- 1State Key Laboratory for Conservation and Utilization of Subtropical Agro-Bioresources, Guangxi University, Nanning, China
- 2College of Animal Science and Technology, Guangxi University, Nanning, China
Siraitia grosvenorii is a kind of medicinal food plant. The mogroside-rich extract (MGE) of its fruits can effectively ameliorate obesity, but the underlying mechanisms remain underexplored. In this study, we aimed to determine whether MGE can ameliorate obesity by protecting against the divergences of gut microbiota. Mice were challenged with a high-fat diet (HFD) and treated with MGE by oral gavage. Then, the characteristics of the gut microbiota were determined by 16S rDNA analysis. Our findings showed that MGE could significantly reduce body weight gain and fat tissue weight of the mice fed with HFD. Moreover, MGE markedly attenuated fatty liver, and improved glucose tolerance and insulin sensitivity. We further found that the gut microbiota structures were disturbed by HFD feeding. In particular, the abundance of Firmicutes was increased and the abundance of Bacteroidetes was decreased, resulting in an increased proportion of Firmicutes to Bacteroidetes (F/B), which contributes to obesity. Interestingly, the abnormal proportion of F/B of HFD feeding mice was restored to the level of control mice by MGE treatment. Additionally, the abundances of obesogenic microbiota, such as Ruminiclostridium and Oscillibacter were also decreased after MGE treatment. In summary, our findings demonstrate that MGE can modulate gut microbiota in obese mice and shed new light on how it alleviates obesity.
Introduction
Obesity is a worldwide pandemic in modern society. It increases the risk of various health problems and has become a leading contributor to diabetes mellitus, cardiovascular diseases, and cancers (1). Given the detrimental effects of obesity on human health, diverse strategies, including surgical operation (2), medicines (3), Chinese medicine conditioning, acupuncture (4, 5), exercise and diet, have been developed to control body weight gain or alleviate obesity (6). Among them, plant extracts with medical and edible properties are receiving increasing attention because they are characterized by effectiveness, safety, and pleasant properties (7, 8).
Siraitia grosvenorii (Luo han guo) is a kind of medicinal food plant primarily grown in South China (9, 10). Mogrosides (MGs), the major bioactive components of S. grosvenorii fruit, have rich medicinal efficiency, namely, anti-inflammation (11, 12), anti-oxidative stress (13, 14), anticancer (15), neuroprotective (16), and promoting reproduction (17–19). For protecting against obesity, in vitro studies have shown that mogrol inhibited adipogenesis in the 3T3L cell line by activating AMP-activated protein kinase (AMPK) activity (20). In vivo studies have shown that MGs can reduce body weight gain and attenuate non-alcoholic fatty liver disease (NAFLD) by enhancing the phosphorylation levels of AMPK in the livers of mice challenged with a high-fat diet (HFD) (21). Similarly, Liu et al. reported that mogroside-rich extract (MGE) can alleviate hyperglycemic and hyperlipidemic syndromes in HFD/streptozotocin-induced diabetic mice, in part due to the improvement of insulin sensitivity and activation of hepatic AMPK signaling (22). Although the effects of MGE on attenuating obesity were widely explored, the underlying mechanisms still have not been sufficiently elucidated.
Microbes settle in the gut and play fundamental roles in maintaining host health. In contrast, intestinal dysbacteriosis is closely linked to a variety of health problems, particularly the pathophysiology of obesity (23). It has been revealed that not only between genetically obese mice and their lean littermates but also between obese and lean human volunteers, gut microbial diversity and gut microbiota compositions are changed, especially the relative abundance of Bacteroidetes and Firmicutes, which are decreased and increased, respectively, in obese individuals (24, 25). Conversely, shaping gut microbiota by colonizing “lean microbiota” or eating certain food can alleviate obesity and metabolic disorders (26–29).
In this study, we speculated that MGs might alleviate obesity associated with the modulation of gut microbiota. To test this hypothesis, male mice were challenged with an HFD to establish a diet-induced obese (DIO) animal model, concomitantly orally administered MGE to determine the effects on alleviating obese phenotypes. Additionally, gut microbial diversities and compositions were characterized to determine how MGE modulates gut microbiota. This study can help us to extend the current understanding of how MGs alleviate obesity.
Materials and Methods
Ethical Approval and Animals
The animal experiments were approved by the Institutional Animal Care and Use Committee (IACUC) of Guangxi University and were conducted in accordance with the animal welfare and ethical rules. Three-week-old male C57BL/6 mice were purchased from Beijing Vital River Laboratory Animal Technology Company (Beijing, China). Mice were maintained in individually ventilated cages (IVCs) under 12 h light/12 h dark cycles at an ambient temperature of 22 ± 2°. Mice were ad libitum accessed to food and water.
Treatments
After acclimating for one week, the mice were randomly divided into four groups with eight mice each in group (control, HFD, HFD + MGE300, and HFD + MGE600). The control mice were fed with a chow diet (10% energy from fat, D12450, Research Diets, New Brunswick, NJ) and the other mice were challenged with an HFD (60% energy from fat, D12492, Research Diets). Mice in the control and HFD groups were given orally 100 μl water at 9:00 a.m., while the HFD + MGE300 and HFD + MGE600 mice were orally administered 300 mg/kg or 600 mg/kg body weight MGE, respectively. During treatment for 18 weeks, the body weight and food intake were recorded weekly during treatment.
TheMGE was provided by Layn Biotech, Inc. (Guilin, China). Its main constituents were described in our previous publication (14).
Body Fat Percentage Measurement
The body fat percentage of mice was assayed by using Small Animal Body Composition Analysis and Imaging System NMR Analyzer (MesoQMR23-060H, Niumag Corporation, Shanghai, China).
Glucose Tolerance Test and Insulin Tolerance Test
Mice were subjected to Glucose Tolerance Test (GTT) (at 19 weeks of age) and Insulin Tolerance Test (ITT) (at 20 weeks of age) as previously described (30). Briefly, mice were fasted for 12 h or 6 h and then intraperitoneally injected with 2 g/kg body weight D-glucose or 1 IU/kg body weight insulin for GTT and ITT, respectively. Blood was collected from the tail tip at 0, 15, 30, 60, and 120 min post-injection. Glucose concentration was measured using an Accu-Chek Performa (Roche Diagnostics, United States).
Liver Triglyceride Content Assay
The triglyceride (TG) content in the liver was measured using a TG colorimetric assay kit (Applygen Technologies, Beijing, China) according to the manufacturer’s instructions. Fifty milligrams of liver tissue were grounded in liquid nitrogen, and then TG was extracted. TG concentration was measured using a BioTek Epoch full-wavelength microplate reader (Epoch, United States) at a 550 nm wavelength.
Hematoxylin and Eosin Staining
Fat and liver tissues were fixed in 4% paraformaldehyde (PFA) overnight at 4°. The samples were embedded in paraffin and cut into 5 μm thick sections according to routine procedures. Hematoxylin and Eosin (H&E) staining was performed according to a standard procedure. Images were captured under a light microscope (Nikon, Eclipse 50i).
Colonic Microbiota Analyses
The structures of the colonic microbiota were assessed by 16S rRNA amplicon gene sequencing, which was performed by Biomarker Technologies (Beijing, China). Samples were collected for the colons and total genomic DNA was extracted by using the TIANamp Stool DNA Kit (TIANGEN Biotech, Beijing, China), and the V3-V4 regions of the 16S rRNA gene were amplified by using the forward primer 338F (5′-ACTCCTACGGGAGGCAGCA-3′) and reverse primer 806R (5′-GGACTACHVGGGTWTCTAAT-3′). The operational taxonomic unit (OTU) table and taxonomic analysis were obtained from Qiime2 (2019.10) software (31).
