- 1College of Pharmacy, Jining Medical University, Jining, China
- 2Tea Research Institute, Chinese Academy of Agricultural Sciences, Hangzhou, China
Gallotannins, characterized with the glycosidic core and galloyl unit, are seemed as vital components of hydrolyzable tannins. Benefit from the more and more discoveries of their bioactivities and edibility, application of gallotannins in food industry, pharmacy industry, and other fields is increasing. Inheriting previous study achievements, chemical structure of gallotannins was illustrated and degradation as well as synthetic routes to gallotannins were summarized. On this basis, distribution in the nature also including the distinction of gallotannins was discussed. More than that, activities involving in antioxidant, anti-inflammatory, enzyme inhibitions, protein binding, and so on, as well as applications in the field of food industry, biopharmaceutical science, agricultural production, etc., were combed. Finally, improvement of bioavailability, chemical modification of the structure, and accurate determination of new gallotannins were pointed out to be the orientation in the future.
Introduction
It had been well known that diet rich in fruits, vegetables, and nuts is beneficial to health. To trace these phenomena to their sources, phytochemicals contained in these products play an essential role in the health benefits. Phytochemicals, especially plant-derived polyphenolic compounds, have been proved to possess a wide range of pharmacological properties. Among the multitudinous food ingredients, tannins occupy an important place. Originally, “tannin” was coined by Seguin to describe the substances present in vegetable extracts (1). In the nature, tannins have been found in a variety of plants, fruits, wines, forages, and tea. With a large number of hydroxyl or other functional groups located on the skeleton, tannins are capable of forming cross-linkages with proteins and other macromolecules. Usually, tannins could covalently bind with alkaloids, gelatin, and proteins to form coagulation. Due to the precipitation with protein, tannins were introduced to leather tanning initially. Roughly divided into hydrolyzable tannins (HTs) and condensed tannins, tannins were reported with multiple health benefits, e.g., antioxidants, anti-inflammatory, anticancer (2–4). Belonging to HTs, gallotannins (GTs) and ellagitannins (ETs) are the most widely occurring tannins. Thereinto, GTs are one of the most often underlined components. In general, GTs are seemed as the simplest HTs (5). Comparatively, GTs were more likely to interact with lipid membranes for its more hydrophobicity (6). Characterized with the glycosidic core and galloyl unit, GTs are present in nearly all the plants in the nature. Herbals, which were rich in gallo- or ellagitannins, had been used to improve vascular health and many other diseases since antiquity. For the nutraceutical and prophylactic potentials, GTs have been of scientific interest. Lots of fruitful pioneer study had been documented (Table 1). According to the results of clinical, interventional, and animal in vivo studies, it could be clearly concluded that GT-containing products possessed anti-inflammatory potential (7). It seems that GTs are beneficial to reduce risk of chronic diseases connected with the elevated inflammatory state, particularly cardiovascular diseases (CVDs), (8) type 2 diabetes, (9) inflammatory bowel diseases (IBD), (10) and other gastrointestinal tract pathological conditions.
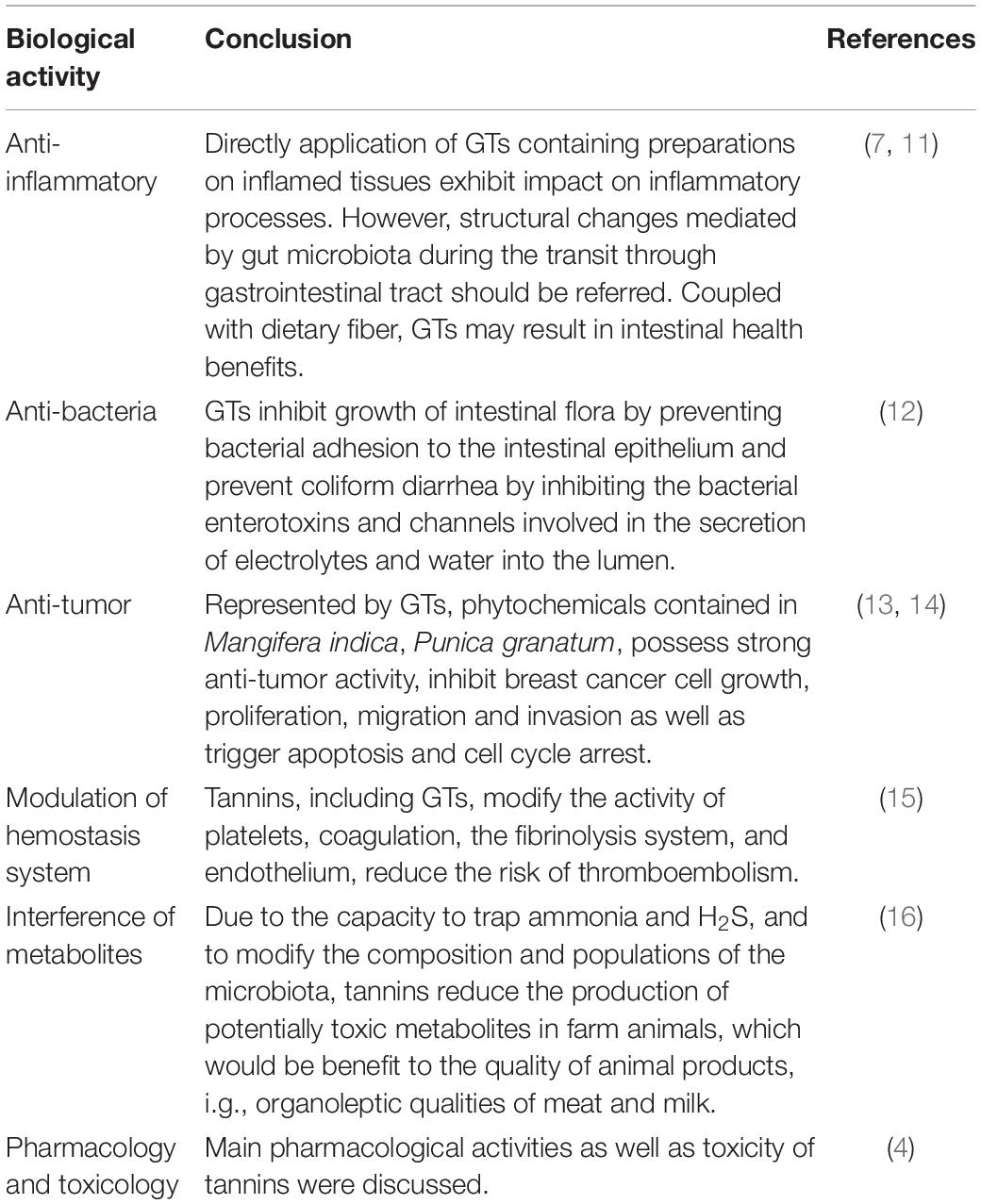
Table 1. Reviews related to physiological activities of gallotannins (GTs) documented in recent 5 years.
