- 1Laboratory of Horticulture, National Agricultural Research Institute of Tunisia (INRAT), University of Carthage, Tunis, Tunisia
- 2Laboratory of Horticulture, Faculty of Agricultural and Environmental Sciences, Horticultural Institute, Szent István University, Budapest, Hungary
- 3Dipartimento di Scienze e Tecnologie Biologiche ed Ambientali, Università del Salento (DiSTeBA), Lecce, Italy
- 4Department of Food Science and Postharvest Technology, Bihar Agricultural University, Bhagalpur, India
- 5Department of Statistics, Mathematics, and Computer Application, Bihar Agricultural University, Bhagalpur, India
Owing to several presumed health-promoting biological activities, increased attention is being given to natural plant chemicals, especially those frequently entering the human diet. Glucosinolates (GLs) are the main bioactive compounds found in broccoli (Brassica oleracea L. var. italica Plenck). Their regular dietary assumption has been correlated with reduced risk of various types of neoplasms (lung, colon, pancreatic, breast, bladder, and prostate cancers), some degenerative diseases, such as Alzheimer's, and decreased incidence of cardiovascular pathologies. GL's synthesis pathway and regulation mechanism have been elucidated mainly in Arabidopsis. However, nearly 56 putative genes have been identified as involved in the B. oleracea GL pathway. It is widely recognized that there are several pre-harvest (genotype, growing environment, cultural practices, ripening stage, etc.) and post-harvest (harvesting, post-harvest treatments, packaging, storage, etc.) factors that affect GL synthesis, profiles, and levels in broccoli. Understanding how these factors act and interact in driving GL accumulation in the edible parts is essential for developing new broccoli cultivars with improved health-promoting bioactivity. In this regard, any systematic and comprehensive review outlining the effects of pre- and post-harvest factors on the accumulation of GLs in broccoli is not yet available. Thus, the goal of this paper is to fill this gap by giving a synoptic overview of the most relevant and recent literature. The existence of substantial cultivar-to-cultivar variation in GL content in response to pre-harvest factors and post-harvest manipulations has been highlighted and discussed. The paper also stresses the need for adapting particular pre- and post-harvest procedures for each particular genotype in order to maintain nutritious, fresh-like quality throughout the broccoli value chain.
General Overview and Commercial Importance of Broccoli
The Brassicaceae family comprises 52 tribes, 351 genera, and 3,977 species, some of great economic importance (BrassiBase, https://brassibase.cos.uni-heidelberg.de). Cultivated varieties of Brassica oleracea L. share a common genome comprising nine chromosomes and present an outstanding diversification of morphotypes, including broccoli (var. italica Plenk), Brussels sprouts [var. gemmifera (DC.) Zenker], cabbage (var. capitata L.), cauliflower (var. botrytis L.), kale (var. medullosa Thell.), kohlrabi (var. gongylodes L.), and several endemisms. All these were likely shaped through a process of human domestication starting from a simple leafy type ancestor (kale, var. viridis L.), resulting in arrested development, enlargement, and morphological alteration of the epigeous organs (inflorescences, leaves, stems, or buds) (1, 2).
Broccoli is a annual, cool season, herbaceous crop with optimum growth temperatures between 13 and 20°C (3). It grows 60–90 cm tall and forms an upright and branching thick green stalk with leathery, oblong gray-blue to green rosette basal leaves. At the end of the central axis and the branches (stems), broccoli has dense green edible clusters of flower buds (florets) that, if left unharvested, bloom in yellow flowers with four petals and produce silique fruits. The florets and the upper stems form the inflorescence (head) of the broccoli, which constitutes the commonly consumed organ (Figure 1). The word broccoli is derived from the Latin word “brachium” (brocco in modern Italian), which means branch and refers to the frequent sprouts making up the head (4).
Although broccoli domestication dates back to the ancient Italian Etruscan civilization, its large-scale commercial cultivation started about a century ago in California and spread widely all over the world after World War II, adapting to local climatic conditions and environments (4–6).
Since then, and particularly in the last 20 years, the import and export of broccoli and cauliflower greatly rose to a value exceeding $1.2 billion USD (Figure 2), alongside a 940% increase in their consumption (The Atlas of Economic Complexity, https://atlas.cid.harvard.edu). Market expansion was primarily driven by a growing global awareness over health and the ecological concept of “green living”; fresh and processed broccoli are, indeed, regularly identified among the vegetables habitually eaten to improve health and well-being (7).
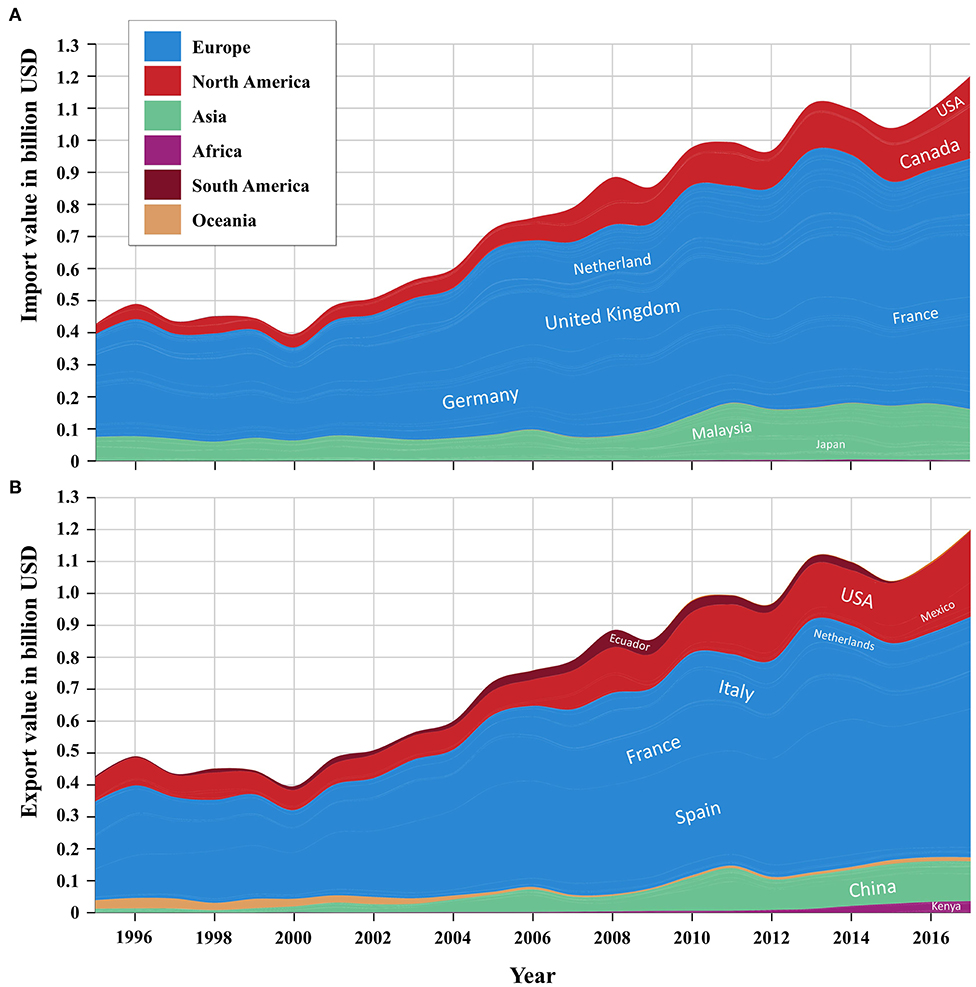
Figure 2. Global trend of broccoli and cauliflower import (A) and export (B) by countries from 1999 to 2017.
Currently, the land cultivated with broccoli and cauliflower worldwide exceeds 1.4 million hectares (ha), with a yield of ~26 million metric tons that has been rising steadily over the years (+14.7% between 2012 and 2017). The top supplying countries are China and India, contributing about 40 and 33%, respectively, to the global production (8). The UK ranks first among importing countries, with a value of ~$210 million USD, followed by Canada, the USA, and Germany (UN Comtrade Database, https://comtrade.un.org/data).
Broccoli is marketed as either a fresh or processed product; however, the fresh market is considerably greater since the quoted price of broccoli for processing is typically 30–60% lower. Fresh broccoli also includes value added through minimally processed products, such as fresh-cut broccoli packed in bunches, bagged florets, and broccoli coleslaw—goods widely appreciated by a growing segment of health-conscious international consumers. Processed broccoli is typically frozen for retail sale and sold as spears or chopped, while only a limited amount is dehydrated and/or canned for ready-to-prepare mills and soups. In addition, the rising adoption of seed and sprout broccoli extracts in the food, cosmeceutical, and nutraceutical industries is estimated to account for significant revenue share in the global market, especially in the emerging economies of the Asia Pacific region. The global broccoli extract market was valued at over 1.9 billion US dollars in 2017 and is expected to reach a valuation in excess of $2.8 billion USD by the end of 2027, reflecting a compound annual growth rate (CAGR) of 3.7% during the forecast period (9).
Broccoli as a Dietary Source of Bioactive Compounds
Broccoli heads have long been identified as a primary part of a well-balanced healthy eating plan. They are a low-calorie vegetable [34 kcal (~142 kJ)/100 g fresh weight (fw)] and a rich dietary source of minerals (calcium, phosphorus, potassium, and sodium), vitamins (B, C, E, K), fibers, and many other health-promoting molecules, including carotenoids (β-carotene and lutein), flavonoids (kaempferol), hydroxycinnamic acids (sinapic and caffeoyl-quinic acid derivatives), and distinctively, glucosinolates (GLs) (10, 11).
Compared with other organs (main stalk, leaves, and roots), edible broccoli heads are particularly rich in vitamin C (~188 mg/100 g fw) and are associated with a high content of polyphenols (64.9 mg/100 g fw) (12). Nevertheless, strong epidemiological evidence associates most of the positive health effects of broccoli consumption to the presence of GLs and their cognate breakdown products, isothiocyanates (13).
GLs represent 0.2–2% of broccoli heads' dry weight (dw) and comprise predominantly 4-methylsulphinylbutyl-GL (23–64%), followed by 3-indolylmethyl-, 4-hydroxy-3-indolylmethyl-, 3-methylsulphinylpropyl-, 1-methoxy-3-indolylmethyl-, and 4-methoxy-3-indolylmethyl-GL. Traces (<0.5 mg/g dw) of 4-methylthiobutyl-GL, 2(S)-2-hydroxy-3-butenyl-GL, 5-methylsulphinylpentenyl-GL, 2-hydroxy-4-pentenyl-GL, and 2-phenylethyl-GL have also been detected (14, 15). However, there is substantial variation regarding the total amount of GLs and the individual components among samples depending on several genetic, physiological, and environmental determinants (16).
GLs and isothiocyanates are potent anti-carcinogenic agents and widely recognized as effective inductors of phase II antioxidant enzymes (17). Particularly interesting in this regard is sulforaphane (4-methylsulfinylbutyl-isothiocyanate), a 4-methylsulphinylbutyl-GL-derived product of which broccoli represents the richest source among Brassica vegetables (13, 18, 19). 4-methylsulfinylbutyl-isothiocyanate inhibits chronic inflammatory processes and plays a multifaceted role in the onset, progression, and pleiotropic invasion of lung, stomach, colon, prostate, and rectal cancers (20–22). It upregulates the expression of some cytoprotective genes encoding for NAD(P)H:quinone oxidoreductase 1 (NQO1), heme oxygenase-1 (HO-1), thioredoxin, and superoxide dismutase (SOD), equilibrating ROS imbalance and inhibiting the expression of several pro-inflammatory mediators (13, 23, 24). Preclinical and clinical studies on animals (rats) and humans (healthy women undergoing breast reduction) provided evidence of a pronounced pharmacodynamic action of oral 4-methylsulfinylbutyl-isothiocyanate administration. A single dose of broccoli sprout preparation (150–200 μmol 4-methylsulfinylbutyl-isothiocyanate) prompted a significant upregulation of the expression of NQO1 and OH-1 transcripts, as well as an increase in NQO1 enzymatic activity. An improved bronchoconstriction with increased NQO1 expression was also observed in 60% of asthmatic patients following the daily oral intake (2 weeks) of 440 mg myrosinase-treated powdered broccoli sprouts extract (equivalent to 100 μmol 4-methylsulfinylbutyl-isothiocyanate) (13, 25). 4-methylsulfinylbutyl-isothiocyanate intake (~420 μmol daily dose in the form of 70 g broccoli sprouts) appears to significantly lessen or completely eradicate Helicobacter pylori infection with a concomitant decrease in gastric lumen inflammation of treated patients during the 8-weeks intervention period (26). Furthermore, a strong correlation between high serum 4-methylsulfinylbutyl-isothiocyanate concentration and (1) reduced fasting glucose levels, (2) stabilization of insulin, and (3) insulin resistance indices was reported in type 2 diabetes patients (27, 28). In addition, the intake of hot aqueous broccoli sprouts extracts (125 mL dose−5.2 μmol/mL of isothiocyanates after myrosinase hydrolysis) prompted the excretion of environmental carcinogens (dithiocarbamates) with a rate of 49 mmol/12 h (29). In vitro and in vivo trials are currently ongoing to assess the efficacy of 4-methylsulfinylbutyl-isothiocyanate intake on several other chronic and degenerative disorders including aging, allergic diseases, adverse drug reactions, cystic fibrosis, and gastrointestinal and smoke-related diseases (13).
Other GLs and isothiocyanates (e.g., 2-phenethyl isothiocyanate) demonstrated preventive properties against cancers and the ability to inhibit the metabolic activation of various carcinogens (30). Following BroccoMax® [a commercial broccoli supplement in pills (Jarrow Formulas, Los Angeles, CA, USA) containing a combination of 121 and 40 μmol 4-methylsulphinylbutyl-GL and 4-methylthiobutyl-GL, respectively, and active myrosinase] consumption, a decrease in ductal carcinoma cell proliferation, with an inverse association of the tumor marker Ki-67, was observed in patients with abnormal breast mammograms (13, 31). Megna et al. (32) reported a novel molecular activity of indole-3-carbinol in combatting various colorectal cells through cytotoxic and pro-apoptotic effects and activation of the aryl hydrocarbon receptor pathway. Quirit et al. (33) demonstrated that indole-3-carbinol prevents human melanoma cell growth by inhibiting NEDD4-1. Dekić et al. (34) found that phenylpentyl isothiocyanates exhibited cytotoxic effect on Caco-2 and HeLa cancer cell lines with 100-fold higher potency than papaverine. Núñez-Iglesias et al. (35) reported a dose-dependent cytotoxic effect of 3-butenyl isothiocyanates on prostate cancer.
However, not all GLs are equal in their biological effects, which rather depend on the specific amount and profile within a vegetable. Thus, a high Brassica consumption cannot be generally regarded as cancer-preventive (36). Recent in vitro and in vivo studies on mammalian cell cultures, bacteria, and animals indicated that broccoli and its extracts might also have adverse consequences for health, including mutagenic and genotoxic activities through the formation of characteristic DNA adducts. Adduct formation appears primarily prompted by the indole GLs [1-methoxy-3-indolylmethyl-GL, 4-methoxy-3-indolylmethyl-GL, and sinalbin (not detected in broccoli)] and requires the presence of myrosinase or gut microbiota thioglucosidase activity, suggesting a causal involvement of their breakdown products (37, 38). However, the relevance of the genotoxic activities to human health is not known yet (16).
Provided the importance of GLs in broccoli quality and human health, several reviews have dealt with various aspects of their chemistry and biosynthesis (20, 39–42), physiologic function in plant (43), and biological activity (13). The effect of post-harvest procedures and processing on GLs levels, as well as the influence of whole supply chain on intake, bioavailability, and human health associated with major Brassica vegetables consumption, were also appropriately reviewed (19, 44–46). However, a systematic summary on the effect of pre- and post-harvest factors/treatments on the levels and profiles of GLs in a large number of broccoli genotypes has never been carried out. Here, a broad overview of the published reports on this subject trying to fill the gap is provided. Despite the awareness given by the amount of recent research on GLs in broccoli, it is far from being comprehensive.
GLs Chemical Structure, Metabolism, and Regulation in Brassica
GLs are cis-N-hydroximinosulfate esters (S-glucopyranosyl thiohydroximates) naturally occurring as S-bound glucosides and consist of a β-thioglucose-moiety, a sulfonated oxime moiety, and a flexible aglycone α-amino acid-deriving side chain (Figure 3) (19, 20, 47). The level of GLs and their composition vary considerably not only between different Brassica crops, but also between different tissues within the same crop (48). More than 17 different GL have been identified in broccoli, classified as aliphatic (derived from the amino acids methionine, alanine, valine, leucine, or isoleucine), indole (derived from tryptophan), and aromatic GLs (derived from phenylalanine or tyrosine) (49).
The biosynthetic pathway of GLs has been thoroughly described in previous reviews (20, 39, 49). Aliphatic GLs are biosynthesized following three key independent steps: (1) the side chain elongation, (2) the GLs core structure building, and (3) the amino acid side chain modification (Figure 4). Aliphatic GLs are characterized by the presence of a side chain of variable length shaped during the first step. Here, a non-polar amino acid, typically methionine, is deaminated to the corresponding 2-oxo-acid by a branched-chain amino acid aminotransferase (BCAT). Then, a sequential addition of single methylene groups (–CH2–) to the side chain of the 2-oxo-acid proceeds through a cycle of three consecutive enzymatic reactions. The reactions involve methylthioalkylmalate synthase (MAM), an isopropylmalate isomerase (IPMI), and isopropylmalate dehydrogenase (IPM-DH), yielding an elongated 2-oxo-acid. The elongated 2-oxo-acid can either proceed through another elongation round or be transaminated by a BCAT to the corresponding chain-elongated amino acid. Unlike indole GLs, a similar step of chain elongation is required for aromatic GL biosynthesis. Subsequently, cytochromes P450 (CYP79s) catalyzes the conversion of the amino acid derivatives to aldoximes, oxidized later by CYP83s into nitrile oxides, transformed to thiohydroximates via glutathione (GSH) S-transferases (GSTFs) and the C-S lyase (SUR1) reaction, and finally converted to the GL core structure by S-glucosyltransferases (UGT74s) and sulfotransferases (SOTs) (Figures 3–5). Simultaneously, GSH is produced by the action of specific ligases (GSH1 and GSH2) from cysteine, glutamate, and glycine (Figure 4, central part) and ensures sulfur supply for GL biosynthesis, yielding GSH conjugates (50, 51). Alkenylation, benzoylation, glycosylation, hydroxylation, methoxylation, oxygenation, and sulfonation may affect the elongated amino acid, increasing the chemical structure diversity of GLs (20, 39, 49). The methylthioalkyl GLs are S-oxygenated in a reaction catalyzed by flavin monooxygenases (FMOGS-OXs) to methylsulfinylalkyl GLs, such as 4-methylsulfinylbutyl-GL (Figure 6). GLs are usually stored in the vacuoles of specific laticifer-like sulfur-rich cells (S-cells) located between the endodermis and phloem along the vasculature and the leaf margins, ensuring the spatial separation of GLs from the enzymes involved in their hydrolysis (42).
