- 1Experimental Center for Preventive Medicine Teaching, College of Public Health, Harbin Medical University, Harbin, China
- 2Department of Nutrition and Food Hygiene, College of Public Health, Harbin Medical University, Harbin, China
- 3Translational Medicine Research and Cooperation Center of Northern China, Heilongjiang Academy of Medical Sciences, Harbin, China
Objective: This study aims to identify dietary branched-chain amino acids (BCAA) consumption trajectories in Chinese adults and to evaluate their association with the risk of hyperuricemia (HU).
Methods: Cohort data from the China Health and Nutrition Survey 1997–2009 were adopted in this research. A total of 6,810 participants aged ≥18 years were included in this study. Participants were designated into four subgroups on basis of the trajectories of dietary BCAA consumption. Cox proportional hazards models were performed to discuss the relationships between varied trajectories and the risk of HU after adjusting potential confounders. The intermediary effect of differential blood indexes between the trajectories and the risk of HU was explored with mediation analysis.
Results: Four distinct trajectory groups of dietary BCAA consumption were identified. Compared with the low stable trajectory group, high to low trajectory group was greatly related to an increased risk of HU (HR 1.35 (95% CI 1.03 to 1.79)) with modification for covariates. Total cholesterol (TC), hemoglobin A1c (HbA1c), fasting blood glucose (FBG), and triglyceride (TG) partially regulated trajectories and HU.
Conclusion: Gradually decreasing dietary BCAA intake increased the risk of HU, which is, at least, partially mediated by TC, HbA1c, FBG, and TG levels.
Introduction
Hyperuricemia (HU) is a disease, in which serum uric acid (UA) exceeds the normal range due to abnormal purine metabolism for various reasons (1). The pooled prevalence of HU was 13.3% in mainland China from 2000 to 2014 (2). HU is considered a risk factor for gout (3), cardiovascular disease (4), stroke (5), diabetes (6), and hypertension (7). HU is becoming a great health issue and is arousing more attention. Currently, most studies on HU have been limited to some regions or nationality, and the pathophysiology of HU has not yet been fully illustrated. Hence, nationally representative research based on the entire population on the epidemiology of HU is needed.
Dietary factor, which is an adjustable element, exerts significant effect on the occurrence and development of HU. Branched-chain amino acids (BCAAs), including Leucine, Isoleucine, and Valine, an important group of basic amino acids, are significant nutrition signals with important roles in protein synthesis, glucose homeostasis, and nutrient-sensitive signaling pathways (8). Previous research has shown that adequate BCAA supplementation can reduce body weight and promote fat metabolism (9, 10). Meanwhile, BCAA can also reduce oxidative stress by restoring mitochondrial function (11). Overweight, obesity, abnormal lipid metabolism, and oxidative stress are potential risk factors for HU (12). However, the association between dietary BCAA consumption and risk of HU are still unknown. Most studies about dietary BCAA intake adopted a single or limited number of measurements. However, little research has been designed to investigate the dynamic change of dietary BCAA levels and the risk of HU. It is necessary to use a time-varying measurement of dietary BCAA to explore the relationship between dietary BCAA trajectory and HU risk.
Therefore, this study firstly used latent class trajectory modeling (LCTM) to identify dietary BCAA consumption trajectories over 12 years in the Chinese adults and to evaluate their association with the risk of HU.
Materials and Methods
Study Population
China Health and Nutrition Survey (CHNS), which stands for 47% of the Chinese population, is designed as prospective household-based research, including various ages and cohorts across different provinces, and five follow-up surveys between 1997 and 2009. Detailed information of CHNS has previously been provided (13). The Institutional Review Committees of the University of North Carolina at Chapel Hill, NC, United States approved the survey protocols, instruments, and process for acquiring informed consent, as well as the China National Institute of Nutrition and Food Safety at the Chinese Center for Disease Control and Prevention, Beijing, China. All participants offered survey data after written informed consent.
A total of 13,575 adults participated in CHNS from 1997 to 2009, with no missing value for BCAA intake were selected in this study. Individuals with missing serum UA information (n = 1,419) and data of demographic or total nutrient intakes dietary interview (n = 228) were excluded. Participates who took part in only one survey (n = 5,118) were excluded. After exclusion, a total of 6,810 adults, including 3,212 men and 3,598 women, with a mean age of 42.5 ± 13.4 years and a mean follow-up time of 9.98 years, met the study criteria and ranged from two to six measurement surveys (two visits, n = 807; three visits, n = 1,148; four visits, n = 1,550; five visits, n = 3,305).
Questionnaire Survey
Detailed in-person interviews were managed by trained personnel with a structured questionnaire, to collect data of demographic features, dietary habits, lifestyle, physical condition, and anthropometric features. In CHNS, the collection of individual dietary intake for three consecutive days was made for every household member. Dietary measurements included total energy (kcal/day), dietary fat (g/day), dietary protein (g/day), dietary carbohydrate (g/day), dietary fiber (g/day), and vitamin C (VC, mg/day), which were calculated by three versions of Chinese food composition table (FCT) according to CHNS project requirements. The 1991 FCT version was adopted in 1997 and 2000. The 2002/2004 (two books integrated) FCT versions were adopted in 2004, 2006, and 2009. Present smoking was defined as a positive answer to the question “do you still smoke now?”. Participants who gave the answer of “never smoker” to the question “Have you ever smoked (such as hand-rolled or device-rolled)?” were categorized into the group of never smoked, and who positively answered the questions “Have you ever smoked (such as hand-rolled or device-rolled)?” and negatively answered “do you still smoke now?” as ex-smoker. Drinks and a standard drink was any drink that contained about 0.6 fluid ounces or 14 grams of pure alcohol were adopted to measure the amount of alcohol consumed (14). Physical activity level (PAL) mainly contained occupational activity, transportation activity, domestic activity, and leisure activity (15). The calculation of total metabolic equivalents (METs) of physical activity MET-h per week was made (15).
