- Soy Nutrition Institute Global, Washington, DC, United States
Feeding a growing global population with projected rising socioeconomic status will require additional sources of calories and especially protein. These sources need to align with the Sustainable Development Goals established by the Food and Agriculture Organization of the United Nations. The soybean is uniquely positioned to meet this challenge based on the following criteria: (1) Global soybean production exceeds by ~4 times the production of all pulses combined (2) Soybeans are higher in protein than other legumes and soy protein quality is similar to animal protein quality (3) Soybeans are an excellent source of healthy fat, including both essential fatty acids (4) Soybeans, like other legumes, symbiotically fix atmospheric nitrogen thereby reducing the need for fertilizer inputs (5) Greenhouse gas emissions per unit protein are lower than for nearly all other foods (6) Soybeans, like other legumes, are also recognized as an affordable food that can be incorporated into diverse diets regardless of economic standing and (7) The range of foods produced from soybeans constitutes an important position in historic and contemporary cuisines, cultures and emerging consumer trends for plant-based protein. Although most soybeans are currently used for animal feed, soybean use is dictated by consumer demand. Therefore, soybeans are well positioned to meet future global needs for energy and protein. Armed with this knowledge, health professionals can feel justified in encouraging greater consumption of soyfoods for both personal and planetary reasons.
Introduction
Sustainably producing sufficient food, and especially protein, for a global population expected to reach 9.7 billion by 2050 represents a significant challenge (1). Sustainable food patterns are those that are nutritionally adequate, economically affordable, socially acceptable, and conserve both agroecosystems and biodiversity (2). Consumers (3) and health and nutrition organizations (4, 5), including the Food and Agriculture Organization (FAO) of the United Nations (6), recognize the importance of meeting this challenge. In our view, the soybean (Glycine max (L.) Merr.) is uniquely positioned to help meet the caloric and protein needs of the growing global population.
The protein content of the soybean is higher (Table 1) than other legumes as is the quality of its protein (7). In fact, soybeans produce the highest protein yield per hectare (8) and are produced at a global scale far exceeding other legumes and pulses. For example, for the year 2020, ~13-fold more soybeans were produced than were produced of the common bean (~353 vs. ~28 million metric tons [MT]), which is the bean produced in the highest quantity aside from soybeans. These attributes are notable because evidence suggests that (1) the US protein recommended dietary allowance [RDA: 4–13 y, 0.95 g/kg; 14–18 y, 0.85 g/kg; ≥19 y, 0.80 g/kg (9)] may be too low for optimal health (10, 11) and (2) if supply and economic conditions allow, populations will elect to consume more protein than the RDA. Additionally, because of its high fat content (~20% of the dry weight), the caloric density of the soybean exceeds that of other beans, so it can more readily contribute to meeting energy requirements and the requirements for both essential fatty acids, the omega-6 fatty acid linoleic acid and the omega-3 fatty acid alpha-linolenic acid, which represent approximately 54.4 and 7.9% of the total fat content, respectively (12).
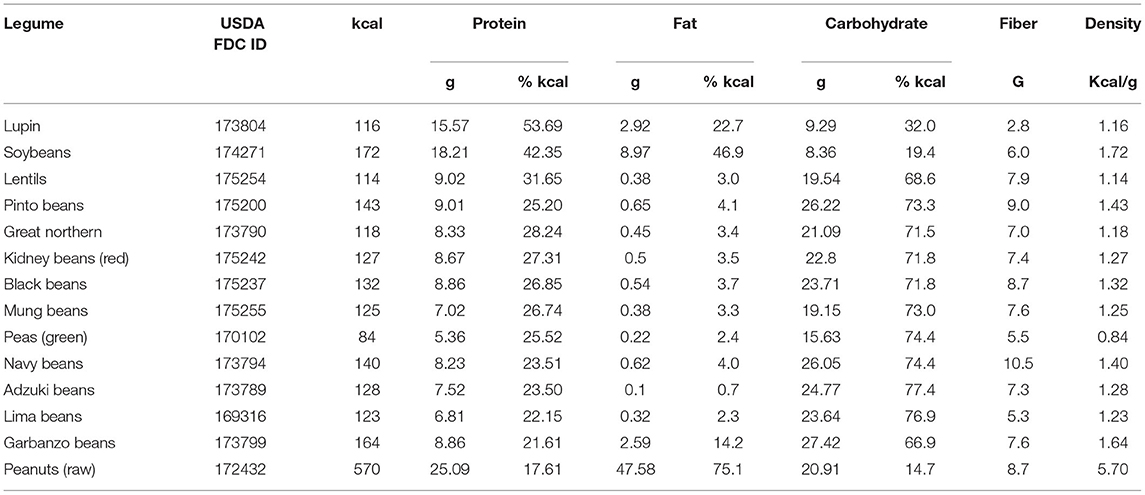
Table 1. Macronutrient, caloric and fiber content per 100 g of legumes (boiled unless otherwise indicated) according to the USDA nutrient database.
Furthermore, the wide range of foods that can be produced from soybeans, from the traditional Asian fermented (miso, natto, tempeh) and unfermented (tofu, soymilk, edamame, soynuts) soyfoods to modern soyfoods that use soy protein as a base to create energy bars, drinks, and meat and dairy alternatives, means the soybean can be embraced by populations with diverse cuisines. Importantly, the acreage devoted to soybean production dwarfs that of other dry beans (8) and growing soybeans has been shown to be a highly energy efficient and sustainable way to produce protein (13). Therefore, the scale, supply, and production systems for soybeans meet the challenge at hand. Finally, legumes in general are considered to be an affordable source of protein, thereby providing a nutrient dense solution for consumers under a range of socioeconomic conditions (14) Table 1.
Dietary Protein Requirements
Population Requirements
Valin et al. (15) estimated that relative to 2005 levels, global food demand would grow by 50 to 100% by 2050 due to an increase in per capita consumption. Protein demand will also substantially increase (16) due to population growth and the positive relationship between increasing incomes and protein intake (especially animal protein) (17). In 2017, Henchion et al. (18) projected that if each person consumes current maximum protein consumption levels (estimated at 103 g/d), protein production would need to increase by 78% to meet the needs of an expected population of 9.6 billion by 2050. Consistent with this estimate, Lieberman et al. (19) found that among 14 developed countries, protein intake was consistently ~16% of total energy, which is nearly twice the percentage of calories derived from protein needed to meet the adult RDA (10). This finding is consistent with the protein leverage hypothesis (20), which maintains there is a strong biological propensity to regulate the amount of protein consumed (21). Even Western vegans derive ~13% of their calories from protein (22, 23).
Individual Protein Requirements
The US adult protein RDA established by the National Academy of Medicine, (NAM) and the requirement established by the FAO and other health agencies is 0.8 g/kg bw (24–26). The RDA, which is based largely on the results of a meta-analysis of nitrogen balance studies (NBS) by Rand et al. (27), assumes an intake of good-quality protein (9). However, the NAM recognized the shortcomings of NBS and called for alternative means of assessing protein requirements (9). One such widely used alternative is the indicator amino acid oxidation method (28). utilization of this method has consistently shown protein requirements are approximately 50% greater than the RDA (29–31).
