- 1Department of Pharmacy, Xiangya Hospital, Central South University, Changsha, China
- 2National Clinical Research Center for Geriatric Disorders, Xiangya Hospital, Institute for Rational and Safe Medication Practices, Central South University, Changsha, China
- 3Center of Clinical Pharmacology, The Third Xiangya Hospital, Central South University, Changsha, China
Depression has become one of the most common mental diseases in the world, but the understanding of its pathogenesis, diagnosis and treatments remains insufficient. Carnitine is a natural substance that exists in organisms, which can be synthesized in vivo or supplemented by intake. Relationships of carnitine with depression, bipolar disorder and other mental diseases have been reported in different studies. Several studies show that the level of acylcarnitines (ACs) changes significantly in patients with depression compared with healthy controls while the supplementation of acetyl-L-carnitine is beneficial to the treatment of depression. In this review, we aimed to clarify the effects of ACs in depressive patients and to explore whether ACs might be the biomarkers for the diagnosis of depression and provide new ideas to treat depression.
Introduction
Depression is a complex mental disease as a result of sociopsychological, and physiological factors combined. Its main manifestations include disorders of emotion, cognition, behavior, sleep, and appetite (1, 2). The transmission of monoaminergic neurotransmitters and the impairment of neuroplasticity are considered as the core in the pathophysiological mechanism of depression (3).
At present, less than half of the patients with depression are receiving effective treatments, with low cure rate and high recurrence rate (4). Meanwhile, it is still difficult to diagnose depression mainly relying on clinical phenomenology and the judgment of psychiatrists (5). Some studies have found that carnitine supplementation, especially acetyl-L-carnitine (LAC), can significantly improve the symptoms of patients with depression, and is expected to become a new treatment for depression (6). Other clinical studies indicated that the level of LAC in patients with major depressive disorder (MDD) was significantly lower than healthy controls (7). It suggests that carnitine might be involved in the pathological process of depression, which also provides the possibility of carnitine serving as a biomarker for the diagnosis and treatment of depression (7). However, it still remains unknown what types of acylcarnitines (ACs) and their optimal concentrations are helpful for the diagnosis and treatment of depression and other mental illnesses. Systematic studies of this topic are therefore needed for further investigation.
This study mainly reviewed the literature on the determination of ACs in patients with depression or other mental illnesses. It summarized potential ACs as biomarkers of depression and aims to improve our understanding of the carnitine-related pathophysiological mechanism of depression.
Methods
Studies were searched in the electronic databases: PubMed, Embase, Cochrane Library, and Web of Science. Moreover, we also searched preprint platform to avoid publication bias. The search was performed by two independent reviewers. Medical Subject-Heading (MeSH) and text words were used as search strategy. And the search strategy was modified according to the database type: (“carnitine” OR “acylcarnitine”) AND (“depression” OR “bipolar disorder” OR “schizophrenia” OR “mood disorders”); (“LAC” OR “Acetyl-L-Carnitine”) AND (“antidepressant” OR “antidepression”) and their matching synonyms were used for an adequate search strategy. Additionally, two independent observers extracted data and resolved differences by discussion to maintain the validity and reliability.
Carnitine and Physiological Function
Carnitine (3-hydroxy-4-N-trimethylaminobutyric acid) is a bioactive, water-soluble and low molecular weight amino acid that is usually considered as a dietary supplement (The structural formula of L-carnitine is shown in Figure 1). L-carnitine and D-carnitine isomers exist in mammals. However, only the L-carnitine isomers participate in the lipid metabolism (8). In humans, 75% of L-carnitine derives from diet, while the 25% endogenous part is synthesized from the essential amino acids, lysine, and methionine in the kidneys and liver (9). Furthermore, carnitine homeostasis is maintained via three pathways, namely dietary absorption, limited synthesis rate, and effective renal reabsorption (10).
The hydroxyl group on carnitine can combine with organic acids or fatty acids to form different short-chain, medium-chain, and long-chain esters, namely ACs. ACs and free carnitine (FC) are the main forms of carnitine in human blood (11). ACs are the products of fatty acid β-oxidation, whose composition and concentration changes are also related to the occurrence and development of many diseases, such as congenital metabolic disorders (12), diabetes (13), atherosclerosis (14), tumors (15), and other diseases (The metabolic process of carnitine is summarized in Figure 2).
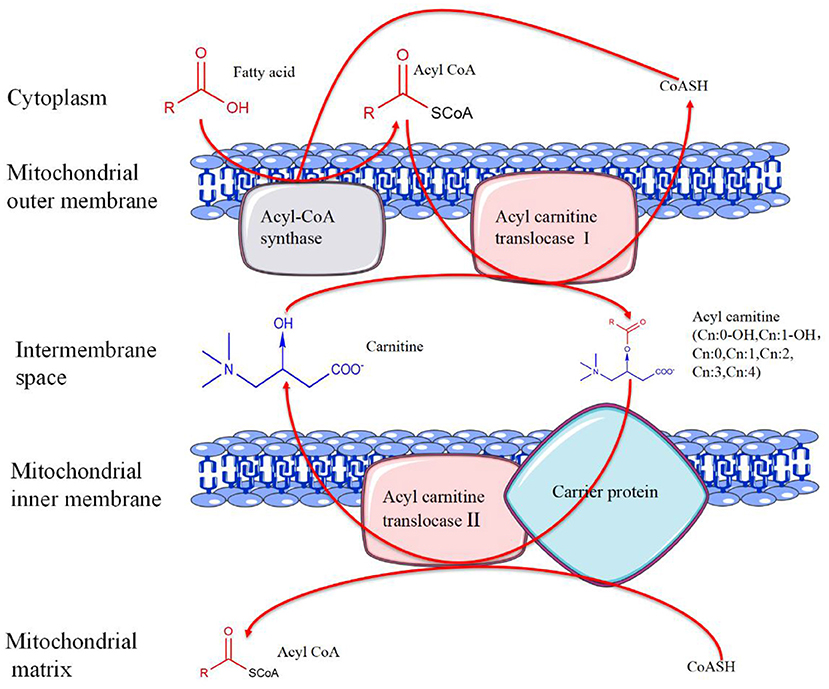
Figure 2. The metabolic process of carnitine. In the presence of coenzyme A (CoA)-SH, fatty acids are catalyzed by acyl-CoA synthase in the outer mitochondrial membrane to generate acyl-CoA. Activated acyl-CoA is transported into the mitochondria under the action of acylcarnitine translocase I with the help of carnitine and carrier protein, and acylcarnitine is produced at the same time. Subsequently, acylcarnitine releases carnitine under the action of acylcarnitine translocase II to produce acyl-CoA. Finally, acyl-CoA enters the mitochondrial matrix for further oxidation.
Carnitine, functioning in the mitochondrial fatty acid oxidation, also plays an important role in regulating the ratio of free coenzyme A (CoA) to acyl-CoA and in the removal of excessive acyl groups (11). In addition, exogenous carnitine derivatives have critical functions in the oxidation of glucose and can improve insulin resistance (16). Carnitine dysfunction may affect the lipid metabolism of mental disorders, suggesting that it may affect the occurrence and development of metabolic syndrome (17). Moreover, the occurrence of carnitine deficiency aggravates the symptoms in some diseases, such as encephalopathy (18), myopathy (19), and cardiomyopathy (20). The symptoms of these diseases are improved after carnitine supplementation (21). For cancer patients, the probability of carnitine deficiency has shown to be very high (22). A research showed that L-carnitine supplementation was safe and might have beneficial effects on fatigue, depressive symptoms and sleep quality in cancer patients (23).
Acetyl-L-Carnitine and Physiological Function
Of the ACs in living organisms, LAC, as the most widely studied endogenous short-chain ACs, has the highest content of ACs and participates in cell anabolism and catabolism pathway (24). It naturally exists in the central nervous system and can travel through the blood-brain barrier (25). ACs have the functions of synthesizing lipids, changing and stabilizing membrane components, regulating gene expressions, improving mitochondrial function, increasing antioxidant activity, and enhancing cholinergic nerve transmission. It promotes acetyl-CoA to enter the mitochondria during fatty acid oxidation, produces acetylcholine, and stimulates the synthesis of protein and membrane phospholipids (26). Furthermore, Acetyl groups provided by LAC and fatty acid β-oxidation can be used to maintain the level of acetyl-CoA (27). LAC also binds with organic acids and free fatty acid fragments, allowing them to be excreted from the cells to prevent them from destroying the cell and tissue structures. In addition to its metabolic activities, LAC also has effects on cytoprotection, antioxidation, and anti-apoptosis in the nervous system (28).
Carnitine and Depression
Increasing data seem to prove the hypothesis that carnitine levels are related to the progression of depression (Studies are summarized in Tables 1, 2). Zhang et al. (29) indicated that the average relative intensity of propionylcarnitine (C3:0), and butyrylcarnitine (C4:0) in plasma of overtired and depressed rats was lower than that of healthy control group. Another study found that most medium-chain and long-chain ACs increased in plasma of depressive rats (details are shown in Table 1). After treatments, most of ACs recovered to the normal level, except for the hydroxyoctadecadienoylcarnitine (C18:2-OH), octadecadienylcarnitine (C18:2), eicosadienoylcarnitine (C20:2). The increase of plasma long-chain ACs indicated that the fatty acid oxidation of depressed rats was incomplete, which may lead to a decrease in activities (30). Interestingly, researchers found that the plasma concentrations of carnitine and LAC were significantly higher in the CUMS-induced rat models than those in the control group, but there was no significant difference in the concentration of carnitine and LAC between the fluoxetine treatment group and the model group (31).
