- 1College of Animal Science and Technology, Northeast Agricultural University, Harbin, China
- 2Department of Animal Nutrition, The University of Agriculture Peshawar, Peshawar, Pakistan
- 3Wellhope Feed Company Limited, Shenyang, China
- 4Department of Ruminant Nutrition, Institute of Animal Husbandry of Heilongjiang Academy of Agricultural Sciences, Harbin, China
The objectives of this study were to evaluate changes in profiles of odd- and branched-chain fatty acids (OBCFA), including pentadecanoic acid (C15:0), 13-methyltetradecanoic acid (iso-C15:0), 12-methyltetradecanoic acid (anteiso-C15:0), 14-methylpentadecanoic acid (iso-C16:0), heptadecanoic acid (C17:0), 15-methylhexadecanoic acid (iso-C17:0), and 14-methylhexadecanoic acid (anteiso-C17:0) during in vitro fermentation of pure carbohydrates mixtures in the buffer-rumen fluid. The second objective was to correlate the changes in the OBCFA profile to the corresponding changes in ruminal fermentation parameters, microbial crude protein (MCP) synthesis, and bacterial populations. Five pure carbohydrates mixtures containing different cellulose: starch (C:S) ratios, i.e., 0:100, 25:75, 50:50, 75:25, and 100:0, were incubated for 6, 12, 18, and 24 h in vitro. The results showed that there was significant interaction (P < 0.05) between C:S and incubation time for changes in all OBCFA profiles, except iso-C17:0. The highest concentration of total OBCFA (3.94 mg/g dry matter; DM) was observed in the residues after 24 h of fermentation when the C:S was 0:100, while the lowest concentration of OBCFA (1.65 mg/g DM) was produced after 6 h of incubation when the C:S was 50:50. The correlation analysis revealed that the concentration of iso-C16:0 might be a potential marker for the estimation of total volatile fatty acids (ρ = 0.78) and MCP synthesis (ρ = 0.82) in the rumen. Compared to starch degrading bacteria, cellulolytic bacteria had stronger correlations with OBCFA concentrations, and the strongest correlation was found between the population of Ruminococcus flavefaciens with C15:0 concentration (ρ = 0.70). Notably, this is the first paper reporting relationship between OBCFA with rumen fermentation products and microbial protein synthesis based on fermentation of pure carbohydrates mixtures in vitro, and thus avoid confounding interference from dietary protein and fat presence in the in vivo studies. However, more in-depth experiments are needed to substantiate the current findings.
Introduction
Odd- and branched-chain fatty acids contents are promising indices for predicting ruminal fermentation characteristics and microbial matter leaving the rumen (1–3). The contents of odd- and branched-chain fatty acids (OBCFA) include pentadecanoic acid (C15:0), 13-methyltetradecanoic acid (iso-C15:0), 12-methyltetradecanoic acid (anteiso-C15:0), 14-methylpentadecanoic acid (iso-C16:0), heptadecanoic acid (C17:0), 15-methylhexadecanoic acid (iso-C17:0), and 14-methylhexadecanoic acid (anteiso-C17:0). The OBCFA are present in microbial membrane lipids (4), and its profile reflects the microbial composition and as such, ruminal fermentation characteristics.
Theoretically, OBCFA is produced from 2-carbon elongation of propionate (C15:0 and C17:0), isobutyrate (iso-C14:0 and iso-C16:0), isovalerate (iso-C15:0 and iso-C17:0), and 2-methyl-butyrate (anteiso-C15:0 and anteiso-C17:0) with the incorporation of malonyl-CoA fatty acid synthetase, which provides the 2-carbons for elongation (4, 5). Therefore, it is not surprising that several studies have reported close relationships between OBCFA and ruminal volatile fatty acids (VFA) composition both in vitro (1) and in vivo (3). For example, the bacterial OBCFA explained 80% of the variations in acetate, propionate, and butyrate under an in vitro culture system (1). However, the study of French et al. (6) demonstrated that infusion of large amounts of VFA failed to alter OBCFA composition of ruminal content in mid-lactation dairy cows.
Earlier studies on the investigations of OBCFA profile have used a variety of natural feedstuffs, and the effect of the content and composition of dietary fat on OBCFA composition was always one of the main components of these studies (1, 6). The variation in content and composition of dietary fat might likely have altered the OBCFA profile by changing the microbial population in the rumen. As a result, these findings could not be attributed clearly to individual dietary constitutes such as starch and fiber; thus, may not extend well to different feedstuffs.
Since most OBCFA arise from microbial membrane lipids (4), greater concentrations of these fatty acids indicate greater microbial yield. A preliminary study (7) has confirmed that the ratios of purine bases:OBCFA (1.16) and uracil:OBCFA (0.34) were constant in mixed rumen bacteria of dairy cows. Similarly, our preliminary study confirmed that concentrations of ruminal OBCFA, especially those of odd-chain fatty acids and isomers of 15 carbon, were significantly correlated with pyrimidine, cytosine, and total nucleic acid bases in dairy cows (8). Although these nucleic acids are of microbial origin and widely used to quantify bacterial protein yield, they are not reliable indices alone because their ratio with nitrogen (N) content considerably varies between solid-associated bacteria and liquid-associated bacteria in the rumen (9). To the best of our knowledge, none of the previous studies has measured the true synthesis of microbial crude protein (MCP) in these experiments. Therefore, the relationship between OBCFA concentration and MCP yield has not been well-determined.
In addition, different bacteria consist of different OBCFA compositions in the rumen (10, 11). As well-reviewed by the study of Vlaeminck et al. (12), fibrolytic bacteria, e.g., Ruminococcus flavefaciens and Ruminococcus albus, are usually enriched in iso-fatty acids, while starch degrading microorganisms, e.g., Selenomonas ruminantium, Ruminobacter amylophilus, and Streptococcus bovis, contain a relatively greater amount of linear odd-chain fatty acids and a small amount of branched-chain fatty acids. In comparison, strains of Butyrivibrio have a more heterogeneous profile of OBCFA (11). Regarding the relationship of OBCFA concentrations with bacterial abundance in the rumen, little information is available, although data from our preliminary work indicated that C17:0 can be used to estimate the cellulolytic bacterial population in mixed rumen bacteria. However, more in-depth studies are required to build up a solid conclusion.
In this study, pure carbohydrates, i.e., cellulose and starch, were selected as fermentation substrates to avoid confounding effects of dietary fat on OBCFA synthesis during rumen fermentation. We hypothesized that the profiles of OBCFA would be different during ruminal fermentation of substrates with different ratios of cellulose and starch (C:S), and the variation in OBCFA composition might be correlated to changes in rumen fermentation characteristics and MCP synthesis in vitro. Therefore, the objectives of this study were to investigate changes in (1) OBCFA profiles, (2) rumen fermentation parameters, (3) MCP synthesis, and (4) relevant bacterial populations during in vitro rumen fermentation of substrates with different C:S ratios. The second objective was to correlate the changes in the OBCFA profile to the corresponding changes in ruminal fermentation parameters, MCP synthesis, and bacterial populations.
Materials and Methods
The animal use protocol was approved by the Animal Care and Use Committee, Northeast Agricultural University (Harbin, China). All the procedures were conducted, following the University standard biosecurity rules.
In vitro Incubation and Sample Collection
Carbohydrate mixtures containing different ratios of pure cellulose and starch (C:S = 0:100, 25:75, 50:50, 75:25, and 100:0) were obtained from Macklin (Macklin Biochemical Co., Ltd, Shanghai, China), and used as fermentation substrates for in vitro incubations. From each of the five substrates, subsamples (1 g) were weighed and transferred into 150 ml culture flasks for subsequent in vitro incubation for 6, 12, 18, and 24 h in a buffered-rumen fluid. For each incubation period, three replicate samples of each substrate were used. The incubations were carried out in two replicate runs within 2 weeks.