Requirements of Plant-Based Consumers
Interest in plant-based diets has raised concern that protein nutriture may suffer because of a lower overall protein intake and lower quality of plant protein in comparison to animal protein (32–36). Therefore, it is important to determine whether the protein RDA is applicable to those consuming plant-based diets. As noted, the US RDA is based on the intake of good quality protein, although no definition of “good” was provided by the NAM (37). Several authors (36, 38–40) although not all (41), have recommended that vegans and adherents of plant-based diets consume at least 10–20% more protein than the RDA to account for the lower digestibility of plant protein (42). The Health Council of the Netherlands recommends that lacto-ovo vegetarians and vegans consume 30% more protein than non-vegetarians (43). These recommendations are prudent, especially if little of the protein consumed is comprised of high-quality protein such as soy protein. Although many individuals will likely reduce their reliance on animal products, few are likely to completely abstain from meat and fewer still from all animal products (44–46).
The potential negative impact of consuming lower quality proteins on meeting protein requirements may be partially mitigated as a result of the efficiency of protein utilization from protein complementarity. When certain lower quality proteins are combined, their combined indispensable amino acid (IAA) profile can result in a higher protein score than the score for either protein alone. The combination of cereals and beans is the prototypical example of protein complementarity in the context of plant-based diets, although many other food combinations also work (47). Cuisines around the world reflect this dietary pattern. Numerous researchers have demonstrated the value of protein combining or fortifying low-quality protein sources with complementary proteins (48–50). In many developing regions of the world, soy flour (51, 52) and even okara (53) (a byproduct of soymilk production) have been combined with traditional protein sources to produce economically affordable foods common to the local cuisine that are higher in protein content and quality.
Soy Protein Quality
Most soy protein quality work has involved soy protein ingredients such as soy protein isolate (SPI), soy protein concentrate (SPC) and soy flour, which are comprised of ≥90%, 65–90% and 50–65% protein, respectively (54). Protein quality scores based on the protein digestibility corrected amino acid score (PDCAAS) for the soy protein ingredients range from 0.86 to 1.05 (Table 2). These scores easily exceed the threshold of ≥0.8 established by the USDA to qualify as a high-quality protein (7).
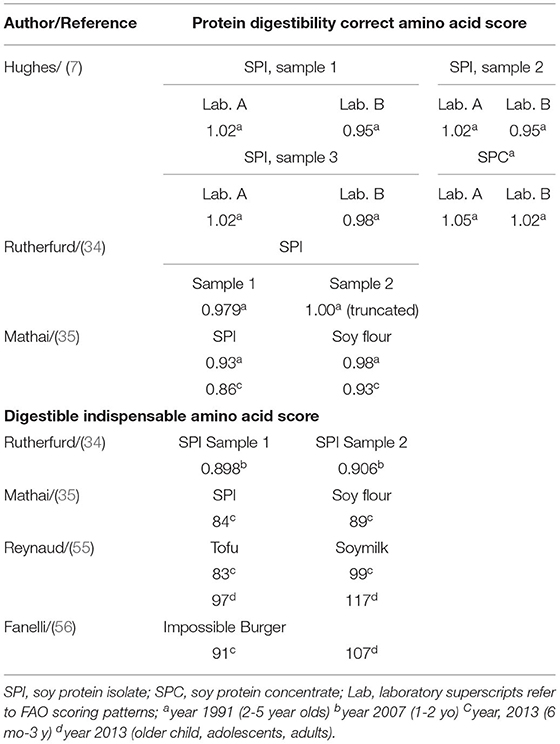
Table 2. Protein quality scores for soy protein derived from different soy products as determined by the PDCAAS and/or the DIAAS using different IAA scoring patterns.
The FAO recently recommended gradually replacing the Protein Digestibility Correct Amino Acid Score (PDCAAS) with the digestible indispensable amino acid score (DIAAS), although it will likely be many years before the DIAAS is adopted by regulatory agencies (57). The FAO developed three new scoring patterns for use with the DIAAS: birth to 6 months, 6 months to 3 years and >3 years (older child, adolescent and adult). Scores based on the DIAAS for the soy protein ingredients range from 84 to 90.6% (Table 2). Although only the younger two scoring patterns were recommended to be used for labeling purposes, the pattern for the older child, adolescent and adult applies to the majority of the population. Protein scores for both the traditional Asian soyfoods, soymilk and tofu (55), exceed the threshold (75%) established by the FAO for high-quality proteins as does the score for the Impossible BurgerTM, which derives most of its protein from SPC (58).
Potential Barriers To Soy Consumption
Non-nutrients
Plant foods contain many biologically active compounds capable of exerting health effects despite not being classified as nutrients (59, 60). The non-nutrients most relevant to the evaluation of soybeans as a source of protein are the protease inhibitors (PIs) and isoflavones. PIs can interfere with protein digestion; but this effect is not straightforward (61). PIs are inactivated by heat, but the extent to which this occurs is a function of temperature, duration, particle size, and moisture conditions. Since heat also denatures protein and thus lowers quality, there is a necessary compromise between the amount used to inactivate PIs and that which does not significantly adversely affect protein quality (62, 63).
Extensive work by Shi et al. (64) showed that soaking and cooking different beans at 95°C in water (1:5 seed:water) in a beaker for 1 h resulted in 100% of the chymotrypsin inhibitor activity and 80 to 100% of the trypsin inhibitor activity being destroyed. Clearly, relatively little PI remains in properly processed pulses. Concentrated sources of soy protein such as SPI are nearly completely devoid of PI content (65, 66). Although higher amounts are found in traditional Asian soyfoods (66–68), evidence indicates these amounts do not appreciably interfere with protein digestion (55) (Table 2). Older research by Rackis et al. (69) showed that reducing the PI content by only 40 to 50% greatly increases protein digestibility.
Isoflavones are a subclass of flavonoids, a larger and more ubiquitous group of polyphenols. Among commonly consumed foods, soybeans are a uniquely rich source of isoflavones (70). In traditional Asian soyfoods, for each gram of soy protein there are approximately 3.5 mg isoflavones (71) whereas SPI and SPC contain very low levels (70)due to losses of 80 to 90% during processing (70). Consequently, health effects of isoflavones pertain mostly to whole soybeans, traditional soyfoods and soy flour.
Isoflavones are classified as phytoestrogens, although they differ significantly from estrogen both clinically and at the molecular level (72, 73). They have been posited to reduce risk of several chronic diseases (74) but are also being investigated for potential adverse effects, especially related to breast cancer, male feminization, and thyroid function. However, the American Cancer Society (75), the American Institute for Cancer Research (76), the World Cancer Research Fund International (77) and the Canadian Cancer Society (78) concluded that women with breast cancer can safely consume soyfoods. Also, the European Food Safety Authority (EFSA) (79) and the Permanent Senate Commission on Food Safety of the German Research Foundation (SKLM) (80) concluded that isoflavone supplements (soyfoods were not evaluated) do not adversely affect breast tissue. The EFSA (79) and the SKLM (SKLM) also concluded that isoflavones do not affect thyroid function, a position consistent with a recently published meta-analysis (81). The notion that soyfoods feminize men is refuted by a 2021 meta-analysis of 41 intervention studies showing neither soyfoods nor isoflavones lower circulating testosterone levels or raise estrogen levels (82) and clinical work showing they do not increase risk of gynecomastia (83, 84) or adversely affect sperm and semen parameters (85–87).
