- Gansu Key Laboratory of Herbivorous Animal Biotechnology, Faculty of Animal Science and Technology, Gansu Agricultural University, Lanzhou, China
Long noncoding RNA (lncRNA) plays an important regulatory role in mammalian adipogenesis and lipid metabolism. However, their function in the longissimus thoracis (LT) muscle of fatty acid metabolism of Tibetan sheep remains undefined. In this study, fatty acid and fat content in LT muscle of Tibetan sheep were determined, and RNA sequencing was performed to reveal the temporal regularity of lncRNA expression and the effect of lncRNA-miRNA-mRNA ceRNA regulatory network on lipid metabolism of LT muscle in Tibetan sheep at four growth stages (4-month-old, 4 m; 1.5-year-old, 1.5 y; 3.5-year-old, 3.5 y; 6-year-old, 6 y). The results indicated that the intramuscular fat (IMF) content was highest at 1.5 y. Moreover, the monounsaturated fatty acid (MUFA) content in 1.5 y of Tibetan sheep is significantly higher than those of the other groups (P < 0.05), and it was also rich in a variety of polyunsaturated fatty acids (PUFA). A total of 360 differentially expressed lncRNAs (DE lncRNAs) were identified from contiguous period transcriptome comparative groups of 4 m vs. 1.5 y, 1.5 y vs. 3.5 y, 3.5 y vs. 6 y, and 4 m vs. 6 y, respectively. Kyoto encyclopedia of genes and genomes (KEGG) enrichment analysis found that the target genes in lncRNA trans-mRNA were significantly related to the protein digestion, absorption, and fatty acid biosynthesis pathways (P < 0.05), which demonstrated that DE lncRNA trans-regulated the target genes, and further regulated the growth and development of the LT muscle and intramuscular fatty acid metabolism in Tibetan sheep. We further analyzed the role of the lncRNA-miRNA-mRNA regulatory network in the lipid metabolism of Tibetan sheep. Additionally, GPD2, LIPE (lipase E hormone-sensitive enzyme), TFDP2, CPT1A, ACACB, ADIPOQ, and other mRNA related to fatty acid and lipid metabolism and the corresponding lncRNA-miRNA regulatory pairs were identified. The enrichment analysis of mRNA in the regulatory network found that the AMPK signaling pathway was the most significantly enriched (P = 0.0000112361). Comprehensive transcriptome analysis found that the LIPE, ADIPOQ, ACACB, and CPT1A that were regulated by lncRNA might change the formation of energy metabolism in Tibetan sheep muscle through the AMPK signaling pathway, and oxidized muscle fibers are transformed into glycolytic muscle fibers, reduced IMF content, and the fatty acid profile also changed.
Introduction
Tibetan sheep is the dominant livestock resource in the Qinghai-Tibet Plateau, living at an altitude above 3,000 m on the Qinghai-Tibet Plateau with high altitude, low temperature, thinner oxygen, and strong UV radiation. Tibetan sheep meat is loved by consumers who like mutton for its unique flavor (1, 2). Skeletal muscle generation is an extremely complex and precise process, which is the result of the interaction between genetics and environment, including sequential steps of muscle stem cell proliferation, myoblast differentiation, and cell fusion to form multinucleated myotubes and so on (3). Mammalian skeletal muscle is a heterogeneous tissue, which is composed of various muscle fibers that exhibit different physiological and metabolic properties, such as glycolysis, oxidative metabolism, and contraction. Skeletal muscle is the major component of body mass accounting for approximately 50% of body mass in a mammal (4). Skeletal muscle growth and development directly influence muscle quality and meat production. Meanwhile, different muscle fiber types of skeletal muscle also have an influence on intramuscular fat (IMF), meat tenderness, water retention, juiciness, and fatty acid composition (5, 6). In addition, the IMF also determines the meat tenderness and fatty acid profiles in the skeletal muscle (7). Fatty acids are an important part of cells and are involved in the energy metabolism of human and animals as signaling molecules (8). Therefore, exploring the mechanism of skeletal muscle growth and development is very important to improve lipid and fatty acid metabolism in Tibetan sheep meat.
Previous studies demonstrated that 80% of the mammalian genome can be transcribed, and RNA is produced from many expressed genomic sites in the sense and antisense DNA strands (9). Additionally, long noncoding RNA (lncRNA) is a type of RNA transcript from the sense strand of DNA, over 200 nucleotides in length, no or weak protein-coding potential, low expression, with specificities of tissue and developmental stage, and low conservation among different species (10–12). It serves as a key functional molecule that mediates a variety of biological processes in organisms, such as histone modification, chromosome remodeling, gene expression regulation, miRNA precursor generation, animal development, cell differentiation, and so on, which is a hot topic of this research (13, 14). lncRNA is essential for the physiological regulation of cell proliferation and differentiation (15). A large number of studies have found that lncRNA has an important regulatory effect on fatty acid and lipid metabolism in human and animals (16, 17).
Long noncoding RNAs can regulate the expression of functional genes in a variety of ways. lncRNAs regulate the expression of neighboring functional genes through cis-mRNA and can also regulate distant target genes through trans-mRNA (18). In addition, lncRNAs could serve as miRNA sponges and thereby impair miRNA-mediated gene silencing (19). Jiang et al. (20) found that differentially expressed lncRNAs (DE lncRNAs) might play an important role in the adipose tissue of cattle at different age groups (20). Zhang et al. (3) found that lnc403 influenced the differentiation of skeletal muscle cells by regulating the expression of neighboring genes and interacting proteins and revealed that lnc403 might participate in the differentiation of bovine myoblasts (3). Yang et al. (21) also demonstrated that Gm16551 was a negative regulator of lncRNA related to SREBP1c activity and adipogenesis in mouse liver (21). Yue et al. (22) constructed a potential function of ceRNA network in bovine skeletal muscle, which indicated that lncRNA had functional specificity for bovine skeletal muscle development, and DE lncRNA was significantly enriched in the biological processes related to muscle development and the Wnt signaling pathway (22). Many studies have demonstrated several lncRNAs involved in mammalian adipogenesis and lipid metabolism. However, there are no studies on the lncRNA in Tibetan sheep muscle development, IMF, and fatty acid metabolism. A total of sixteen Tibetan sheep in four growth stages was selected in this study based on the differences in slaughter performance and meat quality, and the differences in lipid metabolism at different growth stages were analyzed. We further analyzed the function of lncRNA and the mechanism of the lncRNA-miRNA-mRNA ceRNA regulatory network in the longissimus thoracis (LT) muscle lipid metabolism of Tibetan sheep using an RNA sequencing approach. We analyzed the function and regulatory network of lncRNA in lipid metabolism during muscle growth and development, especially with the increase of age, and explored the role of lncRNA in the transformation of oxidized muscle fiber and glycolytic muscle fiber in Tibetan sheep and its influence on the difference of fatty acids, which would benefit to explore the molecular mechanism of Tibetan sheep muscle growth and development.
Materials and Methods
Animals and Muscle Sampling
Sixteen healthy female Tibetan sheep were randomly selected from the same sheep flock of Haiyan County, Qinghai Province, China (3,500 m above sea level), including 4-month-old, 4 m (n = 4); 1.5-year-old, 1.5 y (n = 4); 3.5-year-old, 3.5 y (n = 4); and 6-year-old, 6 y (n = 4). In addition, 4 m, 1.5 y, 3.5 y, and 6 y represent the lambs, the pubertal sheep, the adult sheep, and the old sheep, respectively. All sheep had the same nutrition and were raised under the same environmental conditions with natural light and free access to food and water. Four Tibetan sheep from each growth stage were weighed and immediately slaughtered humanely according to the Islamic practice (exsanguinated, peeled, and split down the midline according to standard operating procedures). The experiment was conducted in accordance with the guidelines of the National Institutes of Health Guide for the Care and Use of Laboratory Animals (NIH Publications No. 8023, revised 1978), and animal welfare and conditions were considered in the use of experimental animals. The carcasses were placed in a chilling room at 4°C before sampling for meat quality traits. LT muscle (from 12th thoracic vertebrae to 5th lumbar vertebrae) samples from the left half carcass were collected from the four different growth stages after slaughtering and immediately frozen in liquid nitrogen and stored at −80°C for RNA isolation.
Meat Quality Measurements
IMF Content
The IMF content was measured using the Soxhlet extraction method with solvent (petroleum ether) and expressed as a weight percentage of wet muscle tissue (AOAC, 2007), with three replicates for each sample (23).
Fatty Acid Profile
Fatty acids were extracted from LT muscle samples of Tibetan sheep and methylated as described by Gao et al. (24). The fatty acid profiles were analyzed by gas chromatography (SRI Model 8610C, USA). The nitrogen flow rate was set to 1.2 ml/min, and the airflow rate was set to 450 ml/min. The column was operated isothermally at 140–240°C at 5°C/min and kept at 240°C for 15 min. The injection and detector temperatures were 260°C and 250°C, respectively. Hydrogen (40 ml/min) was used as the carrier gas. Fatty acids were qualitatively identified by comparing the retention times of 37 fatty acids (Supelco 37 FAME Mix 47885-U, USA) and quantified by comparison of the peak areas of the sample and the internal standard (C11:0). The results were reported as grams of fatty acid per 100 g of LT muscle samples. The nutritional properties of LT muscle samples of Tibetan sheep were evaluated by calculating the ratios of PUFA/SFA and n-6/n-3 fatty acids.
RNA Extraction, Strand-Specific Library Construction, and Sequencing
The total RNA was extracted using the Trizol Reagent Kit (Invitrogen, Carlsbad, CA, USA) according to the manufacturer's protocol. RNA quality was assessed on an Agilent 2100 Bioanalyzer (Agilent Technologies, Palo Alto, CA, USA) and checked using RNase-free agarose gel electrophoresis. After the total RNA was extracted, rRNAs were removed to retain mRNAs and ncRNAs. The enriched mRNAs and ncRNAs were fragmented into short fragments by using fragmentation buffer and reverse transcribed into cDNA with random primers. Second-strand cDNA was synthesized by DNA polymerase I, RNase H, dNTP (dUTP instead of dTTP), and buffer. Then, the cDNA fragments were purified using the QiaQuick PCR Extraction Kit (Qiagen, Venlo, the Netherlands), end-repaired, a base added, and ligated to Illumina sequencing adapters. Then, uracil-N-glycosylase (UNG) was used to digest the second-strand cDNA. The digested products were size selected by agarose gel electrophoresis, PCR amplified, and sequenced using Illumina Novaseq6000 (or other platforms) by Gene Denovo Biotechnology Co., Guangzhou, China.
