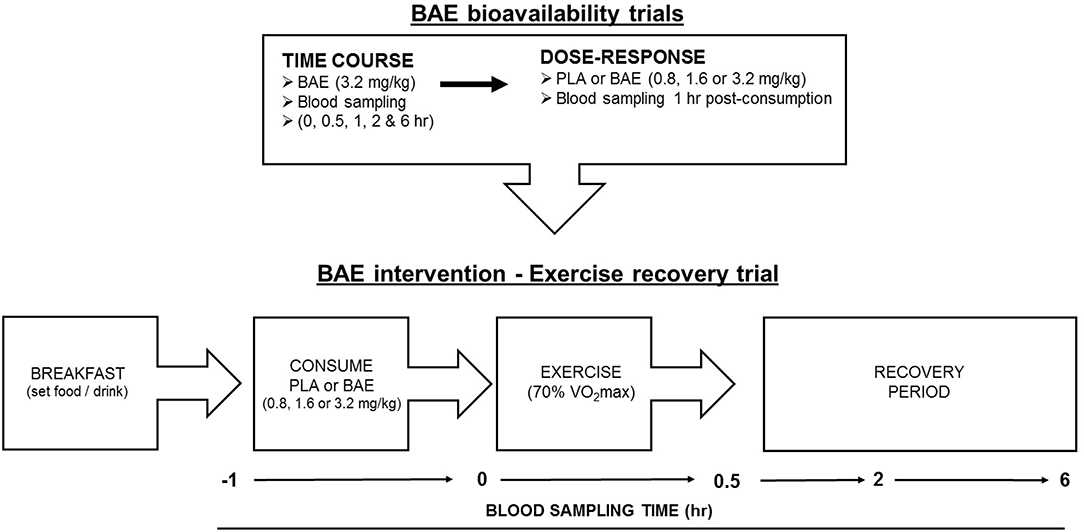
Figure 1. Flow chart of study design involving blackcurrant anthocyanin-rich extract (BAE) bioavailability (time course and dose-response) trials and BAE intervention - exercise recovery trial. PLA = placebo.
Blood Processing
(i) Bioavailability trials. Collected blood samples were immediately centrifuged (300 g, room temperature [RT], 10 min) and the plasma acidified with 5% trifluoroacetic acid and stored at −80°C until liquid chromatography-mass spectrometry (LC-MS) analysis.
(ii) Intervention trial. Blood collected was used to assess blood lactate (Arkray Lactate biosensor Pro™ 2, Baden, Swizerland), circulating neutrophil status, or centrifuged (300 g, RT, 10 min) and the plasma frozen at −80°C for future measurement of oxidative, antioxidant, and inflammatory indices.
Plasma Biochemical Measures
Anthocyanin Bioavailability
Plasma anthocyanins were measured by LC-MS analysis as previously described by Watson et al. (43). Malvidin-3-galactoside (not present in blackcurrants) was used as an internal standard (IS) and data was calculated as total anthocyanins nMequ.
Oxidative Stress Parameters
Plasma oxidative capacity and protein carbonyl levels were used to evaluate exercise-induced oxidative stress.
(i) Oxidative generating capability was assessed using a fluorescence kinetic assay previously described (40). Data were presented as a change in fluorescence intensity (FI) after 5 min (ΔFI5min).
(ii) Protein carbonyls were measured using the modified version of a colorimetric end-point assay previously described (44). Carbonyl levels were calculated as nmol/mg protein.
Antioxidant Measures
Antioxidant capacity was assessed using ferric reducing ability of plasma (FRAP) (45). FRAP was measured against a standard curve of Trolox (Merck NZ), calculated as μM Trolox equivalents and data presented as % pre-exercise values.
Systemic Inflammatory Indices
Plasma C-reactive protein (CRP) was assessed using a specific CRP ELISA kit (R&D systems, Pharmaco, Auckland, New Zealand), calculated as mg/L and presented as % pre-exercise values.
Neutrophil Characteristics and Function
Preparation of Blood Samples
(i) Circulating blood neutrophil levels. Collected blood was lysed (BD-FACS erythrocyte lysis buffer, BD, BioScience, Auckland, New Zealand) and incubated with FITC-CD18 antibodies (BioLegend, San Diego, USA) at RT (in the dark) for 15 min, washed twice and re-suspended in PBS.
(ii) CR3 surface receptor expression. Erythrocyte-lysed blood samples were incubated with FITC-CD18 or PE/Cy7-CD11b antibodies (BioLegend) at RT (in the dark) for 15 min, washed twice and re-suspended in PBS.
(iii) Phagocytic capability. Neutrophil phagocytosis activity was assessed using a modified method described by White-Owen et al (46). Blood samples were incubated with opsonised FITC-conjugated E. coli (Fluorescent BioParticles®, Molecular Probes, Life Technologies) at 37°C for 5 min, erythrocytes lysed and leukocytes incubated with propidium iodide for 10 min. Non-ingested FITC-E. coli were quenched with 10% trypan blue solution immediately prior to flow cytometry analysis.
Flow Cytometry Analysis
All blood samples were prepared for flow cytometry analysis within 2 h of collection. Flow cytometry was performed using a BD FACSCalibur running CellQuest PRO equipped with 488 and 633 nm lasers and four fluorescence detectors. Data files were analyzed following acquisition using FlowJo analysis software as described below
(i) Circulating blood neutrophil levels. Using an initial leukocyte forward (FSC) vs. side (SSC) scatter plot, FITC-CD18 labeled granulocytes were identified (~ 70% of total leukocyte population). Circulating neutrophil levels were then calculated as a % of CD18 positive neutrophils (CD18+) and presented as (a) a single cell scatter plot or (b) expressed as % pre-exercise values in response to exercise and recovery.
(ii) CR3 surface receptor expression. Scatter plots were created to capture CD11b and CD18 expression and used to evaluate changes in combined CD11b/CD18 (CR3+) surface co-expression. CR3+ surface expression on circulating neutrophils were then calculated as % CR3+within a low-moderate and tight high gate (labeled as CR3+high) neutrophil CR3+ population. Results are presented as (a) scatter plots highlighting the two distinct CR3+ neutrophil populations or (b) expressed as % CR3+high neutrophils.
(iii) Phagocytic capability. Application of a scatter plot of blood granulocyte population enabled the selection of FITC positive and negative gates in each subject to determine the shift in FITC-E. coli positive neutrophil population and hence neutrophil phagocytic activity. Results are calculated as (a) scatter plot showing % FITC-E. coli positive and negative neutrophil populations or (b) as % FITC-E. coli positive neutrophils presented as % pre-exercise values.
Statistical Analysis
A one-way ANOVA was applied to analyse the time-dependent plasma anthocyanin bioavailability data sets to examine statistical significance of time within specific individuals. This type of analysis was also applied when analyzing differences in subject physical characteristics and Baêcke questionnaire typical activity scores. Data sets examining multiple comparisons (i.e., dose-dependent plasma anthocyanin bioavailability and nutritional intervention exercise) were assessed by a two-way ANOVA, followed by post hoc paired Student's t-test analysis where appropriate. Comparison between two groups was assessed using a paired Student's t-test. In addition, the original data were transformed (where appropriate) to achieve normality and constant variance in the residuals prior to statistical analysis. Statistical significance for all indices was set at P < 0.05 with a confidence level of 95%.
Results
New Zealand Blackcurrant Anthocyanin Bioavailability Profiles
Time-Dependent Plasma Anthocyanin Bioavailability
All participants showed an increase in plasma anthocyanins as early as 30 min after consuming 3.2 mg/kg BAE (14.6 ± 6.6 nM). This peaked after 2 h, displaying a plasma anthocyanin concentration ranging from 11 to 1,059 nM (Figure 2A).