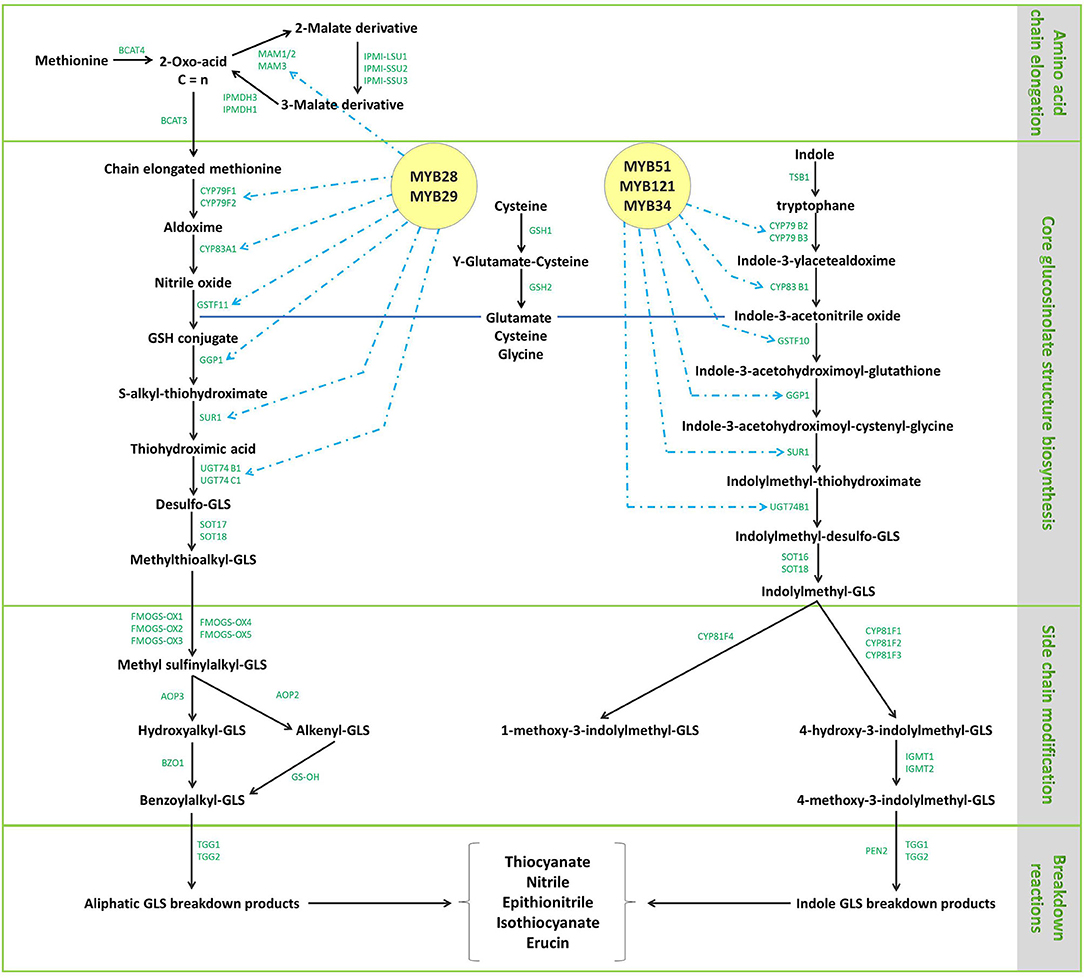
Figure 4. The aliphatic and indole glucosinolate metabolic pathways and related genes in Brassica oleracea L. Amino acid chain elongation is the first step, followed by the biosynthesis of the core glucosinolate structure and finally, side chain elongation of the synthesized structure in parallel with secondary modifications. The enzymes implicated in the different steps are reported in green. MAM1-3, methylthioalkyl malate synthases; BCAT3/4, branched-chain aminotransferases; IPMDH1/3, isopropylmalate dehydrogenases; IPMI-SSU1/2/3, isopropylmalate isomerases; CYP79B2/3, CYP79F1/2, CYP81F1-4, CYP83B1 cytochrome P450 monooxygenases; GSTF10/11, glutathione S-transferases; CYP83A1, non-redundant cytochrome P450. Enzymes metabolizing oximes in the biosynthesis of glucosinolates; GGP1, γ-glutamyl peptidase, SUR1, tyrosine transaminase family protein; UGT74B1/C1, thiohydroximate S-glucosyltransferases; SOT16-18, sulfotransferases; FMOGS-OX1-5, flavin-containing monooxygenase; AOP2/3, 2-oxoglutarate-dependent dioxygenases; BZO1, benzoyl-CoA ligase; GS-OH, 2-oxoglutarate (2OG) and Fe(II)-dependent oxygenase superfamily protein; TGG1/2, myrosinases; TSB1, tryptophan synthesis gene; SUR1, C-S lyase SUPERROOT; UGT74B1, UDP-dependent glycosyl transferases; IGMT1/2, indole glucosinolate methyl tansferases; EN2, atypical myrosinase; GSH, γ-glutamylcysteine synthetase; MYB28/29/34/51/121 and R2R3, transcription factors.
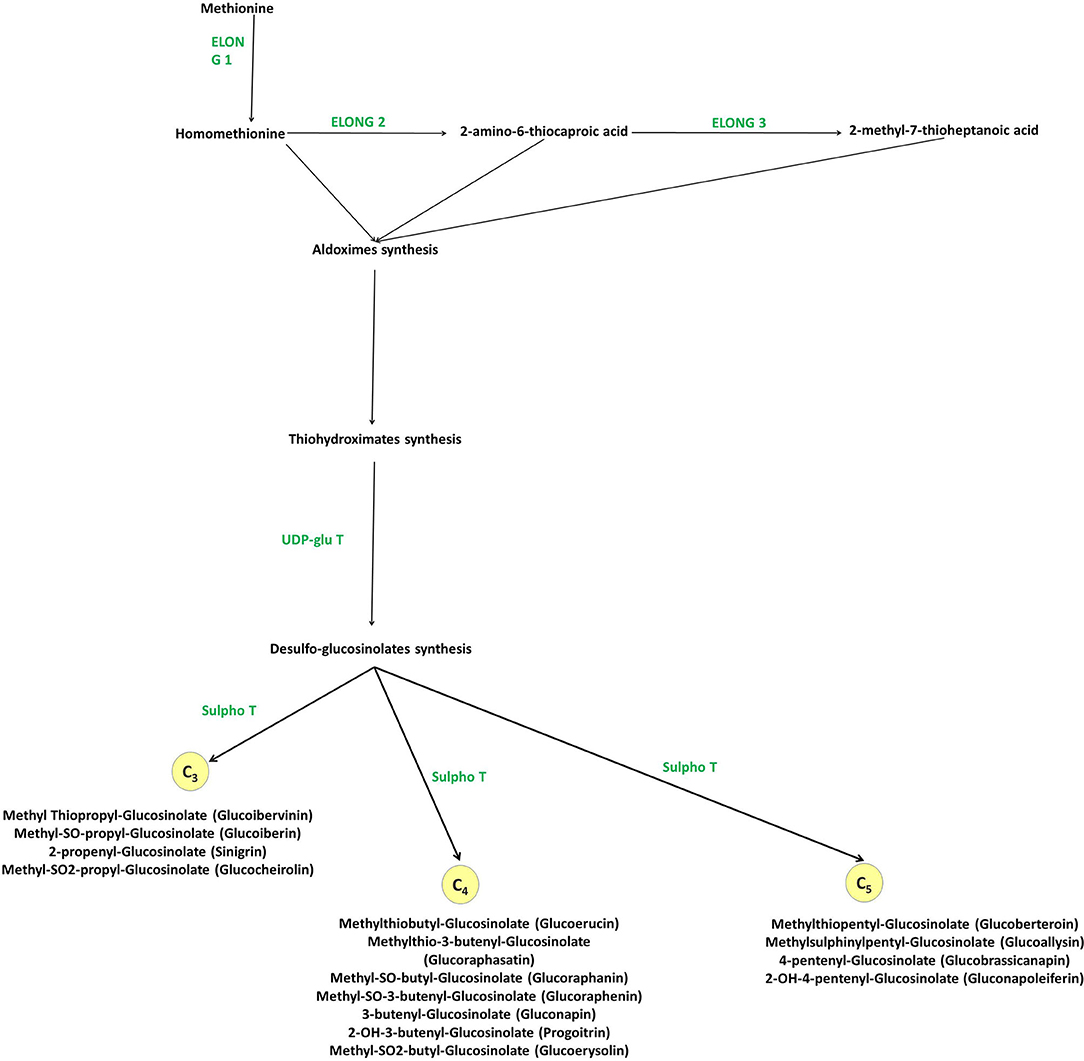
Figure 5. Methionine derived aliphatic GLs biosynthesis in Brassica vegetables. Elong, methionine elongation enzyme; UDP-glu T, UDP-glucose thiohydroximate glucotansferase; Sulfo T, 3′PAPS-5′-phosphosulphate desulphoglucosinolate transferase.
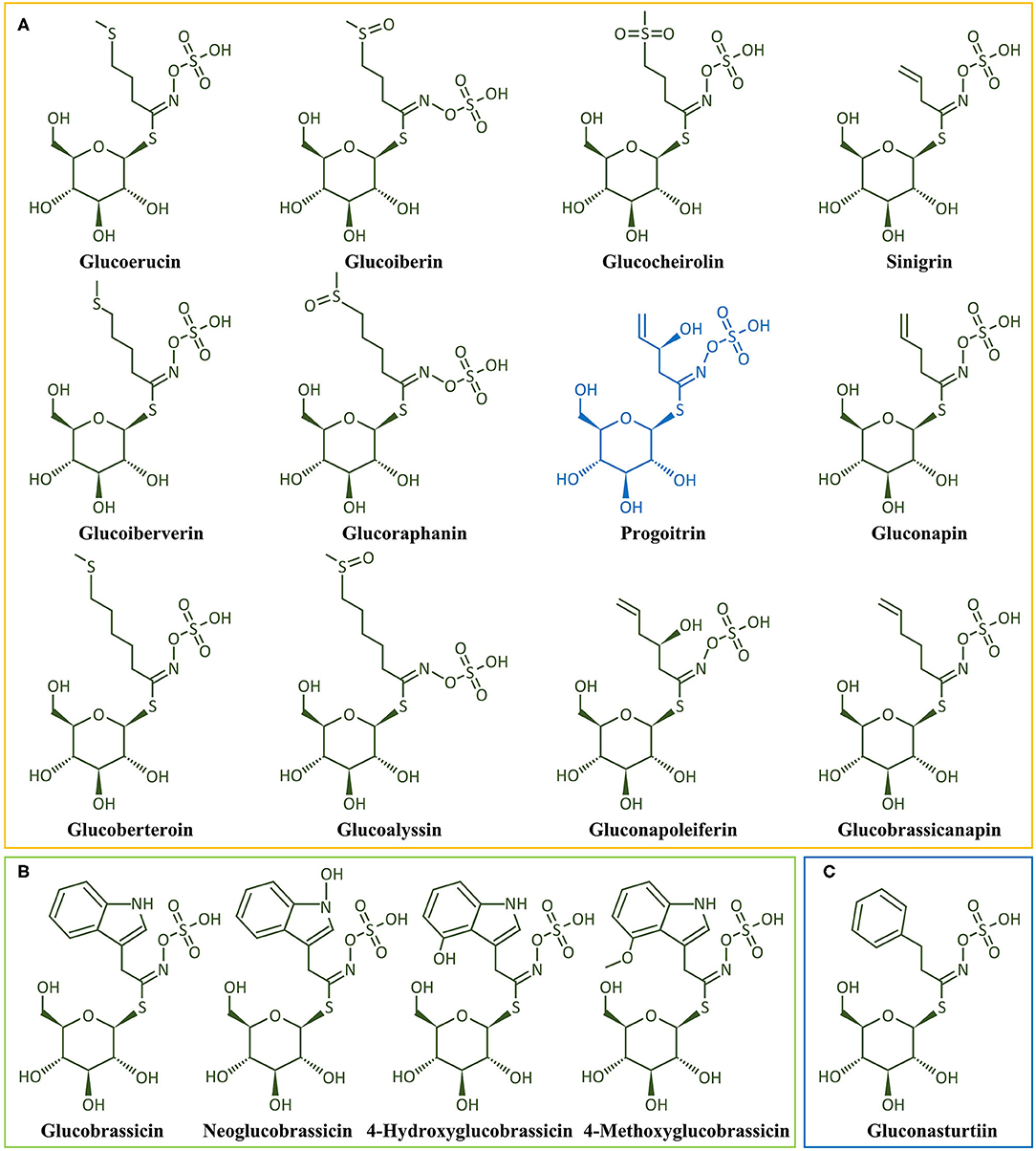
Figure 6. Chemical structure of the main glucosinolates found in broccoli. (A) Aliphatic Gls, (B) Indole GLs, and (C) Aromatic GLs. Glucoerucin, 4-Methylthiobutyl-GL; Glucoiberin, 3-Methylsulphinylpropyl-GL; Sinigrin, 2-Propenyl-GL; Glucoibervirin, 3-Methylthiopropyl-GL; Glucoraphanin, 4-Methylsulphinylbutyl-GL; Progoitrin, 2(R)-2-Hydroxy-3-butenyl-GL; Gluconapin, 3-Butenyl-GL; Glucocheirolin, 3-Methylsufonylpropyl-GL; Glucoberteroin, 5-Methylthiopentyl-GL; Glucoalyssin, 5-Methylsulphinylpentenyl-GL; Gluconapoleiferin, 2-Hydroxy-4-pentenyl-GL; Glucobrassicanapin, 4-Pentenyl-GL; Glucobrassicin, 3-Indolylmethyl-GL; Neoglucobrassicin, 1-Methoxy-3-indolylmethyl-GL; 4-Hydroxyglucobrassicin, 4-Hydroxy-3-indolylmethyl-GL; 4-Methoxyglucobrassicin, 4-Methoxy-3-indolylmethyl-GL; Gluconasturtiin, 2-Phenylethyl-GL.
GL hydrolytic catabolism occurs through the action of myrosinases, specific β-thioglucoside glucohydrolases generating various bioactive products (isothyocyanates, mainly 4-methylsulfinylbutyl isothiocyanate, indole-3-carbinol, allyl isothiocyanate, crambene, iberin, and phenyl isothiocyanate, Figure 7) with recognized nematocidal, fungicidal, and insecticidal activities (52, 53) and controlling key biological process, such as abscisic acid (ABA)-mediated stomatal aperture (54), water regulation under sulfur deficiency (43), jasmonic acid (JA) signaling feedback regulation (55), growth responses (56, 57), flowering (58, 59), and defense reactions against pathogens and herbivores, suggesting a crosstalk with the regulatory networks controlling the developmental and physiological processes (60).
In B. oleracea, 105 genes distributed across the nine chromosomes are putatively related to GL biosynthesis, catabolism, and regulation, with the highest number of loci implicated in aliphatic and indole GL biosynthesis mapping on chromosomes 3 and 4, respectively (61, 62). The genes related to GL enzymatic breakdown, such as PEN and TGG1, and two encodes for β-thioglucoside glucohydrolases are able to cleave the indole (63) and aliphatic GLs, respectively (64). Specifier proteins, such as nitriles specifier proteins (NSPs), epithiospecifier proteins (ESPs), and thiocyanates specifier proteins (TSPs) affect the final breakdown products by inhibiting the synthesis of isothiocyanates in favor of simple nitriles, epithionitriles, and organic isothiocyanates, respectively (65–67).
The biosynthesis of GLs is finely tuned at transcriptional and hormonal levels. Several transcription factors have been identified: IQD1, a gene encoding a calmodulin-binding nuclear protein, and AtDoF1.1, a zinc finger protein, upregulate the biosynthesis of both aliphatic and indole GLs (42, 68, 69); SLIM1, an EIN3-like transcription factor, downregulates the expression of GLs biosynthetic genes (42, 70). Transcription factors, such as MYB28, MYB29, and MYB76 affect specifically the biosynthesis of aliphatic GLs, whereas MYB34, MYB51, and MYB122 are specific for indole GLs (Figure 4). R2R3-MYBs synergize with bHLH05 and bHLH06 to regulate the synthesis of GLs. However, the complex interaction bHLH06/MYC2 was found to negatively regulate the indole GLs biosynthetic pathway. Brassinosteroids (BRs), JA, salicylic acid (SA), and ethylene are known regulators of GL biosynthesis. Ethylene and SA positively affects the synthesis of all GL classes, while ABA is specific for the indole GLs (70). BRs were reported to downregulate CYP79B2 and the biosynthesis of aliphatic- and indole-GL. JA favors the degradation of the transcriptional repressor Jasmonate ZIM domain, which enables the transcription machinery access (42, 71).
Factors Affecting GLs Synthesis and Accumulation
The content and profiles of GLs in broccoli are highly variable and affected by several factors. Genotype is certainly one of the major sources of variability; however, many other pre- and post-harvest aspects often interact, producing substantial effects on the health-promoting properties of broccoli. Although this aspect is central to cultivar recommendation for cancer chemoprevention and raises concerns about the synthesis of compounds reducing broccoli palatability, it also confers prospects for enhancing GL accumulation with no need for metabolic engineering approaches. Thus, it is of great relevance not only for breeders focusing on the development of high-quality genotypes, but also for processors and consumers (72).
