Effect of Maltodextrin and Soy Protein Isolate on the Physicochemical and Flow Properties of Button Mushroom Powder
- 1Department of Food Science and Technology, Sher-e-Kashmir University of Agricultural Sciences and Technology, Jammu, India
- 2Department of Food Processing Technology, Ghani Khan Choudhury Institute of Engineering and Technology, Maligram, India
- 3Department of Food Technology, Islamic University of Science and Technology, Awantipora, India
- 4Department of Food Science and Technology, Government Degree College Shopian, Srinagar, India
- 5Department of Botany, Hindu College Moradabad, Mahatma Jyotiba Phule Rohilkhand University, Bareilly, India
- 6Department of Medical Laboratory Technology, College of Applied Medical Sciences, Taibah University, Medina, Saudi Arabia
- 7Institute of Food Science, University of Debrecen, Debrecen, Hungary
- 8Faculty of Forestry, University of Khartoum, Khartoum North, Sudan
In this investigation, the effect of different drying techniques, such as freeze-drying and cabinet drying, with two different carrier agents, such as maltodextrin (MD) and soy protein isolate (SPI), at different levels (10, 15, and 20%) on button mushrooms has been revealed. The results showed that the button mushroom powders (BMPs) formulated with SPI as a carrier agent had significantly higher powder yield, hygroscopicity, L*, a*, and b* values, whereas BMP formulated with MD had significantly higher water activity, solubility index, tapped density, bulk density, and flowability. The highest retention of bioactive compounds was reported in freeze-dried mushroom powder compared to cabinet dried powder using SPI as a carrier agent. Fourier transform infrared (FTIR) analysis confirmed that certain additional peaks were produced in the mushroom button powder-containing SPI (1,035–3,271 cm−1) and MD (930–3,220 cm−1). Thus, the results revealed that SPI showed promising results for formulating the BMP using the freeze-drying technique.
Introduction
Mushrooms are edible fungi, and their production and consumption have substantially increased due to their nutritive value, flavor, and high delicacy (1, 2). In India, the mushroom industry is dominated by white button mushrooms, which is a largely sophisticated and capital-intensive operation. According to the latest production data (official data from ICAR-DMR, Solan), the total share of button mushrooms in India is the highest at 73%, followed by oyster mushrooms at 16%. Button mushrooms exhibit a soft texture, are perishable, and deteriorate quickly after harvest (3). Mushrooms are an excellent source of protein, vitamins, minerals, and antioxidants (4) and have potential therapeutic applications, such as the prevention of diabetes, cancer, and cardiac ailments (5), but are highly susceptible to microbial growth due to their higher moisture content ranging from 85 to 92%. The nutritional profile and unique umami flavor of button mushrooms are responsible for their extended cultivation and use. Button mushrooms are highly perishable due to their browning reactions and can open their umbrella with a limited shelf life. Therefore, a large proportion of mushrooms are subjected to drying processes for further use (6). Drying of mushrooms by traditional techniques is commonly used to regulate water activity; however, it results in profound nutrition loss (7). Freeze-drying, a low-temperature process, is a suitable method for drying bioproducts, causing a minimal loss of key functional ingredients (8) compared to thermal processing techniques. Despite being a capital-intensive process, it allows the development of high-quality dried food products. In the freeze-drying process, sublimation is responsible for removing water from the frozen product, which decreases bulk and tapped densities and increases particle porosity (9). Fruit-based powder products possess limitations such as enhanced hygroscopicity and flaking attributed to the availability of sugars and acids with lower molecular weight and to having lower glass transition temperature (10). Therefore, the incorporation of carrier agents such as high molecular weight carbohydrates (maltodextrins (MDs), waxy starch, gum arabic, and microcrystalline cellulose), soy protein isolates (SPIs), and organic solvents imbibes significant potential to produce powders with reduced hygroscopicity, increased powder yield, and improved thermal and microstructural properties (11, 12). Further, carrier agents improve the oxidative stability of the powder. Recently, protein-based carrier agents like SPI have been proven to be highly efficient in producing high product yields, even when used at lower concentrations, as compared to polysaccharides such as MD (13). Each of these carriers shows advantages and disadvantages in terms of cost, process efficiency, and the impact on final powder's properties. One of the disadvantage of using MD under storage conditions at high relative humidity is powder stickiness (14, 15).
Mushrooms are used in noodle products because of their unique flavors and health-promoting characteristics. Incorporating mushrooms into noodles would support the development of key organoleptic features, including the appearance, texture, and flavor of the developed product. Based on this, the objective of this research is to formulate a mushroom-based noodle, and the impact of mushrooms was assessed in terms of their physicochemical characteristics and textural aspects.
Materials and Methods
Raw Materials
Fresh button mushrooms were procured from the Division of Plant Pathology, SKUAST-Jammu. Button mushrooms were considered mature when the caps are well-rounded and the partial veil is totally intact. The length-to-thickness ratio of the stipe (stalk) should be small. The length of the drape should be long enough to allow minor trimming without cutting flush with the veil. Button mushroom caps have an average diameter of 4.51 ± 0.87 cm and are linked to short truncated stems with a height of 7.21 ± 1.23 cm. Raw materials were collected, washed, and cut into thin slices followed by drying.
Drying of Button Mushrooms
Freeze-Drying
Button mushrooms (Agaricus bisporus) were selected, washed, and cut into thin slices to provide uniform mixing with carrier agents. The samples were mixed with different carrier agents at different concentrations by adding 25 ml of distilled water to formulate the paste for proper mixing for drying purposes. In freeze-drying, mushroom slices were frozen at a temperature of −80°C in a conventional freezer and then subjected to freeze-drying in a freeze-dryer (SP Scientific VirTis Sentry 2.0, USA) at a pressure of 5 mTorr (0.666 Pa) for a period of 38 h. The obtained flakes were ground using a Mixer Grinder (Philips, India) (as shown in Figures 1–4).
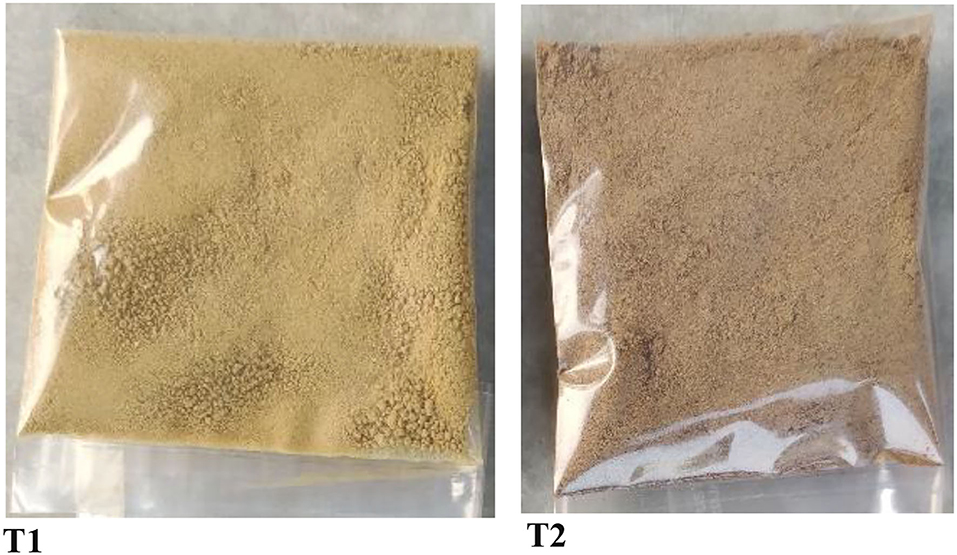
Figure 2. Freeze-dried untreated button mushroom powder (BMP) (T1) and cabinet dried untreated BMP (T2).
Cabinet Drying
In the cabinet drying process, the mushroom samples were washed, cut into thin slices, and dried at 50°C till the constant weight was obtained by grinding using a mixer grinder (Philips, India) in a similar way as in the case of a freeze dryer (as shown in Figures 1–4). The dried mushroom samples were then sealed in plastic pouches and then stored in frozen conditions for further analysis. Sample preparation was carried out using the different formulations (Table 1).