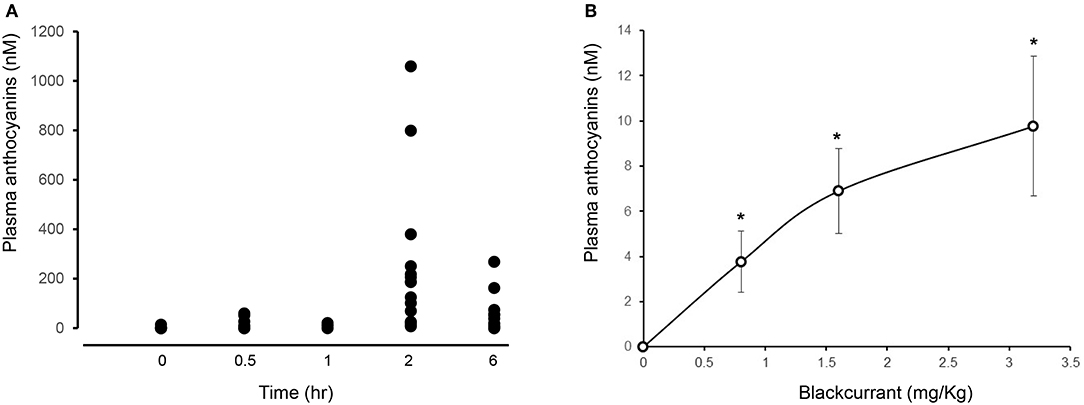
Figure 2. Impact of time (A) and dose (B) of blackcurrant anthocyanin-rich extract (BAE) consumption on plasma anthocyanins. Results are expressed as mean ± SEM of plasma total anthocyanins (nM). *P < 0.05 represents a statistical difference from plasma anthocyanin levels at either (A) time zero or (B) the placebo group.
Dose-Dependent Plasma Anthocyanin Bioavailability
A dose-dependent increase in plasma anthocyanins was observed 1 h after the consumption of BAE (Figure 2B): Participants who had consumed BAE containing 0.8 (3.7 ± 1.3 nM), 1.6 (6.9 ± 1.8 nM), or 3.2 (9.8 ± 3.1 nM) mg/kg anthocyanins showed a significant (P < 0.05) increase in plasma anthocyanins compared to the PLA (0.1 ± 0.3 nM).
Based upon these plasma blackcurrant anthocyanin bioavailability trials, we selected a 1 h pre-feeding intervention strategy, in a pilot trial, to explore the influence of pre-exercise BAE consumption on exercise-induced changes in oxidative stress and innate immunity during recovery.
Exercise Physical Indices, Systemic Antioxidant, and Inflammation Status
Exercise Physical Outcomes
No overall difference was observed in the distance rowed by the trial participants irrespective of intervention group (Figure 3A). Similarly, pre-exercise consumption of BAE had no effect on either baseline heart rates (HR, Figure 3B) or blood lactate (Figure 3C) levels. However, post-exercise HR were significantly (P < 0.05) lower in participants who had consumed 0.8 (126.9 ± 3.5 bpm) or 1.6 (106.6 ± 3.6 bpm) mg/kg BAE compared to the PLA (126.9 ± 3.5 bpm). Furthermore, pre-exercise consumption of 1.6 or 3.2 mg/kg BAE caused a marginal, yet non-significant, 9 and 7% decrease in post-exercise blood lactate levels (Figure 3C); 7.3 ± 1.1 mM (P = 0.16) or 7.5 ± 0.8 mM (P = 0.12), respectively.
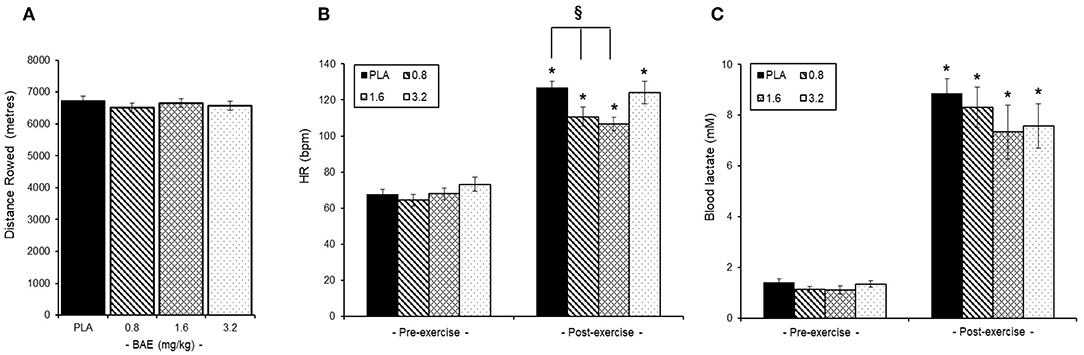
Figure 3. Influence of blackcurrant anthocyanin-rich extract (BAE, mg/kg) consumption 1 h prior to exercise on performance (A), heart rate (B), and blood lactate levels (C). Results are mean ± SEM, n = 8 individuals per group. *P < 0.05 represents a statistical difference from pre-exercise values. §P < 0.05 represents a statistical difference from placebo for the groups highlighted.
Systemic Antioxidant and Inflammation Status
Pre-exercise consumption of BAE had no effect on baseline plasma FRAP or CRP levels and so exercise-induced changes were expressed as % pre-exercise (Figures 4A,B). We found that 30 min exercise in the presence or absence of BAE intervention had no impact upon plasma antioxidant (FRAP) status during exercise recovery (Figure 4A). The 30 min row evoked a transient plasma CRP increase (P < 0.05) in participants who had consumed PLA (1.3 ± 0.7 mg/L), 0.8 (1.4 ± 0.8 mg/L), or 1.6 (1.1 ± 0.4 mg/L) mg/kg BAE, which returned to pre-exercise levels after 2 h post-exercise recovery. No change in plasma CRP levels were observed in participants who had consumed 3.2 mg/kg BAE 1 h prior to the exercise.
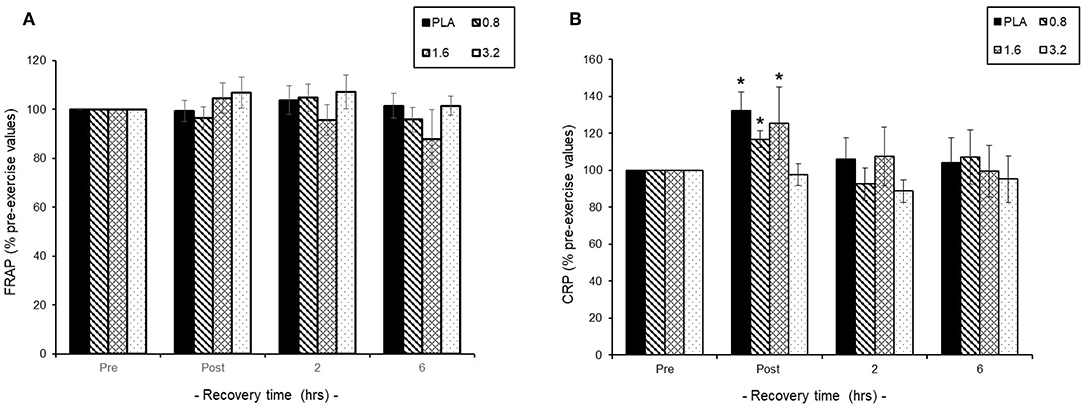
Figure 4. Effect of blackcurrant anthocyanin-rich extract (BAE, mg/kg) consumption 1 h prior to exercise on (A) plasma ferric reducing ability of plasma (FRAP) and (B) C-reactive protein (CRP). Results are expressed as mean ± SEM % pre-exercise values, n = 8 individuals per group. *P < 0.05 represents a statistical difference from pre-exercise CRP levels, respectively, within each treatment group.
Recovery From Exercise-Induced Oxidative Stress
Plasma Oxidative Capacity
Pre-exercise consumption of BAE had a differential influence on both baseline and post-exercise recovery plasma oxidative capacity (Figure 5A). Consumption of either PLA or 0.8 mg/kg BAE 1 h prior to exercise displayed similar plasma oxidative capability; 2491 ± 396 vs. 2129 ± 396 ΔFI5mins, respectively. In contrast, consumption of 1.6 or 3.2 mg/kg BAE prior to exercise showed elevated pre-exercise plasma oxidative capability compared to PLA; 3430 ± 817 or 3500 ± 379 ΔFI5mins, respectively. This increase in plasma oxidative capability within the 1.6 and 3.2 mg/kg BAE groups was still evident immediately post-exercise but by 2 h recovery was lower than post-exercise values. Furthermore, after 6 h post-exercise recovery, the plasma oxidative capability of participants in both the 1.6 and 3.2 mg/kg BAE intervention groups were significantly (P < 0.01) lower than their respective post-exercise values as well as those in the PLA group.
