- 1College of Animal Science and Technology, Yangzhou University, Yangzhou, China
- 2Department of Animal Science, Laboratory of Animal Developmental Biology, Chungbuk National University, Cheongju, Republic of Korea
- 3Joint International Research Laboratory of Agriculture and Agri-Product Safety, Ministry of Education, Yangzhou University, Yangzhou, China
- 4International Joint Research Laboratory in Universities of Jiangsu Province of China for Domestic Animal Germplasm Resources and Genetic Improvement, Yangzhou, China
Lactoferrin (Lf), existing widely in human and mammalian milk, is a multifunctional glycoprotein with many functions, such as immune regulation, anti-inflammation, antibacterial, antiviral, and antioxidant. These extensive functions largely attribute to its ability to chelate iron and interfere with the cellular receptors of pathogenic microorganisms and their hosts. Moreover, it is non-toxic and has good compatibility with other supplements. Thus, Lf has been widely used in food nutrition, drug carriers, biotechnology, and feed development. Although Lf has been continuously explored and studied, a more comprehensive and systematic compendium is still required. This review presents the recent advances in the structure and physicochemical properties of Lf as well as clinical studies on human diseases, with the aim of providing a reference for further research of Lf and the development of its related functional products.
1. Introduction
Human beings use lactoferrin (Lf) for more time than we can imagine. We often see the word lactoferrin on the packaging of dairy products now, but do you know? As early as the war in Europe, knights used whey from colostrum to clean wounds caused by bows, arrows, and swords to avoid worsening infection. Lactoferrin was first discovered in breast milk by scientist Johanson Bengt in 1960 (1). Then scientists discovered the protein in the body fluids and various cells of some organisms and began a lot of research. In 1971, Rümke et al first found that the content of lactoferrin in serum protein of cancer patients was higher (2). This phenomenon was later found in many types of cancer, and gradually lactoferrin became a sign of cancer. In 1994, Mitra first reported the expression of human lactoferrin in plant cells, and subsequently recombinant human lactoferrin was successfully expressed in a variety of plants including tobacco, potato, rice, tomato, and carrot (3). In 2002, Van Berkel et al. pioneered the construction of transgenic cows expressing high levels of recombinant human lactoferrin, which yielded 0.4–3.0 g/L. The recombinant human lactoferrin was found to have similar biological activity to the natural protein (4). Through the large-scale production of recombinant human lactoferrin, human lactoferrin will have important implications in functional food and healthcare.
Lactoferrin, a kind of iron-bound multifunctional cationic glycoproteins (5), belongs to the transferrin (TF) family together with serum transferrin, ovotransferrin, melanin ferritin, and carbonic anhydrase inhibitor (6). Mainly existing in mammalian milk, it is also distributed in tears, nasal and bronchial secretions, saliva, bile, and other body fluids (7). In addition, Lf is also produced by hematopoietic tissue of bone marrow and is present in neutrophil granules. The composition of milk varies considerably between species, because physiologically and structurally, lactation is designed to meet the nutritional requirements of newborns of a particular species. The content of lactoferrin in breast milk and bovine milk also varies significantly. In donkey milk, lactoferrin accounts for 4.48% of the total whey protein, and its molecular weight is about 75ku. Its content in donkey milk is about 0.4 mg/mL, which is between cow milk and human milk. Although bovine lactoferrin (bLf) shares more than 70% homology with human lactoferrin (hLf), the concentration of hLf colostrum is higher, at about 7 g/L. Currently, the versatility of Lf in food and other industries has led to a surge in its demand and a promising application in the market. It has been added to many commercial products, such as nutritional supplements, infant formula, cosmetics, and toothpaste (8). However, the research on the structure, physical and chemical properties of Lf still lacks systematic introduction and prospect (9). This is what this review will especially explore.
In humans and animals, Lf is mainly found in the products of exocrine glands located at the gateways of the digestive, respiratory, and reproductive systems (10). This suggests Lf plays a role in non-specific defense against invading pathogens and is the first line of defense against microbial and viral infections (11). In addition, in plasma, Lf is derived from neutrophils which degranulate and synthesize Lf during the inflammatory process. In general, the expression of the Lf gene is influenced by a variety of factors and has multiple expression specificities (12). Over the past 60 years, Lf has been extensively studied, and its role in numerous biological functions has been accepted by the scientific community. These days Lf is used as a biomarker for COVID-19 (13), inflammatory bowel disease (IBD) (14), aging (15), and neurological disorders (16). Furthermore, Lf has been shown to be involved in a variety of physiological and protective effects. Some of the most studied to date include antimicrobial activity, antiviral, cell proliferation and differentiation, cytokine regulation, inflammatory response, and immunomodulatory activity (17–19). However, the cellular and molecular mechanisms by which Lf regulates inflammatory, antiviral, and immune responses have not been fully elucidated. Hence, this paper reviews the main characteristics and major biological functions of Lf, and particularly clarify the mechanism of action of using exogenous Lf as a therapeutic agent for human diseases to identify new research prospects. We summarize the layout of this paper in Figure 1.
2. Structure of lactoferrin
Lactoferrin, a cationic iron-binding glycoprotein, consists of a single polypeptide chain (approximately 700 amino acid residues) with a relative molecular mass of around 80 ku (20). In humans, mice, and bovine, Lf homology is high. Among them, the homology reaches 70% between humans and mice, 69% between humans and bovine, and 63% between mice and bovine (21). The secondary structure of Lf is mainly composed of alternating arrangements of α-helix and β-fold. On the basis of it, there is a tertiary structure composed of a polypeptide chain according to the three-dimensional structures of hLf solutions and crystals measured by X-rays. This structure folds into two symmetrical spherical lobes with similar structures, namely the N-lobe and the C-lobe (Figure 2). The N-lobe corresponds to the amino acid residues 1-333 in the peptide chain, while the C-lobe corresponds to the amino acid residues 345-692. These two lobes can be further divided into two regions, respectively, N-1 (amino acids 1-90, 251-333) and N-2 (amino acids 91-250), C-1 (amino acids 345-431, 593-689) and C-2 (amino acids 432-592). In addition, the N-lobe and C-lobe are connected by a small peptide bond (amino acids 334-344), forming the overall structure as “two Ginkgo biloba type,” with each lobe having an iron-binding site in the cleft between the N1 and N2, C1 and C2 sub-structural domains. What’s more, iron ions are covalently linked to four amino acid residues (two tyrosine, one aspartate and one histidine) of Lf (22). In the presence of carbonate, each lobe is able to reversibly bind Fe3+ with a maximum binding amount of 1.4 mg Fe/g protein. In addition to Fe3+, some other metal ions such as Zn2+, Cu2+, and Mn3+ can also be bound with Lf.
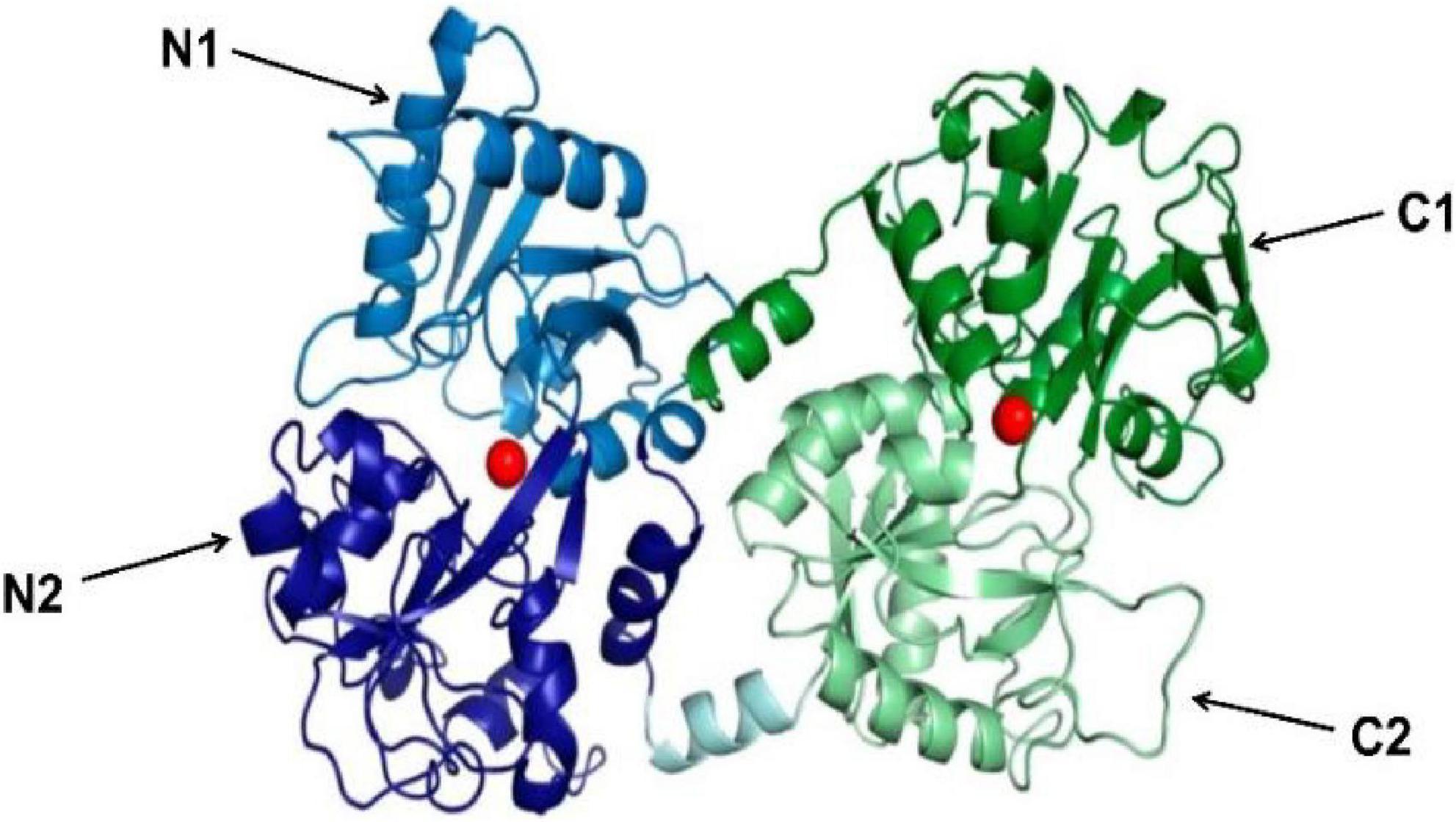
Figure 2. 3D diagram of bovine lactoferrin [adapted from Fabiana Superti et al. (103)]. N-lobe is blue (N1 is light blue, N2 is dark blue), C-lobe is green (C1 is dark green, C2 is light green). The peptide bonds linking N-lobe and C-lobe are expressed in light cyan. The Iron ion is a red sphere.