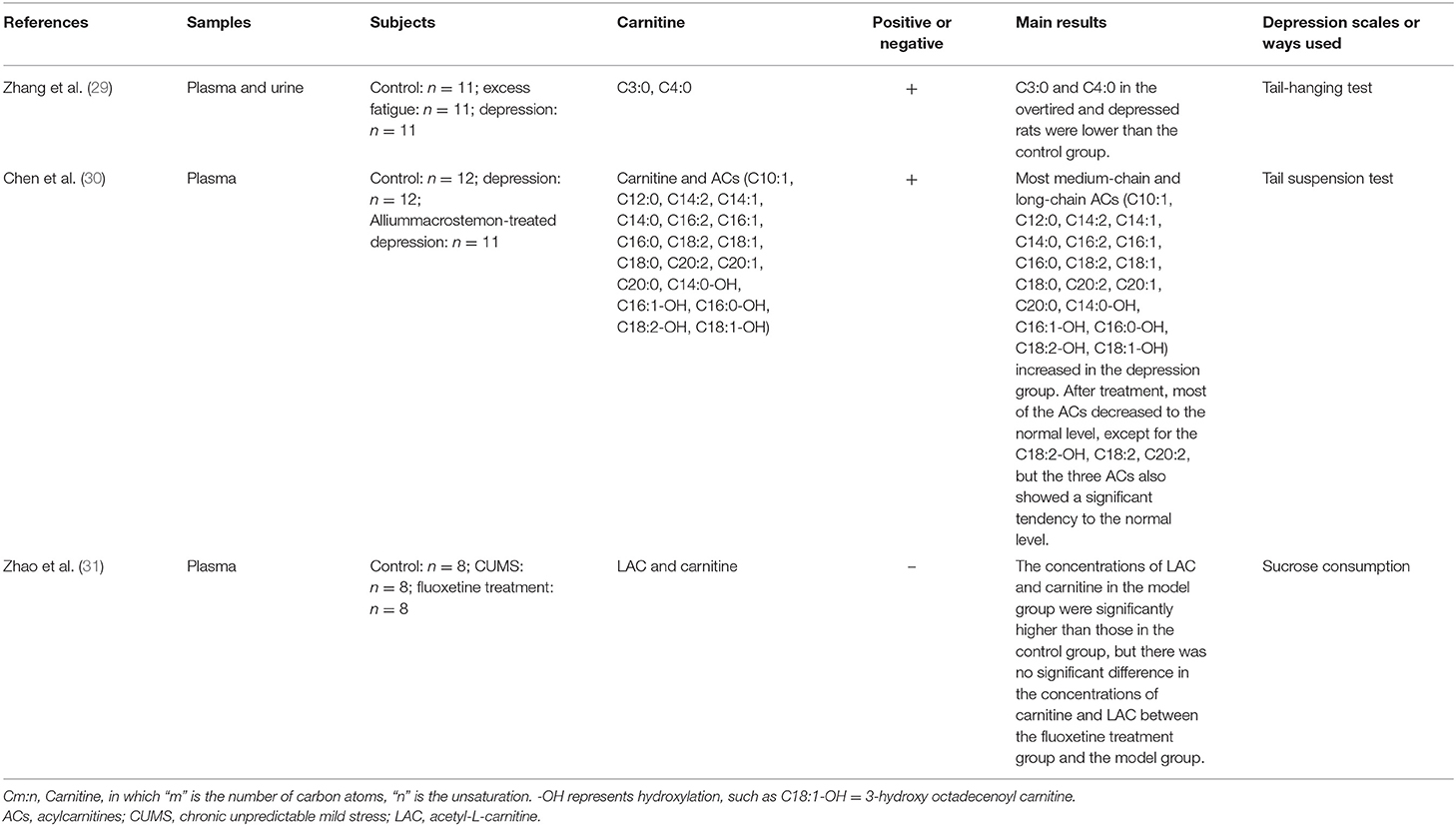
Table 1. Main information of pre-clinical researches studying the correlation between carnitine level and depression in animal.
Furthermore, the relationship of carnitine and depression has also been explored in different clinical studies. Nasca et al. (7) found that LAC levels in patients with MDD decreased compared with healthy controls, but there was no significant difference in free carnitine. However, another study found that free carnitine was decreased in depression group (32). Moreover, LAC declined more sharply in patients with a history of refractory depression. A history of childhood trauma and emotional neglect (only in women) can be used as the factors to predict the concentration of LAC in refractory depression patients (7). One study explored the differences in plasma metabonomics between MDD patients and healthy people, in which 52 identified metabolites showed differences between the two groups, including the levels of decenoylcarnitine (C10:1), decadienylcarnitine (C10:2), tetradecadienylcarnitine (C14:2), tetradecatrienecarnitine (C14:3), hexanoylcarnitine (C6:0), octanoylcarnitine (C8:0), decanoylcarnitine (C10:0), dodecanoylcarnitine (C12:0), and C3:0. The concentration of above carnitines was negatively correlated with the score of the Hamilton scale (33). Mitro et al. (34) reported that C5:0 and C5:1 were associated with lower odds of antepartum depression whereas C4:0 and C5:0 were with continuous Patient Health Questionnare-9 (PHQ-9) score. Ahmed et al. (35) found that acylcarnitine metabolism can be used to distinguish MDD phenotypes. Free carnitine and short-chain acylcarnitines (C3:0, C4:0, C5-M-DC, C5:1) increased in the core depression (CD+) group. Free carnitine and acylcarnitines (C3:0 and C4:0) increased in the CD+ group and anxious depression (ANX+) group. Acylcarnitines (C8:0, C10:0, and C12:0) decreased in the CD+ group and neurovegetative symptoms of melancholia (NVSM+) group. Acylcarnitines (C5-OH, C16:1, C18:1, C18:2) decreased in all three MDD phenotypes. Furthermore, the acylcarnitine levels of MDD patients changed significantly after treatment. For MDD patients after 2 weeks treatment, LC and LAC levels in the effective treatment group increased significantly, but no significant changes were seen in the ineffective treatment group (36). After 8 weeks of citalopram or escitalopram treatment in MDD patients, the levels of three short-chain acylcarnitines (C3:0, C4:0, and C5:0) increased, and the medium-chain and long-chain acylcarnitines (C8:0, C10:0, C12:0, C14:2, C16:0, C16:1, C18:0, C18:1, and C18:2) decreased (37). In a randomized controlled trial (RCT), Moaddel et al. (38) found that LAC decreased less after ketamine treatment in the MDD group than it did in the placebo group. It demonstrated that low molecular weight compounds in the body can be used as biomarkers for diagnosing and prognosis evaluation of depression. However, some studies have found that the level of carnitine is not significantly correlated with depression. Some patients with low serum carnitine level had no significant improvement after oral administration of L-carnitine (39). Levels of carnitine (total carnitine, free carnitine, ACs) in urine did not differ significantly between healthy controls and patients with depression (40). Jerome et al. (41) found no association between baseline carnitine level and response to S-adenosyl methionine (SAMe) or escitalopram treatments.
Many comorbidities of depression are associated with changes of carnitine levels. Depression is common in hemodialysis (HD) patients (47) which may reduce drug compliance and have a negative impact on the quality of life in HD patients (48). Studies showed that the levels of total carnitine, free carnitine, L-carnitine and C3:0 in dialysis patients were lower than those in healthy controls, while those of C4:0, isoamyl C4:0 and C18:1-OH were higher. Another study also found that acylcarnitine C3:0 was decreased in the end-stage renal disease (ESRD) with depression than those without depression and C3:0 had a good ability to diagnose depression in the ESRD (AUC = 0.934) (42). As long-chain ACs are excreted and metabolized in the kidney, it might be the reason for the increase of serum AC levels in male uremic HD patients. Renal diseases generally lead to L-carnitine homeostasis imbalance as long-term hemodialysis leads to an increase in the ratio of L-AC to free L-carnitine. Such changes interfere with the oxidation of fatty acids and the removal of unwanted short-chain acyl groups from tissues (43, 49). Supplementation of carnitine helps in decreasing self-rating depression scale (SDS) score and improving depressive symptoms in the dialysis group. The state of depression was related to the level of carnitine (44).