Especially important insight about potential concerns about soy came in 2017 from the US Food and Drug Administration (FDA) when it concluded that consuming 25 g/d soy protein is safe (88). This conclusion was based on comprehensive evaluation of the scientific literature and an examination of hundreds of comments submitted by the public. Although the primary focus of that review was on soy protein, nearly all safety concerns related to isoflavones. The FDA's conclusion aligns with a 2021 comprehensive technical review that evaluated 417 reports (229 observational studies, 157 clinical studies and 32 systematic reviews and meta-analyses) (89).
Soy Protein Allergy
Regulatory agencies have recognized the need to focus allergen labeling regulations on a limited set of priority allergens. In the US, eight foods (commonly referred to as the “Big 8”) fall under the mandated labeling requirements. These eight foods (milk/dairy, eggs, fish, crustacean shellfish, tree nuts, peanuts, wheat, and soy) are thought to account for 90% of the food allergy reactions among Americans. However, the prevalence of allergy for each of these eight foods differs markedly. North American surveys show that the prevalence of soy allergy is lower than the prevalence reported for each of the other seven major allergens (90). A rough estimate is that three adults per 1,000 are allergic to soy. In comparison, the prevalence of milk/dairy allergy is 5–10x higher than soy allergy. Although soy is one of the Big 14 in Europe, the prevalence of IgE-mediated soy allergy among Europeans appears to be lower than for many commonly-consumed foods not included in the Big 14 (91, 92).
The prevalence of soy allergy among US children/adolescents is also low. Data from the National Health and Nutrition Examination Survey (years 2007–2010) show that among the Big 8 foods, the prevalence of soy allergy (0.25%) was the lowest. The prevalence of milk/dairy allergy was ~8 times greater than soy allergy (93). Furthermore, estimates based on clinical experience are that approximately 70% of children outgrow their soy allergy by age 10 (94).
Environmental Impact of Soy Protein
Calls have been made to consume largely plant-based diets because of their lower overall environmental footprint (95, 96). As concerns about the environment increase, it is probable that interest in soy-based meat and dairy alternatives will also rise. However, there are widely differing opinions about the environmental effects of diet (97–99).
Soybeans, like all legumes, fix nitrogen due to bacterial symbionts (rhizobia) that inhabit soybean root nodules (100), reducing reliance on chemical fertilizer. An estimated half the nitrogen used for crop fertilization globally is lost into the environment, creating environmental concerns (101, 102). It is notable that the environmental impact of soybean production is often portrayed negatively due to its link with deforestation in South America (103, 104). However, production of soybeans in the US, the second leading producer of soybeans in the world (105) does not lead to deforestation (106).
In 2011, Gonzalez et al. (13) determined that of the 22 plant and animal protein sources evaluated, soybeans were the most efficiently produced and provided the most protein (g) per greenhouse gas emissions (GHGE) (kg CO2 equivalents). In Sweden, Carlsson-Kanyama and Gonzalez (107) determined that even when considering the environmental import costs, soybeans provided far more protein per amount of GHGE than nearly all other foods. And based on their analysis, Saarinen et al. (108) concluded that the soybean cements its position as a climate friendly and healthy food based on nutrient density and global warming potential (GWP).
Tessari et al. (109) emphasized that when evaluating the environmental impact of foods, it is important to consider nutritional value and in particular, IAA content. When this metric was used, there was little difference between animal and plant protein sources, except for soybeans, which exhibited the smallest environmental footprint. Moughan (110) also recently expressed the need to consider protein quality when evaluating the environmental impact of protein sources.
In contrast to other legumes, most soy consumed by humans is not eaten in the form of the whole bean. Therefore, it is important to consider both the cost of producing soybeans as well as the cost of producing soybean-based products. van Mierlo et al. (111) concluded soy protein is a key ingredient when trying to mimic the nutrient profile of meat while minimizing environmental impact with regard to climate change, land use, water use and fossil fuel depletion. Other investigators have also documented the environmental advantages of soy protein ingredients (112–116). For example, when evaluating 32 foods based on protein quality as determined by the DIAAS and GWP, Berardy et al. (112) found the three best performing foods were peanuts, whey, and SPI.
Versatility of Soybeans
In 2020, of the slightly more than ~353 million MT of soybeans produced globally, approximately 113 million (31.8%) were produced in the US. Soybean production exceeds the global production of annual oilseeds (minus trees, ~272 million MT) and is much greater than global pulse production (~90 million MT) (117). Although there are concerns about the environmental impact of soybean production in Brazil (118), which is the leading soybean producer in the world, as noted previously, similar concerns are not expressed about the US production of soybeans (106).
The soybean is comprised of approximately 20% oil (by weight); approximately 85% of which is used for human consumption, although most focus is on the non-lipid component. At current production levels (for year 2018, 4.39 billion bushels, 11 pounds protein per bushel), the US soybean crop alone could deliver approximately 6 g/d protein for the entire anticipated 2050 population. Although currently just under 80% of the world's soybean crop is fed to livestock, the amount used directly for human consumption could increase in response to demand for additional dietary diversity (119). Because of the dominant use of soybeans throughout parts of Asia, soyfoods already make a substantial contribution to protein intake. For example, in Japan, soy accounts for approximately 10% of total protein intake (71, 120). Soyfood intake in China varies considerably among regions because Chinese dietary habits are quite heterogenous (121, 122). but in Shanghai, approximately 16% (123) and 13% (124) of total protein intake of men and women, respectively, is derived from soy.
However, legumes play a small role in the diets of developed countries and their direct intake is not expected to increase in the coming years in any region in the world (14, 125–127). Therefore, if soybeans are to play a larger role in meeting global protein needs, it will not likely be via the consumption of whole soybeans but via foods made from soybeans and from soy protein ingredients (concentrated source of soy protein). The vast array of traditional soyfoods that can be made from soybeans underscores the role this legume can play in meeting global protein needs. On the other hand, meat has played an important role in the diet of mankind since the beginning of time and will continue to have a strong cultural and gastronomic significance (128). Research indicates that although vegetarian and vegan consumers will accept plant-based alternatives that lack meat-like sensory properties, omnivorous and flexitarian consumers prefer alternatives that as much as possible resemble meat (129–132).
That is why it is important that the new generation of plant-based meats are formulated to emulate the taste and texture of meat (as opposed to, for example, a black bean burger) and are designed to be used in a similar manner (133). Bianchi et al. (134) recently demonstrated the potential role of meat substitutes in reducing reliance on and changing attitudes toward meat. Finally, hybrid meats, the combination of animal and plant protein, may be an especially appealing and efficacious approach to increasing dietary protein diversity because research has found that to create an effective dietary change, new practices should not diverge too much from consumers' previous behavior (135–137). If hybrid meat products increase in popularity, soy protein ingredients can contribute to their success as manufacturers have considerable experience using these combinations (119, 138).
Conclusion
As the global population grows over the next 30 years, there will an increased need for sustainably produced food and dietary protein. The soybean, which is higher in protein than other legumes, may be in a unique position to help fill this need. It is efficiently produced and versatile as the range of foods that can be produced from this legume can fit within vastly different cuisines. Most notable in this regard are the meat substitutes made using concentrated sources of soy protein. Although other plant sources are also used for this purpose, the high quality of soy protein and the availability of soybeans make it an ideal choice. The affordability of different soyfoods varies considerably but many are less expensive than other commonly consumed sources of protein and their cost is expected to decrease as volume increases. By learning more about the health and environmental advantages of soybeans and soyfoods, nutritionists, dietitians and other health professionals will be better equipped to council their patients and clients about the benefits of incorporating soy into their diet. As consumers become increasingly interested in plant-based diets, such knowledge will become more imperative.