According to its iron saturation, Lf can be classified into iron-deficient lactoferrin (Apo-Lf) (iron saturation is below 5%) and iron-saturated lactoferrin (Holo-Lf) (iron saturation is above 85%). The denaturation temperatures of these two Lfs are 60–66 and 88–92°C, respectively. In addition, they have different tertiary structures: Apo-LF is characterized by an “open” N-lobe and a “closed” C-lobe, whereas both lobes of Holo-LF are “closed,” thus having a more compact structure than iron-free Lf and iron-binding Lf. The iron saturation of Lf is closely associated with its physicochemical properties. For example, iron-free Lf has stronger antibacterial and antioxidant activities than iron-saturated lactoferrin. Furthermore, the osteogenic activity of Lf decreases with the increase of iron saturation in vitro and in vivo (23). Moreover, the isoelectric point of Lf, a basic protein, fluctuates depending on the degree of iron binding and glycosylation.
The isoelectric point of bLf is about 8.0 and that of hLf is about 6.0. As an important regulator of free iron levels in mammalian body fluids, Lf can bind iron with high affinity and maintain it at a low pH value, thus providing proteins with antibacterial and antioxidant properties (24). The retention rate of iron in bLf is as high as 85% when the environmental pH of Lf is 6.5, but only 10% when the environmental pH is 2.0 (25). The solubility, foaminess, and emulsification properties of bLf are improved after high pressure processing. Bellamy et al. first isolated a segment of bLf from the N-terminal polypeptide called bovine lactoferrin active polypeptide (LfcinB), which was hydrolyzed by pepsin under acidic conditions. LfcinB, derived from amino acids 17-41 of bLf, is an antibacterial peptide containing 25 amino acid residues, including 5 Trp, 3 Lys, and several aromatic amino acid residues. Being strongly basic (pH > 8.15), it can be released in the digestive tract under normal physiological conditions. In addition, LfcinB has a hydrophilic lipid structure with two reverse β-folded structures in aqueous solution, which is heat-resistant and not easily degraded in the digestive tract. More importantly, it exhibits higher antibacterial and anticancer activities than natural Lf.
3. Physicochemical properties and applications of lactoferrin
As mentioned above, the broad functions of Lf can be attributed to its ability to chelate iron and interfere with the cellular receptors of pathogenic microorganisms and their hosts. Moreover, the function of Lf is inextricably linked to its own structure and the surrounding environment. Recently, many studies have indicated Lf has potential emerging applications in the clinical treatment of human diseases, agricultural production, and daily use industries. This section summarizes and sorts out the physicochemical properties and applications of Lf.
3.1. Physicochemical properties of lactoferrin: Antibacterial, antiviral, and anti-inflammatory
Lactoferrin has a high affinity for ferric ions, which is 250–300. times higher than that of TF. Acting as an iron-redox homeostasis (Fe-R-H) regulator, Lf, chelating iron and neutralizing iron-mediated free radicals, reduces oxidative stress and improves host defense by optimizing iron metabolism (26). Lf mainly transports iron ions in the intestine, so it plays a crucial role in the absorption of iron ions in the intestine of mammals. Cellular uptake of iron has a negative feedback regulation. When intracellular iron is deficient, the LfRs on the cell surface subsequently increase and so does the cellular uptake of Lf.
Lactoferrin exerts antibacterial effects against a wide range of microorganisms including bacteria, fungi, viruses and parasites through three different mechanisms: (a) Bacteriostatic activity mediated by chelating free iron, which is essential for bacterial growth and proliferation, i.e., “taking away” bacterial nutrients (27). (b) Lf increases the permeability of bacterial cell membrane through the strong cation-binding region at the amino terminus, causing the exudation of bacterial lipopolysaccharide and other cell contents from the outer membrane, thus producing a direct bactericidal effect. (c) Lf hydrolyzes to obtain antimicrobial peptide, which exerts stronger antimicrobial effect (Figure 3) (28). In 1972, Bullen et al. first suggested that Lf has an antibacterial effect which is dependent on iron concentration (29). Using immunofluorescence, Arnold et al. found that apo-Lf could bind to the surface of the bacterium and detach external nutrients, thus preventing them from entering the bacterium and causing their death (30). A prospective randomized trial showed that in 60 women with bacterial vaginitis (BV), the incidence of BV decreased after vaginal administration of 100 mg and 200 mg of Lf for 10 days. Furthermore, the vaginal microbiota of patients with BV altered and proportion of Lactobacillus increased accordingly (31).
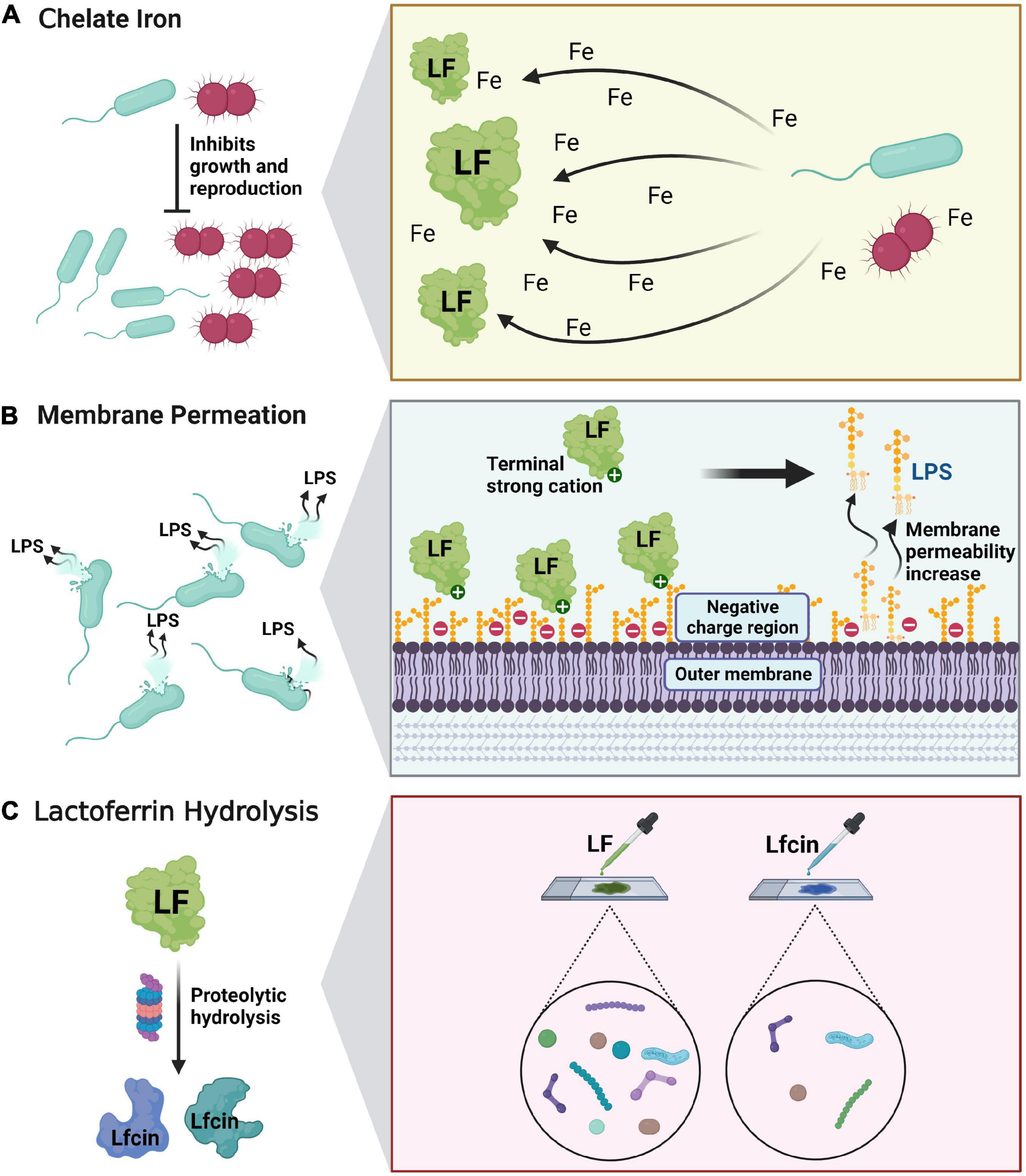
Figure 3. Antibacterial and bactericidal mechanism of lactoferrin. (A) Lactoferrin acts as an antibacterial agent by chelating free iron-mediated activity. (B) Lactoferrin increases the permeability of bacterial cell membranes through the strong cation-binding region at the amino terminus, producing a direct bactericidal effect. (C) Lf hydrolyzes to obtain antimicrobial peptide, which exerts stronger antimicrobial effect.