Despite study for centuries, there are still many confused conclusion and unresolved contradictions waiting to be figured out. For instance, structural definition and scientific classification are still ambiguous. Also, functional activities of GTs were multifarious. Thus, it is necessary to comb the superficial cognition about GTs.
Chemical Characterization of Gallotannins
Before discussing the biosynthetic pathway to GTs, it appears indispensable to insert a few comments on the structural principles and conventional definitions used in this field. First of all, it should be legible with regard to the chemical structure of GTs. Structurally surrounded by several galloyl units, GTs are with a polyol as the core, most often glucose. On the other hand, GTs are identified as polygalloyl esters of glucose. Apart from glucose, diverse polyol, catechin, or triterpenoid cores could be bounded to the galloyl unit (17). Such GTs, containing glucitol core, were identified from Red maple (Acer rubrum) (18). Unnatural GTs, taking 2-C-(hydroxymethyl)-branched aldoses as the central polyol, were designed and synthesized (19). Employing 1,5-anhydroalditol and inositol as the cyclic polyol cores, Machida et al. designed and obtained 14 unnatural gallotannin derivatives (20).
According to Niemeta and Gross (21), GTs could further be subdivided into “simple” and “complex” galloylglucoses. As a natural product, 1-O-galloyl-β-D-glucopyranose (compound 1, Figure 1), the simplest member of GTs, had been isolated and characterized more than a century ago. According to the number of galloyl groups, there are MoGG, one galloyl group; DiGG, two galloyl groups; TriGG, three galloyl groups; and TeGG, four galloyl groups. Fully substitution of galloyl to glucose would lead to 1,2,3,4,6-penta-O-galloyl-β-D-glucopyranose (PGG) (compound 2, Figure 1) (22), which seemed as the prototypical “complex” galloylglucoses (23). Sequentially, gallyol units can be grafted through depside bonds to form “complex” galloylglucoses with high molecular weight (MW). 2-O-digalloyl-1,3,4,6-tetra-O-galloyl-β-D-glucose (compound 3, hexagalloylglucose), the typical representative of these “complex” galloylglucoses, is given in Figure 1. One point to be emphasized was that gallic acid now combined with phenolic hydroxyls via a depsidic bond. As known, chemical properties are significantly different between the aliphatic OH-groups of the glucose and phenolic hydroxyl groups. It had been proved that the depside bonds between galloyl units are considerably easier to broken than the core ester linkages (6). In wake of the presence of an esteric linkage between two galloy moieties, GTs are also considered to be depsidic metabolites.
Degradation and Synthesis of Gallotannins
The degradation of GTs consists of a series of enzymatic catalyzed reactions. These enzymes refer to tannase, decarboxylase, pyrogallol 1,2-dioxygenase, pyrogallol-phloroglucinol isomerase, phloroglucinol reductase, dihydrophloroglucinol hydrolase, phenol oxidase, etc. First, cleavage of depside bonds takes place. Following, hydrolysis of the ester bond was undergone to yield gallic acid. Then, catalyzed by gallic acid decarboxylase, decarboxylation would be taken place on gallic acid to form pyrogallol. Ulteriorly, pyrogallol would convert to open loop secondary metabolites, e.g., pyruvic acid, and finally enters the Krebs cycle (24).
Also, GTs could be easily degraded by bacteria, fungi, and yeasts (25, 26). As reported, microorganisms, present in the rumen and the distal portion of the monogastric intestine of the ruminants, were involved in the hydrolysis of ester and depside linkages of GTs. During the procedure, glucose and gallic acid were released. Furthermore, gallic acid would continue degrade to pyrogallol and phloroglucinol and, ultimately, to acetate and butyrate. In the light of the present situation, biodegradation of GTs is still in an incipient stage. For the treatment with large-scale applications, especially in food, fodder, medicine, and tannery effluent, there is still a long way to go.
When it turns to the synthesis of GTs, biosynthesis would be first discussed. Derived from an intermediate of 5-dehydroshikimate, formation of gallic acid was reported to be through a shikimate pathway (27). Catalyzed by enzyme extracts from oak leaves, esterification between gallic acid and glucose was conducted to form β-glucogallin (1-O-galloyl-β-D-glucose) (28). During this procedure, uridine diphosphate (UDP) glucose served as an activated substrate. In association with the biosynthetic pathway, β-glucogallin is presumed to be the first intermediate and a key metabolite in the synthesis of GTs. Enzyme studies conducted by Grundhofer et al. indicated that β-glucogallin was required as a principal acyl donor for the biosynthesis of GTs (29). Without any other cofactors, the transformation of β-glucogallin, formed in situ, to di- and trigalloylglucose, was catalyzed by these enzymes. β-glucogallin was deemed to function not only as acyl acceptor, but also as efficient acyl donor (24). Demonstrated by immunohistochemical studies, leaf mesophyll cell walls were the typical site of origin and deposition of hydrolyzable tannins.
With extreme regularity, substitution of glucose hydroxyls was not randomly distributed in these conversions. Thus, the metabolic sequence followed the following order: β-glucogallin → 1,6-digalloylglucose → 1,2,6-trigalloylglucose → 1,2,3,6-tetragalloylglucose → 1,2,3,4,6-pentagalloylglucose. Accompanied with the formation of PGG, generally considered to be a simple galloyl glucose ester, the first stage for the biosynthetic pathway of GTs is completed. In all the plants, the pathway to PGG had been regarded to be identical. The transition from “simple” galloylglucoses to “complex” GTs is marked by the attachment of further galloyl residues to PGG to obtain the meta-depside groups (30). Taking PGG as the core, further galloylation reactions were conducted at the phenolic hydroxyl groups. During the second step, high-molecular metabolites containing up to 10 galloyl residues were formed (31).
On the other hand, for the reason of natural materials in short supply and difficulties in the isolation and purification, study work and the practical utilization of GTs were limited. Because of this, chemical synthesis of GTs was documented unremittingly. The first total chemical syntheses of two GTs, hexagalloylglucose and decagalloylglucose, was reported by Sylla et al. (32). Employing commercially 1,2:5,6-di-O-isopropylidene-α-D-glucofuranose as the starting material, the first galloyl moiety was introduced at the O3 position of the glucose unit. Under mild non-acidic Steglich-type conditions, successive galloylation reaction was taken place to give the corresponding 3-O-galloylated D-glucofuranose. Mediated by palladium in tetrahydrofuran (THF) at room temperature for 24 h, the hexagalloyl-β-glucopyranoses, PGG, would be obtained after the final hydrogenolysis of the fully protected hexagalloyl-D-glucopyranoses (32). Employing benzyl-protected gallic acid chloride as the substrate, reaction conditions that lead to high anomeric selectivity for the synthesis of GT were investigated. Accordingly, there is a significant correlation between solvent for the reaction and α:β selectivity. After screening, the highest selectivity was found when acetonitrile (CH3CN) was used as a solvent. Meanwhile, dichloromethane (CH2Cl2) was demonstrated to accelerate the rate of mutarotation relative to the rate of condensation to the ester (33). Starting from slight modification of methyl gallate, coupling with galloylation sequentially, alkyl GTs, which are structurally related with natural compounds, were synthesized (34). And then, protective effects were investigated.