Rumen fluid was obtained from three rumen-fistulated Holstein cows in warm (39°C) thermostat bottles which were pre-flushed with CO2. The cows were fed a balanced ration (Table 1), formulated according to the NRC (13), at 08:00 and 16:00 h daily. The rumen fluid was strained through four layers of cheesecloth and the residues were discarded. The filtered fluid was transferred to a 4 L glass jar and mixed with pre-warmed (39°C) buffer solution in 1:2 (V/V) under a continuous supply of CO2 to ensure an anaerobic environment. The buffer solution was prepared according to the study of Wang et al. (14) and contained NH4HCO3, NaHCO3, Na2HPO4, KH2PO4, MgSO4•7H2O, CaCl2•2H2O, MnCl2•4H2O, CoCl2•6H2O, FeCl3•6H2O, C12H6NO4Na, trypticase peptone, C3H7NO2S•HCl•H2O, and Na2S•9H2O. The buffered rumen fluid was transferred to the dispenser with a volume range of 100 ml and connected with CO2. Before adding the buffered rumen fluid, the incubation flasks containing the samples were flushed with CO2. Afterward, 100 ml of the buffered-rumen fluid was added through the walls to each flask. The flasks were slowly and smoothly stirred to prevent adhesion of particles above the fluid to the bottle walls. The flasks were incubated in a shaking water bath at 39°C with 40–50 movements per minute for 24 h. Triplicate flasks containing only mixed buffered-rumen fluid were also incubated in each run as blank control. After 6, 12, 18, and 24 h of incubation, a total of 18 flasks (15 treatments and 3 blanks) were taken out of the incubator and immediately placed in the ice to end the fermentation. Subsequently, approximately 5 ml of fermented inoculum from each flask was subsampled for the measurements of pH value (Sartorius, pH-Meter PB-10, Goettingen, Germany), ammonia nitrogen (NH3-N), and volatile fatty acids (VFA) concentrations. Then another 4 ml of inoculum was snap-frozen in LN and stored at −80°C for further analysis of rumen bacterial populations. Finally, the remaining fermented samples were freeze-dried, weighed, and analyzed for OBCFA and MCP concentrations.
Measurement of Odd- and Branched-Chain Fatty Acids
The OBCFA were determined according to the procedure described by the study of Sukhija and Palmquist (15). In brief, around 200 mg of freeze-dried fermentation mixture was weighed and added into each nylon-cap glass tube containing 2 mg of non-adecanoic acid (C19:0, internal standard; Macklin Biochemical Co., Ltd, Shanghai, China). Then the samples were treated with 3 ml of 5% methanolic HCl and incubated at 70°C for 2 h. After cooling to room temperature, 5 ml of 6% K2CO3 was added which was followed by 2 ml of benzene and vortexed for 30 s. Following centrifugation at 1,500 rpm for 5 min, the upper organic phase was transferred, dried with N2, and reconstituted in 1 ml of benzene for gas chromatography (GC) analysis.
The methylated fatty acids were analyzed by Shimadzu GC analyzer (GC-2010, Shimadzu, Tokyo, Japan), equipped with flame ionization detector (FID), an Equity™-1 capillary column (15 m ×0.1 mm ×0.1 μm; Supelco, Inc., Sigma-Aldrich, 28039-U), and N2 as the carrier gas. The injector and detector were maintained at 280°C. Individual OBCFA was identified according to the peak and retention time of fatty acid standards, including iso-C14:0, iso-C15:0, anteiso-C15:0, C15:0, iso-C16:0, iso-C17:0, anteiso-C17:0, C17:0, and iso-C18:0 (Larodan Fine Chemicals, Malmo, Sweden).
Analyses of Ruminal Fermentation Parameters
The concentration of NH3-N was determined using the phenol-hypochlorite colorimetric method according to the study of Broderick and Kang (16). The VFA concentrations were measured by using GC-2010 (Shimadzu Laboratory Supplies Co., Ltd, Shanghai, China) equipped with a flame-ionization detector and a capillary column (HP-Innowax, 19091N-133, Agilent Technologies, Santa Clara, CA), as described by the study of Zhang et al. (3).
The MCP synthesis was estimated according to the study of Hall and Herejk (17). Briefly, 300 mg of freeze-dried fermentation residue was weighed, transferred into a 50 ml flask containing 10 ml of trichloroacetic acid (TCA; 19.4%), and the mixtures were then placed on ice for 45 min. Following centrifugation at 7,719 × g for 20 min, the whole flask content was filtered through Whatman 541 filter paper (Fisher Scientific, Atlanta, GA). Subsequently, the filtrate was filtered again through a Whatman GF/A glass fiber filter (Fisher Scientific, Atlanta, GA) using 2% TCA to rinse the flask. Both Whatman 541 and GF/A filters were dried at 55°C overnight and then subjected to Kjeldahl analysis.
DNA Extraction and qPCR Analysis
Total DNA of ruminal microbes was extracted using the cetyl trimethyl ammonium bromide (CTAB) plus bead-beating method (18). The air-dried DNA pellet was re-suspended in Tris-EDTA buffer (10 mM Tris-HCl, 1 mM EDTA, pH 8) and was stored at −20°C until further processing (3). The DNA purity (A260/280) was detected using a microplate spectrophotometer (SpectraMax 190, Sunnyvale, USA). Then the DNA samples were diluted to 10 ng/μl for real-time qPCR amplification. The qPCR primers used in this study (Table 2) were assembled according to previous studies (19–21) and obtained from Sangon Biotech Co. Ltd. (Shanghai, China). By following the manual instruction, the qPCR was performed using Takara SYBR® Premix Ex Taq. Synthesis Kit (Code No. RR420A, Takara, Dalian, China). The relative abundance of bacterial species was expressed as a proportion in total estimated bacterial 16S rDNA by the calculation of relative abundance = 2−(Cttarget−Cttotalbacteria), where Ct represents threshold cycle (23).
Statistical Analysis
The data were analyzed using PROC MIXED model in SAS (version 9.4, SAS Institute Inc., Cary, NC, USA). The model included the fixed effects of different carbohydrate substrates (C:S ratio = 0:100, 25:75, 50:50, 75:25, and 100:0), incubation time (6, 12, 18, and 24 h), their interaction (C:S ratio × incubation time), and random effect of experimental run. Statistical significance was declared at P < 0.05. To investigate the relationships between OBCFA and rumen parameters, a Spearman's rank correlation analysis was performed in this study. A significant correlation was defined P < 0.05 and a strong correlation was defined when −0.5 > ρ > 0.5 and P < 0.05, where ρ is defined as the Spearman rank-order correlation coefficient. In this study, only significant correlations are shown.
Results
Effect of Different Ratios of Cellulose and Starch on Ruminal OBCFA Synthesis During 24 h of Incubation in vitro
Data on the effect of the different pure C:S ratios on ruminal OBCFA synthesis (mg/g DM) during 24 h incubation in vitro are summarized in Table 3. All measured OBCFA including iso-C15:0, anteiso-C15:0, C15:0, iso-C16:0, iso-C17:0, and anteiso-C17:0 was altered (P < 0.001) by C:S ratios and incubation time. However, the significant interactions (P < 0.01) between C:S ratios and incubation time (except for iso-C17:0), prohibit unequivocal comparison of main effects. Among OBCFA, anteiso-C15:0 and C15:0 were the top two abundant fatty acids, accounting for 17.6–23.6% and 15.4–38.1% of the total OBCFA, respectively. The total OBCFA concentration ranged from 1.65 to 3.94 mg/g DM in different residual samples after in vitro incubations. The highest concentration of total OBCFA (3.94 mg/g DM) was recorded in the fermented mixture after 24 h fermentation when the C: S ratio was 0:100, while the lowest concentration of OBCFA (1.65 mg/g DM) was produced after 6 h of incubation when the C:S ratio was 50:50. The concentrations of C15:0, iso-C16:0, iso-C17:0, anteiso-C17:0, and C17:0 were linearly and/or quadratically changed (P < 0.05) as cellulose proportion increased to 100% in the substrates. The OBCFA concentration varied quadratically (P < 0.05), wherein it decreased as the cellulose ratio increased up to 50%, and then increased gradually.
