- 1Development Center for Medical Science and Technology, National Health Commission of the People's Republic of China, Beijing, China
- 2Advanced Medical Research Institute, Shandong University, Jinan, Shandong, China
- 3College of Veterinary Medicine, Qingdao Agricultural University, Qingdao, China
With the increasing global incidence of infertility, the influence of environmental factors, lifestyle habits, and nutrients on reproductive health has gradually attracted the attention of researchers. The quantity and quality of sperm play vital roles in male fertility, and both characteristics can be affected by external and internal factors. In this review, the potential role of genetic, environmental, and endocrine factors; nutrients and trace elements in male reproductive health, spermatozoa function, and fertility potency and the underlying mechanisms are considered to provide a theoretical basis for clinical treatment of infertility.
Introduction
Infertility is a disease with psychological, economic, and medical consequences that can lead to trauma and stress. According to the World Health Organization and the International Committee for Monitoring Assisted Reproductive Technology, infertility is a reproductive system disease defined as the failure to achieve clinical pregnancy after 12 months or more of routine unprotected sex (1). An estimated 45% of infertility cases involve only men while 20% involve both women and men (2). Male infertility is mainly diagnosed via semen analysis, including determination of the sperm concentration/total number, motility, and morphology (3). It is characterized by several features, such as oligospermia, asthenozoospermia, and teratozoospermia. Several factors have been reported to cause male infertility, including genetic, environmental, and endocrine factors (4).
Genetic abnormalities can lead to spermatogenic disorders and account for 15–30% of male infertility (2, 5). Environmental factors, such as exposure to heavy metals, affect male sperm production and fertility. The oxidative stress induced by exposure to heavy metals is thought to affect male sperm production. Endocrine disruptor chemicals (EDCs) alter endocrine system processes and adversely affect the organism itself or its offspring (6). Semen quality is the major index of male fertility and is determined on the basis of various factors; semen quality and quantity vary according to age, disease, sexual activity, and diet. Environmental factors can affect semen quality by impairing spermatogenesis, steroid production, and sperm function, resulting in a decline in male fertility (7). Trace elements play a significant role in sexual health, spermatogenesis, sperm maturation, and capacitation. Human semen contains many trace elements, such as iron, zinc, copper, strontium, selenium, molybdenum, manganese, lead, arsenic, cadmium, vanadium, and cobalt. These elements are essential for normal spermatogenesis and sperm movement, maturation, and capacitation (8). Zinc, manganese, selenium, and copper have antioxidant effects and reduce lipid peroxidation (9). Magnesium and calcium maintain the osmotic balance of the male reproductive system and contribute to nutrient transport and gland function. The lack of some trace elements can also perturb reproductive function and sperm quality, which can negatively impact spermatogenesis, sperm quality, and male fertility (10).
Chemical elements play important roles in male reproduction because imbalance of micronutrients or macronutrients may lead to spermatogenesis defects and decreased libido, resulting in male infertility. Dietary and feed supplements can improve the male reproductive performance and help eliminate infertility. This review provides up-to-date information to better understand the positive and negative effects of selected macronutrients and micronutrients on male fertility.
To our knowledge, this is the first comprehensive review on the effects of nutrients on male infertility. We present the positive and negative effects of macronutrients and micronutrients; genetic, environmental, and endocrine factors; and trace elements on male spermatogenesis and fertility. We also discuss the conclusions and future perspectives on male infertility.
Factors influencing spermatogenesis impairment and male infertility
Spermatogenesis is a complex differentiation process in which cells transform from spermatogonia to mature sperm through many stages and is a key event in male fertility. It is regulated by a myriad of factors that are involved in the development of subfertility. Several genetic abnormalities have been associated with impaired spermatogenesis. Abnormalities in chromosome structure and number have been found in 4% of patients with azoospermia or oligozoospermia, as an abnormal chromosome structure leads to abnormal meiosis and spermatogenesis failure (11). Around 4,000 genes have been reported to participate in human spermatogenesis. Genetic studies have revealed that mutations in the X chromosome and autosomal genes impair spermatogenesis, which has been reported in various animal models, including Saccharomyces cerevisiae, Caenorhabditis elegans, Drosophila (fruit fly), and Mus musculus (mouse) (12).
Sperm dysfunction is the most common cause of infertility in men. Male infertility is diagnosed on the basis of the presence of “oligozoospermia” (reduced sperm count), “asthenospermia” (decreased sperm motility), and “abnormal sperm” (sperm with an abnormal morphology). Oxidative stress leads to sperm dysfunction (13). The disorder related to the balance between oxidants and antioxidants may have a strong toxic effect on spermatogenesis through the production of excessive oxidants and male infertility (14). In humans, male infertility is closely associated with an increase in the exposure to heavy metals that affect semen and a decrease in the antioxidant content. It also results from increased lipid peroxidation induced by reactive oxygen species (ROS higher total plasma peroxidase activity) and the consequent antioxidant depression (lower total antioxidant capacity) observed in these individuals (13). In a previous study, high concentrations of iron and cadmium increased oxidative stress in the seminal plasma and sperm homogenate of the infertile group, decreased the content of antioxidants, and thus had a strong toxic effect on spermatogenesis by producing excessive oxidants and inducing apoptosis (14).
Genetic factors
Genetic factors play an important role in the most serious spermatogenic disorders, such as severe oligozoospermia or azoospermia (15). They cause ~15% of male infertility cases. Karyotype analysis has revealed that genetic factors control spermatogenesis. Chromosomal abnormalities, including deletion, inversion, mutation, aneuploidy, and translocation, are associated with male infertility, and many male infertility-related diseases are governed by various factors, such as chromosomal or monogenic diseases, mitochondrial DNA mutations, Y chromosome deletions, polygenic diseases, imprinted diseases, or hereditary endocrine diseases (16). Epidemiological evidence suggests that male infertility as a precursor for increased risk of diabetes, cardiovascular diseases, and all-cause mortality. Furthermore, studies have shown that abnormal semen parameters may also be linked to non-malignant diseases such as diabetes and ischaemic heart disease.
In infertile men, the incidence of chromosomal abnormalities has been reported to range from 5 to 15%, indicating that these abnormalities lead to non-obstructive azoospermia (NOA) (17). NOA affects about 1% of men in the general population and is characterized by clinical heterogeneity implying the involvement of several different acquired and genetic factors. Epidemiological evidence shows a link between azoospermia and higher morbidity and mortality rate, suggesting a common etiology for NOA and some chronic diseases, including cancer. Macroscopic deletions of the long arm of the Y chromosome can induce azoospermia. In a previous study, the rate of Y chromosome microdeletions in male patients with infertility averaged 8.2%, and the vast majority (84.3%) were associated with azoospermia (18). The azoospermia factor (AZF) region contains three different regions AZFa (792 kb), AZFb (6.2 Mb), and AZFc (4.5 Mb) which contain several genes responsible for male fertility (19). de Llanos et al. (20) reported that there is a partial deletion of 1.6 Mb on the Y chromosome, termed the “gr/gr” deletion, eventually leading to spermatogenic failure (20). Cytogenetic techniques have revealed the link between chromosomal abnormalities and male infertility owing to the presence of an extra X chromosome (47, XXY), which is characteristic of Klinefelter syndrome, and the X chromosome contains multiple genes and plays a significant role in the prophase of meiosis during mammalian spermatogenesis (21). Microdeletions affecting the AZF regions lead to phenotypic changes in infertile men, ranging from oligozoospermia to azoospermia in 2–10% of cases (22). Deletion of the AZFc region containing eight gene families causes progressive deterioration of spermatogenesis and, consequently, azoospermia eventually leads to phenotypic changes in infertile men (19, 22).
Spermatogenesis is thought to be regulated by as many as 2,000 genes, of which 600–900 genes seem to be expressed only in the male reproductive line. Approximately 10% of the genes in the genome are associated with spermatogenesis; some mutations in these genes may cause complete inhibition of spermatogenesis (5). There are many genetic factors related to male infertility, including single-gene and polygene defects. Tüttelmann et al. (23) reported that pathogenic mutations in male infertility genes, such as NR5A1, DMRT1, and TEX11, cause azoospermia in men (23).
Qualitative defects in spermatogenesis are characterized by various parameters, including sperm morphology, motility, and DNA and chromatin integrity, and by various conditions, including oligozoospermia, asthenozoospermia, teratozoospermia, and oligoasthenoteratozoospermia (OAT). Multiple morphological abnormalities of the sperm flagella (MMAF) include weakly malformed spermatozoa characterized by flagellum loss, shortness, curvature, curl, irregularity, and others. They are a mosaic with abnormal morphology and ultrastructural flagellar defects, such as central pair deletion, fibrous sheath dysplasia, double microtubule tissue disorder, or dynamic arm deletion. This suggests that the MMAF phenotype is genetically heterogeneous. Recently, several multiple MMAF pathogenic genes have been identified in humans (24, 25).
Endocrine factors
The endocrine system maintains the balance of the entire body through hormones. Testosterone and sperm are important products of testicular synthesis. Testosterone is necessary for the development and maintenance of several physiological functions. Testicular function depends on the regulation of hormones secreted by the hypothalamus, pituitary gland, and local endocrine and paracrine pathways. Spermatogenesis involves the synergistic action of endocrine hormones, many paracrine and growth factors, closely coordinated gene and protein expression programs, and genomes and epigenetics of different non-coding RNA species (26). Luteinising hormone (LH) and follicle-stimulating hormone (FSH) are the key endocrine factors controlling testicular function and spermatogenesis and are the main hormone regulators of spermatogenesis (27). In particular, LH stimulates high concentrations of testosterone (ITT) in the testis, which is essential for spermatogenesis. Germ cells are in close contact with Sertoli cells at all stages of spermatogenesis and require structural and functional support from Sertoli cells. Unlike germ cells, these somatic cells express sex steroids and FSH receptors, which are the most important hormones that regulate spermatogenesis. The increase in FSH signaling may be sufficient to initiate spermatogenesis by activating Sertoli cell function (28).