In 2009, 12 ml of blood (in three 4-ml tubes) were collected from all individuals on an empty stomach. The measurement of serum UA and fasting blood glucose (FBG) was made by enzymatic colorimetric and glucose oxidase (GOD-PAP) method (Hitachi 7600, Randox, United Kingdom). Total cholesterol (TC) and triglyceride (TG) were measured by cholesterol oxidase (CHOD-PAP) method and glycerol-phosphate oxidase (GPO-PAP) method (Hitachi 7600, Kyowa, Japan). The measurement of HDL-cholesterol (HDL-C) was made by enzymatic approaches (Hitachi 7600, Kyowa, Japan). The measurement of hemoglobin A1c (HbA1c) and high-sensitivity C reactive protein (hs-CRP) was made by high performance liquid chromatography method (HLC-723 G7/D10/PDQ A1c, Japan) and immunoturbidimetric (Hitachi 7600, Denka Seiken, Japan). HU was defined as serum UA ≥7 mg/dl in males and ≥6 mg/dl in females. Hypertension was defined as persistent systolic blood pressure measurements of ≥140 mmHg and/or 90 mmHg of diastolic blood pressure. The calculation of body mass index (BMI) as weight in kilograms divided by the square of height in meters was made. Self-report of a history of diabetes diagnosis, and/or FBG ≥ 7 mmol/L, and/or HbA1c ≥40 mmol/mol (6.5%), and/or receiving treatment for diabetes were adopted to identify Type 2 diabetes (T2D). Details of treating diabetes included special diet, weight control, oral medication, insulin injection, Chinese traditional medicine, and home remedies.
Statistical Analysis
All statistical analyses were conducted with R 4.1.31. A two-sided p-value <0.05 was of statistical significance. Dietary nutrient intake and anthropometric measurements were shown as mean ± standard deviation (SD) for continuous variables and percentage for categorical variables. General linear models and baseline features were compared with Chi-square tests.
The LCTM is a relatively new methodology in epidemiology to describe life-course exposures, which simplifies heterogeneous populations into homogeneous patterns or classes. Model fit was assessed using both the Bayesian information criterion and entropy measures in conjunction with Vuong-Lo-Mendell-Rubin likelihood ratio test. The LCTM has three general advantages. First, it better informs etiological associations by deeply phenotyping certain “at risk” subpopulations. Second, LCTM offers a public health strategy to identify early divergent adverse trajectories as potential intervention targets. Third, the trajectory approach allows a better understanding of the causes of between-individual variation in certain features.
Dietary BCAA consumption trajectories were identified with LCTM, a censored normal model applying the R package lcmm. The best fit and each trajectory class included at least 3% of the sample population, which were decided with statistically rigorous Bayesian information criteria. Once trajectories of dietary BCAA consumption were decided, and a nominal categorical variable was generated to illustrate the trajectory classes of every participant, which was then adopted in Cox multivariate regression models.