Data Availability Statement
The original contributions presented in the study are included in the article/supplementary material, further inquiries can be directed to the corresponding author.
Author Contributions
MM wrote the initial and all subsequent drafts of the manuscript and read and approved the final manuscript.
Conflict of Interest
MM is employed by the Soy Nutrition Institute Global, an organization that receives funding from the United Soybean Board (USB) and from industry members who are involved in the manufacture and/or sale of soyfoods and/or soybean components.
Publisher's Note
All claims expressed in this article are solely those of the authors and do not necessarily represent those of their affiliated organizations, or those of the publisher, the editors and the reviewers. Any product that may be evaluated in this article, or claim that may be made by its manufacturer, is not guaranteed or endorsed by the publisher.
Acknowledgments
The author wishes to thank Lisa M. Balbes, Ph.D., of Balbes Consultants LLC for editorial support.
References
1. United Nations Department Department of Economic and Social Affairs Population Division (2019). World Population Prospects 2019: Highlights (ST/ESA/SER.A/423).
2. Auestad N, Fulgoni V L III. What current literature tells us about sustainable diets: emerging research linking dietary patterns, environmental sustainability, and economics. Adv Nutr. (2015) 6:19–36. doi: 10.3945/an.114.005694
3. Food & Health Survey. International Food Information Council. Available online at: https://foodinsight.org/2020-food-and-health-survey/.
4. Blackstone N T, El-Abbadi N H, McCabe M S, Griffin T S, Nelson ME. Linking sustainability to the healthy eating patterns of the Dietary Guidelines for Americans: a modelling study. Lancet Planet Health. (2018) 2:e3442:e344–52. doi: 10.1016/S2542-5196(18)30167-0
5. Rose D, Heller MC, Roberto CA. Position of the society for nutrition education and behavior: the importance of including environmental sustainability in dietary guidance. J Nutr Educ Behav. (2019) 51:3–15. doi: 10.1016/j.jneb.2018.07.006
6. Burlingame B, Dernini S. Sustainable Diets and Biodiversity Directions and Solutions for Policy, Research and Action. Rome, Italy: FAO: (2012).
7. Hughes GJ, Ryan DJ, Mukherjea R, Schasteen C S. Protein digestibility-corrected amino acid scores (PDCAAS) for soy protein isolates and concentrate: criteria for evaluation. J Agric Food Chemistry. (2011) 59:12707–12. doi: 10.1021/jf203220v
8. Hartman G L, West E D, Herman T K. Crops that feed the World 2. Soybean G L, West E D, Herman T K. Cnd constraints caused by pathogens and pests. Food Security. (2011) 3:5–17. doi: 10.1007/s12571-010-0108-x
9. Institute of Medicine of the National Academies.. Dietary Reference Intakes for Energy, Carbohydrate, Fiber, Fat, Fatty Acids, Cholesterol, Protein, and Amino Acids. Washington, DC: National Academy Press (2005) p. 686.
10. Wolfe RR, Cifelli AM, Kostas G, Kim IY. Optimizing protein intake in adults: interpretation and application of the recommended dietary allowance compared with the acceptable macronutrient distribution range. Adv Nutr. (2017) 8:266–75. doi: 10.3945/an.116.013821
11. Elango R, Humayun M A, Ball R O, Pencharz P B. Evidence that protein requirements have been significantly underestimated. Curr Opin Clin Nutr Metab Care. (2010) 13:52–7. doi: 10.1097/MCO.0b013e328332f9b7
12. Abdelghany AM, Zhang S, Azam M, Shaibu AS, Feng Y, Qi Jet al. Natural variation in fatty acid composition of diverse world soybean germplasms grown in China. Agronomy. (2020) 10:10024. doi: 10.3390/agronomy10010024
13. Gonz024z A D, Frostell B, Carlsson-Kanyama A. Protein efficiency per unit energy and per unit greenhouse gas emissions: potential contribution of diet choices to climate change mitigation. Food Policy. (2011) 36:562–70. doi: 10.1016/j.foodpol.2011.07.003
14. van der Weele C, Feindt P, Jan van der Goot J, van Mierlo B, van Boekel M. Meat alternatives: an integrative comparison. Trends Food Science Technol. (2019) 88:505–12. doi: 10.1016/j.tifs.2019.04.018
15. Valin H, Sands R.D., an der Mensbrugghe D, Nelsond GC, Ahammad H, Blanc E, Bodirsky B, et al. The future of food demand: understanding differences in global economic models Agricultural Economics. (2014) 45:51–67. doi: 10.1111/agec.12089
16. Henchion M, Moloney AP, Hyland J, Zimmermann J, McCarthy S. Review: trends for meat, milk and egg consumption for the next decades and the role played by livestock systems in the global production of proteins. Animal. (2021) 15:100287. doi: 10.1016/j.animal.2021.100287
17. Grigg D. The pattern of world protein consumption. Geoforum. (1995) 26:1–17. doi: 10.1016/0016-7185(94)00020-8
18. Henchion M, Hayes M, Mullen AM, Fenelon M, Tiwari B. Future protein supply and demand: strategies and factors influencing a sustainable equilibrium. Foods. (2017) 6: doi: 10.3390/foods6070053
19. Lieberman HR, Fulgoni VL, Agarwal S, Pasiakos SM, Berryman CE. Protein intake is more stable than carbohydrate or fat intake across various US demographic groups and international populations. Am J Clin Nutr. (2020) 12: 180–6. doi: 10.1093/ajcn/nqaa044
20. Raubenheimer D, Simpson S J; Protein leverage: theoretical foundations and ten points of clarification. Obesity (Silver Spring). (2019) 27:1225–38. doi: 10.1002/oby.22531
21. Simpson SJ, Raubenheimer D. The power of protein. Am J Clin Nutr. (2020) 112:6–7. doi: 10.1093/ajcn/nqaa088
22. Rizzo NS, Jaceldo-Siegl K, Sabate J, Fraser GE. Nutrient profiles of vegetarian and non-vegetarian dietary patterns. J Acad Nutr Diet. (2013) 113:1610–9. doi: 10.1016/j.jand.2013.06.349
23. Sobiecki JG, Appleby PN, Bradbury KE, Key TJ. High compliance with dietary recommendations in a cohort of meat eaters, fish eaters, vegetarians, and vegans: results from the European prospective investigation into cancer and nutrition-oxford study. Nutr Res. (2016) 36:464–77. doi: 10.1016/j.nutres.2015.12.016
24. Picherit C, Coxam V, Bennetau-Pelissero C, Kati-Coulibaly S, Davicco M J, Lebecque P, et al. Daidzein is more efficient than genistein in preventing ovariectomy- induced bone loss in rats. J Nutr. (2000) 130:1675–81. doi: 10.1093/jn/130.7.1675
25. Xu X, Duncan AM, Wangen KE, Kurzer MS. Soy consumption alters endogenous estrogen metabolism in postmenopausal women. Cancer Epidemiol Biomarkers Prev. (2000) 9:781–6.