An imbalance in serum hormone levels causes disturbances in spermatogenesis and male infertility. For example, prolactin (PRL) is a polypeptide hormone, which is primarily secreted by lactotropic cells of the pituitary, which controls the production of LH and FSH via the regulation of gonadotropin-releasing hormone (GnRH) through feedback mechanism on the hypothalamus (29, 30). PRL is involved in various types of functions including lactation, osmoregulation, immune articulation and reproduction (31). FSH balance is regulated by the pituitary. If the sperm-producing potential of the testis decreases, then the pituitary starts producing more FSH to revive normal testicular function (32). Conversely, higher levels of FSH lead to azoospermia and severe-oligozoospermia, which are hallmarks for inactive or damaged seminiferous tubules (33). Studies suggest that increased serum PRL levels are associated with infertility, impotence, and hypogonadism (34, 35). A considerable amount of evidence suggests that several animal and human studies have shown that PRL positively modulates various aspects in testicular function hinting at a crucial role in male reproduction. PRL release is regulated by a wide variety of factors secreted from the hypothalamic pituitary gonadal (HPG) axis (29, 30). PLR receptors (PLR) appear to regulate of sertoli cell (36). High PRL levels lead to hypogonadism and male infertility (37). FSH, LH, thyroid stimulating hormone (TSH), and chorionic gonadotropin (hCG) are known to perform important functions such as Sertoli cell development. Exogenous application of testosterone reduces intra-testicular testosterone and spermatogenesis with consequent oligospermia and sometimes azoospermia (38, 39). Excessive levels of exogenous androgen cause temporary spermatogenic dysfunction, thus aggravating infertility (40). Exogenous testosterone is known for its contraceptive effects in men. Exogenous administration of androgens influences the HPG axis with negative feedback that may lead to a partial or complete cessation of spermatogenesis by decreasing FSH and LH (41). Exogenous testosterone ameliorates hypogonadism in males; conversely, it reduces sperm production and count. An et al. (42) reported that the effect of exogenous testosterone supplementation on spermatogenesis in a rat model of oligoasthenospermia (42). The rats were randomized equally into four groups: control, model, low dose, and high dose. After injection of testosterone undecanoate (TU), body weights, serum reproductive hormone levels, sperm measurements in the epididymis, and testis histology were monitored. The TU injections increased serum testosterone levels steadily. In addition, exogenous testosterone supplemental therapy (TST) increased the intra-testicular testosterone concentration modestly and alleviated the testicular oxidative stress markers. There was a significant improvement in sperm and testicular function due to decreased mitochondrial apoptosis in the testis by modulation of Bcl-2, Bax, caspase-3, and caspase-9 expression. Despite the positive effects of testosterone on sexual function, it has a negative effect on fertility. Exogenous testosterone therapy can negatively affect the HPG axis and inhibit the production of FSH and LH. Therefore, the usage of testosterone remains controversial (43).
Environmental factors and pollutants
Generally, humans are exposed to various hazardous chemicals including carcinogens; toxins; reproductive toxins; irritants; corrosives; neurotoxins; hepatotoxins; and chemicals that damage the lungs, skin, eyes, or mucous membranes. These hazardous chemicals enter human bodies through inhalation, ingestion, injection, and/or absorption through the skin and eyes. The toxic substances potentially damage biological systems, disturbing the functioning of biochemical processes and resulting in detrimental and even fatal effects. Owing to rapid urbanization and the development of chemical industries, numerous xenobiotics have been released into the environment. Among the various systems in the human body, the male reproductive system is highly sensitive to the environmental factors that contribute to infertility (44). The released chemicals mimic estrogen and EDCs and are possible factors responsible for male infertility. Environmental factors, such as living in pollution-enriched urban areas, influence sperm morphological defects and DNA fragmentation and increase the incidence of pear-shaped, slender, round heads; acrosome abnormalities; vacuolar chromatin; asymmetric neck; and ERC (45). Environmental pollutants, such as non-essential heavy metals, lead, cadmium, arsenic, mercury, and barium, can have harmful effects on semen and sperm quality.
EDCs can alter endocrine system processes and adversely affect the organism itself or its offspring. They have oestrogenic, anti-oestrogenic, androgen, or anti-androgen effects, can disrupt the hormonal system, and cause male reproductive dysfunction through a variety of mechanisms (46). Exposure to increasing EDC concentrations leads to decreased semen quality, which increases the incidence of male infertility and impairs male reproductive health (47). Bisphenol A (BPA) is a monomer used to make a wide variety of polycarbonate plastics and resins, which has oestrogenic activity and binds to the α-estrogen receptor (ER-α) and, to a lesser extent, the β-estrogen receptor (ER-β). It reduces serum FSH, LH, and testosterone concentrations. BPA increases the risk of cryptorchidism and azoospermia, decreases semen quality, increases the risk of testicular cancer, and reduces paternal fertility rates (48). Further, a mouse study revealed that BPA exposure leads to a decrease in normal sperm morphology and motility. Men exposed to dioxins exhibit high concentrations of morphologically abnormal sperm and low linear motility. Exposure to polychlorinated biphenyls and phthalates decreases sperm count and motility and alters normal morphology (49). Furthermore, exposure to pesticides alters normal sperm morphology and reduces sperm count, volume, and motility (49). Pesticides affect many organs, including the reproductive system, and related processes and parameters, including spermatogenesis, sperm density, testicular weight, motility, sperm count, sperm survival rate, and other sperm parameters. In particular, they induce sperm DNA damage and abnormal sperm morphology. Pesticides affect the male reproductive system by reducing the testis weight and impairing the function of the seminal vesicles, epididymis, and ventral prostate, leading to degeneration of the seminiferous tubules and changes in the concentrations of hormones, such as testosterone, FSH, and LH. They also affect the antioxidant enzymes in the testes and inhibit testicular steroid production.
Heavy metals refer to any metal chemical elements with a high density and that are toxic at low concentrations. These include mercury, arsenic, cadmium, chromium, lead, and thallium. Heavy metals have bioaccumulation properties, can enter the water supply system through industrial and domestic wastes, and even destroy the soil. They are released through acid rain into streams, rivers, lakes, and groundwater. Different environmental and occupational factors can affect male reproductive function. The adverse effects of exposure to heavy metals on the male reproductive system may be attributed to the disruption of the hypothalamic–pituitary axis or a direct influence on spermatogenesis, resulting in the deterioration of semen quality. There is a correlation between exposure to heavy metals and reduced semen quality. Moderate or high concentrations of lead can reduce sperm count, density, and motility, resulting in fertility problems in humans (50). Exposure of men to high concentrations of cadmium in seminal plasma (65 μg/dL) results in decreased sperm count and activity. Increasing concentrations of mercury in the blood (40.6 mmol/L) lead to harmful effects on sperm and cause a progressive motility rate of < 50% and a normal morphology rate of < 14% (51).
Heavy metal exposure is a potentially harmful factor in male sperm production and fertility (6). Heavy metals have toxic effects on reproductive processes and hormonal regulation. They are endocrine disruptors that interfere with the regulation of endogenous hormones and negatively impact endocrine function (52). The concentrations of heavy metals can be measured in the serum, semen, seminal plasma, and hair, but especially in the blood or urine (52). Among the various types of heavy metals, cadmium, arsenic, and lead are considered to be the major toxins affecting reproductive function.
Heavy metals cause toxicity by inducing oxidative stress, inflammation, endocrine disruption, and signaling pathway disruption (53). Cadmium, mercury, and lead are associated with Sertoli cell dysfunction. Toxicants affecting interstitial cells can lead to abnormal testosterone secretion and seminiferous epithelium reduction, resulting in impaired Sertoli cell function and spermatogenic dysfunction. The main cause of spermatogenesis failure is a change in the adhesion between germ and stem cells (54). Toxins interact with the secretion of LH and FSH, both of which play an important role in sperm quality.
Lead and cadmium are endocrine disruptors that can change male hormone concentrations, leading to impaired semen quality and male infertility (55). Copper sulfate and cadmium chloride significantly reduce sperm motility parameters (56). A high semen viscosity is an important factor leading to poor sperm quality. Sperm motility is impaired; zinc uptake is insufficient, the trace element concentrations change, the semen quality is poor, the leukocyte count increases, and ROS production increases. Oxidative stress leads to imbalances in seminal plasma antioxidants, which is the main mechanism of a high semen viscosity, resulting in sperm DNA damage, sperm chromatin instability, membrane lipid peroxidation, and a low fertilization rate (Figure 1) (58).
Heavy metals found in seminal plasma can also increase the risk of poor sperm survival and abnormal sperm morphology (teratozoospermia) and ultimately affect male fertility by inducing ROS production, resulting in lipid peroxidation and sperm DNA damage (59). Oxidative stress plays an important role in human reproduction. Excessive ROS levels induce deleterious effects on human spermatozoa and damage the plasma membrane of spermatozoa, eventually leading to sperm dysfunction. ROS have potential significance in reproductive biology and play important physiological and pathological roles in male infertility (60). The physiological level of ROS is crucial for sperm maturation, capacitation, acrosome reaction, and fertilization. In fertile and infertile groups, hydrogen peroxide has harmful, dose-dependent effects on sperm DNA integrity and function. ROS regulate sperm chromatin condensation by controlling the number of germ cells and by inducing sperm apoptosis or proliferation (61). They are also involved in the capacitation, acrosome reaction, mitochondrial stabilization, and motility of mature sperm. ROS modify the integrity of DNA in the nucleus of 15 variants by inducing DNA strand breaks, base modification, and chromatin cross-linking (62). They are essential for reproductive function; however, increasing levels of ROS also have a detrimental effect on fertility.
Effects of nutrients on male spermatogenesis and sperm quality
Effect of diet on male spermatogenesis and sperm quality
Nutrients and lifestyle habits play significant roles in reproductive processes/conditions, particularly in male infertility. A hypercaloric diet and foods with low nutritional density have been associated with poorer semen parameters and lower fertility rates (Figure 2). Studies have shown that increased intake of trans-omega-6 fatty acids and decreased intake of omega-4 fatty acids are related to deterioration of testicular endocrine function (63). Intake of saturated fat can lead to decreased sperm density and semen count. For example, caffeinated sweet drinks are associated with reduced semen volume, sperm count, and sperm density (64). Improper diet and obesity can lead to lower semen quality and increase the risk of infertility. The intestinal microbiota plays a significant role in human health. However, an imbalance in the microbiota can lead to various complications, such as immunodeficiencies and a predisposition to metabolic diseases (65). Intake of large amounts of fat and monosaccharides may lead to intestinal biological disorders, resulting in increased intestinal barrier permeability and ultimately poor sperm quality and impaired spermatogenesis (66).
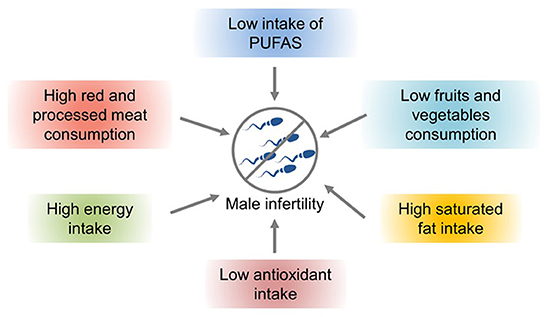
Figure 2. The effects of nutrients and lifestyle factors on male infertility (57).
Lifestyle habits, such as smoking and drinking, can also reduce sperm quality. Studies have shown that obesity and a high-fat diet can lead to impaired reproductive ability, affecting the molecular sperm structure, fetal development, and offspring health (57). High-energy diets, such those with high amounts of trans fat and saturated fat, may affect fat metabolism in the testes, impair spermatogenesis, and alter sperm quality (67). Increased ROS levels have a harmful effect on sperm DNA, resulting in the formation of 8-oxydeoxyguanosine. Excessive consumption of high-calorie foods rich in fatty acids and trans fat leads to the accumulation of fatty acids and liposoluble toxins in the testicular environment, affecting spermatogenesis and testosterone production in Leydig cells (57). Owing to the lack of gonadotropin support, accumulation of a large amount of leptin leads to spermatogenesis disorders and infertility and consequently to a decline in sperm quality (57). A high-cholesterol diet may cause male infertility and reduce semen quality by destroying the blood–testicular barrier (67). The effects of diet and obesity on spermatogenesis are shown in Figure 3.