Statistical Analyses
Post hoc analyses were performed using Prism 7 software (GraphPad, San Diego, CA, United States) when significance was achieved in the main or simple effects model. Multiple comparisons were corrected using Tukey–Kramer or Dunnett–Hsu adjustment in mixed model procedures. Data are presented as means ± SEM. P < 0.05 was considered statistically significant for a null hypothesis of no difference between study test meals. Bioinformatics analyses were carried out at the Multifunction Computer Center of Guangxi University and the R platform (version 4.0.3).
Results
Mogroside-Rich Extract Attenuates Body Weight Gain and Fat Accumulation in Diet-Induced Obese Mice
After challenge with a HFD for eighteen weeks, the mice had vastly higher body weights (Con vs. HFD; 25.1 ± 0.15 vs. 45.9 ± 0.95 g; P < 0.0001) and body fat percentage (Con vs. HFD; 7.7 ± 0.25 vs. 44.4 ± 0.41%; P < 0.0001) than the mice fed the control diet. There were no significant differences in body weight or body fat content between mice in the HFD and HFD + MGE300 groups, indicating that 300 mg/kg MGE does not attenuate obesity. However, when the mice were treated with 600 mg/kg MGE, the body weight and body fat content were significantly reduced compared with those of the HFD group (Figures 1A,B), indicating that 600 mg/kg MGE can attenuate HFD-induced obesity. In addition, compared with the control group, the high-fat diet increased the average weekly calorie intake of mice. However, there was no difference in calorie intake between MGE treated group (MGE300, MGE600) and the HFD group (Figure 1C). For individual adipose tissue, when mice were fed an HFD, the inguinal white adipose tissue (iWAT) and epididymal white adipose tissue (eWAT) weights were notably increased compared with those of the mice fed a control diet. A 300 mg/kg MGE did not decrease the iWAT or eWAT weight in the mice fed an HFD. However, 600 mg/kg MGE significantly reduced iWAT weight but had no impact on eWAT weight in the mice fed a HFD (Figure 1D). Consistently, the HFD challenge obviously increased the adipocyte size of iWAT and eWAT in the mice. Interestingly, 600 mg/kg MGE treatment reduced the adipocyte size of iWAT but did not change the adipocyte size of eWAT in DIO mice (Figures 1E–G). Considering the effects of 600 mg/kg MGE on alleviating obesity, this dose was used in the subsequent analyses.
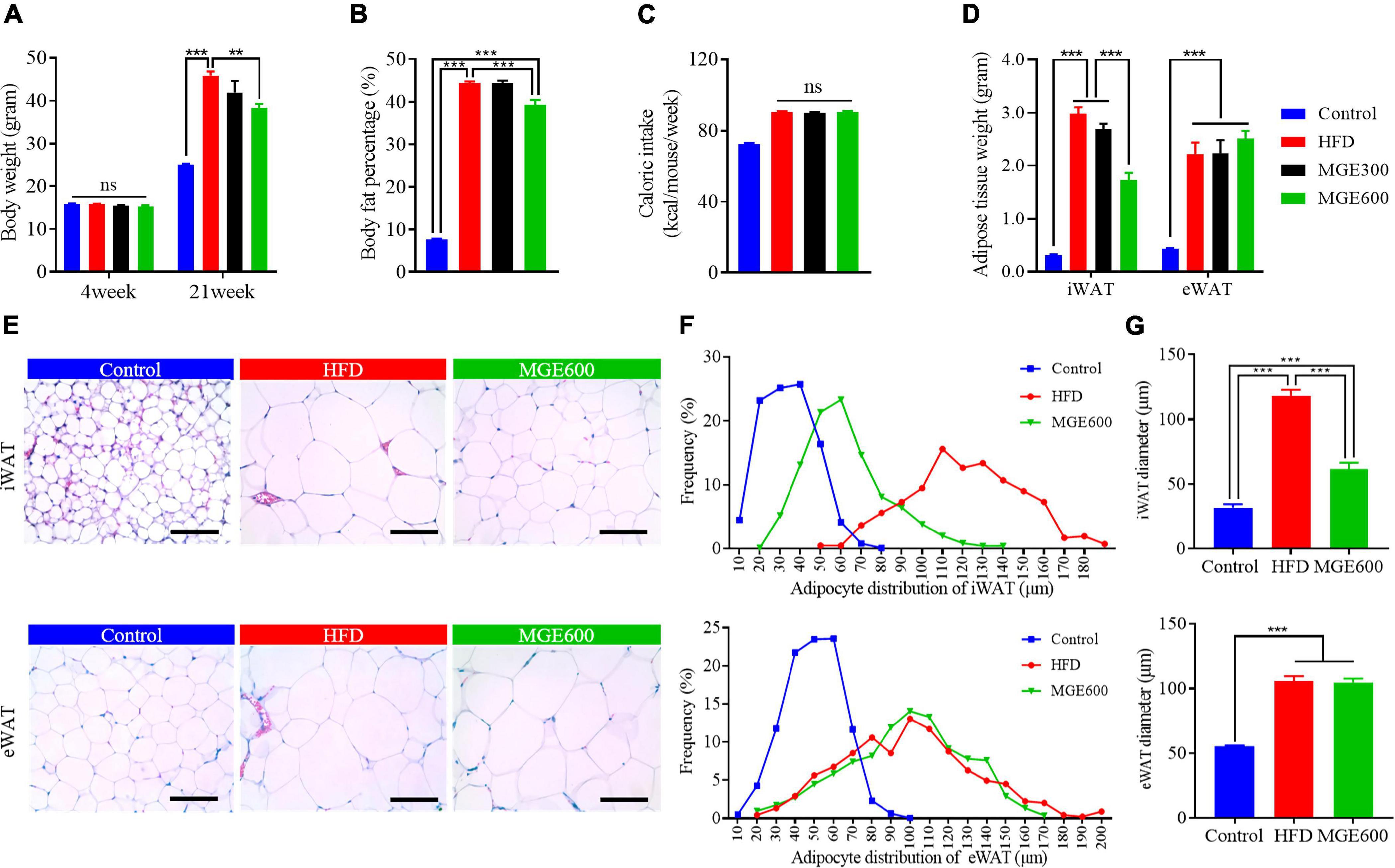
Figure 1. MGE attenuates body weight gain and reduces fat mass in DIO mice. Diet-induced obese (DIO) mice were orally administered 300 and 600 mg body weight MGE from 4 to 21 weeks of age. (A) Body weight at the beginning and end of the experiment (n = 12). (B) Body fat percentage at the end of the experiment (n = 8). (C) Weekly average calorie intake per mice. (D) Inguinal adipose tissue (iWAT) and epididymal adipose tissue (eWAT) weight (n = 6). (E–G) Representative images of H&E staining, adipocyte distribution, and average adipocyte diameter of iWAT and eWAT (n = 6). Scale bar = 100 μm; magnification 20×. **P < 0.01, ***P < 0.001.
Mogroside-Rich Extract Reduces Liver Fat Accumulation and Improves Glucose and Insulin Sensitivity in Diet-Induced Obese Mice
We next determined whether MGE treatment might ameliorate fatty liver in DIO mice. As shown in Figure 2A, the mice in the HFD group had higher liver weights than those in the control group (Con vs HFD; 1.3 ± 0.03 vs 1.7 ± 0.13 g; P < 0.05). H&E staining showed that there were many lipid droplets distributed in the liver of HFD-challenged mice (Figure 2B), suggesting that HFD feeding results in fatty liver. We further assayed TG content in the liver and found that the mice in the HFD group had significantly higher TG content than the mice in the control group (Figure 2C). This further confirmed that the HFD challenge leads to fatty liver in mice. As expected, MGE treatment markedly reduced the liver weight and TG content in the livers of mice challenged with an HFD, indicating that MGE can alleviate fatty liver in DIO mice (Figures 2A–C).