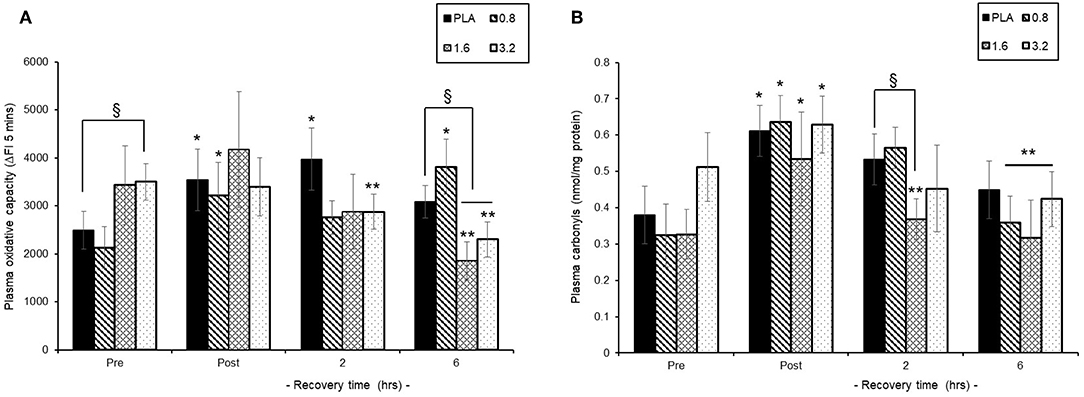
Figure 5. Ability of blackcurrant anthocyanin-rich extract (BAE, mg/kg) consumption 1 h prior to exercise to influence plasma oxidative capability (A) and protein carbonyl levels (B) during post-exercise recovery. Results are mean ± SEM, n = 8 individuals per group. *P < 0.05 and **P < 0.05 represents a statistical difference from pre- and post-exercise values, respectively, for each intervention group. §P < 0.05 represents a statistical difference from placebo for the BAE groups highlighted.
Plasma Protein Carbonyl Levels
Trial participants who consumed 0.8 or 1.6 mg/kg BAE prior to exercise showed a similar pre-exercise plasma carbonyl level to those in the PLA group (Figure 5B), whereas carbonyl levels in the 3.2 mg/kg BAE group was 37% higher. Plasma carbonyls measured immediately post-exercise were elevated (P < 0.05) in PLA (0.61 ± 0.07 nmol/mg protein), 0.8 (0.63 ± 0.07 nmol/mg protein) and 1.6 (0.53 ± 0.12 nmol/mg protein) mg/kg BAE groups and now similar to the levels measured in the 3.2 (0.63 ± 0.07 nmol/mg protein) mg/kg BAE group. However, by 2 h recovery, participants in the 1.6 and 3.2 mg/kg BAE groups showed a 32 and 27%, respective decline in post-exercise plasma carbonyl levels compared to those observed in the PLA group. After 6 h recovery, a decline in plasma carbonyls was observed in all intervention groups, with levels in the 0.8, 1.6, and 3.2 mg/kg BAE groups being significantly (P < 0.05) lower than post-exercise levels.
Maintenance of Circulating Neutrophil Status and Function in Response to Exercise and Recovery
Circulating Neutrophil Population
An initial representative scatter plot (Figure 6A, I) of FITC-CD18 labeled leukocytes showed that 78% of the leukocyte population exhibited CD18 surface expression (CD18+). Further gating of this population enable separation of a discrete CD18+ neutrophil population; 68% (Figure 6A, II) that was then used to evaluate changes in the circulating neutrophil population. Pre-exercise consumption of BAE had no effect on the blood CD18+ neutrophil population and so changes during exercise recovery were evaluated as % pre-exercise levels (Figure 6B). A transient decline in circulating CD18+ neutrophils was observed immediately after exercise (post-exercise) in PLA, 0.8 and 1.6 mg/kg BAE groups. In contrast, by 2 h post-exercise recovery a significant (P < 0.01) increase in blood CD18+ neutrophils was observed in all treatment groups: PLA (23 ± 4%), 0.8 (26 ± 4%), 1.6 (26 ± 3%) and 3.2 (24 ± 7%) mg/kg BAE. This was still evident in the PLA and 0.8 mg/kg BAE groups after 6 h recovery, whereas circulating CD18+ neutrophil levels in participants who had consumed 1.6 or 3.2 mg/kg BAE had returned to pre-exercise values.
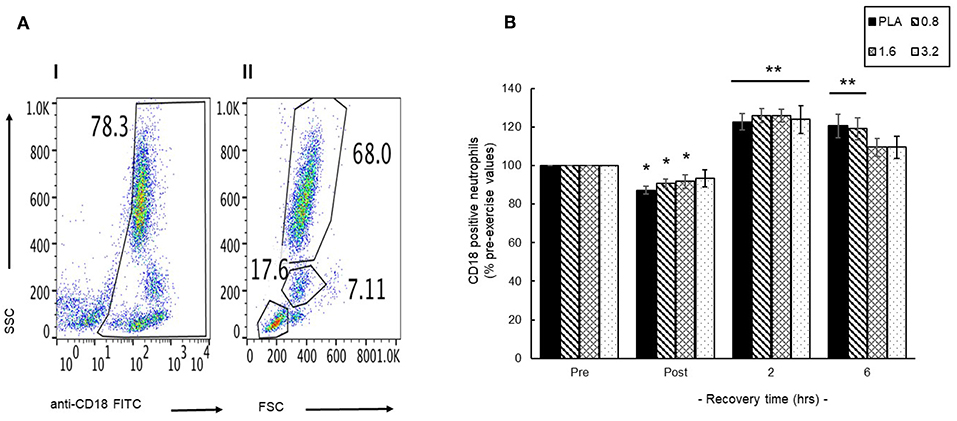
Figure 6. Identification of a blood CD18-labeled neutrophil population (A) and the influence of pre-exercise consumption of blackcurrant anthocyanin-rich extract (BAE, mg/kg) on the distribution of circulating neutrophils during exercise recovery (B). Results are presented as either (A) scatter plots (I /II) highlighting selection of the CD18 neutrophil population or (B) mean ± SEM % pre-exercise values, n = 8 individuals per group. *P < 0.05 and **P < 0.05 represents a statistical difference from pre- and post-exercise circulating neutrophil values, respectively, for each intervention group.
Neutrophil CR3+ Expression
The temporal distribution of CR3+ surface expression in circulating neutrophils in a representative scatter plot (Figure 7A) revealed that following 6 h post-exercise recovery, 88.6% of circulating neutrophils were CR3+high compared to only 2.7% in pre-exercise blood neutrophils. Since CR3+ surface expression is important for neutrophil phagocytosis, we focused on the efficacy of timed BAE consumption on the expression profile of CR3+high neutrophils in response to exercise and recovery (Figure 7B). A significant (P < 0.01) increase in the CR3+high neutrophils was observed in the PLA group (31.3 ± 10.4 %) compared to pre-exercise levels (2.9 ± 1.0 %). This increase in CR3+high neutrophils was also observed in BAE intervention groups, where a dose-dependent increase in circulating CR3+high neutrophils compared to pre-exercise levels was observed (Figure 7B): In addition, pre-exercise consumption of 3.2 mg/kg BAE caused an increase in CR3+high neutrophils (72.1 ± 5.7 %) that was greater (P < 0.01) than that observed in the PLA group.