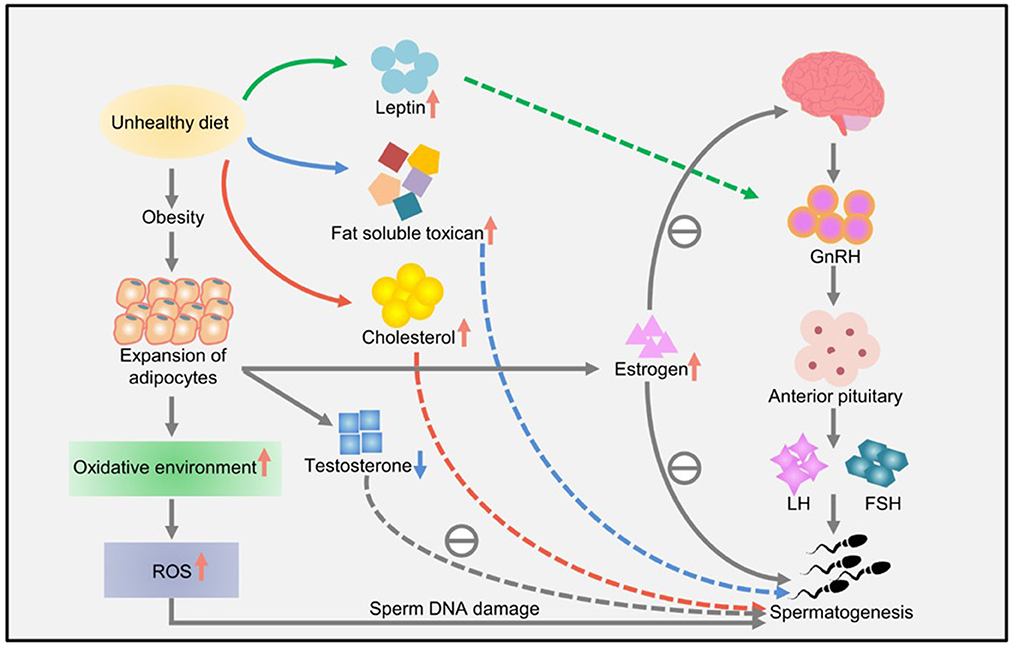
Figure 3. The effects of diet and obesity on spermatogenesis (57).
Effect of lifestyle on male spermatogenesis and sperm quality
At present, lifestyle-related factors such as smoking, alcohol consumption, and use of mobile phones and computers are playing a significant role in male infertility. Studies have shown that sperm quality is essentially determined by obesity, nicotine addiction, heavy exposure to electromagnetic radiation emitting devices and alcohol consumption (68). The immense usage of mobile phones and other devises as a source of low-level radio-frequency electromagnetic fields (RF-EMF) causes poor semen quality. In particular, low-level RF-EMFexerts non-thermal and thermal effects on sperm quality. The non-thermal effect is believed to increase the production of reactive oxygen species, resulting in DNA damage (69, 70). The thermal effect causes testicular dysfunction because the device is carried in trouser pockets near external male reproductive organs; it might augment sperm DNA fragmentation (71). Studies have demonstrated that exposure of human spermatozoa to a laptop connected to the internet via Wi-Fi significantly decreases progressive semen motility and increases semen DNA fragmentation (72). Impaired sperm motility might be the results of EMF induced oxidation of phospholipids and high seminal ROS levels (73, 74). An in vivo study indicated that long lasting exposure to 4G LTE based EMF had deleterious effects on rat spermatogenesis (75).
Among all Word Health Organization regions, ~ 37% of men of reproductive age use tobacco (76). Cigarette smoke contains several hazardous substances, all of which exert harmful effects on male germ cells. Smoking cigarettes has been correlated with reduced semen quality, with impaired motility, decreased concentration, and abnormal morphology (77). Sepaniak et al. reported that there is a direct correlation between cigarette smoking and abnormal sperm chromatin (78). Aboulmaouahib et al. reported significant increases in the DNA fragmentation index and spermatic chromatin decondensation in smokers as well as smokers who consume alcohol (79). Further, a study provided evidence that long-time cigarette smoking leads to increased testosterone metabolism in the liver, concurrently causing secretory dysfunction of Leydig and Sertoli cells (80).
Alcohol consumption is the vital factor that decreases semen quality. Researchers have found a significant correlation between alcohol intake, semen volume, and sperm morphology and motility (81, 82). Another study found more pronounced hormonal changes in infertile patients who drink daily compared with the group of occasional drinkers (83). Alcohol consumption shows a significant impact on semen quality in male offspring and induces DNA damage in male germ cells (84).
Effect of micronutrients on male spermatogenesis and sperm quality
Micronutrients are essential for proper bodily function; therefore, they are necessary in daily food intake. However, excess levels of micronutrients lead to harmful effects. Vitamins are organic micronutrients and play essential roles in the human body (85). Vitamin C is an electron donor vitamin capable of reducing metals and regenerating vitamin E from its oxidized form. Vitamin C prevents agglutination and protects against DNA damage caused by ROS in sperm cells (86). Vitamin E can protect cell membranes and prevents lipid peroxidation (87). Vitamin E has multiple functions in male fertility, such as testosterone biosynthesis and modulation of telomerase activity. An in vivo study demonstrated that in rats subjected to noise-generated stress and exposed to nicotine, vitamin E ameliorates the loss of sperm viability and increases fertilization rates (88, 89). Vitamin B9 is a water soluble compound and essential for DNA metabolism; it also protects DNA from mutations and strand breaks (90, 91). A study revealed that supplementing 5 mg/day of folic acid increases the sperm concentration and the normal sperm count (92).
Trace elements ameliorate impaired male spermatogenesis and reduction in sperm quality
Human semen contains calcium, magnesium, zinc, selenium, copper, manganese, and other trace elements. These substances are essential for sexual health; spermatogenesis; and sperm maturation, motility, capacitation, and function. Deficiency in these trace elements negatively influences spermatogenesis and is responsible for poor sperm quality and low fertility rates in men (8).
Several micronutrients play significant roles in spermatogenesis. Sperm quality is regulated by various nutrients, including zinc, folate, selenium, and vitamins (93). Among these micronutrients, zinc plays multiple roles in the male reproductive system. It is considered a vital trace element for healthy growth, good neurological system function, and normal immune system responses (94).
Zinc
Zinc is an essential micronutrient involved in the electron transfer reactions of a variety of enzymes in the human body (95). It participates in the anatomical development and normal function of the male reproductive system and promotes spermatogenesis by actively participating in the maturation of sperm and the preservation of the germinating epithelium. Low zinc concentrations can delay testicular development and lead to cessation of spermatogenesis. Zinc deficiency leads to a decrease in sperm count; this condition works only at the testicular level, leading to spermatogenic disorders and gonadal dysfunction, such as reduced testicular weight and seminiferous tubule atrophy (96). Zinc supplementation has a protective effect against lead damage, leading to degenerative changes in mature sperm. Zinc is a regulator of semen enzyme activity; it helps stabilize the chromatin and cell membranes of sperm and improves normal flagella, mid-segment formation, and sperm motility (97). Zinc and selenium deficiency in the blood has been linked to poor sperm quality. Huang et al. reported that zinc and selenium counteract cadmium-induced damage, including poor sperm quality and low sperm count (98). Zinc is an important nutrient and essential mineral in human semen. It is necessary for testicular development, normal testicular function, and epididymal and prostate function (99). For example, fertile individuals have significantly higher concentrations of zinc than infertile individuals (8). Zinc deficiency impairs spermatogenesis and lead to abnormal sperm quality (100). It reduces sperm quality through a variety of mechanisms, such as sex hormone deficiency, DNA damage, oxidative stress, apoptosis, spermatogenesis disturbance, and zinc finger protein deficiency. Meanwhile, zinc deficiency leads to male infertility through a variety of mechanisms, including testicular retardation, spermatogenesis disorders, sex hormone deficiency, oxidative stress, inflammation, and cell apoptosis. Furthermore, delayed sexual maturation, hypogonadism, testicular weight loss, Leydig cell damage, testicular atrophy, and seminiferous tubule damage can also occur (101). Zinc plays a significant role in the biosynthesis, storage, and secretion of male sex hormones, such as testosterone; its deficiency induces Leydig cell damage or apoptosis, which is associated with testosterone biosynthesis defects and spermatogenesis failure. Steroidogenesis can also be impaired, including decreased testosterone and progesterone production and increased LH and FSH production (102). Zinc is an important factor affecting sperm formation and regulates DNA replication, transcription, and packaging, as well as protein biosynthesis, cell differentiation, and proliferation. Deficiency in this element can lead to poor sperm quality and male infertility by increasing oxidative stress (8). High levels of oxidative stress, especially lipid peroxidation, have negative effects on spermatogenesis, sperm membrane fluidity, sperm capacitation, the sperm–egg interaction, and sperm transport during female reproduction and fertilization (103). These results indicate that zinc plays an important role in spermatogenesis and development.
Folate is an essential micronutrient required for germ cell development. The antioxidant properties of folate enhance sperm production and count and improve male sterility by inhibiting apoptosis and protecting against DNA damage (104). Vitamin E plays an important role in the survival of the male reproductive organs and sperm cells. It promotes the development of the reproductive organs by increasing epididymal weight, epididymal duct and seminiferous tubule diameter, and spermatogenic cell count and density, which are essential for the smooth progression of spermatogenesis. Vitamin E deficiency can lead to testicular abnormalities, spermatogenesis disorders, epididymal epithelial cell differentiation, incomplete spermatogenesis, and sperm cell degeneration (105). Vitamin C intake is closely associated with sperm concentration, count, and motility. A vitamin-free diet reduces testicular weight, male sex hormone production, and sperm quality (106).
Calcium
Calcium acts as an intracellular messenger and participates in a variety of cellular functions, including sperm motility, hyperactivation, capacitation, the acrosome reaction, and chemotaxis in the female reproductive tract (107). Calcium deficiency impairs sperm motility: it is significantly lower in affected men than in healthy men. Further, calcium regulates spermatogenesis as well as the growth, differentiation, and proliferation of spermatogonia and spermatocytes (108). The seminal plasma calcium concentration is lower in infertile men with oligospermia, azoospermia, or oligoasthenospermia than in healthy men. Furthermore, in the presence of calcium, ATP facilitates flagellar movement and sperm motility (109). Beigi Harchegani et al. reported that calcium deficiency leads to male infertility by activating various mechanisms, such as spermatogenesis failure, impaired steroidogenesis, chemotactic failure, and impaired sperm motility, capacitation, and acrosome reaction (110). Calcium is involved in the induction of steroidogenesis and regulation of high testosterone production in Leydig cells. Collectively, these findings suggest that seminal plasma calcium is associated with male fertility and that lower concentrations lead to male infertility.