Carnitine also plays an important role in other diseases associated with depression, like multiple sclerosis (MS), neuromyelitis optica (NMO), and HIV infection. Decreased levels of total carnitine, free carnitine, and ACs were found in MS and NMO patients. As the most debilitating symptoms, depressive state, and chronic fatigue occur in a majority of patients with MS and NMO (39, 50). Moreover, carnitine deficiency, either from reduced intake or impaired endogenous synthesis, is considered to be the cause of fatigue, which can be treated with oral LAC supplementation (51, 52). Interestingly, there was no difference in carnitine levels between MS patients with or without fatigue. One study revealed that mitochondrial AC deficiency and fatty acid metabolism disorders were not related to fatigue in patients with MS (53). Another study also found no significant correlation between serum carnitine level and scores of three self-evaluation scales in NMO and MS. Determination of serum L-carnitine levels and the benefits of oral administration of L-carnitine remain unclear (39). Depression is also a common complication among patients with HIV infection for their poor treatment outcomes and high mortality (54). Cassol et al. (45) found decreased monoamine metabolites (phenylacetate, 4-hydroxyphenylacetate) and ACs (C3:0, iso C4:0, iso C5:0, 2-methyl C4:0) in plasma distinguished depressed subjects from controls in HIV-positive and HIV-negative cohorts, and these alterations were correlated with the severity of depression. However, a study showed that no significant correlation was found between depression and serum carnitine level as well as dietary carnitine intake (46). In view of the conflicting results presented above, future research should be conducted in larger samples.
Various factors can affect the level of carnitine in vivo. Previous studies have found that age was a factor in determining carnitine level (55, 56). In aged people, the entry of carnitine into cells is negatively affected, thus reducing the metabolism of LAC. Moreover, age has found to be positively correlated with higher AC/FC ratio. Elderly patients with psychosis may suffer secondary carnitine deficiency as higher serum AC/FC ratio is an indicator of secondary carnitine deficiency (21). Besides, the renal reabsorption of LAC may also be impaired, leading to an increase in carnitine level and a decrease in LAC level (57). With regard to gender, there was a significant negative correlation between estrogen level and serum free carnitine in females (58). Therefore, when designing the carnitine determination experiment, in order to avoid the interference of baseline confounding factors, we must pay special attention to the consistency of age, gender, and renal function between the groups, so that the final determination results are comparable.
Carnitine in Bipolar Disorder
Bipolar disorder (BD) is a chronic mental illness characterized by severe bipolar changes, ranging from extreme excitement or mania to severe depression. No effective biomarkers have been found for BD, making accurate diagnosis difficult in clinical practice (59). Abnormal mitochondrial function has been found to be present in patients with BD (60). Therefore, several readily-made dietary supplements, including LAC, have been tested as potential treatments for BD with mitochondrial regulatory functions (61, 62). Although LAC supplement is a simple and cost-effective adjuvant therapy used for the treatment of most neurological diseases, its therapeutic effect on BD is controversial (63). Dosage and duration of LAC and α-lipoic acid (ALA) have shown no antidepressant effect on patients with BD, which also had insignificant enhancement of mitochondrial function in patients (62, 64).
A cross-sectional study found that outpatients had higher total carnitine levels in serum than acutely hospitalized schizophrenia and BD patients, but there was no significant difference in total and free carnitine levels of patients with schizophrenia or BD. Serum carnitine is therefore not a reliable marker to distinguish schizophrenia from BD (65). Valproic acid (VPA) is commonly used in several neurological and psychiatric indications. It was found that the dosage of VPA was negatively correlated with the levels of total carnitine and free carnitine, suggesting that sodium valproate had a negative effect on the bioavailability and metabolism of carnitine (55, 66). Another study indicated that the concentration of C2:0 in BD with hyperammonemia and treated with VPA were significantly lower than epilepsy group under phenytoin treatment. Carnitine had a downward trend. This result showed that the level of human blood ammonia was related to the concentrations of carnitine and LAC (56) (Studies are summarized in Table 3).
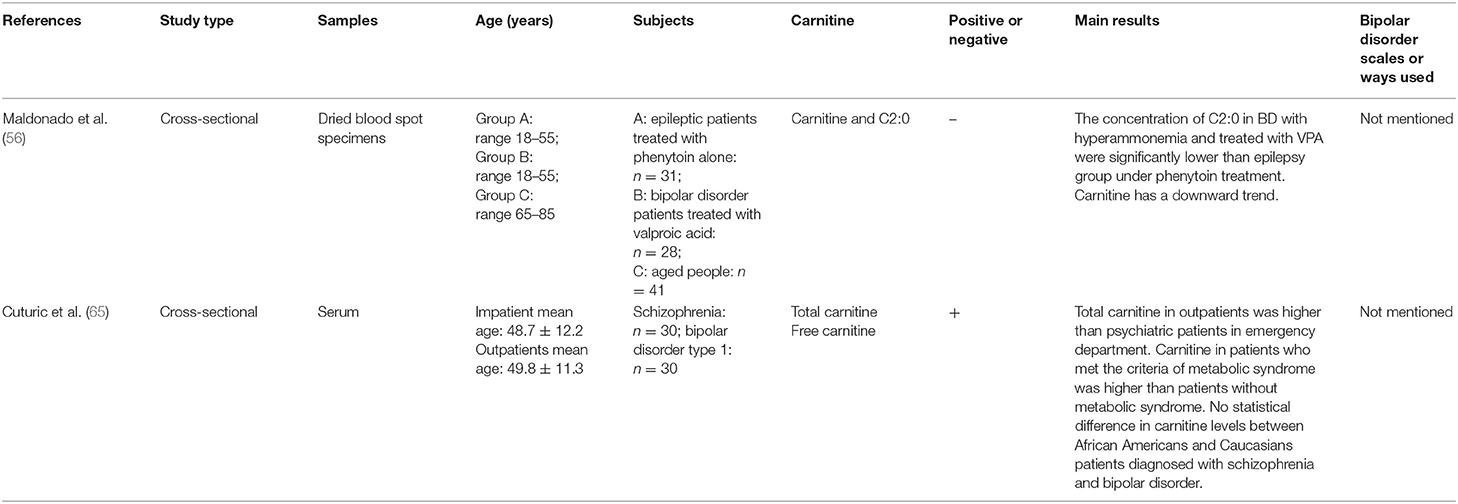
Table 3. Main information of clinical researches studying the correlation between carnitine level and bipolar disorder.
Carnitine in Schizophrenia
Carnitine has potential diagnostic and therapeutic effects on schizophrenia (67, 68). Tasic et al. (69) found that C5:0 was present only in schizophrenics, but not in BD and healthy people, suggesting that carnitine was a biomarker to identify schizophrenia. For patients with schizophrenia and metabolic syndrome (MetS), 8 plasma ACs [C3:0, C4:0, C5:0, hexenoylcarnitine (C6:1), C10:1, C10:2, hydroxytetradecadienylcarnitine (C14:2-OH), hydroxyhexadecadienylcarnitine (C16:2-OH)] were significantly higher than those in schizophrenics without MetS (70). Moreover, concentrations of ACs [hydroxybutyrylcarnitine (C4:0-OH) and hexadecenoylcarnitine (C16:1)] in schizophrenia patients were significantly higher than those in healthy controls, whereas those of C3:0, C8:0, C10:0, C10:1, C10:2, C12:0, hydroxytetradecenoylcarnitine (C14:1-OH), C14:2 and C14:2-OH were lower. After treatment, the levels of acetylcarnitine (C2:0), C8:0, C10:0, C10:1, C10:2, C12:0, C12:1, C14:1, C14:1-OH, C14:2, C14:2-OH, C16:2, C16:2-OH, and C18:0 decreased compared to the controls (71). A longitudinal study demonstrated that the levels of C18:0, L-C16:0, C18:2, and LAC decreased in the first-episode and recurrent patients after 8 weeks treatment (72). However, LAC combined with clozapine was found to not significantly improve the treatment effect of schizophrenic patients who were tolerant to clozapine (73) (Studies are summarized in Table 4).