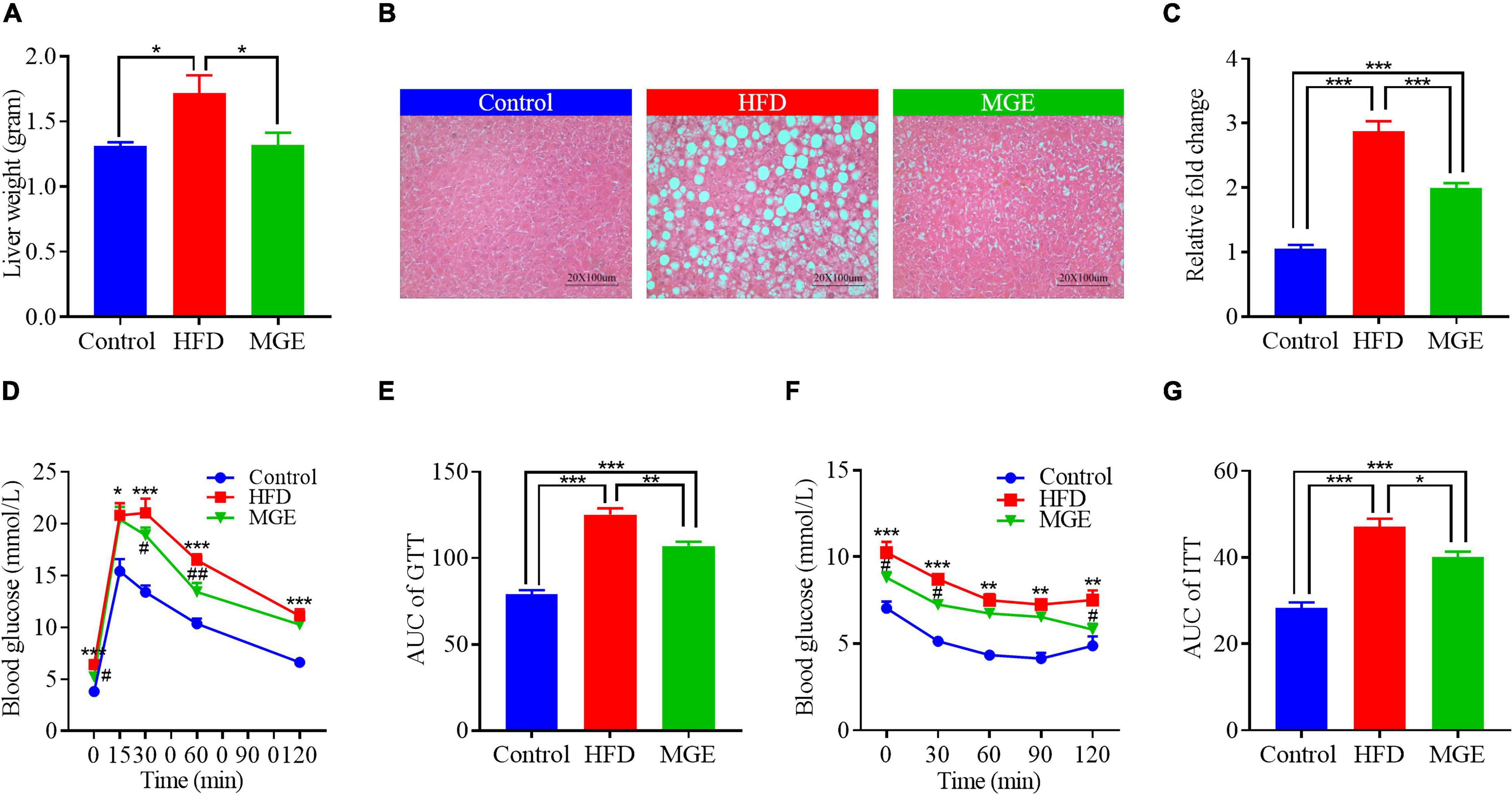
Figure 2. MGE reduces liver fat accumulation and improves glucose and insulin sensitivity in DIO mice. (A) Liver weight of mice (n = 7). (B) Representative images of liver tissue H&E staining. Scale bar = 100 μm. (C) Liver triglyceride concentration relative to the control (n = 7). (D,E) Glucose tolerance test (GTT; n = 6). (F,G) Insulin tolerance test (ITT; n = 5). AUC, area under curve. *P < 0.05, **P < 0.01, ***P < 0.001; #P < 0.05, ##P < 0.01 vs. HFD group.
Compared with the control group, the HFD group had significantly higher AUCs of GTT and ITT (Figures 2D–G), indicating that the HFD challenge reduces glucose and insulin sensitivity in mice. However, MGE treatment notably reduced the AUCs of the GTT and ITT in the mice challenged with a HFD (Figures 2D–G). Taken together, MGE supplementation alleviated fatty liver and improved global metabolic capacities in DIO mice.
Effects of Treatment on Intestinal Microbiota α-Diversity and β-Diversity
High-throughput sequencing generated 1,919,310 raw reads. After removing the low-quality sequences, there were 1,787,645 clean tags, and each sample produced an average of 74,485 clean tags. Based on a 97% similarity cutoff value, all the effective reads were clustered into operational taxonomic units (OTUs).
To identify the differences in intestinal microbiota structure among groups, the α-diversity and β-diversity were compared. As shown in Figures 3A–D, the α-diversity indexes (ACE, Shannon, and phylogenetic diversity) in the HFD group were significantly increased compared with those in the control group, but there were no differences between the HFD and MGE groups. On the other hand, non-metric multidimensional scaling (NMDS) and principal coordinates analysis (PCoA) of the unweighted UniFrac distance matrix were used to identify β-diversity. As shown in Figures 3E,F, NMDS and PCoA showed that the clusters of the HFD group were significantly different from those in the control group, but MGE treatment significantly ameliorated these alters. Taken together, the above results indicate that MGE can restore the changes of community structure in microbiota but not species types in DIO mice.
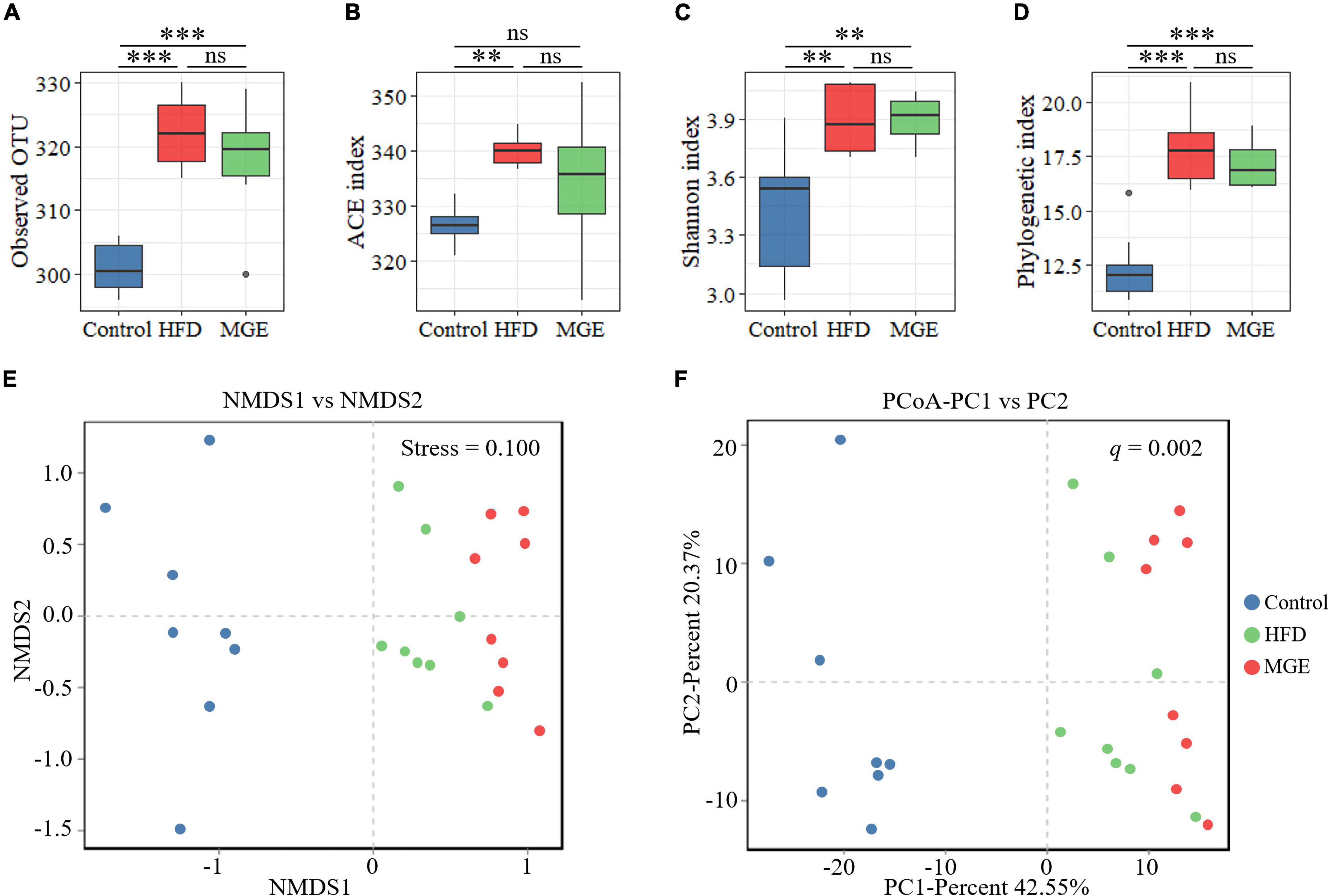
Figure 3. Effects of MGE treatment on intestinal microbiota α-diversity and β-diversity. (A) Observed OTU in each group. (B) Abundance-based coverage estimator (ACE) index. (C) Shannon index. (D) Phylogenetic diversity (PD). (E,F) Beta diversity analysis: (E) non-metric multidimensional scaling (NMDS), (F) principal coordinates analysis (PCoA) of the unweighted UniFrac distance matrix. Group significance was statistically analyzed by using the PERMANOVA method. **P < 0.01, ***P < 0.001.