Sodium and potassium
Sodium and potassium play significant roles in normal sperm function, semen production, and sperm capacitation. They are directly associated with spermatozoa motility and fertilization capacity. Low concentrations of sodium and potassium cause infertility; this is usually observed under normozoospermic, oligozoospermic, oligoasthenozoospermic, asthenozoospermic, and azoospermic conditions (111). A study suggested that male mice lacking sodium/potassium-ATPase were unable to fertilize eggs in vitro (112). Sodium and potassium regulate sperm membrane potential and fertilizing ability and play essential roles in sperm capacitation and testosterone production (111). Deficiency in these elements can lead to spermatogenesis disorders and fertility problems and abnormal progesterone concentrations and acrosome reactions in human sperm, respectively (111). Taken together, these data indicate the importance of sodium and potassium in sperm activity.
Magnesium
Magnesium is an important trace element and activator of many enzymes in the phosphorus transfer reaction; it is involved in spermatogenesis and affects sperm motility (113). Lower concentrations of magnesium are associated with increased nitric oxide production and vasoconstriction, which may lead to premature ejaculation (114). Generally, infertile patients have lower concentrations of magnesium than fertile individuals. This element is directly associated with calcium and plays a significant role in semen transport, sperm motility, and male fertility (114). The energy required for sperm motility is released by magnesium-dependent ATPase (112). Collectively, low magnesium concentrations in seminal plasma play a significant role in infertility.
Selenium
Selenium is an important element for maintaining male fertility. Selenium deficiency leads to increased oxidative stress and has a negative effect on spermatogenesis (115). This element regulates spermatogenesis and is mainly mediated by two selenoproteins, which provide an important link with sperm quality and male fertility (115). It significantly activates the secretion of testosterone and consequently induces spermatogenesis, and its concentrations are closely related to the quantity, vitality, and normal morphology of sperm. Morbat et al. reported that fertile men have higher selenium concentration than in infertile men (116). Lower selenium concentrations are associated with poor sperm quality and an increased risk of male infertility. Selenium has known antioxidant and beneficial effects: it protects sperm against oxidative stress induced DNA damage, which consequently enhances sperm motility and viability (117). Moreover, selenium supplementation significantly improves male fertility. In a previous study, selenium supplementation enhanced the concentrations of magnesium, FSH, testosterone, and glutathione and significantly decreased the concentrations of pro-oxidants, such as malondialdehyde. Scott et al. reported that selenium supplementation at a dose of 100 μg/day for 3 months significantly increased sperm motility in infertile men (118). These findings suggest that selenium potentially influences sperm quality and motility and male fertility.
Manganese
Manganese plays a significant role in human reproductive function. It protects against formaldehyde-induced sperm parameter and testis structure damages in mice (119). Manganese supplementation can maintain thiol concentrations by reducing oxidative stress in human sperm and can enhance the quality and motility of sperm by activating adenylate cyclase activity (120). Decreased manganese concentrations in seminal plasma have been reported to produce adverse effects on semen volume and normal sperm morphology. Guiying reported that high manganese concentrations are associated with erectile dysfunction (121). Another study of infertile men showed that high manganese concentrations in seminal plasma are significantly associated with an increased risk of decreased sperm motility and count (122). High concentrations in seminal plasma are related to increased sperm survival rate and progressive motility rate and abnormal percentage of sperm count (123). These findings suggest that manganese is an essential element and that low concentrations regulate normal function of the human reproductive organs, sperm motility, and fertilization, whereas high concentrations adversely affect human sperm.
Manganese is an essential nutrient for normal human development and activates genes responsible for the production of GnRH (124). Rotter et al. found that the testosterone concentration is negatively correlated with the free androgen index and that an elevated manganese concentration is inversely associated with testosterone production (125). Exposure to manganese may affect the function of the male reproductive system, resulting in increased spontaneous abortion rates, estrogenic hyperactivity, impotence, and infertility among the wives of workers (126). Wang et al. found that long-term exposure to low concentrations of manganese decreased the concentrations of serum PRL, LH, and TSH in 41 welders (127). High serum manganese concentrations in female workers yielded a variety of effects, including increased activity of DNA oxidation products, increased incidence and variability of chromosome polymorphisms characterized by somatic genetic instability, and sex hormone imbalance (128). The manganese exposure level ranged from 0.56 to 34.25 mg/L and the average concentration was 15.92 ± 8.49 mg/L among 84 male workers and 92 referents. GnRH and LH concentrations increased significantly, while the testosterone concentration decreased significantly in the manganese-exposed group. This finding supports the significant positive correlation between urinary manganese and GnRH and LH concentrations and the significant negative correlation between urinary manganese and TSTO concentrations. Ultimately, the manganese-exposed group showed decreased sperm progressive motility and total motility.
Copper
Copper is an essential element for many metalloenzymes involved in energy or antioxidant metabolism and protects sperm cells from oxidative damage. However, its function depends on the plasma concentration. High copper concentrations are harmful to spermatozoa function, sperm quality, sperm motility, and mitochondrial activity (129). One study found that the seminal plasma copper concentration had a positive effect on sperm parameters, including volume and motility (130). The main function of this element is to participate in sperm motility and to act on the hypothalamus-derived progonadoliberin-1 to control the release of LH. High copper concentrations (≥100 μg/mL) are harmful to sperm motility and morphology and DNA (131).
Strontium
Strontium is a silvery metal that naturally occurs as a non-radioactive element. It is found in rocks, soil, dust, coal, and oil. About 99% of the strontium in the human body is concentrated in the bones. Strontium can exist in two oxidation states, namely 0 and +2. The isotopic form of strontium is used as a tracer in geological processes and as an indicator of provenance in archaeological contexts. Strontium has several biomedical applications, including in medicine and dietary supplements. Strontium plays an important role in reproductive health, and exposure to high concentrations is associated with low serum testosterone concentrations and an increased risk of primary male infertility (132). Studies have shown that strontium can improve animal and human sperm motility, capacitation, and the acrosome reaction (133). Clinical studies have shown that the addition of strontium to culture media can activate oocytes, thus improving fertilization and clinical pregnancy rates. Miao et al. proposed that the concentration of strontium in urine was positively correlated with sperm quality (134).
Molybdenum
Molybdenum is an essential trace mineral in animals and humans and is part of more than 50 active enzymes. It is found in foods such as milk, cheese, cereal grains, legumes, nuts, leafy vegetables, and organ meats. Molybdenum is most commonly used for molybdenum deficiency.
Molybdenum occurs in the lithosphere at an average abundance of 1.2 mg/kg and represents one of the scarcest trace elements in biological systems and it exist predominantly in the form of the oxyanion molybdate, which serves as an essential micronutrient in all kingdoms of life. A study showed that moderate doses (25 mg/L) of molybdenum can modulate the epididymal index, sperm motility, and sperm count, whereas high doses (≥ 100 mg/L) can modulate testicular oxidative stress in a complex manner, causing negative effects on sperm (135). It promotes the normal function of cells by catalyzing hydroxylation, oxygen atom transfer, and other redox reactions (136). In a previous study, researchers investigated the effects of different concentrations of molybdenum on sperm parameters and testicular oxidative stress in adult ICR mice. A medium dose of molybdenum (25 mg/L) enhanced sperm parameters (epididymal index, sperm motility, sperm number, and morphology), whereas a high dose (≥ 100 mg/L) had no significant effect on such parameters (137).
Magnesium, calcium, copper, and manganese play significant roles in spermatogenesis and sperm motility. Calcium affects the motility, overactivation, surrender, and acrosome reaction of sperm, resulting in sperm penetration of oocytes. Copper is related to the normal function of sperm, whereas manganese affects sperm motility and fertilization (138). Urinary concentrations of molybdenum are directly proportional to the sperm concentration and total count. Researchers have demonstrated the potential importance of molybdenum in improving human semen quality. While exposure to cadmium, lead, and mercury adversely affects semen quality (139). However, exposure to certain essential elements is beneficial for semen quality because these elements are involved in maintaining normal spermatogenesis and sperm maturation. As a rich alkaline earth element, non-radioactive strontium is abundant in soil and water, as well as in cereals, vegetables, seafood, and other foods.
Effect of nanoparticles/nanometals on spermatogenesis and male infertility
Metal nanoparticles (NPs), also called nanometals, are small particles with a relatively large surface area and a diameter between 1 and 100 nm. Due to recent advancements in nanoscience and nanotechnology, there has been great interest in metal NP applications, including in the area of reproductive biology. For example, cerium oxide (CeO2) NPs, which are able to store oxygen, act as ROS scavengers and protect sperm cell viability during cooling, and supplementting semen extender with CeO2 NPs improves sperm motility and velocity (140). Subcutaneous administration of titanium dioxide (TiO2) NPs at a dose of 400 μg to pregnant mice reduces the number of Sertoli cells, alters testicular morphology, disrupt seminiferous tubules, and significantly decreases daily sperm production (DSP) (141).
Zinc nano-complexes improved the functionality of sperm plasma membranes in a dose dependent manner and increase sperm count and improve sperm characteristics by protecting against oxidative stress (142, 143). Conversely, NPs of silver, TiO2, zinc oxide (ZnO), copper, and nickel induced oxidative stress in male reproductive organs (144–147). Olugbodi et al. reported that sub-dermal administration of AgNPs into male rats for 7 and 28 days produced histological abnormalities in the testis and alter the number of sperm, sperm motility, and testosterone, LH and FSH levels (148). A study found that intragastric exposure to TiO2 NPs induced immunological dysfunction in the mouse testis (149). Oral administration of AgNPs to Wistar rats reduced the number of sperm (150, 151). Exposure to carbon black NPs impaired the reproductive system of male offspring, including a reduction in the number of sperm in F1 males (152, 153). Oral administration of ZnO NPs to male mice significantly and dose-dependently reduced the body weight and increased the relative testicular weight (154). An in vivo study using mice intra peritoneally injected with iron oxide NPs caused histopathological changes such as sloughing, detachment of germ cells, and vacuolisation in seminiferous tubules of the testes (155). Similarly, exposure of adult male albino rats to TiO2 NPs significantly increased the thickness of interstitial spaces, induced blood vessels congestions, and promoted detachment of the germinal epithelium from the basement membrane in the seminiferous tubules (156). Treatment of with both aluminum oxide (Al2O3) NPs and ZnO NPs severely damaged seminiferous tubules and the basement membrane (157). ZnO NPs administered to albino rats induced histological abnormalities including disorganization, vacuolation, and detachment of germ cells in testicular tissues (158). Intragastric administration of TiO2 NPs at doses of 10, 50 and 100 mg/kg body weight induced reproductive toxicity in male mice, increased sperm malformation, and decreased the number of germ cells. Another study found that exposure of TiO2 (159). Xia et al. reported that curcumin functionalised NPs affected the proliferation of testicular cell lines and also causing an acute injury to the testicular functions in male mice (160).
Other causes of infertility in men
Infertility can also caused by other issued, including problems with testicular sperm production, no sperm production, blockage or absence of the duct to transfer sperm from the testes, varicocele, hormonal imbalance, lack of sperm mobility or function, previous injuries, health factors, and ejaculation disorders.
