- 1Department of Sports Medicine, China Medical University, Taichung City, Taiwan
- 2Department of Kinesiology, California State University, Stanislaus, Turlock, CA, United States
- 3Physical Education Office, Central Taiwan University of Science and Technology, Taichung City, Taiwan
- 4School of Nutrition, Chung Shan Medical University, Taichung City, Taiwan
- 5Department of Nutrition, Chung Shan Medical University Hospital, Taichung City, Taiwan
- 6Department of Physical Medicine and Rehabilitation, Changhua Christian Hospital, Changhua City, Taiwan
- 7Department of Post-Baccalaureate Medicine, College of Medicine, National Chung Hsing University, Taichung City, Taiwan
- 8Department of Physical Education, National Taichung University of Education, Taichung City, Taiwan
Aim: Quercetin has been reported to have antioxidant and anti-inflammatory properties on health promotion in human studies. The main purpose of this study was to investigate the effect of short-term oral quercetin supplementation on post-exercise whole-body energy metabolism. This study also aimed to determine the effects of supplementation on oxygen stress, inflammation, muscle damage, and high-intensity cycling exercise performance.
Method: Twelve healthy participants, physically active students, were recruited to perform a randomized, single-blind crossover study. All subjects completed 7-days of quercetin (quercetin:1,000 mg per day for 7-days) and placebo supplementation in a randomized order. Supplement/placebo was combined with exercise consisting of 70% V̇O2max cycling for 60-min, followed by 3-h of recovery, then a subsequent single bout of cycling exercise with 75% V̇O2max to exhaustion. Time to exhaustion, indicators of muscle damage, as well as blood and gaseous parameters relating to energy metabolism, oxidative stress, inflammatory response, respectively, were determined.
Results: The results showed that 7-day quercetin supplementation significantly attenuated the post-exercise glucose-induced insulin response, increased total antioxidant capacity (TAC) and superoxidase dismutase (SOD) activities, and mitigated malondialdehyde (MDA) levels during the recovery period (p < 0.05). While subsequent 75% V̇O2max cycling performance was significantly improved after quercetin treatment and accompanied by lower responses of interleukin 6 and creatine kinase at 24-h. However, it’s noted that there were no significant responses in glucose, respiratory exchange rate, tumor necrosis factor-α (TNF-α), myoglobin, and high sensitivity C-reactive protein between quercetin and placebo trials.
Conclusion: Our findings concluded that 7-day oral quercetin supplementation enhances high-intensity cycling time to exhaustion, which may be due in part to the increase in whole-body insulin-stimulated glucose uptake and attenuation of exercise-induced oxygen stress and pro-inflammation. Therefore, quercetin may be considered an effective ergogenic aid for enhancing high-intensity cycling performance among young adults.
Introduction
Attenuating oxidative stress, inflammation, and muscle damage induced by high-intensity exercise is the key factor for improving exercise performance (1). These effects have been shown in both animal and human models. Uchiyama et al. reported that rats had elevated levels of reactive oxygen species (ROS) and malondialdehyde (MDA) following repeated maximal resistance exercise until fatigue (2). This same study also indicated that muscle damage to the plantar flexor was highly associated with creatine kinase (CK) concentrations (2). As for humans, high-intensity exercise such as running up and down hills for 60 min has been shown to increase oxidative stress, inflammation, and muscle damage (3). Yet another study, involving 18 athletes, found that the concentration of thioredoxin (TRX) and myeloperoxidase (MPO) increased after completing a triathlon (1). In addition, an increase was also observed in CK levels in response to high-intensity triathlon exercise (1). Interestingly, 3-week supplementation of lactobacillus Plantarum PS128, an antioxidant supplement, attenuated exercise-induced oxidative stress and muscle damage levels which resulted in improved performance among the triathletes (1). Therefore, Huang’s study suggests that assuaging oxidative stress, inflammation, and muscle damage induced by mid- and high-intensity exercise may be an important physiological factor for enhancing performance.
Quercetin is a type of phytochemical belonging to the flavonoid family, hence it has antioxidant effects and the ability to decrease free radicals in the body (4). Quercetin can be purified from fruits and vegetables including onion, blueberry, and broccoli. Results from animal cell and human experiments show that quercetin has beneficial physiological effects such as improving insulin-stimulated whole-body glucose uptake, antioxidant capacity, and anti-inflammatory responses (5, 6). Additionally, Nieman et al., demonstrated the positive influence of fourteen days of quercetin (1,000 mg/day) exercise performance of 12-min time trial on the treadmill walking immediately after 60 min of moderate exercise preloads at 60% maximal oxygen uptake (V̇O2max) (7). McAnulty et al., evaluated the chronic quercetin effect on exercise-induced oxidative damage and inflammation, they recruited fourteen trained male cyclists who consumed 1,000 mg quercetin each day for 6 weeks before and during 3 days of cycling at 57% work maximum for 3 h (8). As compared to Nieman’s and McAnulty’s studies, we observe the participants in the present study performing 75% V̇O2max cycling exercise and recorded the time to exhaustion for all subjects following a 3-h recovery period at 70% V̇O2max exercise preload for 60 min under the shorter term of quercetin challenge (1,000 mg/day for 7 days). The questions of short-term quercetin and human exercise study need clarity regarding simulated cycling exercise challenge and post-exercise recovery during multiple races for cyclists in a one-day competition. However, some papers showing the ergogenic properties of quercetin were contrary (9–12). No effect of acute quercetin supplementation (2,000 mg) was found on 15 min cycling time trial following 30 min cycling exercise at a 50% V̇O2 peak under environmental circumstances of 40°C in non-heat-acclimated male volunteers by Cheuvront (9). Short-term (14 days) and long-term (6 weeks) quercetin supplementation (1,000 mg/d) both showed no ergogenic effect on the non-invasive measure of muscle oxidative capacity, physical fitness in healthy males, and V̇O2 peak measurement (10, 11). Moreover, Pelletier indicated that quercetin is unlikely to be evidence ergogenic for aerobic-oriented exercises in trained and untrained individuals by meta-analysis study (12). Nevertheless, whether the supplementation of quercetin alone can also improve the antioxidant enzymes and eliminate the oxidative stress caused by exercise remains unclear. Yet, no published human data are available regarding the effect of quercetin on post-exercise observation on whole-body insulin-stimulated glucose uptake, and indicators of oxidant stress, inflammation, muscle damage is a favor to improve subsequent cycling time to exhaustion. Especially, we wanted to demonstrate the effect of short-term quercetin supplementation (1,000 mg per day for 7 days) on exercise-induced oxidant damage and inflammation levels under simulating cycling competitions after exercise preloading.
It has been shown that the rate at which energy is replenished post-exercise, as well as decreased oxidative stress, inflammation, and muscle damage, is important for subsequent endurance exercise performance (13). Thus, athletes commonly use antioxidant supplements to accelerate energy recovery and eliminate oxidative stress and inflammation induced by exercise. In the present study, we explored whether 7-day quercetin supplementation can affect the blood energy metabolism index [glucose, insulin, and non-esterified fatty acids (NEFA)], oxidative markers [total antioxidant capacity (TAC), superoxidase dismutase (SOD), and MDA], inflammation markers [interleukin 6 (IL-6) and tumor necrosis factor-α (TNF-α)], muscle damage markers [CK, myoglobin (MB), and high sensitivity C-reactive protein (hs-CRP)], and time-to-exhaustion during two consecutive high-intensity cycling exercise bouts.
Materials and Methods
Subjects
The sample size was calculated in the present study using G* power (3.1.9.4) software assuming an effect size of 1.07 as observed previously in the human study regarding the ergogenic property of exercise time to exhaustion (14, 15). Therefore, 12 healthy male participants, physically active students, were recruited for this study supposing that the alpha error and statistic power are set at 0.05 and 90% (16). On average, their age was 20.79 ± 0.53 years old, the height of 171.10 ± 2.52 cm, bodyweight of 66.64 ± 2.67 kg, body mass index (BMI) of 22.86 ± 0.56 kg/m2, and the V̇O2max of 45.18 ± 1.74 mL/kg/min, respectively. Prior to the experimental period, all participants were asked to familiarize with the bicycle ergometer, and perform the V̇O2max test. During the recruiting process, the participants who were excluded included those whose chronic medical history such as cardiovascular disease, diabetes, musculoskeletal, or neuromuscular and were unable to complete the V̇O2max test under trouble respiration. All participants kept their dietary habits and avoid consuming food, supplements, and beverages not including antioxidant and anti-inflammation (e.g., coffee, tea, cola, chocolate, drugs, and nutritional products) during the experimental period. The experimental protocol was approved by the Institutional Review Board at the University of Taipei, Taipei, Taiwan (UT-IRB-2018-085). The testing protocol was thoroughly explained to the participants and informed consent forms were signed in order to participate. Participants were allowed to voluntarily remove themselves from the study at any time without reason.
