- 1Research Center on Aging, Sherbrooke, QC, Canada
- 2Nestlé Health Science, Lausanne, Switzerland
- 3Department of Medicine, Université de Sherbrooke, Sherbrooke, QC, Canada
Ketones provide an alternative brain fuel and may be neuroprotective in older people. Little is known of how to optimize the ketogenic effect of C8:0–C10:0 medium chain triglyceride supplement (kMCT). Metabolic switching (MS) from glucose to ketones as a fuel may have metabolic benefits but has not been extensively studied in humans. The objective of the present study was to use an 8 h metabolic study day protocol to assess the influence of typical components of MS, including a kMCT supplement, low-carbohydrate meal and meal timing, on blood ketones, glucose, insulin and free fatty acids (FFA). In one test, the effect of age was also investigated. Over the 8 h metabolic study day, two 10 g doses of the kMCT increased the plasma ketone response by 19% while reducing overall glycemia by 12% without altering insulin or FFA levels. Moreover, a single early meal (breakfast but no lunch) potentiated the ketogenic effect of MS over 8 h, compared to a single delayed meal (lunch but no breakfast). Age and the low carbohydrate meal did not affect the ketones response. We conclude that an 8-h test period can be used to assess metabolic changes during short-term MS. kMCT provide a robust short-term increase in ketones and might enhance the metabolic effectiveness of short-term or intermittent fasting as a component of MS.
Introduction
Glucose is the brain's main energy substrate but during fasting or energy restriction, plasma ketones (acetoacetate [AcAc] and β-hydroxybutyrate [BHB]) increase and spare brain glucose utilization (1) in part due to their oxidative efficiency and competition with pyruvate to enter the citric acid cycle (2–4). Ketones uptake by the brain is directly proportional to their plasma concentrations (5) which increases as the liver produces ketones from free fatty acids (FFA) in response to low glucose and insulin induced during dietary energy or carbohydrate restriction (6). Mild to moderate nutritional ketonemia has long been known to be beneficial for the management of severe refractory epilepsy (7). Other neurotherapeutic uses of mild-moderate ketonemia are now being investigated, especially in conditions in which brain glucose utilization deteriorates, such as during aging (8–11). Eight carbon medium chain fatty acids cross the blood brain barrier (12) and have anapleurotic and signaling properties independent of ketonemia (13, 14).
Medium chain triglycerides of 8 and 10 carbons are ketogenic (kMCT) because of their rapid absorption and oxidation (15, 16). kMCT transiently raise plasma ketones to 0.5–1.0 mmol/L when taken as a 10–15 g dietary supplement at meals (10, 17, 18). Plasma ketones increase in a direct dose-response relationship to the oral dose of kMCT consumed (1). Emulsifying or consuming MCT without a meal increases their ketogenic effect (1, 18).
Metabolic switching (MS) involves planned recurrent dietary energy or carbohydrate restriction. Typically, the period of reduced energy/carbohydrate intake eliminates 1–2 meals/day or involves total fasting for one to several days at a time. An exercise regimen and exogenous ketogenic supplement may also be involved. During MS, glucose's contribution to the body's energy requirements declines and that of free fatty acid (FFA) and ketones increases (5). During periods of fasting and prolonged exercise, ketones are an important alternative fuel to glucose for the brain (19) and also peripherally (20). The mild to moderate ketonemia associated with MS may improve metabolic outcomes and perhaps brain health (21, 22).
Despite considerable research in animals, few experimental protocols for MS have been reported in humans. We have previously used an 8-h metabolic study protocol to compare the plasma ketone response after kMCT under different test conditions (18, 23, 24), so we used the same 8-h protocol to assess MS. In the present study, we hypothesized that three central aspects of MS, i.e., carbohydrate in the meal, kMCT, and meal timing, would augment the acute plasma ketone response during an 8-h test. Our objective was to use an 8-h study protocol to assess the ketogenic effect of a commercially available kMCT drink in relation to: (i) the dose of kMCT, (ii) carbohydrate content of the meal, (iii) meal timing (missing breakfast vs. missing lunch), and (iv) age. The kMCT dose was in the range we have used previously and permitted a doubling of the dose with minimal side-effects (10–20 g) in one of the experiments. The effect of meal timing was assessed by extending the overnight fast till mid-day and giving the first meal of the day as lunch. In addition to the plasma ketones, glucose, insulin and FFA were also measured.
Methods
Participants
Ethical approval for this study was obtained from the Research Ethics Committee of the Integrated University Health and Social Services of Eastern Townships—Sherbrooke University Hospital Center, which oversees all human research done at the Research Center on Aging (Sherbrooke, QC, Canada) and functions in accordance with the Declaration of Helsinki. All participants provided written informed consent prior to beginning the study and were recruited from June 2017 to May 2018. They underwent a screening visit, including the analysis of a blood sample collected after a 12 h overnight fast. Exclusion criteria included smoking, diabetes (fasting glucose >7.0 mmol/l and glycated hemoglobin >6.9%), strenuous aerobic exercise more than three times a week, untreated hypertension, dyslipidemia, and abnormal renal, liver, heart or thyroid function. Participants on medications known to affect triglyceride or carbohydrate metabolism (i.e., diuretics, beta-blockers, steroids, insulin sensitizers) were also excluded. This project was prospectively registered on ClinicalTrials.gov (NCT03830268).
Metabolic Tests
The metabolic tests are summarized in Table 1. The kMCT drink was a 20% liquid emulsion (45 g MCT in 225 ml water plus an emulsifier and sweetener) containing 60% octanoic acid and 40% decanoic acid (BetaQuik® VitaFlo, Liverpool, UK). It has a caloric density of 1.7 kcal/mL.