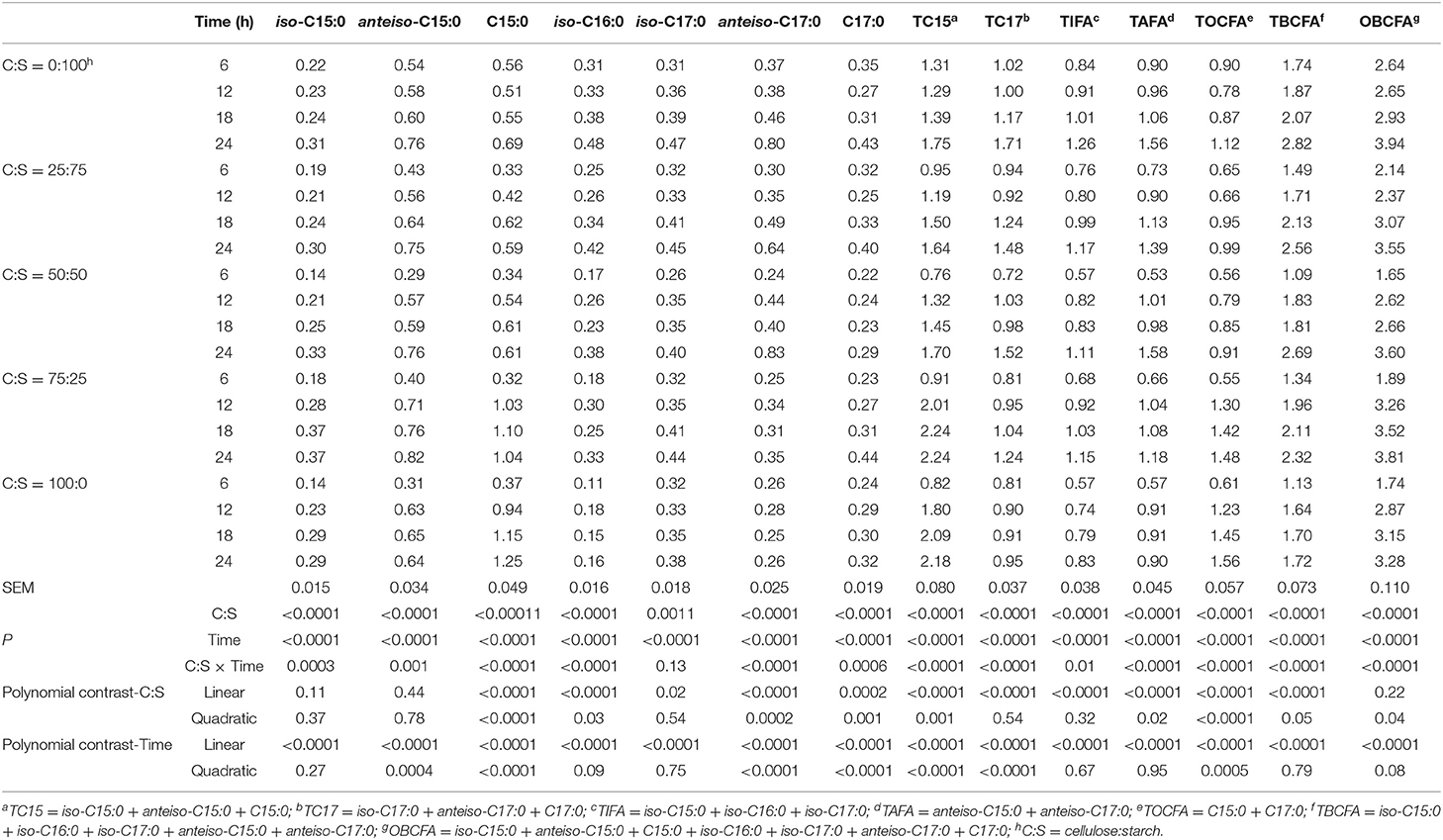
Table 3. Effect of different ratios of cellulose and starch (C:S) on ruminal OBCFA synthesis (mg/g DM) during 24 h of incubation in vitro.
The concentrations of iso-C15:0, iso-C16:0, iso-C17:0, total iso-fatty acids (TIFA), total anteiso-fatty acids (TAFA), total branched-chain fatty acids (TBCFA), and OBCFA linearly increased (P < 0.05), while the remaining OBCFA parameters were altered in both linear and quadratic fashions (P < 0.05) in all fermentation substrates, as incubation time increased within 24 h ruminal incubation in vitro.
Effect of Substrates With Different Ratios of Cellulose and Starch on Ruminal Fermentation Parameters and Microbial Protein Synthesis During 24 h Incubation in vitro
Except for acetate, total VFA (TVFA), pH and MCP synthesis, and all measured ruminal fermentation parameters had significant interactions (P < 0.05) between C:S ratio and incubation time, prohibiting unequivocal comparison of main effects (Table 4). The molar proportions of propionate, isobutyrate, butyrate, isovalerate, and acetate/propionate altered in linear and quadratic fashions (P < 0.05), whereas TVFA concentration remarkably decreased linearly (P < 0.05) as the inclusion of cellulose in the substrates increased. The in vitro ruminal NH3-N concentrations increased (linear or quadratic; P < 0.05) when the starch supply reduced in the substrates. The microbial crude protein synthesis decreased linearly (P < 0.05) as C:S ratio increased. The total VFA concentration increased while pH value and NH3-N concentrations decreased linearly as incubation time increased (P < 0.05).