Genotypic Variability
Genotypic differences are known to significantly affect the content of many secondary metabolites in broccoli, including GLs and their breakdown products (73). Studying the genetic and environmental effects on GL content in the heads of various commercial broccoli cultivars (Everest, Futura, High Sierra, Viking, Marathon), new experimental lines (USVL), and their crosses, Farnham et al. (74) reported a large variability (up to 7.7-fold) in the content of 4-methylsulfinylbutyl-GL, the main aliphatic GL in broccoli florets. This was primarily ascribed to genotypic differences rather than environmental factors (≈22% of the total variance). Instead, genotypic effects were not significant for the concentrations of 4-hydroxy-3-indolylmethyl-, 1-methoxy-3-indolylmethyl, and indole-3-yl-methyl-GL. Even greater variability in the concentration of total GLs (99-fold change) and 4-methylsulfinylbutyl-GL (95-fold change) was reported by Bhandari and Kwak (75), comparing twelve broccoli cultivars (05-C3, AMaGi, BaeRiDom, CheongJae, Diamond, Grace, Grandeur, JikNok No. 28, NokJae, NokYeom No. 1, TS-2319, and YuDoRI No. 1) and reviewed thoroughly in Verkerk et al. (44). Essentially, 60% of aliphatic GLs appear more influenced by genotypic differences than environmental factors, while indole GLs appear concurrently affected by genotype, environmental conditions, and their interaction (44, 74, 76). Consequently, important advances were achieved in breeding for high aliphatic GL content (methionine-derived GLs). However, programs targeting specific indole GLs (tryptophan-derived GLs) appears more problematic (44). Wang et al. (77) focused on the natural variation of GLs in different broccoli genotypes grown under greenhouse conditions. The authors reported variation of 3.8- to 402-fold in the content of 4-methylsulphinylbuty-GL, 26- to 48-fold in the content of 3-indolylmethyl-GL, and 17.7- to 254.3-fold in the content of 1-methoxy-3-indolylmethyl-GL among commercial cultivars and their parental pure lines, respectively. This suggests the possible use of selected lines characterized by higher levels of beneficial GLs in breeding programs. Concurrently, the development of high GLs broccoli cultivars has been successfully achieved via the introgression of genetic material from B. villosa into an ordinary cultivar, with the resulting hybrids accumulating at least 3-fold more 4-methylsulfinylbutyl isothiocyanate than the parental counterpart. In purple broccoli cultivars, the content of 4-methylsulphinylbutyl-GL and 3-methylsulphinylpropyl-GL attained 6.7 and 3.8 mg/100 g fw, respectively (78). Sahamishirazi et al. (79) detected six major GLs in broccoli florets (3-methylsulfinylpropyl-GL, 2-propenyl-GL, 4-methylsulfinylbutyl-GL, indole-3-yl-methyl-GL, 4-methoxy-3-indolylmethyl-GL, and 1-methoxy-3-indolylmethyl-GL). 4-methylsulfinylbutyl-GL (0.03–2.87 μmol/g dw) and indole-3-yl-methyl-GL (0.21–0.73 μmol/g dw) contributed up to 34 and 17% to the total GLs amount, respectively, followed by 4-methoxy-3-indolylmethyl-GL, 1-methoxy-3-indolylmeth-GL, 2-propenyl-GL, and 3-methylsulfinylpropyl-GL, whose concentrations ranged between 0.43 and 0.12 μmol/g dw. The average of total, indole, and aliphatic GLs was 3.27, 1.59, and 1.56 μmol/g dw, respectively. Nicoletto et al. (80) performed a nutritional characterization of five Italian underutilized broccoli landraces (BF, BF4, BFT, BB, and BS) and revealed that BF4 accumulated 95% more 4-methylsulfinylbutyl isothiocyanate than the other lines. Genotypic differences were also evidenced in seeds and sprouts of three commercial broccoli cultivars (Marathon, Nubia, and Viola). 4-methylsulfinylbutyl-GL was the main aliphatic GL in sprouts of Nubia and Marathon but not in Viola, in which 3-methylsulfinylpropyl-GL prevailed. 4-methylsulfinylbutyl-GL ranged from 118.7 to 327.3 mg/100 g fw and from 26.3 to 204.1 mg/100 g fw in seeds and sprouts, respectively, and was more affected by genotype than 4-hydroxy-3-indolylmethyl-GL (142.2–167.9 and 2.3–13.5 mg/100 g fw in seeds and sprouts, respectively) (72, 73). In a fractionate analysis of GL profiles of several commercial and novel broccoli cultivars, 4-methylsulfinylbutyl-GL, 2(R)-2-Hydroxy-3-butenyl-GL, 4-methylthiobutyl-GL and indole-3-yl-methyl-GL were found to prevail in both flowers stalks and florets (81). Some hybrid lines (5,075, 5,078, and 5,079) and the inbred line 5,308 accumulated considerable levels of 4-methylsulfinylbutyl-GL, while other inbred lines (5,307, 5,311, and 5,409) accumulated higher levels of indole-3-yl-methyl-GL, underlying their promising use in further breeding programs to enhance broccoli nutritional quality. Bhandari and Kwak (75) compared the GL profiles and concentrations in the seeds, sprouts, mature roots, and shoots of broccoli. The total GL content was considerably higher in seeds and sprouts (110.8 and 162.2 μmol/g dw) compared to shoots and roots fractions (4.2 and 48.5 μmol/g dw). (2R)-2-Hydroxy-3-butenyl-GL and 4-methylthiobutyl-GL were mostly abundant GLs in seeds and sprouts accounting for 17–27 and 67–76% of their total content, respectively. Rybarczyk-Plonska et al. (82) reported that broccoli buds had 2.61-, 2-, and 12-fold higher concentrations of indole-3-yl-methyl-GL and 1-methoxy-indole-3-yl-methyl-GL contents than stalks with similar concentrations of total aliphatic GLs in all organs. Formica-Oliveira et al. (83) noticed that broccoli leaves presented 2.5/14.5 higher 4-methylsulfinylbutyl-GL/indole-3-yl-methyl-GL ratio compared to florets.
Pre-harvest Factors
Several pre-harvest factors have demonstrated to be effective in altering the synthesis, accumulation, and profile of GLs in broccoli. These include the developmental stage at harvest, environmental, and seasonal variations, and most agricultural practices (irrigation, fertilization, salinity, priming, and elicitation). An overview of the current state of research and key results is given in Table 1.

Table 1. The effect of pre-harvest manipulations on the level of broccoli glucosinolates (GL class, individual GLs, and/or GL biosynthetic enzymes).
Development Stage at Harvest
Broccoli head development is an important factor in defining their phytochemical composition, as substantial changes arise at in this stage (72, 87, 88). However, such data is currently rather limited. Even so, considerable cultivar-to-cultivar variation in GL metabolism has been reported during broccoli development (Table 1). Young broccoli sprouts accumulate up to 20-fold higher GLs (or breakdown products) than late vegetative stages, and their levels progressively decrease with increasing sprout maturity (72, 73, 85). Broccoli seeds show significantly higher content of total and aliphatic GLs (3-methylsulfinylpropyl-GL, 4-methylsulfinylbutyl-GL, and 4-hydroxy-3-indolylmethyl-GL) than 2-weeks-old sprouts but lower levels of indole GLs (indole-3-yl-methyl-GL and 1-methoxy-3-indolylmethyl), suggesting that the two GL classes are differentially used as chemical defense system against aggressors during plant development (72). However, Hanschen and Schreiner (73) reported higher aliphatic GLs in sprouts being progressively replaced by indole GLs in fully developed heads. Additionally, the highest levels of total GLs and isothiocyanates were detected in young heads rather than at the commercial stage. A linear increasing trend for 4-methylsulfinylbutyl-GL content throughout the development stages was instead reported by Rangkadilok et al. (86) in three broccoli cultivars (Claudia, Marathon, and TB-234). In agreement with Rangkadilok et al. (86), Bhandari et al. (87) reported also a linear increase in 4-methylsulphinylbutyl-GL and a decreasing trend for 3-indolylmethyl-GL. However, the dynamic changes affecting 2-Phenylethyl-GL was cultivar-dependent. 2-Phenylethyl-GL peaked at the first stage of ripening in cultivar 12FA-M296 (0.55 μmol/g dw), at the intermediate stage of ripening in cultivars 10FA-M806, 10FA-M853, 12FA-M413, and Koyoshi with levels ranging from 0.24 to 0.36 μmol/g dw, and at the commercial stage in cultivar 09FA-M295 (0.39 μmol/g dw). Some GLs, such as 3-methylsulphinylpropyl-GL, 2(R)-2-hydroxy-3-butenyl-GL, and 3-butenyl-GL were present throughout the ripening stages only in some genotypes. Instead, conflicting results emerged at later stages of development depending on genotype and plant mineral nutrition. Vallejo et al. (88) found that, under poor sulfur fertilization, total GLs peaked at the early stages of development [35 DAT in the cultivar Vencedor (60.3 μmol/g dw) and 42 DAT in Marathon (64.7 4 μmol/g dw) and Monterrey (65.7 μmol/g dw)], but the dynamic of change of the individual GLs was complex and dependent, besides genotype, upon their chemical class. 4-methylsulfinylbutyl-GL peaked at 42 DAT in cultivar Vencendor (2.3 μmol/g dw), at 49 DAT in Marathon (1.8 μmol/g dw), and 56 DAT in Monterrey (1.9 μmol/g dw) under different fertilization regimes. The behavior was even more complex for indole GLs: 1-Methoxy-3-indolylmethyl-GL peaked at 35 DAT in Vencendor (42.1 μmol/g dw), 42 DAT in Marathon (55.0 μmol/g dw), and 49 DAT in Monterrey (49.2 μmol/g dw). Indole-3-yl-methyl-GL peaked at 42 DAT in Monterry and Vencendor with levels of 13.4 and 14.4 μmol/g dw, respectively. However, two peaks were detected at 42 and 49 DAT (15.1 and 15.4 μmol/g dw, respectively) in Marathon. Similarly, 4-methoxy-3-indolylmethyl peaked at 35 DAT in the cultivars Marathon and Vencendor (1 and 1.6 μmol/g dw, respectively), but at 49 DAT in Monterrey (2.5 μmol/g dw). Verkerk et al. (44) reported that 4-methylsulphinylbutyl isothiocyanate content increased until full commercial maturity and declining after flower setting.
Environmental and Seasonal Variation
Broccoli can grow under various climatic conditions and geographical areas; this suggests significant effects, not only on crop production potential, but also on various quality attributes of broccoli (aroma, texture, taste, etc.) and on the levels of their health-promoting compounds (14, 116). It has been reported that the dynamic change in GLs is tightly correlated with temperature and day length and, as a consequence, by seasonal variation. Light induces expression of GL biosynthesis genes, resulting also in diurnal rhythms, while transcriptional profiling revealed genotype-specific gene responses to temperature but only a limited correlation between GL-related gene expression and GL levels (89). The data on field-grown broccoli pointed out that total GL content and individual profile are rather modulated by the interaction temperature × photoperiod (14, 90, 91, 117). During broccoli sprouts growth, Guo et al. (92) detected the highest 4-methylsulfinylbutyl-GL and 4-methylsulfinylbutyl isothiocyanate at 25°C, but lower (20°C) or higher (30°C) temperatures decreased their levels in cultivar Youxiu. This fact might be ascribed to differential activation of aliphatic and indole GL biosynthetic enzymes by light and temperature, respectively (44). Similarly, Verkerk et al. (44) reported that total and aliphatic GLs in Brassica leaves were higher at 32 and 12°C compared to 22°C under constant lighting conditions. However, in broccoli sprouts, their content was higher at 11 and 33°C than intermediate temperatures. Schonhof et al. (118), studying the effect of light radiation and temperature on greenhouse broccoli (Emperor, Shogun and Marathon cultivars) florets grown under high irradiation dose of 8–16 mol/m2/day, detected a peak of 4-methylsulfinylbutyl-GL at low temperatures (6–12°C) with respect to high temperature range (16–22°C). However, a peak of indole-3-yl-methyl-GL content was detected at higher temperature range (16–22°C) and low irradiation dose (2 mol/m2/day). The levels of 2(R)-2-hydroxy-3-butenyl-GL, 2-hydroxy-4-pentenyl-GL, 1-methoxy-3-indolylmethyl-GL, 4-methoxy-3-indolylmethyl-GL, and 4-hydroxy-3-indolylmethyl-GL were not affected by temperature or radiation. However, those of 3-methylsulfinylpropyl-GL and 4-methylsulfinylbutyl-GL were considerably increased under low temperature and high irradiation (91). Conversely, Mølmann et al. (14) detected significantly higher 4-methylsulfinylbutyl-GL levels at 12°C than at 18°C in the florets of broccoli grown under long-day photoperiod, while the differences were non-significant under short-day photoperiod. 3-methylsulfinylpropyl-GL instead exhibited an opposite trend, with significantly higher levels at 18°C than at 12°C under a short-day and non-significant differences under a long-day photoperiod. Generally, total aliphatic GL content, was 33% higher under long-day at 12°C than 18°C, while no-significant difference was found under short-day photoperiod. Regarding indole GLs, the contents of 4-hydroxy-indole-3-yl-methyl-GL and 1-methoxy-indole-3-yl-methyl-GL were greater at 18°C under short-day than under long-day photoperiod, whereas no differences were registered between photoperiods when temperature decreased to 12°C. Generally, total indole GLs were mostly affected by temperature and their content increased by 24% at 18°C with respect to 12°C. The discrepancy in the observed responses may be ascribed to the different optimum preferences for light and temperature of the Gs metabolic enzymes and isoforms (14, 44). It also seems that even the variation between day/night temperatures may also influence the content of GLs in broccoli. Previously, Steindal et al. (93) monitored the contents of individual GLs in broccoli florets grown at different temperatures (15/9 and 21/15°C) and day length (12 and 24 h). The authors revealed that 4-methylsulphinylbuty-GL was unaffected by variations in temperatures (15/9 and 21/15°C). 4-methoxy-3-indolylmethyl-GL and indole-3-yl-methyl-GL were unaffected by day length (12 and 24 h). Generally, high day/night temperatures were associated with peaks in total GLs (31.5–33.2 mg/g dw) regardless of the photoperiod. Under greenhouse conditions at 7–13°C, daily mean temperatures were associated with a radiation of 10–13 mol/m2/day; Schonhof et al. (91) noticed an 8-fold increase in methylsulphinylbuty-GL and 3-methylsulphinylpropyl-GL content and a decrease in 3-indolylmethyl-GL.
With regard to seasonal variation, discrepant results and conclusions are drawn, which reflects the complexity of interacting factors shaping the levels of GLs in broccoli. Charron et al. (94) found that 4-methylsulfinylbutyl isothiocyanate content was higher in fall harvests than in spring in broccoli cultivars Brigadier and Emperor. However, studying the effect of seasonal variation on the content of GLs in florets, leaves, and stems of various broccoli cultivars (05-C3, AMaGi, BaeRiDom, CheongJae, Diamond, Grace, Grandeur, JikNok No. 28, NokJae, NokYeom No. 1, TS-2319, and YuDoRI No. 1), Bhandari and Kwak (75) revealed that the highest total GL content was measured in broccoli florets grown in spring (2.26–17.81 μmol/d dw) compared to the fall season (3.17–13.8 μmol/d dw). Conversely, in broccoli stems, higher total GL content was measured in fall (2.91–7.92 μmol/d dw) compared to spring season (1.04–5.74 μmol/d dw). Nevertheless, 4-methylsulfinylbutyl-GL was the main GL in all cultivars regardless of the growing season. Unlike Bhandari and Kwak (75), Pék et al. (95), studying the effect of harvest season (spring/autumn) on 4-methylsulfinylbutyl isothiocyanate concentration of broccoli florets (cultivar Parthenon) under different irrigation regimes and sulfur supply, revealed greater 4-methylsulfinylbutyl isothiocyanate content in fall (32.2–99.9 mg/kg fw) than in spring (9.9–13.3 mg/kg fw) harvests. Differences were also evidenced among first-fall and last-fall harvests, with the latter showing 164–261% higher methylsulfinylbutyl isothiocyanate depending on the applied treatments. This might suggest differences in optimal temperature required for the functional enzymes required for the synthesis of particular GLs in different cropping seasons. According to Verkerk et al. (44), higher levels of total and individual GLs are measured in late (August–January) compared to early (April–July) cropping seasons.
Agricultural Practices
Irrigation and fertilization are known to alter the balance between nitrogen and sulfur in the soil, thus presumably altering the content and profile of GLs among other secondary metabolites in plants (86, 96, 119). In addition, controlled pre-harvest salinity stresses have also been applied to improve the yield and health-promoting bioactivity of various fresh products and maintain long shelf-life for minimally processed and processed products (120, 121).
Generally, discrepant conclusions are available regarding the effect of water supply on the GL content in Brassica, and it seems that it is species-dependent with implication of other environmental factors. Low rainfall increased the levels of methylsulphinylbuty-GL and methylsulphinylbuty-isothiocyanate in most of the Brassica vegetables but decreased their levels in red radish roots. A similar result was also obtained by Radovich et al. (122), who reported that total and individual GLs were also lower in irrigated than non-irrigated cabbage during head development.
Previously, Vallejo et al. (88) found cultivar-dependent response on total GL content of marketable broccoli heads (56 DAT) under rich and poor sulfur fertilization. In cultivars Monterrey and Vencendor, a ~10% decrease and ~69% increase of total GL content was associated with rich sulfur supply, respectively, while GL content in cultivar Marathon remained statistically unchanged. However, Rangkadilok et al. (86) revealed a significant increase (50–70%) in 4-methylsulfinylbutyl-GL content in florets and whole plant fractions (cultivar Marathon) under low (23 kg S/ha) and high (92 kg S/ha) sulfur supply regimes, respectively, throughout maturity stages of the three broccoli cultivars namely Claudia, Marathon, and TB-234. Additionally, the levels of 4-methylsulfinylbutyl-GL content were more responsive to sulfur supply in cultivar Claudia than cultivar Marathon or TB-234. Zaghdoud et al. (96) found considerable decrease (60–80%) in 3-butenyl-GL content with decreasing /. However, the levels of indole-3-yl-methyl-GL and 1-methoxy-3-indolylmethyl-GL, as well as total indole GLs, significantly increased with decreasing / ratio and elevated CO2 concentrations, suggesting the need for a careful consideration of N-fertilization under projected climatic change for a proper management of broccoli nutritional quality.