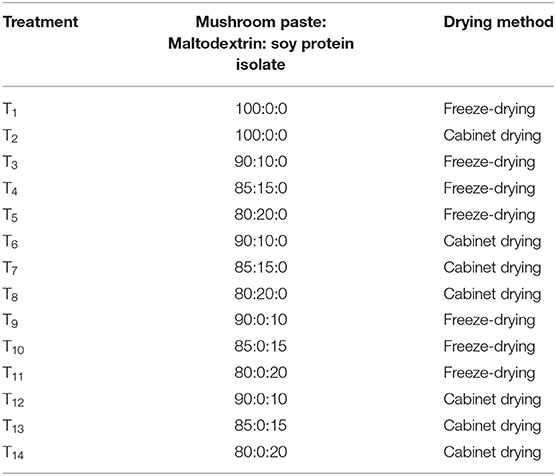
Table 1. Ratio of mushroom paste, maltodextrin (MD), and soy protein isolate (SPI) for making the button mushroom powder (BMP).
Functional Properties
Power Yield
Power yield was determined by calculating product recovery as a percentage ratio of the total mass of product recovery to the mass of the extract injected into the system.
Water Activity (aw)
The sample was filled (3/4th) into a cup of water activity meter (Aqua lab Pre, Decagon Device, USA). The instrument was calibrated as per the instruction manual calibration. The sample was kept in a cup until a constant reading was obtained.
Hygroscopicity
Hygroscopicity was calculated by weighing 1.5 g of the powder in an airtight container in a saturated solution of Na2CO3, and then, weighing was carried out after 1 week. Hygroscopicity was calculated as 1 g of adsorbed moisture per 100 g of the sample (16).
Solubility Index
The solubility index was measured by the method given by Hogekamp and Schubert (17). A 5-g sample was thoroughly mixed with 50 ml of distilled water. The mixture was then placed in a centrifuge tube and incubated in a water bath at 37°C for 30 min followed by centrifugation at 1,140 rpm for 20 m (Model-Remi PR-24). Finally, the supernatant was removed, collected in a pre-weighed Petri plate, and dried at 105°C for 4 h. The solubility index (%) was calculated using Equation (1):
Bulk Density, Tapped Density, Flowability (Carr Index), and Cohesiveness (Hausner Ratio)
The bulk density (ρB, g/ml) and tapped density (ρT, g/ml) of the mushroom powder were determined using the methods given by Santhalakshmy et al. (18) and Ozdikicierler et al. (19), as shown in Equations (2) and (3), respectively. The flowability of the sample was measured using the Carr index (CI), and the cohesiveness was measured using the Hausner ratio (HR), as described by Jinapong et al. (20) and shown in Equations (4) and (5), respectively:
Hunter Color Value
The color parameter of the mushroom dried by different methods was measured with a Hunter Lab color analyzer (Hunter Lab D-25, Ruston, USA) and expressed in terms of L*, a*, and b* values. The parameter L* indicated lightness or whiteness, a* indicated redness or greenness, and b* indicated yellowness or blueness. The instrument was first calibrated using a standard black tile and then calibrated using a standard white tile. The L*, a*, and b* values were obtained by placing a sample handling dish filled with dried powder in the analyzing port. On the other hand, the whiteness index (WI) of samples was calculated using the formula given in Equations (6) and (7):
Bioactive Properties
Estimation of Total Phenolic Content
The total phenolic content (TPC) in the mushroom powder was calculated using the spectrophotometric method given by Sawczuk et al. (21). The Folin–Ciocalteu (FC) method was used to determine the TPC of mushroom noodles. About 100 μl of extract solutions of various strengths are added to 1.5 ml of the FC reagent (10%). Approximately 1.5 ml of sodium carbonate (6%) was added to the liquid after 5 min. The measurement was obtained at 725 nm after allowing the mixture to react for 90 min at room temperature. Gallic acid (0.03–0.3 mg/ml) was used to formulate the calibration curve, from which the total polyphenolic content in the extract was determined. The results were expressed as milligrams of gallic acid equivalents per gram of the extract (mg/g).
Estimation of Total Flavonoid Content
The total flavonoid content (TFC) in the mushroom powder was evaluated using the procedure described by Ravanfar et al. (22). To determine the TFC, 0.50 ml of each extract stock solution (1 mg ml−1) and each dilution of the standard rutin solution (10–100 g ml−1) were used in test tubes. Each test tube was filled with 1.50 ml of methanol, 0.10 ml of aluminum chloride solution, 0.10 ml of potassium acetate solution, and 2.80 ml of distilled water and then shaken. Sample blanks for all extracts and standard rutin dilutions were produced in the same method but with distilled water rather than the aluminum chloride solution. All generated solutions were filtered using Whatmann filter paper No. 1 before being measured for absorbance. The absorbance was measured at 510 nm against a suitable blank. A rutin calibration curve was used to determine the TFC. The results were represented as milligrams of rutin equivalents (RE)/g of the extract.
Estimation of 2,2-Diphenyl-1-Picrylhydrazyl Radical Scavenging Activity
The free radical scavenging capacity of the sample was calculated with 2,2-diphenyl-1-picrylhydrazyl (DPPH) and DPPH radical scavenging activity using the procedure given by Kiselova-Kaneva et al. (23) and was evaluated using Equation (8):
where Ac = absorbance of the control and A = absorbance of the sample at 517 nm. The graph plotting each concentration and percentage inhibition yielded the IC50 value (the concentration of the sample extract required to inhibit 50% DPPH activity).
Reducing Power Activity Assay
The reducing power assay is used to determine the ability of an extract to reduce Fe3+ to Fe2+. The reducing power of the sample was calculated using the procedure given by Oyaizu (24). The reducing power of the sample extract was measured in terms of EC50 (concentration exhibiting 50% absorbance) and compared to that of butylated hydroxytoluene (BHT) and ascorbic acid.
Fourier Transform Infrared Analysis
Infrared (IR) spectra were measured using a Nicolet 360 Fourier transform IR (FTIR) spectrometer (USA) by mixing 0.1 g of the powdered sample with 0.5 g of potassium bromide for pellet preparation to attain the IR spectrum. The IR region was observed between the range of 650 and 4,000 cm−1, representing an average of 32 scans. All spectrums were measured under ambient conditions.
Statistical Analysis
All samples were prepared at various carrier agent concentrations and analyzed three times. The results were presented in the form of means and standard deviations (SDs). Significant differences between samples were analyzed by post-hoc analysis of variance (ANOVA). All statistical analyses were conducted using SPSS software.
Results and Discussion
Functional Properties of Button Mushroom Powder
Powder Yield
Process yield (powder yield) is an important parameter of the drying process because it influences cost and efficiency. It accounts for the total mass losses, including the extraction and drying processes. However, at a higher dosage of SPI, the moisture content of the button mushroom powder (BMP) also showed a significant increase, which may be due to the higher water holding capacity of proteins than polysaccharides, i.e., MD (25). The data in Table 2 show the effect of treatments on powder yield, revealing that different carrier agents have a significant impact on powder yield. BMP treated with MD showed a significantly lower yield than SPI. The powder yield of BMP varied from 15.90 to 19.90% for MD-treated powder and from 21.00 to 62.10% for SPI-treated powder in both cabinet dried and freeze-dried BMPs, respectively, which was lower than the yield of untreated powder at 15.06 and 18.00% for cabinet dried and freeze-dried powders, respectively. The results depicted that powder recovery increased significantly as the concentration of both carrier agents increased; however, the highest treatment yield was observed for SPI than for MD in freeze-drying. The highest yield in SPI was primarily due to the preferential transfer of more proteins to the interface of the feed solution and the formation of glassy skins (formation of protein-rich films) when exposed to dry and hot air, thus overcoming gumminess and stickiness and bonding (26). Cynthia et al. (27) observed the same trend of incorporation of a carrier agent into the powder yield of tamarind pulp extracts.