Experimental Design and Procedure
Twelve healthy participants, physically active students, were recruited for the single-blind crossover study design. Participants were randomly assigned the placebo (P) or Quercetin (Q). The trial order for the 12 subjects was randomized so that six subjects with quercetin trial, while six subjects stared with the placebo. The crossover trial was repeated after completion of the first trial with the 14-day washout period, all subjects accept the implementation of the random allocation sequence occurring without the knowledge of which supplements were received by themselves using the identical appearance of the capsule with quercetin and placebo. The participants received placebo or quercetin for seven consecutive days with a 14-day washout period between the two trials, as shown in Figure 1, the participants’ V̇O2max was measured, and the power for the cycle ergometer was calculated individually. During the trial, subjects were instructed to keep their diet habits, control the energy intake, receive the meals provided by investigators, and avoid strenuous exercise until the day of the experiment. Caffeine intake, smoking, ergogenic supplements, anti-inflammatory drugs, and food items including polyphenol and vitamins were not allowed. All participants received the light breakfast diet and the identical lunch and supper (total energy per meal of lunch/supper is 700.60 kcal, carbohydrate, 77.90 g; fat, 26.60 g; protein, 37.10 g) during the 24 h before trial. To achieve the simulating glycogen depletion was performed in the first cycling exercise challenge, participants fasted for 12-h before the day of the experiment. At 7 am on the next morning, participants reported to the lab and their height and body weight were measured, followed by a cycling challenge at 70% of V̇O2max for 60 min while maintaining 60 rpm (17). Immediately after the first exercise challenge, participants consumed the light diet contained 60% carbohydrate with 1,000 mg quercetin/placebo (60% carbohydrate, 24.12 ± 0.20 g; 25% fat, 16.82 ± 0.21g; 15% protein, 16.12 ± 0.20 g; 304.12 ± 1.20 kcal). Approximately 3 h (post-exercise recovery) later, participants performed a cycling test at 75% of V̇O2max until exhaustion. Time-to-exhaustion was recorded. Blood and gas samples were collected before and after the first cycling bout, during post-exercise recovery, and after exhaustion.
Maximal Oxygen Uptake Test
Participants performed the V̇O2max test on a cycle ergometer (Monark, Varberg, Sweden) while each breath was assessed with gas analyzers (Cortex Biophysik, Non-nenstrasse, Leipzing, Germany). The participants maintained 60 RPM’s throughout the test. The primary workload was at 0.5 kg for 4 min and then increased by 0.5 kg every 2-min until exhaustion. The determination of V̇O2max was based on three criteria: (1) Respiratory exchange ratio (RER) > 1.10; (2) V̇O2max variance < 2 mL/kg/min; and (3) target heart rate reaches a theoretical maximum value at “220-age” (18). Accordingly, the value obtained from the Y-axis was oxygen consumption (ml/kg/min); the value obtained from the X-axis was the workload that corresponded to the oxygen uptake. V̇O2max (100%) was determined when the curve reached the plateau. The value multiplied by 0.70 was defined as 70% V̇O2max as the intensity for the cycling challenge; multiplied by 0.75 was defined as 75% V̇O2max as the intensity for the subsequent time-to-exhaustion test, and the corresponding value from X-axis was the workload used during the formal experiments (19).
Quercetin and Placebo Supplement
The participants received placebo or quercetin for seven consecutive days with a 14-day washout period between the two trials. During seven consecutive days, the participants ingested either two quercetin capsules or two placebo capsules after breakfast at 8 am in the lab. On the exercise challenge experimental morning, the participants ingested either two quercetin capsules or two placebo capsules immediately after the first exercise challenge. Each quercetin capsule (GNC Holdings Inc., United States) was 500 mg while each placebo capsule was 500 mg of cellulose. Thus, the participants received 1,000 mg quercetin or cellulose per day. This dosage of quercetin was within safe limits for human intake (20).
Blood Sample Collection and Analysis
Blood samples were collected before exercise, 1st exercise recovery period, and 2nd post-exercise 0, 1, and 24 h. All blood samples were tested for the exercise-induced status of oxygen stress, inflammation, and muscle damage. Blood samples were collected and centrifuged at 1,000 g for 10 min. Afterward, the supernatant was collected and placed in a freezer at –20°C. The supernatant was used to measure the following: insulin, NEFA, TAC, SOD, MDA, IL-6, TNF-α, CK, MB, and hs-CRP. Blood glucose concentration was determined by an automated glucose analyzer (YSI Life Sciences). Plasma insulin levels were determined using a commercial kit (Roche Diagnostics, Mannheim, Germany). Streptavidin microparticle interacted with anti-insulin AB-biotin and anti-insulin AB-Ru (bpy) 32+ to emit chemiluminescence (Roche Elecsys 1010/2010, Roche Diagnostics; MODULAR ANALYTICS E170, Roche Diagnostics) for analyzing the insulin levels and calculation of insulin sensitivity index (ISI) (21). Plasma NEFA was measured using a commercial kit (Wako, Neuss, Germany) in combination with an automated biochemical analyzer (Hitachi Science Systems, Ltd., Lbaranki, Japan). TAC, MDA, SOD, IL-6, and TNF-α can be used to determine the status of oxidative stress and inflammation. TAC, MDA (Sigma, St. Louis, MO, United States), and SOD (Cayman Chemical Company, ANN Arbor, MI, United States) were measured using commercial kits (Tecan GENios, A -5082, Austria). The levels of TAC, MDA, and SOD were determined by absorbance at 734, 532, and 570 nm wavelength using the standard curve. Cytokine IL-6 (Bio-Legend Inc., San Diego, CA, United States) and TNF-α (Bio-Legend Inc., San Diego, CA, United States) were measured using a commercially available reader (Tecan GENios, A-5082, Austria) to read the absorbance at 450 and 570 nm wavelengths to calculate concentrations in the serum samples. Additionally, CK, MB, and hs-CRP served as the biomarker for muscle damage. CK (Cayman Chemical Company, ANN Arbor, MI, United States), MB (Wuhan Fine Biotech Co., Ltd., Hubei, China), and hs-CRP (Denka Seiken Co., Ltd., Tokyo, Japan) were measured using commercial kits. The concentration was determined using the reader (Tecan GENios, A-5082, Austria) to obtain absorbance at 450 and 570 nm wavelengths as mentioned in the previous section.
Gas Sample Collection and Analysis
Gas samples were collected via the gas analyzer every 60 min for 3 hours (for example, 60, 120, and 180 min) to determine RER during the post-exercise recovery period after subjects were given a carbohydrate-rich diet. RER was calculated based on the amount of CO2 generated/VO2.
Statistics
Values were presented as mean ± standard error (mean ± SE). SPSS software was used for statistical analysis. Paired t-test was used to analyze time-to-exhaustion. Repeated measure two-way ANOVA (trial × time) was used to determine the intra- and inter- difference at different time points and between the two groups with power set at 80%. If significant interaction was observed within and between groups, a simple main effects analysis was conducted. Fisher’s least significant difference (LSD) was used for post hoc analysis. The α value was set at p < 0.05.
Results
Quercetin Enhanced the Subsequence High-Intensity Cycling Performance to Exhaustion After 3-h Post-exercise Recovery of the First Exercise Challenge
We found that seven days of quercetin significantly enhanced the second cycling exercise performance to exhaustion (Quercetin: 37.29 ± 5.20 min; Placebo: 30.72 ± 2.16 min, p < 0.05) (Figure 2). Significantly lower insulin responses were found in quercetin than those of placebo at 60, 120, and 180-min after the first cycling exercise challenge (Figure 3B, p < 0.05). However, there was no significant difference in glucose and NEFA (Figures 3A,C) during the experimental procedures. No significant difference in whole-body carbohydrate and fat oxidation were found between the two trials calculated by gaseous samples during the first cycling exercise recovery period (Figure 4, p > 0.05).