Lactoferrin also has great potential in the antiviral aspect. So far, studies have shown that Lf is effective against human papilloma virus (HPV), herpes simplex virus 1 (HSV-1), herpes simplex virus 2 (HSV-2), cytomegalovirus (CMV), Human Immunodeficiency Virus (HIV), hepatitis B virus (HBV), hepatitis C virus (HCV), respiratory syncytial virus (RSV), hantaan virus (HV), rotavirus (RV), poliovirus (PV), adenovirus (AdV), and the currently spreading SARS-CoV-2 in in vitro and in vivo experiments (32). In several cell lines, Lf is shown to block the attachment of SARS-CoV-2 virus to cytosolic heparan sulfate and enhance the interferon response (33). In vitro experiments, Campione et al. demonstrated that Lf could exert its antiviral activity against SARS-CoV-2 by directly attaching to SARS-CoV-2 and the surface components of recipient cells (34). SARS-CoV-2 infects ACE2-expressing cells and enters the cells through direct plasma membrane fusion or endocytosis. The antiviral mechanisms of Lf include (a) binding to heparan sulfate proteoglycans (HSPGs) on the surface of host cell, thereby reducing viral attachment and subsequent viral entry; (b) binding directly to viral proteins to inhibit viral adsorption to target cells; and (c) interfering with intracellular transport of virus and intercepting the delivery of viral genomes to the cytoplasm (Figure 4). Lf from different sources may show different antiviral and antibacterial activities. Studies have shown that camel lactoferrin (cLf) inhibits hepatitis C virus (HCV) more than human lactoferrin (hLf), bovine lactoferrin (bLf) and sheep lactoferrin (sLf), respectively (35). This may be related to the iron saturation of Lf, as apo-Lf has a higher antiviral potency than holo-Lf.
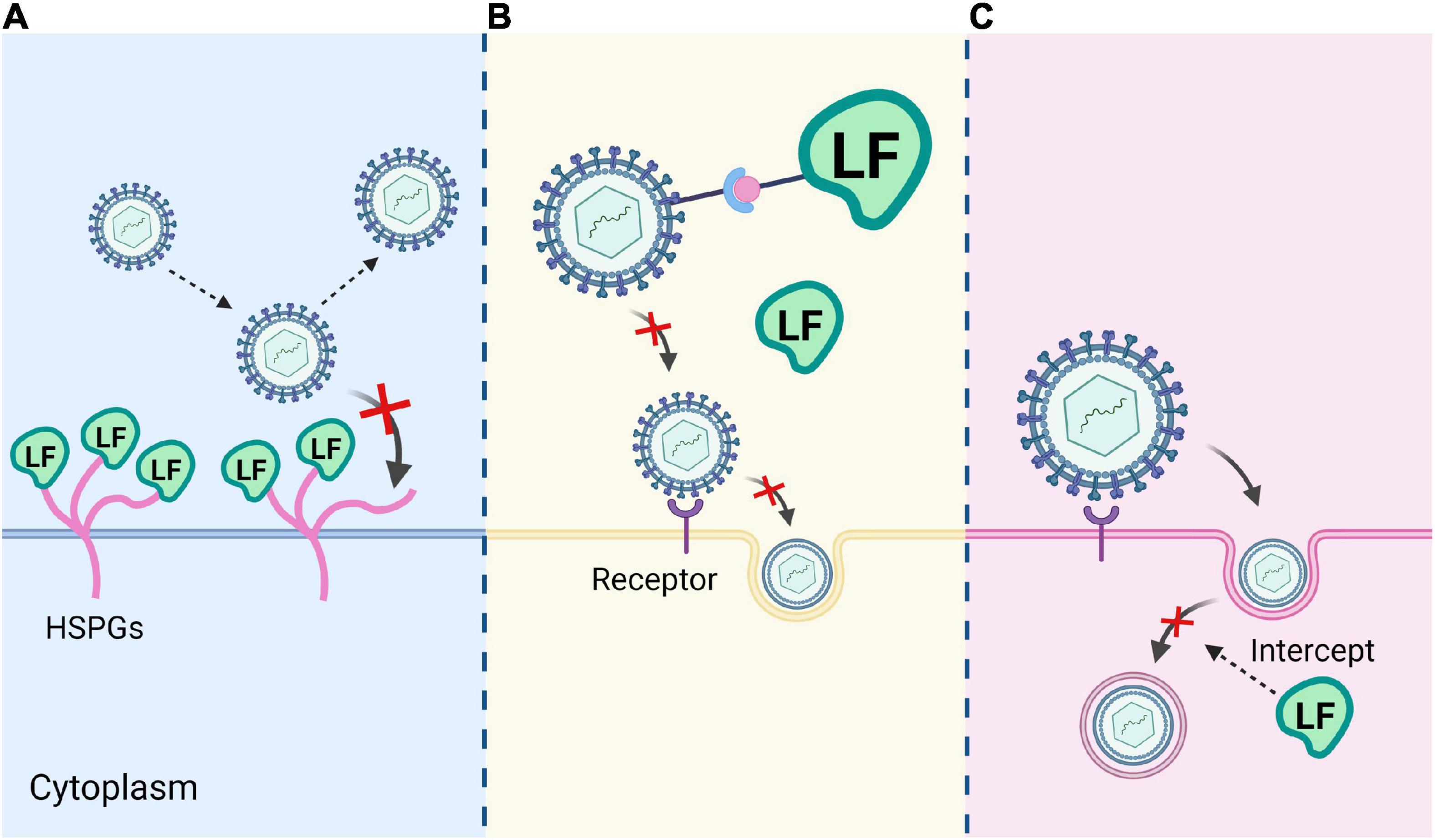
Figure 4. Three mechanisms of Lf antivirus. (A) Lf binds to heparan sulfate proteoglycans (HSPGs) on the host cell surface. (B) Lf binds directly to viral proteins and inhibits viral adsorption to target cells. (C) Lf interferes with intracellular transport of the virus and blocks the transmission of the viral genome to the cytoplasm.
Lactoferrin is a modulator of mammalian innate and acquired immunity, which relies on its ability to bind to conserved structures of pathogens. Lf can be converted into an anti-inflammatory molecule capable of protecting the host from harmful immune responses. Specifically, Lf is immunomodulated by interacting with specific receptors on immune cells such as lymphocytes, monocytes, dendritic cells, macrophages, natural killer cells (NK), etc. Lf interacts with specific receptors on these immune cells. By inhibiting activation of P38 MAPK derived from TLR4 in microglia, bovine lactoferrin (bLf) restrains IL-18 production, thus ameliorating neuropathic pain in mice (36). Oral administration of liposomal bovine lactoferrin (LbLf) effectively prevents the progression of rheumatoid arthritis (RA) in mice by inhibiting tumor necrosis factor (TNF-α) production in the pancreas (37). In addition, Fan et al. found that apo-LF, compared to holo-LF, could significantly attenuate LPS-induced inflammatory bowel injury by modulating the PPAR-γ/PFKFB3/NF-κB inflammatory pathway (38).
3.2. Application in the treatment of human diseases
Lactoferrin has been used in clinical treatment for decades. Since 2020, with the spread of COVID-19, it has been reported that Lf, as a non-toxic, broadly functional “wonder protein,” has great potential in preventing SARS-COV-2. infection and improving patients’ immunity (39). Increased free iron and hyperferritinemia in patients with COVID-19 due to iron dysregulation and overload may exacerbate the inflammatory process through oxidative damage to cellular biomolecules induced by reactive oxygen species (ROS) (13). Facing with a large amount of “cellular debris,” Lf can bind to free iron ions to achieve antioxidant effects, and the antioxidant activity is negatively correlated with iron saturation. Meanwhile, bLf is effective against different variant strains of SARS-COV-2, including South African B.1.351, British B.1.1.7, Brazilian P.1, and Indian Delta variants (40).
3.2.1. Lf and chronic diseases
Chronic diseases, especially cardiovascular disease (CD), diabetes and osteoporosis, have become the focal point of global public health. Khan and co-workers found that the antidiabetic properties of camel milk may be due to the interaction between bLf and the insulin receptor (IR), thereby inducing IR phosphorylation and activating AKT and ERK1/2. signaling pathways (41). Blood pressure is the most important factor in cardiovascular health, and Lf is a good source of orally active antihypertensive peptides (42). The main target of anti-hypertensive food-derived peptides is angiotensin-I-converting enzyme (ACE). After a series of experiments, Ruiz and co-workers found that LfcinB can inhibit ACE activity in mice and reduce systolic blood pressure in spontaneously hypertensive rats (43). In addition, studies have shown that Lfcin significantly prolongs prothrombin kinase and prothrombin time, which is an important entry point for the treatment and prevention of thrombosis (44).
Meanwhile, Lf, a novel bone growth factor, plays a physiological role in bone growth and healing. To be specific, Lf induces the proliferation and differentiation of osteoblasts and inhibits osteoclast formation in bone anabolic activity (45). Guo et al. demonstrated the osteogenic properties of Lf in vivo for the first time and found that Lf has a therapeutic effect on postmenopausal osteoporosis in rats (46). A human whole-transcriptome microarray study, which differentially elucidates the coding and non-coding transcript expression profiles and functional network analysis of human bone marrow mesenchymal stem cells (BMSCs) during osteogenic differentiation, revealed that signaling pathways such as MAPK signaling pathway, TGF-β signaling pathway and Toll-like receptor are associated with osteogenic differentiation of BMSCs (47). Besides, Lf binds to low-density lipoprotein receptor-related protein 1 (LRP1) on the surface of osteoblasts and regulates the ERK1/2 pathway, thus promoting the proliferation of osteoblasts. Lf also induces the activation of p42/44 MAPK signaling in primary osteoblasts. It is reported that LfcinB enables the eradication of Bacillus purpureus and the reduction of Staphylococcus aureus in diabetic mouse wounds. It can also promote migration of keratinocyte and angiogenesis in diabetic mice, being conductive to diabetic wound healing.