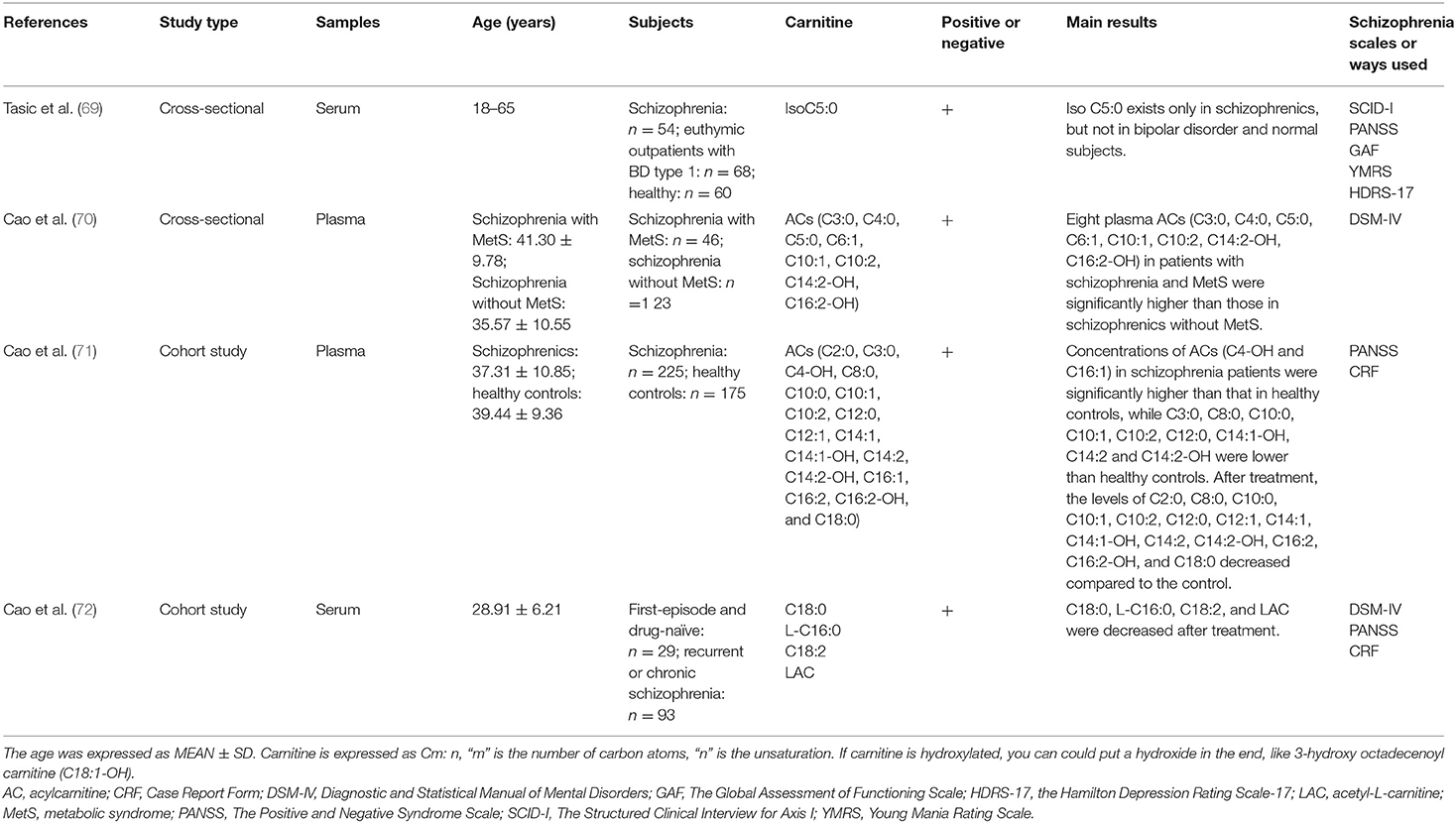
Table 4. Main information of clinical researches studying the correlation between carnitine level and schizophrenia.
The Potential Antidepressant Mechanisms of Acetyl-L-Carnitine
About 2/3 of patients with severe depression are unable to achieve complete remission of symptoms with antidepressants currently available (74, 75). In recent years, there have been a few new and unique antidepressants (76). It has been found that the odds of developing any adverse events are highest for tricyclic antidepressants and serotonin noradrenaline reuptake inhibitors. However, since LAC is an endogenous molecule, it is safer compared with other medicine (77, 78). Unlike some currently used antidepressants that take effect within 2–3 weeks, LAC can take effect within 2–3 days (79). LAC can be used in patients with MDD as a cognitive enhancer (80) and carnitine supplementation is beneficial to depression. LAC supplementation, with the same effect as antidepressants, can significantly reduce depressive symptoms compared with placebo/no intervention (81). Therefore, it is expected to become the next generation of new antidepressants with fewer side effects (79). Previous studies have proposed a variety of mechanisms of LAC in the treatment of depression. Most studied potential mechanisms include neuroplasticity effect, oxidative stress and neurotransmitter regulation. The antidepressant mechanisms of LAC are summarized in Figure 3.
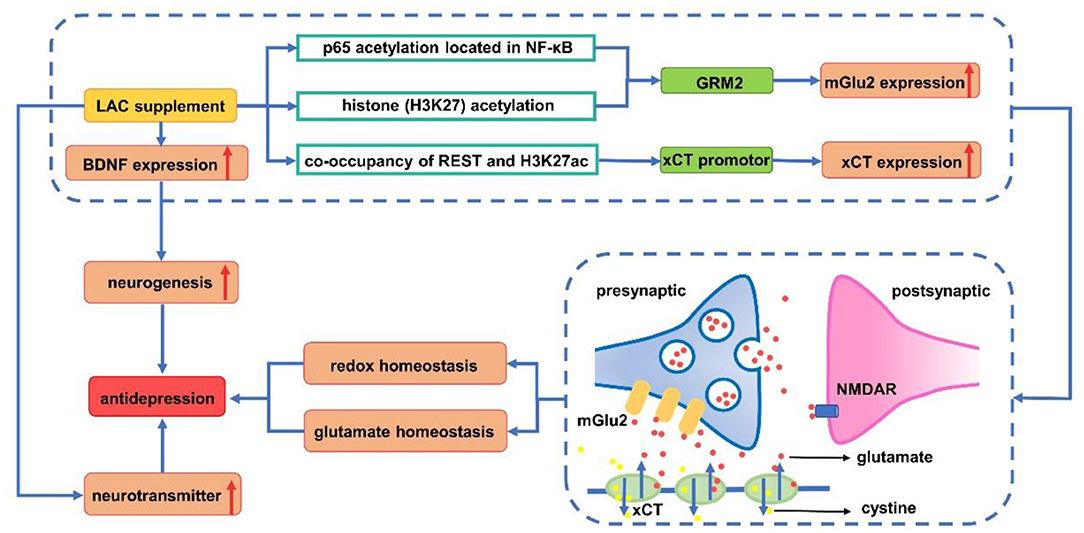
Figure 3. Antidepressant mechanism of acetyl-L-carnitine. LAC supplement increases the expression of mGlu2 (via up-regulating transcription of GRM2) by acetylating p65 on NF-κB and H3K27. REST (repressor element 1 silencing transcription factor) and H3K27ac are enriched in the xCT promoter, promoting the expression of xCT. This in turn causes the release of glutamate out of the cell, which activates the mGlu2 receptor, and reduces the spillover of glutamate between synapses to enhance glutamate homeostasis. Meanwhile, xCT transports cystine into cells for the synthesis of glutathione to avoid oxidative stress damage. Upregulation of BDNF expression induced by LAC increases neurogenesis to exert antidepressant effects. Increase of monoamine neurotransmitters (such as 5-HT, DA, and NE) induced by LAC has demonstrated its antidepressant effect from the pathogenesis of depression. BDNF, brain derived neurotrophic factor; DA, dopamine; GRM2, glutamate receptor metabotropic 2; H3K27ac, acetylated H3K27; 5-HT, serotonin; LAC, acetyl-L-carnitine; mGlu2, metabotropic glutamate 2; NE, norepinephrine; NMDAR, N-methyl-D-aspartic acid receptor; REST, repressor element silencing transcription factor; xCT: Xc-cystine/glutamate antiporter.
Neuroplasticity Effect
The adult brain can adapt to internal and external stressors through structural plasticity, and failed resilience is likely to cause depression and anxiety. Behaviorally, the LAC treatment during the last part of chronic restraint stress (CRS) enhanced resilience and reversed the effect of CRS, which is shown by increased social interaction and reduced passive behavior in a forced swim test (82).
Disorders of metabotropic glutamate (mGlu) receptors and neurotrophins have significant negative effects on neuroplasticity, leading to depression, but LAC regulate brain type 2 metabotropic glutamate (mGlu2) receptors and N-methyl-D-aspartic acid (NMDA) receptors to induce antidepressant effect (83–85). In detail, it up-regulated level of acetylated H3K27 induced by LAC that binds to the glutamate receptor metabotropic 2 (GRM2) promoter and acetylation of the NF-κB p65 subunit, which enhanced the transcription of the GRM2 gene encoding mGlu2 receptor in the hippocampus and prefrontal cortex (7, 86). While improving central energy regulation and playing a rapid antidepressant-like effect, LAC corrected systemic hyperinsulinemia and hyperglycemia in responder Flinders Sensitive Line (rFSL). As LAC can regulate metabolic factors and reduce glutamate spillover, it can quickly improve depression and also be considered for the treatment of insulin resistance in patients with depression (85).
Neurotrophic factors are associated with the pathogenesis of depression (87, 88). The decreased concentrations of BDNF (89), glial cell line-derived neurotrophic factor (GDNF), Artemin (ARTN) (90), nerve growth factor (NGF) (91), and vascular endothelial growth factor (VEGF) (92) are a good repetitive finding in depression. Supplementation of LAC can induce neuroprotective and neurotrophic effects of the peripheral nervous system by increasing the level of brain-derived neurotrophic factor (BDNF), especially in the hippocampus and prefrontal cortex (93–95). Meanwhile, it could promote the growth of dendrites and increase the number of intersections in the medial amygdala (82). In addition, it has a positive effect on the differentiation of neural progenitor cells in hippocampus, proliferation, and long-term survival of neural progenitor cells (84, 96). Studies have shown that LAC can reverse the decrease in NGF caused by pressure exposure in the central nervous system (97), and normalize the levels of GDNF and ARTN in the peripheral and central nervous systems (98), but there is still a lack of relevant research on the effect of LAC on VEGF in mental illness.