Mogroside-Rich Extract Restores the Relative Abundance of Firmicutes and Bacteroidetes in Diet-Induced Obese Mice
Combining the unweighted pair-group method with arithmetic mean (UPGMA), the relative abundance of the gut microbiota at the phylum level was identified by QIIME2 taxonomic analysis. As shown in Figure 4A, the HFD group had an apparent difference in taxonomic composition compared with the control group, but there was an apparent similarity between the MGE group and the control group. For individual phyla, the relative abundance of Firmicutes was significantly increased, but that of Bacteroidetes was significantly decreased due to HFD feeding, resulting in an increase in the ratio of Firmicutes to Bacteroidetes (F/B) in DIO mice (Figure 4B). However, MGE treatment restored the relative abundance of Firmicutes and Bacteroidetes, as well as the ratio of F/B to control levels, in DIO mice (Figure 4B). In addition, the linear discriminant analysis effect size (LEfSe) method was used to further determine differences in microbiota abundances (Figure 4C). There was 67 differentially abundant microbiota, and 49 out of 67 (73.1%) belonged to Bacteroidetes and Firmicutes, which further confirmed that MGE can restore the abundance of Firmicutes and Bacteroidetes in DIO mice.
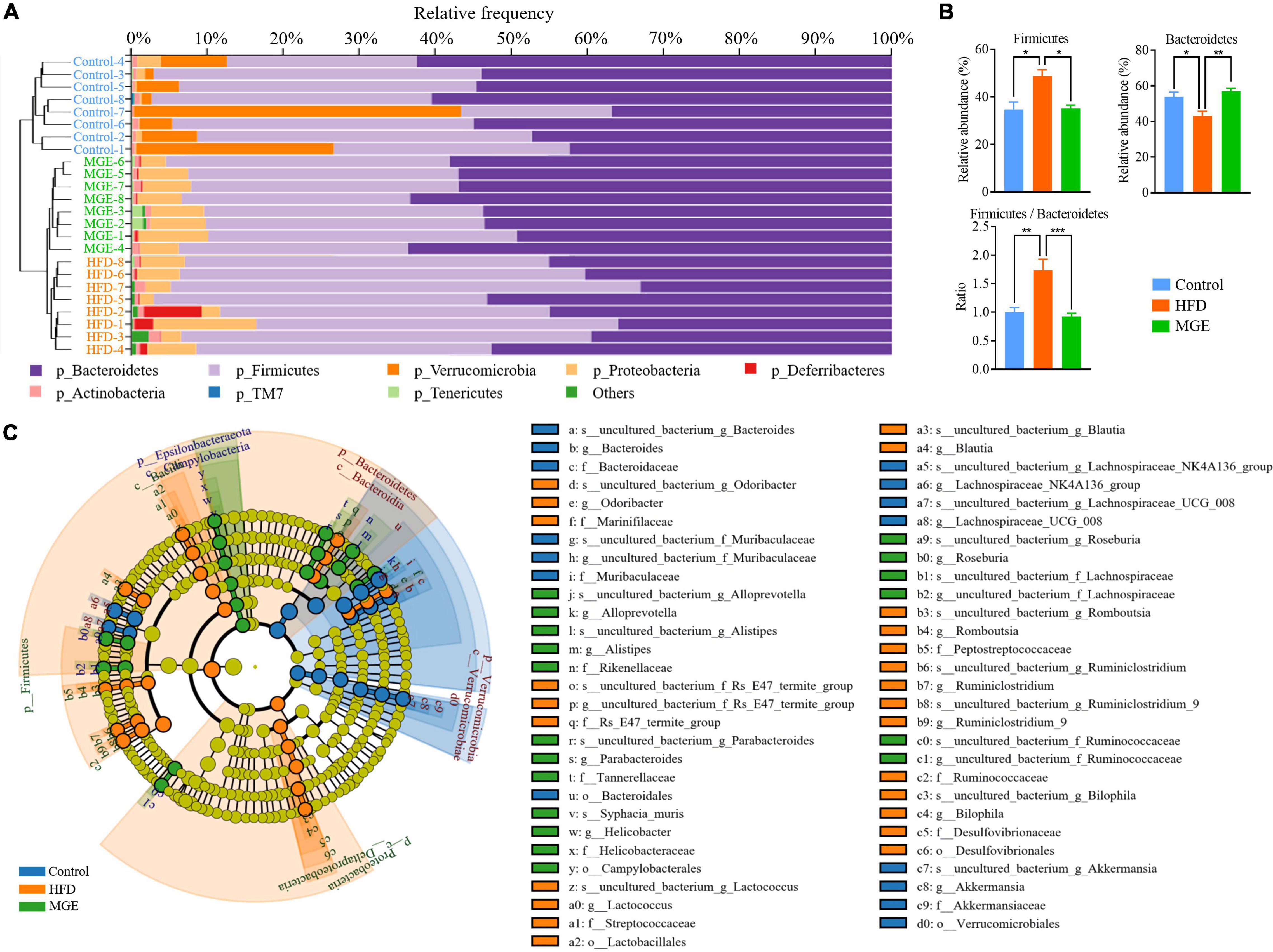
Figure 4. MGE restores the relative abundance of Firmicutes and Bacteroidetes in DIO mice. (A) Microbiota taxonomic composition clustering histogram at the phylum level; left: unweighted pair-group method with arithmetic mean (UPGMA) tree; right: histogram of microbiota abundance. (B) Relative abundance of Firmicutes and Bacteroidetes and the ratio of Firmicutes to Bacteroidetes. (C) Taxonomic cladogram obtained from linear discriminant analysis effect size (LEfSe) sequence analysis. Species taxonomy from phyla to genus (inside-outside). The diameter of each circle represents the relative abundance of the taxon, and the color corresponds to the grouping. Undifferentiated microbiota components (yellow). The predominant microbiota component at each taxonomic level is represented by different colors. *P < 0.05, **P < 0.01, ***P < 0.001.