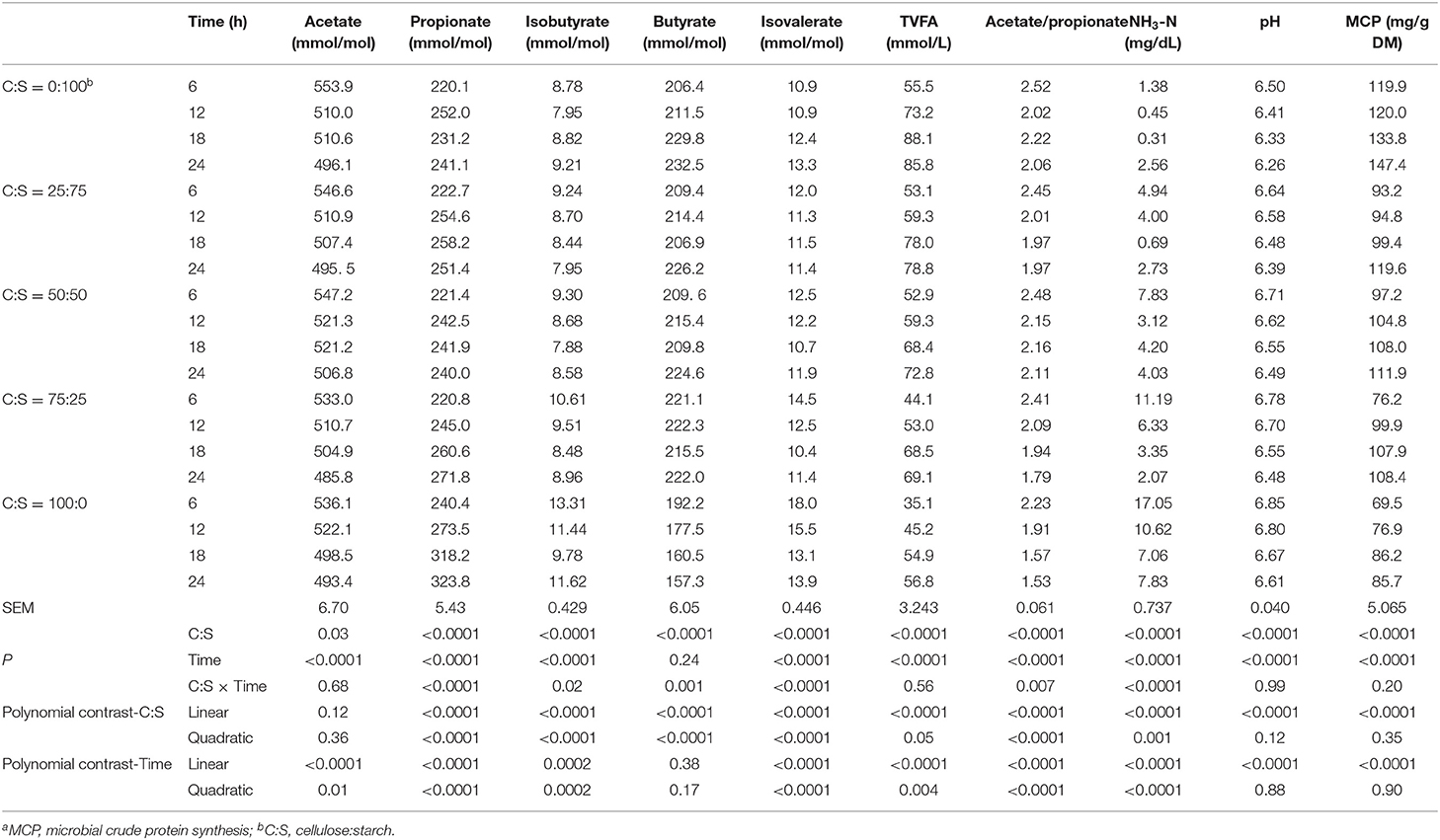
Table 4. Effect of different ratios of cellulose and starch (C:S) on ruminal fermentation parameters and MCPa concentration during 24 h of incubation in vitro.
Effect of Substrates With Different Ratios of Cellulose and Starch on Bacterial Population Composition During 24 h Incubation in vitro
In the present study, three cellulolytic bacteria (R. albus, R. flavefaciens, and B. fibrisolvens) and three amylolytic bacteria (S. ruminantium, R. amylophilus, and S. bovis) populations were measured using the real-time qPCR system (Table 5). Among the bacterial population, R. flavefacien (2.23–29.28%) and R. amylophilus (0.3–28.91%) were the top two abundant bacteria during 24 h of in vitro incubation. The third predominant bacterium that is S. ruminantium which accounted for.42–1.11%, was not affected (P > 0.05) by C:S ratio and incubation time. Except for S. ruminantium, significant interactions (P < 0.05) between C:S ratio and incubation time was observed for all bacterial population. The proportions of investigated cellulolytic bacteria in the present in vitro study increased linearly or quadratically (P < 0.05), while those of Ramylophilus and S. bovis decreased (P < 0.05) as cellulose inclusion increased in the substrates. For each kind of mixed carbohydrate substrates, the relative abundances of all the bacteria (except S. ruminantium) had marked reductions overtime during the 24-h incubation, in linear or quadratic fashions (P < 0.05).
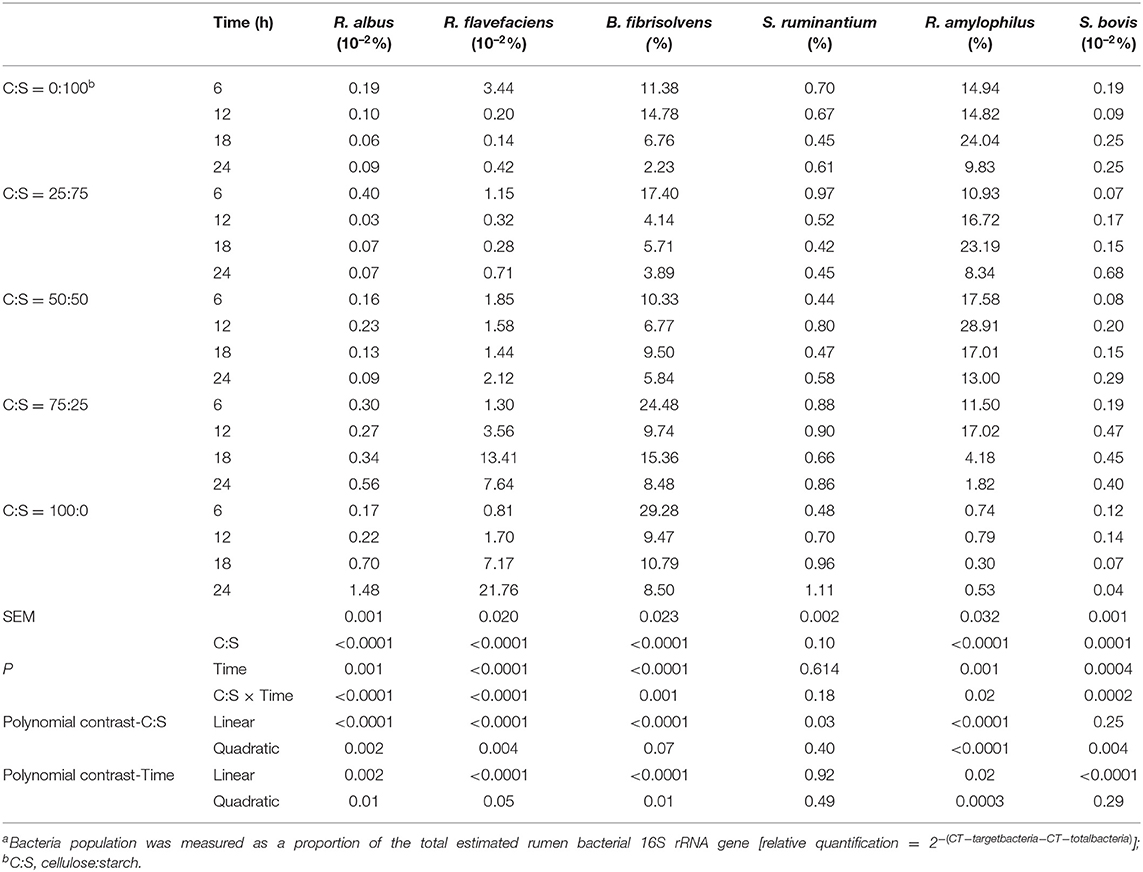
Table 5. Effect of different ratios of cellulose and starch (C:S) on ruminal bacteria populationsa during 24 h of incubation in vitro.
Correlation of OBCFA Synthesis With Fermentation Parameters and Bacterial Populations During 24 h Incubation in vitro
Data used for correlation analysis are summarized in Table 6. Figure 1 shows results of Spearman's rank correlation analysis between ruminal fermentation products and OBCFA synthesis during 24 h in vitro rumen fermentation of the different mixtures of pure carbohydrates. In general, strong correlations were observed between the molar proportion of acetate and concentration of OBCFA than between other individual VFA molar proportion and concentration of OBCFA (Figure 1). The acetate molar proportion was negatively correlated (P < 0.05; ρ = −0.32 to −0.69) with all measured OBCFA concentrations. Within these correlations, 10 were relatively stronger (ρ < −0.50). The molar proportion of propionate was positively correlated with concentrations of C15:0 (P < 0.05; ρ = 0.77), TC15 (TC15 = iso-C15:0 + anteiso-C15:0 + C15:0; P < 0.05; ρ = 0.67) and TOCFA (TOCF = C15:0 + C17:0; P < 0.05; ρ = 0.73), while the molar proportion of isobutyrate (P < 0.05; ρ = −0.60) and butyrate (P < 0.05; ρ = 0.65) had significant correlation with iso-C16:0 concentration. The concentration of TVFA had positive correlation with all measured OBCFA parameters (P < 0.05; ρ = 0.49–0.78), except C15:0, TC15 and TOCFA (P > 0.05).