Experimental Design
The protocol involved six separate metabolic studies in young adults, and one comparing young and older adults aged 65 y old. On each metabolic study day, participants arrived at 7:30 a.m. after a 12 h overnight fast and a minimum of 24 h without alcohol intake. A forearm venous catheter was installed, and a baseline blood sample withdrawn. Participants then consumed the kMCT drink with or without a meal. The meal consisted of two pieces of toast with strawberry jam, a piece of cheese, and two scrambled eggs.
To simplify identifying the different tests and reporting the results, we will refer to MS as the tests with kMCT and the midday meal, as distinct from the tests with kMCT and the low carbohydrate (LC) or breakfast meal. To test if the post-prandial increase in blood glucose or insulin influenced changes in plasma ketones after kMCT, we provided an iso-caloric LC meal instead of the regular high carbohydrate meal (Table 2). The LC meal consisted of three pieces of cheese and three scrambled eggs providing 3 g instead of 49 g carbohydrate. A second dose of kMCT was given at noon, with or without a meal depending on the test.
During the MS, participants had no meal or kMCT at T0 followed by the meal alone T4 (MS-CTL) or kMCT but no meal at T0 followed by the meal and kMCT at T4 (MS-kMCT). Water was available ad libitum throughout the test day. Blood samples were taken at baseline (immediately before the intervention) and every 30 min during 8 h with the first post-dose sample being taken 30 min after the kMCT drink was consumed.
Plasma Metabolite Analyses
Blood samples were centrifuged at 2,846 g for 10 min at 4°C and plasma stored at −80°C until analyzed. Plasma BHB and AcAc were measured by an automated colorimetric assay. For AcAc, 25 μL of plasma was mixed with 330 μL of fresh reagent (Tris buffer, pH 7.0, 100 mmol/L, 20 mmol/L sodium oxamate; 0.15 mmol/L NADH and 1 U/mL β-hydroxybutyrate dehydrogenase [BHBDH]). For BHB, the reagent was Tris buffer (pH 9.0; 20 mM sodium oxamate, 1 mmol/L NAD, and 1 U/mL BHBDH). Tris, oxamic acid, DL-BHB sodium salt, Li-AcAc standard, and NAD were purchased from Sigma (St. Louis, MO, USA), NADH, from Roche (Mannheim, Germany), and BHBDH from Toyobo (Osaka, Japan). The change in absorbance at 340 nm between 15 and 120 s after the addition of the reagent was measured on an automated clinical chemistry analyzer (Dimension Xpand Plus; Siemens, Deerfield, IL, USA). The assay was calibrated with freshly diluted standards from frozen aliquots of a 10 mmol/L standard of Li-AcAc or DL-BHB sodium salt, which is stable at −20°C for 2 and 6 months, respectively. Calibrations and quality controls were performed for each assay to ensure the precision of the kits (coefficient of variation between tests 5 ± 1%). Where “ketones” are mentioned in the results, it refers to AcAc and BHB combined.
Plasma glucose and triglycerides (Siemens Medical Solutions USA, Inc., Deerfield, IL, USA) and FFA (Randox Laboratories Limited, West Virginia, USA) were analyzed using commercial kits. Plasma insulin was analyzed by enzyme-linked immunosorbent assay (Alpco Diagnostics Ltd., Salem, NH, USA) with a microplate reader (Victor multi-label plate reader 2030; Perkin Elmer, MA, USA). Glycated hemoglobin was measured by HPLC-723G7, a fully automated high-performance liquid chromatography instrument-reagent system (Tosoh Bioscience, King of Prussia, PA, USA). Plasma insulin was analyzed by ELISA (Alpco, Salem, NH, USA).
Statistical Analysis
All statistical analyses were carried out using SPSS 23.0 software (SPSS Inc., Chicago, IL, USA). On metabolic test days, the test dose of kMCT was given at T0 or T4, so the half-day periods are reported as T0–4 and T4–8 to compare the different timing of the meal and kMCT drink. Areas-under-the-curve (AUC) from T0 to T4 and T4 to T8 were calculated according the trapezoid method (25). The Shapiro-Wilks test demonstrated that the plasma metabolite data were not normally distributed, so results were compared using the non-parametric Friedman's test, and the effect of the treatments was determined in each group using Wilcoxon's signed rank test. The Young and Older groups were treated as independent groups and compared using the Mann-Whitney test. Differences were considered statistically significant at P ≤ 0.05. Graphs were prepared using Prism version 6.0 (GraphPad Software Inc., San Diego, CA, USA). Preliminary analysis of the AcAc and BHB data did not show any major changes in this ratio across the treatments so these data are not reported. All results are given as the mean ± SD.
Results
Six to ten young participants completed all seven metabolic tests (see Table 1 and figure legends). Baseline anthropometry and plasma metabolites corresponded to reference values from the Sherbrooke University Hospital Center (Sherbrooke, QC). No significant difference was found in anthropometric and plasma measures between the Young and Older groups, except for age, glucose and glycated hemoglobin (Table 3), and were within the normal range for age in both groups (26, 27). No adverse events related to the kMCT drink were reported.