Mogroside-Rich Extract Restores the Abundance of Intestinal Microbiota at the Genus Level in Diet-Induced Obese Mice
To further explore the effect of MGE on bacterial taxonomic composition at the genus level, a clustering heatmap was constructed. Figure 5A displays the top 72 OTUs with significant differences (P < 0.01) selected for clustering analysis. Fifty-eight out of 72 OTUs were significantly less abundant in the control group and MGE group than in the HFD group. Interestingly, Lactococcus, Blautia, Lactobacillus, Ruminiclostridium_9, Oscillibacter, and Ruminiclostridium belong to Firmicutes and are obesogenic microbiota (Figure 5B). In contrast, 14 out of 72 OTUs, including Bacteroides, were significantly more abundant in the control group and MGE group than in the HFD group (Figure 5B). Taken together, MGE can restore the intestinal microbiota at the genus level in DIO mice.
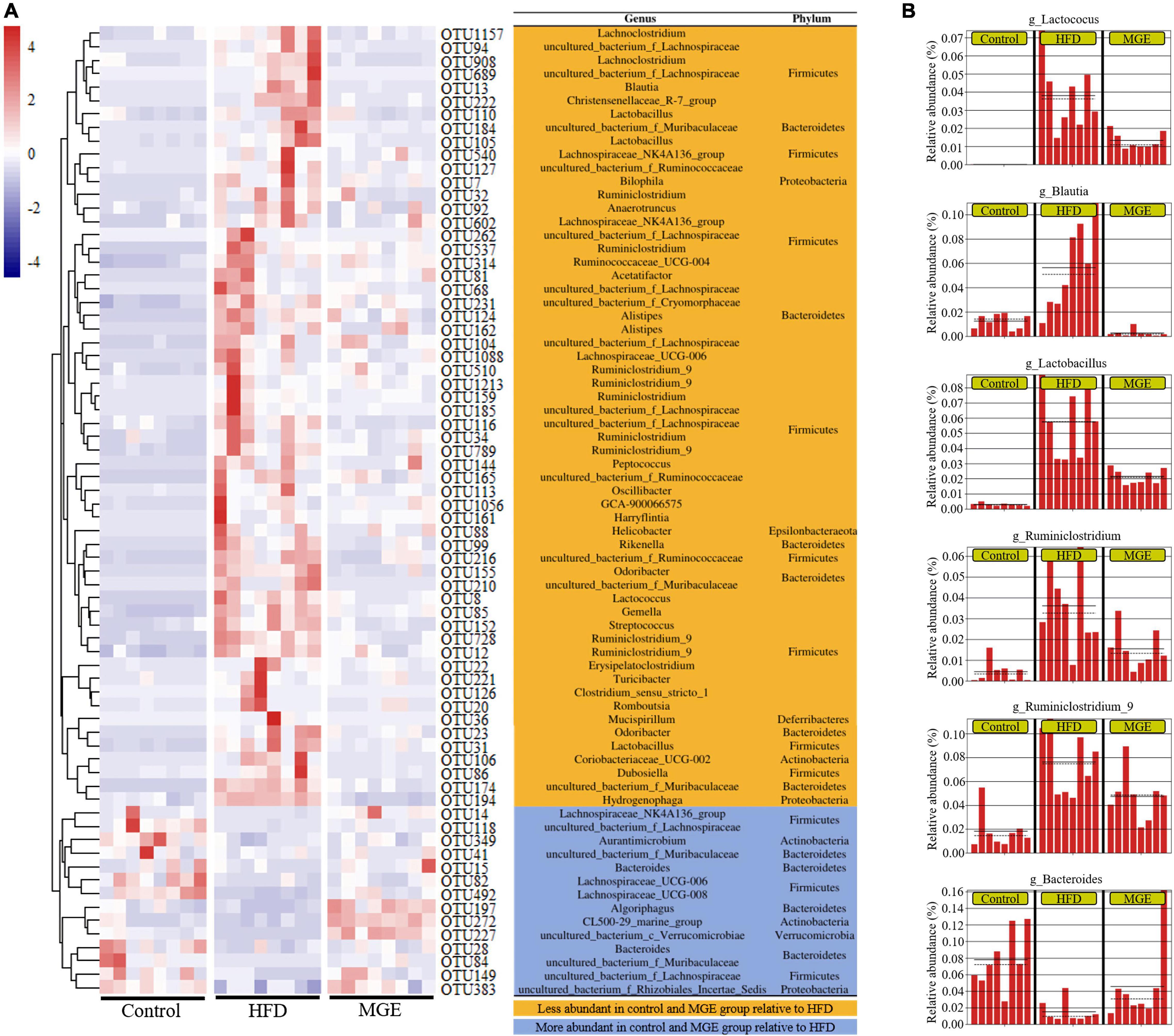
Figure 5. MGE restores the abundance of intestinal microbiota at the genus level in DIO mice. (A) OTU differential clustering heatmap with representative bacterial taxon information (genus and phylum). (B) The relative abundance of bacterial genera significantly recovered by MGE treatment (P < 0.01); solid and dashed lines indicate the mean and median, respectively.
Correlation Analyses of Gut Microbiota Structures and Obese Phenotypes
We further performed a correlation analysis between the gut microbiota structures and obese phenotypes. Based on the Spearman index, we found that the abundances of Firmicutes, Lactobacillus, and Lactococcus were positively correlated with obese phenotypes, such as body weight, iWAT weight, body fat content, liver TG concentration, and AUC of GTT and ITT. However, the abundances of Bacteroidetes and Bacteroides were negatively correlated with the above obesity parameters (Figure 6A). The results of correlation analysis show that MGs alleviate obesity and maybe associated with the gut microbiota modulation (Figure 6B).
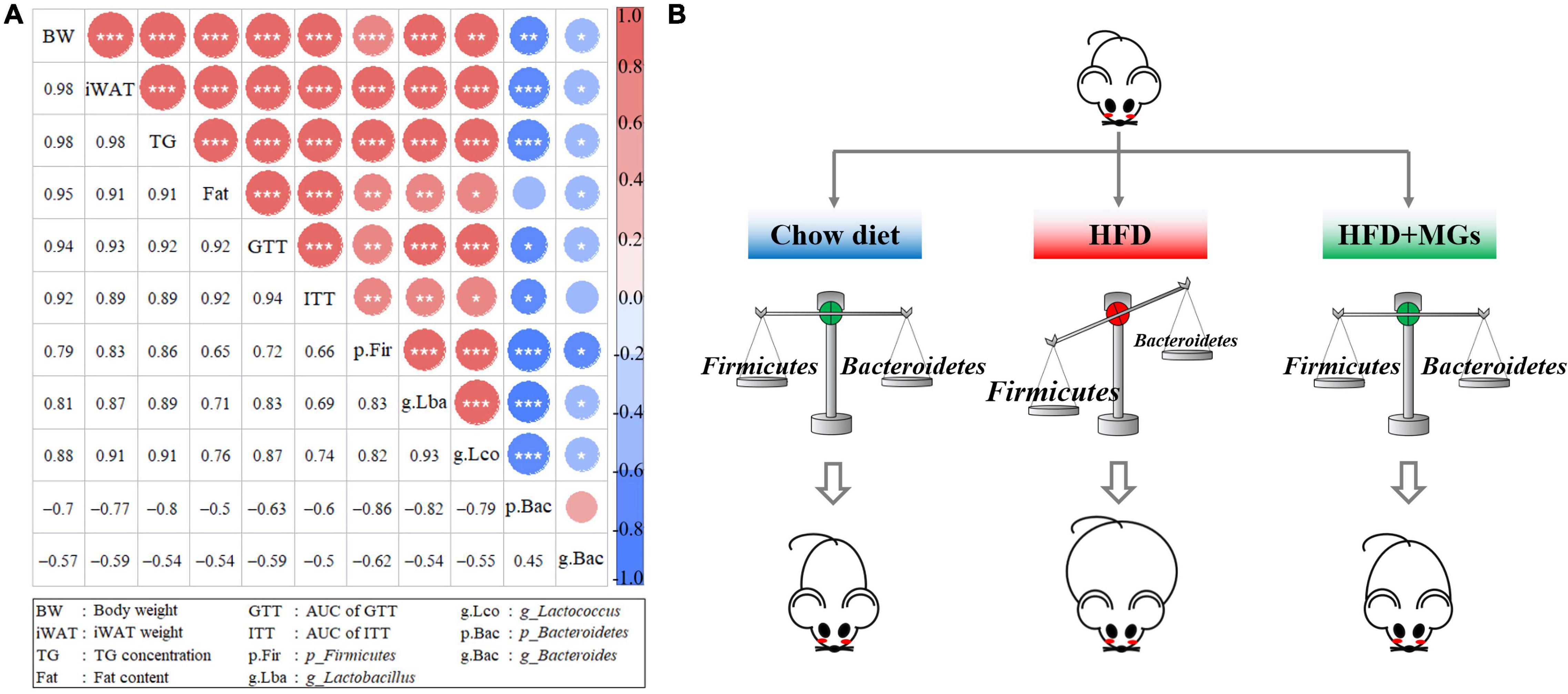
Figure 6. Correlation analyses of gut microbiota structures and obese phenotypes. (A) The diagonals represent the indicator; positive correlation (red), negative correlation (blue); the circle diameters correspond to the absolute value of correlation coefficients; the asterisk corresponds to significance: *P < 0.05, **P < 0.01, ***P < 0.001. (B) The cartoon represents how MGs attenuate obesity by shaping gut microbiota.