Water Activity
The basic preservation principle in drying is to reduce water activity to the level where biochemical reactions and microbial growth are no longer supported. The data in Table 2 show that the water activity of both cabinet dried and freeze-dried BMPs were significantly reduced with the incorporation of carrier agents. The water activity (aw) of the samples decreased from 0.62 to 0.36 for MD-treated powder and from 0.58 to 0.37 for SPI-treated powder in both cabinet dried and freeze-dried BMPs, which was less than that of untreated powder, which had 0.67 and 0.53 in cabinet dried and freeze-dried powders, respectively. The drying technique also significantly influenced the water activity of the samples. Water activity may directly affect the shelf life of the powder. The results depicted that the BMP prepared using MD as a carrier agent possessed significantly lower water activity than the powder produced using SPI. The variation in water activity may be attributed to the high hydrophilic attraction of SPI as compared to MD. The water activity value of 0.20–0.40 for dried foods is regarded as microbiologically safe, increasing the shelf stability of the products (28). Higher water activity may hamper the shelf stability of the products (29). Most bacteria, yeasts, and molds cannot grow below water activity values of 0.87, 0.88, and 0.80, respectively, as a result of decreased water activity (30). However, with a further decrease in water activity, powders produced with carrier agents confer a stable environment due to lower water activity as it is a key factor in maintaining the chemical reaction or chemical stability of the samples (31). However, freeze-dried powders with water activity <0.3 were comparatively less sensitive to microbial damage and more stable for bioactive compounds (32). Gurak et al. (33) observed the same trend, with a water activity of 0.43 for grape juice treated with MD by freeze-drying.
Hygroscopicity
Hygroscopicity can affect the powder's storage stability. With the incorporation of carrier agents, the hygroscopicity decreased. The data in Table 2 depict that the hygroscopicity of BMP decreased from 9.5 to 6.8% for MD-treated powder and from 9.8 to 6.4% in SPI-treated powder in both cabinet dried and freeze-dried BMPs, respectively, which was lower than that of untreated powder having hygroscopicity values of 9.8 and 11.2% for cabinet dried and freeze-dried powders, respectively. It was observed that freeze-dried powders had lower values than cabinet dried powders. The hygroscopicity of the powder can explain the water adsorption mechanism in the powders as being attributed to the presence of hydrophilic groups in the structure of each carrier agent. Differences in the values of hygroscopicity might be attributed to the rate at which they absorb water molecules from the environment and the nature of the powder (34). The large particle size, due to increased viscosity of the feed material, can reduce the total surface area, resulting in decreased hygroscopicity (10). The same trend was observed by Etzbach et al. (35) in carotenoid-rich goldenberry, where the hygroscopicity decreased from 17.2 to 14.1%.
Solubility Index
Solubility is an essential functional parameter of edible powders, which affects the powder behavior when reconstituted in water (36). The data presented in Table 2 depict that the addition of carrier agents had a significant effect on the solubility. The solubility index increased from 81.7 to 96.0% for MD-treated and from 84.2 to 93.2% for SPI-treated powders in both cabinet and freeze-dried BMPs, than that of untreated powder having solubility index of 78.8 and 80.1% for the cabinet and freeze-dried powders, respectively. Solubility is the key factor in determining the dispersibility and wettability of powders in water (37). Powder solubility may depend on the presence of hydrophilic groups (10). Powder solubility is an essential functional property that has a significant effect on other functional properties such as gelation, emulsification, and foaming properties (38). Zhang et al. (39) also reported that powders prepared with only 20% MD depicted higher solubility due to the high polarity of the polysaccharide. The same trend was also reported by Pudziuvelyte et al. (40) who revealed the solubility of freeze-dried powders in the range of 42.50–92.50%. Grabowski et al. (41) also observed that the solubility of sweet potato powder increased with increasing MD concentration. Freeze-dried powders showed a higher solubility index than cabinet dried powders. It has been also reported that solubility and bulk density are inversely proportional and that protein–protein interaction may increase solubility (15).
Flow Properties of BMP
Bulk Density
Bulk density is defined as the material density when packed or stacked in bulk. Bulk density is commonly used to characterize the end product obtained by drying or milling (42). The bulk density of the dried BMP is essential for determining the powder qualities and industrial operations associated with BMP production, packaging, storage, and distribution (43). The data presented in Table 3 show that the incorporation of carrier agents decreased the bulk density of BMP. The bulk density decreased from 0.510 to 0.400 g/ml for MD-treated powder and from 0.510 to 0.370 g/ml for SPI-treated powder for both cabinet dried and freeze-dried BMPs, respectively, which was less than that of the untreated powder having 0.526 and 0.405 g/ml for the cabinet dried and freeze-dried powders, respectively. The decrease in bulk density with the incorporation of carrier agents might be attributed to the increase in feed viscosity, which resulted in an increase in particle size (44). The rise in feed viscosity with increased protein percentage in the feed material, leading to the creation of aggregates and thus a reduction in bulk density, could be the reason for the decrease in bulk density with the increase in the addition rate of SPI compared to MD (24). Spray-dried bottle gourd and tamarind showed a similar pattern, with bulk density values in the range of 0.36–0.48 and 0.391–0.685 g/ml, respectively (10) (44).
Tapped Density
The tapped density of the BMP was based on the true solid density; however, it did not take into account the spaces between the particles (45). Table 3 demonstrates that the tapped density of cabinet dried and freeze-dried BMPs range from 0.43 to 0.65 g/ml for MD-incorporated powder and from 0.40 to 0.63 g/ml for SPI-incorporated powder. The results were lower than those for powders produced without MD and SPI, which had 0.68 and 0.49 g/ml for cabinet dried and freeze-dried BMPs, respectively. The decrease in tapped density with the incorporation of carrier agents indicates an increase in particle size. Low moisture content (reduced water activity) played an essential role in reducing the bulk and tapped density. The increase in the carrier agent concentration provides a large number of ramifications with hydrophilic groups, leading to lower residual moisture content and resulting in low bulk density (10). Bhat et al. (10) also reported reduction in the tapped density from 1.20 to 1.37 g/ml with the incorporation of carrier agents. The same pattern was observed by Sarabandi et al. (46), stating that, as the protein ratio increases, the viscosity of the feed increases, causing a decrease in density and an increase in particle size.
Flowability (Carr Index) and Cohesiveness (HR)
The Carr Index (CI) and HR are important in giving information regarding the flowability of the powder (47). Flowability and cohesiveness are the essential handling characteristics of dry powder. The flowability of the powder can be expressed as the Carr index (CI), while the cohesiveness is expressed as the HR (48). The Carr index (CI) and HR for better flowability of powders must be <15 and 1.25%, respectively. Table 3, pertaining to the HR and CI of both freeze-dried and cabinet dried BMPs, shows that, with the incorporation of carrier agents, CI decreased significantly from 21.53 to 6.97% in MD-treated powder and from 19.04 to 5.00% in SPI-treated powder in both cabinet dried and freeze-dried BMPs, respectively, which was less than that of the untreated powder having 22.64 and 17.34% for cabinet dried and freeze-dried BMPs, respectively. HR also decreased with the addition of carrier agents from 1.28 to 1.08 in MD-treated powder and from 1.25 to 1.07 in SPI-treated powder in both cabinet dried and freeze-dried BMPs, respectively, which was less than that of the untreated powder having 1.32 and 1.20 for cabinet dried and freeze-dried powders, respectively. It is evident from Table 3 that the freeze-dried powder will flow better than the powder after cabinet drying. After freeze-drying, the protein particle size increased, and the higher the particle size, the smaller the surface area per unit mass. The lower the possibility of surface interaction, the lower the cohesion, and the greater the flowability (49). HR and CI are highly sensitive to differences in shape and particle size distribution (10). Powder particle flowability was mostly influenced by surface characteristics (globular and smooth, or rough surface with dents) and particle size distribution (50). Tontul et al. (48) also revealed a similar pattern in tomato powder samples.