3.2.2. Lf and neurodegenerative diseases
Iron imbalance and oxidative stress (OS) are common in patients with neurodegenerative diseases, such as Parkinson’s disease (PD), Alzheimer’s disease (AD), dementia, depression, and multiple sclerosis (MS) (48). Due to the blood-brain barrier (BBB), most central nervous system drugs cannot enter the brain. However, Lf can rapidly cross the blood-brain barrier via receptor-mediated transcytosis and accumulate in brain capillary endothelial cells. Lactoferrin receptors (LfRs) are present on glioma cells, brain microvessels, and neurons. By binding to specific lipoprotein receptors, Lf regulates neuronal differentiation to stimulate the intracellular phosphatidylinositol kinase (PI3K) and extracellular signal-regulated kinase (ERK) transduction pathways, thus upregulating neuron-specific enolase (NSE) expression and promoting neuronal cell differentiation (49). Sokolov et al. found that salivary and tear lactoferrin levels are significantly higher in PD patients than in controls (non-PD patients), and the plasma Lf levels are inversely correlated with the severity of PD (50). Nevertheless, it is noteworthy that although Alzheimer’s patients have the same neurodegenerative disease, their salivary Lf levels are significantly lower compared to controls (non-AD patients). Researchers have explored receptor-mediated approaches to target drug and non-drug molecules to the brain, thereby achieving exogenous gene expression across a wide range of brain regions. Gothwal et al. coupled Lf to polyamidoamine generation 3.0. (PAMAM G3.0) dendrimers to deliver rivastigmine (RIV) to the mouse brain, thus significantly enhancing acetylcholine levels, and improving overall memory in mice drastically (51).
3.2.3. Lf and gastrointestinal health
While inhibiting the growth of harmful intestinal bacteria, Lf promotes the growth of beneficial bacteria such as Lactobacillus acidophilus and Bifidobacterium bifidum, thereby maintaining intestinal health by regulating the balance of intestinal flora. A study of 48. term and preterm infants found that the number of fecal bifidobacteria and lactobacilli correlates significantly with fecal Lf concentrations 3 days after delivery, suggesting that Lf may promote the growth of beneficial intestinal microbiota (52). Although Lf inhibits the growth of harmful bacteria, some researchers have found large amounts of Lf in the resected stomachs of patients with gastritis, speculating that H. pylori takes up iron through specific Lf receptors, and more importantly, Lf may play a major role in the virulent effects of H. pylori infection (53).
On the other hand, Lf is used as a biomarker for inflammatory bowel disease along with fecal calprotectin and serum C-reactive protein in clinical care (54). Compared to patients with inactive inflammation and irritable bowel syndrome (IBS), patients with ulcerative colitis or Crohn’s disease have higher levels of Lf in stool and serum. A double-blind randomized clinical trial in Japanese patients with colorectal polyps showed that bLf suppresses colorectal polyps by enhancing immune responsiveness. To be specific, the polyp growth is significantly inhibited in patients with colorectal polyps who consumed 3.0 g bLf per day 1 year later. Meanwhile, serum hLf levels also increase (enhanced neutrophil activity), along with enhanced systemic NK cell activity and reinforced polyps with CD4+ and CD161+ cells (55).
Additionally, Lf also plays a role in establishing the gut microbiota in newborns. A series of randomized clinical trials were conducted to test the supplementation of enteral bLf, results of which demonstrated a significant reduction in late-onset sepsis (LOS) in preterm or low birth weight infants (56, 57). In another large randomized controlled trial, 2,203 preterm infants (less than 32 weeks) with gestation from 37 hospitals in the United Kingdom were randomly assigned to receive either bLf or an equivalent dose of sucrose placebo until 34 weeks of gestation. Surprisingly, the results showed that enteral supplementation with bLf does not reduce the risk of LOS infection in very preterm infants (58), which is also supported by subsequent studies (59, 60). Studies also indicate that LfcinB is conductive in maintaining and repairing intestinal mucosal tissues and preventing intestinal infection caused by rotavirus (61). For example, in a clinical trial in Japan, researchers found that intake of LfcinB could inhibite human norovirus infection (62).
3.2.4. Lf and cancer cells
A variety of cancer cells are significantly damaged by Lf action, and it has been shown that Lf leads to cell cycle arrest, cytoskeleton damage and apoptosis induction, as well as cell migration reduction through regulation of cell cycle-associated proteins (63). Chea and co-workers reported that bLf selectively inhibits proliferation and induces apoptosis in oral squamous cell carcinoma (OSCC) cells via the mTOR/S6K and JAK/STAT3. pathways (64). In the assay, bLf inhibited phosphorylation of the growth and survival-associated kinases p65 and Akt in OSCC cells, which in turn inhibited activation of the STAT3 signaling pathway and induced G1/S cell cycle arrest, ultimately contributing to OSCC apoptosis. The combination of Lf and linolenic acid, on the one hand, activates the AMPK/JNK-related apoptotic pathway to inhibit growth of colorectal tumor. On the other hand, inhibition of JAK2/STAT3-related pathway suppressed the growth of xenograft esophageal tumors in mice (65).
3.2.5. Absorption and utilization of Lf
The most common Lf on the market is bovine lactoferrin (BLf), which, as a bioactive and nutritional health protein, is non-toxic to animals. Currently, its global production is about 280. tons, of which Lf is ≥95% pure. Another common product of Lf is dairy-derived Lf, which is obtained by freeze-drying and spray-drying after being extracted from skim milk or whey cheese by ion exchange and ultrafiltration separation. The realization of Lf function is highly dependent on its structural integrity. To a large extent, oral Lf is digested in the stomach by gastric juices with only 1% utilization rate, and only trace amounts of intact Lf reach the small intestine (66). Researchers have also developed liposomal bovine lactoferrin (LbLf), a multilayer phospholipid vesicle containing a large number of Lf molecules, which can ensure effective Lf activity during transport. Ishikado and co-workers found that liposomal lactoferrin (LbLf) could improve the resistance of bLf to artificial gastric juice digestion (67). Lf is also incorporated into hyaluronic acid-coated liposomes and then homogenized under high pressure. In vitro and in vivo experiments found that it could reverse the symptoms of dry eye and anti-inflammatory properties without causing eye irritation (68). Xu and coworkers developed a recombinant human lactoferrin (rhLf) transgenic silk for the fabrication of silk sericin nanospheres systems (SS-NS-rhLf). When ambient pH ≥ 5.5, the negatively charged silk gliadin achieves specific targeting of SS-NS-rhLf to the positively charged colonic sites (14).
3.3. Application of lactoferrin in other industries
In agricultural production, the abuse of antibiotics leads to the development of drug-resistant pathogens and drug residues in the body, posing a great threat to human health and public health. BLf can be used as a new animal-derived antimicrobial agent with superior properties such as low residue, non-toxicity and non-resistance, and can be used as a dietary supplement for livestock, poultry and fish. Hu et al. found that early Lf intervention could modulate the composition of the colonic microbiota and improve the intestinal function of suckling pigs (69). Shao et al. reported that supplementation of 100. mg/kg/day of LfcinB in the diet of lactating dairy goats could reduce oxidative stress and inhibit mammary gland inflammation (70). Hashem et al. found that Lf could improve the immune characteristics and antioxidant status of Oreochromis niloticus when added to the diet, showing a better improvement than hyoscine (71). On the whole, it can be seen that Lf is a dietary supplement with excellent properties, playing positive effects on immune regulation, disease resistance and antioxidant in animals.
Lactoferrin is generally recognized as safe (GRAS) by the United States Food and Drug Administration (FDA). Approved by the U.S. Department of State in 2002, activated lactoferrin (aLf) can be sprayed on carcasses to prevent bacterial contamination during processing, or applied to the base or finished beef surface prior to final packaging to inhibit bacterial growth and extend shelf life (72). Using 0.5% Lf solution as a disinfectant has 90–100% killing effect on tobacco mosaic virus after spraying (73). Kobayashi et al. reported that the addition of Lf can effectively improve sperm activity and prolong the survival time of frozen-thawed semen after thawing, because Lf maintains the integrity of sperm plasma membrane and reduces the level of reactive oxygen species (ROS) (74). This has also been reported in horses (75), goats (76), and porcine (77).
Above discusses the role of Lf as an exogenous substance in dietary additives, food processing, and sperm quality maintenance. What is the result of inserting Lf genes into plants and animals using transgenic technology? In 1994, Mitra et al. first reported the introduction of hLf gene into tobacco and other plants to obtain transgenic plants by Agrobacterium tumefaciens-mediated method (3). These transgenic plants showed superior antibacterial and disease resistance as well as iron content. Li et al. first created hLf transgenic dairy cattle by microinjecting 150 kb bacterial artificial chromosome (BAC) containing the entire hLf gene into bovine fetal fibroblasts via plasmid encoding marker gene (78). Afterward, hLf transgenic dairy goats were born on demand. It has been shown that neo-lactoferrin, carrying the full-length hLf gene, is a natural combination of recombinant hLf (90%) and goat lactoferrin (10%) isolated from the milk of transgenic goats, which can enhance IL-1β production. Moreover, Neo-lactoferrin saturated with iron ions increases the synthesis of the pro-inflammatory cytokine TNF-α (Figure 5).
4. What factors influence the regulation of lactoferrin gene expression?
The expression of Lf gene is influenced by many factors, and Lf was first identified as an estrogen-sensitive protein (79). To date, researchers have conducted a series of studies on the mechanisms of lactoferrin gene expression regulation in terms of its structure, nuclear receptors, transcription factors, and external factors such as nutrition.