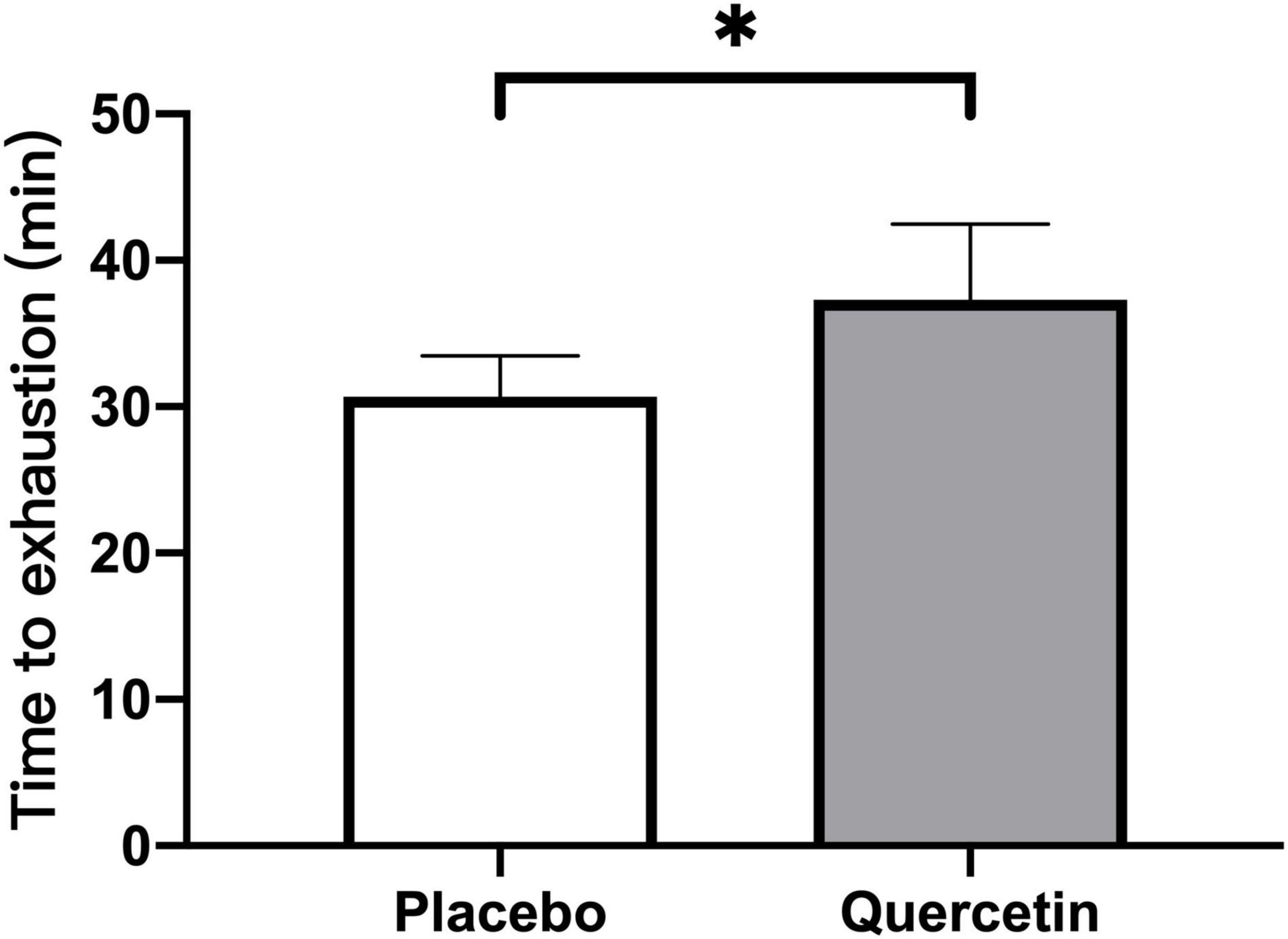
Figure 2. Mean values data of all participants for the time-to-exhaustion tests of the second cycling exercise with 75% VO2max. *Significant difference between quercetin and placebo (p < 0.05). Values are expressed as mean ± SE, N = 12.
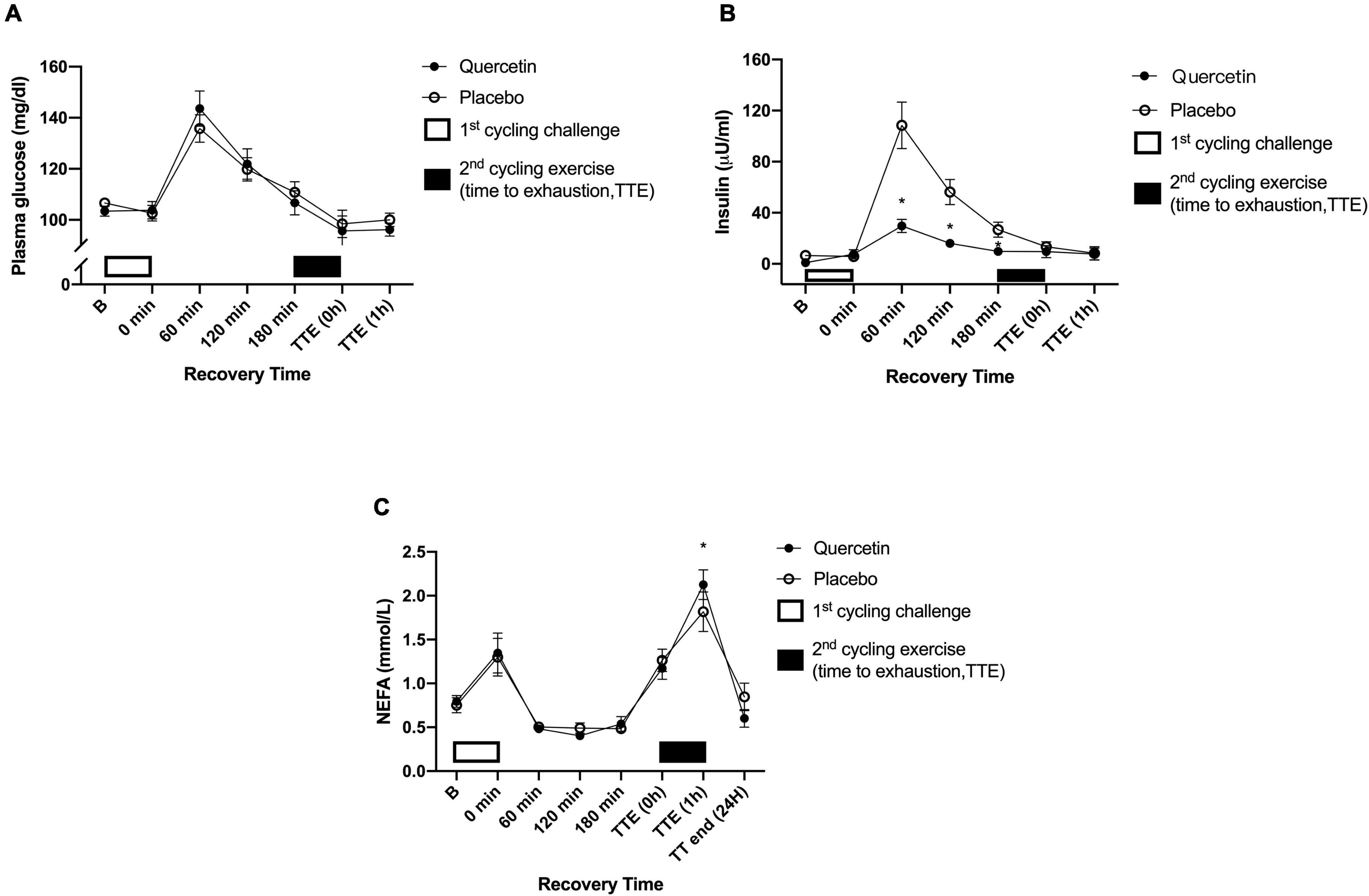
Figure 3. Blood glucose (A), plasm insulin (B), and plasma non-esterified fatty acids (NEFA) (C) concentrations in (-•-) quercetin and (-◦-) placebo trials. B represents before the first cycling challenge. TTE (0,1 h,24 h): represents immediately, 1-h, and 24-h after the second cycling exercise, respectively. *Significant difference between quercetin and placebo (p < 0.05). Values are expressed as mean ± SE, N = 12.