Plasma Ketones
Oral intake of a 10 or 20 g dose of kMCT (kMCT10 and kMCT20, respectively) at T0 significantly elevated plasma ketones over about 3 h in a dose-dependent fashion compared to the CTL (Figure 1A). The ketone Cmax was 455 ± 125 and 612 ± 160 μmol/L, for kMCT10 and kMCT20, respectively (P = 0.028). During the CTL test, plasma ketone levels remained between 49 and 97 μmol/L between T0 and T4 but increased to about 400 μmol/L from T6.5 to T8 (Figure 1A). The T0–4 AUCs for kMCT10 and kMCT20 were both significantly higher than the CTL test (P = 0.028; Figure 2). During the MS test, the second dose of kMCT raised plasma ketones over baseline between T4 and T8. The increase in plasma ketones after kMCT10 was essentially independent of the carbohydrate content from the meal between T0 and T4 (Figure 1B, Table 4).
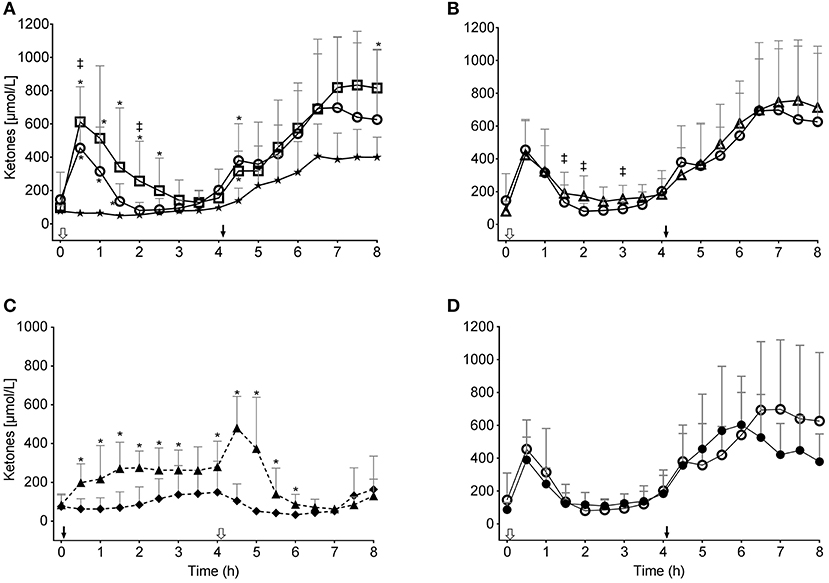
Figure 1. Plasma total ketone (β-hydroxybutyrate and acetoacetate combined) concentration during the tests: (A) without consuming kMCT (CTL, ★) or after 10 g (kMCT10, ) or 20 g (kMCT20, □); (B) after 10 g of kMCT with a carbohydrate-rich (kMCT10,
) or low carbohydrate (kMCT10LC, Δ) meal; (C) after no meal or kMCT at T0 but a meal at T4 (MS-CTL; ⧫– –⧫) or no meal at T0 but a meal plus KMCT10 at T4 (MS-kMCT; ▴– –▴); (D) Older (•) vs. Young (
) after KMCT10 at T0. The black arrow indicates when the meal plus kMCT were consumed and the white arrow indicates when kMCT alone was consumed without the accompanying meal. Values are presented as mean ± SD (n = 6–10). *Difference with CTL; ‡Difference between the different test conditions (P < 0.05).
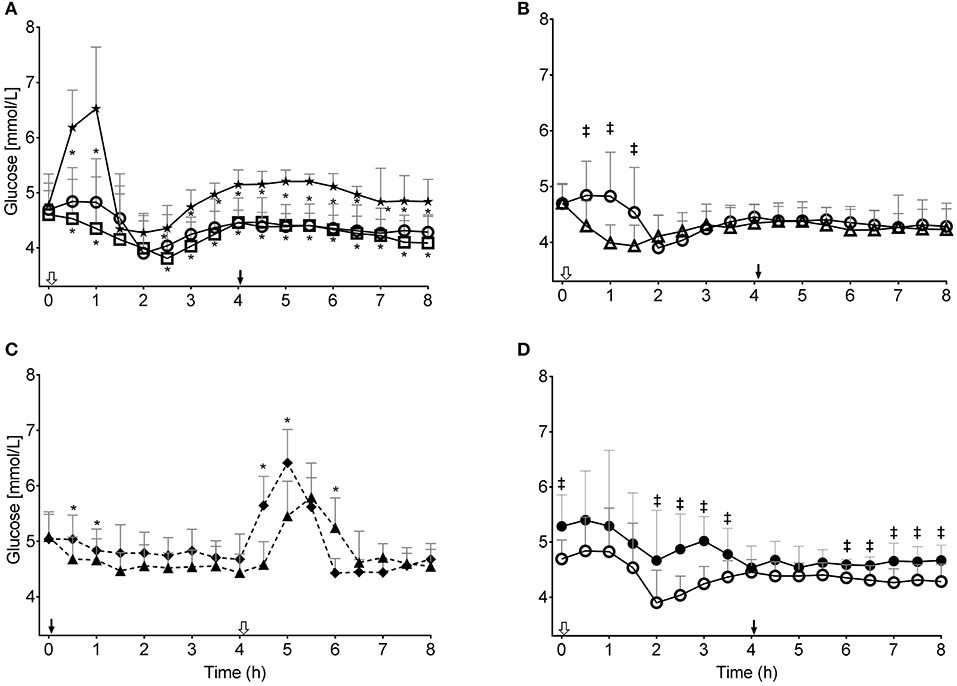
Figure 2. Plasma glucose concentration during the tests: (A) without consuming kMCT (CTL, ★) or after 10 g of kMCT (kMCT10, ) or 20 g of kMCT (kMCT20, □); (B) after 10 g of kMCT with a carbohydrate-rich (kMCT10,
) or low carbohydrate (kMCT10LC, Δ) meal; (C) after no meal or kMCT at T0 but a meal at T4 (MS-CTL; ⧫– –⧫) or no meal at T0 but a meal plus kMCT10 at T4 (MS-kMCT; ▴– –▴); (D) Older (•) vs. Young (
) after kMCT10 at T0. The black arrow indicates when the meal plus kMCT were consumed and the white arrow indicates when kMCT alone was consumed without the accompanying meal. Values are presented as mean ± SD (n = 6–10). *Difference with CTL; ‡Difference between the different test conditions (P < 0.05).