Discussion
Previous studies have demonstrated that MGs can attenuate obesity, but their effect on modulating gut microbiota in this condition remains unknown. In this study, we first observed that MGE treatment can alleviate obesity by inhibiting body weight gain and fat accumulation in adipose tissues and liver, improving insulin and glucose sensibilities in DIO mice. Importantly, we further found that MGE treatment modulates gut microbiota, particularly restores the ratio of Firmicutes to Bacteroidetes and reduces the relative abundance of obesogenic microbiota. Our findings depict that MGE attenuates obesity at least in part by gut microbiota modulation.
First, we used a DIO mouse model to determine the beneficial effects of MGs on obese animals. When the mice were fed an HFD from 4 to 21 weeks of age, the body weight, global fat mass, and adipose tissue weight were vastly increased. In addition, the increased adipocyte size and accumulated fat content in the liver indicated that the HFD challenge induced hypertrophic adipose tissues and NAFLD, respectively. Moreover, HFD feeding also impairs global metabolic capacities by reducing glucose and insulin sensibilities. Combined with previous reports (32–35), all the above defective parameters demonstrated that a DIO animal model was successfully established in this study. As expected, when DIO mice were orally administered MGE, body weight gain and fat accumulation were attenuated, and global metabolic capacities were also improved. Our findings reconfirmed that MGs can alleviate obesity (21, 22, 36, 37); therefore, this model can be used to explore the modulation of gut microbiota by MGE.
We next used 16S sequencing to investigate how MGE affects gut microbial compositions and diversities in DIO mice. α-Diversity and β-diversity are two key indicators that reflect the differences in gut microbial communities (38, 39). We found that the α-diversity of the gut microbiota was increased in DIO mice but was not altered by MGE treatment. Similarly, the β-diversity of the gut microbiota was vastly different between the control and HFD groups. Interestingly, MGE treatment could partly restore this alteration in DIO mice. Consistently, a previous study showed that MG can alleviate metabolic disorders by regulating the gut microbiota in T2DM rats (40). This study and a previous report (40) show that MGs can regulate gut microbiota communities, which is a potential mechanism by which MGs attenuate metabolic disorders.
We further found that the abundance of Firmicutes was increased while the abundance of Bacteroidetes was decreased in DIO mice, whereas these changes were recovered by MGE treatment. It has been revealed that Firmicutes are positively associated with the pathogenesis of obesity; in contrast, Bacteroidetes exhibit anti-obesity activity (41). Moreover, the abundance of Firmicutes is increased and that of Bacteroidetes is reduced in obese individuals (42). Thus, on the one hand, the reduction of Firmicutes abundance by antibiotics (vancomycin and bacitracin) can improve insulin resistance in DIO mice (43). On the other hand, Bacteroidetes abundance is significantly decreased in leptin-deficient obese mice compared with lean mice (44) because Bacteroidetes can obtain energy from indigestible polysaccharides and produce SCFAs to regulate energy metabolism (45). Therefore, MGs attenuate obesity was associated with the intestinal microbiota of specific Firmicutes and Bacteroidetes at the phylum level.
We next deeply explored the effects of MGE on the intestinal microbiota at the genus level in DIO mice. We observed decreased abundances of Bacteroides and increased abundances of Lactobacillus, Lactococcus, Ruminiclostridium, Ruminiclostridium_9, Oscillibacter, and Blautia in DIO mice. Interestingly, these changes were effectively restored by MGE treatment. Consistently, germ-free mice colonized with gut microbiota from DIO mice showed NAFLD, suggesting that certain gut microbiota, such as the genus Bacteroides, are responsible for hepatic lipid accumulation (46–48). In addition, Lactobacillus was positively correlated with insulin resistance and body weight gain. Furthermore, studies have revealed that Lactococcus, Ruminiclostridium, Ruminiclostridium_9, Oscillibacter and Blautia are associated with obesity in animal models and humans (49–51). Furthermore, our correlation analyses show that HFD-mediated gut microbiota abundance changes are significantly related to obesity phenotypes, e.g., body weight gain, fat mass, and insulin sensitivity.
Conclusion
Siraitia grosvenorii is a kind of medicinal food plant, and its fruit extracts have anti-obesity effects. Our findings reveal that MGE can alleviate intestinal dysbacteriosis in obese mice. Of note, fecal microbiota transplantation (FMT) or co-housing experiments should further strengthen the conclusion that MGE alleviates obesity by gut microbiota modulation.
Data Availability Statement
The datasets presented in this study can be found in online repositories. The name of the repository and accession number can be found below: National Center for Biotechnology Information (NCBI) BioProject, https://www.ncbi.nlm.nih.gov/bioproject/, PRJNA811511.
Ethics Statement
The animal study was reviewed and approved by Institutional Animal Care and Use Committee (IACUC) of Guangxi University.
Author Contributions
SW, KC, and XL conceived the study. SW, KC, JL, JH, KY, and PX performed the experiments. SW, XY, YL, and XL analyzed the data and wrote the manuscript. All authors reviewed the manuscript and approved the final version of the manuscript.
Funding
This work was supported by the National Natural Science Foundation of China (No. 82160287), the Scientific Research Foundation of Guangxi University (XTZ170099), the Guangxi Natural Science Foundation Program (2018GXNSFAA138125 and 2020GXNSFAA159099), and the One-hundred Talent Program of Guangxi and the Funding from State Key Laboratory for Conservation and Utilization of Subtropical Agro-bioresources (SKLCUSA-b201913).
Conflict of Interest
The authors declare that the research was conducted in the absence of any commercial or financial relationships that could be construed as a potential conflict of interest.
Publisher’s Note
All claims expressed in this article are solely those of the authors and do not necessarily represent those of their affiliated organizations, or those of the publisher, the editors and the reviewers. Any product that may be evaluated in this article, or claim that may be made by its manufacturer, is not guaranteed or endorsed by the publisher.