Distribution and Distinction
Various galloyl-glucopyranoses occur naturally in lots of plants. Representatively, occurrence of GTs was found in the extracts of Chinese galls (Rhus semialata) and Turkish galls (Gallae turcicea). Also, presence of GTs in plants, such as Paeonia (23) and Schinus terebinthifolius, the leaves of Sicillian sumac (Rhus coriora L.) and the common smoke tree (Cotinus coggygria Scop.) were at sufficient levels to allow direct isolation and utilization (17). Among 42 edible beans, red sword bean (Canavalia gladiata) was found to have the highest content of GTs (35). Also, first report of the presence of GTs in nutshell of camellia tree (Camellia oleifera C. Abel) was disclosed by He et al. (36). From the byproducts of mango, barks, kernels, leaves, etc., GTs were identified and quantitated (37).
As to the refined structure, the various sources resulted in GTs with different chemical structures. As well, the concentration of GTs varies with plant genotype, tissue developmental stage, and environmental conditions (38). Several inconsistent theories had been developed regarding the chemical composition of GTs distributed in different plants. Although the contents varied greatly, the presence of GTs was fairly consistent across a range of cultivars. Under the circumstances, general assays were much preferred to elucidate specific structures of GTs. Chelation with metal ions and the formation of complexes guaranteed the detection of GTs by UV–Vis spectroscopy. Advances in describing GTs structural variation using high-performance liquid chromatography–mass spectrometry (HPLC–MS) had been made (39). Undoubtedly, simple colorimetric assays for the measurement of tannins and other phenolics facilitated many ecological studies of GTs, whereas the appropriate standard was crucial for the assay. Quantitatively, huge errors might be obtained if an inappropriate standard was used, for example, the commonly used quebracho tannin. Stumbling blocks for controlled studies on specific GTs were still existed due to the lack of commercially available standards. It is delightful, with the extraction of crude tannin and additional purification, a growing number of standards had been prepared by more and more researchers in a related field. Taking the degree of hydrolysis into consideration, amounts of free gallic acids were also indeterminate in quantity. According to the report, ratio of glucose to gallic acid was 1–9 or 10 in oriental sumac GTs in contrast to 1–5 or 6 in Turkish gall nuts (39).
Certainly, extraction would make a big difference in analysis of GTs. Despite the lack of commercially available standards, pioneer articles related to extraction, optimization, identification, and quantification of GTs were documented constantly. In respect to separation of GTs from mango kernels, extract process comprised of degreasing of seeds, extraction with aqueous acetone [80% (v/v)], and use of high-speed countercurrent chromatography for purification (40). With the crude extract in hand, subsequent purification was applied by being partitioned against ethyl acetate. According to the degree of galloylation (tetra-O-galloylglucose to deca-O-galloylglucose), solvent system employing hexane/ethyl acetate/methanol/water [0.5:5:1:5 (v/v/v/v)] was used in the head-to-tail mode to elute tannins. It had been proved that the using of hexane for the extraction of mango kernel fat would not affect GTs in respect to the profile and contents (41). In contrast, when methanol was used, significant changes were observed, which might result from the methanolysis of GTs to PGG. Methyl gallate and PGG would be yielded due to simultaneous extraction and methanolytic conversion of GTs. Degradation of higher GTs took place when methanol was used merely for the extraction of mango seeds. All these constituted important facets with respect to the utilization of GTs present in byproducts derived from mango fruit processing.
The rapid development of instruments as well as the innovation in analytical methods facilitates the discovery of new novel GTs. Meanwhile, improved detection accuracy guaranteed the detection of GTs in a wider range of plant species. Based on the flow-injection analysis–electrospray ionization–ion trap–tandem mass spectrometry (FIA-ESI-IT-MS-MS) and matrix-assisted laser desorption/ionization-time-of-flight mass spectrometry (MALDI-TOF-MS), a rapid and reliable analytical approach was proposed to investigate the full file of HTs present in the extracts of Astronium species and evidenced the existence of GTs in Astronium genus first (42). According to Boulekbache-Makhlouf, 18 GTs were present in fruit of Eucalyptus globulus growing in Algeria (43). By means of high-performance liquid chromatography–electrospray ionization mass spectrometry (HPLC–ESI-MS) method, assisted by diode array detection, 14 GTs could be isolated from birch (Betula pubescens) leaves (44). With the occurrence of absorption maxima between 275 and 280 nm on UV spectra, LC-MS molecular ion and fragment ion peaks, combining with NMR survey to the proton and carbon signals, five GTs were identified from Sapria himalayana f. albovinosa in Myanmar (45). Study result revealed that monogalloyl to hexagalloyl hexosides comprised the GTs film. Characterized by MALDI-TOF-MS, relying on the alteration of cationization reagents, Cs+, K+, and Na+, a different spectrum of Chinese GTs was given (46).
Physiological Activities of Gallotannins
There is one consensus to be emphasized that GT structural details are fateful for many of their biological effects. Equipped with the amount of hydroxyl groups (-OH) as well as the high molecular complexity, GTs were documented with several pro- and antiphysiological effects, such as antioxidant (47), antidiabetic (9), precipitating proteins (48), and antimicrobial (49). Theoretically, cooperative effects of hydrogen bonding and hydrophobic association were responsible for the GT–protein and GT–phospholipid interactions (48). Likewise, hydrogen bonding was pointed to be the predominant effect in the interactions between GTs and sugars.
Antioxidant
It is important to note that health benefits mentioned above all associated with the antioxidant capacity of GTs. Studies showed that these properties were result of the number of the galloyl groups and their position (50). Consistent with the number of the galloyl groups, interactions with proteins and ability to scavenge 2,2-diphenyl-1-picrylhydrazyl (DPPH) radicals improved (24). According to the study, antioxidative activity of GTs increased proportionally to the number of galloyl moieties (51). Determined antioxidative capacities of GTs on lipid peroxidation were PGG > TeGG > TGG > DiGG > GA (45). With regard to the placement of the galloyl groups, a study to investigate the inhibitory effects of 1,2, 6-,1,3, 6-, and 3,4,6-TriGG on lipid peroxidation in mitochondria and microsomes of the liver was conducted (52). The comparative antioxidative effect came to the conclusion: 1,3,6-TriGG > 1,2,6-TriGG > 3,4,6-TriGG. In contrast to inactive of 1,3,4,6-TeGG on the affinity of GTs to galloyltransferase, strong effect was shown by 1,2,3,6-TeGG (53). On the other hand, the galloyl groups of GTs were detected as the hydrophobic sites, through which interaction with aliphatic side chains of amino acids was established (29). Diversely, evaluation of antioxidant, antimicrobial, and antibiofilm activities indicated that type of sugar played an important role on the inhibitory effect (19).