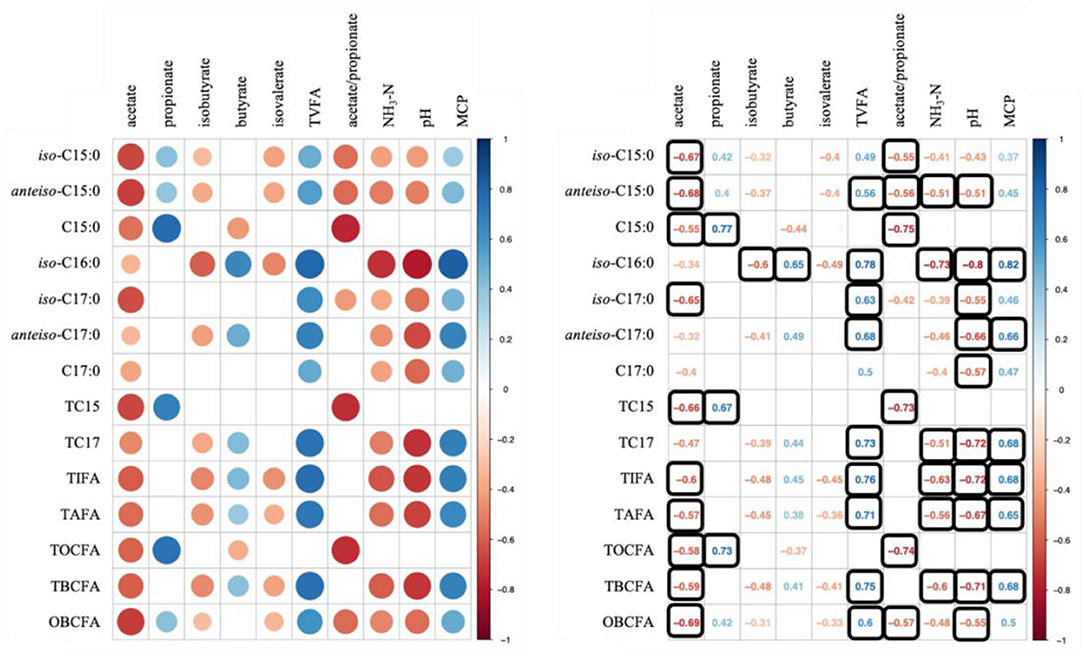
Figure 1. Correlation analysis between ruminal fermentation parameters/MCP and OBCFA concentrations during 24 h of incubation in vitro. Color in round shape represents significant correlations (P < 0.05) and black square means −0.50 > ρ > 0.50.
Except C15:0, the concentrations of OBCFA were negatively correlated with NH3-N (P < 0.05; ρ = −0.39 to −0.73) and positively correlated with MCP synthesis (P < 0.05; ρ = 0.37–0.82). In our study, the strong correlation of MCP concentration was observed with iso-C16:0 (ρ = 0.82) and anteiso-C17:0 (ρ = 0.66) concentrations.
We further investigated the correlations between OBCFA and bacterial populations (Figure 2). Stronger correlations were observed between OBCFA production and cellulolytic bacteria (R. albus, R. flavefaciens, and B. fibrisolvens) than between OBCFA and starch-degrading bacteria (S. ruminantium, R. amylophilus, and S. bovis). Notably, the relative abundance of R. albus (P < 0.05; ρ = 0.58) and R. flavefaciens (P < 0.05; ρ = 0.70) were positively correlated with C15:0, while that of B. fibrisolvens abundance was negatively correlated with anteiso-C15:0 (P < 0.05; ρ = −0.57) and iso-C16:0 (P < 0.05; ρ = −0.56). Although statistically significant, the correlation coefficient between OBCFA and S. ruminantium, R. amylophilus, and S. bovis populations were all lower than.50.
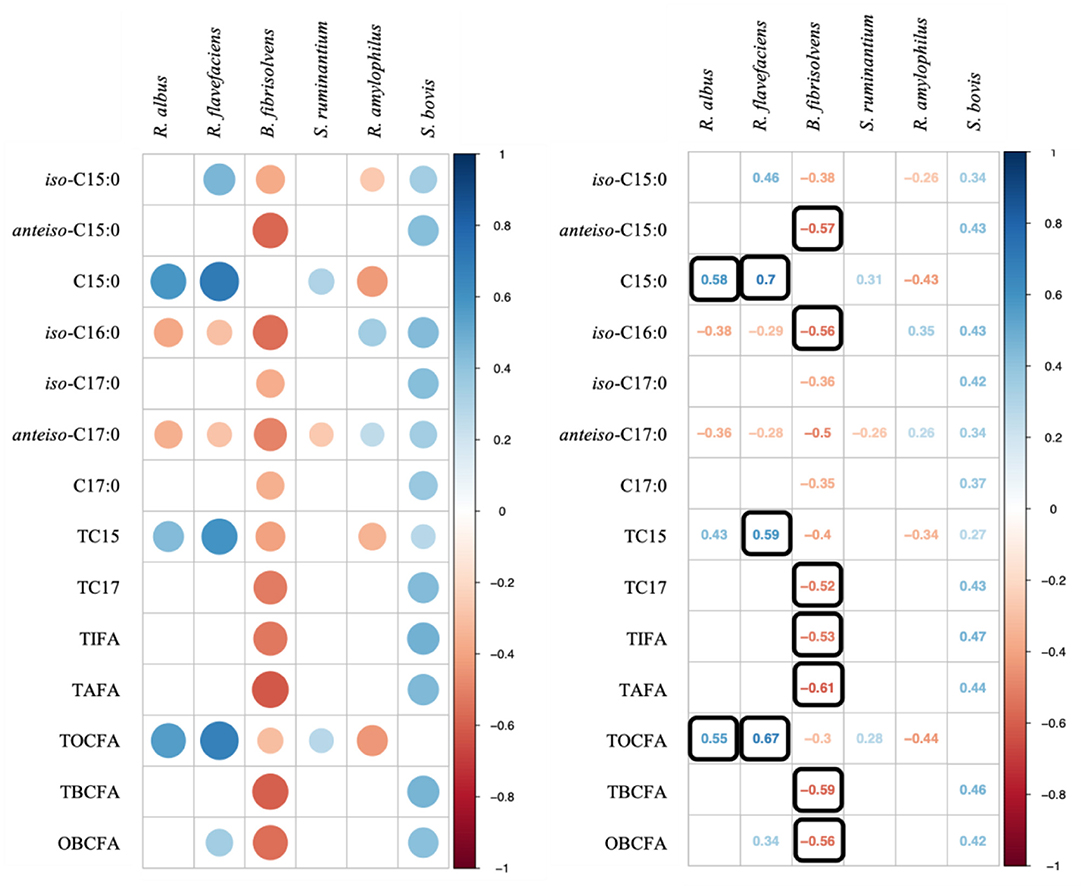
Figure 2. Correlation analysis on ruminal bacterial populations and OBCFA concentrations during 24 h of incubation in vitro. Color in Q22 round shape represents significant correlations (P < 0.05) and black square means −0.50 > ρ > 0.50.