Oxidative Stress
LAC promoted the removal of oxidation products and provided acetyl groups to regulate the expression of neuro-nutrients and glutamate genes (25, 99). xCT (Xc− cystine/glutamate antiporter) is an important component of antioxidant activity in vivo, which is associated with glutathione synthesis (100). Pharmacologically, modulating histone acetylation with LAC rapidly increased xCT and activated a network with mGlu2 receptors to prime enhanced glutamate homeostasis that promotes both pro-resilient and antidepressant-like responses (101).
Neurotransmitter Regulation
The neurotransmitters associated with depression mainly include monoamine, 5-methyl-hydroxytryptamine, and dopamine (DA) (102). Long-term LAC supplementation reduces glucose metabolism to lactic acid, resulting in an increment in energy metabolites and changes in norepinephrine (NE), DA and serotonin (5-HT) in the brain of mice (96, 103). WAY-100635, a 5-HT1A antagonist, could significantly inhibit the antidepressant effects of acetyl-L-carnitine. It is suggested that 5-HT1A may be the target of LAC treatment. Effects of long-term administration of LAC on rats increased dopamine production in the marginal division of the rat cortex and protective effects on acute stress exposure (96). Moreover, Up-regulation of γ-aminobutyric acid (GABA) levels in the brain is considered to be related to the pathological mechanism of depression (104). Long-term LAC can significantly reduce the GABA level in the hippocampus of mice, but the GABA level in the cortex did not change significantly (103).
Discussion
Although many antidepressants with different mechanisms are being used, a number of patients have not achieved appropriate therapeutic effects. The monoamine hypothesis of depression has been the main pathophysiology and drug therapy target of depression over the past few decades, but the current monoamine theory has serious limitations (105). Therefore, new antidepressants with different mechanisms are needed to enable clinicians to diversify the treatment options for MDD. Perhaps LAC may be the next potentially effective and tolerable treatment with new mechanisms for patients with depression, especially for elderly and female patients, as well as patients with concomitant diseases who are vulnerable to the adverse effects of antidepressants. However, compared with other antidepressants, its hypothetical benefits must be thoroughly studied. More large-scale clinical trials are needed to determine whether LAC as a single drug or enhancer is effective and clinically beneficial for the treatment of depression.
The relationship of multiple ACs in vivo and psychiatric disorders such as depression has yet to be further investigated. Most of the current studies on depression have found that the levels of free carnitine and several short-chain acylcarnitines decreased in patients with depression. The changes of medium- and long-chain acylcarnitines are not well-studied but their metabolism may have to do with comorbiditic diseases (43, 49). One Study have found that iso C5:0 exists only in patients with schizophrenia, not in those with bipolar disorder and normal subjects (69), but it still exists in depressive patients (45). Therefore, iso C5:0 may be an important biomarker to distinguish between depression, schizophrenia and BD. Interestingly, it is found that C8:0, C10:0, C10:1, C12:0, and C14:2 have opposite changes in depression and schizophrenia compared with healthy controls (30, 37, 71). The differences in the metabolism of these ACs may potentially become important biomarkers for distinguishing depression and schizophrenia, but these possibilities are yet to be explored in further research (Table 5).
Meanwhile, the determination of carnitine concentrations in serum or plasma did not seem to be significantly different. The comprehensive screening and evaluation of carnitine changes in patients with depression is of great significance to studying the development of depression in depth. It is believed that ACs, especially LAC, might become potential biomarkers for the diagnosis of depression and effective drugs for their treatment of depression in the future given their important clinical value. Comprehensive studies on the relationships of depression and carnitine metabolism can lend support to the diagnosis and treatment of other psychiatric diseases such as BD and schizophrenia, so as to further study the similarities and differences of carnitine metabolism in psychiatric diseases.
Author Contributions
TL and KD wrote the manuscript. YX collected the relevant literature and organized it. RuY and RoY checked the content of the manuscript. ZG and MT reviewed and amended the manuscript. All authors read and approved the final manuscript.
Funding
The work was supported by National Natural Science Foundation of China (No. 81803233), the Natural Science Foundation of Hunan Province (No. 2018JJ3834), the Key Research Project of Ningxia Hui Autonomous Region in 2021 (Major Project) (2021BEG01001), Science Foundation of Xiangya Hospital for Young Scholar (No. 2017Q13), and China Postdoctoral Science Foundation (No. 2021M693561).
Conflict of Interest
The authors declare that the research was conducted in the absence of any commercial or financial relationships that could be construed as a potential conflict of interest.
Publisher's Note
All claims expressed in this article are solely those of the authors and do not necessarily represent those of their affiliated organizations, or those of the publisher, the editors and the reviewers. Any product that may be evaluated in this article, or claim that may be made by its manufacturer, is not guaranteed or endorsed by the publisher.
References
1. Potter GG, Mcquoid DR, Steffens DC. Appetite loss and neurocognitive deficits in late-life depression. Int J Geriatr Psychiatry. (2015) 30:647–54. doi: 10.1002/gps.4196
2. Rice F, Riglin L, Lomax T, Souter E, Potter R, Smith DJ, et al. Adolescent and adult differences in major depression symptom profiles. J Affect Disord. (2019) 243:175–81. doi: 10.1016/j.jad.2018.09.015
3. Blugeot A, Rivat C, Bouvier E, Molet J, Mouchard A, Zeau B, et al. Vulnerability to depression: from brain neuroplasticity to identification of biomarkers. J Neurosci. (2011) 31:12889–99. doi: 10.1523/JNEUROSCI.1309-11.2011
4. Kessler RC, Bromet EJ. The epidemiology of depression across cultures. Annu Rev Public Health. (2013) 34:119–38. doi: 10.1146/annurev-publhealth-031912-114409
5. Mitchell AJ, Vaze A, Rao S. Clinical diagnosis of depression in primary care: a meta-analysis. Lancet. (2009) 374:609–19. doi: 10.1016/S0140-6736(09)60879-5
6. Wang S-M, Han C, Lee S-J, Patkar AA, Masand PS, Pae C-U. A review of current evidence for acetyl-l-carnitine in the treatment of depression. J Psychiatr Res. (2014) 53:30–7. doi: 10.1016/j.jpsychires.2014.02.005
7. Nasca C, Bigio B, Lee FS, Young SP, Kautz MM, Albright A, et al. Acetyl-l-carnitine deficiency in patients with major depressive disorder. Proc Natl Acad Sci USA. (2018) 115:8627–32. doi: 10.1073/pnas.1801609115
8. Tsoko M, Beauseigneur F, Gresti J, Niot I, Demarquoy J, Boichot J, et al. Enhancement of activities relative to fatty acid oxidation in the liver of rats depleted of L-carnitine by D-carnitine and a gamma-butyrobetaine hydroxylase inhibitor. Biochem Pharmacol. (1995) 49:1403–10. doi: 10.1016/0006-2952(95)00019-V
9. Chiechio S, Copani A, Nicoletti F, Gereau RWT. L-acetylcarnitine: a proposed therapeutic agent for painful peripheral neuropathies. Curr Neuropharmacol. (2006) 4:233–7. doi: 10.2174/157015906778019509
10. Rebouche CJ. Kinetics, pharmacokinetics, and regulation of L-carnitine and acetyl-L-carnitine metabolism. Ann N Y Acad Sci. (2004) 1033:30–41. doi: 10.1196/annals.1320.003
11. Reuter S, Evans A. Carnitine and acylcarnitines: pharmacokinetic, pharmacological and clinical aspects. Clin Pharmacokinet. (2012) 51:553–72. doi: 10.1007/BF03261931