Anti-inflammatory
Anti-inflammatory effects were shown by GT-containing products, especially in treatment with inflammatory bowel disease (IBD) and cardiovascular diseases (CVDs). In ulcerative colitis (UC), a primary component of IBD, GTs also exhibited significant advantages (54). Protective effect on dextran sulfate sodium (DSS)-induced colitis was witnessed by treatment with corilagin, a gallotannin present in many medical plants. Acting on the nuclear factor-kappa B (NF-κB) pathway, corilagin could mitigate colon inflammatory responses and the apoptosis of intestinal epithelial cells. By a self-assembly technique, Turkish galls GTs (TGTs)-FeIII microcapsules were prepared (55). Ex vivo and in vivo adhesion experiments showed that accumulation of the microcapsules was more likely to occur on the inflammatory surface and UC symptoms were effectively alleviated. To get a better sense of the postulated effectiveness, new perspective was given (7). Expression and activity of inducible nitric oxide synthase (iNOS), as well as the production of nitric oxide (NO), were inhibited by PGG (24). As a result, the production of proinflammatory cytokines and the adhesive molecules in endothelial cells would be decreased. Also, it would reduce the aggregation of thrombocytes and induce relaxation of contracted aortic rings. As documented, GTs could dose-dependently decrease gene expression and production of iNOS. As the result, lipopolysaccharide (LPS)-induced NO production was significantly reduced in macrophages. In other words, GTs isolated from Euphorbia species (Euphorbiaceae) showed an inhibitory effect on the LPS-induced inflammatory reaction (56).
Sensory Reinforcement
Being the predominant substance contributed to the orosensory sensation of food, GTs were employed to investigate the aversive behavior on astringency of foods by naïve mice (57). Undergoing a one-bottle preference test, ingestion of serial dilutions of GTs by inbred mice was assessed. The result indicated concentration-dependently inhibition of GTs on daily drink consumption. For this reason, drink intake was far predominant at night (circadian rhythm). Sometimes, astringency was tasted when we drink tea. A combination of tannin and oral salivary protein was responsible for the sensual experience.
Antidiabetic
The aggregation propensity of GTs equipped them with the potential to be used as antidiabetic drugs. With the numerous hydroxyl groups located on GTs skeleton, gallotannin-enzyme interactions were witnessed at the pancreatic lipase (PL)-colipase complex interface (58). According to molecular docking, GTs were provided with strong affinity toward the enzyme-substrate complex (uncompetitive inhibition). IC50 22.4 and 64.6 μM, respectively, implied GTs to be effective inhibitors, which endowed GTs to be used to treat dyslipidemias and obesity. Moreover, potential beneficial activity toward diabetes and metabolic syndrome was exhibited by PGG (24). Applying to adipocytes, stimulation of glucose transport and suppression of adipocyte differentiation were caused by PGG. Administration of glucitol-core containing gallotannins (GCGs)-enriched red maple leaves extract would be a benefit to colon-derived short-chain fatty acids (SCFAs) production and metabolic improvement (18). In turn, this supported the utilization of red maple GTs as a dietary ingredient for preventing obesity and related metabolic diseases. As a poly (ADP-ribose) glycohydrolase (PARG) inhibitor, protection of glomerular damage was suggested with the treatment of GTs. Through aggregation, GTs originated from Aleppo oak exerted the nature as non-specific promiscuous α-amylase inhibitors (9). Being inhibitor of α-glucosidase, GTs were reported to possess antidiabetic properties (51). As a hallmark for apoptotic cell death, protection in poly (ADP-ribose) polymerase (PARP) cleavage signified the protective role of GTs in cell death signaling (59). The result portended GTs to be used as an alternative approach to ameliorate the development of streptozotocin-induced diabetic nephropathy.
Induction of Apoptosis
Gallotannins were proved to be potent inhibitors and disruptors of a series of bacteria (19). As documented, the outer membrane conferred bacterial resistance to microbicide (60). Benefiting from the strong affinity for iron, GTs showed inhibitory activities against the growth of bacteria. In response to the shin-whitening effect, GTs isolated from Chinese galls showed inhibitory activity against tyrosinase and inhibited the biosynthesis of melanin, which is related to hyperpigmentation (61). In a study on the antitumor mechanism, cytotoxicity against HepG2 and Chang hepatocellular carcinoma cells was exhibited by GTs (21). By inhibiting cell adhesion and suppressing cell repair motility, GTs would induce apoptosis of hepatocellular carcinoma cells.
Application of Gallotannins
Food Processing
Hypothetically, GTs would be oxidized to reactive quinone by laccase (Lac) in laccase-mediator system (LMS) (62). Compared to the direct catalyzation of Lac, quinone could attack acid-swollen collagen (ASC) more easily. This would result in an efficient crosslinking and related performance of ASC. For the reinforcement, GTs were introduced as a mediator for application in ASC films. In the food industry, especially meat processing, LMS containing GTs would be option to modify collagenous material greenly. High selectivity on the regulation of the growth of bacteria was showed by penta-, hexa-, and heptagalloylglucose (63). In contrast to the inhibition of several pathogenic bacteria, growth of non-pathogenic lactic acid bacteria is not affected. This predicted the application of GTs as biopreservatives in foods. Fabricating insoluble gallotannin-pectin complexes, GTs could be bound by pectin (64). According to report, maximum mass ratios of combination GT to pectin for hydrochloric acid-extracted pectin (HEP) and chelating agent-extracted pectin (ChEP) were 0.28 and 0.47, respectively. It leads to ChEP was a better additive in beverage containing GTs, while HEP had advantage in emulsifying stability. In a dose-dependent manner, GTs improved collagen synthesis and reduced matrix metalloproteinase-1 (MMP-1) expression. In UVB-irradiated human cells, GTs downregulated MMP-1 levels through the extracellular signal-regulated kinase/c-Jun N-terminal kinase (ERK/JNK) signaling pathway (65). Also, glutathione rather than transforming growth factor β-1, which would induce fibrosis, would be increased by GTs.
Food Package
To extend shelf-life and reduce the risk from foodborne bacteria, many efforts had been tried on packing films/coasting. For the sake of the food packaging legislation of many regions and given the inactivation or evaporation of the antimicrobial agents under the harsh conditions (high temperature, pressure, and shear forces), allyl isothiocyanate or nanosilver films were circumscribed for use in food packing. Furthermore, the negative impact on organoleptic qualities of food was also an important reason. Thus, packaging film that was universally approved and commercialized available for antimicrobial of food was desired. For the proven antibacterial properties and food contact permit, GTs were introduced into food packaging films (66). The thermal process would trigger the release of free gallic acid from GTs and the high concentration of gallic acid would lead to an increase of antioxidant ability (67). Proudly, this character guaranteed GTs the inhibitory effect on lipid oxidation and convinced the use in the food package.