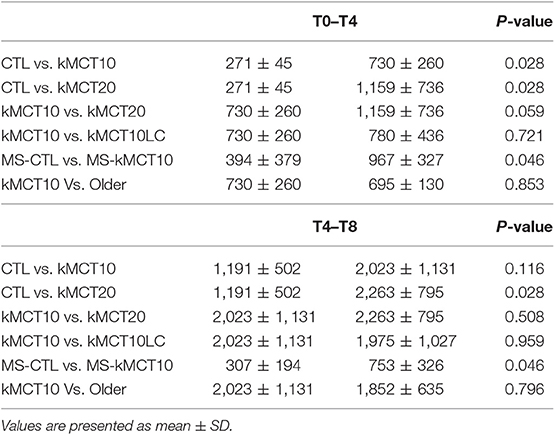
Table 4. Areas-under-the-curve for total ketones (μmol h/L; β-hydroxybutyrate + acetoacetate) during the first 4 h (T0–T4) or the second 4 h (T4–T8) of the metabolic study days (see Table 1 for legend).
The MS-CTL test showed constantly low blood ketones between 33 and 150 μmol/L over 8 h (Figure 1C) and did not display the same ketone increase between T4-T8 as seen with the CTL baseline (Figure 1A). Without the meal at T0, kMCT10 produced a more prolonged increase in ketones up to the meal taken at T4 (MS-kMCT). The second 10 g dose of kMCT in the MS-kMCT10 test transiently raised ketones followed by a rapid decrease to baseline like the change in ketones after the first dose of kMCT. There was no significant difference in plasma ketones in the Young compared to Older group after kMCT10 (Figure 1D, Table 4).
Plasma Glucose
The post-prandial plasma glucose response to a standard meal showed that kMCT10 and kMCT20 reduced glucose values at most time points compared to CTL without a difference between the plasma glucose response to kMCT10 vs. kMCT20 (P < 0.05), and also unaffected by the second dose of kMCT taken at T4 (Figure 2A). The AUC for plasma glucose between T0 and T4 was reduced by 30–42% by concomitant consumption of kMCT10 (Figure 3) or kMCT20. During the MS-kMCT10 test, post-prandial glucose between T4 and T8 was significantly lower than MS-CTL at T4.5 and T5 (P < 0.017), and the Tmax was 30 min later (T 5.5 vs. T5; Figure 2C). The post-prandial glucose response was lower over the first 4 h when the kMCT10 was taken with a LC meal (Figures 2B, 3). The post-prandial glucose response to the standard meal at T0 was significantly higher in the Older vs. the Young group at T0 (fasting), from T2 to T3.5 and from T6 to T8 (Figure 2D; P < 0.05), with a correspondingly higher T0 to T4 AUC in the Older group as well (Figure 3).
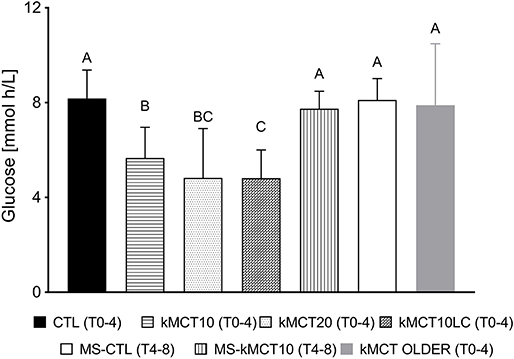
Figure 3. Area-under-the-curve (mmoLh/L) for the post-prandial plasma glucose response at T0-T4 without consuming kMCT (CTL), after 10 g of kMCT (kMCT10), with no meal at T0 but a meal at T4 without kMCT (MS-CTL) and no meal at T0 but a meal at T4 with 10 g of kMCT (MS-kMCT10). The time period of the area-under-the-curve for each bar is shown in brackets. Values are presented as mean ± SD (n = 6–10). Means without a common letter differ significantly (A < B); P < 0.05.
Plasma Insulin
During the CTL, kMCT10 and KkMCT20 tests, plasma insulin peaked at 311 ± 134, 193 ± 93, and 166 ± 98 pmol/L (all at T1), respectively, with no significant difference across the three tests (Figure 4A). The insulin response to kMCT20 was significantly higher at most T4–8 time points compared to CTL (P < 0.046). During kMCT10LC, plasma insulin was significantly lower than either CTL or kMCT10 at several time points (P < 0.038; Figure 4B). During the two MS tests, plasma insulin peaked at 287 ± 80 (T5.5) and at 148 ± 52 pmol/L (T5.5; P < 0.046; Figure 4C). On the kMCT10 test, plasma insulin only differed between the Young and Older groups at T4.5 (P < 0.05; Figure 4D). Insulin AUC did not differ between tests (data not shown).