12. Sánchez-Pintos P, De Castro M, Roca I, Rite S, López M, Couce M. Similarities between acylcarnitine profiles in large for gestational age newborns and obesity. Sci Rep. (2017) 7:16267. doi: 10.1038/s41598-017-15809-4
13. Batchuluun B, Al Rijjal D, Prentice K, Eversley J, Burdett E, Mohan H, et al. Elevated medium-chain acylcarnitines are associated with gestational diabetes mellitus and early progression to type 2 diabetes and induce pancreatic β-cell dysfunction. Diabetes. (2018) 67:885–97. doi: 10.2337/db17-1150
14. Vilskersts R, Kuka J, Liepinsh E, Makrecka-Kuka M, Volska K, Makarova E, et al. Methyl-γ-butyrobetaine decreases levels of acylcarnitines and attenuates the development of atherosclerosis. Vascul Pharmacol. (2015) 72:101–7. doi: 10.1016/j.vph.2015.05.005
15. Fujiwara N, Nakagawa H, Enooku K, Kudo Y, Hayata Y, Nakatsuka T, et al. CPT2 downregulation adapts HCC to lipid-rich environment and promotes carcinogenesis via acylcarnitine accumulation in obesity. Gut. (2018) 67:1493–504. doi: 10.1136/gutjnl-2017-315193
16. Malaguarnera M. Carnitine derivatives: clinical usefulness. Curr Opin Gastroenterol. (2012) 28:166–76. doi: 10.1097/MOG.0b013e3283505a3b
17. Cuturic M, Abramson RK, Moran RR, Hardin JW. Carnitine and metabolic correlates in hospitalized psychiatric patients: a follow-through report. J Psychiatr Pract. (2011) 17:35–40. doi: 10.1097/01.pra.0000393842.63841.bc
18. Rasmussen J, Nielsen O, Lund A, Køber L, Djurhuus H. Primary carnitine deficiency and pivalic acid exposure causing encephalopathy and fatal cardiac events. J Inherit Metab Dis. (2013) 36:35–41. doi: 10.1007/s10545-012-9488-8
19. Zhang W, Miao J, Zhang G, Liu R, Zhang D, Wan Q, et al. Muscle carnitine deficiency: adult onset lipid storage myopathy with sensory neuropathy. Neurol Sci. (2010) 31:61–4. doi: 10.1007/s10072-009-0128-5
20. Lahrouchi N, Lodder E, Mansouri M, Tadros R, Zniber L, Adadi N, et al. Exome sequencing identifies primary carnitine deficiency in a family with cardiomyopathy and sudden death. Eur J Hum Genet. (2017) 25:783–7. doi: 10.1038/ejhg.2017.22
21. Lheureux PER, Penaloza A, Zahir S, Gris M. Science review: carnitine in the treatment of valproic acid-induced toxicity - what is the evidence? Critical Care. (2005) 9:431–40. doi: 10.1186/cc3742
22. Matsui H, Einama T, Shichi S, Kanazawa R, Shibuya K, Suzuki T, et al. L-Carnitine supplementation reduces the general fatigue of cancer patients during chemotherapy. Mol Clin Oncol. (2018) 8:413–6. doi: 10.3892/mco.2018.1557
23. Cruciani RA, Dvorkin E, Homel P, Culliney B, Malamud S, Shaiova L, et al. L-carnitine supplementation for the treatment of fatigue and depressed mood in cancer patients with carnitine deficiency: a preliminary analysis. Ann N Y Acad Sci. (2004) 1033:168–76. doi: 10.1196/annals.1320.016
24. Flanagan JL, Simmons PA, Vehige J, Willcox MD, Garrett Q. Role of carnitine in disease. Nutr Metab. (2010) 7:30–30. doi: 10.1186/1743-7075-7-30
25. Scafidi S, Fiskum G, Lindauer SL, Bamford P, Shi D, Hopkins I, et al. Metabolism of acetyl-L-carnitine for energy and neurotransmitter synthesis in the immature rat brain. J Neurochem. (2010) 114:820–31. doi: 10.1111/j.1471-4159.2010.06807.x
26. Parnetti L, Gaiti A, Mecocci P, Cadini D, Senin U. Pharmacokinetics of IV and oral acetyl-L-carnitine in a multiple dose regimen in patients with senile dementia of Alzheimer type. Eur J Clin Pharmacol. (1992) 42:89–93. doi: 10.1007/BF00314926
27. Pettegrew JW, Levine J, Mcclure RJ. Acetyl-L-carnitine physical-chemical, metabolic, and therapeutic properties: relevance for its mode of action in Alzheimer's disease and geriatric depression. Mol Psychiatry. (2000) 5:616–32. doi: 10.1038/sj.mp.4000805
28. Madiraju P, Pande SV, Prentki M, Madiraju SRM. Mitochondrial acetylcarnitine provides acetyl groups for nuclear histone acetylation. Epigenetics. (2009) 4:399–403. doi: 10.4161/epi.4.6.9767
29. Zhang F, Jia Z, Gao P, Kong H, Li X, Lu X, et al. Metabonomics study of urine and plasma in depression and excess fatigue rats by ultra fast liquid chromatography coupled with ion trap-time of flight mass spectrometry. Mol Biosyst. (2010) 6:852–61. doi: 10.1039/b914751a
30. Chen S, Wei C, Gao P, Kong H, Jia Z, Hu C, et al. Effect of Allium macrostemon on a rat model of depression studied by using plasma lipid and acylcarnitine profiles from liquid chromatography/mass spectrometry. J Pharm Biomed Anal. (2014) 89:122–9. doi: 10.1016/j.jpba.2013.10.045
31. Zhao L, Xiong Z, Lu X, Zheng S, Wang F, Ge L, et al. Metabonomic evaluation of chronic unpredictable mild stress-induced changes in rats by intervention of fluoxetine by HILIC-UHPLC/MS. PLoS ONE. (2015) 10:e0129146. doi: 10.1371/journal.pone.0129146
32. Nie LJ, Liang J, Shan F, Xu YY, Yan CY, Zhou X, et al. A UPLC-MS/MS method for determination of endogenous l-carnitine and acetyl-l-carnitine in serum of patients with depression. Biomed Chromatogr. (2021) 35:e4991. doi: 10.1002/bmc.4991
33. Liu X, Zheng P, Zhao X, Zhang Y, Hu C, Li J, et al. Discovery and validation of plasma biomarkers for major depressive disorder classification based on liquid chromatography-mass spectrometry. J Proteome Res. (2015) 14:2322–30. doi: 10.1021/acs.jproteome.5b00144
34. Mitro SD, Larrabure-Torrealva GT, Sanchez SE, Molsberry SA, Williams MA, Clish C, et al. Metabolomic markers of antepartum depression and suicidal ideation. J Affect Disord. (2020) 262:422–8. doi: 10.1016/j.jad.2019.11.061
35. Ahmed AT, Mahmoudiandehkordi S, Bhattacharyya S, Arnold M, Liu D, Neavin D, et al. Acylcarnitine metabolomic profiles inform clinically-defined major depressive phenotypes. J Affect Disord. (2020) 264:90–7. doi: 10.1016/j.jad.2019.11.122
36. Nie LJ, Liang J, Shan F, Wang BS, Mu YY, Zhou XH, et al. L-carnitine and acetyl-L-carnitine: potential novel biomarkers for major depressive disorder. Front Psychiatry. (2021) 12:671151. doi: 10.3389/fpsyt.2021.671151
37. Mahmoudiandehkordi S, Ahmed AT, Bhattacharyya S, Han X, Baillie RA, Arnold M, et al. Alterations in acylcarnitines, amines, and lipids inform about the mechanism of action of citalopram/escitalopram in major depression. Transl Psychiatry. (2021) 11:153. doi: 10.1038/s41398-020-01097-6
38. Moaddel R, Shardell M, Khadeer M, Lovett J, Kadriu B, Ravichandran S, et al. Plasma metabolomic profiling of a ketamine and placebo crossover trial of major depressive disorder and healthy control subjects. Psychopharmacology. (2018) 235:3017–30. doi: 10.1007/s00213-018-4992-7
39. Akaishi T, Nakashima I, Misu T, Fujihara K, Aoki M. Depressive state and chronic fatigue in multiple sclerosis and neuromyelitis optica. J Neuroimmunol. (2015) 283:70–3. doi: 10.1016/j.jneuroim.2015.05.007
40. Jones MG, Goodwin CS, Amjad S, Chalmers RA. Plasma and urinary carnitine and acylcarnitines in chronic fatigue syndrome. Clin Chim Acta. (2005) 360:173–7. doi: 10.1016/j.cccn.2005.04.029
41. Sarris JI, Papakostas G, Vitolo O, Fava M, Mischoulon D. S-adenosyl methionine (SAMe) versus escitalopram and placebo in major depression RCT: Efficacy and effects of histamine and carnitine as moderators of response. J Affect Disord. (2014) 164:76–81. doi: 10.1016/j.jad.2014.03.041
42. Yuan D, Kuan T, Ling H, Wang H, Feng L, Zhao Q, et al. Serum metabolomics of end-stage renal disease patients with depression: potential biomarkers for diagnosis. Ren Fail. (2021) 43:1479–91. doi: 10.1080/0886022X.2021.1994995
43. Fukami K, Yamagishi S-I, Sakai K, Kaida Y, Minami A, Nakayama Y, et al. Carnitine deficiency is associated with late-onset hypogonadism and depression in uremic men with hemodialysis. Aging Male. (2014) 17:238–42. doi: 10.3109/13685538.2014.888053
44. Tashiro K, Kaida Y, Yamagishi S-I, Tanaka H, Yokoro M, Yano J, et al. L-carnitine supplementation improves self-rating depression scale scores in uremic male patients undergoing hemodialysis. Lett Drug Des Discov. (2017) 14:737–42. doi: 10.2174/1570180814666170216102632