Agricultural management is also known to quantitatively and qualitatively affect crop production and the content of secondary metabolites GLs among others. Once again, the effect on GLs is controversial between conventional and organic farming practices. The simple application of olive tree pruning-based biochar increased GL content in broccoli florets with respect to conventionally fertilized treatments, which registered the lowest concentration of 1-methoxy-3-indolylmethyl-GL and methylsulphinylbuty-GL (97). A similar result was also reported by Meyer and Adam (98), where organically grown broccoli exhibited significantly higher amounts of 3-indolylmethyl-GL than their conventionally grown counterparts. However, Vicas et al. (99) noticed 240 and 6% higher 3-indolylmethyl-GL and 1-methoxy-3-indolylmethyl-GL content under conventional compared to organic farming.
Priming and elicitation are strongly recommended eco-friendly agricultural approaches for agricultural production under actual global climate change (123). A number of agents, such as cold, drought, water, inorganic salts, sugars, solid medium with water and various nutrients, beneficial microbes, micronutrients, hormones (JA, MeJA, SA, and ABA), rhizobacteria, as well as organic sources (112, 124–128), are used for fruit and vegetable seed priming. Discrepant conclusions are drawn regarding the effect of NaCl for different broccoli cultivars even when the same treatments are applied. Under moderate salinity stress, Machado and Serralheiro (100) reported an accumulation of GL content and polyphenol contents, leading to an overall improvement of the nutritional quality of broccoli florets. Conversely, López-Berenguer et al. (101) noticed an increase in GL levels in broccoli florets (cultivar Marathon) disregarding the concentrations of NaCl applied (40 or 80 mM). This discrepancy can be ascribed to the date of application of the salt stress and the resistance of the cultivar to such stress. Similarly, Zaghdoud et al. (102) even found an opposite response among two broccoli cultivars (Parthenon and Naxos) to salinity stress (0, 30, 60, and 90 mM NaCl). In fact, while the content of total GL significantly decreased in Naxos due to a sharp decline in the content of indole GLs, it was practically unaffected in Parthenon. Nevertheless, under the applied stress, florets of Naxos accumulate and store higher aliphatic GLs compared to Parthenon, suggesting the possible use of this cultivar with improved health-promoting bioactivity under salinity stress. Similarly, Del Carmen Martínez-Ballesta et al. (43), also using the cultivar Parthenon, found that the content of aliphatic GLs (methylsulphinylbuty-GL and 4-methylthiobutyl-GL) generally increased whereas indole GLs (4-hydroxy-3-indolylmethyl-GL, 3-indolylmethyl-Gl, 4-methoxy-3-indolylmethyl-GL, and 1-methoxy-3-indolylmethyl-GL) decreased in plant grown under salinity stress. However, Sarikamiş and Cakir (103) noticed a decrease in 4-methylsulfinylbutyl-GL and 4- 4-methoxy-3-indolylmethyl-GL in cultivar Marathon by 61 and 74%, respectively, 1 day following 80 mM saline treatment, whereas indole-3-yl-methyl-GL and 3-methylsulfinylpropyl-GL decreased by 65 and 70% respectively, at day 6 compared to the control plants. Generally, except for 4-methoxy-3-indolylmethyl-GL, the authors noticed a decreasing trend for all GLs between 40 and 80 mM followed by a slight increase of all individual GLs. The increase might be due to de novo synthesis of GLs once input substrates are available following catabolic reactions affecting other molecules. Moreno et al. (104) pointed out that the combination of salinity and foliar sprayed elicitors (methionine, tryptophan, and chitosan) may increase the content of various secondary metabolites in broccoli florets produced under hydroponic conditions. The effect of salinity stress on GLs seems also to be dependent upon the growth stage of broccoli head. Di Gioia et al. (105) reported an increase in indole GLs (3-indolylmethyl-GL and 1-methoxy-3-indolylmethyl-GL) following irrigation of broccoli florets with saline water (4 dS/m) from transplanting to appearance of the inflorescence. However, total GLs remained unchanged in broccoli heads exposed to the same salinity stress from transplanting to harvest.
Methyl jasmonate and salycilic acid function as signaling molecules; their synthesis is triggered following tissue wounding and aggressor attacks (44), eliciting cascades of reactions leading to the accumulation of various secondary metabolites, such as GLs, among others, as a defensive response. At sprouting stage, Baenas et al. (106, 107) comparatively evaluated the effect of phytohormones (25–250 μM JA or MeJA), sugars (277 mM glucose, 146 mM sucrose), methionine (1–10 mM). and SA (100 μM) in eliciting GL biosynthesis and revealed that most of the applied treatments increased the total GL contents, though differentially, with MeJA resulting the most effective response even at low concentrations. Aliphatic GLs, such as 4-methylsulfinylbutyl-GL and indole GLs, such as indole-3-yl-methyl-GL, 4-methoxy-3-indolylmethyl-GL, and 1-methoxy-3-indolylmethyl-GL levels were, interestingly, increased by 48–247% following 25 μM MeJA treatments, with 4-methylsulfinylbutyl-GL rising from 183 to 294 mg/100 g fw. Following broccoli microgreens treatment with 10 mM CaCl2, a significant increase in indole GLs (indole-3-yl-methyl-GL, 1-methoxy-3-indolylmethyl-GL, and 4-hydroxy-3-indolylmethyl-GL) and aliphatic GLs (4-methylthiobutyl-GL, 3-methylsulfinylpropyl-GL, 3-methylthiopropyl-GL, and 4-methylsulfinylbutyl-GL) content was observed, while a low concentration of 1 mM CaCl2 was found ineffective (108, 109). A similar response was observed in broccoli florets by Ku et al. (110) who reported an increase in the biosynthetic activity of some GL degradation products like 4-methylsulfinylbutyl isothiocyanate, neoascorbigen, N-methoxyindole-3-carbinol, and phenethyl isothiocyanate in broccoli florets subjected to pre-harvest application of 500 ppb MeJA and post-harvest treatments with 1-methylcyclopropene assessed in fresh- and in 10–30 days post-harvest stored florets at 4°C, with particular increase in the levels of β-thioglucoside glucohydrolases after application of MeJA (110), whereas Yang et al. (111) noticed a 50% increase in 4-methylthiobutyl-GL as compared to the control in 10 mM CaCl2-treated broccoli sprouts. Hassini et al. (112), applying various priming/eliciting agents (K2SO4 and NaCl solutions, MeJA, SA and methionine), to broccoli plants, reported a 19% increase in total aliphatic GL content after K2SO4 application, mainly ascribed to a peak in methylsulphinylbuty-GL and 765% increase in total indole GL content following MeJA foliar spray application. However, a 99% decrease in indole-3-yl-methyl-GL levels occurred following all the treatments with the greatest decrease (99%) following KCl priming. A similar trend was also monitored following the pre-harvest treatment of broccoli (Diplomat and Gypsy cultivars) sprouts, young leaves, and florets, with 25 μM sodium selenate (Na2SeO4), which decreased the levels of amino acids involved in the synthesis of both aliphatic- and indole GLs in broccoli florets, and a 50% decrease in 4-methylsulfinylbutyl-GL and 1-methoxy-3-indolylmethyl-GL were consequently observed (113). This fact may be ascribed to competitive uptake between Se and S in plants.
Under prolonged UV-B exposure, at least four genes involved in GL biosynthesis were downregulated in Arabidopsis, whereas short-term exposure led to enhanced GL content, probably following the activation of jasmonic acid and salicylic acid pathways (129). Pre-harvest and/or supplementary UV radiation might be also beneficial in increasing levels of health beneficial substances and altering the pathways related to the biosynthesis of various secondary metabolites, particularly nitrogen-containing molecules (115, 130, 131). The pre-harvest treatment of broccoli sprouts treated with UV-B radiation at doses ranging from 0.3 to 1 kJ/m2/d prompted an increase in the levels of both aliphatic and indole GLs (114). Using a combined treatment (10 mM CaCl2 and 0.18 Wh/m2 UV-B) on young broccoli microgreens (shoots of the young plant), an increase in GL content correlated essentially with an increase in singrin content at 8.8 kJ/m2/d was obtained (115).
Post-harvest Factors Affecting GL Content
Generally, fresh is used for freshly harvested products. However, those sold in various markets and shops were already prone to various post-harvest factors at different levels. Several post-harvest factors have significant effect in altering broccoli GLs in terms of quality and quantity. These include the storage, processing, and packaging. An overview of the current state of research and of the key results is given in Table 2.

Table 2. The effect of post-harvest manipulations on the level of broccoli glucosinolates (GL class, individual GLs, and/or GL biosynthetic enzymes).
Storage
Broccoli is a highly perishable crop due to its high respiration rate. This results in important economic losses and poses a challenge to post-harvest storage, logistics, and supply management. Therefore, storage conditions play a fundamental role in slowing down fresh product decay and preserving quality. Contrasting reports regarding the effect of storage on GLs are available and it seems that the effect of storage conditions on GL content differs among heads and florets. An increase in various GLS was observed in broccoli florets and heads stored under different conditions. Increased levels of 4-hydroxy-3-indolylmethy-GL and 4-methoxy-3-indolylmethyl-GL were detected in 48 h stored broccoli heads at room temperature and in broccoli (cultivar 1997) florets stored under controlled atmosphere at three different temperatures (5, 10, and 18°C) (132). 4-methylsulfinylbutyl-GL content increased in broccoli florets after 6-days storage followed by a decreasing trend regardless of temperature (0, 5, and 10°C), and their content can be preserved for 12 days' storage under 0–5°C (133). Similarly, Baenas et al. (134) monitored a 10-fold increase in the levels of 1-methoxy-3-indolylmethyl-GL in broccoli heads stored for 9 days at 1–2°C and 85–90% RH.
The content of 4-methylsulfinylbutyl-GL isothiocyanate exhibited a post-harvest increase in 6-benzylaminopurine (6-BA) treated (135) and in 500 μL/L ethanol treated (136) broccoli florets, suggesting the advantageous application of such procedures for double purpose of extending shelf-life while increasing the nutritional quality of broccoli florets. Ku et al. (110) reported also an increase in 4-methylsulphinylbutyl-GL and 2-Phenylethyl-GL after pre-harvest MeJA and a preservation of 3-indolylmethyl-GL content after 1-methylcyclopropene (1-MCP) application to 10–30 days-stored broccoli florets at 4°C, which suggests the useful application of those treatments in controlling the nutritional quality of stored broccoli. 4-hydroxy-3-indolylmethyl-GL content exhibited an 84% increase when whole local market broccoli heads were stored at 20°C for 24 h, but the application of exogenous ethylene (1,000 ppm) for 24 h increased the concentration of 4-methylsulfinylbutyl-GL and 4-hydroxy-3-indolylmethyl by ~52 and ~223%, respectively, compared to freshly harvested samples (137). Also, considerable increase in the levels of 1-methoxy-3-indolylmethyl-GL, attaining 193 and 286%, respectively, was detected in wounded heads in response to ethylene or MeJA treatments. This is mainly explained by the activation of CYP81F genes transcription producing methoxylated derivatives of indole-3-yl-methyl-GL and hydroxylated derivatives (4-hydroxy-3-indolylmethyl-GL) following ethylene and MeJA application (137).
The use of melatonin (N-acetyl-5-methoxytryptamine) in agriculture has increased particularly since it protects plant from various biotic and abiotic stresses by modulating key physiological process (183, 184). The application of melatonin to broccoli florets at concentrations ranging from 1 to 100 μM has been associated with significant increase in 4-methylsulfinylbutyl-GL and 4-methylsulfinylbutyl isothiocyanate contents and was also detected with synchronized upregulation of various GL biosynthetic genes, such as Elong, CYP83A1, MYB28, UGT74B1, and FMOGS-OX1, and downregulation of AOP2. Miao et al. (138) noted the additional activation of BoTGG1 expression in broccoli florets treated with 1 μM melatonin and stored at room temperature.
Under other particular conditions, a decrease affecting different classes of GLs and individual GLs was also reported. Vallejo et al. (139) noticed up to an 80% decrease in total GL content of broccoli florets (cultivar Marathon) subjected to 1 week of pre-storage at 1°C and 3 days' storage at 15°C. Similarly, Yuan et al. (140) found a considerable decrease in total GLs in 1-methylcyclopropene-treated broccoli florets and stored for 5 days at 20°C. Xu et al. (141) reported that the decrease in GLs and 4-methylsulfinylbutyl isothiocyanate contents of broccoli (cultivar Youxiu) florets were suppressed by sucrose treatment throughout the 4-days storage period at 20°C and 95% RH. Similarly, Baenas et al. (134) noticed a 50% decrease in indole-3-yl-methyl-GL and 4-methoxy-3-indolylmethyl-GL, with concomitant increase (up to 10-fold) in the level of 1-methoxy-3-indolylmethyl-GL throughout 9 days' storage of broccoli heads stored at 1–2°C and HR of 85–90%.
In other experiments, the content of GLs remained unchanged or little affected, such as cold storage of broccoli florets for 5 days at 4°C, for 7 days at 4–8°C, or for 2 days at 25°C with subsequent exposure to fluorescent light (142–145). Similarly, 4-methylsulfinylbutyl-GL content remained unchanged in broccoli (cultivar Marathon) heads stored at temperatures of 1 or 4°C, 99% relative humidity (RH), for 2, 7, 14, or 28 days, followed by exposure to 8, 15, or 20°C, with 99, 90, or 70% RH, respectively, for 3 days (146). No significant changes in the contents of total GLs were found during pre-storage of broccoli florets (cultivar Marathon) at 0 or 4°C for 4 or 7 days (82). Therefore, the β-thioglucoside glucohydrolases' hydrolytic activity and the GLs' biosynthetic activity are key forces driving the GL level of stored broccoli florets (140, 182). The proper use and application of different post-harvest treatments might ensure a better control of GL content and fresh produce with high nutritional value and beneficial health effects. Treatment with essential oils is also a promising eco-friendly practice to improve the storability and conservation of many horticultural crops (185). El-Awady et al. (147) evaluated the feasibility in using fennel, caraway, basil, thyme, and sage essential oils to preserve the horticultural traits and the GL levels in broccoli sprouts during storage at 4°C and 95% RH. The authors noticed an increase in antioxidant content of broccoli sprouts following the application of all tested essential oils compared to the control. In thyme essential oil-treated sprouts, there was 2.18% loss in GLs compared to more than 49% in non-treated sprout samples at the end of the storage period (2 weeks).
Post-harvest light treatments of broccoli sprouts and florets under storage were reported to trigger changes affecting GL biosynthesis (109, 186). At early stages, it also seems that the beneficial effect of UV-B treatment is dependent upon CaCl2 concentration when combined. There was a decrease (57%) in 4-methylthiobutyl-GL content after the treatment of microgreens with 1 mM CaCl2 spray and UV-B. However, when microgreens were treated by higher CaCl2 concentration of 10 mM, an increase in aliphatic GLs and 35–43% decrease in GL degradation rate throughout 21 days was noticed regardless of the applied light treatment (109). In mature florets, 3-methylsulfinylpropyl-GL, 4-methylsulfinylbutyl-GL, and total aliphatic GL content increased, respectively by 130, 99, and 102% after exposure to 7 days' pre-storage at 0°C followed by 3 days' storage at 10°C under visible light of 13 and 25 μmol/m2/s, compared to freshly harvested samples. However, under higher visible light intensity (25 μmol/m2/s) and higher storage temperature (18°C), 4-hydroxy-3-indolylmethyl-GL increased considerably in broccoli heads exposed to a 7-days pre-storage period at 0°C and 3-days' storage at 10°C (82). Darré et al. (148) reported differential response of GLs according to the applied treatment, storage condition, and duration while assessing the effect of various UV-B irradiation intensities (low to high) ranging from 0 to 5 W/m2 and doses ranging from 0 to 12 kJ/m2 on the overall nutritional quality of broccoli florets throughout 17 days of storage at 4°C. The short-term response was an accumulation of 4-methylsulfinylbutyl-GL in broccoli florets until 18 h after UV-B treatment. However, UV treatments, UV-B treatments, or their combination was ineffective in altering total GLs for samples subjected to 4–7 days' dark pre-storage, subsequent 10°C 3-days storage, and left in the dark.
Processing and Minimal Processing
Before being consumed, vegetables crops of the Brassica groups are jeopardized by different home and industrial processing. It has been reported that treatments, such as slicing, cooking, steaming, frying, and microwaving significantly affect the levels of GL content in broccoli. Naturally, postharvest physical injuries of plant tissue after grinding, slicing, mixing, juicing, cooking, and frosting/defrosting prompt an activation of the myrosinase-GL system, which leads to the accumulation of isothiocyanates and GLs degradation products (149–152). Torres-Contreras et al. (150) reported that cutting of broccoli heads into florets and subsequent incubation for 24 h at 20°C triggered an increase attaining 490% in total GLs, 4,200% in 4-hydroxy-3-indolylmethyl-GL, and 1,300% in 1-methoxy-3-indolylmethyl-GL contents. Similarly, Verkerk et al. (44, 153) observed a 3.5- and 2-fold increase in 4-hydroxy- and 4-methoxy-3-indolylmethyl-GL, respectively, after chopping and storage of broccoli florets, but a significant decline of most GLs. This increase was reported as wound-induced de novo GL biosynthesis triggered by ethylene (4-hydroxy-3-indolylmethyl-GL and 1-methoxy-3-indolylmethyl-GL) and by ROS (4-hydroxy-3-indolylmethyl-GL, indole-3-yl-methyl-GL, and 1-methoxy-3-indolylmethyl-GL) (150).
The use of ultrasound leads to microorganisms' and enzymes' inactivation, but might also damage plant tissues if applied at high frequencies and trigger a stress response accordingly. Aguilar-Camacho et al. (154) reported an increase of 187% in 4-hydroxy-3-indolylmethyl-, 112% in 4-methylthiobutyl-, 755% in 2-phenylethyl-, and 232% in 1-methoxy-3-indolylmethyl-GL by a combined treatment with 250 ppm MeJA and ultrasound treatment (20 min, frequency 24 kHz, amplitude 100 μm) after 3 days' storage at 15°C. Collazo et al. (151) noted that 2-phenylethyl- and 2-phenylethyl-, 4-methylsulfinylbutyl-, and 2(R)-2-hydroxy-3-butenyl-GLs were significantly increased 24 h after pulsed-light treatment (1.5 kJ/m2 UV-C), water-washing, or chlorine-sanitizing of fresh-cut broccoli florets. The exposure of fresh-cut broccoli to three UV-C light pulses of 1.5 kJ/m2 revealed a 132% increase in 4-methylsulphinylbutyl-GL and 122% in 4-methylsulphinylbutyl isothiocyanate but almost 50% decrease in 2-phenylethyl-GL contents after 24 h. A single treatment using UV-B (15 kJ/m2) and a combination of UV-B (15 kJ/m2) + UV-C (9 kJ/m2) induced the highest 4-methylsulphinylbutyl-GL content (131 and 117 mg kg−1) in Bimi broccoli florets after 72 h (83). It is widely recognized that UV-B treatment upregulates the biosynthesis of GLs (114).