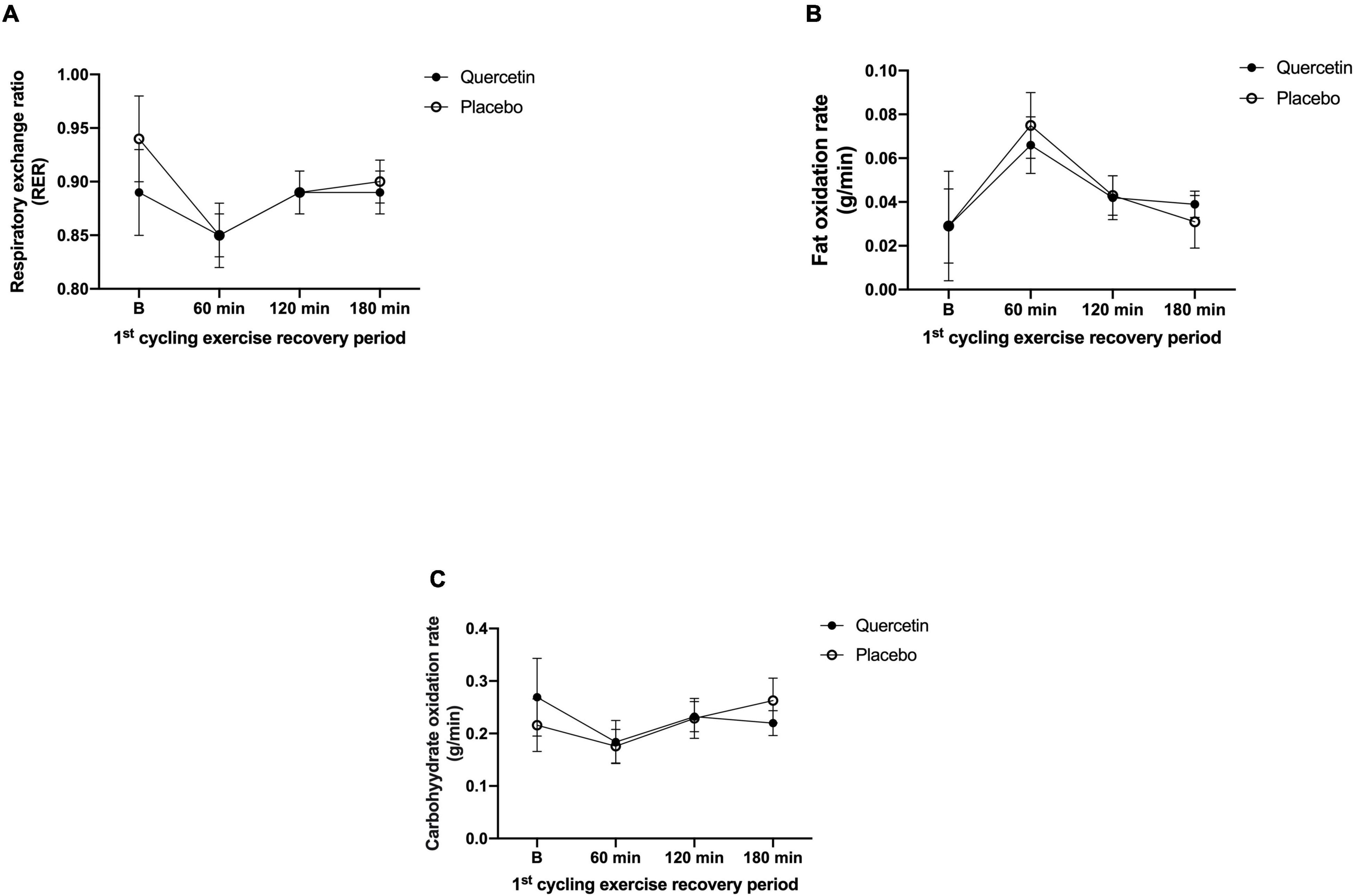
Figure 4. Respiratory exchange rate (A), fat oxidation rate (B), and carbohydrate oxidation rate (C) in (-•-) and quercetin trials (-◦-) placebo. B represents before the first cycling challenge; *Significant difference between quercetin and placebo (p < 0.05). Values are expressed as mean ± SE, N = 12.
Quercetin Enhanced Antioxidant Capacity and Resulted in Decreased Oxidative Stress After the Cycling Exercise Challenge
Total antioxidant capacity activity was enhanced by quercetin, evidenced by the response after the cycling exercise challenge (Figure 5A, p < 0.05). Similarly, the SOD concentrations were significantly higher in the quercetin than those of placebo (Figure 5B, p < 0.05). In addition, we measured oxidative stress MDA markers, which were significantly lower at 60, and 180-min during the first exercise recovery period and at the end of the second cycling exercise performance to exhaustion (Figure 5C, p < 0.05).
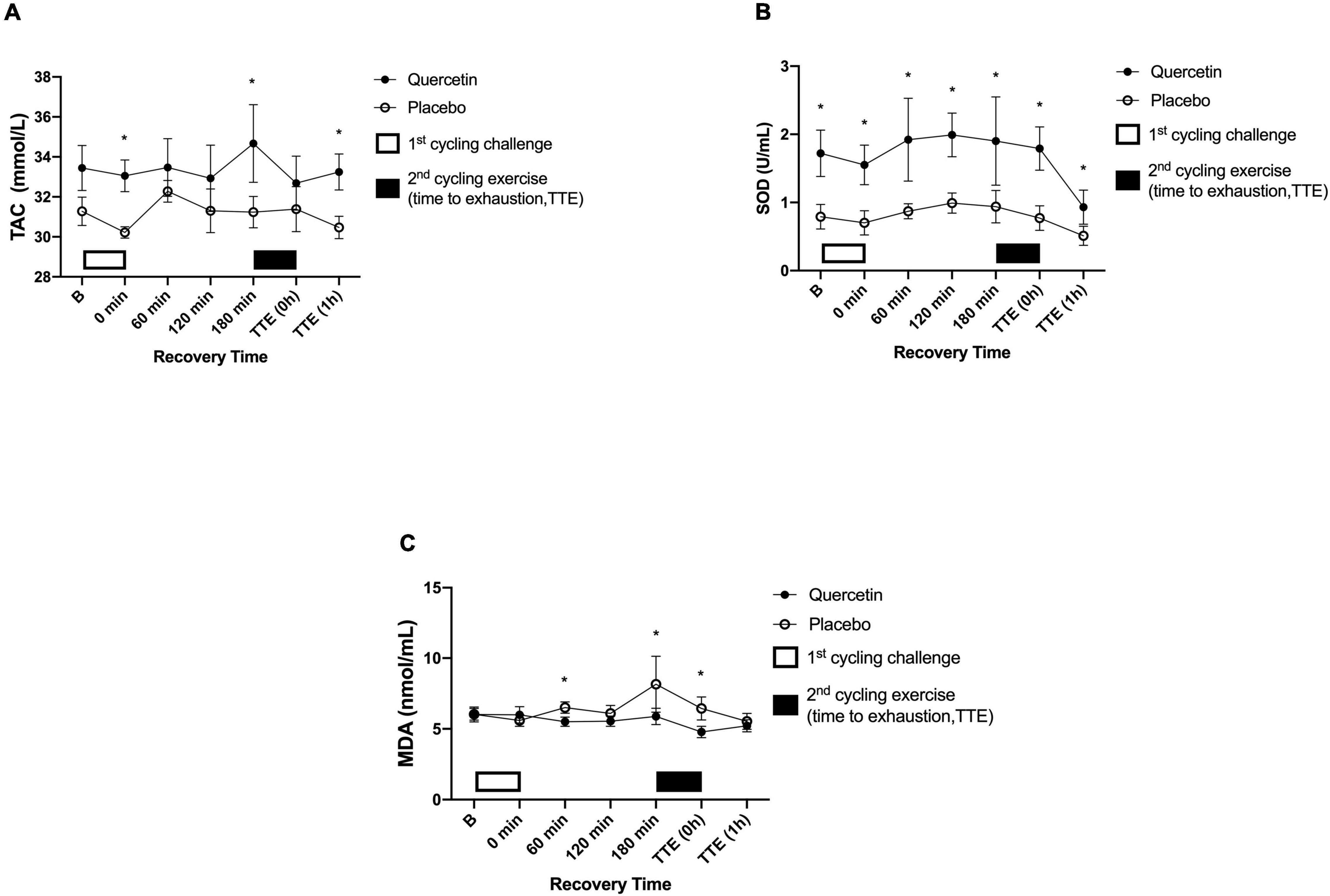
Figure 5. Serum total antioxidant capacity (TAC) (A), superoxidase dismutase (SOD) (B), and malondialdehyde (MDA) (C) concentrations in (-◦-) placebo and (-•-) quercetin trials. B represents before the first cycling challenge. TTE (0h,1h): represents immediately, and 1-h after the second cycling exercise, respectively. *Significant difference between quercetin and placebo (p < 0.05). Values are expressed as mean ± SE, N = 12.