Conclusions and future perspectives
Male reproduction and fertility are necessary for the production of mature, healthy sperm. Proper nutrition is essential for spermatogenesis, good sperm motility and quality, and the development of Sertoli and interstitial cells. Some nutrients are involved in capacitation, hyperactivation, and the acrosome reaction. Trace elements are essential for sperm production and quality and could affect male fertility in distinct ways. Abnormal or insufficient concentrations of certain elements can cause issues during human reproduction, embryogenesis, and pregnancy. Human semen contains various micronutrients that are necessary for normal spermatogenesis and sperm maturation, motility, and capacitation. Reduced concentrations of trace elements can potentially affect sperm quality and cause infertility. Increased or decreased concentrations of trace elements can lead to sperm parameters that are thought to be the main cause of idiopathic male infertility. Environmental factors also play an important role in male fertility. Adverse environmental factors can lead to poor semen quality, an increase in the sperm DNA fragment index, and mitochondrial dysfunction, all of which can lead to male infertility. Increased EDC concentrations cause male reproductive disorders and infertility, eventually leading to a decline in male reproductive health.
However, the harmful effects of excessive trace elements are still not completely understood. Further, the molecular mechanisms underlying the positive and negative effects of many chemical and trace elements involved in male fertility are not clear. Future research should focus on identifying the key concentrations and mechanisms of these elements in relation to promoting or stopping sperm production and motility. It is necessary to investigate the complex relationships between chemical elements, enzymatic and non-enzymatic mechanisms, and other proteins and/or biomolecules. Determination of the total seminal concentration of minerals in fertile and infertile men is necessary to determine the sperm fertilization potential. In addition, it is important to determine the standards and optimal dietary nutrient concentrations required to achieve the best testicular growth, spermatogenesis, and semen quality outcomes. Additional studies on the sufficient amount of trace minerals needed for mammalian fertility could provide a solid foundation to explore the best combination of chemical elements and appropriate doses for the nutritional prevention or treatment of male infertility are needed. Future research should also focus on large consortia of well-funded organizations, including research institutes in different regions of the world. This infrastructure could allow researchers to play unbiased genomics methods and the relevant functional verification analyses on large patient cohorts. Birth cohort studies are also important to address the effects of maternal EDC exposure on semen quality and reproductive hormone concentrations.
Author contributions
X-FZ and SG designed the study and proof read this manuscript. H-HC, YZ, and P-YD prepared this review. All authors contributed to the article and approved the submitted version.
Funding
This work was supported by the High-Level Talents Research Fund Project of Qingdao Agricultural University in China (1120043).
Conflict of interest
The authors declare that the research was conducted in the absence of any commercial or financial relationships that could be construed as a potential conflict of interest.
Publisher's note
All claims expressed in this article are solely those of the authors and do not necessarily represent those of their affiliated organizations, or those of the publisher, the editors and the reviewers. Any product that may be evaluated in this article, or claim that may be made by its manufacturer, is not guaranteed or endorsed by the publisher.
References
1. Zegers-Hochschild F, Adamson GD, de Mouzon J, Ishihara O, Mansour R, Nygren K, et al. The international committee for monitoring assisted reproductive technology (ICMART) and the world health organization (WHO) revised glossary on ART terminology, 2009. Hum Reprod. (2009) 24:2683–7. doi: 10.1093/humrep/dep343
2. Leaver RB. Male infertility: an overview of causes and treatment options. Br J Nurs. (2016) 25:S35–40. doi: 10.12968/bjon.2016.25.18.S35
3. Wang C, Swerdloff RS. Limitations of semen analysis as a test of male fertility and anticipated needs from newer tests. Fertil Steril. (2014) 102:1502–7. doi: 10.1016/j.fertnstert.2014.10.021
4. Babakhanzadeh E, Nazari M, Ghasemifar S, Khodadadian A. Some of the factors involved in male infertility: A prospective review. Int J Gen Med. (2020) 13:29–41. doi: 10.2147/IJGM.S241099
5. Yoshida A, Miura K, Shirai M. Cytogenetic survey of 1,007 infertile males. Urol Int. (1997) 58:166–76. doi: 10.1159/000282975
6. Ilieva DM, Argirova M, Angelova LY, Gradinaru RV, Drochioiu G, Surleva AR. Application of chemical and biological tests for estimation of current state of a tailing dump and surrounding soil from the region of Tarnita, Suceava, Romania. Environ Sci Pollut Res Int. (2020) 27:1386–96. doi: 10.1007/s,11356-019-06919-9
7. Selvaraju V, Baskaran S, Agarwal A, Henkel R. Environmental contaminants and male infertility: effects and mechanisms. Andrologia. (2021) 53:e13646. doi: 10.1111/and.13646
8. Colagar AH, Marzony ET, Chaichi MJ. Zinc levels in seminal plasma are associated with sperm quality in fertile and infertile men. Nutr Res. (2009) 29:82–8. doi: 10.1016/j.nutres.2008.11.007
9. Tvrda E, Luka N, Lukanova J, Kazicka Z, Massanyi P. Stimulating and protective effects of vitamin E on bovine spermatozoa. J Microbiol Biotechnol Food Sci. (2013) 2:1386–95.
10. Marzec-Wroblewska U, Kaminski P, Lakota P. Influence of chemical elements on mammalian spermatozoa. Folia Biol. (2012) 58:7–15.
11. Chandley AC. The chromosomal basis of human infertility. Br Med Bull. (1979) 35:181–6. doi: 10.1093/oxfordjournals.bmb.a071567
12. Hackstein JH, Hochstenbach R, Pearson PL. Towards an understanding of the genetics of human male infertility: lessons from flies. Trends Genet. (2000) 16:565–72. doi: 10.1016/S0168-9525(00)02140-5
13. Agarwal A, Virk G, Ong C, du Plessis SS. Effect of oxidative stress on male reproduction. World J Mens Health. (2014) 32:1–17. doi: 10.5534/wjmh.2014.32.1.1
14. Raghda H, Sara AW, Amany MG, Samar SA. Infliximab abrogates cadmium-induced testicular damage and spermiotoxicity via enhancement of steroidogenesis and suppression of inflammation and apoptosis mediators. Ecotoxicol Environ Saf. (2019) 182. doi: 10.1016/j.ecoenv.2019.109398
15. Krausz C, Riera-Escamilla A. Genetics of male infertility. Nat Rev Urol. (2018) 15:369–84. doi: 10.1038/s41585-018-0003-3
16. Shamsi MB, Kumar K, Dada R. Genetic and epigenetic factors: role in male infertility. Indian J Urol. (2011) 27:110–20. doi: 10.4103/0970-1591.78436
17. Vincent JL, Sakr Y, Sprung CL, Ranieri VM, Reinhart K, Gerlach H, et al. Sepsis in European intensive care units: results of the SOAP study. Crit Care Med. (2006) 34:344–53. doi: 10.1097/01.CCM.0000194725.48928.3A
18. Sharma A. Male infertility; evidences, risk factors, causes, diagnosis and management in human. Ann Clin Lab Res. 3:188 (2017). doi: 10.21767/2386-5180.1000188
19. Deng CY, Zhang Z, Tang WH, Jiang H. Microdeletions and vertical transmission of the Y-chromosome azoospermia factor region. Asian J Androl. (2022). doi: 10.4103/aja2021130. [Epub ahead of print].
20. de Llanos M, Ballesca JL, Gazquez C, Margarit E, Oliva R. High frequency of gr/gr chromosome Y deletions in consecutive oligospermic ICSI candidates. Hum Reprod. (2005) 20:216–20. doi: 10.1093/humrep/deh582
21. Jacobs PA, Strong JA. A case of human intersexuality having a possible XXY sex-determining mechanism. Nature. (1959) 183:302–3. doi: 10.1038/183302a0
22. Krausz C, Hoefsloot L, Simoni M, Tuttelman, F. EAA/EMQN best practice guidelines for molecular diagnosis of Y-chromosomal microdeletions: state-of-the-art 2013. Andrology. (2014) 2:5–19.
23. Tuttelmann F, Ruckert C, Ropke A. Disorders of spermatogenesis: perspectives for novel genetic diagnostics after 20 years of unchanged routine. Med Genet. (2018) 30:12–20. doi: 10.1007/s11825-018-0181-7
24. Gao Y, Tian S, Sha Y, Zha X, Cheng H, Wang A, et al. Novel bi-allelic variants in DNAH2 cause severe asthenoteratozoospermia with multiple morphological abnormalities of the flagella. Reprod Biomed Online. (2021) 42:963–72. doi: 10.1016/j.rbmo.2021.01.011
25. Shen Y, Zhang F, Li F, Jiang X, Yang Y, Li X, et al. Loss-of-function mutations in QRICH2 cause male infertility with multiple morphological abnormalities of the sperm flagella. Nat Commun. (2019) 10:433. doi: 10.1038/s41467-018-08182-x
26. Holt JE, Stanger SJ, Nixon B, McLaughlin EA. Non-coding RNA in spermatogenesis and epididymal maturation. Adv Exp Med Biol. (2016) 886:95–120. doi: 10.1007/978-94-017-7417-8_6
27. Oduwole OO, Peltoketo H, Huhtaniemi IT. Role of follicle-stimulating hormone in spermatogenesis. Front Endocrinol. (2018) 9:763. doi: 10.3389/fendo.2018.00763
28. Santi D, Crepieux P, Reiter E, Spaggiari G, Brigante G, Casarini L, et al. Follicle-stimulating hormone (FSH) action on spermatogenesis: a focus on physiological and therapeutic roles. J Clin Med. (2020) 9:1014. doi: 10.3390/jcm9041014
29. Bole-Feysot C, Goffin V, Edery M, Binart N, Kelly PA. Prolactin (PRL) and its receptor: actions, signal transduction pathways and phenotypes observed in PRL receptor knockout mice. Endocr Rev. (1998) 19:225–68. doi: 10.1210/edrv.19.3.0334
30. Freeman ME, Kanyicska B, Lerant A, Nagy G. Prolactin: structure, function, and regulation of secretion. Physiol Rev. (2000) 80:1523–631. doi: 10.1152/physrev.2000.80.4.1523
31. Guillaumot P, Benahmed M. Prolactin receptors are expressed and hormonally regulated in rat Sertoli cells. Mol Cell Endocrinol. (1999) 149:163–8. doi: 10.1016/S0303-7207(98)00246-9
32. Luboshitzky R, Shen-Orr Z, Nave R, Lavi S, Lavie P. Melatonin administration alters semen quality in healthy men. J Androl. (2002) 23:572–8. doi: 10.1002/j.1939-4640.2002.tb02281.x
33. Bergmann M, Behre HM, Nieschlag E. Serum FSH and testicular morphology in male infertility. Clin Endocrinol. (1994) 40:133–6. doi: 10.1111/j.1365-2265.1994.tb02455.x
34. Gonzales GF. Functional structure and ultrastructure of seminal vesicles. Arch Androl. (1989) 22:1–13. doi: 10.3109/01485018908986745
35. Segal S, Yaffe H, Laufer N, Ben-David M. Male hyperprolactinemia:effects on fertility. Fertil Steril. (1979) 32:556–61. doi: 10.1016/S0015-0282(16)44359-1
36. Guillaumot P, Tabone E, Benahmed M. Sertoli cells as potential targets of prolactin action in the testis. Mol Cell Endocrinol. (1996) 122:199–206. doi: 10.1016/0303-7207(96)03891-9
37. Scarabelli L, Caviglia D, Bottazzi C, Palmero S. Prolactin effect on pre-pubertal Sertoli cell proliferation and metabolism. J Endocrinol Invest. (2003) 26:718–22. doi: 10.1007/BF03347352
38. Kliesch S. [Testosterone and infertility]. Urologe A. (2010) 49:32–6. doi: 10.1007/s00120-009-2195-x
39. Moss JL, Crosnoe LE, Kim ED. Effect of rejuvenation hormones on spermatogenesis. Fertil Steril. (2013) 99:1814–20. doi: 10.1016/j.fertnstert.2013.04.003
40. Dean A, Sharpe RM. Clinical review: anogenital distance or digit length ratio as measures of fetal androgen exposure: relationship to male reproductive development and its disorders. J Clin Endocrinol Metab. (2013) 98:2230–8. doi: 10.1210/jc.2012-4057
41. Fusco F, Verze P, Capece M, Napolitano L. Suppression of spermatogenesis by exogenous testosterone. Curr Pharm Des. (2021) 27:2750–3. doi: 10.2174/1381612826666201207104340
42. An Q, Zhang K, Fu L, Guo Y, Zhang C, Ge Z, et al. The impact of exogenous testosterone supplementation on spermatogenesis in a rat model of oligoasthenospermia. Int J Clin Exp Pathol. (2020) 13:1287–99.