References
1. Bluher M. Obesity: global epidemiology and pathogenesis. Nat Rev Endocrinol. (2019) 15:288–98. doi: 10.1038/s41574-019-0176-8
2. Wright SM, Aronne LJ. Obesity in 2010: the future of obesity medicine: where do we go from here? Nat Rev Endocrinol. (2011) 7:69–70. doi: 10.1038/nrendo.2010.231
3. Msika S, Castel B. Present indications for surgical treatment of morbid obesity: how to choose the best operation? J Visc Surg. (2010) 147:e47–51. doi: 10.1016/j.jviscsurg.2010.08.015
4. Gong S, Ye T, Wang M, Wang M, Li Y, Ma L, et al. Traditional Chinese medicine formula Kang Shuai lao pian improves obesity, gut dysbiosis, and fecal metabolic disorders in high-fat diet-fed mice. Front Pharmacol. (2020) 11:297. doi: 10.3389/fphar.2020.00297
5. Abdi H, Zhao B, Darbandi M, Ghayour-Mobarhan M, Tavallaie S, Rahsepar AA, et al. The effects of body acupuncture on obesity: anthropometric parameters, lipid profile, and inflammatory and immunologic markers. ScientificWorldJournal. (2012) 2012:603539. doi: 10.1100/2012/603539
6. Chen Y, Ma G, Hu Y, Yang Q, Deavila JM, Zhu MJ, et al. Effects of maternal exercise during pregnancy on perinatal growth and childhood obesity outcomes: a meta-analysis and meta-regression. Sports Med. (2021) 51:2329–47. doi: 10.1007/s40279-021-01499-6
7. Trigueros L, Pena S, Ugidos AV, Sayas-Barbera E, Perez-Alvarez JA, Sendra E. Food ingredients as anti-obesity agents: a review. Crit Rev Food Sci Nutr. (2013) 53:929–42. doi: 10.1080/10408398.2011.574215
8. Wang HN, Xiang JZ, Qi Z, Du M. Plant extracts in prevention of obesity. Crit Rev Food Sci Nutr. (2020) 62:2221–34. doi: 10.1080/10408398.2020.1852171
9. Liu C, Dai L, Liu Y, Dou D, Sun Y, Ma L. Pharmacological activities of mogrosides. Future Med Chem. (2018) 10:845–50. doi: 10.4155/fmc-2017-0255
10. Gong X, Chen N, Ren K, Jia J, Wei K, Zhang L, et al. The fruits of Siraitia grosvenorii: a review of a chinese food-medicine. Front Pharmacol. (2019) 10:1400. doi: 10.3389/fphar.2019.01400
11. Qi X, Chen W, Liu L, Yao P, Xie B. Effect of a Siraitia grosvenori extract containing mogrosides on the cellular immune system of type 1 diabetes mellitus mice. Mol Nutr Food Res. (2006) 50:732–8. doi: 10.1002/mnfr.200500252
12. Sung YY, Kim SH, Yuk HJ, Yang WK, Lee YM, Son E, et al. Siraitia grosvenorii residual extract attenuates ovalbumin-induced lung inflammation by down-regulating Il-4, Il-5, Il-13, Il-17, and MUC5AC expression in mice. Phytomedicine. (2019) 61:152835. doi: 10.1016/j.phymed.2019.152835
13. Li Y, Zou L, Li T, Lai D, Wu Y, Qin S. Mogroside V inhibits LPS-Induced COX-2 expression/ROS production and overexpression of HO-1 by blocking phosphorylation of AKT1 in RAW264.7 Cells. Acta Biochim Biophys Sin. (2019) 51:365–74. doi: 10.1093/abbs/gmz014
14. Du Y, Liu J, Liu S, Hu J, Wang S, Cui K, et al. Mogroside-rich extract from Siraitia grosvenorii fruits protects against the depletion of ovarian reserves in aging mice by ameliorating inflammatory stress. Food Funct. (2022) 13:121–30. doi: 10.1039/d1fo03194e
15. Liu C, Dai L, Liu Y, Rong L, Dou D, Sun Y, et al. Antiproliferative activity of triterpene glycoside nutrient from monk fruit in colorectal cancer and throat cancer. Nutrients. (2016) 8:360. doi: 10.3390/nu8060360
16. Ju P, Ding W, Chen J, Cheng Y, Yang B, Huang L, et al. The protective effects of mogroside V and its metabolite 11-oxo-mogrol of intestinal microbiota against MK801-induced neuronal damages. Psychopharmacology. (2020) 237:1011–26. doi: 10.1007/s00213-019-05431-9
17. Nie J, Yan K, Sui L, Zhang H, Zhang H, Yang X, et al. Mogroside V improves porcine oocyte in vitro maturation and subsequent embryonic development. Theriogenology. (2019) 141:35–40. doi: 10.1016/j.theriogenology.2019.09.010
18. Yan K, Cui K, Nie J, Zhang H, Sui L, Zhang H, et al. Mogroside V protects porcine oocytes from lipopolysaccharide-induced meiotic defects. Front Cell Dev Biol. (2021) 9:639691. doi: 10.3389/fcell.2021.639691
19. Sui L, Yan K, Zhang H, Nie J, Yang X, Xu CL, et al. Mogroside V alleviates oocyte meiotic defects and quality deterioration in benzo(a)pyrene-exposed mice. Front Pharmacol. (2021) 12:722779. doi: 10.3389/fphar.2021.722779
20. Harada N, Ishihara M, Horiuchi H, Ito Y, Tabata H, Suzuki YA, et al. Mogrol derived from Siraitia grosvenorii mogrosides suppresses 3T3-L1 adipocyte differentiation by reducing camp-response element-binding protein phosphorylation and increasing amp-activated protein kinase phosphorylation. PLoS One. (2016) 11:e0162252. doi: 10.1371/journal.pone.0162252
21. Zhang X, Song Y, Ding Y, Wang W, Liao L, Zhong J, et al. Effects of mogrosides on high-fat-diet-induced obesity and nonalcoholic fatty liver disease in mice. Molecules. (2018) 23:1894. doi: 10.3390/molecules23081894
22. Liu H, Qi X, Yu K, Lu A, Lin K, Zhu J, et al. AMPK activation is involved in hypoglycemic and hypolipidemic activities of mogroside-rich extract from Siraitia grosvenorii (Swingle) fruits on high-fat diet/streptozotocin-induced diabetic mice. Food Funct. (2018) 10:151–62. doi: 10.1039/c8fo01486h
23. Ley RE, Turnbaugh PJ, Klein S, Gordon JI. Microbial ecology: human gut microbes associated with obesity. Nature. (2006) 444:1022–3. doi: 10.1038/4441022a
24. Turnbaugh PJ, Ley RE, Mahowald MA, Magrini V, Mardis ER, Gordon JI. An obesity-associated gut microbiome with increased capacity for energy harvest. Nature. (2006) 444:1027–31. doi: 10.1038/nature05414
25. Yin J, Li Y, Han H, Chen S, Gao J, Liu G, et al. Melatonin reprogramming of gut microbiota improves lipid dysmetabolism in high-fat diet-fed mice. J Pineal Res. (2018) 65:e12524. doi: 10.1111/jpi.12524
26. Cotillard A, Kennedy SP, Kong LC, Prifti E, Pons N, Le Chatelier E, et al. Dietary intervention impact on gut microbial gene richness. Nature. (2013) 500:585–8. doi: 10.1038/nature12480
27. Mardinoglu A, Wu H, Bjornson E, Zhang C, Hakkarainen A, Rasanen SM, et al. An integrated understanding of the rapid metabolic benefits of a carbohydrate-restricted diet on hepatic steatosis in humans. Cell Metab. (2018) 27:559–71.e5. doi: 10.1016/j.cmet.2018.01.005
28. Liu D, Huang J, Luo Y, Wen B, Wu W, Zeng H, et al. Fuzhuan brick tea attenuates high-fat diet-induced obesity and associated metabolic disorders by shaping gut microbiota. J Agric Food Chem. (2019) 67:13589–604. doi: 10.1021/acs.jafc.9b05833