Color
Color is an essential quality parameter reflecting the sensory attractiveness and the quality of the powder (51). In dried foods, color is a quality indicator that provides information on the relative color change of fresh and dried materials (52). The incorporation of carrier agents significantly affected the color values (L*, a*, and b*). The data presented in Table 4 show that, as the level of carrier agents increased, the L* value increased, which can be due to the inherent whitish color of the carrier agent, while a* and b* decreased. The L* value increased in both MD- (65.16–85.26) and SPI-treated powders (63.87–76.23) in both cabinet dried and freeze-dried BMPs, which was higher than that of the untreated powder having 60.32 and 73.39 for the cabinet dried and freeze-dried powders, respectively. The addition of different carrier agents significantly reduced a* and b* in both drying procedures. The a* value decreased in both MD- (3.12–0.66) and SPI-treated powders (3.96–0.73) in both cabinet dried and freeze-dried BMPs, respectively, which were less than that of the untreated powder having 3.04 and 0.89 for the cabinet dried and freeze-dried powders, respectively. The b* value decreased in both MD- (11.37–7.87) and SPI-treated powders (12.01–10.37) in both cabinet dried and freeze-dried BMPs, respectively, which was less than that of the untreated powder having 12.26 and 10.22 for the cabinet dried and freeze-dried powders, respectively. Meanwhile, the WI value increased in both MD- (63.21–83.27) and SPI-treated (61.70–74.05) powders in both cabinet dried and freeze-dried BMPs, respectively, which was less than that of the untreated powder having 58.35 and 71.48 for the cabinet dried and freeze-dried powders, respectively. The changes in color parameters largely depend on the type and concentration of carrier agents in the feed solutions, which can be related to polyphenolic changes during drying (53). Ahmed et al. (54) showed that, in purple sweet potato powder, the L* value increased with the incorporation of MD as compared with untreated samples. The color values were attributed to the formation of polymeric anthocyanins. Padzil et al. (28) also observed that the L* value of the treated purple sweet potato extract increased with the incorporation of MD as the MD initially has a white color (L* = 98.18 ± 0.15), whereas the redness (+a*) of the treated purple sweet potato extract was significantly decreased as the concentration of MD increased. The increase in lightness and a reduction in chroma (color saturation) could be attributed to the dilution effect (55). The same trend was obtained for powdered pink guava with MD (56) and tamarind pulp with MD–SPI (57). Rocha-Parra also observed a decrease in a* (redness) and an increase in L* (lightness) with the incorporation of carrier agents (58). Bhusari et al. also observed similar results stating that tamarind pulp powder (TPP) with protein concentrate at all levels showed lower values of lightness (L) as compared to TPPs with MD (44). This may be due to the inherent color of the protein concentrate. Also, the a* and b* values decreased as the concentration of carrier agents increased. TPPs with protein concentrate showed high b* values than TPPs with MD (44).
Bioactive Properties of BMP
Total Phenolic Content
Determination of the TPC of carrier agent-treated powder was needed to calculate the powder efficiency. Different concentrations of carrier agents had a significant impact on the phenolic content. The data in Table 5 show that, with an increase in the concentration of carrier agents, the phenolic content decreased from 15.81 to 12.84 mg GAE/g in MD-treated powder and from 15.96 to 12.89 mg GAE/g in SPI-treated powder in both freeze-dried and cabinet dried BMPs, respectively, which was lower than that of the untreated powder having 17.06 and 20.30 mg GAE/g for the cabinet dried and freeze-dried powders, respectively. The powder produced with SPI depicted high retention, while the use of MD caused low TPC retention. This can be attributed to the strong interactions between polyphenols–proteins as compared to polyphenols–carbohydrates (10). TPC methodology is used to determine the number of phenolic compounds based on the formation of molybdate- and phosphotungstate-containing complexes and the electron transfer shown as a blue color in the solution (59). Wang et al. also observed that the TPC of mulberry juice powders was significantly decreased with an increased concentration of MD (49). A similar tendency was found for protein concentrate; TPC decreased significantly with an increase in the total solid content of proteins in the feed solution (60). Leyva-Porras et al. also showed a significant decrease in TPC with the incorporation of MD strawberry juice powder. Boonchu and Utama-ang also found that the TPC decreased in red grape pomace with an increase in MD concentration (59). Muzaffar and Kumar also found similar results stating that an increase in SPI concentration significantly reduced the DPPH radical scavenging activity of powders from 61.91 to 45.81 mg GAE/g, which could be correlated with the concentration effect of SPI at each concentration level (61). Mishra et al. also found a reduction in TPC as the concentration of carrier agents increased. It has been proven that increasing MD concentration in amla juice decreased the TPC, which further led to the decrease in radical scavenging capacity of the prepared powder (62). The overall decline in TPC can be due to enzymatic and oxidative degradation of polyphenols to subunits.
Total Flavonoid Content
The data in Table 5 depict that, with an increase in the concentration of carrier agents, the TFC decreased from 13.74 to 12.46 mg RE/g for MD-treated powder and from 13.92 to 12.49 mg RE/g for SPI-treated powder in both freeze-dried and cabinet dried BMPs, respectively, which was less than that of the untreated powder having 16.08 and 17.31 mg RE/g for the cabinet dried and freeze-dried powders, respectively. Similar results were also reported by Ahmed et al. (54), stating that the lowest flavonoid content was observed in purple sweet potato powders treated with an increased concentration of MD. In contrast, flavonoid content was high in powders treated with lower MD concentration. Muzaffar et al. (57) also observed a similar trend with the TFC in TPP of 5.96 CE mg/100 g, which was lower than fresh ripe tamarind pulp (12.22, 5.96 CE mg/100 g flavonoid content). TFC is highly susceptible to heating and oxidation (26). The TFC of the sample was highly affected by both the concentration of carrier agents and the drying method. More flavonoid retention was attained with freeze-drying than with cabinet drying; this change can be attributed to the temperature variation in the two different drying techniques.
DPPH Scavenging Activity
The data present in Table 5 show that, as the concentration of carrier agents increased, the scavenging activity of BMP decreased from 3.68 to 3.37 mg/ml in MD-treated powder and from 3.76 to 3.50 mg/ml in SPI-treated powder in both cabinet dried and freeze-dried BMPs, respectively, which was less than that of the untreated powder having 3.98 and 4.06 mg/ml for the cabinet dried and freeze-dried powders, respectively. In the DPPH assay, the freeze-dried powders achieved better antioxidant activity than cabinet dried samples, which may be because the higher temperature of cabinet drying led to the significant damage of thermolabile antioxidant compounds responsible for hydrogen or electron donors to the DPPH free radical, such as flavonoids, and similar results were reported by Miron et al. (63). Muzaffar and Kumar (61) also found similar results stating that an increase in SPI concentration significantly reduced the DPPH radical scavenging activity of the TPP samples from 61.91 to 45.81%, which can be related to the concentration effect of SPI at each concentration level. The scavenging activity of tamarind pulp can be related to polyphenols and flavonoids contributing to electron transfer or hydrogen donating ability.
Reducing Power Activity Assay
The EC50 value of the extract represents the concentration depicting 0.5 absorbances. The data present in Table 5 show that, with the incorporation of carrier agents, the reducing power decreased from 0.72 to 0.46 mg/ml in MD-treated powder and from 0.73 to 0.51 mg/ml in SPI-treated powder in both cabinet dried and freeze-dried BMPs, respectively, which was less than that of the untreated powder having 0.76 and 0.83 mg/ml for cabinet dried and freeze-dried powders, respectively. Silva et al. (64) also obtained similar results, stating that the reducing capacity was higher for powders treated with 15% MD and 30% gum arabic and lower for powders treated with 30% MD. The reduction in the reducing capacity of powders prepared with carrier agents was mainly due to component dilution (64). Variations in the values of bioactive components in samples can be due to the different carrier agents and different drying methods.
The influence of drying conditions on the antioxidant activity of the dried products was investigated by examining the relationship between TPC, TFC, DPPH and reducing power activity of the samples obtained under various drying conditions. Table 6 shows the Pearson correlation between TPC, TFC, DPPH, and the reducing power activity of the samples obtained under various drying conditions. TPC and TFC were shown to have a significant (p < 0.05) and positive association with antioxidant activity under different drying conditions.
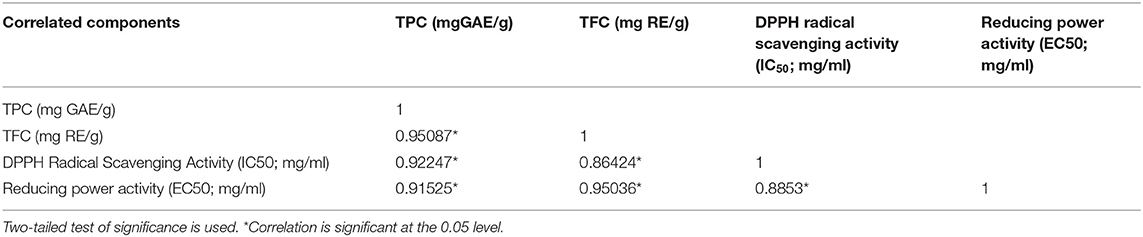
Table 6. Pearson's correlation coefficient for total phenolic content (TPC), total flavonoid content (TFC), 2,2-diphenyl-1-picrylhydrazyl (DPPH), and reducing power activity of BMP.