Quercetin Attenuated IL-6 and CK Levels Induced by the High-Intensity Cycling Exercise to Exhaustion After 3 h Post-eercise Recovery of 75% VO2max Exercise Challenge
The effect of quercetin on circulating response regarding inflammation and muscle damage indicators was measured before and after two bouts of cycling exercise challenge. Figures 6A, 7A showed the significant response of pro-inflammatory markers IL-6 and muscle damage indicators CK after Quercetin supplementation (p < 0.05). In this human study, IL-6 concentrations were significantly lower immediately, and 1-h after the second cycling exercise (Figure 6A, p < 0.05). The peak serum CK responded at 24-h after the second cycling challenge (Figure 7A). Identically, the concentrations of the serum CK were significantly lower at 24-h in the quercetin trial compared to the placebo trial (Figure 7A, p < 0.05). However, there were no differences in TNF-α (Figure 6B), MB (Figure 7B), and hs-CRP (Figure 7C) between the two trials. Therefore, we speculate that oral quercetin supplementation tends to attenuate IL-6 and CK concentrations after high-intensity cycling exhaustion challenges but no other inflammation and muscle damage indicators.
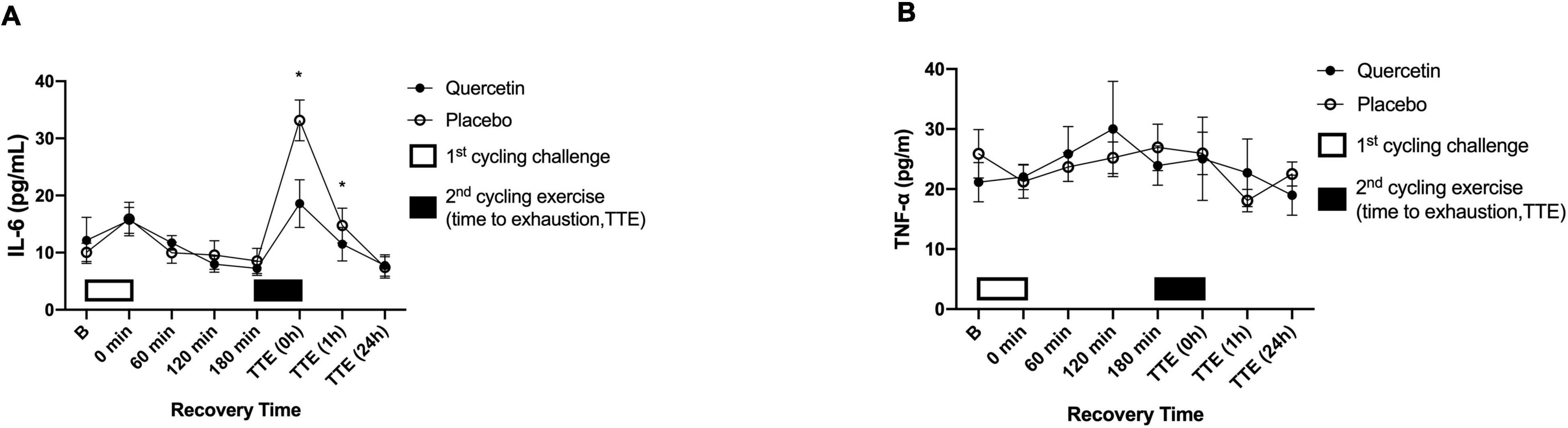
Figure 6. Serum interleukin-6 (IL-6) (A) and tumor necrosis factor-α (TNF-α) (B) concentrations in (-◦-) placebo and (-•-) quercetin trials. B represents before the first cycling challenge. TTE (0 h, 1 h, 24 h): represents immediately, 1-h, and 24-h after the second cycling exercise, respectively. *Significant difference between quercetin and placebo (p < 0.05). Values are expressed as mean ± SE, N = 12.
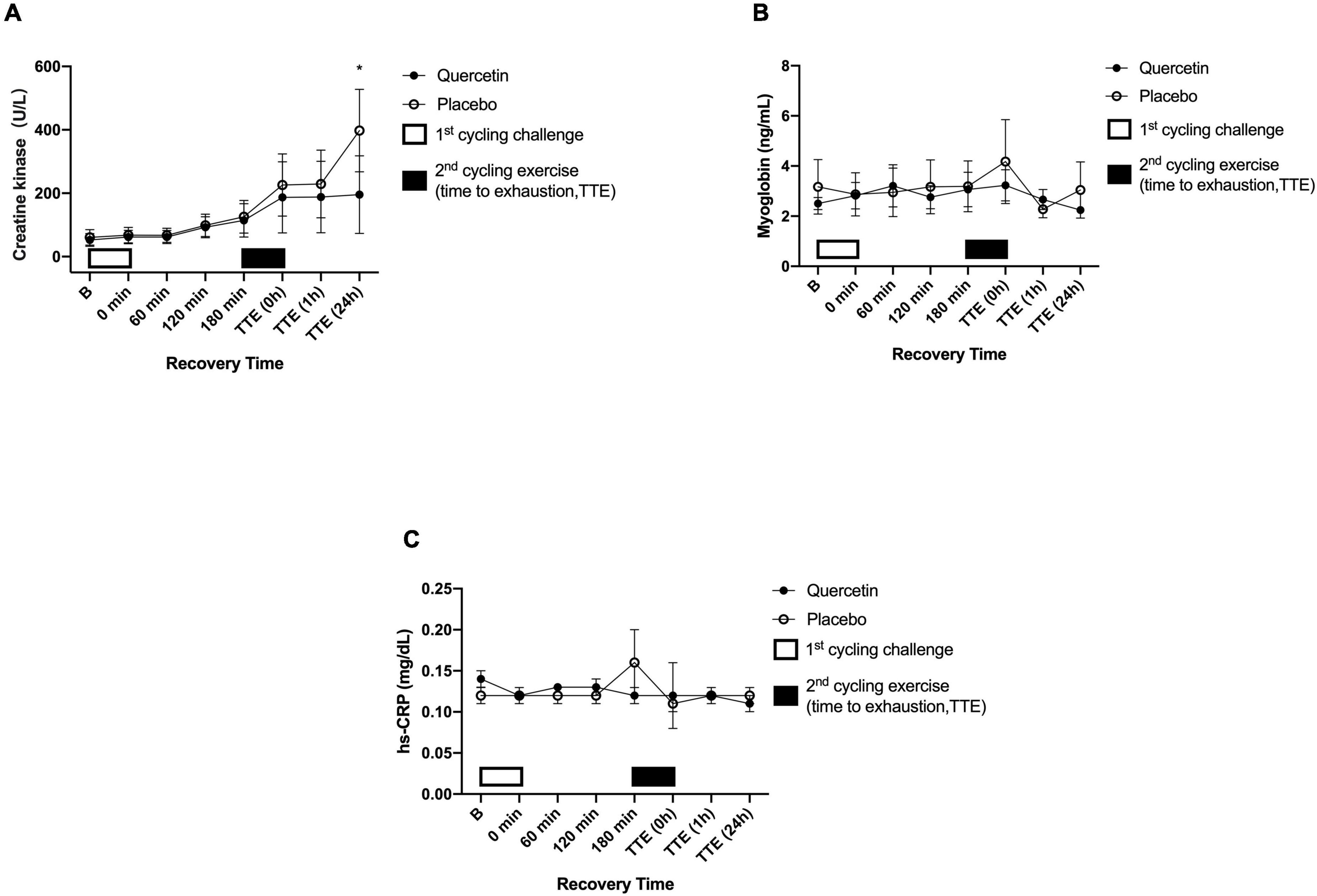
Figure 7. Serum creatine kinase (CK) (A), myoglobin (B), and hs-CRP (C) concentrations in (-◦-) placebo and (-•-) quercetin trials. B represents before the first cycling challenge. TTE (0 h, 1 h, 24 h): represents immediately, 1-h, and 24-h after the second cycling exercise, respectively. *Significant difference between quercetin and placebo (p < 0.05). Values are expressed as mean ± SE, N = 12.
Discussion
Quercetin is a polyphenolic flavonoid with antioxidant and anti-inflammatory properties (22–25). In the present human study, we hypothesized that 7-days of oral quercetin supplementation can boost antioxidant and anti-inflammatory responses as well as mitigate oxidative stress and inflammation caused by high-intensity cycling for 60 min. Furthermore, we hypothesized that supplementation would increase time to exhaustion during the second high-intensity cycling challenge. The major finding in the present study was significantly increased time-to-exhaustion during the second cycling challenge after a short-term (3 h) break following the first cycling bout (Figure 2). The compatible papers showing the ergogenic effect of quercetin are reported (7, 22, 23, 26). Nevertheless, some comparisons were made where the quercetin supplement in four published papers indicating the ergogenic properties of quercetin on exercise performance was contrary to the present study (9–12). The short-term model of quercetin supplementation in the present study is not the same as the acute condition as Cheuvront’s study and the blood sampling about oxidant stress, inflammation, and muscle damage indicators in the present study are different from the non-invasive measurement of muscle oxidative capacity, physical fitness in Cureton or Bigelman studies. Nonetheless, we conservatively inferred that 1,000 mg/day of quercetin supplementation for a week improved a time trial performance following a moderate aerobic exercise, evidenced on post-exercise blood samples regarding increased systemic insulin-stimulated glucose uptake, antioxidant capacity, and decreased IL-6, CK levels.