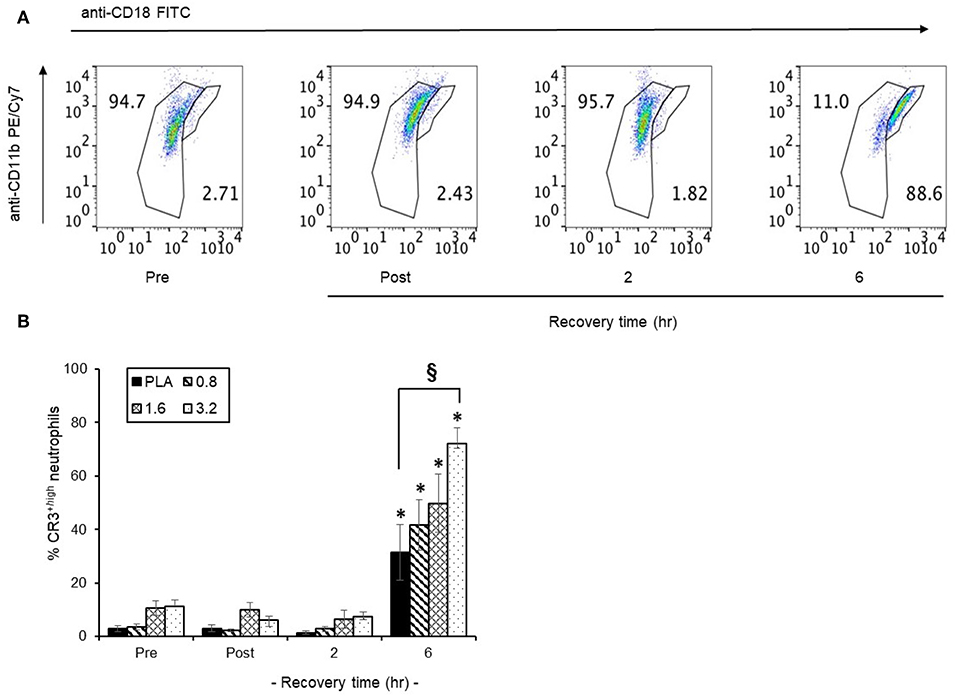
Figure 7. Influence of blackcurrant anthocyanin-rich extract (BAE, mg/kg) consumption 1 h prior to exercise on neutrophil complement receptor 3 (CR3+) surface expression during exercise recovery. Results are presented as CD18 neutrophil populations exhibiting CR3+ surface expression in the form of (A) a series of scatter plots or (B) mean ± SEM % CR3+high neutrophils, n = 8 individuals per group, *P < 0.01 represents a statistical difference from respective pre-exercise values for each intervention group. §P < 0.01 represents a statistical difference from the placebo group.
Phagocytic Capability of Circulating Neutrophils
In a representative scatter plot (Figure 8A), we found that baseline phagocytosis (90.4 %) of FITC-E. coli by blood neutrophils became lower (86.4%) following a 30 min row, and returned to pre-exercise levels (92.1%) by 6 h recovery. We applied this model to examine the efficacy of pre-exercise BAE consumption on the phagocytic capability of circulating neutrophils during exercise recovery. Baseline blood neutrophil phagocytosis capability was similar in all intervention groups and so results are presented as % pre-exercise values (Figure 8B). In the PLA group, exercise caused a gradual decline in the ability of blood neutrophils to phagocytosis opsonised FITC-E. coli during recovery, which by 6 h was 17% (P < 0.05) lower than pre-exercise values. Pre-exercise consumption of BAE exhibited a dose-dependent ability to preserve neutrophil phagocytic capability during post-exercise recovery. Whilst a 12% (P < 0.01) drop in blood neutrophil phagocytic capability was observed 6 h post-exercise in the 0.8 mg/kg BAE group, no decrease was observed in the 1.6 or 3.2 mg/kg BAE intervention groups: By 6 h post-exercise recovery, blood neutrophil phagocytosis capability within these intervention groups was still 97.4 ± 4.4 and 110.5 ± 6.4 % pre-exercise values, respectively, and a significantly different (P < 0.05) phagocytosis capability was observed in the PLA and 0.8 mg/kg BAE groups.
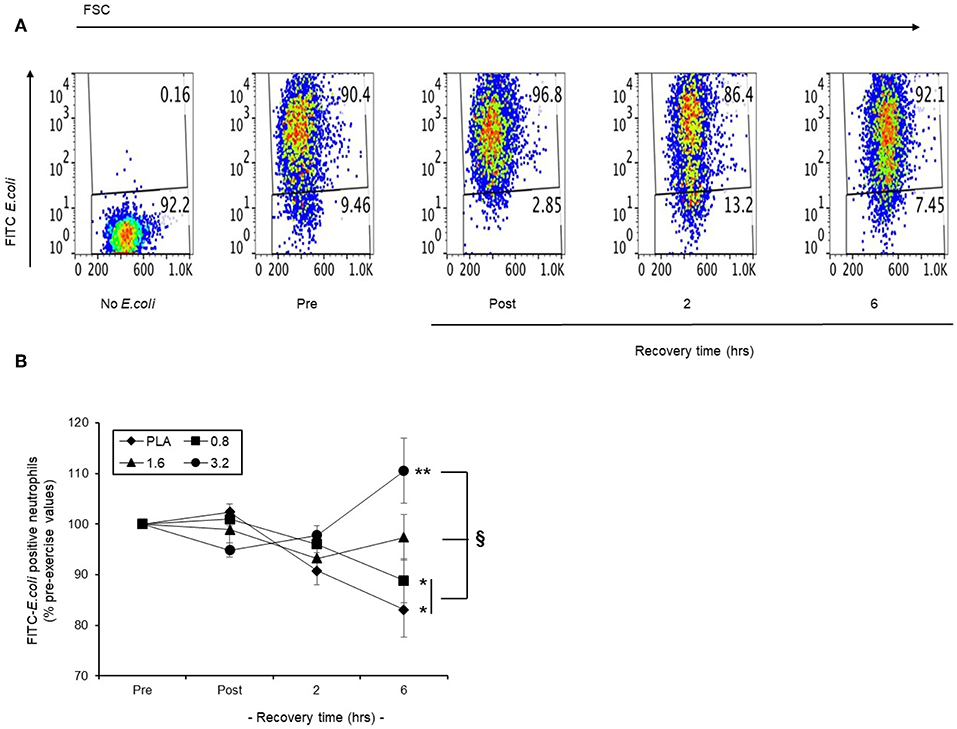
Figure 8. Capability of pre-exercise blackcurrant anthocyanin-rich extract (BAE, mg/kg) consumption to preserve neutrophil phagocytic activity during exercise recovery. Results are presented as the distribution of FITC-E.coli positive neutrophils in the form of (A) a series of scatter plots or (B) mean ± SEM % pre-exercise values, n = 8 individuals per group. *P < 0.05 represents a statistical difference from pre-exercise values for each intervention group. §P < 0.05 represents a statistical difference from the placebo group.
Discussion
The data reported here as a whole demonstrate that a single, timed consumption of BAE dose-dependently prior to exercise supported the recovery from exercise-induced oxidative stress, and maintained neutrophil function, thus reducing the risk for opportunistic infections. Previous studies show that the consumption of anthocyanin rich foods/supplements results in a rapid increase in plasma anthocyanin concentration, usually peaking 1–2 h post-consumption (47, 48). This early discrete plasma bioavailability profile enables the nutritional efficacy of these anthocyanins to be evaluated in short-term intervention studies. In this current study we found a time-dependent increase in plasma anthocyanins following the consumption of an anthocyanin extract from 3.2 mg/kg total anthocyanins that peaked at 2 h. In contrast, Matsumoto et al. (49) reported that plasma anthocyanin levels after the consumption of a New Zealand blackcurrant extract (containing 17 mg/kg anthocyanins) peaked at 1 h post-consumption. The temporal bioavailability profile of the plasma anthocyanins and their concentrations were similar to our findings here. The differences in the time of the peak in plasma anthocyanin levels observed between these two studies is unclear and may be due to a number of factors including the different chemical anthocyanin compositions of the extracts, plasma anthocyanin extraction and analyses methods used, the amount of anthocyanins consumed by trial participants, and variation in the absorption kinetics by different participant cohorts (47). In our study, the peak plasma anthocyanin bioavailability at 2 h post-consumption was similar in both studies irrespective of the initial blackcurrant extract dose consumed. However, despite the reported anthocyanin bioavailability differences, the findings from our current study and that of others (36, 49) demonstrate a close relationship between optimal plasma anthocyanin bioavailability and functional efficacy.