26. EFSA Panel on Dietetic Products Nutrition and Allergies (NDA). Scientific opinion on dietary reference values for protein. EFSA J. (2012) 10:2557. doi: 10.2903/j.efsa.2012.2557
27. Rand WM, Pellett PL, Young VR. Meta-analysis of nitrogen balance studies for estimating protein requirements in healthy adults. Am J Clin Nutr. (2003) 77:109–27. doi: 10.1093/ajcn/77.1.109
28. Elango R, Ball RO, Pencharz PB. Indicator amino acid oxidation: concept and application. J Nutr. (2008) 138:243–6. doi: 10.1093/jn/138.2.243
29. Bandegan A, Courtney-Martin G, Rafii M, Pencharz PB, Lemon PWR. Indicator amino acid oxidation protein requirement estimate in endurance-trained men 24 h postexercise exceeds both the EAR and current athlete guidelines. Am J Physiol Endocrinol Metab. (2019) 316:E741–8. doi: 10.1152/ajpendo.00174.2018
30. Rafii M, Chapman K, Elango R, Campbell W W, Ball R O, Pencharz P B, et al. Dietary protein requirement of men >65 years old determined by the indicator amino acid oxidation technique is higher than the current estimated average requirement. J Nutr. (2016) 146:681–7. doi: 10.3945/jn.115.225631
31. Rafii M, Chapman K, Owens J, Elango R, Campbell W W, Ball R O, et al. Dietary protein requirement of female adults >65 years determined by the indicator amino acid oxidation technique is higher than current recommendations. J Nutr. (2015) 145:18–24. doi: 10.3945/jn.114.197517
32. Marinangeli CPF, Fabek H, Ahmed M, Sanchez-Hernandez D, Foisy S, House JD. The effect of increasing intakes of plant protein on the protein quality of Canadian diets. Appl Physiol Nutr Metab. (2021) 46:771–80. doi: 10.1139/apnm-2020-1027
33. Nosworthy MG, Neufeld J, Frohlich P, Young G, Malcolmson L, House JD. Determination of the protein quality of cooked Canadian pulses. Food Sci Nutr. (2017) 5:896–903. doi: 10.1002/fsn3.473
34. Rutherfurd SM, Fanning AC, Miller BJ, Moughan PJ. Protein digestibility-corrected amino acid scores and digestible indispensable amino acid scores differentially describe protein quality in growing male rats. J Nutr. (2015) 145:372–9. doi: 10.3945/jn.114.195438
35. Mathai JK, Liu Y, Stein HH. Values for digestible indispensable amino acid scores (DIAAS) for some dairy and plant proteins may better describe protein quality than values calculated using the concept for protein digestibility-corrected amino acid scores (PDCAAS). Br J Nutr. (2017) 117:490–9. doi: 10.1017/S0007114517000125
36. Domic J, Grootswagers P, van Loon LJC, de Groot L. Perspective: vegan diets for older adults? A perspective on the potential impact on muscle mass and strength. Adv Nutr. (2022). doi: 10.1093/advances/nmac009. [Epub ahead of print].
37. Institute of Medicine. Dietary Reference Intakes for Energy, Carbohydrate, Fiber, Fat, Fatty Acids, Cholesterol, Protein, and Amino Acids. Washington, DC: The National Academies Press. (2005). doi: 10.17226/10490
38. Mariotti F Plant protein animal protein and protein quality in Vegetarian and plant-based diets in health and disease prevention F. Mariotti, editor, Cambridge, MA: Academic Press. (2017) p. 621–42. doi: 10.1016/B978-0-12-803968-7.00035-6
39. Mangels R, Messina V, Messina M The The Dietitian's Guide to Vegetarian Diets. 3rd ed. Burlington, Massachusetts: Jones and Bartlett (2011).
40. Ciuris C, Lynch H M, Wharton C, Johnston CS. A comparison of dietary protein digestibility, based on DIAAS scoring, in vegetarian and non-vegetarian athletes. Nutrients. (2019) 11:23016. doi: 10.3390/nu11123016
41. Mariotti F, Gardner CD. Dietary protein and amino acids in vegetarian diets-a review. Nutrients. (2019) 11:2661. doi: 10.3390/nu11112661
42. Millward D J, Layman D K, Tome D, Schaafsma G. Protein quality assessment: impact of expanding understanding of protein and amino acid needs for optimal health. Am J Clin Nutr. (2008) 87:1576S–81S. doi: 10.1093/ajcn/87.5.1576S
43. Health Council of the Netherlands. Dietary Reference Intakes: Energy, Proteins, Fats and Digestible Carbohydrates. The Hague: Health Council of the Netherlands. (2001). Available online at: https://www.healthcouncil.nl/binaries/healthcouncil/documents/advisory-reports/2001/07/18/dietary-reference-intakes-energy-proteins-fats-and-digestible-carbohydrates/advisory-report-dietary-reference-intakes-energy-proteins-fats-and-digestible-carbohydrates.pdf
44. Moreno LA, Meyer R, Donovan SM, Goulet O, Haines J, Kok FJ, Van't Veer P. Perspective: striking a balance between planetary and human health: is there a path forward? Adv Nutr. (2021) 13:355–75. doi: 10.1093/advances/nmab139
45. Neff R A, Edwards D, Palmer A, Ramsing R, Righter A, Wolfson J. Reducing meat consumption in the USA: a nationally representative survey of attitudes and behaviours. Public Health Nutr. (2018) 21:1835–44. doi: 10.1017/S1368980017004190
46. Dagevos H, Voordouw J; Sustainability and meat consumption: is reduction realistic? Sustainabil: Sci, Prac, Pol. (2013) 9:60–9. doi: 10.1080/15487733.2013.11908115
47. Woolf PJ, Fu LL, Basu A. vProtein: identifying optimal amino acid complements from plant-based foods. PLoS ONE. (2011) 6:e18836. doi: 10.1371/journal.pone.0018836
48. Muoki PN, de Kock HL, Emmambux MN. Effect of soy flour addition and heat-processing method on nutritional quality and consumer acceptability of cassava complementary porridges. J Sci Food Agric. (2012) 92:1771–9. doi: 10.1002/jsfa.5545
49. Rafii M, Pencharz P B, Ball R O, Tomlinson C, Elango R, Courtney-Martin G. Bioavailable methionine assessed using the indicator amino acid oxidation method is greater when cooked chickpeas and steamed rice are combined in healthy young men. J Nutr. (2020) 150:1834–44. doi: 10.1093/jn/nxaa086
50. Fanelli NS, Bailey HM, Guardiola LV, Stein HH. Values for digestible indispensable amino acid score (DIAAS) determined in pigs are greater for milk than for breakfast cereals, but DIAAS values for individual ingredients are additive in combined meals. J Nutr. (2021) 151:540–7. doi: 10.1093/jn/nxaa398
51. Maziya-Dixon B, Alamu EO, Popoola IO, Yomeni M. Nutritional and sensory properties: snack food made from high-quality cassava flour and legume blend. Food Sci Nutr. (2017) 5:805–11. doi: 10.1002/fsn3.464
52. Twum LA, Ocloo FCK, Duah-Bisiw D, Odai BT. Determining the effect of heat treatment on iron fortified soybean gari blend and its bioavailability. Sci African. (2021):12 e00763. doi: 10.1016/j.sciaf.2021.e00763
53. Atuna RA, Amagloh FC, Denwar NN, Asase VR, Faisal S, Baako E, et al. Filling the protein gap in Ghana: the role of soy. Front Sustain Food Syst. (2022) 5:781571. doi: 10.3389/fsufs.2021.781571
55. Reynaud Y, Buffiere C, Cohade B, Vauris M, Liebermann K, Hafnaoui N, et al. True ileal amino acid digestibility and digestible indispensable amino acid scores (DIAASs) of plant-based protein foods. Food Chem. (2020) 338:128020. doi: 10.1016/j.foodchem.2020.128020
56. Fanelli NS, Bailey HM, Thompson TW, Delmore R, Nair MN, Stein HH. Digestible indispensable amino acid score (DIAAS) is greater in animal-based burgers than in plant-based burgers if determined in pigs. Eur J Nutr. (2022) 61:461–75. doi: 10.1007/s00394-021-02658-1
57. FAO (2013). Dietary protein Quality Evaluation in Human Nutrition. Report of an FAO Expert Consultation. Rome: FAO Food and Nutrition Paper 92.