Discussion
The concentration of anteiso-C15:0 (17.6–23.6%) and C15:0 (15.4–38.1%) observed in the current study are consistent with those (anteiso-C15:0, 24.7–29.6%; C15:0, 24.9–27.9%) observed by the study of Vlaeminck et al. (1) in fermented mixtures after 21 h incubation (in vitro) of four types of dairy diets. Moreover, the concentration of anteiso-C15:0 and C15:0 observed in this study was consistent with those observed in rumen bacteria (anteiso-C15:0, 23.76%; C15:0, 25.7%) collected from Holstein cows under different feeding regimes (24, 25). The study of Vlaeminck et al. (26) reported a close relationship (r = −0.77) between anteiso-C15:0 concentration and dietary forage inclusion levels in the diet of dairy cows. However, large changes in anteiso-C15:0 concentrations were not observed in this study with increasing levels of cellulose during in vitro incubation. Generally, the odd-chain fatty acids were formed using propionate as a primer (4). As for the branched-chain fatty acids, the iso-fatty acids were produced from isobutyrate and isovalerate, while anteiso-fatty acids originated from 2-methybutyrate in ruminants (12). The synthesis of these short-chain fatty acids was not only influenced by diet composition and feeding strategies (3, 27), but also highly dependent on the ruminal environment and physiological conditions of dairy cows (28). Indeed, studies regarding ruminal OBCFA synthesis in cows fed rations with different ratios of forage and concentrate have shown contrasting results. With increasing inclusion levels of forages in the diet, total OBCFA synthesis has been reported to be linearly increased in the study of Vlaeminck et al. (26). However, the study of Zhang et al. (3) observed that with increasing inclusion levels of forages in the diet OBCFA synthesis changed quadratically in rumen contents after 6 h morning feeding, which is partially in line with our data. As known, the OBCFA, especially BCFA, was mainly derived from bacteria and they are the unique components of cell membrane lipids in bacteria (4), but also exist in rumen protozoa (24, 25). Therefore, the increase in OBCFA synthesis during the 24 h incubation in the present study, indicated that the microorganisms were keeping a strong reproducibility and proliferation during the whole in vitro incubation. This represented a good condition for microbial metabolic process and fermentation activities. However, the decreases in ruminal OBCFA synthesis during 12–17 h after feeding (2, 29) mainly disagree with our results. This might be due to the characteristics of the ruminal in vitro culture system, in which the fermentation products, e.g., VFAs, could not outflow nor be absorbed, as done by rumen mucosal epithelia, which might have contributed to the accumulation of OBCFA over time, as observed in our study.
The observed VFA profiles in the present study are in accordance with recent studies of Wang et al. (23) and Li et al. (30) who all reported that increasing dietary forage proportion decreased TVFA production in the rumen of dairy cows. The increasing proportion of fermented carbohydrates, such as starch, enhanced the microbial population and they capture more of the released NH3-N to synthesize MCP (30), which partly explained our results. As well-known, the short-chain fatty acids (e.g., VFA) produced during rumen fermentation were readily absorbed by rumen epithelial papilla in ruminants (31). However, failure of VFA absorption in the batch in vitro system resulted in higher accumulation of VFA and a consequent decrease in pH values as incubation time progresses, which explained our findings. Furthermore, the decrease in NH3-N concentrations overtime might suggest that these released nutrients were utilized by microorganisms, which corresponded well with the increased production of MCP overtime during the 24 h of incubation in our in vitro study.
Increasing dietary forage proportion promotes the growth of cellulose-degrading bacteria and reduces the relative abundance of amylolytic bacteria in the rumen (23, 32), which supported the findings of our study. The observed reductions of the relative abundance of all the bacteria (except S. ruminantium) during 24 h incubation indicated microbial lysis and decomposition, as previously reported for a batch in vitro system by the study of Wells and Russell (33). Moreover, the study of Cone et al. (34) observed that microbial activities in rumen fluid stored anaerobically at 39°C for 24 h was considerably decreased as compared with fresh inoculum.
Rumen fermentation products, namely short-chain fatty acids, are usually regarded as precursors for estimation of OBCFA synthesis (12). The quantities of OBCFA have been reported to be a potential predictor for microbial growth (2), since these fatty acids, especially branched-chain fatty acids, are the consistent components of bacterial membrane lipids (4). However, studies exploring the relationship between rumen VFA and OBCFA, have shown mixed results. Despite this, our results are in part consistent with previous findings of an in vitro incubation trial with nine mixed dairy cow rations (1), which reported a negative correlation of acetate with C15:0 and iso-C17:0, and a positive correlation of propionate with C15:0. The study of French et al. (6) observed that ruminal OBCFA response was minor, following infusion of large amounts of acetate and propionate. The findings of this study extrapolated that extracellular VFA concentrations did not alter rumen OBCFA concentration. Furthermore, the rumen OBCFA profiles have been reported to be largely determined by the relative abundance of specific microbial strains rather than the availability of precursors (12, 35).
The correlations of OBCFA concentrations with NH3-N should be largely interpreted concerning the utilization and incorporation of NH3-N by rumen microorganisms into MCP, which contain OBCFA in their cell membranes (4). Similarly, the study of Zhang et al. (3) showed that there was a negative relationship between NH3-N and OBCFA in the rumen contents of Holstein cows. Even though data on MCP synthesis was not available in previous works of literature, indirect comparisons could be made. For example, the studies conducted by Vlaeminck et al. (2) and Liu et al. (8) demonstrated that the total OBCFA or the sum of some specific OBCFA, e.g., C13:0, C15:0, iso-C15:0, and C17:0, was closely related to microbial markers, such as uracil and purine bases (2), and pyrimidine (8). The synthesis of microbial protein is a very complex biological process, which is related to many factors such as the activity of the different enzymes, VFA production and ammonia utilization. All these factors influence the activities of the microorganisms, including their reproduction and lysis as well as OBCFA concentration which are present in microbial membrane lipids. Therefore, the strong correlation of MCP concentration with iso-C16:0 and anteiso-C17:0 concentrations observed in our study indicated that these two fatty acids, especially iso-C16:0 were a good index for estimation of microbial flow from the rumen.
Changes in rumen microbial population, which were driven by altered diet composition or environmental conditions (36, 37), might lead to variations in OBCFA concentrations. Based on the available data from pure culture studies, it was evident that the OBCFA compositions were always species-specific (10, 38, 39), or even varied widely in microbial strains (11, 39). Some strains of R. albus spp., e.g., R albus 7, contained a high amount of linear odd-chain fatty acids (40), and this is also supported by the positive correlation between the concentration of C15:0 and TOCFA with R. albus relative abundance in the present study. Several studies have reported that iso-C15:0 is the predominant OBCFA in the pure cultured strains of R. flavefaciens (R. flavefaciens FD1 and C94), accounting for 37–41% of total fatty acids (35, 40). Combining these results, along with the positive correlation between iso-C15:0 and R. flavefaciens in our experiment, indicated that more abundance of this bacteria might produce a high level of iso-acids in the rumen. Increasing dietary forage provision would lead to the rapid growth of cellulolytic bacteria such as R. flavefaciens (23, 41), and results in higher production of iso-C15:0 in the rumen, as expected (3). However, the strongest correlation of R. flavefaciens was with C15:0 concentration in the present study, which seemed to be discrepant with measured cellular fatty acid compositions in pure culture studies (35, 40). As summarized by the study of Vlaeminck et al. (12), B. fibrisolvens had a heterogeneous profile of OBCFA, which might partly explain the negative relationship observed in our study. The observed weak to moderate correlations between amylolytic bacteria and OBCFA in the present study are consistent with the results of the study by Zhang et al. (3), who found a negative correlation between S. ruminantium with anteiso-C17:0 (ρ = 0.21, P < 0.05), and a positive relationship between S. bovis and C15:0 (ρ = 0.44, P < 0.05) and total OBCFA (ρ = 0.37, P < 0.05) in 108 rumen samples obtained from dairy cows fed rations with different ratios of forage and concentrate.