Transcriptome Assemble
The reads containing adapters were filtered using fastp (version 0.18.0) (25), removing reads containing more than 10% of unknown nucleotides (N) and low-quality reads containing more than 50% of low quality (Q-value ≤ 20) bases, to get high-quality clean reads. Short read alignment tool Bowtie 2 (version 2.2.8) was used for mapping reads to the ribosome RNA (rRNA) database (26). The rRNA mapped reads were then removed. The remaining clean reads were mapped to the reference genome Oar_rambouillet_v1.0 using HISAT2 (version 2.1.0) (27). The reconstruction of transcripts was carried out using StringTie (version 1.3.4) (28, 29).
lncRNA Identification
The filter criteria of lncRNAs were as follows: (a) seven classes of transcripts (“i,” “j,” “x,” “u,” “c,” “e,” and “o”) were defined as a novel transcript; (b) the length of the transcript was longer than 200 bp, and the exon number more than 2 was identified a reliable novel gene; (c) transcripts that overlap protein-coding mRNAs were removed; (d) novel transcripts were then aligned to the Nr, Kyoto encyclopedia of genes and genomes (KEGG), and gene ontology (GO) database to obtain protein functional annotation and exclude known mRNA and other noncoding RNAs (such as rRNA, tRNA, snoRNA, and snRNA). Transcripts without coding potential, as predicted by CNCI (version 2) (30), CPC (version 0.9-r2) (31), and the intersection of both nonprotein-coding potential results were chosen as novel lncRNAs.
Identifying DE lncRNAs and Function Analysis
For each transcription region, the Fragment Per Kilobase of transcript per Million mapped reads (FPKM) value was calculated to quantify its expression abundance and variations, using RSEM software (32). The genes/transcripts with the parameter of corrected P-value (FDR) < 0.05 and |log2 (fold change)| > 1 were considered DE lncRNAs. The cis target genes of lncRNAs were predicted according to the location of gene transcripts (around 100 kb of lncRNA) in genomics. The correlation of expression between lncRNAs and protein-coding genes was analyzed to identify trans-target genes of lncRNAs and protein-coding genes with an absolute correlation over 0.999. In this study, DAVID (http://david.abcc.ncifcrf.gov/) online analysis software was used for GO function annotation and KEGG pathway enrichment analysis (33).
Construction of lncRNA-miRNA-mRNA ceRNA Network
Based on our previous Illumina HiSeq miRNA and mRNA sequencing data from the same samples, the lncRNA-miRNA-mRNA ceRNA regulatory network was constructed as per the following ceRNA theory: (a) expression correlation between mRNA and miRNA or lncRNA and miRNA was evaluated using the Spearman rank correlation coefficient (SCC). Pairs with SCC < −0.7 were selected as co-expressed negatively lncRNA-miRNA pairs or mRNA-miRNA pairs; (b) expression correlation between lncRNA and mRNA was evaluated using the Pearson correlation coefficient (PCC). Pairs with PCC > 0.9 were selected as co-expressed lncRNA-mRNA pairs; and (c) the hypergeometric cumulative distribution function test was used to test whether the common miRNA sponges between the two genes were significant. As a result, only the gene pairs with a P < 0.05 were selected. The ceRNA network related to muscle development was visualized using Cytoscape 3.7.1.
Real-Time Quantitative PCR Analysis
The total RNA was extracted from LT muscle samples of Tibetan sheep with TRIzol reagent (Invitrogen, Carlsbad, CA, USA), which was used for RNA-Seq and synthesized cDNA using a real-time quantitative PCR (RT-PCR) Kit (Takara, Dalian, China). RT-qPCR analysis was used to validate the authenticity transcriptome results. The primer sequences of genes were designed for RT-qPCR analysis (Table 1). The RNA samples that were the same as those used for the RNA-Seq, the RT-qPCR was conducted in triplicate using the SYBR Green Pro Taq HS qPCR Kit (Accurate Biology, Hunan, China) on an Applied Biosystems QuantStudio® 6 Flex (Thermo Lifetech, MA, United States). The relative expression levels of these genes were analyzed using the 2−ΔΔCt method. Sheep β-actin was used as an internal reference gene.
Correlation Analysis
To further verify the function of lncRNAs in fatty acid metabolism of the LT muscle in Tibetan sheep at different ages, Pearson correlation analysis was performed between 10 lncRNAs and fatty acids with significant differences. In this study, a two-tailed test was used for correlation analysis.
Statistical Analysis
All statistical analyses were performed using IBM SPSS 22.0 (SPSS, Inc., Chicago, IL, USA). The differences between the mean values were compared using the Duncan's multiple range test (P < 0.05). Each experiment was replicated at least three times.
Results
IMF and Fatty Acid Profile in Tibetan Sheep Meat at Different Ages
Figure 1 shows the IMF and fatty acid content of Tibetan sheep at different ages. It seems that the IMF content in the LT muscles of Tibetan sheep increased first and then decreased with the growth of age (Figure 1A), it was higher of 1.5 y and 3.5 y than that of 4 m and 6 y (P < 0.05), and there was no significant difference between 1.5 y and 3.5 y (P > 0.05) and 4 m and 6 y (P > 0.05). A total of 26 fatty acids were detected in the LT muscle of Tibetan sheep at different ages (Figure 1B). The SFA content of 4 m and 1.5 y sheep was lower than the other two groups (P < 0.05), and there was no significant difference between 4 m and 1.5 y (P > 0.05), while SFA contents increased obviously at 3.5 y (P = 0.032). The content of monounsaturated fatty acid (MUFA), C14:1, C17:1, and C18:2n6t in Tibetan sheep meat of 1.5 y was significantly higher than that of other groups (P < 0.05), and it was also rich in polyunsaturated fatty acids.
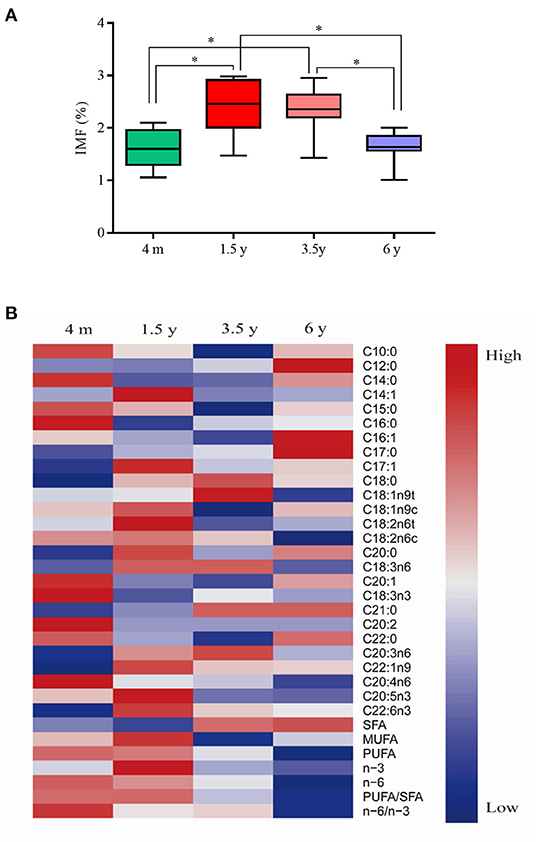
Figure 1. Intramuscular fat (IMF) and fatty acid content in Tibetan sheep meat at different ages. (A) Box plot of IMF content in Tibetan sheep meat at different ages. (B) Heat map of fatty acid composition in Tibetan sheep meat at different ages. *Difference is significant at P < 0.05.
Quality Analysis of RNA-Seq Data
In this study, sixteen libraries were using the LT muscle tissues from four growth stages of Tibetan sheep. The summary statistics of the RNA-Seq data are shown in Table 2. The average of 91,167,071, 95,150,638, 90,313,673, and 87,472,429 raw reads were generated from four growth stages, respectively, after lower-quality reads were filtered, and more than 87 million clean reads were generated from each age. The correlation coefficient between four samples within 4 m, 1.5 y, 3.5 y, and 6.0 y of Tibetan sheep were 0.983, 0.890, 0.962, and 0.990, respectively. After removing the rRNA mapped reads, more than 86 million remaining clean reads were generated for each group. Of the remaining clean reads, more than an average of 82 million (92.04%) were mapped to the reference genome, and more than 74 million (91.75%) were unique mapped reads.
The mapping sequence of each library was reconstructed and assembled using StringTie software, and a total of 6,131 transcripts were obtained. The intersection of CPC2 and CNCI predicted that nonprotein-coding potential results were chosen as novel lncRNAs. A total of 890 novel lncRNAs were identified in this study (Figure 2A). According to the position of lncRNA in the genome relative to the protein-coding gene, lncRNAs were classified into five classes, namely, intergenic lncRNAs, bidirectional lncRNAs, intronic lncRNAs, antisense lncRNAs, and sense overlapping lncRNAs. The number of known lncRNAs and novel lncRNAs, i.e., 2,448 and 403, respectively, were the largest in the intergenic region (Figure 2B).
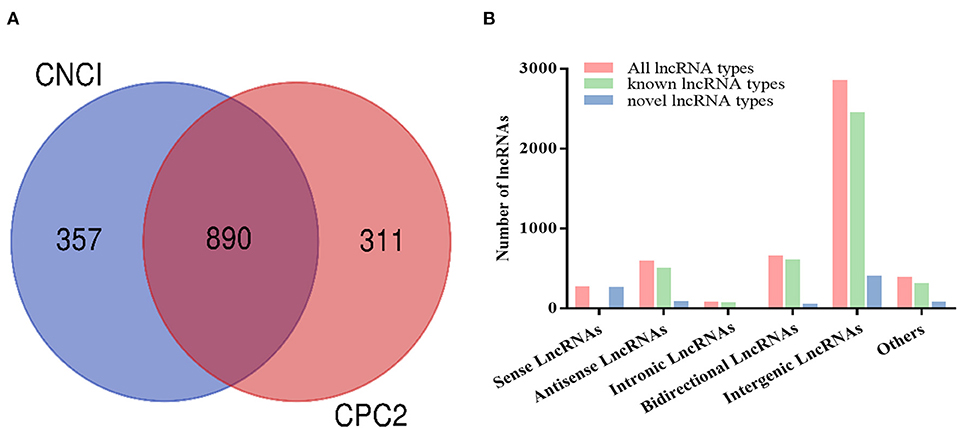
Figure 2. (A) Identification of 890 novel long noncoding RNAs (lncRNAs) without protein-coding potential evaluated by CNCI and CPC2. (B) LncRNA type distribution.