After calculating the follow-up time of non-HU and HU, Cox proportional-hazards model was adopted to assess the relationship between dietary BCAA consumption trajectories and the risk of HU. A set of potential confounders and effect modifiers, which were age, sex, smoking, education, PAL, urban index, total dietary energy, fat, protein, carbohydrate, VC intake, BMI, and disease state of hypertension and T2D, were controlled.
After categorizing participants into varied dietary BCAA consumption trajectories, the association between acquired dietary BCAA consumption trajectories and blood indexes modified with the above co-variables was determined with subgroup analyses by generalized linear models, which could recognize HU-associated blood indexes that were statistically different in varied trajectories.
At last, the R package lavaan was adopted to make mediation analysis models, to investigate whether these biomarkers with modification for the above covariates were adopted to mediate the relationship between dietary BCAA consumption trajectories and risk of HU.
Sensitivity Analysis
Two sets of sensitivity analyses were made below: in set 1, blood samples from the participants were first collected in 2009, the relationship between dietary BCAA intake and the risk of HU for participants in 2009 was analyzed using logistic regression model; and in set 2, the association between mean dietary BCAA intake during follow-ups and the risk of HU from 1997 to 2009 was analyzed with logistic regression models.
Results
Trajectories of Dietary Branched-Chain Amino Acids Intake
In this cohort of 6,810 Chinese adults, the trajectories of BCAA intake were displayed in Figure 1. Every trajectory group was named based on dynamic variations of the BCAA intake levels. Figure 1 corresponded with the first trajectory, labeled “T1: Low stable,” to participants who kept low BCAA intake throughout the survey period. The second trajectory, “T2: High to low,” corresponded to participants whose BCAA intake gradually decreased from high to low by comparing with T1. The third trajectory, “T3: Moderate stable,” corresponded to participants whose BCAA intake remained in moderate level by comparing with T1. The fourth trajectory, “T4: Moderate to high then decline,” corresponded to participants whose intake of BCAA gradually increased to moderate level and then decreases. It was estimated that the trajectories from T1 to T4 include 12.1, 7.8, 76.1, and 4% of participants.
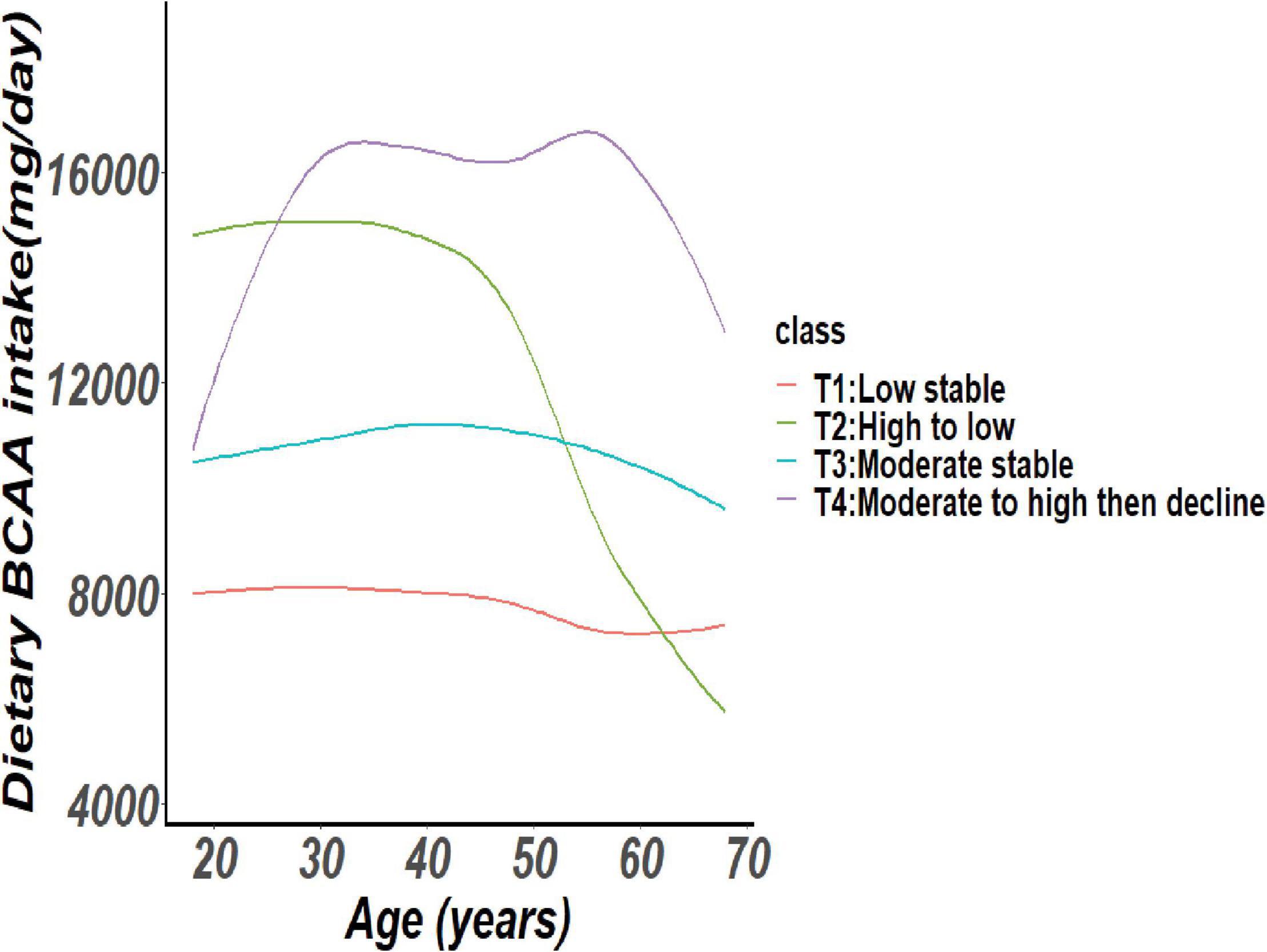
Figure 1. Trajectories of dietary branched-chain amino acids (BCAA) consumption (n = 6,810) in the Chinese adults from the CHNS by LCTM. BCAA, Branched chain amino acids; CHNS, China Health and Nutrition Survey; LCTM, Latent class trajectory modelling.
Baseline Features by Varied Trajectories of Dietary Branched-Chain Amino Acids Intake
The baseline characteristics of different dietary BCAA intake trajectories were displayed in Table 1. There were great diversities in age, gender, smoking, drinks, education levels, total energy, fat, protein, carbohydrate, VC intake, BMI, T2D, and hypertension among the four trajectories (p < 0.05). There was no great diversity in PAL among the different dietary BCAA intake trajectories (p > 0.05).
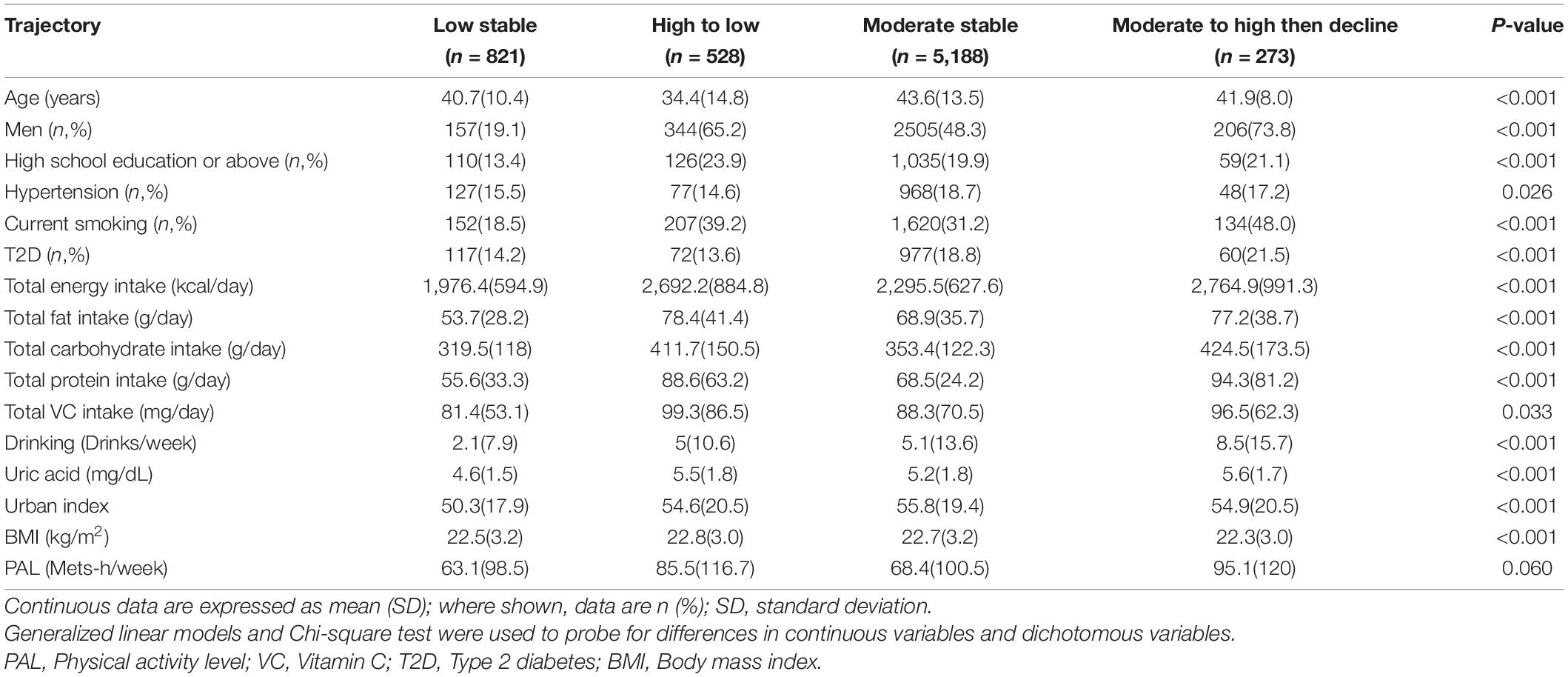
Table 1. Baseline characteristics of study variables by different trajectories of dietary branched-chain amino acids (BCAA) consumption (N = 6,810).