29. Chang CJ, Lin CS, Lu CC, Martel J, Ko YF, Ojcius DM, et al. Ganoderma lucidum reduces obesity in mice by modulating the composition of the gut microbiota. Nat Commun. (2015) 6:7489. doi: 10.1038/ncomms8489
30. Liang X, Yang Q, Fu X, Rogers CJ, Wang B, Pan H, et al. Maternal obesity epigenetically alters visceral fat progenitor cell properties in male offspring mice. J Physiol. (2016) 594:4453–66. doi: 10.1113/JP272123
31. Bolyen E, Rideout JR, Dillon MR, Bokulich NA, Abnet CC, Al-Ghalith GA, et al. Reproducible, interactive, scalable and extensible microbiome data science using QIIME 2. Nat Biotechnol. (2019) 37:852–7. doi: 10.1038/s41587-019-0209-9
32. Liang X, Yang Q, Zhang L, Maricelli JW, Rodgers BD, Zhu MJ, et al. Maternal high-fat diet during lactation impairs thermogenic function of brown adipose tissue in offspring mice. Sci Rep. (2016) 6:34345. doi: 10.1038/srep34345
33. Tian Q, Zhao J, Yang Q, Wang B, Deavila JM, Zhu MJ, et al. Dietary alpha-ketoglutarate promotes beige adipogenesis and prevents obesity in middle-aged mice. Aging Cell. (2020) 19:e13059. doi: 10.1111/acel.13059
34. Zhang F, Ai W, Hu X, Meng Y, Yuan C, Su H, et al. Phytol stimulates the browning of white adipocytes through the activation of amp-activated protein kinase (AMPK) alpha in mice fed high-fat diet. Food Funct. (2018) 9:2043–50. doi: 10.1039/C7FO01817G
35. Zhang F, Su H, Song M, Zheng J, Liu F, Yuan C, et al. Calcium supplementation alleviates high-fat diet-induced estrous cycle irregularity and subfertility associated with concomitantly enhanced thermogenesis of brown adipose tissue and browning of white adipose tissue. J Agric Food Chem. (2019) 67:7073–81. doi: 10.1021/acs.jafc.9b02663
36. Lin GP, Jiang T, Hu XB, Qiao XH, Tuo QH. Effect of Siraitia grosvenorii polysaccharide on glucose and lipid of diabetic rabbits induced by feeding high fat/high sucrose chow. Exp Diabetes Res. (2007) 2007:67435. doi: 10.1155/2007/67435
37. Zhang Y, Zhou G, Peng Y, Wang M, Li X. Anti-hyperglycemic and anti-hyperlipidemic effects of a special fraction of luohanguo extract on obese T2DM rats. J Ethnopharmacol. (2020) 247:112273. doi: 10.1016/j.jep.2019.112273
38. Li J, Deng Q, Zhang Y, Wu D, Li G, Liu J, et al. Three novel dietary phenolic compounds from pickled Raphanus sativus L. Inhibit lipid accumulation in obese mice by modulating the gut microbiota composition. Mol Nutr Food Res. (2021) 65:e2000780. doi: 10.1002/mnfr.202000780
39. Zhang XY, Chen J, Yi K, Peng L, Xie J, Gou X, et al. Phlorizin ameliorates obesity-associated endotoxemia and insulin resistance in high-fat diet-fed mice by targeting the gut microbiota and intestinal barrier integrity. Gut Microbes. (2020) 12:1–18. doi: 10.1080/19490976.2020.1842990
40. Zhang Y, Peng Y, Zhao L, Zhou G, Li X. Regulating the gut microbiota and scfas in the faeces of T2DM rats should be one of antidiabetic mechanisms of mogrosides in the fruits of Siraitia grosvenorii. J Ethnopharmacol. (2021) 274:114033. doi: 10.1016/j.jep.2021.114033
41. Cao S-Y, Zhao C-N, Xu X-Y, Tang G-Y, Corke H, Gan R-Y, et al. Dietary plants, gut microbiota, and obesity: effects and mechanisms. Trends Food Sci Technol. (2019) 92:194–204. doi: 10.1016/j.tifs.2019.08.004
42. Jumpertz R, Le DS, Turnbaugh PJ, Trinidad C, Bogardus C, Gordon JI, et al. Energy-balance studies reveal associations between gut microbes, caloric load, and nutrient absorption in humans. Am J Clin Nutr. (2011) 94:58–65. doi: 10.3945/ajcn.110.010132
43. Hwang I, Park YJ, Kim YR, Kim YN, Ka S, Lee HY, et al. Alteration of gut microbiota by vancomycin and bacitracin improves insulin resistance via glucagon-like peptide 1 in diet-induced obesity. FASEB J. (2015) 29:2397–411. doi: 10.1096/fj.14-265983
44. Ley RE, Backhed F, Turnbaugh P, Lozupone CA, Knight RD, Gordon JI. Obesity alters gut microbial ecology. Proc Natl Acad Sci USA. (2005) 102:11070–5. doi: 10.1073/pnas.0504978102
45. Johnson EL, Heaver SL, Walters WA, Ley RE. Microbiome and metabolic disease: revisiting the bacterial phylum bacteroidetes. J Mol Med. (2017) 95:1–8. doi: 10.1007/s00109-016-1492-2
46. Le Roy T, Llopis M, Lepage P, Bruneau A, Rabot S, Bevilacqua C, et al. Intestinal microbiota determines development of non-alcoholic fatty liver disease in mice. Gut. (2013) 62:1787–94. doi: 10.1136/gutjnl-2012-303816
47. Qiao S, Bao L, Wang K, Sun S, Liao M, Liu C, et al. Activation of a specific gut bacteroides-folate-liver axis benefits for the alleviation of nonalcoholic hepatic steatosis. Cell Rep. (2020) 32:108005. doi: 10.1016/j.celrep.2020.108005
48. Turnbaugh PJ, Hamady M, Yatsunenko T, Cantarel BL, Duncan A, Ley RE, et al. A core gut microbiome in obese and lean twins. Nature. (2009) 457:480–4. doi: 10.1038/nature07540
49. Hippe B, Remely M, Aumueller E, Pointner A, Magnet U, Haslberger AG. Faecalibacterium prausnitzii phylotypes in type two diabetic, obese, and lean control subjects. Benef Microbes. (2016) 7:511–7. doi: 10.3920/BM2015.0075
50. Pekkala S, Munukka E, Kong L, Pollanen E, Autio R, Roos C, et al. Toll-like receptor 5 in obesity: the role of gut microbiota and adipose tissue inflammation. Obesity. (2015) 23:581–90. doi: 10.1002/oby.20993
Keywords: Siraitia grosvenorii, mogroside, obesity, high fat diet, gut microbiota
Citation: Wang S, Cui K, Liu J, Hu J, Yan K, Xiao P, Lu Y, Yang X and Liang X (2022) Mogroside-Rich Extract From Siraitia grosvenorii Fruits Ameliorates High-Fat Diet-Induced Obesity Associated With the Modulation of Gut Microbiota in Mice. Front. Nutr. 9:870394. doi: 10.3389/fnut.2022.870394
Received: 06 February 2022; Accepted: 05 May 2022;
Published: 13 June 2022.
Edited by:
Riadh Hammami, University of Ottawa, CanadaReviewed by:
Francesco Suriano, University of Gothenburg, SwedenYi-Chen Lo, National Taiwan Ocean University, Taiwan
Copyright © 2022 Wang, Cui, Liu, Hu, Yan, Xiao, Lu, Yang and Liang. This is an open-access article distributed under the terms of the Creative Commons Attribution License (CC BY). The use, distribution or reproduction in other forums is permitted, provided the original author(s) and the copyright owner(s) are credited and that the original publication in this journal is cited, in accordance with accepted academic practice. No use, distribution or reproduction is permitted which does not comply with these terms.
*Correspondence: Xingwei Liang, eHdsaWFuZ0BneHUuZWR1LmNu; Xiaogan Yang, eGd5YW5nQGd4dS5lZHUuY24=
†These authors have contributed equally to this work