The interest in non-thermal food processing, such as high-pressure processing, by consumers is increasing due to an increasing concern about the beneficial effect of natural antioxidants generally found in fresh produce. The proper application of this technology helps inactivate food pathogens at ambient temperature, preserving nutritional quality and therefore the shelf-life of the final product. Inconsistent conclusions are also drawn regarding the effect of high-pressure processing on GL content. Using a high pressure in the range of 100–600 MPa, Westphal et al. (152) noticed their beneficial effect on sprouts' GLs-myrosinase system, with a conversion rate of GLs to isothiocyanate attaining 18% until 300 MPa and 85% upwards. It has been reported that broccoli myrosinase remains active until 45°C but loses more than 90% activity after 10 min at 70°C (155). Van Eylen et al. (155) reported that the highest conversion rate of 4-methylsulphinylbuty-GL to methylsulphinylbuty-isothiocyanate in broccoli florets was attained after 15 min using a pressure of 200–500 MPa at 40°C and after 35 min using 300 MPa. However, the conversion rate significantly decreased, most likely due to enzymatic inactivation of myrosinase. Besides, it has been reported that 4-methylsulphinylbutyl-isothioyanate in vegetal matrix remains stable at room temperature or at 75°C and up to 600 MPa pressure (156). However, Vallejo et al. (157), comparing ordinary and high-pressure cooking, reported significant (33–55%) decrease in total GLs after both treatments, affecting mostly the indole rather than the aliphatic class. This may be attributed to cooking temperature rather than pressure intensity. Martínez-Hernández et al. (158) found a 40–80% decrease in total GLs after low-pressure steaming as well as boiling and sous vide of a new kailan-hybrid broccoli. Tríska et al. (159) observed a significant decrease in isothiocyanates content of different cruciferous vegetables-based juices with pasteurization. However, their level remained practically unchanged after freezing. In an innovative broccoli (cultivars Parthenon and Bimi) hummus prepared and subjected to microwaving of 9 kW/40 s or high-pressure processing of 550 MPa/10 min/23°C, Klug et al. (160) noticed 110% higher 4-methylsulphinylbuty-GL after high-pressure processing of Parthenon-based hummus, while 4-methylsulphinylbutyl-isothiocyanate was better maintained until 4 weeks at 5°C after microwaving.
Pulsed electric fields (PEF) have been also proposed as a non-thermal food-grade technology to improve bioactive compounds biosynthesis and reduce microbial load without deleterious effect on the processed product (161, 187, 188). An increase of 187–212% in GL content in broccoli florets and 111–203% in stalks was reported after exposure to 4 kV/cm for 525 and 1,000 μs (161). However, Frandsen et al. (162) noted an increasing enzymatic activity of β-thioglucoside glucohydrolases after PEF treatment of broccoli purée and juice, leading to the degradation of most GLs. The decrease affecting 4-methylsulfinylbutyl-GL attained 71% 24 after juicing, which stresses the need for the application of stabilization agents, such as cyclodextrins to encapsulate valuable compounds in broccoli juice (163).
Thermal processing induces changes in cell-wall structural components, nutrients (including proteins and vitamins), and non-nutritive bioactive components, such as GLs and polyphenols. GLs are relatively stable under heat treatment and their hydrolysis is mainly enzymatic. Nevertheless, it should be highlighted that GL degradation products are responsible for their bioactivity. It seems that the effect of heat treatment on GL content is dependent upon genotype, food matrix, the chemical class of GL, the temperature, and the duration of the treatment (166, 189). At mild heat treatment, aliphatic GLs are unaffected but indole GLs decreased considerably (90%) (164, 190). The loss in total GLs was 27–30% after blanching and increasing to 72% after cooking with a complete inhibition of β-thioglucoside glucohydrolases activity (19, 158, 165, 166). It has been noted that 4-methylsulfinylbutyl-isothiocyanate and the nutritional value of broccoli florets was better preserved after steaming for 1–3 min than after blanching, microwaving, heating, or boiling (45, 77, 166–169). Similarly, boiling of green and purple cauliflower for 15 min at 100°C significantly reduced not only GLs, but also their degradation products (170). In other experiments, treatment at 41°C for 180 min or 47°C for 12 min significantly increased indole GLs in processed broccoli (cultivar Diplomat) florets (171), most likely due to an ROS-induced accumulation triggered by heat stress. At a higher temperature of 60°C a significant loss in total GLs occurred (134). The effect of temperature and other factors on GL content in broccoli is complex since other food matrixes present might also influence the final content of GLs during processing. The effect of temperature on GL content is also dependent on the type of vegetal matrix. In fact, in different pak choi cultivars, boiling for 15 min, reduced the GL content by 54–82.2% with respect to control, while their content remained unchanged after steaming. Dekker et al. (172) also reported differential thermal stability of the same GLs in different Brassica crops consisting of red cabbage, broccoli and Brussels sprouts, pak choi, and Chinese cabbage. 3-indolylmethyl-GL and 4-methoxy-3-indolylmethyl were most stable in red cabbage, while 3-butenyl-GL was most stable in broccoli after 120 min at 100°C.
Giambanelli et al. (173) reported a positive and protective interaction of onion with broccoli florets at boiling temperature leading to significantly higher preservation of GLs in a processed broccoli/onion system than in broccoli, suggesting the possible use of such systems in improving the health-promoting bioactivity of processed broccoli products. Under sous-vide boiling, Martínez-Hernández et al. (158) found an 80% loss in GL content of new kailan-hybrid broccoli. Generally, hydroxylated GLs are less stable than aliphatic under high temperature (100–130°C) and more degraded in basic medium than neutral and slightly acidic medium (174).
Packaging
Controlled atmosphere storage and modified atmosphere packaging are also a practical alternative for preserving Brassica vegetables' quality and marketability during a longer shelf-life (44, 191) with respect to ordinary canning leading to considerable loss of GLs following their thermal degradation (44). It has been reported that polyethylene-, polypropylene-, and polystyrene-MAP better preserved aliphatic, indole, and aromatic GLs as well as the nutritional quality of broccoli florets of various cultivars (Parthenon, Aishwarya, and Lord F1) stored until 3 weeks, even at relatively higher temperatures (144, 175–177). Similarly, acceptable sensory quality texture and antioxidant composition of broccoli florets was maintained up to 25 days' storage after using an innovative MAP made of gas promoter-gas barrier blending materials, maintaining an internal atmosphere of 8.2% CO2 and 2.0% O2 (178). Hansen et al. (179) monitored a 21–42% increase in total GLs, respectively, after storage under controlled atmosphere of 0.5% O2 + 20% CO2 and air with respect to fresh florets. Individual GLs, such as 3-indolylmethyl-GL, levels declined by 35% in broccoli stored 7 days at 10°C under 0.5% O2 + 20% CO2 or 20% CO2, whereas 4-methoxy-3-indolylmethyl-GL levels increased during storage under low O2 atmosphere and increased further after transfer to air. Rangkadilok et al. (192) measured a 55–56% decrease in 4-methylsulphinylbuty-GL in broccoli stored in open boxes after 3 days and plastic bags after 7 days at 20°C, respectively. However, modified atmosphere packaging at 4°C preserved the 4-methylsulphinylbuty-GL level practically unchanged for up to 10 days with respect to air control packaging. Dissimilar dynamic changes in GL content of 7-days stored broccoli and cauliflower mix under controlled atmosphere packaging has been also reported by Schreiner et al. (180). Aliphatic GLs were preserved in cauliflower florets but decreased in broccoli florets under 1% O2 + 21% CO2 and 8% O2 + 14% CO2 at 8°C. Similarly, Schreiner et al. (181) reported discrepant response to storage under modified atmosphere between mini broccoli and mini cauliflower since aliphatic and indole GLs were better preserved under 8% O2 + 14% CO2 in mini broccoli for 7 days but at 1% O2 + 21% CO2 in mini cauliflower for 7 days, which highlights the suitable separate packaging of such agricultural crops to avoid the decrease in health-promoting bioactivity. Modified atmosphere packaging treatments maintained the levels of individual aliphatic and indole GLs in broccoli florets relative to those unwrapped florets stored in open boxes, and longer shelf-life with improved GL content was better achieved using less perforated polyethylene bags and lower temperature (13 days at 4°C) (182).
Conclusions
GLs and their hydrolytic products isothiocyanates are increasingly attracting interest due to their various health benefits since their consumption was correlated with lower risks of various chronic degenerative diseases. As demonstrated, the levels of total or individual GLs from different classes may possibly fluctuate differently when exposed to various pre- (genotypes, growing environment, cultural practices, ripening stage, etc.) and post-harvest manipulations and agricultural procedures (harvesting, post-harvest treatments, packaging, storage, etc.) (Figures 8, 9). The understanding of these traits is crucial to improve, preserve, and maintain the nutritional quality of broccoli florets. These data are critical for breeders, processors, and producers willing to (1) improve the levels of GLs in broccoli genotypes via new breeding programs integrating large number of broccoli lines and good agricultural practices, such as salinity, water stress, and various elicitors; (2) adopt post-harvest procedures maintaining fresh-like quality and significant gain in GLs, such as refrigerated storage and UV treatments; and (3) adopt processing techniques and treatments leading to minimal loss of these highly bioactive compounds in broccoli genotype, such as pulsed light treatment, pulsed electric field, and UV radiation.
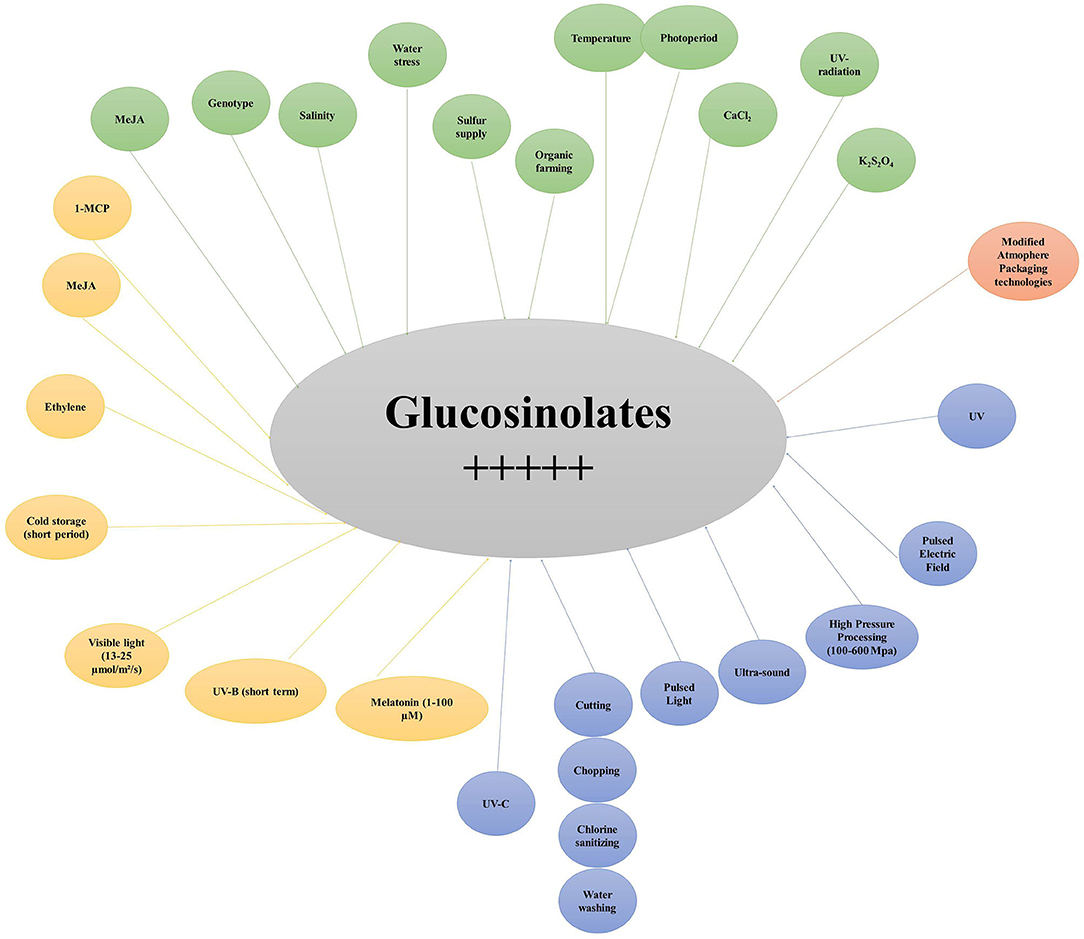
Figure 8. Major pre- and post-harvest factors positively affecting glucosinolate content in broccoli. MeJA, Methyl Jasmonate; 1-MCP, 1-Methylcyclopropene; UV, ultraviolet; UV-B, ultraviolet B; UVC, ultraviolet C; K2SO4, Potassium sulfate.
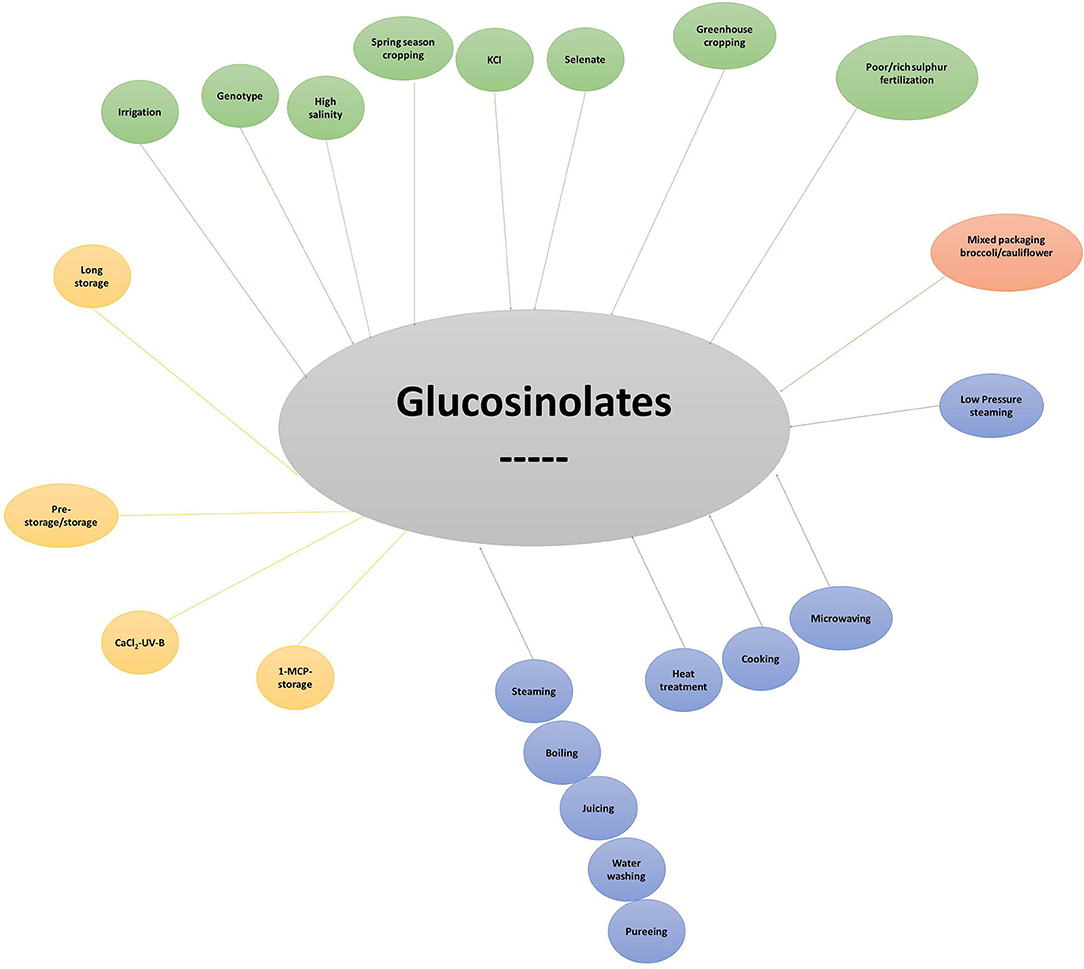
Figure 9. Major pre- and post-harvest factors negatively affecting glucosinolate content in broccoli. KCl, Potassium chloride; 1-MCP, 1-Methylcyclopropene, UV-B, ultraviolet B; CaCl2, Calcium Chloride.
Author Contributions
RI conceptualized the idea of this review. RI, IT, ZP, MS, ML, AM, TR'H, CH, HL, and FH scanned the literature, retrieved and processed papers referenced in the review, and wrote the manuscript. All authors critically reviewed the manuscript, enriched, developed the main parts, and contributed during the preparation of the tables and figures before submission.
Funding
This research was supported by the Higher Education Institutional Excellence Program (NKFIH-1159-6/2019/FEKUTSTRAT) awarded by the Ministry for Innovation and Technology within the framework of the water related research of Szent István University, Hungary.
Conflict of Interest
The authors declare that the research was conducted in the absence of any commercial or financial relationships that could be construed as a potential conflict of interest.