As shown in Figure 3B, insulin levels by quercetin were significantly lower than those of placebo during the first post-exercise recovery period. The calculation of area under the curve for glucose/insulin and insulin sensitivity index (ISI) are shown (IACU: Quercetin:3248.88 ± 1643.56 min × μU/ml; Placebo:13085.78 ± 6178.03 min × μU/ml, p < 0.05; GACU: Quercetin:671.50 ± 13.63 min × mg/ml; Placebo: 670.70 ± 17.51 min × mg/dl, p > 0.05; ISI: Quercetin: 24.85 ± 14.7; Placebo: 4.95 ± 1.32, p < 0.05). Presumably, these data led to increased muscle glycogen levels and endurance performance during subsequent exercises (27). In previous studies, Cheng et al. performed a single-blind, randomized, crossover study that involved post-exercise supplementation of hydroxycitric acid or placebo for a group of male subjects. The supplement significantly accelerated the rate of glycogen synthesis twofold in exercised human skeletal muscle compared to placebo; this increase occurred in parallel with increased whole-body insulin-stimulated glucose uptake, evidenced by reduced post-meal insulin response to the same carbohydrate meal challenge (28). Therefore, the synthesis of glycogen from glucose was promoted which is especially meaningful to energy replenishment during post-exercise recovery (13, 29). Guo et al. study showed that quercetin can positively regulate insulin receptor substrate 1 (IRS-1) serine/tyrosine phosphorylation and regulate insulin signaling through the downstream protein kinase B (Akt)/endothelial nitric oxide synthase (eNOS)-phosphoinositide 3-kinase (PI3K) pathway (30). Unfortunately, the present study did not perform the muscle biopsy to assess muscle glycogen results, and systemic fat oxidation utilization was not significantly enhanced by quercetin during post-exercise recovery (Figure 4). Therefore, we suggest that the higher insulin-stimulated glucose uptake found in this study enhanced replenishment function which would be conducive to the rapid restoration of muscle glycogen. This would then have a synergistic effect to improve time-to-exhaustion during exercise.
Following 7-days of quercetin supplementation antioxidant enzymes, TAC and SOD were changed significantly in the present study. Quercetin has been shown the most potent scavenger of ROS (31). The anti-oxidative capacities of quercetin are due to the presence of two antioxidant pharmacophores within the molecule that have the optimal configuration for free radical scavenging, i.e., the catechol group in the B ring and the OH group at position 3 of the AC ring (32). In the present study, the SOD activity and TAC by quercetin were significantly higher than those of placebo during the experimental period in parallel with significantly decreased levels of MDA, a plasma lipid peroxidation product caused by exercise (Figures 5A–C). TAC and SOD are considered the first line of defense against ROS, catalyzing the decomposition of superoxide anions into hydrogen peroxide (33). These findings appear to be in line with the supplement itself. Quercetin, a natural polyphenolic flavonoid, has been shown to provide a combination of antioxidant and anti-inflammatory properties in both animal and human studies (5, 6). The antioxidant capacity of quercetin has been attributed to its chemical structure, especially the presence and position of the catechol group in the B ring and the hydroxyl (–OH) group, which regulate the redox mechanism and enhance the ability of free radical scavenging (34). It has been reported that high oxidative stress in the human body significantly postpones or reduces the mitochondrial synthesis in skeletal muscle (35) and affects the rate of cellular adenosine triphosphate (ATP) synthesis (36). Previous human studies showed that mitochondrial synthesis and ATP production play important physiological roles to improve human exercise performance (7). Additionally, Paschalis et al. reported that antioxidant supplements significantly decrease oxidative stress as measured by F2-isoprostanes and protein carbonyls, which effectively improved the aerobic performance (V̇O2max test), anaerobic power test (Wingate), and 5-min time trial test (37). Almeida et al. reported that quercetins absorption was variated by their gut microbiota in the small intestine and low bioavailability in humans (38). Diksha et al., study was to investigate the absorption of quercetin in 18 healthy human subjects administered. The Cmax of quercetin was highest achieved within 3.3 h (39). The present study was designed to perform multiple exercises over a long period of time, consisting of an initial 60-min 70% V̇O2max test followed by a 3 h post-exercise recovery period, and finishing with high-intensity cycling exercise to exhaustion. Similar to Paschalis’ human study, the increased antioxidant enzymatic activity, coinciding with reduced oxidative stress, supports quercetin supplementation for improving high-intensity exercise performance.
Physiological inflammation levels and increased muscle damage are related to both the muscles exercised and for how long the muscles were actively engaged. For instance, whole-body muscle movement has been reported to significantly increase the concentration of IL-6, TNF-α, CK, MB, and hs-CRP (40–42). A previous study from our lab indicated that IL-6 levels significantly increased in response to a cycling challenge at 80% V̇O2max (43). The same exercise was applied in the present and significantly increased levels of IL-6 after exhaustion for both the quercetin and placebo groups (Figure 6A), indicating that treatment with exercise at 75% V̇O2max could induce pro-inflammatory marker IL-6 levels in the human body. Quercetin has been shown the property of anti-inflammation via the inhibition of activation of the transcript factors such as nuclear factor-κB (NF-κB) and activator protein (AP-1) (44, 45). Consequently, the property of quercetin on scavenging ROS would not only prevent the occurrence of oxidative stress but also help mitigate inflammation. Indeed, it has already been shown that quercetin can inhibit pro-inflammation via modulation of NF-κB in human peripheral blood mononuclear cells (46). The present study monitored physiological reactions throughout the trial in participants treated with quercetin (1,000 mg/day) for 7 days. The IL-6 levels caused by exercises were significantly reduced by 7-day quercetin supplementation (Figure 6A, p < 0.05). Together, we were able to infer that 7-day quercetin supplementation was capable of inhibiting the muscle pro-inflammatory cytokine IL-6 concentration induced by high-intensity exercise to exhaustion, suggesting that quercetin supplementation can assert positive physiological effects. In addition, blood CK and MB are sensitive to muscle damage and serve as biomarkers for such damage. Creatine kinase participates in the reversible reaction that catalyzes the phosphorylation of creatine, ultimately forming new ATP, which occurs primarily in skeletal muscle (47), the effect of quercetin on CK response involving AMP activated protein kinase (AMPK) itself a regulation in the heart muscle of rats (48, 49). In the present study, we speculated the conservation of the adenine nucleotide pools in the skeletal muscle may be a supplementary advantage of quercetin regarding ergogenic strategy for athletes. The present study investigated the positive effects of quercetin supplementation for 7 days on muscle damage biomarkers and showed that CK was significantly lower than placebo at 24-hr after the exercise-to-exhaustion challenge (Figure 7A, p < 0.05), not in MB, and hs-CRP (Figures 7B,C, p > 0.05). The authors wondered that unsuitable blood sample timing to correctly measure MB, and hs-CRP was likely the reason for our inability to detect changes. Cunniffe et al. showed that serum cortisol and IL-6 levels increased immediately after an International Rugby League game. While CK and the CRP index reached their peaks 14 and 38 hours after exercise respectively, perhaps revealing the true pattern of these physiological biomarkers in the acute phase post-exercise (50), suggesting that the time at which blood samples are obtained is crucial. Nevertheless, the last time-point of blood sampling in the present human study was 24 h after exhaustion, which may not be appropriate to observe the course of change among inflammation and muscle damage markers such as MB, and hs-CRP.
In conclusion, this study examined the effects of quercetin (1,000 mg/day for 7 days) supplementation on insulin sensitivity, antioxidant capacity, muscle damage, and exercise performance in human subjects. Quercetin supplementation was found to improve whole-body insulin-stimulated glucose uptake and antioxidant capacity, which attenuated exercise-induced oxygen stress during two consecutive high-intensity cycling bouts. Following a 3-h recovery period from the first high-intensity exercise session, quercetin improved time to exhaustion for the subsequent cycling test. Actually, there were limitations in the present study, including without performing a muscle biopsy, due to the COVID-19 pandemic, the muscle biopsy could not be performed on the exercised subjects to obtain muscle glycogen data, therefore, we speculated the effect of muscle glycogen use on cycling time to exhaustion only based on insulin data without to outline the possible underlying mechanisms for a relationship between muscle glycogen and cycling time to exhaustion. Of course, we also were lack of records about physical activity and dietary diaries during the experimental periods for all participants. This useful information could elucidate the exclusive benefit effect of quercetin in the ergogenic property on exercise competition. However, the novel findings and new knowledge the present study provided was quercetin supplementation enhanced the post-exercise whole-body insulin-stimulated glucose uptake and antioxidant capacity, in parallel with a decrease in exercise-induced MDA, IL-6, and CK levels throughout the post-exercise period. The beneficial effects of quercetin are likely due to its glucogenic, antioxidant, and anti-inflammatory properties. Thus, short-term quercetin supplementation may be considered an effective ergogenic aid for enhancing high-intensity cycling performance among young adults.