58. Fanelli NS, Bailey HM, Thompson TW, Delmore R, Nair MN, Stein HH. Digestible indispensable amino acid score (DIAAS) is greater in animal-based burgers than in plant-based burgers if determined in pigs. Eur J Nutr. (2021) 61:461–75.
59. Dinkova-Kostova AT, Kostov RV; Glucosinolates and isothiocyanates in health and disease. Trends Mol Med. (2012) 18:337–47. doi: 10.1016/j.molmed.2012.04.003
60. Kunyanga CN, Imungi JK, Okoth M, Momanyi C, Biesalski HK, Vadivel V. Antioxidant and antidiabetic properties of condensed tannins in acetonic extract of selected raw and processed indigenous food ingredients from Kenya. J Food Sci. (2011) 76:C56076:1 doi: 10.1111/j.1750-3841.2011.02116.x
61. Hernandez-Infante M, Sousa V, Montalvo I, Tena E. Impact of microwave heating on hemagglutinins, trypsin inhibitors and protein quality of selected legume seeds. Plant Foods Hum Nutr. (1998) 52:199–208. doi: 10.1023/A:1008033610737
62. Rackis J J; Biological and physiological factors in soybeans. J Am Oil Chem Soc. (1974) 51:161A–74A. doi: 10.1007/BF02542123
63. Liener IE. Effects of processing on antinutritional factors in legumes: the soybean case. Arch Latinoam Nutr. (1996) 44:48S–54S.
64. Shi L, Mu K, Arntfield SD, Nickerson MT. Changes in levels of enzyme inhibitors during soaking and cooking for pulses available in Canada. J Food Sci Technol. (2017) 54:1014–22. doi: 10.1007/s13197-017-2519-6
65. Friedman M, Brandon DL. Nutritional and health benefits of soy proteins. J Agric Food Chem. (2001) 49:1069–86. doi: 10.1021/jf0009246
66. Xiao CW, Wood CM, Robertson P, Gilani GS. Protease inhibitor activities and isoflavone content in commercial soymilks and soy-based infant formulas sold in Ottawa, Canada. J Food Comp Anal. (2011) 25:130–6. doi: 10.1016/j.jfca.2011.10.001
67. Guo QC, Liang HH, Qin WH. The research on trypsin inhibitor activity for commercial soy beverage. China Dairy Ind. (1997) 25:8–10.
68. DairyMiyagi Y, Shinjo S, Nishida R, Miyagi C, Takamatsu K, Yamamoto T, et al. Trypsin inhibitor activity in commercial soybean products in Japan. J Nutr Sci Vitaminol. (1997) 43:575–80. doi: 10.3177/jnsv.43.575
69. Rackis J J, McGhee J E, Booth A N. Biological threshold levels of soybean trypsin inhibitors by rat bioassay. Cereal Chem. (1975) 52:85–92. doi: 10.1007/BF02545077
70. Murphy PA, Barua K, Hauck CC. Solvent extraction selection in the determination of isoflavones in soy foods. J Chromatogr B Analyt Technol Biomed Life Sci. (2002) 777:129–38. doi: 10.1016/S1570-0232(02)00342-2
71. Messina M, Nagata C, Wu A H. Estimated Asian adult soy protein and isoflavone intakes. Nutr Cancer. (2006) 55:1–12. doi: 10.1207/s15327914nc5501_1
72. Kuiper GG, Lemmen JG, Carlsson B, Corton JC, Safe SH, van der Saag PT, et al. Interaction of estrogenic chemicals and phytoestrogens with estrogen receptor beta. Endocrinology. (1998) 139:4252–63. doi: 10.1210/endo.139.10.6216
73. Paruthiyil S, Parmar H, Kerekatte V, Cunha GR, Firestone GL, Leitman DC. Estrogen receptor beta inhibits human breast cancer cell proliferation and tumor formation by causing a G2 cell cycle arrest. Cancer Res. (2004) 64:423–8. doi: 10.1158/0008-5472.CAN-03-2446
74. Messina M. A brief historical overview of the past two decades of soy and isoflavone research. J Nutr. (2010) 140:1350S–4S. doi: 10.3945/jn.109.118315
75. Rock CL, Doyle C, Demark-Wahnefried W, Meyerhardt J, Courneya KS, Schwartz AL, et al. Nutrition and physical activity guidelines for cancer survivors. CA Cancer J Clin. (2012) 62:242–74. doi: 10.3322/caac.21142
76. American Institute for Cancer Research; Soy is safe for breast cancer survivors (2012). Available online at: http://www.aicr.org/cancer-research-update/november_21_2012/cru-soy-safe.html (accessed Feburary 5, 2013).
77. World Cancer Research Fund International. Continuous Update Project Report: Diet, Nutrition, Physical Activity, and Breast Cancer Survivors. 2014 (2014). Available online at: www.wcrf.org/sites/default/files/Breast-Cancer-Survivors-2014-Report.pdf (accessed December 10, 2014).
78. Canadian, Cancer Society,. Eating well after breast cancer. Available online at: https://www.cancer.ca/en/cancer-information/cancer-type/breast/supportive-care/eating-well-after-breast-cancer/?region=on (accessed October 25, 2019).