Identification and Characteristics of lncRNAs During Intramuscular Lipid Metabolism in Tibetan Sheep
To comprehensively explore the characteristics of lncRNAs during fat and fatty acid metabolism of Tibetan sheep meat, transcript length, the exon number, and open reading frame (ORF) length to explore differences of known and novel lncRNAs and mRNAs were characterized, as shown in Figures 3A–C. Similar to lncRNAs, the transcript length and ORF length of novel lncRNAs were significantly shorter than those of the mRNA transcript. In addition, the number of exons was also less than that of mRNA (P < 0.05). The expression level of lncRNA was much lower than that of mRNA (Figure 3D). The expression level (FPKM) of novel lncRNAs was higher than that of known lncRNAs (P < 0.001) but lower than that of mRNAs (P > 0.05) (Figure 3E). The novel lncRNAs possessed a coding potential score similar to that of known lncRNAs and a lower coding potential score than that of mRNAs (P < 0.001) (Figure 3F).
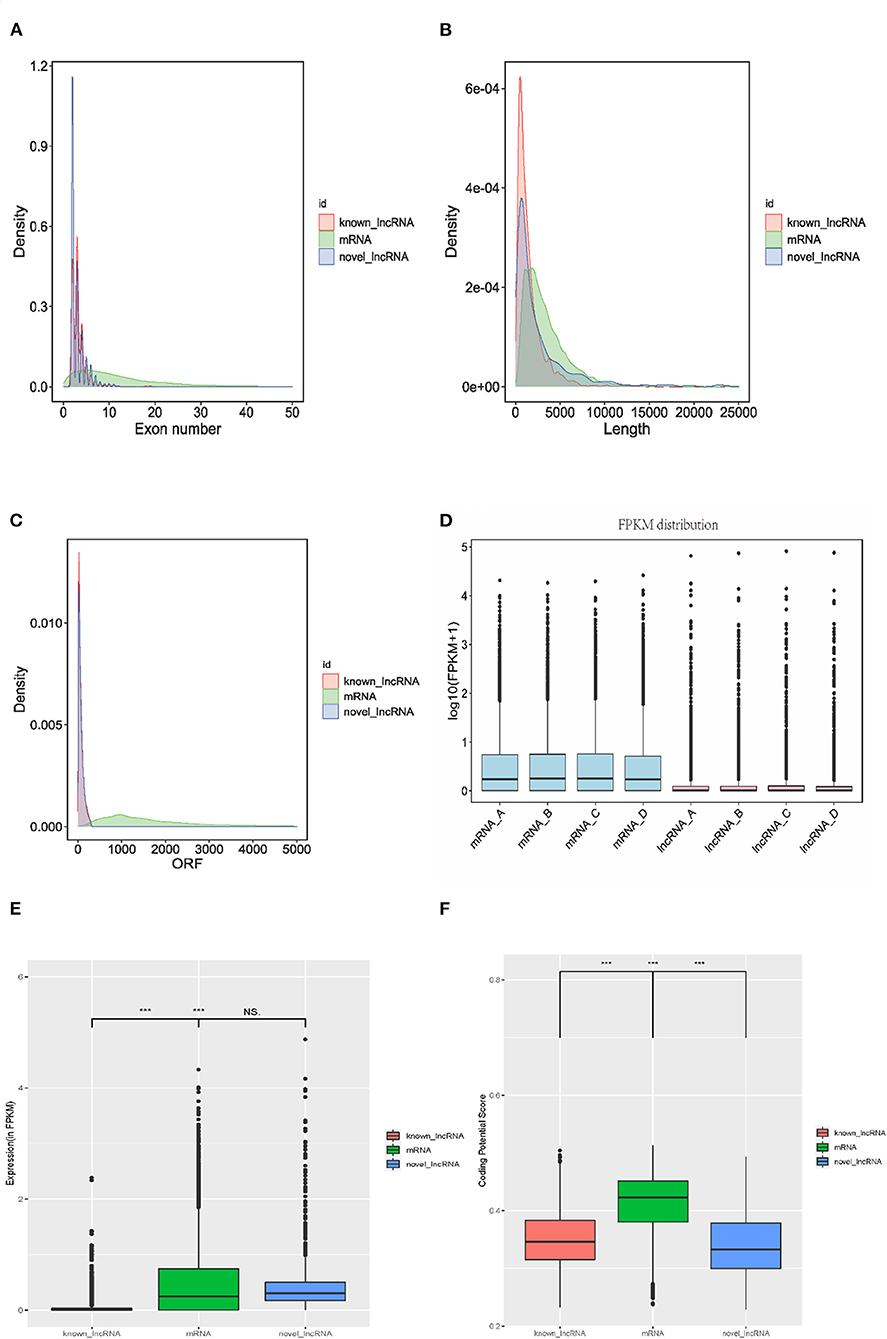
Figure 3. Characteristics of identified lncRNAs. (A) Distribution of exon numbers of mRNA, known lncRNAs, and novel lncRNAs. (B) Distribution of transcript lengths of mRNA, known lncRNAs, and novel lncRNAs. (C) Distribution of open reading frame (ORF) length of mRNA, known lncRNAs, and novel lncRNAs. (D) Box plot of log10 (FPKM+1) expression levels of lncRNA and mRNA in Tibetan sheep. mRNA_A, mRNA_B, mRNA_C, and mRNA_D was mRNA in 4-month-old, 4 m; 1.5-year-old, 1.5 y; 3.5-year-old, 3.5 y; and 6-year-old, 6 y of Tibetan sheep, respectively. lncRNA_A, lncRNA_B, lncRNA_C, and lncRNA_D were lncRNA in 4 m, 1.5 y, 3.5 y, and 6 y of Tibetan sheep, respectively. (E) Comparison of the expression levels of mRNA (green), known lncRNAs (red), and novel lncRNAs (blue), plotted as Fragments Per Kilobase of exon per Million fragments mapped (FPKM). ***P < 0.001; NS, no significant difference. (F) Coding potential scores of mRNAs, known lncRNAs, and novel lncRNAs calculated by coding potential calculator (CPC). ***P < 0.001.
DE lncRNAs During Intramuscular Lipid Metabolism in Tibetan Sheep
To find lncRNAs that were related to lipid metabolism, we compared the lncRNA expression levels of LT muscle of Tibetan sheep at different ages. Overall, 116 (65 upregulated; 51 downregulated), 39 (22 upregulated; 17 downregulated), 37 (17 upregulated; 20 downregulated), and 168 (85 upregulated; 83 downregulated) DE lncRNAs were identified in 4 m vs. 1.5 y, 1.5 y vs. 3.5 y, 3.5 y vs. 6 y, and 4 m vs. 6 y groups, respectively (Figure 4A). There was a lncRNA co-expressed in four contiguous period transcriptome comparative groups (Figure 4B). In addition, the heat map results of DE lncRNAs showed that there were obvious differences in lncRNA among the four ages (Figure 4C). The results demonstrated that the differences in lipid metabolism in the muscles of Tibetan sheep at different ages might be caused by these DE lncRNAs.
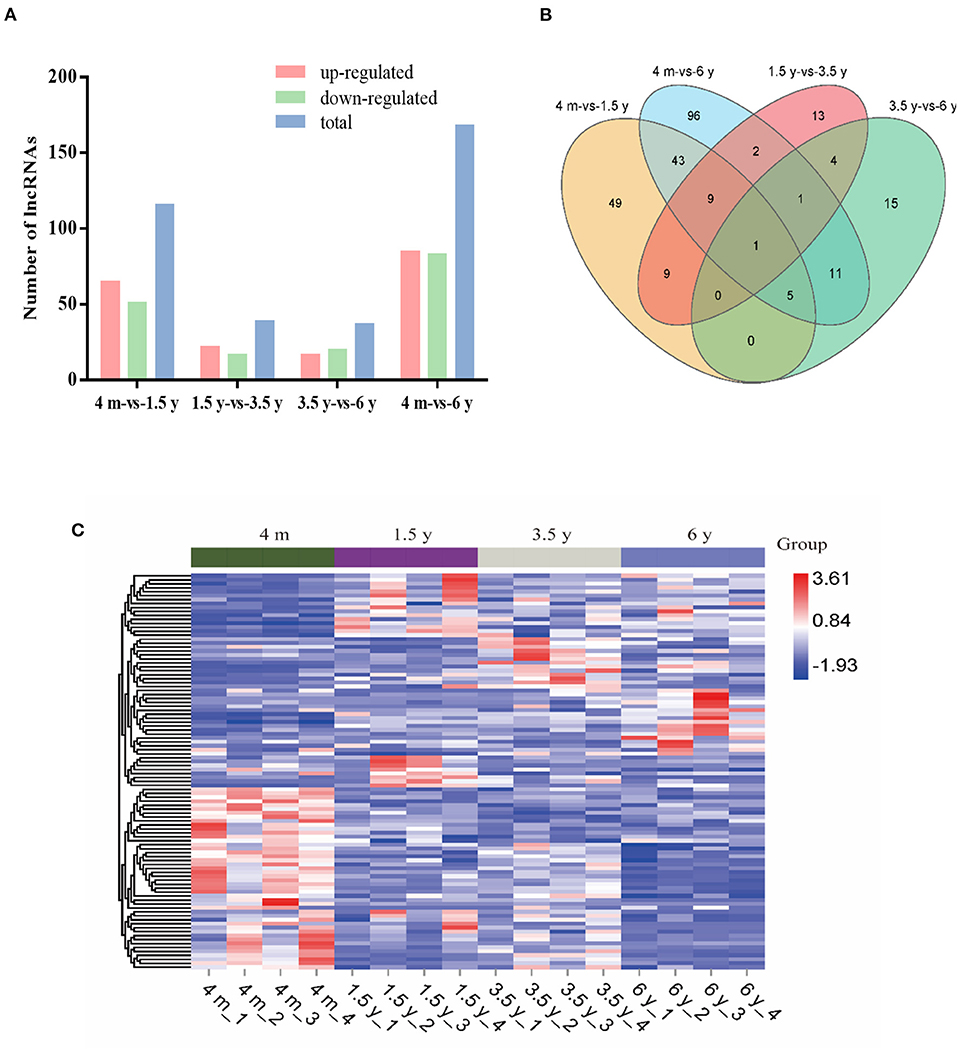
Figure 4. Analysis of the differentially expressed (DE) lncRNAs in the muscle of Tibetan sheep at different ages. (A) Statistical histogram of DE lncRNA in four contiguous period transcriptome comparative groups. (B) The Venn diagrams of the shared and unique differential lncRNAs in the four comparative groups. (C) Cluster heat map of DE lncRNA expression. Red means upregulation and blue means downregulation.