FTIR Analysis
Fourier transform IR analysis of the formulated powder products was carried out in the wavenumber range 650–4,000 cm−1 to study the chemical bond structures and identify the functional groups of the products. The IR spectrum of the developed products is shown in Figure 5. The freeze-dried powder product of the mushroom paste–MD showed significant peaks at 930, 1,019, 1,080, 1,147, 1,303–1,458, 1,635, 2,935, and 3,220 cm−1. The cabinet dried powder product of the mushroom paste–MD showed characteristic peaks at 930, 994, 1,077, 1,148, 1,305–1,458, 1,628, 2,925, and 3,265 cm−1. Major peaks of the cabinet dried powder product of the mushroom paste–SPI were observed at 1,035, 1,394, 1,525, 1,626, 2,927, and 3,271 cm−1. For the freeze-dried mushroom paste–SPI, significant peaks were observed at 884, 927, 952, 1,019, 1,078, 1,388, 1,524, 1,625, 2,939, and 3,277 cm−1.
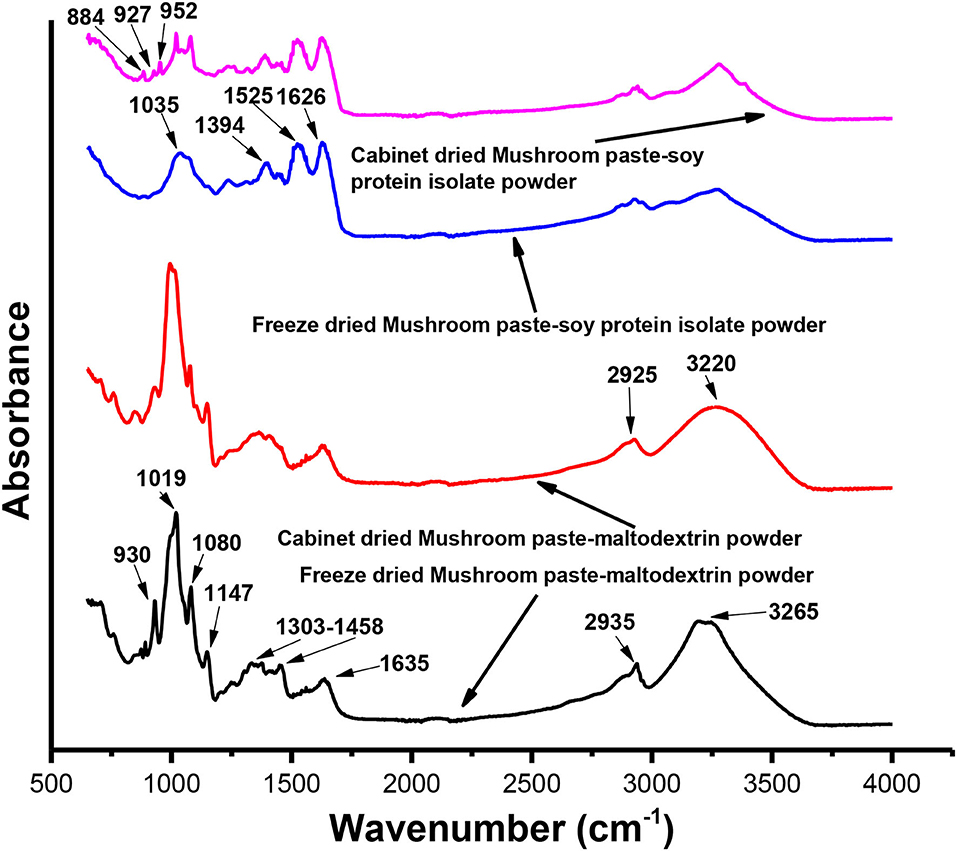
Figure 5. Fourier transform infrared (FTIR) analysis of freeze-dried and cabinet dried mushroom powders.
The wavenumber absorption band in the range of 800–1,300 cm−1 has been considered the “carbohydrate region” and is characterized by sharp overlapping peaks resulting from glycosidic linkages consisting of CO stretching, CC stretching, and COH bending vibrations (65–70). The wavenumber absorption peak in the range of 1,303–1,358 cm−1 could be ascribed to the N–H bending and C–N stretching of the “amide III group” of tertiary aromatic amines. The peak wavenumber in the range of 1,625–1,635 cm−1 could be C=O stretching of the “amide I group” of the antiparallel β-pleated sheet protein structures present in mushrooms (70–79). A characteristic absorption peak in the wavenumber range of 2,927–2,939 cm−1 could be assigned due to the CH stretching and denotes the presence of carbohydrates. The peak at 3,188–3,277 cm−1 could be assigned to the O–H stretching vibrations or N–H stretching groups (70, 71, 73, 80–82).
The reduction in the peak intensity of the freeze-dried mushroom–MD sample at wavenumbers of 930 and 1,077 cm−1 and a slight absorption peak shift from 1,080 to 1,077 cm−1 were observed. The reduction of peak intensities and the absorption peak shift from higher to lower wavenumbers indicates that the freeze-dried powder product has a weaker bonding structure than the cabinet-dried powder product. Deformation and weakening of the bonding structure could make the powder product less stable, resulting in a shortened shelf life. Absorption peaks of the mushroom–SPI powder at wavenumbers of 1,524–1,525 cm−1 could be assigned to the amide II region in proteins (83, 84). This bonding type of protein might be from the SPI (which is also very nutritious due to its high protein content) component of the powder. The amide II band at 1,524–1,525 cm−1 is very stable under different conditions (85). The N–H groups and O–H in the SPI component could form intra- and interhydrogen bonding with the C=O moiety of the protein structure (amino acids) of SPI and the mushroom. The formation of the –C–H bond was confirmed by the appearance of additional peaks at wavenumbers of 884, 927, and 952 cm−1 in cabinet dried mushroom paste and SPI powder, confirming the formation of undesirable different complex compounds. The formation of different complex compounds was undesirable for our target powder product. Hence, due to the presence of SPI without the formation of additional complex compounds, the freeze-dried powder product of the mushroom paste incorporated with SPI could be considered the best product because it is relatively more stable in addition to its higher protein content compared to others.
Conclusion
The present study investigated the performance of different carrier agents, such as MD and SPI, and button mushrooms during both freeze-drying and cabinet drying. The influence of incorporation of carrier agents at different concentrations on functional, flow, bioactive, and chemical parameters was investigated. SPI showed a positive increase in powder recovery. MD showed better solubility, and SPI displayed higher hygroscopicity. The results of this study also confirmed the efficacy of the addition of carrier agents to retain bioactive compounds. The highest recovery of phenolic compounds, calculated using FTIR of treated samples, depicted that the incorporation of carrier agents was proven to be effective, and freeze-drying was the most appropriate drying technique. It was concluded that SPI had higher potential as a carrier agent to produce BMPs during drying when compared with MD. BMPs are high in bioactive components that have antioxidant characteristics, such as phenolic and flavonoid compounds. Mushroom powders can be used as a supplement for a variety of staple foods because of their capacity to boost protein content and also to provide valuable health benefits from bioactive compounds.
Data Availability Statement
The original contributions presented in the study are included in the article/supplementary material, further inquiries can be directed to the corresponding author/s.
Author Contributions
RS, JS, KD, and AD contributed to the conception, design of the study, and wrote the first draft of this manuscript. GN and AD proposed the title of this manuscript. RS, JS, and KD wrote the sections of this manuscript. BK, AS, AA, HH, and MA have critically revised this manuscript. All authors collated papers, read, and approved the final version of this manuscript.
Conflict of Interest
The authors declare that the research was conducted in the absence of any commercial or financial relationships that could be construed as a potential conflict of interest.