Association Between Dietary Branched-Chain Amino Acids Intake Trajectories and Risk of Hyperuricemia
The relationships between dietary BCAA intake trajectories and risk of HU were displayed in Table 2. By comparing with “T1,” the trajectory labeled “T2” was greatly related to growing risk of HU (HR 1.35 (95% CI 1.03, 1.79)) with adjustment for covariates.
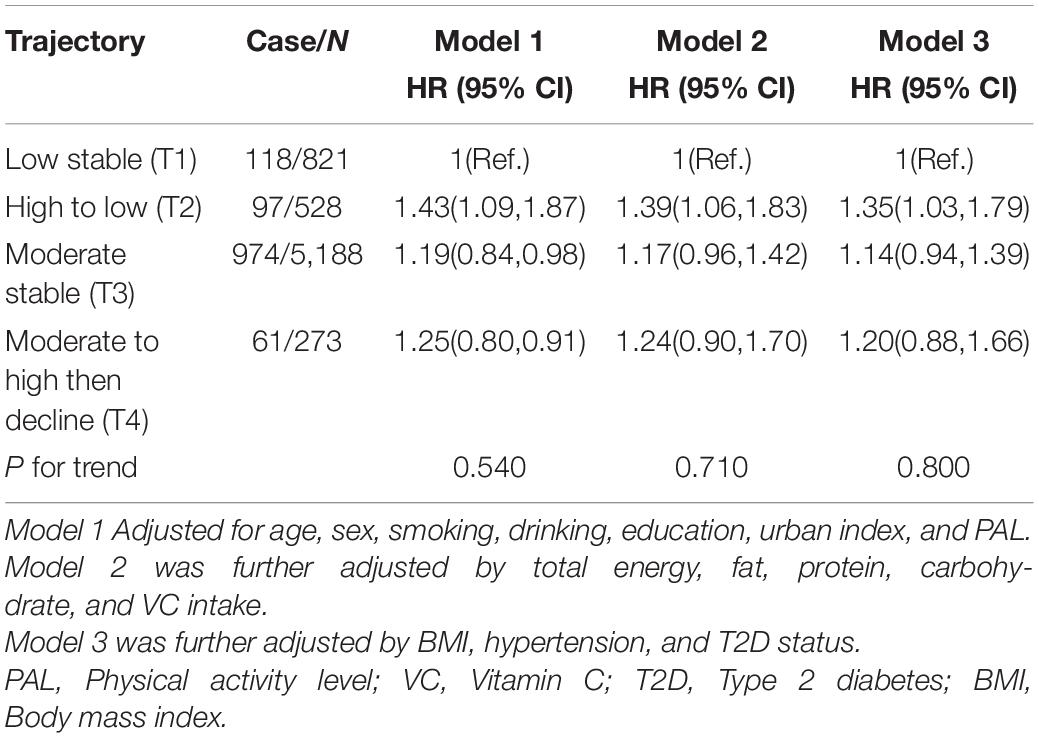
Table 2. Association between dietary branched-chain amino acids (BCAA) consumption trajectories and hyperuricemia (HU) by Cox regression models.
Trajectories of Dietary Branched-Chain Amino Acids Intake and Biomarkers of Hyperuricemia
The biomarkers across different dietary BCAA intake trajectories were shown in Table 3. TG, TC, FBG, and HbA1c in the T2 trajectory were higher than the other three trajectories (T1, T3, and T4) (all p for trend <0.05). HDL-C and hs-CRP in the T2 trajectory were not statistically different from the other trajectories.
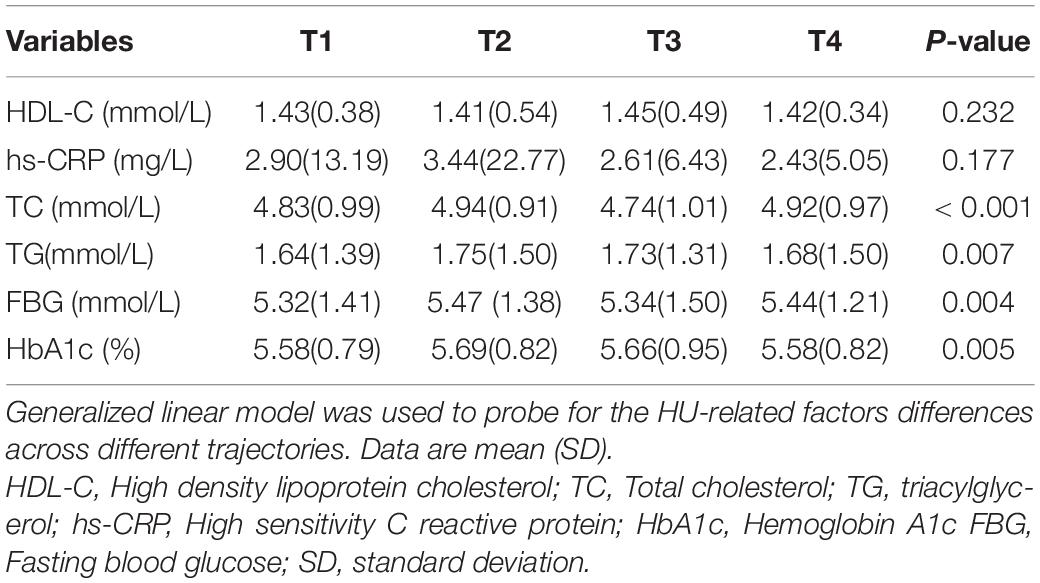
Table 3. Difference for hyperuricemia (HU)-related factors across dietary branched-chain amino acids (BCAA) consumption trajectories in 2009.
Mediation Analysis
Figure 2 demonstrates mediation results of TC, HbA1c, FBG, and TG in the relationship between dietary BCAA trajectory (T2) and risk of HU. The total effect of dietary BCAA intake trajectories was estimated at 7.5%. The total indirect role for four factors was calculated with β1 to β8. It was estimated that the percentages of the total effect mediated by TC, HbA1c, FBG, and TG are 10.2%, 4.2%, 10.2%, and 12.7%, respectively.