It is still debatable whether or not consuming foods that exhibit powerful inherent antioxidant properties actually results in increased human plasma antioxidant capacity that is capable of attenuating oxidative stress resulting from exercise (2). In this pilot study, we found that the consumption of the BAE had no impact on overall plasma antioxidant capacity prior to, or during recovery from, a 30 min rowing exercise at 70% VO2max. Our preliminary findings are supported by Morillas-Ruiz et al. (50), who demonstrated that the ingestion of a fruit drink enriched in polyphenolics 15 min prior to a 90 min cycle (70% VO2max) had no effect on overall plasma antioxidant status despite mediating a significant decrease in post-exercise plasma protein carbonyls. These results indicate that the facilitated recovery from exercise-induced oxidative stress is unlikely to be due to the inherent antioxidant properties of the ingested anthocyanin extract. Others have shown that similar plasma levels of anthocyanins did not exhibit any antioxidant properties in chemical and in vitro antioxidant studies (22, 23). However, Matsumoto et al. (51) showed that the consumption of a blackcurrant extract containing 33 mg/kg of anthocyanins, exhibited similar plasma anthocyanin concentrations to that found in our current study, and was able to mediate a detectable increase in plasma antioxidant capacity; although a different chemical assay, compared to the one we used in this current study, was used to detect the changes in plasma antioxidant capacity. Alternatively blackcurrant anthocyanins may activate cellular redox-sensitive mechanisms that up-regulate antioxidant systems to increase antioxidant capacity (20, 25, 50). In this pilot trial, we observed an increase in plasma oxidative capacity 1 h following consumption of either 1.6 or 3.2 mg/kg BAE (Figure 4A) prior to exercise that was greater than the placebo group. Since anthocyanins and their metabolites exhibit electrophilic properties (52), it is feasible that the pre-exercise increase in plasma oxidative capability observed may be the consequence of an increase in plasma anthocyanins. This in turn may lead to the activation of cellular redox-sensitive processes, such as nuclear redox factor-2/antioxidant response element (Nrf2/ARE) transcription, and the up-regulation of cellular antioxidant systems that contribute to the lower plasma oxidative stress indices observed during recovery. Berryfruit anthocyanins in particular, have been shown to activate a number of cellular transcription factors, especially the Nrf2 pathway that up-regulate the expression of a number of redox-sensitive cellular defense pathways including redox-sensitive antioxidant enzymes (e.g., HO-1, NQO1) to enhance overall cellular antioxidant capacity (53, 54). Other plant-derived polyphenols have been shown to activate cAMP-respective element (CRE) signaling, which mediates the up-regulation of the master antioxidant reduced glutathione (GSH) (55). It is thought that certain anthocyanin glycosides and/or primary metabolites such as protocatecheuic acid and/or gallic acid indirectly activate cellular Nrf2/ARE via the chemical conversion of diphenols to quinines through auto-oxidation to an electrophile quinine-type molecule or reduce proteasome ubiquitination activity that in turn causes fluctuations in oxidative and/or redox status (52, 56–58). Moreover, recent cell experiments conducted within our group found that blackcurrant anthocyanins enhance tBHQ- and redox (copper II)-activation of Nrf2/ARE transcription using an ARE-luciferase reporter HepG2 cell-line and up-regulate antioxidant enzymes, HO-1 and thioredoxin reductase (data not shown). Our findings support Thoppil et al. (30) who reported in a rat hepatocellular carcinoma study that daily consumption of blackcurrant anthocyanins (100 or 500 mg/kg) for 18 weeks increased the antioxidant capacity via the stress-induced activation of Nrf2/ARE. It is therefore feasible that the auto-oxidation of bioavailable blackcurrant-derived anthocyanins, either alone or in conjunction with exercise-induced ROS (or redox fluctuations), leads to the activation of cellular stress-sensitive signaling mechanisms, such as Nrf2/ARE, enabling the up-regulation of cell antioxidant capacity and the improved recovery from exercise-induced oxidative stress observed in this initial study.
Maintaining functional innate immunity in response to exercise-evoked oxidative stress and acute inflammation, especially during post-exercise recovery, is vital to limiting susceptibility to opportunistic pathogen infection (10–12, 59). Neutrophil recruitment in response to exercise and during recovery is related to a number of factors, including haemodynamic changes, increased cardiac output, circulating stress hormones (e.g., adrenaline) or increased inflammation-driven chemokines, e.g., IL-8, GM-CSF (60–62). This tightly coordinated event creates a dynamic neutrophil population that responds to inflammatory status and so nutritional strategies designed to facilitate exercise recovery, also need to support (and not suppress) flexibility in circulating neutrophil numbers (12). In this pilot study, we found timed consumption of BAE (3.2 mg/kg) prior to exercise attenuated the transient post-exercise decline in circulating neutrophils as well as supported the increase in circulating neutrophils during recovery. Although the underlying mechanism is unknown, it is feasible that the bioavailability of blackcurrant anthocyanins prior to exercise may improve blood flow (36) to support rapid neutrophil influx during recovery (outflux after recovery) and/or maintain the existing neutrophil population through the removal of potential oxidants such as lactic acid (63). Since neutrophils are the first line of defense against microbial infection, it is also important that circulating neutrophils possess and maintain their functional capacity in response to exercise and subsequent recovery. The functionality of circulating neutrophils in response to exercise is influenced by fluctuations in intracellular ROS—redox status (64, 65) and neutrophil phagocytic function in response to exercise and during recovery is dependent upon the intensity and duration of the exercise and the fitness of the individual (66–68). The 30 min row at 70% VO2max employed in our study caused a time-dependent decrease in blood neutrophil phagocytosis (opsonised E. coli) capability during post-exercise recovery. A similar decrease in neutrophil function has been observed in other exercise models (59, 65–67), with is being attributed to exercise-induced oxidative damage within the neutrophil, resulting in a loss of antioxidant defense mechanisms causing defective chemotaxis and phagocytosis. Here, in this preliminary study, we found that the consumption of the BAE (1.6 or 3.2 mg/kg) 1 h prior to exercise prevented the decline in neutrophil phagocytic capability. The master antioxidant regulator, Nrf2 is highly expressed in neutrophils enabling the leukocyte to respond quickly to changes in oxidative and redox status by up-regulating its antioxidant status (69). Since plant polyphenols, including anthocyanins (20, 25, 50) activate cellular Nrf2/ARE transcription (direct or indirect mechanism), it is conceivable that by timing the plasma bioavailability of blackcurrant anthocyanins prior to exercise may facilitate the up-regulation of neutrophil antioxidant defenses (i.e., priming event) to preserve functionality against exercise-induced oxidative stress, however a large study is required to support this hypothesis. This notion, however, is supported in a study by Suzuki (65) who suggested that certain polyphenols may enhance neutrophil antioxidant capacity leading to a retention of their functionality. Furthermore, acute exercise has been shown to influence neutrophil function via altering functional membrane receptor profiles of circulating neutrophils (60, 61, 66, 70). Complement receptors (CRs), CIqR, CR1, CR3 (CD11b/CD18) and CR4 (CD11c/CD18), expressed on the surface of neutrophils efficiently recognize microbes bound with complement components. These surface receptors are sensitive to fluctuations in exercise-induce changes in oxidative and redox status, which can result in either a down-regulation or membrane cleaving resulting in loss of neutrophil function (71). Since CR3 expression is critical for phagocytosis it becomes important to maintain expression levels of CR3 during exercise recovery to minimize susceptibility to infection. In our current pilot study, we found the consumption of BAE caused a dose-dependent enhancement in the surface expression of CR3 during post-exercise recovery, which paralleled the increased phagocytosis capability observed 6 h post-exercise. These preliminary findings are supported by Pizza et al. (72) who demonstrated that neutrophil and monocyte CR3 and CD64 expression together with neutrophil functionality fluctuated over 96 h during adaptation to eccentric exercise; an increase in post-exercise neutrophil CR3 was observed after 6 h recovery following the first eccentric exercise bout. In contrast, Gavrieli et al. (68), found no change in neutrophil CR3 surface expression 24 h after the completion of a 30 min treadmill run (75% VO2max) in physically active males. Despite the exercise-induced differences in neutrophil receptor/function relationship, regular exercise, especially as we age (70), is generally shown to preserve circulating neutrophil numbers and function, including maintaining the surface expression of CR3, for appropriate anti-microbial chemotaxis, phagocytosis and oxidative burst function. Whilst our current findings suggest that timing the bioavailability of blackcurrant anthocyanins prior to a moderate exercise (i.e., consuming ≥ 1.6 mg/kg BAE 1 h prior to exercise) preserves innate neutrophil immunity reducing the susceptibility to opportunistic microbial infection during post-exercise recovery, a larger human study is required to define efficacy.