Data Availability Statement
The raw data supporting the conclusions of this article will be made available by the authors, without undue reservation.
Ethics Statement
The studies involving human participants were reviewed and approved by Institutional Review Board at the University of Taipei, Taipei, Taiwan (UT-IRB-2018-085). The patients/participants provided their written informed consent to participate in this study.
Author Contributions
I-SC designed the experiments. J-PT, S-FL, and H-CH carried out the laboratory experiments. J-PT and C-LH contributed reagents, materials, and analysis platforms. S-FL, C-LH, and I-SC analyzed the data. JB helped with the polishing of English writing. J-PT and I-SC interpreted the results, prepared the figures, and wrote and revised the manuscript. All authors are qualified and approved this final submitted version of this study.
Funding
This work was supported by grants from the Ministry of Science and Technology (MOST 108-2410-H-142-012-) of Taiwan.
Conflict of Interest
The authors declare that the research was conducted in the absence of any commercial or financial relationships that could be construed as a potential conflict of interest.
The handling editor MK declared a past collaboration with the authors S-FL, I-SC, and J-PT at the time of review.
Publisher’s Note
All claims expressed in this article are solely those of the authors and do not necessarily represent those of their affiliated organizations, or those of the publisher, the editors and the reviewers. Any product that may be evaluated in this article, or claim that may be made by its manufacturer, is not guaranteed or endorsed by the publisher.
Acknowledgments
We sincerely appreciate all subjects and researchers for contributing to this study.
References
1. Huang WC, Wei CC, Huang CC, Chen WL, Huang HY. The beneficial effects of Lactobacillus plantarum Ps128 on high-intensity, exercise-induced oxidative stress, inflammation, and performance in triathletes. Nutrients. (2019) 11:353. doi: 10.3390/nu11020353
2. Uchiyama S, Tsukamoto H, Yoshimura S, Tamaki T. Relationship between oxidative stress in muscle tissue and weight-lifting-induced muscle damage. Pflugers Arch. (2006) 452:109–16. doi: 10.1007/s00424-005-0012-y
3. Peake JM, Suzuki K, Hordern M, Wilson G, Nosaka K, Coombes JS. Plasma cytokine changes in relation to exercise intensity and muscle damage. Eur J Appl Physiol. (2005) 95:514–21. doi: 10.1007/s00421-005-0035-2
4. Loke WM, Proudfoot JM, McKinley AJ, Needs PW, Kroon PA, Hodgson JM, et al. Quercetin and its in vivo metabolites inhibit neutrophil-mediated low-density lipoprotein oxidation. J Agric Food Chem. (2008) 56:3609–15. doi: 10.1021/jf8003042
5. Boots AW, Drent M, de Boer VC, Bast A, Haenen GR. Quercetin reduces markers of oxidative stress and inflammation in Sarcoidosis. Clin Nutr. (2011) 30:506–12. doi: 10.1016/j.clnu.2011.01.010
6. Yang H, Yang T, Heng C, Zhou Y, Jiang Z, Qian X, et al. Quercetin improves nonalcoholic fatty liver by ameliorating inflammation, oxidative stress, and lipid metabolism in Db/Db mice. Phytother Res. (2019) 33:3140–52. doi: 10.1002/ptr.6486
7. Nieman DC, Williams AS, Shanely RA, Jin F, McAnulty SR, Triplett NT, et al. Quercetin’s influence on exercise performance and muscle mitochondrial biogenesis. Med Sci Sports Exerc. (2010) 42:338–45. doi: 10.1249/MSS.0b013e3181b18fa3
8. McAnulty SR, McAnulty LS, Nieman DC, Quindry JC, Hosick PA, Hudson MH, et al. Chronic quercetin ingestion and exercise-induced oxidative damage and inflammation. Appl Physiol Nutr Metab. (2008) 33:254–62. doi: 10.1139/h07-177
9. Cheuvront SN, Ely BR, Kenefick RW, Michniak-Kohn BB, Rood JC, Sawka MN. No effect of nutritional adenosine receptor antagonists on exercise performance in the heat. Am J Physiol Regul Integr Comp Physiol. (2009) 296:R394–401. doi: 10.1152/ajpregu.90812.2008
10. Cureton KJ, Tomporowski PD, Singhal A, Pasley JD, Bigelman KA, Lambourne K, et al. Dietary quercetin supplementation is not ergogenic in untrained men. J Appl Physiol. (2009) 107:1095–104. doi: 10.1152/japplphysiol.00234.2009
11. Bigelman KA, Fan EH, Chapman DP, Freese EC, Trilk JL, Cureton KJ. Effects of six weeks of quercetin supplementation on physical performance in Rotc cadets. Mil Med. (2010) 175:791–8. doi: 10.7205/milmed-d-09-00088
12. Pelletier DM, Lacerte G, Goulet ED. Effects of quercetin supplementation on endurance performance and maximal oxygen consumption: a meta-analysis. Int J Sport Nutr Exerc Metab. (2013) 23:73–82. doi: 10.1123/ijsnem.23.1.73
13. Betts J, Williams C, Duffy K, Gunner F. The influence of carbohydrate and protein ingestion during recovery from prolonged exercise on subsequent endurance performance. J Sports Sci. (2007) 25:1449–60. doi: 10.1080/02640410701213459
14. Hou CW, Lee SD, Kao CL, Cheng IS, Lin YN, Chuang SJ, et al. Improved inflammatory balance of human skeletal muscle during exercise after supplementations of the ginseng-based steroid Rg1. PLoS One. (2015) 10:e0116387. doi: 10.1371/journal.pone.0116387
15. Gurton WH, Gough LA, Sparks SA, Faghy MA, Reed KE. Sodium bicarbonate ingestion improves time-to-exhaustion cycling performance and alters estimated energy system contribution: a dose-response investigation. Front Nutr. (2020) 7:154. doi: 10.3389/fnut.2020.00154
16. Serdar CC, Cihan M, Yücel D, Serdar MA. Sample size, power and effect size revisited: simplified and practical approaches in pre-clinical, clinical and laboratory studies. Biochem Med (Zagreb). (2021) 31:010502. doi: 10.11613/bm.2021.010502
17. Huang CC, Liu CC, Tsao JP, Hsu CL, Cheng IS. Effects of oral resveratrol supplementation on glycogen replenishment and mitochondria biogenesis in exercised human skeletal muscle. Nutrients. (2020) 12:3721. doi: 10.3390/nu12123721
18. Zuniga JM, Housh TJ, Camic CL, Bergstrom HC, Traylor DA, Schmidt RJ, et al. Metabolic parameters for ramp versus step incremental cycle ergometer tests. Appl Physiol Nutr Metab. (2012) 37:1110–7. doi: 10.1139/h2012-098
19. Kuipers H, Verstappen FT, Keizer HA, Geurten P, van Kranenburg G. Variability of aerobic performance in the laboratory and its physiologic correlates. Int J Sports Med. (1985) 6:197–201. doi: 10.1055/s-2008-1025839
20. Wang L, Morris ME. Liquid chromatography-tandem mass spectroscopy assay for quercetin and conjugated quercetin metabolites in human plasma and urine. J Chromatogr B Anal Technol Biomed Life Sci. (2005) 821:194–201. doi: 10.1016/j.jchromb.2005.05.009
21. Matsuda M, DeFronzo RA. Insulin sensitivity indices obtained from oral glucose tolerance testing: comparison with the euglycemic insulin clamp. Diabetes Care. (1999) 22:1462–70. doi: 10.2337/diacare.22.9.1462
22. Davis JM, Murphy EA, Carmichael MD. Effects of the dietary flavonoid quercetin upon performance and health. Curr Sports Med Rep. (2009) 8:206–13. doi: 10.1249/JSR.0b013e3181ae8959
23. Davis JM, Murphy EA, Carmichael MD, Davis B. Quercetin increases brain and muscle mitochondrial biogenesis and exercise tolerance. Am J Physiol Regul Integr Comp Physiol. (2009) 296:R1071–7. doi: 10.1152/ajpregu.90925.2008