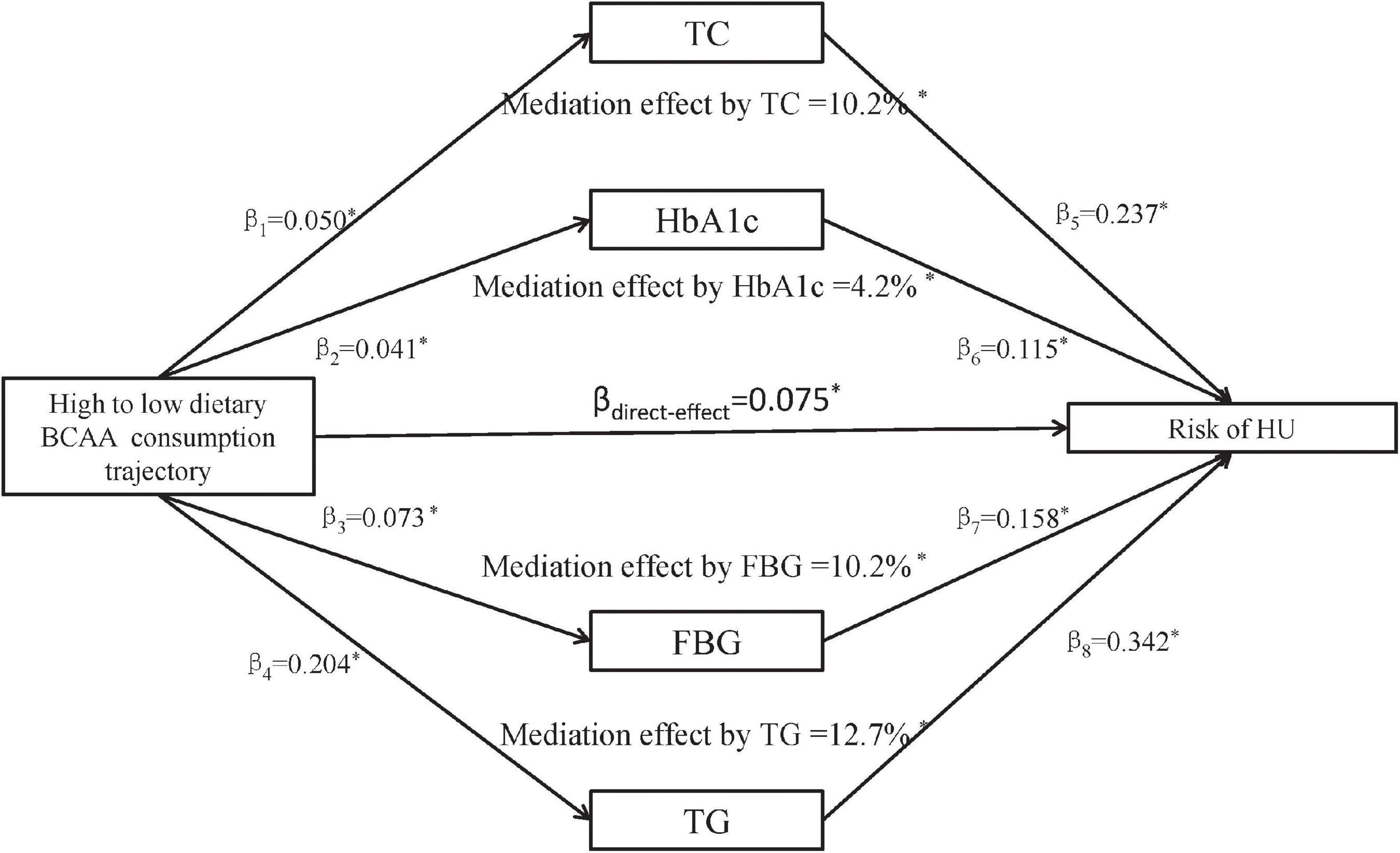
Figure 2. Mediation effects of TC, HbA1c, FBG, and TG on the association between dietary branched-chain amino acids (BCAA) consumption trajectories and risk of HU. Data were standardized regression coefficients with adjustment for covariates; *p < 0.05 for coefficients different from 0. TC, Total cholesterol; HbA1c, Hemoglobin A1c; FBG, Fasting blood glucose; TG, Triglyceride; BCAA, Branched chain amino acids; HU, Hyperuricemia.
Sensitivity Analysis
Sensitivity analyses demonstrated that compared to the lowest quintile, the highest quintile of dietary BCAA intake was not greatly related to the risk of HU in 2009 (OR, 1.16, 95%CI (0.76, 1.33)) in Table 4. Similarly, the highest quintile of mean dietary BCAA intake during follow-ups was also not significantly associated with the risk of HU from 1997 to 2009 (OR, 1.18, 95%CI (0.94, 1.49)) in Table 5.

Table 4. Association between dietary branched-chain amino acids (BCAA) consumption and hyperuricemia (HU) by Logistic regression models in 2009 (N = 6,810).

Table 5. Association between Mean dietary branched-chain amino acids (BCAA) intake during follow-ups and hyperuricemia (HU) by Logistic regression model (N = 6,810).
Discussion
In this prospective cohort of Chinese adults with five surveys, four unique dietary BCAA intake trajectories were identified, in which the high to low trajectory group was greatly related to increased risk of HU. In addition, high to low trajectory has higher TC, HbA1c, FBG, and TG than other trajectories, which may explain the association between trajectory and HU.
Obesity is considered as an independent risk factor for HU. Obesity can cause HU by increasing UA synthesis and inhibiting its excretion. Changes in obesity measure indices levels are independently associated with subsequent changes in UA concentrations. Previous studies have shown a strong association between obesity and HU (16–18). Higher dietary BCAA intake was related to lower prevalence of overweight and obesity in Asia (19). BCAA contributes to the oxidation of fat, and decreases fat in the body (20). Increased dietary leucine intake significantly reduced body weight and improved glucose and cholesterol metabolism (21). It has been found that adenosine monophosphate-activated protein kinase (AMPK) is involved in the basic control of whole-body energy balance by controlling food intake and energy expenditure in response to hormonal and nutritional signals in the central nervous system and peripheral tissues (22). Negative AMPK expression in the hypothalamus is clearly enough to decrease food intake and body weight (23). Mammalian target of rapamycin (mTOR), which is a serine/threonine kinase, takes part in a lot of cellular processes, such as protein synthesis, cell metabolism, and growth (24). Studies have shown that activation of mTOR can inhibit food intake (25). However, dietary leucine intake can lead to weight loss by reducing AMPK or increasing mTOR activity (26). Meanwhile, the BMI of the high to low trajectory group was greatly higher than the other trajectory groups in this research, which confirmed the results of previous studies.