Functional Characterization of DE lncRNAs During Intramuscular Lipid Metabolism in Tibetan Sheep
To systematically study the functions of lncRNA and fatty acid metabolism in LT muscle of Tibetan sheep, the target genes of DE lncRNA were analyzed. In total, 39 significantly different cis-mRNA pairs and 62 significantly different trans-mRNA pairs were identified. To further analyze the potential function of DE lncRNA in regulating lipid metabolism in Tibetan sheep LT muscle, GO function annotation and KEGG enrichment analysis were performed on cis-regulated and trans-regulated target genes, respectively. The results indicated that most of the target genes in the cis-mRNA pairs were significantly enriched into specific functional groups (P < 0.05), including those that were related to muscle development, external stimuli, and immune (Figure 5A). GO term involved in the function of muscle structure development (GO:0061061) and antibacterial peptide production (GO:0002778). Most of the target genes in the trans-mRNA pairs were significantly enriched into protein modification and defense functions (P < 0.05) (Figure 5B). GO term involved in the function of protein palmitoylation (GO:0018345) and defense response to fungus (GO:0050832). These enriched functions were all related to muscle development.
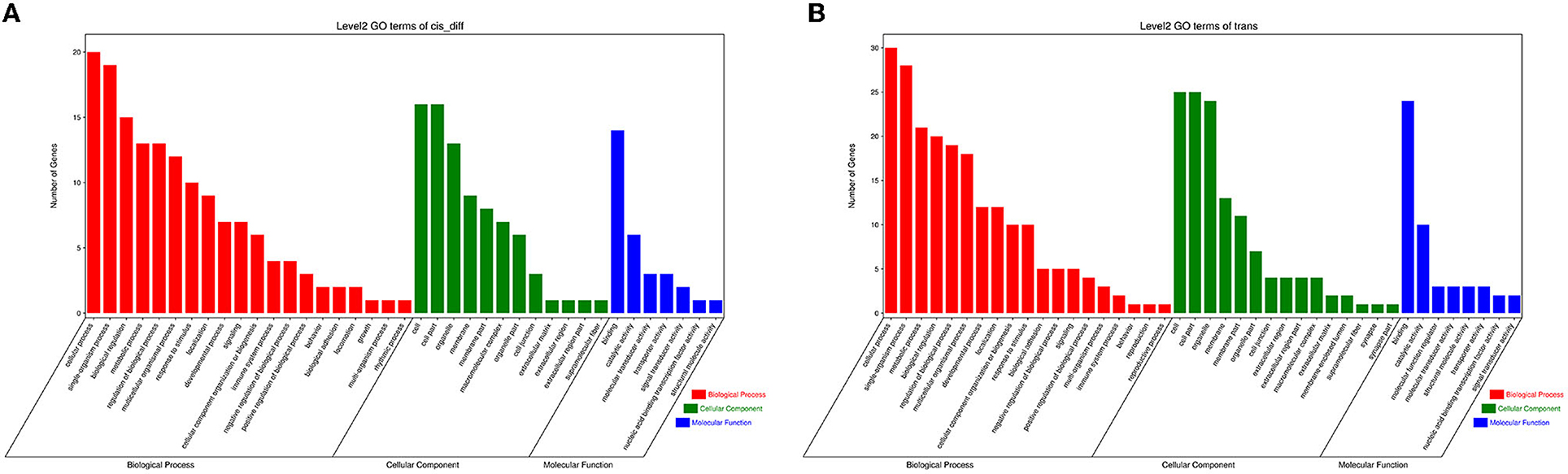
Figure 5. Histogram of DE lncRNA target genes of gene ontology (GO) functional annotation. The GO annotation included biological process (BP), molecular function (MF), and cellular component (CC). (A) The target genes in the cis-mRNA pairs. (B) The target genes in the trans-mRNA pairs.
The KEGG pathway was used to further analyze the potential functional signaling pathways of target genes in the four compare groups of the LT muscle of Tibetan sheep (Table 3), which is a substitute method of gene function classification focusing on biochemical pathways. The results showed that most of the target genes in the cis-mRNA pairs were significantly enriched in the immune system, development, and signal transduction (P < 0.05). However, most of the target genes in the trans-mRNA pairs were significantly enriched in the protein digestion and absorption and fatty acid biosynthesis (P < 0.05). FASN was significantly enriched in fatty acid biosynthesis (P < 0.05). The results demonstrated that DE lncRNA trans-regulate target genes played an important biological function in the LT muscle growth and development and intramuscular fatty acid metabolism of Tibetan sheep at different ages.
Construction of lncRNA-miRNA-mRNA Regulatory Network
To further analyze the biological functions of these DE lncRNAs, based on the ceRNA hypothesis mechanism, by integrating the results of our previous miRNA and mRNA sequencing data, a regulatory network of lncRNA-miRNA-mRNA was constructed. The ceRNA network contained 166 lncRNA-miRNA pairs and 250 miRNA-mRNA pairs and included 29 lncRNAs, 53 miRNAs, and 81 mRNAs (Figure 6). Among them, the ncbi_443090 (GPD2), ncbi_100169699 (lipase E hormone-sensitive enzyme (LIPE)), ncbi_101105870 (TFDP2), ncbi_443434 (CPT1A), ncbi_101114816 (ACACB), and ncbi_101111848 (ADIPOQ) and other mRNAs related to fatty acid and lipid metabolism were identified. Finally, some important regulatory relationships related to fatty acid metabolism were obtained through connectivity and correlation: MSTRG.41.1-miR-381-3p-GPD2, XR_001040849.2-miR-136-GPD2, XR_003590307.1-miR-485-3p-TFDP2, XR_003587341.1-miR-127-5p-TFDP2, MSTRG.1451.4-novel-m0170-3p-ACACB, XR_003585597.1-miR-376c-3p-ADIPOQ, MSTRG.5551.2-miR-105-5p-CPT1A, and XR_001041923.2-miR-412-5p-LIPE. We speculated that these lncRNAs regulated the corresponding miRNAs and further regulated genes related to fatty acids and IMF, which played an important biological role in fatty acid and fat metabolism in Tibetan sheep muscle.
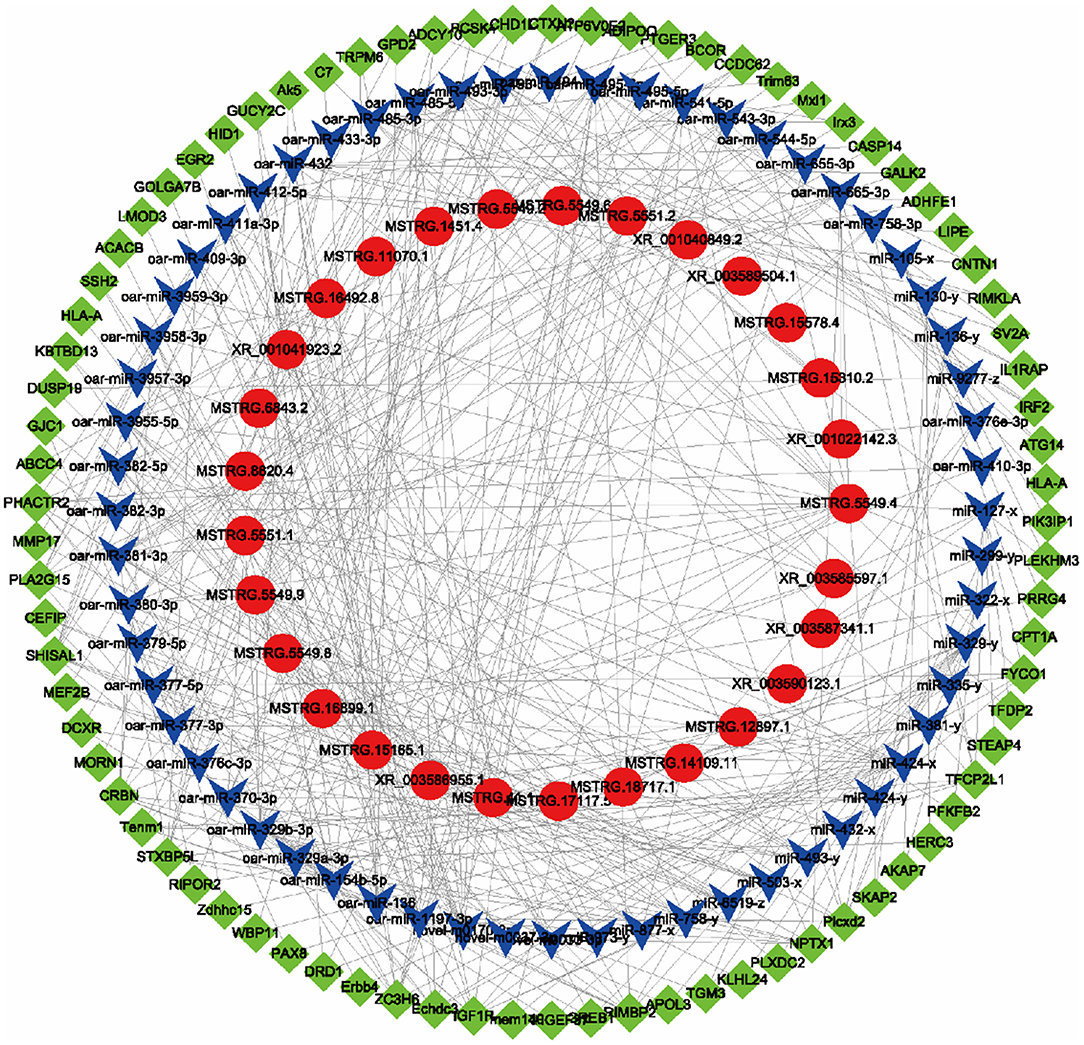
Figure 6. The lncRNA-miRNA-mRNA ceRNA regulatory network related to fat and fatty acid metabolism in Tibetan sheep muscle. The red circle represents lncRNAs. Blue V represents miRNAs. Green diamonds represent mRNAs.