In conclusion, it is becoming apparent that the beneficial outcomes of anthocyanin supplementation on innate immunity (including alleviating oxidative stress), may be tailored to an individual's needs. Nutritional studies report that regular consumption of foods high in polyphenols protect against and alleviate the inflammatory symptoms of cardiovascular disease, type 2 diabetes, obesity and other inflammation-driven diseases (73, 74). Further, timed consumption of these foods/supplements, demonstrated in this study and by others (25, 33, 75) can support exercise recovery and maintain natural immune defenses, which is independent of the food products inherent antioxidant property. Although the cellular mechanisms are currently unconfirmed, it is most likely to involve the modulation of innate immune systems (via the activation of cellular stress and/or redox sensitive processes) such as antioxidant; Nrf2/ARE (53), inflammation regulation; Nuclear factor (NF)-κB, Activator protein-1 (AP-1) (34, 73, 76) and/or immune homeostasis; Forkhead box class O (FOXO) (77) signaling, rather than through the direct antioxidant properties of anthocyanins. Moreover, the potential adaptive action of plant polyphenols to maintain innate immunity is supported by others (20, 25, 50), including a study by McAulty et al (75) who found that the consumption of blueberries 2 h prior to exercise facilitated recovery from exercise-induced oxidative stress and natural killer cell function. Our preliminary findings support the inclusion of appropriately timed and dosed nutritional intervention of ≥ 1.6 mg/kg BAE to aid post-exercise recovery and contributes to on-going meta-analysis collection that pre-exercise consumption of anthocyanin-rich foods may facilitate exercise recovery from oxidative stress and preserve innate immune function.
Ethics Statement
The human trials conducted in this study were approved by either the Northern (NTY/09/10/105, Hamilton) or Central (CEN/09/11/088, Wellington) Ethical Regional Committees of New Zealand.
Author Contributions
RH, KL, and SH designed the study and gained human ethical approval from Human Disability Ethics Committees (HDEC). RH, KL, and RW recruited the participants and performed the human trials. SH, KL, JR, AP, RW, JC, and DJ carried out the experimental analysis on collected blood samples and the interpretation of results. KL, SH, and RW developed and performed the biochemical assays. JR and AP designed, performed, and analyzed flow cytometry experiments. JC and DJ developed and performed the anthocyanin LC-MS analysis. RH, NB, and SH drafted the manuscript. JR, AP, and JC revised the manuscript critically for important intellectual content. SH approved the final version of the manuscript submitted.
Funding
Financial support for this study was provided by a New Zealand Ministry for Business Innovation and Employment (MBIE) program (C06X0807) led by RH, entitled New Healthy and Flavorsome Berries and Products (2008-2013).
Conflict of Interest Statement
The authors are employees of the New Zealand Institute for Plant and Food Research Ltd which has no royalty agreement associated with sales of New Zealand blackcurrant products. The authors declare that there is no individual personal financial relationship. The data sets for this manuscript are not publicly available because of commercial sensitivity. Requests to access the datasets should be directed to the corresponding author.
Acknowledgments
The authors thank the individuals within Plant and Food Research and the wider community who kindly agreed to be participants in the various trials. We also thank Drs. David Stevenson, Chris Clark and Dawei Deng, and Ms. Selena Holmes for their expert technical advice and support as well as Dr. Lee Huffman and the Food Solutions team of Plant and Food Research for overseeing the food safe packaging of both the blackcurrant anthocyanin extract and sugar placebo into capsules. The authors thank Drs Odette Shaw and Gunaranjan Paturi, for their constructive guidance on the construction of the manuscript.
References
1. Meo SD, Reed TT, Venditti P, Victor VM. Role of ROS and RNS sources in physiological and pathological conditions. Oxid Med Cell Longev. (2016) 2016:1245049. doi: 10.1155/2016/1245049
2. Merry TL, Ristow M. Do antioxidant supplements interfere with skeletal muscle adaptation to exercise training? J Physiol. (2016) 594:5135–47. doi: 10.1113/JP270654
3. Powers SK, Jackson MJ. Exercise-induced oxidative stress: cellular mechanisms and impact on muscle force production. Physiol Rev. (2008) 88:1243–76. doi: 10.1152/physrev.00031.2007
4. Gomez-Cabrera MC, Domenech E, Romagnoli M, Arduini A, Borras C, Pallardo FV, et al. Oral administration of vitamin C decreases muscle mitochondrial biogenesis and hampers training-induced adaptations in endurance performance. Am J Clin Nutr. (2008) 87:142–9. doi: 10.1093/ajcn/87.1.142
5. Ristow M, Zarse K, Oberbach A, Kloting N, Birringer M, Kiehntopf M, et al. Antioxidants prevent health-promoting effects of physical exercise in humans. Proc Natl Acad Sci USA. (2009) 106:8665–70. doi: 10.1073/pnas.0903485106
7. Yang Y, Bazhin AV, Werner J, Karakhanova S. Reactive oxygen species in the immune system. Int Rev Immunol. (2013) 32:249–70. doi: 10.3109/08830185.2012.755176
8. Nieman DC, Henson DA, Austin MD, Brown VA. Immune response to a 30 min walk. Med Sci Sports Exerc. (2005) 37:57–62. doi: 10.1249/01.MSS.0000149808.38194.21
9. Nieman DC, Henson DA, Austin MD, Sha W. Upper respiratory tract infection is reduced in physically fit and active adults. Br J Sports Med. (2011) 45:987–92. doi: 10.1136/bjsm.2010.077875
11. Schwellnus M, Soligard T, Alonso JM, Bahr R, Clarsen B, Dijkstra HP, et al. How much is too much? (Part 2) International Olympics Committee consensus statement on load in sport and risk of illness. Br J Sports Med. (2016) 50:1043–52. doi: 10.1136/bjsports-2016-096572
12. Nieman DC, Mitmesser SH. Potential impact of nutrition on immune system recovery from heavy exertion: a metabolomics perspective. Nutrients. (2017) 9:513. doi: 10.3390/nu9050513
13. Benestad HB, Laerum OD. The neutrophilic granulocyte. Curr Topics Pathol. (1989) 79:7–36. doi: 10.1007/978-3-642-73855-5_2
14. Calder PC. Feeding the immune system. Proc Nutr Soc. (2013) 72:299–309. doi: 10.1017/S0029665113001286
15. Rankin P, Landy A, Stevenson E, Cockburn E. Milk: an effective recovery drink for female athletes. Nutrients. (2018) 10:228. doi: 10.3390/nu10020228
16. Beelen M, Burke LM, Gibala MJ, van Loon L JC. Nutritional strategies to promote postexercise recovery. Int J Sport Nutr Exerc Metab. (2010) 20:515–32.