Plant Protection
Furthermore, as protein-binding agents, GTs are still often referred in the field of plant–insect interactions. Benefiting from the inherent affinity for proteins, GTs endowed the defense against pathogenic microbes to plants chemically. In addition, in blocking the infection of viral pathogens against insects and herbivores, GTs also played an important role. Both of these advantages might be via protein binding and enzyme inactivation caused by GTs. For instance, GTs in oak could reduce the infectivity of naturally occurring nuclear polyhedrosis viruses in gypsy moth caterpillars (68–70). Against Ralstonia solanacearum (R. solanacearum), potent antibacterial activity, in vitro and in vivo, was shown by GTs extracted from Sedum takesimense. Even more, GTs displayed broad-spectrum activity against various plant-pathogenic bacteria. This made it possible for the use of GTs as natural bactericides for the control of tomato bacterial wilt (71). Antibacterial activities in vitro showed that the strongest was against R. solanacearum. As documented, 1,2,6-tri-O-galloyl-β-D-glucopyranose isolated from Terminalia chebula fruit exhibited antibacterial action against multidrug-resistant uropathogenic Escherichia coli (E. coli) (72). Possibly, efflux pump inhibitory activity may be one of the mechanisms. Besides, revealed by Tuominen and Salminen that contents of polyphenol, GTs, e.g., differed significantly among ontogenic phases. According to this, evaluation of the role of polyphenols in plant–herbivore interactions would be feasible and render it plausible for the planned best collection times of Geranium sylvaticum with compound isolation purpose (73).
Phylaxiology
To some extent, GTs could be identified as the molecular basis of red wine and green tea for their benefits in prevention of cardiovascular disease and antisecretory action (74). According to documents, the calcium-activated Cl–1 channel (CaCC) plays an important role in cell physiology, in particular the treatment of hypertension, diarrhea, and cystic fibrosis. Being a modulator, GTs strongly inhibited TMEM16A with IC50 was 10.0 μM, which indicated potential utility for the treatment mentioned above.
Safety and Toxicity
Despite the diverse physiological activities as well as the extensive application, especially in food industry, double-edged sword of GTs should not be neglected. Intake of GTs might lead to damage of intestine and digestion, especially in susceptible species, e.g., sheep (75). In severe case, failure of kidney and liver would be resulted. Experimental data revealed that synthetic GTs, methyl 2,3,4,6-tetra-O-galloyl-α-D-glucoside (G4Glc), methyl 2,3,4,6-tetra-O-galloyl-α-Dmannoside (G4Man), and methyl 2,3,4-tri-O-galloyl-α-L-rhamnoside (G3Rham), possessed significant antioxidant activities (24). Whereas, very low genotoxic effect on human peripheral blood mononuclear cells (PBMCs) was manifested. Moreover, profiting from the protection of these compounds, damage induced by hydrogen peroxide or Fe2+ on DNA would be alleviated considerably. Based on these results, it could be concluded that synthetic GTs represent non-toxic agents, which implied the potential biomedical applications of GTs. At another level, it would be useful for the subsequent design of new antioxidants. Because of the binding with protein of GTs, bioavailability of both the proteins and polyphenols would be reduced. Previous studies suggested that vegetable tannins possess a high binding affinity to proline-rich proteins (76). Hydrophobic stacking of the planar phenolic ring against the pyrrolidine ring of the proline was pointed out to be the primary between PGG and proline-rich peptides. The molecular weight as well as structural flexibility of GTs was related to the ability of precipitation of protein. Depending on GTs concentration and nutrient levels, it would be beneficial for mammals for the ability to bind with proteins in the guts (41).
Conclusion and Prospect
Stand on the foundation of pioneer study, perception of GTs was refreshed. The precise chemical structure about GTs was illustrated to define the structural characteristics accurately. The degradation of GTs, with the presence of enzymes, was summarized cumulatively. The result would be beneficial for the understanding of the synthesis of GTs. Subsequently, distribution as well as the distinction of GTs distributed in different resources was proposed, which would be constructive for the utilization. Sequentially, the advantages of GTs, performed on antioxidant, anti-inflammatory, sensory reinforcement, antidiabetic, induction of apoptosis, etc., were combed systematically. All these endowed the good prospects of GTs for scale application. Following, applications of GTs in the food process, food package, plant protection, and phylaxiology were enumerated. In view of the dual property of phytochemicals, safety and toxicity of GTs were also referred.
Despite amount of excellent study and sufficient attention had been paid, accompanied with massive literature reports, insight into GTs is still at the very beginning stage. Concerted efforts are still needed to achieve more extensive and in-depth utilization of GTs.
Improvement of the Bioavailability
Due to the limited bioavailability of GTs, intake of GT-containing products orally can influence immune response at the level of the gastrointestinal tract. Modulating effects on the gut microbiota composition would be affected as well. More accurate delivery to affected areas as well as more efficient absorption and utilization is still in front of the urgent need to solve the problem.
Chemical Modification of the Structure
At the level of action mechanism, structure–function relationship indicated among polyphenolated templates, naturally occurring GTs may be not the optimal protein recognition agents in fact (77). For this reason, modification of the structure of GTs with the help of chemical synthesis was desired. From another perspective, assays for detection of GTs require the access to standard monomeric substance and chemical synthesis would be a powerful means. Development of the chemical synthetic methodology to construct GTs awaits to be optimized.
Accurate Determination of New Gallotannins
With the advantage of analytical instruments, it becomes possible for the determination of GTs with more accuracy and faster. Taking ultramodern instruments and technique into application, characterization of monomers and analogs of GTs remains to be clarified systemically. Also, content of trace in new resources would be no longer the obstacle for verification. As a result, it is worth looking forward to see the increase of the membership of GTs family.
Author Contributions
The author confirms being the sole contributor of this work and has approved it for publication.
Funding
I have grateful for financial support from the National Natural Science Foundation of China (Grant No. 32102443), the Key Research and Development Project of Hainan Province (Grant No. ZDYF2020108), and the Science and Technology Innovation Program of the Chinese Academy of Agricultural Sciences (CAAS-ASTIP-TRICAAS).
Conflict of Interest
The author declares that the research was conducted in the absence of any commercial or financial relationships that could be construed as a potential conflict of interest.
Publisher’s Note
All claims expressed in this article are solely those of the authors and do not necessarily represent those of their affiliated organizations, or those of the publisher, the editors and the reviewers. Any product that may be evaluated in this article, or claim that may be made by its manufacturer, is not guaranteed or endorsed by the publisher.
References
2. Watson RR. Nutrition and Functional Foods for Healthy Aging, Chapter 18-Polyphenols and Intestinal Health. Cambridge, MA: Academic Press (2017). p. 191–210.
3. Tollefsbol TO. Medical Epigenetics, Chapter 39-Natural Epigenetic-Modifying Molecules in Medical Therapy. Cambridge, MA: Academic Press (2016). p. 747–98.