GO Function Annotation and KEGG Enrichment Analysis of mRNA in ceRNA Network
To further analyze the potential function of mRNA in the ceRNA network in fatty acid and fat metabolism in Tibetan sheep muscle, we performed GO function annotation and KEGG enrichment analysis with 81 mRNAs in the ceRNA network. The results showed that most of the genes were enriched into specific functional groups, mainly including processes such as metabolism and protein modification (Figure 7A). GO term involved in the function of lipid metabolic process (GO:0019216), where ADIPOQ, and ACACB, and lipid metabolism-related genes were significantly enriched in this pathway (P < 0.05). In addition, it was also significantly enriched in the regulation of fatty acid metabolic process (GO:0019217) and cellular lipid metabolic process (GO:0044255) (P < 0.05). These functions were all related to lipid metabolism. The genes in these GO functions might further regulate fatty acid metabolism during the growth and development of Tibetan sheep. The results of KEGG enrichment analysis showed that the most significant enrichment was the AMPK signaling pathway (P = 0.0000112361) (Figure 7B), and LIPE, ADIPOQ, ACACB, and CPT1A were enriched in the AMPK signaling pathway (P < 0.05). Additionally, our previous study found that the AMPK signaling pathway was related to the transformation of muscle fiber types in LT muscles of Tibetan sheep (Figure 7C). Therefore, we speculated that these genes might be involved in the AMPK signaling pathway, changed the types of muscle fibers in Tibetan sheep muscles, and ultimately lead to differences in fatty acid composition and IMF content in LT muscles of Tibetan sheep (Figure 7D). In addition, the signaling pathways that were significantly enriched include the adipocytokine signaling pathway, PPAR signaling pathway, and fatty acid biosynthesis signaling pathway. These signaling pathways were related to lipid metabolism and further regulate fatty acid metabolism in LT muscle of Tibetan sheep at different ages.
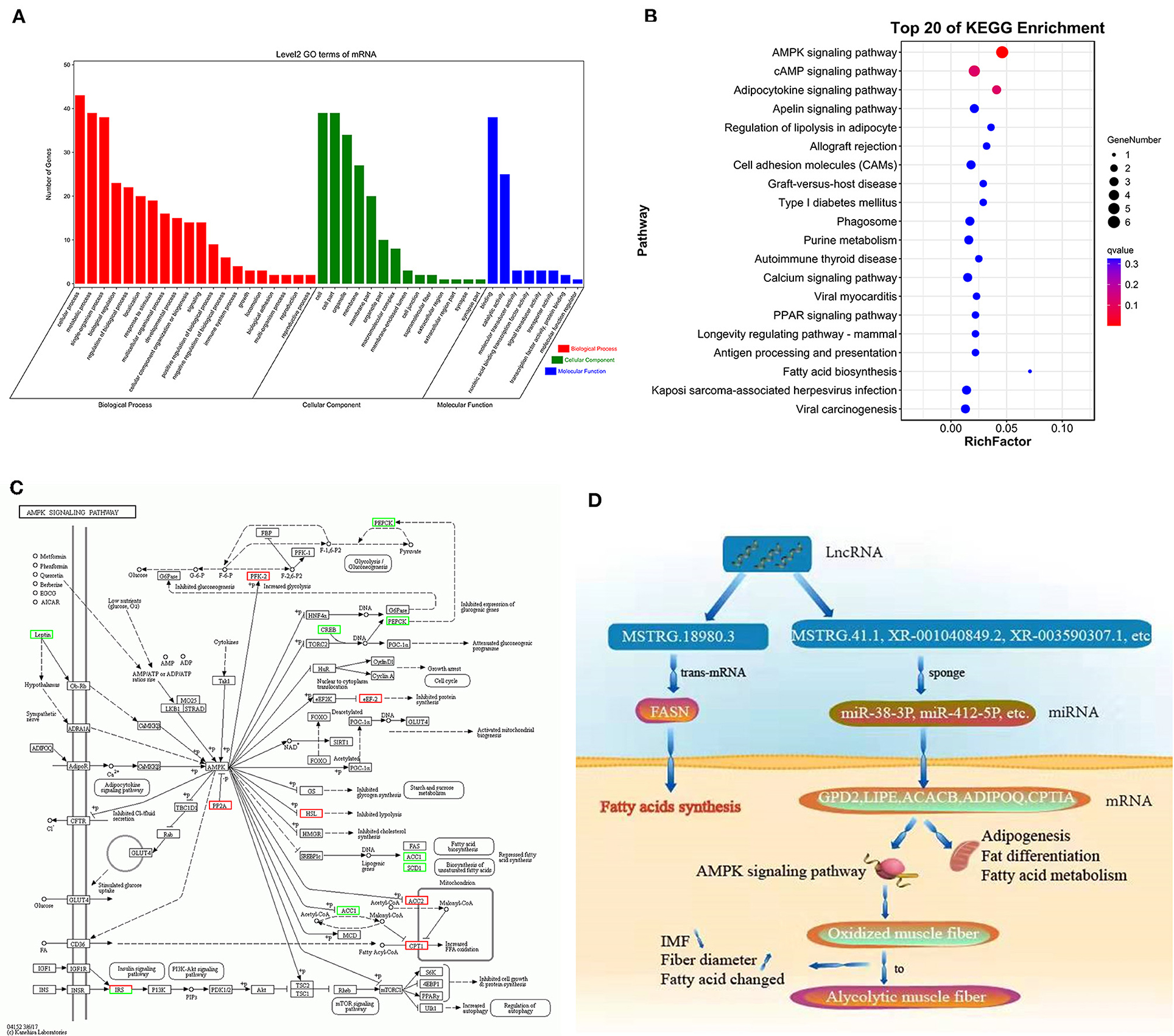
Figure 7. (A) Histogram of mRNA GO function annotated in ceRNA network. (B) Bubble chart of Kyoto encyclopedia of genes and genomes (KEGG) enrichment analysis. (C) AMPK signaling pathway during the growth and development of Tibetan sheep. (D) Model of lncRNA regulating lipid metabolism in Tibetan sheep.
Validation of lncRNA Expression by RT-qPCR
To verify the RNA-Seq results, 10 DE lncRNAs were randomly selected and verified by RT-qPCR (Figure 8). The RT-qPCR expression pattern of selected genes was consistent with the results of RNA-Seq analysis, and this demonstrated the reliability and accuracy of the RNA-Seq method used in this study.
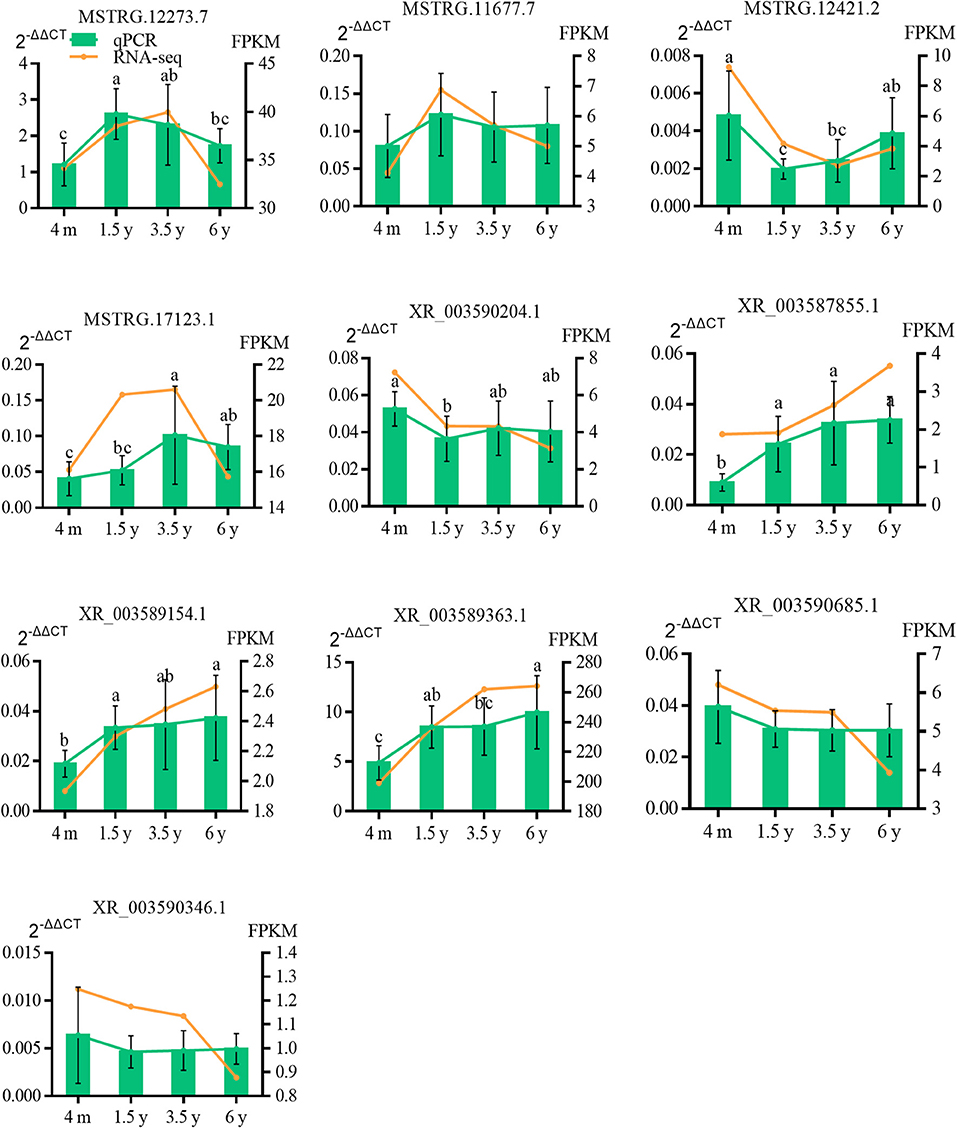
Figure 8. Comparison of the expression levels of lncRNA between RNA-Seq and RT-qPCR. RT-qPCR data were shown as the means ± S.D. Different letters (a–c) indicate significant difference between different ages (P < 0.05). 2−ΔΔCT was the result of RT-qPCR, and FPKM was the result of RNA-Seq.