The systemic inflammatory response index (SIRI), a potent indicator of HU, is independently associated with the risk of HU (27). Studies have also shown that decreasing the intake of proinflammatory diet decreases the incidence of HU in women (28). However, BCAA plays an anti-inflammatory role in the body or indirectly regulates inflammatory states (29). Reducing the intake of BCAA may increase inflammation in the body and lead to the occurrence of HU. The hs-CRP is widely used laboratory markers of systemic inflammation. Previous studies demonstrated no correlation between hs-CRP levels and valine (30), and there was no statistical difference in hs-CRP between different trajectories in this study, which was consistent with previous research result.
Insulin resistance (IR) is closely associated with the occurrence and growth of HU by inhibiting UA excretion and increasing sodium reabsorption in renal tubules (31, 32). It is well-known that increased glucose content in the liver is a major characteristic of IR. Elevated liver glucose content is due to the activation of c-Jun N-terminal kinase (JNK) caused by the produced reactive oxygen species (ROS), leading to phosphorylation of FoxO1 and nuclear accumulation of FoxO1, and ultimately leading to increased liver glucose content (33). Intake of BCAA can improve IR by activating antioxidant mechanisms and can reduce ROS production in the liver by improving albumin metabolism (34). A cross-sectional study has demonstrated that higher intakes of BCAA were associated with lower IR, inflammation, blood pressure, and adiposity-related metabolites (35). Therefore, the risk of HU might be reduced by BCAA intake.
Blood glucose control is of great significance for the prevention of HU (36). Studies have shown that increased isoleucine intake can stimulate the uptake of glucose by skeletal muscle, leading to a reduction in blood glucose (37). Growing leucine intake can enhance glucose metabolism, decrease the insulin resistance induced by diets, and lower levels of plasma glucagon and the expression of hepatic glucose 6 phosphatase, which is the key enzyme for regulating hepatic glucose production (38). In addition, previous studies have shown that UA levels increase significantly with the increase of FBG in the non-diabetic phase (39). Leucine levels were inversely correlated with FBG, and FBG increased when leucine intake was reduced (40). Reduced BCAA intake will lead to increased FBG, which can increase UA levels in the body.
The alteration of blood lipid may be another mechanism to explain our observations. Higher TG and TC levels were significantly correlated with the increase of serum UA (41). The risk of HU in patients with hypertriglyceridemia was higher than those in patients with normal TG (42). High TG may disrupt the metabolism of free fatty acids and accelerate the breakdown of adenosine triphosphate, which ultimately results in a growth in UA (43). Isoleucine reduces TG accumulation by affecting fatty acid oxidation (44), which decreases the incidence of HU. Animal studies have shown that compared to the control group, when mice consumed more isoleucine, the adiposity of liver and skeletal muscle was less, which indicated that the TG levels in both tissues were lowered. Several studies have shown that higher intake of BCAA presents lower occurrence of hypertriglyceridemia (45).
There are several strengths in this study. Primarily, this is the first research in this subject area that was made with a relatively great cohort size and long follow-up duration. Secondly, this research firstly explored the association between the dynamic trajectory of dietary BCAA intake and the risk of HU by using LCTM in Chinese adults. Thirdly, results of this study using dietary trajectory analysis were not presented in cross-sectional data, which meant that the study using dietary trajectory method to discuss the association between dietary BCAA intake, and the incidence of HU might provide additional information in cohort study and emphasized the significance of utilizing LCTM to scientific research. Nevertheless, it was recognized that there were several restrictions in this research. First, the dietary data were acquired by questionnaires in CHNS, and the respondents might have misreported the mount and kinds of food intake, leading to inaccurate mean value for BCAA measurement in three consecutive days. Secondly, several confounding elements, which were not measured or recognized, might have affected the outcomes.
Conclusion
Gradually decreasing dietary BCAA intake increased the risk of HU, which is, at least, partially mediated by TC, HbA1c, FBG, and TG levels.
Data Availability Statement
The original contributions presented in this study are included in the article/supplementary material, further inquiries can be directed to the corresponding author/s.
Author Contributions
YL and SY conceived the concept. XR designed the research and prepared the original manuscript. WX researched and explained data. SW made the validation analyses. All authors have read and agreed to the published version of the manuscript.
Acknowledgments
We express sincere thanks to the University of North Carolina, Chapel Hill; the National Institutes of Health (R01-HD-30880, DK-056350, R24-HD-050924, and R01-HD-38700); the National Institute for Nutrition and Health, China Center for Disease Control and Prevention, Carolina Population Center (P2C-HD-050924 and T32-HD-007168); and the National Institutes of Health Fogarty International Center (D43-TW-009077 and D43-TW-007709) for economic support for the CHNS data collection.
Conflict of Interest
The authors declare that the research was conducted in the absence of any commercial or financial relationships that could be construed as a potential conflict of interest.
Publisher’s Note
All claims expressed in this article are solely those of the authors and do not necessarily represent those of their affiliated organizations, or those of the publisher, the editors and the reviewers. Any product that may be evaluated in this article, or claim that may be made by its manufacturer, is not guaranteed or endorsed by the publisher.
Footnotes
References
1. Lu X, Li X, Zhao Y, Zheng Z, Guan S, Chan P. Contemporary epidemiology of gout and hyperuricemia in community elderly in Beijing. Int J Rheumat Dis. (2014) 17:400–7. doi: 10.1111/1756-185x.12156
2. Liu R, Han C, Wu D, Xia X, Gu J, Guan H, et al. Prevalence of hyperuricemia and gout in mainland China from 2000 to 2014: a systematic review and meta-analysis. Biomed Res Int. (2015) 2015:762820. doi: 10.1155/2015/762820
3. Richette P, Frazier A, Bardin T. Pharmacokinetics considerations for gout treatments. Expert Opin Drug Metab Toxicol. (2014) 10:949–57. doi: 10.1517/17425255.2014.915027
4. Kim SY, Guevara JP, Kim KM, Choi HK, Heitjan DF, Albert DA. Hyperuricemia and coronary heart disease: a systematic review and meta-analysis. Arthritis Care Res (Hoboken). (2010) 62:170–80. doi: 10.1002/acr.20065
5. Tu W, Wu J, Jian G, Lori J, Tang Y, Cheng H, et al. Asymptomatic hyperuricemia and incident stroke in elderly Chinese patients without comorbidities. Eur J Clin Nutr. (2019) 73:1392–402. doi: 10.1038/s41430-019-0405-1
6. Mallat SG, Al Kattar S, Tanios BY, Jurjus A. Hyperuricemia, hypertension, and chronic kidney disease: an emerging association. Curr Hypertens Rep. (2016) 18:74. doi: 10.1007/s11906-016-0684-z
7. Li C, Hsieh MC, Chang SJ. Metabolic syndrome, diabetes, and hyperuricemia. Curr Opin Rheumatol. (2013) 25:210–6. doi: 10.1097/BOR.0b013e32835d951e
8. Yoshiharu S, Kitaura Y, Kadota Y. [Diversity of physiological functions of branched-chain amino acids]. Seikagaku. (2012) 84:938–42.