Conclusions
In vitro ruminal incubation of pure carbohydrate substrates with different C:S ratios, i.e., 0:100, 25:75, 50:50, 75:25, and 100:0, produced different amounts of OBCFA. Except for iso-C17:0, the concentrations of all individual OBCFA were affected (P < 0.05) by the interaction of the C:S ratio and incubation time. The highest concentration of total OBCFA (3.94 mg/g DM) was observed in the fermented mixture after 24 h incubation when the C:S was 0:100, while the lowest concentration of OBCFA (1.65 mg/g DM) was produced after 6 h of incubation when the C:S was 50:50. The odd- and branched-chain fatty acids concentrations were closely correlated to rumen VFA, MCP, and bacterial populations. In particular, the iso-C16:0 concentration might have potential as a marker of estimation of total VFA and MCP concentrations with ρ being.78 and.82, respectively. Compared with starch degrading bacteria, cellulolytic bacteria had relatively stronger correlations with OBCFA profiles. However, to achieve a final accurate conclusion on the relationship of OBCFA with rumen fermentation profiles, more in-depth experiments are needed to substantiate the current findings.
Data Availability Statement
The original contributions presented in the study are included in the article/supplementary material, further inquiries can be directed to the corresponding authors.
Ethics Statement
The animal study was reviewed and approved by the Ethical Committee of the College of Animal Science and Technology of Northeast Agricultural University.
Author Contributions
HX, NK, XLiu, and XLi: supervision, conceptualization, funding acquisition, project administration, and writing of the original draft. XJ, FS, SZ, YS, and YZ: conceived, designed investigation, conceptualization and formal analysis, review, and editing. All authors contributed to the article and approved the submitted version.
Funding
This study was supported by the National Natural Science Foundation of China (Grant No. 31702135) and the China Agricultural Research System of MOF and MARA and Heilongjiang Provincial Dairy Industry and Technology System.
Conflict of Interest
XLiu and SZ were employed by the company Wellhope Feed Company Limited.
The remaining authors declare that the research was conducted in the absence of any commercial or financial relationships that could be construed as a potential conflict of interest.
Publisher's Note
All claims expressed in this article are solely those of the authors and do not necessarily represent those of their affiliated organizations, or those of the publisher, the editors and the reviewers. Any product that may be evaluated in this article, or claim that may be made by its manufacturer, is not guaranteed or endorsed by the publisher.
Abbreviations
anteiso-C15:0, 12-methyltetradecanoic acid; anteiso-C17:0, 14-methylhexadecanoic acid; C15:0, pentadecanoic acid; C17:0, heptadecanoic acid; DM, dry matter; C:S, cellulose:starch ratio; iso-C15:0, 13-methyltetradecanoic acid; iso-C16:0, 14-methylpentadecanoic acid; iso-C17:0, 15-methylhexadecanoic acid; MCP, microbial crude protein; NH3-N, ammonia nitrogen; OBCFA, odd- and branched-chain fatty acids; TCA, trichloroacetic acid; TIFA, total iso-fatty acids; TAFA, total anteiso-fatty acids; TOCFA, total odd-chain fatty acids; TBCFA, total branched-chain fatty acids; TVFA, total volatile fatty acids; VFA, volatile fatty acids.
References
1. Vlaeminck B, Fievez V, Van Laar H, Demeyer D. Rumen odd and branched chain fatty acids in relation to in vitro rumen volatile fatty acid productions and dietary characteristics of incubated substrates. J Anim Physiol An N. (2004) 88:401–11. doi: 10.1111/j.1439-0396.2004.00497.x
2. Vlaeminck B, Dufour C, Van Vuuren AM, Cabrita ARJ, Dewhurst RJ, Demeyer D, et al. Use of odd and branched-chain fatty acids in rumen contents and milk as a potential microbial marker. J Dairy Sci. (2005) 88:1031–42. doi: 10.3168/jds.S0022-0302(05)72771-5
3. Zhang Y, Liu K, Hao X, Xin H. The relationships between odd-and branched-chain fatty acids to ruminal fermentation parameters and bacterial populations with different dietary ratios of forage and concentrate. J Anim Physiol An N. (2017) 101:1103–14. doi: 10.1111/jpn.12602
4. Kaneda TO. Iso-and anteiso-fatty acids in bacteria: biosynthesis, function, and taxonomic significance. Microbiol Mol Biol R. (1991) 55:288–302. doi: 10.1128/mr.55.2.288-302.1991
5. Kaneda T. Fatty acids of the genus Bacillus: an example of branched-chain preference. Bacteriol. R. (1977) 41:391. doi: 10.1128/br.41.2.391-418.1977
6. French EA, Bertics SJ, Armentano LE. Rumen and milk odd-and branched-chain fatty acid proportions are minimally influenced by ruminal volatile fatty acid infusions. J Dairy Sci. (2012) 95:2015–26. doi: 10.3168/jds.2011-4827
7. Vlaeminck B, Gervais R, Rahman MM, Gadeyne F, Gorniak M, Doreau M, et al. Postruminal synthesis modifies the odd-and branched-chain fatty acid profile from the duodenum to milk. J Dairy Sci. (2015) 98:4829–40.
8. Liu K, Hao X, Li Y, Luo G, Zhang Y, Xin H. The relationship between odd-and branched-chain fatty acids and microbial nucleic acid bases in rumen. Asian Austral J Anim. (2017) 30:1590. doi: 10.5713/ajas.16.0966
9. Broderick GA, Merchen NR. Markers for quantifying microbial protein synthesis in the rumen. J. Dairy Sci. (1992) 75:2618–32. doi: 10.3168/jds.S0022-0302(92)78024-2
10. Ifkovits RW, Ragheb HS. Cellular fatty acid composition and identification of rumen bacteria. Appl Microbiol. (1968) 16:1406–13. doi: 10.1128/am.16.9.1406-1413.1968
11. Miyagawa E. Cellular fatty acid and fatty aldehyde composition of rumen bacteria. J Gen Appl Microbiol. (1982) 28:389–408. doi: 10.2323/jgam.28.389
12. Vlaeminck B, Fievez V, Cabrita AR, Fonseca AJ, Dewhurst RJ. Factors affecting odd-and branched-chain fatty acids in milk: a review. Anim Feed Sci Tech. (2006) 131:389–417. doi: 10.1016/j.anifeedsci.2006.06.017
13. NRC. Nutrient Requirements of Dairy Cattle. 7th rev. ed. Washington, DC: National Academy of Science (2001).
14. Wang Y, Frutos P, Gruber MY, Ray H, McAllister TA. In vitro ruminal digestion of anthocyanidin-containing alfalfa transformed with the maize Lc regulatory gene. Can J Plant Sci. (2006) 86:1119–30. doi: 10.4141/P06-001
15. Sukhija PS, Palmquist DL. Rapid method for determination of total fatty acid content and composition of feedstuffs and feces. J Agr Food Chem. (1988) 36:1202–6. doi: 10.1021/jf00084a019
16. Broderick GA, Kang JH. Automated simultaneous determination of ammonia and total amino acids in ruminal fluid and in vitro media1. J Dairy Sci. (1980) 63:64–75. doi: 10.3168/jds.S0022-0302(80)82888-8
17. Hall MB, Herejk C. Differences in yields of microbial crude protein from in vitro fermentation of carbohydrates. J Dairy Sci. (2001) 84:2486–93. doi: 10.3168/jds.S0022-0302(01)74699-1
18. Yu Z, Morrison M. Improved extraction of PCR-quality community DNA from digesta and fecal samples. Biotechniques. (2004) 36:808–12. doi: 10.2144/04365ST04
19. Denman SE, McSweeney CS. Development of a real-time PCR assay for monitoring anaerobic fungal and cellulolytic bacterial populations within the rumen. FEMS Microbiol Ecol. (2006) 58:572–82. doi: 10.1111/j.1574-6941.2006.00190.x
20. Yang S. Effects of soybean oil and linseed oil supplementation on population of ruminal bacteria and fermentation parameters in dairy cows (PhD thesis). Chinese Academy of Agricultural Sciences, Beijing, China (2007).