43. Knuth UA, Nieschlag E. Endocrine approaches to male fertility control. Baillieres Clin Endocrinol Metab. (1987) 1:113–31. doi: 10.1016/S0950-351X(87)80055-1
44. Singh V, Pakhiddey R. Current scenario on genetic basis of infertility-a review. Acta Medica Int. (2015) 2:149. doi: 10.5530/ami.2015.4.7
45. Kleshchev M, Osadchuk A, Osadchuk L. Impaired semen quality, an increase of sperm morphological defects and DNA fragmentation associated with environmental pollution in urban population of young men from Western Siberia, Russia. PLoS ONE. (2021) 16:e0258900. doi: 10.1371/journal.pone.0258900
46. De Coster S, van Larebeke N. Endocrine-disrupting chemicals: associated disorders and mechanisms of action. J Environ Public Health. (2012) 2012:713696. doi: 10.1155/2012/713696
47. Abdelouahab N, Ainmelk Y, Takser L. Polybrominated diphenyl ethers and sperm quality. Reprod Toxicol. (2011) 31:546–50. doi: 10.1016/j.reprotox.2011.02.005
48. Helena EV, Jorma T. Cryptorchidism and fertility. Endocrinol Metab Clin North Am. (2015) 44:751–60. doi: 10.1016/j.ecl.2015.07.013
49. Natalie HAH, Gloria WS, Maria SB, Paulina F, Christiaan DJ. Impaired semen quality associated with environmental DDT exposure in young men living in a malaria area in the Limpopo Province, South Africa. J Androl. (2007) 28:423–34. doi: 10.2164/jandrol.106.001701
50. Telisman S, Cvitković P, Jurasović J, Pizent A, Gavella M, Rocić B. Semen quality and reproductive endocrine function in relation to biomarkers of lead, cadmium, zinc, and copper in men. Environ Health Perspect. (2000) 108:45–53. doi: 10.1289/ehp.0010845
51. Badr FM, El-Habit O. Heavy Metal Toxicity Affecting Fertility and Reproduction of Males. Ismailia; Cairo: Academic Press (2018).
52. Lopez-Botella A, Velasco I, Acien M, Saez-Espinosa P, Todoli-Torro JL, Sanchez-Romero R, et al. Impact of heavy metals on human male fertility-an overview. Antioxidants. (2021) 10:1473. doi: 10.3390/antiox10091473
53. de Angelis C, Galdiero M, Pivonello C, Salzano C, Gianfrilli D, Piscitelli P, et al. The environment and male reproduction: the effect of cadmium exposure on reproductive function and its implication in fertility. Reproduct Toxicol. (2017) 73:105–27. doi: 10.1016/j.reprotox.2017.07.021
54. Reis MMS, Moreira AC, Sousa M, Mathur PP, Oliveira PF, Alves MG. Sertoli cell as a model in male reproductive toxicology: advantages and disadvantages. J Appl Toxicol. (2015) 35:870–83. doi: 10.1002/jat.3122
55. Pant N, Kumar G, Upadhyay AD, Gupta YK, Chaturvedi PK. Correlation between lead and cadmium concentration and semen quality. Andrologia. (2015) 47:887–91. doi: 10.1111/and.12342
56. Mendiola J, Stahlhut RW, Jørgensen N, Liu F, Swan SH. Shorter anogenital distance predicts poorer semen quality in young men in Rochester, New York. Environ Health Perspect. (2011) 119:958–63. doi: 10.1289/ehp.1103421
57. Giahi L, Mohammadmoradi S, Javidan A, Sadeghi MR. Nutritional modifications in male infertility: a systematic review covering 2 decades. Nutr Rev. (2016) 74:118–30. doi: 10.1093/nutrit/nuv059
58. Harchegani AB, Khor A, Niha MM, Kaboutaraki HB, Shirvani H, Shahriary A. The hepatoprotective and antioxidative effect of saffron stigma alcoholic extract against vincristine sulfate induced toxicity in rats. Interdiscip Toxicol. (2019) 12:186–91. doi: 10.2478/intox-2019-0023
59. Maneesh M, Jayalekshmi H. Role of reactive oxygen species and antioxidants on pathophysiology of male reproduction. Indian J Clin Biochem. (2006) 21:80–9. doi: 10.1007/BF02912918
60. Hillary W, Julie WC, Edmund YK. Role of reactive oxygen species in male infertility: an updated review of literature. Arab J Urol. (2018) 16:35–43. doi: 10.1016/j.aju.2017.11.001
61. Baskaran S, Finelli R, Agarwal A, Henkel R. Reactive oxygen species in male reproduction: a boon or a bane? Andrologia. (2020) 53:e13577. doi: 10.1111/and.13577
62. Said TM, Agarwal A, Sharma RK, Thomas AJ, Sikka SC. Impact of sperm morphology on DNA damage caused by oxidative stress induced by beta-nicotinamide adenine dinucleotide phosphate. Fertil Steril. (2005) 83:95–103. doi: 10.1016/j.fertnstert.2004.06.056
63. Lidia M, Jorge EC, Jaime M, Manuela R, Cigdem T, Jesús V, et al. Fatty acid intake in relation to reproductive hormones and testicular volume among young healthy men. Asian J Androl. (2017) 19:184–90. doi: 10.4103/1008-682X.190323
64. Kinga S, Piotr E, Liliana A, Agnieszka D, Iwona K. Diet and nutritional factors in male (In)fertility—underestimated factors. J Clin Med. (2020) 9:1400. doi: 10.3390/jcm9051400
65. Nagpal G, Usmani SS, Dhanda SK, Kaur H, Singh S, Sharma M, et al. Computer-aided designing of immunosuppressive peptides based on IL-10 inducing potential. Sci Rep. (2017) 7:42851. doi: 10.1038/srep42851
66. Bibbo S, Ianiro G, Giorgio V, Scaldaferri F, Masucci L, Gasbarrini A, et al. The role of diet on gut microbiota composition. Eur Rev Med Pharmacol Sci. (2016) 20:4742–9.
67. Ding GL, Liu Y, Liu ME, Pan JX, Guo MX, Sheng JZ, et al. The effects of diabetes on male fertility and epigenetic regulation during spermatogenesis. Asian J Androl. (2015) 17:948–53. doi: 10.4103/1008-682X.150844
68. Balawender K, Orkisz S. The impact of selected modifiable lifestyle factors on male fertility in the modern world. Cent European J Urol. (2020) 73:563–8. doi: 10.5173/ceju.2020.1975
69. Challis LJ. Mechanisms for interaction between RF fields and biological tissue. Bioelectromagnetics Suppl. (2005) 7:S98–106. doi: 10.1002/bem.20119
70. Rago R, Salacone P, Caponecchia L, Sebastianelli A, Marcucci I, Calogero AE, et al. The semen quality of the mobile phone users. J Endocrinol Invest. (2013) 36:970–4. doi: 10.3275/8996
71. Agarwal A, Singh A, Hamada A, Kesari K. Cell phones and male infertility: a review of recent innovations in technology and consequences. Brazilian J Urol. (2011) 37:432–54. doi: 10.1590/S1677-55382011000400002
72. Avendaño C, Mata A, Sanchez Sarmiento CA, Doncel GD. Use of laptop computers connected to internet through Wi-Fi decreases human sperm motility and increases sperm DNA fragmentation. Fertil Steril. (2012) 97:39–45.e2. doi: 10.1016/j.fertnstert.2011.10.012
73. Cooper TG. The epididymis, cytoplasmic droplets and male fertility. Asian J Androl. (2011) 13:130–8. doi: 10.1038/aja.2010.97
74. Tremellen K. Oxidative stress and male infertility–a clinical perspective. Hum Reprod Update. (2008) 14:243–58. doi: 10.1093/humupd/dmn004
75. Oh JJ, Byun SS, Lee SE, Choe G, Hong SK. Effect of electromagnetic waves from mobile phones on spermatogenesis in the era of 4G-LTE. Biomed Res Int. (2018) 2018:1801798. doi: 10.1155/2018/1801798
76. Sharma R, Harlev A, Agarwal A, Esteves SC. Cigarette smoking and semen quality: a new meta-analysis examining the effect of the 2010 world health organization laboratory methods for the examination of human semen. Eur Urol. (2016) 70:635–45. doi: 10.1016/j.eururo.2016.04.010
77. Mostafa RM, Nasrallah YS, Hassan MM, Farrag AF, Majzoub A, Agarwal A. The effect of cigarette smoking on human seminal parameters, sperm chromatin structure and condensation. Andrologia. (2018) 50. doi: 10.1111/and.12910
78. Sepaniak S, Forges T, Gerard H, Foliguet B, Bene MC, Monnier-Barbarino P. The influence of cigarette smoking on human sperm quality and DNA fragmentation. Toxicology. (2006) 223:54–60. doi: 10.1016/j.tox.2006.03.001
79. Aboulmaouahib S, Madkour A, Kaarouch I, Sefrioui O, Saadani B, Copin H, et al. Impact of alcohol and cigarette smoking consumption in male fertility potential: looks at lipid peroxidation, enzymatic antioxidant activities and sperm DNA damage. Andrologia. (2018) 50. doi: 10.1111/and.12926
80. Dai J, Zhan C, Xu W, Wang Z, Nie D, Zhao X, et al. Nicotine elevates sperm motility and induces Pfn1 promoter hypomethylation in mouse testis. Andrology. (2015) 3:967–78. doi: 10.1111/andr.12072
81. Li Y, Lin H, Li Y, Cao J. Association between socio-psycho-behavioral factors and male semen quality: systematic review and meta-analyses. Fertil Steril. (2011) 95:116–23. doi: 10.1016/j.fertnstert.2010.06.031
82. Ricci E, Al BS, Cipriani S, Candiani M, Chiaffarino F, Vigano P, et al. Semen quality and alcohol intake: a systematic review and meta-analysis. Reprod Biomed Online. (2017) 34:38–47. doi: 10.1016/j.rbmo.2016.09.012
83. Condorelli RA, Calogero AE, Vicari E, La Vignera S. Chronic consumption of alcohol and sperm parameters: our experience and the main evidences. Andrologia. (2015) 47:368–79. doi: 10.1111/and.12284
84. Hansen ML, Thulstrup AM, Bonde JP, Olsen J, Håkonsen LB, Ramlau-Hansen CH. Does last week's alcohol intake affect semen quality or reproductive hormones? A cross-sectional study among healthy young Danish men. Reprod Toxicol. (2012) 34:457–62. doi: 10.1016/j.reprotox.2012.06.004
85. Berger MM, Shenkin A. Vitamins and trace elements: practical aspects of supplementation. Nutrition. (2006) 22:952–5. doi: 10.1016/j.nut.2006.06.004
86. Angulo C, Maldonado R, Pulgar E, Mancilla H, Cordova A, Villarroel F, et al. Vitamin C and oxidative stress in the seminiferous epithelium. Biol Res. (2011) 44:169–80. doi: 10.4067/S0716-97602011000200009
87. Ahmadi S, Bashiri R, Ghadiri-Anari A, Nadjarzadeh A. Antioxidant supplements and semen parameters: an evidence based review. Int J Reprod Biomed. (2016) 14:729–36. doi: 10.29252/ijrm.14.12.729
88. Bisong SA, Ukoh IE, Nna VU, Ebong PE. Vitamin E attenuates nicotine- and noise-induced reproductive impairment in male albino Wistar rats. Andrologia. (2018) 50:e13050. doi: 10.1111/and.13050
89. Miyazawa T, Burdeos GC, Itaya M, Nakagawa K, Miyazawa T. Vitamin E: regulatory redox interactions. IUBMB Life. (2019) 71:430–41. doi: 10.1002/iub.2008
90. Fenech M. Folate (vitamin B9) and vitamin B12 and their function in the maintenance of nuclear and mitochondrial genome integrity. Mutat Res. (2012) 733:21–33. doi: 10.1016/j.mrfmmm.2011.11.003
91. Merrell BJ, McMurry JP. folic Acid. Int J Fertil Steril. (2019) 12:267–72. doi: 10.22074/ijfs.2019.5420
92. Wong WY, Merkus HMWM, Thomas CMG, Menkveld R, Regine GA, Theunissen PMS. Effects of folic acid and zinc sulfate on male factor subfertility: a double-blind, randomized, placebo-controlled trial. Fertil Steril. (2002) 77:491–8.