The Relationship Between lncRNAs and Fatty Acids
In this study, fatty acids with significant differences were selected, and correlation analysis was performed with the 10 lncRNAs. It was found that there was a very significant correlation between most fatty acids and lncRNAs (Figure 9). Positive correlation was found between the C14:0 and MSTRG.12421.2 (0.63, P < 0.001), XR_003590204.1 (0.53, P < 0.001), while negative correlation was found between C14:0 and MSTRG.12273.7 (−0.67, P < 0.001), XR_003587855.1 (−0.50, P < 0.001). It was found between C16:0 and MSTRG.12421.2 (0.50, P < 0.001), while it was found between C16:0 and MSTRG.12273.7 (−0.56, P < 0.001). A positive correlation was found between C17:1 and MSTRG.12273.7 (0.59, P < 0.001); however, a negative correlation was found between C17:1 and MSTRG.12421.2 (−0.51, P < 0.001). A positive correlation was found between C18:0 and XR_003587855.1 (0.73, P < 0.001), and a negative correlation was also found between C20:4n6 and XR_003587855.1 (−0.73, P < 0.001).
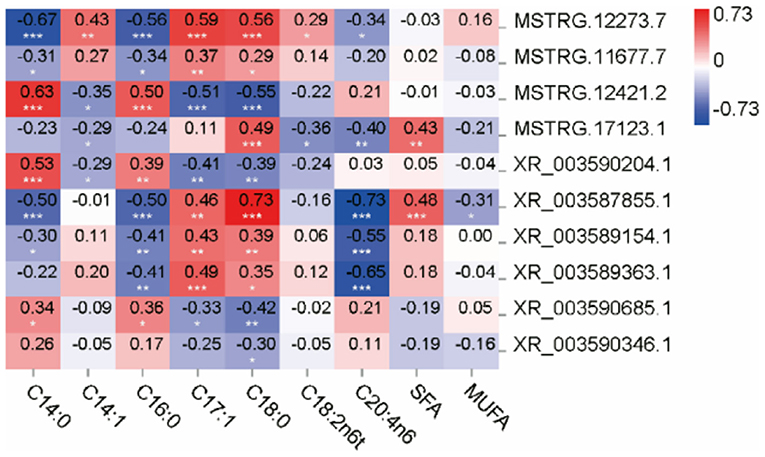
Figure 9. Pearson correlations between expression of lncRNAs and content of fatty acids in the muscle of Tibetan sheep. *Correlation is significant at P < 0.05, **correlation is significant at P < 0.01, and ***correlation is significant at P < 0.001.
Discussion
Lipid metabolism in animal skeletal muscle has a significant impact on metabolic energy and homeostasis. In addition, TMF and fatty acid profiles have positive impacts on flavor, juiciness, tenderness, and nutrients. Skeletal muscle accounts for 40–50% of animal body weight, and the skeletal muscle development process of mammal prenatal had been studied more clearly (34, 35). The skeletal muscle development of animal postnatal mainly involved an increase in muscle fiber diameter and transformation of muscle fiber types (36, 37). Additionally, oxidized muscle fibers gradually transformed into glycolytic muscle fibers with the increase of age. In this study, the IMF content of Tibetan sheep increased first and then decreased with the increase of age. In addition, it was largest at 1.5 y, which might be related to the transformation of oxidized muscle fibers into glycolytic muscle fibers. In addition, higher MUFA and PUFA contents were observed in 1.5 y of Tibetan sheep. The above results demonstrated that 1.5 y was a more suitable slaughter age of Tibetan sheep for a healthy human diet.
Fatty acids are important nutrients for human health and have important physiological functions. MUFA can reduce total cholesterol, enhance antioxidant enzyme activity, and reduce blood pressure and blood sugar level, preventing memory loss and promoting growth and development. It is of great significance for reducing cardiovascular diseases (38). More studies have demonstrated that lncRNA has a significant effect on lipid metabolism. Analysis of the functional role of lncRNA in the regulation of lipid and fatty acid metabolism is helpful to explore the mechanisms of lipid deposition and adipose tissue development.
In this study, we systematically analyzed the lncRNA profile related to fat and fatty acid metabolism in the LT muscles of Tibetan sheep at different growth and developmental stages. A total of 890 novel lncRNAs were identified, with similar characteristics to that of 3,943 known lncRNAs, in order to further analyze the molecular mechanism that caused the differences in IMF and fatty acids profile in the LT muscle growth and development of Tibetan sheep. We analyzed the DE lncRNAs in the four contiguous period transcriptome comparative groups, and a total of 360 DE lncRNAs were identified. KEGG enrichment analysis results found that DE lncRNA trans-regulated the target genes to regulate LT muscle development and fatty acid biosynthesis. In addition, COL13A1, COL11A1, COL22A1, and FASN were significantly enriched in muscle development-related signaling pathways and fatty acid biosynthesis pathway. Among them, COL13A1, COL11A1, and COL22A1 are important members of the collagen superfamily. Previous studies found that the mutation of COL13A1 during muscle development might cause myasthenia syndrome (39). Nallanthighal et al. (40) found that COL11A1 upregulated fatty acid β oxidation in ovarian cancer cells. In addition, COL11A1 had been demonstrated to enhance the expression of proteins involved in fatty acid synthesis and induce upregulation of fatty acid biosynthesis (40). Charvet et al. (41) found that knocking out COL22A1 caused zebrafish muscle weakness and contraction-induced fiber detachment (41). Moreover, FASN had also been significantly enriched in fatty acid biosynthesis signaling pathways, which are a fatty acid synthase and a key rate-limiting enzyme in the fatty acid synthesis process de novo (42). These studies confirmed that MSTRG.18980.3, XR_003585779.1 (LOC105606646), MSTRG.11734.2, and MSTRG.18980.3 trans-regulated COL13A1, COL11A1, COL22A1, and FASN, respectively, and were involved in LT muscle growth and development and fatty acid metabolism, which played an important biological function.
Previous studies have demonstrated that lncRNA could serve as a miRNA sponge to indirectly regulate the expression of downstream target genes of miRNA (43). Song et al. (44) found that lncRNA-KRTAP5-AS1 and lncRNA-TUBB2A could serve as competing endogenous RNA to influence the function of Claudin-4 (44). Therefore, we constructed the lncRNA-miRNA-mRNA ceRNA regulatory network to reveal the functions of these DE lncRNAs in IMF and fatty acid metabolism in Tibetan sheep muscle at different growth and development stages. A total of 81 mRNAs were identified in the ceRNA network. Among them, GPD2 could catalyze the esterification of fatty acids to triglycerides (45). In addition, GPD2 was potentially regulated by the MSTRG.41.1-miR-381-3p and XR_001040849.2-miR-136 pairs. The study about miR-381-3p mainly focuses on diseases. Previous studies suggested that miR-381-3p was a dual inhibitor of apoptosis and necrosis, and it could also regulate downstream target genes to inhibit smooth muscle growth of human (46). However, miR-136 may be related to fat metabolism, one of the evidence is that overexpression of miR-136 could inhibit the expression of LRH-1 and lead to dyslipidemia and liver steatosis (47). The results of this study showed that MSTRG.41.1-miR and XR_001040849.2 regulated miR-381-3p and miR-136, respectively, relieved the inhibition of GPD2 by miRNA, and played an important biological role in fat metabolism. TFDP2 blocked the differentiation of adipocytes by inhibiting the binding of CEBPA to the promoters of target genes (48). TFDP2 was potentially regulated by the relationship pair of XR_003590307.1-miR-485-3p and XR_003587341.1-miR-127-5p in this study. There were many studies on miR-485-3p in diseases. Previous studies showed that miR-485-3p was involved in the regulation, apoptosis, and migration of metastatic tumors. Overexpression of miR-485-3p in fibroblasts could promote fibrosis (49). Ji et al. (50) demonstrated that miR-127-5p plays an important regulatory role in fat deposition by regulating downstream target genes (50). CPT1A is a key enzyme for carnitine-dependent transport across the inner mitochondrial membrane. Lack of CPT1A would reduce the rate of fatty acid oxidation (51). MSTRG.5551.2 acted as a miR-105-5p sponge to further regulate CPT1A in this study. miR-105-5p played an important role in regulating the proliferation, migration, and apoptosis of cancer cells (52). ACACB is acetyl-CoA carboxylase β and played an important role in fatty acid metabolism (53). ACACB was potentially regulated by the MSTRG.1451.4-novel-m0170-3p relationship pair in this study. The novel-m0170-3p was a novel miRNA identified, and there was no study on novel-m0170-3p. ADIPOQ is related to lipid metabolism and adipocyte differentiation (54, 55). ADIPOQ was potentially regulated by the XR_003585597.1-miR-376c-3p relationship pair in this study. Zhang et al. (56) found that LncRNA-LINC00152 was downregulated by miR-376c-3p to further limit the viability of rectal cancer cells and promote cell apoptosis (56). In addition, LIPE is a kind of lipid decomposing enzyme, which plays a key role in regulating the deposition of adipose tissue (57). The LIPE gene was potentially regulated by the XR_001041923.2-miR-412-5p relationship pair in this study. There were few studies on the lipid metabolism of miR-412-5p; however, previous studies suggested that the inactivation of miR-412-5p in vascular endothelial cells lead to the high expression of Xpo1 and the inhibition of the p53-p66SHC-p16 pathway, which ultimately promoted the formation of vascular endothelial cells and hemorrhoid blood vessels (58). These genes were involved in fatty acid and lipid metabolism during the growth and development of Tibetan sheep.
Long noncoding RNA acts as a sponge of miRNA and played an important role in the process of lipid metabolism. The enrichment analysis of mRNA in the ceRNA regulatory network found that the AMPK signaling pathway was most significantly enriched. There have been numerous reports on the function of the AMPK signaling pathway. van der Vaart et al. (59) found that AMPK signaling played an important role in brown adipose tissue activation (59). Dan et al. (60) found AMPK signaling and inhibiting complex I in the mitochondria, leading to a reduction in mitochondrial respiration and elevated ATP production (60). In addition, the latest studies found that the AMPK signaling pathway played a key role in the regulation of skeletal muscle fiber type transformation (61, 62). Therefore, combined with our previous study, we speculated that the oxidized muscle fibers gradually transformed into glycolytic muscle fibers in Tibetan sheep muscle were the direct cause of poor meat quality. Moreover, it was significantly enriched into adipocytokine signaling pathway, PPAR signaling pathway, and fatty acid biosynthesis signal pathway.
The function of lncRNA in fatty acid metabolism was estimated through the correlation analysis between lncRNA and fatty acid. Negative correlations were found between XR_003589363.1 (RPS28) and C20:4n6 (r = −0.65, P < 0.001), and positive correlations were found between XR_003589363.1 (RPS28) and C17:1 (r = 0.49, P < 0.001). RPS28 is a kind of Ribosomal proteins that regulate protein biosynthesis and may be associated with muscle development. Jiao et al. (63) found that Rps28a is regulating the levels of a subset of proteins with known antiaging roles in skeletal muscle, while there was less study on fatty acid metabolism (63). The above studies demonstrated that lncRNA played a direct or indirect biological role in the metabolism of lipids and fatty acids in the LT muscles of Tibetan sheep during growth and development. However, these potential regulatory mechanisms still need to be further studied.