21. Stevenson DM, Weimer PJ. Dominance of Prevotella and low abundance of classical ruminal bacterial species in the bovine rumen revealed by relative quantification real-time PCR. Appl Microbiol Biotechnol. (2007) 75:165–74. doi: 10.1007/s00253-006-0802-y
22. Khafipour E, Li S, Plaizier JC, Krause DO. Rumen microbiome composition determined using two nutritional models of subacute ruminal acidosis. Appl Environ Microb. (2009) 75:7115–24. doi: 10.1128/AEM.00739-09
23. Wang L, Zhang G, Li Y, Zhang Y. Effects of high forage/concentrate diet on volatile fatty acid production and the microorganisms involved in VFA production in cow rumen. Animals. (2020) 10:223. doi: 10.3390/ani10020223
24. Or-Rashid MM, Odongo NE, McBride BW. Fatty acid composition of ruminal bacteria and protozoa, with emphasis on conjugated linoleic acid, vaccenic acid, and odd-chain and branched-chain fatty acids. J Anim Sci. (2007) 85:1228–34. doi: 10.2527/jas.2006-385
25. Bainbridge ML, Saldinger LK, Barlow JW, Alvez JP, Roman J, Kraft J. Alteration of rumen bacteria and protozoa through grazing regime as a tool to enhance the bioactive fatty acid content of bovine milk. Front Microbiol. (2018) 9:904. doi: 10.3389/fmicb.2018.00904
26. Vlaeminck B, Fievez V, Demeyer D, Dewhurst RJ. Effect of forage: concentrate ratio on fatty acid composition of rumen bacteria isolated from ruminal and duodenal digesta. J. Dairy Sci. (2006) 89:2668–78. doi: 10.3168/jds.S0022-0302(06)72343-8
27. Olijhoek DW, Løvendahl P, Lassen J, Hellwing AL, Höglund JK, Weisbjerg MR, et al. Methane production, rumen fermentation, and diet digestibility of Holstein and Jersey dairy cows being divergent in residual feed intake and fed at 2 forage-to-concentrate ratios. J Dairy Sci. (2018) 101:9926–40. doi: 10.3168/jds.2017-14278
28. Roman-Garcia Y. Assessing dietary conditions influencing the requirements by rumen bacteria for branched chain volatile fatty acids (PhD Dissertation). The Ohio State University, Columbus, OH, United States (2019).
29. Liu K. Odd- and branched-chain fatty acids profiles in relation to rumen microbial population and fermentation patterns in dairy cows (PhD Dissertation). Northeast Agricultural University, Harbin, China (2016).
30. Li C, Beauchemin KA, Yang W. Feeding diets varying in forage proportion and particle length to lactating dairy cows: I. Effects on ruminal pH and fermentation, microbial protein synthesis, digestibility, milk production. J Dairy Sci. (2020) 103:4340–54. doi: 10.3168/jds.2019-17606
31. Storm AC, Kristensen NB, Hanigan MD. A model of ruminal volatile fatty acid absorption kinetics and rumen epithelial blood flow in lactating Holstein cows. J Dairy Sci. (2012) 95:2919–34. doi: 10.3168/jds.2011-4239
32. Vlaeminck B, Dewhurst RJ, Demeyer D, Fievez V. Odd and branched chain fatty acids to estimate proportions of cellulolytic and amylolytic particle associated bacteria. J Anim Feed Sci. (2004) 13:235–8. doi: 10.22358/jafs/73848/2004
33. Wells JE, Russell JB. Why do many ruminal bacteria die and lyse so quickly? J Dairy Sci. (1996) 79:1487–95. doi: 10.3168/jds.S0022-0302(96)76508-6
34. Cone JW, Van Gelder AH, Bachmann H. Influence of inoculum source, dilution and storage of rumen fluid on gas production profiles. In: Gas Production: Fermentation Kinetics for Feed Evaluation and to Assess Microbial Activity. Proceedings of the EAAP Satellite Symposium on Gas Production. Wageningen. (2000). p. 15–16.
35. Saluzzi L, Smith A, Stewart CS. Analysis of bacterial phospholipid markers and plant monosaccharides during forage degradation by Ruminococcus flavefaciens and Fibrobacter succinogenes in co-culture. Microbiology. (1993) 139:2865–73. doi: 10.1099/00221287-139-11-2865
36. Xie X, Yang C, Guan LL, Wang J, Xue M, Liu JX. Persistence of cellulolytic bacteria Fibrobacter and Treponema after short-term corn stover-based dietary intervention reveals the potential to improve rumen fibrolytic function. Front Microbiol. (2018) 26:1363. doi: 10.3389/fmicb.2018.01363
37. Dill-McFarland KA, Weimer PJ, Breaker JD, Suen G. Diet influences early microbiota development in dairy calves without long-term impacts on milk production. Appl Environ Microb. (2019) 85:e02141-18. doi: 10.1128/AEM.02141-18
38. Logar RM, Zorec M, Kopečn, ý J. Reliable identification of Prevotella and Butyrivibrio spp. from rumen by fatty acid methyl ester profiles. Folia Microbiol. (2001) 46:57–9. doi: 10.1007/BF02825886
39. Kopečný J, Zorec M, Mrazek J, Kobayashi Y, Marinšek-Logar R. Butyrivibrio hungatei sp. nov. and Pseudobutyrivibrio xylanivorans sp. nov., butyrate-producing bacteria from the rumen. Int J Syst Evol Microbiol. (2003) 53:201–9. doi: 10.1099/ijs.0.02345-0
40. Minato H, Ishibashi S, Hamaoka T. Cellular fatty acid and sugar composition of representative strains of rumen bacteria. J Gen Appl Microbiol. (1988) 34:303–19. doi: 10.2323/jgam.34.303
Keywords: odd- and branched-chain fatty acids, rumen fermentation products, microbial protein, volatile fatty acids, bacterial population
Citation: Xin H, Khan NA, Liu X, Jiang X, Sun F, Zhang S, Sun Y, Zhang Y and Li X (2021) Profiles of Odd- and Branched-Chain Fatty Acids and Their Correlations With Rumen Fermentation Parameters, Microbial Protein Synthesis, and Bacterial Populations Based on Pure Carbohydrate Incubation in vitro. Front. Nutr. 8:733352. doi: 10.3389/fnut.2021.733352
Received: 30 June 2021; Accepted: 23 August 2021;
Published: 22 September 2021.
Edited by:
Yangchun Cao, Northwest A and F University, ChinaReviewed by:
Miao Lin, Yangzhou University, ChinaGang Guo, Shanxi Agricultural University, China
Xing Liu, Boston University, United States
Copyright © 2021 Xin, Khan, Liu, Jiang, Sun, Zhang, Sun, Zhang and Li. This is an open-access article distributed under the terms of the Creative Commons Attribution License (CC BY). The use, distribution or reproduction in other forums is permitted, provided the original author(s) and the copyright owner(s) are credited and that the original publication in this journal is cited, in accordance with accepted academic practice. No use, distribution or reproduction is permitted which does not comply with these terms.
*Correspondence: Hangshu Xin, aGFuZ3NodS54aW5AbmVhdS5lZHUuY24=; Xiang Li, eGxpQG5lYXUuZWR1LmNu
†These authors have contributed equally to this work and share first authorship