4. Maugeri A, Lombardo GE, Cirmi S, Süntar I, Barreca D, Laganà G, et al. Pharmacology and toxicology of tannins. Arch Toxicol. (2022) 96:1257–77. doi: 10.1007/s00204-022-03250-0
5. Haslam E, Haworth RD, Jones K, Rogers HJ. Gallotannins. Part I. Introduction and the Fractionation of Tannase. J Chem Soc. (1961) 1829–35. doi: 10.1039/jr9610001829
6. Virtanen V, Karonen M. Partition Coecients (logP) of Hydrolysable Tannins. Molecules. (2020) 25:3691. doi: 10.3390/molecules25163691
7. Kiss AK, Piwowarski JP. Ellagitannins, gallotannins and their metabolites- the contribution to the anti-inflammatory effect of food products and medicinal plants. Curr Med Chem. (2018) 25:4946–67. doi: 10.2174/0929867323666160919111559
8. Burton-Freeman BM, Sandhu AK, Edirisinghe I. Mangos and their bioactive components: addingvariety to the fruit plate for health. Food Funct. (2017) 8:3010–32. doi: 10.1039/c7fo00190h
9. Szabo K, Hamori C, Gyemant G. Gallotannins are non-specific inhibitors of α-amylase: aggregates are the active species taking part in inhibition. Chem Biol Drug Des. (2021) 97:349–57. doi: 10.1111/cbdd.13787
10. Kim H, Venancio VP, Fang C, Dupont AW, Talcott ST, Mertens-Talcott SU. Mango (Mangifera indica L.) polyphenols reduce IL-8, GRO, and GM-SCF plasma levels and increase Lactobacillus species in a pilot study in patients with inflammatory bowel disease. Nutr Res. (2020) 75:85–94. doi: 10.1016/j.nutres.2020.01.002
11. Kim H, Castellon-Chicas MJ, Arbizu S, Talcott ST, Drury NL, Smith S, et al. Mango (Mangifera indica L.) polyphenols: anti-inflammatory intestinal microbial health benefits, and associated mechanisms of actions. Molecules. (2021) 26:2732. doi: 10.3390/molecules26092732
12. Girard M, Bee G. Invited review: tannins as a potential alternative to antibiotics to prevent coliform diarrhea in weaned pigs. Animal. (2020) 14:95–107. doi: 10.1017/S1751731119002143
13. Yap KM, Sekar M, Seow LJ, Gan SH, Bonam SR, Mat Rani NNI, et al. Mangifera indica (Mango): a promising medicinal plant for breast cancer therapy and understanding its potential mechanisms of action. Breast Cancer. (2021) 13:471–503. doi: 10.2147/BCTT.S316667
14. Moga MA, Dimienescu OG, Balan A, Dima L, Toma SI, Bîgiu NF, et al. Pharmacological and therapeutic properties of Punica granatum phytochemicals: possible roles in breast cancer. Molecules. (2021) 26:1054. doi: 10.3390/molecules26041054
15. Marcińczyk N, Gromotowicz-Popławska A, Tomczyk M, Chabielska E. Tannins as hemostasis modulators. Front Pharmacol. (2022) 12:806891. doi: 10.3389/fphar.2021.806891
16. Gasaly N, Gotteland M. Interference of dietary polyphenols with potentially toxic amino acidmetabolites derived from the colonic microbiota. Amino Acids. (2021) 54:311–24. doi: 10.1007/s00726-021-03034-3
17. Tuohy K, Del Rio D. Diet-Microbe Interactions in the Gut, Chapter 6 - Bioactivation of High-Molecular-Weight Polyphenols by the Gut Microbiome. Cambridge, MA: Academic Press (2015). p. 73–101.
18. Li L, Ma H, Liu T, Ding Z, Liu W, Gu Q, et al. Glucitol-core containing gallotannins-enriched red maple (Acer rubrum) leaves extract alleviated obesity via modulating short-chain fatty acid production in high-fat diet-fed mice. J Funct Foods. (2020) 70:103970. doi: 10.1016/j.jff.2020.103970
19. Hricovíniová Z, Mascaretti Š, Hricovíniová J, Čížek A, Jampílek J. New unnatural gallotannins: a way toward greenantioxidants, antimicrobials and antibiofilm agents. Antioxidants. (2021) 10:1288. doi: 10.3390/antiox10081288
20. Han HJ, Kwon HY, Sohn EJ, Ko H, Kim B, Jung K, et al. Suppression of E-cadherin mediates gallotannin induced apoptosis in hep G2 hepatocelluar carcinoma cells. Int J Biol Sci. (2014) 10:490–9. doi: 10.7150/ijbs.7495
21. Niemeta R, Gross GG. Enzymology of gallotannin and ellagitannin biosynthesis. Phytochem. (2005) 66:2001–11. doi: 10.1016/j.phytochem.2005.01.009
22. Torres-Leon C, Ventura-Sobrevilla J, Serna-Cock L, Ascacio-Valdés JA, Contreras-Esquivel J, Aguilar CN. Pentagalloylglucose (PGG): a valuable phenolic compound with functional properties. J Funct Foods. (2017) 37:176–89. doi: 10.1016/j.jff.2017.07.045
23. Li B, Ge J, Liu W, Hu D, Li P. Unveiling spatial metabolome of Paeonia suffruticosa and Paeonia lactiflora roots using MALDI MS imaging. New Phytol. (2021) 231:892–902. doi: 10.1111/nph.17393
24. Gross GG. Synthesis of mono-, di- and trigalloyl-β-D-glucose by β-glucogallin-dependent galloyltransferases from oak leaves. Z Naturforsch. (1983) 38c:519–23. doi: 10.1515/znc-1983-7-804
25. Niemetz R, Niehaus JU, Gross GG. Biosynthesis and biodegradation of complex gallotannins. In: GG Gross, RW Hemingway, T Yoshida editors. Plant polyphenols 2: Chemistry, Biology, Pharmacology and Ecology. New York, NY: Plenum Press (1999). p. 63–82. doi: 10.1007/978-1-4615-4139-4_4
26. Li M, Yao K, He Q, Dongying J. Biodegradation of gallotannins and ellagitannins. J Basic Microbiol. (2006) 46:68–84. doi: 10.1002/jobm.200510600
27. Werner I, Bacher A, Eisenreich W. Retrobiosynthetic NMR studies with 13C-labelled glucose. Formation of gallic acid in plants and fungi. J Biol Chem. (1997) 272:25474–82. doi: 10.1074/jbc.272.41.25474
28. Gross GG. Partial purification and properties of UDP-glucose: vanillate 1-O-glucosyl transferase from oak leaves. Phytochemistry. (1983) 22:2179–82. doi: 10.1016/s0031-9422(00)80141-7
29. Grundhofer P, Niemetz R, Schilling G, Gross GG. Biosynthesis and subcellular distribution of hydrolyzable tannins. Phytochemistry. (2001) 57:915–27. doi: 10.1016/s0031-9422(01)00099-1
30. Arapitsas P. Hydrolyzable tannin analysis in food. Food Chem. (2012) 135:1708–17. doi: 10.1016/j.foodchem.2012.05.096
31. Buzzini P, Arapitsas P, Goretti M, Branda E, Turchetti B, Pinelli P, et al. Antimicrobial and antiviral activity of hydrolysable tannins. Mini Rev Med Chem. (2008) 8:1179–87. doi: 10.2174/138955708786140990