17. Myburgh KH. Polyphenol supplementation: benefits for exercise performance or oxidative stress? Sports Med. (2014) 44:S57–70. doi: 10.1007/s40279-014-0151-4
18. McLeay Y, Stannard S, Houltham S, Starck C. Dietary thiols in exercise: oxidative stress defence, exercise performance, and adaptation. J Int Soc Sports Nutr. (2017) 14:12. doi: 10.1186/s12970-017-0168-9
19. Yavari A, Javadi M, Mirmiran P, Bahadoran Z. Exercise-induced oxidative stress and dietary antioxidants. Asian J Sports Med. (2015) 6:e24898. doi: 10.5812/asjsm.24898
20. Mankowski RT, Anton SD, Buford TW, Leeuwenburgh C. Dietary antioxidants as modifiers of physiologic adaptations to exercise. Med Sci Sports Exerc. (2015) 47:1857–68. doi: 10.1249/MSS.0000000000000620
21. Csepregi K, Neugart S, Schreiner M, Hideg E. Comparative evaluation of total antioxidant capacities of plant polyphenols. Molecules. (2016) 21:e208. doi: 10.3390/molecules21020208
22. Diaconeasa Z, Leopold L, Rugina D, Ayvaz H, Socaciu C. Ant-proliferative and antioxidant properties of anthocyanin rich extracts from blueberry and blackcurrant juice. Int J Mol Sci. (2015) 16:2352–65. doi: 10.3390/ijms16022352
23. Huang W, Zhu Y, Li C, Sui Z, Min W. Effect of blueberry anthocyanins malvidin and glycosides on the antioxidant properties in endothelial cells. Oxid Med Cell Longev. (2016) 2016:1591803. doi: 10.1155/2016/1591803
24. McLeay Y, Barnes MJ, Mundel T, Hurst SM, Hurst RD, Stannard SR. Effect of New Zealand blueberry consumption on recovery from eccentric exercise-induced muscle damage. Int Soc Sports Nutr. (2012) 9:19. doi: 10.1186/1550-2783-9-19
25. Trinity JD, Pahnke MD, Trombold JR, Coyle EF. Impact of polyphenol antioxidants on cycling performance and cardiovascular function. Nutrients. (2014) 6:1273–92. doi: 10.3390/nu6031273
26. Hussain T, Tan B, Yin Y, Blachier F, Tossou MC, Rahu N. Oxidative stress and inflammation: what polyphenols can do for us? Oxid Med Cell Longev. (2016) 2016:7432797. doi: 10.1155/2016/7432797
27. Yoon JH, Baek SJ. Molecular targets of dietary polyphenols with anti-inflammatory properties. Yonsei Med J. (2005) 46:585–96. doi: 10.3349/ymj.2005.46.5.585
28. Kevers C, Pincemail J, Defraigne JO, Dommes J. Antioxidant capacity of small dark fruits: influence of cultivars and harvest time. J Berry Res. (2014) 4:97–105. doi: 10.3233/JBR-140071
29. Lee SG, Vance TM, Man TG, Kim DO, Koo SI, Chun OK. Contribution of anthocyanin composition to total antioxidant capacity of berries. Plant Foods Hum Nutr. (2015) 70:427–32. doi: 10.1007/s11130-015-0514-5
30. Thoppil RJ, Bhatia D, Barnes KF, Haznagy-Radnai E, Hohmann J, Darvesh AS, et al. Black currant anthocyanins abrogate oxidative stress through Nrf2-mediated antioxidant mechanisms in a rat model of hepatocellular carcinoma. Curr Cancer Drug Targets. (2012) 12:1244–57. doi: 10.2174/156800912803987968
31. Edirisinghe I, Banaszewski K, Cappozzo J, McCarthy D, Burton-Freeman BM. Effect of blackcurrant anthocyanins on the activation of endothelial nitric oxide synthase (eNOS) in vitro in human endothelial cells. J Agric Food Chem. (2011) 59:8616–24. doi: 10.1021/jf201116y
32. Kim B, Bae M, Park YK, Ma H, Yuan T, Seeram NP, et al. Blackcurrant anthocyanins stimulated cholesterol transport via post-transcriptional induction of LDL receptor in Caco-2 cells. Eur J Nutr. (2018) 57:405–15. doi: 10.1007/s00394-017-1506-z
33. Lyall KA, Hurst SM, Cooney J, Jensen D, Lo K, Hurst RD, et al. Short-term blackcurrant extract consumption modulates exercise-induced oxidative stress and lipopolysaccharide-stimulated inflammatory responses. Am J Physiol Regul Integr Comp Physiol. (2009) 297:R70–81. doi: 10.1152/ajpregu.90740.2008
34. Karlsen A, Retterstøl L, Laake P, Paur I, Kjølsrud-Bøhn S, Sandivk L, et al. Anthocyanins inhibit nuclear factor-kappaB activation in monocytes and reduce plasma concentrations of pro-inflammatory mediators in healthy adults. J Nutr. (2007) 137:1951–4. doi: 10.1093/jn/137.8.1951
35. Moller P, Loft S, Alfthan G, Freese R. Oxidative DNA damage in circulating mononuclear blood cells after ingestion of blackcurrant juice or anthocyanin-rich drink. Mutation Res Funda Molec Mech Mutagen. (2004) 551:119–26. doi: 10.1016/j.mrfmmm.2004.02.020
36. Ohguro I, Ohguro H, Nakazawa M. Effects of anthocyanins in blackcurrant on retinal blood flow circulation of patients with normal tension glaucoma. A pilot study. Hirosaki Med J. (2007) 59:23–32. doi: 10.1159/000335961
37. Cook MD, Myers SD, Gault ML, Willems ME. Blackcurrants alters physiological responses and femoral artery diameter during sustained isometric contraction. Nutrients. (2017) 9:e556. doi: 10.3390/nu9060556
38. Willems ME, Meyers SD, Gault ML, Cook MD. Beneficial physiological effects with blackcurrant intake in endurance athletes. Int J Sport Nutr Exerc Metab. (2015) 25:367–74. doi: 10.1123/ijsnem.2014-0233
39. Baecke JA, Burema J, Frijters JE. A short questionnaire for the measurement of habitual physical activity in epidemiological studies. Am J Clin Nutr. (1982) 36:936–42. doi: 10.1093/ajcn/36.5.936
40. Hurst SM, Lyall KA, Hurst RD, Stevenson LM. Exercise-induced elevation in plasma oxidative generating capability augments the temporal inflammatory response stimulated by lipopolysaccharide. Euro J Appl Physiol. (2009) 107:61–72. doi: 10.1007/s00421-009-1099-1
41. Karvonen M, Kentala K, Mustala O. The effects of training on heart rate: a longitudinal study. Ann Med Exp Biol Fenn. (1957) 35:307–15.
42. Swain DP, Abernathy KS, Smith CS, Lee SJ, And Bunn SA. Target heart rates for the development of cardiorespiratory fitness. Med Sci Sports Exerc. (1994) 26:112. doi: 10.1249/00005768-199401000-00019
43. Watson AW, Haskell-Ramsay CF, Kennedy DO, Cooney JM, Trower T, Scheepens A. Acute supplementation with blackcurrant extracts modulates cognitive functioning and inhibits monoamine oxidase-B in healthy young adults. J Funct Foods. (2015) 17:524–39. doi: 10.1016/j.jff.2015.06.005
44. Levine RL, Williams JA, Stadtman ER, Shacter E. Carbonyl assays for determination of oxidatively modified proteins. Methods Enzymol. (1994) 233:346–57. doi: 10.1016/S0076-6879(94)33040-9
45. Benzie IF, Strain JJ. The ferric reducing ability of plasma (FRAP) as a measure of “antioxidant power”: the FRAP assay. Ana Biochem. (1996) 239:70–6. doi: 10.1006/abio.1996.0292
46. White-Owen C, Alexander JW, Sramkoski RM, Babcock GF. Rapid whole blood microassay using flow cytometry for measuring neutrophil phagocytosis. J Clin Microbiol. (1992) 30:2071–6.