93. Tarapore P, Ouyang B. Perfluoroalkyl chemicals and male reproductive health: do PFOA and PFOS increase risk for male infertility? Int J Environ Res Public Health. (2021) 18:3794. doi: 10.3390/ijerph18073794
94. Alonge S, Melandri M, Leoci R, Lacalandra GM, Caira M, Aiudi GG. The effect of dietary supplementation of vitamin e, selenium, zinc, folic acid, and N-3 polyunsaturated fatty acids on sperm motility and membrane properties in dogs. Animals. (2019) 9:34. doi: 10.3390/ani9020034
95. Chasapis CT, Ntoupa PA, Spiliopoulou CA, Stefanidou ME. Recent aspects of the effects of zinc on human health. Arch Toxicol. (2020) 94:1443–60. doi: 10.1007/s00204-020-02702-9
96. Evans P, Halliwell B. Micronutrients: oxidant/antioxidant status. Br J Nutr. (2001) 85(Suppl. 2):S67–74. doi: 10.1079/BJN2000296
97. Liu DY, Sie BS, Liu ML, Agresta F, Baker HW. Relationship between seminal plasma zinc concentration and spermatozoa-zona pellucida binding and the ZP-induced acrosome reaction in subfertile men. Asian J Androl. (2009) 11:499–507. doi: 10.1038/aja.2009.23
98. Fallah A, Mohammad-Hasani A, Colagar AH. Zinc is an essential element for male fertility: a review of Zn roles in men's health, germination, sperm quality, and fertilization. J Reprod Infertil. (2018) 19:69–81.
99. Kothari RP, Chaudhari AR. Zinc levels in seminal fluid in infertile males and its relation with serum free testosterone. J Clin Diagn Res. (2016) 10:CC05-8. doi: 10.7860/JCDR/2016/14393.7723
101. Chu Q, Chi ZH, Zhang X, Liang D, Wang X, Zhao Y, et al. A potential role for zinc transporter 7 in testosterone synthesis in mouse Leydig tumor cells. Int J Mol Med. (2016) 37:1619–26. doi: 10.3892/ijmm.2016.2576
102. Egwurugwu JN, Ifedi CU, Uchefuna RC, Ezeokafor EN, Alagwu EA. Effects of zinc on male sex hormones and semen quality in rats. Niger J Physiol Sci. (2013) 28:17–22.
103. Talevi R, Barbato V, Fiorentino I, Braun S, Longobardi S, Gualtieri R. Protective effects of in vitro treatment with zinc, d-aspartate and coenzyme q10 on human sperm motility, lipid peroxidation and DNA fragmentation. Reprod Biol Endocrinol. (2013) 11:81. doi: 10.1186/1477-7827-11-81
104. Huang YL, Tseng WC, Cheng SY, Lin TH. Trace elements and lipid peroxidation in human seminal plasma. Biol Trace Elem Res. (2000) 76:207–15. doi: 10.1385/BTER:76:3:207
105. Ebisch IM, Peters WH, Thomas CM, Wetzels AM, Peer PG, Steegers-Theunissen RP. Homocysteine, glutathione and related thiols affect fertility parameters in the (sub)fertile couple. Hum Reprod. (2006) 21:1725–33. doi: 10.1093/humrep/del081
106. Saito H, Hara K, Kitajima S, Tanemura K. Effect of vitamin E deficiency on spermatogenesis in mice and its similarity to aging. Reprod Toxicol. (2020) 98:225–32. doi: 10.1016/j.reprotox.2020.10.003
107. James V, Kalanghot PS, Pulikkal SK, Kalanghot PSA, Skandhan A, Meenaxi G. Calcium and magnesium in male reproductive system and in its secretion. I level in normal human semen, seminal plasma and spermatozoa. Urologia J. (2015) 82:174–8. doi: 10.5301/urologia.5000039
108. Golpour A, Pšenička M, Niksirat H. Subcellular distribution of calcium during spermatogenesis of zebrafish, Danio rerio. J Morphol. (2017) 278:1149–59. doi: 10.1002/jmor.20701
109. Bassey IE, Essien OE, Udoh AE, Imo IU, Effiong IO. Seminal plasma selenium, calcium, magnesium and zinc levels in infertile men. J Med Sci. (2013) 1:196–204. doi: 10.3923/jms.2013.483.487
110. Beigi HA, Irandoost A, Mirnamniha M, Rahmani H, Tahmasbpour E, Shahriary A. Possible mechanisms for the effects of calcium deficiency on male infertility. Int J Fertil Steril. (2019).
111. Hamad A-WR, Al-Dadhistani HI, Shquirat WD, Abdel-Dayem M, Al-Swaif M. Sodium, potassium, calcium and copper levels in seminal plasma are associated with sperm quality in fertile and infertile men. Biochem Pharmacol. (2014) 3:4. doi: 10.4172/2167-0501.1000141
112. Jimenez T, McDermott JP, Sánchez G, Blanco G. Na,K-ATPase alpha4 isoform is essential for sperm fertility. Proc Natl Acad Sci U S A. (2011) 108:644–9. doi: 10.1073/pnas.1016902108
113. Pascoal GDFL, Geraldi MV, Maróstica MR, Ong TP. Effect of paternal diet on spermatogenesis and offspring health: focus on epigenetics and interventions with food bioactive compounds. Nutrients. (2022) 14:2150. doi: 10.3390/nu14102150
114. Omu AE, Al-Bader AA, Dashti H, Oriowo MA. Magnesium in human semen: possible role in premature ejaculation. Arch Androl. (2001) 46:59–66. doi: 10.1080/01485010150211164
115. Ursini F, Heim S, Kiess M, Maiorino M, Roveri A, Wissing J, et al. Dual function of the selenoprotein PHGPx during sperm maturation. Science. (1999) 285:1393–6. doi: 10.1126/science.285.5432.1393
116. Morbat MM, Hadi AM, Hadri DH. Effect of selenium in treatment of male infertility. Exp Tech Urol Nephrol. (2018) 5:1–4. doi: 10.31031/ETUN.2018.01.000521
117. Asadi N, Bahmani M, Kheradmand A, Rafieian-Kopaei M. The impact of oxidative stress on testicular function and the role of antioxidants in improving it: a review. J Clin Diagn Res. (2017) 11:IE01–5. doi: 10.7860/JCDR/2017/23927.9886
118. Asri-Rezaei S, Nourian A, Shalizar-Jalali A, Najafi G, Nazarizadeh A, Koohestani M, et al. Selenium supplementation in the form of selenium nanoparticles and selenite sodium improves mature male mice reproductive performances. Iran J Basic Med Sci. (2018) 21:577–85. doi: 10.22038/IJBMS.2018.26023.6397
119. Tajaddini S, Ebrahimi S, Behnam B, Bakhtiyari M, Joghataei MT, Abbasi M, et al. Antioxidant effect of manganese on the testis structure and sperm parameters of formalin-treated mice. Andrologia. (2014) 46:246–53. doi: 10.1111/and.12069
120. Bansal MP, Jaswal S. Hypercholesterolemia induced oxidative stress is reduced in rats with diets enriched with supplement from dunaliella salina algae. Am J Biomed Sci. (2009) 85:880–5. doi: 10.5099/aj090300196
121. Avila DS, Puntel RL, Aschner M. Manganese in health and disease. Met Ions Life Sci. (2013) 13:199–227. doi: 10.1007/978-94-007-7500-8_7
122. Li P, Zhong Y, Jiang X, Wang C, Zuo Z, Sha A. Seminal plasma metals concentration with respect to semen quality. Biol Trace Elem Res. (2012) 148:1–6. doi: 10.1007/s12011-012-9335-7
123. Kumar N, Singh AK. Trends of male factor infertility, an important cause of infertility: A review of literature. J Hum Reprod Sci. (2015) 8:191–6. doi: 10.4103/0974-1208.170370
124. Pine M, Lee B, Dearth R, Hiney JK, Dees WL. Manganese acts centrally to stimulate luteinizing hormone secretion: a potential influence on female pubertal development. Toxico Sci. (2005) 61:673–80. doi: 10.1093/toxsci/kfi134
125. Rotter I, Kosik-Bogacka DI, Dołegowska B, Safranow K, Kuczyńska M, Laszczyńska M. Analysis of the relationship between the blood concentration of several metals, macro- and micronutrients and endocrine disorders associated with male aging. Environ Geochem Health. (2016) 38:749–61. doi: 10.1007/s10653-015-9758-0
126. Fengdi W, Haibo Y, Yanan L, Xinxin Y, Bin X, Wei L, et al. Manganese exposure caused reproductive toxicity of male mice involving activation of GnRH secretion in the hypothalamus by prostaglandin E2 receptors EP1 and EP2. Ecotoxicol Environ Saf. (2020) 201:110712. doi: 10.1016/j.ecoenv.2020.110712
127. Wang C, Lu JP, Jiang YM, Ma NH, Qin WP, Luo HL, et al. [Effects of low level manganese exposure on the serum neuroendocrine hormones in the welders]. Zhonghua Lao Dong Wei Sheng Zhi Ye Bing Za Zhi. (2011) 29:94–7.