The Lf gene is species-specific, and at least 60 Lf gene sequences have been identified in 11 mammalian species, of which hLf and bLf share 67% primary structure homology. The hLf gene is located on human chromosome 3p21.3 (80), the bLf gene is localized on chromosome 22 and U12 (81), and the rat Lf gene is localized on chromosome 9 (82) (Table 1). Researchers have cloned lactoferrin gene from a number of mammals, including humans, murine, bovine and porcine. It is found that all these genes are highly conserved and consist of 17 exons with cDNA lengths ranging from 23 to 35 kb. Studies of the promoter region of the lactoferrin gene reveal that the basic promoter of the mouse Lf gene contains a non-canonical TATA box, GATAAA and AP1/CEBP element. Near the promoter are GC-rich sequences that can bind to Sp1, Sp3, and IKLF. Bioinformatics analysis showed that the transcription factor binding sites in −2000 bp promoter region of bLf are concentrated in the −1300 to −1800 and −200 to −600 regions. Interestingly, there are substantial differences in DNA sequences among different individuals in the human population (83). Teng et al. investigated restriction endonuclease polymorphisms of the hLf gene and established common SNPs of the lactoferrin gene in 91 healthy blood donors from different races (including European, American, African, and Asian). As a result, 60 SNPs are detected in the Lf gene (promoter, exons, and introns). In addition, Asians and Africans have a higher proportion of pure-hybrid variants in amino acids 11, 382 and 523 (84).
Lactoferrin is present in a variety of tissues and is specifically recognized by the cellular components in tissues. Overall, lactoferrin genes are complexly regulated in different tissues in multiple ways to achieve different biological activities. This is largely dependent on lactoferrin receptors (LfRs) on the surface of specific target cells, and LfRs attach to the cell membrane surface relying on glycophosphatidylinositol and bind to different target cells to exert their respective effects (85). In 1979, Cox and coworkers first identified the presence of Lf receptors (LfRs) in the human small intestine (86), and in addition, receptors at the mRNA level for transporter Lf were also expressed in brain, heart, and liver tissues (Table 2). The concentrations of Lf in the fallopian tubes, uterus, vagina, vas deferens or epididymis are much higher than that of serum, while the Lf concentrations in the ovaries, testes, seminal vesicles and prostate are comparable to that of serum (87). The Lf gene is classical estrogen target gene, and the regulation of its expression involves estrogen response elements (EREs), estrogen receptors (ERs), estrogen-related receptors (ERRs), retinoic acid receptors (RARs) and chicken ovalbumin upstream promoter transcription factor (Coup-TF) (88). Various in vitro in vivo experiments have demonstrated that estrus, menstruation, and pregnancy are associated with changes in the expression levels of the Lf gene. The concentration of Lf in breastmilk is related to the duration of lactation, with 5∼7 g/L of Lf in colostrum, then decreasing to 2∼3 g/L in mature milk (89). Using ultra performance liquid chromatography (UPLC), Cai et al. measured Lf levels in the breast milk of 248 lactating women from eight different regions in China, and the results showed that Lf levels gradually decreased from day 1 to day 30 (p < 0.01) and remained constant from day 31 to day 330 (90). Thus, Lf gene expression is also spatiotemporally specific.
The expression levels of Lf genes also vary considerably at the cellular level. Compared to normal cells, Lf expression is absent or reduced in leukemia and breast cancer cells (91). Through DNA sequencing of the human lactoferrin gene, Teng et al. found that this phenomenon is due to increased methylation of the CpG site of the lactoferrin gene and the presence of non-CpG methylation in tumor cells, i.e., an altered methylation pattern at the CpG site of the Lf gene in tumor cells (92). The regulation of Lf synthesis depends on the type of cells that produce Lf. The amount of Lf synthesized in the mammary gland is controlled by prolactin, while its synthesis in reproductive tissues is determined by estrogen. In addition, cell-derived miRNAs can also affect lactoferrin gene expression (93, 94). MiR-130a regulates C/EBP-ε protein expression in mouse and human granulocyte precursors, and overexpression downregulates C/EBP-ε and Lf (95). MiR-584 can upregulate lactoferrin receptor expression, and miR-214 directly targets Lf gene expression in cells (96, 97).
5. Discussion and prospect
Many studies have now shown that Lf is a reliable multifunctional protein with anti-inflammatory, antibacterial and antiviral effects. Because of its immune enhancing effects, it is also used in infant formulas. The immunomodulatory effect of Lf lies in its ability to modulate innate and adaptive immunity. One of the most interesting properties of Lf is that it can reach the nucleus as Lf possesses the ability to bind specifically to the LfR, which also gives Lf great potential to deliver macromolecules to the cytoplasm and nucleus, such as nucleic acids (siRNA, mRNA, shRNA, and DNA) (98). Lf is also used as a non-viral vector to deliver anti-cancer and anti-bacterial drugs for targeted therapeutic purposes. Currently researchers are investigating the role of Lf in post-operative infections such as organ transplants, prosthetic fittings, and skin grafts (99–102). Transgenic cows and goats are also being tried to produce recombinant hLf on a large-scale. Transgenic plants have improved antibacterial and disease resistance properties and can be used to the production of hypoallergenic lactoferrin. However, there is still a huge controversy about Genetically Modified (GM) foods, and the acceptance of GM foods has yet to be improved. In neurodegenerative diseases, Lf is specifically increased in saliva in patients with Alzheimer compared to Parkinson, dementia, and depression. So, we speculate that this may be related to a certain specific LfR. Lf also alters the tumor microenvironment and inhibits the metastasis of tumor cells. By evaluating effect of oral and intravenous (IV) administration of recombinant human Lf (rhLf) on blood cells, transcriptomic analysis showed that the majority of genes (72.8%) altered by oral rhLf are identical to IV treatment. Pathway mapping reveals a similar trend of upregulation of specific genes involved in oxidative stress and inflammatory responses in both treatment pathways, nevertheless the binding of Lf to cellular receptors and the mechanism of action of Lf in different diseases currently remain unknown. Furthermore, Lf also has different effects in diverse environments with various iron saturation, which is an area worth further exploring.
Author contributions
XC contributed to the original draft, investigation, data curation, and drawing. KW, YW, and YHW contributed to the investigation. YR, QL, YZ, and ZC revised the manuscript. X-SC, ZY, and ZC contributed to the funding acquisition and resources. All authors have read and agreed to the published version of the manuscript.
Funding
This work was supported by the Postgraduate Research and Practice Innovation Program of Jiangsu Province (KYCX22_3533), Independent Innovation in Jiangsu Province of China [CX(21)3119], and Yangzhou University “Blue Project,” and the Open Project Program of International Joint Research Laboratory in Universities of Jiangsu Province of China for Domestic Animal Germplasm Resources and Genetic Improvement.
Conflict of interest
The authors declare that the research was conducted in the absence of any commercial or financial relationships that could be construed as a potential conflict of interest.
Publisher’s note
All claims expressed in this article are solely those of the authors and do not necessarily represent those of their affiliated organizations, or those of the publisher, the editors and the reviewers. Any product that may be evaluated in this article, or claim that may be made by its manufacturer, is not guaranteed or endorsed by the publisher.
References
1. Johanson B. Isolation of an iron-containing red protein from human milk. Acta Chem Scand. (1960) 14:510–2.
2. Rümke P, Visser D, Kwa H, Hart A. Radio-immuno assay of lactoferrin in blood plasma of breast cancer patients, lactating and normal women; prevention of false high levels caused by leakage from neutrophile leucocytes in vitro. Folia Med Neerl. (1971) 14:156–68.
3. Mitra A, Zhang Z. Expression of a human lactoferrin cDNA in tobacco cells produces antibacterial protein(s). Plant Physiol. (1994) 106:977–81.