24. Li Y, Yao J, Han C, Yang J, Chaudhry MT, Wang S, et al. Quercetin, inflammation and immunity. Nutrients. (2016) 8:167. doi: 10.3390/nu8030167
25. Shi G-J, Li Y, Cao Q-H, Wu H-X, Tang X-Y, Gao X-H, et al. In vitro and in vivo evidence that quercetin protects against diabetes and its complications: a systematic review of the literature. Biomed Pharmacother. (2019) 109:1085–99. doi: 10.1016/j.biopha.2018.10.130
26. Quindry JC, McAnulty SR, Hudson MB, Hosick P, Dumke C, McAnulty LS, et al. Oral quercetin supplementation and blood oxidative capacity in response to ultramarathon competition. Int J Sport Nutr Exerc Metab. (2008) 18:601–16. doi: 10.1123/ijsnem.18.6.601
27. Widrick JJ, Costill DL, Fink WJ, Hickey MS, McConell GK, Tanaka H. Carbohydrate feedings and exercise performance: effect of initial muscle glycogen concentration. J Appl Physiol. (1993) 74:2998–3005. doi: 10.1152/jappl.1993.74.6.2998
28. Cheng IS, Huang SW, Lu HC, Wu CL, Chu YC, Lee SD, et al. Oral hydroxycitrate supplementation enhances glycogen synthesis in exercised human skeletal muscle. Br J Nutr. (2012) 107:1048–55. doi: 10.1017/s0007114511003862
29. Lawrence JC Jr, Roach PJ. New insights into the role and mechanism of glycogen synthase activation by insulin. Diabetes. (1997) 46:541–7. doi: 10.2337/diab.46.4.541
30. Guo XD, Zhang DY, Gao XJ, Parry J, Liu K, Liu BL, et al. Quercetin and quercetin-3-O-glucuronide are equally effective in ameliorating endothelial insulin resistance through inhibition of reactive oxygen species-associated inflammation. Mol Nutr Food Res. (2013) 57:1037–45. doi: 10.1002/mnfr.201200569
31. Cushnie TP, Lamb AJ. Antimicrobial activity of flavonoids. Int J Antimicrob Agents. (2005) 26:343–56. doi: 10.1016/j.ijantimicag.2005.09.002
32. Heijnen CG, Haenen GR, Oostveen RM, Stalpers EM, Bast A. Protection of flavonoids against lipid peroxidation: the structure activity relationship revisited. Free Radic Res. (2002) 36:575–81. doi: 10.1080/10715760290025951
33. Wang Y, Branicky R, Noë A, Hekimi S. Superoxide dismutases: dual roles in controlling ROS damage and regulating ROS signaling. J Cell Biol. (2018) 217:1915–28. doi: 10.1083/jcb.201708007
34. Santos MR, Mira L. Protection by flavonoids against the peroxynitrite-mediated oxidation of dihydrorhodamine. Free Radic Res. (2004) 38:1011–8. doi: 10.1080/10715760400003384
35. Di Meo S, Venditti P. Mitochondria in exercise-induced oxidative stress. Biol Signals Recept. (2001) 10:125–40. doi: 10.1159/000046880
36. Reichmann D, Voth W, Jakob U. Maintaining a Healthy proteome during oxidative stress. Mol Cell. (2018) 69:203–13. doi: 10.1016/j.molcel.2017.12.021
37. Paschalis V, Theodorou AA, Margaritelis NV, Kyparos A, Nikolaidis MGN-. Acetylcysteine supplementation increases exercise performance and reduces oxidative stress only in individuals with low levels of glutathione. Free Radic Biol Med. (2018) 115:288–97. doi: 10.1016/j.freeradbiomed.2017.12.007
38. Almeida AF, Borge GIA, Piskula M, Tudose A, Tudoreanu L, Valentová K, et al. Bioavailability of quercetin in humans with a focus on interindividual variation. Compr Rev Food Sci Food Saf. (2018) 17:714–31. doi: 10.1111/1541-4337.12342
39. Kaushik D, O’Fallon K, Clarkson PM, Dunne CP, Conca KR, Michniak-Kohn B. Comparison of quercetin pharmacokinetics following oral supplementation in humans. J Food Sci. (2012) 77:H231–8. doi: 10.1111/j.1750-3841.2012.02934.x
40. Fallon KE. The acute phase response and exercise: the ultramarathon as prototype exercise. Clin J Sport Med. (2001) 11:38–43. doi: 10.1097/00042752-200101000-00007
41. Fischer CP. Interleukin-6 in acute exercise and training: what is the biological relevance? Exerc Immunol Rev. (2006) 12:6–33.
42. Hody S, Rogister B, Leprince P, Wang F, Croisier JL. Muscle fatigue experienced during maximal eccentric exercise is predictive of the plasma creatine kinase (Ck) response. Scand J Med Sci Sports. (2013) 23:501–7. doi: 10.1111/j.1600-0838.2011.01413.x
43. Tsao JP, Liu CC, Wang HF, Bernard JR, Huang CC, Cheng IS. Oral resveratrol supplementation attenuates exercise-induced interleukin-6 but not oxidative stress after a high intensity cycling challenge in adults. Int J Med Sci. (2021) 18:2137–45. doi: 10.7150/ijms.55633
44. MacNee W. Oxidative stress and lung inflammation in airways disease. Eur J Pharmacol. (2001) 429:195–207. doi: 10.1016/s0014-2999(01)01320-6
45. Rahman I, Gilmour PS, Jimenez LA, MacNee W. Oxidative stress and Tnf-alpha induce histone acetylation and Nf-Kappab/Ap-1 activation in alveolar epithelial cells: potential mechanism in gene transcription in lung inflammation. Mol Cell Biochem. (2002) 234-235:239–48. doi: 10.1007/978-1-4615-1087-1_28
46. Nair MP, Mahajan S, Reynolds JL, Aalinkeel R, Nair H, Schwartz SA, et al. The flavonoid quercetin inhibits proinflammatory cytokine (tumor necrosis factor alpha) gene expression in normal peripheral blood mononuclear cells via modulation of the Nf-kappa beta system. Clin Vaccine Immunol. (2006) 13:319–28. doi: 10.1128/cvi.13.3.319-328.2006
47. McLeish MJ, Kenyon GL. Relating structure to mechanism in creatine kinase. Crit Rev Biochem Mol Biol. (2005) 40:1–20. doi: 10.1080/10409230590918577
48. Ponticos M, Lu QL, Morgan JE, Hardie DG, Partridge TA, Carling D. Dual regulation of the Amp-activated protein kinase provides a novel mechanism for the control of creatine kinase in skeletal muscle. EMBO J. (1998) 17:1688–99. doi: 10.1093/emboj/17.6.1688
49. Zakaria N, Khalil SR, Awad A, Khairy GM. Quercetin reverses altered energy metabolism in the heart of rats receiving adriamycin chemotherapy. Cardiovasc Toxicol. (2018) 18:109–19. doi: 10.1007/s12012-017-9420-4
Keywords: exercise, time to exhaustion, ergogenic aids, quercetin, insulin-stimulated glucose uptake
Citation: Tsao J-P, Bernard JR, Hsu H-C, Hsu C-L, Liao S-F and Cheng I-S (2022) Short-Term Oral Quercetin Supplementation Improves Post-exercise Insulin Sensitivity, Antioxidant Capacity and Enhances Subsequent Cycling Time to Exhaustion in Healthy Adults: A Pilot Study. Front. Nutr. 9:875319. doi: 10.3389/fnut.2022.875319
Received: 14 February 2022; Accepted: 06 April 2022;
Published: 28 April 2022.
Edited by:
Mallikarjuna Korivi, Zhejiang Normal University, ChinaReviewed by:
Mousa Khalafi, University of Kashan, IranPeter Stehle, University of Bonn, Germany
Zhi Xia, Chengdu Sport University, China
Copyright © 2022 Tsao, Bernard, Hsu, Hsu, Liao and Cheng. This is an open-access article distributed under the terms of the Creative Commons Attribution License (CC BY). The use, distribution or reproduction in other forums is permitted, provided the original author(s) and the copyright owner(s) are credited and that the original publication in this journal is cited, in accordance with accepted academic practice. No use, distribution or reproduction is permitted which does not comply with these terms.
*Correspondence: Chin-Lin Hsu, Y2xoc3VAY3NtdS5lZHUudHc=; Su-Fen Liao, c3VlbGlhbzNAZ21haWwuY29t; I-Shiung Cheng, aXNjaGVuZzE5NjVAbWFpbC5udGN1LmVkdS50dw==