Publisher's Note
All claims expressed in this article are solely those of the authors and do not necessarily represent those of their affiliated organizations, or those of the publisher, the editors and the reviewers. Any product that may be evaluated in this article, or claim that may be made by its manufacturer, is not guaranteed or endorsed by the publisher.
Acknowledgments
Project no. TKP2021-NKTA-32 has been implemented with the support of the National Research, Development, and Innovation Fund of Hungary, funded under the TKP2021-NKTA funding scheme.
References
1. Usman M, Murtaza G, Ditta A. Nutritional, medicinal, and cosmetic value of bioactive compounds in button mushroom (Agaricus bisporus): a review. Appl Sci. (2021) 11:5943. doi: 10.3390/app11135943
2. Giri SK, Prasad S. Optimization of microwave-vacuum drying of button mushrooms using response-surface methodology. Dry Technol. (2007) 25:901–11. doi: 10.1080/07373930701370407
3. Walde SG, Velu V, Jyothirmayi T, Math RG. Effects of pretreatments and drying methods on dehydration of mushroom. J Food Eng. (2006) 74:108–15. doi: 10.1016/j.jfoodeng.2005.02.008
4. Liu J, Jia L, Kan J, Jin C. In vitro and in vivo antioxidant activity of ethanolic extract of white button mushroom (Agaricus bisporus). Food Chem Toxicol. (2013) 51:310–16. doi: 10.1016/j.fct.2012.10.014
5. Loganathan J, Krishnan V, Ramalingam S, Kaviyarasan V. Comparitive study on the antioxidant, anticancer and antimicrobial property of Agaricus bisporus (J. E. Lange) Imbach before and after boiling. Afr J Biotechnol. (2009) 8:654–51.
6. Pei F, Yang W, Shi Y, Sun Y, Mariga AM, Zhao L, et al. Comparison of freeze-drying with three different combinations of drying methods and their influence on colour, texture, microstructure and nutrient retention of button mushroom (Agaricus bisporus) slices. Food Bioprocess Technol. (2014) 7:702–10. doi: 10.1007/s11947-013-1058-z
7. Argyropoulos D, Heindl A, Müller J. Assessment of convection, hot-air combined with microwave-vacuum and freeze-drying methods for mushrooms with regard to product quality. Int J Food Sci Technol. (2011) 46:333–42. doi: 10.1111/j.1365-2621.2010.02500.x
8. Xu D, Wei L, Guangyue R, Wenchao L, Yunhong L. Comparative study on the effects and efficiencies of three sublimation drying methods for mushrooms. Int J Agric Biol Eng. (2015) 8:91–7. doi: 10.3965/j.ijabe.20150801.012
9. Kaur M, Modi VK, Sharma HK. Effect of carbonation and ultrasonication assisted hybrid drying techniques on physical properties, sorption isotherms and glass transition temperature of banana (Musa) peel powder. Powder Technol. (2022) 396:519–34. doi: 10.1016/j.powtec.2021.11.006
10. Bhat S, Saini CS, Kumar V, Sharma HK. Spray drying of bottle gourd juice: effect of different carrier agents on physical, antioxidant capacity, reconstitution, and morphological properties. ACS Food Sci Technol. (2021) 1:282–91. doi: 10.1021/acsfoodscitech.0c00041
11. Pal S, Bhattacharjee P. Spray dried powder of lutein-rich supercritical carbon dioxide extract of gamma-irradiated marigold flowers: process optimization, characterization and food application. Powder Technol. (2018) 327:512–23. doi: 10.1016/j.powtec.2017.12.085
12. Zhang J, Zhang C, Chen X, Quek SY. Effect of spray drying on phenolic compounds of cranberry juice and their stability during storage. J Food Eng. (2020) 269:109744. doi: 10.1016/j.jfoodeng.2019.109744
13. Muzaffar K, Dinkarrao BV, Kumar P. Optimization of spray drying conditions for production of quality pomegranate juice powder. Cogent Food Agric. (2016) 2:1127583. doi: 10.1080/23311932.2015.1127583
14. Bae EK, Lee SJ. Microencapsulation of avocado oil by spray drying using whey protein and maltodextrin. J Microencapsul. (2008) 25:549–60. doi: 10.1080/02652040802075682
15. Wang W, Jiang Y, Zhou W. Characteristics of soy sauce powders spray-dried using dairy whey proteins and maltodextrins as drying aids. J Food Eng. (2013) 119:724–30. doi: 10.1016/j.jfoodeng.2013.06.047
16. Cai YZ, Corke H. Production and properties of spray-dried amaranthus betacyanin pigments. J Food Sci. (2000) 65:1248–252. doi: 10.1111/j.1365-2621.2000.tb10273.x
17. Hogekamp S, Schubert H. Rehydration of food powders. Food Sci Technol Int. (2003) 9:223–35. doi: 10.1177/1082013203034938
18. Santhalakshmy S, Don Bosco SJ, Francis S, Sabeena M. Effect of inlet temperature on physicochemical properties of spray-dried jamun fruit juice powder. Powder Technol. (2015) 274:37–43. doi: 10.1016/j.powtec.2015.01.016
19. Ozdikicierler O, Dirim SN, Pazir F. The effects of spray drying process parameters on the characteristic process indices and rheological powder properties of microencapsulated plant (Gypsophila) extract powder. Powder Technol. (2014) 253:474–80. doi: 10.1016/j.powtec.2013.12.004
20. Jinapong N, Suphantharika M, Jamnong P. Production of instant soymilk powders by ultrafiltration, spray drying and fluidized bed agglomeration. J Food Eng. (2008) 84:194–205. doi: 10.1016/j.jfoodeng.2007.04.032
21. Sawczuk R, Karpinska J, Filipowska D, Bajguz A, Hryniewicka M. Evaluation of total phenols content, anti-DPPH activity and the content of selected antioxidants in the honeybee drone brood homogenate. Food Chem. (2022) 368:130745. doi: 10.1016/j.foodchem.2021.130745
22. Ravanfar SA, Karimi E, Mehrabanjoubani P, Ebrahimi M. Enhancement of phenolic and flavonoids compounds, antioxidant and cytotoxic effects in regenerated red cabbage by application of Zeatin. Nat Prod Res. (2020) 34:898–902. doi: 10.1080/14786419.2018.1508145
23. Kiselova-Kaneva Y, Galunska B, Nikolova M, Dincheva I, Badjakov I. High resolution LC-MS/MS characterization of polyphenolic composition and evaluation of antioxidant activity of Sambucus ebulus fruit tea traditionally used in Bulgaria as a functional food. Food Chem. (2022) 367:130759. doi: 10.1016/j.foodchem.2021.130759
24. Oyaizu M. Studies on products of browning reaction antioxidative activities of products of browning reaction prepared from glucosamine. Jpn J Nutr. (1986) 44:307–316. doi: 10.5264/eiyogakuzashi.44.307
25. Ronkart S, Blecker C, Fougnies C, Van Herck JC, Wouters J, Paquot M. Determination of physical changes of inulin related to sorption isotherms: an X-ray diffraction, modulated differential scanning calorimetry and environmental scanning electron microscopy study. Carbohydr Polym. (2006) 63:210–17. doi: 10.1016/j.carbpol.2005.08.030
26. Fang Z, Bhandari B. Effect of spray drying and storage on the stability of bayberry polyphenols. Food Chem. (2011) 129:1139–47. doi: 10.1016/j.foodchem.2011.05.093
27. Cynthia SJ, Bosco JD, Bhol S. Physical and structural properties of spray dried tamarind (Tamarindus indica L.) pulp extract powder with encapsulating hydrocolloids. Int J Food Prop. (2015) 18:1793–800. doi: 10.1080/10942912.2014.940536
28. Padzil AM, Aziz AA, Muhamad I. I.Physicochemical properties of encapsulated purple sweet potato extract; effect of maltodextrin concentration, and microwave drying power. Malays J Anal Sci. (2018) 22:612–18. doi: 10.17576/mjas-2018-2204-06
29. Vardin H, Yasar M. Optimisation of pomegranate (Punica Granatum L.) juice spray-drying as affected by temperature and maltodextrin content. Int J Food Sci Technol. (2012) 47:167–76. doi: 10.1111/j.1365-2621.2011.02823.x
30. Bourdoux S, Li D, Rajkovic A, Devlieghere F, Uyttendaele M. Performance of drying technologies to ensure microbial safety of dried fruits and vegetables. Compr Rev Food Sci Food Saf. (2016) 15:1056–66. doi: 10.1111/1541-4337.12224
31. Bell LN. Moisture effects on food's chemical stability. In: Barbosa-Cánovas GV, Fontana AJ Jr., Schmidt SJ, Labuza TP, editors. Water Activity in Foods. Chicago, IL: John Wiley and Sons (2020). p. 227–53.