45. Cassol E, Misra V, Morgello S, Kirk GD, Mehta SH, Gabuzda D. Altered monoamine and acylcarnitine metabolites in HIV-positive and HIV-negative subjects with depression. J Acquired Immune Deficiency Syndromes. (2015) 69:18–28. doi: 10.1097/QAI.0000000000000551
46. Rezaee H, Khalili H, Hatamkhani S, Dashti-Khavidaki S, Khazaeipour Z. Frequency of depression and its correlation with serum carnitine level in HIV/AIDS patients. Curr HIV Res. (2013) 11:226–30. doi: 10.2174/1570162X11311030007
47. Agganis BT, Weiner DE, Giang LM, Scott T, Tighiouart H, Griffith JL, et al. Depression and cognitive function in maintenance hemodialysis patients. Am J Kidney Dis. (2010) 56:704–12. doi: 10.1053/j.ajkd.2010.04.018
48. Park HC, Yoon HB, Son MJ, Jung ES, Joo KW, Chin HJ, et al. Depression and health-related quality of life in maintenance hemodialysis patients. Clin Nephrol. (2010) 73:374–80. doi: 10.5414/CNP73374
49. Evans A. Dialysis-related carnitine disorder and levocarnitine pharmacology. Am J Kidney Dis. (2003) 41:S13–26. doi: 10.1016/S0272-6386(03)00113-6
50. Tomassini V, Pozzilli C, Onesti E, Pasqualetti P, Marinelli F, Pisani A, et al. Comparison of the effects of acetyl L-carnitine and amantadine for the treatment of fatigue in multiple sclerosis: results of a pilot, randomised, double-blind, crossover trial. J Neurol Sci. (2004) 218:103–8. doi: 10.1016/j.jns.2003.11.005
51. Tejani AM, Wasdell M, Spiwak R, Rowell G, Nathwani S. Carnitine for fatigue in multiple sclerosis. Cochr Datab Syst Rev. (2012) 2012:CD007280. doi: 10.1002/14651858.CD007280.pub3
52. Yang T-T, Wang L, Deng X-Y, Yu G. Pharmacological treatments for fatigue in patients with multiple sclerosis: a systematic review and meta-analysis. J Neurol Sci. (2017) 380:256–61. doi: 10.1016/j.jns.2017.07.042
53. Fukazawa T, Sasaki H, Kikuchi S, Hamada T, Tashiro K. Serum carnitine and disabling fatigue in multiple sclerosis. Psychiatry Clin Neurosci. (1996) 50:323–5. doi: 10.1111/j.1440-1819.1996.tb00573.x
54. Do AN, Rosenberg ES, Sullivan PS, Beer L, Strine TW, Schulden JD, et al. Excess burden of depression among HIV-infected persons receiving medical care in the united states: data from the medical monitoring project and the behavioral risk factor surveillance system. PLoS ONE. (2014) 9:e92842. doi: 10.1371/journal.pone.0092842
55. Cuturic M, Abramson RK, Moran RR, Hardin JW, Hall AV. Clinical correlates of low serum carnitine levels in hospitalized psychiatric patients. World J Biol Psychiatry. (2011) 12:73–9. doi: 10.3109/15622975.2010.489619
56. Maldonado C, Guevara N, Queijo C, González R, Fagiolino P, Vázquez M. Carnitine and/or acetylcarnitine deficiency as a cause of higher levels of ammonia. Biomed Res Int. (2016) 2016:2920108–2920108. doi: 10.1155/2016/2920108
57. Opalka JR, Gellerich FN, Zierz S. Age and sex dependency of carnitine concentration in human serum and skeletal muscle. Clin Chem. (2001) 47:2150–3. doi: 10.1093/clinchem/47.12.2150
58. Takiyama N, Matsumoto K. Age-and sex-related differences of serum carnitine in a Japanese population. J Am Coll Nutr. (1998) 17:71–4. doi: 10.1080/07315724.1998.10720458
59. Göteson A, Isgren A, Sparding T, Holmén-Larsson J, Jakobsson J, Pålsson E, et al. A serum proteomic study of two case-control cohorts identifies novel biomarkers for bipolar disorder. Transl Psychiatry. (2022) 12:55. doi: 10.1038/s41398-022-01819-y
60. Scaini G, Rezin GT, Carvalho AF, Streck EL, Berk M, Quevedo J. Mitochondrial dysfunction in bipolar disorder: evidence, pathophysiology and translational implications. Neurosci Biobehav Rev. (2016) 68:694–713. doi: 10.1016/j.neubiorev.2016.06.040
61. Nierenberg AA, Kansky C, Brennan BP, Shelton RC, Perlis R, Iosifescu DV. Mitochondrial modulators for bipolar disorder: a pathophysiologically informed paradigm for new drug development. Aust N Z J Psychiatry. (2013) 47:26–42. doi: 10.1177/0004867412449303
62. Jamilian H, Jamilian M, Samimi M, Afshar Ebrahimi F, Rahimi M, Bahmani F, et al. Oral carnitine supplementation influences mental health parameters and biomarkers of oxidative stress in women with polycystic ovary syndrome: a randomized, double-blind, placebo-controlled trial. Gynecol Endocrinol. (2017) 33:442–7. doi: 10.1080/09513590.2017.1290071
63. Maldonado C, Vázquez M, Fagiolino P. Potential therapeutic role of carnitine and acetylcarnitine in neurological disorders. Curr Pharmaceut Design. (2020) 26:1277–85. doi: 10.2174/1381612826666200212114038
64. Brennan BP, Jensen JE, Hudson JI, Coit CE, Beaulieu A, Pope HG, et al. A placebo-controlled trial of acetyl-L-carnitine and alpha-lipoic acid in the treatment of bipolar depression. J Clin Psychopharmacol. (2013) 33:627–35. doi: 10.1097/JCP.0b013e31829a83f5
65. Cuturic M, Abramson RK, Breen RJ, Edwards AC, Levy EE. Comparison of serum carnitine levels and clinical correlates between outpatients and acutely hospitalised individuals with bipolar disorder and schizophrenia: a cross-sectional study. World J Biol Psychiatry. (2016) 17:475–9. doi: 10.1080/15622975.2016.1178803
66. Vázquez M, Fagiolino P, Maldonado C, Olmos I, Ibarra M, Alvariza S, et al. Hyperammonemia associated with valproic acid concentrations. Biomed Res Int. (2014) 2014:217269. doi: 10.1155/2014/217269
67. Cui G, Qing Y, Hu X, Wang P, Sun L, Yang X, et al. Serum metabolomic profiling based on fourier transform-ion cyclotron resonance-mass spectrometry: do the dysfunctions of metabolic pathways reveal a universal risk of oxidative stress in schizophrenia? Antioxid Redox Signal. (2020) 33:679–88. doi: 10.1089/ars.2020.8141
68. Ni P, Noh H, Park G, Shao Z, Guan Y, Park J, et al. iPSC-derived homogeneous populations of developing schizophrenia cortical interneurons have compromised mitochondrial function. Mol Psychiatry. (2020) 25:2873–88. doi: 10.1038/s41380-019-0423-3
69. Tasic L, Larcerda ALT, Pontes JGM, Da Costa TBBC, Nani JV, Martins LG, et al. Peripheral biomarkers allow differential diagnosis between schizophrenia and bipolar disorder. J Psychiatr Res. (2019) 119:67–75. doi: 10.1016/j.jpsychires.2019.09.009
70. Cao B, Chen Y, Mcintyre R, Yan L. Acyl-Carnitine plasma levels and their association with metabolic syndrome in individuals with schizophrenia. Psychiatry Res. (2020) 293:113458. doi: 10.1016/j.psychres.2020.113458
71. Cao B, Wang D, Pan Z, Brietzke E, Mcintyre R, Musial N, et al. Characterizing acyl-carnitine biosignatures for schizophrenia: a longitudinal pre- and post-treatment study. Transl Psychiatry. (2019) 9:19. doi: 10.1038/s41398-018-0353-x
72. Cao B, Jin M, Brietzke E, Mcintyre R, Wang D, Rosenblat J, et al. Serum metabolic profiling using small molecular water-soluble metabolites in individuals with schizophrenia: a longitudinal study using a pre-post-treatment design. Psychiatry Clin Neurosci. (2019) 73:100–8. doi: 10.1111/pcn.12779
73. Bruno A, Pandolfo G, Crucitti M, Lorusso S, Zoccali R, Muscatello M. Acetyl-L-carnitine augmentation of clozapine in partial-responder schizophrenia: a 12-week, open-label uncontrolled preliminary study. Clin Neuropharmacol. (2016) 39:277–80. doi: 10.1097/WNF.0000000000000170
74. Rush AJ, Trivedi MH, Wisniewski SR, Nierenberg AA, Stewart JW, Warden D, et al. Acute and longer-term outcomes in depressed outpatients requiring one or several treatment steps: a STAR*D report. Am J Psychiatry. (2006) 163:1905–17. doi: 10.1176/ajp.2006.163.11.1905
75. De Zwart PL, Jeronimus BF, De Jonge P. Empirical evidence for definitions of episode, remission, recovery, relapse and recurrence in depression: a systematic review. Epidemiol Psychiatr Sci. (2019) 28:544–62. doi: 10.1017/S2045796018000227
76. Yao X, Li Y, Yu Z, Mo C, Pan H, Li C. The efficacy and safety of agomelatine, sertraline, and escitalopram for senile post-stroke depression: a randomized double-blind placebo-controlled trial. Clin Neurol Neurosurg. (2021) 205:106651. doi: 10.1016/j.clineuro.2021.106651