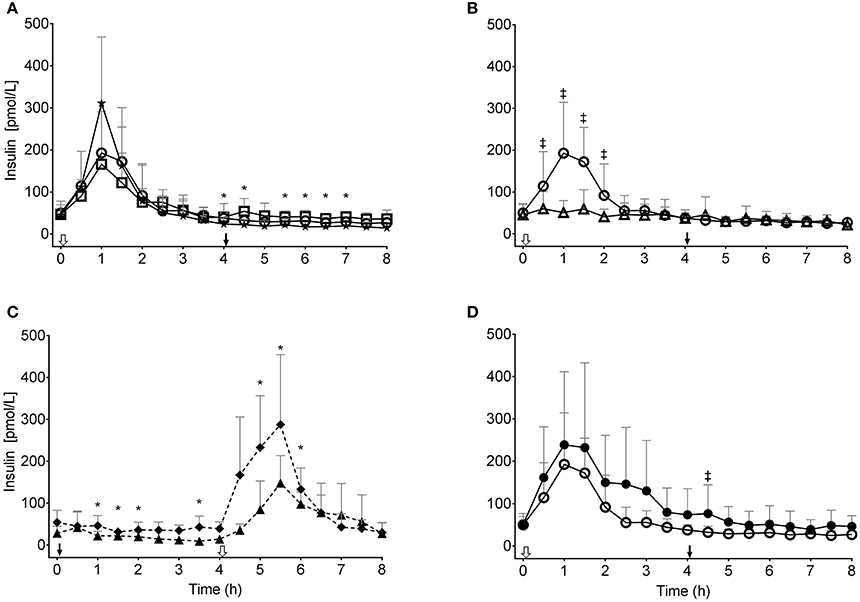
Figure 4. Plasma insulin concentration during the tests: (A) without consuming kMCT (CTL, ★) or after 10 g or 20 g of kMCT (kMCT10, ; kMCT20, □); (B) after 10 g of kMCT with a carbohydrate-rich (kMCT10,
) or low carbohydrate (kMCT10LC, Δ) meal; (C) after no meal or kMCT at T0 but a meal at T4 (MS-CTL;⧫– –⧫), or no meal at T0 but a meal plus kMCT10 at T4 (MS-kMCT; ▴– –▴; (D) Older (•) vs. Young (
) after kMCT10 at T0. The black arrow indicates when the meal plus kMCT were consumed and the white arrow indicates when kMCT alone was consumed without the accompanying meal. Values are presented as mean ± SD (n = 6–10). *Difference with CTL; ‡Difference between the different test conditions (P < 0.05).
Plasma Free Fatty Acids
The plasma FFA response differed very little from CTL after either dose of kMCT (Figure 5A), with the MS (Figure 5C) or in the Older group (Figure 5D). There was a significantly lower trough and peak plasma FFA after the LC meal (Figure 5B).
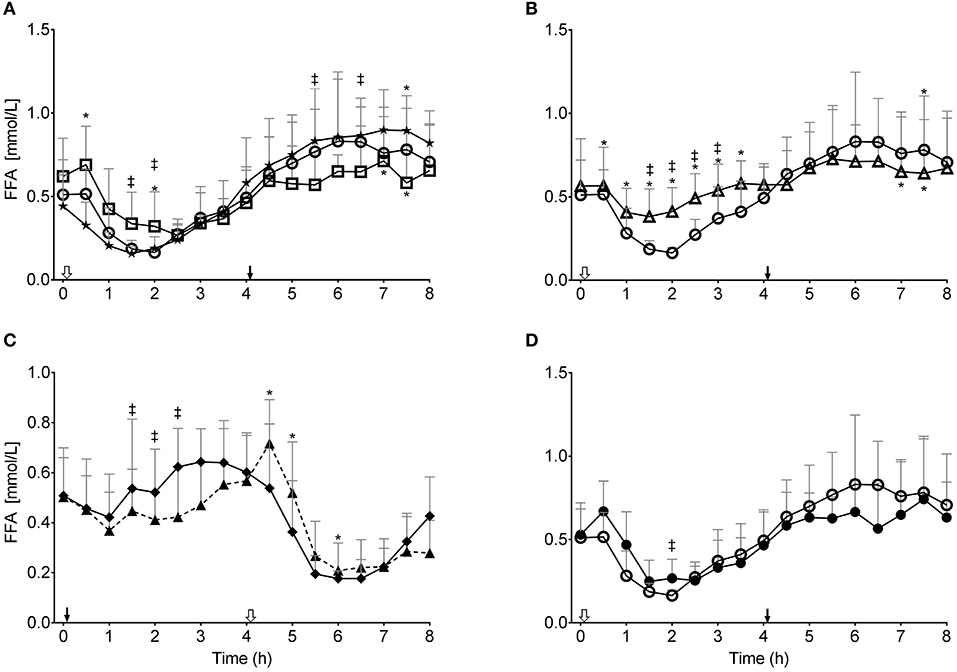
Figure 5. Plasma free fatty acid (FFA) concentration during the tests: (A) without consuming kMCT (CTL, ★) or after 10 g or 20 g of kMCT (kMCT10, ; kMCT20, □); (B) after 10 g of kMCT with a carbohydrate-rich (kMCT0,
) or low carbohydrate (kMCT10LC, Δ) meal; (C) after no meal or kMCT at T0 but a meal at T4 (MS-CTL; ⧫– –⧫) or no meal at T0 but a meal plus kMCT10 at T4 (MS-kMCT; ▴– –▴); (D) Older (•) vs. Young (
) after kMCT10 at T0. The black arrow indicates when the meal plus kMCT were consumed and the white arrow indicates when kMCT alone was consumed without the accompanying meal. Values are presented as mean ± SD (n = 6–10). *Difference with CTL; ‡Difference between the different test conditions (P < 0.05).