47. de Ferrars RM, Czank C, Zhang Q, Botting NP, Kroon PA, Cassidy A, et al. The pharmacokinetics of anthocyanins and their metabolites in humans. Br J Pharmacol. (2014) 171:3268–82. doi: 10.1111/bph.12676
48. McGhie TK, Walton MC. The bioavailability and absorption of anthocyanins: towards a better understanding. Mol Nutr Food Res. (2007) 51:702–13. doi: 10.1002/mnfr.200700092
49. Matsumoto H, Takenami E, Iwasaki-Kurashige K, Osada T, Katsumura T, Hamaoka T. Effects of blackcurrant anthocyanin intake on peripheral muscle circulation during typing work in humans. Eur J Appl Physiol. (2005) 94:36–45. doi: 10.1007/s00421-004-1279-y
50. Morillas-Ruiz J, Zafrilla P, Almar M, Cuevas MJ, López FJ, Abellán P, et al. The effects of an antioxidant-supplemented beverage on exercise-induced oxidative stress: results from a placebo-controlled double-blind study in cyclists. Eur J Appl Physiol. (2005) 95:543–9. doi: 10.1007/s00421-005-0017-4
51. Matsumoto H, Nakamura Y, Hirayama M, Yoshiki Y, Okubo K. Antioxidant activity of black currant anthocyanin aglycons and their glycosides measured by chemiluminesence in a neutral pH region and in human plasma. J Agri Food Chem. (2002) 50:5034–7. doi: 10.1021/jf020292i
52. Dangles O, Fenger J-A. The chemical reactivity of anthocyanins and its consequences in food science and nutrition. Molecules. (2018) 23:1970. doi: 10.3390/molecules23081970
53. Kropat C, Mueller D, Boettler U, Zimmermann K, Heiss EH, Dirsch VM, et al. Modulation of Nrf2-dependent gene transcription by billerry anthocyanins in vivo. Mol Nutr Food Res. (2013) 57:545–50. doi: 10.1002/mnfr.201200504
54. Yan F, Chen Y, Azat R, Zheng X. Mulberry Anthocyanin extract ameliorates oxidative damage in HepG2 cells and prolongs the lifespan of Caenorhabditis elegans through MAPK and Nrf2 pathways. Oxid Med Cell Longev. (2017) 2017:7956158. doi: 10.1155/2017/7956158
55. Zhu W, Jia Q, Wang Y, Zhang Y, Xia M. The anthocyanin cyanidin-3-O-β-glucoside, a flavonoid, increases hepatic glutathione synthesis and protects hepatocytes against reactive oxygen species during hyperglycemia: Involvement of a cAMP-PKA-dependent signaling pathway. Free Rad Biol Med. (2012) 52:314–27. doi: 10.1016/j.freeradbiomed.2011.10.483
56. Vari R, D'Archivio M, Filesi C, Cartenuto S, Scazzocchio B, Santangelo C, et al. Protocatechuic acid induces antioxidant/detoxifying enzyme expression through JNK-mediated Nfr2 activation in murine macrophages. J Nutr Biochem. (2011) 22:409–17. doi: 10.1016/j.jnutbio.2010.03.008
57. Li H, Wu S, Shi N, Lian S, Lin W. Nrf2/HO-1 pathway activation by manganese is associated with reactive oxygen species and ubiquitin-proteasome pathway, not MAPKs signaling. J Appl Toxicol. (2001) 31:690–7. doi: 10.1002/jat.1654
58. Dreiseitel A, Schreier P, Oehme A, Locher S, Rogler G, Piberger HG, et al. Inhibition of proteasome activity by anthocyanins and anthocyanidins. Biochem Biophys Res Com. (2008) 373:57–61. doi: 10.1016/j.bbrc.2008.04.140
59. Lagranha CJ, Levada-Pires AC, Sellitti DF, Procopio J, Curi R, Pithon-Curi TC. The effect of glutamine supplementation and physical exercise on neutrophil function. Amino Acids. (2008) 34:337–46. doi: 10.1007/s00726-007-0560-x
60. Ali S, Ullah F, Jan R. Effects of intensity and duration of exercise on differential leukocyte count. J Ayub Med Coll Abbottabad. (2003) 15:35–7.
61. Quindry JC, Stone WL, King J, Broeder CE. The effects of acute exercise on neutrophils and plasma oxidative stress. Med Sci Sports Exerc. (2003) 35:1139–45. doi: 10.1249/01.MSS.0000074568.82597.0B
62. Pedersen BK, Hoffman-Goetz L. Exercise and the immune system: regulation, integration, and adaptation. Physiol Rev. (2000) 80:1055–81. doi: 10.1152/physrev.2000.80.3.1055
63. Deley G, Guillemet D, Allaert F-A, Babault N. An acute dose of specific grape and apple polyphenols improve endurance performance: a randomized, crossover, double-blind verses placebo controlled study. Nutrients. (2017) 9:917–26. doi: 10.3390/nu9080917
64. Peake J, Suzuki K. Neutrophil activation, antioxidant supplements and exercise-induced oxidative stress. Exerc Immunol Rev. (2004) 10:129–41.
65. Suzuki K. Exhaustive exercise-induced neutrophil-associated tissue damage and possibility of its prevention. J Nanomed Biotherap Discov. (2017) 7:156. doi: 10.4172/2155-983X.1000156
66. Chinda D, Nakaji S, Umeda T, Shimoyama T, Kurakake S, Okamura N, et al. A competitive marathon race decreases neutrophil functions in athletes. Luminescence. (2003) 18:324–9. doi: 10.1002/bio.744
67. Syu GD, Chen HI, Jen CJ. Differential effects of acute and chronic exercise on human neutrophil functions. Med Sci Sports Exerc. (2012) 44:1021–7. doi: 10.1249/MSS.0b013e3182408639
68. Gavrieli R, Ashlagi-Amiri T, Eliakim A, Nemet D, Zigel L, Berger-Archituv S, et al. The effect of aerobic exercise on neutrophil functions. Med Sci Sports Exerc. (2008) 40:1623–8. doi: 10.1249/MSS.0b013e318176b963
69. Joshi N, Werner S. Nrf2 is highly expressed in neutrophils, but myeloid cell-derived Nrf2 is dispensable for wound healing in mice. PLoS ONE. (2017) 12:e0187162. doi: 10.1371/journal.pone.0187162
70. Bartlett DB, Fox O, McNulty CL, Greenwood HL, Murphy L, Sapey E, et al. Habitual physical activity is associated with the maintenance of neutrophil migratory dynamics in healthy older adults. Brain Behav Immun. (2016) 56:12–20. doi: 10.1016/j.bbi.2016.02.024
71. Futosi K, Fodor S, Mócsai A. Neutrophil cell surface receptors and their intracellular signaling transduction pathways. Int Immunopharm. (2013) 17:638–50. doi: 10.1016/j.intimp.2013.06.034
72. Pizza FX, Davis BH, Henrickson SD, Mitchell JB, Pace JF, Bigelow N, et al. Adaptation to eccentric exercise: Effect on CD64 and CD11b/CD18 expression. J Appl Physiol. (1996) 80:47–55. doi: 10.1152/jappl.1996.80.1.47
73. Lee YM, Yoon Y, Yoon H, Park HM, Song S, Yeum KJ. Dietary anthocyanins against obesity and inflammation. Nutrients. (2017) 9:e1089. doi: 10.3390/nu9101089
74. Smith RE, Tran K, Smith CC, McDonald M, Shejwalkar P, Hara K. The role of the Nrf2/ARE antioxidant system in preventing cardiovascular diseases. Diseases. (2016) 4:e34. doi: 10.3390/diseases4040034
75. McAulty LS, Nieman DC, Dumke CL, Shooter LA, Henson DA, Utter AC, et al. Effect of blueberry ingestion on natural killer cell count, oxidative stress, and inflammation prior to and after 2.5 h of running. Appl Physiol Nutr Metab. (2011) 36:976–84. doi: 10.1139/h11-120
76. Lu H, Li J, Zhang D, Stoner GD, Huang C. Molecular mechanism involved in chemoprevention of black raspberry extracts: from transcription factors to their target genes. Nutr Cancer. (2006) 54:69–78. doi: 10.1207/s15327914nc5401_8
Keywords: anthocyanin bioavailability, pilot exercise intervention study, oxidative stress, neutrophil phagocytosis, exercise recovery
Citation: Hurst RD, Lyall KA, Roberts JM, Perthaner A, Wells RW, Cooney JM, Jensen DJ, Burr NS and Hurst SM (2019) Consumption of an Anthocyanin-Rich Extract Made From New Zealand Blackcurrants Prior to Exercise May Assist Recovery From Oxidative Stress and Maintains Circulating Neutrophil Function: A Pilot Study. Front. Nutr. 6:73. doi: 10.3389/fnut.2019.00073
Received: 28 November 2018; Accepted: 01 May 2019;
Published: 29 May 2019.
Edited by:
Joanna Bowtell, University of Exeter, United KingdomReviewed by:
Katsuhiko Suzuki, Waseda University, JapanSophie C. Killer, English Institute of Sport, United Kingdom
Copyright © 2019 Hurst, Lyall, Roberts, Perthaner, Wells, Cooney, Jensen, Burr and Hurst. This is an open-access article distributed under the terms of the Creative Commons Attribution License (CC BY). The use, distribution or reproduction in other forums is permitted, provided the original author(s) and the copyright owner(s) are credited and that the original publication in this journal is cited, in accordance with accepted academic practice. No use, distribution or reproduction is permitted which does not comply with these terms.
*Correspondence: Roger D. Hurst, cm9nZXIuaHVyc3RAcGxhbnRhbmRmb29kLmNvLm56; Suzanne M. Hurst, c3V6YW5uZS5odXJzdEBwbGFudGFuZGZvb2QuY28ubno=