4. van Berkel P, Welling M, Geerts M, van Veen H, Ravensbergen B, Salaheddine M, et al. Large scale production of recombinant human lactoferrin in the milk of transgenic cows. Nat Biotechnol. (2002) 20:484–7. doi: 10.1038/nbt0502-484
5. Elzoghby A, Abdelmoneem M, Hassanin I, Abd Elwakil M, Elnaggar M, Mokhtar S, et al. Lactoferrin, a multi-functional glycoprotein: active therapeutic, drug nanocarrier & targeting ligand. Biomaterials. (2020) 263:120355. doi: 10.1016/j.biomaterials.2020.120355
6. Morgenthau A, Pogoutse A, Adamiak P, Moraes T, Schryvers A. Bacterial receptors for host transferrin and lactoferrin: molecular mechanisms and role in host-microbe interactions. Future Microbiol. (2013) 8:1575–85. doi: 10.2217/fmb.13.125
7. Kell D, Heyden E, Pretorius E. The biology of lactoferrin, an iron-binding protein that can help defend against viruses and bacteria. Front Immunol. (2020) 11:1221. doi: 10.3389/fimmu.2020.01221
8. Lin T, Meletharayil G, Kapoor R, Abbaspourrad A. Bioactives in bovine milk: chemistry, technology, and applications. Nutr Rev. (2021) 79(Suppl. 2):48–69. doi: 10.1093/nutrit/nuab099
9. Shende P, Khanolkar B. Human breast milk-based nutritherapy: a blueprint for pediatric healthcare. J Food Drug Anal. (2021) 29:203–13. doi: 10.38212/2224-6614.3352
10. Dierick M, Vanrompay D, Devriendt B, Cox E. Lactoferrin, a versatile natural antimicrobial glycoprotein that modulates the host’s innate immunity. Biochem Cell Biol. (2021) 99:61–5. doi: 10.1139/bcb-2020-0080
11. Albar A, Almehdar H, Uversky V, Redwan E. Structural heterogeneity and multifunctionality of lactoferrin. Curr Protein Peptide Sci. (2014) 15:778–97. doi: 10.2174/1389203715666140919124530
12. Teng C. Factors regulating lactoferrin gene expression. Biochem Cell Biol. (2006) 84:263–7. doi: 10.1139/o06-034
13. Habib H, Ibrahim S, Zaim A, Ibrahim W. The role of iron in the pathogenesis of COVID-19 and possible treatment with lactoferrin and other iron chelators. Biomed Pharmacother. (2021) 136:111228. doi: 10.1016/j.biopha.2021.111228
14. Xu S, Yang Q, Wang R, Tian C, Ji Y, Tan H, et al. Genetically engineered pH-responsive silk sericin nanospheres with efficient therapeutic effect on ulcerative colitis. Acta Biomater. (2022) 144:81–95. doi: 10.1016/j.actbio.2022.03.012
15. Li B, Zhang B, Liu X, Zheng Y, Han K, Liu H, et al. The effect of lactoferrin in aging: role and potential. Food Funct. (2022) 13:501–13. doi: 10.1039/d1fo02750f
16. Bazarnyi V, Sidenkova A, Sosnin D. Lactoferrin of oral fluid is normal and in Alzheimer’s disease: laboratory and diagnostic aspects (review of literature) [Лактоферрин ротовой жидкости в норме и при болезни Альцгеймера: лабораторно-диагностические аспекты(обзор литературы)]. Klin Lab Diagn. (2022) 67:207–12. doi: 10.51620/0869-2084-2022-67-4-207-212
17. Ostan N, Moraes T, Schryvers A. Lactoferrin receptors in Gram-negative bacteria: an evolutionary perspective. Biochem Cell Biol. (2021) 99:102–8. doi: 10.1139/bcb-2020-0079
18. Moreno-Expósito L, Illescas-Montes R, Melguizo-Rodríguez L, Ruiz C, Ramos-Torrecillas J, de Luna-Bertos E. Multifunctional capacity and therapeutic potential of lactoferrin. Life Sci. (2018) 195:61–4. doi: 10.1016/j.lfs.2018.01.002
19. Ramírez-Sánchez D, Arredondo-Beltrán I, Canizalez-Roman A, Flores-Villaseñor H, Nazmi K, Bolscher J, et al. Bovine lactoferrin and lactoferrin peptides affect endometrial and cervical cancer cell lines. Biochem Cell Biol. (2021) 99:149–58. doi: 10.1139/bcb-2020-0074
20. Song N, Luo J, Huang L, Tian H, Chen Y, He Q. miR-204-5p and miR-211 synergistically downregulate the α(S1)-casein content and contribute to the lower allergy of goat milk. J Agric Food Chem. (2021) 69:5353–62. doi: 10.1021/acs.jafc.1c01147
21. Baker H, Baker EN. A structural perspective on lactoferrin function. Biochem Cell Biol. (2012) 90:320–8. doi: 10.1139/o11-071
22. Singh J, Maurya A, Singh P, Viswanathan V, Ahmad M, Sharma P, et al. Peptide bond from the inter-lobe segment in the bilobal lactoferrin acts as a preferred site for cleavage for serine proteases to generate the perfect C-lobe: structure of the pepsin hydrolyzed lactoferrin C-lobe at 2.28 Å resolution. Protein J. (2021) 40:857–66. doi: 10.1007/s10930-021-10028-3
23. Wang X, Guo H, Zhang W, Wen P, Zhang H, Guo Z, et al. Effect of iron saturation level of lactoferrin on osteogenic activity in vitro and in vivo. J Dairy Sci. (2013) 96:33–9. doi: 10.3168/jds.2012-5692
24. Baker H, Baker E. Lactoferrin and iron: structural and dynamic aspects of binding and release. Biometals. (2004) 17:209–16. doi: 10.1023/b:biom.0000027694.40260.70
25. Rastogi N, Singh A, Singh P, Tyagi T, Pandey S, Shin K, et al. Structure of iron saturated C-lobe of bovine lactoferrin at pH 6.8 indicates a weakening of iron coordination. Proteins. (2016) 84:591–9. doi: 10.1002/prot.25004
26. Naidu S, Clemens R, Naidu A. SARS-CoV-2 infection dysregulates host iron (Fe)-redox homeostasis (Fe-R-H): role of fe-redox regulators, ferroptosis inhibitors, anticoagulants, and iron-chelators in COVID-19 control. J Diet Suppl. (2022) 1–60. doi: 10.1080/19390211.2022.2075072
27. Dierick M, Van der Weken H, Rybarczyk J, Vanrompay D, Devriendt B, Cox E. Porcine and bovine forms of lactoferrin inhibit growth of porcine enterotoxigenic Escherichia coli and degrade its virulence factors. Appl Environ Microbiol. (2020) 86:e00524-20. doi: 10.1128/aem.00524-20
28. Gruden Š, Poklar Ulrih N. Diverse mechanisms of antimicrobial activities of lactoferrins, lactoferricins, and other lactoferrin-derived peptides. Int J Mol Sci. (2021) 22:11264. doi: 10.3390/ijms222011264
29. Bullen J, Rogers H, Leigh L. Iron-binding proteins in milk and resistance to Escherichia coli infection in infants. Br Med J. (1972) 1:69–75. doi: 10.1136/bmj.1.5792.69
30. Arnold R, Russell J, Champion W, Gauthier J. Bactericidal activity of human lactoferrin: influence of physical conditions and metabolic state of the target microorganism. Infect Immun. (1981) 32:655–60. doi: 10.1128/iai.32.2.655-660.1981
31. Pino A, Giunta G, Randazzo C, Caruso S, Caggia C, Cianci A. Bacterial biota of women with bacterial vaginosis treated with lactoferrin: an open prospective randomized trial. Microb Ecol Health Dis. (2017) 28:1357417. doi: 10.1080/16512235.2017.1357417
32. Gallo V, Giansanti F, Arienzo A, Antonini G. Antiviral properties of whey proteins and their activity against SARS-CoV-2 infection. J Funct Foods. (2022) 89:104932. doi: 10.1016/j.jff.2022.104932
33. Mirabelli C, Wotring J, Zhang C, McCarty S, Fursmidt R, Pretto C, et al. Morphological cell profiling of SARS-CoV-2 infection identifies drug repurposing candidates for COVID-19. Proc Natl Acad Sci U.S.A. (2021) 118:e2105815118. doi: 10.1073/pnas.2105815118
34. Campione E, Lanna C, Cosio T, Rosa L, Conte M, Iacovelli F, et al. Lactoferrin against SARS-CoV-2: in vitro and in silico evidences. Front Pharmacol. (2021) 12:666600. doi: 10.3389/fphar.2021.666600
35. Jittavisutthikul S, Thanongsaksrikul J, Thueng-In K, Chulanetra M, Srimanote P, Seesuay W, et al. Humanized-VHH transbodies that inhibit HCV protease and replication. Viruses. (2015) 7:2030–56. doi: 10.3390/v7042030
36. Horie K, Watanabe M, Chanbora C, Awada T, Kunimatsu R, Uchida T, et al. Bovine lactoferrin reduces extra-territorial facial allodynia/hyperalgesia following a trigeminal nerve injury in the rat. Brain Res. (2017) 1669:89–96. doi: 10.1016/j.brainres.2017.04.015
37. Oda H, Wakabayashi H, Yamauchi K, Sato T, Xiao J, Abe F, et al. Isolation of a bifidogenic peptide from the pepsin hydrolysate of bovine lactoferrin. Appl Environ Microbiol. (2013) 79:1843–9. doi: 10.1128/aem.03343-12
38. Fan L, Yao Q, Wu H, Wen F, Wang J, Li H, et al. Protective effects of recombinant lactoferrin with different iron saturations on enteritis injury in young mice. J Dairy Sci. (2022) 105:4791–803. doi: 10.3168/jds.2021-21428
39. Piacentini R, Centi L, Miotto M, Milanetti E, Di Rienzo L, Pitea M, et al. Lactoferrin inhibition of the complex formation between ACE2 receptor and SARS CoV-2 recognition binding domain. Int J Mol Sci. (2022) 23:5436. doi: 10.3390/ijms23105436
40. Wotring J, Fursmidt R, Ward L, Sexton J. Evaluating the in vitro efficacy of bovine lactoferrin products against SARS-CoV-2 variants of concern. J Dairy Sci. (2022) 105:2791–802. doi: 10.3168/jds.2021-21247
41. Khan F, Anwar I, Redwan E, Palakkott A, Ashraf A, Kizhakkayil J, et al. Camel and bovine milk lactoferrins activate insulin receptor and its related AKT and ERK1/2 pathways. J Dairy Sci. (2022) 105:1848–61. doi: 10.3168/jds.2021-20934