Metabolic Changes Over 8 h
To evaluate the overall impact of the main metabolic tests (kMCT, MS) on plasma ketone, glucose, insulin and FFA, AUCs were compared for the full T0-T8 period (Figure 6). kMCT10 induced a significantly lower glycemic response, no change in ketones or insulin response, and higher FFA response when compared with MS kMCT10.
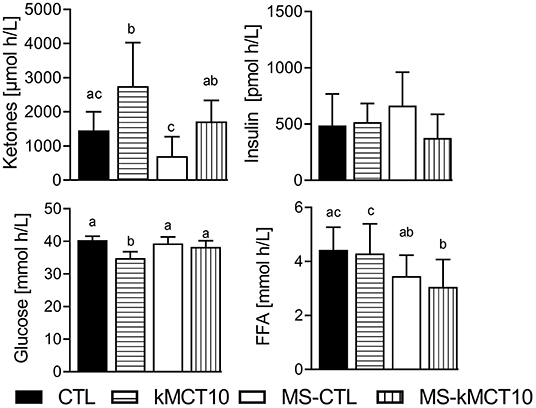
Figure 6. Area-under-the-curve for the plasma ketone, glucose, insulin and free fatty acid (FFA) response over the full 8 h of the metabolic study day without consuming kMCT (CTL), after 10 g of kMCT (kMCT10), with no meal at T0 but a meal at T4 without kMCT (MS-CTL) or with no meal at T0 but a meal at T4 with 10 g of kMCT (MS-kMCT10). Values are presented as mean ± SD (n = 6–10). Means without a common letter differ significantly (A < B); P < 0.05.
Discussion
The main observation was that over the 8 h test period, two 10 g doses of kMCT increased the plasma ketone response by 88% while reducing glycemic response by 12%, without altering insulin or FFA levels. After an overnight fast, an early meal (breakfast) was more effective at potentiating the ketogenic effect of MS over 8 h, compared to a delayed meal (lunch) without breakfast. When compared to our standard high carbohydrate meal, a LC meal lowered plasma glucose and insulin but had similar plasma ketone response from kMCT.
Like other kMCT preparations reported previously (18, 28), the kMCT drink used here rapidly increased plasma ketones with an equivalent ketogenic effect observed whether it was taken with a meal in the morning or at midday (Figure 1C). The ketonemia induced by kMCT lasted 4–5 h so projected over a typical intake of three meals/day, would result in mild ketosis for at least 12–15 h/d. The ketogenic response to kMCT20 was higher than for kMCT10 in the 30 min after consuming the drink (Figure 1A), but the 20 g dose did not actually double the ketogenic response. These results confirm that although the plasma ketone response increases with the dose of MCT taken orally over a large range (10–70 g) (5), the response is not necessarily linear over a small range (10 to 20 g) and may well-depend on how it is provided with the volume of the drink playing a role (29). The timing of macronutrient absorption varies with energy density, volume and digestibility of meal and differs significantly between liquid and solid foods (30, 31).
Few experimental protocols for MS have been reported in humans. Our MS protocol described here with a first dose of kMCT in the morning without breakfast and a second dose of kMCT at noon with a meal increased the plasma ketone response by 145%. Plasma glucose changes were inversely related to those of ketones suggesting that MS conditions were being met albeit over only 8 h making this protocol relatively simple to test in other study conditions.
During healthy aging, fasting plasma glucose tends to be higher and brain glucose uptake declines but brain ketone uptake does not change (32, 33). After consuming a ketogenic drink containing kMCT, older people in good health have previously been shown to have a similar plasma ketone response and oxidize carbon-13-labeled BHB at the same rate as middle-aged or young adults (34). Here we confirm that the plasma ketone response induced with kMCT while consuming a high-carbohydrate meal was unaffected by aging and this despite the usual trend toward higher plasma glucose and mild insulin resistance in older people (Figures 1–3) (33, 35). A daily 30 g dose of MCT taken for 6 months was not associated with more side-effects than a high oleic acid placebo and did not change compliance or cardiovascular risk markers in an older population (10), suggesting good long-term acceptability, safety and validity of the 10 and 20 g doses of kMCT tested here.
This study had some limitations. First, the metabolic response to a single dose of kMCT may not be extrapolatable to longer term consumption of MCT. However, one report showed that consumption of a ketogenic MCT drink for 2–3 months did not significantly change the plasma ketone response compared to before starting the MCT (11). Second, we did not have a CTL metabolic test for the Older group, but the plasma ketone response of older people is very similar to that in young people (34). Third, although adequately powered for a significant effect of the dose of MCT on plasma ketones in young adults, the number of participants was still small making the study vulnerable to type II error.
We conclude that an 8-h MS protocol involving two doses of kMCT, the first taken with breakfast and the second without lunch, increases the plasma ketone response independent of carbohydrate in the meal or age, and without affecting the insulin or FFA level. Thus, a kMCT drink can provide a robust short-term increase in ketones under various feeding conditions and might enhance the metabolic effectiveness of short-term MS or intermittent fasting.
Data Availability Statement
The datasets generated for this study are available on request to the corresponding author.
Ethics Statement
The studies involving human participants were reviewed and approved by Research Ethics Committee of the Integrated University Health and Social Services of Eastern Townships—Sherbrooke University Hospital Center. The patients/participants provided their written informed consent to participate in this study.
Author Contributions
SC, BC, C-AC, and MF designed the study and CV, VS-P, C-AC, and MF conducted it. CV, VS-P, and SC analyzed the data. All authors contributed to the final article.
Funding
Nestlé provided the kMCT drink for this project which was funded by the Vitae Foundation, Sherbrooke, Quebec, Canada. The funder had no role in the study design, data collection or analysis reported in the present manuscript.