32. Daza LD, Fujita A, Fávaro-Trindade CS, Rodrigues-Ract JN, Granato D, Genovese MI. Effect of spray drying conditions on the physical properties of Cagaita (Eugenia dysenterica DC.) fruit extracts. Food Bioprod Process. (2016) 97:20–29. doi: 10.1016/j.fbp.2015.10.001
33. Gurak PD, Cabral LMC, Rocha-Leão MH. Production of grape juice powder obtained by freeze-drying after concentration by reverse osmosis. Braz Arch Biol Technol. (2013) 56:1011–17. doi: 10.1590/S1516-89132013005000008
34. Adetoro AO, Opara UL, Fawole OA. Effect of carrier agents on the physicochemical and technofunctional properties and antioxidant capacity of freeze-dried pomegranate juice (Punica granatum) powder. Foods. (2020) 9:1388. doi: 10.3390/foods9101388
35. Etzbach L, Meinert M, Faber T, Klein C, Schieber A, Weber F. Effects of carrier agents on powder properties, stability of carotenoids, and encapsulation efficiency of goldenberry (Physalis peruviana L.) powder produced by co-current spray drying. Curr Res Food Sci. (2020) 3:73–81. doi: 10.1016/j.crfs.2020.03.002
36. Tomsone L, Galoburda R, Kruma Z, Durrieu V, Cinkmanis I. Microencapsulation of horseradish (Armoracia rusticana L.) juice using spray-drying. Foods. (2020) 9:1332. doi: 10.3390/foods9091332
37. Du J, Ge Z-Z, Xu Z, Zou B, Zhang Y, Li C-M. Comparison of the efficiency of five different drying carriers on the spray drying of persimmon pulp powders. Dry Technol. (2014) 32:1157–66. doi: 10.1080/07373937.2014.886259
38. Meinlschmidt P, Schweiggert-Weisz U, Brode V, Eisner P. Enzyme assisted degradation of potential soy protein allergens with special emphasis on the technofunctionality and the avoidance of a bitter taste formation. LWT Food Sci Technol. (2016) 68:707–16. doi: 10.1016/j.lwt.2016.01.023
39. Zhang H, Yu D, Sun J, Liu X, Jiang L, Guo H, et al. Interaction of plant phenols with food macronutrients: characterisation and nutritional-physiological consequences. Nutr Res Rev. (2014) 27:1–15. doi: 10.1017/S095442241300019X
40. Pudziuvelyte L, Marksa M, Sosnowska K, Winnicka K, Morkuniene R, Bernatoniene J. Freeze-drying technique for microencapsulation of Elsholtzia ciliata ethanolic extract using different coating materials. Molecules. (2020) 25:2237. doi: 10.3390/molecules25092237
41. Grabowski JA, Truong V-D, Daubert CR. Spray-drying of amylase hydrolyzed sweetpotato puree and physicochemical properties of powder. J Food Sci. (2006) 71:E209–17. doi: 10.1111/j.1750-3841.2006.00036.x
42. Yousefi S, Emam-Djomeh Z, Mousavi SM. Effect of carrier type and spray drying on the physicochemical properties of powdered and reconstituted pomegranate juice (Punica granatum L.). J Food Sci Technol. (2011) 48:677–84. doi: 10.1007/s13197-010-0195-x
43. Shi Q, Fang Z, Bhandari B. Effect of addition of whey protein isolate on spray-drying behavior of honey with maltodextrin as a carrier material. Dry Technol. (2013) 31:1681–92. doi: 10.1080/07373937.2013.783593
44. Bhusari SN, Muzaffar K, Kumar P. Effect of carrier agents on physical and microstructural properties of spray dried tamarind pulp powder. Powder Technol. (2014) 266:354–64. doi: 10.1016/j.powtec.2014.06.038
45. Ferrari CC, Germer SPM, Alvim ID, Vissotto FZ, de Aguirre JM. Influence of carrier agents on the physicochemical properties of blackberry powder produced by spray drying. Int J Food Sci Technol. (2012) 47:1237–45. doi: 10.1111/j.1365-2621.2012.02964.x
46. Sarabandi KH, Peighambardoust SH, Sadeghi Mahoonak AR, Samaei SP. Effect of different carriers on microstructure and physical characteristics of spray dried apple juice concentrate. J Food Sci Technol. (2018) 55:3098–109. doi: 10.1007/s13197-018-3235-6
47. Barbosa-Cánovas GV, Juliano P. Physical and chemical properties of food powders. In: Oneulata C, editor. Encapsulated and Powdered Foods. Boca Raton, FL: CRC Press (2005).
48. Tontul I, Topuz A, Ozkan C, Karacan M. Effect of vegetable proteins on physical characteristics of spray-dried tomato powders. Food Sci Technol Int. (2016) 22:516–24. doi: 10.1177/1082013216629528
49. Wang R, Zhao Y, Zhu L, Fang Z, Shi Q. Effect of carrier types on the physicochemical and antioxidant properties of spray-dried black mulberry juice powders. J Food Meas Charact. (2020) 14:1201–12. doi: 10.1007/s11694-019-00369-0
50. Lumay G, Boschini F, Traina K, Bontempi S, Remy J-C, Cloots R, et al. Measuring the flowing properties of powders and grains. Powder Technol. (2012) 224:19–27. doi: 10.1016/j.powtec.2012.02.015
51. Quek SY, Chok NK, Swedlund P. The physicochemical properties of spray-dried watermelon powders. Chem Eng Process Process Intensif. (2007) 46:386–92. doi: 10.1016/j.cep.2006.06.020
52. Bansal S, Kumar S, Alam MS, Kumar M. Optimization of process parameters for multi-layer-cum microwave drying of oyster mushrooms (Pleurotus sajor caju). Int J Eng Res App. (2013) 3:206–17.