128. Chen X, Liu Z, Ge X, Luo X, Huang S, Zhou Y, et al. Associations between manganese exposure and multiple immunological parameters in manganese-exposed workers healthy cohort. J Trace Elem Med Biol. (2020) 59:126454. doi: 10.1016/j.jtemb.2020.126454
129. Tvrda E, Peer R, Sikka SC, Agarwal A. Iron and copper in male reproduction: a double-edged sword. J Assist Reprod Genet. (2015) 32:3–16. doi: 10.1007/s10815-014-0344-7
130. Saleh A, Alvarez-Venegas R, Avramova Z. An efficient chromatin immunoprecipitation (ChIP) protocol for studying histone modifications in Arabidopsis plants. Nat Protoc. (2008) 3:1018–25. doi: 10.1038/nprot.2008.66
131. Seven I, Tatli SP, Gul BB, Parlak AT, Ozer KS, Yaman M. Bee glue (propolis) improves reproductive organs, sperm quality and histological changes and antioxidant parameters of testis tissues in rats exposed to excess copper. Andrologia. (2020) 52:e13540. doi: 10.1111/and.13540
132. Xu XF, Wang B, Lou Y, Han WJ, Lu JY, Li DD, et al. Magnesium transporter 5 plays an important role in Mg transport for male gametophyte development in Arabidopsis. Plant J. (2015) 84:925–36. doi: 10.1111/tpj.13054
133. Marín-Briggiler CI, Vazquez-Levin MH, Gonzalez-Echeverría F, Blaquier JA, Tezón JG, Miranda PV. Strontium supports human sperm capacitation but not follicular fluid-induced acrosome reaction. Biol Reprod. (1999). doi: 10.1095/biolreprod61.3.673
134. Miao Y, Liu L, Liu C, Deng Y, Chen P, Luo Q, et al. Urinary biomarker of strontium exposure is positively associated with semen quality among men from an infertility clinic. Ecotoxicol Environ Saf. (2021) 208. doi: 10.1016/j.ecoenv.2020.111694
135. Zhai X, Zhang Y, Qi Q, Bai Y, Chen X, Jin L, et al. Effects of molybdenum on sperm quality and testis oxidative stress. Syst Biol Reprod Med. (2013) 59. doi: 10.3109/19396368.2013.791347
136. Russ H, János R, Ulrike B, Wolfram R, Bernhard S. Mechanistic aspects of molybdenum-containing enzymes. FEMS Microbiol Rev. (1998) 22:489–501. doi: 10.1111/j.1574-6976.1998.tb00383.x
137. Jurkowska K, Kratz EM, Sawicka E, Piwowar A. The impact of metalloestrogens on the physiology of male reproductive health as a current problem of the XXI century. J Physiol Pharmacol. (2019) 70. doi: 10.26402/jpp.2019.3.02
138. Mirnamniha M, Faroughi F, Tahmasbpour E, Ebrahimi P, Beigi HA. An overview on role of some trace elements in human reproductive health, sperm function and fertilization process. Rev Environ Health. (2019) 34:339–48. doi: 10.1515/reveh-2019-0008
139. Eibensteiner L, Del CSA, Frumkin H, Gonzales C, Gonzales GF. Lead exposure and semen quality among traffic police in Arequipa, Peru. Int J Occup Environ Health. (2005) 11:161–6. doi: 10.1179/oeh.2005.11.2.161
140. Falchi L, Khalil WA, Hassan M, Marei WFA. Perspectives of nanotechnology in male fertility and sperm function. Int J Vet Sci Med. (2018) 26:265–9. doi: 10.1016/j.ijvsm.2018.09.001
141. Takeda K, Suzuki K, Ishihara A, Kubo-Irie M, Fujimoto R, Tabata M, et al. Nanoparticles transferred from pregnant mice to their offspring can damage the genital and cranial nerve systems. J Health. (2009) 55:95–102. doi: 10.1248/jhs.55.95
142. Afifi M, Almaghrabi OA, Kadasa NM. Ameliorative effect of zinc oxide nanoparticles on antioxidants and sperm characteristics in streptozotocin-induced diabetic rat testes. Biomed Res Int. (2015) 2015:153573. doi: 10.1155/2015/153573
143. Jahanbin R, Yazdanshenas P, Afshar MA, Sangcheshmeh AM, Varnaseri H, Chamani M, et al. Effect of zinc nano-complex on bull semen quality after freeze-thawing process. Anim Prod. (2015) 17:371–80. doi: 10.22059/jap.2015.54040
144. Ema M, Gamo M, Honda K. Developmental toxicity of engineered nanomaterials in rodents. Toxicol Appl Pharmacol. (2016) 299:47–52. doi: 10.1016/j.taap.2015.12.015
145. Ogunsuyi OM, Ogunsuyi OI, Akanni O, Alabi OA, Alimba CG, Adaramoye OA, et al. Alteration of sperm parameters and reproductive hormones in Swiss mice via oxidative stress after co-exposure to titanium dioxide and zinc oxide nanoparticles. Andrologia. (2020) 52:e13758. doi: 10.1111/and.13758
146. Opris R, Toma V, Olteanu D, Baldea I, Baciu AM, Lucaci FI, et al. Effects of silver nanoparticles functionalized with Cornus mas L. extract on architecture and apoptosis in rat testicle. Nanomedicine. (2019) 14:275–99. doi: 10.2217/nnm-2018-0193
147. Han Z, Yan Q, Ge W, Liu ZG, Gurunathan S, De Felici M, et al. Cytotoxic effects of ZnO nanoparticles on mouse testicular cells. Int J Nanomedicine. (2016) 11:5187–203. doi: 10.2147/IJN.S111447
148. Olugbodi JO, David O, Oketa EN, Lawal B, Okoli BJ, Mtunzi F. Silver nanoparticles stimulates spermatogenesis impairments and hematological alterations in testis and epididymis of male rats. Molecules. (2020) 25:1063. doi: 10.3390/molecules25051063
149. Hong F, Zhao X, Chen M, Zhou Y, Ze Y, Wang L, et al. TiO2 nanoparticles-induced apoptosis of primary cultured Sertoli cells of mice. J Biomed Mater Res A. (2016) 104:124–35. doi: 10.1002/jbm.a.35548
150. Miresmaeili SM, Halvaei I, Fesahat F, Fallah A, Nikonahad N, Taherinejad M. Evaluating the role of silver nanoparticles on acrosomal reaction and spermatogenic cells in rat. Iran J Reprod Med. (2013) 11:423–30.
151. Sleiman HK, Romano RM, Oliveira CAD, Romano MA. Effects of prepubertal exposure to silver nanoparticles on reproductive parameters in adult male Wistar rats. J Toxicol Environ Health A. (2013) 76:1023–32. doi: 10.1080/15287394.2013.831723
152. Boisen AM, Shipley T, Jackson P, Wallin H, Nellemann C, Vogel U, et al. In utero exposure to nanosized carbon black (Printex90) does not induce tandem repeat mutations in female murine germ cells. Reprod Toxicol. (2013) 41:45–8. doi: 10.1016/j.reprotox.2013.06.068
153. Skovmand A, Jensen AC, Maurice C, Marchetti F, Lauvås AJ, Koponen IK, et al. Effects of maternal inhalation of carbon black nanoparticles on reproductive and fertility parameters in a four-generation study of male mice. Part Fibre Toxicol. (2019) 16:1–13. doi: 10.1186/s12989-019-0295-3
154. Tang Y, Chen B, Hong W, Chen L, Yao L, Zhao Y, et al. ZnO Nanoparticles induced male reproductive toxicity based on the effects on the endoplasmic reticulum stress signaling pathway. Int J Nanomedicine. (2019) 14:9563–76. doi: 10.2147/IJN.S223318
155. Sundarraj K, Manickam V, Raghunath A, Periyasamy M, Viswanathan MP, Perumal E. Repeated exposure to iron oxide nanoparticles causes testicular toxicity in mice. Environ Toxicol. (2017) 32:594–608. doi: 10.1002/tox.22262
156. Soliman AHM, Ibrahim IA, Shehata MA, Mohammed HO. Histopathological and genetic study on the protective role of β-Carotene on testicular tissue of adult male albino rats treated with titanium dioxide nanoparticles. Afr J Pharm Pharmaco. (2020) 14:9–19. doi: 10.5897/AJPP2019.5109
157. Yousef MI, Al-Hamadani M, Kamel MA. Reproductive toxicity of aluminum oxide nanoparticles and zinc oxide nanoparticles in male rats. Nanoparticle. (2019) 1:3. doi: 10.35702/nano.10003
158. Hussein MM, Ali HA, Saadeldin IM, Ahmed MM. Querectin alleviates zinc oxide nanoreprotoxicity in male albino rats. J Biochem Mol Toxicol. (2016) 30:489–96. doi: 10.1002/jbt.21812
159. Song G, Lin L, Liu L, Wang K, Ding Y, Niu Q, et al. Toxic effects of anatase titanium dioxide nanoparticles on spermatogenesis and testicles in male mice. Pol J Environ Stud. (2017) 26:2739–45. doi: 10.15244/pjoes/70807
Keywords: spermatogenesis, reproductive health, sperm, nutrients, fertility
Citation: Chao H-H, Zhang Y, Dong P-Y, Gurunathan S and Zhang X-F (2023) Comprehensive review on the positive and negative effects of various important regulators on male spermatogenesis and fertility. Front. Nutr. 9:1063510. doi: 10.3389/fnut.2022.1063510
Received: 10 October 2022; Accepted: 23 December 2022;
Published: 16 January 2023.
Edited by:
Francesco Galli, University of Perugia, ItalyReviewed by:
Elena Eugeni, University of Perugia, ItalyLuigi Napolitano, University of Naples Federico II, Italy
Copyright © 2023 Chao, Zhang, Dong, Gurunathan and Zhang. This is an open-access article distributed under the terms of the Creative Commons Attribution License (CC BY). The use, distribution or reproduction in other forums is permitted, provided the original author(s) and the copyright owner(s) are credited and that the original publication in this journal is cited, in accordance with accepted academic practice. No use, distribution or reproduction is permitted which does not comply with these terms.
*Correspondence: Xi-Feng Zhang, emhhbmd4ZjEwNkBxYXUuZWR1LmNu;
emhhbmd4Zjk0NjVAMTYzLmNvbQ==
†These authors share first authorship