Conflict of Interest
SC acted as an external consultant to Nestlé, Bulletproof and Accera and received research funding and/or research materials from Abitec and Nestlé. SC is founder and CEO of Senotec Ltd.
The remaining authors declare that the research was conducted in the absence of any commercial or financial relationships that could be construed as a potential conflict of interest.
Acknowledgments
We thank Marie-Christine Morin and Étienne Croteau for their assistance in participant screening, blood sampling, and care of the participants.
References
1. Courchesne-Loyer A, Lowry CM, St-Pierre V, Vandenberghe C, Fortier M, Castellano CA, et al. Emulsification increases the acute ketogenic effect and bioavailability of medium-chain triglycerides in humans: protein, carbohydrate, and fat metabolism. Curr Dev Nutr. (2017) 1:e000851. doi: 10.3945/cdn.117.000851
2. Veech RL. The therapeutic implications of ketone bodies: the effects of ketone bodies in pathological conditions: ketosis, ketogenic diet, redox states, insulin resistance, and mitochondrial metabolism. Prostagland Leukot Essent Fatty Acids. (2004) 70:309–19. doi: 10.1016/j.plefa.2003.09.007
3. Cox PJ, Clarke K. Acute nutritional ketosis: implications for exercise performance and metabolism. Extrem Physiol Med. (2014) 3:17. doi: 10.1186/2046-7648-3-17
4. Cunnane SC, Courchesne-Loyer A, St-Pierre V, Vandenberghe C, Pierotti T, Fortier M, et al. Can ketones compensate for deteriorating brain glucose uptake during aging? Implications for the risk and treatment of Alzheimer's disease. Ann N Y Acad Sci. (2016) 1367:12–20. doi: 10.1111/nyas.12999
5. Cunnane SC, Courchesne-Loyer A, Vandenberghe C, St-Pierre V, Fortier M, Hennebelle M, et al. Can ketones help rescue brain fuel supply in later life? Implications for cognitive health during aging and the treatment of Alzheimer's disease. Front Mol Neurosci. (2016) 9:53. doi: 10.3389/fnmol.2016.00053
6. Cahill GF. Fuel metabolism in starvation. Annu Rev Nutr. (2006) 26:1–22. doi: 10.1146/annurev.nutr.26.061505.111258
8. Henderson ST, Vogel JL, Barr LJ, Garvin F, Jones JJ, Costantini L, et al. Study of the ketogenic agent AC-1202 in mild to moderate Alzheimer's disease: a randomized, double-blind, placebo-controlled, multicenter trial. Nutr Metab. (2009) 6:31. doi: 10.1186/1743-7075-6-31
9. Krikorian R, Shidler MD, Dangelo K, Couch SC, Benoit SC, Clegg D, et al. Dietary ketosis enhances memory in mild cognitive impairment. Neurobiol Aging. (2012) 33:425.e419–27. doi: 10.1016/j.neurobiolaging.2010.10.006
10. Fortier M, Castellano AC, Croteau E, Langlois F, Bocti C, St-Pierre V, et al. A ketogenic drink improves brain energy and some measures of cognition in mild cognitive impairment. Alzheimers Dement. (2019) 5:625–34. doi: 10.1016/j.jalz.2018.12.017
11. Ota M, Matsuo J, Ishida I, Takano H, Yokoi Y, Hori H, et al. Effects of a medium-chain triglyceride-based ketogenic formula on cognitive function in patients with mild-to-moderate Alzheimer's disease. Neurosci Lett. (2019) 690:232–6. doi: 10.1016/j.neulet.2018.10.048
12. Ebert D, Haller RG, Walton ME. Energy contribution of octanoate to intact rat brain metabolism measured by 13C nuclear magnetic resonance spectroscopy. J Neurosci. (2003) 23:5928–35. doi: 10.1523/JNEUROSCI.23-13-05928.2003
13. Robinson AM, Williamson DH. Physiological roles of ketone bodies as substrates and signals in mammalian tissues. Physiol Rev. (1980) 60:143–87. doi: 10.1152/physrev.1980.60.1.143
14. Tefera TW, Borges K. Metabolic dysfunctions in amyotrophic lateral sclerosis pathogenesis and potential metabolic treatments. Front Neurosci. (2017) 10:611. doi: 10.3389/fnins.2016.00611
15. Schönfeld P, Wojtczak L. Short- and medium-chain fatty acids in energy metabolism: the cellular perspective. J Lipid Res. (2016) 57:943–54. doi: 10.1194/jlr.R067629
16. Bach AC, Babayan VK. Medium-chain triglycerides: an update. Am J Clin Nutr. (1982) 36:950–62. doi: 10.1093/ajcn/36.5.950
17. St-Onge MP, Ross R, Parsons WD, Jones PJ. Medium-chain triglycerides increase energy expenditure and decrease adiposity in overweight men. Obes Res. (2003) 11:395–402. doi: 10.1038/oby.2003.53
18. Vandenberghe C, St-Pierre V, Pierotti T, Fortier M, Castellano CA, Cunnane SC, et al. Tricaprylin alone increases plasma ketone response more than coconut oil or other medium-chain triglycerides: an acute crossover study in healthy adults. Curr Dev Nut. (2017) 1:e000257. doi: 10.3945/cdn.116.000257
19. Courchesne-Loyer A, Croteau E, Castellano CA, St-Pierre V, Hennebelle M, Cunnane SC, et al. Inverse relationship between brain glucose and ketone metabolism in adults during short-term moderate dietary ketosis: a dual tracer quantitative positron emission tomography study. J Cereb Blood Flow Metab. (2017) 37:2485–93. doi: 10.1177/0271678X16669366