79. EFSA ANS Panel (EFSA Panel on Food Additives and Nutrient Sources added to Food). Scientific opinion on the risk assessment for peri- and post-menopausal women taking food supplements containing isolated isoflavones. EFSA J. (2015) 13:4246. doi: 10.2903/j.efsa.2015.4246
80. Huser S, Guth S, Joost H G, Soukup S T, Kohrle J, Kreienbrock L, et al. Effects of isoflavones on breast tissue and the thyroid hormone system in humans: a comprehensive safety evaluation. Arch Toxicol. (2018) 92:2703–48. doi: 10.1007/s00204-018-2279-8
81. Otun J, Sahebkar A, Ostlundh L, Atkin SL, Sathyapalan T. Systematic review and meta-analysis on the effect of soy on thyroid function. Sci Rep. (2019) 9:3964. doi: 10.1038/s41598-019-40647-x
82. Reed K E, Camargo J, Hamilton-Reeves J, Kurzer M, Messina M. Neither soy nor isoflavone intake affects male reproductive hormones: an expanded and updated meta-analysis of clinical studies. Reprod Toxicol. (2021) 100:60–7. doi: 10.1016/j.reprotox.2020.12.019
83. Fleshner NE, Kapusta L, Donnelly B, Tanguay S, Chin J, Hersey K, et al. Progression from high-grade prostatic intraepithelial neoplasia to cancer: a randomized trial of combination vitamin-E, soy, and selenium. J Clin Oncol. (2011) 29:2386–90. doi: 10.1200/JCO.2010.32.0994
84. Sathyapalan T, Rigby AS, Bhasin S, Thatcher NJ, Kilpatrick ES, Atkin SL. Effect of soy in men with type 2 diabetes mellitus and subclinical hypogonadism: a randomized controlled study. J Clin Endocrinol Metab. (2017) 102:425–33. doi: 10.1210/jc.2016-2875
85. Mitchell JH, Cawood E, Kinniburgh D, Provan A, Collins AR, Irvine DS. Effect of a phytoestrogen food supplement on reproductive health in normal males. Clin Sci (Lond). (2001) 100:613–8. doi: 10.1042/CS20000212
86. Beaton LK, McVeigh BL, Dillingham BL, Lampe JW, Duncan AM. Soy protein isolates of varying isoflavone content do not adversely affect semen quality in healthy young men. Fertil Steril. (2010) 94:1717–22. doi: 10.1016/j.fertnstert.2009.08.055
87. Messina M, Watanabe S, Setchell K D; Report on the 8th international symposium on the role of soy in health promotion and chronic disease prevention and treatment. J Nutr. (2009) 139:796S–802S. doi: 10.3945/jn.108.104182
88. Food Labeling: Health Claims. Soy Protein and Coronary Heart Disease. A Proposed Rule by the Food and Drug Administration on 10/31/2017. Available online at: https://www.federalregister.gov/documents/2017/10/31/2017-23629/food-labeling-health-claims-soy-protein-and-coronary-heart-disease.
89. Messina M, Mejia SB, Cassidy A, Duncan A, Kurzer M, Nagato C, et al. Neither soyfoods nor isoflavones warrant classification as endocrine disruptors: a technical review of the observational and clinical data. Crit Rev Food Sci Nutr. (2021) 1–57. doi: 10.1080/10408398.2021.1895054
90. Messina M. and Venter C. Recent surveys on food allergy prevalence. Nutr Today. (2020) 55:22–9. doi: 10.1097/NT.0000000000000389
91. Bartra J, Garcia-Moral A, Enrique E. Geographical differences in food allergy. Bundesgesundheitsblatt Gesundheitsforschung Gesundheitsschutz. (2016) 59:755–63. doi: 10.1007/s00103-016-2357-0
92. Burney P, Summers C, Chinn S, Hooper R, van Ree R, Lidholm J. Prevalence and distribution of sensitization to foods in the European community respiratory health survey: a EuroPrevall analysis. Allergy. (2010) 65:1182–8. doi: 10.1111/j.1398-9995.2010.02346.x
93. McGowan EC, Keet CA. Prevalence of self-reported food allergy in the national health and nutrition examination survey (NHANES) 2007–2010. J Allergy Clin Immunol. (2013) 132:1216–9. doi: 10.1016/j.jaci.2013.07.018
94. Savage JH, Kaeding AJ, Matsui EC, Wood RA. The natural history of soy allergy. J Allergy Clin Immunol. (2010) 125:683–6. doi: 10.1016/j.jaci.2009.12.994
95. Willett W, Rockstrom J, Loken B, Springmann M, Lang T, Vermeulen S, et al. Food in the Anthropocene: the EAT-Lancet Commission on healthy diets from sustainable food systems. Lancet. (2019) 393:447–92. doi: 10.1016/S0140-6736(18)31788-4
96. Clark M A, Domingo N G G, Colgan K, Thakrar S K, Tilman D, Lynch J, et al. Global food system emissions could preclude achieving the 15 degrees and 2 degrees C climate change targets. Science. (2020) 370:705–8. doi: 10.1126/science.aba7357
97. Barnsley JE, Chandrakumar C, Gonzalez-Fischer C, Eme PE, Bourke BEP, Smith NW, et al. Lifetime climate impacts of diet transitions: a novel climate change accounting perspective. Sustainability. (2021) 13:5568. doi: 10.3390/su13105568
98. Ridoutt BG, Baird D, Hendrie GA. Diets within planetary boundaries: what is the potential of dietary change alone? Sustainable Production Consumption. (2021) 28:802–10. doi: 10.1016/j.spc.2021.07.009
99. Leroy F, Abraini F, Beal T, Dominguez-Salas P, Gregorini P, Manzano P, et al. Animal board invited review: animal source foods in healthy, sustainable, and ethical diets - An argument against drastic limitation of livestock in the food system. Animal. (2022) 16:100457. doi: 10.1016/j.animal.2022.100457
100. Leip A, Bodirsky B L, Kugelberg S. The role of nitrogen in achieving sustainable food systems for healthy diets. Glob Food Sec. (2021) 28:100408. doi: 10.1016/j.gfs.2020.100408
101. Lassaletta L, Billen G, Grizzetti B, Anglade J, Garnier J. 50 year trends in nitrogen use efficiency of world cropping systems: the relationship between yield and nitrogen input to cropland. Environ Res Lett. (2014) 9:105011. doi: 10.1088/1748-9326/9/10/105011
102. Melillo JM. Disruption of the global nitrogen cycle: a grand challenge for the twenty-first century: This article belongs to Ambio's 50th anniversary collection. Theme: Eutrophication. Ambio. (2021) 50: 759–63 doi: 10.1007/s13280-020-01429-2
103. Wilkinson J M, Young R H; Strategies to reduce reliance on soya bean meal and palm kernel meal in livestock nutrition. J Appl Animal Nutr. (2020) 8:75l Nut doi: 10.3920/JAAN2020.0007
104. Song XP, Hansen MC, Potapov P, Adusei B, Pickering J, Adami M, et al. Massive soybean expansion in South America since 2000 and implications for conservation. Nat Sustain. (2021) 2021:729. doi: 10.1038/s41893-021-00729-z
105. Statista. Leading soybean producing countries worldwide from 2012/13 to 2020/21 (in million metric tons) Available online at: https://www.statista.com/statistics/263926/soybean-production-in-selected-countries-since-1980/ (accessed January 6, 2022).