32. Sylla T, Pouysegu L, Costa GD, Deffieux D, Monti JP, Quideau S. Gallotannins and tannic acid: first chemical syntheses and in vitro inhibitory activity on AlzheimerÏs amyloid β-peptide aggregation. Angew Chem Int Ed. (2015) 54:8217–21. doi: 10.1002/anie.201411606
33. Binkley RC, Ziepfel JC, Himmeldirk KB. Anomeric selectivity in the synthesis of galloyl esters of D-glucose. Carbohyd Res. (2009) 344:237–9. doi: 10.1016/j.carres.2008.10.024
34. Hricoviniova J, Sevcovicova A, Hricoviniova Z. Evaluation of the genotoxic, DNA-protective and antioxidant profile of synthetic alkyl gallates and gallotannins using in vitro assays. Toxicol In Vitro. (2020) 65:104789. doi: 10.1016/j.tiv.2020.104789
35. Gan R-Y, Kong K-W, Li H-B, Wu K, Ge YY, Chan CL, et al. Separation, identification, and bioactivities of the main gallotannins of red sword bean (Canavalia gladiata) coats. Front Chem. (2018) 6:39. doi: 10.3389/fchem.2018.00039
36. He Y-C, Wu M-J, Lei X-L, Yang J-F, Gao W, Bae Y-S, et al. Gallotannins from nut shell extractives of Camellia oleifera. J Korean Wood Sci. Technol. (2021) 49:267–73. doi: 10.5658/wood.2021.49.3.267
37. Barreto JC, Trevisan MTS, Hull WE, Erben G, de Brito ES, Pfundstein B, et al. Characterization and quantitation of polyphenolic compounds in bark, kernel, leaves, and peel of mango (Mangifera indica L.). J Agric Food Chem. (2008) 56:5599–610. doi: 10.1021/jf800738r
38. Barbehenn RV, Constabel CP. Tannins in plant–herbivore interactions. Phytochemistry. (2011) 72:1551–65. doi: 10.1016/j.phytochem.2011.01.040
39. Beaseley TH, Zlegler HW, Bell AD. Determination and characterization of gallotannin by high performance liquid chromatography. Anal Chem. (1977) 49:238–43. doi: 10.1021/ac50010a016
40. Engels C, Ganzle MG, Schieber A. Fractionation of gallotannins from mango (Mangifera indica L.) kernels by high-speed counter-current chromatography and determination of their antibacterial activity. J Agric Food Chem. (2010) 58:775–80. doi: 10.1021/jf903252t
41. Engels C, Ganzle MG, Schieber A. Fast LC–MS analysis of gallotannins from mango (Mangifera indica L.) kernels and effects of methanolysis on their antibacterial activity and iron binding capacity. Food Res Int. (2012) 45:422–6. doi: 10.1016/j.foodres.2011.11.008
42. da Silva VC, Napolitano A, Eletto D, Rodrigues CM, Pizza C, Vilegas W, et al. Characterization of gallotannins from Astronium species by flow injection analysis-electrospray ionization-ion trap-tandem mass spectrometry and matrix-assisted laser desorption/ionization time-of-flight mass spectrometry. Eur. J. Mass Spectrom. (2011) 17:365–75. doi: 10.1255/ejms.1141
43. Boulekbache-Makhlouf L, Meudec E, Chibane M, Mazauric JP, Slimani S, Henry M, et al. analysis by high-performance liquid chromatography diode array detection mass spectrometry of phenolic compounds in fruit of Eucalyptus globulus cultivated in Algeria. J Agric Food Chem. (2010) 58:12615–24. doi: 10.1021/jf1029509
44. Salminen J-P, Ossipov V, Loponen J, Haukioja E, Pihlaja K. Characterisation of hydrolysable tannins from leaves of Betula pubescens by high-performance liquid chromatography-mass spectrometry. J Chromatogr A. (1999) 864:283–91. doi: 10.1016/s0021-9673(99)01036-5
45. Iwashina T, Tanaka N, Aung MM, Devkota HP, Mizuno T. Phenolic compounds from parasitic Sapria himalayana f. albovinosa and Sapria myanmarensis (Rafflesiaceae) in Myanmar. Biochem Syst Ecol. (2020) 93:104179. doi: 10.1016/j.bse.2020.104179
46. Xiang P, Lin Y, Lin P, Xiang C, Yang Z, Lu Z. Effect of cationization reagents on the matrix-assisted laser desorption/ionization time-of-flight mass spectrum of Chinese gallotannins. J Appl Polym Sci. (2007) 105:859–64. doi: 10.1002/app.26373
47. Olivas-Aguirre FJ, Wall-Medrano A, Gonzalez-Aguilar GA, López-Díaz JA, Álvarez-Parrilla E, de la Rosa LA, et al. Hydrolyzable tannins; biochemistry, nutritional & analytical aspects and health effects. Nutr Hosp. (2015) 31:55–66.
48. He Q, Shi B, Yao K. Interactions of gallotannins with proteins, amino acids, phospholipids and sugars. Food Chem. (2006) 95:250–4. doi: 10.1016/j.foodchem.2004.11.055
49. Ekambaram SP, Perumal SS, Balakrishnan A. Scope of hydrolysable tannins as possible antimicrobial agent. Phytother Res. (2016) 30:1035–45. doi: 10.1002/ptr.5616
50. Hatano T, Yasuhara T, Yoshihara R, Agata I, Noro T, Okuda T. Effects of interaction of tannins with co-existing substances. VII. inhibitory effects of tannins and related polyphenols on xanthine oxidase. Chem Pharm Bull. (1990) 38:1224–9. doi: 10.1248/cpb.38.1224
51. Machida S, Sugaya M, Saito H, Uchiyama T. Synthesis and evaluation of gallotannin derivatives as antioxidants and ɑ-glucosidase inhibitors. Chem Pharm Bull. (2021) 69:1209–12. doi: 10.1248/cpb.c21-00566
52. Kimura Y, Okuda H, Okuda T, Hatano T, Okuda H, Arichi S. Studies on the activities of tannins and related compounds; V. Inhibitory effects on lipid peroxidation in mitochondria and microsomes of liver. Plant Med. (1984) 50:473–7. doi: 10.1055/s-2007-969776
53. Niemetz R, Gross GG. Gallotannin biosynthesis: purification of β-glucogallin: 1,2,3,4,6-pentagalloyl-β-d-glucose galloyltransferase from sumac leaves. Phytochemistry. (1998) 49:327–32. doi: 10.1016/s0031-9422(98)00014-4
54. Xiao H-T, Lin C-Y, Ho DHH, Peng J, Chen Y, Tsang SW, et al. Inhibitory effect of the gallotannin corilagin on dextran sulfate odium-induced murine ulcerative colitis. J Nat Prod. (2013) 76:2120–5. doi: 10.1021/np4006772
55. Zhang X, Zang J, Ma S, Yu W, Long F, Qi R, et al. Hollow microcapsules with ulcerative colitis therapeutic effects made of multifunctional turkish galls extraction. ACS Appl Mater Inter. (2019) 11:25054–65. doi: 10.1021/acsami.9b07557