20. Puchalska P, Crawford PA. Multi-dimensional roles of ketone bodies in fuel metabolism, signaling, and therapeutics. Cell Metab. (2017) 25:262–84. doi: 10.1016/j.cmet.2016.12.022
21. Anton SD, Moehl K, Donahoo WT, Marosi K, Lee SA, Mainous AG III, et al. (2018). Flipping the metabolic switch: understanding and applying the health benefits of fasting. Obesity. 26:254–68. doi: 10.1002/oby.22065
22. Mattson MP, Moehl K, Ghena N, Schmaedick M, Cheng A. Intermittent metabolic switching, neuroplasticity and brain health. Nat Rev Neurosci. (2018) 19:63–80. doi: 10.1038/nrn.2017.156
23. St-Pierre V, Courchesne-Loyer A, Vandenberghe C, Hennebelle M, Castellano AC, Cunnane SC, et al. Butyrate is more ketogenic than leucine or octanoate-monoacylglycerol in healthy adult humans. J Funct Foods. (2017) 32:170–5. doi: 10.1016/j.jff.2017.02.024
24. St-Pierre V, Vandenberghe C, Lowry MC, Fortier M, Castellano AC, Wagner R, et al. Plasma ketone and medium chain fatty acid response in humans consuming different medium chain triglycerides during a metabolic study day. Front Nut. (2019) 6:46. doi: 10.3389/fnut.2019.00046
25. Gagnon RC, Peterson JJ. Estimation of confidence intervals for area under the curve from destructively obtained pharmacokinetic data. J Pharmacokinet Biopharm. (1998) 26:87–102. doi: 10.1023/A:1023228925137
26. Pani LN, Korenda L, Meigs JB, Driver C, Chamany S, Fox CS, et al. Effect of aging on A1C levels in individuals without diabetes: evidence from the framingham offspring study and the national health and nutrition examination survey 2001–2004. Diabetes Care. (2008) 31:1991–96. doi: 10.2337/dc08-0577
27. Golderngerg R, Punthakee Z. Lignes directrices de pratique clinique. Can J Diabetes. (2013) 37:S36–372. doi: 10.1016/j.jcjd.2013.07.031
28. Courchesne-Loyer A, Fortier M, Tremblay-Mercier J, Chouinard-Watkins R, Roy M, Nugent S, et al. Stimulation of mild, sustained ketonemia by medium-chain triacylglycerols in healthy humans: estimated potential contribution to brain energy metabolism. Nutrition. (2013) 29:635–40. doi: 10.1016/j.nut.2012.09.009
29. Okabe T, Terashima H, Sakamoto A. What is the manner of gastric emptying after ingestion of liquids with differences in the volume under uniform glucose-based energy content? Clin Nutr. (2017) 36:1283–87. doi: 10.1016/j.clnu.2016.08.014
30. Stull AJ, Apolzan JW, Thalacker-Mercer AE, Iglay HB, Campbell WW. Liquid and solid meal replacement products differentially affect postprandial appetite and food intake in older adults. J Am Diet Assoc. (2008) 108:1226–30. doi: 10.1016/j.jada.2008.04.014
31. Müller M, Canfora EE, Blaak EE. Gastrointestinal transit time, glucose homeostasis and metabolic health: modulation by dietary fibers. Nutrients. (2018) 10:E275. doi: 10.3390/nu10030275
32. Nugent S, Tremblay S, Chen KW, Ayutyanont N, Roontiva A, Castellano CA, et al. Brain glucose and acetoacetate metabolism: a comparison of young and older adults. Neurobiol Aging. (2014) 35:1386–95. doi: 10.1016/j.neurobiolaging.2013.11.027
33. Castellano CA, Hudon C, Croteau E, Fortier M, St-Pierre V, Vandenberghe C, et al. Links between metabolic and structural changes in the brain of cognitively normal older adults: a 4-year longitudinal follow-up. Front Aging Neurosci. (2019) 11:15. doi: 10.3389/fnagi.2019.00015
34. Freemantle E, Vandal M, Tremblay Mercier J, Plourde M, Poirier J, Cunnane SC, et al. Metabolic response to a ketogenic breakfast in the healthy elderly. J Nutr Health Aging. (2009) 13:293–98. doi: 10.1007/s12603-009-0026-9
Keywords: medium chain triglycerides, ketone, glucose, insulin, free fatty acids
Citation: Vandenberghe C, St-Pierre V, Fortier M, Castellano C-A, Cuenoud B and Cunnane SC (2020) Medium Chain Triglycerides Modulate the Ketogenic Effect of a Metabolic Switch. Front. Nutr. 7:3. doi: 10.3389/fnut.2020.00003
Received: 03 September 2019; Accepted: 10 January 2020;
Published: 31 January 2020.
Edited by:
Jennie Cecile Brand-Miller, University of Sydney, AustraliaReviewed by:
Stephen F. Burns, Nanyang Technological University, SingaporeDominic D'Agostino, University of South Florida, United States
Copyright © 2020 Vandenberghe, St-Pierre, Fortier, Castellano, Cuenoud and Cunnane. This is an open-access article distributed under the terms of the Creative Commons Attribution License (CC BY). The use, distribution or reproduction in other forums is permitted, provided the original author(s) and the copyright owner(s) are credited and that the original publication in this journal is cited, in accordance with accepted academic practice. No use, distribution or reproduction is permitted which does not comply with these terms.
*Correspondence: Valérie St-Pierre, valerie.r.st-pierre@usherbrooke.ca