106. Spangler K, Burchfield EK, Schumacher B. Past and current dynamics of US agricultural land use and policy. Front Sust Food Sys. (2020) 4:98. doi: 10.3389/fsufs.2020.00098
107. Carlsson-Kanyama A, Gonzalez AD. Potential contributions of food consumption patterns to climate change. Am J Clin Nutr. (2009) 89:1704S–9S. doi: 10.3945/ajcn.2009.26736AA
108. Saarinen M, Fogelholm M, Tahvonen R, Kurppa S. Taking nutrition into account within the life cycle assessment of food products. J Clean Prod. (2017) 149:828–44. doi: 10.1016/j.jclepro.2017.02.062
109. Tessari P, Lante A, Mosca G. Essential amino acids: master regulators of nutrition and environmental footprint? Sci Rep. (2016) 6:26074. doi: 10.1038/srep26074
110. Moughan P J. Population protein intakes and food sustainability indices: the metrics matter. Global Food Security. (2021) 29:100548. doi: 10.1016/j.gfs.2021.100548
111. van Mierlo K, Rohmer S, Gerdessen J C. A model for composing meat replacers: reducing the environmental impact of our food consumption pattern while retaining its nutritional value. J Cleaner Production. (2017) 165:930–50. doi: 10.1016/j.jclepro.2017.07.098
112. Berardy A, Johnston CS, Plukis A, Vizcaino M, Wharton C. Integrating protein quality and quantity with environmental impacts in life cycle assessment. Sustainability. (2019) 11:2747. doi: 10.3390/su11102747
113. Braun M, Muñoz I, Schmidt JH, Thrane M. Sustainability of soy protein from life cycle assessment. FASEB J. (2016) 30:894–5. doi: 10.1096/fasebj.30.1_supplement.894.5
114. Smetana S, Mathys A, Knoch A, Heinz V. Meat alternatives: life cycle assessment of most known meat substitutes. Int J Life Cycle Assess. (2015) 20:1254–67. doi: 10.1007/s11367-015-0931-6
115. Mejia M A, Frese Assessoch A, Heinz V. Meat alternatives: life cycle assessment of most known meat oduction of a large variety of meat analogs by three diverse factories. J Hunger Environmental Nutr. (2020) 15:699–711. doi: 10.1080/19320248.2019.1595251
116. Fres2518 EnvironmA, Craig WJ, Jaceldo-Siegl K, Sabadt, Jaceldo-Siegl K, SabautrA, Heinz V. Meat altes: a comparison of their sustainability and nutritional content. Sustainability. (2019) 11:3231. doi: 10.3390/su11123231
117. FAOUN (2022). Production of Crops and Livestock Products. FAOSTAT. Rome, Italy: Available online at: 3/15/2022 from http://www.fao.org/faostat/en/#home
118. Rajao R, Soares-Filho B, Nunes F, Borner J, Machado L, Assis D, et al. The rotten apples of Brazil's agribusiness. Science. (2020) 369:246–8. doi: 10.1126/science.aba6646
119. Thrane M, Paulsen P V, Orcutt M W, Krieger T M Soy protein: Impacts production and applications in Sustainable Protein Sources S.R. Nadathur, J.P.D. Wanasundara, and L. Scanlin, editors (2017), United Kingdom: Academic Press:. p. 23–46. doi: 10.1016/B978-0-12-802778-3.00002-0
120. Sasatani D. Utilization of food-grade soybeans in Japan. Report number JA2021-0040. 2021. Tokyo: USDA FAS.
121. Zhao W, Hasegawa K, Chen J. The use of food-frequency questionnaires for various purposes in China. Public Health Nutr. (2002) 5:829–33. doi: 10.1079/PHN2002374
122. Liu Z, Li W, Sun J, Liu C, Zeng Q, Huang J, et al. Intake of soy foods and soy isoflavones by rural adult women in China. Asia Pac J Clin Nutr. (2004) 13:204–9. doi: 10.1201/9781439822203.ch3
123. Lee SA, Wen W, Xiang YB, Barnes S, Liu D, Cai Q, et al. Assessment of dietary isoflavone intake among middle-aged Chinese men. J Nutr. (2007) 137:1011–6. doi: 10.1093/jn/137.4.1011
124. Yang G, Shu XO, Jin F, Zhang X, Li HL, Li Q, et al. Longitudinal study of soy food intake and blood pressure among middle-aged and elderly Chinese women. Am J Clin Nutr. (2005) 81:1012–7. doi: 10.1093/ajcn/81.5.1012
125. McDermott J, Wyatt AJ. The role of pulses in sustainable and healthy food systems. Ann N Y Acad Sci. (2017) 1392:30–42. doi: 10.1111/nyas.13319
126. Gardner CD, Hartle JC, Garrett RD, Offringa LC, Wasserman AS. Maximizing the intersection of human health and the health of the environment with regard to the amount and type of protein produced and consumed in the United States. Nutr Rev. (2019) 77:197–215. doi: 10.1093/nutrit/nuy073
127. Figueira N, Curtain F, Beck E, Grafenauer S. Consumer understanding and culinary use of legumes in Australia. Nutrients. (2019) 11:1575. doi: 10.3390/nu11071575
128. Cordain L, Miller JB, Eaton SB, Mann N, Holt SH, Speth JD. Plant-animal subsistence ratios and macronutrient energy estimations in worldwide hunter-gatherer diets. Am J Clin Nutr. (2000) 71:682–92. doi: 10.1093/ajcn/71.3.682
129. Michel F, Hartman C, Siegrist M. Consumers' associations, perceptions and acceptance of meat and plant-based meat alternatives. Food Quality Preference. (2021) 87:104063. doi: 10.1016/j.foodqual.2020.104063
130. Reipurth MFS, Hurth Mty PreferenceG, Bonke A, Perez Cueto FJA. Barriers and facilitators towards adopting a more plant-based diet in a sample of Danish consumers. Food Qual Prefer. (2019) 73:288–92. doi: 10.1016/j.foodqual.2018.10.012
131. Curtain F, Grafenauer S; Plant-based meat substitutes in the flexitarian age: an audit of products on supermarket shelves. Nutrients. (2019) 11:2603. doi: 10.3390/nu11112603
132. van Vliet S, Kronberg SL, Provenza FD. Plant-based meats, human health, and climate change. Front Sust Food Sys. (2020) 4:128. doi: 10.3389/fsufs.2020.00128
133. Sadler MJ. Meat alternatives - market developments and health benefits. Trends Food Sci Technol. (2004) 15:250–60. doi: 10.1016/j.tifs.2003.09.003
134. Bianchi F, Stewart C, Astbury NM, Cook B, Aveyard P, Jebb SA. Replacing meat with alternative plant-based products (RE-MAP): a randomized controlled trial of a multicomponent behavioral intervention to reduce meat consumption. Am J Clin Nutr. (2021). doi: 10.1093/ajcn/nqab414. [Epub ahead of print].
135. Ryan RM, Deci EL. Self-determination theory and the facilitation of intrinsic motivation, social development, and well-being. Am Psychol. (2000) 55:68–78. doi: 10.1037/0003-066X.55.1.68
136. Grasso S, Jaworska S.Part meat and part plant: are hybrid meat products fad or future? Foods. (2020) 9:1888. doi: 10.3390/foods9121888
137. Askew K. Making 'healthier' meat: consumer attitudes towards to better-for-you-burgers and bangers. (2020). Available online at: https://www.foodnavigator.com/Article/2020/07/02/Making-healthier-meat-Consumer-attitudes-to-better-for-you-burgers-and-bangers (accessed July 2, 2020).
Keywords: soybeans, protein, versatility, efficiency, sustainable, requirements, soyfoods
Citation: Messina M (2022) Perspective: Soybeans Can Help Address the Caloric and Protein Needs of a Growing Global Population. Front. Nutr. 9:909464. doi: 10.3389/fnut.2022.909464
Received: 31 March 2022; Accepted: 13 April 2022;
Published: 06 May 2022.
Edited by:
Gianluca Rizzo, Independent Researcher, Messina, ItalyReviewed by:
Emmanuel Oladeji Alamu, International Institute of Tropical Agriculture, ZambiaJustyna Godos, University of Catania, Italy
Copyright © 2022 Messina. This is an open-access article distributed under the terms of the Creative Commons Attribution License (CC BY). The use, distribution or reproduction in other forums is permitted, provided the original author(s) and the copyright owner(s) are credited and that the original publication in this journal is cited, in accordance with accepted academic practice. No use, distribution or reproduction is permitted which does not comply with these terms.
*Correspondence: Mark Messina, bWFya2pvaG5tZXNzaW5hQGdtYWlsLmNvbQ==