42. Timmis A, Townsend N, Gale C, Torbica A, Lettino M, Petersen S, et al. European society of cardiology: cardiovascular disease statistics 2019. Eur Heart J. (2020) 41:12–85. doi: 10.1093/eurheartj/ehz859
43. Ruiz-Gimenez P, Ibanez A, Salom J, Marcos J, Lopez-Diez J, Valles S, et al. Antihypertensive properties of lactoferricin B-derived peptides. J Agric Food Chem. (2010) 58:6721–7. doi: 10.1021/jf100899u
44. Xu S, Fan F, Liu H, Cheng S, Tu M, Du M. Novel anticoagulant peptide from lactoferrin binding thrombin at the active site and exosite-I. J Agric Food Chem. (2020) 68:3132–9. doi: 10.1021/acs.jafc.9b08094
45. Shi P, Fan F, Chen H, Xu Z, Cheng S, Lu W, et al. A bovine lactoferrin-derived peptide induced osteogenesis via regulation of osteoblast proliferation and differentiation. J Dairy Sci. (2020) 103:3950–60. doi: 10.3168/jds.2019-17425
46. Guo H, Jiang L, Ibrahim S, Zhang L, Zhang H, Zhang M, et al. Orally administered lactoferrin preserves bone mass and microarchitecture in ovariectomized rats. J Nutr. (2009) 139:958–64. doi: 10.3945/jn.108.100586
47. Zhang W, Dong R, Diao S, Du J, Fan Z, Wang F. Differential long noncoding RNA/mRNA expression profiling and functional network analysis during osteogenic differentiation of human bone marrow mesenchymal stem cells. Stem Cell Res Ther. (2017) 8:30. doi: 10.1186/s13287-017-0485-6
48. Li Y, Guo C. A review on lactoferrin and central nervous system diseases. Cells. (2021) 10:1810. doi: 10.3390/cells10071810
49. Li H, Wang Y, Yang H, Liu L, Wang J, Zheng N. Lactoferrin induces the synthesis of vitamin B6 and protects HUVEC functions by activating PDXP and the PI3K/AKT/ERK1/2 pathway. Int J Mol Sci. (2019) 20:587. doi: 10.3390/ijms20030587
50. Sokolov A, Miliukhina I, Belsky Y, Belska N, Vasilyev V. Potential role of lactoferrin in early diagnostics and treatment of Parkinson disease [Потенциальная роль лактоферрина для ранней диагностики и лечения болезни Паркинсона]. Med Acad J. (2020) 20:37–44. doi: 10.17816/maj33848
51. Gothwal A, Nakhate K, Alexander A, Ajazuddin, Gupta U. Boosted memory and improved brain bioavailability of rivastigmine: targeting effort to the brain using covalently tethered lower generation PAMAM dendrimers with lactoferrin. Mol Pharm. (2018) 15:4538–49. doi: 10.1021/acs.molpharmaceut.8b00537
52. Mastromarino P, Capobianco D, Campagna G, Laforgia N, Drimaco P, Dileone A, et al. Correlation between lactoferrin and beneficial microbiota in breast milk and infant’s feces. Biometals. (2014) 27:1077–86. doi: 10.1007/s10534-014-9762-3
53. Lu J, Haley K, Francis J, Guevara M, Doster R, Craft K, et al. The innate immune glycoprotein lactoferrin represses the Helicobacter pylori cag type IV Secretion system. Chembiochem. (2021) 22:2783–90. doi: 10.1002/cbic.202100249
54. Mosli M, Zou G, Garg S, Feagan S, MacDonald J, Chande N, et al. C-reactive protein, fecal calprotectin, and stool lactoferrin for detection of endoscopic activity in symptomatic inflammatory bowel disease patients: a systematic review and meta-analysis. Am J Gastroenterol. (2015) 110:802–19; quiz 820. doi: 10.1038/ajg.2015
55. Iigo M, Alexander D, Xu J, Futakuchi M, Suzui M, Kozu T, et al. Inhibition of intestinal polyp growth by oral ingestion of bovine lactoferrin and immune cells in the large intestine. Biometals. (2014) 27:1017–29. doi: 10.1007/s10534-014-9747-2
56. Gao Y, Hou L, Lu C, Wang Q, Pan B, Wang Q, et al. Enteral lactoferrin supplementation for preventing sepsis and necrotizing enterocolitis in preterm infants: a meta-analysis with trial sequential analysis of randomized controlled trials. Front Pharmacol. (2020) 11:1186. doi: 10.3389/fphar.2020.01186
57. Razak A, Hussain A. Lactoferrin supplementation to prevent late-onset sepsis in preterm infants: a meta-analysis. Am J Perinatol. (2021) 38:283–90. doi: 10.1055/s-0039-1696676
58. Elfin trial investigators group. Enteral lactoferrin supplementation for very preterm infants: a randomised placebo-controlled trial. Lancet. (2019) 393:423–33. doi: 10.1016/s0140-6736(18)32221-9
59. Berrington J, McGuire W, Embleton ND. ELFIN, the United Kingdom preterm lactoferrin trial: interpretation and future questions (1). Biochem Cell Biol. (2021) 99:1–6. doi: 10.1139/bcb-2020-0073
60. Strunk T, Hibbert J, Doherty D, Nathan E, Simmer K, Patole S, et al. Lactoferrin expression is not associated with late-onset sepsis in very preterm infants. Neonatology. (2020) 117:606–11. doi: 10.1159/000509404
61. Jeong Y, Lee G, Yoo Y. Bovine lactoferricin induces intestinal epithelial cell activation through phosphorylation of FAK and paxillin and prevents rotavirus infection. J Microbiol Biotechnol. (2021) 31:1175–82. doi: 10.4014/jmb.2106.06044
62. Oda H, Kolawole A, Mirabelli C, Wakabayashi H, Tanaka M, Yamauchi K, et al. Antiviral effects of bovine lactoferrin on human norovirus. Biochem Cell Biol. (2021) 99:166–72. doi: 10.1139/bcb-2020-0035
63. Gibbons J, Kanwar R, Kanwar J. Lactoferrin and cancer in different cancer models. Front Biosci. (2011) 3:1080. doi: 10.2741/212
64. Chea C, Miyauchi M, Inubushi T, Febriyanti Ayuningtyas N, Subarnbhesaj A, Nguyen P, et al. Molecular mechanism of inhibitory effects of bovine lactoferrin on the growth of oral squamous cell carcinoma. PLoS One. (2018) 13:e0191683. doi: 10.1371/journal.pone.0191683
65. Li H, Yao Q, Min L, Huang S, Wu H, Yang H, et al. The combination of two bioactive constituents, lactoferrin and linolenic acid, inhibits mouse xenograft esophageal tumor growth by downregulating lithocholyltaurine and inhibiting the JAK2/STAT3-related pathway. ACS Omega. (2020) 5:20755–64. doi: 10.1021/acsomega.0c01132
66. Gajda-Morszewski P, Śpiewak-Wojtyła K, Oszajca M, Brindell M. Strategies for oral delivery of metal-saturated lactoferrin. Curr Protein Peptide Sci. (2019) 20:1046–51. doi: 10.2174/1389203720666190513085839
67. Ishikado A, Imanaka H, Takeuchi T, Harada E, Makino T. Liposomalization of lactoferrin enhanced it’s anti-inflammatory effects via oral administration. Biol Pharm Bull. (2005) 28:1717–21. doi: 10.1248/bpb.28.1717
68. López-Machado A, Díaz-Garrido N, Cano A, Espina M, Badia J, Baldomà L, et al. Development of lactoferrin-loaded liposomes for the management of dry eye disease and ocular inflammation. Pharmaceutics. (2021) 13:1698. doi: 10.3390/pharmaceutics13101698
69. Hu P, Zhao F, Wang J, Zhu W. Early-life lactoferrin intervention modulates the colonic microbiota, colonic microbial metabolites and intestinal function in suckling piglets. Appl Microbiol Biotechnol. (2020) 104:6185–97. doi: 10.1007/s00253-020-10675-z
70. Shao Y, Zhang X, Zhang H, Tian B, Weng Y, Huang J, et al. Effects of dietary supplementation of bovine lactoferricin on rumen microbiota, lactation, and health in dairy goats. Front Nutr. (2021) 8:722303. doi: 10.3389/fnut.2021.722303
71. Hashem N, El-Son M, Ateya A, Saleh R. Impact of lactoferrin supplementation on oxidative stress, gene expression and immunity dysfunction induced by Aeromonas veronii in Nile tilapia (Oreochromis niloticus). Aquac Res. (2022) 53:2392–407. doi: 10.1111/are.15757
73. Chanda B, Shamimuzzaman M, Gilliard A, Ling K. Effectiveness of disinfectants against the spread of tobamoviruses: tomato brown rugose fruit virus and Cucumber green mottle mosaic virus. Virol J. (2021) 18:7. doi: 10.1186/s12985-020-01479-8
74. Kobayashi J, Sasaki A, Watanabe A, Komine K, Kuroishi T, Takada N, et al. Effects of exogenous lactoferrin on characteristics and functions of bovine epididymal, ejaculated and frozen-thawed sperm. Anim Sci J. (2021) 92:e13538. doi: 10.1111/asj.13538
75. Martins H, da Silva G, Cortes S, Paes F, Martins Filho O, Araujo M, et al. Lactoferrin increases sperm membrane functionality of frozen equine semen. Reprod Domest Anim. (2018) 53:617–23. doi: 10.1111/rda.13148
76. Su J, Wang C, Song Y, Yang Y, Cao G. Effect of lactoferrin on ram sperm motility after cryopreservation. Anim Biosci. (2022) 35:1351–9. doi: 10.5713/ab.21.0561
77. Pearl C, Roser J. Expression of lactoferrin in the boar epididymis: effects of reduced estrogen. Domest Anim Endocrinol. (2008) 34:153–9. doi: 10.1016/j.domaniend.2007.01.001
78. Yang P, Wang J, Gong G, Sun X, Zhang R, Du Z, et al. Cattle mammary bioreactor generated by a novel procedure of transgenic cloning for large-scale production of functional human lactoferrin. PLoS One. (2008) 3:e3453. doi: 10.1371/journal.pone.0003453
79. Teng C. Regulation of lactoferrin gene expression by estrogen and epidermal growth factor - Molecular mechanism. Cell Biochem Biophys. (1999) 31:49–64. doi: 10.1007/bf02738154
80. Kim S, Yu D, Pak K, Jeong S, Kim S, Lee K. Structure of the human lactoferrin gene and its chromosomal localization. Mol Cells. (1998) 8:663–8.