77. Meister R, Von Wolff A, Mohr H, Härter M, Nestoriuc Y, Hölzel L, et al. Comparative safety of pharmacologic treatments for persistent depressive disorder: a systematic review and network meta-analysis. PLoS ONE. (2016) 11:e0153380. doi: 10.1371/journal.pone.0153380
78. Chiechio S, Canonico PL, Grilli M. l-Acetylcarnitine: a mechanistically distinctive and potentially rapid-acting antidepressant drug. Int J Mol Sci. (2017) 19:11. doi: 10.3390/ijms19010011
79. Russo SJ, Charney DS. Next generation antidepressants. Proc Natl Acad Sci USA. (2013) 110:4441–2. doi: 10.1073/pnas.1301593110
80. Bortolato B, Miskowiak KW, Köhler CA, Maes M, Fernandes BS, Berk M, et al. Cognitive remission: a novel objective for the treatment of major depression? BMC Med. (2016) 14:9–9. doi: 10.1186/s12916-016-0560-3
81. Veronese N, Stubbs B, Solmi M, Ajnakina O, Carvalho A, Maggi S. Acetyl-L-carnitine supplementation and the treatment of depressive symptoms: a systematic review and meta-analysis. Psychosom Med. (2018) 80:154–9. doi: 10.1097/PSY.0000000000000537
82. Lau T, Bigio B, Zelli D, Mcewen BS, Nasca C. Stress-induced structural plasticity of medial amygdala stellate neurons and rapid prevention by a candidate antidepressant. Mol Psychiatry. (2017) 22:227–34. doi: 10.1038/mp.2016.68
83. Zarate C, Machado-Vieira R, Henter I, Ibrahim L, Diazgranados N, Salvadore G. Glutamatergic modulators: the future of treating mood disorders? Harv Rev Psychiatry. (2010) 18:293–303. doi: 10.3109/10673229.2010.511059
84. Cuccurazzu B, Bortolotto V, Valente MM, Ubezio F, Koverech A, Canonico PL, et al. Upregulation of mGlu2 receptors via NF-κB p65 acetylation is involved in the Proneurogenic and antidepressant effects of acetyl-L-carnitine. Neuropsychopharmacology. (2013) 38:2220–30. doi: 10.1038/npp.2013.121
85. Bigio B, Mathé AA, Sousa VC, Zelli D, Svenningsson P, Mcewen BS, et al. Epigenetics and energetics in ventral hippocampus mediate rapid antidepressant action: implications for treatment resistance. Proc Natl Acad Sci USA. (2016) 113:7906–11. doi: 10.1073/pnas.1603111113
86. Nasca C, Xenos D, Barone Y, Caruso A, Scaccianoce S, Matrisciano F, et al. L-acetylcarnitine causes rapid antidepressant effects through the epigenetic induction of mGlu2 receptors. Proc Natl Acad Sci USA. (2013) 110:4804–9. doi: 10.1073/pnas.1216100110
87. Zhang X, Ru B, Sha W, Xin W, Zhou H, Zhang Y. Performance on the Wisconsin card-sorting test and serum levels of glial cell line-derived neurotrophic factor in patients with major depressive disorder. Asia Pac Psychiatry. (2014) 6:302–7. doi: 10.1111/appy.12120
88. Kerling A, Kück M, Tegtbur U, Grams L, Weber-Spickschen S, Hanke A, et al. Exercise increases serum brain-derived neurotrophic factor in patients with major depressive disorder. J Affect Disord. (2017) 215:152–5. doi: 10.1016/j.jad.2017.03.034
89. Youssef MM, Underwood MD, Huang YY, Hsiung SC, Liu Y, Simpson NR, et al. Association of BDNF Val66Met polymorphism and brain BDNF levels with major depression and suicide. Int J Neuropsychopharmacol. (2018) 21:528–38. doi: 10.1093/ijnp/pyy008
90. Otsuki K, Uchida S, Watanuki T, Wakabayashi Y, Fujimoto M, Matsubara T, et al. Altered expression of neurotrophic factors in patients with major depression. J Psychiatr Res. (2008) 42:1145–53. doi: 10.1016/j.jpsychires.2008.01.010
91. Wiener CD, De Mello Ferreira S, Pedrotti Moreira F, Bittencourt G, De Oliveira JF, Lopez Molina M, et al. Serum levels of nerve growth factor (NGF) in patients with major depression disorder and suicide risk. J Affect Disord. (2015) 184:245–8. doi: 10.1016/j.jad.2015.05.067
92. Sideromenos S, Lindtner C, Zambon A, Horvath O, Berger A, Pollak DD. VEGF treatment ameliorates depression-like behavior in adult offspring after maternal immune activation. Cells. (2020) 9:1048. doi: 10.3390/cells9041048
93. Wang W, Lu Y, Xue Z, Li C, Wang C, Zhao X, et al. Rapid-acting antidepressant-like effects of acetyl-l-carnitine mediated by PI3K/AKT/BDNF/VGF signaling pathway in mice. Neuroscience. (2015) 285:281–91. doi: 10.1016/j.neuroscience.2014.11.025
94. Peng S, Li W, Lv L, Zhang Z, Zhan X. BDNF as a biomarker in diagnosis and evaluation of treatment for schizophrenia and depression. Discov Med. (2018) 26:127–36.
95. Rolim LC, Da Silva EM, Flumignan RL, Abreu MM, Dib SA. Acetyl-L-carnitine for the treatment of diabetic peripheral neuropathy. Cochrane Datab Syst Rev. (2019) 6:CD011265. doi: 10.1002/14651858.CD011265.pub2
96. Tolu P, Masi F, Leggio B, Scheggi S, Tagliamonte A, De Montis M, et al. Effects of long-term acetyl-L-carnitine administration in rats: I. increased dopamine output in mesocorticolimbic areas and protection toward acute stress exposure. Neuropsychopharmacology. (2002) 27:410–20. doi: 10.1016/S0893-133X(02)00306-8
97. Piovesan P, Pacifici L, Taglialatela G, Ramacci MT, Angelucci L. Acetyl-L-carnitine treatment increases choline acetyltransferase activity and NGF levels in the CNS of adult rats following total fimbria-fornix transection. Brain Res. (1994) 633:77–82. doi: 10.1016/0006-8993(94)91524-5
98. Vivoli E, Di Cesare Mannelli L, Salvicchi A, Bartolini A, Koverech A, Nicolai R, et al. Acetyl-L-carnitine increases artemin level and prevents neurotrophic factor alterations during neuropathy. Neuroscience. (2010) 167:1168–74. doi: 10.1016/j.neuroscience.2010.03.017
99. Chiappelli J, Rowland LM, Wijtenburg SA, Muellerklein F, Tagamets M, Mcmahon RP, et al. Evaluation of myo-inositol as a potential biomarker for depression in schizophrenia. Neuropsychopharmacology. (2015) 40:2157–64. doi: 10.1038/npp.2015.57
100. Miyazaki I, Murakami S, Torigoe N, Kitamura Y, Asanuma M. Neuroprotective effects of levetiracetam target xCT in astrocytes in parkinsonian mice. J Neurochem. (2016) 136:194–204. doi: 10.1111/jnc.13405
101. Nasca C, Bigio B, Zelli D, De Angelis P, Lau T, Okamoto M, et al. Role of the astroglial glutamate exchanger xCT in ventral hippocampus in resilience to stress. Neuron. (2017) 96:402–13.e405. doi: 10.1016/j.neuron.2017.09.020
102. Pan J, Xia J, Deng F, Liang W, Wu J, Yin B, et al. Diagnosis of major depressive disorder based on changes in multiple plasma neurotransmitters: a targeted metabolomics study. Transl Psychiatry. (2018) 8:130. doi: 10.1038/s41398-018-0183-x
103. Smeland O, Meisingset T, Borges K, Sonnewald U. Chronic acetyl-L-carnitine alters brain energy metabolism and increases noradrenaline and serotonin content in healthy mice. Neurochem Int. (2012) 61:100–7. doi: 10.1016/j.neuint.2012.04.008
104. Bradley K, Alonso C, Mehra L, Xu J, Gabbay V. Elevated striatal γ-aminobutyric acid in youth with major depressive disorder. Prog Neuropsychopharmacol Biol Psychiatry. (2018) 86:203–10. doi: 10.1016/j.pnpbp.2018.06.004
Keywords: carnitine, acylcarnitines, depression, bipolar disorder, schizophrenia
Citation: Liu T, Deng K, Xue Y, Yang R, Yang R, Gong Z and Tang M (2022) Carnitine and Depression. Front. Nutr. 9:853058. doi: 10.3389/fnut.2022.853058
Received: 12 January 2022; Accepted: 11 February 2022;
Published: 14 March 2022.
Edited by:
Anna Maria Giudetti, University of Salento, ItalyReviewed by:
Stefan Kabisch, Charité Universitätsmedizin Berlin, GermanyIlaria Riboldi, University of Milano-Bicocca, Italy
Copyright © 2022 Liu, Deng, Xue, Yang, Yang, Gong and Tang. This is an open-access article distributed under the terms of the Creative Commons Attribution License (CC BY). The use, distribution or reproduction in other forums is permitted, provided the original author(s) and the copyright owner(s) are credited and that the original publication in this journal is cited, in accordance with accepted academic practice. No use, distribution or reproduction is permitted which does not comply with these terms.
*Correspondence: Zhicheng Gong, Z29uZ3poaWNoZW5nMjAxM0AxNjMuY29t; Mimi Tang, dGFuZ21pbWkxOTg5QDE2My5jb20=
†These authors have contributed equally to this work and share first authorship