Conclusion
In summary, the results of this study indicated that the higher MUFA and PUFA content were observed in 1.5 y of Tibetan sheep, which demonstrated that 1.5 y was a more suitable slaughter age of Tibetan sheep for a healthy human diet. Furthermore, the reasons for this difference through the deep sequencing and bioinformatic analysis of the skeletal muscles of Tibetan sheep at different growth and development stages were analyzed. The results showed that DE lncRNA trans-regulated FASN, to regulate fatty acid metabolism during the growth and development of Tibetan sheep. Moreover, lncRNA also acted as a miRNA sponge, regulated GPD2, TFDP2, LIPE, CPT1A, ACACB, and ADIPOQ, and played an important biological role in lipid and fatty acid metabolism. Among them, LIPE, ADIPOQ, ACACB, and CPT1A changed the formation of energy metabolism in skeletal muscle through the AMPK signaling pathway, and oxidized muscle fibers were gradually transformed into glycolytic muscle fibers, which caused an increase in Tibetan sheep muscle fiber diameter and reduction in the IMF content, meat tenderness, and meat juiciness. In addition, it also changed the metabolism of fatty acid composition, resulting in differences in fatty acid profiles in Tibetan sheep muscles at different growth stages.
Data Availability Statement
The datasets presented in this study can be found in online repositories. The names of the repository/repositories and accession number(s) can be found in the article/supplementary material.
Ethics Statement
The animal study was reviewed and approved by the Faculty Animal Policy and Welfare Committee of Gansu Agricultural University (Ethic approval file No. GSAU-Eth-AST-2021-001).
Author Contributions
GB did the data analysis and wrote the manuscript. SL and FZ performed the investigation and collected the samples. JW, XL, JH, BS, YW, and LZ performed the formal analysis, methodology, and software. YL and SL did the project administration and revised the manuscript. All authors contributed to this study and approved the submitted version.
Funding
This study was supported by the fund of Distinguished Young Scholars Fund of Gansu Province (21JR7RA857), the Fuxi Young Talents Fund of Gansu Agricultural University (Gaufx-03Y04), the Projects of Gansu Agricultural University (GSAU-ZL-2015-033), and the Key R&D Projects in Gansu Province (18YF1WA082).
Conflict of Interest
The authors declare that the research was conducted in the absence of any commercial or financial relationships that could be construed as a potential conflict of interest.
Publisher's Note
All claims expressed in this article are solely those of the authors and do not necessarily represent those of their affiliated organizations, or those of the publisher, the editors and the reviewers. Any product that may be evaluated in this article, or claim that may be made by its manufacturer, is not guaranteed or endorsed by the publisher.
References
1. Bertol TM, de Campos RM, Ludke JV, Terra NN, de Figueiredo EA, Coldebella A, et al. Effects of genotype and dietary oil supplementation on performance, carcass traits, pork quality and fatty acid composition of backfat and intramuscular fat. Meat Sci. (2013) 93:507–16. doi: 10.1016/j.meatsci.2012.11.012
2. Jung JH, Shim KS, Na CS, Choe HS. Studies on intramuscular fat percentage in live swine using real-time ultrasound to determine pork quality. Asian Austral J Anim. (2015) 28:318–22. doi: 10.5713/ajas.14.0927
3. Zhang XJ, Chen MM, Liu XF, Zhang LL, Ding XB, Guo YW, et al. A novel lncRNA, lnc403, involved in bovine skeletal muscle myogenesis by mediating KRAS/Myf6. Gene. (2020) 751:144706. doi: 10.1016/j.gene.2020.144706
4. Li R, Li B, Jiang A, Cao Y, Hou L, Zhang Z, et al. Exploring the lncRNAs related to skeletal muscle fiber types and meat quality traits in pigs. Genes. (2020) 11:883. doi: 10.3390/genes11080883
5. Ryu YC, Kim BC. The relationship between muscle fiber characteristics, postmortem metabolic rate, and meat quality of pig longissimus dorsi muscle. Meat Sci. (2005) 71:351–7. doi: 10.1016/j.meatsci.2005.04.015
6. Anne L, Gagaoua M, Andueza D, Gruffat D, Hocquette JF. What are the drivers of beef sensory quality using metadata of intramuscular connective tissue, fatty acids and muscle fiber characteristics? Livest Sci. (2020) 240:104209. doi: 10.1016/j.livsci.2020.104209
7. Han H, Wei W, Chu W, Liu K, Tian Y, Jiang Z, et al. Muscle conditional medium reduces intramuscular adipocyte differentiation and lipid accumulation through regulating insulin signaling. Int J Mol Sci. (2017) 18:1799. doi: 10.3390/ijms18081799
8. Currie E, Schulze A, Zechner R, Walther TC, Farese R Jr. Cellular fatty acid metabolism and cancer. Cell Metab. (2013) 18:153–61. doi: 10.1016/j.cmet.2013.05.017
9. Cui XY, Zhan JK, Liu YS. Roles and functions of antisense lncRNA in vascular aging. Ageing Res Rev. (2021) 72:101480. doi: 10.1016/j.arr.2021.101480
10. Guttman M, Rinn JL. Modular regulatory principles of large non-coding RNAs. Nature. (2012) 482:339–46. doi: 10.1038/nature10887
11. Zhang M, Ma X, Zhai Y, Zhang D, Sui L, Li W, et al. Comprehensive transcriptome analysis of lncRNAs reveals the role of lncAD in chicken intramuscular and abdominal adipogenesis. J Agr Food Chem. (2020) 68:3678–88. doi: 10.1021/acs.jafc.9b07405
12. Yu X, Zhang Y, Li T, Ma Z, Jia H, Chen Q, et al. Long non-coding RNA Linc-RAM enhances myogenic differentiation by interacting with MyoD. Nat Commun. (2017) 8:14016. doi: 10.1038/ncomms14016
13. Fatica A, Bozzoni I. Long non-coding RNAs: new players in cell differentiation and development. Nat Rev Genet. (2014) 15:7–21. doi: 10.1038/nrg3606
14. Song C, Wang J, Ma Y, Yang Z, Dong D, Li H, et al. Linc-smad7 promotes myoblast differentiation and muscle regeneration via sponging miR-125b. Epigenetics. (2018) 13:591–604. doi: 10.1080/15592294.2018.1481705
15. Zhou J, Xu J, Zhang L, Liu S, Ma Y, Wen X, et al. Combined single-cell profiling of lncRNAs and functional screening reveals that H19 is pivotal for embryonic hematopoietic stem cell development. Cell Stem Cell. (2019) 24:285–98.e285. doi: 10.1016/j.stem.2018.11.023
16. Zhang M, Li F, Sun JW Li DH, Li WT, Jiang RR, et al. LncRNA IMFNCR promotes intramuscular adipocyte differentiation by sponging miR-128-3p and miR-27b-3p. Front Genet. (2019) 10:42. doi: 10.3389/fgene.2019.00042
17. Wu H, Liu B, Chen Z, Li G, Zhang Z. MSC-induced lncRNA HCP5 drove fatty acid oxidation through miR-3619-5p/AMPK/PGC1α/CEBPB axis to promote stemness and chemo-resistance of gastric cancer. Cell Death Dis. (2020) 11:233. doi: 10.1038/s41419-020-2426-z
18. Zhang B, Yan Z, Wang P, Yang Q, Huang X, Shi H, et al. Identification and characterization of lncRNA and mRNA in testes of landrace and hezuo boars. Animals. (2021) 11:2263. doi: 10.3390/ani11082263
19. Cesana M, Cacchiarelli D, Legnini I, Santini T, Sthandier O, Chinappi M, et al. A long noncoding RNA controls muscle differentiation by functioning as a competing endogenous RNA. Cell. (2011) 147:358–69. doi: 10.1016/j.cell.2011.09.028
20. Jiang R, Li H, Huang Y, Lan X, Lei C, Chen H. Transcriptome profiling of lncRNA related to fat tissues of Qinchuan cattle. Gene. (2020) 742:144587. doi: 10.1016/j.gene.2020.144587
21. Yang L, Li P, Yang W, Ruan X, Kiesewetter K, Zhu J, et al. Integrative transcriptome analyses of metabolic responses in mice define pivotal lncRNA metabolic regulators. Cell Metab. (2016) 24:627–39. doi: 10.1016/j.cmet.2016.08.019
22. Yue B, Li H, Liu M, Wu J, Li M, Lei C, et al. Characterization of lncRNA-miRNA-mRNA network to reveal potential functional ceRNAs in bovine skeletal muscle. Front Genet. (2019) 10:91. doi: 10.3389/fgene.2019.00091
23. AOAC. Official Methods of Analysis, 18th ed. Gaithersburg, MD, USA: Association of official analytical chemists (2007).