56. Kim M-S, Park S-B, Suk K, Kim IK, Kim SY, Kim JA, et al. Gallotannin isolated from Euphorbia species, 1,2,6-Tri-O-galloyl-β -dallose, decreases nitric oxide production through inhibition of nuclear factor-κB and Downstream inducible nitric oxide synthase expression in macrophages. Biol Pharm Bull. (2009) 32:1053–6. doi: 10.1248/bpb.32.1053
57. Ramirez M, Toledo H, Obreque-Sliver E, Peña-Neira A, López-Solís RO. Aversive effect of tannic acid on drinking behavior in mice of an inbred strain: potential animal model for assessing astringency. J Agric Food Chem. (2011) 59:11744–51. doi: 10.1021/jf2029972
58. Moreno-Cordova EN, Arvizu-Flores AA, Valenzuela-Soto EM, García-Orozco KD, Wall-Medrano A, Alvarez-Parrilla E, et al. Gallotannins are uncompetitive inhibitors of pancreatic lipase activity. Biophys Chem. (2020) 264:106409. doi: 10.1016/j.bpc.2020.106409
59. Chandak PG, Gaikwad AB, Tikoo K. Gallotannin ameliorates the development of streptozotocin-induced diabetic nephropathy by preventing the activation of PARP. Phytother Res. (2009) 23:72–7. doi: 10.1002/ptr.2559
60. Engels C, Schieber A, Ganzle MG. Inhibitory spectra and modes of antimicrobial action of gallotannins from mango Kernels (Mangifera indica L.). Appl Environ Microb. (2011) 77:2215–23. doi: 10.1128/AEM.02521-10
61. Chen L-G, Chang W-L, Lee C-J, Lee LT, Shih CM, Wang CC. Melanogenesis inhibition by gallotannins from Chinese galls in B16 mouse melanoma cells. Biol Pharm Bull. (2009) 32:1447–52. doi: 10.1248/bpb.32.1447
62. Duan S, Wang W, Li S, Zhang K, Guo Y, Ma Y, et al. Moderate laccase-crosslinking improves the mechanical and thermal properties of acid-swollen collagen-based films modified by gallotannins. Food Hydrocoll. (2020) 106:105917. doi: 10.1016/j.foodhyd.2020.105917
63. Engels C, Knodler M, Zhao Y-Y, Carle R, Gänzle MG, Schieber A. Antimicrobial activity of gallotannins isolated from mango (Mangifera indica L.) kernels. J Agric Food Chem. (2009) 57:7712–8. doi: 10.1021/jf901621m
64. Zhang W, Xie F, Liu X, Luo J, wu J, Wang Z. Pectin from black tomato pomace: characterization, interaction with gallotannin, and emulsifying stability properties. Starch Stärke. (2019) 71:1800172. doi: 10.1002/star.201800172
65. Ryeom GGM, Bang WJ, Kim YB, Ge L. Gallotannin improves the photoaged-related proteins by extracellular signal-regulated kinases/c-Jun N-terminal kinases signalling pathway in human epidermal keratinocyte cells. J Med Food. (2018) 21:785–92. doi: 10.1089/jmf.2017.4096
66. Widsten P, Mesic BB, Cruz CD, Fletcher GC, Chycka MA. Inhibition of foodborne bacteria by antibacterial coatings printedonto food packaging films. J Food Sci Technol. (2017) 54:2379–86. doi: 10.1007/s13197-017-2678-5
67. Gonzalez MJ, Torres JL, Medina I. Impact of thermal processing on the activity of gallotannins and condensed tannins from Hamamelis virginiana used as functional ingredients in seafood. J Agric Food Chem. (2010) 58:4274–83. doi: 10.1021/jf904032y
68. Haslam E. Plants Polyphenols-Vegetable Tannins Revisited. Cambridge: Cambridge University Press (1989). 2 p.
69. Hemingway RW, Laks PE. Plant Polyphenols. Synthesis, Properties, Significance. New York, NY: Plenum Press (1992). p. 621–37.
70. Barbosa P, Krischik VA, Jones CG. Microbial mediation of plant-herbivore interactions. Wiley N Y. (1991) 16:489–506. doi: 10.1007/s00442-014-2950-6
71. Vu TT, Kim J-C, Choi YH, Choi GJ, Jang KS, Choi TH, et al. Effect of gallotannins derived from Sedum takesimense on tomato bacterial wilt. Plant Dis. (2013) 97:1593–8. doi: 10.1094/PDIS-04-13-0350-RE
72. Bag A, Chattopadhyay RR. Efflux-pump inhibitory activity of a gallotannin from Terminalia chebula fruit against multidrug-resistant uropathogenic Escherichia coli. Nat Prod Res. (2014) 28:1280–3. doi: 10.1080/14786419.2014.895729
73. Tuominen A, Salminen J-P. Hydrolyzable tannins, flavonol glycosides, and phenolic acids show seasonal and ontogenic variation in Geranium sylvaticum. J Agric Food Chem. (2017) 65:6387–403. doi: 10.1021/acs.jafc.7b00918
74. Namkung W, Thiagarajah JR, Phuan P-W, Verkman AS. Inhibition of Ca2+-activated Cl–1 channels by gallotannins as a possible molecular basis for health benefits of red wine and green tea. FASEB J. (2010) 24:4178–86. doi: 10.1096/fj.10-160648
75. Drozdzewska K, Potocnik E, Schwarz BC. Suspected acorn poisoning in a donkey – a case report. Wien Tierarztl Monat. (2018) 105:221–5.
76. Zhang H, Zhang L, Tang L, Hu X, Xu M. Effects of metal ions on the precipitation of penta-O-galloyl-β-D-glucopyranose by protein. J Agric Food Chem. (2021) 69:5059–66. doi: 10.1021/acs.jafc.1c01185
Keywords: gallotannins, structural characteristic, resource distribution, physiological activities, industrial application
Citation: He H-F (2022) Recognition of Gallotannins and the Physiological Activities: From Chemical View. Front. Nutr. 9:888892. doi: 10.3389/fnut.2022.888892
Received: 03 March 2022; Accepted: 15 April 2022;
Published: 01 June 2022.
Edited by:
Miguel Angel Prieto Lage, University of Vigo, SpainReviewed by:
Aurora Silva, Chemistry and Technology Network (REQUIMTE), PortugalFranklin Chamorro, University of Vigo, Spain
Marta Barral Martínez, University of Vigo, Spain
Copyright © 2022 He. This is an open-access article distributed under the terms of the Creative Commons Attribution License (CC BY). The use, distribution or reproduction in other forums is permitted, provided the original author(s) and the copyright owner(s) are credited and that the original publication in this journal is cited, in accordance with accepted academic practice. No use, distribution or reproduction is permitted which does not comply with these terms.
*Correspondence: Hua-Feng He, Y2FwdGFpbmh1YWZlbmdAdHJpY2Fhcy5jb20=