References
1. Gad N, Abd El-Moez MR. Broccoli growth, yield quantity and quality as affected by cobalt nutrition. Agric Biol J N Am. (2011) 2:226–31. doi: 10.5251/abjna.2011.2.2.226.231
2. Maggioni L, von Bothmer R, Poulsen G, Lipman E. Domestication, diversity and use of Brassica oleracea L., based on ancient Greek and Latin texts. Genet Resour Crop Evol. (2018) 65:137–59. doi: 10.1007/s10722-017-0516-2
3. Díaz-Pérez JC. Root zone temperature, plant growth and yield of broccoli [Brassica oleracea (Plenck) var. italica] as affected by plastic film mulches. Sci Hort. (2009) 123:156–63. doi: 10.1016/j.scienta.2009.08.014
4. Nooprom K, Santipracha Q. Growth and yield of broccoli planted year round in Songkhla province, Thailand. AJABS. (2014) 7:4157–61. doi: 10.19026/rjaset.7.781
5. Kałuzewicz A, Krzesiński W, Knaflewski M, Lisiecka J, Spizewski T, Fraszczak B. Effect of temperature on the growth of broccoli (Brassica oleracea L. var. italica Plenck) cv. Fiesta. Veg Crop Res Bull. (2012) 77:129–41. doi: 10.2478/v10032-012-0021-2
6. Nooprom K, Santipracha Q. Planting times and varieties on incidence of bacterial disease and yield quality of broccoli during rainy season in southern Thailand. Mod Appl Sci. (2013) 7:9–14. doi: 10.5539/mas.v7n12p9
7. Fahey JW. Brassica: characteristics and properties. In: Caballero B, Finglas PM, Toldrá F, editors. Encyclopedia of Food and Health. Florida, FL: Academic Press, Inc. (2016). p. 43–62.
8. FAO STAT. (2020). Available online at: http://www.fao.org/faostat/en/#data/QC (accessed May 28, 2020).
9. Broccoli Extract Market. Global Market Study on Broccoli Extract: Seed Extract Product Type to Exhibit Relatively High Market Attractiveness Index During 2017–2027. New York, NY: Persistence Market Research (2018).
10. Moreno DA, Carvajal M, López-Berenguer C, García-Viguera C. Chemical and biological characterisation of nutraceutical compounds of broccoli. J Pharmaceut Biomed. (2006) 41:1508–22. doi: 10.1016/j.jpba.2006.04.003
11. Kaulmann A, Jonville MC, Schneider YJ, Hoffmann L, Bohn T. Carotenoids, polyphenols and micronutrient profiles of Brassica oleraceae and plum varieties and their contribution to measures of total antioxidant capacity. Food Chem. (2014) 155:240–50. doi: 10.1016/j.foodchem.2014.01.070
12. Ueda K, Tsukatani T, Murayama K, Kurata Y, Takeda E, Otsuka T, et al. Determination of vitamin C, S-methylmethionine and polyphenol contents, and functional activities of different parts of broccoli (Brassica oleracea var. Italica). Nippon Shokuhin Kagaku Kogaku Kaishi. (2015) 62:242–9. doi: 10.3136/nskkk.62.242
13. Mazarakis N, Snibson K, Licciardi PV, Karagiannis TC. The potential use of l-sulforaphane for the treatment of chronic inflammatory diseases: a review of the clinical evidence. Clin Nutr. (2020) 39:664–75. doi: 10.1016/j.clnu.2019.03.022
14. Mølmann JA, Steindal AL, Bengtsson GB, Seljåsen R, Lea P, Skaret J, et al. Effects of temperature and photoperiod on sensory quality and contents of glucosinolates, flavonols and vitamin C in broccoli florets. Food Chem. (2015) 172:47–55. doi: 10.1016/j.foodchem.2014.09.015
15. Thomas M, Badr A, Desjardins Y, Gosselin A, Angers P. Characterization of industrial broccoli discards (Brassica oleracea var. italica) for their glucosinolate, polyphenol and flavonoid contents using UPLC MS/MS and spectrophotometric methods. Food Chem. (2018) 245:1204–11. doi: 10.1016/j.foodchem.2017.11.021
16. Latté KP, Appel K-E, Lampen A. Health benefits and possible risks of broccoli–an overview. Food Chem Toxicol. (2011) 49:3287–309. doi: 10.1016/j.fct.2011.08.019
17. Ezzat SM, El-Halawany AM, Hamed AR, Abdel-Sattar E. Role phytochemicals play in the activation of antioxidant response elements (AREs) and phase II enzymes and their relation to cancer progression and prevention. Stud Nat Products Chem. (2018) 60:345–69. doi: 10.1016/b978-0-444-64181-6.00009-7
18. Keck AS, Finley JW. Cruciferous vegetables: cancer protective mechanisms of glucosinolate hydrolysis products and selenium. Integr Cancer Ther. (2004) 3:5–12. doi: 10.1177/1534735403261831
19. Lafarga T, Bobo G, Viñas I, Collazo C, Aguiló-Aguayo I. Effects of thermal and non-thermal processing of cruciferous vegetables on glucosinolates and its derived forms. J Food Sci Technol. (2018) 55:1973–81. doi: 10.1007/s13197-018-3153-7
20. Ishida M, Hara M, Fukino N, Kakizaki T, Morimitsu Y. Glucosinolate metabolism, functionality and breeding for the improvement of Brassicaceae vegetables. Breed Sci. (2014) 64:48–59. doi: 10.1270/jsbbs.64.48
21. Fuentes F, Paredes-Gonzalez X, Kong ANT. Dietary glucosinolates sulforaphane, phenethyl isothiocyanate, indole-3-carbinol/3,3′-diindolylmethane: anti-oxidative stress/inflammation, Nrf2, epigenetics/epigenomics and in vivo cancer chemopreventive efficacy. Curr Pharmacol Rep. (2015) 1:179–96. doi: 10.1007/s40495-015-0017-y
22. Liu P, Wang W, Tang J, Bowater RP, Bao Y. Antioxidant effects of sulforaphane in human HepG2 cells and immortalised hepatocytes. Food Chem Toxicol. (2019) 128:129–36. doi: 10.1016/j.fct.2019.03.050
23. Kim JK, Park SU. Current potential health benefits of sulforaphane. EXCLI J. (2016) 15:571–7. doi: 10.17179/excli2016-485
24. Mokhtari RB, Baluch N, Homayouni TS, Morgatskaya E, Kumar S, Kazemi P, et al. The role of sulforaphane in cancer chemoprevention and health benefits: a mini-review. J Cell Commun Signal. (2018) 12:91–101. doi: 10.1007/s12079-017-0401-y
25. Brown RH, Reynolds C, Brooker A, Talalay P, Fahey JW. Sulforaphane improves the bronchoprotective response in asthmatics through Nrf2-mediated gene pathways. Respir Res. (2015) 16:106. doi: 10.1186/s12931-015-0253-z
26. Yanaka A. Role of sulforaphane in protection of gastrointestinal tract against H. pylori and NSAID-induced oxidative stress. Curr Pharm Des. (2017) 23:4066–75. doi: 10.2174/1381612823666170207103943
27. Bahadoran Z, Mirmiran P, Hosseinpanah F, Rajab A, Asghari G, Azizi F. Broccoli sprouts powder could improve serum triglyceride and oxidized LDL/LDL-cholesterol ratio in type 2 diabetic patients: a randomized double-blind placebo-controlled clinical trial. Diabetes Res Clin Pract. (2012) 96:348–54. doi: 10.1016/j.diabres.2012.01.009
28. Axelsson AS, Tubbs E, Mecham B, Chacko S, Nenonen HA, Tang Y, et al. Sulforaphane reduces hepatic glucose production and improves glucose control in patients with type 2 diabetes. Sci Transl Med. (2017) 9:eaah4477. doi: 10.1126/scitranslmed.aah4477
29. Kensler TW, Chen JG, Egner PA, Fahey JW, Jacobson LP, Stephenson KK, et al. Effects of glucosinolate-rich broccoli sprouts on urinary levels of aflatoxin-DNA adducts and phenanthrene tetraols in a randomized clinical trial in He Zuo township, Qidong, People's Republic of China. Cancer Epidemiol Biomarkers Prev. (2005) 14:2605–13. doi: 10.1158/1055-9965.EPI-05-0368
30. Boldry EJ, Yuan JM, Carmella SG, Wang R, Tessier K, Hatsukami DK, et al. Effects of 2-phenethyl isothiocyanate on metabolism of 1,3-butadiene in smokers. Cancer Prev Res (Phila). (2020) 13:91–100. doi: 10.1158/1940-6207.capr-19-0296
31. Ganguly A, Frank D, Kumar N, Cheng YC, Chu E. Cancer biomarkers for integrative oncology. Curr Oncol Rep. (2019) 21:32. doi: 10.1007/s11912-019-0782-6
32. Megna BW, Carney PR, Nukaya M, Geiger P, Kennedy GD. Indole-3-carbinol induces tumor cell death: function follows form. J Surg Res. (2016) 204:47–54. doi: 10.1016/j.jss.2016.04.021
33. Quirit JG, Lavrenov SN, Poindexter K, Xu J, Kyauk C, Durkin KA, et al. Indole-3-carbinol (I3C) analogues are potent small molecule inhibitors of NEDD4-1 ubiquitin ligase activity that disrupt proliferation of human melanoma cells. Biochem Pharmacol. (2017) 127:13–27. doi: 10.1016/j.bcp.2016.12.007
34. Dekić MS, Radulović NS, Stojanović NM, Randjelović PJ, Stojanović-Radić ZZ, Najman S, et al. Spasmolytic, antimicrobial and cytotoxic activities of 5-phenylpentyl isothiocyanate, a new glucosinolate autolysis product from horseradish (Armoracia rusticana P. Gaertn, B Mey & Scherb, Brassicaceae). Food Chem. (2017) 232:329–39. doi: 10.1016/j.foodchem.2017.03.150
35. Núñez-Iglesias MJ, Novio S, García-Santiago C, Cartea ME, Soengas P, Velasco P, et al. Effects of 3-butenyl isothiocyanate on phenotypically different prostate cancer cells. Int J Oncol. (2018) 53:2213–23. doi: 10.3892/ijo.2018.4545
36. Lippmann D, Lehmann C, Florian S, Barknowitz G, Haack M, Mewis I, et al. Glucosinolates from pak choi and broccoli induce enzymes and inhibit inflammation and colon cancer differently. Food Funct. (2014) 5:1073–81.
37. Baasanjav-Gerber C, Monien BH, Mewis I, Schreiner M, Barillari J, Iori R, et al. Identification of glucosinolate congeners able to form DNA adducts and to induce mutations upon activation by myrosinase. Mol Nutr Food Res. (2011) 55:783–92. doi: 10.1002/mnfr.201000352
38. Schumacher F, Florian S, Schnapper A, Monien BH, Mewis I, Schreiner M, et al. A secondary metabolite of Brassicales, 1-methoxy-3-indolylmethylglucosinolate, as well as its degradation product, 1-methoxy-3-indolylmethyl alcohol, forms DNA adducts in the mouse, but in varying tissues and cells. Arch Toxicol. (2013) 88:823–36. doi: 10.1007/s00204-013-1149-7
39. Sønderby IE, Geu-Flores F, Halkier BA. Biosynthesis of glucosinolates–gene discovery and beyond. Trends Plant Sci. (2010) 15:283–90. doi: 10.1016/j.tplants.2010.02.005
40. Hanschen FS, Lamy E, Schreiner M, Rohn S. Reactivity and stability of glucosinolates and their breakdown products in foods. Angew Chem Int Ed. (2014) 53:11430–50. doi: 10.1002/anie.201402639
41. Avato P, Argentieri MP. Brassicaceae: a rich source of health improving phytochemicals. Phytochem Rev. (2015) 14:1019–33. doi: 10.1007/s11101-015-9414-4
42. Malka SK, Cheng Y. Possible interactions between the biosynthetic pathways of indole glucosinolate and auxin. Front Plant Sci. (2017) 8:2131. doi: 10.3389/fpls.2017.02131
43. Del Carmen Martínez-Ballesta M, Moreno D, Carvajal M. The physiological importance of glucosinolates on plant response to abiotic stress in Brassica. Int J Mol Sci. (2013) 14:11607–25. doi: 10.3390/ijms140611607
44. Verkerk R, Schreiner M, Krumbein A, Ciska E, Holst B, Rowland I, et al. Glucosinolates in Brassica vegetables: the influence of the food supply chain on intake, bioavailability and human health. Mol Nutr Food Res. (2009) 53:S219–S219. doi: 10.1002/mnfr.200800065
45. Soares A, Carrascosa C, Raposo A. Influence of different cooking methods on the concentration of glucosinolates and vitamin C in broccoli. Food Bioproc Tech. (2017) 10:1387–411. doi: 10.1007/s11947-017-1930-3
46. Fernandes L, Saraiva JA, Pereira JA, Casal S, Ramalhosa E. Post-harvest technologies applied to edible flowers: a review: edible flowers preservation. Food Rev Int. (2019) 35:132–54. doi: 10.1080/87559129.2018.1473422
47. Possenti M, Baima S, Raffo A, Durazzo A, Giusti AM, Natella F. Glucosinolates in food. In: Mérillon JM, Ramawat K, editors. Glucosinolates. Cham: Springer (2016). p. 87–132. doi: 10.1007/978-3-319-26479-0_4-1
48. Kushad MM, Brown AF, Kurilich AC, Juvik JA, Klein BP, Wallig MA, et al. Variation of glucosinolates in vegetable crops of Brassica oleracea. J Agric Food Chem. (1999) 47:1541–8. doi: 10.1021/jf980985s
49. Miao H, Wang J, Cai C, Chang J, Zhao Y, Wang Q. Accumulation of glucosinolates in broccoli. In: Mérillon J-M, Ramawat KG, editors. Glucosinolates. Cham: Springer (2017). p. 133–62. doi: 10.1007/978-3-319-25462-3_16
50. Geu-Flores F, Møldrup ME, Böttcher C, Olsen CE, Scheel D, Halkier BA. Cytosolic γ-glutamyl peptidases process glutathione conjugates in the biosynthesis of glucosinolates and camalexin in Arabidopsis. Plant Cell. (2011) 23:2456–69. doi: 10.1105/tpc.111.083998
51. Gao J, Yu X, Ma F, Li J. RNA-seq analysis of transcriptome and glucosinolate metabolism in seeds and sprouts of broccoli (Brassica oleracea var. italic). PLoS ONE. (2014) 9:e88804. doi: 10.1371/journal.pone.0088804
52. Hanschen FS, Klopsch R, Oliviero T, Schreiner M, Verkerk R, Dekker M. Optimizing isothiocyanate formation during enzymatic glucosinolate breakdown by adjusting pH value, temperature and dilution in Brassica vegetables and Arabidopsis thaliana. Sci Rep. (2017) 7:40807. doi: 10.1038/srep40807
53. Palliyaguru DL, Yuan JM, Kensler TW, Fahey JW. Isothiocyanates: translating the power of plants to people. Mol Nutr Food Res. (2018) 62:e1700965. doi: 10.1002/mnfr.201700965
54. Zhao Z, Zhang W, Stanley BA, Assmann SM. Functional proteomics of Arabidopsis thaliana guard cells uncovers new stomatal signaling pathways. Plant Cell. (2008) 20:3210–26. doi: 10.1105/tpc.108.063263
55. Burow M, Atwell S, Francisco M, Kerwin RE, Halkier BA, Kliebenstein DJ. The glucosinolate biosynthetic gene AOP2 mediates feed-back regulation of jasmonic acid signaling in Arabidopsis. Mol Plant. (2015) 8:1201–12. doi: 10.1016/j.molp.2015.03.001
56. Francisco M, Joseph B, Caligagan H, Li B, Corwin JA, Lin C, et al. Genome wide association mapping in Arabidopsis thaliana identifies novel genes involved in linking allyl glucosinolate to altered biomass and defense. Front Plant Sci. (2016) 7:1010. doi: 10.3389/fpls.2016.01010
57. Francisco M, Joseph B, Caligagan H, Li B, Corwin JA, Lin C, et al. The defense metabolite, allyl glucosinolate, modulates Arabidopsis thaliana biomass dependent upon the endogenous glucosinolate pathway. Front Plant Sci. (2016) 7:774. doi: 10.3389/fpls.2016.00774
58. Atwell S, Huang YS, Vilhjalmsson BJ, Willems G, Horton M, Li Y, et al. Genome-wide association study of 107 phenotypes in Arabidopsis thaliana inbred lines. Nature. (2010) 465:627–31. doi: 10.1038/nature08800
59. Jensen LM, Jepsen HKS, Halkier BA, Kliebenstein DJ, Burow M. Natural variation in cross-talk between glucosinolates and onset of flowering in Arabidopsis. Front Plant Sci. (2015) 6:697. doi: 10.3389/fpls.2015.00697
60. Burow M. Complex environments interact with plant development to shape glucosinolate profiles. Adv Bot Res. (2016) 80:15–30. doi: 10.1016/bs.abr.2016.06.001
61. Liu S, Liu Y, Yang X, Tong C, Edwards D, Parkin IA, et al. The Brassica oleracea genome reveals the asymmetrical evolution of polyploid genomes. Nat Commun. (2014) 5:3930. doi: 10.1038/ncomms4930
62. Yi GE, Robin A, Yang K, Park JI, Kang JG, Yang TJ, et al. Identification and expression analysis of glucosinolate biosynthetic genes and estimation of glucosinolate contents in edible organs of Brassica oleracea subspecies. Molecules. (2015) 20:13089–111. doi: 10.3390/molecules200713089
63. Bednarek P, Pislewska-Bednarek M, Svatos A, Schneider B, Doubsky J, Mansurova M, et al. A glucosinolate metabolism pathway in living plant cells mediates broad-spectrum antifungal defense. Science. (2009) 323:101–6. doi: 10.1126/science.1163732
64. Barth C, Jander G. Arabidopsis myrosinases TGG1 and TGG2 have redundant function in glucosinolate breakdown and insect defense. Plant J. (2006) 46:549–62. doi: 10.1111/j.1365-313x.2006.02716.x
65. De Vos M, Kriksunov KL, Jander G. Indole-3-acetonitrile production from indole glucosinolates deters oviposition by Pieris rapae. Plant Physiol. (2008) 146:916–26. doi: 10.1104/pp.107.112185
66. Kuchernig JC, Burow M, Wittstock U. Evolution of specifier proteins in glucosinolate-containing plants. BMC Evol Biol. (2012) 12:127. doi: 10.1186/1471-2148-12-127
67. Eisenschmidt-Bönn D, Schneegans N, Backenköhler A, Wittstock U, Brandt W. Structural diversification during glucosinolate breakdown: mechanisms of thiocyanate, epithionitrile and simple nitrile formation. Plant J. (2019) 99:329–43. doi: 10.1111/tpj.14327