9. Novin ZS, Ghavamzadeh S, Mehdizadeh A. The weight loss effects of branched chain amino acids and vitamin b6: a randomized controlled trial on obese and overweight women. Int J Vitam Nutr Res. (2018) 88:80–9. doi: 10.1024/0300-9831/a000511
10. Mourier A, Bigard AX, de Kerviler E, Roger B, Legrand H, Guezennec CY. Combined effects of caloric restriction and branched-chain amino acid supplementation on body composition and exercise performance in elite wrestlers. Int J Sports Med. (1997) 18:47–55. doi: 10.1055/s-2007-972594
11. Korenaga M, Nishina S, Korenaga K, Tomiyama Y, Yoshioka N, Hara Y, et al. Branched-chain amino acids reduce hepatic iron accumulation and oxidative stress in hepatitis C virus polyprotein-expressing mice. Liver Int. (2015) 35:1303–14. doi: 10.1111/liv.12675
12. Qian DF, Fan GL, Chen P, He DC, Fan JD, Feng C, et al. [Risk factors for hyperuricemia in active and retired employees underwent physical examination]. Zhonghua Xin Xue Guan Bing Za Zhi. (2013) 41:60–4.
13. Zhang B, Zhai FY, Du SF, Popkin BM. The China health and nutrition survey, 1989-2011. Obesity Rev. (2014) 15(Suppl. 1):2–7. doi: 10.1111/obr.12119
14. Almoosawi S, Prynne CJ, Hardy R, Stephen AM. Time-of-day of energy intake: association with hypertension and blood pressure 10 years later in the 1946 British birth cohort. J Hypertens. (2013) 31:882–92. doi: 10.1097/HJH.0b013e32835ecc06
15. Ng SW, Howard AG, Wang HJ, Su C, Zhang B. The physical activity transition among adults in China: 1991-2011. Obesity Rev. (2014) 15(Suppl. 1):27–36. doi: 10.1111/obr.12127
16. Duan Y, Liang W, Zhu L, Zhang T, Wang L, Nie Z, et al. Association between serum uric acid levels and obesity among university students (China). Nutr Hospitalar. (2015) 31:2407–11. doi: 10.3305/nh.2015.31.6.8734
17. Oyama C, Takahashi T, Oyamada M, Oyamada T, Ohno T, Miyashita M, et al. Serum uric acid as an obesity-related indicator in early adolescence. Tohoku J Experiment Med. (2006) 209:257–62. doi: 10.1620/tjem.209.257
18. Ogura T, Matsuura K, Matsumoto Y, Mimura Y, Kishida M, Otsuka F, et al. Recent trends of hyperuricemia and obesity in Japanese male adolescents, 1991 through 2002. Metabolism. (2004) 53:448–53. doi: 10.1016/j.metabol.2003.11.017
19. Qin LQ, Xun P, Bujnowski D, Daviglus ML, Van Horn L, Stamler J, et al. Higher branched-chain amino acid intake is associated with a lower prevalence of being overweight or obese in middle-aged east Asian and western adults. J Nutr. (2011) 141:249–54. doi: 10.3945/jn.110.128520
20. Kainulainen H, Hulmi JJ, Kujala UM. Potential role of branched-chain amino acid catabolism in regulating fat oxidation. Exerc Sport Sci Rev. (2013) 41:194–200. doi: 10.1097/JES.0b013e3182a4e6b6
21. Zhang Y, Guo K, LeBlanc RE, Loh D, Schwartz GJ, Yu YH. Increasing dietary leucine intake reduces diet-induced obesity and improves glucose and cholesterol metabolism in mice via multimechanisms. Diabetes. (2007) 56:1647–54. doi: 10.2337/db07-0123
22. Xu J, Ji J, Yan XH. Cross-talk between ampk and mTOR in regulating energy balance. Crit Rev Food Sci Nutr. (2012) 52:373–81. doi: 10.1080/10408398.2010.500245
23. Minokoshi Y, Alquier T, Furukawa N, Kim YB, Lee A, Xue B, et al. AMP-kinase regulates food intake by responding to hormonal and nutrient signals in the hypothalamus. Nature. (2004) 428:569–74. doi: 10.1038/nature02440
24. Martínez de Morentin PB, Martinez-Sanchez N, Roa J, Ferno J, Nogueiras R, Tena-Sempere M, et al. Hypothalamic mTOR: the rookie energy sensor. Curr Mol Med. (2014) 14:3–21. doi: 10.2174/1566524013666131118103706
25. Zhang W, Zhang C, Fritze D, Chai B, Li J, Mulholland MW. Modulation of food intake by mTOR signalling in the dorsal motor nucleus of the vagus in male rats: focus on ghrelin and nesfatin-1. Exp Physiol. (2013) 98:1696–704. doi: 10.1113/expphysiol.2013.074930
26. Ropelle ER, Pauli JR, Fernandes MF, Rocco SA, Marin RM, Morari J, et al. A central role for neuronal AMP-activated protein kinase (AMPK) and mammalian target of rapamycin (mTOR) in high-protein diet-induced weight loss. Diabetes. (2008) 57:594–605. doi: 10.2337/db07-0573
27. Chen MQ, Wang HY, Shi WR, Sun YX. Estimate of prevalent hyperuricemia by systemic inflammation response index: results from a rural Chinese population. Postgrad Med. (2021) 133:242–9. doi: 10.1080/00325481.2020.1809870
28. Kim HS, Kwon M, Lee HY, Shivappa N, Hébert JR, Sohn C, et al. Higher pro-inflammatory dietary score is associated with higher hyperuricemia risk: results from the case-controlled korean genome and epidemiology study_cardiovascular disease association study. Nutrients. (2019) 11:1803. doi: 10.3390/nu11081803
29. Nicastro H, da Luz CR, Chaves DF, Bechara LR, Voltarelli VA, Rogero MM, et al. Does branched-chain amino acids supplementation modulate skeletal muscle remodeling through inflammation modulation? Possible mechanisms of action. J Nutr Metab. (2012) 2012:136937. doi: 10.1155/2012/136937
30. Hamaya R, Mora S, Lawler PR, Cook NR, Ridker PM, Buring JE, et al. Association of plasma branched-chain amino acid with biomarkers of inflammation and lipid metabolism in women. Circ Genom Precis Med. (2021) 14:e003330. doi: 10.1161/circgen.121.003330