53. Horuz E, Altan A, Maskan M. Spray drying and process optimization of unclarified pomegranate (Punica granatum) juice. Dry Technol. (2012) 30:787–98. doi: 10.1080/07373937.2012.663434
54. Ahmed M, Akter MstS, Lee J-C, Eun J-B. Encapsulation by spray drying of bioactive components, physicochemical and morphological properties from purple sweet potato. LWT Food Sci Technol. (2010) 43:1307–12. doi: 10.1016/j.lwt.2010.05.014
55. Vargas-Muñoz DP, Kurozawa LE. Influence of combined hydrolyzed collagen and maltodextrin as carrier agents in spray drying of cocona pulp. Braz J Food Technol. (2020) 23:e2019254. doi: 10.1590/1981-6723.25419
56. Shishir MRI, Taip FS, Aziz NA, Talib RA. Physical properties of spray-dried pink guava (Psidium guajava) powder. Agric Agric Sci Proc. (2014) 2:74–81. doi: 10.1016/j.aaspro.2014.11.011
57. Muzaffar K, Dar BN, Kumar P. Assessment of nutritional, physicochemical, antioxidant, structural and rheological properties of spray dried tamarind pulp powder. J Food Meas Charact. (2017) 11:746–57. doi: 10.1007/s11694-016-9444-7
58. Rocha-Parra DF, Lanari MC, Zamora MC, Chirife J. Influence of storage conditions on phenolic compounds stability, antioxidant capacity and colour of freeze-dried encapsulated red wine. LWT. (2016) 70:162–70. doi: 10.1016/j.lwt.2016.02.038
59. Leyva-Porras C, Saavedra-Leos MZ, López-Martinez LA, Espinosa-Solis V, Terán-Figueroa Y, Toxqui-Terán A, et al. Strawberry juice powders: effect of spray-drying conditions on the microencapsulation of bioactive components and physicochemical properties. Molecules. (2021) 26:5466. doi: 10.3390/molecules26185466
60. Le Bourvellec C, Renard CMGC. Interactions between polyphenols and macromolecules: quantification methods and mechanisms. Crit Rev Food Sci Nutr. (2012) 52:213–248. doi: 10.1080/10408398.2010.499808
61. Muzaffar K, Kumar P. Effect of soya protein isolate as a complementary drying aid of maltodextrin on spray drying of tamarind pulp. Dry Technol. (2016) 34:142–8. doi: 10.1080/07373937.2015.1042586
62. Mishra P, Mishra S, Mahanta CL. Effect of maltodextrin concentration and inlet temperature during spray drying on physicochemical and antioxidant properties of amla (Emblica officinalis) juice powder. Food Bioprod Process. (2014) 92:252–8. doi: 10.1016/j.fbp.2013.08.003
63. Miron TL, Plaza M, Bahrim G, Ibáñez E, Herrero M. Chemical composition of bioactive pressurized extracts of Romanian aromatic plants. J Chromatogr A. (2011) 1218:4918–27. doi: 10.1016/j.chroma.2010.11.055
64. Silva VM, Kurozawa LE, Park KJ, Hubinger MD. Influence of carrier agents on the physicochemical properties of mussel protein hydrolysate powder. Dry Technol. (2012) 30:653–63. doi: 10.1080/07373937.2012.657727
65. Sritham E, Gunasekaran S. FTIR spectroscopic evaluation of sucrose-maltodextrin-sodium citrate bioglass. Food Hydrocoll. (2017) 70:371–82. doi: 10.1016/j.foodhyd.2017.04.023
66. Kokoszka S, Debeaufort F, Hambleton A, Lenart A, Voilley A. Protein and glycerol contents affect physico-chemical properties of soy protein isolate-based edible films. Innov Food Sci Emerg Technol. (2010) 11:503–10. doi: 10.1016/j.ifset.2010.01.006
67. Wang Z, Kang H, Zhao S, Zhang W, Zhang S, Li J. Polyphenol-induced cellulose nanofibrils anchored graphene oxide as nanohybrids for strong yet tough soy protein nanocomposites. Carbohydr Polym. (2018) 180:354–64. doi: 10.1016/j.carbpol.2017.09.102
68. Zhang S, Xia C, Dong Y, Yan Y, Li J, Shi SQ, et al. Soy protein isolate-based films reinforced by surface modified cellulose nanocrystal. Ind Crops Prod. (2016) 80:207–13. doi: 10.1016/j.indcrop.2015.11.070
69. Qin Z, Mo L, Liao M, He H, Sun J. Preparation and characterization of soy protein isolate-based nanocomposite films with cellulose nanofibers and nano-silica via silane grafting. Polymers. (2019) 11:1835. doi: 10.3390/polym11111835
70. Movasaghi Z, Rehman S, ur Rehman DrI. Fourier Transform Infrared (FTIR) spectroscopy of biological tissues. Appl Spectrosc Rev. (2008) 43:134–79. doi: 10.1080/05704920701829043
71. Fazio A, La Torre C, Caroleo MC, Caputo P, Plastina P, Cione E. Isolation and purification of glucans from an Italian cultivar of Ziziphus jujuba mill and in vitro effect on skin repair. Molecules. (2020) 25:968. doi: 10.3390/molecules25040968
72. Ciolacu D, Ciolacu F, Popa V. Amorphous cellulose - structure and characterization. Cellul Chem Technol. (2011) 45:13–21.
73. Oldenhof H, Wolkers WF, Fonseca F, Passot S, Marin M. Effect of sucrose and maltodextrin on the physical properties and survival of air-dried Lactobacillus bulgaricus: an in situ fourier transform infrared spectroscopy study. Biotechnol Prog. (2005) 21:885–92. doi: 10.1021/bp049559j
74. Balan V, Mihai C-T, Cojocaru F-D, Uritu C-M, Dodi G, Botezat D, et al. Vibrational spectroscopy fingerprinting in medicine: from molecular to clinical practice. Materials. (2019) 12:2884. doi: 10.3390/ma12182884
75. Tang C-H, Ma C-Y. Effect of high pressure treatment on aggregation and structural properties of soy protein isolate. LWT Food Sci Technol. (2009) 42:606–11. doi: 10.1016/j.lwt.2008.07.012
76. Wood BR, Kiupel M, McNaughton D. Progress in fourier transform infrared spectroscopic imaging applied to venereal cancer diagnosis. Vet Pathol. (2014) 51:224–37. doi: 10.1177/0300985813501340
77. Hu X, Lu Q, Sun L, Cebe P, Wang X, Zhang X, et al. Biomaterials from ultrasonication-induced silk fibroin–hyaluronic acid hydrogels. Biomacromolecules. (2010) 11:3178–88. doi: 10.1021/bm1010504
78. Lefèvre T, Subirade M, Pézolet M. Molecular description of the formation and structure of plasticized globular protein films. Biomacromolecules. (2005) 6:3209–19. doi: 10.1021/bm050540u
79. Lefèvre T, Subirade M. Molecular differences in the formation and structure of fine-stranded and particulate β-lactoglobulin gels. Biopolymers. (2000) 54:578–86. doi: 10.1002/1097-0282(200012)54:7<578::AID-BIP100>3.0.CO;2-2
80. Oliveira RN, Mancini MC, Oliveira FCS de, Passos TM, Quilty B, Thiré RM da SM, et al. FTIR analysis and quantification of phenols and flavonoids of five commercially available plants extracts used in wound healing. Matér Rio Jan. (2016) 21:767–79. doi: 10.1590/S1517-707620160003.0072
81. Bekiaris G, Tagkouli D, Koutrotsios G, Kalogeropoulos N, Zervakis GI. Pleurotus mushrooms content in glucans and ergosterol assessed by ATR-FTIR spectroscopy and multivariate analysis. Foods. (2020) 9:535. doi: 10.3390/foods9040535
82. Su J-F, Huang Z, Yuan X-Y, Wang X-Y, Li M. Structure and properties of carboxymethyl cellulose/soy protein isolate blend edible films crosslinked by Maillard reactions. Carbohydr Polym. (2010) 79:145–53. doi: 10.1016/j.carbpol.2009.07.035
83. Guerrero P, Kerry JP, de la Caba K. FTIR characterization of protein–polysaccharide interactions in extruded blends. Carbohydr Polym. (2014) 111:598–605. doi: 10.1016/j.carbpol.2014.05.005
84. Santiago-Adame R, Medina-Torres L, Gallegos-Infante JA, Calderas F, González-Laredo RF, Rocha-Guzmán NE, et al. Spray drying-microencapsulation of cinnamon infusions (Cinnamomum zeylanicum) with maltodextrin. LWT Food Sci Technol. (2015) 64:571–7. doi: 10.1016/j.lwt.2015.06.020
Keywords: maltodextrin, soy protein isolate, freeze-drying, cabinet drying, bioactive compounds, powder efficiency, button mushroom
Citation: Shams R, Singh J, Dash KK, Dar AH, Nayik GA, Ansari MJ, Hemeg HA, Ahmed AEM, Shaikh AM and Kovács B (2022) Effect of Maltodextrin and Soy Protein Isolate on the Physicochemical and Flow Properties of Button Mushroom Powder. Front. Nutr. 9:908570. doi: 10.3389/fnut.2022.908570
Received: 30 March 2022; Accepted: 25 April 2022;
Published: 27 May 2022.
Edited by:
R. Pandiselvam, Central Plantation Crops Research Institute (ICAR), IndiaReviewed by:
Ayon Tarafdar, Indian Council of Agricultural Research (ICAR), IndiaFeng Xue, Nanjing University of Chinese Medicine, China
Rakesh Kumar, Central University of South Bihar, India
Copyright © 2022 Shams, Singh, Dash, Dar, Nayik, Ansari, Hemeg, Ahmed, Shaikh and Kovács. This is an open-access article distributed under the terms of the Creative Commons Attribution License (CC BY). The use, distribution or reproduction in other forums is permitted, provided the original author(s) and the copyright owner(s) are credited and that the original publication in this journal is cited, in accordance with accepted academic practice. No use, distribution or reproduction is permitted which does not comply with these terms.
*Correspondence: Abdelhakam Esmaeil Mohamed Ahmed, ahmed.abdelhakam@agr.unideb.hu