68. Levy M, Wang Q, Kaspi R, Parrella MP, Abel S. Arabidopsis IQD1, a novel calmodulin-binding nuclear protein, stimulates glucosinolate accumulation and plant defense. Plant J. (2005) 43:79–96. doi: 10.1111/j.1365-313x.2005.02435.x
69. Skirycz A, Reichelt M, Burow M, Birkemeyer C, Rolcik J, Kopka J, et al. DOF transcription factor AtDof1.1 (OBP2) is part of a regulatory network controlling glucosinolate biosynthesis in Arabidopsis. Plant J. (2006) 47:10–24. doi: 10.1111/j.1365-313x.2006.02767.x
70. Frerigmann H, Gigolashvili T. Update on the role of R2R3-MYBs in the regulation of glucosinolates upon sulfur deficiency. Front Plant Sci. (2014) 5:626. doi: 10.3389/fpls.2014.00626
71. Frerigmann H, Berger B, Gigolashvili T. bHLH05 is an interaction partner of MYB51 and a novel regulator of glucosinolate biosynthesis in Arabidopsis. Plant Physiol. (2014) 166:349–69. doi: 10.1104/pp.114.240887
72. Pérez-Balibrea S, Moreno DA, García-Viguera C. Improving the phytochemical composition of broccoli sprouts by elicitation. Food Chem. (2011) 129:35–44. doi: 10.1016/j.foodchem.2011.03.049
73. Hanschen FS, Schreiner M. Isothiocyanates, nitriles, and epithionitriles from glucosinolates are affected by genotype and developmental stage in Brassica oleracea varieties. Front Plant Sci. (2017) 8:1095. doi: 10.3389/fpls.2017.01095
74. Farnham MW, Wilson PE, Stephenson KK, Fahey JW. Genetic and environmental effects on glucosinolate content and chemoprotective potency of broccoli. Plant Breed. (2004) 123:60–5. doi: 10.1046/j.0179-9541.2003.00912.x
75. Bhandari SR, Kwak JH. Seasonal variation in phytochemicals and antioxidant activities in different tissues of various Broccoli cultivars. Afr J Biotechnol. (2014) 13:604–15. doi: 10.5897/ajb2013.13432
76. Brown AF, Yousef GG, Jeffery EH, Klein BP, Wallig MA, Kushad MM, et al. Glucosinolate profiles in broccoli: variation in levels and implication in breeding for cancer chemoprotection. J Am Soc Hortic Sci. (2002) 127:807–13. doi: 10.21273/jashs.127.5.807
77. Wang GC, Farnham M, Jeffery EH. Impact of thermal processing on sulforaphane yield from broccoli (Brassica oleracea L. ssp italica). J Agric Food Chem. (2012) 60:6743–8. doi: 10.1021/jf2050284
78. Sarikamis G, Marquez J, MacCormack R, Bennett RN, Roberts J, Mithen R. High glucosinolate broccoli: a delivery system for sulforaphane. Mol Breed. (2006) 18:219–28. doi: 10.1007/s11032-006-9029-y
79. Sahamishirazi S, Zikeli S, Fleck M, Claupein W, Graeff-Hoenninger S. Development of a near-infrared spectroscopy method (NIRS) for fast analysis of total, indolic, aliphatic and individual glucosinolates in new bred open pollinating genotypes of broccoli (Brassica oleracea convar. botrytis var italica). Food Chem. (2017) 232:272–7. doi: 10.1016/j.foodchem.2017.04.025
80. Nicoletto C, Santagata S, Pino S, Sambo P. Caracterização antioxidante de diferentes variedades crioulas de brócolis italiano. Hortic Brasil. (2016) 34:74–9. doi: 10.1590/s0102-053620160000100011
81. Jo JS, Bhandari SR, Kang GH, Lee JG. Comparative analysis of individual glucosinolates, phytochemicals, and antioxidant activities in broccoli breeding lines. Hortic Environ Biotechnol. (2016) 57:392–403. doi: 10.1007/s13580-016-0088-7
82. Rybarczyk-Plonska A, Hagen SF, Borge GIA, Bengtsson GB, Hansen MK, Wold AB. Glucosinolates in broccoli (Brassica oleracea L. var italica) as affected by postharvest temperature and radiation treatments. Postharvest Biol Technol. (2016) 116:16–25. doi: 10.1016/j.postharvbio.2015.12.010
83. Formica-Oliveira AC, Martínez-Hernández GB, Díaz-López V, Artés F, Artés-Hernández F. Use of postharvest UV-B and UV-C radiation treatments to revalorize broccoli byproducts and edible florets. Innov Food Sci Emerg Technol. (2017) 43:77–83. doi: 10.1016/j.ifset.2017.07.036
84. Bhandari SR, Jo JS, Lee JG. Comparison of glucosinolate profiles in different tissues of nine Brassica crops. Molecules. (2015) 20:15827–41. doi: 10.3390/molecules200915827
85. Fahey JW, Zhang Y, Talalay P. Broccoli sprouts: an exceptionally rich source of inducers of enzymes that protect against chemical carcinogens. Proc Natl Acad Sci USA. (1997) 94:10367–72. doi: 10.1073/pnas.94.19.10367
86. Rangkadilok N, Nicolas ME, Bennett RN, Eagling DR, Premier RR, Taylor PW. The effect of sulfur fertilizer on glucoraphanin levels in broccoli (B. oleracea L. var italica) at different growth stages. J Agric Food Chem. (2004) 52:2632–9. doi: 10.1021/jf030655u
87. Bhandari SR, Kwak JH, Jo JS, Lee JG. Changes in phytochemical content and antioxidant activity during inflorescence development in broccoli. Chil J Agric Res. (2019) 79:36–47. doi: 10.4067/s0718-58392019000100036
88. Vallejo F, García-Viguera C, Tomás-Barberán FA. Changes in broccoli (Brassica oleracea L. var italica) health-promoting compounds with inflorescence development. J Agric Food Chem. (2003) 51:3776–82. doi: 10.1021/jf0212338
89. Kissen R, Øverby A, Winge P, Bones AM. Allyl-isothiocyanate treatment induces a complex transcriptional reprogramming including heat stress, oxidative stress and plant defence responses in Arabidopsis thaliana. BMC Genomics. (2016) 17:740. doi: 10.1186/s12864-016-3039-x
90. Pérez-Balibrea S, Moreno DA, García-Viguera C. Influence of light on health-promoting phytochemicals of broccoli sprouts. J Sci Food Agric. (2008) 88:904–10. doi: 10.1002/jsfa.3169
91. Schonhof I, Blankenburg D, Müller S, Krumbein A. Sulfur and nitrogen supply influence growth, product appearance, and glucosinolate concentration of broccoli. J Soil Sci Plant Nutr. (2007) 170:65–72. doi: 10.1002/jpln.200620639
92. Guo L, Yang R, Wang Z, Guo Q, Gu Z. Glucoraphanin, sulforaphane and myrosinase activity in germinating broccoli sprouts as affected by growth temperature and plant organs. J Funct Foods. (2014) 9:70–7. doi: 10.1002/s12864-016-3039-x
93. Steindal ALH, Mølmann J, Bengtsson GB, Johansen TJ. Influence of day length and temperature on the content of health-related compounds in broccoli (Brassica oleracea L. var italica). J Agric Food Chem. (2013) 61:10779–86. doi: 10.1021/jf403466r
94. Charron CS, Saxton AM, Sams CE. Relationship of climate and genotype to seasonal variation in the glucosinolate-myrosinase system. I. Glucosinolate content in ten cultivars of Brassica oleracea grown in fall and spring seasons. J Sci Food Agric. (2005) 85:671–81. doi: 10.1002/jsfa.1880
95. Pék Z, Daood H, Nagyné M, Neményi A, Helyes L. Effect of environmental conditions and water status on the bioactive compounds of broccoli. Open Life Sci. (2013) 8:777–87. doi: 10.2478/s11535-013-0172-7
96. Zaghdoud C, Carvajal M, Moreno DA, Ferchichi A, del Carmen Martínez-Ballesta M. Health-promoting compounds of broccoli (Brassica oleracea L. var. italica) plants as affected by nitrogen fertilisation in projected future climatic change environments. J Sci Food Agric. (2016) 96:392–403. doi: 10.1002/jsfa.7102
97. Garcia-Ibañez P, Sanchez-Garcia M, Sánchez-Monedero MA, Cayuela ML, Moreno DA. Olive tree pruning derived biochar increases glucosinolate concentrations in broccoli. Sci Hortic. (2020) 267:109329. doi: 10.1016/j.scienta.2020.109329
98. Meyer M, Adam ST. Comparison of glucosinolate levels in commercial broccoli and red cabbage from conventional and ecological farming. Eur Food Res Technol. (2008) 226:1429–37. doi: 10.1007/s00217-007-0674-0
99. Vicas SI, Teusdea AC, Carbunar M, Socaci SA, Socaciu C. Glucosinolates profile and antioxidant capacity of Romanian Brassica vegetables obtained by organic and conventional agricultural practices. Plant Foods Hum Nutr. (2013) 68:313–21. doi: 10.1007/s11130-013-0367-8
100. Machado RMA, Serralheiro RP. Soil salinity: effect on vegetable crop growth. Management practices to prevent and mitigate soil salinization. Horticulturae. (2017) 3:30. doi: 10.3390/horticulturae3020030
101. Lopez-Berenguer C, Martinez-Ballesta MDC, Moreno DA, Carvajal M, Garcia-Viguera C. Growing hardier crops for better health: salinity tolerance and the nutritional value of broccoli. J Agric Food Chem. (2009) 57:572–8. doi: 10.1021/jf802994p
102. Zaghdoud C, Alcaraz-Lopez C, Mota-Cadenas C, Martínez-Ballesta MDC, Moreno DA, Ferchichi A, et al. Differential responses of two broccoli (Brassica oleracea L. var Italica) cultivars to salinity and nutritional quality improvement. Sci World J. (2012) 2012:291435. doi: 10.1100/2012/291435
103. Sarikamiş G, Cakir A. Influence of salinity on aliphatic and indole glucosinolates in broccoli (Brassica oleracea var. italica). Appl Ecol Environ Res. (2017) 15:1781–8. doi: 10.15666/aeer/1503_17811788
104. Moreno DA, López-Berenguer C, Martínez-Ballesta MC, Carvajal M, García-Viguera C. Basis for the new challenges of growing broccoli for health in hydroponics. J Sci Food Agric. (2008) 88:1472–81. doi: 10.1002/jsfa.3244
105. Di Gioia F, Rosskopf EN, Leonardi C, Giuffrida F. Effects of application timing of saline irrigation water on broccoli production and quality. Agric Water Manag. (2018) 203:97–104. doi: 10.1016/j.agwat.2018.01.004
106. Baenas N, García-Viguera C, Moreno DA. Biotic elicitors effectively increase the glucosinolates content in Brassicaceae sprouts. J Agric Food Chem. (2014) 62:1881–9. doi: 10.1021/jf404876z
107. Baenas N, Villaño D, García-Viguera C, Moreno DA. Optimizing elicitation and seed priming to enrich broccoli and radish sprouts in glucosinolates. Food Chem. (2016) 204:314–9. doi: 10.1016/j.foodchem.2016.02.144
108. Sun J, Kou L, Geng P, Huang H, Yang T, Luo Y, et al. Metabolomic assessment reveals an elevated level of glucosinolate content in CaCl2 treated broccoli microgreens. J Agric Food Chem. (2015) 63:1863–8. doi: 10.1021/jf504710r
109. Lu Y, Dong W, Alcazar J, Yang T, Luo Y, Wang Q, et al. Effect of preharvest CaCl2 spray and postharvest UV-B radiation on storage quality of broccoli microgreens, a richer source of glucosinolates. J Food Compost Anal. (2018) 67:55–62. doi: 10.1016/j.jfca.2017.12.035
110. Ku KM, Choi JH, Kim HS, Kushad MM, Jeffery EH, Juvik JA. Methyl jasmonate and 1-methylcyclopropene treatment effects on quinone reductase inducing activity and post-harvest quality of broccoli. PLoS ONE. (2013) 8:e77127. doi: 10.1371/journal.pone.0077127
111. Yang R, Hui Q, Gu Z, Zhou Y, Guo L, Shen C, et al. Effects of CaCl2 on the metabolism of glucosinolates and the formation of isothiocyanates as well as the antioxidant capacity of broccoli sprouts. J Funct Foods. (2016) 24:156–63. doi: 10.1016/j.jff.2016.04.007
112. Hassini I, Rios JJ, Garcia-Ibañez P, Baenas N, Carvajal M, Moreno DA. Comparative effect of elicitors on the physiology and secondary metabolites in broccoli plants. J Plant Physiol. (2019) 239:1–9. doi: 10.1016/j.jplph.2019.05.008
113. Tian M, Yang Y, Ávila FW, Fish T, Yuan H, Hui M, et al. Effects of selenium supplementation on glucosinolate biosynthesis in broccoli. J Agric Food Chem. (2018) 66:8036–44. doi: 10.1021/acs.jafc.8b03396
114. Mewis I, Schreiner M, Nguyen CN, Krumbein A, Ulrichs C, Lohse M, et al. UV-B irradiation changes specifically the secondary metabolite profile in broccoli sprouts: induced signaling overlaps with defense response to biotic stressors. Plant Cell Physiol. (2012) 53:1546–60. doi: 10.1093/pcp/pcs096
115. Topcu Y, Dogan A, Sahin-Nadeem H, Polat E, Kasimoglu Z, Erkan M. Morphological and biochemical responses of broccoli florets to supplemental ultraviolet-B illumination. Agric Ecosyst Environ. (2018) 259:1–10. doi: 10.1016/j.agee.2018.02.027
116. Francisco M, Moreno DA, Cartea ME, Ferreres F, GarciaViguera C, Velasco P. Simultaneous identification of glucosinolates and phenolic compounds in a representative collection of vegetable Brassica rapa. J Chromatogr A. (2009) 1216:6611–9. doi: 10.1016/j.chroma.2009.07.055
117. Huseby S, Koprivova A, Lee BR, Saha S, Mithen R, Wold AB, et al. Diurnal and light regulation of sulphur assimilation and glucosinolate biosynthesis in Arabidopsis. J Exp Bot. (2013) 64:1039–48. doi: 10.1093/jxb/ers378
118. Schonhof I, Krumbein A, Brückner B. Genotypic effects on glucosinolates and sensory properties of broccoli and cauliflower. Food Nahrung. (2004) 48:25–33. doi: 10.1002/food.200300329
119. Zaghdoud C, Carvajal M, Ferchichi A, del Carmen Martínez-Ballesta M. Water balance and N-metabolism in broccoli (Brassica oleracea L. var. Italica) plants depending on nitrogen source under salt stress and elevated CO2. Sci Total Environ. (2016) 571:763–71. doi: 10.1016/j.scitotenv.2016.07.048
120. Watada AE, Ko NP, Minott DA. Factors affecting quality of fresh-cut horticultural products. Postharvest Biol Technol. (1996) 9:115–25. doi: 10.1016/s0925-5214(96)00041-5
121. Giuffrida F, Agnello M, Mauro RP, Ferrante A, Leonardi C. Cultivation under salt stress conditions influences postharvest quality and glucosinolates content of fresh-cut cauliflower. Sci Hortic. (2018) 236:166–74. doi: 10.1016/j.scienta.2018.03.049
122. Radovich TJ, Kleinhenz MD, Streeter JG. Irrigation timing relative to head development influences yield components, sugar levels, and glucosinolate concentrations in cabbage. J Am Soc Hortic Sci. (2005) 130:943–9. doi: 10.21273/jashs.130.6.943
123. Xiao W, LIU FL, Jiang D. Priming: a promising strategy for crop production in response to future climate. J Integr Agric. (2017) 16:2709–16. doi: 10.1016/s2095-3119(17)61786-6
124. Sher A, Sarwar T, Nawaz A, Ijaz M, Sattar A, Ahmad S. Methods of Seed Priming. In: Hasanuzzaman M, Fotopoulos V, editors. Priming and Pretreatment of Seeds and Seedlings: Implication in Plant Stress Tolerance and Enhancing Productivity in Crop Plants. Singapore: Springer (2019). p. 1–10. doi: 10.1007/978-981-13-8625-1_1
125. Hussain M, Farooq M, Lee DJ. Evaluating the role of seed priming in improving drought tolerance of pigmented and non-pigmented rice. J Agron Crop Sci. (2017) 203:269–76. doi: 10.1111/jac.12195
126. Verma V, Ravindran P, Kumar PP. Plant hormone-mediated regulation of stress responses. BMC Plant Biol. (2016) 16:86. doi: 10.1186/s12870-016-0771-y
127. Yuan S, Lin H-H. Role of salicylic acid in plant abiotic stress. Z Naturforsch. (2008) 63:313–20. doi: 10.1515/znc-2008-5-601
128. Belt K, Huang S, Thatcher LF, Casarotto H, Singh KB, Van Aken O, et al. Salicylic acid-dependent plant stress signaling via mitochondrial succinate dehydrogenase. Plant Physiol. (2017) 173:2020–40. doi: 10.1104/pp.16.00060
129. Jansen MA, Hectors K, O'Brien NM, Guisez Y, Potters G. Plant stress and human health: Do human consumers benefit from UV-B acclimated crops? Plant Sci. (2008) 175:449–58. doi: 10.1016/j.plantsci.2008.04.010
130. Cisneros-Zevallos L. The use of controlled postharvest abiotic stresses as a tool for enhancing the nutraceutical content and adding-value of fresh fruits and vegetables. J Food Sci. (2003) 68:1560–5. doi: 10.1111/j.1365-2621.2003.tb12291.x
131. Topcu Y, Dogan A, Kasimoglu Z, Sahin-Nadeem H, Polat E, Erkan M. The effects of UV radiation during the vegetative period on antioxidant compounds and postharvest quality of broccoli (Brassica oleracea L.). Plant Physiol Biochem. (2015) 93:56–65. doi: 10.1016/j.plaphy.2015.02.016
132. Schouten RE, Zhang X, Verkerk R, Verschoor JA, Otma EC, Tijskens LMM, et al. Modelling the level of the major glucosinolates in broccoli as affected by controlled atmosphere and temperature. Postharvest BioTech. (2009) 53:1–10. doi: 10.1016/j.postharvbio.2009.03.001