31. Nakamura K, Sakurai M, Miura K, Morikawa Y, Nagasawa SY, Ishizaki M, et al. Homa-Ir and the risk of hyperuricemia: a prospective study in non-diabetic Japanese men. Diabetes Res Clin Pract. (2014) 106:154–60. doi: 10.1016/j.diabres.2014.07.006
32. Bonora E, Capaldo B, Perin PC, Del Prato S, De Mattia G, Frittitta L, et al. Hyperinsulinemia and insulin resistance are independently associated with plasma lipids, uric acid and blood pressure in non-diabetic subjects Gisir Database. Nutr Metabol Cardiovasc Dis. (2008) 18:624–31. doi: 10.1016/j.numecd.2007.05.002
33. Iwasa M, Kobayashi Y, Mifuji-Moroka R, Hara N, Miyachi H, Sugimoto R, et al. Branched-chain amino acid supplementation reduces oxidative stress and prolongs survival in rats with advanced liver cirrhosis. PLoS One. (2013) 8:e70309. doi: 10.1371/journal.pone.0070309
34. Deng L, Shoji I, Ogawa W, Kaneda S, Soga T, Jiang DP, et al. Hepatitis C virus infection promotes hepatic gluconeogenesis through an Ns5a-mediated, foxo1-dependent pathway. J Virol. (2011) 85:8556–68. doi: 10.1128/jvi.00146-11
35. Jennings A, MacGregor A, Pallister T, Spector T, Cassidy A. Associations between branched chain amino acid intake and biomarkers of adiposity and cardiometabolic health independent of genetic factors: a twin study. Int J Cardiol. (2016) 223:992–8. doi: 10.1016/j.ijcard.2016.08.307
36. Shi W, Xing L, Jing L, Tian Y, Liu S. Usefulness of triglyceride-glucose index for estimating hyperuricemia risk: insights from a general population. Postgrad Med. (2019) 131:348–56. doi: 10.1080/00325481.2019.1624581
37. Doi M, Yamaoka I, Nakayama M, Mochizuki S, Sugahara K, Yoshizawa F. Isoleucine, a blood glucose-lowering amino acid, increases glucose uptake in rat skeletal muscle in the absence of increases in AMP-activated protein kinase activity. J Nutr. (2005) 135:2103–8. doi: 10.1093/jn/135.9.2103
38. Ji L, Weng J, Lu J, Guo X, Yang W, Jia W, et al. Hyperglycemia and duration of diabetes as risk factors for abnormal lipids: a cross sectional survey of 19,757 patients with type 2 diabetes in China. J Endocrinol Invest. (2014) 37:843–52. doi: 10.1007/s40618-014-0115-4
39. Hairong N, Zengchang P, Shaojie W, Weiguo G, Lei Z, Jie R, et al. Serum uric acid, plasma glucose and diabetes. Diab Vasc Dis Res. (2010) 7:40–6. doi: 10.1177/1479164109347408
40. Barceló A, Morell-Garcia D, Salord N, Esquinas C, Pérez G, Pérez A, et al. A randomized controlled trial: branched-chain amino acid levels and glucose metabolism in patients with obesity and sleep APNEA. J Sleep Res. (2017) 26:773–81. doi: 10.1111/jsr.12551
41. Peng TC, Wang CC, Kao TW, Chan JY, Yang YH, Chang YW, et al. Relationship between hyperuricemia and lipid profiles in US adults. Biomed Res Int. (2015) 2015:127596. doi: 10.1155/2015/127596
42. Hou YL, Yang XL, Wang CX, Zhi LX, Yang MJ, You CG. Hypertriglyceridemia and hyperuricemia: a retrospective study of urban residents. Lipids Health Dis. (2019) 18:81. doi: 10.1186/s12944-019-1031-6
43. Balasubramanian T. Uric acid or 1-methyl uric acid in the urinary bladder increases serum glucose, insulin, true triglyceride, and total cholesterol levels in wistar rats. ScientificWorldJournal. (2003) 3:930–6. doi: 10.1100/tsw.2003.90
44. Nishimura J, Masaki T, Arakawa M, Seike M, Yoshimatsu H. Isoleucine prevents the accumulation of tissue triglycerides and upregulates the expression of Pparalpha and uncoupling protein in diet-induced obese mice. J Nutr. (2010) 140:496–500. doi: 10.3945/jn.109.108977
45. Cogate PG, Natali AJ, de Oliveira A, Alfenas RC, Hermsdorff HH. Consumption of branched-chain amino acids is inversely associated with central obesity and cardiometabolic features in a population of Brazilian middle-aged men: potential role of leucine intake. J Nutr Health Aging. (2015) 19:771–7. doi: 10.1007/s12603-015-0521-0
Keywords: branched chain amino acids, hyperuricemia, latent class trajectory model, China health and nutrition survey, mediation analysis
Citation: Ren X, Wu S, Xie W, Liu Y and Yang S (2022) Association Between the Risk of Hyperuricemia and Changes in Branched-Chain Amino Acids Intake Over Twelve Years: A Latent Class Trajectory Analysis From the China Health and Nutrition Survey, 1997–2009. Front. Nutr. 9:916446. doi: 10.3389/fnut.2022.916446
Received: 09 April 2022; Accepted: 23 June 2022;
Published: 11 August 2022.
Edited by:
Mainul Haque, National Defence University of Malaysia, MalaysiaCopyright © 2022 Ren, Wu, Xie, Liu and Yang. This is an open-access article distributed under the terms of the Creative Commons Attribution License (CC BY). The use, distribution or reproduction in other forums is permitted, provided the original author(s) and the copyright owner(s) are credited and that the original publication in this journal is cited, in accordance with accepted academic practice. No use, distribution or reproduction is permitted which does not comply with these terms.
*Correspondence: Ying Liu, bGl1eWluZzAyMjNAc29odS5jb20=; Shucai Yang, eWFuZ3NodWNhaTNAaHJibXUuZWR1LmNu
†These authors have contributed equally to this work