81. Schwerin M, Solinas Toldo S, Eggen A, Brunner R, Seyfert H, Fries R. The bovine lactoferrin gene (LTF) maps to chromosome 22 and syntenic group U12. Mamm Genome. (1994) 5:486–9. doi: 10.1007/bf00369317
82. Innes B, Dorin J. Submucosal gland distribution in the mouse has a genetic determination localized on chromosome 9. Mamm Genome. (2001) 12:124–8. doi: 10.1007/s003350010244
83. Liu L, Gladwell W, Teng C. Detection of exon polymorphisms in the human lactoferrin gene. Biochem Cell Biol. (2002) 80:17–22. doi: 10.1139/o01-207
84. Teng C, Gladwell W. Single nucleotide polymorphisms (SNPs) in human lactoferrin gene. Biochem Cell Biol. (2006) 84:381–4. doi: 10.1139/o06-035
85. Suzuki Y, Shin K, Lonnerdal B. Molecular cloning and functional expression of a human intestinal lactoferrin receptor. Biochemistry. (2001) 40:15771–9. doi: 10.1021/bi0155899
86. Cox T, Mazurier J, Spik G, Montreuil J, Peters T. Iron binding proteins and influx of iron across the duodenal brush border. Evidence for specific lactotransferrin receptors in the human intestine. Biochim Biophys Acta. (1979) 588:120–8. doi: 10.1016/0304-4165(79)90377-5
87. Yu L, Chen Y. The developmental profile of lactoferrin in mouse epididymis. Biochem J. (1993) 296(Pt 1):107–11. doi: 10.1042/bj2960107
88. Lee M, Liu Y, Zhang XK. A retinoic acid response element that overlaps an estrogen response element mediates multihormonal sensitivity in transcriptional activation of the lactoferrin gene. Mol Cell Biol. (1995) 15:4194–207. doi: 10.1128/mcb.15.8.4194
89. Czosnykowska-Łukacka M, Orczyk-Pawiłowicz M, Broers B, Królak-Olejnik B. Lactoferrin in human milk of prolonged lactation. Nutrients. (2019) 11:2350. doi: 10.3390/nu11102350
90. Cai X, Duan Y, Li Y, Wang J, Mao Y, Yang Z, et al. Lactoferrin level in breast milk: a study of 248 samples from eight regions in China. Food Funct. (2018) 9:4216–22. doi: 10.1039/c7fo01559c
91. Panella T, Liu Y, Huang A, Teng C. Polymorphism and altered methylation of the lactoferrin gene in normal leukocytes, leukemic cells, and breast cancer. Cancer Res. (1991) 51:3037–43.
92. Teng C, Gladwell W, Raphiou I, Liu E. Methylation and expression of the lactoferrin gene in human tissues and cancer cells. Biometals. (2004) 17:317–23. doi: 10.1023/B:BIOM.0000027711.13818.8a
93. Zhou J, Ji X, Wang Y, Wang X, Mao Y, Yang Z. Long intergenic noncoding RNAs differentially expressed in Staphylococcus aureus-induced inflammation in bovine mammary epithelial cells. Biocell. (2021) 45:1033–44. doi: 10.32604/biocell.2021.015586
94. Serwas N, Huemer J, Dieckmann R, Mejstrikova E, Garncarz W, Litzman J, et al. CEBPE-mutant specific granule deficiency correlates with aberrant granule organization and substantial proteome alterations in neutrophils. Front Immunol. (2018) 9:588. doi: 10.3389/fimmu.2018.00588
95. Larsen M, Häger M, Glenthøj A, Asmar F, Clemmensen S, Mora-Jensen H, et al. miRNA-130a regulates C/EBP-ε expression during granulopoiesis. Blood. (2014) 123:1079–89. doi: 10.1182/blood-2013-08-523233
96. Liao Y, Lonnerdal B. miR-584 mediates post-transcriptional expression of lactoferrin receptor in Caco-2 cells and in mouse small intestine during the perinatal period. Int J Biochem Cell Biol. (2010) 42:1363–9. doi: 10.1016/j.biocel.2009.07.019
97. Liao Y, Du X, Lonnerdal B. miR-214 Regulates lactoferrin expression and pro-apoptotic function in mammary epithelial cells. J Nutr. (2010) 140:1552–6. doi: 10.3945/jn.110.124289
98. Bukowska-Ośko I, Sulejczak D, Kaczyńska K, Kleczkowska P, Kramkowski K, Popiel M, et al. Lactoferrin as a human genome “guardian”-an overall point of view. Int J Mol Sci. (2022) 23. doi: 10.3390/ijms23095248
99. Koca C, Yıldırım B, Ozmen O, Dikilitas A, Cicek M, Simsek A, et al. Effect of single-dose locally applied lactoferrin on autograft healing in peri-implant bone in rat models. Injury. (2022) 53:858–67. doi: 10.1016/j.injury.2021.11.065
100. Pugh C, Hathwar V, Richards J, Stonehuerner J, Ghio A. Disruption of iron homeostasis in the lungs of transplant patients. J Heart Lung Transpl. (2005) 24:1821–7. doi: 10.1016/j.healun.2005.03.016
101. Chua M, Barry C, Chen X, Salvatierra O, Sarwal M. Molecular profiling of anemia in acute renal allograft rejection using DNA microarrays. Am J Transpl. (2003) 3:17–22. doi: 10.1034/j.1600-6143.2003.30104.x
102. Martinez L, Casadevall A. Specific antibody can prevent fungal biofilm formation and this effect correlates with protective efficacy. Infect Immun. (2005) 73:6350–62. doi: 10.1128/iai.73.10.6350-6362.2005
103. Superti F. Lactoferrin from bovine milk: a protective companion for life. Nutrients. (2020) 12:2562. doi: 10.3390/nu12092562
104. Cheng H, Chen X, Huan L. cDNA cloning and sequence analysis of human lactoferrin. Chin J Biotechnol. (2001) 17:385–7.
105. Barber M, Kronenberg Z, Yandell M, Elde N. Antimicrobial functions of lactoferrin promote genetic conflicts in ancient primates and modern humans. PLoS Genet. (2016) 12:e1006063. doi: 10.1371/journal.pgen.1006063
106. Goodman R, Schanbacher F. Bovine lactoferrin mRNA: sequence, analysis, and expression in the mammary gland. Biochem Biophys Res Commun. (1991) 180:75–84. doi: 10.1016/s0006-291x(05)81257-4
107. Liu Y, Teng C. Characterization of estrogen-responsive mouse lactoferrin promoter. J Biol Chem. (1991) 266:21880–5.
108. Wang Y, Han F, Xu Z. Developmental gene expression of lactoferrin in duodenum and effect of weaning age on gene expression of lactoferrin in piglets. Arch Anim Nutr. (2006) 60:1–9. doi: 10.1080/17450390500468255
109. Lydon J, O’Malley B, Saucedo O, Lee T, Headon D, Conneely O. Nucleotide and primary amino acid sequence of porcine lactoferrin. Biochim Biophys Acta. (1992) 1132:97–9. doi: 10.1016/0167-4781(92)90061-4
110. Kang J, Li X, Zheng H, Zhou R, Li L, Zhao H. Sequence analysis of the lactoferrin gene and variation of g.7605C→T in 10 Chinese indigenous goat breeds. Biochem Genet. (2011) 49:63–72. doi: 10.1007/s10528-010-9385-5
111. Le Provost F, Nocart M, Guerin G, Martin P. Characterization of the goat lactoferrin cDNA: assignment of the relevant locus to bovine U12 synteny group. Biochem Biophys Res Commun. (1994) 203:1324–32. doi: 10.1006/bbrc.1994.2327
112. Pereira S, Rhodes J, Campbell B, Kumar D, Bain I, Murphy G, et al. Biliary lactoferrin concentrations are increased in active inflammatory bowel disease: a factor in the pathogenesis of primary sclerosing cholangitis? Clin Sci. (1998) 95:637–44. doi: 10.1042/cs0950637
113. Du Y, Li D, Chen J, Li Y, Zhang Z, Hidayat K, et al. Lactoferrin improves hepatic insulin resistance and pancreatic dysfunction in high-fat diet and streptozotocin-induced diabetic mice. Nutr Res. (2022) 103:47–58. doi: 10.1016/j.nutres.2022.03.011
114. Sherman M. Lactoferrin and necrotizing enterocolitis. Clin Perinatol. (2013) 40:79–91. doi: 10.1016/j.clp.2012.12.006
115. Gill K, Horsley H, Swamy S, Khasriya R, Malone-Lee J. A prospective observational study of urinary cytokines and inflammatory response in patients with overactive bladder syndrome. BMC Urol. (2021) 21:39. doi: 10.1186/s12894-021-00809-4
116. Markus C, Olivier B, Panhuysen G, Van Der Gugten J, Alles M, Tuiten A, et al. The bovine protein alpha-lactalbumin increases the plasma ratio of tryptophan to the other large neutral amino acids, and in vulnerable subjects raises brain serotonin activity, reduces cortisol concentration, and improves mood under stress. Am J Clin Nutr. (2000) 71:1536–44. doi: 10.1093/ajcn/71.6.1536
Keywords: lactoferrin, antimicrobial, anticancer, immunoregulation, expression regulation
Citation: Cao X, Ren Y, Lu Q, Wang K, Wu Y, Wang Y, Zhang Y, Cui X, Yang Z and Chen Z (2023) Lactoferrin: A glycoprotein that plays an active role in human health. Front. Nutr. 9:1018336. doi: 10.3389/fnut.2022.1018336
Received: 13 August 2022; Accepted: 21 November 2022;
Published: 05 January 2023.
Edited by:
Ken Ng, University of Melbourne, AustraliaReviewed by:
Wang Bing, Tianjin Medical University, ChinaGiovanni Antonini, Roma Tre University, Italy
Copyright © 2023 Cao, Ren, Lu, Wang, Wu, Wang, Zhang, Cui, Yang and Chen. This is an open-access article distributed under the terms of the Creative Commons Attribution License (CC BY). The use, distribution or reproduction in other forums is permitted, provided the original author(s) and the copyright owner(s) are credited and that the original publication in this journal is cited, in accordance with accepted academic practice. No use, distribution or reproduction is permitted which does not comply with these terms.
*Correspondence: Zhi Chen, ✉ emhpY2hlbkB5enUuZWR1LmNu