133. Xu CJ, Guo DP, Yuan J, Yuan GF, Wang QM. Changes in glucoraphanin content and quinone reductase activity in broccoli (Brassica oleracea var. italica) florets during cooling and controlled atmosphere storage. Postharvest Biol Technol. (2006) 42:176–84. doi: 10.1016/j.postharvbio.2006.06.009
134. Baenas N, Cartea ME, Moreno DA, Tortosa M, Francisco M. Processing and cooking effects on glucosinolates and their derivatives. In: Galanakis CM, editor. Glucosinolates: Properties, Recovery, and Applications. Cambridge, MA: Elsevier Inc.; Academic Press (2020). p. 181–212. doi: 10.1016/b978-0-12-816493-8.00006-8
135. Xu F, Chen X, Yang Z, Jin P, Wang K, Shang H, et al. Maintaining quality and bioactive compounds of broccoli by combined treatment with 1-methylcyclopropene and 6-benzylaminopurine. J Sci Food Agric. (2013) 93:1156–61. doi: 10.1002/jsfa.5867
136. Xu F, Chen X, Jin P, Wang X, Wang J, Zheng Y. Effect of ethanol treatment on quality and antioxidant activity in postharvest broccoli florets. Eur Food Res Technol. (2012) 235:793–800. doi: 10.1007/s00217-012-1808-6
137. Villarreal-García D, Nair V, Cisneros-Zevallos L, Jacobo-Velázquez DA. Plants as biofactories: postharvest stress-induced accumulation of phenolic compounds and glucosinolates in broccoli subjected to wounding stress and exogenous phytohormones. Front Plant Sci. (2016) 7:45. doi: 10.3389/fpls.2016.00045
138. Miao H, Zeng W, Zhao M, Wang J, Wang Q. Effect of melatonin treatment on visual quality and health-promoting properties of broccoli florets under room temperature. Food Chem. (2020) 126498. doi: 10.1016/j.foodchem.2020.126498
139. Vallejo F, Tomas-Barberan FA, Garcia-Viguera C. Health-promoting compounds in broccoli as influenced by refrigerated transport and retail sale period. J Agric Food Chem. (2003) 51:3029–34. doi: 10.1021/jf021065j
140. Yuan G, Sun B, Yuan J, Wang Q. Effect of 1-methylcyclopropene on shelf life, visual quality, antioxidant enzymes and health-promoting compounds in broccoli florets. Food Chem. (2010) 118:774–81. doi: 10.1016/j.foodchem.2009.05.062
141. Xu F, Tang Y, Dong S, Shao X, Wang H, Zheng Y, et al. Reducing yellowing and enhancing antioxidant capacity of broccoli in storage by sucrose treatment. Postharvest Biol Technol. (2016) 112:39–45. doi: 10.1016/j.postharvbio.2015.09.038
142. Rodrigues AS, Rosa EAS. Effect of post-harvest treatments on the level of glucosinolates in broccoli. J Sci Food Agric. (1999) 79:1028–32. doi: 10.1002/(sici)1097-0010(19990515)79:7%3C1028::aid-jsfa322%3E3.0.co;2-i
143. Song L, Thornalley PJ. Effect of storage, processing and cooking on glucosinolate content of Brassica vegetables. Food Chem Toxicol. (2007) 45:216–24. doi: 10.1016/j.fct.2006.07.021
144. Fernández-León MF, Fernández-León AM, Lozano M, Ayuso MC, González-Gómez D. Different postharvest strategies to preserve broccoli quality during storage and shelf life: controlled atmosphere and 1-MCP. Food Chem. (2013) 138:564–73. doi: 10.1016/j.foodchem.2012.09.143
145. Jin P, Yao D, Xu F, Wang H, Zheng Y. Effect of light on quality and bioactive compounds in postharvest broccoli florets. Food Chem. (2015) 172:705–9. doi: 10.1016/j.foodchem.2014.09.134
146. Winkler S, Faragher J, Franz P, Imsic M, Jones R. Glucoraphanin and flavonoid levels remain stable during simulated transport and marketing of broccoli (Brassica oleracea var. italica) heads. Postharvest Biol Technol. (2007) 43:8994. doi: 10.1016/j.postharvbio.2006.08.001
147. El-Awady AA, Saber WI, Hamid NMA, Hassan HA. Increasing antioxidant content of broccoli sprouts using essential oils during cold storage. Agriculture (Polnohospodárstvo). (2016) 62:111–26. doi: 10.1515/agri-2016-0012
148. Darré M, Valerga L, Araque LCO, Lemoine ML, Demkura PV, Vicente AR, et al. Role of UV-B irradiation dose and intensity on color retention and antioxidant elicitation in broccoli florets (Brassica oleracea var. Italica). Postharvest Biol Technol. (2017) 128:76–82. doi: 10.1016/j.postharvbio.2017.02.003
149. Sánchez-Vega R, Elez-Martínez P, Martín-Belloso O. Influence of high-intensity pulsed electric field processing parameters on antioxidant compounds of broccoli juice. Innov Food Sci Emerg Technol. (2015) 29:70–7. doi: 10.1016/j.ifset.2014.12.002
150. Torres-Contreras AM, González-Agüero M, Cisneros-Zevallos L, Jacobo-Velázquez DA. Role of reactive oxygen species and ethylene as signaling molecules for the wound-induced biosynthesis of glucosinolates in broccoli (Brassica oleracea L. ‘Italica’). Acta Hortic. (2018) 1194:909–14. doi: 10.17660/actahortic.2018.1194.128
151. Collazo C, Charles F, Aguiló-Aguayo I, Marín-Sáez J, Lafarga T, Abadias M, et al. Decontamination of Listeria innocua from fresh-cut broccoli using UV-C applied in water or peroxyacetic acid, and dry-pulsed light. Innov Food Sci Emerg Technol. (2019) 52:438–49. doi: 10.1016/j.ifset.2019.02.004
152. Westphal A, Riedl KM, Cooperstone JL, Kamat S, Balasubramaniam VM, Schwartz SJ, et al. High-pressure processing of broccoli sprouts: influence on bioactivation of glucosinolates to isothiocyanates. J Agric Food Chem. (2017) 65:8578–85. doi: 10.1021/acs.jafc.7b01380
153. Verkerk R, Dekker M, Jongen WMF. Post-harvest increase of indolyl glucosinolates in response to chopping and storage of Brassica vegetables. J Sci Food Agric. (2001) 81:953–8. doi: 10.1002/jsfa.854
154. Aguilar-Camacho M, Welti-Chanes J, Jacobo-Velázquez DA. Combined effect of ultrasound treatment and exogenous phytohormones on the accumulation of bioactive compounds in broccoli florets. Ultrason Sonochem. (2019) 50:289–301. doi: 10.1016/j.ultsonch.2018.09.031
155. Van Eylen D, Oey I, Hendrickx M, Van Loey A. Effects of pressure/temperature treatments on stability and activity of endogenous broccoli (Brassica oleracea L. cv Italica) myrosinase and on cell permeability. J Food Eng. (2008) 89:178–86. doi: 10.1016/j.jfoodeng.2008.04.016
156. Butz P, Tauscher B. Recent studies on pressure-induced chemical changes in food constituents. Int J High Press Res. (2000) 19:11–8. doi: 10.1080/08957950008202529
157. Vallejo F, Tomás-Barberán F, García-Viguera C. Glucosinolates and vitamin C content in edible parts of broccoli florets after domestic cooking. Eur Food Res Technol. (2002) 215:310–6. doi: 10.1007/s00217-002-0560-8
158. Martínez-Hernández GB, Gómez PA, García-Talavera NV, Artés-Hernández F, Monedero-Saiz T, Sánchez-Álvarez C, et al. Human metabolic fate of glucosinolates from kailan-hybrid broccoli. Differences between raw and microwaved consumption. Food Res Int. (2013) 53:403–8. doi: 10.1016/j.foodres.2013.05.002
159. Tríska J, Vrchotová N, Houška M, Strohalm J. Comparison of total isothiocyanates content in vegetable juices during high pressure treatment, pasteurization and freezing. High Pressure Res. (2007) 27:147–9. doi: 10.1080/08957950601082565
160. Klug TV, Martínez-Hernández GB, Collado E, Artés F, Artés-Hernández F. Effect of microwave and high-pressure processing on quality of an innovative broccoli hummus. Food Bioprocess Tech. (2018) 11:1464–77. doi: 10.1007/s11947-018-2111-8
161. Aguiló-Aguayo I, Suarez M, Plaza L, Hossain MB, Brunton N, Lyng JG, et al. Optimization of pulsed electric field pre-treatments to enhance health-promoting glucosinolates in broccoli flowers and stalk. J Sci Food Agric. (2015) 95:1868–75. doi: 10.1002/jsfa.6891
162. Frandsen HB, Markedal KE, Martín-Belloso O, Sánchez-Vega R, Soliva-Fortuny R, Sørensen H, et al. Effects of novel processing techniques on glucosinolates and membrane associated myrosinases in broccoli. Pol J Food Nutr Sci. (2014) 64:17–25. doi: 10.2478/pjfns-2013-0005
163. Martínez-Hernández GB, Venzke-Klug T, Carrión-Monteagudo MDM, Artés Calero F, López-Nicolás JM, Artés-Hernández F. Effects of α-, β- and maltosyl-β-cyclodextrins use on the glucoraphanin–sulforaphane system of broccoli juice. J Sci Food Agric. (2019) 99:941–6. doi: 10.1002/jsfa.9269
164. Florkiewicz A, Ciska E, Filipiak-Florkiewicz A, Topolska K. Comparison of Sous-vide methods and traditional hydrothermal treatment on GL content in Brassica vegetables. Eur Food Res Technol. (2017) 243:1507–17. doi: 10.1007/s00217-017-2860-z
165. Cieślik E, Leszczyńska T, Filipiak-Florkiewicz A, Sikora E, Pisulewski PM. Effects of some technological processes on glucosinolate contents in cruciferous vegetables. Food Chem. (2007) 105:976–81. doi: 10.1016/j.foodchem.2007.04.047
166. Tiwari U, Sheehy E, Rai D, Gaffney M, Evans P, Cummins E. Quantitative human exposure model to assess the level of glucosinolates upon thermal processing of cruciferous vegetables. LWT Food Sci Technol. (2015) 63:253–61. doi: 10.1016/j.lwt.2015.03.088
167. Volden J, Borge GIA, Bengtsson GB, Hansen M, Thygesen IE, Wicklund T. Effect of thermal treatment on glucosinolates and antioxidant-related parameters in red cabbage (Brassica oleracea L. ssp capitata f rubra). Food Chem. (2008) 109:595–605. doi: 10.1016/j.foodchem.2008.01.010
168. Bongoni R, Verkerk R, Steenbekkers B, Dekker M, Stieger M. Evaluation of different cooking conditions on broccoli (Brassica oleracea var. italica) to improve the nutritional value and consumer acceptance. Plant Food Hum Nutr. (2014) 69:228–34. doi: 10.1007/s11130-014-0420-2
169. Deng Q, Zinoviadou KG, Galanakis CM, Orlien V, Grimi N, Vorobiev E, et al. The effects of conventional and non-conventional processing on glucosinolates and its derived forms, isothiocyanates: extraction, degradation, and applications. Food Eng Rev. (2014) 7:357–81. doi: 10.1007/s12393-014-9104-9
170. Kapusta-Duch J, Kusznierewicz B, Leszczyńska T, Borczak B. Effect of cooking on the contents of glucosinolates and their degradation products in selected Brassica vegetables. J Funct Foods. (2016) 23:412–22. doi: 10.1016/j.jff.2016.03.006
171. Duarte-Sierra A, Forney CF, Michaud D, Angers P, Arul J. Influence of hormetic heat treatment on quality and phytochemical compounds of broccoli florets during storage. Postharvest Biol Technol. (2017) 128:44–53. doi: 10.1016/j.postharvbio.2017.01.017
172. Dekker M, Hennig K, Verkerk R. Differences in thermal stability of glucosinolates in five Brassica vegetables. Czech J Food Sci. (2009) 27:S85–S88. doi: 10.17221/1079-cjfs
173. Giambanelli E, Verkerk R, Fogliano V, Capuano E, D'Antuono L, Oliviero T. Broccoli glucosinolate degradation is reduced performing thermal treatment in binary systems with other food ingredients. RSC Adv. (2015) 5:66894–900. doi: 10.1039/c5ra11409h
174. Hanschen FS, Rohn S, Mewis I, Schreiner M, Kroh LW. Influence of the chemical structure on the thermal degradation of the glucosinolates in broccoli sprouts. Food Chem. (2012) 130:1–8. doi: 10.1016/j.foodchem.2011.05.109
175. Paulsen E, Barrios S, Baenas N, Moreno DA, Heinzen H, Lema P. Effect of temperature on glucosinolate content and shelf life of ready-to-eat broccoli florets packaged in passive modified atmosphere. Postharvest Biol Technol. (2018) 138:125–33. doi: 10.1016/j.postharvbio.2018.01.006
176. Chanbisana C, Banik AK. Studies on effectiveness of packaging on storability of broccoli Cv. Aishwarya. Int J Chem Stud. (2019) 7:5112–8.
177. Kapusta-Duch J, Leszczyńska T, Borczak B, Florkiewicz A, Ambroszczyk A. Impact of different packaging systems on selected antioxidant properties of frozen-stored broccoli. Ecol Chem Eng Sci. (2019) 26:383–96. doi: 10.1515/eces-2019-0027
178. He Q, Xiao K. Quality of broccoli (Brassica oleracea L. var italica) in modified atmosphere packaging made by gas barrier-gas promoter blending materials. Postharvest Biol Technol. (2018) 144:63–9. doi: 10.1016/j.postharvbio.2018.05.013
179. Hansen M, Møller P, Sørensen H, de Trejo MC. Glucosinolates in broccoli stored under controlled atmosphere. J Am Soc Hortic Sci. (1995) 120:1069–74. doi: 10.21273/jashs.120.6.1069
180. Schreiner M, Peters P, Krumbein A. Changes of glucosinolates in mixed fresh-cut broccoli and cauliflower florets in modified atmosphere packaging. J Food Sci. (2007) 72:S585–9. doi: 10.1111/j.1750-3841.2007.00506.x
181. Schreiner MC, Peters PJ, Krumbein AB. Glucosinolates in mixed-packaged mini broccoli and mini cauliflower under modified atmosphere. J Agric Food Chem. (2006) 54:2218–22. doi: 10.1021/jf0525636
182. Jia CG, Xu CJ, Wei J, Yuan J, Yuan GF, Wang BL, et al. Effect of modified atmosphere packaging on visual quality and glucosinolates of broccoli florets. Food Chem. (2009) 114:28–37. doi: 10.1016/j.foodchem.2008.09.009
183. Wang LY, Liu JL, Wang WX, Sun Y. Exogenous melatonin improves growth and photosynthetic capacity of cucumber under salinity-induced stress. Photosynthetica. (2016) 54:19–27. doi: 10.1007/s11099-015-0140-3
184. Zhang N, Sun Q, Zhang H, Cao Y, Weeda S, Ren S, et al. Roles of melatonin in abiotic stress resistance in plants. J Exp Bot. (2015) 66:647–56. doi: 10.1093/jxb/eru336
185. El-Awady AAEF. Effect of essential oils on storability and preservation of some vegetable crops. In: El-Shemy H, editor. Essential Oils-Oils of Nature. London: IntechOpen (2019). p. 1–20. doi: 10.5772/intechopen.87213
186. Aiamla-or S, Shigyo M, Yamauchi N. UV-B treatment controls chlorophyll degradation and related gene expression in broccoli (Brassica oleracea L. Italica Group) florets during storage. Sci Hortic. (2019) 243:524–7. doi: 10.1016/j.scienta.2018.09.009
187. Donsì F, Ferrari G, Pataro G. Applications of pulsed electric field treatments for the enhancement of mass transfer from vegetable tissue. Food Eng Rev. (2010) 2:109–30. doi: 10.1007/s12393-010-9015-3
188. Siddiqui MW, Lara I, Ilahy R, Tlili I, Ali A, Homa F, et al. Dynamic changes in health-promoting properties and eating quality during off-vine ripening of tomatoes. Compr Rev Food Sci Food Saf . (2018) 17:1540–60. doi: 10.1111/1541-4337.12395
189. Sarvan I, Verkerk R, van Boekel M, Dekker M. Comparison of the degradation and leaching kinetics of glucosinolates during processing of four Brassicaceae (broccoli, red cabbage, white cabbage, Brussels sprouts). I Innov Food Sci Emerg Technol. (2014) 25:58–66. doi: 10.1016/j.ifset.2014.01.007
190. Oerlemans K, Barrett DM, Suades CB, Verkerk R, Dekker M. Thermal degradation of GLS in red cabbage. Food Chem. (2006) 95:19–29. doi: 10.1016/j.foodchem.2004.12.013
191. Sandhya. Modified atmosphere packaging of fresh produce: current status and future needs. LWT Food Sci Technol. (2010) 43:381–92. doi: 10.1016/j.lwt.2009.05.018
Keywords: aliphatic, indole and aromatic glucosinolates, biosynthetic pathways, broccoli, Brassica oleracea L var. italica, pre-harvest and post-harvest management
Citation: Ilahy R, Tlili I, Pék Z, Montefusco A, Siddiqui MW, Homa F, Hdider C, R'Him T, Lajos H and Lenucci MS (2020) Pre- and Post-harvest Factors Affecting Glucosinolate Content in Broccoli. Front. Nutr. 7:147. doi: 10.3389/fnut.2020.00147
Received: 28 April 2020; Accepted: 23 July 2020;
Published: 10 September 2020.
Edited by:
Antonello Santini, University of Naples Federico II, ItalyReviewed by:
Inga Mewis, Humboldt University of Berlin, GermanyJohn A. Juvik, University of Illinois at Urbana-Champaign, United States
Copyright © 2020 Ilahy, Tlili, Pék, Montefusco, Siddiqui, Homa, Hdider, R'Him, Lajos and Lenucci. This is an open-access article distributed under the terms of the Creative Commons Attribution License (CC BY). The use, distribution or reproduction in other forums is permitted, provided the original author(s) and the copyright owner(s) are credited and that the original publication in this journal is cited, in accordance with accepted academic practice. No use, distribution or reproduction is permitted which does not comply with these terms.
*Correspondence: Riadh Ilahy, Ym4ucmlhZGhAZ21haWwuY29t