24. Gao Y, Li M, Zhang L, Wang Z, Han L. Preparation of rapeseed oil oleogels based on beeswax and its application in beef heart patties to replace animal fat. LWT. (2021). doi: 10.1016/j.lwt.2021.111986
25. Chen S, Zhou Y, Chen Y, Gu J. fastp: an ultra-fast all-in-one FASTQ preprocessor. Bioinformatics. (2018) 34:i884–90. doi: 10.1093/bioinformatics/bty560
26. Langmead B, Salzberg SL. Fast gapped-read alignment with Bowtie 2. Nat Methods. (2012) 9:357–9. doi: 10.1038/nmeth.1923
27. Kim D, Langmead B, Salzberg SL. HISAT: a fast spliced aligner with low memory requirements. Nat Methods. (2015) 12:357–60. doi: 10.1038/nmeth.3317
28. Trapnell C, Williams BA, Pertea G, Mortazavi A, Kwan G, van Baren MJ, et al. Transcript assembly and quantification by RNA-Seq reveals unannotated transcripts and isoform switching during cell differentiation. Nat Biotechnol. (2010) 28:511–5. doi: 10.1038/nbt.1621
29. Pertea M, Pertea GM, Antonescu CM, Chang TC, Mendell JT, Salzberg SL. StringTie enables improved reconstruction of a transcriptome from RNA-seq reads. Nat Biotechnol. (2015) 33:290–5. doi: 10.1038/nbt.3122
30. Sun L, Luo H, Bu D, Zhao G, Yu K, Zhang C, et al. Utilizing sequence intrinsic composition to classify protein-coding and long non-coding transcripts. Nucleic Acids Res. (2013) 41:e166. doi: 10.1093/nar/gkt646
31. Kong L, Zhang Y, Ye ZQ, Liu XQ, Zhao SQ, Wei L, et al. CPC: assess the protein-coding potential of transcripts using sequence features and support vector machine. Nucleic Acids Res. (2007) 35:345–9. doi: 10.1093/nar/gkm391
32. Li B, Dewey CN. RSEM: accurate transcript quantification from RNA-Seq data with or without a reference genome. BMC Bioinformatics. (2011) 12:323. doi: 10.1186/1471-2105-12-323
33. Kanehisa M, Araki M, Goto S, Hattori M, Hirakawa M, Itoh M, et al. KEGG for linking genomes to life and the environment. Nucleic Acids Res. (2008) 36:480–4. doi: 10.1093/nar/gkm882
34. Du M, Wang B, Fu X, Yang Q, Zhu MJ. Fetal programming in meat production. Meat Sci. (2015) 109:40–7. doi: 10.1016/j.meatsci.2015.04.010
35. Oksbjerg N. New aspects of meat quality || Myogenesis and muscle growth and meat quality (2017).
36. Hopkins DL, Stanley DF, Martin LC, Toohey ES, Gilmour AR. Genotype and age effects on sheep meat production 3. meat quality. Aust J Exp Agr. (2007) 47:1155–64. doi: 10.1071/EA06299
37. Kim GD, Ryu YC, Jo C, Lee JG, Yang HS, Jeong JY, et al. The characteristics of myosin heavy chain-based fiber types in porcine longissimus dorsi muscle. Meat Sci. (2014) 96:712–8. doi: 10.1016/j.meatsci.2013.09.028
38. Legako JF, Dinh TT, Miller MF, Brooks JC. Effects of USDA beef quality grade and cooking on fatty acid composition of neutral and polar lipid fractions. Meat Sci. (2015) 100:246–55. doi: 10.1016/j.meatsci.2014.10.013
39. Beeson D, Cossins J, Rodriguez Cruz PM, Maxwell S, Liu WW, Palace J. Myasthenic syndromes due to defects in COL13A1 and in the N-linked glycosylation pathway. Ann Ny Acad Sci. (2018) 1413:163–9. doi: 10.1111/nyas.13576
40. Nallanthighal S, Rada M, Heiserman JP, Cha J, Sage J, Zhou B, et al. Inhibition of collagen XI alpha 1-induced fatty acid oxidation triggers apoptotic cell death in cisplatin-resistant ovarian cancer. Cell Death Dis. (2020) 11:258. doi: 10.1038/s41419-020-2442-z
41. Charvet B, Guiraud A, Malbouyres M, Zwolanek D, Guillon E, Bretaud S, et al. Knockdown of col22a1 gene in zebrafish induces a muscular dystrophy by disruption of the myotendinous junction. Development. (2013) 140:4602–13. doi: 10.1242/dev.096024
42. Morris CA, Cullen NG, Glass BC, Hyndman DL, Manley TR, Hickey SM, et al. Fatty acid synthase effects on bovine adipose fat and milk fat. Mamm Genome. (2007) 18:64–74. doi: 10.1007/s00335-006-0102-y
43. Du Z, Sun T, Hacisuleyman E, Fei T, Wang X, Brown M, et al. Integrative analyses reveal a long noncoding RNA-mediated sponge regulatory network in prostate cancer. Nat Commun. (2016) 7:10982. doi: 10.1038/ncomms10982
44. Song YX, Sun JX, Zhao JH, Yang YC, Shi JX, Wu ZH, et al. Author correction: non-coding RNAs participate in the regulatory network of CLDN4 via ceRNA mediated miRNA evasion. Nat Commun. (2021) 12:3149. doi: 10.1038/s41467-021-23211-y
45. Sadri H, Mielenz M, Morel I, Bruckmaier RM, van Dorland HA. Plasma leptin and mRNA expression of lipogenesis and lipolysis-related factors in bovine adipose tissue around parturition. J Anim Physiol Anim Nutr. (2011) 95:790–7. doi: 10.1111/j.1439-0396.2010.01111.x
46. Zhao C, Zhou Y, Ran Q, Yao Y, Zhang H, Ju J, et al. MicroRNA-381-3p functions as a dual suppressor of apoptosis and necroptosis and promotes proliferation of renal cancer cells. Front Cell Dev Biol. (2020) 8:290. doi: 10.3389/fcell.2020.00290
47. Góralska J, Razny U, Polus A, Dziewońska A, Gruca A, Zdzienicka A, et al. Enhanced GIP secretion in obesity is associated with biochemical alteration and miRNA contribution to the development of liver steatosis. Nutrients. (2020) 12:476. doi: 10.3390/nu12020476
48. Zaragoza K, Bégay V, Schuetz A, Heinemann U, Leutz A. Repression of transcriptional activity of C/EBPalpha by E2F-dimerization partner complexes. Mol Cell Biol. (2010) 30:2293–304. doi: 10.1128/MCB.01619-09
49. Solé C, Domingo S, Ferrer B, Moliné T, Ordi-Ros J, Cortés-Hernández J. MicroRNA expression profiling identifies miR-31 and miR-485-3p as regulators in the pathogenesis of discoid cutaneous lupus. J Invest Dermatol. (2019) 139:51–61. doi: 10.1016/j.jid.2018.07.026
50. Ji H, Wang H, Ji Q, Ji W, Luo X, Wang J, et al. Differential expression profile of microRNA in yak skeletal muscle and adipose tissue during development. Genes Genom. (2020) 42:1347–59. doi: 10.1007/s13258-020-00988-8
51. Briant LJB, Dodd MS, Chibalina MV, Rorsman NJG, Johnson PRV, Carmeliet P, et al. CPT1a-dependent long-chain fatty acid oxidation contributes to maintaining glucagon secretion from pancreatic islets. Cell Rep. (2018) 23:3300–11. doi: 10.1016/j.celrep.2018.05.035
52. Suo ST, Gong P, Peng XJ, Niu D, Guo YT. Knockdown of long non-coding RNA VIM-AS1 inhibits glioma cell proliferation and migration, and increases the cell apoptosis via modulation of WEE1 targeted by miR-105-5p. Eur Rev Med Pharmaco. (2020) 24:6834–47. doi: 10.26355/eurrev_202006_21673
53. Costa ASH, Costa P, Alves SP, Alfaia CM, Prates JAM, Vleck V, et al. Correction: Does growth path influence beef lipid deposition and fatty acid composition? PLoS ONE. (2018) 13:e0201997. doi: 10.1371/journal.pone.0201997
54. Bakhtiarizadeh MR, Alamouti AA. RNA-Seq based genetic variant discovery provides new insights into controlling fat deposition in the tail of sheep. Sci Rep. (2020) 10:13525. doi: 10.1038/s41598-020-70527-8
55. Zhao X, Hu H, Lin H, Wang C, Wang Y, Wang J. Muscle transcriptome analysis reveals potential candidate genes and pathways affecting intramuscular fat content in pigs. Front Genet. (2020) 11:877. doi: 10.3389/fgene.2020.00877
56. Zhang YH, Fu J, Zhang ZJ, Ge CC, Yi Y. LncRNA-LINC00152 down-regulated by miR-376c-3p restricts viability and promotes apoptosis of colorectal cancer cells. Am J Transl Res. (2016) 8:5286–97.
57. Al-Thuwaini TM, Al-Shuhaib MBS, Lepretre F, Mahdi ZA. Co-inherited novel SNPs of the LIPE gene associated with increased carcass dressing and decreased fat-tail weight in Awassi breed. Trop Anim Health Pro. (2020) 52:3631–8. doi: 10.1007/s11250-020-02400-9
58. Wang C, Lu H, Luo C, Song C, Wang Q, Peng Y, et al. miR-412-5p targets Xpo1 to regulate angiogenesis in hemorrhoid tissue. Gene. (2019) 705:167–76. doi: 10.1016/j.gene.2019.04.058
59. van der Vaart JI, Boon MR, Houtkooper RH. The role of AMPK signaling in brown adipose tissue activation. Cells. (2021) 10:1122. doi: 10.3390/cells10051122
60. Yi D, Yu H, Lu K, Ruan CS, Ding CH, Tong LP, et al. Signaling in energy control, cartilage biology, and osteoarthritis. Front Cell Dev Biol. (2021) 9:696602. doi: 10.3389/fcell.2021.696602
61. Xu M, Chen X, Huang Z, Chen D, Chen H, Luo Y, et al. Procyanidin B2 promotes skeletal slow-twitch myofiber gene expression through the AMPK signaling pathway in C2C12 myotubes. J Agr Food Chem. (2020) 68:1306–14. doi: 10.1021/acs.jafc.9b07489
62. Xu M, Chen X, Huang Z, Chen D, Yu B, Chen H, et al. Grape seed proanthocyanidin extract promotes skeletal muscle fiber type transformation via AMPK signaling pathway. J Nutr Biochem. (2020) 84:108462. doi: 10.1016/j.jnutbio.2020.108462
Keywords: lncRNA, skeletal muscle development, fatty acid metabolism, Tibetan sheep, regulation of nutrient metabolism
Citation: Bao G, Li S, Zhao F, Wang J, Liu X, Hu J, Shi B, Wen Y, Zhao L and Luo Y (2022) Comprehensive Transcriptome Analysis Reveals the Role of lncRNA in Fatty Acid Metabolism in the Longissimus Thoracis Muscle of Tibetan Sheep at Different Ages. Front. Nutr. 9:847077. doi: 10.3389/fnut.2022.847077
Received: 01 January 2022; Accepted: 11 February 2022;
Published: 14 March 2022.
Edited by:
Jinsong Bao, Zhejiang University, ChinaCopyright © 2022 Bao, Li, Zhao, Wang, Liu, Hu, Shi, Wen, Zhao and Luo. This is an open-access article distributed under the terms of the Creative Commons Attribution License (CC BY). The use, distribution or reproduction in other forums is permitted, provided the original author(s) and the copyright owner(s) are credited and that the original publication in this journal is cited, in accordance with accepted academic practice. No use, distribution or reproduction is permitted which does not comply with these terms.
*Correspondence: Shaobin Li, bGlzYkBnc2F1LmVkdS5jbg==; Yuzhu Luo, bHVveXpAZ3NhdS5lZHUuY24=
†These authors have contributed equally to this work